- 1ICAR Research Complex for NEH Region, Umiam, Meghalaya, India
- 2ICAR Research Complex for NEH Region, Tripura Centre, Lembucherra, Meghalaya, India
- 3ICAR-Indian Institute of Framing Systems Research, Modipuram, Meerut, India
- 4Faculty of Agricultural Sciences, Aligarh Muslim University, Aligarh, India
Introduction: Organic farming is a promising solution for mitigating environmental burdens related to input-intensive agricultural practices. The major challenge in organic agriculture is the non-availability of large quantities of organic inputs required for crop nutrition and sustaining soil health, which can be resolved by efficient recycling of the available on- and off-farm resources and the integration of the components as per the specific locations.
Methods: An integrated organic farming system (IOFS) model comprising agricultural and horticultural crops, rainwater harvesting units, livestock components, and provisions for nutrient recycling was developed and disseminated in the adopted organic villages Mynsain, Pynthor, and Umden Umbathiang in the Ri-Bhoi District, Meghalaya, India, to improve the income and livelihood of farmers. Harvested rainwater in farm ponds and Jalkunds was used for live-saving irrigation in the winter months and diversified homestead farming activities, such as growing high-value crops and rearing cattle, pigs, and poultry.
Results: Maize, french bean, potato, ginger, tomato, carrot, and chili yields in the IOFS model increased by 20%−30%, 40%−45%, 25%−30%, 33%−40%, 45%−50%, 37%−50%, and 27%−30%, respectively, compared with traditional practices. Some farmers produced vermicompost in vermibeds (made of high-density polyethylene) and cement brick chambers, generating 0.4−1.25 tons per annum. Two individual farmers, Mr. Jrill Makroh and Mrs. Skola Kurbah obtained net returns (without premium price) of Rs. 46,695 ± 418 and Rs. 31,102 ± 501 from their respective 0.27- and 0.21-ha IOFS models, which is equivalent to Rs. 172,944 ± 1,548/ha/year and Rs. 148,105 ± 2,385/ha/year, respectively. The net returns obtained from the IOFS models were significantly higher than those obtained from the farmers' practice of maize-fallow or cultivation of maize followed by vegetable (~30% of the areas). It is expected that, with the certification of organic products, the income and livelihood of the farmers will improve further over the years. While Mr. Jrill Makroh's model supplied 95.1%, 82.0%, and 96.0% of the total N, P2O5, and K2O, respectively, needed by the system, Mrs. Skola Kurbah's model supplied 76.0%, 68.6%, and 85.5% of the total N, P2O5, and K2O, respectively.
Discussion: Thus, IOFS models should be promoted among hill farmers so that they can efficiently recycle farm resources and increase their productivity, net returns, and livelihood while reducing their dependence on external farm inputs.
1. Introduction
Organic farming emerged as a solution to the input-driven industrialization of agricultural practices and its associated environmental and social problems. Organic farming combines tradition, innovation, and science to benefit the environment and the quality of life for all involved (Pleguezuelo et al., 2018). Organic farming that relies mostly on animal manure, organic waste, crop rotation, legumes, and biological pest control methods is practiced in the majority of the areas of North-East India, especially in the hill region (Das et al., 2017a,b). In the north-eastern hill (NEH) region of the country, the application of chemical fertilizers is very low and most of them are used in the valley ecosystem (Layek et al., 2023), but the upland ecosystem is free from the use of chemical fertilizers (Layek et al., 2018). Similarly, the use of pesticides in the region is very low because the farmers practice traditional methods for controlling insect pests and diseases (Das et al., 2017a,b). As such, the farmers have shown an inclination toward organic farming, which is being harnessed for the development of the region and has ecological benefits (Layek et al., 2020). It is estimated that 18 million hectares of such land are available in the NEH region, which can be exploited for organic production (Das et al., 2018). Agriculture in North-East India, especially in Meghalaya, is characterized by the limited use of external inputs, such as fertilizers and pesticides (14.0 kg N + P + K/ha and 0.032 kg/ha, respectively), the cultivation of traditional varieties, subsistence in nature, and low productivity (Das et al., 2017a,b; Devi et al., 2017). Most areas of Meghalaya (>70%) and the north-eastern region are hilly and mountainous tracks with moderate to steep slopes, <30% of which constitutes valley areas (Layek et al., 2019; Choudhury et al., 2022). Conventional farming with monocropping of rice and maize along with the cultivation of a few vegetables in the kitchen garden with inadequate inputs leads to very low productivity (Ansari et al., 2021). Vegetables constitute an important part of the diet of the Eastern Himalayan population (Pandey, 2002). This North-Eastern Region (NER) is not only rich in vegetable diversity but also in spices and fruits, which are an integral part of the farming system there (Deka et al., 2012). Growing vegetables after kharif maize not only increases cropping intensity but also utilizes the land efficiently while providing employment and economic benefits to the farmers, who are mostly small and marginal in nature (Layek et al., 2020). There is a yield gap of 25%−40% for most of the vegetables, such as okra, French bean, carrot, tomato, and potato, between their farm yield and the yield obtained from the ICAR experimental organic farms (Panwar et al., 2022). However, the NER, especially Meghalaya, has a lot of potential for improving agricultural productivity. The region is one of the mega-biodiversity zones of the world (Layek et al., 2019). The region receives high rainfall (>2,450 mm). The climate varies from tropical to temperate, and as a result, most of the crops could be grown in one or other part of the region. By virtue of the lower amounts of chemical inputs imported and utilized, the state of Meghalaya has a great scope for successful organic farming (Patel D. P. et al., 2014; Das et al., 2017a,b). The soils of the region are highly degraded due to cultivation on steep slopes, negligible nutrient supplementation, and biomass burning under traditional practices (Roy et al., 2018; Ansari et al., 2022b). Organic farming is considered one of the best options for protecting/sustaining soil health and producing healthy foods. The objectives of environmental, social, and economic sustainability can be met through organic farming (Saldarriaga-Hernandez et al., 2020). It is assumed that the difference in the production gap due to the adoption of organic agriculture will be negligible in the region. There is scope for enhancing productivity with good organic management since most of the households are maintaining livestock (pig, poultry, cattle, goats, etc.) and producing enough on-farm manures, which could be efficiently used for organic agriculture (Ravisankar et al., 2021, 2022).
Most people are non-vegetarians and rear animals, especially pigs and poultry, so a good amount of animal excreta is generated, which is essential for successful organic farming (Das et al., 2017a,b). However, the major constraint to the success of ‘organic farming' is the non-availability of huge quantities of organic inputs, and the application of animal excreta is not sufficient alone to meet the demand for nutrients for the crops (Das et al., 2017a,b; Layek et al., 2019). The favorable climatic conditions and high concentration of soil organic carbon (SOC) allows the huge growth of plant biomass (weeds, shrubs, residues, etc.), which can be recycled in crop production as a vital source of nutrient supply (Patel D. P. et al., 2014; Layek et al., 2023). The adoption of organic agriculture in an integrated farming system approach, viz., an integrated organic farming system (IOFS), which utilizes all the on-farm and off-farm resources judiciously by using the byproducts or output of one as the input for the other, can make organic farming sustainable and profitable (Das et al., 2019). Thus, the focus should be on integrating complementary and supplementary enterprises, such as crops, fruits, vegetables, livestock, poultry, fish, multipurpose tree species, and mushrooms, along with adequate nutrient recycling strategies (Panwar et al., 2021a,b; Ravisankar et al., 2021). One such IOFS model has been developed at the ICAR Research Complex for North Eastern Hill Region, Umiam, Meghalaya, India through the scientific integration of different enterprises, such as crops, fruits, vegetables, livestock, poultry, and fish, along with adequate nutrient recycling strategies (composting/vermicomposting) and the use of water from farm ponds (Das et al., 2017a,b; Layek et al., 2020). The net income of IOFS model was enhanced from farmer's practice I and II by 355% and 191%, respectively. The IOFS model could meet 92%, 82%, and 96% (N, P2O5, and K2O, respectively) of its nutrient demand within the system.
Although the region gets substantial rainfall during the months of April to November, there is virtually no rainfall during the winter season, especially from November to March (Layek et al., 2022). The creation of water harvesting structures is essential in the hills to supply water in the winter season for livestock and crops maintained in an organic farming system. Owing to a lack of water harvesting structures/irrigation facilities in the hills, the farmers are cultivating only one crop per year, leading to low cropping intensity and limited income (Bujarbaruah, 2004; Layek et al., 2020). However, water conservation in hills is very difficult as traditional farm ponds in the hill regions are exposed to very high water loss through infiltration, percolation, and seepage loss (Lairenjam et al., 2014; Das et al., 2017a,b). The seepage and percolation from the dug-out ponds/tanks can be prevented using UV-resistant polyethylene films that have high tensile strength, are durable, and are resistant to external pressure, e.g., Silpaulin (200 GSM or more). These low-cost rainwater harvesting structures, known as “Jalkunds,” have storage capacities of 30,000–45,000 L and can be key to the success of an IOFS model (Samuel and Satapathy, 2008; Layek et al., 2020). Major emphasis should also be placed on the management of livestock components, such as dairy, pigs or poultry, and compost preparation for the supply of year-round quality manure and income generation in an IOFS. For disseminating the IOFS technology, a model village concept in line with the Network Project on Organic Farming-Tribal Sub Plan (NPOF-TSP) was implemented in the village of Mynsain in the Ri-Bhoi District of Meghalaya with financial assistance from the ICAR-Indian Institute of Farming Systems Research, Modipuram. Several farmers in the village started practicing organic farming in an IOFS model. This practice increased crop productivity and diversified homestead farming to grow remunerable crops and rear cattle, pigs, poultry, etc.
2. Materials and methods
For disseminating organic production technologies developed by the ICAR Research Complex for NEH Region, Umiam, a model village concept for organic farming using a cluster approach in line with the Network Project on Organic Farming-Tribal Sub Plan (NPOF-TSP) was initiated between 2013 and 2014 in the village of Mynsain (25°44′21,61″ N−92°1′1,73″ E, 853–901 AMSL) in the Ri-Bhoi District of Meghalaya with financial assistance from the ICAR-Indian Institute of Farming Systems Research, Modipuram. To disseminate the IOFS technology in a cluster approach (group of neighboring farmers), areas where farmers were either not using or using a meager amount of synthetic fertilizers and pesticides were identified. A sensitization meeting with the villagers, including the village head (Headman), members of self-help groups, and the Department of Agriculture (Gram Sabha), was organized before the work was initiated, and subsequently, a group of farmers visited the ICAR, Umiam to obtain first-hand experience of the various technologies that would be used in the program. A participatory rural appraisal (PRA) was undertaken at the project site at Mynsain village to obtain information about the local agrosystem, resources, farming practices, and social structure and identify problems within the farming community. The village has 132 households with a population of 600 people. Most people in the village are Christians. The main occupation in the village is agriculture. Paddy (Oryza sativa), maize (Zea mays), and ginger (Zingiber officinale) are the main crops that are cultivated. Ginger is the cash crop and is the most profitable as it is a non-perishable crop, and it has become a major source of income. Paddy is mostly cultivated for self-consumption. There are other crops and vegetables that the villagers grow, such as sweet potatoes, potato, pumpkin, yam, corn, tomato, beans, and chili. There are also few households that rear livestock, including cows, pigs, and hens. The prevailing soil in the lowland and upland regions of the village were sampled at a depth of 0–15 and 15–30 cm, and the average nitrogen (N) content, phosphorus (P) content, soil organic content (SOC), and pH content were analyzed using standard procedures. The available N, P, SOC, and pH of the soil in the lowland region was 210.1 ± 27.8 kg/ha, 9.1 ± 6.4 kg/ha, 16.0 ± 3.2 mg/kg, and 4.97 ± 0.62, respectively, for a depth of 0–15 cm. Similarly, the available N, P, SOC, and pH of the soil in the upland region was 201.9 ± 35.7 kg/ha, 21.2 ± 12.5 kg/ha, 13.1 ± 3.9 mg/kg, and 4.81 ± 0.67 pH, respectively, for a depth of 0–15 cm.
Organic farming using the cluster approach was implemented in three villages over a total area of 110 ha comprising 315 farmers, 100% of whom belonged to tribal communities. In these three villages, IOFS technology had been adopted by 35 farmers. A georeferenced characterization of the All India-Network Programme on Organic Farming (AI-NPOF) adopted villages revealed that most of the farmers grew crops such as rice, maize, ginger, and turmeric only in the rainy season and produced a very low acreage of winter crops, especially vegetables, due to a lack of irrigation water. Additionally, crop productivity was much lower in the villages due to a lack of improved varieties, limited availability of manure or animal excreta, and the absence of synthetic fertilizers and pesticides. However, most of the farmers from the villages maintained farm animals (poultry, pigs, goats, or cattle) in an isolated way but paid very little attention to the production of quality manure or vermicompost. The rationale was that the adoption of an IOFS would not reduce productivity but rather would enhance it due to the fact that farmers in the villages were previously using a significant amount of manure or fertilizers and pesticides for crop production. Multidisciplinary programs covering agriculture, horticulture, fishery, livestock, food-feed crops, rainwater harvesting, composting/vermicomposting, green manuring, etc., were integrated into the IOFS model to consider the problems that farmers face and the resources available to them. Emphasis was placed on local demand, socio-economic issues, ecology, and the effective recycling of on-farm resources to minimize dependency on external resources and generate continuous income and employment while supplying nutritious food to the farmers' families.
Within the program, seeds of improved varieties of crops and vegetables, planting materials, lime, rock phosphate, neem cake, and other organic inputs were provided to the adopted farmers. Effective soil fertility management through the application of well-decomposed organic manures, such as farmyard manure, green leaf manure, and composts, was promoted. For pest and disease management, the use of neem oil, Trichoderma, derisome (bio-insecticide prepared from extract of Pongamia glabra/Pongamia pinnata), and indigenous technical knowledge (ITK) was emphasized. In terms of ITK, farmers mixed cow dung with water in rice fields to control rice hispa (Dicladispa armigera), smoked pumpkin fields to control fruit flies (Bactocera cucurbitae), placed red tree ant (Oecophylla smoragdina) nests on citrus plants to control citrus trunk borers (Anoplophora verstegii), and placed dried Artemisia vulgaris leaves and/or branches in and around granaries to control stored insects and rats (Deka et al., 2006). For promoting small-scale mechanization, implements and tools, such as paddy threshers, rice mills, sprayers, tulu pumps, and cono weeders, were provided to the village and a custom hiring center was established. Additionally, farmers were trained in various aspects of organic farming and the conservation of natural resources and residue recycling.
2.1. Weather parameters of the experimental area
The meteorological data of the villages (2014–2016) is graphically presented in Figure 1. The temperature was moderate for the most of the year except for a few months in winter. The daily minimum temperatures tended to rise from January and this trend was maintained the until June, decreasing from July onwards. For most of the year the maximum relative humidity was more than 75%. The mean annual evaporation was ~850 mm. Although the area received an annual average rainfall of 2,450 mm, most of the rainfall occurred between April and November, and there was little or no rainfall between December and March.
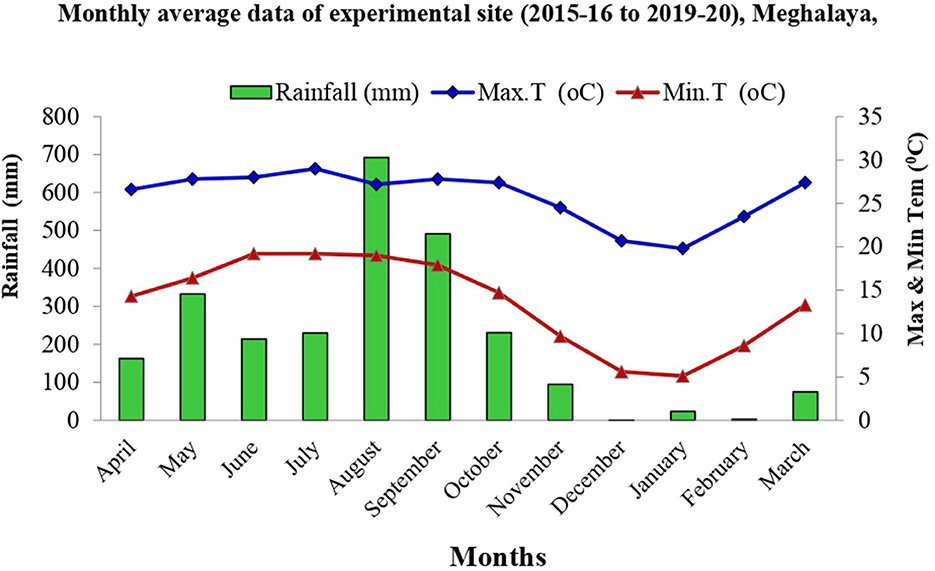
Figure 1. Monthly average rainfall and maximum and minimum temperatures during crop growing seasons in Umiam (average of 2015–16, 2016–17, 2017–18, 2018–19, and 2019–20).
2.2. Integrated organic farming system model development in the village using a cluster approach
Several IOFS models were developed from 2014 onwards in the village according to the situation and crop and livestock preferences. A flowchart showing the developmental steps of the IOFS model in the fields of farmers is presented in Figure 2. They integrated crops, viz., maize (Zea mays L.), vegetables, viz., tomato (Solanum lycopersicum), French bean (Phaseolus vulgaris), cabbage (Brassica oleracea var. capitata), cauliflower (Brassica oleracea var. botrytis), broccoli (Brassica oleracea var. italica), potato (Solanum tuberosum), lettuce (Lactuca sativa), and carrot (Daucus carota), spice crops, viz., ginger (Zingiber rubens), turmeric (Curcuma longa), chili (Capsicum annuum), fruit trees, viz., Assam lemon (Citrus limon L. Burmf ), papaya (Carica papaya), banana (Musa paradisiaca), guava (Psidium guajava), etc., livestock (dairy, pigs, and poultry), water harvesting (Jalkund), compost units, etc. (Figures 4A, B). Water from a micro water-harvesting structure, such as a Jalkund, was used for live-saving irrigation during the winter months. These structures increased crop productivity and diversified their homestead farming to growing remunerable crops and rearing cattle, pigs, poultry, etc. The IOFS model promotes crop diversification, thereby providing food security and employment for the farmers around the year. The different components in the model practiced by farmers on their farm itself are depicted in Figure 3. This approach involved the use of outputs of one enterprise component as inputs for other related enterprises wherever feasible, e.g., cattle dung mixed with crop residues and farm waste was converted into nutrient-rich vermicompost. Therefore, there was less dependence on organic manure from external sources. A judicious mixture of livestock enterprises, such as dairy, poultry, fish, goat-rearing, and vermicomposting, helped to generate additional income. Climbing vegetables, such as bottle gourd, chow-chow, cucumber, and ridge gourd, were grown on a structure created above water bodies on one side of the Jalkund for vertical intensification. Pumpkin was raised on another side of the Jalkund and allowed to crawl on the ground. During the rainy season, roof water was harvested and stored in a Jalkund. Jalkunds in the model have multipurpose uses, such as irrigation, supply to the piggery and poultry, and mushroom block making. Before the distribution of improved vegetable seeds, beneficiary farmers were trained in nursery raising and the scientific methods of vegetable cultivation. A community nursery was formed in the villages for raising seedlings of cole crops, such as cabbage, broccoli, and cauliflower. This activity was crucial for obtaining strong and healthy vegetable seedlings.
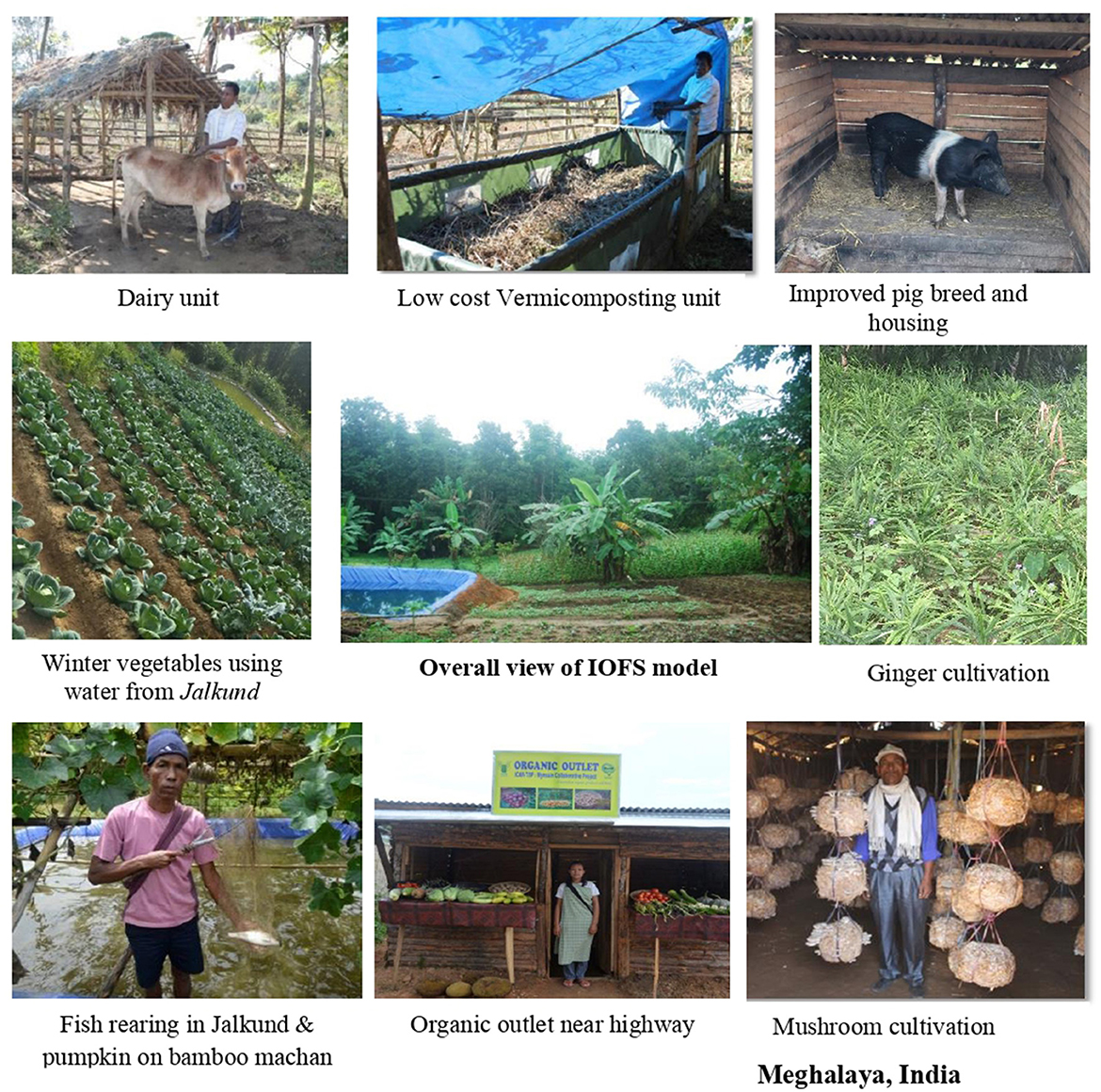
Figure 3. Different components of the IOFS model developed in the farmers' fields in Meghalaya, India.
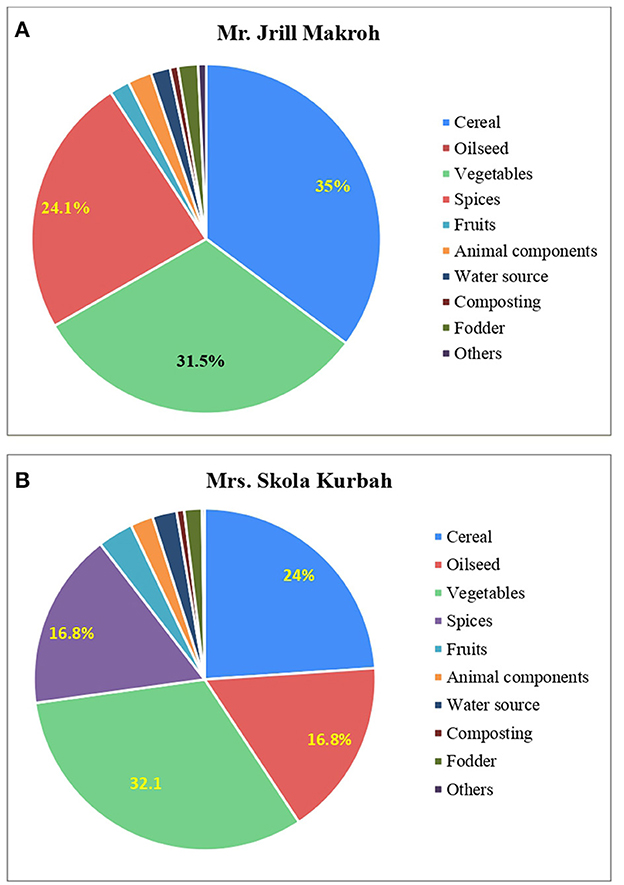
Figure 4. Allocation of different enterprises in the IOFS models of two farmers in the adopted villages. (A) Mr. Jrill Makroh and (B) Mrs. Skola Kurbah.
New interventions, such as mushroom houses and honey boxes, were also implemented to obtain additional income and use farm resources more efficiently. Although paddy straw was used to produce organic mushrooms, honeybee plays an important role in pollination and overall crop performance. Oyster mushroom cultivation was carried out, except in July and August when the incidence of insect pests and competitor molds is very high. The PL-14-02 oyster mushroom (Pleurotus florida) strain was used as it has very high biological efficiency (~106%) and therefore produces a very high yield in comparison with other strains. During the summer season, Pleurotus pulmonarius (Pleurotus sajor-caju) was grown on paddy straw. Mushroom cultivation not only provides additional income but is also a source of nutrient security in rural areas because of its high protein content, and in addition, it also possesses many nutraceutical properties.
2.3. Construction of the Jalkund water harvesting structure and farm ponds
To promote efficient water conservation and its multiple uses, several farm ponds were constructed and existing farm ponds were renovated in the adopted villages. Initiatives were also undertaken to popularize the low-cost rainwater harvesting structures known as Jalkunds (5 × 4 × 1.5 m), which were constructed using 250 GSM Silpaulin sheets, had storage capacities of 30,000 L, and were used to harvest rainwater in the IOFS fields of farmers. These structures were constructed to enable the farmers to harvest rainwater during the rainy season and subsequently use the water during dry periods to irrigate high-value winter crops. Farmers diversified their farming activities throughout the year and cultivated high-value crops, such as broccoli, tomato, and French bean. Climbing vegetables, such as pumpkin, bottle gourd, chow-chow, cucumber, and ridge gourd, were grown on a structure created above the water harvesting structure on one side of the pond dyke for vertical intensification. Additionally, the stored water was used in daily activities, such as cleaning and giving water to livestock; previously, the farmers had to fetch water from distant places. Some farmers also used the Jalkunds for rearing fish, which provided them with additional income.
2.4. Animal components
In addition to the improved technology of the housing system, the improved pig variety “Lumsniang” (with 25% genetic inheritance of Khasi local and 75% genetic inheritance of Hampshire) was also introduced to the village. These pigs attained a higher body weight at an early age, as well as a larger litter size at weaning, than the local non-descriptive pigs in the low input tribal production system. The deep litter housing system provides a better micro-environment during both summer and winter, with better physiological adaptation. Approximately 1,000–1,500 kg of well-decomposed manure/year was produced by replacing the bedding material in the pigsty. In Meghalaya, backyard poultry farming has emerged as an important alternative livelihood option for farm women, providing income and household nutritional security. Most of the backyard poultry production involves the rearing of indigenous birds with poor production performance. Dual-purpose backyard poultry birds (the Vanaraja and Gramapriya varieties) with high production potential were introduced into the IOFS models developed in the village. Feed for the poultry and pigs was the major constraint. Emphasis was placed on the production of maize grain for feed purposes and the cultivation of fodder (hybrid bajra napier, congo-signal, broom grass, etc.) and the multipurpose tree Colocasia for cattle and pigs.
2.5. Pisciculture
For composite fish culture, fingerlings consisting of catla (30%), grass carp (30%), and common carp (40%) were released according to the size of the pond and Jalkund. Lime (500 kg/ha) and well-decomposed FYM (10 t/ha) were applied after the pond was constructed to enhance soil fertility. Sun-dried cow dung was used to manure the pond (100 kg/ha per month in weekly splits).
2.6. Compost preparation
Vermicomposting units were constructed to recycle on-farm biomass to increase the fertility of the soil. Vermibeds are the latest unique technology for earthworm farming and are 12′ × 4′ × 2′ and each can produce ~500–1,000 kg of vermicompost in a year. They are very portable, low cost, easy to handle and install, and allow the collection of vermiwash. Vermibeds can be used on a small scale by farmers with household organic waste. Crop residues and agricultural waste were collected by the farmers and used to fill in the vermibeds for decomposition processes. Even bio-enriched compost or enriched compost was used in these areas as it increases nutrient availability and suppresses diseases. The Trichoderma-based formulation was used for preparing bio-enriched compost. Trichoderma formulation (1 kg) was mixed with 100 kg of well-decomposed FYM and kept for 10 days under the polythene cover. The mixture was turned every 3 days. For the management of bacterial wilt in chili and brinjal, Pseudomonas fluorescence-based formulations were used for soil drenching. Other seeds were also treated with Trichoderma-based formulations, which help to enhance germination, provide protection from damping off and other soil-borne diseases, and increase seedling vigor. The slurry method was used for seed treatment; 5 g of Trichoderma formulation was prepared in 10 ml of water and this slurry was sufficient for 1 kg of seed. The compost was dried in the shade after treatment.
2.7. Organic management of insect pests and diseases
Pest management for various crops in an IOFS involved proper sanitation, clean cultivation, and the manual collection and destruction of egg masses and larvae of lepidopteron pests at the initial stage of incidence. Therefore, insect pest infestation was avoided in very severe conditions on most of the crops. However, the infestation of fruit borers in tomatoes, cabbage butterflies and aphids in cole crops, lepidopteron borers in maize, and aphids in beans was as a major problem. For keeping the pest population below the economic damage level, neem oil 0.03% (5 ml/L) and Bacillus thuringinesis (2 g/L) were sprayed alternatively at 10-day intervals to manage lepidopteron pests in cabbage, tomato, maize, and other crops, whereas neem oil and Lecanicillium lecanii (5 g/L) were sprayed to manage aphids and other sucking pests. In addition, a mixture of vermiwash (1 L) and 10–15-day-old cow urine (1 L) in 10 L of water was used as a biopesticide and liquid manure spray on vegetable crops. Diseases were managed within the system using Trichoderma- and P. fluorescence-based formulations. Seed/rhizome treatment was carried out in many cases, e.g., for ginger and French bean. For ginger, rhizome treatment was performed by preparing a suspension of Trichoderma formulation (3 g/L), and 10 L of this suspension was used for treating 10 kg of seed rhizomes. Rhizomes were kept in the suspension for 45 min and then shade-dried for 24 h before sowing. During July and August, the incidence and severity of Pythium soft rot and bacterial wilt caused by Ralstonia solanacearum is high; therefore, the affected spots and nearby healthy clumps were soil drenched with Trichoderma and P. fluorescence-based formulations (4 g/L).
2.8. Nutrient budgeting
While compost pits were dug in Mrs. Skola Kurbah's field, vermicompost was prepared in Mr. Jrill Makroh's field. Product or waste generation from one enterprise was judiciously used as input for the others. The requirement of nutrients, such as nitrogen (N), phosphorous (P2O5), and potassium (K2O), for crops cultivated in the model was calculated, and nutrients recycled within the system through manure, compost, and vermicompost were determined. The economic and byproduct samples of IOFS models were collected and their total N concentrations were determined using the micro-Kjeldahl method (Bremner and Mulvaney, 1982), while total P and K concentrations were measured using a di-acid mixture (HNO3:HClO4 at a 3:1 ratio) (Tandon, 1995). Soil pH was measured in a 1:2.5 soil:water suspension (Jackson, 1973) and the SOC was estimated using the Walkley–Black method (Walkley and Black, 1934). While available soil N concentrations were measured using the alkaline potassium permanganate method (Subbiah and Asija, 1956), available P and K were measured using Bray's method (Bray and Kurtz, 1945) and the ammonium acetate method (Knudsen et al., 1982), respectively. While the farmyard manure (FYM) contained 0.73 ± 0.04% N, 0.24 ± 0.03% P2O5, and 0.98 ± 0.05% K2O, the vermicompost prepared in the model contained 1.74 ± 0.07% N, 0.69 ± 0.05% P2O5, and 1.03 ± 0.06% K2O. Nutrient balance was calculated by subtracting the amount of nutrients recycled from the nutrient requirement within the IOFS model (Das et al., 2019).
2.9. Statistical analysis
We undertook descriptive statistical analysis of the data in the IOFS models, and the year was considered as replication. Standard error of the mean is shown in Tables 2–6. Similarly, descriptive statistical analysis was also conducted for the figures and the vertical bars represent the standard error (SE), with p < 0.05 considered significant (Figure 5).
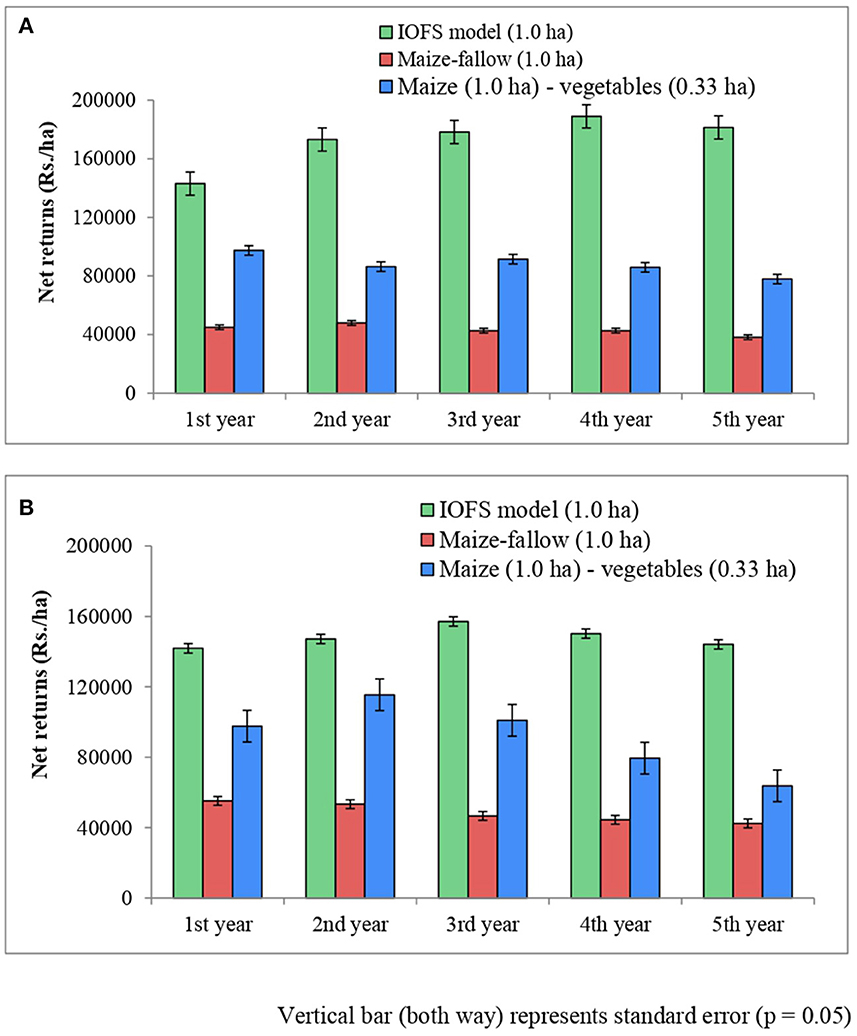
Figure 5. Net return/ha over 5 years from the IOFS models of Mr. Jrill Makroh (A) and Mrs. Skola Kurbah (B) compared with common farming practices.
3. Results
3.1. System productivity, profitability, and water harvesting in a participatory IOFS model
The IOFS models have achieved success by providing diversified food, year-round employment, and improving the income of farmers. As the farmers were traditionally growing crops for decades without using any synthetic fertilizer, yield levels increased significantly after the adoption of organic practices in a systematic manner. Maize-French bean and rice-pea cropping systems were found to be popular among the farming communities as they promote crop diversification and provided additional income. As the farmers were given training on improved crop production techniques, which including field visits to ICAR Research Complex farms, farmers become confident in applying organic farming methods in their field. The IOFS model promotes crop diversification, thereby providing food security and employment for the farmers all year round. The IOFS provides better means for year-round employment in these sections of rural mass through the use of different crops in a sequence mode, livestock management, mushroom rearing, compost preparation, and pisciculture. Maize, French bean, potato, ginger, tomato, carrot, and chili yields in the IOFS were increased by approximately 20–30%, 40–45%, 25–30%, 33–40%, 45–50%, 37–50%, and 27–30%, respectively, compared with conventional practice. Additionally, the average productivity of the fruit trees pineapple, Assam lemon, and guava increased in the organic system by 35–40%, 27–30%, and 30–35%, respectively, compared with the conventional system. A small shop was constructed near the highway so that the farmers could sell organic produce (vegetables, fruits, and spices) from the village/Institute at a relatively higher price. The organic certification (PGS mode) process for the farmers of the adopted villages was also initiated (provisional registration number given) so that they could demand the premium price for organic produce, thus increasing their income further. Emphasis was placed on producing seeds in the farm itself to reduce the dependency on external seeds and reduce the cost of production. Certified organic or chemically untreated seeds of some crops were also not available all the time. Successful IOFS models can generate 75–80% of its total requirement for seeds (rice, maize, ginger, turmeric, soybean, pea, lentil, French bean, pumpkin, bottle gourd, squash, leafy mustard, coriander, spinach, brinjal, chili, etc.) and 20–25% of seeds (tomato, cauliflower, cabbage, broccoli, carrot, beetroot, radish, etc.) are purchased from the market. Improved pig breeds (75% Hampshire and 25% local) and local breeds were integrated with improved husbandry practices. A deep litter model of pig housing was introduced to increase productivity and use resources more efficiently. The pig attained a higher body weight of 90–100 kg at 12 months of age and produced a larger litter size at weaning than local non-descriptive pigs in the low-input tribal production system. The deep litter housing system provides a better micro-environment during both summer and winter and better physiological adaptation. In this housing system, little or no liquid effluent is produced, and odor is greatly reduced. Approximately 1,000–1,500 kg of well-decomposed manure/year was produced by replacing the bedding material in the pigsty. The adult upgraded pigs were sold by the farmers for Rs. 10,000–12,000 per pig, whereas the local adult pigs were sold for Rs. 6,000–7,000 per pig. The breeding farmers harvested two or three extra piglets per farrowing, compared with the earlier system, and sold each piglet for Rs. 2,500–3,000. After 1 year of stocking 2,000 fingerlings in her pond of Mrs. Pretywon of Rynghang harvested 200 kg of fish, which she sold at Rs 180 per kilogram and generated an income of Rs. 36,000. Mr. Lamare, one of the beneficiaries, said that he was able to harvest ~7 kg of fish from his 20-m2 Jalkund. He sold them at Rs. 180 per kilogram and generated an income of Rs. 1,260. Additionally, he used the azolla generated from the Jalkund as fish feed and a source of manure for raising his crops. Nutrient recycling through vermicomposting using animal excreta, weed biomass, kitchen waste, and the leaves of peripheral trees, along with FYM application, fulfilled most of the nutritional requirement of all the crops in the organic farming system model and sustained the overall productivity of the farm. The farmers produced vermicompost in vermibeds and cement brick chambers and generated 0.4 to 1.25 tons per annum. The data from eight IOFS models from the villages are shown in Table 1. They integrated cereal crops (rice and maize), vegetables (tomato, French bean, potato, lettuce, and carrot), livestock (dairy, pigs, and poultry), and a water harvesting structure (Jalkund) into the system. The average yearly income of the farmers with IOFS models of 0.18–0.35 ha was recorded within the range of Rs. 18,750–46,695 per annum without any premium price. However, the farmers could get a 20% premium price for their organic produce, and in this instance, income was increased to a range of Rs. 22,500–56,034 (Table 1).
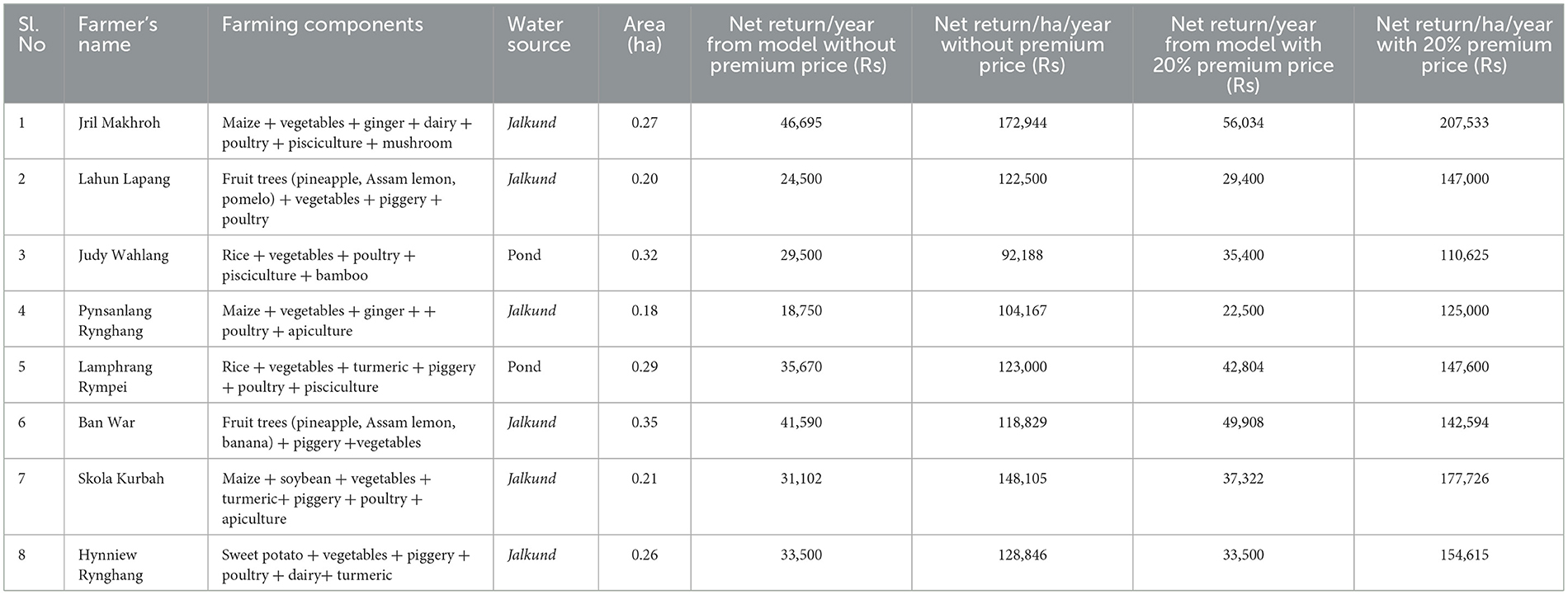
Table 1. Farmers who adopted the IOFS model in Meghalaya, India and their economic return (average of 5 years).
3.2. Case studies of livelihood assessments of two farmers
3.2.1. Livelihood assessments
Two progressive farmers, Mr. Jrill Makroh and Mrs. Skola Kurbah, were the pioneers of the IOFS model in the village and started their IOFS model in March 2014. The results/performance of the systems of both farmers was analyzed after 1 year and from 2015 onwards. The IOFS model in Mr. Jrill Makroh's field covered an area of 0.27 ha and consisted of cereals (maize), vegetables, spices (turmeric and chili), fruit crops (papaya and Assam lemon), dairy, a piggery, mushroom units, composting units, and a water harvesting unit (a Jalkund; Table 1). The largest area was covered by cereal (35%), followed by vegetables (31.5%) and spices (24.1%); the other components (animal, water source, composting, fodder, oilseeds, etc.) covered 9.4% of the total area (Figure 4A). Mrs. Skola Kurbah's field (0.21 ha), in addition to cereals (maize [24%]), vegetables (32%) and spices (16.8%), was used for growing oilseed in the form of soybean (16.8%), and 10.3% of the total area was used for growing fruit, composting, water sources, animals, and fodder (Figure 4B). The average cost of cultivation, gross return, and net return of five consecutive years (2014–2019) from the 0.27 ha area of Mr. Jrill Makroh's IOFS model were Rs. 71,670 ± 985, Rs. 118,365 ± 1,001, and Rs. 46,695 ± 418, respectively (Table 2). For Mrs. Skola Kurbah's 0.21-ha IOFS model, the average cost of cultivation, gross return, and net return of five consecutive years (2014–19) were Rs. 40,900 ± 973, Rs. 72,002 ± 1,159 and Rs. 31,102 ± 501, respectively (Table 3). On a 1-ha basis, the net return was assumed to be Rs. 172,944 per year (Rs. 474 per day) for Mr. Jrill Makroh and Rs. 148,105 per year (Rs. 406 per day) for Mrs. Skola Kurbah, which is a modest amount for a four- to five-member family. The two farmers, Mr. Jrill Makroh and Mrs. Skola Kurbah, could get a total net return per annum of Rs. 56,034 ± 502 and Rs. 37,322 ± 601 with a 20% premium price. The higher net return recorded in the former farmer's field compared with the latter was due to the maximum enterprises included and the greater IOFS area. The water source, composting, and fodder were the inputs for the other component in the IOFS model; therefore, a negative net return was recorded (Tables 1, 2). The net return (Rs./ha) over the years obtained from the IOFS models of the two farmers were significantly higher (p = 0.05) than the farmers' practice-I of maize-fallow or farmers' practice-II (cultivation of maize followed by the cultivation of vegetables in 30% of the areas; Figures 5A, B).
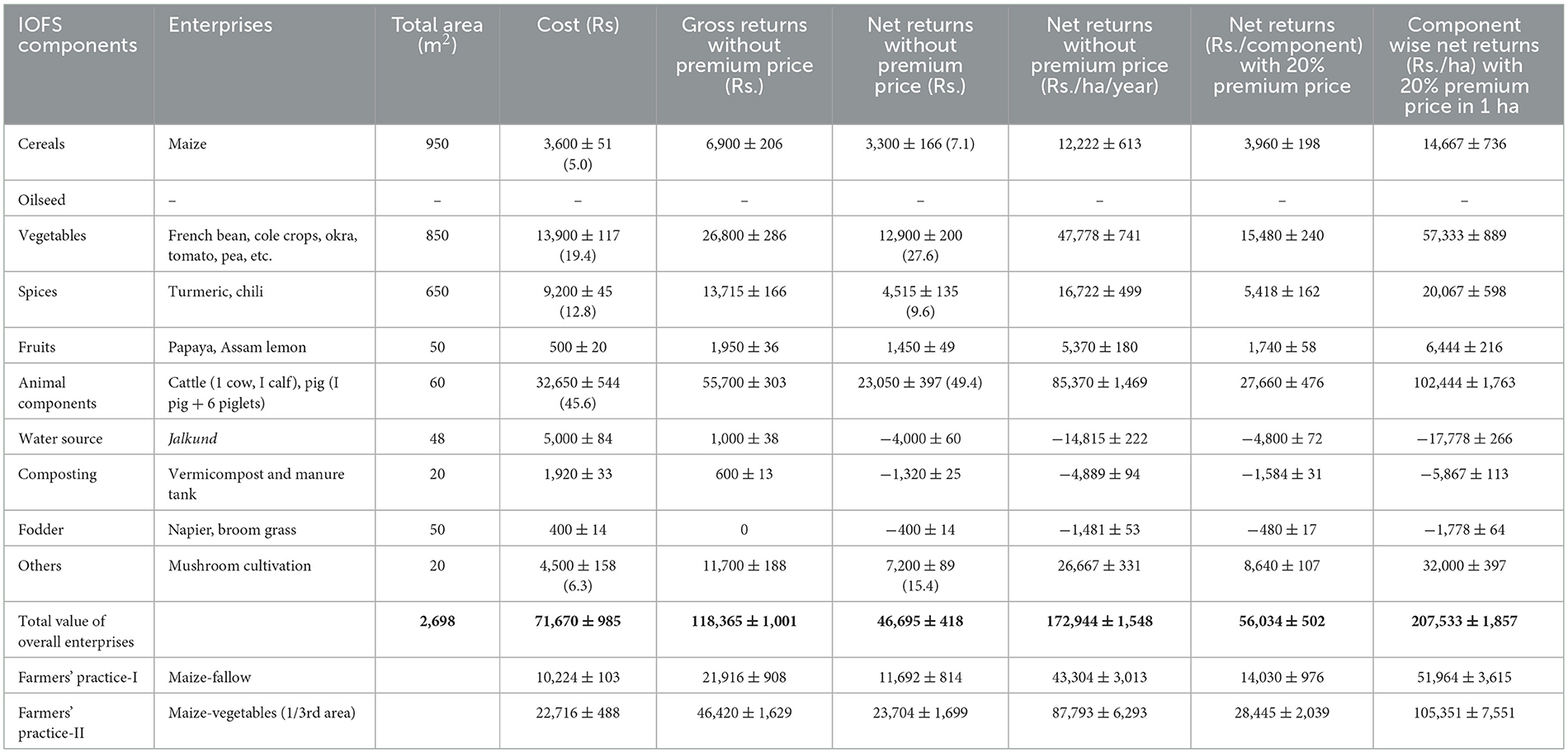
Table 3. Detailed analysis of the IOFS model developed at Mr. Jril Makroh's farm (average of 5 years).
The system productivity (SP) of the IOFS models of the two farmers was calculated based on rice equivalent yield (REY) in terms of kg/ha. The total SP of Mr. Jrill Makroh and Mrs. Skola Kurbah was 21,919 ± 185 kg/ha and 17,143 ± 276 kg/ha, respectively (Table 4). The average highest SP for 5 consecutive years was reported for animal components in both the farmer's IOFS models (Mr. Jrill Makroh as 10,315 ± 56 kg/ha and Mrs. Skola Kurbah as 7,405 ± 83 kg/ha; Table 4), which may be due to the higher pricing of animal products and meat and the high rate of livestock. The total average production efficiency for 5 consecutive years under the IOFS model was 60.1 ± 0.51 kg/ha/day for Mr. Jrill Makroh and 47.0 ± 0.76 kg/ha/day for Mrs. Skola Kurbah (Table 4). System productivity (kg/ha) and production efficiency (kg/ha/day) was significantly higher with IOFS models than with farmers' practice-I and farmers' practice-II (Table 4). This shows that the adoption of an IOFS model in farmer's fields can achieve a premium farm income, reduce poverty, and provide better food security for the farmers.
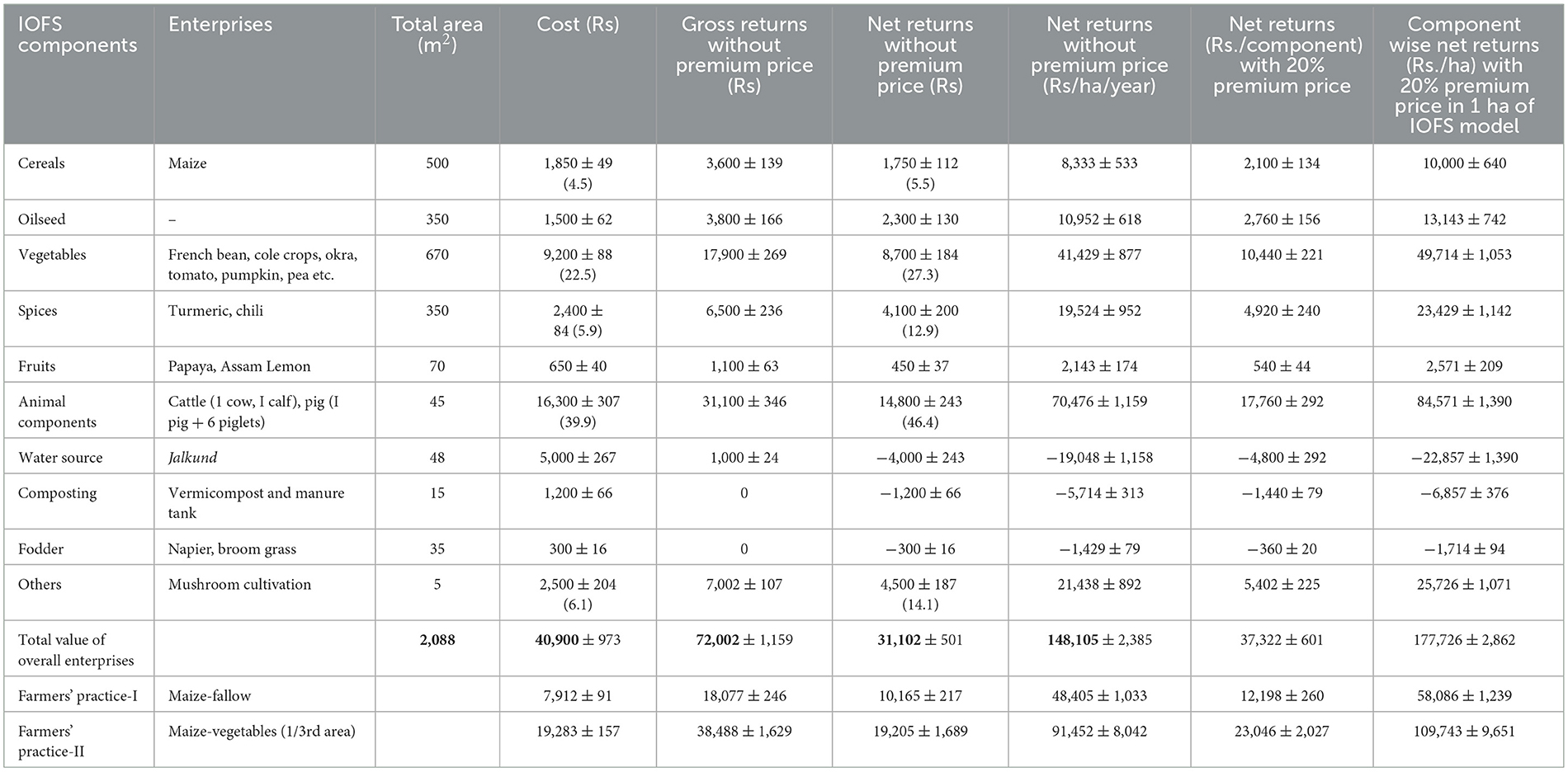
Table 4. Detailed analysis of the IOFS model developed at Mrs. Skola Kurbah's farm (average of 5 years).
3.2.2. Residue recycling and nutrient balance
The two IOFS models of Mr. Jrill Makroh and Mrs. Skola Kurbah had different on-farm nutrient supply balance sheets. The sheets were categorized under five modules (module I, module II, module III, module IV, and module V). Module I comprised cereals and oilseeds; module II comprised horticultural crops; module III comprised dairy; module IV comprised pigs and poultry; and module V comprised others (Tables 5, 6). All the modules of the two IOFS models generated on-farm nutrients, such as N, P2O5, and K2O, through the recycling of crop residues, livestock excreta, and leftovers except module III for Mrs. Skola Kurbah. The highest on-farm nutrients recycled was recorded under module V for both the IOFS models (23.1 ± 0.11 kg N, 7.4 ± 0.32 kg P2O5, and 20.0 ± 0.63 kg K2O for Mr. Jrill Makroh, and 22.9 ± 0.36 kg N, 6.9 ± 0.2 kg P2O5, and 20.1 ± 0.34 kg K2O for Mrs. Skola Kurbah). The average lowest N and P2O5 on-farm nutrients recycled for five consecutive years was recorded under module I and module IV for K2O for Mr. Jrill Makroh. However, the IOFS model of Mrs. Skola Kurbah had the lowest N under module I and P2O5 and K2O under module II. The above results show that module V has a higher potential for supplying macronutrients (N, P, and K) than other modules in the IOFS model and macronutrient content in the residues of module I and module II were less. The more on-farm nutrients are recycled, the less off-farm nutrients will be needed; therefore, modules I and II for both farmers had a negative nutrient balance. Modules III and IV for Mr. Jrill Makroh and module IV for Mrs. Skola Kurbah required no off-farm nutrients and they were the major source of on-farm nutrients. Furthermore, adopting the IOFS model resulted in only 1.8 ± 0.24 kg of N, 3.8 ± 0.21 kg of P2O5, and 0.9 ± 0.11 kg of K2O being needed from off-farm sources to achieve a nutrient balance for Mr. Jrill Makroh, i.e., the model could supply 95.1% of N, 82.0% of P2O5, and 96.0 % of the total K2O requirement of the model, and only 4.9% of the total N, 18% of P2O5, and 4.0% of K2O was needed from outside sources (Table 5). This means the IOFS model is highly sustainable. For Mrs. Skola Kurbah, the IOFS could generate 76.0 % of total N, 68.6% of P2O5, and 85.5% of K2O needed within the system (Table 6); 24% of N, 31.4% of P2O5, and 14.5% of the total K2O requirement was supplied from external sources, such as through the purchase of FYM.
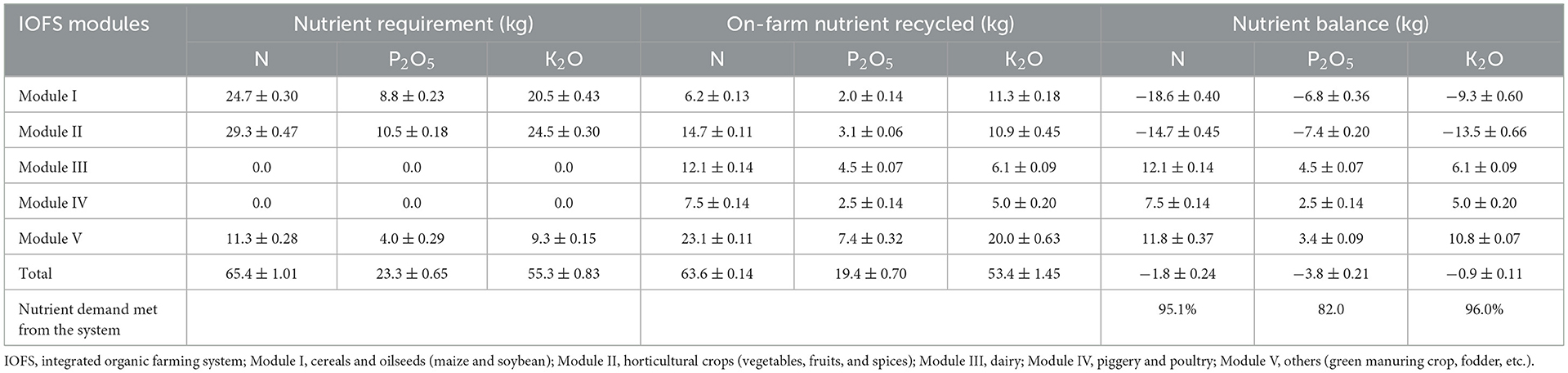
Table 5. On-farm nutrient supply balance sheet in the IOFS model of Mr. Jrill Makroh (after 5 years).
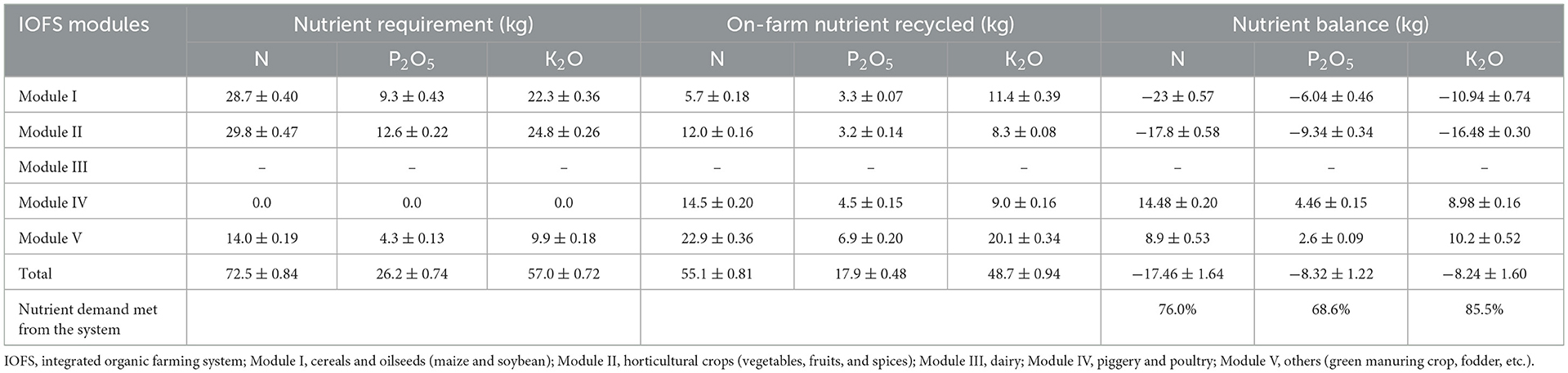
Table 6. On-farm nutrient supply balance sheet in the IOFS model of Mrs. Skola Kurbah (after 5 years).
4. Discussion
4.1. Impact of the IOFS on system productivity and profitability
The IOFS integrates the management of all the production systems to achieve a sustainable result economically and environmentally (Manhoudt et al., 2002). An increase in system productivity and net returns due to the scientific integration of farming system enterprises, such as livestock, cereals, pulses, and vegetables, with the in situ production of compost or vermicompost by efficient recycling of farm resources was recorded by many workers (Ansari et al., 2017, 2023; Das et al., 2019; Layek et al., 2020). The sustainable rice-based integrated farming system (IFS) in an irrigated agro-ecosystem reported that the cropping system of rice-pea-okra achieved a higher rice equivalent yield (REY) (17.88 t/ha), greater system productivity, and higher employment than the conventional rice-wheat cropping system (Ansari et al., 2013; Layek et al., 2017). A crop rotation system that includes a mixture of soil fertility building leguminous crops and cash crops, such as vegetables and spices, was the main mechanism for long-term nutrient supply and reducing the pest and disease load within organic systems. Leguminous crops also have the potential for biological nitrogen fixation (BNF), which helps in supplying nitrogen and improves the organic production system (Connor, 2021). The inclusion of legumes in cropping systems or farming systems as intercrops or in sequence to prevent monocropping is very much needed to improve system productivity and soil health (Ansari et al., 2017; Layek et al., 2018). The small and marginal farmers faced under-employment due to the seasonal nature of their crop production (Ramrao et al., 2005; Ansari et al., 2014). However, IOFSs comprising crops (cereal and horticultural) and livestock (poultry, piggery, dairy, apiculture, pisciculture, and rabbit) are more economic in terms of net returns than crop production only (Das et al., 2014; Ravisankar et al., 2021). This helps to ensure that the farmers' income is above the poverty line. The concept of the IOFS is to increase the income and employment of the marginal and small land holdings by integrating various farm components (livestock, pisciculture, crop production, apiculture, vermicomposting etc.) and residue management, in which the waste of one source is the input of the other, for sustainable agriculture (Soni et al., 2014; Meena et al., 2022). For example, cattle dung mixed with crop residues and farm waste can be converted into nutrient-rich vermicompost, thereby, there is less dependence on organic manure from external sources.
There is always a chance to reduce the production cost of individual enterprises, such as livestock rearing, crop production, and pisciculture, and subsequently the overall cost of a farming system (Layek et al., 2020; Das et al., 2021). Different resources generated within the farm viz., crop residues (rice straw, maize stalk, pulses biomass, etc.), weed biomass, vegetable waste, livestock dung and urine, and poultry/duck droppings can be efficiently recycled through composting or vermicomposting and subsequently can be used in an IOFS model (Das et al., 2019, 2021). The application of organic amendments, such as vermicompost, enhances the activity of soil microorganisms, thereby improving soil health long term, i.e., the physical, chemical, and biological properties of the soil (Pierre-Louis et al., 2021). The inclusion of animal components in the system has a positive link to sustainability by generating cash income, improving family nutrition, and recycling crop residues into feed. A judicious mixture of livestock enterprises, such as dairy, poultry, fish, goat-rearing, and vermicomposting, will help to generate additional income (Panwar et al., 2018). Before the initiation of the IOFS program, the pigs reared by farmers had a very high mortality rate due to diseases and poor management. The inclusion of livestock components (cattle, pig, poultry, duck, etc.) and high value vegetables (broccoli, cauliflower, tomato, brinjal, carrot, lettuce, etc.) has the potential to improve the net return of the IOFS system due to the prevailing high market demand for organic produce and price (Layek et al., 2020). The productivity of pigs and poultry was low due to low feed conversion efficiency. Farmers were also motivated by the performance of the dual-purpose improved poultry varieties as they thrived even when poorly fed and subjected to the low-intensive management practices followed by the farmers of the village. By integrating livestock units, such as cattle, pigs, or poultry/duck, with fish ponds, the input cost of fish feed, manure, fertilizers, etc., can be minimized (Layek et al., 2020; Das et al., 2021). Unlike conventional practices, organic farming practices meet the biological and ethological needs of livestock (Von Borell and Sorensen, 2004). Poultry in the organic farming system increases the renewable and local inputs for the other components (Castellini et al., 2006). According to Lepcha et al. (2018), the gross return per annum (Rs. 165,800) of backyard poultry from organic farming systems is significantly higher than that from conventional farming systems (Rs. 95,695). Among the fish species reared in ponds and Jalkunds, the performance of common carp was superior as it had a faster growth rate, high tolerance, was easy to handle, could be raised at a high density, and was associated with high production per square unit. Moreover, the Jalkund facilitated crop diversification (legumes, vegetables, spices, etc.) and increased net income to Rs. 43,074 per annum by increasing the yield (26.9%), with a B:C ratio of 2.1 (Lepcha et al., 2018). The rate of return from the farming system integrated with pisciculture, in which fish were reared organically by recycling the byproducts of dairy, poultry, and duckery, was Rs. 5.46/rupee invested (Behera et al., 2010). The performance of the IOFS components was 11.1%, 75%, and 16.8% better for dairy, poultry, and pigs, respectively, than that with conventional practices (Lepcha et al., 2018). The integration of poultry and livestock with a conventional farming system increases crop productivity, thereby making the enterprise more profitable and increasing farmers' income (Ali and Shivalingaiah, 2022). Apart from the increased income, farmers consume a variety of nutritional vegetables and fruits, milk, and eggs throughout the year, which leads to nutritional security.
4.2. The impact of water harvesting and the use of water in an IOFS
The availability of fresh water per person in the Himalayan area was estimated as 1,473 m3 year−1, 1,757 m3 year−1, and 18,417 m3 year−1 for the Ganges, Indus, and Brahmaputra basins, respectively, while for India as a whole, the average fresh water per capita is 2,214 m3 year−1 (Das et al., 2018). The per capita availability of water is decreasing day by day. Although the per hectare and per capita fresh water availability in the NER is the highest in India, < 5% of the available water is being tapped for use. The success of a farming practice is dependent on the availability of irrigation water, which can be harvested in a farm pond or Jalkunds. These structures play a significant role in crop and vegetable production, providing drinking water for livestock, and kitchen gardening, thus increasing crop productivity, farmers' income, and employment (Chowdhury et al., 2020). Moreover, the harvested water can be used for duckery and pisciculture practices (Patel L. C. et al., 2014). The IOFS model has been shown to be climatically resilient, particularly in sustaining crops and livestock during the lean period through the development of a water harvesting unit (Jalkund), which operates by storing the excess water during the rainy season and then supplying the stored water during the dry season. The improved integrated farming system, including the integration of different farm enterprises in tribal population regions, can potentially be more productive, achieve greater net returns, improve nutritional value, and increase employment more than a conventional farming system in Manipur (Ansari et al., 2013). In monocropping systems, employment opportunities for farmers and laborers are limited and seasonal. In an IOFS system, labor is needed for year-round diversified farm activities (crop cultivation, livestock rearing, etc.), which has a high potential for increasing employment. One such IFS model has increased employment by 434 man days in a year for 1.0 ha over the traditional monocropping system on hills in the eastern Himalayas (Das et al., 2021). The IOFS model enables the farmer to generate income and produce from various components at various seasons of the year. This system reduces the dependence on one specific component and minimizes the overall loss of the system in case one component fails to perform (Panwar et al., 2018).
4.3. Residue recycling and nutrient balance in an IOFS
Year-round feed, fodder, labor, manure, and water are needed for a successful and sustainable IFS/IOFS model in any particular region (Das et al., 2021). Crop residue management, such as soil surface retention, has a positive impact on soil health (Mishra and Nayak, 2004; Turmel et al., 2015; Ansari et al., 2022a). The regular addition of residue as organic input maintains the soil organic matter (SOM) level for better soil health (FAO, 2011). The quantity and quality of crop residues generated within a farm and their efficient utilization influence soil fertility build-up over time and its subsequent release of nutrients to the crops that follow (Jarvis et al., 1996; Panwar et al., 2021a; Ansari et al., 2022c). The main strengths of an IOFS lie in better resource recycling as an organic farm mainly relies on internal resources and restricts or limits the input of external materials (Nemecek et al., 2011; Panwar et al., 2020). In an organic farming system, the application of crop residue based-vermicompost to the soil is biologically better than the direct application of manure or crop residue (Aynehband et al., 2017; Mukherjee et al., 2023). The integration of poultry or ducks, by virtue of creating artificial structures over farm ponds or in pond dikes, and the cultivation of year-round high value vegetables using water from Jalkunds or ponds can increase system productivity by up to 750% and income by 850% (Babu et al., 2019). The transfer of vermiculture technology was highly successful and widely adopted by the farmers of the village. The farmers were happy due to the growing demand for worms from other groups and they were convinced of the superiority of the farm produce due to the use of compost in their own fields. Soil fertility is degraded with the continuous use of synthetic agro-inputs, which impacts sustainable agriculture. An increase in soil organic matter content and soil microbial activity are indicators of crop and livestock productivity (Biswas et al., 2014). The adoption of IOFS also enhances soil fertility by maintaining biodiversity (Mader et al., 2002; Panwar et al., 2021b). An IOFS model includes all types of crop production and soil management systems without disturbing environmental factors and only uses organic inputs. The major fundamental differences between the management of conventional and integrated organic systems are that while conventional agriculture mostly relies on short-term solutions, such as the application of a readily available nutrient, e.g., synthetic fertilizer, IOFS mostly relies on long-term solutions at the systems level, e.g., nutrient cycling and conservation. Meta-analysis results showed that the organic farming system has a higher SOM content with a minimal loss of soil nutrients and increased soil organic and inorganic carbon sequestration (Foereid and Hogh-Jensen, 2004; Tuomisto et al., 2012). One important principle of an integrated farming system is to reduce the dependence on external inputs, especially nutrients, to sustain the model in the long run (emphasis was placed on establishing the IOFS model in the villages by increasing residue recycling and the preparation of quality compost in a compost pit or through vermicomposting) (Das et al., 2019). Livestock components, such as dairy and pigs, generate enough animal manure, and the efficient recycling of crop and weed biomass helps to generate sufficient nutrients within the system. Organic inputs in the form of FYM, compost, and vermicompost have the potential to increase the macronutrient (N, P, and K) content of the soil and act as a store house of various soil nutrients and as a soil conditioner, unlike the inorganic fertilizers, which only supply major nutrients (Mishra and Nayak, 2004). Through the efficient recycling of farm and kitchen wastes and vermicomposting, nutrient requirements can be reduced substantially in the near future. There is enough scope to increase the nutrient supply from the model by intercropping with a legume, using biofertilizers, efficiently collecting poultry manure, and adopting vermicomposting.
4.4. Integration of an IOFS model
The integration of different enterprises within the IOFS and efficient recycling of the resources may be the causes for the increase in productivity and income (Layek et al., 2019). It can be assumed that with the certification of organic products, farmers' income from the IOFS models will be increased further. Organic certification is recommended for a strict closed cycle restricting external farm inputs and achieving a standard farm production system (Reganold and Wachter, 2016). The models took some time to operate at their full potential, and once the model was established, gross and net returns increased, particularly from the second or third year (Das et al., 2019). Moreover, the introduction of a premium price for certified organic produce can increase the profitability of organic produce, i.e., 22 to 35% more profitable than the current price and a significant improvement in the B:C ratio of 20 to 24% compared with conventional farming (Crowder and Reganold, 2015). Even though crop productivity in the organic farming system was reduced by 9.2%, the farmers' net profit increased by 22.0% due to the 20–40% higher premium price for the certified organic produce (Ramesh et al., 2010).
As there is a need to employ labor on a daily basis to maintain the livestock and a need to supply costly animal feed, such as rice bran and oil cake the variable cost increased (Panwar et al., 2018). The introduction of dairy components significantly increased the gross and net income of the farmers by providing milk, with less dependence on outside feed and fodder (Panwar et al., 2018). High quality dairy production depends on feedstuff or fodder of high nutritive value, with high protein content and roughage, and that can be easily processed (Rahmann and Bohm, 2005). The cultivation of fodder in the model supplies a good amount of forage to support the cattle, especially during the lean period between November and March.
5. Constraints on the adoption of the IOFS model
Major constraints on the adoption of the IOFS models by resource-poor farmers in large areas include: (i) limited availability of quality organic pesticides; (ii) high cost of seeds of improved varieties of vegetables, such as cabbage, tomato, and cauliflower; (iii) unavailability of quality manure in sufficient quantities; and (iv) high feed cost for pigs and poultry. As the marketing for organic produce and demand in the state is still not high, farmers are not being paid premium prices for their produce. However, with the efficient management of different enterprises within the system, such as the cultivation of fodder and multi-purpose trees, efficient recycling of resources for quality manure production, and organic certification to obtain a premium price, IOFS technologies may become very popular among farming communities.
6. Conclusions
The efficient use of byproducts or waste product from one enterprise as the input of others is the major principle underpinning a farming system that significantly reduces the demand for external inputs. This study successfully demonstrated that organic agriculture in farmers' fields significantly increases the average productivity of different agricultural and horticultural crops and livestock compared with the conventional system used previously. The adoption of Jalkund- or pond-based IOFS with demand-based location-specific scientific integration of agricultural and horticultural crops, cattle, pigs, poultry, fish, etc., increased system productivity, production efficiency, and the net returns of farmers compared with traditional practices, such as rice monocropping or rice followed by a vegetable. On a 1-ha area basis, the net returns from the IOFS models were Rs. 172,944 per year for Mr. Jrill Makroh and Rs. 148,105 per year for Mrs. Skola Kurbah, which were significantly higher than those of their fellow farmers who only practiced crop-based farming. Efficient recycling of available farm resources by small and marginal farmers in the IOFS models through vermicomposting/composting with animal excreta, weed biomass, tree leaves, kitchen wastes, etc., fulfilled most of the nutritional requirement (76.0 to 95.1% of N, 68.6 to 82% of P, and 85.5 to 96.0% of K) and sustained the overall productivity of the farm. Extensive efforts should be made to transfer this IOFS technology to larger farm communities practicing organic agriculture to fulfill the demand for organic inputs within the farm and improve the livelihood of poor rural households.
Data availability statement
The original contributions presented in the study are included in the article/supplementary material, further inquiries can be directed to the corresponding authors.
Ethics statement
Written informed consent was obtained from the participant/patient(s) for the publication of this article.
Author contributions
JL: conceptualization, methodology, investigation, monitoring, data curation, and writing of the original and final draft. AD: monitoring, data curation, and review and editing. MAA: data analysis, writing of the original and final draft, and review and editing. VM, NR, and AP: review and editing and project administration. KR, SP, PB, TR, SH, SD, MHA, and BP: review and editing. All authors contributed to the article and approved the submitted version.
Acknowledgments
We are thankful to the ICAR-Indian Institute of Farming Systems Research, Modipuram, and the ICAR Research Complex for NEH Region, Umiam, Meghalaya (TSP component) for providing technical and financial support to conduct the study in farmers' fields of Meghalaya. We are also thankful to the Director of ICAR-IIFSR for financial assistance for publishing the research outcome under the AI-NPOF project. We are also thankful to the farmers and villagers, who has given their consent to conduct the participatory experiment in their field and use their data, images and other information for publication.
Conflict of interest
The authors declare that the research was conducted in the absence of any commercial or financial relationships that could be construed as a potential conflict of interest.
Publisher's note
All claims expressed in this article are solely those of the authors and do not necessarily represent those of their affiliated organizations, or those of the publisher, the editors and the reviewers. Any product that may be evaluated in this article, or claim that may be made by its manufacturer, is not guaranteed or endorsed by the publisher.
References
Ali, H., and Shivalingaiah, Y. N. (2022). Comparative analysis of farmers practicing different farming systems in lower Subansiri District of Arunachal Pradesh. Pharma Innov. J. 11, 535–538. Available online at: https://www.thepharmajournal.com/special-issue?year=2022&vol=11&issue=4S&ArticleId=11892
Ansari, M. A., Babu, S., Choudhary, J., Ravisankar, N., and Panwar, A. S. (2022a). Soil quality restoration and yield stabilization in acidic soils of northeastern Himalayas: five years impact of green manuring and crop residue management. Front. Environ. Sci. 10, 940349. doi: 10.3389/fenvs.2022.940349
Ansari, M. A., Choudhury, B. U., Layek, J., Das, A., Lal, R., and Mishra, V. K. (2022c). Green manuring and crop residue management: effect on soil organic carbon stock, aggregation, and system productivity in the foothills of Eastern Himalaya (India). Soil Tillage Res. 218, 105318. doi: 10.1016/j.still.2022.105318
Ansari, M. A., Choudhury, B. U., Mandal, S., Jat, S. L., and Meitei, C. B. (2022b). Converting primary forests to cultivated lands: long-term effects on the vertical distribution of soil carbon and biological activity in the foothills of Eastern Himalaya. J. Environ. Manage. 301, 113886. doi: 10.1016/j.jenvman.2021.113886
Ansari, M. A., Prakash, N., Baishya, L. K., Punitha, P., Sharma, P. K., Yadav, J., et al. (2014). Integrated farming system: an ideal approach for developing more economically and environmentally sustainable farming systems for the Eastern Himalayan Region. Indian J. Agric. Sci. 84, 356–362. Available online at: https://epubs.icar.org.in/index.php/IJAgS/article/view/38572
Ansari, M. A., Prakash, N., Baishya, L. K., Punitha, P., Yadav, J. S., Sharma, P. K., et al. (2013). Comparative study on conventional and improved integrated farming systems for sustainable production, income generation and employment opportunity among the tribal farmers in hilly Regions of Manipur. Indian J. Agric. Sci. 83, 765–72. Available online at: https://epubs.icar.org.in/index.php/IJAgS/article/view/31174
Ansari, M. A., Ravisankar, N., Ansari, M. H., Babu, S., Layek, J., Panwar, A. S., et al. (2023). Integrating conservation agriculture with intensive crop diversification in the maize-based organic system: impact on sustaining food and nutritional security. Front. Nutr. 10, 1137247. doi: 10.3389/fnut.2023.1137247
Ansari, M. A., Saha, S., Das, A., Lal, R., Das, B., Choudhury, B. U., et al. (2021). Energy and carbon budgeting of traditional land use change with groundnut based cropping system for environmental quality, resilient soil health and farmers income in eastern Indian Himalayas. J. Environ. Manage. 293, 112892. doi: 10.1016/j.jenvman.2021.112892
Ansari, M. A., Saraswat, P. K., Roy, S. S., Sharma, S. K., Punitha, P., Ansari, M. H., et al. (2017). Significance and strategies of legume production for achieving nutritional security in North East Indian Himalayan Region. J. Food Legum. 30, 56–63.
Aynehband, A., Gorooei, A., and Moezzi, A. A. (2017). Vermicompost: an eco-friendly technology for crop residue management in organic agriculture. Energy Procedia 141, 667–671. doi: 10.1016/j.egypro.2017.11.090
Babu, S., Das, A., Mohapatra, K. P., Yadav, G. S., Singh, R., Tahashildar, M., et al. (2019). Pond dyke utilization: an innovative means for enhancing productivity and income under integrated farming system in north East Hill region of India. Indian J. Agric. Sci. 89, 117–122. doi: 10.56093/ijas.v89i1.86190
Behera, U. K., Rautaray, S. K., and Sahoo, P. K. (2010). Farming system-based composite pisciculture in harvested pond water under eastern Indian situations. J. Soil Water Conserv. 9, 143–147. Available online at: http://www.indiaenvironmentportal.org.in/content/322053
Biswas, S., Ali, M. N., Goswami, R., and Chakraborty, S. (2014). Soil health sustainability and organic farming: a review. J Food Agri. Environ. 12, 237–243. Available online at: https://www.researchgate.net/publication/267038963
Bray, R. H., and Kurtz, L. T. (1945). Determination of total, organic, and available forms of phosphorus in soils. Soil Sci. 59, 39–46. doi: 10.1097/00010694-194501000-00006
Bremner, J. M., and Mulvaney, C. S. (1982). “Nitrogen-total,” in Methods of Soil Analysis. Part 2, eds A. L. Page, R. H. Miller, D. R. Keeney, 2nd ed. Agron. Monogr. 9. (Madison, WI: ASA and SSSA), 595–624. doi: 10.2134/agronmonogr9.2.2ed.c31
Bujarbaruah, K. M. (2004). Organic Farming: Opportunities and Challenges in North Eastern Region. Umiam, Meghalaya: ICAR Research Complex for NEH Region.
Castellini, C., Bastianoni, S., Granai, C., Dal, B. A., and Brunetti, M. (2006). Sustainability of poultry production using the energy approach: comparison of conventional and organic rearing systems. Agric. Ecosyst. Environ. 114, 343–350. doi: 10.1016/j.agee.2005.11.014
Choudhury, B. U., Nengzouzam, G., Ansari, M. A., and Islam, A. (2022). Causes and consequences of soil erosion in northeastern Himalaya, India. Curr. Sci. 122, 772–789. doi: 10.18520/cs/v122/i7/772-789
Chowdhury, P., Kumar, R. S., Kumar, M., Meena, K. L., and Rajkhowa, D. J. (2020). Livelihood Improvement through Artificial Rainwater Harvesting Pond Based Farming Systems-Success Stories in Nagaland, India. Available online at: https://www.researchgate.net/publication/341804347
Connor, D. J. (2021). What is the real productivity of organic farming systems? Outlook Agric. 50, 125–129. doi: 10.1177/00307270211017151
Crowder, D. W., and Reganold, J. P. (2015). Financial competitiveness of organic agriculture on a global scale. Proc. Natl Acad. Sci. USA 112, 7611–7616. doi: 10.1073/pnas.1423674112
Das, A., Datta, D., Samajdar, T., Idapuganti, R. G., Islam, M., Choudhury, B. U., et al. (2021). Livelihood security of small holder farmers in eastern Himalayas, India: pond based integrated farming system a sustainable approach. Curr. Res. Environ. Sustain. 3, 100076. doi: 10.1016/j.crsust.2021.100076
Das, A., Layek, J., Ramkrushna, G. I., Babu, S., Dey, U., Suting, D., et al. (2019). Integrated organic farming system: an innovative approach for enhancing productivity and income of farmers in north eastern hill region of India. Indian J. Agric. Sci. 89, 1267–1272. doi: 10.56093/ijas.v89i8.92843
Das, A., Layek, J., Yadav, G. S., Babu, S., Sarkar, D., Meena, R. S., et al. (2018). “Managing nitrogen in small landholder hill farms of North Eastern Indian Himalayas,” in Soil Nitrogen Uses and Environmental Impacts, edsR. Lal, and B. A. Stewart (Boca Raton, FL: CRC Press), 257. doi: 10.1201/b22044-10
Das, A., Patel, D. P., Kumar, M., Ramkrushna, G. I., Mukherjee, A., Layek, J., et al. (2017a). Impact of seven years of organic farming on soil and produce quality and crop yields in eastern Himalayas, India. Agric. Ecosyst. Environ. 236, 142–153. doi: 10.1016/j.agee.2016.09.007
Das, A., Patel, D. P., Kumar, M., Ramkrushna, G. I., Ngachan, S. V., Layek, J., et al. (2014). Influence of cropping systems and organic amendments on productivity and soil health at mid altitude of North East India. Indian J. Agric. Sci. 84, 1525–30. Available online at: https://epubs.icar.org.in/index.php/IJAgS/article/view/45300
Das, A., Singh, R. K., Ramkrushna, G. I., Layek, J., Tripathi, A. K., Ngachan, S. V., et al. (2017b). Roof water harvesting in hills–innovations for farm diversification and livelihood improvement. Curr. Sci. 113, 292–298. doi: 10.18520/cs/v113/i02/292-298
Deka, B. C., Thirugnanavel, A., Patel, R. K., Nath, A., and Deshmukh, N. (2012). Horticultural diversity in Northeast India and its improvement for value addition. Indian J. Genet. Plant Breed. 72, 157. Available online at: https://www.researchgate.net/publication/283044354
Deka, M. K., Bhuyan, M., and Hazarika, L. K. (2006). Traditional pest management practices of Assam. Indian J. Tradit. Knowl. 5, 75–78. Available online at: https://www.researchgate.net/publication/255988483
Devi, P. I., Thomas, J., and Raju, R. K. (2017). Pesticide consumption in India: a spatiotemporal analysis §. Agric. Econ. Res. Rev. 30, 163–172. doi: 10.5958/0974-0279.2017.00015.5
Foereid, B., and Hogh-Jensen, H. (2004). Carbon sequestration potential of organic agriculture in northern Europe – a modelling approach. Nutr. Cycling Agroecosyst. 68, 13–24. doi: 10.1023/B:FRES.0000012231.89516.80
Jarvis, S. C., Stockdale, E. A., Shepherd, M. A., and Powlson, D. S. (1996). Nitrogen mineralization in temperate agricultural soils: processes and measurement. Adv. Agron. 57, 187–235. doi: 10.1016/S0065-2113(08)60925-6
Knudsen, D., Peterson, G. A., and Pratt, P. F. (1982). “Lithium, sodium, and potassium.” in Methods of Soil Analysis, Part 2: Chemical and Microbiological Properties, eds A. L. Page, R. H. Miller, and D. R. Keeney (Madison, WI: American Society of Agronomy).
Lairenjam, C., Singh, R. K., Huidrom, S., and Nongthombam, J. (2014). Economic analysis of integrated farming system using jalkund and micro-irrigation system to harvest rainwater in north east india. Int. J. Agric. Ext. 2, 219–226. Available online at: https://www.researchgate.net/publication/272238413
Layek, J., Das, A., Dey, U., Krishnappa, R., Marak, M. R., Babu, S., et al. (2019). “Integrated organic farming system (IOFS) for livelihood security and doubling of farmers' income in hill agriculture,” in Souvenir of Krishi Unnati Mela on “Rural livelihood improvement options for the tribal farmers in Mizoram” at ICAR RC Mizoram Centre, Kolasib, Mizoram on 28-19 January 2019, 29–31.
Layek, J., Das, A., Ghosh, P. K., Rangappa, K., Lal, R., Idapuganti, R. G., et al. (2022). Double no-till and rice straw retention in terraced sloping lands improves water content, soil health and productivity of lentil in Himalayan foothills. Soil Tillage Res. 221, 105381. doi: 10.1016/j.still.2022.105381
Layek, J., Das, A., Mitran, T., Nath, C., Meena, R. S., Yadav, G. S., et al. (2018). “Cereal+ legume intercropping: an option for improving productivity and sustaining soil health,” in Legumes for Soil Health and Sustainable Management, eds R. S. Meena, A. Das, and G. S. Yadav (New York, NY: Springer), 347–386. doi: 10.1007/978-981-13-0253-4_11
Layek, J., Das, A., Ramkrushna, G. I., Hazarika, S., Krishnappa, R., Dey, U. Kandpal BK., et al. (2020). “Integrated organic farming system (IOFS): a success story of technology demonstration in cluster approach,” in ICAR Research Complex for North Eastern Hill (NEH) Region, Umiam-793 103 (Meghalaya, India), 106.
Layek, J., Das, A., Ramkrushna, G. I., Sarkar, D., Ghosh, A., Zodape, S. T., et al. (2017). Seaweed extract as organic bio-stimulant improves productivity and quality of rice in eastern Himalayas. J. Appl. Phycol. 30, 547–558. doi: 10.1007/s10811-017-1225-0
Layek, J., Dutta, S. K., Krishnappa, R., Das, A., Ghosh, A., Mishra, V. K., et al. (2023). Productivity, quality and profitability enhancement of French bean, okra and tomato with seaweed extract application under North-Eastern Himalayan condition. Sci. Hortic. 309, 111626. doi: 10.1016/j.scienta.2022.111626
Lepcha, B., Avasthe, R., Singh, R., Phukan, P., and Singh, N. J. (2018). Enhancing livelihood of tribal farmers of sikkim through integrated organic farming system: A case study. Indian Res. J. Extens. Educ. 18, 84–87. Available online at: http://www.kiran.nic.in/pdf/whats%20new/2017/IOFS-Casestudy.pdf
Mader, P., Fliessbach, A., Dubois, D., Gunst, L., Fried, P., Niggli, U., et al. (2002). Soil fertility and biodiversity in organic farming. Science 296, 1694–1697. doi: 10.1126/science.1071148
Manhoudt, A. G. E., Van De Van, G. W. J., De Haes, H. A. U., and Snoo, G. R. (2002). Environmental labelling in the Netherands: a framework for integrated farming. J. Environ. Manage. 65, 269–283. doi: 10.1006/jema.2002.0548
Meena, A. L., Pandey, R. N., Kumar, D., Dutta, D., Sharma, V. K., Karwal, M., et al. (2022). The long-term (13 years) effect of rice based organic farming on soil sulphur dynamics in a typic ustochrept soil of indo Gangetic plain of India. J. Soil. Sci. Plant Nutr. 23, 651–667. doi: 10.1007/s42729-022-01072-8
Mishra, B. B., and Nayak, K. C. (2004). Organic farming for sustainable agriculture. Orissa Rev. 10, 42–45. Available online at: https://www.academia.edu/3176400
Mukherjee, S., Basak, A., Chakraborty, A., Goswami, R., Ray, K., Ali, M. N., et al. (2023). Revisiting the oldest manure of India, Kunapajala: Assessment of its animal waste recycling potential as a source of plant biostimulant. Front. Sustain. Food Syst. 6, 1073010. doi: 10.3389/fsufs.2022.1073010
Nemecek, T., Dubois, D., Huguenin-Elie, O., and Gaillard, G. (2011). Life cycle assessment of Swiss farming systems: I. Integrated and organic farming. Agric. Syst. 104, 217–232. doi: 10.1016/j.agsy.2010.10.002
Pandey, G. (2002). Popularizing Under Exploited Fruits for Consumptions, India. Indian Horticulture, Oct–Dec., 18–21. Available online at: https://www.researchgate.net/publication/265468886
Panwar, A. S., Ansari, M. A., Ravisankar, N., Babu, S., Prusty, A. K., Ghasal, P. C., et al. (2022). Effect of organic farming on the restoration of soil quality, ecosystem services, and productivity in rice-wheat agro-ecosystems. Front. Environ. Sci. 10, 972394. doi: 10.3389/fenvs.2022.97239
Panwar, A. S., Prusty, A. K., Shamim, M., Ravisankar, N., Ansari, M. A., Singh, R., et al. (2021a). Nutrient recycling in integrated farming system for climate resilience and sustainable income. Indian J. Fertilizers 17, 1126–1137. Available online at: https://www.researchgate.net/publication/356355396
Panwar, A. S., Ravishankar, M., Samim, S., Bhaskar, B. P., Bhatt, R. S., Prusty, A. K., et al. (2020). Organic Farming Packages for Major Cropping System. New Delhi: Indian Council of Agriculture Research.
Panwar, A. S., Shamim, M., Ravisankar, N., Ansari, M. A., Singh, R., Prusty, A. K., et al. (2021b). Influence of long term fertilization with organic sources on crop productivity and soil quality in rice-wheat system under arid and sub humid conditions. Indian J. Fertilizers 16, 544–554. Available online at: https://www.researchgate.net/publication/285383656
Panwar, A. S., Syiemlieh, H., Kalita, L., Tahashildar, M., and Layek, J. (2018). “Food and nutritional security through farming system approach,” in Conservation Agriculture for Advancing Food Security in Changing Climate, Volume I (Crop Production, Farming System and Soil Health), eds A. Das, K. P. Mohapatra, S. V. Ngachan, A. S. Panwar, D. J. Rajkhowa, G. I. Ramkrushna et al. (New Delhi: Today and Tomorrow's Printers and Publishers), 223–244.
Patel, D. P., Das, A., Kumar, M., Ngachan, S. V., Ramkrushna, G. I., Layek, J., et al. (2014). Continuous application of organic amendments enhance soil health, produce quality and system productivity of vegetable based cropping systems at subtropical eastern Himalayas. Exp. Agric. 51, 85–106. doi: 10.1017/S0014479714000167
Patel, L. C., Nath, D., Islam, N., Biswas, S., Shil, S., De, D., et al. (2014). Dissemination of outcome of climate resilient agricultural technologies in a tribal village of Tripura. Int. J. Farm Sci. 4, 272–278. Available online at: https://www.researchgate.net/publication/342872142
Pierre-Louis, R. C., Kader, M. A., Desai, N. M., and John, E. H. (2021). Potentiality of vermicomposting in the South Pacific Island countries: a review. Agriculture 11, 876. doi: 10.3390/agriculture11090876
Pleguezuelo, C. R. R., Zuazo, V. H. D., Martinez, J. R. F., Peinado, F. J. M., Martín, F. M., Tejero, I. F. G., et al. (2018). Organic olive farming in Andalusia, Spain. A review. Agron. Sustain. Dev. 38, 20. doi: 10.1007/s13593-018-0498-2
Rahmann, G., and Bohm, H. (2005). “Organic fodder production in intensive organic livestock production in Europe: recent scientific findings and the impact on the development of organic farming,” in Integrating Livestock-Crop Systems to Meet the Challenges of Globalisation, eds P. Rowlinson, G. Wachirapakorn, P. Pakdee, and M. Wanapat, Vol. 1 (Thailand: Poultry Industry), 471–485.
Ramesh, P., Panwar, N. R., Singh, A. B., Ramana, S., Yadav, S. K., Shrivastava, R., et al. (2010). Status of organic farming in India. Curr. Sci. 1190–1194. Available online at: https://www.currentscience.ac.in/Volumes/98/09/1190.pdf
Ramrao, W. Y., Tiwari, S. P., and Singh, P. (2005). Crop-livestock integrated farming system for augmenting socio-economic status of smallholder tribal farmers of Chhattisgarh in Central India. Livest. Res. Rural. Dev. 17, 8. Available online at: https://www.lrrd.cipav.org.co/lrrd17/8/ramr17090.htm
Ravisankar, N., Ansari, M. A., Panwar, A. S., Aulakh, C. S., Sharma, S. K., Suganthy, M., et al. (2021). Organic farming research in India: potential technologies and way forward. Indian J. Agron. 66 (5th IAC Special issue): S142–S162. Available online at: https://www.researchgate.net/publication/356000991
Ravisankar, N., Ansari, M. A., Shamim, M., Prusty, A. K., Singh, R., Panwar, A. S. Bal., et al. (2022). Sustainable livelihood security of small farmers improved through resilient farming systems in the Semi-Arid Region of India. Land Degrad. Dev. 33, 2830–2842. doi: 10.1002/ldr.4358
Reganold, J. P., and Wachter, J. M. (2016). Organic agriculture in the twenty-first century. Nat. Plants 2, 1–8. doi: 10.1038/nplants.2015.221
Roy, S. S., Ansari, M. A., Sharma, S. K., Sailo, B., Devi, C. B., Singh, I. M., et al. (2018). Climate resilient agriculture in Manipur: status and strategies for sustainable development. Curr. Sci. 115, 1342–1350. doi: 10.18520/cs/v115/i7/1342-1350
Saldarriaga-Hernandez, S., Hernandez-Vargas, G., Iqbal, H. M. N., Barcelo, D., and ParraSaldivar, R. (2020). Bioremediation potential of Sargassum sp. biomass to tackle pollution in coastal ecosystems: circular economy approach. Sci. Total Environ. 715, 136978. doi: 10.1016/j.scitotenv.2020.136978
Samuel, M. P., and Satapathy, K. K. (2008). Concerted rainwater harvesting technologies suitable for hilly agro-ecosystems of Northeast India. Curr. Sci. 1130–1132. Available online at: https://www.researchgate.net/publication/255614015
Soni, R. P., Katoch, M., and Ladohia, R. (2014). Integrated farming systems-a review. IOSR J. Agric. Vet. Sci. 7, 36–42. doi: 10.9790/2380-071013642
Subbiah, B. V., and Asija, G. L. (1956). A rapid procedure for the estimation of available N in soils. Curr. Sci. 25, 259–260.
Tandon, H. L. S. (1995). Methods of Analysis of Soils, Plants, Waters and Fertilizers. New Delhi, India: FDCO.
Tuomisto, H. L., Hodge, I. D., Riordan, P., and Macdonald, D. W. (2012). Does organic farming reduce environmental impacts? – a meta-analysis of European research. J. Environ. Manage. 112, 309–320. doi: 10.1016/j.jenvman.2012.08.018
Turmel, M. S., Speratti, A., Baudron, F., Verhulst, N., and Govaerts, B. (2015). Crop residue management and soil health: a systems analysis. Agric. Syst. 134, 6–16. doi: 10.1016/j.agsy.2014.05.009
Von Borell, E., and Sorensen, J. T. (2004). Organic livestock production in Europe: aims, rules and trends with special emphasis on animal health and welfare. Livest. Prod. Sci. 90, 3–9. doi: 10.1016/j.livprodsci.2004.07.003
Keywords: integrated organic farming system, nutrient balance, profitability, system productivity, water harvesting, residue recycling
Citation: Layek J, Das A, Ansari MA, Mishra VK, Rangappa K, Ravisankar N, Patra S, Baiswar P, Ramesh T, Hazarika S, Panwar AS, Devi S, Ansari MH and Paramanik B (2023) An integrated organic farming system: innovations for farm diversification, sustainability, and livelihood improvement of hill farmers. Front. Sustain. Food Syst. 7:1151113. doi: 10.3389/fsufs.2023.1151113
Received: 25 January 2023; Accepted: 14 March 2023;
Published: 17 April 2023.
Edited by:
Sanjay Singh Rathore, Indian Agricultural Research Institute (ICAR), IndiaReviewed by:
Kapila Shekhawat, Indian Council of Agricultural Research (ICAR), IndiaOwais Ali Wani, Sher-e-Kashmir University of Agricultural Sciences and Technology of Kashmir, India
Copyright © 2023 Layek, Das, Ansari, Mishra, Rangappa, Ravisankar, Patra, Baiswar, Ramesh, Hazarika, Panwar, Devi, Ansari and Paramanik. This is an open-access article distributed under the terms of the Creative Commons Attribution License (CC BY). The use, distribution or reproduction in other forums is permitted, provided the original author(s) and the copyright owner(s) are credited and that the original publication in this journal is cited, in accordance with accepted academic practice. No use, distribution or reproduction is permitted which does not comply with these terms.
*Correspondence: Meraj A. Ansari, merajiari@gmail.com; Natesan Ravisankar, n.ravisankar@icar.gov.in
†These authors have contributed equally to this work and share first authorship