- 1Department of Agriculture, Minab Higher Education Center, University of Hormozgan, Bandar Abbas, Iran
- 2Plant Protection Research Group, University of Hormozgan, Bandar Abbas, Iran
- 3Department of Horticultural Sciences, University of Hormozgan, Bandar Abbas, Iran
Sweet pepper (Capsicum annum) is an important vegetable with high economic and nutritional value. The present study was conducted to evaluate the effectiveness of biological treatments on the nutritional, nutraceutical and functional status of greenhouse sweet peppers cv. Nirvin. Plants were divided into two categories: Peppers that were biologically treated included five microbial-based fertilizers and insecticide and those that were chemically treated contained a large number of chemical fertilizers and pesticides. The results showed that the total phenolic content, antioxidant activity, and leaf chlorophyll content were significantly higher (1.16, 1.14, and 1.09-fold) in the biologically treated plants compared to those that received the chemical treatment. The concentration of Fe, K, Mg, P, Ca, Cu, Si, and Mn also increased in the fruits of biologically treated sweet pepper plants. Fe, Mg, and P content of the leaves was higher in the chemically treated plants, while, the concentration of Zn and Cu showed the higher values in the leaves of the biologically treated plants. There was no significant difference between biological and chemical treatments in plant height as well as the number of flowers and fruits per plant. In conclusion, biological treatment could significantly improve the nutritional, nutraceutical and functional values of sweet peppers. Considering the risk of environmental pollution, the high cost of chemical fertilizers and pesticides, as well as their adverse impact on human health and the ecosystem, biological treatment can be a suitable alternative for sweet pepper management programs.
Introduction
In recent years, greenhouse crops have gained more attention and importance due to numerous advantages such as high productivity, possibility of out-of-season production, high quality of products, low water consumption, and job creation (Naderi et al., 2019). Sweet pepper (Capsicum annuum) belongs to the nightshade family (Solanaceae). It is considered worldwide as an important vegetable plant with high nutritional value, mainly used for fresh consumption, cooking, and processing (Krasnow and Ziv, 2022). In addition, this crop is used in food and cosmetics, pharmaceuticals, as well as nutraceuticals and has beneficial impact on human health (Murariu et al., 2019; Brezeanu et al., 2022). These beneficial effects of sweet peppers on human health are attributed to the high concentration of ascorbic acid, carotenoids, tocopherols, and phenolic compounds that scavenge free radicals and protect against oxidative damage (Sreeramulu and Raghunath, 2010; Chen and Kang, 2013; Brezeanu et al., 2022). Greenhouse crops have a high commercial value due to production costs. Consequently, the damage caused by pests and pathogens in greenhouses is of great importance from an economic point of view (Nasiri and Nikzadfar, 2007). Chemicals such as pesticides and fertilizers are extensively used yearly in greenhouse production worldwide. However, due to the contamination of water, air, and soil, the increased use of these chemical inputs can pose a significant risk to the environment. These agents can also lead to resistance in insects and pathogens, reduce populations of beneficial insects, contribute to high greenhouse gas emissions and incur high costs (Arias-Estevez et al., 2008; Alvarez et al., 2017; Brezeanu et al., 2022). Due to the environmental and health risks associated with the use of chemical fertilizers and pesticides in agriculture, the production and consumption of safe products have become a practical alternative that attracted the attention of investors in the agricultural sector worldwide (Mishra et al., 2013). Biopesticides and biofertilizers, as environmentally friendly and cost-effective means, contain the natural microflora (beneficial bacteria and fungi). They provide micro- and macronutrients through the varieties of mechanisms including fixation of nitrogen, solubilization of phosphate and potassium and production of plant growth regulators, antibiotics, siderophores, hydrogen cyanide, lytic acid and indole acetic acid (Sinha et al., 2010; Babalola and Glick, 2012). In other words, the biological agents can improve plant diseases and pests control, soil fertility, microflora, plant growth, and yield (Deepak et al., 2014; Fasusi et al., 2021). Recently, a plant protein hydrolysate-based biostimulant increased growth rate and fruit yield and promoted faster and more efficient plant recovery in stressed sweet pepper plants (Agliassa et al., 2021). Additionally, biostimulant treatment improved yield parameters, vitamin C, phenolic contents and antioxidant activities in sweet pepper fruits (Paradiković et al., 2011). Moreover, a seaweed liquid extract biostimulant increased the yield, antioxidant activity and composition of C. annuum (Ashour et al., 2021). Therefore, there is increasing consumer demand for safe and residue-free crop production by applying biopesticides and biofertilizers. Hormozgan province is located in the southern region of Iran and is considered the production hub of sweet pepper. Considering the importance of this crop in the agricultural economy of Hormozgan province, the present study aimed to evaluate the effectiveness of the application of biological agents (biofertilizers and biopesticides) in the management program of sweet pepper under greenhouse conditions.
Materials and methods
Study location
The present study was conducted in 2021 and 2022 in a commercial greenhouse in a completely randomized design in the Minab region (longitude and latitude of 57.01758671 and 27.14917768 and 27 m elevation), Hormozgan province, Iran. Healthy and uniform sweet peppers cv. Nirvin transplants with four to five fully expanded leaves were planted with the row spacing and inter-row spacing of 50 and 55 cm, respectively. Three rows (each 55 m long) were selected for each treatment. Plants were irrigated every other day for 6 h using a tape drip irrigation system. Drip irrigation involved distinct tubes for each treatment and the treatments were separated based on a drip-lock end cap. In this case, the special tubes for each treatment were opened and the treatment was applied. After each treatment, tubes were washed with water to eliminate any residual materials. The insolation for growing plants in the greenhouse ranged from 5,000 to 6,000 lux. The average maximum and minimum temperature (October 2021-March 2022) was between 14 and 28°C, respectively. Greenhouse soil and water samples were collected and analyzed before the start of the experiment; their characteristics are shown in Tables 1, 2. The soil sample was taken at a depth of 0–30 cm. Sheep manure was applied to improve the quality of the greenhouse soil.
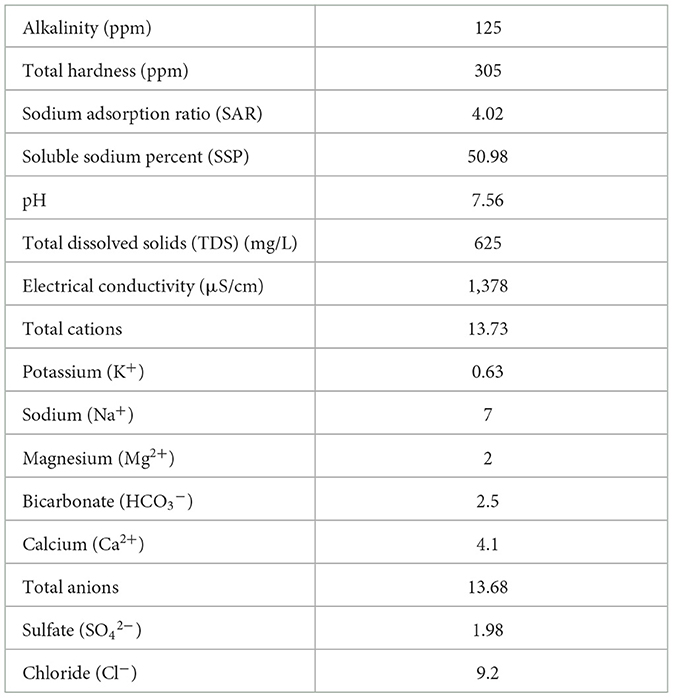
Table 1. Characteristics of chemical parameters of the irrigation water sample in the greenhouse used for sweet pepper cultivation.
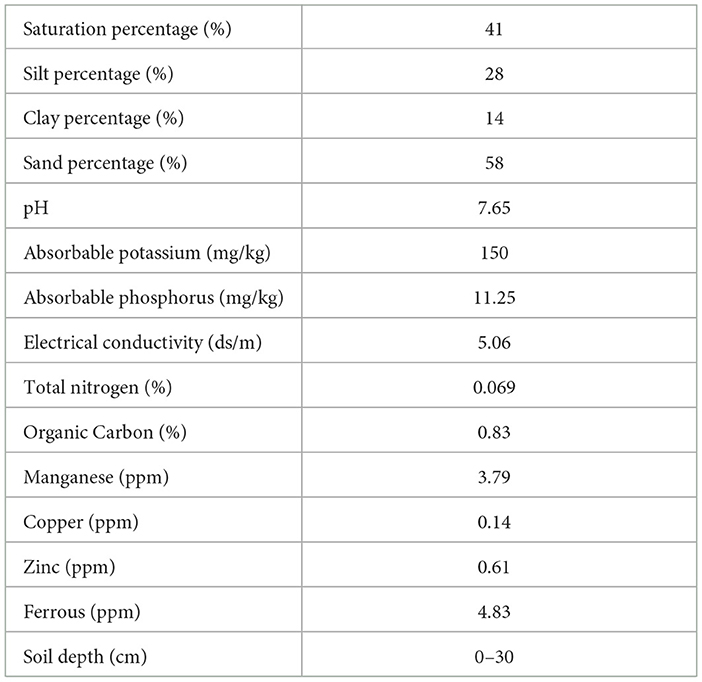
Table 2. Characteristics of the chemical and structural parameters of the greenhouse soil sample used for sweet pepper cultivation.
Treatments and experimental design
Plants were divided into two categories under the same conditions; sweet peppers which received biological treatments and those that were chemically treated. Nature Biotechnology Company (Biorun) products were used as the biological treatment, including: “Probio 96” (a biological fertilizer containing Bacillus subtilis), “Biofarm” [a biological fertilizer formulated based on several nitrogen fixing PGPRs, such as Azospirillum and Pseudomonas (2 × 107 CFU per mL)], “RoshdAfza” (a biological fertilizer formulated based on several phyllosphere bacteria), “Bioguard” [a water-soluble fertilizer based on beneficial microorganisms such as Trichoderma harzianum and Bacillus velezensis, humic acid (potassium humate), and seaweed extract (Ascophyllum nodosum, Laminaria, and Sargassum)], and Biolep (a biological insecticide formulated based on the entomopathogenic bacterium, Bacillus thuringiensis subsp. kurstaki). Probio 96 (three liters per hectare), Biofarm (one liter per hectare), and Bioguard (1.5 kg per hectare) were added with the adequate volume of water to irrigate plants. RoshdAfza (three liters per hectare) and Biolep (two liters per hectare) were added to the appropriate volume of water and sprayed on sweet pepper plants. Probio 96 and Biofarm fertilizers were used four times during the growth stage. RoshdAfza fertilizer was applied to sweet pepper plants at two stages (30 and 60 days after cultivation). Bioguard was used monthly during the cultivation cycle. Biolep insecticide was applied on a weekly basis once insects started appearing on the plants.
During their growth cycle, plants in the second category received common conventional management programs (application of fertilizers and pesticides). Chemical fertilizers included ammonium sulfate, khazra iron, zinc, and boron chelate fertilizers, humic acid, ammonium nitrate, calcium nitrate, codamin radicular rooting fertilizer, super liquid fertilizer, liquid sulfur fertilizer, frutaliv fertilizer, NPK 20-20-20 (10 Kg per hectare), super micro plus fertilizer, isabion amino acids. Chemical pesticides in this category included beltanol, berdofix, ortiva top, signum, potassium phosphite, dichlorvos, abamectin, starkle, avaunt, acetamiprid, dursban, nissorun, sponsor, chess, methoxyfenozide, and adjuvants.
Leaf chlorophyll content
For each treatment, ten leaf samples were selected from mature leaves and below the growing point with similar growth in ten different places of three rows in the greenhouse at 90 days after transplanting and their leaf chlorophyll concentration was determined using a SPAD meter (Konica-Minolta, Japan).
Plant height, number of flowers and fruits per plant
For each treatment, 10 plants were selected from 10 different places in three rows of each treatment to measure plant height, number of flowers and fruits per plant at the end of the experiment, flowering stage and harvest period, respectively. Their height was determined using a measuring tape. A number of fruits and flowers per plant were counted.
Mineral composition
The plant samples (leaves and fruits) were dried. Then the amount of 0.5 g of the powdered samples from 10 plants in three rows of each treatment were mixed with 12.5 ml of HNO3 and kept on a heater at a temperature of 100°C for 2 h. A yellow solution was formed, and the residue was filtered through filter paper. Finally, the element concentrations in the leaf and fruit samples of sweet peppers were determined by inductively coupled plasma optical emission spectrometry (ICP-OES, Agilent 700 Series, USA).
Total phenolic content
One gram of sweet pepper fruit tissue was homogenized with 10 ml of distilled water and then filtered. The amount of 700 μl of the extract was mixed with 900 μl sodium carbonate solution (2% w/v). Then 180 μl Folin-Ciocalteau reagent (50%) was added to the mixture and the samples were kept at room temperature for 30 min. The absorbance of the samples was then evaluated using a spectrophotometer (DR3900, Hach, Germany) at 750 nm. The total phenolic content was measured using a calibration curve and expressed as mg gallic acid per 100 g fresh weight (Etemadipoor et al., 2020). Four replicates of each treatment were randomized on the fruits of test plants in three rows at the harvest period.
Antioxidant activity
One gram of sweet pepper fruit tissue was homogenized with 10 ml of methanol (80%) and then filtered. One ml of DPPH (0.1 mM) was added to 100 μl of the methanolic fruit extract and mixed with one ml of Tris-HCl (pH = 7.5). The samples were vortexed and kept in the dark. Four replicates of each treatment were randomized on the fruits of test plants in three rows at the harvest period. The absorbance of the samples was evaluated using a spectrophotometer (DR3900, Hach, Germany) at 517 nm. Antioxidant activity was measured according to the equation (Etemadipoor et al., 2020):
Antioxidant activity (%): [1 – Abs sample/Abs control × 100]
Statistical analysis
The independent-sample t-test was used to compare the effects of treatments at 5% probability level (2-tailed) using SPSS 21.0 (SPSS Inc., Chicago, IL, USA).
Results and discussion
Soil and water analysis
According to soil and water analysis conducted before the start of the experiment (Tables 1, 2), water EC showed an optimum value (1,378 μS/cm). The soil organic carbon, P, Fe, Mn, Zn, and Cu concentrations were relatively low and the soil demonstrated a deficiency in nitrogen element.
Effect of biological and chemical treatments on some growth and physiological parameters
Leaf chlorophyll concentration increased by the biological treatment compared to the chemical treatment (Figure 1A). SPAD values have been shown to be positively correlated with nitrogen concentration and leaf chlorophyll content (Murdock et al., 1997). Lozo et al. (2022) reported that Bacillus bacteria can produce indole acetic acid and aminocyclopropane carboxylate deaminase, which increases plant growth and inhibits chlorophyll degradation. Aminocyclopropane carboxylate deaminase reduces the formation of ethylene hormone in plants by degrading aminocyclopropane carboxylate, a precursor of ethylene (Ghorai et al., 2021), which may inhibit plant reactions associated with senescence, such as chlorophyll degradation. There was no significant difference between biological and chemical treatments in plant height and the number of flowers and fruits per plant (Figures 1B–D). Our findings were in accordance with another study that showed the application of biostimulants did not affect marketable yield but improved pepper fruit quality (Arthur et al., 2022). Additionally, the application of microbial-based biostimulants did not improve the morphological characteristics and yield of pepper fruit (Majkowska-Gadomska et al., 2021).
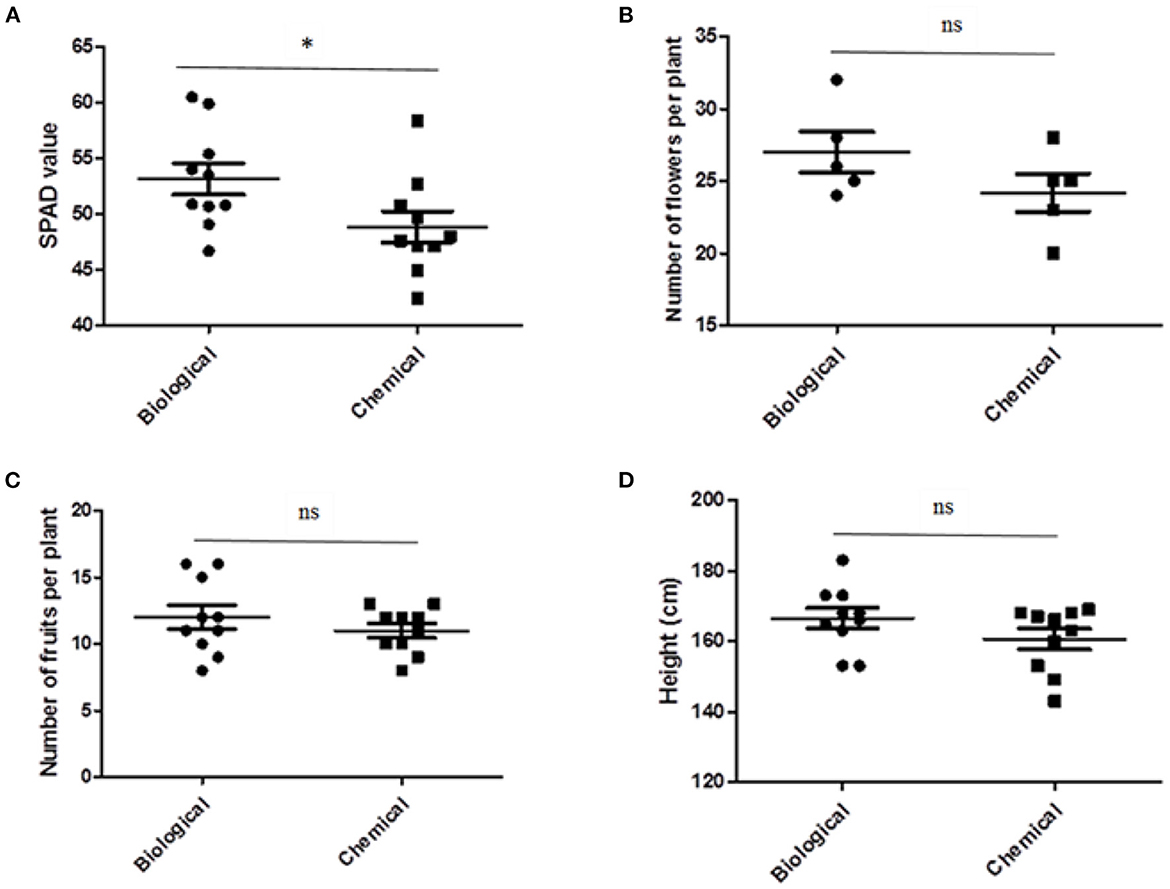
Figure 1. The effect of biological and chemical treatments on leaf SPAD value (A), number of flowers (B) and fruits (C) per plant and height (D) of sweet pepper plants. Asterisk indicates a statistically significant effect (*p < 0.05), whereas “ns” indicates not significant (p > 0.05) between biological and chemical treatments.
Effect of biological and chemical treatments on the element concentration of sweet pepper plants
The concentration of Fe, K, Mg, P, Ca, Cu, Si, and Mn increased in sweet pepper fruits when the plants were treated with the biological treatment. Satisfactory fruit quality and productivity require the adequate supply of macro- and micronutrients (Gruda, 2005). In our experiment, the higher concentration of macro- and micronutrients in the biologically treated plants demonstrated a beneficial impact of this treatment on fruit quality. Compared with the chemical treatment, the concentration of Zn and Cu was higher in the leaves of the biologically treated plants (Table 3). The beneficial effects of plant growth-promoting bacteria as a significant component of our biological treatments may be due to the induction of phytohormone production, nitrogen fixation, and enhancement of mineral nutrient uptake by plants (Del Amor and Cuadra-Crespo, 2011; Backer et al., 2018). Cisternas-Jamet et al. (2020) indicated that inoculation of the roots of bell pepper plants with Bacillus amyloliquefaciens bacteria led to increased Ca and Fe concentrations, antioxidant activity in fruits, and also an improved growth. Similarly, the use of growth-promoting bacteria with a mixture of compost and Trichoderma harzianum fungus has been shown to increase the concentration of Cu in tomato (Khan et al., 2017). It has been reported that B. amyloliquefaciens can affect Ca transport in plants through the production of auxin (Tadesse, 1997; Salvatierra-Martinez et al., 2018). Application of B. subtilis in cauliflower resulted in an increase in Ca concentration (Ekinci et al., 2014). Inoculation of green bell pepper seeds with B. amyloliquefaciens resulted in an increase in Ca and Fe concentration (Cisternas-Jamet et al., 2020). The pH of the soil in the greenhouse was 7.65 (Table 2), which could affect the availability of several micronutrients such as Fe, Zn, Mn, etc., which were deficient in this study. Under iron deficiency conditions, chelating compounds such as siderophores are synthesized by some bacteria. These compounds help in the uptake of macronutrients and have a beneficial effect on plant growth and productivity (Shahriari and Moradi, 2018).
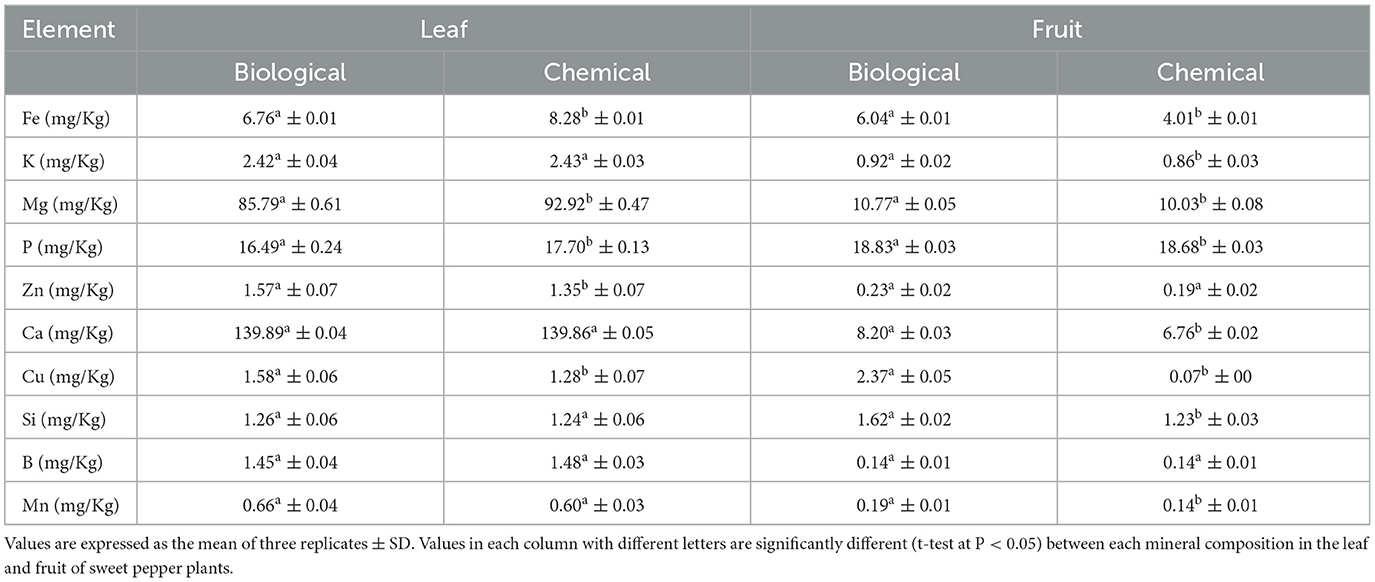
Table 3. Concentration of some macro- and micronutrients in the leaf and fruit of sweet pepper plants.
Effect of biological and chemical treatments on the total phenols and antioxidant activity of sweet peppers
In our experiment the concentration of total phenols and antioxidant activity showed a significant increase in plants treated with the biological treatment in comparison with the chemical treatment (Figure 2). These results are in agreement with previous investigations, which showed that inoculation of green pepper seeds with B. amyloliquefaciens bacteria caused an increase in vitamin C, total phenols and antioxidant activity (Cisternas-Jamet et al., 2020). The results of another study showed that the use of biological agents could lead to a higher yield of sweet pepper (El Arnaouty et al., 2020). Higher concentration of micronutrients such as Fe, Mn, Zn and Cu can increase the antioxidant activity within plant tissues as these essential elements are cofactors of enzymatic antioxidants such as superoxide dismutase. In addition, zinc plays an important role (cofactor of transcription factors) in the expression of genes encoding antioxidant enzymes (Homayoonzadeh et al., 2022). There was a significant positive correlation between total phenolic content and antioxidant activity (r = 0.983, significant at the 0.05 level). Therefore, the high antioxidant activity in biologically treated sweet pepper plants in our experiment, could be attributed to the higher concentration of phenolic compounds in the fruit extract.
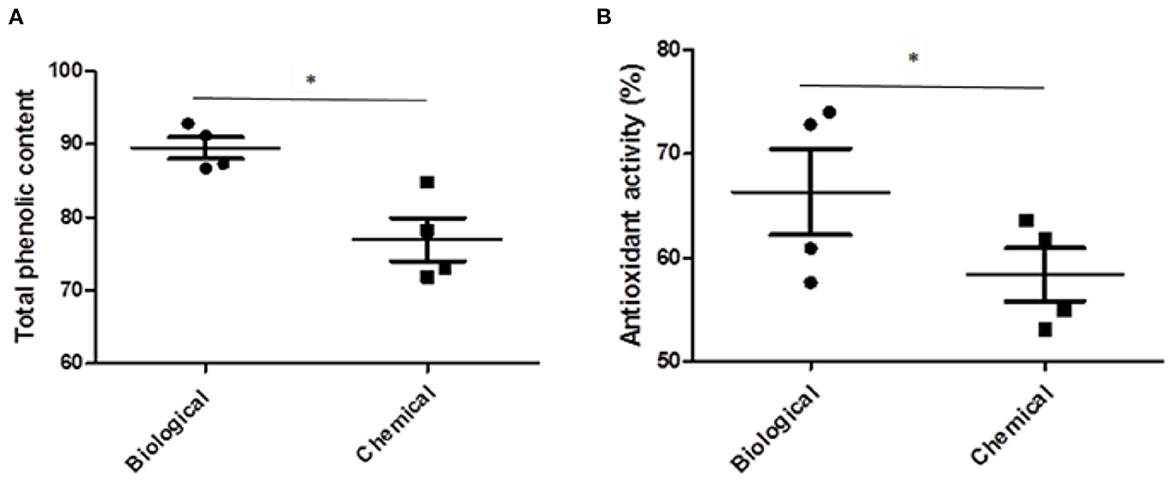
Figure 2. The effect of biological and chemical treatments on the total phenolic content (A) and antioxidant activity (B) of sweet pepper fruits. Asterisk indicates a statistically significant effect (*p < 0.05) between biological and chemical treatments.
Conclusion
This study provides new information on the effects of microbial-based biological treatments on the nutritional, nutraceutical and functional properties of greenhouse sweet pepper. Biological treatments significantly improved the nutritional, nutraceutical and functional composition of sweet pepper fruits compared to chemical treatments. These results indicate that biological treatments have great potential to increase the concentration of elements, total phenolic content, and antioxidant activity in sweet pepper fruits, compared with the chemical control program under the same conditions. However, the exact mechanisms of these processes need further investigation. Therefore, biological treatments can be used as a practical, safe, and promising alternative to chemical agents in sweet pepper management programs for growers seeking environmentally friendly strategies to improve sweet pepper fruit quality.
Data availability statement
The original contributions presented in the study are included in the article/supplementary material, further inquiries can be directed to the corresponding author.
Author contributions
AT conceived the study, performed the experiments, analyzed the data, and mainly wrote the manuscript. AM performed some experiments. BJ wrote some parts of the manuscript. All authors edited and approved the final version of the manuscript.
Funding
This study was funded by the Minab Higher Education Center, University of Hormozgan.
Acknowledgments
The authors express their gratitude to Minab Higher Education Center, University of Hormozgan to provide laboratory facilities and equipment.
Conflict of interest
The authors declare that the research was conducted in the absence of any commercial or financial relationships that could be construed as a potential conflict of interest.
Publisher's note
All claims expressed in this article are solely those of the authors and do not necessarily represent those of their affiliated organizations, or those of the publisher, the editors and the reviewers. Any product that may be evaluated in this article, or claim that may be made by its manufacturer, is not guaranteed or endorsed by the publisher.
References
Agliassa, C., Mannino, G., Molino, D., Cavalletto, S., Contartese, V., Bertea, C. M., et al. (2021). A new protein hydrolysate-based biostimulant applied by fertigation promotes relief from drought stress in Capsicum annuum L. Plant Physiol. Biochem. 166, 1076–1086. doi: 10.1016/j.plaphy.2021.07.015
Alvarez, A., Saez, J. M., Costa, J. S. D., Colin, V. L., Fuentes, M. S., Cuozzo, S. A., et al. (2017). Actinobacteria: current research and perspectives for bioremediation of pesticides and heavy metals. Chemosphere 166, 41–62. doi: 10.1016/j.chemosphere.2016.09.070
Arias-Estevez, M., Lopez-Periago, E., Mart'inez-Carballo, E., Simal-G'andara, J., Mejuto, J. C., and Garc'ia-R'io, L. (2008). The mobility and degradation of pesticides in soils and the pollution of groundwater resources. Agric. Ecosyst. Environ. 123, 247–260. doi: 10.1016/j.agee.2007.07.011
Arthur, J. D., Li, T., and Bi, G. (2022). Plant Growth, Yield, and quality of containerized heirloom chile pepper cultivars affected by three types of biostimulants. Horticulturae 9, 12. doi: 10.3390/horticulturae9010012
Ashour, M., Hassan, S. M., Elshobary, M. E., Ammar, G. A., Gaber, A., Alsanie, W. F., et al. (2021). Impact of commercial seaweed liquid extract (TAM®) biostimulant and its bioactive molecules on growth and antioxidant activities of hot pepper (Capsicum annuum). Plants 10,1045. doi: 10.3390/plants10061045
Babalola, O. O., and Glick, B. R. (2012). The use of microbial inoculants in African agriculture: current practice and future prospects. J. Food Agric. Environ. 10, 540–549.
Backer, R., Rokem, J. S., Ilangumaran, G., Lamont, J., Praslickova, D., Ricci, E., et al. (2018). Plant growth-promoting rhizobacteria: context, mechanisms of action, and roadmap to commercialization of biostimulants for sustainable agriculture. Front. Plant Sci. 9, 1473. doi: 10.3389/fpls.2018.01473
Brezeanu, C., Brezeanu, P. M., Stoleru, V., Irimia, L. M., Lipşa, F. D., Teliban, G. C., et al. (2022). Nutritional value of new sweet pepper genotypes grown in organic system. Agriculture 12, 1863. doi: 10.3390/agriculture12111863
Chen, L., and Kang, Y. H. (2013). Anti-inflammatory and antioxidant activities of red pepper (Capsicum annum L.) stalk extracts: comparison of pericarp and placenta extract. J. Funct. Food 5, 1724–1731. doi: 10.1016/j.jff.2013.07.018
Cisternas-Jamet, J., Salvatierra-Martínez, R., Vega-Gálvez, A., Stoll, A., Uribe, E., and Goñi, M. G. (2020). Biochemical composition as a function of fruit maturity stage of bell pepper (Capsicum annum) inoculated with Bacillus amyloliquefaciens. Sci. Hortic. 263, 109107. doi: 10.1016/j.scienta.2019.109107
Deepak, B., Mohammad, W. A., Ranjan, K. S., and Narendra, T. (2014). Biofertilizers function as a key player in sustainable agriculture by improving soil fertility, plant tolerance, and crop productivity. Microb. Cell Fact. 13, 66. doi: 10.1186/1475-2859-13-66
Del Amor, F. M., and Cuadra-Crespo, P. (2011). Plant growth-promoting bacteria as a tool to improve salinity tolerance in sweet pepper. Funct. Plant Biol. 39, 82–90. doi: 10.1071/FP11173
Ekinci, M., Turan, M., Yildirim, E., Günes, A., Kotam, R., and Dursun, A. (2014). Effect of plant growth promoting Rhizobacteria on growth, nutrient, organic acid, amino acid and hormone content of cauliflower (Brassica oleracea L. var. botrytis) transplants. Acta Sci. Pol. Hortorum. Cultus. 13, 71–85.
El Arnaouty, S. A., El-Heneidy, A. H., Afifi, A. I., Heikal, I. H., and Kortam, M. N. (2020). Comparative study between biological and chemical control programs of certain sweet pepper pests in greenhouses. Egypt J. Biol. Pest Control 30, 1–7. doi: 10.1186/s41938-020-00226-z
Etemadipoor, R., Dastjerdi, A. M., Ramezanian, A., and Ehteshami, S. (2020). Ameliorative effect of gum arabic, oleic acid and/or cinnamon essential oil on chilling injury and quality loss of guava fruit. Sci. Hortic. 266, 109255. doi: 10.1016/j.scienta.2020.109255
Fasusi, O. A., Cruz, C., and Babalola, O. O. (2021). Agricultural sustainability: microbial biofertilizers in rhizosphere management. Agriculture 11, 163. doi: 10.3390/agriculture11020163
Ghorai, A. K., Patsa, R., Jash, S., and Dutta, S. (2021). “Microbial secondary metabolites and their role in stress management of plants,” in Biocontrol Agents and Secondary Metabolites (Cambridge: Woodhead Publishing), 283–319. doi: 10.1016/B978-0-12-822919-4.00012-0
Gruda, N. (2005). Impact of environmental factors on product quality of greenhouse vegetables for fresh consumption. Crit. Rev. Plant Sci. 24, 227–274. doi: 10.1080/07352680591008628
Homayoonzadeh, M., Haghighi, S. R., Hosseininaveh, V., Talebi, K., Roessner, U., and Winters, A. (2022). Effect of spirotetramat application on salicylic acid, antioxidative enzymes, amino acids, mineral elements, and soluble carbohydrates in Cucumber (Cucumis sativus L.). Biol. Life Sci Forum 11, 3. doi: 10.3390/IECPS2021-11921
Khan, Y. M., Manjurul Haque, M., Molla, A. H., Rahman, M., and Alam, M. Z. (2017). Antioxidant compounds and minerals in tomatoes by Trichoderma enriched bio- fertilizer and their relationship with the soil environments. J. Integr. Agric. 16, 691–703. doi: 10.1016/S2095-3119(16)61350-3
Krasnow, C., and Ziv, C. (2022). Non-chemical approaches to control postharvest gray mold disease in bell peppers. Agronomy 12, 216. doi: 10.3390/agronomy12010216
Lozo, J., Danojević, D., Jovanović, Ž., Nenadović, Ž., Fira, D., Stanković, S., et al. (2022). Genotype-Dependent antioxidative response of four sweet pepper cultivars to water deficiency as affected by drought-tolerant Bacillus safensis SS-2.7 and Bacillus thuringiensis SS-29.2 Strains. Horticulturae 8, 236. doi: 10.3390/horticulturae8030236
Majkowska-Gadomska, J., Dobrowolski, A., Jadwisieńczak, K. K., Kaliniewicz, Z., and Francke, A. (2021). Effect of biostimulants on the growth, yield and nutritional value of Capsicum annuum grown in an unheated plastic tunnel. Sci. Rep. 11, 1–14. doi: 10.1038/s41598-021-01834-x
Mishra, D. J., Singh, R., Mishra, U. K., and Kumar, S. S. (2013). Role of bio-fertilizer in organic agriculture: a review. Res. J. Recent Sci. 2, 39–41.
Murariu, F., Voda, A. D., and Murariu, O. C. (2019). Researches on food safety assessment—Supporting a healthy lifestyle for the population from NE of Romania. J. Biotechnol. 5, S68. doi: 10.1016/j.jbiotec.2019.05.238
Murdock, L., Jones, S., Bowely, C., Needham, P., James, J., and Howe, P. (1997). Using a Chlorophyll Meter to Make Nitrogen Recommendations on Wheat. Louisville, KY: Cooperative Extension Service University of Kentucky-college of Agriculture.
Naderi, S. A., Dehkordi, A. L., and Taki, M. (2019). Energy and environmental evaluation of greenhouse bell pepper production with life cycle assessment approach. Environ. Sustain. Indic. 3, 100011. doi: 10.1016/j.indic.2019.100011
Nasiri, A., and Nikzadfar, M. (2007). Assessment of the Current Situation and Prospects of Greenhouse Cultivation. Isfahan: The First National Conference of Hydroponics and Greenhouse Production, Isfahan University of Technology.
Paradiković, N., Vinković, T., Vinković Vrček, I., Žuntar, I., Bojić, M., and Medić-Šarić, M. (2011). Effect of natural biostimulants on yield and nutritional quality: an example of sweet yellow pepper (Capsicum annuum L.) plants. J. Sci. Food Agric. 91, 2146–2152. doi: 10.1002/jsfa.4431
Salvatierra-Martinez, R., Arancibia, W., Araya, M., Aguilera, S., Olalde, V., Bravo, J., et al. (2018). Colonization ability as an indicator of enhanced biocontrol capacity—an example using two Bacillus amyloliquefaciens strains and Botrytis cinerea infection of tomatoes. J. Phytopathol. 166, 601–612. doi: 10.1111/jph.12718
Shahriari, F., and Moradi, R. (2018). Siderophores in environmental research: roles and applications. J Bio Safety 12, 1–20.
Sinha, R. K., Valani, D., Chauhan, K., and Agarwal, S. (2010). Embarking on a second green revolution for sustainable agriculture by vermiculture biotechnology using earthworms: reviving the dreams of Sir Charles Darwin. J. Agric. Biotech. Sustain. Dev. 2, 113–128. doi: 10.5897/JABSD.9000017
Keywords: beneficial microorganisms, clean production, fruit quality, greenhouse, vegetable crop
Citation: Tahmasebi A, Mirzaalian Dastjerdi A and Jamali B (2023) Microbial-based biological treatments improved the nutritional, nutraceutical and functional properties of greenhouse sweet pepper (Capsicum annuum L.). Front. Sustain. Food Syst. 7:1145972. doi: 10.3389/fsufs.2023.1145972
Received: 18 January 2023; Accepted: 24 March 2023;
Published: 06 April 2023.
Edited by:
Matteo Balderacchi, Independent Researcher, Piacenza, ItalyReviewed by:
Lin Xu, Ghent University, BelgiumGiuseppe Mannino, University of Turin, Italy
Sławomir Swierczyński, Poznan University of Life Sciences, Poland
Copyright © 2023 Tahmasebi, Mirzaalian Dastjerdi and Jamali. This is an open-access article distributed under the terms of the Creative Commons Attribution License (CC BY). The use, distribution or reproduction in other forums is permitted, provided the original author(s) and the copyright owner(s) are credited and that the original publication in this journal is cited, in accordance with accepted academic practice. No use, distribution or reproduction is permitted which does not comply with these terms.
*Correspondence: Aminallah Tahmasebi, YS50YWhtYXNiaSYjeDAwMDQwO2hvcm1vemdhbi5hYy5pcg==