- 1Institute for the Advanced Study of Sustainability (UNU-IAS), United Nations University, Tokyo, Japan
- 2Tokyo College, The University of Tokyo, Tokyo, Japan
- 3Future Earth Global Hub Japan, Tokyo, Japan
- 4Institute for Future Initiatives, The University of Tokyo, Tokyo, Japan
- 5International Research Programme Coordinating Unit, Armenian National Agrarian University, Yerevan, Armenia
- 6Florida Museum of Natural History, University of Florida, Gainesville, FL, United States
- 7Faculty of Foreign Languages, Southwest Forestry University, Kunming, China
- 8Institute for Global Environmental Strategies, Hayama, Japan
- 9Subtropical Field Science Center, Faculty of Agriculture, The University of the Ryukyus, Nishihara, Japan
- 10Madagascar Forests and Communities Caretakers Association, Antananarivo, Madagascar
- 11Mention Foresterie et Environnement, Ecole Supérieure des Sciences Agronomiques, Université d'Antananarivo, Antananarivo, Madagascar
- 12Ocean One Social Research Centre, Soroti, Uganda
- 13National Institute for Environmental Studies of Japan, Tsukuba, Japan
- 14Research Institute for Humanity and Nature, Kyoto, Japan
- 15Stockholm Resilience Centre, Stockholm University, Stockholm, Sweden
Although biodiversity is a central component of food systems, conventional food systems have become one of the major drivers of biodiversity loss globally. There is an increasing need to transform food systems to provide sufficient and nutritious food, but with minimal negative impacts on the environment and society. One of the possible avenues to enable the sustainable transformation of food systems might be through the development of locally appropriate biodiversity-based solutions. In this paper we report the insights and lessons learned during the design and implementation of transdisciplinary projects that employed the concept of Sustainable Development Goals labs (SDG-Labs) to create biodiversity-based solutions to transform food systems. The six SDG-Labs outlined in this paper were implemented in Armenia, China, Japan, Madagascar, Thailand, and Uganda. Collectively they developed very diverse biodiversity-based solutions that used different components of biodiversity, ranging from novel cultivation systems with endangered plants, to gardens using tree species for wind breaks, or novel tea-forestry production systems. Beyond their ability to leverage different components of biodiversity to transform local food systems (also conserving biodiversity in the process), all solutions had multiple co-benefits such as climate change adaptation/mitigation and livelihoods generation, among other sustainability domains. Through a Strengths-Weaknesses-Opportunities-Threats (SWOT) analysis we synthesized the experiences gained during the design and implementation of all six SDG-Labs. The findings suggest the great promise of these transdisciplinary approaches for developing solutions at the biodiversity-food-climate nexus. However, this synthesis paper also points to the multiple context-specific challenges that should be overcomed to maximize the potential of SDG-Labs to both enable the sustainable transformation of (local) food systems and/or be scaled up effectively.
1. Introduction
Biodiversity plays an essential role for food systems, planetary health, and human wellbeing (Hawkins, 2018; García-Martín et al., 2022). On the one hand biodiversity is crucial for the functioning of agroecosystems and the stability of food production (Crist et al., 2017). For example, biodiversity is essential for soil health and fertility (Frąc et al., 2018) and the stability of the hydrological cycle (Rolls et al., 2018), which are in turn very important for sustainable food production. On the other hand genetic diversity in crops and animals can ensure the production of sufficient and nutritious food (Dawson et al., 2019), and the resilience of agroecosystems against different environmental shocks, including climate change (Martin and Magne, 2015; Córdoba Vargas et al., 2020).
However, biodiversity loss has been accelerating globally in the last decades (IPBES, 2019). There are major concerns that this biodiversity loss could compromise the resilience of food systems and the stability of food production (Roe, 2019), with possible negative ripple effects for food security (Sunderland, 2011; Fischer et al., 2017). In fact many studies have identified that biodiversity loss is one of the main threats for the sustainability of food systems, and essentially for meeting multiple Sustainable Development Goals (SDGs) and targets, such as “No Hunger” (SDG2; Krause and Tilker, 2022).
Paradoxically food systems are one of the major threats to biodiversity (Leclère et al., 2020; Rockström et al., 2020). Modern food systems are the most important driver of land use change (Springmann et al., 2018) and major sources of greenhouse gases (GHGs) emissions (Crippa et al., 2021), both important drivers of biodiversity loss (IPBES, 2019). Beyond land use change and GHG emissions, industrialized agriculture systems have multiple other negative effects on biodiversity through pollution from excessive fertiliser and agrochemical use (Eliasson et al., 2023), agrodiversity loss due to the dominance of few crop and animal species (Zimmerer et al., 2019), and the introduction of invasive species that threaten native (agro)ecosystems (Shabani et al., 2020). There is a vast literature exploring the association between conventional food systems and biodiversity loss: from population growth (Crist et al., 2017) to urbanization and lifestyle changes (Evans and Gawlik, 2020; Pawera et al., 2020).
This creates the paradoxical situation that on the one hand biodiversity is essential for food systems, but on the other hand conventional food systems are one of the most important threats to biodiversity. This calls for an urgent need to transform food systems in order to safeguard biodiversity, and essentially ensure sustainable food supply to meet the accelerating global demand. However, despite the many sustainability initiatives aiming at transforming food systems in a biodiversity-friendly manner, social and economic inequality persist (El Bilali et al., 2021).
In the context of the post-2020 Global Biodiversity Framework, many studies have pointed that biodiversity can indeed provide solutions for sustainable food systems (Gassner et al., 2020; Pimm, 2022). This has been in tandem with the growing popularity of concepts seeking to leverage the role of biodiversity to offer sustainable solutions. One such example is the concept of nature-based solutions (NbS), which refers to the sustainable management and utilization of nature to address sustainability challenges (IUCN, 2020). NbS are inspired and supported by nature, are (often) cost-effective, tend to deliver multiple environmental, social, and economic co-benefits, and could enhance resilience (Haase et al., 2017), while at the same time have the potential to catalyse sustainability transitions (Subedi et al., 2020). In this sense NbS could essentially offer opportunities to generate positive sustainability outcomes and at the same time conserving biodiversity (Chausson et al., 2020). However, there is still no standard way on how NbS should be mobilized, leveraged and used in the real world (Sowińska-Świerkosz and García, 2022).
When looking critically at the above, biodiversity protection and sustainable use could be a “source” of solutions for sustainable food systems. This can arguably provide synergistic ‘win–win’ opportunities, as well as “co-generate” opportunities for wider sustainability transitions (Jacob et al., 2021). Such ‘win-win’ opportunities could be initiated and implemented in different scales through the application of innovative technologies and/or the integration of local knowledge (Béné et al., 2019). Despite the fundamental need for broader changes to legal, political, economic, and other social structures at the national and international scale to attain such transitions (Esquinas-Alcázar, 2005; Kaufman et al., 2021), scholars have argued that under the right circumstances it might be possible to unlock and accelerate transitions through local-scale actions (Loorbach et al., 2017). This is because under the right conditions scalable small-scale actions can evolve to “long-term, multi-dimensional, and fundamental transformation processes” that lead to desired sustainability transitions (Markard et al., 2016, p. 956).
As a possible approach for designing such local solutions in real-world context, sustainability-oriented labs have emerged (McCrory et al., 2020). These types of labs have originated in the efforts to conceptualize real-life experiments1 in the sustainability transitions literature (Sengers et al., 2019). This notion of sustainability-oriented labs encompasses a broad family of participatory, multi-stakeholder and transdisciplinary research approaches seeking to enable transformation (Nevens et al., 2013; Schäpke et al., 2018). According to McCrory et al. (2020), there are seven types of sustainability-oriented labs, namely Urban Living Labs, Living Labs, Real-World Labs, Evolutionary Learning Labs, Urban Transition Labs/Transition Management, Change Laboratories, and Transformation Labs (T-Labs; see Box S2 in the Supplementary material for a brief introduction and distinction). Depending on how they engage with sustainability and their approach to designing and implementing sustainable solutions they can be sub-divided as (a) fix and control; (b) (re-)design and optimize, (c) make and relate, (d) educate and engage, (e) empower and govern; and (f) explore and shape (McCrory et al., 2022; see Box S3 in Supplementary material).
Despite their differences, all these types of sustainability-oriented labs are transdisciplinary venues that test potential solutions to sustainability issues through collaboration between different actors (Bulkeley and Castán Broto, 2013). Their aim is to design and implement bottom-up and context-specific solutions mobilizing the input of different actors, including those that can be generally marginalized from decision-making in some contexts such as women and young people but can be quite innovative and entrepreneurial thinking outside the box (e.g., Aernouts et al., 2020; Wrangsten et al., 2022).
Sustainability-oriented labs have been implemented in very different thematic contexts, including microfinance for youth and clean water (Leist et al., 2018), climate change adaptation (Snyman-van der Walt et al., 2020), or low carbon cities (Voytenko et al., 2016). Similarly wide have been the geographical contexts of such labs, with examples coming from both urban (Bulkeley et al., 2016; von Wirth et al., 2019) and rural settings (Zavratnik et al., 2019), as well as from developed (Voytenko et al., 2016) and developing countries (Leist et al., 2018; Pereira et al., 2022). The above examples clearly show that sustainability-oriented labs have followed very diverse methodological approaches to develop equally diverse solutions (e.g., technologies, agricultural systems, or social institutions). However, what they have in common is the attempt to design context-specific solutions and involve multiple relevant stakeholders.
Sustainability-oriented labs have also been used in the context of food systems. For example, Wolfert et al. (2010) implemented a living lab in the Dutch agri-food sector integrating sustainable farm management through optimal information supply. Pereira et al. (2022) conducted a transformation lab in the Western Cape (South Africa) to identify how local underutilized food products can be used to increase the sustainability and inclusivity of the local food system. Hvitsand et al. (2022) implemented a living lab to strengthen agri-food systems associated with organic vegetables in the Vestfold region in Norway. Gamache et al. (2020) critically discussed through a bibliometric analysis whether agri-food living labs offer support to local transition pathways. Such attempts have emphasized the need of developing context-specific solutions and integrating the perspectives and knowledge of different stakeholders. This inclusivity is particularly important as many studies have pointed the necessity of including very diverse voices and stakeholders in food system transformation towards greater sustainability (Herens et al., 2022; UNEP, FAO, and UNDP, 2023), including young people (Glover and Sumberg, 2020; WFF, 2023).
In the literature there have been few examples of sustainability-oriented labs that use biodiversity as the source of solutions, including for sustainable food systems. Furthermore, when looking at specific types of sustainability-oriented labs (see Box S2, Supplementary material), there have been few efforts in the literature to provide a comprehensive understanding of context-specific approaches to SDG-Labs, let alone in the context of food systems (see below).
Hence the aim of the paper is to synthesise the lessons learned from the design and implementation of very diverse sustainability-oriented labs that developed biodiversity-focused NbS, dubbed as “biodiversity-based solutions,” for sustainable food systems. In particular, it focuses on six Living Labs, which emphasized a strong connection to the SDGs as a guiding principle since their inception (Section 2.2; referred to as SDG-Labs for the remainder of the paper). The SDG-Labs were funded and implemented in the period 2020–2021, and here are comparatively analysed. We aim to distil the main insights generated from these transdisciplinary processes, as a means of enhancing their applicability in other contexts and unlocking their potential for transforming food systems through biodiversity-based solutions.
The papers starts with a section that outlines the timeline of the SDG-Labs (Section 2.1) and describes the comparative methodology (Section 2.2). The results section systematizes the objectives (Section 3.1) and transdisciplinary research approaches of the six SDG-Labs (Section 3.2), shows how they contributed to sustainable food systems (Section 3.3) and sustainability more broadly (Section 3.4), and summarizes the lessons learned from their design and implementation (Section 3.4). The discussion (Section 4) critically addresses some the main insights in view of the literature, and offers recommendations for future improvements for tackling sustainability challenges for food systems, biodiversity conservation and sustainability more broadly.
2. Methodology
2.1. Timeline of the SDG-Labs
The six SDG-Labs outlined in this paper were funded through the 8th International Conference on Sustainability Science (8th ICSS). The 8th ICSS was co-organized by the (a) Institute of Future Initiatives (IFI), University of Tokyo, (b) Tokyo College, University of Tokyo, (c) Institute for Global Environmental Strategies (IGES), (d) Stockholm Resilience Centre, Stockholm University, Sweden, (e) Future Earth, (f) Secretariat of the Convention on Biological Diversity, (g) Kunming Institute of Botany, Chinese Academy of Sciences, and (h) International Union of Biological Sciences. The 8th ICSS was held on January 18–20, 2022 (online), and an online Open Call (10 November 2019 to 8 December 2019) invited proposals for SDG-Labs on the broad topic of “biodiversity-solutions for change.”
The selection criteria were the SDG-Lab’s ability to: (a) find solutions for sustainability problems through leveraging local biodiversity (i.e., through existing local knowledge, innovative solutions or their combination); (b) address multiple SDGs beyond “Life below Water” (SDG14) or “Life on Land” (SDG15), which were the foci of the Open Call; (c) follow a transdisciplinary approach that was defined as “interdisciplinary approach with multi-stakeholder engagement”), and (d) have the capacity to trigger positive change at the local scale (and with the potential to be scaled up). Thematic, disciplinary and geographical diversity were also criteria in the selection process. However, the type of solution, and the format and implementation approach were decided independently by each proposed SDG-Lab.
Ten SDG-Labs were selected for funding (17 January 2020) but only six managed to launch successfully due to the challenges posed by the COVID-19 pandemic (Figure 1). Each of these six SDG-Labs were awarded USD 5,000 in seed funding in order to undertake their activities. The design and implementation of the SDG-Labs lasted much longer as explained below.
The implementation process of the SDG-Labs sought to enable vertical and horizontal information exchange with international researchers in the form of coaching and presentations at international conferences and between groups (see below). Each SDG-Lab group worked with one coach from the Institute of Future Initiatives (IFI; University of Tokyo), Tokyo College (University of Tokyo), Institute for Global Environmental Strategies (IGES), Stockholm Resilience Centre (Stockholm University), and Future Earth. The role of the coach was to provide advice related to the design and implementation of the SDG-Lab, with all coaches being knowledgeable on aspects related to sustainability science, biodiversity/ecosystem services, and social-ecological systems.
Although each SDG-Lab had the freedom to approach its design and implementation individually, the overall timeline of all SDG-Labs was aligned with the different activities of the 8th ICSS (Figure 2). In particular, each SDG-Lab presented the early results of their activities during a virtual session of the 5th Forum for Biodiversity and the 8th ICSS (23 April 2021). Mid-term reporting occurred in an interactive session of the Sustainability Research and Innovation Congress 2021 (SRI 2021; 3 June 2021). The presentation of the final results was held during a dedicated session of the 8th ICSS (19 January 2022). Each SDG-Lab provided an interim and final report that summarized its characteristics, design/implementation process, and final outcomes.
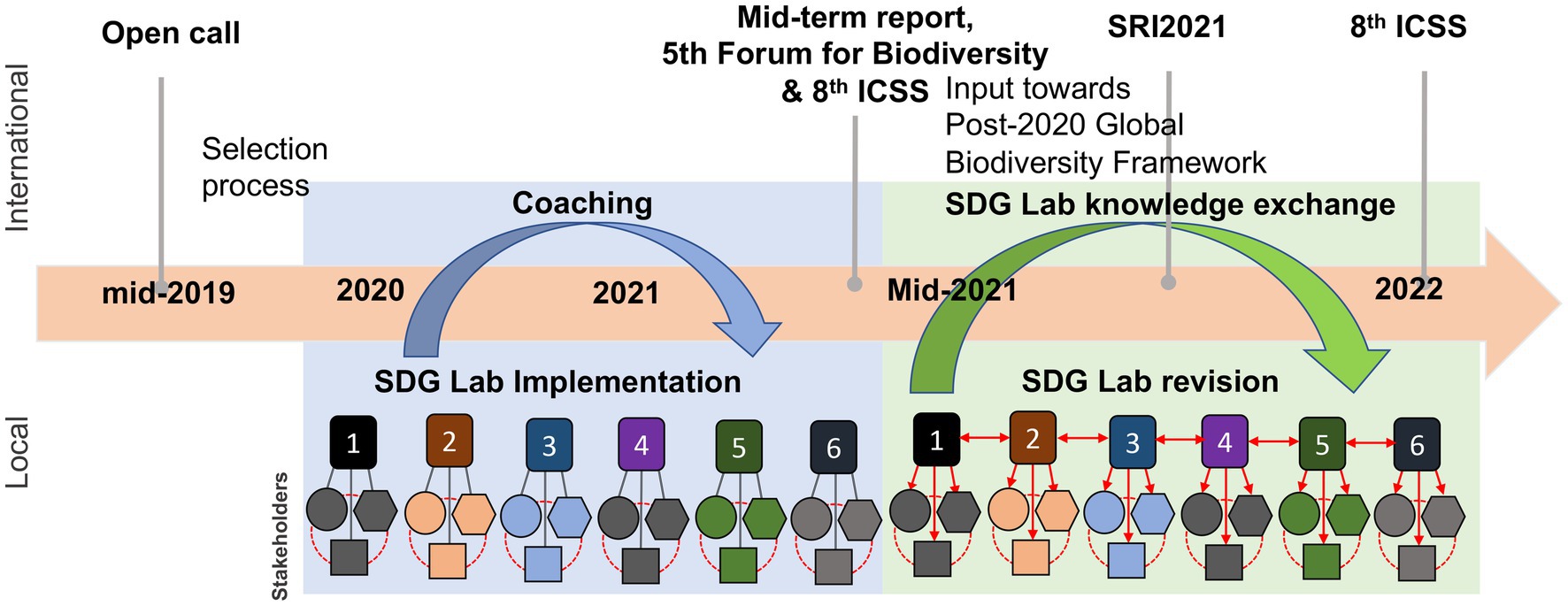
Figure 2. Design and implementation processes of the SDG-Labs. For visualization purposes the main arrow length (in pink) is not proportional to the duration of each activity/process.
The originally expected implementation period was January to September 2020, but due to difficulties posed by the COVID-19 pandemic (including the postponement of the 8th ICSS in person in Kunming, China), the implementation of the SDG-Labs was extended up to October 2021. Each SDG-Lab individually negotiated extension periods to allow for sufficient time to complete their activities.
2.2. Data collection and analysis
In this study we provide a comparative synthesis of the main characteristics of the six SDG-Labs, in terms of their (a) thematic focus, (b) transdisciplinarity, (c) outcomes, and (d) lessons learned.
For (a) we summarise the focus and objectives of each SDG-Lab (Section 3.1). For (b) we track the types of stakeholders involved in each SDG-Lab and the methods used for stakeholder engagement (Section 3.2). For (c) we track the characteristics of the biodiversity-based solutions in each SDG-Lab, as well as their beneficiaries, contributions to food systems, climate co-benefits and broader sustainability outcomes (Section 3.3–3.4). For (d) we track the advantages and disadvantages of the SDG-Lab approach through a Strengths-Weaknesses-Opportunities-Threats (SWOT) analysis (Ioppolo et al., 2013; Section 3.5). In the SWOT analysis the Strengths and Weaknesses refer to the internal characteristics of the SDG-Labs technique itself as an approach for developing sustainability solutions, while the Opportunities and Threats refer to the wider system characteristics that support or hinder the design and implementation of SDG-Labs for local sustainable transformations (Longsheng et al., 2022).
For (a)-(c) the information is reported for each SDG-Lab individually in appropriate figures and tables. For (d) the SWOT analysis was conducted first for each SDG-Lab and then synthesized for all six labs to identify general patterns.
The reported information is elicited through the critical analysis of the interim and final reporting documents of each SDG-Lab, as well as the insights of the main organisers of each SDG-Lab and the entire process that serve as co-authors in this paper. In particular, AA coordinated the SDG-Lab Armenia, JL coordinated the SDG-Lab China, JK coordinated the SDG-Lab Thailand, BC coordinated the SDG-Lab Japan, R.N.N.A coordinated the SDG-Lab Madagascar, and IO coordinated SDG-Lab Uganda. MJ coordinated the overall SDG-Lab process through the University of Tokyo.
3. Results
3.1. Thematic focus and objectives of the SDG-Labs
Table 1 outlines the thematic focus and objectives of the six SDG-Labs that were finally implemented. It becomes obvious that although all of them mobilized biodiversity as a source of solutions for sustainable food systems, they did so from radically different angles.
The SDG-Lab in Armenia was named “Biodiversity in Action: Accelerating data digitization for innovative cropping systems.” It was led by an academic team and had a two-fold objective. First, was to develop an innovative cropping system based on inter-, over-, and cover-cultivation to increase yield stability (by up to 30%), agroecosystem quality, and sustainability. Second was to enhance the conservation and sustainable use of wild endangered medicinal plant species through innovative “on farm” conservation.
The SDG-Lab in China was named “Forestry-tea ecosystem services and influence on local livelihoods: The case of tea forests in Yunnan, China.” It was led by an academic team and had two main objectives, namely to (a) develop forestry-tea garden production models to conserve biodiversity, and (b) to use efficiently natural resources and achieve good yields in terms of quantity and quality.
The SDG-Lab in Japan was named “Conserving biodiversity of homestead windbreaks and home gardens for food security and rural development.” It was led by an academic team and had two objectives. First, was to raise local community awareness about the significance of homestead windbreaks. Second, was to maintain homestead windbreaks and sustain their home garden production for food security in remote island regions (Okinawa, Japan).
The SDG-Lab in Madagascar was led by a practitioner and was named “Immersive ecotourism in Tampolo Protected Area towards sustainable development.” It sought to develop immersive ecotourism activities by connecting visitors with local biodiversity.
The SDG-Lab in Thailand was titled “Promoting traditional agroforestry farming systems for sustainable watershed forest management in the north-eastern region of Thailand.” It was led by a researcher and a practitioner and had two main goals. First, was to explore and demonstrate the potential of traditional agroforestry farming systems for degraded land rehabilitation and management for communities living inside a national park. Second was to improve the livelihoods and food security of the local communities.
Finally, the SDG-Lab in Uganda was led by a practitioner and was titled “Establish the role of the Karimojong Nomadic Indigenous Pastoralist in the conservation and sustainable use of biodiversity.” Its focus was to establish how pastoralists contribute to the sustainability of semiarid ecosystems and the sustainable use of biodiversity.
3.2. Transdisciplinary engagement across the SDG-Labs
A central part of each SDG-Lab was the conscious effort to mobilise and integrate the perspectives of multiple stakeholders from local communities, businesses/private sector, local government, civil society, and academia/research in the design, testing and implementation of the biodiversity-based solutions. Table 2 suggests that almost all SDG-Labs involved at least one stakeholder from each of these stakeholder categories. The only exceptions were the SDG-Lab from Thailand and China that did not involve any stakeholder from the private sector and the local government, respectively. On average, each SDG-Lab engaged >10 stakeholders. The most commonly engaged stakeholder group were local community members (n = 16), which is to be expected considering the very local focus of SDG-Labs, and the fact that most of the solutions were geared towards local communities. In order of declining prevalence, the other stakeholders involved in the SDG-Labs came from the businesses/private sector (n = 13), local governments (n = 12), civil society (n = 12), and academia/research (n = 11; Table 2).
However, the relative mix of these stakeholders differed between SDG-Labs (Table 2). For example, in the SDG-Lab Armenia, most of the engaged stakeholders came from civil society (33.3%), while in SDG-Lab China came from the private sector/businesses (46.2%). The representation of stakeholder types in SDG-Lab Uganda and Japan was relatively even (though low in absolute numbers, Table 2), and included stakeholders coming from all five categories. Stakeholder representation in other SDG-Labs such as China, Madagascar and Thailand were higher (Table 2), but also more unevenly distributed.
The engagement method for the different stakeholders also varied among the six SDG-Labs. In particular, there was a large variation between SDG-Labs in the type of the participatory approaches employed to engage stakeholders for the co-design and co-development of the solutions (Table 3). The specific participatory approaches included: (a) online and offline interviews; (b) onsite workshops and training; (c) online workshops, (d) field research (forest inventory, plant species count, etc., including a participatory field research), and (e) laboratory experiments.
Similar, to the stakeholder groups, the different SDG-Labs mobilized different participatory processes to engage with stakeholders. For example, while overall the six SDG-Labs applied 6 types of approaches, the SDG-Labs of China and Uganda employed each a total of five participatory methods, and the other SDG-labs employed fewer participatory approaches (Table 3).
The above suggest that although all SDG-Labs adopted highly transdisciplinary approaches, they did not necessarily follow the same template in terms of engagement approaches or data collection/analysis methods. What was important instead across all labs was to select the most appropriate stakeholders and participatory methods to reflect the local problem, needs, and social-ecological system characteristics. For example, the SDG-Labs in Uganda and China adopted many participatory approaches to engage stakeholders in a relatively balanced manner. These SDG-Labs applied interviews onsite and online, workshop online, and field research. Instead, SDG-Labs in Armenia, Madagascar and Thailand tried to adopt different participatory approaches, but with one of them being more dominant (e.g., onsite interviews). Finally, SDG-Lab Japan adopted relatively fewer participatory processes, but with some degree of balance between them, focusing on interviews, field research, and workshops.
3.3. Contribution of the SDG-Labs in the biodiversity-food-climate nexus
The six SDG-Labs have a good variability in terms of ecosystem focus and biodiversity-based solutions (Table 4). In terms of the biodiversity component used for the solutions, species diversity was the most common, and was present across all six SDG-Labs (Table 4). Diversified production systems were also prevalent including diversified cropping systems (e.g., Armenia), diversified agroforestry systems (e.g., Thailand), and diversified home garden (e.g., Japan). Conversely ecosystem diversity and genetic diversity were less utilized in the biodiversity-based solutions (Table 4). In terms of the food system focus, although some SDG-Labs did not target food production systems (e.g., Madagascar), all biodiversity-based solutions contributed to local communities’ food and nutrition security. This was mainly through enhanced food availability (e.g., increased or more stable food production) and/or increased food accessibility due to increased income (Table 4). Interestingly, although it was not part of the Open Call (Section 2.1), all SDG-Labs identified climate change as an inherently cross-cutting issue in this interface of biodiversity and food systems. It is worth noting that three SDG-Labs specified their solutions as NbS for climate change mitigation and/or adaptation (Table 4). In this sense, all SDG-Labs contribute to the biodiversity-food-climate nexus in different extent. Below we summarise in detail the biodiversity-based solution for each SDG-Lab and how it contributes to the biodiversity-food-climate nexus (Table 4).
In SDG-Lab Armenia, the biodiversity-based solution aimed at enhancing local food availability through a diverse mixed-species cropping system that generated in the process income for improving farmers’ food accessibility (via the sales of endangered medicinal plant to tea making companies or other local markets) and enabled the conservation and sustainable use of wild endangered medicinal plant biodiversity. This latter was achieved through innovative “on farm” conservation approaches. This diversified agro-ecosystem essentially helped to raise the resilience of farmlands toward climate change, which is significant in the Ararat region which was the geographical focus of this SDG-Lab.
In SDG-Lab China, the biodiversity-based solution focused towards conserving biodiversity through mixed-species tree and tea culture through forestry-tea garden models. It was expected that the development of such forestry-tea gardens led to higher yields and better quality of tea, which eventually would generate higher income for tea farmers and employees in the tea industry to improve food accessibility. In addition, the increased abundance and coverage of trees in the forestry area provides shade for the tea plants, reduce temperature and absorb dust, which collectively enhance the adaptation of tea production to the impact of global warming.
In the SDG-Lab Japan the biodiversity-based solution was aimed at creating homestead windbreaks in traditional village landscapes. These were created outside residences and in home gardens inside residences through the conservation of local biodiversity. On the one hand, home gardens with diverse species of leafy vegetables, roots and fruit trees enhances food availability for local residents for self-consumption. Considering that this solution was implemented in a chain of isolated islands, a home garden for local food provision could further reduce the substantial GHG emission from importing food from the mainland. On the other hand, the previously planted homestead windbreaks around the residences were used as a NbS to protect the house from strong typhoons and monsoonal winds during the winter. It was also estimated that homestead trees planted in an orderly line have a high potential for biomass accumulation, carbon sequestration, climate change mitigation and adaptation.
In SDG-Lab Madagascar, the biodiversity-based solution related to utilizing local biodiversity for ecotourism and a series of related ancillary activities. In this case the benefits of the rich local biodiversity (including aquatic and terrestrial) were leveraged by increasing local awareness (including from business and households) about the value of the biodiversity that could be appreciated by tourists, and how it could be translated into income generation for improving food accessibility and alleviating poverty. Furthermore, restoration activities in the protected area will further restore terrestrial and aquatic biodiversity but also increase the potential of carbon storage for climate change mitigation.
In the SDG-Lab Thailand the biodiversity-based solution related to enhancing species diversity in watershed forests, community forests, illegal logging areas and degraded forest areas near local communities through traditional agroforestry farming system, e.g., planting native food crop species and local tree species. This system can increase local food availability as well as food accessibility through income generation from marketable products such as native food plant species like Pak Wan. In the meantime, the promotion of traditional agroforestry farming systems in watershed forests was perceived by local villagers as a NbS for water regulation and drought mitigation, especially during the dry season, which helped local climate change adaptation.
In the SDG-Lab Uganda, the biodiversity-based solution related to the promotion of indigenous pastoral systems that had multiple benefits, including biodiversity conservation. In this SDG-Lab different biodiversity components were used namely genetic diversity (e.g., locally adapted livestock breeds and crop varieties), species diversity (e.g., grass species diversity), and ecosystem diversity (e.g., habitat diversity). This sustainable pastoralism model relying on the different components of local biodiversity enhances, on the one hand, the food availability of pastoralists and generates income from the sales of livestock products that can increase food accessibility. On the other hand, the indigenous knowledge of species, ecosystems and climate were used by the pastoralists to better adapt the pastoral system to disease outbreaks, droughts, and climate change. Furthermore, it was also observed that the vegetation maintained through grazing activities could in turn store carbon, which had positive ripple effects for climate change mitigation.
3.4. Broader sustainability benefits of the SDG-Labs
Figure 3 shows the contribution of the six SDG-Labs to the different SDGs. As discussed in Section 3.3, given their approach of developing biodiversity-based solutions through conservation and sustainable use, all SDG-Labs could contribute to “Life on Land” (SDG15), and some to “Life below Water” (SDG14; i.e., Japan, Madagascar, Thailand; Table 4). Here it is worth noting that by protecting the watershed, the SDG-Lab in Thailand could also contribute to “Clean water and sanitation for all” (SDG6). Furthermore, as climate change was perceived as a cross-cutting challenge in practically all SDG-Lab contexts (Section 3.3), the proposed solutions could also have a positive effect for “Climate Action” (SDG13) via offering opportunities for climate change mitigation and/or adaptation (Table 4). This suggests that the SDG-Labs contributed substantially to all environment-related SDGs, and especially SDG15 and SDG13.
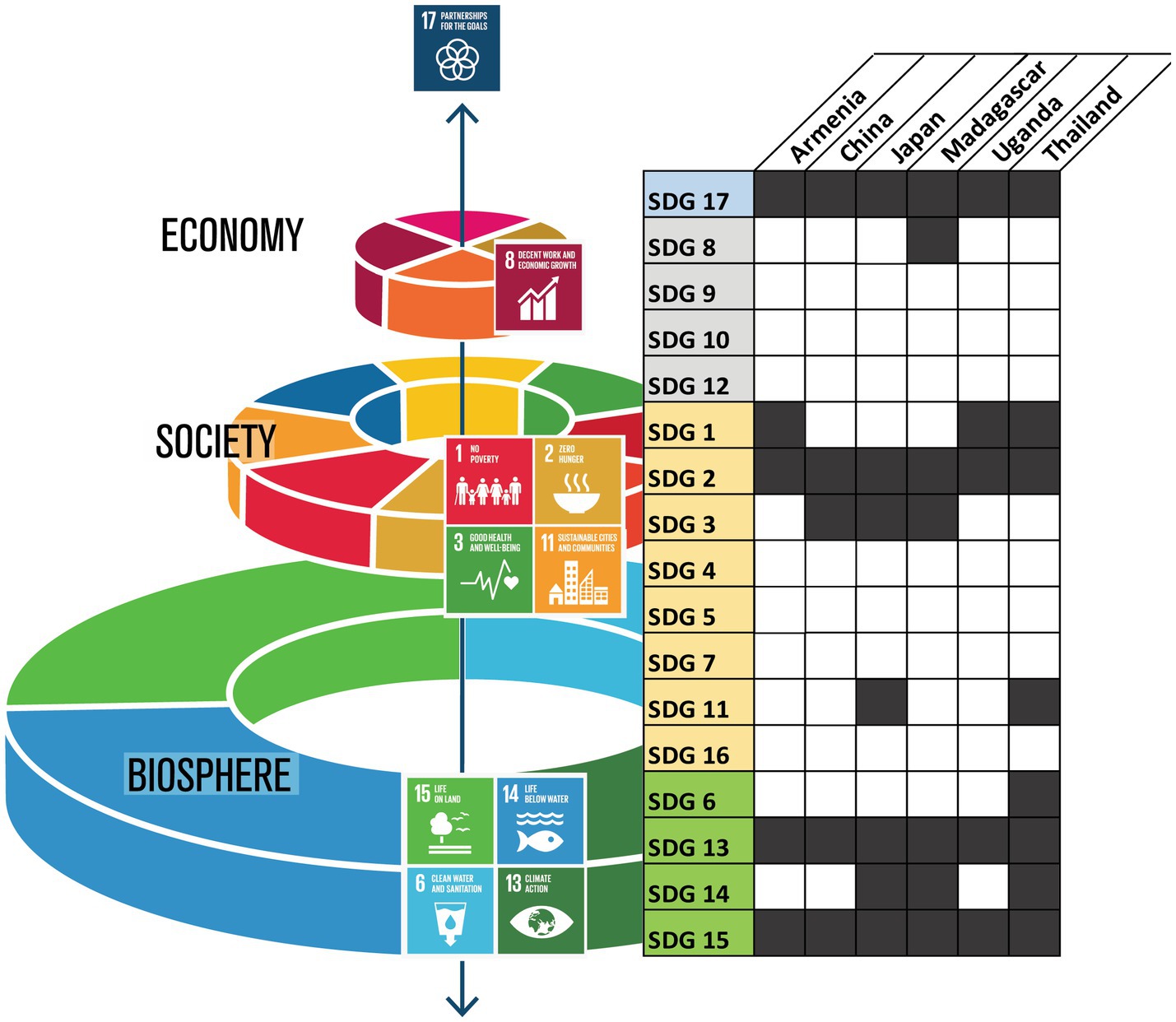
Figure 3. Broader sustainability contributions of each SDG-Lab. The division of SDGs across the three thematic categories (i.e., biosphere, society, economy) follows Rockström and Sukhdev (2016).
When it comes to social SDGs, as the focus of the biodiversity-based solutions was to enhance the sustainability of food systems (Table 4), all SDG-Labs contributed to “Zero Hunger” (SDG2). However, all SDG-Labs contributed to at least one more SDG. For example, the China, Japan, and Madagascar SDG-Labs contributes to “Good Health and Well-being” (SDG3). Air pollution in Madagascar could be prevented by the proposed ecotourism-driven forest conservation and restoration. By conserving densely planted Fukugi trees (Garcinia subelliptica) around homes and alleys, the SDG-Lab in Japan promotes a solution to heatwaves. Conversely the SDG-Labs in Uganda, Madagascar, and Thailand contributed to “No Poverty” (SDG1). Poverty alleviation effects in the Uganda SDG-Lab were expected through livelihood improvements via improving use of pastoralist knowledge systems on managing and using the diverse vegetation of semi-arid ecosystems. In the Madagascar SDG-Lab, poverty alleviation effects for the local community were expected through income generation via ecotourism, whereas in the Thailand SDG-Lab via the enhanced capacity of local communities for sustainable forest management and biodiversity conservation. Finally, the SDG-Labs in Japan and Thailand are expected to contribute to “Sustainable cities and communities” (SDG11). In Japan, this contribution was expected through building the community value among new residents in the area who do not know the local traditions and have no social network; and among the new generation who had lost the connection with local traditions and biodiversity. The SDG-Lab project provided a space for communal and intergenerational interactions and rediscovery of the use of forementioned Fukugi Tree (Garcinia subelliptica), as a natural wind shield and shade, and source of dyeing material and food additive. Conversely, in the SDG-Lab, the contributions to SDG11 were expected through the support to local communities’ reliance on sustainable practices such as agroforestry, with engagement in forest rehabilitation.
However, the SDG-Labs had a much lower potential to tackle economy-related SDGs (Figure 3). Only the Madagascar SDG-Lab had such a potential, and only for “Decent work and economic growth” (SDG8). The expected pathway to contribute to SDG8 was via enhancing self-employment in immersive ecotourism, as well as supporting local sustainable tourism-related businesses.
Finally, all projects can contribute to “Partnership for the goals” (SDG17; Figure 3). As outlined in Section 3.2 very diverse stakeholders participated in all six SDG-Lab projects in order to co-design and implement the proposed biodiversity-based solutions for sustainable food systems.
Overall, each SDG-Lab can potentially contribute to multiple SDGs. However, the focus and approaches can vary substantially. This shows the very flexible nature of these transdisciplinary processes in terms of their ability to tackle multiple sustainability challenges through locally-driven solutions.
3.5. Strengths and weaknesses of the SDG-Labs
Overall, the SWOT analysis suggests that the methodology and design/implementation of the SDG-Labs had diverse strengths and weaknesses, while the prevailing local and national context offered various opportunities and threats (Table 5). The major identified strengths related to ability of the SDG-Labs to provide a platform for knowledge generation and dissemination for food production and biodiversity conservation, as well as an opportunity to generate income and/or food. Collectively these had several positive ripple effects as outlined in Table 4. In more details, SDG-Labs offered an opportunity to accumulate and disseminate local knowledge about the use of native plants as food sources (in Armenia, Japan, Uganda), and use local biodiversity of plants and animals as food and income sources (in Uganda, Japan, Thailand). In many SDG-Labs the local and national governments, as well as local communities members (especially farmers), showed an active interest in engaging and supporting biodiversity-based solution for food security, new cropping systems, and agrobiodiversity conservation (in SDG-Lab Armenia). Moreover, the SDG-labs in all communities increased the understanding of local communities about resource depletion and environmental deterioration (all SDG-Labs). Furthermore, in many local contexts the businesses and governments recognized the income generation potential of the sustainable practices encapsulated in the SDG-Labs as a big strength of these local transdisciplinary approaches (in SDG-Lab Thailand, SDG-Lab Madagascar, SDG-Lab Armenia, SDG-Lab China).
However, SDG-Labs also demonstrated a number of Weaknesses (Table 5). Although in some SDG-Labs local communities and farmers participated actively in the decision-making process, in other SDG-Labs farmers were less active as they were familiar only to a top-down implementation and were thus less determined to participate in bottom-up initiatives (e.g., in Madagascar). Another weakness of the SDG-Lab model related to the lower capacity of local farmers to harness modern technologies and/or to comprehend that some practices, despite offering higher profits, are essentially not sustainable (in Armenia, China, Thailand). Another weakness of the SDG-Lab model was the inability to engage properly the younger generation that lacked knowledge of local farming and ecosystem management practices (e.g., in Japan), which in the end prevented the revival of some local food production practices. In some cases the SDG-Lab was misaligned with other local interests and priorities, as for example stakeholders from the growing tourism industry and expanding plantations did not have local sustainability transformation high up on their priorities (e.g., in Madagascar). Finally, in some contexts local governments also had a limited capacity for environmental policy enforcement, which generated certain difficulties in the design and implementation of some SDG-Labs, e.g., through ongoing ecosystem degradation in the surrounding areas (in SDG-Lab Madagascar, SDG-Lab Uganda).
The most apparent Opportunities for SDG-Labs were the amenable environment created from some of the main stakeholders (Table 5). For example, the growing tourism sector in some countries/localities encouraged the rediscovery and preservation of local food and biodiversity (in Japan, Thailand, Madagascar, China). Such a case was the SDG-Lab in Madagascar that benefited from (a) the growing demand from the national market for locally and sustainably produced food (which provided a strong incentive for local food production), and (b) the alignment with the interests of other funders (which provided an opportunity to replicate the showcased model for local change in other geographical contexts). In almost all SDG-Labs (with the exception of Madagascar), there was a notion that the SDG-Labs benefited from existing environmental policies and central government initiatives that recognized the value of local agriculture and ecosystems. Finally, in most SDG-Labs the interest of research and academic organizations in conducting research on food security and biodiversity conservation, was seen major opportunity, benefiting the SDG-Lab implementation teams (in Armenia, China).
Finally, all SDG-Labs encountered a number of negative context-specific circumstances that acted as Treats (Table 5). For example, in SDG-Lab Madagascar, the prevailing policies had an insufficient alignment with the SDGs, which was perceived as hindering the design, implementation and uptake of the SDG-Lab. In SDG-Lab Uganda, despite the reportedly amenable environmental policies that facilitated the groundwork for the implementation of the project, their weak implementation posed certain challenges. Furthermore, the competition for land and labour with other industries such as mining, created a major challenge in acquiring a critical mass of people to enable the sustainability transformation through the SDG-Lab (in Uganda). Similar threats were identified in SDG-Lab Japan, where the lack of effective policies to support the younger generation to stay in the area and work on sustainable transitions (including local food production) was perceived to be a major threat to implementing the biodiversity-based solutions identified through the SDG-Lab.
4. Discussion
4.1. SDG-Labs as transdisciplinary solution spaces
Our SDG-Labs drew upon the concept of Living Labs (Section 1), which have been used for the transdisciplinary design and implementation of sustainable solutions in different thematic and geographical contexts (Lupp et al., 2020). The experiences from the six SDG-Labs reported here demonstrate in practice the application of participatory and multi-stakeholder transition governance models (Nevens et al., 2013; Schäpke et al., 2018), with a particular focus on biodiversity-based solutions for sustainable food systems (Section 3.3). While there is an emerging (but still limited) literature on comparative studies about the performance and effectiveness of Living Labs (see Hossain et al., 2019; Kok et al., 2021), here we elaborate how such transdisciplinary approaches are able to facilitate the creation of models for local seeds of transformation using biodiversity as the “source” of the solution.
Overall, the six SDG-Labs outlined in this study had very diverse thematic foci in terms of the proposed and/or implemented biodiversity-based solution. These ranged from the promotion of traditional food systems that mobilized different components of biodiversity (e.g., Thailand, Japan Uganda) to the development of innovative food systems (e.g., Armenia, China) or ecotourism models (e.g., Madagascar; Section 3.1–3.2). Furthermore, equally wide was the scale within which these biodiversity-based solutions were expected to “operate,” that ranged from the level of the agroecosystem (e.g., Armenia, China) to the landscape level (e.g., Japan, Madagascar, Thailand), to the regional scale (e.g., Uganda). It is also interesting to note that all of the SDG-Labs did not only focus on food systems, but other interlinked challenges in the specific local contexts, including biodiversity loss (e.g., China), land use conversion (e.g., Thailand), or climate change (e.g., Uganda). Interlinkages of food systems challenges with other persistent sustainability challenges have been addressed in other Living Labs focusing on for example sustainable food-water-energy innovations (Offermans et al., 2020), wild food for health, food security and livelihoods (Pereira et al., 2022), and organic agriculture for soil health (Hvitsand et al., 2022).
Despite their thematic divergences, when looking at the sub-categorisation of McCrory et al. (2022), all SDG-Labs generally adopted a “make and relate” approach, as they sought to create social innovations, which were constructed and bound at the local level, but were also informal and self-organizing in nature (see Section 1 and Box S3, Supplementary material). Furthermore, they were highly transdisciplinary in the sense that each SDG-Lab during the solution design and implementation phases involved participants from four to five major stakeholder groups, including stakeholders from local communities, private sector, local government, academia, civil society, and other organizations (Section 3.2). On average, each SDG-Lab engaged over 10 stakeholders (Section 3.2), highlighting the ability of these processes to mobilise local interest about the development of sustainable solutions. In addition, the beneficiaries of these six SDG-Labs varied from solely local community beneficiaries (e.g., Thailand) to beneficiaries from a broader industrial sector (e.g., China) and even non-local beneficiaries such as tourists (e.g., Madagascar).
The implementation teams of the SDG-Labs were equally diverse, with some SDG-Labs led by practitioners (i.e., Madagascar and Uganda), others by academic researchers (i.e., Armenia, China and Japan), and some by joint teams (i.e., Thailand; Section 3.1). Transdisciplinarity was further enhanced through the overall design of SDG-Labs that allowed vertical and horizontal information exchange with international researchers in the form of coaching and presentations at international conference venues as well as between SDG-Lab groups (Section 2.1). Similarly, the SDG-Labs used very diverse techniques to design/implement the biodiversity-based solutions and engage with stakeholders (Section 3.2).
The above show clearly how SDG-Labs can follow very divergent approaches anchored on principles of transdisciplinarity (Lawrence et al., 2022). This further reflects the observation there is no universally applied approach for their implementation, but can be rather flexible in terms of methods and approaches, as long as they embrace a participatory and multi-stakeholder mindset to transition governance (Nevens et al., 2013; Schäpke et al., 2018) with a good balance between scientific and societal goals while addressing requirements, interests, and limits of practitioners (Bergmann et al., 2021).
However, we need to also point, although young people can play a critical role for sustainable food transformation and living labs (see Section 1), the six SDG-Labs moderately achieved to include youth as stakeholders. This does not come as a surprise as in many areas youth was simply underrepresented in the general population due to population ageing or migration (Section 3.5). Furthermore, many of the solutions were geared towards agriculture, where younger generations are generally less involved compared to their older cohorts (Christiaensen et al., 2021).
4.2. SDG-Labs as sources of multi-functional solutions
Beyond transdisciplinarity, another common characteristic of all SDG-Labs was the multifunctionality of the proposed biodiversity-based solutions. All six SDG-Labs had strong connections with multiple SDGs (Section 3.4) and potential for climate change adaptation and/or mitigation (Section 3.3).
In terms of SDGs linkages, the biodiversity-based solutions proposed by practically all SDG-Labs did not only contribute to the biosphere-related SDGs, but also to the society-related SDGs (Figure 3). In particular, while SDG15 and 14 (pertaining to life on land and water respectively) were by definition the main SDGs targeted by the proposed solutions, all SDG-Labs also contributed to SDG13 associated with climate change. Similarly, all SDG-Labs contributed to SDG2 on food, and many contributed to SDG3 on health. Finally, the highly transdisciplinary nature of all SDG-Labs (see Section 3.2 and 4.1), implies their possible positive ripple benefits towards the creation of partnership for the SDGs and strengthening locally the means of implementation for sustainable development (SDG17).
A very noteworthy observation was that climate change emerged as a strong cross-cutting theme in all SDG-Labs. This was largely unplanned at the Open Call level (see Section 2.1). In particular climate change featured in two main ways, as discussed below.
On the one hand, in the Armenia, Japan and Uganda SDG-Labs, climate change was perceived as an emerging threat for the food production systems and the wellbeing of the local communities. Therefore, the proposed and implemented biodiversity-based solutions sought to: (a) reduce the impact of climate change and extreme weather events (e.g., conservation of windbreaks: SDG-Lab Japan) or (b) improve the resilience and adaptive capacity of food systems (e.g., innovative cropping system: SDG-Lab Armenia; indigenous pastoralist system: SDG-Lab Uganda) and local communities (i.e., SDG-Lab Japan; Table 4). These actions contributed to enhancing local food availability through higher species diversity (Table 4), which in turn contributed to climate change mitigation via increased carbon sequestration (Section 3.3). Similar efforts to use biodiversity to combat climate change impacts on food production systems have been widely reported in the literature (Duarte et al., 2020; Henry, 2020; Ojea et al., 2020; Tschora and Cherubini, 2020; Dhyani et al., 2021; Miralles-Wilhelm, 2021). Particularly, some of the recent discussions about NbS have encouraged tackling societal challenges not only related to disaster risk reduction and climate change, but also food security and human health (Cohen-Shacham et al., 2016).
On the other hand, in some other SDG-Labs (i.e., China, Madagascar, and Thailand), climate change was not directly mentioned/identified as a major challenge but was inherently interconnected with the proposed and implemented solutions. For instance, the development of forestry-tea gardens in the SDG-Lab China could also help tea trees adapt to the rising temperature. Similarly in the SDG-Lab Thailand, the reforestation targeted native food tree species that have higher tolerance to drought, which could also help the local community and ecosystem adapt better to drought under climate change. The restoration activities in the protected areas of SDG-Lab Madagascar could also contribute to increasing carbon sequestration from restored native biomass (Table 4).
Such types of climate co-benefits are not an uncommon feature of biodiversity-based solutions for food systems. For example, the mobilization of neglected and underutilized crop species (or orphan crops) can enhance local food and nutrition security via agrodiversity conservation, and at the same time help build the resilience of local communities and agricultural systems due to their better adaptation to challenging climatic conditions (Mabhaudhi et al., 2019). Similarly conserving agrobiodiversity for sustainable food systems in the Hindu Kush Himalaya has been argued to provide a good basis for achieving food security and agricultural sustainability, as well as for adapting to climate change (Rasul et al., 2022).
Finally, when looking critically at the biodiversity-based solutions and their design/implementation approaches, beyond their multi-functionality, most SDG-Labs followed multiple pathways to the targeted impact. For example some biodiversity-based solutions aimed at creating societal impact via building individual capacity (e.g., capacity building workshops in SDG-Lab Armenia; re-discovering the value of homestead windbreaks and home gardens in SDG-Lab Japan). Other SDG-Labs sought to create impact via usable products or innovative production models (e.g., tree-garden cultivation systems in SDG-Lab China; mixed-crop cultivation systems and medicinal plants in SDG-Lab Armenia). Other SDG-Labs sought to create impact through novel institutional arrangements (e.g., “immersive tourism model” in SDG-Lab Madagascar). Other SDG-Labs sought to restore traditional systems (e.g., traditional agroforestry farming system for degraded land rehabilitation and management in SDG-Lab Thailand; indigenous pastoralism for ecosystem conservation in SDG-Lab Uganda; homestead windbreaks in SDG-Lab Japan). Finally, practically all SDG-Labs sought to create impact by building networks and relationships considering their highly transdisciplinary nature (Section 3.2).
4.3. Lessons learned and future research directions
When looking critically the SWOT analysis (Section 3.5) and other observations from the design and implementation of the SDG-Labs (both individually and collectively), it is possible to identify some key lessons learned to improve chances of success of future similar initiatives. These lessons relate to the (a) knowledge/capacity/willingness of different stakeholders and community buy-in to engage in transdisciplinary bottom-up processes, (b) productive engagement of the private sector, (c) underlying institutional environment and implementation.
A factor that seemed to benefit greatly the design and implementation of several SDG-Labs was the accumulated body of knowledge in the local communities about the use of local resources and ecosystems. This included for example plant species (SDG-Lab Japan) or livestock and rangelands (SDG-Lab Uganda). It was also encouraging that the participating farmers, local government agencies, and even businesses in most SDG-Lab locations were aware of resource scarcity and environmental degradation, which created a good basis to initiate these activities. Furthermore, many local communities and other stakeholders were already interested in joining forces in actions towards achieving locally important goals such as food security, improved agricultural production, income generation or biodiversity conservation. On many occasions the local residents had some level of capacity and willingness to participate in these bottom-up processes and the overall decision-making process. Conversely, in some SDG-Labs local communities were aware of resource degradation and were willing to participate, but their capacity to initiate/engage in bottom-up initiatives or harness modern technologies were limited, which hindered the implementation of some of the innovations (e.g., SDG-Lab in Armenia). Similarly in some cases local governments also had limited capacity for the enforcement of sustainability policies due to different context-specific reasons such as the decreasing reliance of local communities on local food compared to imported food (SDG-Lab Japan). This existing knowledge/capacity of local communities and buy in/willingness to participate in local actions has been identified as a major factor of success or failure in other sustainability-oriented labs (Bergmann et al., 2021) and more broadly transdisciplinary research (Lawrence et al., 2022). Awareness of this capacity and buy-in could potentially help to identify promising locations to implement such approaches targeting local sustainability transformation.
A second factor benefiting some SDG-Labs was the productive engagement of local businesses. In particular, some of the proposed sustainable practices were recognized to be new potential opportunities to generate profits, especially in relation to tourism and local food production (e.g., SDG-Labs in Madagascar and China). For example, several of the processes in favor of capitalizing on local actions were driven by the growing tourism sector in Madagascar that seeks the rediscovery and preservation of local food and biodiversity, leading to growing demand from the national market for local and sustainably grown food. Conversely, in some cases the private sector played knowingly or unknowingly an unproductive role for the implementation of some SDG-Labs. For example, the conventional tourism industry, expanding plantations or mining industries operating in the broader areas did not have sustainability on their agenda, and often acted as better income opportunities especially for young people, reducing to some extent local interest for engaging in SDG-Labs (e.g., in Uganda and Madagascar). Many studies have discussed how buy-in from the private sector can be an important factor for facilitating local sustainability transformation, considering its capacity to mobilise funds and political support (Schmidt et al., 2013; Masuda et al., 2022). However, as has been pointed in other sustainability-oriented labs and transdisciplinary processes it is important to ensure that the engagement of the private sector does not trample other local needs and sensibilities, considering the generally high power of such stakeholders (Di Maddaloni and Sabini, 2022).
A third factor that facilitated the design and implementation of some SDG-Labs were the existing environmental policies and the general recognition of national and local governments about the value of local agriculture and ecosystems (e.g., SDG-Labs in Armenia, China, and Thailand). This often helped to promote sustainable farming systems with climate change and biodiversity conservation as the main goal. However, there were also cases where the persistently not firm enforcement of national environmental policies or the lack of policies aligned with the SDGs had a negative impact on the SDG-Labs. For example, this took a toll through continuing deforestation (SDG-Lab Madagascar) and ongoing illegal mining (SDG-Lab Uganda), which threatened biodiversity. Here we need to re-iterate that conducive institutional environments have been identified as an important factor affecting the success or underperformance of sustainability-oriented labs, and transdisciplinary research processes more generally (Bergmann et al., 2021).
In terms of future research, despite their diverse thematic foci on biodiversity-based solutions for food systems, all six SDG-Labs were implemented in rural settings, and mostly revolved around food production. However, there is a growing experience of sustainability-oriented labs in the context of peri-urban areas (Kok et al., 2019) or urban food system transformation (Brons et al., 2022), though few that actually develop biodiversity-based solutions. It is worth noting that biodiversity and other food system components are also closely interlinked, such as food consumption (Crenna et al., 2019) or transportation of agricultural products (Puppim de Oliveira et al., 2011). Future SDG-Labs related to food systems can further explore possible opportunities to develop and leverage biodiversity-based solutions in different component of food systems and urban or peri-urban settings.
Furthermore, species diversity was the main biodiversity component leveraged in practically all SDG-Labs, with other components such as genetic diversity only considered in few cases (Section 3.3; Table 4). Genetic diversity plays a substantial role for ecosystem resilience and maintaining species diversity (Hoban et al., 2020), and although crucial for food security, it is being lost at an alarming rate (Oliver et al., 2015). Even though genetic diversity was a smaller focus among the six SDG-Labs, it can arguably to provide solutions to enhance resilience to climate change and achieve food security goals (Wani et al., 2022), including from crops (Mujeeb-Kazi et al., 2013; Begna, 2021), livestock (Mottet et al., 2018) and aquatic food (Lind et al., 2012). At the same time there have been calls for more attention to genetic diversity (Hoban et al., 2020) including through dedicated goals in the recent post-2020 Global Biodiversity Framework (CBD, 2021). In this respect, future sustainability-oriented labs could develop possible biodiversity-based solution for food systems leveraging on genetic diversity.
5. Conclusion
In this paper we reported the experiences and lessons learned from the implementation of six SDG-Labs that developed biodiversity-based solutions as a means of transforming food systems. The results show that these transdisciplinary and solutions-oriented techniques can follow very diverse approaches and use very different biodiversity components to target equally diverse and context-specific sustainability challenges of local food systems. Furthermore, and depending on the context, all SDG-Labs followed quite different approaches when designing the biodiversity-based solutions and engaging with relevant stakeholders. This suggests that there is no universally applicable approach for the design and implementation of SDG-Labs. Instead, what is important is to embrace actively and unreservedly a solutions-oriented mentality and a willingness to engage meaningfully diverse local stakeholders during the design, implementation and eventual uptake of the solutions.
Through the SWOT analysis we synthesized the experiences and lesson learned from all six SDG-Labs. Some of the Strengths include knowledge accumulation in local communities regarding the use of local plants for food or an increase in farmer awareness about resource scarcity and environmental degradation. Opportunities include broader market developments, for example, on the tourism sector or on alternative food markets for locally and sustainably produced food. Conversely some of the most important Weaknesses include the low initiative of farmers to engage in bottom-up activities (as they are often used to top-down project implementation), the low capacity of local farmers to harness modern technologies, and the lack of knowledge among young generations on local farming and other traditional practices. Commonly mentioned Threats include the weak implementation of environmental policies at different levels, and the fact that many policies are not yet well aligned with SDGs.
Collectively, the synthesis exercise reported in this paper (as well as the broader experience from the entire process of the SDG-Labs) suggests the great promise of this type of transdisciplinary research approach for developing solutions at the biodiversity-food-climate nexus. However, our accumulated experience also points to the very diverse and context-specific challenges that must be overcome to maximize the potential of SDG-Labs to both enable the sustainable transformation of local food systems or be scaled up effectively in other geographical and/or thematic contexts.
Data availability statement
The original contributions presented in the study are included in the article/Supplementary material, further inquiries can be directed to the corresponding author.
Author contributions
MJ: conceptualization, methodology, results, and writing. AG: conceptualization, structuring, discussion, editing, and writing. OS and TE: conceptualization. JS: analysis, writing, and editing. AA, JL, JK, BC, RA, and IO: coordination and results of SDG-Labs. GS and RL: discussion. All authors contributed to the article and approved the submitted version.
Funding
The Alliance for Global Sustainability (AGS) Endowment fund at the University of Tokyo supported SDG-Labs. MJ, BC, and AG acknowledge the financial support of the Japan Society for the Promotion of Science (JSPS) through the Grant-in-Aid for Scientific Research (C), project 23K11530 “Aging and shrinking population-driven transition of the nature conservation (ASP-NC)”. AG acknowledges the financial support of the Japan Science and Technology Agency (JST) through the AJ-Core programme (Project FORENS, JPMJSC20A3).
Acknowledgments
The general SDG-Lab approach was designed during the 7th ICSS (Stockholm, 2018). We acknowledge the inspiration of the organising team of this event.
Conflict of interest
The authors declare that the research was conducted in the absence of any commercial or financial relationships that could be construed as a potential conflict of interest.
Publisher’s note
All claims expressed in this article are solely those of the authors and do not necessarily represent those of their affiliated organizations, or those of the publisher, the editors and the reviewers. Any product that may be evaluated in this article, or claim that may be made by its manufacturer, is not guaranteed or endorsed by the publisher.
Supplementary material
The Supplementary material for this article can be found online at: https://www.frontiersin.org/articles/10.3389/fsufs.2023.1144506/full#supplementary-material
Footnotes
1. ^There is a wide variety of real-life experiments including “niche experiments,” “bounded socio-technical experiments,” “grassroots experiments,” “transition experiments,” and “sustainability experiments.” Box S1 in the Supplementary material provides a brief introduction and distinction between these concepts.
References
Aernouts, N., Maranghi, E., and Ryckewaert, M. (2020). Towards a definition of socially-oriented LivingLabs, Brussels: Soholab, p, 163.
Begna, T. (2021). Role and economic importance of crop genetic diversity in food security. Int. J. Agric. Sci. Food Technol. 7, 164–169. doi: 10.17352/2455-815X.000104
Béné, C., Oosterveer, P., Lamotte, L., Brouwer, I. D., de Haan, S., Prager, S. D., et al. (2019). When food systems meet sustainability – current narratives and implications for actions. World Dev. 113, 116–130. doi: 10.1016/j.worlddev.2018.08.011
Bergmann, M., Schäpke, N., Marg, O., Stelzer, F., Lang, D. J., Bossert, M., et al. (2021). Transdisciplinary sustainability research in real-world labs: success factors and methods for change. Sustain. Sci. 16, 541–564. doi: 10.1007/s11625-020-00886-8
Brons, A., van der Gaast, K., Awuh, H., Jansma, J. E., Segreto, C., and Wertheim-Heck, S. (2022). A tale of two labs: rethinking urban living labs for advancing citizen engagement in food system transformations. Cities 123:103552. doi: 10.1016/j.cities.2021.103552
Bulkeley, H., and Castán Broto, V. (2013). Government by experiment? Global cities and the governing of climate change: government by experiment? Trans. Inst. Br. Geogr. 38, 361–375. doi: 10.1111/j.1475-5661.2012.00535.x
Bulkeley, H., Coenen, L., Frantzeskaki, N., Hartmann, C., Kronsell, A., Mai, L., et al. (2016). Urban living labs: governing urban sustainability transitions. Curr. Opin. Environ. Sustain. 22, 13–17. doi: 10.1016/j.cosust.2017.02.003
CBD (2006). Article 2. Use of terms. Available at: https://www.cbd.int/convention/articles/?a=cbd-02 (Accessed January 4, 2023).
CBD (2021). First draft of the post-2020 global biodiversity framework, CBD/WG2020/3/3, Montreal. Available at: https://www.cbd.int/doc/c/abb5/591f/2e46096d3f0330b08ce87a45/wg2020-03-03-en.pdf (Accessed January 7, 2023).
Chausson, A., Turner, B., Seddon, D., Chabaneix, N., Girardin, C. A. J., Kapos, V., et al. (2020). Mapping the effectiveness of nature-based solutions for climate change adaptation. Glob. Chang. Biol. 26, 6134–6155. doi: 10.1111/gcb.15310
Christiaensen, L., Rutledge, Z., and Taylor, J. E. (2021). Viewpoint: the future of work in agri-food. Food Policy 99:101963. doi: 10.1016/j.foodpol.2020.101963
Cohen-Shacham, E., Walters, G., Janzen, C., and Maginnis, S. (2016). Nature-based solutions to address global societal challenges. Gland, Switzerland: IUCN International Union for Conservation of Nature.
Córdoba Vargas, C. A., Hortúa Romero, S., and León Sicard, T. (2020). Key points of resilience to climate change: a necessary debate from agroecological systems. Clim. Dev. 12, 564–574. doi: 10.1080/17565529.2019.1664376
Crenna, E., Sinkko, T., and Sala, S. (2019). Biodiversity impacts due to food consumption in Europe. J. Clean. Prod. 227, 378–391. doi: 10.1016/j.jclepro.2019.04.054
Crippa, M., Solazzo, E., Guizzardi, D., Monforti-Ferrario, F., Tubiello, F. N., and Leip, A. (2021). Food systems are responsible for a third of global anthropogenic GHG emissions. Nat. Food 2, 198–209. doi: 10.1038/s43016-021-00225-9
Crist, E., Mora, C., and Engelman, R. (2017). The interaction of human population, food production, and biodiversity protection. Science 356, 260–264. doi: 10.1126/science.aal2011
Dawson, I. K., Powell, W., Hendre, P., Bančič, J., Hickey, J. M., Kindt, R., et al. (2019). The role of genetics in mainstreaming the production of new and orphan crops to diversify food systems and support human nutrition. New Phytol. 224, 37–54. doi: 10.1111/nph.15895
Dhyani, S., Murthy, I. K., Kadaverugu, R., Dasgupta, R., Kumar, M., and Adesh Gadpayle, K. (2021). Agroforestry to achieve global climate adaptation and mitigation targets: are south Asian countries sufficiently prepared? Forests 12:303. doi: 10.3390/f12030303
Di Maddaloni, F., and Sabini, L. (2022). Very important, yet very neglected: where do local communities stand when examining social sustainability in major construction projects? Int. J. Proj. Manag. 40, 778–797. doi: 10.1016/j.ijproman.2022.08.007
Duarte, C. M., Agusti, S., Barbier, E., Britten, G. L., Castilla, J. C., Gattuso, J.-P., et al. (2020). Rebuilding marine life. Nature 580, 39–51. doi: 10.1038/s41586-020-2146-7
El Bilali, H., Strassner, C., and Ben Hassen, T. (2021). Sustainable agri-food systems: environment, economy, society, and policy. Sustainability 13:6260. doi: 10.3390/su13116260
Eliasson, K., West, C. D., Croft, S. A., and Green, J. M. H. (2023). A spatially explicit approach to assessing commodity-driven fertilizer use and its impact on biodiversity. J. Clean. Prod. 382:135195. doi: 10.1016/j.jclepro.2022.135195
Esquinas-Alcázar, J. (2005). Protecting crop genetic diversity for food security: political, ethical and technical challenges. Nat. Rev. Genet. 6, 946–953. doi: 10.1038/nrg1729
Evans, B. A., and Gawlik, D. E. (2020). Urban food subsidies reduce natural food limitations and reproductive costs for a wetland bird. Sci. Rep. 10:14021. doi: 10.1038/s41598-
Fischer, J., Abson, D. J., Bergsten, A., French Collier, N., Dorresteijn, I., Hanspach, J., et al. (2017). Reframing the food–biodiversity challenge. Trends Ecol. Evol. 32, 335–345. doi: 10.1016/j.tree.2017.02.009
Frąc, M., Hannula, S. E., Bełka, M., and Jędryczka, M. (2018). Fungal biodiversity and their role in soil health. Front. Microbiol. 9:707. doi: 10.3389/fmicb.2018.00707
Gamache, G., Anglade, J., Feche, R., Barataud, F., Mignolet, C., and Coquil, X. (2020). Can living labs offer a pathway to support local Agri-food sustainability transitions? Environ. Innov. Soc. Transit. 37, 93–107. doi: 10.1016/j.eist.2020.08.002
García-Martín, M., Huntsinger, L., Ibarrola-Rivas, M. J., Penker, M., D’Ambrosio, U., Dimopoulos, T., et al. (2022). Landscape products for sustainable agricultural landscapes. Nat. Food 3, 814–821. doi: 10.1038/s43016-022-00612-w
Gassner, A., Dobie, P., Harrison, R., Vidal, A., Somarriba, E., Pythoud, F., et al. (2020). Making the post-2020 global biodiversity framework a successful tool for building biodiverse, inclusive, resilient and safe food systems for all. Environ. Res. Lett. 15:101001. doi: 10.1088/1748-9326/abae2b
Glover, D., and Sumberg, J. (2020). Youth and food systems transformation. Front. Sustain. Food Syst. 4:101. doi: 10.3389/fsufs.2020.00101
Haase, D., Kabisch, S., Haase, A., Andersson, E., Banzhaf, E., Baró, F., et al. (2017). Greening cities – to be socially inclusive?: about the alleged paradox of society and ecology in cities. Habitat Int. 64, 41–48. doi: 10.1016/j.habitatint.2017.04.005
Hawkins, I. W. ed. (2018). Promoting biodiversity in food systems. 1st edition. Boca Raton, Florida: CRC Press.
Henry, R. J. (2020). Innovations in plant genetics adapting agriculture to climate change. Curr. Opin. Plant Biol. 56, 168–173. doi: 10.1016/j.pbi.2019.11.004
Herens, M. C., Pittore, K. H., and Oosterveer, P. J. M. (2022). Transforming food systems: multi-stakeholder platforms driven by consumer concerns and public demands. Glob. Food Sec. 32:100592. doi: 10.1016/j.gfs.2021.100592
Hoban, S., Bruford, M., D’Urban Jackson, J., Lopes-Fernandes, M., Heuertz, M., Hohenlohe, P. A., et al. (2020). Genetic diversity targets and indicators in the CBD post-2020 global biodiversity framework must be improved. Biol. Conserv. 248:108654. doi: 10.1016/j.biocon.2020.108654
Hossain, M., Leminen, S., and Westerlund, M. (2019). A systematic review of living lab literature. J. Clean. Prod. 213, 976–988. doi: 10.1016/j.jclepro.2018.12.257
Hvitsand, C., Raanaas, R. K., Gjøtterud, S., and Nicolaysen, A. M. (2022). Establishing an Agri-food living lab for sustainability transitions: methodological insight from a case of strengthening the niche of organic vegetables in the Vestfold region in Norway. Agric. Syst. 199:103403. doi: 10.1016/j.agsy.2022.103403
Ioppolo, G., Saija, G., and Salomone, R. (2013). From coastal management to environmental management: the sustainable eco-tourism program for the mid-western coast of Sardinia (Italy). Land Use Policy 31, 460–471. doi: 10.1016/j.landusepol.2012.08.010
IPBES (2019). Summary for policymakers of the global assessment report on biodiversity and ecosystem services. Zenodo.
IUCN (Ed.) (2020). IUCN global standard for nature-based solutions: A user-friendly framework for the verification, design and scaling up of NbS. 1st Edn. Gland, Switzerland: IUCN, International Union for Conservation of Nature.
Jacob, M. C. M., Chaves, V. M., and Rocha, C. (2021). “Biodiversity towards sustainable food systems: four arguments” in Local food plants of Brazil ethnobiology. ed. M. C. M. Jacob (U P. Albuquerque, Cham: Springer International Publishing), 3–18.
Kaufman, S., Saeri, A., Raven, R., Malekpour, S., and Smith, L. (2021). Behaviour in sustainability transitions: a mixed methods literature review. Environ. Innov. Soc. Transit. 40, 586–608. doi: 10.1016/j.eist.2021.10.010
Kok, K. P. W., den Boer, A. C. L., Cesuroglu, T., van der Meij, M. G., de Wildt-Liesveld, R., Regeer, B. J., et al. (2019). Transforming research and innovation for sustainable food systems—a coupled-systems perspective. Sustainability 11:7176. doi: 10.3390/su11247176
Kok, K. P. W., Gjefsen, M. D., Regeer, B. J., and Broerse, J. E. W. (2021). Unraveling the politics of ‘doing inclusion’ in transdisciplinarity for sustainable transformation. Sustain. Sci. 16, 1811–1826. doi: 10.1007/s11625-021-01033-7
Krause, T., and Tilker, A. (2022). How the loss of forest fauna undermines the achievement of the SDGs. Ambio 51, 103–113. doi: 10.1007/s13280-021-01547-5
Lawrence, M. G., Williams, S., Nanz, P., and Renn, O. (2022). Characteristics, potentials, and challenges of transdisciplinary research. One Earth 5, 44–61. doi: 10.1016/j.oneear.2021.12.010
Leclère, D., Obersteiner, M., Barrett, M., Butchart, S. H. M., Chaudhary, A., de Palma, A., et al. (2020). Bending the curve of terrestrial biodiversity needs an integrated strategy. Nature 585, 551–556. doi: 10.1038/s41586-020-2705-y
Leist, A., Avidar, O., Szelest, L., Chapet, J., and Tenikue, M. (2018). Supporting youth in African countries to advance local economies and community health: The SDG lab on microfinance for youth and clean water. Available at: https://orbilu.uni.lu/handle/10993/40781 (Accessed December 12, 2022).
Lind, C. E., Brummett, R. E., and Ponzoni, R. W. (2012). Exploitation and conservation of fish genetic resources in Africa: issues and priorities for aquaculture development and research. Rev. Aquac. 4, 125–141. doi: 10.1111/j.1753-5131.2012.01068.x
Longsheng, C., Shah, S. A. A., Solangi, Y. A., Ahmad, M., and Ali, S. (2022). An integrated SWOT-multi-criteria analysis of implementing sustainable waste-to-energy in Pakistan. Renew. Energy 195, 1438–1453. doi: 10.1016/j.renene.2022.06.112
Loorbach, D., Frantzeskaki, N., and Avelino, F. (2017). Sustainability transitions research: transforming science and practice for societal change. Annu. Rev. Environ. Resour. 42, 599–626. doi: 10.1146/annurev-environ-102014-021340
Lupp, G., Zingraff-Hamed, A., Huang, J. J., Oen, A., and Pauleit, S. (2020). Living labs—a concept for co-designing nature-based solutions. Sustainability 13:188. doi: 10.3390/su13010188
Mabhaudhi, T., Chimonyo, V. G. P., Hlahla, S., Massawe, F., Mayes, S., Nhamo, L., et al. (2019). Prospects of orphan crops in climate change. Planta 250, 695–708. doi: 10.1007/s00425-019-03129-y
Markard, J., Suter, M., and Ingold, K. (2016). Socio-technical transitions and policy change – advocacy coalitions in Swiss energy policy. Environ. Innov. Soc. Transit. 18, 215–237. doi: 10.1016/j.eist.2015.05.003
Martin, G., and Magne, M. A. (2015). Agricultural diversity to increase adaptive capacity and reduce vulnerability of livestock systems against weather variability – a farm-scale simulation study. Agric. Ecosyst. Environ. 199, 301–311. doi: 10.1016/j.agee.2014.10.006
Masuda, H., Kawakubo, S., Okitasari, M., and Morita, K. (2022). Exploring the role of local governments as intermediaries to facilitate partnerships for the sustainable development goals. Sustain. Cities Soc. 82:103883. doi: 10.1016/j.scs.2022.103883
McCrory, G., Holmén, J., Schäpke, N., and Holmberg, J. (2022). Sustainability-oriented labs in transitions: an empirically grounded typology. Environ. Innov. Soc. Transit. 43, 99–117. doi: 10.1016/j.eist.2022.03.004
McCrory, G., Schäpke, N., Holmén, J., and Holmberg, J. (2020). Sustainability-oriented labs in real-world contexts: an exploratory review. J. Clean. Prod. 277:123202. doi: 10.1016/j.jclepro.2020.123202
Miralles-Wilhelm, F. (2021). Nature-based solutions in agriculture: Sustainable management and conservation of land, water and biodiversity. Virginia: Food & Agriculture Org.
Mottet, A., Teillard, F., Boettcher, P., Besi, G. D., and Besbes, B. (2018). Review: domestic herbivores and food security: current contribution, trends and challenges for a sustainable development. Animal 12, s188–s198. doi: 10.1017/S1751731118002215
Mujeeb-Kazi, A., Kazi, A. G., Dundas, I., Rasheed, A., Ogbonnaya, F., Kishii, M., et al. (2013). “Chapter four - genetic diversity for wheat improvement as a conduit to food security” in Advances in agronomy. Ed. D. L. Sparks (United States: Academic Press), 179–257.
Nevens, F., Frantzeskaki, N., Gorissen, L., and Loorbach, D. (2013). Urban transition labs: co-creating transformative action for sustainable cities. J. Clean. Prod. 50, 111–122. doi: 10.1016/j.jclepro.2012.12.001
Offermans, A., Forrest, N., Wahl, D., Dalla Fontana, M., Bernert, P., de Araujo Moreira, F., et al. (2020). Urban living labs on food, water and energy: Evaluative scheme and manual.
Ojea, E., Lester, S. E., and Salgueiro-Otero, D. (2020). Adaptation of fishing communities to climate-driven shifts in target species. One Earth 2, 544–556. doi: 10.1016/j.oneear.2020.05.012
Oliver, T., Isaac, N., August, T., Woodcock, B. A., Roy, D. B., and Bullock, J. M. (2015). Declining resilience of ecosystem functions under biodiversity loss. Nat. Commun. 6:10122. doi: 10.1038/ncomms10122
Pawera, L., Khomsan, A., Zuhud, E. A. M., Hunter, D., Ickowitz, A., and Polesny, Z. (2020). Wild food plants and trends in their use: from knowledge and perceptions to drivers of change in West Sumatra, Indonesia. Foods 9:1240. doi: 10.3390/foods9091240
Pereira, L. M., Kushitor, S. B., Cramer, C., Drimie, S., Isaacs, M., Malgas, R., et al. (2022). Leveraging the potential of wild food for healthy, sustainable, and equitable local food systems: learning from a transformation lab in the Western Cape region. Sustain. Sci. doi: 10.1007/s11625-022-01182-3
Pimm, S. (2022). We can have biodiversity and eat too. Nat. Food 3, 310–311. doi: 10.1038/s43016-022-00503-0
Puppim de Oliveira, J. A., Balaban, O., Doll, C. N. H., Moreno-Peñaranda, R., Gasparatos, A., Iossifova, D., et al. (2011). Cities and biodiversity: perspectives and governance challenges for implementing the convention on biological diversity (CBD) at the city level. Biol. Conserv. 144, 1302–1313. doi: 10.1016/j.biocon.2010.12.007
Rasul, G., Hussain, A., Adhikari, L., and Molden, D. J. (2022). Conserving agrobiodiversity for sustainable food systems in the Hindu Kush Himalaya. Int. J. Agric. Sustain. 20, 1117–1135. doi: 10.1080/14735903.2022.2057642
Rockström, J., Edenhofer, O., Gaertner, J., and DeClerck, F. (2020). Planet-proofing the global food system. Nat. Food 1, 3–5. doi: 10.1038/s43016-019-0010-4
Rockström, J., and Sukhdev, P. (2016). New way of viewing the sustainable development goals and how they are all linked to food. Stockholm Resilience Centre/Stockholm University. Available at: https://www.stockholmresilience.org/research/research-news/2016-06-14-how-food-connects-all-the-sdgs.html (Accessed August 12, 2022).
Roe, D. (2019). Biodiversity loss—more than an environmental emergency. Lancet Planet. Health 3, e287–e289. doi: 10.1016/S2542-5196(19)30113-5
Rolls, R. J., Heino, J., Ryder, D. S., Chessman, B. C., Growns, I. O., Thompson, R. M., et al. (2018). Scaling biodiversity responses to hydrological regimes. Biol. Rev. 93, 971–995. doi: 10.1111/brv.12381
Schäpke, N., Stelzer, F., Caniglia, G., Bergmann, M., Wanner, M., Singer-Brodowski, M., et al. (2018). Jointly experimenting for transformation? Shaping real-world laboratories by comparing them. GAIA - Ecol. Perspect. Sci. Soc. 27, 85–96. doi: 10.14512/gaia.27.S1.16
Schmidt, T. S., Blum, N. U., and Wakeling, R. S. (2013). Attracting private investments into rural electrification — a case study on renewable energy based village grids in Indonesia. Energy Sustain. Develop. 17, 581–595. doi: 10.1016/j.esd.2013.10.001
Sengers, F., Wieczorek, A. J., and Raven, R. (2019). Experimenting for sustainability transitions: a systematic literature review. Technol. Forecast. Soc. Change 145, 153–164. doi: 10.1016/j.techfore.2016.08.031
Shabani, F., Ahmadi, M., Kumar, L., Solhjouy-fard, S., Shafapour Tehrany, M., Shabani, F., et al. (2020). Invasive weed species’ threats to global biodiversity: future scenarios of changes in the number of invasive species in a changing climate. Ecol. Indic. 116:106436. doi: 10.1016/j.ecolind.2020.106436
Snyman-van der Walt, L., Schreiner, G., Laurie, S., Audouin, M., Lochner, P., Marivate, R., et al. (2020). “Pathways for mainstreaming resilience-thinking into climate change adaptation and planning in the City of Cape Town” in The Palgrave handbook of climate resilient societies (Cham: Springer International Publishing), 1–22. doi: 10.1007/978-3-030-32811-5_48-1
Sowińska-Świerkosz, B., and García, J. (2022). What are nature-based solutions (NBS)? Setting core ideas for concept clarification. Nat. Based Solut. 2:100009. doi: 10.1016/j.nbsj.2022.100009
Springmann, M., Clark, M., Mason-D’Croz, D., Wiebe, K., Bodirsky, B. L., Lassaletta, L., et al. (2018). Options for keeping the food system within environmental limits. Nature 562, 519–525. doi: 10.1038/s41586-018-0594-0
Subedi, R., Karki, M., and Panday, D. (2020). Food system and water–energy–biodiversity Nexus in Nepal: a review. Agronomy 10:1129. doi: 10.3390/agronomy10081129
Sunderland, T. C. H. (2011). Food security: why is biodiversity important? Int. For. Rev. 13, 265–274. doi: 10.1505/146554811798293908
Tschora, H., and Cherubini, F. (2020). Co-benefits and trade-offs of agroforestry for climate change mitigation and other sustainability goals in West Africa. Glob. Ecol. Conserv. 22:e00919. doi: 10.1016/j.gecco.2020.e00919
UNEP, FAO, and UNDP. (2023). Rethinking our food systems: A guide for multi-stakeholder collaboration. Nairobi, Rome, and New York.
von Wirth, T., Fuenfschilling, L., Frantzeskaki, N., and Coenen, L. (2019). Impacts of urban living labs on sustainability transitions: mechanisms and strategies for systemic change through experimentation. Eur. Plan. Stud. 27, 229–257. doi: 10.1080/09654313.2018.1504895
Voytenko, Y., McCormick, K., Evans, J., and Schliwa, G. (2016). Urban living labs for sustainability and low carbon cities in Europe: towards a research agenda. J. Clean. Prod. 123, 45–54. doi: 10.1016/j.jclepro.2015.08.053
Wani, S. H., Khan, H., Riaz, A., Joshi, D. C., Hussain, W., Rana, M., et al. (2022). “Chapter six - genetic diversity for developing climate-resilient wheats to achieve food security goals” in Advances in agronomy. Ed. D. L. Sparks (United States: Academic Press), 255–303.
WFF (2023). Opportunities and barriers for advancing Agrifood systems: Empowering Young people for a sustainable future. Rome: FAO.
Wolfert, J., Verdouw, C. N., Verloop, C. M., and Beulens, A. J. M. (2010). Organizing information integration in Agri-food—a method based on a service-oriented architecture and living lab approach. Comput. Electron. Agric. 70, 389–405. doi: 10.1016/j.compag.2009.07.015
Wrangsten, C., Ferlander, S., and Borgström, S. (2022). Feminist urban living labs and social sustainability: lessons from Sweden. Urban Transform. 4:5. doi: 10.1186/s42854-022-00034-8
Zavratnik, V., Superina, A., and Stojmenova Duh, E. (2019). Living labs for rural areas: contextualization of living lab frameworks, concepts and practices. Sustainability 11:3797. doi: 10.3390/su11143797
Keywords: sustainability-oriented experiment, transdisciplinarity, solutions-oriented approach, biodiversity-food-climate nexus, sustainability, co-benefits, agriculture
Citation: Jarzebski MP, Su J, Abrahamyan A, Lee J, Kawasaki J, Chen B, Andriatsitohaina RNN, Ocen I, Sioen GB, Lambino R, Saito O, Elmqvist T and Gasparatos A (2023) Developing biodiversity-based solutions for sustainable food systems through transdisciplinary Sustainable Development Goals Labs (SDG-Labs). Front. Sustain. Food Syst. 7:1144506. doi: 10.3389/fsufs.2023.1144506
Edited by:
Johannes S. C. Wiskerke, Wageningen University and Research, NetherlandsReviewed by:
Cécil J. W. Meulenberg, Scientific Research Center Koper, SloveniaDinesh Panday, Rodale Institute, United States
Copyright © 2023 Jarzebski, Su, Abrahamyan, Lee, Kawasaki, Chen, Andriatsitohaina, Ocen, Sioen, Lambino, Saito, Elmqvist and Gasparatos. This is an open-access article distributed under the terms of the Creative Commons Attribution License (CC BY). The use, distribution or reproduction in other forums is permitted, provided the original author(s) and the copyright owner(s) are credited and that the original publication in this journal is cited, in accordance with accepted academic practice. No use, distribution or reproduction is permitted which does not comply with these terms.
*Correspondence: Marcin Pawel Jarzebski, marcin.p.jarzebski@unu.edu