- 1College of Forestry and Prataculture, Ningxia University, Yinchuan, China
- 2State Key Laboratory of Grassland Agro-ecosystems, Lanzhou University, Lanzhou, China
- 3College of Grassland Agriculture, Northwest A & F University, Yangling, Shaanxi, China
- 4College of Pastoral Agriculture Science and Technology, Lanzhou University, Lanzhou, China
- 5National Demonstration Center for Experimental Grassland Science Education, Lanzhou University, Lanzhou, China
Understanding the relationships of productivity performance and water utilization and soil nitrogen dynamics after annual forage planting during the fallow period (F) in winter wheat (Triticum aestivum L.; W) mono-cropping is critically important for maintaining sustainable livestock and grain production in semiarid regions. We used 2 years (2017–2019) of data to investigate soil nitrogen dynamics, production, water utilization, and fallow efficiency when forage rape (Brassica campestris L.; R) and common vetch (Vicia sativa L.; V) were planted in a 3-month summer fallow of the W-F-W-F cropping system. Three cropping systems were comprised of winter wheat-summer fallow-winter wheat-summer fallow (W-F-W-F), winter wheat-forage rape-winter wheat-forage rape (W-R-W-R), and winter wheat-forage rape-winter wheat-common vetch (W-R-W-V). The results showed that the annual forage planting decreased the average NO3−-N content by 54.8% compared with the W-F-W-F cropping system. Compared with the W-F-W-F cropping system, planting annual forage in summer fallow increased the average system forage production by 4.93 t ha−1. Local total annual precipitation can meet crop-water requirements, and the limiting factor for agricultural production was the drought due to the uneven seasonal distribution of precipitation. In comparison to the W-F-W-F cropping system, annual forage planting decreased the average available soil moisture storage by 50.3 mm above the 80 cm soil layer. Compared with that in the W-R-W-R (23.21 t ha−1) and W-F-W-F (30.25 t ha−1) cropping systems, the crop productivity in the W-R-W-V cropping system (33.23 t ha−1) was relatively stable and high because the reduction in subsequent winter wheat yield (2.96 t ha−1) was adequately offset by the forage yield (5.15 t ha−1). Adding forage rape to the W-F-W-F cropping system decreased system crop-water productivity (CWP) by 40.9%. However, the CWP, precipitation use efficiency (PUE), and soil nitrate in the W-R-W-V cropping system increased by 30.4, 30.1, 110.9, and 82.0%, respectively, compared with those in the W-R-W-R cropping system. Therefore, the W-R-W-V cropping system is recommended for better water and fertility management as well as grain and forage production in semiarid regions. However, further study is required to involve drought years for better evaluation of the effect of long-term precipitation variability on the crop productivity.
1. Introduction
Given the increasing global population, the function of water and nitrogen fertilizer on agricultural production is irreplaceable (Li et al., 2019). In many arid and semiarid areas of the world, agricultural production activities will face severe challenges related to water and fertilizer shortages (Gan et al., 2015). Therefore, a more sustainable farming system for food production that could better meet the demand of society with a less detrimental impact on the environment is needed (Jensen et al., 2012).
In the region of Northwest China, winter wheat monoculture is the traditional crop production system, mainly practiced on the Chinese Loess Plateau. This cropping system involves a fallow of 3 months during the summer (July–September). Generally, because the availability of water and nitrogen are the main limiting factors for crop production in semiarid regions, summer fallow is considered a way to conserve soil moisture and soil nutrients, especially soil nitrate for subsequent crops (Grant et al., 2002; Campbell et al., 2008; Nielsen and Vigil, 2010). However, summer fallow may cause negative impacts on sustainable production (Unger et al., 1997; Farahani et al., 1998), such as nitrate leaching (Yang et al., 2017), loss of soil organic matter (Lemke et al., 2012), and low crop-water productivity (CWP) and precipitation use efficiency (PUE; Zhu et al., 1994). These negative impacts of summer fallow occurred because substantial rainfall happened during this period, which is accompanied by large amount of soil moisture evaporation because the soil is bare (Kang et al., 2001; Yang et al., 2018). In addition, there was an obvious enhancement in production systems in recent years due to the increased demand for food and animal products (Zhang et al., 2019a). However, meeting this demand poses a significant challenge in this region because of variable precipitation distribution, water scarcity and low quality and shortages of forage supply for animal feeding. These have been the typical constraints on crop production and farmer incomes, and these factors subsequently stall the regional livestock industry’s economic development in semiarid regions (Hou et al., 2008; Komarek et al., 2015; Zhang et al., 2019b). Integrating forage crops to replace fallow is an effective way to alleviate erosion, improve PUE, and enhance forage yields (Aiken et al., 2013; Blanco-Canqui et al., 2015; Nielsen et al., 2015). Li et al. (2000) reported a 17% increase in precipitation utilization with a continuous wheat production system with a forage crop compared with continuous wheat production system on the Loess Plateau. In semiarid climates, Li et al. (2000) found that the success of integrating grain-forage production systems depends on the choice of forage crops. Cultivars with fast seedling emergence and short growth spans that can quickly achieve complete cover are considered most appropriate. Abate et al. (2003) reported a 2.0 t ha−1 increase in forage yield when forage vetch was planted during a short period of fallow. As an annual forage crop, forage rape resulted in a relatively high yield within a short period (Liu et al., 2014). However, Unger and Vigil (1998) reported that there is limited adoption of cover crops in dry regions, chiefly due to poor soil nutrients and low CWP. The availability of water strongly influences forage rape and common vetch production, as well as the yield stability of subsequent winter wheat in semiarid environments (Nielsen et al., 2016; Zhang et al., 2018). In the semiarid Loess Plateau of China, the annual precipitation varies considerably, from 300 to 600 mm (Ao et al., 2007). The precipitation ratio during summer fallow is as great as 62% compared to the annual value, although with a considerable variation from 35 to 88% (Wang et al., 2020). Therefore, it is necessary to investigate how forage production and soil moisture utilization are affected by precipitation.
Agriculture production is largely determined by the amount of soil moisture stored and residual nitrate in rainfed regions. When a single crop-fallow system is practiced, the amount of water lost from the soil due to evapotranspiration could be recouped by precipitation during the fallow season (Li et al., 2013). A modest reduction in yield (0–16%) could occur in normal precipitation years when a spring broadleaf crop replaces fallow. Non-summer fallowing practices also have an obvious impact on soil nitrogen, and the integration of pulse crops [dry pea (Pisum sativum L.) and lentil (Lens culinaris Medikus)] could enhance soil available nitrogen (Herridge et al., 2008). Yang et al. (2015) reported that nitrates were highly mobile. Therefore, due to the concentration of precipitation during the summer fallow period, the residual nitrates could be susceptible to leaching into the deeper layers of the soil. Hence, the availability of soil nitrate for crop production in rainfed regions primarily relies on the amount of available water (Gan et al., 2015). Therefore, the differences in the soil nitrate, water balance, and system CWP mainly rely on the precipitation amount and distribution, fallow period, cropping patterns, and evaporative demand (Lyon et al., 2004). However, little research has conducted the effects of integrating forage into winter wheat monocultures on evapotranspiration (ET). In addition, Deng et al. (2020) studied the crude protein productivity in relation to CWP and PUE and the economic return of replacing summer fallow with either forage rape and/or common vetch on the Chinese Loess Plateau. However, soil nitrate dynamics, soil moisture storage and water utilization during crop development in different cropping systems need to be explored.
Therefore, the main goal of the present study was to determine the effect of integrating forage rape and common vetch into the winter wheat monoculture as a replacement for summer fallow and specifically: (1) evaluate the crop yields as affected by the cropping system; (2) evaluate the effect of cropping system on soil nitrate dynamics, CWP, and PUE; (3) investigate the ET characteristics of the cropping system during crop development; and (4) assess soil moisture utilization sustainability under different cropping systems on the Loess Plateau.
2. Materials and methods
2.1. Experimental site and design description
The experimental site was located on the Chinese Loess Plateau at 1298 m above sea level. The area has a temperate, semiarid continental monsoon climate. The mean annual precipitation, solar radiation, and air temperature are 527.6 mm, 5543.0 MJ m−2, and 9.2°C, respectively. The annual precipitation exhibits an uneven distribution, with 60–70% of it concentrated in July, August, and September. The field trial was conducted on level terrain, and the soil type is a typical Loess Plateau soil classified as the Heilu soil type (U.S. classification; Zhu et al., 1983). The properties of the soil and the nutrient profile at a depth of 0–160 cm in late September 2017 are shown in Supplementary Table 1. The soil from the 0–160 cm layer contained 4.1 g kg−1 organic matter, 31.1 mg kg−1 available nitrogen, 2.6 mg kg−1 available phosphorus, and 88.9 mg kg−1 available potassium. The field experiment was conducted in late September 2015 at the Lanzhou University Research Station located near Xifeng district, Qingyang, Gansu, China (35°39′N, 107°51′E) and was implemented for two cycles during the growing seasons of 2015–2019. Because the soil moisture measuring instrument was installed in 2017, the experimental data in this paper are from the two winter wheat growing seasons of 2017–2019. The field experiment contained three treatments: winter wheat-summer fallow-winter wheat-summer fallow (W-F-W-F), winter wheat-forage rape-winter wheat-forage rape (W-R-W-R), and winter wheat-forage rape-winter wheat-common vetch (W-R-W-V).
2.2. Experimental field management
A randomized complete block design was adopted in this study, and it was replicated three times, with each experimental plot measuring 4 m by 5 m. The seeding rate of the wheat was 225 kg ha−1, while the spacing between rows was 15 cm. Furthermore, annual forage was sown in late June or early July immediately after the winter wheat harvest. The seeding rate for common vetch (Lanjian 3) was 120 kg ha−1, and the inter-row spacing was 20 cm. Forage rape (Siyou 1) was surface-broadcasted at a rate of 15 kg ha−1. All the plots were ploughed to a 30 cm depth using a chisel plough before planting. Nitrogen and phosphorus fertilizers were applied according to the local fertilization recommendations, which suggest an application of available N (70 kg ha−1) and P (100 kg ha−1) prior to planting. N and P fertilization was applied to the winter wheat and forage rape, but P fertilization was only applied to the common vetch. Manual hoeing was used to ensure all the experimental plots were weed free throughout the experiment and no irrigation was given in this study.
2.3. Measurements and methods
2.3.1. Weather conditions
During the experimental years, daily information on precipitation, radiation, and air temperature was recorded by an onsite weather station (Campbell Sci., Inc. Logan, UT, United States) located within the experimental station.
2.3.2. Yield measurements
The grain and dry matter yields of winter wheat were estimated at the physiological maturity of the crop. Samples were collected using three 0.3 m2 (2 m × 0.15 m) quadrats that were randomly placed within each plot. The estimation of dry matter yield was carried out following the harvest of forage rape and common vetch at 50% flowering. Three quadrats (1 × 1 m) that were randomly placed in each plot were used for sampling. A distance of approximately 50 cm between sampling areas was used for all crops, and the sampling positions were away from the plot edges. The collected samples were oven dried at an initial temperature of 105°C for 30 min to kill the fresh tissues and subsequently dried to a constant weight at 80°C. The oven-dried samples were used to calculate grain and dry matter yield, which were extrapolated to tons per hectare (Deng et al., 2020).
2.3.3. Soil available nitrogen
When the winter wheat and forage crop harvest occurred, three soil samples were taken randomly at depths of 0–10, 10–20, 20–40, 40–60, 60–80, 80–100, and 100–120 cm with an auger in the middle of each plot. The soil samples were used for calorimetric determination of NO3−–N (soil nitrate-nitrogen) and NH4+–N (ammonium nitrogen) concentrations by using the salicylate-nitroprusside method with an automated continuous flow analyzer (AA3, Seal, Norderstedt, Germany; Luce et al., 2020).
2.3.4. Soil moisture
Soil moisture content was measured approximately every 7–13 days during the entire study. The soil moisture up to the 160 cm depth was measured at an interval of 10 cm using a Diviner-2000 device (Sentek Pty Ltd., Australia). PVC access tubes (internal diameter 40 mm) were installed in the center of each plot at the beginning of this experiment in late September 2015.
Soil water storage (SWS; mm) in the 0–160 cm soil layer was determined according to Zhang et al. (2017) as follows:
Available soil water storage (ASWS) was calculated by multiplying the depth soil layer (10 cm) by the difference between the calculated volumetric water and the bottom limit of available water at each depth of water measurement (Ritchie, 1981; Ratliff et al., 1983). The bottom limit of available water at each of the 16 measurement intervals was initially determined in late September 2017 in the plot area as the lowest volumetric water value. The profile plant-available soil water storage was calculated by adding the individual values of available water at the 16 depths in each plot.
The ET (mm) from stage j (i.e., its starting time (d)) to stage j + 1 (d) was calculated as follows (Ding et al., 2018):
The evapotranspiration rate (ETR, mm d−1) from stage i to stage i + 1 was calculated as follows:
2.4. Data analysis
The variables of soil nitrogen dynamics, production, water utilization, and fallow efficiency were subjected to analysis of variance using GenStat 17.0 (VSN International, Hemel Hempstead, United Kingdom). Significant differences between treatments were analyzed using Fisher’s protected least significant difference test at a probability level of 5%. SigmaPlot 12.2 was used for drawing of figures.
3. Results
3.1. Precipitation and temperature
The precipitation in the 2017–2018 and 2018–2019 growing seasons, was 808.6 mm (398.5 mm for wheat and 410.1 mm for the summer fallow season) and 670.9 mm (278.3 mm for wheat and 392.6 mm for the summer fallow season), respectively (Figure 1; Supplementary Table 2). The long-term average (LTA) precipitation (2007–2017) was 557.8 mm (260.9 mm for wheat and 296.9 mm for the summer fallow season). The air temperature of LTA (2007–2017) was 10.0°C (7.0°C for winter wheat and 19.1°C for the summer fallow season). The air temperature was 10.1°C (6.8°C for winter wheat and 20.6°C for the summer fallow season) and 9.6°C (6.5°C for winter wheat and 19.9°C for the summer fallow season) for the growing seasons of 2017–2018 and 2018–2019, respectively.
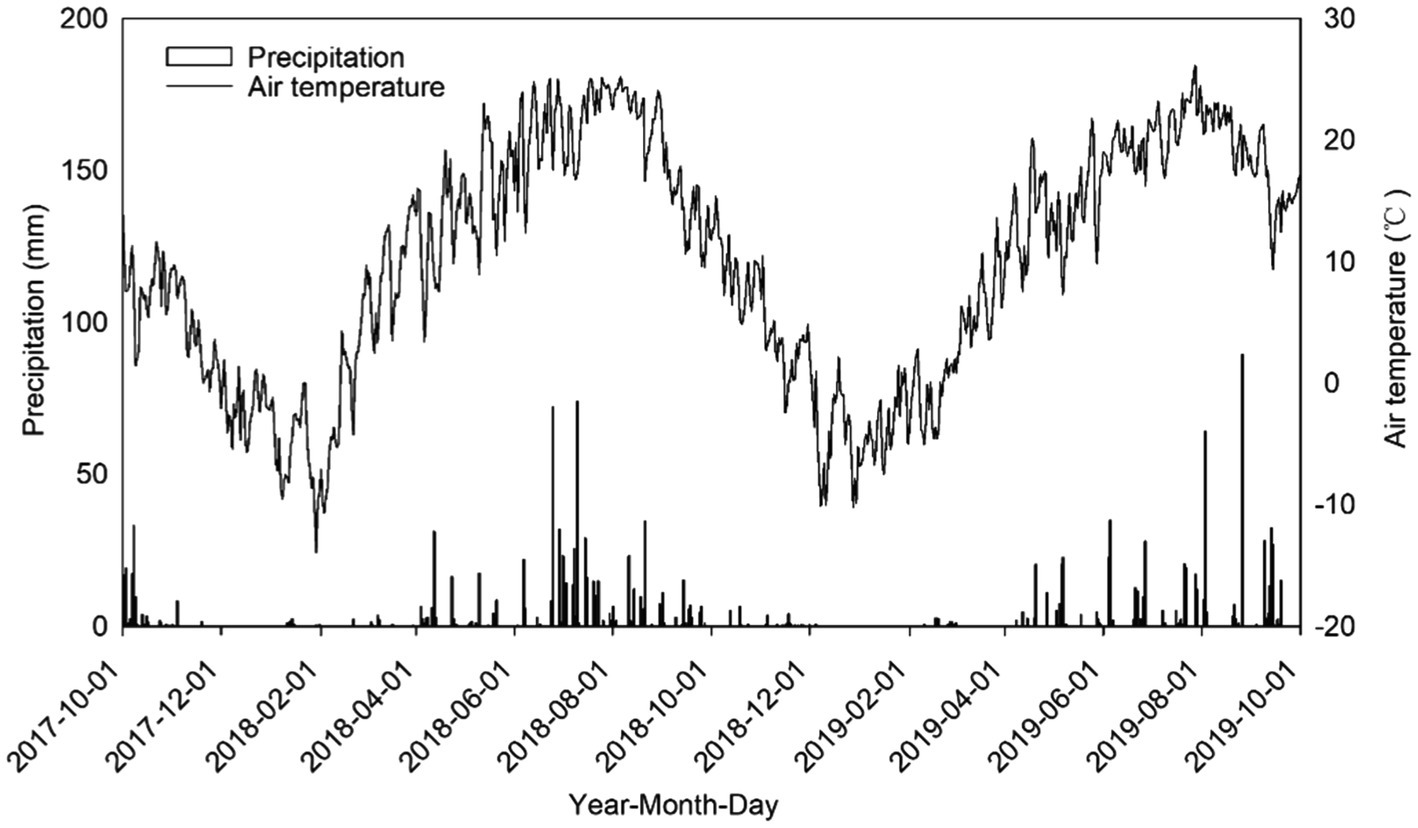
Figure 1. Daily precipitation distribution and air temperature during the growing seasons (October 2017–September 2019).
3.2. Production
During the growing season of 2017–2018, the grain yield for winter wheat had no significant difference and the average was 5.2 t ha−1 across the cropping systems (Table 1). No significant difference was found in forage rape yield and the average was 4.0 t ha−1 across the W-R-W-R and W-R-W-V treatments. During the 2018–2019 growing season, the winter wheat grain yield in the W-R-W-R treatment had the lowest value, which was 82.6 and 58.7% lower than that in the W-F-W-F and W-R-W-V treatments, respectively (p < 0.05). The forage yield in the W-R-W-R treatment was 46.7% greater than that in the W-R-W-V treatment (p < 0.05). The system DM was significant (p < 0.05). The system DM yield in the W-R-W-V treatment was 30.2% greater than that in the W-R-W-R treatment (p < 0.05), but the difference with that in the W-F-W-F treatment was not statistically significant.
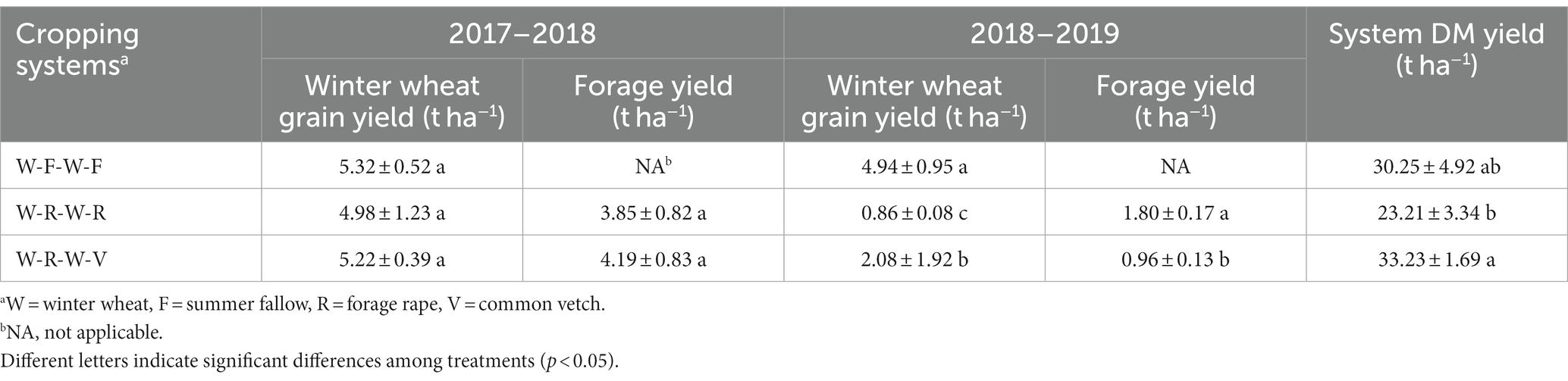
Table 1. Winter wheat grain yield, forage yield, and dry matter (DM) yield of the system under different cropping systems during the growing seasons (October 2017–September 2019) at Xifeng, Gansu, China.
3.3. Soil available nitrogen in the 0–120 cm layer
The differences in the distribution of available soil nitrogen in the 0–120 cm layer among the cropping systems are presented in Figure 2. The NO3−–N content obtained among the different cropping systems after the wheat growing season in 2017–2018 was not significant (p > 0.05). After forage rape planting (24 September 2018), the NO3−–N content decreased by 54.8% (p < 0.05) compared to that in the W-F-W-F treatment. On 3 July 2019, the NO3−–N content in the W-R-W-R treatment had the highest average value (23.9 mg kg−1) in the 0–60 cm soil layer, 50.0% higher than that in the W-F-W-F treatment (p < 0.05). At the forage harvesting stage (22 September 2019), no significant difference was found in the NO3−-N content in the 0–70 cm soil layer. However, the NO3−-N content in the W-R-W-V treatment was 51.0% greater than that in the W-F-W-F treatment in 70–120 cm soil layer (p < 0.05). There was no obvious difference in the NH4+-N content in the 0–120 cm soil layer among the treatments on 22 September 2017, 27 June 2018, 24 September 2018, and 3 July 2019. However, in the 30–70 cm soil layer, the NH4+-N content in the W-R-W-V treatment was 82.0% greater than that in the W-F-W-F treatment on 22 September 2019 (p < 0.05).
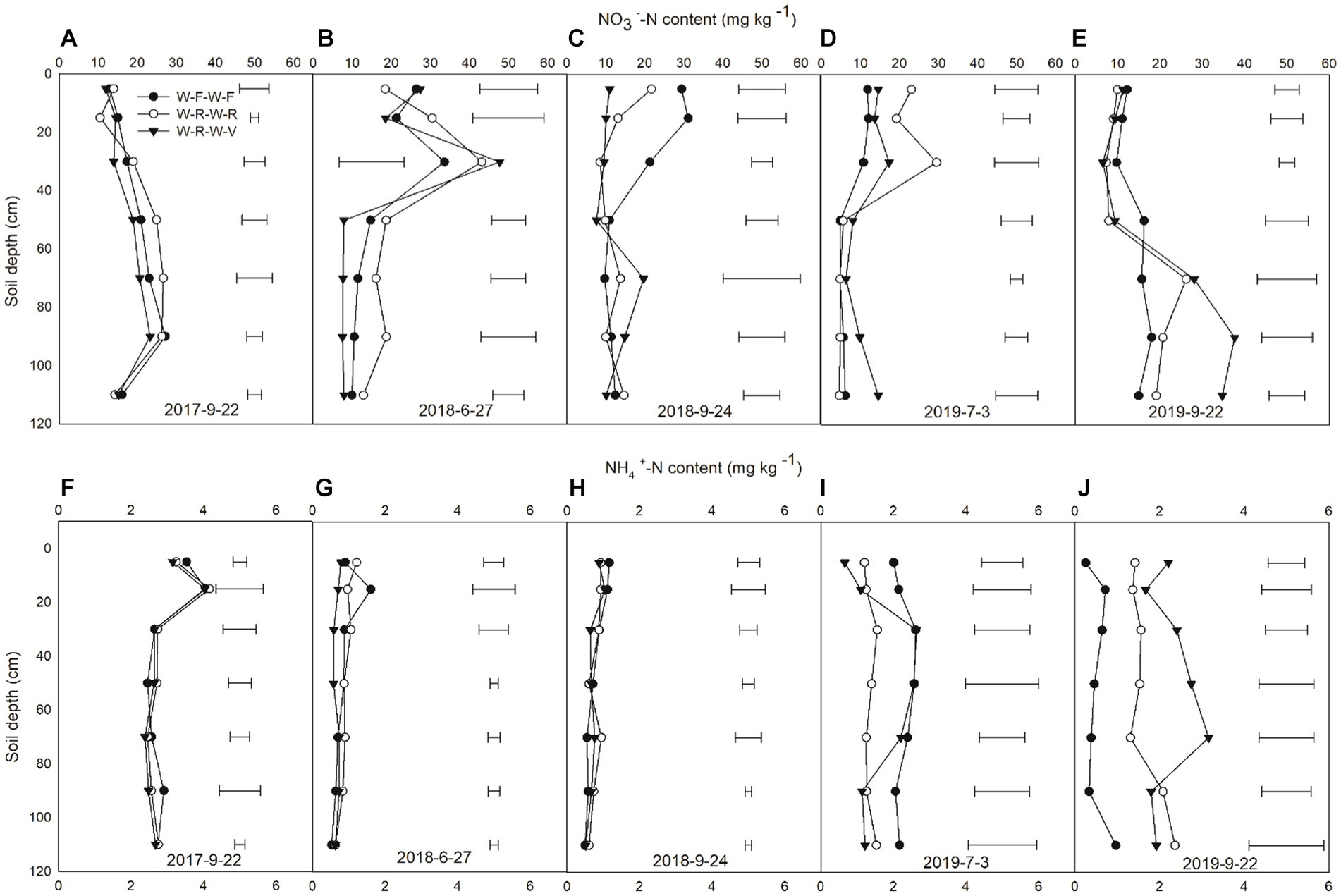
Figure 2. Vertical distribution of NO3−–N and NH4+–N content in the 0–120 cm soil layer for different sowing and harvesting dates of each crop among the cropping systems during the growing seasons (October 2017–September 2019). The bars adjacent to each soil layer represent the least significant difference (p = 0.05). W = winter wheat, F = summer fallow, R = forage rape, V = common vetch.
3.4. Evapotranspiration characteristics and soil water storage
During the experimental period of 2017–2018, there was no significant difference in winter wheat ET among the different cropping systems at the early stage (Figure 3). However, in April 2018, the average ET in the W-F-W-F treatment was 7.8% higher than that in the W-R-W-R and W-R-W-V treatments (p < 0.05), and the average ET in the W-F-W-F treatment was 10.2% lower than that in the W-R-W-R and W-R-W-V treatments in June 2018 (p < 0.05). During the summer fallow period in 2018, the average ET in the W-F-W-F treatment was lowest in July, which was 13.5% lower than that in the W-R-W-R and W-R-W-V treatments (p < 0.05). In August 2018, the average ET in the W-F-W-F treatment was 24.0% less than that in the W-R-W-R and W-R-W-V treatments (p < 0.05). There was no cropping system effect on ET in September (average 18.6 mm). During the experimental period of 2018–2019, the average ET in the W-F-W-F treatment had the highest value, 29.7, 60.1, 21.9, 10.4, and 61.1% greater than that in the other treatments in November, December, January, February, March, and April, respectively (p < 0.05). The cropping system had no significant effect on the ET of winter wheat at the emergence and harvesting stages. During the summer fallow period in 2019, there was no significant difference in July and August. In September, ET in the W-F-W-F treatment was lowest, which was 10.7 and 10.3% lower than that in the W-R-W-R and W-R-W-V treatments, respectively (p < 0.05).
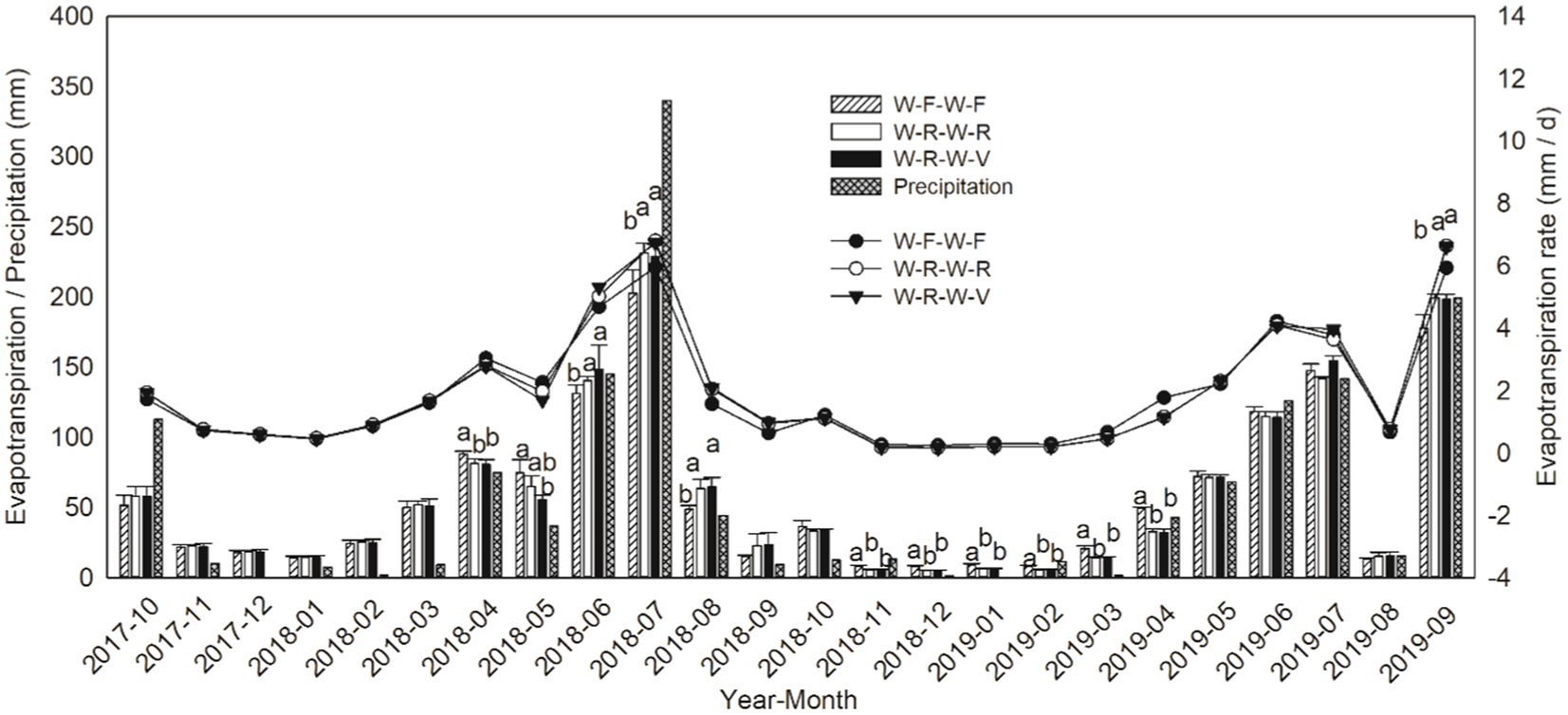
Figure 3. Effects of different cropping systems on evapotranspiration and the corresponding evapotranspiration rate during the growing seasons (October 2017–September 2019). The vertical bars represent the least significant difference (p = 0.05). Different letters indicate significant differences among treatments (p < 0.05). W = winter wheat, F = summer fallow, R = forage rape, V = common vetch.
Table 2 shows the soil water supply and demand (SWSD) of the system under different cropping systems across the experimental period from 2017 to 2019. The system SWSD in the W-F-W-F treatment had the greatest value, followed by that in W-R-W-V and finally that in W-R-W-R.

Table 2. System soil water supply and demand (SWSD) under different cropping systems during the experimental period of 2017–2019 during the growing seasons (October 2017–September 2019) in Xifeng, Gansu, China.
The ASWS in the 0–80 cm soil layer declined gradually, and then increased rapidly but without a cropping system effect in the wheat-growing season of 2017–2018 (Figure 4A). After 1 August 2018, the ASWS in the W-F-W-F treatment in the 0–80 cm soil layer was an average 40.5% higher than that in the W-R-W-R treatment (p < 0.05). A similar ASWS trend was found in the 80–160 cm soil layer during the growing season but without a cropping system effect among the W-F-W-F, W-R-W-R, and W-R-W-V treatments. At the regreening stage of winter wheat (2 April 2019), the ASWS in the W-F-W-F treatment in the 0–80 cm soil layer was 36.2 and 29.8% greater than that in the W-R-W-R and W-R-W-V treatments, respectively (p < 0.05; Figure 4B). After May, there was no significant difference in the ASWS among the cropping systems (average = 56.1 mm). A similar ASWS trend was found in the 80–160 cm soil layer during the growing season but without a cropping system effect among the W-F-W-F, W-R-W-R, and W-R-W-V treatments.
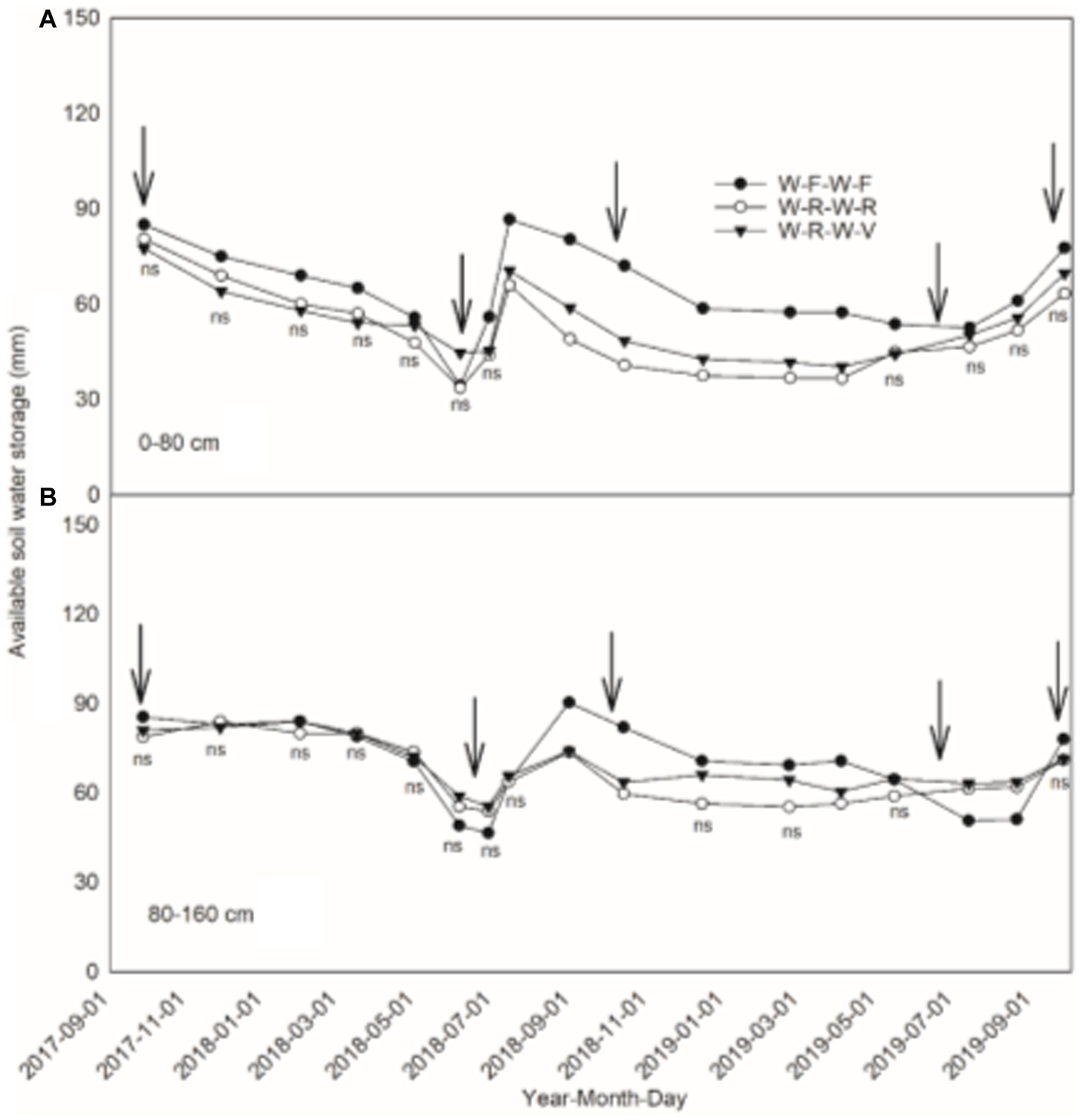
Figure 4. Variation in available soil water storage in the 0–80 cm (A) and 80–160 cm (B) soil layers under different cropping systems during the growing seasons (October 2017–September 2019). Vertical bars represent the least significant difference (p = 0.05). W = winter wheat, F = summer fallow, R = forage rape, V = common vetch.
3.5. Crop water productivity and fallow efficiency
The system CWP in the W-R-W-V treatment was 30.4% greater than that in the W-R-W-R treatment (p < 0.05) but statistically similar to that in the W-F-W-F treatment (Figure 5A). The system PUE in the W-R-W-V treatment had the highest value, which was 8.9 and 30.1% greater than that in the W-F-W-F and W-R-W-R treatments, respectively (p < 0.05; Figure 5B). The FE in the W-F-W-F treatment was 53.1 and 21.7% greater than that in the W-R-W-R and W-R-W-V treatments, respectively (p < 0.05; Figure 5C).
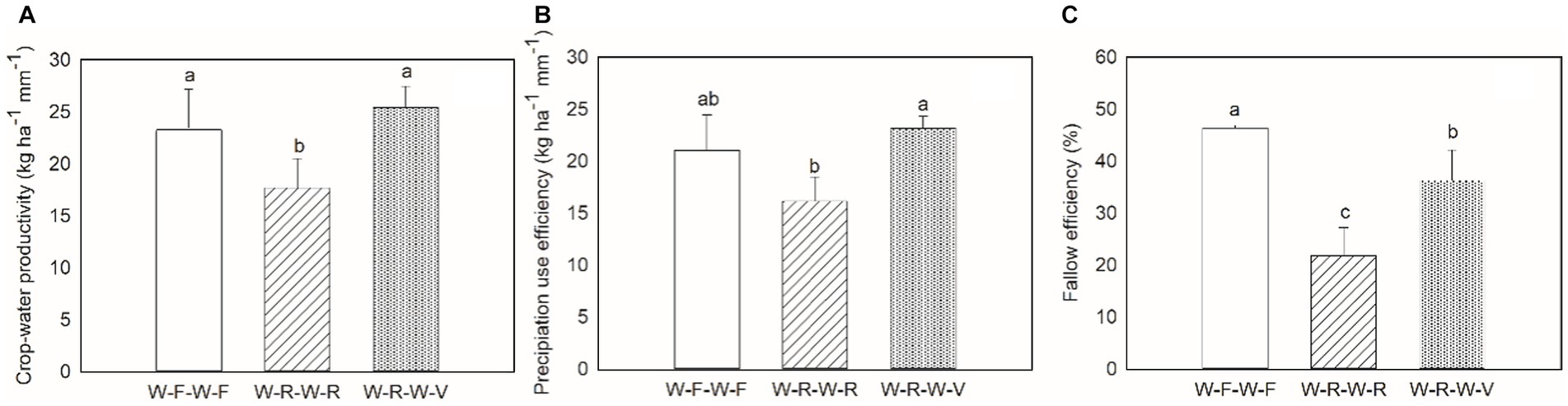
Figure 5. System crop-water productivity (A), precipitation use efficiency (B), and fallow efficiency (C) among the different cropping systems. Different letters indicate significant differences between treatments (p < 0.05). W = winter wheat, F = summer fallow, R = forage rape, V = common vetch.
4. Discussion
4.1. Relationship of production performance with soil moisture and nitrogen
In the Chinese Loess Plateau region, Li et al. (2000) reported that the rainy season occurs around the forage growing periods (July to September), and the growth of such crops relies on growing-period precipitation. Therefore, forages, such as soybean [Glycine max L. (Merr.)] and common vetch, has relatively less influence on soil moisture storage. However, our results showed that forage in the W-R-W-R and W-R-W-V treatments sharply decreased the ASWS in 2017–2018 (Figure 4). Water depletion obviously occurred in the 0–80 cm soil layer (Figure 4; Supplementary Figure 1), which hindered the subsequent wheat from completing the regreening stage in the next year, thereby resulting in a significant reduction in winter wheat grain yield compared to that in the W-F-W-F treatment (Table 1; Figure 5). Similarly, Nielsen et al. (2017) also found up to a 17% reduction in the yield of subsequent winter wheat due to the depletion of soil moisture storage to 47 mm by planting the forage triticale (X Triticosecale rimpaui Wittm.) in a wheat-maize (Zea mays L.)—fallow rotation system. Precipitation during the summer fallow period in 2018 was 23.2% higher than that in the LTA; the precipitation variability degree was approximately 38.1% (Supplementary Table 2), and 85.8% of the precipitation was mainly concentrated in the late growing season of winter wheat and the early forage rape growing season (Figure 1). Hence, forage rape grew fast, and the strong root system had a negative impact on the 0–80 cm soil moisture content in the late forage rape growing season. Moreover, the soil moisture consumption by forage rape might not be offset by precipitation (snow and rain) from November to April, which could result in the failure of the subsequent crop. Therefore, it is necessary to implement supplementary irrigation to ensure successful crop development and ultimately achieve optimum yield. In addition, the wheat grain yield in the W-R-W-V treatment were better than those in the W-R-W-R treatment when drought occurred (Table 1; Figure 5); this result was likely due to the impact of the different preceding forage crops between W-R-W-V and W-R-W-R treatments in 2017. Our results showed that eliminating summer fallow with common vetch had a relatively small impact on subsequent wheat production and obtained a relatively high market price compared to that for forage rape. Parihar et al. (1999) analyzed the net return among different crop rotations and found a similar result: the net return in a rice (Oryza sativa L.)-sunflower (Helianthus annuus L.) system was 16.2% lower than that in a rice-peanut (Arachis hypogaea L.) system. Compared to that of forage rape, the shallow root system of common vetch (Gan et al., 2011) can preserve soil moisture for subsequent deeper-rooted crops in the rotation, decreasing the risk of reducing yield (Wang et al., 2012). Figure 4 also shows that the ASWS in the W-R-W-V treatment was greater than that in the W-R-W-R treatment, although no significant difference was found. In addition, in comparison to when wheat monoculture is continuous, when forage legumes are integrated into wheat monoculture, there was a significant increase in N content through biological N fixation (Li et al., 2002; Luce et al., 2016). A similar result was found in the current study, where enhanced NO3−-N and NH4+-N contents were obtained when common vetch was planted in the fallow period. However, planting forage rape had an obvious negative impact on the NO3−-N content in the 0–30 cm soil layer (Figure 2). Of the treatment, the W-F-W-F cropping system had the lowest NO3−-N content, which also explained the reduction in wheat production in the 2018–2019 growing season.
4.2. Evapotranspiration characteristics
In the rainfed regions on the Loess Plateau, precipitation is sufficient but uneven, resulting in frequent drought (Ding et al., 2016). To mitigate the effect of extreme weather and environmental conditions on soil moisture utilization and crop-forage production, ET characteristics of the crop growing season under different cropping systems need to be explored. Our study showed that ET among the cropping systems had no obvious difference during early winter wheat growth, but there was a significant difference in ET during late wheat growth (Figure 3). This difference was mainly related to the precipitation distribution and soil moisture storage. Ding et al. (2018) showed that the amount of local precipitation and its spatiotemporal distribution greatly affect the ET and soil moisture content during winter wheat growing seasons. Fallow practices result in substantial soil evaporation (267–397 mm) because 55.3% of annual precipitation was concentrated in the summer fallow period during the experimental period and the air temperatures in the summer fallow period were relatively high (Figure 1; Supplementary Table 2). However, there was no significant difference in ET between the W-R-W-R and W-R-W-V treatments, indicating that in comparison to the cropping system, precipitation had a much larger impact on ET when the rainfall amount was relatively high on the Chinese Loess Plateau. Ding et al. (2018) reported that relatively low air temperatures in the wheat-growing season result in a decline in soil evaporation, thereby explaining the present study results.
4.3. Crop-water productivity and sustainability
Water is an important driving factor in agriculture, and the efficient utilization of precipitation determines agriculture sustainability (Peterson et al., 1996). Therefore, a system that enhances water storage efficiency and CWP must be developed to ensure agricultural sustainability on the Chinese Loess Plateau. In comparison with the W-F-W-F treatment, all other rotation treatments significantly increased the period of crop coverage in the rainy season and the PUE of the system (Huang et al., 2003). Our results showed that the system PUE in the W-R-W-V treatment presented a greater value than that in the W-R-W-R treatment but no significant difference from that in the W-F-W-F treatment (Figure 5B). This result was primarily because the W-R-W-R treatment had the lowest system DM yield among the treatments, and no significant difference in system DM yield was found between the W-F-W-F and W-R-W-V treatments (Table 1). Evans (1993) also reported that a reduction in the individual crop yield in alternative cropping system intensification system with at least two crops could be offset by a cumulative increase in the annual yield. Our study revealed that the decline in the subsequent winter wheat grain yield in 2018–2019 was adequately offset by the common vetch yield in 2019. The result suggests that compared to the W-R-W-R system, the W-R-W-V system is a plausible alternative cropping system that can ensure Loess Plateau farming system sustainability. Similarly, in an environment with water limitations, efficiency and high production in relation to water use are important to select cropping systems that could be sustainable (Neal et al., 2011). In addition, the SWSD averaged 64.9 mm across the cropping system (Table 2). Although planting forage crops in the summer fallow decreased the FE (Figure 5C), precipitation during the summer fallow period still restored the soil moisture content well. Because precipitation was generally sufficient during the experimental years in this semiarid region of the Loess Plateau compared with the long-term average (Figure 1; Supplementary Table 2), losses in agricultural production do not solely depend on rainfall scarcity but also the seasonal variability in rainfall distribution (Li, 1998). Furthermore, the difference in soil moisture was significant among the cropping systems in 2018 when the forage crop was harvested, but no significant difference was found in 2019 (Figure 4; Supplementary Figure 1), indicating that the effect of substituting summer fallow with forage crops mainly depends on the precipitation distribution on the Loess Plateau. However, Wang et al. (2020) reported 135.9 mm of evaporated water from bare soil for the corresponding period from planting to late harvesting. This value is almost half of the water used by synchronous cover crops in a dry year. Therefore, common vetch should be planted solely or intercropped in a greater proportion when there is limited rainfall during the fallow period. In addition, our study showed that the W-R-W-R cropping system posed a threat to subsequent winter wheat production based on soil moisture consumption. Hence, in comparison to the other treatments, the W-R-W-V system was had a better performance for maintaining the sustainable development of agricultural production. However, since the experimental years in the present study fell above the long term precipitation mean, quantification of soil moisture should be conducted prior to planting fallow crops so as to prevent moisture shortage during the subsequent winter wheat production in the event of low precipitation year (below the long time average). In addition, further study with a longer experimental period that could capture the long term rainfall variability in the Loess Plateau is required.
5. Conclusion
Based on this two-year field study, we found that the challenge to sustainable agricultural production is not necessarily the absolute scarcity of precipitation but rather its uneven seasonal distribution on the Chinese Loess Plateau. In comparison to the W-F-W-F cropping system, planting forage rape and common vetch in summer fallow could increase the average system forage production by 4.93 t ha−1. However, planting these crops decreased the available soil moisture storage and NO3–_N content by an average of 50.3 mm and 54.8%, respectively, resulting in a decline in subsequent winter wheat yield due to the drought resulting from the erratic monthly precipitation distribution in the 2018–2019, especially in the W-R-W-R cropping system. The reduced yield of winter wheat in the system had been offset by the forage yield in the W-R-W-V cropping system which helped to maintain the productivity of the system. Adding forage rape into the W-F-W-F cropping system decreased the CWP of the total system by 40.9%, without a significant difference in PUE. However, the PUE of the W-R-W-V system were 30.1% higher than those in the W-R-W-R system. Therefore, the W-R-W-V cropping system has shown a decreased soil evaporation and could be a stable pattern for grain and forage production that will significantly contribute to the sustainability of soil moisture and fertility on the Chinese Loess Plateau. Further study with a longer experimental period that could capture the long-term rainfall variability in the Loess Plateau is required.
Data availability statement
The original contributions presented in the study are included in the article/Supplementary material, further inquiries can be directed to the corresponding author.
Author contributions
All authors listed have made a substantial, direct, and intellectual contribution to the work and approved it for publication.
Funding
The work was supported by the Natural Science Foundation of China (Grant number: 31872416) and the Program for Gansu Provincial Agriculture and Animal Husbandry Department-Pattern and Key Technology Research Program (Grant number: 160230).
Acknowledgments
We are grateful to thank Longshuai Ma for his valuable time at data analysis and revision. We acknowledge Zhiting Liang, Xingfa Lai, and Quan Cao for help with field data collection.
Conflict of interest
The authors declare that the research was conducted in the absence of any commercial or financial relationships that could be construed as a potential conflict of interest.
Publisher’s note
All claims expressed in this article are solely those of the authors and do not necessarily represent those of their affiliated organizations, or those of the publisher, the editors and the reviewers. Any product that may be evaluated in this article, or claim that may be made by its manufacturer, is not guaranteed or endorsed by the publisher.
Supplementary material
The Supplementary material for this article can be found online at: https://www.frontiersin.org/articles/10.3389/fsufs.2023.1139453/full#supplementary-material
References
Abate, T., Berhanu, T., Bogale, S., and Worku, D. (2003). Potential of forages legumes to replace the traditional fallow-barley rotation system in the cool-high land of bale. Challenges and opportunities of livestock marketing in Ethiopia proceedings of the 10th annual conference of the Ethiopian Society of Animal Production. Addis Ababa, Ethiopia, 21–23 August 2003.
Aiken, R. M., O’Brien, D. M., Olson, B. L., and Murray, L. (2013). Replacing fallow with continuous cropping reduces crop water productivity of semiarid wheat. Agron. J. 105, 199–207. doi: 10.2134/agronj2012.0165
Ao, Y. H., Wen, J., and Lü, S. H. (2007). A study of the water and energy transfer at the soil surface in cropped field on the loess plateau of northwestern China. Environ. Earth Sci. 52, 595–603. doi: 10.1007/s00254-006-0490-z
Blanco-Canqui, H., Shaver, T. M., Lindquist, J. L., Shapiro, C. A., Elmore, R. W., Francis, C. A., et al. (2015). Cover crops and ecosystem services: insights from studies in temperate soils. Agron. J. 107, 2449–2474. doi: 10.2134/agronj15.0086
Campbell, C. A., Zentner, R. P., Basnyat, P., Jong, R. D., Lemke, R., and Desjardins, R. L. (2008). Nitrogen mineralization under summer fallow and continuous wheat in the semiarid Canadian prairie. Can. J. of Soil Sci. 88, 681–696. doi: 10.4141/CJSS07115
Deng, J. Q., Zhang, Z. X., Liang, Z. T., Li, Z., Yang, X. L., Wang, Z. K., et al. (2020). Replacing summer fallow with annual forage improves crude protein productivity and water use efficiency of the summer fallow-winter wheat cropping system. Agric. Water Manag. 230:105890. doi: 10.1016/j.agwat.2019.105980
Ding, D. Y., Feng, H., Zhao, Y., Liu, W. Z., Chen, H. X., and He, J. Q. (2016). Impact assessment of climate change and later-maturing cultivars on winter wheat growth and soil water deficit on the loess plateau of China. Clim. Chang. 138, 157–171. doi: 10.1007/s10584-016-1714-1
Ding, D. Y., Zhao, Y., Feng, H., Hill, R. L., Chu, X. S., Zhang, T. B., et al. (2018). Soil water utilization with plastic mulching for a winter wheat-summer maize rotation system on the loess plateau of China. Agric. Water Manag. 201, 246–257. doi: 10.1016/j.agwat.2017.12.029
Farahani, H. J., Peterson, G. A., and Westfall, D. G. (1998). Dryland cropping intensification: a fundamental solution to efficient use of precipitation. Adv. Agron. 64, 197–223. doi: 10.1016/S0065-2113(08)60505-2
Gan, Y. T., Hamel, C., O’Donovan, J. T., Cutforth, H., Zentner, R. P., Campbell, C. A., et al. (2015). Diversifying crop rotations with pulses enhances system productivity. Sci. Rep. 5:14625. doi: 10.1038/srep14625
Gan, Y. T., Liu, L. P., Cutforth, H., Wang, X. Y., and Ford, G. (2011). Vertical distribution profiles and temporal growth patterns of roots in selected oilseeds, pulses and spring wheat. Crop Pasture Science 62, 457–466. doi: 10.1071/CP10406
Grant, C. A., Peterson, G. A., and Campbell, C. A. (2002). Nutrient considerations for diversified cropping systems in the northern Great Plains. Agron. J. 94, 186–198. doi: 10.2134/agronj2002.1860
Herridge, D. F., Peoples, M. B., and Boddey, R. M. (2008). Global inputs of biological nitrogen fixation in agricultural systems. Plant Soil 311, 1–18. doi: 10.1007/s11104-008-9668-3
Hou, F. J., Nan, Z. B., Xie, Y. Z., Li, X. L., Lin, H. L., and Ren, J. Z. (2008). Integrated crop-livestock production systems in China. Rangel. J. 30, 221–231. doi: 10.1071/RJ08018
Huang, M. B., Shao, M. A., Zhang, L., and Li, Y. S. (2003). Water use efficiency and sustainability of different long-term crop rotation systems in the loess plateau of China. Soil Till. Res. 72, 95–104. doi: 10.1016/S0167-1987(03)00065-5
Jensen, E. S., Peoples, M. B., Boddey, R. M., Gresshoff, P. M., Hauggaard-Nielsen, H., Alves, B. J. R., et al. (2012). Legumes for mitigation of climate change and the provision of feedstock for biofuels and biorefineries. A review. Agron. Sustain. Dev. 32, 329–364. doi: 10.1007/s13593-011-0056-7
Kang, S. Z., Zhang, L., Song, X. Y., Zhang, S. H., Liu, X. Z., Liang, Y. L., et al. (2001). Runoff and sediment loss responses to rainfall and land use in two agricultural catchments on the loess plateau. Hydrol. Process. 15, 977–988. doi: 10.1002/hyp.191
Komarek, A. M., Bell, L. W., Whish, J. P. M., Robertson, M. J., and Bellotti, W. D. (2015). Whole-farm economic, risk and resource-use trade-offs associated with integrating forages into crop–livestock systems in western China. Agric. Syst. 133, 63–72. doi: 10.1016/j.agsy.2014.10.008
Lemke, R. L., Vandenbygaart, A. J., Campbell, C. A., Lafond, G. P., McConkey, B. G., and Grant, B. (2012). Long-term effects of crop rotations and fertilization on soil C and N in a thin black Chernozem in southeastern Saskatchewan. Can. J. Soil Sci. 92, 449–461. doi: 10.4141/cjss2010-047
Li, F. R. (1998). Studies on arid agricultural ecosystems (In Chinese). Shanxi Science and Technology Press, Xian.
Li, F. R., Gao, C. Y., Zhao, H. L., and Li, X. Y. (2002). Soil conservation effectiveness and energy efficiency of alternative rotations and continuous wheat cropping in the loess plateau of Northwest China. Agric. Ecosyst. Environ. 91, 101–111. doi: 10.1016/S0167-8809(01)00265-1
Li, Y., Li, Z., Cui, S., Chang, S. X., Jia, C. L., and Zhang, Q. P. (2019). A global synthesis of the effect of water and nitrogen input on maize (Zea mays) yield, water productivity and nitrogen use efficiency. Agric. For. Meteorol. 268, 136–145. doi: 10.1016/j.agrformet.2019.01.018
Li, S. X., Wang, Z. H., Li, S. Q., Gao, Y. J., and Tian, X. H. (2013). Effect of plastic sheet mulch, wheat straw mulch, and maize growth on water loss by evaporation in dryland areas of China. Agric. Water Manag. 116, 39–49. doi: 10.1016/j.agwat.2012.10.004
Li, Z., Yang, X., Cui, S., Yang, Q., Yang, X. L., Li, J. C., et al. (2018). Developing sustainable cropping systems by integrating crop rotation with conservation tillage practices on the loess plateau, a long-term imperative. Field Crops Res. 222, 164–179. doi: 10.1016/j.fcr.2018.03.027
Li, F. R., Zhao, S. L., and Geballe, G. T. (2000). Water use patterns and agronomic performance for some cropping systems with and without fallow crops in a semi-arid environment of Northwest China. Agric. Ecosyst. Environ. 79, 129–142. doi: 10.1016/S0167-8809(99)00149-8
Liu, Y. B., Tian, L. H., Zhang, Q. P., and Shen, Y. Y. (2014). Grain and forage performances of winter wheat in a rape and wheat rotation system. Pratacultural Sci. 31, 1336–1342.
Luce, M. S., Grant, C. A., Ziadi, N., Zebarth, B. J., O’Donovan, J. T., Blackshaw, R. E., et al. (2016). Preceding crops and nitrogen fertilization influence soil nitrogen cycling in no-till canola and wheat cropping systems. Field Crops Res. 191, 20–32. doi: 10.1016/j.fcr.2016.02.014
Luce, M. S., Lemke, R., Gan, Y. T., Mcconkey, B., May, W., Campbell, C., et al. (2020). Diversifying cropping systems enhances productivity, stability, and nitrogen use efficiency. Agron. J. 112, 1517–1536. doi: 10.1002/agj2.20162
Lyon, D. J., Baltensperger, D. D., Blümenthal, J. M., Burgener, P. A., and Harveson, R. M. (2004). Eliminating summer fallow reduces winter wheat yields but not necessarily system proftability. Crop Sci. 44, 855–860. doi: 10.2135/cropsci2004.8550
Neal, J. S., Fulkerson, W. J., and Hacker, R. B. (2011). Differences in water use efficiency among annual forages used by the dairy industry under optimum and deficit irrigation. Agric. Water Manag. 98, 759–774. doi: 10.1016/j.agwat.2010.11.011
Nielsen, D. C., Lyon, D. J., Hergert, G. W., Higgins, R. K., and Holman, J. D. (2015). Cover crop biomass production and water use in the central Great Plains. Agron. J. 107, 2047–2058. doi: 10.2134/agronj15.0186
Nielsen, D. C., Lyon, D. J., Higgins, R. K., Hergert, G. W., Holman, J. D., and Vigil, M. F. (2016). Cover crop effect on subsequent wheat yield in the central Great Plains. Agron. J. 108, 243–256. doi: 10.2134/agronj2015.0372
Nielsen, D. C., Lyon, D. J., and Miceli-Garcia, J. J. (2017). Replacing fallow with forage triticale in a dryland wheat-corn-fallow rotation may increase profitability. Field Crops Res. 203, 227–237. doi: 10.1016/j.fcr.2016.12.005
Nielsen, D. C., and Vigil, M. F. (2010). Precipitation storage efficiency during fallow in wheat-fallow systems. Agron. J. 102, 537–543. doi: 10.2134/agronj2009.0348
Parihar, S. S., Pandey, D., Shukla, R. K., Verma, V. K., Chaure, N. K., Choudhary, K. K., et al. (1999). Energetics, yield, water use and economics of rice-based cropping system[J]. Indian J. Agron. 44, 205–209.
Peterson, G. A., Schlegel, A. J., Tanaka, D. L., and Jones, O. R. (1996). Precipitation use efficiency as affected by cropping and tillage systems. J. Prod. Agric. 9, 180–186. doi: 10.2134/jpa1996.0180
Ratliff, L. F., Ritchie, J. T., and Cassel, D. K. (1983). A survey of field-measured limits of soil water availability as related to laboratory-measured properties. Soil Sci. Soc. Am. J. 47, 770–775. doi: 10.2136/sssaj1983.03615995004700040032x
Unger, P. W., Schomberg, H., Dao, T. H., and Jones, O. R. (1997). Tillage and crop residue management practices for sustainable dryland farming systems. Ann. Arid Zone 36, 209–232.
Unger, P. W., and Vigil, M. F. (1998). Cover crops effects on soil water relationships. J. Soil Water Conserv. 53, 200–207.
Wang, X. Y., Gan, Y. T., Hamel, C., Lemke, R., and McDonald, C. (2012). Water use profiles across the rooting zones of various pulse crops. Field Crops Res. 134, 130–137. doi: 10.1016/j.fcr.2012.06.002
Wang, Z. K., Jiang, H. L., and Shen, Y. Y. (2020). Forage production and soil water balance in oat and common vetch sole crops and intercrops cultivated in the summer-autumn fallow season on the Chinese loess plateau. Eur. J. Agron. 115:126042. doi: 10.1016/j.eja.2020.126042
Yang, X. L., Lu, Y. L., Tong, Y. A., and Yin, X. F. (2015). A 5-year lysimeter monitoring of nitrate leaching from wheat–maize rotation system: comparison between optimum N fertilization and conventional farmer N fertilization. Agric. Ecosyst. Environ. 199, 34–42. doi: 10.1016/j.agee.2014.08.019
Yang, X. L., Lu, Y. L., Yan, D., Yin, X. F., Raza, S., and Tong, Y. A. (2017). Optimising nitrogen fertilisation: a key to improving nitrogen-use efficiency and minimising nitrate leaching losses in an intensive wheat/maize rotation (2008-2014). Field Crops Res. 206, 1–10. doi: 10.1016/j.fcr.2017.02.016
Yang, X., Zheng, L. N., Yang, Q., Wang, Z. K., Cui, S., and Shen, Y. Y. (2018). Modelling the effects of conservation tillage on crop water productivity, soil water dynamics and evapotranspiration of a maize-winter wheat-soybean rotation system on the loess plateau of China using APSIM. Agric. Syst. 166, 111–123. doi: 10.1016/j.agsy.2018.08.005
Zhang, Q. P., Bell, L. W., Shen, Y. Y., and Whish, J. P. M. (2018). Indices of forage nutritional yield and water use efficiency amongst spring-sown annual forage crops in north-West China. Eur. J. Agron. 93, 1–10. doi: 10.1016/j.eja.2017.11.003
Zhang, Z. X., Christensen, M., Nan, Z. B., Whish, J. P. M., Lindsay, W. B., Wang, J. F., et al. (2019b). Plant development and solar radiation interception of four annual forage plants in response to sowing date in a semi-arid environment. Ind. Crop. Prod. 131, 41–53. doi: 10.1016/j.indcrop.2019.01.028
Zhang, Z. X., Whish, J. P. M., Bell, L. W., and Nan, Z. B. (2017). Forage production, quality and water-use-efficiency of four warm-season annual crops at three sowing times in the loess plateau region of China. Eur. J. Agron. 84, 84–94. doi: 10.1016/j.eja.2016.12.008
Zhang, Z. X., Yu, K. L., Jin, X. L., Nan, Z. B., Wang, J. F., Niu, Y. N., et al. (2019a). Above- and below-ground dry matter partitioning of four warm-season annual crops sown on different dates in a semiarid region. Eur. J. Agron. 109:125918. doi: 10.1016/j.eja.2019.125918
Zhu, X. M., Li, Y. S., Peng, X. L., and Zhang, S. G. (1983). Soils of the loess region in China. Geoderma 29, 237–255. doi: 10.1016/0016-7061(83)90090-3
Keywords: dryland farming system, soil nitrogen, yield, water management, crop water productivity
Citation: Deng J, Zhang Z, Usman S, Yang X, An D and Shen Y (2023) Productivity, water and nitrogen utilization of intensified dryland farming with annual forages on the Chinese Loess plateau. Front. Sustain. Food Syst. 7:1139453. doi: 10.3389/fsufs.2023.1139453
Edited by:
Bin Yao, Institute of Desertification Studies, Chinese Academy of Forestry, ChinaReviewed by:
Haiming Zhao, Hebei Academy of Agricultural and Forestry Sciences, ChinaPeng Zhang, Northwest A&F University, China
Miaochun Fan, Northwest A&F University, China
Copyright © 2023 Deng, Zhang, Usman, Yang, An and Shen. This is an open-access article distributed under the terms of the Creative Commons Attribution License (CC BY). The use, distribution or reproduction in other forums is permitted, provided the original author(s) and the copyright owner(s) are credited and that the original publication in this journal is cited, in accordance with accepted academic practice. No use, distribution or reproduction is permitted which does not comply with these terms.
*Correspondence: Yuying Shen, eXkuc2hlbkBsenUuZWR1LmNu