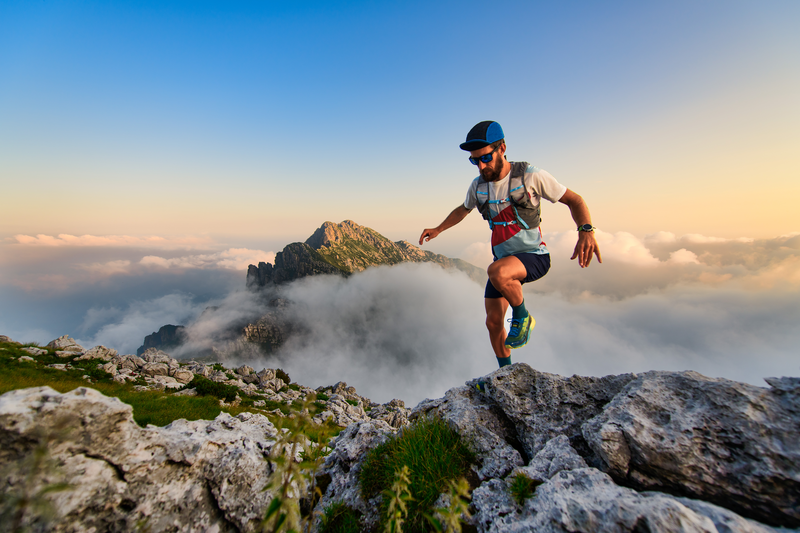
95% of researchers rate our articles as excellent or good
Learn more about the work of our research integrity team to safeguard the quality of each article we publish.
Find out more
REVIEW article
Front. Sustain. Food Syst. , 15 June 2023
Sec. Crop Biology and Sustainability
Volume 7 - 2023 | https://doi.org/10.3389/fsufs.2023.1137683
This article is part of the Research Topic Integrated Pest Management Strategies for Sustainable Food Production View all 6 articles
The environment in which postharvest crops are processed and stored is a dynamic ecosystem influenced not only by environmental factors such as temperature and humidity, but also by biotic influences such as humans and insects. Abiotic influences such as variation in landscapes of warehouses, processing facilities, storage and shipping containers, and urban and agricultural settings can also drive changes in ecosystem processes for insects living in a postharvest system. Principles of community ecology can help to tease apart broad interactions among the environment including succession, interactions with conspecifics that lead to competition and niche partitioning, behavioral ecology variation, and physiology and developmental changes. Focusing on these concepts for integrated pest management (IPM) for stored product insect pests can help pest managers to better predict risk thresholds and develop targeted approaches for treatments. Typically, pest management decisions focus on single species without regards to the interactions with other species. Without consideration of the entire ecosystem, targeted treatments for one species can have both direct and indirect impacts on other species that may have equally detrimental effects on stored products. Current knowledge of stored product ecosystems lags behind what is known for field pest ecosystems, and hinders our ability to design effective control strategies for the whole system. Here, we present a review of work on stored product insect pests using a community ecology lens. We analyze how the current state of the knowledge regarding species interactions and variation and incorporating factors such as movement, species interactions, energy transfer models in succession, behavior, and effects of climate change in ecological modeling can be used to better develop and implement more effective postharvest IPM. Implementing these concepts will significantly improve management of these insects and can help reduce time and cost associated with managing and treating insect infestations.
Odum (1969) defines an ecosystem as an area where living and nonliving organisms exchange materials and interact based on biotic and abiotic conditions. Within an ecosystem there are communities, species, and populations; populations are usually comprised of the same species that occur at the same time and space, although spatial and temporal scales can have a wide range of values, and communities are groups of populations of different species that coexist in the same area and are dependent on one another (Begon et al., 2014). Within a stored-grain environment, there can be a variety of different communities that include insects, mites, fungi and even rodents or birds (White, 1992). Compared to an ecosystem like a forest, stored-grain ecosystems are relatively short-lived (van Bronswijk and Sinha, 1971) but can still be complex and dynamic (Fleurat-Lessard, 2002). In addition, unlike most ecosystems that have a continuous input of energy, stored grain as a resource is limited to the energy that is available when the grain is initially stored. Although new grain can be added to storage environments, and product is moved into and out of warehouses and processing systems, every energy change to the grain itself comes from the ecosystem changes driven by the subsequent reproduction, development and immigration or emigration of insects, fungi, and other community members (Campbell and Sinha, 1978).
All species within a stored product ecosystem have three characteristics, including a strong association with humans across different continents and cultures; the ability to reproduce and increase their populations quickly; and the ability to infest and utilize a variety of foods and processed products (Sinha, 1991). Within any ecosystem there are producers and decomposers, as well as the primary, secondary, and tertiary consumers. Stored product pests can also fill multiple roles in these trophic systems. Humans are included in this food web, as they can remove grain at any time and disrupt further decomposition or succession events. Similar to other natural ecosystems, environmental variables also have a large impact on the niche spaces and successional events. For example, stored wheat in temperate climates tend to have a greater diversity and population level of mite species compared to insects and microorganisms while in tropical regions mites are rarely found and insects are more dominant. A drier climate tends to favor insect only infestations, while in sub-tropical regions, microbes, insects, and mites can all coexist. With the opportunity of coexistence in the sub-tropical regions, dominance of any one type of organism is then highly dependent on the cultural practices before and during storage (Sinha, 1991; Tran et al., 2021).
In much of the stored product literature, organisms are often researched individually for presence, absence, function, or management within the system. However, as noted above, the stored product ecosystem is dynamic and ever-changing, where influences of culture, environment, and commodity can have large impacts on the species and population levels that can colonize and persist in the product. In this manuscript, we discuss how understanding stored product ecosystems is dependent on evaluating the webs of interactions of species and how communities of different species interact within this energy-limited, but easily disturbed, environment. We highlight what research has been done on ecological succession; niche space concepts; behavioral ecology of communities within stored products; physiology, ecology, and development within stored product ecosystems; how community ecology can be impacted by chemical treatments or risk management; how reduced risk management can impact community ecology; and what key gaps there are within this research that could help us better understand stored product ecosystems to best manage pests. This review aims to provide a comprehensive picture of why community ecology is important to the IPM of stored products, and where essential knowledge gaps exist.
Ecological succession is defined as the establishment and extinction of different species in an ecosystem over time (Cain et al., 2008). Establishment of stored-grain ecosystems are driven by changes in temperature and humidity (Sinha, 1973, 1982; White et al., 2011) but are greatly impacted by human-caused disruption as grain is sold, transported, mixed with other grains, or processed (Sinha, 1982). In addition, stored grain is moved, processed, and stored at different times of the year, which can introduce disruptions and impact ecosystem stability (Odum, 1969, 1971; Imura, 1981). In areas where high intensity and high frequency changes occur, there is little chance for establishment of a steady state population of insects. With high intensity and low frequency disturbances, primary succession or pioneer life where no life occurred previously is likely; with medium intensity and medium frequency disturbance events, secondary succession or the reestablishment of individuals is likely, since not all individuals were removed from the environment during these events (Cain et al., 2008).
Stored grain can be subjected to a variety of different intensities and frequencies of disturbance since each stored-grain environment is unique (Figure 1). In addition, climate and the type of commodity plays a significant role in which insect species become established and ultimately how succession proceeds (Loschiavo and Okumura, 1979; Arbogast and Mullen, 1987). In addition, the location within a facility can play a role in altering the composition of the insect community (Tilley et al., 2017). This makes it difficult to predict the outcome of ecological succession in stored product ecosystems with certainty; however, the impacts of certain IPM and storage practices on specific stored product insect populations are relatively well-known. For example, grain is often treated before it is put into storage, which often reduces all live insects or inhibits growth of early life stages (White, 1992; Arthur, 2012). Pretreatment of the grain leaves the grain open to primary succession, mostly by primary pests that feed on intact grains (Dunkel, 1992). If grain is free of mechanical damage or deterioration when it goes into storage, there is a general pattern of succession that flows (Figure 2), starting from primary feeders such as Sitophilus spp. or Rhyzopertha spp. As grain is damaged by primary feeders, secondary pests such as Cryptolestes spp. and Oryzaephilus spp. (Coombs and Woodroffe, 1963, 1968; Arbogast and Mullen, 1988; Trematerra et al., 1999; Mason and McDonough, 2012) can then move into the environment leading to a successional process. As grain continues to deteriorate, scavengers such as dermestids are established, which feed on carcasses, frass, and other waste products of primary and secondary pests as well as other materials left behind (Dunkel, 1992; Mason and McDonough, 2012).
Figure 1. The importance of ecological succession of community colonization by stored product insects, degradation of commodity, and decreasing IPM tactic efficacy in bulk storage over time.
Figure 2. Summary figure of disturbance over time and the effect on quality, kernel (or product) condition, and energy loss in the system. As quality decreases, the number of intact or whole kernels within the system decreases. Energy loss represents the energy within the stored product itself; as energy is transferred to pests, it decreases within the commodity itself. Examples of insects or microbes present over time and in response to different levels of disturbance is also included.
In comparison, if grain is aerated or cooled during storage, insect population growth will be slowed. Once cooling treatment is complete and temperatures rise again, the system is vulnerable to secondary succession and reestablishment of both primary and secondary pests, depending on the deterioration of the grain. Research on grain fumigated after weevil damage also showed that secondary pests recolonized and established larger population numbers because the environment contained damaged grains and was primed for their arrival (Kiritani, 1958). Likewise, if insecticide or fumigant-resistant insect populations are present after fumigation, pests can reestablish quickly. The recovery of species following a disturbance such as insecticide treatment is also dependent on other species present. For example, pest resurgences can occur quickly after a disturbance if a species is suddenly released from a competitive interaction (Cordeiro et al., 2014).
Depending on the initial moisture content of the grain and whether moisture levels increase during storage which can happen due to high insect infestations or structural leaks, fungal communities can also develop (Sinha et al., 1969; van Bronswijk and Sinha, 1971; Hunter et al., 1973; Toews et al., 2006), which can then support populations of fungivorous insects such as Mycetophagidae spp. (Nansen et al., 2004; Mason and McDonough, 2012). Based on these basic successional patterns (Table 1), the presence of the different species in general successional patterns can serve as an indicator of grain quality (Coombs and Woodroffe, 1973; Allotey, 1991). For example, the presence of hairy fungus beetles and psocids can indicate deteriorating grain quality and they may also hasten deterioration as they create metabolic hot spots that contribute to grain warming, moisture increases, and subsequent fungal growth (Sinha and Wallace, 1966; Stejskal et al., 1999; Tsai et al., 2007; Mason and McDonough, 2012). The same pattern has also been observed with other stored product species such as S. oryzae (Fourar-Belaifa et al., 2011). However, this may also be reciprocal, as fungal hotspots can also cause grain temperatures to elevate which draws in insects from surrounding areas (Mason, 2019). Succession can also appear to skip steps, depending on human interventions. For example, Kiritani (1958) reported secondary pests infesting recently fumigated rice, but it suggested this was likely due to previous infestation by weevils that had damaged the kernels enough for the secondary feeders before being killed by fumigation.
Table 1. Summary of successional patterns in stored product pest insects with each study having an independent timeline of new and old grain aging.
Bulk storage of post-harvest products can entail containers of a variety of sizes and shapes. For most low-income countries, most grains (75–85%) are stored on-farm or by small-volume traders (McFarlane and Prevett, 1984; Allotey, 1991). However, specific successional patterns of infestation have rarely been documented in these post-harvest ecosystems. Coombs and Woodroffe (1963, 1968) established some baseline ecological succession patterns both in the laboratory and field. Testing the generalized patterns as described above, Coombs and Freeman (1955) did find that grain that is mostly whole, supported high population levels of the internal feeders Sitophilus granarius and the moth Hofmannophila pseudospretella (Stainton) (Lepidoptera: Oecophoridae). As grain deteriorated and became less whole, spider beetles (Ptinus spp.) and Tenebrio molitor (Linnaeus) (Coleoptera: Tenebrionidae) gradually rose in population numbers and at even poorer grain quality, scavengers such as Attagenus pellio (Linnaeus) (Coleoptera: Dermestidae) and Scenopinus fenestralis (Linnaeus) (Diptera: Scenopinidae) dominated the ecosystem (Coombs and Freeman, 1955). Researchers also noted that dominance was on a sliding scale based on nutritional properties of the grain – as nutrition declined, total numbers of insects also declined (Coombs and Woodroffe, 1963).
Although much work has been done surveying and recording insects found within bulk post-harvest storage environment (Horton, 1981; Toews et al., 2005; Arthur et al., 2006; Toews et al., 2006; Tilley et al., 2017), integrating these findings into a context of ecological succession is challenging. First, long-term (longer than 1–2 years) storage and monitoring is challenging as markets fluctuate and grain is sold at different time intervals. However, there are a few long-term studies that have specifically monitored succession in bulk grain. Sinha et al. (1969) took monthly samples for 8 years from wheat bulks in Winnipeg, Manitoba and used principal-component analysis (PCA) to assess the variables that impacted grain storage most. The top PCA variables were month or time since grain was put into storage bins or the environments where samples were taken (two bins total), depth of sampling, germination or seed viability, temperature (which corresponded mostly to ambient air temperature), and moisture content and was taken at each grain sample. The next most important variables were fungi or actinomycetes species or species groups followed by arthropods that were associated with the grain. These arthropods mostly included mites and lice of aging grain and Cryptolestes ferrugineus (Stephens) (Coleoptera: Laemophloeidae), and a combination of Cryptophagus spp. and Ahasverus spp., which are a group of fungivorous beetles that are most often found in late-season samplings in grain in Canada (Sinha et al., 1969). Overall, these researchers concluded that temperature, moisture, and depth of grain bulk can play the largest role in mite communities but made few conclusions on the impacts and changes to the overall insect community within the grain bulk.
A monthly sampling conducted over an 8-year time period of a corn bulk in southeastern Georgia (USA) provides another nice field example for ecological succession (Arbogast and Mullen, 1988). Nine species were dominant during the sampling period with Sitotroga cereallela (Olivier) (Lepidoptera: Gelechiidae) initially dominant followed by Oryzaephilus surinamensis (Linnaeus) (Coleoptera: Silvanidae), which died out by year three of sampling (Figure 2). During the first four years, Sitophilus zeamais (Motschulsky) (Coleoptera: Curculionidae) was dominant for years 2–3, with C. ferrugineus dominant periodically. A parasitoid of S. zeamais, Anisopteromalus calandrae (Howard) (Hymenoptera: Pteromalidae), had a single peak of dominance in year three and as grain deteriorated in years 3–5, Tribolium castaneum Herbst (Coleoptera: Tenebrionidae) populations colonized the commodity, followed by Latheticus oryzae Waterhouse (Coleoptera: Tenebrionidae). By year eight, the larger black flour beetle, Cyaneus angustus LeConte (Coleoptera: Tenebrionidae) was briefly dominant along with Trogoderma inclusum LeConte (Coleoptera: Dermestidae). Comparisons to work by Coombs and Woodroffe (1963, 1968) also showed similar patterns of succession of insects with O. surinamensis early, then lessening O. surinamensis followed by T. castaneum, which have been known to feed on O. surinamensis (LeCato, 1975a). Similarly, S. granarius, a weevil of cooler temperatures, dominated early storage grain in Canada. Similarly, Horton (1981) and Reed et al. (2003) found populations of Sitophilus, Tribolium, Oryzaephilus, S. cereallela, Plodia interpunctella Hübner (Lepidoptera: Pyralidae), and Rhyzopertha dominica (Fabricius) (Coleoptera: Bostrichidae) in grain sampled early in storage (7 months and 2 years, respectively).
Ecological succession during processing may not be as relevant as ecological succession in bulk storage, as grain is quickly changing form and being moved on to warehouses or packaged for retail. However, the insect species within processing environments are affected by the machinery within the processing facility and seasonal fluctuations (Tilley et al., 2017), as well as the diversity of stages or types of processed commodity present. Feed mills typically support insects with higher thermal tolerances due to heat produced by the milling and pelleting equipment (Mills, 1992). For example, since the use of thermal controls in milling facilities has increased, Ephestia kuehniella (Zeller) (Lepidoptera: Pyralidae) have been found more often in roller mills and T. castaneum, which could not survive colder, unheated mills, are now found more in this environment than the more cold hardy Tribolium confusum duVal (Coleoptera: Tenebrionidae) (Halstead, 1975). Similarly, in Canadian facilities, P. interpunctella, Tribolium spp., and dermestids have shown significant preference and unlimited population growth potential in heated, indoor facilities such as mills and warehouses (White et al., 2011). For the quarantine pest Trogoderma granarium Everts (Coleoptera: Dermestidae), several factors such as the modernization of the kiln environment, which has reduced the overall temperature, have driven a decrease in numbers in malting facilities (Hunter et al., 1973; Halstead, 1975). Monitoring for insects in malt-producing facilities found over 100 different types of arthropod species, but certain species were found in different areas such as on growing floors, where the grain is germinated (Cryptophagus saginatus Sturm (Coleoptera: Cryptophagidae), Cryptophagus cellaris Scopoli (Coleoptera: Cryptophagidae), Cryptophagus pilosus Gyllenhal (Coleoptera: Cryptophagidae), Mycetaea hirta (Marsham) (Coleoptera: Mycetaeidae), Aridius nodifer (Westwood) (Coleoptera: Latridiidae), Lathridius minutus (Linnaeus) (Coleoptera: Latridiidae), Pentarthrum huttoni Wollaston (Coleoptera: Curculionidae), Psychoda spp., and Drosophila spp.), malt stores, where malted grain is stored temporarily (T. granarium, T. castaneum, and T. confusum), or in maltings themselves (Ptinus tectus Boieldieu (Coleoptera: Ptinidae)) (Hunter et al., 1973), indicating different insect successional stages based on stage of production in each area of the processing facility.
Processing also results in more dust, and hidden areas under floorboards or cracks and crevices, which may be consistent environments in terms of temperature and humidity and may harbor insects at a variety of successional stages depending on the age of the residue (Arthur et al., 2006). These microhabitats contain residual dust or grains that fall under floorboards or in spaces that cannot easily be cleaned, and that can become reservoirs for insect pests (Coombs and Woodroffe, 1968, 1973; Athanassiou et al., 2002; Stejskal, 2002; Holditch and Smith, 2020). In a commercial elevator, stable environments such as the boot pit and tunnel support populations such as Sitophilus spp. (Arthur et al., 2006). Coombs and Freeman (1955) found succession in hidden areas, which supported S. granarius and H. pseudospretella with whole kernels but as grain quality deteriorated, Ptinus spp., T. molitor, A. pellio, and S. fenestralis increased in population. Hunter et al. (1973) also documented the transition of S. granarius to P. tectus in a flour mill that had recently been shut down and had ceased production. Because these microhabitats can provide shelter from insecticides and ideal temperature and moisture conditions, understanding when and where these areas can develop can be key to controlling resident populations of insects.
The studies detailed above mainly focus on bulk stored grain since it can be stored for the longest duration (Figure 3). High protein products, such as pet food, can also support populations of stored product insect pests, but few studies have explicitly examined the successional patterns associated with these products. However, several studies focusing on protein rich products note some interesting patterns including a higher prevalence of insect species associated with scavenging. Lasioderma serricorne Fabricius (Coleoptera: Ptinidae), which can have a diverse diet (Strong and Okumura, 1958), was common in feed and pet food stores in Hawaii (Loschiavo and Okumura, 1979) and also occurred in high numbers near dog food, cat food, and horse feed in three department stores in Kansas (Arbogast et al., 2000). The Indian meal moth P. interpunctella was also a common pest of pet food stores (Arbogast et al., 2000; Roesli et al., 2003), grocery stores near the pet food aisles (Platt et al., 1998), and near dried fruits, nuts, cereals and oilseeds (Williams, 1964; Cox and Bell, 1991). The red-legged ham beetle, Necrobia rufipes (De Geer) (Coleoptera: Cleridae), prefers high protein diets and was prevalent in pet food stores and was found near dog food, cat food, and pig ears (Roesli et al., 2003), where it can feed on larvae of other stored product insect pests (Simmons and Ellington, 1925; Gonzalez et al., 1957; Ashman, 1963), and even reproduce on animal carcasses in other situations (De Souza and Linhares, 1997; Roesli et al., 2003). Other insects that had high numbers in feed mills, were scavengers like Attagenus, Trogoderma, Dermestes, and P. tectus as they prefer high protein diets like fish meal, bran, and yeast (Coombs and Woodroffe, 1963; Loschiavo and Okumura, 1979).
Figure 3. At food facilities, there may be intact commodities, processed commodities, and those rich in protein that form the base of food webs. Both insects and microbes use these commodities as food. Some insects specifically use microbes as food, while predators and parasitoids use most of these other organisms as prey. Some predators may also eat other predators (e.g., intraguild predation), while you have species competing for food, undergoing apparent competition with a shared predator, and showing many other food web typologies.
Despite the information described above there has been little application of community ecology tools to develop modeling tools to make management predictions on how these communities can change over time. For example, there are relatively few descriptions of stored product communities in any postharvest ecosystem using common ecology terms such as the Shannon-Wiener Index (H′), in terms of the evenness, species richness (S), or beta diversity (β). These tools have been used successfully for decades to provide information about community structure in other agroecosystems. There has also been little use of community ecology statistical tools such as multidimensional scaling (MDS), principal component analysis (PCA), redundancy analysis (RA), or network analysis. With these tools, calculations of energy transfer or grain deterioration may be useful to managers to decide treatment options and plans. Diversity within a habitat (alpha) compared to between habitats (beta) can also be used to estimate how communities vary in different stored product ecosystems (storage vs. processing) or even within different areas of the same facility which could be considered as the entire landscape (gamma). This may be useful to stakeholders in targeting management to certain parts of a facility that are known to be abundant, for example. At these multiple levels in a stored-product system, alpha diversity can be measured within each on-farm storage bin, beta diversity can be used to compare each bin to one another, and gamma diversity can be used to measure diversity within the entire system of on-farm storage bins, for instance. Accounting for sampling techniques including Monte Carlo resampling, an unbiased method to generate a larger sample size to make better predictions, also needs to be implemented to ensure measures of dominance go hand-in-hand with diversity. Since species abundance and significance in food webs and trophic cascades (Figure 3) can provide alternative targets for treating pests in a stored-product ecosystem, researchers need to understand the interplay of each species to each other instead of a single species being identified as more important than another.
Understanding insect species composition in stored grain can be based on lessons and tools from community ecology on niche space and species adaptations. A niche is defined as a habitat that supplies an organism with the environmental conditions where survival and reproduction can occur (Hutchinson, 1959). Viewed another way, a niche can classically be thought of as an n-dimensional hypervolume, where n represents one of a high-order number of parameters that could define the niche space (Hutchinson, 1959; Blonder et al., 2018). Within the definition of a niche, there is also a fundamental niche or a niche where given the environmental conditions, the organism has unlimited potential, and a realized niche, where the organism can live and reproduce in the same space as competitors and predators. For stored-product insect pests, grain with an appropriate microclimate or moisture content and temperature is fundamentally suitable for survival and reproduction. However, the microclimate can be influenced by insect aggregations, which can increase temperature and moisture and change the quality of the grain as they are consumed (Plarre and Burkholder, 2009) and can drive insects into a more limited realized niche. Microclimate can also be influenced by colonization of grain with microbes and the formation of hotspots of activity (Ponce et al., 2021). Species competition and interaction can also limit niche space. For example, populations of the S. cereallela are often suppressed if other internal feeders such as maize weevils and lesser grain borers are present (Table 2; Mason and McDonough, 2012). Behavioral adaptations by C. ferrugineus, which can hide under seed coats, allow this species to outcompete T. castaneum in many environments (Alabi et al., 2008). Competitive exclusion can also occur in some stored product species, when two species with the same niche compete for a limiting resource (Crombie, 1945; Park, 1948) and ultimately cannot survive together (DeBach, 1966; Ayala, 1970; Pianka, 1974; Allotey and Kumar, 1985). One example of this is Ephestia cautella (Walker) (Lepidoptera: Pyralidae) which competitively excludes Corcyra cephalonica (Stainton) (Lepidoptera: Pyralidae) when raised together (Allotey and Kumar, 1985).
Competition can take on two forms, as defined by Pianka (1974): interference competition where organisms are directly interacting with one another, even exhibiting aggression; and exploitation competition, also known as indirect or apparent competition, when organisms are competing for the same resource or have the same predator (Figure 3). For exploitation competition, leaving or emitting semiochemicals can drive insects to avoid potentially costly interactions with other species (Giunti et al., 2018). For example, T. confusum actively avoided rice infested with C. ferrugineus, as they share a niche space. However, this insect was attracted to chemical cues associated with rice infested with S. zeamais since T. confusum benefits from the damaged kernels of S. zeamais conspecifics (Giunti et al., 2018). Saliva from both conspecifics and different species of larvae can also affect competitive interactions (Mudd, 1973; Mossadegh, 1978, 1980; Poirier and Borden, 1995, 2000) by reducing egg-laying behaviors (Anderson and Löfqvist, 1996).
Founder effects also sometimes influence the outcomes of competition often via interference competition. For example, simultaneous introductions of T. castaneum and T. confusum resulted in T. castaneum becoming dominant; however, allowing the latter species to infest the grain for two weeks prior to the introduction of T. castaneum reduced its dominance (Holditch and Smith, 2020). In some laboratory cases where founder effects have been tested, these do not affect the outcome of competition. Prostephanus truncatus (Horn) (Coleoptera: Bostrichidae), for example, was always found to outcompete Sitophilus oryzae (L.) (Coleoptera: Curculionidae), even when the latter was given a week head start (Baliota et al., 2022). Importantly, not every competitive interaction has clear or predictable outcomes. Many competitive environments result in a scramble type competition, where any insect within the environment experiences reductions in populations due to resource limitations (Nicholson, 1954). This often results in unstable population dynamics, where predictions of the most successful populations are difficult to make, or depend on underlying resource concentrations (Tilman, 1977; Giga and Canhao Sr., 1993; May, 2004). Behavioral differences can also drive what appears to be competitive interactions. For example, in laboratory assays Acanthoscelides obtectus (Say) (Coleoptera: Chrysomelidae) scatter their eggs among stored beans while Zabrotes subfasciatus (Boheman) (Coleoptera: Chrysomelidae) lay their eggs directly on the surfaces of the beans. Although A. obtectus can colonize beans without Z. subfasciatus, the co-occurrence of this species can expedite the ability of A. obectus to colonize beans after hatching or when dispersing to a new patch (Mallqui et al., 2013).
Scramble type competitive interactions occurred when the number of adult P. truncatus and R. dominica in the same space declined significantly when both species were present (Nicholson, 1954; Cordeiro et al., 2014). An example of competitive exclusion occurs when S. oryzae consistently drives out S. zeamais from shared niche space (Birch, 1954; Floyd and Newsom, 1959; Imura, 1981). Temperature, insecticide treatments, and space can influence competitive outcomes greatly (Imura, 1981; Vowotor et al., 2005; Papanikolaou et al., 2018; Quellhorst et al., 2020). Heat and CO2 diffusion (Jayas et al., 1988; Alagusundaram et al., 1990a,b) and rate of cooling of bagged products (Flinn et al., 2015) vary even within a single grain bulk or pallet of product, which creates environmental gradients, which can affect the outcome of competition between species. For example, under laboratory conditions, S. zeamais outcompeted P. truncatus when the insects were at 25°C, but at 30°C there were no consistent patterns (Quellhorst et al., 2020). Trogoderma granarium also reproduces and outcompetes other species such as S. oryzae and R. dominica at higher temperatures, but when reared together at 25°C, seemingly does not impact population growth of the other species (Kavallieratos et al., 2017a,b). In malting facilities, O. surinamensis was able to outcompete T. granarium due to changes in both heating and the increased use of insecticides (Hunter et al., 1973). As household heating began to become common, species like Tribolium destructor Uyttenboogaart (Coleoptera: Tenebrionidae), Oryzaephilus mercator (Fauvel) (Coleoptera: Silvanidae) (Halstead, 1975), and O. surinamensis (Gourgouta et al., 2021) outcompeted other species in households. In moths, S. cereallela and E. cautella have an unstable equilibrium at 25°C and S. cereallela is dominant at 32°C, but declines by 20°C (Odeyemi, 1993). Similarly, when an insecticide was used on competing populations of R. dominica and S. zeamais, R. dominica became the dominant species instead of S. zeamais (Cordeiro et al., 2014). Diet or host also impacts species dominance with R. dominica more successful than S. oryzae on rice but S. oryzae dominating on wheat (Kavallieratos et al., 2017b).
Most of the studies performed to date have only studied competition between two stored product species at any one time (Table 2). However, adding more than two species can significantly impact population success as evidenced by the coexistence of S. cerealella and O. surinamensis. When just the two species were present, S. cerealella coexisted, but when other internal feeders such as S. zeamais and R. dominica were added, the moth population declined (Mason and McDonough, 2012). A handful of studies do consider three or more species (e.g., LeCato, 1975b; Alabi et al., 2008; Yu, 2015; Athanassiou et al., 2017a) but they are relatively few. Even so, it is not possible to conduct factorial tests with the 70 or so common, economically important stored product pests that invade the world’s food supply. A much more practical option would be to model communities of interacting stored product species that could be parameterized from 1-, 2-, or 3-way species studies using different factors. This is yet another very common community ecology tool that could be adopted to speed our understanding of large interacting assemblages of insects in grain bins or at food facilities. Several studies have also detailed spatio-temporal dynamics within feed mills and storage facilities, demonstrating significant variation in preferred locations of different species, which could be a result of a multitude of factors including species interactions, food availability, temperature, and humidity (Trematerra et al., 2004; Gerken and Campbell, 2019). The distribution of species detailed in these studies could lend baseline hypotheses to test for species interactions and competitive exclusion in these ecosystems.
Intraspecific competition, by contrast, generally occurs when a population becomes overcrowded, and is nearing carrying capacity. Cannibalism and the consumption of eggs is common in many Tribolium spp. and they also prefer eggs of conspecifics rather than heterospecifics (Alabi et al., 2008). Eating eggs and pupae regulates population numbers and provides nutrition when food may be scarce (Elgar and Crespi, 1992; Alabi et al., 2008; Holditch and Smith, 2020). Intraspecific competition is also often ameliorated by chemical cues, similarly to interspecific competition (Latifian et al., 2021) and individuals may choose to emigrate away from high density areas (Alabi et al., 2008) or delay pupation (Nakakita, 1982). Of note, however, is that in most laboratory-based assays, emigration away from high density areas may not be possible and this bias should be accounted for in conclusions for competitive outcomes. Jones et al. (1990) also proposed that long-term waste accumulation in stored product insect populations can take on a form of competition, as some insects, such as P. interpunctella, can survive primarily on this waste, but other species will die out via competitive exclusion. For populations with high densities, insecticide treatments also had a much stronger effect on mortality and population levels as evidenced in S. zeamais (Cordeiro et al., 2014), which suggests that the stress of intraspecific competition enhances the effectiveness of insecticide treatments, and possibly other IPM tactics that place a stress on the organism.
Within ecosystems, understanding how predation and parasitism shape interactions with pests is another added layer that can impact community assembly. For stored product insect pests, there are several known predators and research has focused on the potential use of predators as biological control. Predators, microorganisms, and parasites for biocontrol in stored product ecosystems can include both insects and fungi. There are typically three types of functional responses when considering predator–prey relationships (Holling, 1959a,b) and stored product pests (Rahman et al., 2009). Type I is classified with a linear response of predator search rate no matter the density of prey, with a random search pattern until satiation is reached (Hassel, 1978). For Type II, the most common functional response includes an initial constant rate of searching, followed by a decreasing rate that increases as satiation is reached. Finally, Type III has a slow rate of increase in searching followed by a decreased rate of attacks at satiation. A common predator of stored product pests, Xylocoris flavipes (Reuter) (Hemiptera: Anthocoridae), was shown to follow the Type III functional response and researchers found that females will have higher levels of predation compared to males to acquire suitable energy for reproduction (Rahman et al., 2009). However, functional response has rarely been evaluated for other predators and parasitoids (but see, Menon et al., 2002).
In addition to understanding the functional response rates of these predators, other factors such as predator or parasitoid strain, host physiological state, nutritional quality of prey, defensive mechanisms, and cuticle and epicuticular microorganisms, may all affect predator effectiveness and community composition. Carrying capacity associated with body size and nutritional content can have a huge impact on the long-term success and establishment of these predators or fungal communities (Cebolla et al., 2009). For example, in the mite, Cheyletus malaccensis (Oudemans) (Acari: Cheyletidae), which is common in stored grain habitats in central Europe, conspecifics preferred to prey on other mite species but not E. kuehniella eggs. The mites preyed on T. castaneum eggs, but were more likely to increase their cannibalism rates (Pulpán and Verner, 1959) and suffer increased mortality rather than consume insect eggs (Athanassiou and Palyvos, 2006; Palyvos et al., 2006). Consideration of prey developmental times and life history parameters is critical for determining the success of predators (Cebolla et al., 2009). For example, X. flavipes is a well-suited predator for smaller insects but cannot prey on small internal grain feeders (Imamura et al., 2008). Larval stages are the most susceptible life stage of many of these insect predators (Ramos-Rodríguez et al., 2007), but different species carry different proportions of life stages (e.g., more adults versus fewer larvae at any given time) which ultimately contributes to population success.
There may be several undervalued community ecology tools in understanding the natural enemy communities. For example, one tool here may be molecular gut content analysis, wherein rapid PCR tests are used to screen the gut contents of predators or parasitoids to identify prey that they have recently consumed. This has been used successfully to reconstruct networks and guilds of prey consumed by predator groups (e.g., Saqib et al., 2021). In addition, this technique can be used to determine the host range of pest groups when there is uncertainty or switching behavior in the field, such as in the case of the spotted lanternfly, Lycorma delicatula (White) (Hemiptera: Fulgoridae) (Avanesyan and Lamp, 2020). A related but different tool that may help illuminate the community ecology and movement of natural enemies and their pests is the use of in situ protein-marking (e.g., Blaauw et al., 2017; reviewed in Hagler, 2019).
There may be different types of ecological relationships in stored products. Some of these relationships may be classified as commensalism, where one species benefits while the other is neither harmed nor benefits (Cain et al., 2008). For primary pests, initial breakdown of whole kernels is a substantial benefit to secondary feeders, who rely on byproducts to feed and survive (Kiritani, 1958; Coombs and Woodroffe, 1963). Primary pests do not receive any apparent benefits from the secondary feeders, but in many cases there is no real direct competition for niche space or food, as they do not share the same dietary and life history requirements. For stored product insects, there may also be adaptations to promote commensalism relationships as evidenced by T. castaneum, a secondary feeder, with positive chemotaxis toward rice that had previously been infested by S. zeamais, a primary feeder. Interestingly, positive chemotaxis was not observed toward rice that had been colonized by C. ferrugineus, a secondary feeder, which could have resulted in a competitive environment (Giunti et al., 2018). Coombs and Woodroffe (1973) also detailed how the relationship between S. granarius and P. tectus shifts from competition to commensalism. In this situation, S. granarius suppresses populations of P. tectus when whole kernels are present; however, over time, as S. granarius turns whole wheat into frass and husk and begins to experience higher mortality, P. tectus populations expand by feeding on dead S. granarius (Coombs and Woodroffe, 1973). In addition to commensalism, symbiotic relationships need further exploration in stored product ecosystems. A famous example is the mutualism between the symbiont Symbiotaphrina spp. and the stored product pests, Stegobium paniceum (Linnaeus) (Coleoptera: Ptinidae) and L. serricorne (Martinson, 2020). The symbiont produces important B vitamins for the hosts, while obtaining shelter and other nutrients. Martinson (2020) proposed this system as a model for studying symbiotic mutualisms. Additionally, in association with mites in stored products, bacterial communities can thrive in areas where moisture and dead organisms build up over time (Hubert et al., 2006). In one assay, Sitophilus and Tribolium communities were assessed for niche space competition and the researchers found that the buildup of frass promoted bacterial growth, which only heightened the deterioration of stored products (van Bronswijk and Sinha, 1971) and promoted further fungal or mite growth.
Populations of the same species in the same specific location interbreed freely if they are valid biological species. However, isolation by distance, or allopatric speciation, has been known to separate lineages (Toon et al., 2016; Cordeiro et al., 2019) and create locally adapted populations, including those with specific resistance to insecticides (Daglish et al., 2014; Holloway et al., 2016; Toon et al., 2016). These insects can complete their life cycles on very small scales, even for multiple generations confined to a single package of food (Blanc et al., 2006). However, with stored product insect pests comes a large degree of human influence through movement of product and sanitation which in turn influences gene flow and spreads resistance. For the mite, Liposcelis bostrychophila Badonnel (Psocodea: Liposcelididae), there can be clear habitat fragmentation and individuals have little immigration potential. However, distinct populations maintain a medium level of genetic differentiation due to human transport of individuals, with isolation incomplete and periodic gene flow keeping genetic differentiation from reaching higher levels (Wang et al., 2016). Even across 116 populations of L. bostrychophila in Britain, genetic differentiation appeared random, even though there was a large degree of genetic diversity within a population (Ali and Turner, 2001; Nayak et al., 2014). Similarly, for 16 populations of L. serricorne from 15 different countries, there did not appear to be a significant pattern of genetic clustering based on geographic origin (Blanc et al., 2006), suggesting anthropogenic-mediated gene flow among these populations.
For T. castaneum and R. dominica populations in the USA and Australia, gene flow also appears to be substantial (Beeman, 2003; Ridley et al., 2011; Daglish et al., 2014; Cordeiro et al., 2019). Distance can play a factor in population differentiation, but commodity does not seem to be a factor (Cordeiro et al., 2019). However, shipping routes and processing facilities can help track populations, as products such as peanuts and unprocessed rice are rarely shipped north in the USA, and this barrier can contribute to significant population differentiation in T. castaneum (Beeman, 2003). In the moth, E. kuehniella, shipping routes between two mills demonstrated shared population genetics with a distinct population in a third mill that was not along the shipping route (Ryne and Bensch, 2008). However, the lack of anthropogenic mediated movement between two populations does not mean that these populations cannot maintain gene flow if the opportunity presents itself as evidenced by highly divergent lineages of C. ferrugineus, which were able to freely share phosphine resistant alleles through random mating (Toon et al., 2016). By contrast, sympatric speciation, or the formation of new species de novo in the same place, is more controversial (reviewed in Bolnick and Fitzpatrick, 2007) and far less likely with stored product insects given the abundant movement and many common, shared niches.
Behavioral ecology writ large includes the movement, distribution, chemical ecology, mating, and foraging of stored product insects at food facilities. Ideally, improved knowledge about the behavioral ecology of stored product insects will allow food facility managers to better manipulate pest and natural enemy populations to improve protection of postharvest commodities (see Morrison et al., 2021; enumerating the advantages of this approach is beyond this contribution). However, the behavioral ecology of stored product insects may also pose significant challenges to the management of commodities throughout the postharvest agricultural supply chain. One challenge has been weak or inconsistent response to commercially available kairomone or pheromone lures by stored product insects. To this point, eight strains of T. castaneum were assessed for response to kairomone- and pheromone-based lures in three behavioral assays. The study found large variation in attraction to the stimuli among strains, ranging from no attraction to the lures to strong attraction with greater than 80% of individuals moving toward the lures (Gerken et al., 2018). The authors conclude that there is evidence for heritability in behavioral response, but that there may be a large genotype by environment component. Interestingly, the authors did not find any consistent sex-specific effects for preference to either stimuli in any of their behavioral assays. Phillips et al. (1993) found that food kairomones were more attractive when combined with a pheromone synergist to S. oryzae and T. castaneum. A food-based trapping oil was not attractive to T. castaneum in the predecessor to the Dome® Trap when used without a pheromone synergist (Doud and Phillips, 2020). Bringing an ecological lens to bear on this matter will entail calculating the plume reach of these stimuli and determining trapping circumferences for traps that are important for stored product pests (e.g., Miller et al., 2015), as has been done for important invasive species like Halyomorpha halys (Stål) (Hemiptera: Pentatomidae) (Kirkpatrick et al., 2019).
Another challenge may be that semiochemicals in traps may vary in efficacy over time, especially as the traps capture new insects, potentially affecting efficacy of the lures to the traps. For instance, when common commercial traps with kairomone oil contained prior captures of conspecifics, captures of T. castaneum and T. confusum increased, but when the opposite species was first in the trap, captures did not increase (Athanassiou et al., 2016). In a broader study, Athanassiou et al. (2017b) found that prior captures of different but related species of stored product beetles in traps with an otherwise attractive grain oil modulated attraction to traps. By contrast, Gourgouta et al. (2021) found that previous captures of T. variabile or the quarantine pest, T. granarium, did not negatively affect subsequent capture in traps.
While human-mediated movement of insects in and around food facilities is well-understood and appreciated, the dynamics and relative importance of insect immigration from the landscape is less well understood. Mean dispersal distance in wooded habitats and open habitats for R. dominica were 337–375 m and 261–333 m, respectively (Mahroof et al., 2010). Scheff et al. (2021a) found R. dominica abundantly many kilometers away from the nearest food facility on native Kansas tallgrass prairie. Results from Mahroof and Phillips (2012) suggest R. dominica may have a diet that includes both stored products and natural hosts such as acorns. Self-marked T. variabile were able to move across multiple floors in a food facility and between 7–216 m in a warehouse (Campbell et al., 2002). During a heat treatment, Semeao et al. (2013) also found that T. castaneum was willing to move 1–2 floors up or down from the level on which they were marked with the heat treatment. Finally, T. castaneum actively disperses through flight (with prior work documenting flight between 20 and 300 m; Gurdasani et al., 2019) with lower genetic differentiation than historically suspected, and conspecifics have been captured at least 1 km away from the nearest food facility in Australia (Ridley et al., 2011). Thus, it appears that insect immigration from the landscape is an important factor at food facilities. To combat this propensity for movement, work has been evaluating IPM tools to intercept immigrating insects, including long-lasting insecticide netting (Morrison et al., 2018; Rumbos et al., 2018; Wilkins et al., 2021; Scheff et al., 2021b; Ranabhat et al., 2022) and insecticide packaging (Kavallieratos et al., 2017b; Scheff et al., 2020). However, other IPM tools that can exploit the behavior of stored product insects or at least be behaviorally compatible will surely be welcome.
Another challenge is that the behavioral traits of stored product insects make them ideal hitchhikers in global commerce. Most global alien interceptions of foodstuffs via mail were found to be Coleoptera, and Coleoptera comprised three-quarters of interceptions of foodstuffs via baggage and cargo (Fenn-Moltu et al., 2022). Indeed, T. granarium, the khapra beetle, is of particular quarantine concern to many countries around the world (Athanassiou et al., 2018) and in a synthesis of interceptions, T. granarium was the 72nd most intercepted species in biosecurity programs worldwide from 1995–2019 (Turner et al., 2021). Recently, there has been a push to develop new traps and attractants for T. granarium (e.g., Domingue et al., 2020, 2022; Morrison et al., 2020b; Sakka et al., 2023). The resemblance of T. granarium to non-quarantined dermestids, its propensity to engage in diapause under unfavorable conditions, and its inconspicuous nature lend itself to easily escaping notice. Another invasive stored product species is P. truncatus, the larger grain borer, which is endemic to much of Latin America, but has invaded many countries in Africa (reviewed in Quellhorst et al., 2021). Under climate change, the range for P. truncatus may be expected to expand polewards (Arthur et al., 2019). This pest is of particular concern to maize production globally, so it is important that biosecurity programs on the edge of P. truncatus’ current range monitor for this pest (Adler et al., 2022). As P. truncatus expands, it may encounter other maize pests that are already established, so there has been interest in predicting the outcome of competition (e.g., Sakka and Athanassiou, 2018; Quellhorst et al., 2020, 2023). However, the outcomes of competition may be done more cost-effectively using a modeling framework as many species in the community can be parameterized from primary data in the literature. In this way, more complex simulations can be carried out without necessarily needing to juxtapose every pairwise combination (or more) of species against each other. Models can also be used to simulate efficacy of IPM tactics (e.g., Fontenot et al., 2012). In particular, a modeling framework will need to take into account some essential parameters of each species, including the intrinsic rate of increase, survival rate, the carrying capacity, the deleterious effect each species has on the other, and starting population size, among others.
Finally, many stored product insects are excellent at using disparate and temporally variable food sources at food facilities. Unfortunately, sites of spillage and food dust accumulation frequently occur during the normal operation of a facility, and unless there is a strict sanitation protocol, this is likely to benefit stored product pests (Trematerra and Fleurat-Lessard, 2015; Gerken and Campbell, 2021). In a review, there was a 1.3–17-fold decrease in IPM tactic efficacy under poor sanitation compared to improved sanitation conditions (Morrison et al., 2019). The presence of food has been shown to decrease the efficacy of residual insecticides for stored product insects (Arthur, 2013). Some possible explanations for this may be (1) that food allows stored product insects to detoxify insecticides, (2) food helps absorb and disperse the insecticide, or (3) food provides a refuge from insecticide applications. Campbell and Hagstrum (2002) observed that outside food patches T. castaneum tend to be at edges of the environment where they are more often inactive, tend to move along perimeters, and to also move more slowly when at edges. As a consequence, those authors found that flour along edges was more often infested with T. castaneum. Thus, it is possible T. castaneum or other stored product insects may be using these patches as a refuge. Indeed, a two-year survey of spatial and temporal dynamics within a feed mill found strong influences of food availability and species interactions driving species distribution, with T. castaneum found only with raw materials, O. surinamensis and S. paniceum by the conveyer belt and the storeroom, and S. oryzae, and T. confusum near storage bins (Trematerra and Sciarretta, 2004). Sitophilus granarius was also found to oviposit more in larger grains than smaller and actually laid more eggs when fewer grains were available (Stejskal and Kucerova, 1996). More to the point, sanitation affects almost every IPM tactic, including potentially the reliability of monitoring programs when used in conjunction with aerosols (Toews et al., 2010).
On a global scale, there are large variations in climate, storage condition, processing facilities, spatial scales, or temporal scales for postharvest grain. Variation in any one of these factors can create significant influences on the insect and fungal communities, and therefore, impact recommendations for treatment and management. However, insects that infest stored grain share developmental processes, physiological adaptations, and ecological characteristics regardless of their geographic origin. For example, the presence of cryptonephridia in the tenebrionid and dermestid hindgut, offer an adaptation to absorb water one additional time before it is excreted and Tribolium use quinones as antifungal compounds that can reduce fungal populations within their environment (Dunkel, 1992). In comparison, hairy fungus beetles and psocids, which both prefer grain that is deteriorated heavily, can use metabolic heating and moisture to enhance fungal growth, creating a better environment for themselves (Sinha and Wallace, 1966; Mason and McDonough, 2012). The use of diapause, retromolting, or retrogressive molting by over 40 different species of stored product insects is also a major adaptation for the types of environments that these insects live in Bell (1994), Wilches et al. (2016), and Wilches et al. (2017), including an increase in diapause at higher latitudes (Wijayaratne and Fields, 2012). Diapause can help insects avoid a variety of unfavorable conditions, including cold extremes (Wilches et al., 2016, 2017; Andreadis and Athanassiou, 2017) or delay development after phosphine fumigation (Hole et al., 1976).
Another challenge with the management of these pests is the development of insecticide and fumigant resistance, which is increasing globally every year (Nayak et al., 2014). This can be due to improper use, physiological adaptations such as cuticular changes, respiration, or even weak resistance that can increase in frequency with each generation (Stadler et al., 2003; Pimentel et al., 2007; Talukder, 2009; Pimentel et al., 2010; Boyer et al., 2012; Holloway et al., 2016; Attia et al., 2020). Resistance to many insecticides has increased in recent years and across the globe (Attia et al., 2020) and across many different species (Talukder, 2009; Pimentel et al., 2010), especially as monitoring tools have become easier to use and access (Agrafioti et al., 2019). Physiologically, respiration rate, which can change depending on different environmental variables such as temperature, is often correlated with phosphine resistance and can drive resistant populations to increase (Pimentel et al., 2007). Genetic correlations with resistant populations also suggest mitochondrial functions and cuticular components can also have a significant impact on phosphine resistance (Oppert et al., 2015), and human influences can contribute to the spread of these resistance genes (Dunkel, 1992; Holloway et al., 2016; Toon et al., 2016).
Grain storage, without disturbance, is a short-lived system with non-regenerating energy that can appear stable. However, due to environmental and human influences, stored grain has a random lifespan that can be punctuated by disruptions including influences of weather, addition of newer grain, partial removal of grain, or insecticide treatments (Sinha, 1982, 1995). Energy transfer or consumption within the environment changes significantly due to what species are present but energy flow is ultimately low (van Bronswijk and Sinha, 1971). For example, most energy that is consumed by insects is used to make eggs with C. ferrugineus assimilating 66–79% of food in the larval stage but only 3–23% converted to tissue development while R. dominica assimilated almost 38% of food into tissues (Campbell and Sinha, 1978). Initial grain temperature can affect heat of respiration significantly with high contribution of respiration heat from T. castaneum, T. confusum, and R. dominica as temperatures increased, but decreases in heat production for S. granarius under the same conditions (Cofie-Agblor et al., 1995). Although these measurements of energy assimilation and heat production are important for each species, Sinha (1982) also points out that the energy at population, community, and ecosystem levels is necessary for understanding energy transfer in the entire system and extrapolations are limited by a known quantity of grain and a single, specific species. Intrinsic rates of increases at a given temperature can also provide a starting point for using insect energetics to predict food degradation and losses, but only with thermal and humidity information can this information be applied to a variety of climate zones (Howe, 1965; Sinha, 1982; Bjørge et al., 2018). As beneficial as information on energy assimilation, grain deterioration, and correlates to species presence seem to be for making predictions, information is lacking. Tracking grain energy loss and deterioration via species presence and energy assimilation properties at different temperatures and humidities deserves significant attention as a potential method to develop thresholds for treating, rotating, or selling stored products.
Furthermore, climate plays an important role in the development, ecology, and physiology of stored product insect pests (Arbogast and Mullen, 1987). Although microclimates may exist throughout a facility or packaged good, general climatic conditions in the environment surrounding the commodity can have a significant impact on the survival and control of insect pests (Gerken and Morrison, 2022). Thermal preferences among different species can drive population growth rates and ultimately the number of populations that can be produced within each growing season. Long-term monitoring datasets are few but show that there is significant correlation between insects captured in traps outdoors and indoors (Doud and Phillips, 2000; Gerken and Campbell, 2019), suggesting climate will continue to have a large impact on how insects are infesting stored products. Predictions associated with climate change and stored product pests suggest shifts in life history, habitats, and competitive interactions (Stathers et al., 2013) including shifts in insect populations to higher latitudes or toward the poles (Bale, 2002; Arthur et al., 2019). Monitoring insects in the face of a changing climate requires a team effort to gather spatially large, long-term datasets. In addition, prediction involves a multidisciplinary approach, with thermal preferences, behavior, genetic variation, ecology, and physiology all key players in development of risk models (White, 1992; Gerken and Morrison, 2022).
Many decades of community ecology have been spent in pursuit of fundamental truths about how disturbance and perturbations affect ecosystems and ecosystem function (O’Gorman and Emmerson, 2009). Some moderate amount of disturbance has been historically hypothesized to produce the greatest biodiversity (Roxburgh et al., 2004) and ecosystem services (e.g., Morrison and Bohlen, 2010; Kimoto et al., 2012). This has been termed the intermediate disturbance hypothesis, but it has recently fallen out of favor with ecologists, because of its lack of empirical evidence across systems and lack of cogent reasoning for why it should be so (Fox, 2013). Conversely, the resilience of a system can be measured by its propensity to return to the same steady state equilibrium it had prior to a perturbation. Ecosystem function and properties before and after a disturbance may be collectively measuring how resilient a system is (Yi and Jackson, 2021). Writ large, integrated pest management (IPM) tactics may be thought of as forms of disturbance in postharvest food ecosystems (Figure 4). In various ways, an IPM tactic may distort certain parameters in the ecosystem (Table 3). For example, fumigation temporarily but pronouncedly, elevates mortality in the system (Buckman et al., 2013), reducing populations and population growth. But, because fumigation only acts for as long as it is actively applied, mortality soon returns to background levels in the food system, and the remaining insects continue growing as before at their prior steady state, just at lower initial population numbers (Campbell and Arbogast, 2004). Most chemical control treatments, whether organic or conventional, act to increase mortality as a form of disturbance in the system.
Figure 4. Factors controlling the effect size in an IPM tactic created disturbance, including intensity, duration, and frequency. Intensity is controlled by properties of IPM tactic and method of application, while duration is primarily controlled by the properties of the IPM tactic, and frequency is controlled by method of application.
Table 3. Summary of IPM tactics parsed by disturbance parameters, including intensity, duration, and frequency.
Instead of acting on mortality, other IPM tactics may act on other population or ecosystem parameters (Table 3). For example, grain aeration or grain chilling acts on population growth rate and reproduction measures instead of mortality by modifying the abiotic environment. In this case, grain aeration or chilling brings in cooler air in the bulk grain mass to reduce the number of generations produced (Arthur et al., 2020; Morrison et al., 2020a). Insect growth regulators (IGRs) also reduce the number of progeny produced, but instead of accomplishing this by modifying the external abiotic environment, IGRs perform this by creating a hormonal imbalance internally in exposed individuals (Wijayaratne et al., 2018). Even food packaging may modify population or ecosystem parameters, for example decreasing population growth by preventing access to food, increasing mortality (if an insecticide is incorporated; Scheff and Arthur, 2018), or perhaps increasing metabolic stress on individuals by virtue of extra handling time of invading packaging (Scheff et al., 2018).
Each of these IPM tactics represents a disturbance (Figure 4), but depending on the tactic, the disturbance intensity, duration, and frequency may vary between each. For example, depending on type of facility, packaging may just be in one section, but it may be used consistently there. Thus, disturbance from packaging may be low intensity, but long-lasting where it occurs. On the other hand, grain aeration may only be used in the cooler months (Arthur et al., 2003), so may be temporally isolated, but it may have a very intense effect on the environment, and may be employed on a daily basis for months at a time. Therefore, this would be an example of an intense disturbance that is also long-lasting, with the caveat that it only occurs in certain parts of the year. By contrasts, aerosols are typically used on a weekly or every other week basis (Arthur, 2012), and only kill insects actively moving, and have a limited duration of activity. However, aerosols can cover most surfaces and can thus be very intense. Bringing an ecological lens to these important IPM tactics may illuminate new questions and be able to bind them to ecological modeling literature in ways not yet done for stored product insects. For instance, once one knows how a disturbance impacts a population or ecosystem parameter it is targeting, one can model it, and understand how it may vary under conditions that are hard to simulate in the laboratory. This may bring a new, more comprehensive picture about the fundamental and applied biology of stored product insects to the fore, especially in the light of pressing issues such as climate change (Gerken and Morrison, 2022).
While researchers have made progress in understanding generalized patterns of community ecology in stored product ecosystems, the dynamics of the system require more long-term data gathering and a general overall synthesis of the data that is available for stored product pests. Although human influences may seem arbitrary and sudden, many processors, elevator managers, and cooperative units have important deadlines, patterns, and thresholds that they operate by and thus, disturbances to ecosystems are somewhat predictable. Other factors such as climate can also be predictable, although extreme events such as drought, flooding, severe weather, and changes in temperature extremes can be less predictable and increasingly so in the future (Gerken and Morrison, 2022). In the future, pest modeling, incorporating niche space, behavioral ecology, climate, and successional factors up to and including timing of grain deterioration and energy transfer are necessary to provide a full estimate and plan for management of pests (Figure 2).
Although there are programs that have been developed using population demography models to estimate insect populations and predict timelines to certain treatment thresholds (Stejskal, 2000; Flinn et al., 2007; Rossini et al., 2020), some of these older programs are now defunct, and future modeling efforts need to incorporate the ecology and biology of the insects within the population and must be freely available to stakeholders to provide relevant management recommendations for insect populations and associated microbial populations. The quality of these models and predictions also relies on a continuous collection and monitoring of these stored product systems either by researchers or through a network of scientist and citizen scientist validation efforts. General patterns from monitoring information combined with local climatic information from around the globe can provide a baseline for these models, followed by expanded simulations and laboratory assays to expand on given environmental parameters. Incorporating economic impacts associated with population growth and management tactics using these predictive models are also important for implementation and adoption. If storage professionals can demonstrate that using community ecology metrics and models results in reduced costs of treatments and less product lost to insect damage, expanded adoption and refining of models to new climates, commodities, and facilities can expand the success and applicability of these management predictions.
Increased appreciation and uptake of community ecology statistical tools will help stored product entomologists and stakeholders better understand stored product ecosystem processes after harvest. This includes using multivariate statistical tools (Stratton et al., 2023), as well as machine learning, and the gamut of alpha, beta, and gamma species richness to describe a community. Their consistent use will help develop novel information about a variety of stored product hosts, food facilities, community interactions, and successional states. This, in turn, will hopefully help support the development of more robust postharvest IPM programs for a variety of commodities. In addition, mathematical and theoretical models can be used to understand population dynamics in these ecosystems. These models can range from simple models that invoke “if-then” scenarios to agent-based models that use complex neural networks (DeAngelis and Diaz, 2019), including neural ordinary differential equations (Bonnaffé et al., 2021). Species abundance models are also gaining popularity in understanding species distributions over time and space (Antão et al., 2021; Tsafack et al., 2021). These models can also play a role in economic estimations with information from stakeholders to inform on decision-making within a particular market scenario (Heckbert et al., 2010). Models using impulsive control strategies could also be implemented using differential timing of selling or moving a stored commodity to understand species’ interactions (Yu et al., 2009).
There are several frontiers to be explored in behavioral ecology. One of these includes looking at fine-scale movement and dispersal of insects that takes place to and from the landscape within a food facility. Newer techniques such as miniaturized harmonic radar, protein-marking, and gut-content analysis can generate new information about dispersal and dispersal pathways into food facilities. These techniques have successfully been used to ask questions about movement and dispersal between cash and cover crops in fruit orchards before harvest (Blaauw et al., 2017). It is also necessary to understand how outside landscapes alter pest communities at food facilities, and if scale (1–2 km diameter habitat mosaic) around a facility is the relevant driver of pest community composition. Similar questions have been asked successfully about the natural enemy community in pre-harvest agriculture in field crops (e.g., Gardiner et al., 2009). Finally, future-looking technology that enhances precision agriculture after harvest is increasingly important. Automated monitoring systems including artificial intelligence for species identification and robotics or drones to facilitate scouting for insect distribution will use internet or satellite-enabled pheromone-traps to inform food facility managers of pest infestations. Acoustic monitoring, including for specific life stages, should also continue to be explored as an automated method of insect detection (Fleurat-Lessard et al., 2006). These tools will increasingly support the tailoring of management tactics to specific areas of a facility and allow managers to respond more quickly.
Understanding energy dynamics associated with stored products is also an area that could greatly improve models, and empirical data for energy consumption and transfer needs expansion to more insects and more environments. Various groups have quantified energetic capacities in some insects (Campbell and Sinha, 1978; Sinha, 1982; Cofie-Agblor et al., 1995) and these estimates can be used as a baseline for further investigations and implementation into understanding how energy changes can help predict the life cycle of the grain as the producer and the other successional stages associated with insects, fungi, or other microorganisms (Sinha, 1982; White, 1992). Some research associated with grain deterioration with and without S. oryzae has shown promise in developing a “biodeterioration susceptibility index” and extensions of this with community ecology factors can provide more information on how the entire ecosystem contributes to deterioration and infestation of grain (Fourar-Belaifa et al., 2011). How energy is transferred in an energy limited ecosystem can also provide an understanding of the potential of deterioration and how preparation of stored products by limiting moisture or preventing a certain percentage threshold of insect infestation can impact the lifetime success of storage processes.
As small and closed as these stored product ecosystems may appear, research has demonstrated that there is significant gene flow associated with insects infesting grain products (Blanc et al., 2006; Ryne and Bensch, 2008; Daglish et al., 2014; Toon et al., 2016; Wang et al., 2016; Cordeiro et al., 2019). Thus, understanding paths of the grain from harvest to storage would provide a contact-tracing monitoring system that could be accessed when significant pests are found within a given product. By identifying the larger network of grain movement from harvest to storage to processing, events that result in extreme costs or damage could be avoided by triggering increased monitoring or treatment efforts. A large, coordinated effort of this scale would require a network of both local storage to large-scale storage and may prove difficult based on customs and cultural influences. However, it would provide pathway information with which to manage intense outbreaks or focused treatments as needed.
As discussed by Ehler and Bottrell (2000) there can be an “Illusion of Integrated Pest Management” as they highlight that using a variety of tactics does not constitute IPM. Instead, it may even exacerbate problems, where managers do not understand the consequences of removing one pest species and the impact that it has on the rest of the trophic cascade (White, 1992; Ehler and Bottrell, 2000). Other pest management tactics, such as intermittent sanitation, may flush pests into a commodity storage area. In addition, other pests can arrive and fill the open niche space, or secondary pests can invade more quickly and cause greater damage, costing the producers and consumers more money. However, consistent sanitation has been shown to reduce residual populations and could also remove key members of a trophic cascade (Morrison et al., 2019), aiding in long-term insect pest management. Although there is an extensive amount of literature relating to concepts of community ecology in stored-product insects, more synthesis is needed to gauge general trends from specific circumstances and understand the impacts of a wider range of influences on the system from humans to climate to other insects within the ecosystem. Understanding stored product community ecology can significantly impact the utilization and success of IPM and can save time and resources by having focused targets and understanding of the potential consequences of manipulating the ecosystem.
AG and WM participated equally in the conception, outlining, writing, editing, and approval of this manuscript. All authors contributed to the article and approved the submitted version.
This work was funded, in part, by a United States Department of Agriculture, National Institute of Food and Agriculture, Crop Protection and Pest Management grant #2020-70006-33000.
The authors thank the Gerken and Morrison Laboratories. The use of trade names is for the purposes of providing scientific information only and does not constitute endorsement by the United States Department of Agriculture. The USDA is an equal opportunity employer.
The authors declare that the research was conducted in the absence of any commercial or financial relationships that could be construed as a potential conflict of interest.
All claims expressed in this article are solely those of the authors and do not necessarily represent those of their affiliated organizations, or those of the publisher, the editors and the reviewers. Any product that may be evaluated in this article, or claim that may be made by its manufacturer, is not guaranteed or endorsed by the publisher.
Adler, C., Athanassiou, C., Carvalho, M. O., Emekci, M., Gvozdenac, S., Hamel, D., et al. (2022). Changes in the distribution and pest risk of stored product insects in Europe due to global warming: need for pan-European pest monitoring and improved food-safety. J. Stored Prod. Res. 97:101977. doi: 10.1016/j.jspr.2022.101977
Agrafioti, P., Athanassiou, C. G., and Nayak, M. K. (2019). Detection of phosphine resistance in major stored-product insects in Greece and evaluation of a field resistance test kit. J. Stor. Prod. Res, 82, 40–47. doi: 10.1016/j.jspr.2019.02.004
Alabi, T., Michaud, J., Arnaud, L., and Haubruge, E. (2008). A comparative study of cannibalism and predation in seven species of flour beetle. Ecol. Entomol. 33, 716–726. doi: 10.1111/j.1365-2311.2008.01020.x
Alagusundaram, K., Jayas, D., White, N., and Muir, W. (1990a). Finite difference model of three-dimensional heat transfer in grain bins. Can. Agric. Eng. 32, 315–321.
Alagusundaram, K., Jayas, D., White, N., and Muir, W. (1990b). Three-dimensional, finite element, heat transfer model of temperature distribution in grain storage bins. Trans. ASAE 33, 577–0584.
Ali, N., and Turner, B. (2001). Allozyme polymorphism and variability in permethrin tolerance in British populations of the parthenogenetic stored product pest Liposcelis bostrychophila (Liposcelididae, Psocoptera). J. Stored Prod. Res. 37, 111–125. doi: 10.1016/S0022-474X(00)00013-8
Allotey, J. (1991). Storage insect pests of cereal in small scale farming community and their control. Int. J. Trop. Insect Sci. 12, 679–693. doi: 10.1017/S1742758400013187
Allotey, J., and Kumar, R. (1985). Competition between Corcyra cephalonica (Stainton) and Ephestia cautella (Walker) in cocoa beans. Int. J. Trop. Insect Sci. 6, 627–632. doi: 10.1017/S1742758400009231
Anderson, P., and Löfqvist, J. (1996). Asymmetric oviposition behaviour and the influence of larval competition in the two pyralid moths Ephestia kuehniella and Plodia interpunctella. Oikos 76, 47–56. doi: 10.2307/3545747
Andreadis, S. S., and Athanassiou, C. G. (2017). A review of insect cold hardiness and its potential in stored product insect control. Crop Prot. 91, 93–99. doi: 10.1016/j.cropro.2016.08.013
Antão, L. H., Magurran, A. E., and Dornelas, M. (2021). The shape of species abundance distributions across spatial scales. Front. Ecol. Evol. 9:626730. doi: 10.3389/fevo.2021.626730
Arbogast, R. T., Kendra, P. E., Mankin, R. W., and McGovern, J. E. (2000). Monitoring insect pests in retail stores by trapping and spatial analysis. J. Econ. Entomol. 93, 1531–1542. doi: 10.1603/0022-0493-93.5.1531
Arbogast, R. T., and Mullen, M. A. (1987). Dynamics of Sitotroga cerealella (Olivier)(Lepidoptera: Gelechiidae) and Sitophilus zeamais Motschulsky (Coleoptera: Curculionidae) populations in a small bulk of stored corn. Popul. Ecol. 29, 1–15. doi: 10.1007/BF02515421
Arbogast, R., and Mullen, M. (1988). Insect succession in a stored-corn ecosystem in Southeast Georgia. Ann. Entomol. Soc. Am. 81, 899–912. doi: 10.1093/aesa/81.6.899
Arthur, F. H. (2012). Aerosols and contact insecticides as alternatives to methyl bromide in flour mills, food production facilities, and food warehouses. J. Pest. Sci. 85, 323–329. doi: 10.1007/s10340-012-0439-9
Arthur, F. H. (2013). Dosage rate, temperature, and food source provisioning affect susceptibility of Tribolium castaneum and Tribolium confusum to chlorfenapyr. J. Pest. Sci. 86, 507–513. doi: 10.1007/s10340-013-0495-9
Arthur, F. H., Hagstrum, D. W., Flinn, P. W., Reed, C. R., and Phillips, T. W. (2006). Insect populations in grain residues associated with commercial Kansas grain elevators. J. Stored Prod. Res. 42, 226–239. doi: 10.1016/j.jspr.2005.02.003
Arthur, F., Morrison, W., and Morey, A. C. (2019). Modeling the potential range expansion of larger grain borer, Prostephanus truncatus (Coleoptera: Bostrichidae). Sci. Rep. 9:6862. doi: 10.1038/s41598-019-42974-5
Arthur, F. H., Morrison, W. R., and Trdan, S. (2020). Feasibility of using aeration to cool wheat stored in Slovenia: a predictive modeling approach using historical weather data. Appl. Sci. 10, 1–11. doi: 10.3390/app10176066
Arthur, F. H., Takahashi, K., Hoernemann, C. K., and Soto, N. (2003). Potential for autumn aeration of stored rough rice and the potential number of generations of Sitophilus zeamais Motschulsky in milled rice in Japan. J. Stored Prod. Res. 39, 471–487. doi: 10.1016/S0022-474X(02)00038-3
Ashman, F. (1963). Factors affecting the abundance of the copra beetle, Necrobia rufipes (Deg.) (Col., Cleridae). Bull. Entomol. Res. 53, 671–680. doi: 10.1017/S0007485300048422
Athanassiou, C. G., Kavallieratos, N. G., and Campbell, J. F. (2016). Capture of Tribolium castaneum and Tribolium confusum (Coleoptera: Tenebrionidae) in floor traps: the effect of previous captures. J. Econ. Entomol. 109, 461–466. doi: 10.1093/jee/tov307
Athanassiou, C. G., Kavallieratos, N. G., and Campbell, J. F. (2017a). Competition of three species of Sitophilus on rice and maize. PLoS One 12:e0173377. doi: 10.1371/journal.pone.0173377
Athanassiou, C. G., Kavallieratos, N. G., and Campbell, J. F. (2017b). Effect of the presence of live or dead insects on subsequent captures of six stored-product beetle species: the relative species matters. J. Econ. Entomol. 110, 770–775. doi: 10.1093/jee/tow213
Athanassiou, C. G., and Palyvos, N. E. (2006). Laboratory evaluation of two diatomaceous earth formulations against Blattisocius keegani fox (Mesostigmata, Ascidae) and Cheyletus malaccensis oudemans (Prostigmata, Cheyletidae). Biol. Control 38, 350–355. doi: 10.1016/j.biocontrol.2006.04.007
Athanassiou, C., Palyvos, N., Eliopoulos, P., and Papadoulis, G. T. (2002). Mites associated with stored seed cotton and related products in Greece. Phytoparasitica 30, 387–394. doi: 10.1007/BF02979686
Athanassiou, C. G., Phillips, T. W., and Wakil, W. (2018). Biology and control of the khapra beetle, Trogoderma granarium, a major quarantine threat to global food security. Annu. Rev. Entomol. 64, 1–18. doi: 10.1146/annurev-ento-011118-111804
Attia, M. A., Wahba, T. F., Shaarawy, N., Moustafa, F. I., Guedes, R. N. C., and Dewer, Y. (2020). Stored grain pest prevalence and insecticide resistance in Egyptian populations of the red flour beetle Tribolium castaneum (Herbst) and the rice weevil Sitophilus oryzae (L.). J. Stored Prod. Res. 87:101611. doi: 10.1016/j.jspr.2020.101611
Avanesyan, A., and Lamp, W. O. (2020). Use of molecular gut content analysis to decipher the range of food plants of the invasive spotted lanternfly, Lycorma delicatula. Insects 11:215. doi: 10.3390/insects11040215
Ayala, F. (1970). “Competition, co-existence and evolution” in Essays in evolution and genetics. eds. M. L. Hetch and W. C. Steere (New York, NY: Appleton-Century-Crofts), 121–158.
Bale, J. (2002). Insects and low temperatures: from molecular biology to distributions and abundance. Philos. Trans. R. Soc. Lond. Ser. B Biol. Sci. 357, 849–862. doi: 10.1098/rstb.2002.1074
Baliota, G. V., Scheff, D. S., Morrison, W. R., and Athanassiou, C. G. (2022). Competition between Prostephanus truncates and Sitophilus oryzae on maize: the species that gets there first matters. Bull. Entomol. Res. 112, 520–527. doi: 10.1017/S000748532100105X
Beeman, R. W. (2003). Distribution of the Medea factor M4 in populations of Tribolium castaneum (Herbst) in the United States. J. Stored Prod. Res. 39, 45–51. doi: 10.1016/S0022-474X(02)00016-4
Begon, M., Howarth, R. W., and Townsend, C. R. (2014). Essentials of ecology. 4th Edn, Wiley: Danvers, MA.
Bell, C. (1994). A review of diapause in stored-product insects. J. Stored Prod. Res. 30, 99–120. doi: 10.1016/0022-474X(94)90189-9
Birch, L. (1954). Experiments on the relative abundance of two sibling species of grain weevils. Aust. J. Zool. 2, 66–74. doi: 10.1071/ZO9540066
Bjørge, J. D., Overgaard, J., Malte, H., Gianotten, N., and Heckman, L.-H. (2018). Role of temperature on growth and metabolic rate in the tenebrionid beetles Alphitobius diaperinus and Tenebrio molitor. J. Insect Phys. 107, 89–96. doi: 10.1016/j.jinsphys.2018.02.010
Blaauw, B. R., Morrison, III W. R., Mathews, C., Leskey, T. C., and Nielsen, A. L. (2017). Measuring host plant selection and retention of Halyomorpha halys by a trap crop. Entomol. Exp. Appl. 163, 197–208. doi: 10.1111/eea.12571
Blanc, M., Lugon-Moulin, N., Panighini, C., Pijnenburg, H., and Rossi, L. (2006). Structure of worldwide populations of Lasioderma serricorne (Coleoptera: Anobiidae) as revealed by amplified fragment length polymorphism profiles. Bull. Entomol. Res. 96, 111–116. doi: 10.1079/BER2005405
Blonder, B., Babich Morrow, C., Maitner, B., Harris, D. J., Lamanna, C., Violle, C., et al. (2018). New approaches for delineating n-dimensional hypervolumes. Methods Ecol. Evol. 9, 305–319. doi: 10.1111/2041-210X.12865
Bolnick, D. I., and Fitzpatrick, B. M. (2007). Sympatric speciation: models and empirical evidence. Annu. Rev. Ecol. Evol. Syst. 38, 459–487. doi: 10.1146/annurev.ecolsys.38.091206.095804
Bonnaffé, W., Sheldon, B. C., and Coulson, T. (2021). Neural ordinary differential equations for ecological and evolutionary time-series analysis. Methods Ecol. Evol. 12, 1301–1315. doi: 10.1111/2041-210X.13606
Boyer, S., Zhang, H., and Lempérière, G. (2012). A review of control methods and resistance mechanisms in stored-product insects. Bull. Entomol. Res. 102, 213–229. doi: 10.1017/S0007485311000654
Buckman, K. A., Campbell, J. F., and Subramanyam, B. (2013). Tribolium castaneum (Coleoptera: Tenebrionidae) associated with rice mills: fumigation efficacy and population rebound. J. Econ. Entomol. 106, 499–512. doi: 10.1603/EC12276
Cain, M. L., Bowman, W. D., and Hacker, S. D. (2008). Ecology. Sunderland, MA: Sinauer Associates, Inc.
Campbell, J. F., and Arbogast, R. T. (2004). Stored-product insects in a flour mill: population dynamics and response to fumigation treatments. Entomol. Exp. Appl. 112, 217–225. doi: 10.1111/j.0013-8703.2004.00197.x
Campbell, J. F., and Hagstrum, D. W. (2002). Patch exploitation by Tribolium castaneum: movement patterns, distribution, and oviposition. J. Stored Prod. Res. 38, 55–68. doi: 10.1016/S0022-474X(00)00042-4
Campbell, J. F., Mullen, M. A., and Dowdy, A. K. (2002). Monitoring stored-product pests in food processing plants with pheromone trapping, contour mapping, and mark-recapture. J. Econ. Entomol. 95, 1089–1101. doi: 10.1093/jee/95.5.1089
Campbell, A., and Sinha, R. (1978). Bioenergetics of granivorous beetles, Cryptolestes ferrugineus and Rhyzopertha dominica (Coleoptera: Cucujidae and Bostrichidae). Can. J. Zool. 56, 624–633. doi: 10.1139/z78-090
Cebolla, R., Pekár, S., and Hubert, J. (2009). Prey range of the predatory mite Cheyletus malaccensis (Acari: Cheyletidae) and its efficacy in the control of seven stored-product pests. Biol. Control 50, 1–6. doi: 10.1016/j.biocontrol.2009.03.008
Cofie-Agblor, R., Muir, W., and Sinha, R. (1995). Comparative heat of respiration of five grain beetles in stored wheat. Postharvest Biol. Technol. 5, 167–175. doi: 10.1016/0925-5214(94)00007-F
Coombs, C., and Freeman, J. (1955). The insect fauna of an empty granary. Bull. Entomol. Res. 46, 399–417. doi: 10.1017/S0007485300030996
Coombs, C., and Woodroffe, G. (1963). An experimental demonstration of ecological succession in an insect population breeding in stored wheat. J. Anim. Ecol. 32, 271–279. doi: 10.2307/2540
Coombs, C., and Woodroffe, G. (1968). Changes in the arthropod fauna of an experimental bulk of stored wheat. J. Appl. Ecol. 5, 563–574. doi: 10.2307/2401632
Coombs, C., and Woodroffe, G. (1973). Evaluation of some of the factors involved in ecological succession in an insect population breeding in stored wheat. J. Anim. Ecol. 42, 305–322. doi: 10.2307/3287
Cordeiro, E. M., Campbell, J. F., Phillips, T., and Akhunov, E. (2019). Isolation by distance, source-sink population dynamics and dispersal facilitation by trade routes: impact on population genetic structure of a stored grain pest. G3-Genes Genom. Genet. 9, 1457–1468. doi: 10.1534/g3.118.200892
Cordeiro, E. M. G., Correˆa, A. S., and Guedes, R. N. C. (2014). Insecticide-mediated shift in ecological dominance between two competing species of grain beetles. PloS One 9:e100990. doi: 10.1371/journal.pone.0100990
Cox, P., and Bell, C. (1991). “Biology and ecology of moth pests of stored foods” in Ecology and Management of Food-Industry Pests. ed. J. R. Gorham (Gaithersburg, MD: The Association of Official analytical Chemists), 181–193.
Crombie, A. (1945). On competition between different species of graminivorous insects. Proc. Royal Soc. Lond. B Biol. Sci. 132, 362–395. doi: 10.1098/rspb.1945.0003
Daglish, G., Ridley, A., Hereward, J., Emery, R., Holloway, J., Raghu, S., et al. (2014). “Investigation of dispersal and spatio-temporal distributions of stored grain insects in Australia using ecological and molecular tools” in Proceedings of the 11th international working conference on stored product protection. eds. F. H. Arthur, R. Kengkanpanich, W. Chayaprasert, and D. Suthisut, IWCSPP Organizing Committee, (Chiang Mai, Thailand), 74–78.
DeAngelis, D. L., and Diaz, S. G. (2019). Decision-making in agent-based modeling: a current review and future prospectus. Front. Ecol. Evol. 6:237. doi: 10.3389/fevo.2018.00237
DeBach, P. (1966). The competitive displacement and coexistence principles. Annu. Rev. Entomol. 11, 183–212. doi: 10.1146/annurev.en.11.010166.001151
Domingue, M. J., Morrison, W. R., Yeater, K., and Myers, S. W. (2020). Oleic acid emitted from frozen Trogoderma spp. larvae causes conspecific behavioral aversion. Chemoecology 30, 161–172. doi: 10.1007/s00049-020-00307-3
Domingue, M. J., Scheff, D. S., Leva, N., and Myers, S. W. (2022). Climbing ability of Trogoderma granarium larvae on artificial household and insecticide-treated materials. J. Stored Prod. Res. 95:101922. doi: 10.1016/j.jspr.2021.101922
Domingue, M. J., Wu, Y., Vieira, K. A., McGraw, A. R., Furtado, M., Athanassiou, C. G., et al. (2023). Direct competition and potential displacement involving managed Trogoderma stored product pests. Sci. Rep. 13:3656. doi: 10.1038/s41598-023-30618-8
Doud, C. W., and Phillips, T. W. (2000). Activity of Plodia interpunctella (Lepidoptera: Pyralidae) in and around flour mills. J. Econ. Entomol. 93, 1842–1847. doi: 10.1603/0022-0493-93.6.1842
Doud, C. W., and Phillips, T. W. (2020). Responses of red flour beetle adults, Tribolium castaneum (Coleoptera: Tenebrionidae), and other stored product beetles to different phermone trap designs. Insects 11:733. doi: 10.3390/insects11110733
Dunkel, F. V. (1992). The stored grain ecosystem: a global perspective. J. Stored Prod. Res. 28, 73–87. doi: 10.1016/0022-474X(92)90017-K
Ehler, L. E., and Bottrell, D. G. (2000). The illusion of integrated pest management. Issues Sci. Technol. 16, 61–64.
Elgar, M. A., and Crespi, B. J. (1992). Cannibalism: Ecology and evolution among diverse taxa. Oxford: Oxford University Press.
Fenn-Moltu, G., Ollier, S., Caton, B., Liebhold, A. M., Nahrung, H., Pureswaran, D. S., et al. (2022). Alien insect dispersal mediated by the global movement of commodities. Ecol. Appl. 33:e2721. doi: 10.1002/eap.2721
Fleurat-Lessard, F. (2002). Qualitative reasoning and integrated management of the quality of stored grain: a promising new approach. J. Stor. Prod. Res. 38, 593–613. doi: 10.1016/S0022-474X(01)00022-4
Fleurat-Lessard, F., Tomasini, B., Kostine, L., and Fuzeau, B. (2006). "Acoustic detection and automatic identification of insect stages activity in grain bulks by noise spectra processing through classification algorithms. I. Lorini, B. Bacaltchuk, and H. Beckel, Deckers, E., Sundfeld, J. P., dos Santos, J. D., et al. Proceedings of the 9th International Working Conference on Stored-Product Protection. Brazilian Post-harvest Association: Campinas, São Paulo, 476–486.
Flinn, P., Arthur, F., Throne, J. E., Friesen, K., and Hartzer, K. (2015). Cold temperature disinfestation of bagged flour. J. Stored Prod. Res. 63, 42–46. doi: 10.1016/j.jspr.2015.06.001
Flinn, P., Hagstrum, D., Reed, C., and Phillips, T. (2007). Stored grain advisor pro: decision support system for insect management in commercial grain elevators. J. Stored Prod. Res. 43, 375–383. doi: 10.1016/j.jspr.2006.09.004
Floyd, E., and Newsom, L. (1959). Biological study of the rice weevil complex. Ann. Entomol. Soc. Am. 52, 687–695. doi: 10.1093/aesa/52.6.687
Fontenot, E. A., Arthur, F. H., Nechols, J. R., and Throne, J. E. (2012). Using a population growth model to simulate response of Plodia interpunctella Hübner to temperature and diet. J. Pest. Sci. 85, 163–167. doi: 10.1007/s10340-011-0411-0
Fourar-Belaifa, R., Fleurat-Lessard, F., and Bouznad, Z. (2011). A systemic approach to qualitative changes in the stored-wheat ecosystem: prediction of deterioration risks in unsafe storage conditions in relation to relative humidity level, infestation by Sitophilus oryzae (L.), and wheat variety. J. Stor. Prod. Res. 47, 48–61. doi: 10.1016/j.jspr.2010.09.002
Fox, J. W. (2013). The intermediate disturbance hypothesis should be abandoned. Trends Ecol. Evol. 28, 86–92. doi: 10.1016/j.tree.2012.08.014
Gardiner, M. M., Landis, D. A., Gratton, C., DiFonzo, C. D., O’neal, M., Chacon, J. M., et al. (2009). Landscape diversity enhances biological control of an introduced crop pest in the north‐central USA. Ecol. Appl, 19, 143–154. doi: 10.1890/07-1265.1
Gerken, A. R., and Campbell, J. F. (2019). Using long-term capture data to predict Trogoderma variabile Ballion and Plodia interpunctella (Hübner) population patterns. Insects 10:93. doi: 10.3390/insects10040093
Gerken, A. R., and Campbell, J. F. (2021). Spatial and temporal variation in stored-product insect pest distributions and implications for pest management in processing and storage facilities. Ann. Entomol. Soc. Am. 115, 239–252. doi: 10.1093/aesa/saab049
Gerken, A. R., and Morrison, W. R. (2022). Pest management in the postharvest agricultural supply chain under climate change. Front. Agron. 4:918845. doi: 10.3389/fagro.2022.918845
Gerken, A. R., Scully, E. D., and Campbell, J. F. (2018). Red flour beetle (Coleoptera: Tenebrionidae) response to volatile cues varies with strain and behavioral assay. Environ. Entomol. 47, 1252–1265. doi: 10.1093/ee/nvy107
Giga, D., and Canhao, J. Sr. (1993). Competition between Prostephanus truncatus (horn) and Sitophilus zeamais (Motsch.) in maize at two temperatures. J. Stored Prod. Res. 29, 63–70. doi: 10.1016/0022-474X(93)90023-W
Giunti, G., Palmeri, V., Algeri, G. M., and Campolo, O. (2018). VOC emissions influence intra-and interspecific interactions among stored-product Coleoptera in paddy rice. Sci. Rep. 8, 1–9. doi: 10.1038/s41598-018-20420-2
Gonzalez, J., Quilae, J., and Manalo, N. (1957). Study of the biology of the copra beetle. Araneta J. Agric. 4, 69–83.
Gourgouta, M., Baliota, G. V., Morrison, W. R., Domingue, M. J., and Athanassiou, C. G. (2021). Comparative capture of Trogoderma granarium (Coleoptera: Dermestidae) and T. variabile in floor traps in single species releases with previously captured conspecific or heterospecific individuals. J. Econ. Entomol. 114, 2591–2597. doi: 10.1093/jee/toab174
Gurdasani, K., Rafter, M. A., Daglish, G. J., and Walter, G. H. (2019). The dispersal flight of Tribolium castaneum – a field test of laboratory generated predictions. J. Stored Prod. Res. 83, 25–33. doi: 10.1016/j.jspr.2019.05.009
Hagler, J. R. (2019). Super mark it! A review of the protein immunomarking technique. Ann. Entomol. Soc. Am, 112, 200–210. doi: 10.1093/aesa/say039
Halstead, D. (1975). “Changes in the status of insect pests in storage and domestic habitats,” in Proceedings 1st International Conference on Stored Product Entomology, eds. E. U. Brady, J. H. Brower, P. E. Hunter, E. G. Jay, P. T. M. Lund, and M. A. Mullen, et al., 7-11 October, 1974 (Savannah, GA), 142–153.
Hassel, M. P. (1978). The dynamics of arthropod predator prey systems monographs in population biology 13. Princeton, NJ: Princeton University Press.
Heckbert, S., Baynes, T., and Reeson, A. (2010). Agent-based modeling in ecological economics. Acad. Sci. 1185, 39–53. doi: 10.1111/j/1749-6632.2009.05286.x
Holditch, Z., and Smith, A. D. (2020). Priority determines Tribolium competitive outcome in a food-limited environment. PloS One 15:e0235289. doi: 10.1371/journal.pone.0235289
Hole, B. D., Bell, C., Mills, K., and Goodship, G. (1976). The toxicity of phosphine to all developmental stages of thirteen species of stored product beetles. J. Stored Prod. Res. 12, 235–244. doi: 10.1016/0022-474X(76)90039-4
Holling, C. S. (1959a). Some characteristics of simple types of predation and parasitism. Can. Entomol. 91, 385–398. doi: 10.4039/Ent91385-7
Holling, C. S. (1959b). The components of predation as revealed by a study of small-mammal predation of the European pine sawfly 1. Can. Entomol. 91, 293–320. doi: 10.4039/Ent91293-5
Holloway, J., Falk, M., Emery, R., Collins, P., and Nayak, M. (2016). Resistance to phosphine in Sitophilus oryzae in Australia: a national analysis of trends and frequencies over time and geographical spread. J. Stored Prod. Res. 69, 129–137. doi: 10.1016/j.jspr.2016.07.004
Horton, P. M. (1981). Identification and control of on-farm stored product insect problems in South Carolina [dissertation]. Auburn, AL: Auburn University.
Howe, R. (1965). A summary of estimates of optimal and minimal conditions for population increase of some stored products insects. J. Stored Prod. Res. 1, 177–184. doi: 10.1016/0022-474X(65)90018-4
Hubert, J., Műnzbergová, Z., Kučerová, Z., and Stejskal, V. (2006). Comparison of communities of stored product mites in grain mass and grain residues in the Czech Republic. Exp. Appl. Acarol. 39, 149–158. doi: 10.1007/s10493-006-0026-y
Hunter, F., Tulloch, J. B., and Lambourne, M. (1973). Insects and mites of maltings in the east midlands of England. J. Stored Prod. Res. 9, 119–141. doi: 10.1016/0022-474X(73)90019-2
Hutchinson, G. E. (1959). Homage to Santa Rosalia or why are there so many kinds of animals? Am. Nat. 93, 145–159. doi: 10.1086/282070
Imamura, T., Murata, M., and Miyanoshita, A. (2008). Biological aspects and predatory abilities of hemipterans attacking stored-product insects. JARQ 42, 1–6. doi: 10.6090/jarq.42.1
Imura, O. (1981). Stored-product insects in a barley mill: II. Fauna and seasonal changes of species diversity. J. Ecol. 31, 139–146. doi: 10.18960/seitai.31.2_139
Jayas, D., Muir, W., and White, N. (1988). Modelling the diffusion of carbon dioxide in stored grain. American Society of Agricultural Engineers (Microfiche collection): St. Joseph, MI.
Jones, A., Gurney, W., Nisbet, R., and Gordon, D. (1990). Food degradation as a mechanism of intraspecific competition among the larvae of secondary stored-product pests. Funct. Ecol. 4, 629–638. doi: 10.2307/2389730
Kavallieratos, N. G., Athanassiou, C. G., Guedes, R. N., Drempela, J. D., and Boukouvala, M. C. (2017a). Invader competition with local competitors: displacement or coexistence among the invasive khapra beetle, Trogoderma granarium Everts (Coleoptera: Dermestidae), and two other major stored-grain beetles? Front. Plant Sci. 8:1837. doi: 10.3389/fpls.2017.01837
Kavallieratos, N. G., Athanassiou, C. G., Nika, E. P., and Boukouvala, M. C. (2017b). Efficacy of alpha-cypermethrin, chlorfenapyr and pirimiphos-methyl applied on polypropylene bags for the control of Prostephanus truncatus (horn), Rhyzopertha dominica (F.) and Sitophilus oryzae (L.). J. Stored Prod. Res. 73, 54–61. doi: 10.1016/j.jspr.2017.06.005
Kimoto, C., DeBano, S. J., Thorp, R. W., Taylor, R. V., Schmalz, H., Del Curto, T., et al. (2012). Short-term responses of native bees to livestock and implications for managing ecosystem services in grasslands. Ecosphere 3:art 88. doi: 10.1890/ES12-00118.1
Kiritani, K. (1958). On the distribution and seasonal prevalence of stored grain insects in a farm premises. Botyu. Kagaku 23, 164–172.
Kirkpatrick, D. M., Acebes-Doria, A. L., Rice, K. B., Short, B. D., Adams, C. G., Gut, L. J., et al. (2019). Estimating monitoring trap plume reach and trapping area for nymphal and adult Halyomorpha halys (Hemiptera: Pentatomidae) in crop and non-crop habitats. Environ. Entomol. 48, 1104–1112. doi: 10.1093/ee/nvz093
Latifian, M., Moghadam, M. J., and Jahromi, S. R. (2021). Competition and overlap of Oryzaephilus surinamensis and Plodia interpunctella populations under condition of stored date fruits. J. Asia Pac. Entomol. 24, 201–207. doi: 10.1016/j.aspen.2020.12.013
LeCato, G. L. (1975a). Predation by red flour beetle on sawtoothed grain beetle. Environ. Entomol. 4, 504–506. doi: 10.1093/ee/4.3.504
LeCato, G. L. (1975b). Interactions among four species of stored-product insects in corn: a multifactorial study. Ann. Entomol. Soc. Am. 68, 677–679.
Loschiavo, S., and Okumura, G. (1979). A survey of stored product insects in Hawaii. Proc. Hawaiian Entomol. Soc. 23, 95–118.
Mahroof, R. M., Edde, P. A., Robertson, B., Puckette, J. A., and Phillips, T. W. (2010). Dispersal of Rhyzopertha dominica (Coleoptera: Bostrichidae) in different habitats. Environ. Entomol. 39, 930–938. doi: 10.1603/EN09243
Mahroof, R. M., and Phillips, T. W. (2012). Use of macro and trace elements as biological markers in the lesser grain borer, Rhyzopertha dominica (F.) (Coleoptera: Bostrichidae). J. Stored Prod. Res. 48, 126–131. doi: 10.1016/j.jspr.2011.10.008
Mallqui, K. S. V., Oliveira, E. E., and Guedes, R. N. C. (2013). Competition between the bean weevils Acanthoscelides obtectus and Zabrotes subfasciatus in common beans. J. Stored Prod. Res. 55, 32–35. doi: 10.1016/j.jspr.2013.07.004
Martinson, V. G. (2020). Rediscovering a forgotten system of symbiosis: historical perspective and future potential. Genes 11:1063. doi: 10.3390/genes11091063
Mason, L. J. (2019). “Chapter 8 – effect and control of insects, molds and rodents affecting corn quality” in Corn. ed. S. O. Serna-Saldivar . 3rd ed (St. Paul, MN: AACC International Press), 213–234.
Mason, L. J., and McDonough, M. (2012). “Biology, behavior, and ecology of stored grain and legume insects” in Stored product protection. eds. D. W. Hagstrum, T. W. Phillips, and G. W. Cuperus (Manhattan, KS: Kansas State University Research and Extension), 7–20.
May, R. M. (2004). “Simple mathematical models with very complicated dynamics” in The theory of chaotic attractors. eds. B. R. Hunt, T.-Y. Li, J. A. Kennedy, and H. E. Nusse (New York, NY: Springer), 85–93.
McFarlane, J., and Prevett, F. (1984). “Training and extension for storage improvements through the establishment of special technical units,” in Proceedings of the 3rd international working conference on stored-product entomology, eds. R. B. Mills, V. F. Wright, J. R. Pedersen, W. H. McGaughey, R. W. Beeman, and K. J. Kramer, et al. 23-28 October, 1983, Manhattan, KS, 698–710.
Menon, A., Flinn, P. W., and Dover, B. A. (2002). Influence of temperature on the functional response of Anisopteromalus calandrae (Hymenoptera: Pteromalidae), a parasitoid of Rhyzopertha dominica (Coleoptera: Bostrichidae). J. Stored Prod Res, 38, 463–469. doi: 10.1016/S0022-474X(01)00050-9
Miller, J. R., Adams, C. G., Weston, P. A., and Schenker, J. H. (2015). Trapping of small organisms moving randomly: Principles and applications to Pest monitoring and management. New York: Springer.
Mills, J. (1992). “Ecological aspects of feed-mill operation” in Stored-Grain Ecosystems. eds. D. S. Jayas, N. D. G. White, and W. E. Muir (New York: Marcel Dekker), 677–707.
Morrison, W. R. III, Arthur, F. H., Wilson, L. T., Yang, Y., Wang, J., and Athanassiou, C. G. (2020a). Aeration to manage insects in wheat stored in the Balkan peninsula: computer simulations using historical weather data. Agronomy 10:1927. doi: 10.3390/agronomy10121927
Morrison, W. R. III, and Bohlen, P. J. (2010). Influence of vegetation on invertebrate communities in grazed freshwater wetlands in south-Central Florida. Southeast. Nat. 9, 453–464. doi: 10.1656/058.009.0304
Morrison, W. R. III, Bruce, A., Wilkins, R. V., Albin, C. E., and Arthur, F. H. (2019). Sanitation improves stored product insect pest management. Insects 10:77. doi: 10.3390/insects10030077
Morrison, W. R. III, Grosdidier, R. F., Arthur, F. H., Myers, S. W., and Domingue, M. J. (2020b). Attraction, arrestment, and preference by immature Trogoderma variabile and Trogoderma granarium to food and pheromonal stimuli. J. Pest. Sci. 93, 135–147. doi: 10.1007/s10340-019-01171-z
Morrison, W. R. III, Scully, E. D., and Campbell, J. F. (2021). Towards developing areawide semiochemical-mediated, behaviorally-based integrated pest management programs for stored product insects. Pest Manag. Sci. 77, 2667–2682. doi: 10.1002/ps.6289
Morrison, W. R. III, Wilkins, R. V., Gerken, A. R., Scheff, D. S., Zhu, K. Y., Arthur, F. H., et al. (2018). Mobility of adult Tribolium castaneum (Coleoptera: Tenebrionidae) and Rhyzopertha dominica (Coleoptera: Bostrichidae) after exposure to long-lasting insecticide-incorporated netting. J. Econ. Entomol. 111, 2443–2453. doi: 10.1093/jee/toy173
Mossadegh, M. (1978). Mechanism of secretion of the contents of the mandibular glands of Plodia interpunctella larvae. Physiol. Entomol. 3, 335–340. doi: 10.1111/j.1365-3032.1978.tb00167.x
Mossadegh, M. (1980). Inter-and intra-specific effects of the mandibular gland secretion of larvae of the Indian-meal moth, Plodia interpunctella. Physiol. Entomol. 5, 165–173. doi: 10.1111/j.1365-3032.1980.tb00223.x
Mudd, A. (1973). Mandibular gland secretion of larvae of stored products pests Anagasta kuehniella, Ephestia cautella, Plodia interpunctella and Ephestia elutella. Entomol. Exp. Appl. 16, 291–293. doi: 10.1111/j.1570-7458.1973.tb00274.x
Nakakita, H. (1982). Effect of larval density on pupation of Tribolium freemani Hinton (Coleoptera: Tenebrionidae). Appl. Entomol. Zool. 17, 269–276. doi: 10.1303/aez.17.269
Nansen, C., Phillips, T. W., and Palmer, M. W. (2004). Analysis of the insect community in a stored-maize facility. Ecol. Res. 19, 197–207. doi: 10.1111/j.1440-1703.2003.00624.x
Nayak, M. K., Collins, P. J., Throne, J. E., and Wang, J.-J. (2014). Biology and management of psocids infesting stored products. Annu. Rev. Entomol. 59, 279–297. doi: 10.1146/annurev-ento-011613-161947
Nicholson, A. J. (1954). An outline of the dynamics of animal populations. Aust. J. Zool. 2, 9–65. doi: 10.1071/ZO9540009
O’Gorman, E. J., and Emmerson, M. C. (2009). Perturbations to trophic interactions and the stability of complex food webs. Proc. Natl. Acad. Sci. U. S. A. 106, 13393–13398. doi: 10.1073/pnas.0903682106
Odeyemi, O. (1993). Competitive ability of two moths, Sitotroga cerealella (Oliv.) and Ephestia cautella (Wlk) in sorghum. Appl. Entomol. Zool. 28, 387–389. doi: 10.1303/aez.28.387
Odum, E. P. (1969). The strategy of ecosystem development: an understanding of ecological succession provides a basis for resolving man's conflict with nature. Science 164, 262–270. doi: 10.1126/science.164.3877.262
Oppert, B., Guedes, R. N., Aikins, M. J., Perkin, L., Chen, Z., Phillips, T. W., et al. (2015). Genes related to mitochondrial functions are differentially expressed in phosphine-resistant and-susceptible Tribolium castaneum. BMC Genomics 16, 1–10. doi: 10.1186/s12864-015-2121-0
Palyvos, N. E., Athanassiou, C. G., and Kavallieratos, N. G. (2006). Acaricidal effect of a diatomaceous earth formulation against Tyrophagus putrescentiae (Astigmata: Acaridae) and its predator Cheyletus malaccensis (Prostigmata: Cheyletidae) in four grain commodities. J. Econ. Entomol. 99, 229–236. doi: 10.1603/0022-0493(2006)099[0229:AEOADE]2.0.CO;2
Papanikolaou, N. E., Kavallieratos, N. G., Boukouvala, M. C., and Malesios, C. (2018). Do temperature, relative humidity and interspecific competition alter the population size and the damage potential of stored-product insect pests? A hierarchical multilevel modeling approach. J. Therm. Biol. 78, 415–422. doi: 10.1016/j.jtherbio.2018.10.022
Park, T. (1948). Interspecies competition in populations of Trilobium confusum Duval and Trilobium castaneum Herbst. Ecol. Monogr. 18, 265–307. doi: 10.2307/1948641
Phillips, T. W., Jiang, X. L., Burkholder, W. E., Phillips, J. K., and Tran, H. Q. (1993). Behavioral responses to food volatiles by two species of stored-product coleoptera, Sitophilus oryzae (Curculionidae) and Tribolium castaneum (Tenebrionidae). J. Chem. Ecol. 19, 723–734. doi: 10.1007/BF00985004
Pimentel, M. A., Faroni, L. R. D. A., da Silva, F. H., Batista, M. D., and Guedes, R. N. (2010). Spread of phosphine resistance among Brazilian populations of three species of stored product insects. Neotrop. Entomol. 39, 101–107. doi: 10.1590/S1519-566X2010000100014
Pimentel, M. A. G., Faroni, L. R. D. A., Tótola, M. R., and Guedes, R. N. C. (2007). Phosphine resistance, respiration rate and fitness consequences in stored-product insects. Pest Manag. Sci.: formerly. Pestic. Sci. 63, 876–881. doi: 10.1002/ps.1416
Plarre, R., and Burkholder, W. E. (2009). “Chapter 245- stored products as habitats” in Encyclopedia of insects. eds. V. H. Resh and R. T. Carde. 2nd ed (Camridge, MA: Academic Press), 967–970.
Platt, R., Cuperus, G., Payton, M., Bonjour, E., and Pinkston, K. (1998). Integrated pest management perceptions and practices and insect populations in grocery stores in south-Central United States. J. Stored Prod. Res. 34, 1–10. doi: 10.1016/S0022-474X(97)00036-2
Poirier, L., and Borden, J. (1995). Oral exudate as a mediator of behavior in larval eastern and western spruce budworms (Lepidoptera: Tortricidae). J. Insect Behav. 8, 801–811. doi: 10.1007/BF02009507
Poirier, L., and Borden, J. (2000). Influence of diet on repellent and feeding-deterrent activity of larval oral exudate in spruce budworms (Lepidoptera: Tortricidae). Can. Entomol. 132, 81–89. doi: 10.4039/Ent13281-1
Ponce, M. A., Kim, T. N., and Morrison, W. R. III (2021). A systematic review of the behavioral responses by stored-product arthropods to individual or blends of microbially produced volatile cues. Insects 12:391. doi: 10.3390/insects12050391
Pulpán, J., and Verner, P. (1959). The mites of the stored grain and their control (Acari). Bohem. Cent. 1, 169–292.
Quellhorst, H. E., Athanassiou, C. G., Bruce, A., Scully, E. D., and Morrison, W. R. III (2020). Temperature-mediated competition between the invasive larger grain borer (Coleoptera: Bostrichidae) and the cosmopolitan maize weevil (Coleoptera: Curculionidae). Environ. Entomol. 49, 255–264. doi: 10.1093/ee/nvz151
Quellhorst, H., Athanassiou, C. G., Zhu, K. Y., and Morrison, W. R. III (2021). The biology, ecology, and management of the larger grain borer, Prostephanus truncatus (horn) (Coleoptera: Bostrichidae). J. Stored Prod. Res. 94:101860. doi: 10.1016/j.jspr.2021.101860
Quellhorst, H. E., Kim, T. N., Zhu, K. Y., and Morrison, W. R. III (2023). Niche partitioning among the local competitors, larger grain borer and maize weevil in grain masses. Environ. Entomol. In press
Rahman, M., Islam, W., and Ahmed, K. (2009). Functional response of the predator Xylocoris flavipes to three stored product insect pests. Int. J. Agric. Biol. 11, 316–320.
Ramos-Rodríguez, O., Campbell, J. F., and Ramaswamy, S. B. (2007). Efficacy of the entomopathogenic nematode Steinernema riobrave against the stored-product insect pests Tribolium castaneum and Plodia interpunctella. Biol. Control 40, 15–21. doi: 10.1016/j.biocontrol.2006.09.007
Ranabhat, S., Zhu, K. Y., Bingham, G. V., and Morrison, W. R. (2022). Mobility of phosphine-susceptible and -resistant Rhyzopertha dominica (Coleoptera: Bostrichidae) and Tribolium castaneum (Coleoptera: Tenebrionidae) after exposure to controlled release materials with existing and novel active ingredients. J. Econ. Entomol. 115, 888–903. doi: 10.1093/jee/toac033
Reed, C. R., Hagstrum, D., Flinn, P., and Allen, R. (2003). Wheat in bins and discharge spouts, and grain residues on floors of empty bins in concrete grain elevators as habitats for stored-grain beetles and their natural enemies. J. Econ. Entomol. 96, 996–1004. doi: 10.1093/jee/96.3.996
Ridley, A. W., Hereward, J. P., Daglish, G. J., Raghu, S., Collins, P. J., and Walter, G. H. (2011). The spatiotemporal dynamics of Tribolium castaneum (Herbst): adult flight and gene flow. Mol. Ecol. 20, 1635–1646. doi: 10.1111/j.1365-294X.2011.05049.x
Roesli, R., Subramanyam, B., Campbell, J. F., and Kemp, K. (2003). Stored-product insects associated with a retail pet store chain in Kansas. J. Econ. Entomol. 96, 1958–1966. doi: 10.1093/jee/96.6.1958
Rossini, L., Severini, M., Contarini, M., and Speranza, S. (2020). Ento Sim, a ROOT-based simulator to forecast insects’ life cycle: description and application in the case of Lobesia botrana. Crop Prot. 129:105024. doi: 10.1016/j.cropro.2019.105024
Roxburgh, S. H., Shea, K., and Wilson, J. B. (2004). The intermediate disturbance hypothesis: patch dynamics and mechanisms of species coexistence. Ecology 85, 359–371. doi: 10.1890/03-0266
Rumbos, C. I., Sakka, M., Athanassiou, C. G., Schaffert, S., Sterz, T., Austin, J. W., et al. (2018). Evaluation of Carifend®, an alpha-cypermethrin-coated polyester net, for the control of Lasioderma serricorne and Ephestia elutella in stored tobacco. J. Pest. Sci. 91, 751–759. doi: 10.1007/s10340-017-0947-8
Ryne, C., and Bensch, S. (2008). Do anthropogenic transports facilitate stored-product pest moth dispersal? A molecular approach. Sci. Nat. 95, 155–159. doi: 10.1007/s00114-007-0299-y
Sakka, M. K., and Athanassiou, C. G. (2018). Competition of three stored-product bostrychids on different temperatures and commodities. J. Stored Prod. Res. 79, 34–39. doi: 10.1016/j.jspr.2018.07.002
Sakka, M., Gourgouta, M., Morrison, W. R., Domingue, M. J., Grosdidier, R. F., Wilkins, R. V., et al. (2023). Different trap designs and attractants affect the capture of multiple life stages of Trogoderma granarium Everts and Trogoderma variabile Ballion in the laboratory. J. Stored Prod. Res. 102:102108. doi: 10.1016/j.jspr.2023.102108
Saqib, H. S. A., Liang, P., You, M., and Gurr, G. M. (2021). Molecular gut content analysis indicates the inter- and intra-guild predation patterns of spiders in conventionally managed vegetable fields. Ecol. Evol. 11:9543–9552. doi: 10.1002/ece3.7772
Scheff, D. S., and Arthur, F. H. (2018). Fecundity of Tribolium castaneum and Tribolium confusum adults after exposure to deltamethrin packaging. J. Pest. Sci. 91, 717–725. doi: 10.1007/s10340-017-0923-3
Scheff, D. S., Arthur, F. H., Myers, S. W., and Domingue, M. J. (2020). Effcacy determination of commercial deltamethrin-treated storage bags on Trogoderma granarium Everts adults and larvae. Agronomy 10:814. doi: 10.3390/agronomy10060814
Scheff, D. S., Campbell, J. F., and Arthur, F. H. (2021a). Seasonal, landscape, and attractant effects on lesser grain borer, Rhyzopertha dominica (F.), captures in Northeast Kansas. Agronomy 12:99. doi: 10.3390/agronomy12010099
Scheff, D. S., Gerken, A. R., Morrison, W. R., Campbell, J. F., Arthur, F. H., and Zhu, K. Y. (2021b). Assessing repellency, movement, and mortality of three species of stored product insects after exposure to deltamethrin-incorporated long-lasting polyethylene netting. J. Pest. Sci. 94, 885–898. doi: 10.1007/s10340-020-01326-3
Scheff, D. S., Subramanyam, B., Arthur, F. H., and Dogan, H. (2018). Plodia interpunctella and Trogoderma variabile larval penetration and invasion of untreated and methoprene-treated foil packaging. J. Stored Prod. Res. 78, 74–82. doi: 10.1016/j.jspr.2018.05.002
Semeao, A. A., Campbell, J. F., Whitworth, R. J., and Sloderbeck, P. E. (2013). Movement of Tribolium castaneum within a flour mill. J. Stored Prod. Res. 54, 17–22. doi: 10.1016/j.jspr.2013.03.004
Simmons, P., and Ellington, G. W. (1925). The ham beetle, Necrobia rufipes DE GEER. J. Agric. Res. 30, 845–863.
Sinha, R. (1982). Food losses through energy transfer from cereal grains to stored-product insects. Food Nutr. Bull. 4, 1–9. doi: 10.1177/156482658200400205
Sinha, R. N. (1991). “Storage Ecosystems” in Ecology and Management of Food-Industry Pests. ed. J. R. Gorham (Arlington, VA: Association of Official Analytical Chemists), 17–30.
Sinha, R. N. (1995). “The stored-grain ecosystem” in Stored-grain ecosystems. eds. D. S. Jayas, N. D. G. White, and W. E. Muir (New York: Dekker), 1–32.
Sinha, R., and Wallace, H. (1966). Ecology of insect-induced hot spots in stored grain in western Canada. Popul. Ecol. 8, 107–132. doi: 10.1007/BF02518801
Sinha, R., Wallace, H., and Chebib, F. (1969). Principal-component analysis of interrelations among fungi, mites, and insects in grain bulk ecosystems. Ecology 50, 536–547. doi: 10.2307/1936244
Souza, A. M., and Linhares, A. X. (1997). Diptera and Coleoptera of potential forensic importance in southeastern Brazil: relative abundance and seasonality. Med. Vet. Entomol. 11, 8–12. doi: 10.1111/j.1365-2915.1997.tb00284.x
Stadler, T., Subramanyam, B., and Ferrero, A. (2003). Monitoring for insecticide resistance in major stored product pests in Argentina: a review. Agri 20, 99–110. doi: 10.31047/1668.298x.v20.n0.2838
Stathers, T., Lamboll, R., and Mvumi, B. M. (2013). Post-harvest agriculture in a changing climate. Rural 21. Int. J. Rural. Dev. 47, 12–14.
Stejskal, V. (2000). Cumulative and actual population size of stored-product pests: effect of initial numbers and rate of increase. Plant Prot. Sci. 36, 65–68.
Stejskal, V. (2002). “Metapopulation concept and the persistence of urban pests in buildings,” in Proceeding of the 4th international conference on urban pests, eds. S. Jones, J. Zhai, and W. Robinson, Charleston, South Carolina, USA, 75–85.
Stejskal, V., and Kucerova, Z. (1996). The effect of grain size on the biology of Sitophilus granrius L. (Col., Curculionidae). I. Oviposition, distribution of eggs and adult emergence. J. Appl. Ent. 120, 143–146. doi: 10.1111/j.1439-0418.1996.tb01581.x
Stejskal, V., Zuska, J., Werner, P., and Kucerova, Z. (1999). Survival over the winter of Rhyzopertha dominica F. (Coleoptera: Bostrichidae) in hot spots caused by improper grain storage technology: the first record in the Czech Republic. Plant Prot. Sci. 35, 23–25. doi: 10.17221/9668-PPS
Stratton, C., Hansen, P., Thompson, Y., Zio, K., Morrison, III W. R., and Murrell, E. (2023). uafR: Automates mass spectrometry data processing. GitHub Repository. Available at: https://github.com/castratton/uafR, https://castratton.github.io/uafR/.
Strong, R., and Okumura, G. (1958). Insects and mites associated with stored foods and seeds in California. Bull. Calif. Dep. Agric. 47, 233–249.
Talukder, F. (2009). Pesticide resistance in stored-product insects and alternative biorational management: a brief review. J. Agric. Mar. Sci. 14, 9–15.
Tilley, D. R., Casada, M. E., Subramanyam, B., and Arthur, F. H. (2017). Temporal changes in stored-product insect populations associated with boot, pit, and load-out areas of grain elevators and feed mills. J. Stored Prod. Res. 73, 62–73. doi: 10.1016/j.jspr.2017.07.002
Tilman, D. (1977). Resource competition between plankton algae: an experimental and theoretical approach. Ecol. 58, 338–348. doi: 10.2307/1935608
Toews, M., Campbell, J., and Arthur, F. (2006). Temporal dynamics and response to fogging or fumigation of stored-product Coleoptera in a grain processing facility. J. Stored Prod. Res. 42, 480–498. doi: 10.1016/j.jspr.2005.08.003
Toews, M. D., Campbell, J. F., and Arthur, F. H. (2010). The presence of flour affects the efficacy of aerosolized insecticides used to treat the red flour beetle, Tribolium castaneum. J. Insect Sci. 10:Article 196-Article 196. doi: 10.1673/031.010.19601
Toews, M. D., Phillips, T. W., and Payton, M. E. (2005). Estimating populations of grain beetles using probe traps in wheat-filled concrete silos. Environ. Entomol. 34, 712–718. doi: 10.1603/0046-225X-34.3.712
Toon, A., Daglish, G., Ridley, A., Emery, R., Holloway, J., and Walter, G. (2016). Random mating between two widely divergent mitochondrial lineages of Cryptolestes ferrugineus (Coleoptera: Laemophloeidae): a test of species limits in a phosphine-resistant stored product pest. J. Econ. Entomol. 109, 2221–2228. doi: 10.1093/jee/tow178
Tran, T. M., Ameye, M., Phan, L. T.-K., Devlieghere, F., De Saeger, S., Eeckhout, M., et al. (2021). Post-harvest contamination of maize by Fusarium verticillioides and fumonisins linked to traditional harvest and post-harvest practices: a case study of small-holder farms in Vietnam. Int. J. Food Microbiol. 339:109022. doi: 10.1016/j.ijfoodmicro.2020.109022
Trematerra, P., and Fleurat-Lessard, F. (2015). Food industry practices affecting pest management. Stewart Postharvest Rev. 1, 1–7. doi: 10.2212/spr.2015.1.2
Trematerra, P., Fontana, F., Mancini, M., and Sciarretta, A. (1999). Influence of intact and damaged cereal kernels on the behaviour of rice weevil, Sitophilus oryzae (L.) (Coleoptera: Curculionidae). J. Stored Prod. Res. 35, 265–276. doi: 10.1016/S0022-474X(99)00010-7
Trematerra, P., Paula, M. C. Z., Sciarretta, A., and Lazzari, S. M. N. (2004). Spatio-temporal analysis of insect pests infesting a paddy rice storage facility. Neotrop. Entomol. 33, 469–479. doi: 10.1590/S1519-566X2004000400012
Trematerra, P., and Sciarretta, A. (2004). Spatial distribution of some beetles infesting a feed mill with spatio-temporal dynamics of Oryzaephilus surinamensis, Tribolium castaneum, and Tribolium confusum. J. Stored Prod. Res. 40, 363–377. doi: 10.1016/S0022-474X(03)00027-4
Tsafack, N., Borges, P. A. V., Xie, Y., Wang, X., and Fattorini, S. (2021). Emergent rarity properties in Carabid communities from Chinese steppes with different climatic conditions. Front. Ecol. Evol. 9:603436. doi: 10.3389/fevo.2021.603436
Tsai, W.-T., Mason, L. J., and Woloshuk, C. P. (2007). Effect of three stored-grain fungi on the development of Typhaea stercorea. J. Stored Prod. Res. 43, 129–133. doi: 10.1016/j.jspr.2006.01.001
Turner, R. M., Brockerhoff, E. G., Bertelsmeier, C., Blake, R. E., Caton, B., James, A., et al. (2021). Worldwide border interceptions provide a window into human-mediated global insect movement. Ecol. Appl. 31:e02412. doi: 10.1002/eap.2412
Van Bronswijk, J., and Sinha, R. (1971). Interrelations among physical, biological, and chemical variates in stored-grain ecosystems; a descriptive and multivariate study. Ann. Entomol. Soc. Am. 64, 789–803. doi: 10.1093/aesa/64.4.789
Vowotor, K., Meikle, W., Ayertey, J., and Markham, R. (2005). Distribution of and association between the larger grain borer Prostephanus truncatus (horn)(Coleoptera: Bostrichidae) and the maize weevil Sitophilus zeamais Motschulsky (Coleoptera: Curculionidae) in maize stores. J. Stored Prod. Res. 41, 498–512. doi: 10.1016/j.jspr.2004.08.002
Wang, Y., Sun, E., Wang, W., Wang, K., Wang, H., and Ge, M. (2016). Effects of habitat fragmentation on genetic diversity and population differentiation of Liposcelis bostrychophila badonnel (Psocoptera: Liposcelididae) as revealed by ISSR markers. J. Stored Prod. Res. 68, 80–84. doi: 10.1016/j.jspr.2016.04.008
White, N. (1992). A multidisciplinary approach to stored-grain research. J. Stored Prod. Res. 28, 127–137. doi: 10.1016/0022-474X(92)90020-Q
White, N. D. G., Fields, P. G., Demianyk, C. J., Timlick, B., and Jayas, D. S. (2011). “Arthropods of stored cereals, oilseeds, and their products in Canada: artificial ecosystems on grasslands” in Athropods of Canadian grasslands (volume 2): Inhabitants of a changing landscape. ed. K. D. Floate (Sackville, NB: Biological Survey of Canada), 267–289.
Wijayaratne, L. K. W., Arthur, F. H., and Whyard, S. (2018). Methoprene and control of stored-product insects. J. Stored Prod. Res. 76, 161–169. doi: 10.1016/j.jspr.2016.09.001
Wijayaratne, L. K., and Fields, P. G. (2012). Effects of rearing conditions, geographical origin, and selection on larval diapause in the Indianmeal moth, Plodia interpunctella. J. Insect Sci. 12:119. doi: 10.1673/031.012.11901
Wilches, D. M., Laird, R. A., Floate, K. D., and Fields, P. G. (2016). A review of diapause and tolerance to extreme temperatures in dermestids (Coleoptera). J. Stored Prod. Res. 68, 50–62. doi: 10.1016/j.jspr.2016.04.004
Wilches, D. M., Laird, R. A., Floate, K. D., and Fields, P. G. (2017). Effects of acclimation and diapause on the cold tolerance of Trogoderma granarium. Entomol. Exp. Appl. 165, 169–178. doi: 10.1111/eea.12632
Wilkins, R. V., Campbell, J. F., Zhu, K. Y., Starkus, L. A., McKay, T., and Morrison, W. R. (2021). Long-lasting insecticide-incorporated netting and interception traps at pilot-scale warehouses and commercial facilities prevents infestation by stored product beetles. Front. Sust. Food Syst. 4, –561820. doi: 10.3389/fsufs.2020.561820
Williams, G. C. (1964). The life-history of the Indian meal moth Plodia interpunctella (Hubner) (Lep. Phycitidae) in a warehouse in Britain and on different foods. Ann. Appl. Biol. 53, 459–475. doi: 10.1111/j.1744-7348.1964.tb07259.x
Yi, C., and Jackson, N. (2021). A review of measuring ecosystem resilience to disturbance. Environ. Res. Lett. 16:053008. doi: 10.1088/1748-9326/abdf09
Yu, G. X. (2015). Influence of temperature, relative humidity and interspecific competition on the population growth dynamics of three pests of stored product, Oryzaephilus surinamensis (L.), Sitophilus oryzae (L.), and Tribolium castaneum Herbst [thesis]. Penang: Universiti Sains Malaysia.
Keywords: niche, succession, insect pest, stored grain, ecosystem, behavior, competition
Citation: Gerken AR and Morrison WR (2023) Farm2Fork through the lens of community ecology: concepts and applications in postharvest storage. Front. Sustain. Food Syst. 7:1137683. doi: 10.3389/fsufs.2023.1137683
Received: 04 January 2023; Accepted: 24 May 2023;
Published: 15 June 2023.
Edited by:
Dipayan Sarkar, Sugarbeet and Potato Research Unit, United StatesReviewed by:
Tanya Stathers, University of Greenwich, United KingdomCopyright © 2023 Gerken and Morrison. This is an open-access article distributed under the terms of the Creative Commons Attribution License (CC BY). The use, distribution or reproduction in other forums is permitted, provided the original author(s) and the copyright owner(s) are credited and that the original publication in this journal is cited, in accordance with accepted academic practice. No use, distribution or reproduction is permitted which does not comply with these terms.
*Correspondence: Alison R. Gerken, YWxpc29uLmdlcmtlbkB1c2RhLmdvdg==
†These authors have contributed equally to this work
Disclaimer: All claims expressed in this article are solely those of the authors and do not necessarily represent those of their affiliated organizations, or those of the publisher, the editors and the reviewers. Any product that may be evaluated in this article or claim that may be made by its manufacturer is not guaranteed or endorsed by the publisher.
Research integrity at Frontiers
Learn more about the work of our research integrity team to safeguard the quality of each article we publish.