- 1Agrobiosciences Program, Plant and Soil Microbiome Subprogram, Mohammed VI Polytechnic University, Benguerir, Morocco
- 2Department of Agriculture, Ecotrophology and Landscape Development, Institute of Bioanalytical Sciences (IBAS), Anhalt University of Applied Sciences, Bernburg, Germany
- 3Agrobiotechnology and Bioengineering Center, Research Unit CNRST Labeled, Faculty of Sciences and Techniques, Cadi Ayyad University, Marrakech, Morocco
- 4Situation Innovation-OCP Group, El Jadida, Morocco
- 5African Sustainable Agriculture Research Institute (ASARI), Mohammed VI Polytechnic University (UM6P), Laayoune, Morocco
The rhizosphere is a hot spot and a source of beneficial microorganisms known as plant growth-promoting rhizobacteria (PGPR). From the alfalfa (Medicago sativa) rhizosphere, 115 bacteria were isolated, and from the screening for PGP traits, 26 interesting isolates were selected as PGP rhizobacteria for the next tests. The objective of this study was to use a consortium of PGPR to enhance the growth of faba-bean under phosphate (P) deficiency by taking advantage of their ability to release phosphorus from rock phosphate (RP). Several examined strains were found to have a relatively high activity on P solubilization, auxin, siderophore, ammoniac production, antifungal activity, and the ability to tolerate hypersalinity and water stress. 16S rRNA gene sequencing of the collection revealed six different genera, including Bacillus (46.15%), Siccibacter (23.07%), and Acinetobacter (15.38%) which were identified as the most abundant. Three of the interesting strains (Siccibacter colletis, Enterobacter huaxiensis, and Pantoea sp.) showed high plant growth promotion traits and no antagonism with Rhizobium laguerreae. These three bacteria were retained to establish a rhizobia-including consortium. The inoculation of faba-bean plants with the consortium improved growth parameters as root and shoot dried biomasses and some physiological criteria (chlorophyll content and P uptake under low P availability conditions), and the increase reached 40%. Our study could be the first report of faba-bean growth promotion by a multi-strain PGPR-rhizobia consortium involving S. colletis, E. huaxiensis, and Pantoea sp. Thus, this consortium could be recommended for faba-bean inoculation, particularly under P-limiting conditions.
1. Introduction
Soil mineral nutrients are essential for crop production. The limitations of soil nutrient availability as well as other abiotic and biotic stresses impact plant growth and its performance (Choudhary et al., 2016). In recent times, to deal with these constraints, sustainable biological strategies were implemented to increase the availability of nutrients and the ability of plants to tolerate stress (Umesha et al., 2018). For this purpose, microbial communities draw more attention and are involved due to their beneficial interactions with plants, such as the rhizobacteria that colonize the rhizosphere and are characterized by plant growth-promoting traits (Marschner et al., 2004; Msimbira and Smith, 2020). The majority of recognized PGPR for the enhancement of crop production were isolated from the rhizosphere (a hotspot of multi-beneficial microorganisms colonization) and have a generally positive impact on plants (Chamkhi et al., 2022). Indeed, the PGPR are a group of bacteria that can be found in the rhizosphere in interaction with the plant root systems and that can improve plant performance by multiple direct mechanisms such as biological nitrogen (N) fixation, phosphorus (P) solubilization, and phytohormone production such as cytokinins (CKs) and indole-3-acetic acid (IAA) (Goswami et al., 2016; Chamkhi et al., 2022). There are also PGPR-stimulating substances such as bacterial volatile compounds like 1-hexanol, pentadecane, and indole that are capable of promoting plant growth and its regulation (Blom et al., 2011; Kanchiswamy et al., 2015). Moreover, PGPR can induce the production of hydrogen cyanide (HCN), ammonia, and bioactive metabolites (biosurfactant, siderophore, and phenazine) (Patil et al., 2017). In addition to their indirect actions related to enzyme production, such as synthesizing stress-alleviating enzymes, such as the 1-aminocyclopropane-1-carboxylate (ACC) deaminase (Chandra et al., 2018), antibiotic production, and induced systematic resistance, the PGPR can modulate plant stress markers under abiotic stress (Goswami et al., 2016). Moreover, they can induce numerous metabolic changes in host plants, including the accumulation of secondary metabolites (Chamkhi et al., 2021).
Low soil P availability has a negative impact on legume performance, mainly when the growth is dependent on biological N fixation, which is a high energy-demanding process (Oukaltouma et al., 2021). Furthermore, under conditions of P deficiency in the soil, P solubilization is one of the most common modes of action implicated in increasing the amount of PGPR available in the soil that could be easily absorbed by the plants. The increase in P solubilization involves some physiological mechanisms, such as the acidification of the rhizosphere and the excretion of phosphatases into the rhizosphere (Vance et al., 2003).
Indeed, rhizospheric bacterial communities contain highly diverse populations of P-solubilizing bacteria such as Bacillus, Pseudomonas, Acinetobacter, Burkholderia, Serratia, Enterobacter, Paenibacillus, Pantoea, Methylobacterium, Azotobacter, Ochrobactrum, Rhizobium, and Acetobacter (Kour et al., 2021).
Similarly, it was shown that PGPR, individually or in combination (consortium), increased the nutrient contents (N, P, K, Fe, Zn, and Mn) and improved the absorption of macronutrients and micronutrients in the host plant (Rana et al., 2012; Behera et al., 2021). Indeed, Kumawat et al. (2021) reported that, in comparison to the uninoculated control treatment, the inoculation of spring mung bean plants with a bacterial dual combination enhanced the shoot contents of N (170.54%), P (79.01%), K (20.98%), Mn (100%), Fe (49.65%), Zn (65.96%), and Cu (89.52%) under salt stress conditions.
While soils of many legume-growing areas are affected by drought, low P availability further reduces the performance of these crops. To alleviate stress effects, inoculation with efficient rhizobia and synergistic PGPR could be a promising approach. Thus, this research aimedto isolate beneficial multi-trait rhizobacteria from legume rhizosphere in order to evaluate their potential effectiveness in promoting growth, and all bacterial isolates were tested for their in vitro putative PGP traits (direct and indirect PGP traits), including P solubilization, biological N-fixation, tolerance to osmotic stress, auxin production, siderophore production, ammonia production, antifungal activity, and phytase activity. Through this original research article, we screened the most promising PGPR that colonize the rhizosphere of Medicago sativa, testing their beneficial biological activities and the molecular identification of these bacteria to determine their species. The promising rhizobacteria were selected for the conception of a consortium to test their ability under stressful conditions. This study highlights the impact of PGPR as a biologically sustainable fertilizer to enhance plants under P deficiency by direct application of phosphate rock (PR) as a source of phosphorus.
2. Material and methods
2.1. Prospected legume areas and rhizobacteria isolation
Soil samples were collected from M. sativa rhizosphere from two different agricultural regions, namely, Daraa-Tafilalet and Chaouia of Morocco. Two zones, Imiter (WT3E5) and Tizagazouine (WT2E4), were sampled from the Daraa-Tafilalet region. From the Chaouia area, two zones, Ouled Said (CH3E5) and Sidi El Aydi (CH2E3), were sampled. Soil samples were conserved at −20°C until they were used.
Rhizospheric soil samples (4 g) were suspended in 16 ml of physiological sterile water, diluted serially, and homogenized for 30 min. Petri dishes containing TY medium [tryptone (5 g), yeast extract (3 g), and CaCl2 H2O (0.8 g)] were spiked with 0.1 ml of each soil dilution and incubated at 28°C for 48 h (Becerra-Castro et al., 2012).
2.2. Screening and characterization of rhizobacteria isolates for their PGP traits
2.2.1. Biological nitrogen fixation ability
The isolates were tested for their biological nitrogen fixation ability on a selected medium of biological nitrogen fixation (NFB) (KH2PO4 1.20 g, K2HPO4 0.80 g, glucose 5.0 g, MgSO4·7H2O 0.20 g, NaCl 0.20 g, CaCl2·2H2O 0.02 g, FeSO4·7H2O 0.002 g, distilled water 1 L, and 2.0 ml of metal solution (0.40 g CaCl2·2H2O, 0.30 g H3BO3, 0.04 g CuSO4·5H2O, 0.10 g KI, 0.20 g FeSO4·7H2O, 0.40 g MnSO4·7H2O, 0.20 g NaMoO4·2H2O, and 10.0 ml concentrated HCl in 1.0 L of distilled water). Adjusted fresh culture (DO = 0.1) of the isolates were inoculating plates containing NFb medium with or without the addition of NH4Cl (positive control). Plates were incubated at 28°C for 7 days (Zhou et al., 2013).
2.2.2. Phosphate solubilization ability on solid medium
The obtained isolates were tested for their phosphate solubilization potential in a selective Pikovskaya medium (PVK) [glucose 10 g, Ca3(PO4)2 5 g, (NH4)2SO4 0.5 g, NaCl 0.2 g, MgSO4·7H2O 0.1 g; KCl 0.2 g, yeast extract 0.5 g, MnSO4·H2O 0.002 g, FeSO4·7H2O 0.002 g; and Bromocresol Purple 0.1 g in 1.0 L of distilled water] supplemented with (0.5 g/L) of rock phosphate (RP) (Pikovskaya, 1948). A sample of 10 μl of each bacterial suspension (DO = 0.1) was dispensed into the center of Petri dishes containing the medium and incubated for 7 days at 28°C. The presence of a clearing zone around bacterial colonies (halo zone) is an indicator of positive phosphate solubilization (Xie et al., 2009).
2.2.3. Osmotic potential tolerance
The isolates obtained were screened for their osmotolerance levels by assessing their ability to grow in a medium supplemented with 30% (−1.69 MPa), 40% (−2.25 MPa), and 0% (0 MPa) as the control of polyethylene glycol (PEG) (8000). Appropriate uninoculated controls were maintained. The tubes were inoculated with 200 μl inoculum of each isolate (DO = 0.1) and incubated at 28°C for 2 days on an orbital shaker. Later, the O.D. of the broth was measured at 600 nm (Ilyas et al., 2020). The selected isolates were purified and stored at −80°C in 40% glycerol.
2.3. Assessment of isolated rhizobacteria for PGP traits
2.3.1. Quantification of phosphate solubilization
The P solubilization activity was determined according to Murphy and Riley (1962). In 250-ml Erlenmeyer flasks, 100 ml of liquid PVK medium were inoculated with 100 μl of fresh pre-cultures of each isolate (DO = 0.1). The incubation was done for 7 days at 28°C in the Shaker-Incubator at 158 rpm. A volume of 2 ml of the culture was centrifuged, and the pH of the culture rest was determined. Soluble P concentrations were measured spectrophotometrically by using the molybdenum blue method at 665 nm (Murphy and Riley, 1962).
2.3.2. Auxin quantification
The Salkowski colorimetric method was used to determine the auxin production of the isolates. Liquid pre-cultures (DO = 0.1) were inoculated into liquid YEM medium (Vincent, 1970) supplemented with tryptophan (YEM-Try) (5 g Mannitol, 3H2O, 0.12 g KH2PO4, 0.2 g MgSO4·7H2O, 0.1 g NaCl, 1 g yeast extract, 0.46 g KH2PO4, 1 L distilled water, and 0.5 mg tryptophan) and then incubated at 28°C for 7 days. At 12,000 rpm for 10 min, 2 ml of each culture were centrifuged, and the supernatants were used to determine the auxin concentration following the method of Glickmann and Dessaux (1995).
2.3.3. Siderophore production
A sample of 100 μl of 24-h broth culture (DO = 0.1) was inoculated into 20 ml of iron-free succinic acid broth medium. The flask was incubated at 28°C for 7 days. According to Rachid and Ahmed, the concentration was calculated using the absorption maximum (400 nm) and the molar extinction coefficient (ε = 20,000/M/cm) (Rachid and Ahmed, 2005).
2.3.4. Ammoniac production
2.3.4.1. Qualitative test
The purified isolates (DO = 0.1) were grown in 10 ml of peptone broth in a test tube and placed on a rotary shaker at 120 rpm for 72 h at 28°C. After this incubation, 0.5 ml of Nessler's reagent was added to the culture test tube. The change in color from yellow to brown indicated that ammonia production was occurring (Mukherjee et al., 2017).
2.3.4.2. Quantitative test
The ammonia concentration was estimated for the best bacteria selected in the qualitative test. The supernatant of the isolates grown on peptone medium was used to quantify the ammonia produced by each bacterium using NH4Cl as standard and measured at 420 nm (Zhao et al., 2019).
2.3.5. Halotolerance test
Bacterial isolates were screened for their salinity tolerance levels using TY media supplemented with NaCl at different concentrations (1%, 5%, 10%, and 15%). The plates were inoculated with 20 μl volumes of inoculum (DO = 0.1), and the cultures were incubated for 7 days at 28°C (Albdaiwi et al., 2019).
2.3.6. Antifungal activity
The isolates were tested against Fusarium oxysporum on potato dextrose agar (PDA) to test their antifungal activity. Hence, fungal disks were placed in the middle of agar plates, which is 2 cm away from the isolated spots. The antagonistic activity was observed after incubation at 28°C for 7 days. The percentage of inhibition was measured using the following formula: I (%) = (C – T/C) × 100, where I is the percentage of growth reduction, C is the diameter of the control hyphal growth (without bacterial spot), and T is the diameter of the treated hyphal growth (with bacterial spot) (Petatán-Sagahón et al., 2011).
2.3.7. Phytase activity
Bacterial isolates were assessed for their phytase activity by plate assay using phytase-specific medium agar in Petri plates after incubation at 28°C for 14 days. Bacterial isolates able to hydrolyze calcium phytate can grow on a specific medium (Singh et al., 2013).
2.4. Diversity analysis of selected PGPR
2.4.1. DNA extraction and PCR amplification
The genomic DNA was extracted (Rodríguez et al., 2014), and the quantity of extracted DNA was checked by using a NanoDrop spectrophotometer. PCR amplification of partial nucleotide sequences of the 16S rRNA gene was performed using the universal primers 7f (5′-AGA GTT TGA TYM TGG CTC AG-3′)/1510r (5′-ACG GYT ACC TTG TTA CGA CTT-3′). The PCR procedure used was as follows: initial denaturation for 3 min at 96°C, followed by 35 cycles of denaturation at 98°C for 10 s, annealing at 64°C for 20 s, and extension at 72°C for 45 s. Purified PCR products were then sequenced in both senses by using 1510r and 7 f based on the Sanger sequencing platform.
2.4.2. Phylogenetic analysis
The sequences of sense 1510r were converted into inverse complements by using the bioedit software and then aligned with the sequences of sense 7f by using the MEGA11 software and the ClustalW tool. The almost complete 16S rRNA gene sequence (2,400 bp) was obtained by assembling the sequences that were then compared to the 16S rRNA gene sequences obtained from the GenBank database (https://blast.ncbi.nlm.nih.gov/Blast.cgi). The phylogenetic tree was constructed from the ClustalX results and the maximum likehood test with the Kimura 2-parameter model by using the MEGA11 program. The bootstrap method was used as the phylogeny test. Reference sequences were added to optimize the comparison (Tamura et al., 2011).
2.5. Bacterial inoculation, consortium preparation, and trial assessment
2.5.1. Rhizobacteria selection for consortium establishment
The isolates showing highly interesting PGPR traits were selected to establish a rhizobacterial consortium with rhizobia for faba-bean inoculation under P deficiency. The isolates 20WT2E4, 21WT2E4, and 25WT2E4 were selected for their highest PGPR traits and then tested for their eventual antagonism before consortium establishment with rhizobia R. laguerreae from the collection of Cadi Ayyad University, Team of Biotechnology and Symbiosis Agrophysiology, Faculty of Sciences and Techniques, Marrakech, Morocco. Primary screening of three rhizobacterial strains (20WT2E4, 21WT2E4, and 25WT2E4) and R. laguerreae was carried out for the selection for the consortium and evaluated by the Cross-Streak method. Each of the four isolates was streaked on a YEM medium in a straight line and incubated at 28°C for 6 days. The plates were then seeded with four other similar isolates by a single streak at a 90° angle to the previous streaked isolates and incubated at 28°C for 24 h. The absence of antagonistic interactions among the tested isolates was confirmed by the absence of an inhibition (clear) zone around the crosses between streaked lines of colonies (Velho-Pereira and Kamat, 2011).
For secondary screening based on the disk-diffusion method, strain inocula of 0.1 ml (final concentration of 106 CFU ml) was swabbed on the TY agar plates, and the isolates were directly spot inoculated onto sterilized Whatman paper disks of 7 mm diameter, which were deposited on the agar surface, and 15 μl of each strain was added on the top of the disk. The disks were then incubated at 28°C for 24 h. The antagonistic interaction was detected by the presence of the inhibition zone (Tendencia, 2004).
2.5.2. Inoculation of faba-bean plants with a selected rhizobacterial consortium
2.5.2.1. Bacterial inoculum production
Each bacterial strain was grown in a liquid YEM medium shaken at 180 rpm for 24 h at 28°C. The bacterial inoculum concentration used was approximately 108 UFC ml for each strain. The mixed consortium inoculum was prepared by mixing equal amounts of the three strain solutions (20WT2E4, 21WT2E4, and 25WT2E4) and R. laguerreae (the consortium) compared with the inoculation only with R. laguerreae.
2.5.2.2. Plantlets growing and inoculation
Seeds of the faba-bean (Vicia faba), Alfia variety, were surface sterilized using acidified 0.2% mercury chloride for 3 min, rinsed five times thoroughly with sterile distilled water, and allowed to germinate on sterile agar plates. After 4 days, seedlings were transferred into plastic pots (10 cm × 12 cm × 12 cm) containing 1 kg of a mixture of sterilized agricultural soil with a low available P level (3 ppm). Rock phosphate was added to the substrate as the only P source at a rate of 1.5 g per 1 kg. Two plantlets per pot were considered, and each one was inoculated with 5 ml of inoculum consisting of rhizobia only (R. laguerreae) or the bacterial consortium [R. laguerreae + rhizobacteria (20WT2E4 + 21WT2E4 + 25WT2E4)]. The pots were designed in triplicate, with two seeds per pot and one inoculated pot without seed as a control for each inoculated treatment. Four treatments were tested: the individual strain [Rhizobia (R. laguerreae) and the consortium of the three rhizobacteria and rhizobia] and the controls with non-inoculated plants [a negative control (T–), pots without Hoagland nutrient solution, and a positive control (T+), pots were watered when required with Hoagland nutrient solution]. The pots were transferred to a growth chamber (phytotron) under controlled conditions (25°C, 70% humidity, 16:8 h photoperiod, and illumination intensity of 240 mmol m−2 s−1). A second reminder inoculation was conducted after 4 days of the first one.
2.6. Assessment of inoculation effect on agrophysiological parameters
2.6.1. Pre-harvest studies (late vegetative parameters)
Stomatal conductance was measured (mmol m−2 s) by the SC-1 leaf porometer of treated plants (T+, T–, R. laguerreae, and consortium) to estimate the rate of gas exchange and transpiration through the leaf stomata was determined by the degree of stomatal opening. The Chlorophyll Content Index (CCI) was assessed on the central part of well-extended young leaves in plants 30 days after inoculation using the chlorophylometer CL-01 (Hansatech Instruments Ltd., UK) (Shrestha et al., 2012).
2.6.2. Dry weight determinations
Root and shoot parts of the plants were dried in an oven at 70°C for 48 h to determine their dry weights.
2.6.3. Determination of P and minerals contents in plants
2.6.3.1. Dosage of total P in the plant
Samples of 0.5 g of dry matter from different parts of the faba-bean plant were incinerated at 600°C for 6 h. The ashes were recovered and dissolved in 3 ml of HCl (10 N), and after filtration, the filtrate was adjusted to 100 ml with distilled water. A volume of 1 ml of the filtrate was added to 4 ml of distilled water and 5 ml of the ascorbic acid reagent and then incubated in a water bath at 95°C for 10 min. The OD was measured with a spectrophotometer at 825 nm (Wieczorek et al., 2022).
2.6.3.2. Dosage of other minerals in the plant
Mineral elements (K and Ca) in the different parts of the plants were determined on the same filtrates used for the P assay with a spectrophotometer (model AFP100, Biotech Management Engineering Co. Ltd., UK) (Liu et al., 2021).
2.6.4. Roots morphological parameters
After harvesting the plants, the roots were first washed with distilled water, and then, their architecture was immediately analyzed using the LA2400 scanner and WinRHIZO software (Regent Instruments Inc., Canada). Total root length, average root diameter, root volume, and root surface area were measured as the main root parameters (Gaudin et al., 2011).
2.6.5. Soil available P and acid phosphatase activity analysis
Available P content in the rhizosphere soil was measured using the Olsen (1954) method. Available P was determined after its extraction from the soil with 0.5 M NaHCO3, adjusted to pH 8.5. The acid phosphatase (APase) activity of the soils was determined according to Tabatabai and Bremner (1969) using the para-nitrophenolate colorimetric assay method and buffer acetate (pH <6.5). The reaction was stopped by adding 1 ml of NaOH (0.5 N). The APase activity was determined by measuring the formation of para-nitrophenolate at a wavelength of 410 nm.
2.7. Statistical analysis
The experimental data for different assessments were statistically analyzed using an analysis of variance (ANOVA) by the SPSS version 20 software (IBM-SPSS Inc., Chicago, IL). The collected data were analyzed by the HSD Tukey's test with a 5% probability threshold. The graphs were performed using the GraphPad Prism 8 software.
3. Results
3.1. Rhizobacterial isolation and screening of PGP rhizobacteria
In total, 115 bacteria were isolated from the collected legume rhizospheric soils, 34 were obtained from the CH2E3 area of Sidi El Ayedi, 24 from the CH3E5 area of Ouled Said, 26 from the WT3E5 of Imiter, and 31 from the WT2E4 area of Tizagazouine. The isolate that demonstrated a halo around the colonies as an indication of phosphate solubilization depends on the biological N fixation activity, the tolerance level to osmotic stress, and the qualitative phosphate solubilization in Pikovskaya medium (PVK) and NBRIP medium. A collection of 26 isolates were selected and conserved for subsequent tests.
3.1.1. Phosphate solubilization ability on Pikovskaya medium
Halo production is an indication of natural phosphate solubilization, as demonstrated. All selected bacteria can produce phosphate solubilizing halo (Figure 1). The test was done in Pikovskaya medium (PVK) agar supplemented with natural rock phosphate. As a result, 11 isolates showed a high ability for P solubilization. The isolates 20WT2E4, 1CH2E3, and 5CH2E3 revealed a strong ability for P solubilization with the presence of a clear halo around the colonies measuring 30 ± 1.0 mm, 27.7 ± 0.6 mm, and 28.3 ± 1.2 mm, respectively.
3.1.2. Biological nitrogen fixation
The results of the biological N fixation screening showed that 42.30% of the tested collection presented high N fixation potential (+++), 38.46% fixed N moderately (++), and 19.23% had weak potential (+) for N fixation (Table 1).
3.1.3. Effect of osmotic stress
The effect of different levels of osmotic stress on the growth of the selected isolates was determined (Figure 2). All selected isolates were able to grow at higher osmotic stress induced by 30% (−1.5 MPa) and 40% (−1.99 MPa) of PEG 8000, although growth was drastically reduced. The highest growth of 30% PEG 8000 was obtained by the isolates 25WT2E4, 1CH2E4, 21WT2E4, 17WT2E4, and 16WT2E4, respectively. However, the highest growth of 40% PEG 8000 was recorded in the isolates 25WT2E4, 26WT2E4, 13WT2E4, 17WT2E4, and 1CH2E4, respectively. Moreover, it was also noticed that the isolates 25WT2E4, 17WT2E4, and 1CH2E4 were ranked, respectively, as being tolerant to high osmotic stress. In addition, the highest reduction in growth as compared to control conditions was observed for the isolate 7WT2E4, in which the growth declined remarkably with the increase in PEG concentration.
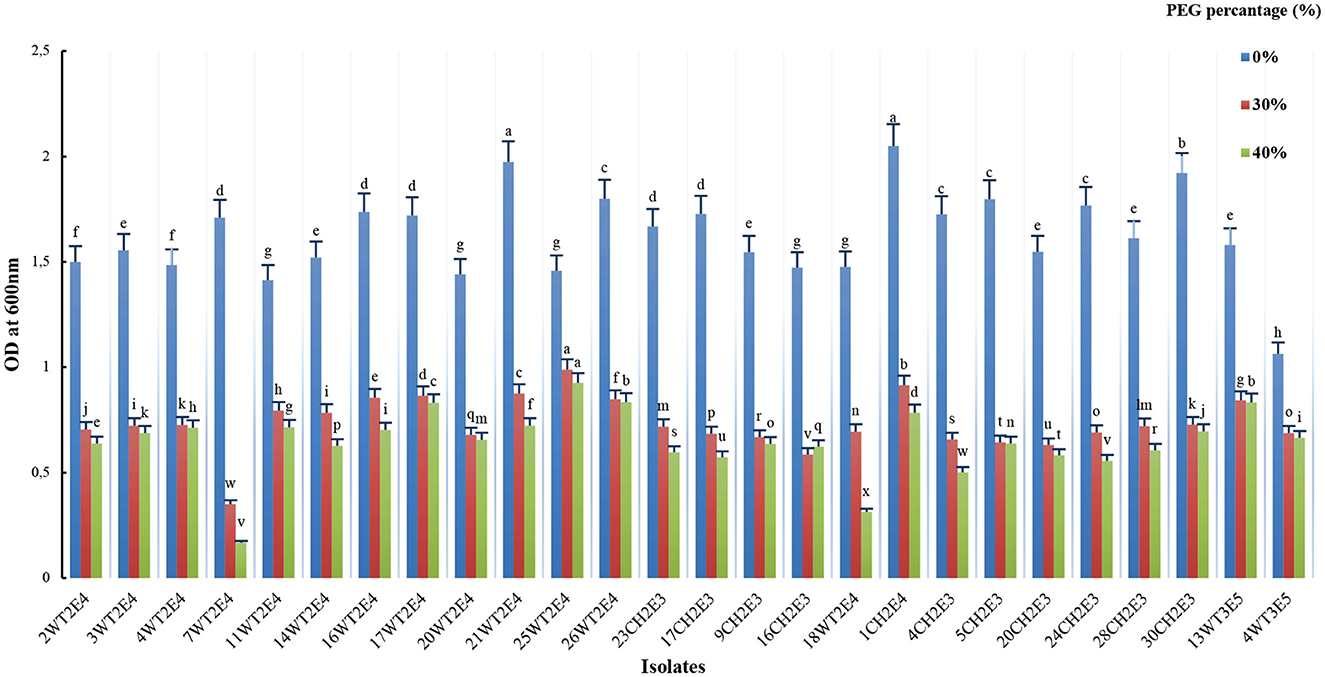
Figure 2. Effect of osmotic stress on the growth of the tested isolates. Growth obtained by the isolates on different concentrations of (0%, 30%, and 40%) PEG 8000. Different letters indicate that values are statistically different at a P-value of ≤ 0.0.
3.2. Evaluation of isolates for PGP traits
3.2.1. Quantification of released phosphate in the NBRIP medium
All isolated rhizobacteria solubilize the P in the NBRIP medium. The isolates 20WT2E4, 25WT2E4, 18WT2E4, and 1CH2E3 were the most P solubilizing bacteria, with concentrations of released P of 79.15 ± 0.2a mg/L, 61.95 ± 0.3b mg/L, 60.60 ± 0.2bc mg/L, and 60.37 ± 0.2bc mg/L, respectively. The weakest concentration of released P was approximately 26.90 mg/L, corresponding to isolate 17 CH2E3 (Figure 3). However, other isolates presented moderate P solubilization activities.
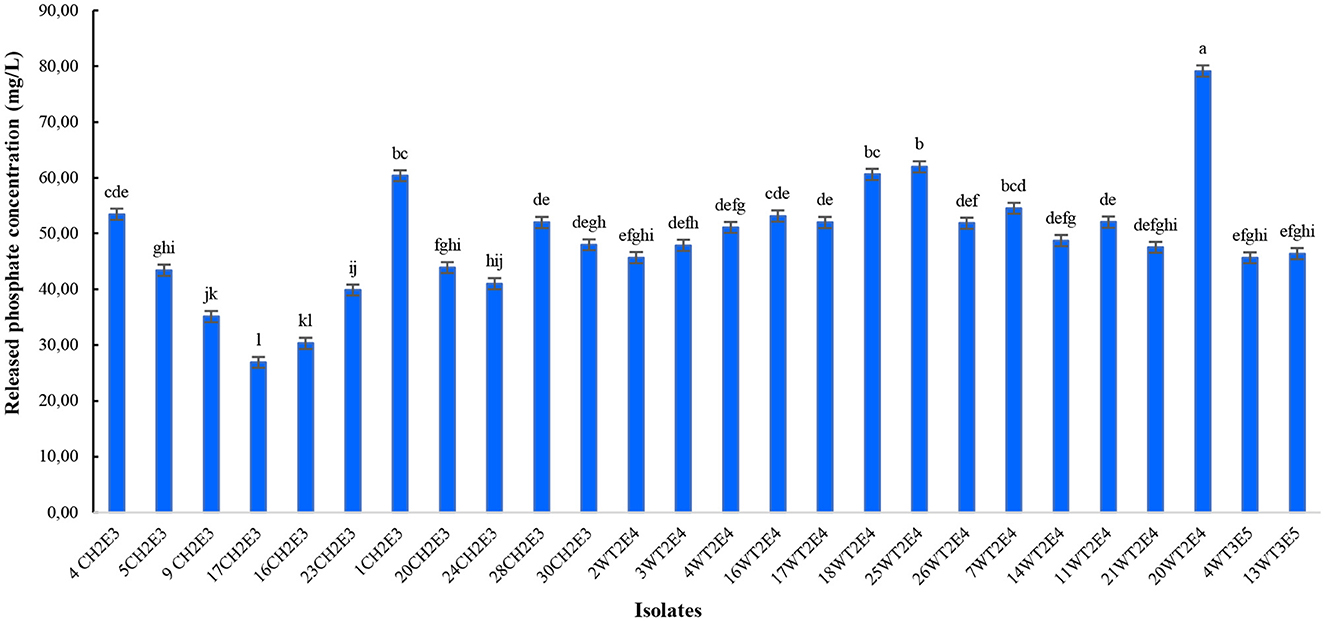
Figure 3. Quantification of soluble P content released by different selected isolates. Different letters indicate that values are statistically different at a P-value of ≤0.05.
3.2.2. Auxin production
The quantification of auxin biosynthesis by the rhizobacteria showed that the concentrations varied between the isolates. It was noticed that the isolate 20WT2E4 was more performing in auxin production and revealed the highest concentration of 126.14 ± 4.1a μg/ml followed by the isolate 21WT2E4 with 46.22 ± 5.3b μg/ml and then the isolates 28CH2E3 and 24CH2E3 with auxin concentrations of 25.64 ± 6.1c μg/ml and 25.42 ± 4.4d μg/ml, respectively (Figure 4). In general, the remaining isolates were low producers of auxin, with concentrations that did not exceed 10 μg/ml.
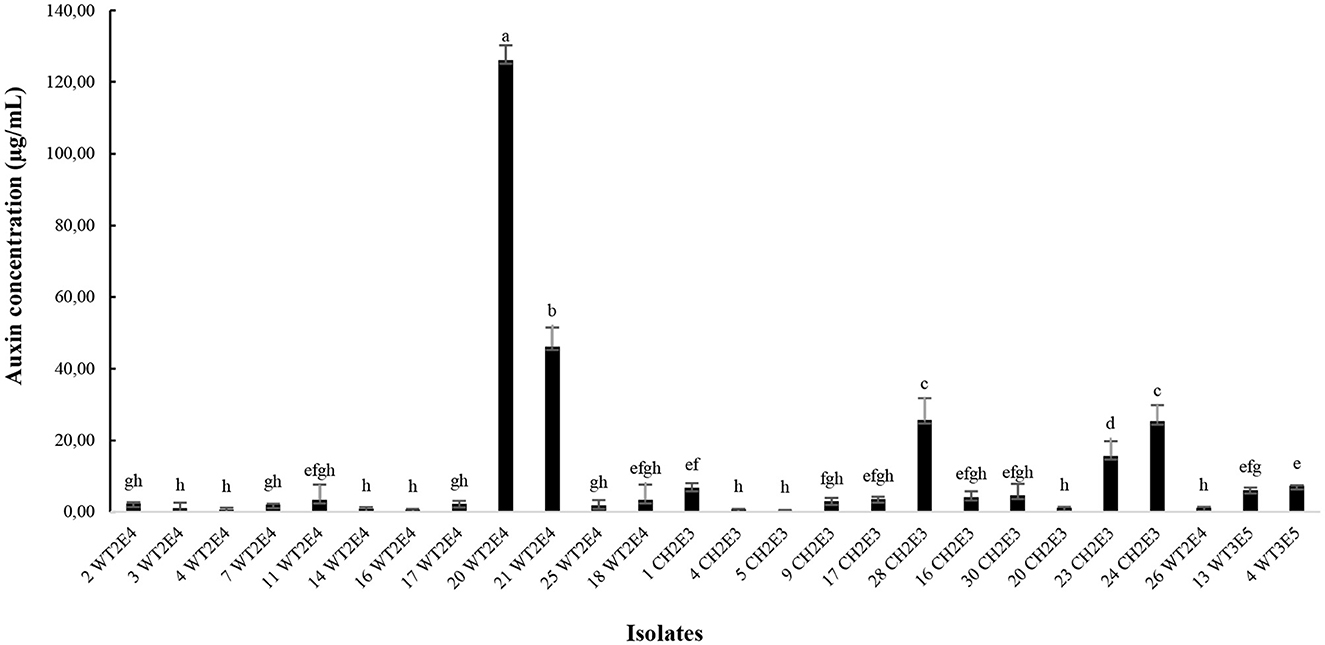
Figure 4. Auxin production by different selected isolates. Different letters indicate that values are statistically different at a P-value of ≤0.05.
3.2.3. Siderophore production
The results presented in Figure 5 show a significant variation in siderophore production, on a standard succinate medium, between the tested isolates. Indeed, the isolates 5CH2E3 and 11WT2E4 produced the highest siderophore concentrations, with more than 3,500 μM. The isolates obtained with 2WT2E4, 20WT2E4, 20CH2E3, and 13WT3E5 showed relatively high concentrations of siderophore between 1,700 and 2,000 μM. Seven isolates produced very low siderophore concentrations that did not exceed 400 μM (Figure 5).
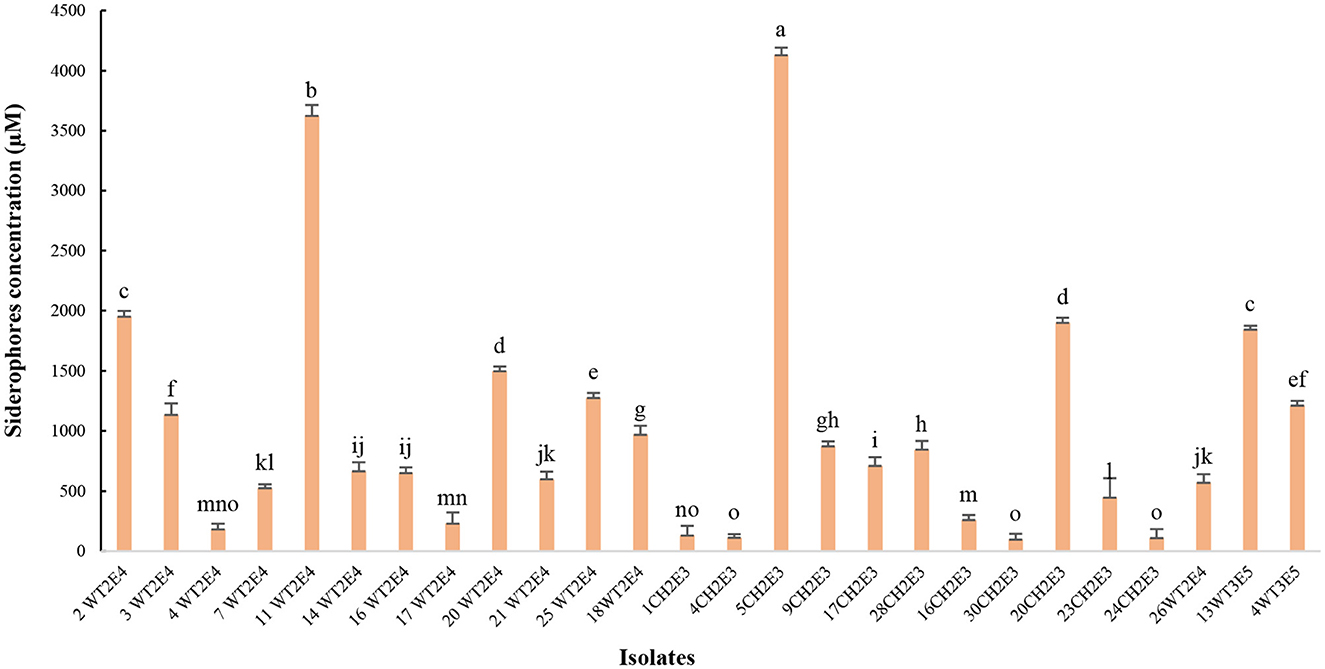
Figure 5. Siderophores concentrations (μM) produced by different selected isolates. Different letters indicate that values are statistically different at a P-value of ≤0.05.
3.2.4. Ammoniac production
3.2.4.1. Qualitative test
As indicated by the results in Table 2, the ammonia production test showed that 23.07% of the isolates produced was highly (+++) ammoniac as indicated by the intensity of the brown color. Moreover, it was revealed that 42.30% of the isolates produced was moderately (++) ammoniac and 34.61% produced was weakly (+) ammoniac. The results of this qualitative test allowed us to select isolates with higher ammonia revelation for the quantitative test.
3.2.4.2. Quantitative test
From the qualitative test, six isolates (20WT2E4, 4CH2E3, 30CH2E3, 20CH2E3, 23CH2E3, and 24CH2E3) were selected as the most producer of ammonia. As detailed in Figure 6, the quantitative test demonstrated that the isolate 23CH2E3 registered the highest concentration of ammonia (242.72 ± 4.9a μg/L), followed by the isolates 30CH2E3 (221.41 ± 0.3b μg/L), 24CH2E3 (199.17 ± 2.2c μg/L), 4CH2E3 (186.08 ± 1.19d μg/L), 20WT2E4 (181.17 ± 1.8e μg/L), and 20CH2E3 (199.17 ± 2.2f μg/L).
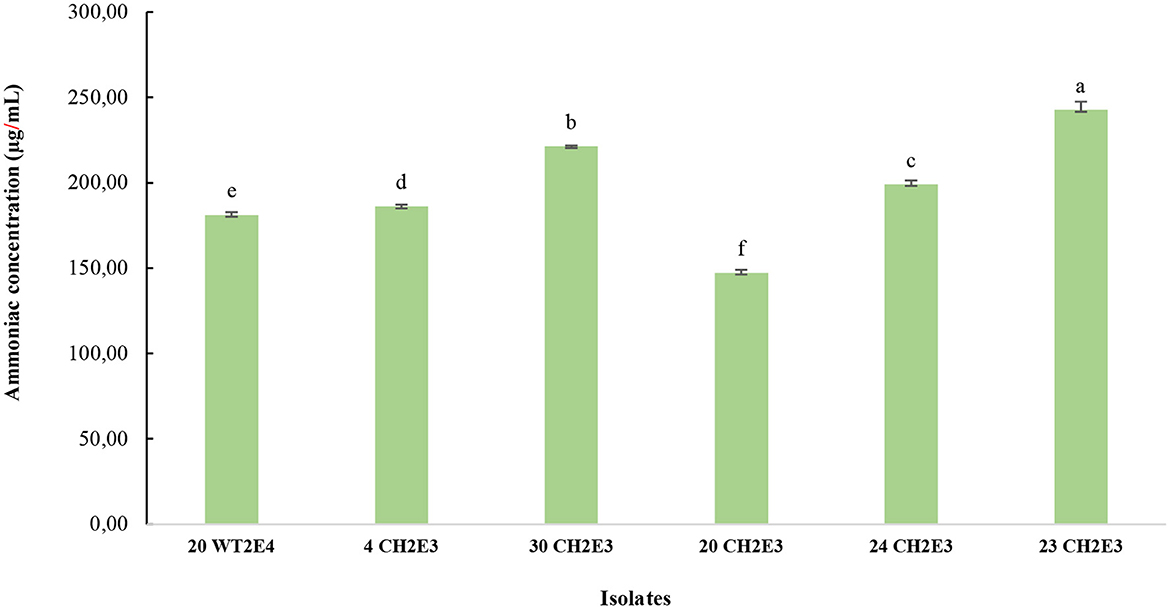
Figure 6. Ammoniac production by the selected isolates for the quantitative test. Different letters indicate that values are statistically different at a P-value of ≤0.05.
3.2.5. Assessment of isolates for their halotolerant levels
The selected collection demonstrated an interesting halotolerant ability, as detailed in Table 3. All 26 tested isolates were able to tolerate a salinity of up to 5% NaCl. The majority of them, 21 isolates, were able to tolerate salinity levels up to 10% NaCl, and only 6 isolates tolerated the concentration of 15% NaCl (Table 3).
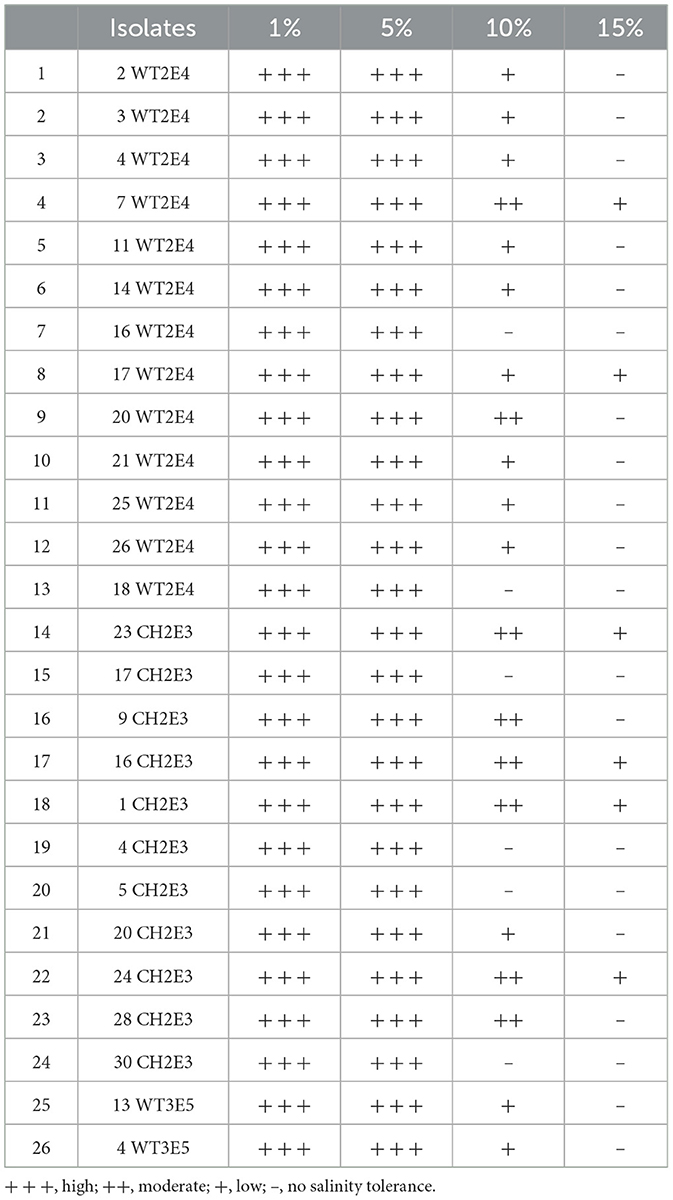
Table 3. Results of the halotolerance test corresponding to the selected isolates at four salinity levels (1%, 5%, 10%, and 15% NaCl).
3.2.6. Antifungal activity against Fusarium oxysporum
The bacterial strain 21WT2E4 showed the highest antifungal activity against F. oxysporum, restricting mycelial growth in a dual culture plate assay with an inhibition percentage of up to 70% (Figure 7). The isolates 13WT3E5, 20CH2E3, and 4WT2E4 also presented interesting inhibition percentages of F. oxysporum at 46.42%, 44.04%, and 40.47%, respectively. Nine other isolates tested did not present any antagonistic activity against F. oxysporum.
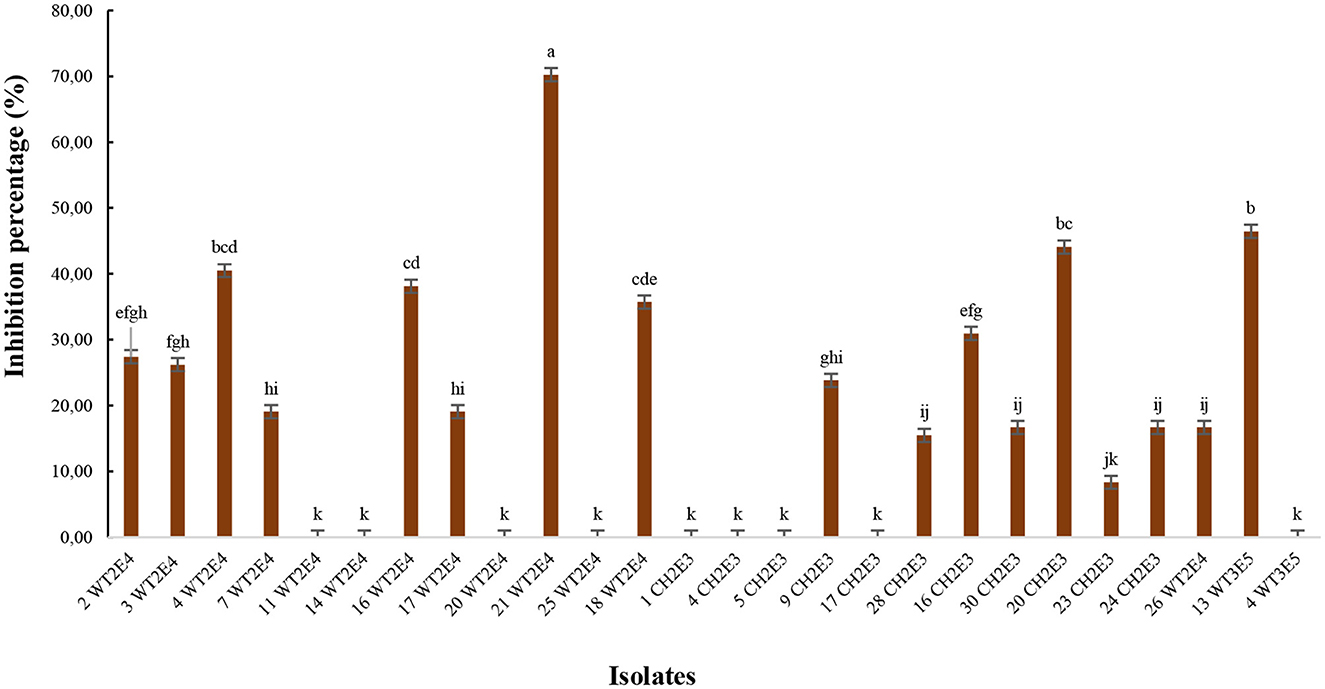
Figure 7. Antifungal effect of bacterial isolates against F. oxysporum. Different letters indicate that values are statistically different at a P-value of ≤0.05.
3.2.7. Phytase activity
Out of 26 isolates, only isolate 21WT2E4 grew on phytase-specific medium agar.
3.3. Phylogenetic analysis
The 16S rRNA gene sequences obtained were analyzed using bioinformatic tools (Figure 8). Bacterial isolates belonged to 13 different bacterial species, namely, Siccibacter colletis, Siccibacter turicensis, Enterobacter sp., Enterobacter huaxiensis, Pantoea sp., Pseudomonas punonensis, Acinetobacter dijkshoorniae, Acinetobacter calcoaceticus, Acinetobacter lactucae, Bacillus qingshengii, Bacillus sp., Bacillus huizhouensis, and Brevibacterium frigoritolerans.
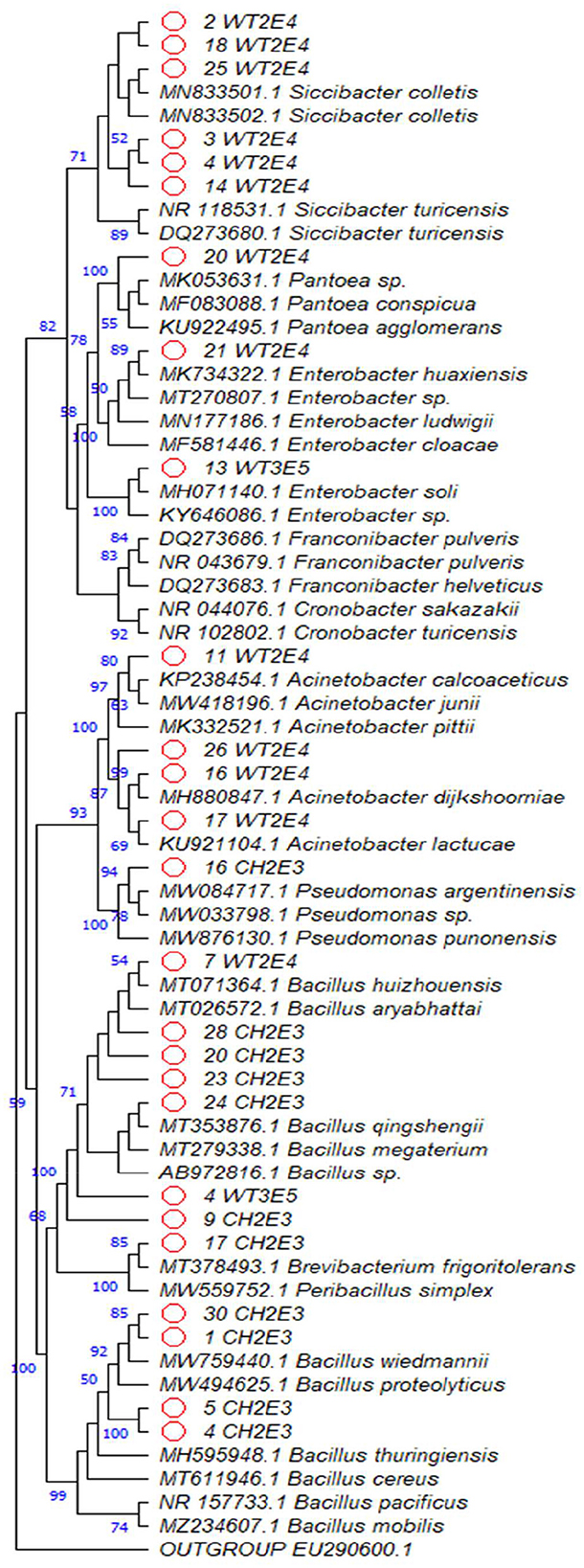
Figure 8. Phylogenetic tree based on the 16S rRNA gene sequences (consensus alignment) corresponding to selected PGPR from alfalfa rhizosphere. The evolutionary history was inferred by using the maximum likelihood method based on the Tamura–Nei model with 1,000 bootstrap replications. The percentage of trees in which the associated taxa clustered together are shown next to the branches. Evolutionary analyses were conducted in MEGA11.
Among the 6 genera identified, Bacillus is the predominant genus with 46.15%, followed by the genus Siccibacter with 23.07%, Acinetobacter with 15.38%, Enterobacter with 7.69%, and then Pantoea and Pseudomonas with the same percentage of 3.84%.
3.4. Effect of inoculation test
Different parameters of the faba-bean plant growth were determined to assess the impact of the inoculation on the individual strains and the consortium.
3.4.1. Effect of inoculation on stomatal conductance and chlorophyll content index as physiological parameters affecting biomass accumulation
The bacterial inoculation impacted positively the stomatal conductance of the plant compared with non-inoculated plants. Notably, the consortium ameliorates the plant stomatal conductance up to 207 mmol/m2 s, followed by the plant inoculated with rhizobia only up to 160 mmol/m2 s compared with the positive control (129 mmol/m2 s) and the negative control (98 mmol/m2 s) (Supplementary Figure S1).
On the contrary, the results of the Chlorophyll Content Index (CCI) estimation showed a positive effect of the inoculation compared to non-inoculated plants. The index of chlorophyll content increased significantly in the faba leaves inoculated with the consortium. As recorded, the index of chlorophyll was about 54.8, followed by the plant inoculated with R. laguerreae (36.15) compared to the non-inoculated plants, the positive control (26.03), and the negative control (20.43) (Supplementary Figure S2).
3.4.2. Determination of minerals in plants
As summarized in Figure 9, inoculation impacts the content of some minerals (K and Ca) and P in the plants. The measurement of P in the faba-bean shows that the consortium enhances the P in the inoculated plants (0.224 ± 0.04 B mg/g) compared to the rhizobia (0.074 ± 0.043 C mg/g) and the negative control (0.074 ± 0.02 C mg/g). In addition, the measurement that the inoculation impacts the content of K and Ca. While the inoculation with bacterial inoculum influences the content of minerals, the plant inoculated with R. laguerreae had k = 60.49 ± 3.5b mg/gDM and Ca = 12.51 ± 0.05b mg/gDM and the plant inoculated with consortium had k = 31.69 ± 2.1b mg/gDM and Ca=5.59 ± 0.2c mg/gDM.
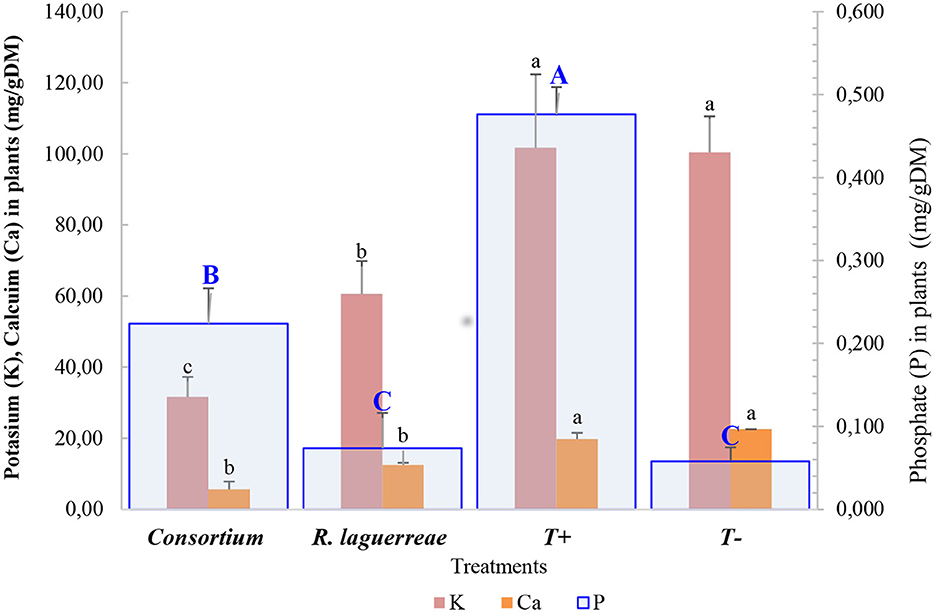
Figure 9. Minerals (K and Ca) and P content in the faba-bean plant in response to different treatments. For each element, different letters indicate that values are statistically different at a P-value of ≤0.05.
3.4.3. Dry weight determination
The inoculation of the faba-bean plants with rhizobia alone induced a slight increase in root biomass and shoot biomass compared to the non-inoculated negative control (T–) and positive control (T+) (Figure 10A). Meanwhile, an important increase in this parameter was noticed when the plants were inoculated with the consortium compared to the other treatments (R. laguerreae, positive and negative controls). As shown in Figure 10B, the consortium significantly improved shoot biomass, and it induced an increase of 1.83 times more than the positive control and an increase of 1.29 times more than R. laguerreae.
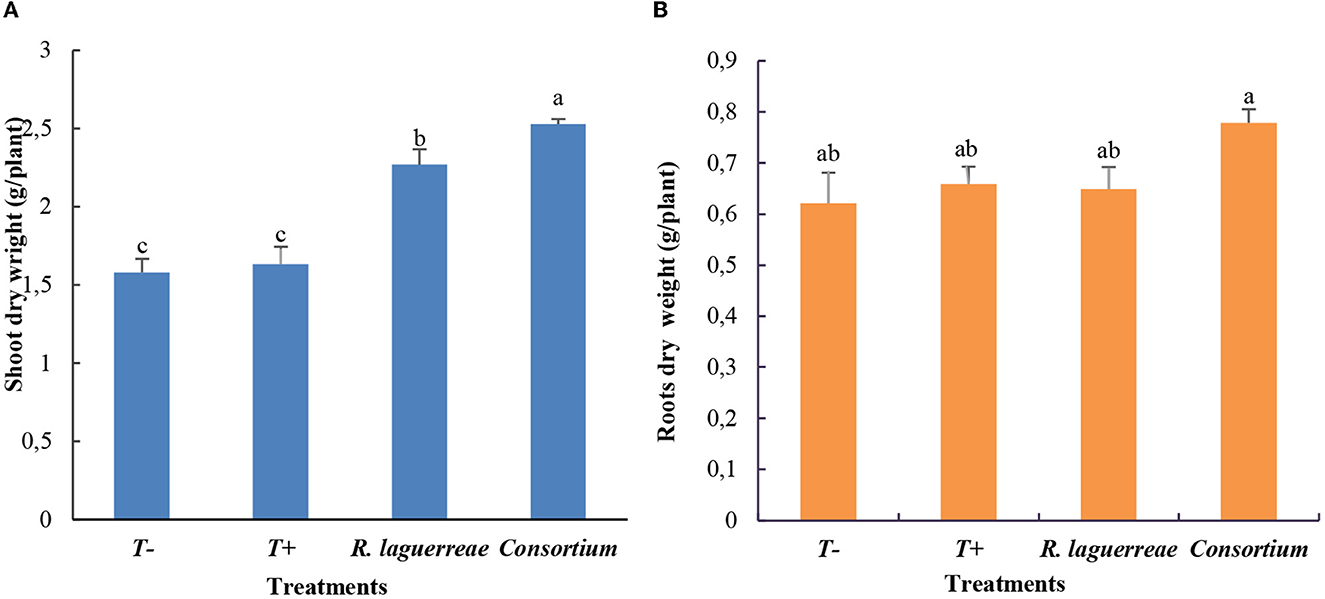
Figure 10. Root (A) and shoot (B) biomasses of faba-bean in response to different treatments. Each value represents the mean ± of three independent replicates; different letters indicate that values by Tukey's test are statistically different at a P-value of ≤0.05.
In contrast, we noticed a slight increase of root biomass in plants inoculated with the consortium (0.77 g) compared to those inoculated with R. laguerreae (0.64 g) and with the positive control (0.65 g), respectively.
3.4.4. Roots morphology parameters
The observation and analysis of root morphological traits of the faba-bean plants submitted to different treatments were done using WinRHIZO (LA2400 scanner). The data collected showed the difference between the inoculated and the non-inoculated control plants. Based on the root architecture observation, the inoculation of plants improved root growth, especially in the plants inoculated with the consortium. The analysis of morphological traits revealed that the plants' inoculation with consortium shows a significant promoter effect on the total length of roots (Supplementary Figure S3). Similarly, inoculation of plants with these rhizobia including consortium significantly improved the surface area (Supplementary Figure S3A) and the length of the roots (Supplementary Figure S3B), compared to controls “T (+) 440 cm2” and “T (–) 360 cm2” for surface area and “1.33 mm” and “1.45 mm” compared to controls “T (+) 1.05 mm” and “T (–) 0.80 mm” for diameter, respectively.
3.4.5. Available P and acid phosphatase analysis
The inoculation of the faba-bean plants with the consortium or rhizobia induced an increase of released available P in the rhizosphere compared to the non-inoculated negative control (T–) (no nutrient solution added). We noticed that there was no significant difference between the rhizosphere of plants inoculated with rhizobia alone and those of plants inoculated with the consortium.
The analysis of acid phosphatase (APase) activity showed that the rhizospheric soil of plants inoculated with rhizobia alone showed an increase in APase activity compared with non-inoculated plants and exceeded 50 nmol/g/min (Figure 11). The soil of plants inoculated with rhizobia-including consortium showed the highest APase activity that exceeded 85 nmol/g/min, indicating the synergistic effect between PGPRs and rhizobia under low availability of P.
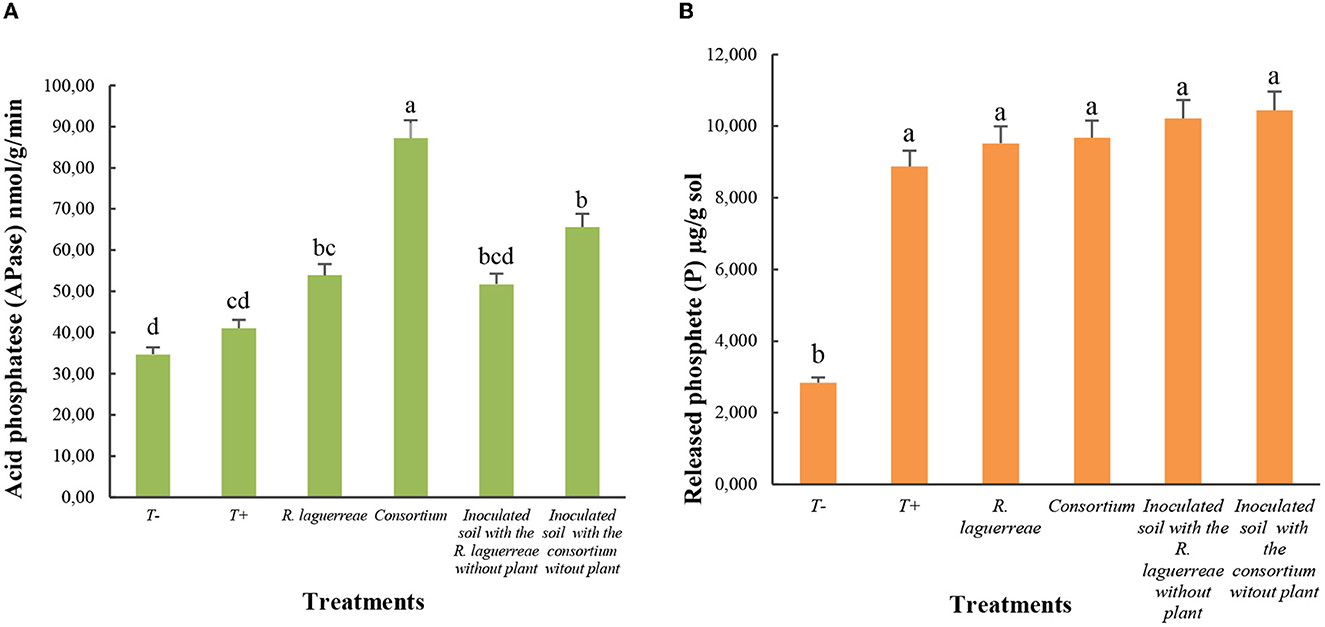
Figure 11. Available P (A) and acid phosphatase activity (B) and analysis in rhizospheric soil of plants inoculated with rhizobia alone or with the consortium. Each value represents the mean ± of three independent replicates; different letters indicate that the values by Tukey's test are statistically different at a P-value of ≤0.05.
4. Discussion
The use of beneficial plant growth-promoting rhizobacteria for plant inoculation to enhance crop production, even under stress conditions, is an important practice for agriculture sustainability.
In the present study, rhizospheric soils were collected from the alfalfa plant grown on low P fertilized for the isolation of PGP rhizobacteria. In addition, in vitro assays and physiological characterizations were carried out to evaluate PGP traits. Based on the screening tests of PGP traits, including P solubilization, N fixation, and osmotolerance ability it allowed the obtention of a collection of 26 PGPR selected for further studies and molecular identification. The physiological traits were chosen to establish a bacterial consortium based on specific biological activities to create complementarity among its members and act in a synergistic way for promoting plant growth under stress.
The most important biological activity focused on the rhizobacteria, as a PGP trait, is the inorganic P solubilization due to the importance of this nutrient for biological N fixation, plant growth, and productivity (Etesami and Maheshwari, 2018; Elhaissoufi et al., 2021). In this study, all the selected rhizobacteria solubilized the complex rock phosphate (RP) with important levels of released, available P that presented variation between isolates. Different studies identified several bacterial isolates that can promote plant growth, improve rhizosphere area, and solubilize different sources of immobilized P (Chen et al., 2006; Granada et al., 2018). In addition, as a complementary activity of P solubilization, there is auxin production. This phytohormone is responsible for the different stages of plant development (Jahn et al., 2021). In addition to their effect on the architecture and the development of the roots, the auxin also enhances nutrient absorption, especially P (Talboys et al., 2014). Our study demonstrated that the selected rhizobacteria produced the auxin-indole-3-acetic acid (IAA) with varying concentrations between them, and this result was reported for 80% of the tested bacteria by Gilbert et al. (2018). Nutrient availability in the soil is a crucial condition as is the availability of iron. For this objective, the PGPR isolates were tested for their ability to siderophores production. As chelates of insoluble iron, siderophores can have alternative functions such as non-iron metal transport, toxic metal sequestration, protection from oxidative stress, and antibiotic activity (Kramer et al., 2020). In our study, it was noted that the majority of the selected isolates from the alfalfa rhizosphere in arid areas showed siderophores production. This behavior may be related to the adaptation against stressing conditions prevailing in these poor soils and their pedological characteristics. Indeed, Nicolitch et al. (2016) confirmed the hypothesis of a variable selection of specific rhizosphere bacterial communities according to the soil conditions and the plant's nutritional requirements.
In contrast, among the isolated PGPR collection, 23.07% of the isolates produced highly ammoniac. In addition, from the 26 tested isolates, 21 isolates were able to tolerate salinity levels up to 10% NaCl and only 6 isolates tolerated it up to 15%. This can be an interesting PGP trait that impacts the growth of plants under extreme conditions of saline and/or dry areas. For this issue, the PGPR collection was also tested for its ability to resist water deficit, and our isolates grew in a medium containing 40% of PEG 8000. Our PGPR isolates were also tested for their antifungal activity and some of them showed antagonistic activity against F. oxysporum, isolated from field-infected faba-bean plants, and caused high inhibition that reached 70% of the mycelial growth.
Based on the interesting PGP traits and the test of antagonistic activity between the retained isolates and the rhizobia strain, a bacterial consortium was established. The three interesting rhizobacteria were selected as candidates for the PGPR rhizobia consortium and the inoculation test on the faba-bean plants.
The results of molecular studies, based on the 16S rRNA gene, demonstrated a diverse taxonomic structure, while 6 genera were identified, noting that Bacillus was the predominant genus (46.15%), followed by the Siccibacter (23.07%) and Acinetobacter (15.38%) genera. The Siccibacter genus is a new phylum with some well-defined characteristics as described by Stephan et al. (2014). As revealed by our study, one of the retained PGPR members of the consortium (25WT2E4) is Siccibacter colletis, a species characterized by important PGPR traits such as P solubilization, N fixation, and its halotolerant. The first description of Siccibacter colletis sp. as a novel species was reported in 2015 and has been isolated from poppy seeds and tea leaves (Jackson et al., 2015). Up to the present time, the study of Salazar-Ramírez et al. (2021) reported the isolation of Siccibacter colletis from the candelilla rhizosphere (Euphorbia antisyphilitica). This is the first study to isolate Siccibacter colletis from the alfalfa rhizosphere and assess their PGP traits such as siderophores production, P solubilization, indole-3-acetic acid (IAA) production, and tolerance to water and salt stresses, all of which make them useful for enhancing crop production under stress. Thus, this species was included in our established rhizobacterial consortium for inoculation. The second candidate, 20WT2E4, was identified as Pantoea sp. and the third one, 21WT2E4, was identified as Enterobacter huaxiensis. Recently, Ka-Ot and Joshi (Ka-Ot and Joshi, 2022) reported that the strain E. huaxiensis was able to be resistant to acidic conditions as well as Fe, Cd, and Cr and was able to remove 89%, 90%, and 82.45% of Fe, Cd, and Cr, respectively. Therefore, it could be used for the inoculation of plants in heavy metal-contaminated soils. However, the synergistic effect of more than one PGPR strain gathered as a consortium could be very interesting as a biofertilizer compared to as an individual strain. Eventually, the positive interaction between Enterobacter sp. Z1 and Klebsiella sp. Z2 exhibited great capacities for heterotrophic nitrification-aerobic denitrification (HNAD) and intracellular P accumulation. Strikingly, the co-cultured strains enhanced the removal efficiency of total N and P, with removal efficiencies of ammonia, nitrate, nitrite, and soluble P of 99.64%, 99.85%, 96.94%, and 66.7%, respectively (Zhang et al., 2019). In addition, the cooperation of the two strains belonging to the Pantoea and Enterobacter genera showed their potential for improving plant tolerance to stress and promoting maximum plant growth (PGP). It was found that they possess the nifH and acdS genes associated with N-fixation, ethylene production, and nitrogenase activity. The application of these strains to two sugarcane varieties increased several sugarcane physiological parameters, i.e., plant height, shoot weight, root weight, leaf area, chlorophyll content, and photosynthesis, in plants grown under greenhouse conditions (Singh et al., 2021). These findings support our choice to retain the three species, namely, Siccibacter colletis, Pantoea sp., and Enterobacter huaxiensis, for consortium establishment with R. laguerreae for the inoculation of the faba-bean plant under P deficiency. Such an inoculation increased the root parameters (weight, volume, diameter, length, and surface) and the shoot growth and other physiological parameters of the faba-bean inoculated plants under this nutrient constraint. Furthermore, the inoculation with this consortium increased the availability of inorganic P in the rhizosphere of inoculated plants. This P solubilization could be due to the production of organic acids, which are ubiquitous among rhizosphere P-solubilizing rhizobacteria in P-deficient soils (Elhaissoufi et al., 2021). Based on our knowledge, our study is the first report on faba-bean growth promotion by Siccibacter colletis, Pantoea sp., and Enterobacter huaxiensis as synergistic PGPRs with rhizobia in a consortium, highlighting their PGPR traits particularly under the P-limiting conditions. Our results also point to the suggested role that our selected PGPR-rhizobia consortium could play, besides the nutritional effects (N and P), in helping inoculated plants cope with other abiotic stresses such as salt stress and water deficit, which are the most prevailing stresses affecting legume crops in the Mediterranean basin and Africa.
5. Conclusion
The isolation of rhizobacteria from the M. sativa rhizosphere and their characterization allowed us to select a collection of 26 isolates with interesting PGP traits. Based on 16S rRNA gene sequencing, we identified eight different genera. In addition, the molecular characterization revealed for the first time the identification and isolation, from the M. sativa rhizosphere, of the S. colletis species and the identification of its interesting PGPR traits, and thus, it could be used for legume inoculation as a member of the rhizobia-including consortium. The results of the inoculation of faba-bean with the rhizobacterial consortium (S. colletis, E. huaxiensis, Pantoea sp., and R. laguerreae) under P deficiency significantly improved the plant growth compared to non-inoculated controls or plants inoculated only with rhizobia, confirming thus the synergy between the PGPRs strains and the rhizobia for their beneficial effects for faba-bean inoculation under P deficiency conditions.
Data availability statement
The datasets presented in this study can be found in online repositories. The names of the repository/repositories and accession number(s) can be found in the article/Supplementary material.
Author contributions
IC planned and designed the research, investigation, methodology, resources, software, visualization, and writing—original draft. JZ performed the isolates' molecular identification. AI performed soil analysis and conducted data analysis. SC performed plant analysis and conducted data analysis. RS conducted data analysis and prepared figures. JG performed project administration, conceptualization, and review and editing. YZ and LK performed funding acquisition, investigation, and project administration. AB conducted investigation and project administration. CG performed conceptualization, project administration, funding acquisition, supervision, and writing—review and editing. All authors contributed to the article and approved the submitted version.
Funding
This study was financially supported by the Innovation-OCP Group, represented by YZ, within the framework of the AS1-Anhalt-UM6p project (2020–2023).
Conflict of interest
The authors declare that the research was conducted in the absence of any commercial or financial relationships that could be construed as a potential conflict of interest.
Publisher's note
All claims expressed in this article are solely those of the authors and do not necessarily represent those of their affiliated organizations, or those of the publisher, the editors and the reviewers. Any product that may be evaluated in this article, or claim that may be made by its manufacturer, is not guaranteed or endorsed by the publisher.
Supplementary material
The Supplementary Material for this article can be found online at: https://www.frontiersin.org/articles/10.3389/fsufs.2023.1134809/full#supplementary-material
References
Albdaiwi, R. N., Khyami-Horani, H., Ayad, J. Y., Alananbeh, K. M., and Al-Sayaydeh, R. (2019). Isolation and characterization of halotolerant plant growth promoting rhizobacteria from durum wheat (Triticum turgidum subsp. durum) cultivated in saline areas of the Dead Sea region. Front. Microbiol. 10, 1639. doi: 10.3389/fmicb.2019.01639
Becerra-Castro, C., Monterroso, C., Prieto-Fernández, A., Rodríguez-Lamas, L., Loureiro-Viñas, M., Acea, M. J., et al. (2012). Pseudometallophytes colonising Pb/Zn mine tailings: a description of the plant-microorganism-rhizosphere soil system and isolation of metal-tolerant bacteria. J. Hazard. Mater. 217–218, 350–359. doi: 10.1016/j.jhazmat.2012.03.039
Behera, B., Das, T. K., Raj, R., Ghosh, S., Raza, M. B., and Sen, S. (2021). Microbial consortia for sustaining productivity of non-legume crops: prospects and challenges. Agric. Res. 10, 1–14. doi: 10.1007/s40003-020-00482-3
Blom, D., Fabbri, C., Connor, E. C., Schiestl, F. P., Klauser, D. R., Boller, T., et al. (2011). Production of plant growth modulating volatiles is widespread among rhizosphere bacteria and strongly depends on culture conditions. Environ. Microbiol. 13, 3047–3058. doi: 10.1111/j.1462-2920.2011.02582.x
Chamkhi, I., Benali, T., Aanniz, T., El Menyiy, N., Guaouguaou, F.-E., El Omari, N., et al. (2021). Plant-microbial interaction: the mechanism and the application of microbial elicitor induced secondary metabolites biosynthesis in medicinal plants. Plant Physiol. Biochem. 167, 269–295. doi: 10.1016/j.plaphy.2021.08.001
Chamkhi, I., El Omari, N., Balahbib, A., El Menyiy, N., Benali, T., and Ghoulam, C. (2022). Is the rhizosphere a source of applicable multi-beneficial microorganisms for plant enhancement? Saudi J. Biol. Sci. 29, 1246–1259. doi: 10.1016/j.sjbs.2021.09.032
Chandra, D., Srivastava, R., and Sharma, A. K. (2018). Influence of IAA and ACC deaminase producing fluorescent pseudomonads in alleviating drought stress in wheat (Triticum aestivum). Agric. Res. 7, 290–299. doi: 10.1007/s40003-018-0305-y
Chen, Y. P., Rekha, P. D., Arun, A. B., Shen, F. T., Lai, W.-A., and Young, C. C. (2006). Phosphate solubilizing bacteria from subtropical soil and their tricalcium phosphate solubilizing abilities. Appl. Soil Ecol. 34, 33–41. doi: 10.1016/j.apsoil.2005.12.002
Choudhary, D. K., Kasotia, A., Jain, S., Vaishnav, A., Kumari, S., Sharma, K. P., et al. (2016). Bacterial-mediated tolerance and resistance to plants under abiotic and biotic stresses. J. Plant Growth Regul. 35, 276–300. doi: 10.1007/s00344-015-9521-x
Elhaissoufi, W., Ghoulam, C., Barakat, A., Zeroual, Y., and Bargaz, A. (2021). Phosphate bacterial solubilization: a key rhizosphere driving force enabling higher P use efficiency and crop productivity. J. Adv. Res. 38, 13–28. doi: 10.1016/j.jare.2021.08.014
Etesami, H., and Maheshwari, D. K. (2018). Use of plant growth promoting rhizobacteria (PGPRs) with multiple plant growth promoting traits in stress agriculture: action mechanisms and future prospects. Ecotoxicol. Environ. Saf. 156, 225–246. doi: 10.1016/j.ecoenv.2018.03.013
Gaudin, A. C. M., Mcclymont, S. A., Holmes, B. M., Lyons, E., and Raizada, M. N. (2011). Novel temporal, fine-scale and growth variation phenotypes in roots of adult-stage maize (Zea mays L.) in response to low nitrogen stress. Plant Cell Environ. 34, 2122–2137. doi: 10.1111/j.1365-3040.2011.02409.x
Gilbert, S., Xu, J., Acosta, K., Poulev, A., Lebeis, S., and Lam, E. (2018). Bacterial production of indole related compounds reveals their role in association between duckweeds and endophytes. Front. Chem. 6, 265. doi: 10.3389/fchem.2018.00265
Glickmann, E., and Dessaux, Y. (1995). A critical examination of the specificity of the salkowski reagent for indolic compounds produced by phytopathogenic bacteria. Appl. Environ. Microbiol. 61, 793–796. doi: 10.1128/aem.61.2.793-796.1995
Goswami, D., Thakker, J. N., and Dhandhukia, P. C. (2016). Portraying mechanics of plant growth promoting rhizobacteria (PGPR): a review. Cogent Food Agric. 2, 1127500. doi: 10.1080/23311932.2015.1127500
Granada, C. E., Passaglia, L. M. P., de Souza, E. M., and Sperotto, R. A. (2018). Is phosphate solubilization the forgotten child of plant growth-promoting rhizobacteria? Front. Microbiol. 9, 2054. doi: 10.3389/fmicb.2018.02054
Ilyas, N., Mumtaz, K., Akhtar, N., Yasmin, H., Sayyed, R. Z., Khan, W., et al. (2020). Exopolysaccharides producing bacteria for the amelioration of drought stress in wheat. Sustainability 12, 8876. doi: 10.3390/su12218876
Jackson, E. E., Masood, N., Ibrahim, K., Urvoy, N., Hariri, S., and Forsythe, S. J. (2015). Description of Siccibacter colletis sp. nov., a novel species isolated from plant material, and emended description of Siccibacter turicensis. Int. J. Syst. Evol. Microbiol. 65, 1335–1341. doi: 10.1099/ijs.0.000108
Jahn, L., Hofmann, U., and Ludwig-Müller, J. (2021). Indole-3-acetic acid is synthesized by the endophyte cyanodermella asteris via a tryptophan-dependent and -independent way and mediates the interaction with a non-host plant. Int. J. Mol. Sci. 22, 2651. doi: 10.3390/ijms22052651
Kanchiswamy, C. N., Malnoy, M., and Maffei, M. E. (2015). Chemical diversity of microbial volatiles and their potential for plant growth and productivity. Front. Plant Sci. 6, 151. doi: 10.3389/fpls.2015.00151
Ka-Ot, A. L., and Joshi, S. R. (2022). Application of acid and heavy metal resistant bacteria from rat-hole coal mines in bioremediation strategy. J. Basic Microbiol. 62, 480–488. doi: 10.1002/jobm.202100241
Kour, D., Rana, K. L., Kaur, T., Yadav, N., Yadav, A. N., Kumar, M., et al. (2021). Biodiversity, current developments and potential biotechnological applications of phosphorus-solubilizing and -mobilizing microbes: a review. Pedosphere 31, 43–75. doi: 10.1016/S1002-0160(20)60057-1
Kramer, J., Özkaya, Ö., and Kümmerli, R. (2020). Bacterial siderophores in community and host interactions. Nat. Rev. Microbiol. 18, 152–163. doi: 10.1038/s41579-019-0284-4
Kumawat, K. C., Sharma, P., Nagpal, S., Gupta, R. K., Sirari, A., Nair, R. M., et al. (2021). Dual microbial inoculation, a game changer? Bacterial biostimulants with multifunctional growth promoting traits to mitigate salinity stress in spring mungbean. Front. Microbiol. 11, 600576. doi: 10.3389/fmicb.2020.600576
Liu, Z., Bai, Y., Luo, L., Wan, J., Wang, W., and Zhao, G. (2021). Effects of high dose copper on plant growth and mineral nutrient (Zn, Fe, Mg, K, Ca) uptake in spinach. Environ. Sci. Pollut. Res. 28, 37471–37481. doi: 10.1007/s11356-021-13395-7
Marschner, P., Crowley, D., and Yang, C. H. (2004). Development of specific rhizosphere bacterial communities in relation to plant species, nutrition and soil type. Plant Soil 261, 199–208. doi: 10.1023/B:PLSO.0000035569.80747.c5
Msimbira, L. A., and Smith, D. L. (2020). The roles of plant growth promoting microbes in enhancing plant tolerance to acidity and alkalinity stresses. Front. Sustain. Food Syst. 4, 106. doi: 10.3389/fsufs.2020.00106
Mukherjee, P., Roychowdhury, R., and Roy, M. (2017). Phytoremediation potential of rhizobacterial isolates from Kans grass (Saccharum spontaneum) of fly ash ponds. Clean. Techn. Environ. Policy 19, 1373–1385. doi: 10.1007/s10098-017-1336-y
Murphy, J., and Riley, J. P. (1962). A modified single solution method for the determination of phosphate in natural waters. Anal. Chim. Acta 27, 31–36. doi: 10.1016/S0003-2670(00)88444-5
Nicolitch, O., Colin, Y., Turpault, M.-P., and Uroz, S. (2016). Soil type determines the distribution of nutrient mobilizing bacterial communities in the rhizosphere of beech trees. Soil Biol. Biochem. 103, 429–445. doi: 10.1016/j.soilbio.2016.09.018
Olsen, S. R. (1954). Estimation of Available Phosphorus in Soils by Extraction with Sodium Bicarbonate. Washington, DC: U.S. Department of Agriculture.
Oukaltouma, K., Moukhtari, A. E., Lahrizi, Y., Mouradi, M., Farissi, M., Willems, A., et al. (2021). Phosphorus deficiency enhances water deficit impact on some morphological and physiological traits in four faba bean (Vicia faba L.) varieties. Ital. J. Agron. 16. doi: 10.4081/ija.2020.1662
Patil, S., Nikam, M., Anokhina, T., Kochetkov, V., and Chaudhari, A. (2017). Multi-stress tolerant plant growth promoting Pseudomonas spp. MCC 3145 producing cytostatic and fungicidal pigment. Biocatal. Agric. Biotechnol. 10, 53–63. doi: 10.1016/j.bcab.2017.02.006
Petatán-Sagahón, I., Anducho-Reyes, M. A., and Silva-Rojas, H. V. (2011). Isolation of bacteria with antifungal activity against the phytopathogenic fungi Stenocarpella maydis and Stenocarpella macrospora. Int. J. Mol. Sci. 12, 5522–5537. doi: 10.3390/ijms12095522
Pikovskaya, R. I. (1948). Mobilization of phosphorus in soil in connection with vital activity of some microbial species. Mikrobiologiya 17, e370.
Rachid, D., and Ahmed, B. (2005). Effect of iron and growth inhibitors on siderophores production by Pseudomonas fluorescens. Afr. J. Biotechnol. 4, 697–702. doi: 10.5897/ajb2005.000-3129
Rana, A., Saharan, B., Nain, L., Prasanna, R., and Shivay, Y. S. (2012). Enhancing micronutrient uptake and yield of wheat through bacterial PGPR consortia. Soil Sci. Plant Nutr. 58, 573–582. doi: 10.1080/00380768.2012.716750
Rodríguez, I., Fraga, J., Noda, A. A., Mayet, M., Duarte, Y., Echevarria, E., et al. (2014). An alternative and rapid method for the extraction of nucleic acids from ixodid ticks by potassium acetate procedure. Braz. Arch. Biol. Technol. 57, 542–547. doi: 10.1590/S1516-8913201402005
Salazar-Ramírez, M. T., Sáenz-Mata, J., Preciado-Rangel, P., Fortis-Hernández, M., Rueda-Puente, E. O., Yescas-Coronado, P., et al. (2021). Plant growth-promoting rhizobacteria associated to candelilla rhizosphere (Euphorbia antisyphilitica) and its effects on Arabidopsis thaliana seedlings. Notulae Bot. Horti Agrobotan. Cluj-Napoca 49, 12294–12294. doi: 10.15835/nbha49212294
Shrestha, S., Brueck, H., and Asch, F. (2012). Chlorophyll index, photochemical reflectance index and chlorophyll fluorescence measurements of rice leaves supplied with different N levels. J. Photochem. Photobiol. B Biol. 113, 7–13. doi: 10.1016/j.jphotobiol.2012.04.008
Singh, N., Joshi, D., and Gupta, R. (2013). Isolation of phytase producing bacteria and optimization of phytase production parameters. Jundishapur J. Microbiol. 6, 6419. doi: 10.5812/jjm.6419
Singh, P., Singh, R. K., Li, H.-B., Guo, D.-J., Sharma, A., Lakshmanan, P., et al. (2021). Diazotrophic bacteria Pantoea dispersa and enterobacter asburiae promote sugarcane growth by inducing nitrogen uptake and defense-related gene expression. Front. Microbiol. 11, 600417. doi: 10.3389/fmicb.2020.600417
Stephan, R., Grim, C. J., Gopinath, G. R., Mammel, M. K., Sathyamoorthy, V., Trach, L. H., et al. (2014). Re-examination of the taxonomic status of Enterobacter helveticus, Enterobacter pulveris and Enterobacter turicensis as members of the genus Cronobacter and their reclassification in the genera Franconibacter gen. nov. and Siccibacter gen. nov. as Franconibacter helveticus comb. nov., Franconibacter pulveris comb. nov. and Siccibacter turicensis comb. nov., respectively. Int. J. Syst. Evol. Microbiol. 64, 3402–3410. doi: 10.1099/ijs.0.059832-0
Tabatabai, M. A., and Bremner, J. M. (1969). Use of p-nitrophenyl phosphate for assay of soil phosphatase activity. Soil Biol. Biochem. 1, 301–307. doi: 10.1016/0038-0717(69)90012-1
Talboys, P. J., Owen, D. W., Healey, J. R., Withers, P. J., and Jones, D. L. (2014). Auxin secretion by Bacillus amyloliquefaciens FZB42 both stimulates root exudation and limits phosphorus uptake in Triticum aestivum. BMC Plant Biol. 14, 51. doi: 10.1186/1471-2229-14-51
Tamura, K., Peterson, D., Peterson, N., Stecher, G., Nei, M., and Kumar, S. (2011). MEGA5: molecular evolutionary genetics analysis using maximum likelihood, evolutionary distance, and maximum parsimony methods. Mol. Biol. Evol. 28, 2731–2739. doi: 10.1093/molbev/msr121
Tendencia, E. A. (2004). “Disk diffusion method,” in Laboratory Manual of Standardized Methods for Antimicrobial Sensitivity Tests for Bacteria Isolated From Aquatic Animals and Environment (Washington, DC: Aquaculture Department, Southeast Asian Fisheries Development Center), 13–29.
Umesha, S. K., Singh, P., and Singh, R. (2018). “Chapter 6—Microbial Biotechnology and Sustainable Agriculture,” in Biotechnology for Sustainable Agriculture, eds R. L. Singh and S. Mondal (Philadelphia, PA: Woodhead Publishing), 185–205. doi: 10.1016/B978-0-12-812160-3.00006-4
Vance, C. P., Uhde-Stone, C., and Allan, D. L. (2003). Phosphorus acquisition and use: critical adaptations by plants for securing a nonrenewable resource. New Phytol. 157, 423–447. doi: 10.1046/j.1469-8137.2003.00695.x
Velho-Pereira, S., and Kamat, N. M. (2011). Antimicrobial screening of actinobacteria using a modified cross-streak method. Indian J. Pharm. Sci. 73, 223–228. doi: 10.4103/0250-474x.91566
Vincent, J. M. (1970). A Manual for the Practical Study of the Root-Nodule Bacteria. Available online at: https://www.cabdirect.org/cabdirect/abstract/19710700726 (accessed April 16, 2022).
Wieczorek, D., Zyszka-Haberecht, B., Kafka, A., and Lipok, J. (2022). Determination of phosphorus compounds in plant tissues: from colourimetry to advanced instrumental analytical chemistry. Plant Methods 18, 22. doi: 10.1186/s13007-022-00854-6
Xie, J., Knight, J. D., and Leggett, M. E. (2009). Comparison of media used to evaluate Rhizobium leguminosarum bivar viciae for phosphate-solubilizing ability. Can. J. Microbiol. 55, 910–915. doi: 10.1139/W09-034
Zhang, Y., Xu, Z., Li, J., Liu, D., Yuan, Y., Chen, Z., et al. (2019). Cooperation between two strains of Enterobacter and Klebsiella in the simultaneous nitrogen removal and phosphate accumulation processes. Bioresour. Technol. 291, 121854. doi: 10.1016/j.biortech.2019.121854
Zhao, Y., Shi, R., Bian, X., Zhou, C., Zhao, Y., Zhang, S., et al. (2019). Ammonia detection methods in photocatalytic and electrocatalytic experiments: how to improve the reliability of NH3 production rates? Adv. Sci. 6, 1802109. doi: 10.1002/advs.201802109
Zhou, G.-C., Wang, Y., Zhai, S., Ge, F., Liu, Z.-H., Dai, Y.-J., et al. (2013). Biodegradation of the neonicotinoid insecticide thiamethoxam by the nitrogen-fixing and plant-growth-promoting rhizobacterium Ensifer adhaerens strain TMX-23. Appl. Microbiol. Biotechnol. 97, 4065–4074. doi: 10.1007/s00253-012-4638-3
Keywords: rhizosphere, plant growth-promoting rhizobacteria, 16S rRNA, inoculation, phosphate deficient
Citation: Chamkhi I, Zwanzig J, Ibnyasser A, Cheto S, Geistlinger J, Saidi R, Zeroual Y, Kouisni L, Bargaz A and Ghoulam C (2023) Siccibacter colletis as a member of the plant growth-promoting rhizobacteria consortium to improve faba-bean growth and alleviate phosphorus deficiency stress. Front. Sustain. Food Syst. 7:1134809. doi: 10.3389/fsufs.2023.1134809
Received: 30 December 2022; Accepted: 20 February 2023;
Published: 16 March 2023.
Edited by:
Abdelilah Meddich, Cadi Ayyad University, MoroccoReviewed by:
Raja Ben-Laouane, Cadi Ayyad University, MoroccoMohamed Anli, Cadi Ayyad University, Morocco
Mohammed Mouradi, Université Sultan Moulay Slimane, Morocco
Copyright © 2023 Chamkhi, Zwanzig, Ibnyasser, Cheto, Geistlinger, Saidi, Zeroual, Kouisni, Bargaz and Ghoulam. This is an open-access article distributed under the terms of the Creative Commons Attribution License (CC BY). The use, distribution or reproduction in other forums is permitted, provided the original author(s) and the copyright owner(s) are credited and that the original publication in this journal is cited, in accordance with accepted academic practice. No use, distribution or reproduction is permitted which does not comply with these terms.
*Correspondence: Imane Chamkhi, imane.chamkhi@um6p.ma