- 1Food and Nutrition Sciences Laboratory, International Institute of Tropical Agriculture, Southern Africa Research and Administration Hub Campus, Lusaka, Zambia
- 2Food and Nutrition Sciences Laboratory, International Institute of Tropical Agriculture, Ibadan, Nigeria
- 3Cassava Breeding Unit, International Institute of Tropical Agriculture, Southern Africa Research and Administration Hub (SARAH) Campus, Lusaka, Zambia
- 4Food Science Laboratory, International Institute of Tropical Agriculture, Eastern Africa Hub, Dar es Salaam, Tanzania
Introduction: Cassava (Manihot esculanta) is becoming an important crop in most developing countries, especially Zambia. However, cassava roots deteriorate quickly after harvest, producing flour or chips of sub-optimal quality. Hence the evaluation of processing procedures to extend the product's shelf-life cannot be overemphasized. This study investigated the effects of different drying methods on the chemical, functional and pasting properties of selected advanced cassava clones grown in Zambia.
Methods: Fresh Roots of the seven cassava clones (harvested 12 months after planting) were sampled, peeled, washed and dried using the three drying methods (sun-drying, oven-drying and freeze-drying). The flour samples from these drying methods were analyzed using standard laboratory methods for primary chemical, functional and pasting properties. The data generated were statistically analyzed for descriptive, analysis of variance (ANOVA), and the means were separated at p < 0.05.
Results and discussion: The clone and drying method had significant effects (p < 0.001) on most chemical, functional and pasting properties. Clone 00/0093 had consistently lower ΔE* values for each drying method than other clones. The overall mean of total color difference (ΔE*) across the genotypes revealed the ranking ΔE* for drying techniques in the order of oven > sun > freeze drying. Generally, the highest sugar content was recorded for the freeze-dried samples. Similarly, the total starch content was higher in the sun-dried samples of six out of the seven samples than in the oven-dried samples. The functional properties ranged from 31.29–61.04%, 2.31–5.41%, 119.68–206.78% and 54–70 for solubility, swelling power, water absorption capacity and dispersibility, respectively.
Conclusion: In conclusion, the clonal effect of the cassava and drying method significantly affected the cassava flours' color, chemical, functional, and pasting properties.
1. Introduction
Cassava (Manihot esculenta) is one of the most important crop commodities globally, owing to its versatility in utilization. In 2017, the world cassava trade share was estimated at 15.7% from a total production of 278 million tons (FAO, 2017). Demand for cassava flour and starch, mainly in Africa's food markets, has been attributed to increased cassava production (Droppelmann et al., 2018). Cassava is believed to have originated from South American countries and was introduced to Africa around the eighteenth century by Portuguese traders (Uchechukwu-Agua et al., 2015). Despite being the largest producer (FAO, 2017) and having the fastest production growth rate of ~4% (Lukuyu et al., 2014), Africa lags in terms of trade share, with only Egypt occupying the top 10 list of cassava exporters (Otekunrin and Sawicka, 2019). This is because most cassava produced in Africa is for consumption, as reported in the FAO's food outlook report (FAO, 2017), where sub-Saharan Africa's per capita consumption is 105.1 kg/year compared to the world average of 20 kg/year. Various items, such as food, beer, textiles, paper, adhesives, chemicals, glucose, detergents, ethanol, cosmetic powders, pharmaceuticals, and pesticides, are produced using cassava flour and starch directly or as raw material. The processing suitability of cassava roots for authentic food and non-food applications is a function of their functional properties, which depend on their chemical, functional, and pasting properties.
In its traditional form, cassava roots have low nutritional value (Montagnac et al., 2009), and most cassava-consuming households have limited access to nutrient-dense supplementary foods, such as fruits and vegetables. The dietary guidelines (USDHHS, 2019) estimate that half of the adult population suffers from one or more preventable chronic diseases. Many of these diseases are a result of poor nutrition (Simon, 2014). The situation is the same for most adult populations worldwide and is exacerbated in resource-poor households, typical in cassava-consuming communities.
Cassava roots deteriorate rather quickly after harvest. Thus, they are often sun-dried or oven-dried to extend the shelf life to prevent postharvest physiological deterioration (PPD) that usually develops a few days after harvest. This deterioration usually leads to the production of flour or chips of sub-optimal quality. Processing fresh cassava roots into flour reduces bulkiness and losses and adds value (Dufour et al., 2002; Silayo et al., 2013; Alamu et al., 2017). Cassava roots are cut into small pieces to speed up the drying by increasing surface area (Udoro et al., 2013; Olowoyeye and Evbuomwan, 2014). Sun drying is the most prevalent method because it is the most straightforward and affordable for smallholder farmers. Sun-drying involves drying food by direct exposure to the sun's rays and causes water evaporation with the assistance of the surrounding air's movement (Charlotte, 1994). However, it is imperative to note that sun-drying is relatively slow because the absorbed solar radiation does not cause rapid moisture evaporation from the product compared with other drying techniques (Charlotte, 1994; Silayo et al., 2013). However, studies have been reported to improve the efficiency of sun-drying cassava roots (Charlotte, 1994; Silayo et al., 2013; Olowoyeye and Evbuomwan, 2014). These studies assess the efficiency of drying on different surfaces and drying depths. Efficient removal of moisture can be provided artificially by generating hot air. However, an expensive but fast artificial drying [such as oven (hot air) and freeze-drying] could also be used, provided the drying air temperature is maintained below appropriate temperatures. Hot air drying (convectional) is mainly affected by temperature, airflow, relative humidity, and surface area. Vacuum freeze-drying is a drying process for the long-term preservation of heat-sensitive food and other biological materials based on the phenomena of sublimation. It comprises three main stages: freezing, primary, and secondary (Udomkun et al., 2018). The ability of freeze-drying to protect the primary structure and minimize shrinkage is high. Most deterioration, microbiological reactions, and enzyme deactivation are terminated due to the process's absence of liquid water and the low temperature required (Udomkun et al., 2018). The final product from the freeze-drying process is satisfied with qualities such as nutritional properties, color, flavor, and aroma compared to conventional drying (Ceballos et al., 2012; Udomkun et al., 2018). It has been reported that processing affects cassava roots' nutritional value and quality through modification and losses in nutrients of high value and causes changes to the physicochemical properties (Yousif et al., 2012; Olowoyeye and Evbuomwan, 2014; Alamu et al., 2017; Ikeogu et al., 2017). Various processing methods are associated with modification and nutrient loss, with sun-drying having the most negative impact (Olowoyeye and Evbuomwan, 2014). The increased cassava utilization by the food and feed industries will depend on the suitable variety and effective drying processes that produce the appropriate quality of cassava flour and stimulate the demand for cassava products. Cassava flour is popular in the food industry because of its unique characteristics—clarity of appearance, low flavor overtones, and ideal viscosity (Alamu et al., 2017). Tamiru (2018) reported the influence of drying methods on flour quality and the cyanide content of cassava root from Ethiopia. However, he used only one variety and sun-drying and oven-drying methods, unlike this study which used a more significant number of elite genotypes and selected varieties. Another method employed was the freeze-drying system to dehydrate the cassava roots.
However, the stability of cassava roots' chemical, functional, and pasting properties, when different drying methods are employed to process the cassava root, has not been adequately investigated, especially for elite cassava clones and varieties grown in Zambia. There is a need to examine whether there is a significant quality difference between sun-dried, oven-dried, and freeze-dried cassava grown in Zambia, especially the elite clones (advanced and promising pipeline clones). Therefore, this study aimed to assess the impact of drying methods on the chemical, functional, and pasting properties of flours produced from cassava varieties from Zambia. There are many opportunities for cassava flour in the wheat flour market, which depends on the availability of good-quality cassava flour. The information from this study will be helpful for processors to know the appropriate drying techniques that will give quality cassava flours, especially for the Zambia market.
2. Materials and methods
2.1. The location
The experiment was conducted at the International Institute of Tropical Agriculture's (IITA) Kabangwe farm, located at 28°18′26.9′′E and 15°18′11.6′′S in Chongwe district, Lusaka province, in Zambia. The site is in the agroecological region II and receives an average rainfall of 800 to 1,000 mm per annum. The area gets unimodal rain with an average crop-growing period of 100 to 140 days. The soil texture at Kabangwe is sandy loam and slightly acidic (pH 5.7). The soil organic matter and total nitrogen are below critical values at 2.0 and 0.061 percent, respectively (Fenton et al., 2008; Biratu et al., 2018).
2.2. Materials and methods
Cassava genotypes were harvested from a Uniform Yield Trial at IITA's Kabangwe farm in Lusaka, Zambia, in the 2018/2019 season. Mature cassava roots were harvested 12 months after planting (MAP) from seven elite slightly yellow-fleshed clones (01/1235, 99/3575, 01/1551, 99/0395, PHN2K18, MM96/1757, and 00/0093). five plants were carefully selected from the middle rows for each clone, and the five roots (of different sizes) were sampled from pool roots according to the prescribed protocol (Rodriguez-Amaya and Kimura, 2004). Three representative roots for each clone were selected, washed, and peeled at the laboratory. The peeled samples were longitudinally cut into four sectors. Two diagonally opposite sectors were then re-selected, chopped into small cubes of approximately 0.5–1 cm3, and thoroughly mixed to achieve a homogeneous sample (Figure 1).
2.3. Drying methods
Samples were dried using three different methods.
i. Oven-drying
The BINDER™ model ED 400 drying chamber was preheated to a uniform 60°C for an hour. Then, samples were introduced into tassel bags—typically high-quality, unbleached paper bags free of chemicals and other contaminants. They are also breathable, allowing moisture to escape during the drying process. The samples were dried for 72 h for moisture content to reduce to 14%.
ii. Freeze-drying
Samples were lyophilized using the LABCONCO™ freeze dryer set at Vacuum: Hi MBars pressure, wait for the collector: 22°C, and Run Collector: −41°C for 48 cumulative hours. The LABCONCO™ freeze dryer was checked every 3 h until the moisture content of the samples was at 14%.
iii. Sun-drying
Samples were put in tassel bags and displayed in direct sunlight on a raised platform for three sunny days with temperatures ranging from 18 to 25°C and humidity ranging from 60 to 75%.
2.4. Sample milling
All samples were milled to 0.5 mm particle size with a Laboratory Mill 3100 (PERTEN Inc., 3200 Robbins Road Suite 100A Springfield, IL 62704). Before analysis, the milled sample was stored in the refrigerator in airtight ziplock bags. All samples were analyzed in duplicate within 1 week.
2.5. Color measurements (HunterLab color)
A PCE Instruments' COLOR METER model PCE-CSM 2 (Color Tec Associates, Inc., 28 Center Street, Clinton, NJ 08809) was used to determine the color variation of the dried samples using the three different drying methods described by Alamu et al. (2020). The L*, a*, and b* readings were recorded in an Excel sheet. The color meter operates on the Commission Internationale de l'Eclairage (CIE) L*, a*, b* color scheme. The maximum for L* is 100, representing a perfect reflecting diffuser, the value for the standard white paper. The positive a* value is red, the negative a* value is green, the positive b* value is yellow, and the negative b* indicates blueness. The total color difference, ΔE*, was calculated using the L*, a*, and b* values. The ΔE* is a single value considering the differences between the sample's and standard's L*, a*, and b*.
2.6. Fundamental chemical analysis: proximate (moisture, ash, fat, amylose, total starch, and sugar) composition determinations
2.6.1. Moisture content
The cassava flour's moisture content (MC) was determined by weighing 5 g of the cassava samples in a cleaned and pre-weighed moisture can and placed in an oven at 103°C for 16 h until the constant weight was obtained (AOAC, 2006). The % MC was estimated using the equation shown below:
Where
M2 = weight in (g) of dish, lid, and sample after drying
M1 = weight in (g) of dish, lid, and sample before drying
M0 = weight in (g) of dish and lid only.
2.6.2. Ash content
For ash content determination, 10 g of the sample was weighed into a previously weighed crucible and placed into a muffle furnace at 500°C for 4 h (Akoto et al., 2015), and % total ash was calculated as shown below:
2.6.3. Fat content
Fat content was determined by the conventional Soxhlet extraction method using n-hexane as the solvent, following the approved methods 920.87 described by AOAC (Rodriguez-Amaya and Kimura, 2004). The crude fat content was estimated using the equation below:
Weight of the can with the extracted oil = W3
Weight of the empty can = W2
Weight of the sample = W1
2.6.4. Protein content
The Kjeldahl method was used to estimate the total nitrogen content of the samples. The crude protein (%) was obtained using the following equation (AOAC, 2006).
2.6.5. Amylose content
Amylose content was determined using the method described by Onitilo et al. (2007). This spectrophotometric method is based on forming the deep blue-colored complex with iodine, the absorbance of which is read at 620 nm. The total amylose was calculated using the equation below:
2.6.6. Starch and sugar content
The starch and sugar contents of the samples were determined using the method described in detail by Onitilo et al. (2007). Approximately 0.02 g of the sample was weighed into centrifuge tubes, wetted with 1 ml of ethanol, and 2 ml of distilled water was added, followed by 10 ml of 95% ethanol. The mixture was vortexed and centrifuged at 2,000 rpm for 10 min. The supernatant was collected and used for a free sugar assay. An aliquot of 0.01 to 2 ml extract was used for the assay; 0.8 ml of distilled water with 0.5 ml of 5% phenol was mixed using a vortex mixer (Thomas Scientific, USA) with 2.5 ml of concentrated H2SO4, vortex, cooled, and read absorbance using a spectrophotometer (Thermo-Fisher Scientific, USA) at 490 nm.
At the same time, the residue was hydrolyzed using 7.5 ml perchloric acid to estimate the starch content. phenol–sulfuric acid was used for color development, and glucose standards were used for sugar determination after reading the absorbance of color developed at 490 nm using a spectrophotometer (Thermo-Fischer Scientific, USA). The sugar obtained after hydrolysis of the residue was converted to starch by multiplying by 0.9.
Calculation
where A = Absorbance of sample
I = Intercept of sample
D.F = Dilution factor (depends on aliquot taken for assay).
2.7. Functional properties determination
The swelling powers of the samples from the various drying methods were determined according to the method described by Appiah et al. (2011). In total, 1 g of the sample was weighed into 10 ml of distilled water in a 25-ml centrifuge tube. The mixture was heated at 85°C for 30 min while continuously stirring. The supernatant was decanted after centrifuging the sample for 15 min at 1,000 rpm. The sample's swelling capacity was calculated after recording the sediment weight. Percentage dispersibility was determined according to the method described by the American Association of Cereal Chemists (AACC) (American Association of Cereal Chemists, 2000) method. Water absorption capacity (WAC) was determined according to the method described by Akoto et al. (2015). A total of 1 g of the sample was weighed into a 25-ml centrifuge tube that contained 10 ml of distilled water and allowed to stand at room temperature for 1 h. The suspension was centrifuged at 500 rpm for 30 min. The volume of water on the sediment was measured, and the water absorbed was expressed as the percentage of water absorption based on the original weight of the sample (Appiah et al., 2011).
2.8. Pasting properties determination
The pasting properties of the samples were determined using the protocol described by Alamu et al. (2017). The Rapid Visco Analyzer (RVA, model 3C; Newport Scientific Pty Ltd, Sydney, NSW, Australia) was used. Peak viscosity, holding strength, breakdown, final viscosity, setback, peak time, and the pasting temperature were recorded using a computer (Thermocline for Windows Software; Newport Scientific; AOAC, 2006).
All chemicals used for the analysis were of analytical grade, and analyses were run in duplicates.
3. Statistical analysis
The data generated were analyzed for descriptive and analysis of variance (ANOVA) at a 95% significance level using XLSTAT statistical and data analysis solution, New York, USA, version 2022.3.2 (https://www.xlstat.com/en). The differences between means were tested using the Duncan multiple range test (P < 0.05, P < 0.01, and P < 0.001).
4. Results and discussions
Table 1 presents the mean squares from the analysis of variance (ANOVA) for color, proximate, functional, and pasting properties of flours produced from elite cassava clones using different drying methods. There was a significant effect (p < 0.005) of the clone, drying method, and clone-by-method interaction on all the color properties, including the total color difference (ΔE). The results suggest that the color changes in the cassava flour could be genetic and depend on the processing techniques, especially drying methods. Color is a critical trait in determining cassava flour's quality, and industries prefer white and odorless high-quality cassava flour. There were also highly significant (p < 0.01 and p < 0.001) effects of clone, drying method, and second-level interaction of clone-by-drying method on the proximate (except fat), functional, and pasting properties of the cassava flour samples studied. It implies that factors including genetic and drying processing techniques affect the quality of cassava flour, which in turn affects the value of the usage of the flour. This finding agrees with Tamiru (Tamiru, 2018) who reported that drying techniques had an impact on the quality of the flour of Ethiopian cassava root. However, he used only one variety and sun-drying and oven-drying methods, unlike this study which used a more significant number of elite genotypes and selected varieties. According to a few studies, processing alters cassava roots' nutritional content and quality, resulting in the loss of high-value nutrients and their physicochemical qualities (Yousif et al., 2012; Ikeogu et al., 2017). The appropriate cassava varieties and efficient drying techniques that produce flour of good quality could raise demand for cassava products. They will be necessary for the expanded use of cassava by the food and feed industries.
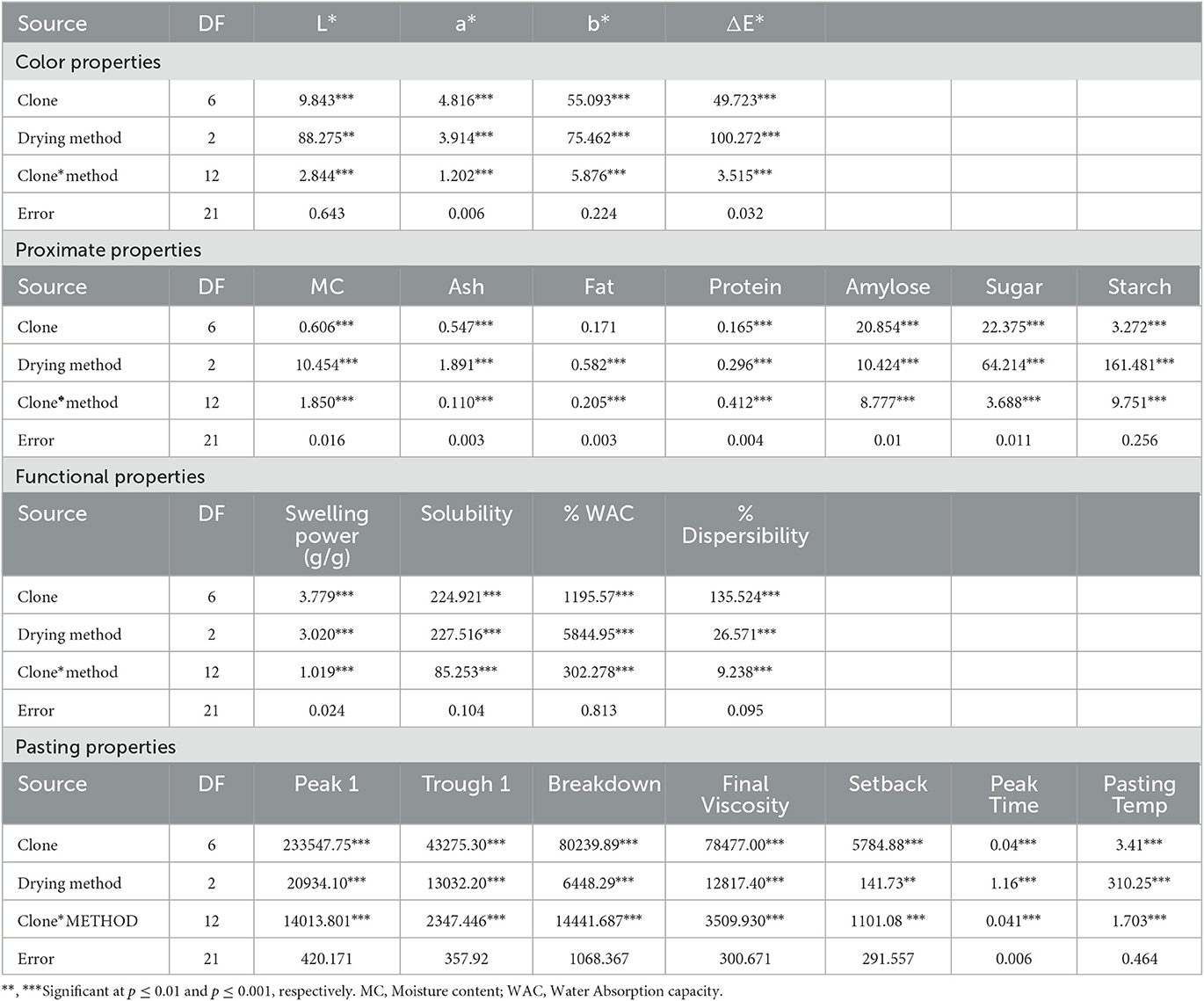
Table 1. Mean squares from the analysis of variance for color, proximate, functional, and pasting properties of flours produced from elite cassava clones using different drying methods.
4.1. Effect of the drying methods on the total color difference (ΔE*) of cassava flour samples
Table 2 shows ΔE*, calculated using the L*, a*, and b* values of the color scheme for the cassava flour from the three different drying methods studied. There was a significant effect of drying processes on the color property of the cassava clones. The ΔE* values of the oven-dried flours range between 16.85 ± 0.05 for 00/0093 and 22.08 ± 0.03 for PHN2K18. The sun-dried cassava samples had the ΔE* values range between 16.37 ± 0.01 (00/0093) and 24.78 ± 0.00 (MM96/1757), while the freeze-dried cassava flour samples had ΔE* values range between 20.56 ± 0.04 (00/0093) and 27.18 ± 0.14 (01/1235). Clone 00/0093 had consistently lower ΔE* values for each drying method than other clones. The overall mean of ΔE* across the genotypes revealed the ranking ΔE* for drying methods in the order of oven-drying > sun-drying > freeze-drying. This indicates that the most significant change in flour color among the tested clones occurred in the oven-dried samples. The best drying method with the lowest color change effect was the freeze-drying method, and this could be due to the drying carried out under low temperatures compared to sun- and oven-drying methods. Also, it could be that the ability of freeze-drying to protect the primary structure and minimize shrinkage is high (Udomkun et al., 2018). It has been reported that the final product from the freeze-drying process is satisfied with qualities such as color compared to conventional drying (Ceballos et al., 2012; Udomkun et al., 2018). However, regarding the color change effect, the sun-drying method is the next best way to freeze-drying. This implies that the sun-drying method could still be used as a sustainable, cost-effective, and simple drying method.
4.2. Effect of drying methods on chemical properties of cassava flour samples
The mean values of the chemical properties of cassava flour from the three methods are shown in Table 3. The clones' overall mean moisture content (MC) was as follows: freeze-drying > sun-drying > oven-drying. All samples showed MC below 10%, irrespective of the drying methods. The result implies that all samples may have a high shelf life because of the low MC. Moreover, the freeze-dried sample from 99/3575 had the highest (9.77 ± 0.01) MC, while an oven-dried sample from 99/3575 had the lowest (6.22 ± 0.02).
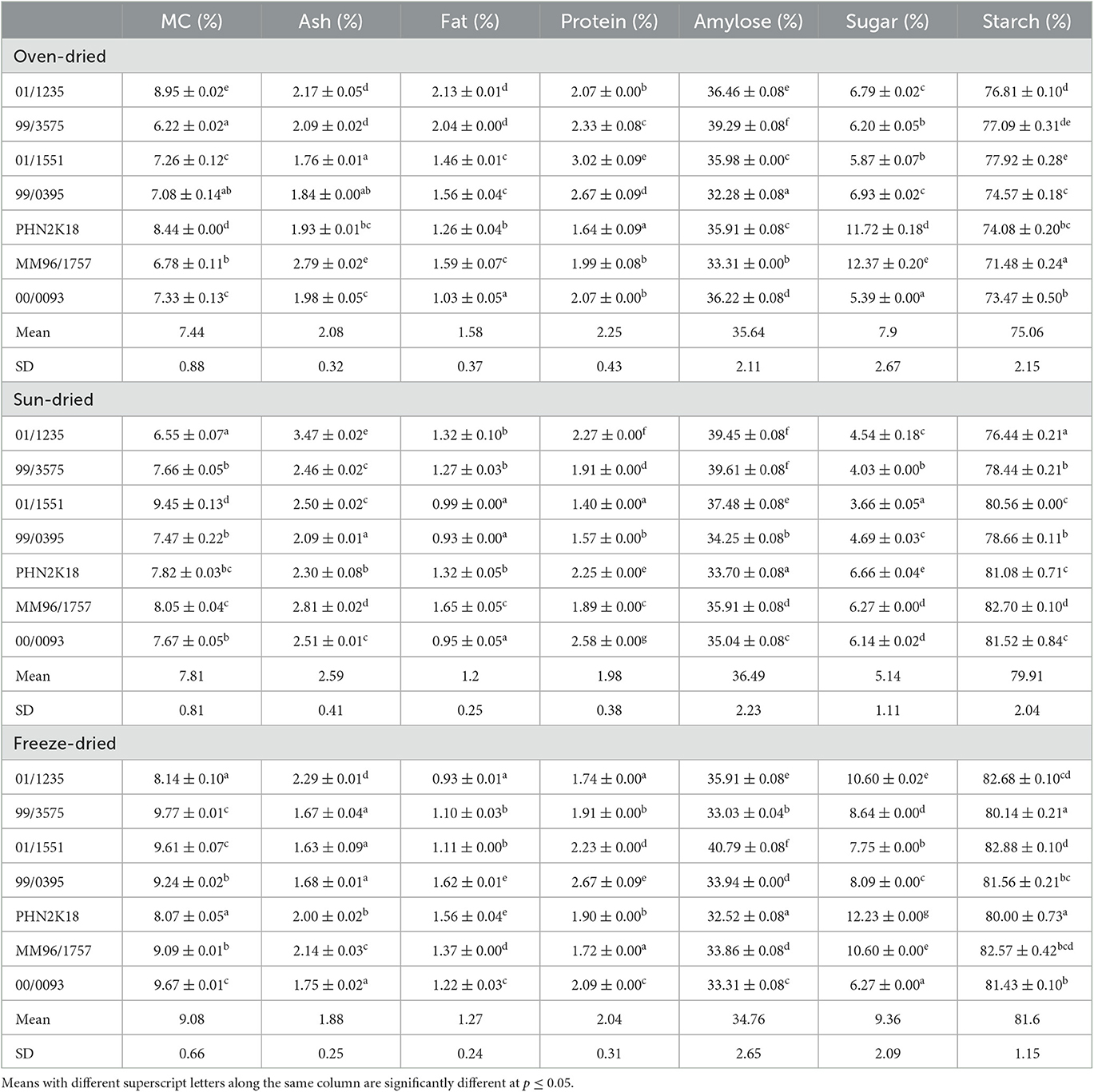
Table 3. Proximate properties of flours produced from elite cassava clones using different drying methods.
The ash content of the oven-dried samples ranged from 1.76 (01/1551) to 2.79% (MM96/1757) and that of sun-dried samples ranged from 2.09 to 3.47%. The highest values were for the clones 99/0395 and 01/1235, respectively, while the ash of freeze-dried samples was from 1.63 (01/1551) to 2.29% (01/1235). The freeze-drying method had the highest overall mean ash content, while the oven-drying method showed the lowest. Moreover, the fat content of the cassava flour samples from the three drying methods ranged from 1.03 to 2.13% (oven-dried), 0.93 to 1.65%(sun-dried), and 0.93 to 1.62 %(freeze-dried). The oven-drying method showed the highest mean fat content, but the sun-drying method had the lowest mean value. The low-fat content is expected for cassava as it is reported to be a low-fat content crop (Yousif et al., 2012). However, the oven-drying method had the highest protein mean value among the drying methods, while the sun-drying method showed the lowest.
Regarding the amylose content of the oven-dried samples, clone 99/0395 had the lowest (32.28%), and clone 99/3575 had the highest (39.29%). Among the sun-dried samples clone, PHN2K18 had amylose of 33.70% (lowest), and clone 99/3575 had 39.61% (highest), while for freeze-dried samples, clone PHN2K18 had 32.52% (lowest) and clone 01/1551 had 40.79 % (highest). The samples' moisture, ash, and starch contents fell within the acceptable limit of 12%, a maximum of 3%, and a minimum of 70%, respectively, as reported by Sanni et al. (2016) and Eke et al. (2010). The freeze-dried sample from 01/1552 had the lowest ash content and the highest starch value. It has been reported that the lower the ash content and MC, the better the quality of the cassava flour (Sanni et al., 2016; Alamu et al., 2017). The freeze-dried sample from clone 01/1552 was the best because of the high starch and low ash contents. Because freeze-drying can protect the primary structure and reduce shrinkage, high-quality samples from the process are anticipated.
4.3. Effect of the drying methods on functional properties of cassava flour samples
Table 4 shows the mean values of the functional properties of cassava flour samples subjected to different drying methods. The analysis of variance (ANOVA) showed that there was a highly significant (p < 0.001) effect of drying methods on the functional properties of cassava flour samples. The interaction of clones by the drying method was also found to have a highly significant (p < 0.001) effect among all the functional property parameters.
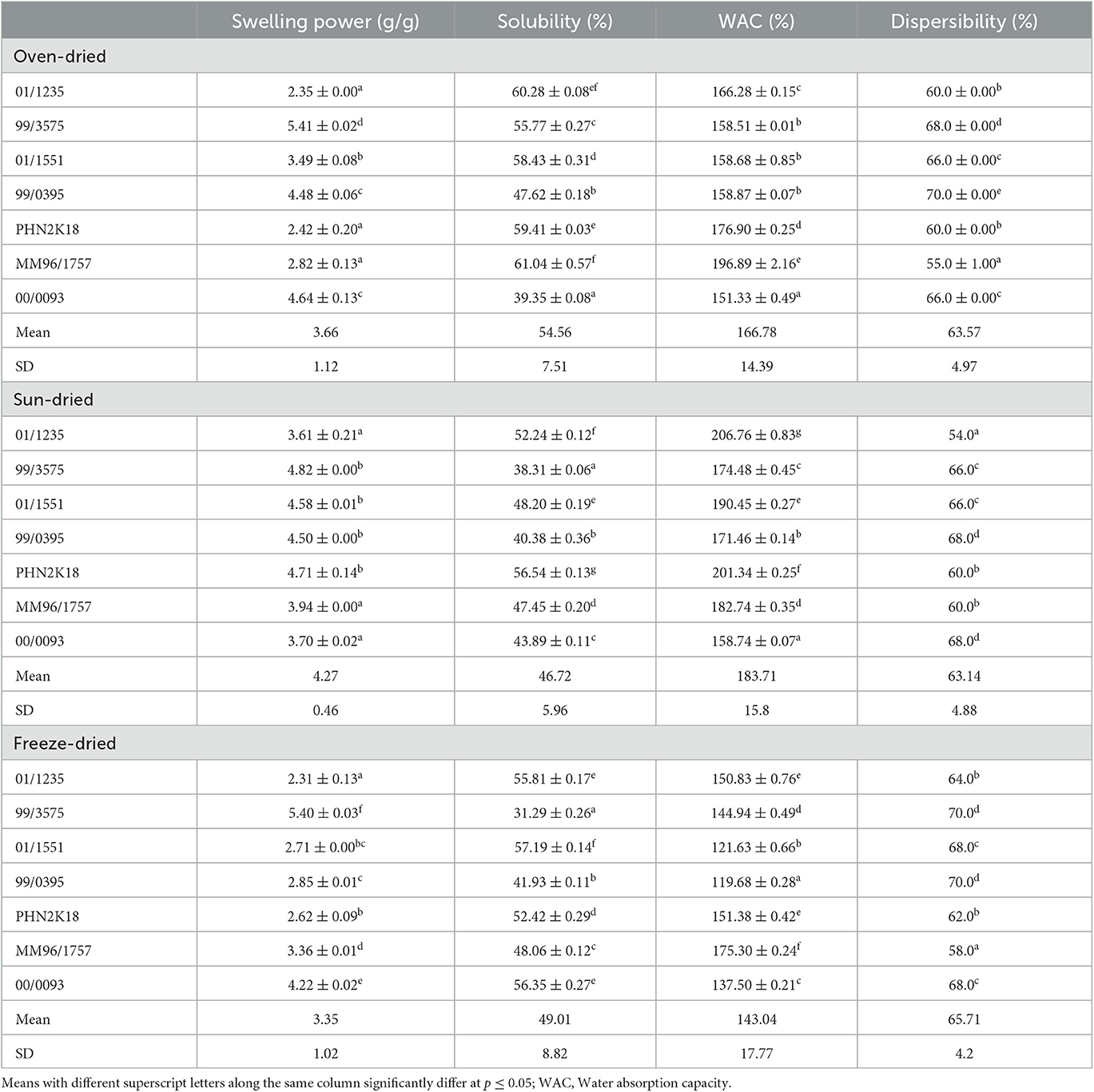
Table 4. Functional properties of flours produced from elite cassava clones using different drying methods.
The swelling power varied from 2.35 to 5.14%, 3.61 to 4.82%, and 2.31 to 5.40% in the oven-dried, sun-dried, and freeze-dried methods for 01/1235 and 99/3575, respectively. Clone 01/1235 showed comparatively lower swelling power values among the clones across the drying methods. The opposite was true for clone 99/3575, implying the varietal effect was stronger than the drying method effect on the swelling power functional property. Swelling power is influenced by the flour's amylose and amylopectin characteristics (Kusumayanti et al., 2015). Sasaki and Matsuki (1998) established a strong correlation between amylose content and swelling power. Therefore, the finding of this study may imply that clone 01/1235, with the least swelling power values, could have the highest amylose content among the observed clones, and the opposite is true for clone 99/3575. The solubility mean values were 54.56, 46.72, and 49.01% for oven-dried, sun-dried, and freeze-dried methods, as shown in Table 4. The highest solubility values were recorded in clones MM96/1757 (61.04%), PHN2K18 (56.54%), and 01/1551 (57.19%), whereas the least solubility values were observed in clones 00/0093 (39.35%) and 99/3575 (38.31% and 31.29%) for oven-dried, sun-dried, and freeze-dried methods, respectively. The clone 99/3575 exhibited negligible solubility for both sun-dried and freeze-dried methods, indicating low amylose and protein content (Kusumayanti et al., 2015).
The water absorption capacity (WAC) is a function of protein and starch content. It indicates the amount of water held by the starch extract. The mineral composition, such as phosphorous in starches, influences the water-binding capacity, where high starch content results in high binding capacity (Kacou et al., 2018). WAC ranged from 151.33 to 196.89%, 158.74 to 206.76%, and 119.68 to 175.30% for the oven-dried, sun-dried, and freeze-dried methods, respectively. Clone 99/3575 had the least WAC for oven-dried and freeze-dried methods, implying that starch and protein content may be low and that the varietal effect was more robust than the drying method. The degree of the starch association with water under conditions of limitation of water dough and pastes describes the WAC. This increased water absorption capacity for cassava flour samples obtained from oven-drying may also be due to ionizable esterified phosphate groups, resulting in mutual electrical repulsion, which weakens the association between starch molecules, increasing the water-binding capacity (EAC, 2012). Adeola et al. (2020) referred to dispersibility as a measure to which extent flour reconstitutes in water. Flour reconstitution in water is directly proportional to the dispersibility value. Clone 99/3575 had the highest dispersibility values for both oven and freeze-dried methods (70 %). The least values for the oven and freeze-dried methods were observed for the same clone MM96/1757 (58 %). Clones with higher dispersibility are easier to work with to achieve a uniform consistency because less energy is spent stirring to prevent product lump formation. Therefore, 99/3575 is a preferred clone from the dispersibility standpoint, regardless of which drying method was used.
The sun-dried samples had the highest swelling power and water absorption capacity values, followed by oven-dried samples. In contrast, the oven-dried samples had the highest solubility, followed by the sun-dried samples. However, freeze-dried samples had the lowest swelling power, solubility, and water absorption capacity. The higher swelling power and solubility values for sun-dried and oven-dried samples could be due to the micellar network's strength within the starch granules being a significant factor contributing to the swelling behavior of starch (Gujska et al., 1994). Thus, a highly associated starch with extensive strongly bonded micellar structure and character is readily resistant to swelling. This indicates that the increase in temperature for the oven-drying method results in a decrease in the strength and character of the micellar network. This is similar to Hoover's (Roca and Thro, 1993) observations concerning pinto and navy bean starch temperature. Roca and Thro (1993) also observed that swelling power and solubility are associated with the granules' binding force and degree of association. Thus, the relative increase in swelling power as the drying temperature increases suggests that starch structural damage is directly proportional to the increase in drying temperature. The increase in solubility as drying temperature increases may be associated with a higher granule swelling capacity that allows the exudation of amylose. The arrangement of the starch granule was assumed to influence the swelling behavior and invariably solubility. Therefore, the greater the structural damage, the easier the breakdown of starch. This may be because the high swelling power obtained with higher drying temperature weakened associated forces, increasing the swelling capacity as indicated by Olanipekun et al. (2009).
4.4. Effect of the drying methods on the pasting properties of cassava flour samples
Pasting qualities result from the heat-mediated transition of starch granules in excess water from a stable ordered state to an unordered state (Kusumayanti et al., 2015). Starch granules eventually rupture entirely due to the seepage of their molecular components. Swelling occurs due to the binding of additional water by starch granules. As a result, the starch granule's potential to bind water is reduced, leading to physical contact. As a result of these physical interactions, viscosity increases. The process is accelerated by heat, which enables water to be significantly ingested and aids in the melting of the starch granules' crystalline regions. Heat introduces the necessary energy for the water molecules in the starch granules to move quickly, upsetting the order through shearing action and resulting in random interactions among starch granules (Van Hung and Morita, 2005; Kumar and Khatkar, 2017).
Table 5 shows the effect of oven-dried, sun-dried, and freeze-dried methods on the pasting properties of seven cassava flour samples. Peak 1 viscosity ranged from 190.04 to 853.92 RVU for the cassava flours, with 01/1235 having the lowest value while clone 99/3575 was recorded as the highest for the oven-dried method, respectively. For the sun-dried method, peak 1 viscosity varied from 451.33 to 738.4 RVU, with clone 01/1235 having the lowest and clone 99/3575 with the highest value. Peak 1 viscosity for the freeze-dried method was observed in clone 01/1235, the lowest value, and 01/1551, the highest value. The trough viscosity varied from 10.38 to 198.71 RVU. The oven-dried method obtained the highest value in clone 99/0395 cassava flour. The sun-dried method's trough viscosity varied from 76.96 to 277.75 RVU (Table 5). The highest and lowest values were obtained in clone 99/0395 and PHN2K18 flour samples, while the freeze-dried method reviewed the range of 5.71 to 234.21 RVU of trough viscosity. The setback viscosity ranged from 138.34 to 1.33 RVU, with the clone 99/3575 having the highest value. The peak time varied from 3.24 to 3.57 min, with PHN2K18 having the lowest value and clone 99/0395 having the highest (Table 5). The peak temperature varied from 68.63 to 71.08°C, with flour samples from 99/3575 and 00/0093 varieties having the lowest temperature. In contrast, the 01/1235 clone had the highest temperature, as shown in Table 5 for the oven-dried method. The highest trough viscosity for sun-dried (237.80) and freeze-dried (234.21) methods were recorded in clones 99/3575 and 01/1551, respectively, while the respective least values (76.96 and 10.88) were observed in clone PHN2K18.
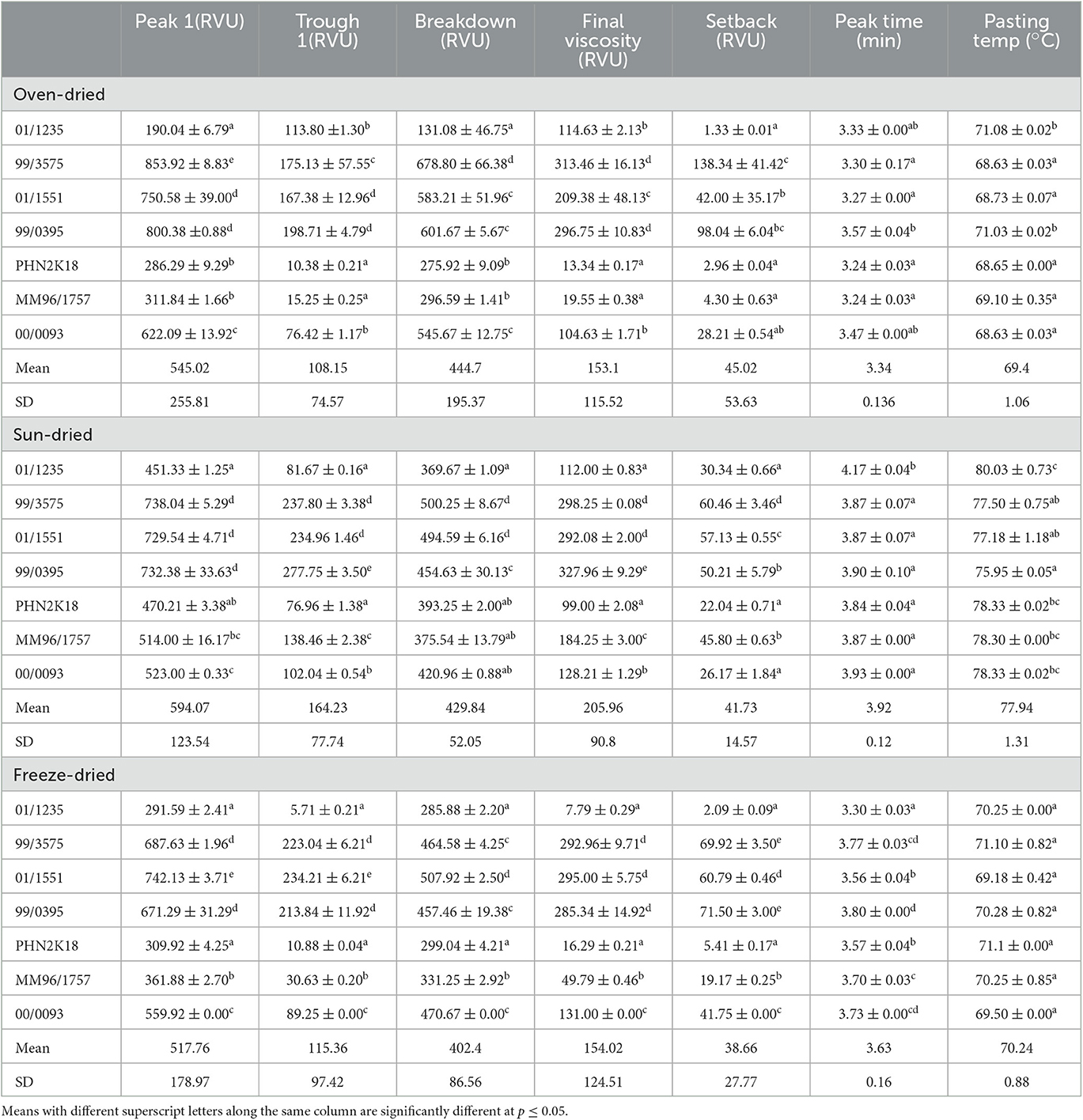
Table 5. Pasting properties of flours produced from elite cassava clones using different drying methods.
The sun-dried samples had the highest values for all the pasting properties except setback viscosity. However, oven-dried samples showed the lowest values for trough 1, final viscosity, peak time, and pasting temperature. The samples' pasting temperatures for oven-dried and freeze-dried fell within the acceptable limit of 75°C as reported by Adebowale et al. (2005) and Alamu et al. (2017) for high-quality cassava flour (HQCF) from white and yellow varieties, respectively. This implies that samples will form a paste with hot water below the boiling temperature, suggesting low cooking time and thus cost-saving. The oven-dried samples from clone 99/3575 showed superior Peak 1 (initial viscosity), breakdown viscosity, and setback viscosity than all other samples. However, the sample from 99/0395 using the sun-drying method had the highest Trough 1 and final viscosities values. However, the sun-dried 01/1235 and oven-dried 99/3576 samples had the highest (4.20 min) and the lowest (3.13 min) peak times, respectively. The sun-dried 01/1235 sample had the highest pasting temperature. Yousif et al. (2012) opined that gelatinization and pasting properties of flours are essential in the food industry because they influence the texture, uses, and digestibility of starch-based foods. For instance, the flour's high peak viscosity may suggest its suitability for products requiring high gel strength and elasticity. Sanni et al. (2016) defined peak viscosity as the ability of starches to swell freely before their physical breakdown, and this property is of the utmost importance in the food industry.
5. Conclusion
In summary, drying methods, clones, and the interaction of the drying methods and clones influenced the cassava samples' proximate composition. The flour properties' values differed significantly with the cassava clones, the drying method, and the interaction of cassava clones and the drying method. The variation due to clones and the drying method indicates that the clones and the drying method used for flour production affect its properties. Thus, clones and drying methods are essential for various uses of cassava flour. The freeze-drying method had the highest Δ E* values observed from the sample MM96/1757, making it the best sample for the best color properties. However, freeze-dried samples showed the highest MC, sugar, and starch contents among all the samples; oven-dried samples had the highest fat, protein, and amylose contents, indicating that the shelf life might be lower than the oven and sun-drying methods. The opposite was true for sun-dried samples that had the lowest values for all the chemical properties except amylose content. As the significant chemical property of cassava is starch, the freeze-drying method could be considered best for drying cassava for optimum starch retention. Thus, the freeze-dried sample from 01/1552 was the best because of the high starch and low ash contents. However, freeze-dried samples had the lowest swelling power, solubility, and water absorption capacity. The oven-dried samples from clone 99/3575 showed superior Peak 1 (initial viscosity), breakdown viscosity, and setback viscosity than all other samples.
Data availability statement
The raw data supporting the conclusions of this article will be made available by the authors, without undue reservation.
Author contributions
EA: conceptualization, formal analysis, and data curation. EA and NM: methodology, software, investigation, and writing—original draft preparation. PN, AA, and BM-D: validation, resources, supervision, and project administration. EA, NM, PN, AA, and BM-D: writing—review and editing. EA, PN, AA, and BM-D: visualization. AA and BM-D: funding acquisition. All authors have read and agreed to the published version of the manuscript.
Funding
This study was supported with funds from the CGIAR Research Program on Roots, Tubers, and Bananas (RTB) and the APC funded by the Bill & Melinda Gates Foundation (BMGF) OPP1019962.
Acknowledgments
The authors acknowledged the support from the CGIAR Research Program - Roots, Tubers and Bananas (CRP-RTB) and the Food and Nutrition Sciences Laboratory staff, especially Mr. Adesokan Michael.
Conflict of interest
The authors declare that the research was conducted in the absence of any commercial or financial relationships that could be construed as a potential conflict of interest.
Publisher's note
All claims expressed in this article are solely those of the authors and do not necessarily represent those of their affiliated organizations, or those of the publisher, the editors and the reviewers. Any product that may be evaluated in this article, or claim that may be made by its manufacturer, is not guaranteed or endorsed by the publisher.
References
Adebowale, A. A., Sanni, L. O., and Awonorin, S. O. (2005). Effect of texture modifiers on the physicochemical and sensory properties of dried fufu. Food Sci. Technol. Int. 11, 373–382. doi: 10.1177/1082013205058531
Adeola, A. A., Idowu, M. A., Oyatogun, R. M., Adebowale, A. A., Afolabi, W. A. O., and Adigbo, S. O. (2020). Quality of cassava flour as affected by age at harvest, cropping system and Clone. Agric. Trop. Subtrop. 53, 187–198. doi: 10.2478/ats-2020-0019
Akoto, O., Borquaye, L. S., Howard, A. S., and Konwuruk, N. (2015). Nutritional and mineral composition of the fruits of Solanum torvum from Ghana. Int. J. Mol. Sci. 4, 222–226.
Alamu, E. O., Maziya-Dixon, B., and Dixon, G. A. (2017). Evaluation of proximate composition and pasting properties of high-quality cassava flour (HQCF) from cassava genotypes (Manihot esculenta Crantz) of β-carotene-enriched roots. LWT Food Sci. Technol. 86, 501–506. doi: 10.1016/j.lwt.2017.08.040
Alamu, E. O., Maziya-Dixon, B., Menkir, A., Ogunlade, A. O., and Olaofe, O. (2020). Harvesting time and roasting effects on colour properties, xanthophylls, phytates, tannins and vitamin C contents of orange maize hybrid. Sci. Rep. 10, 21327. doi: 10.1038/s41598-020-78433-9
American Association of Cereal Chemists (2000). Approved Methods of Analysis, 10th Edn. St. Paul, MN: American Association of Cereal Chemists. Available online at: https://www.sciepub.com/ref/ (accessed August 16, 2021).
Appiah, F., Asibuo, J. Y., and Kumah, P. (2011). Physicochemical and functional properties of bean flours of three cowpea (Vigna unguiculata L. Walp) varieties in Ghana. Afr. J. Food Sci. 5, 100–104.
Biratu, G. K., Eyasu, E., Ntawuruhunga, P., and Nhamo, N. (2018). Effect of chicken manure application on cassava biomass and root yields in two agro-ecologies of Zambia. Agriculture 8. doi: 10.3390/agriculture8040045
Ceballos, A. M., Giraldo, G. I., and Orrego, C. E. (2012). Effect of freezing rate on quality parameters of freeze-dried soursop fruit pulp. J. Food Eng. 111, 360–365. doi: 10.1016/j.jfoodeng.2012.02.010
Charlotte, P. B. (1994). Home Drying of Food. Available online at: https://extension.usu.edu/preserve-the-harvest/research/drying-methods (accessed 06 August 2021).
Droppelmann, K., Günther, P., Kamm, F., Rippke, U., Voigt, C., and Walenda, B. (2018). Cassava, the 21st Century Crop for Smallholders? doi: 10.18452/19137
Dufour, D., O'Brien, G. M., and Best, R. (2002). Cassava Flour and Starch: Progress in Research and Development. Centro Internacional de Agricultura Tropical (CIAT), Départment des Systèmes Agroalimentaires et Ruraux, Cali.
EAC (2012). High Quality Cassava Flour-Specification East African Community. Available online at: http://www.eac-quality.net/fileadmin/eac_quality/ (accessed August 16, 2021).
Eke, J., Achinewhu, S. C., and Sanni, L. O. (2010). Functional properties of cassava tapioca grits. Int. J. Food Prop. 13, 427–440. doi: 10.1080/10942910802571737
FAO (2017). Biannual Report on Global Food Markets. Food outlook: Biannual Report on Global Food Markets.
Fenton, M., Albers, C., and Ketterings, Q. (2008). Soil organic matter agronomy fact sheet series. Agron. Fact Sheet Ser. 41, 1–2. doi: 10.1016/0378-4290(80)90012-X
Gujska, E., D.-Reinhard, W., and Khan, K. (1994). Physicochemical properties of field pea, pinto and navy bean starches. J. Food Sci. 59, 634–636. doi: 10.1111/j.1365-2621.1994.tb05580.x
Ikeogu, U. N., Davrieux, F., Dufour, D., Ceballos, H., Egesi, C. N., and Jannink, J. (2017). Rapid Analyses of Dry Matter Content and Carotenoids in Fresh Cassava Roots Using a Portable Visible and Near Infrared Spectrometer NextGen Cassava Breeding Project Workshop Report. Available online at: http://www.nextgencassava.org/meeting-2017/ (accessed August 16, 2021).
Kacou, M. A., Ekissi, E. G. S., Ebbah Djedji, B. C., N'zué, B., and Kouamé, P. L. (2018). Assessment of Functional Properties Flours from Seven Local Varieties of Cassava (Manihot esculenta Crantz) Consumed in Côte d'Ivoire'. Int. J. Curr. Microbiol. Appl. Sci. 7, 815–826. doi: 10.20546/ijcmas.2018.710.090
Kumar, R., and Khatkar, B. S. (2017). Thermal, pasting and morphological properties of starch granules of wheat (Triticum aestivum L.) varieties. J. Food Sci. Technol. 54, 2403–2410. doi: 10.1007/s13197-017-2681-x
Kusumayanti, H., Handayani, N. A., and Santosa, H. (2015). Swelling power and water solubility of cassava and sweet potatoes flour. Proc. Environ. Sci. 23, 164–167. doi: 10.1016/j.proenv.2015.01.025
Lukuyu, B., Okike, I., Duncan, A., Beveridge, M., and Blümmel, M. (2014). Use of Cassava in Livestock and Aquaculture Feeding Programs. Available online at: https://cgspace.cgiar.org/DiscussionPaper25/se (accessed August 16, 2021).
Montagnac, J. A., Davis, C. R., and Tanumihardjo, S. A. (2009). Nutritional value of cassava for use as a staple food and recent advances for improvement. Comp. Rev. Food Sci. Food Saf. 8, 181–194. doi: 10.1111/j.1541-4337.2009.00077.x
Olanipekun, B. F., Otunola, E. T., Adelakun, O. E., and Oyelade, O. J. (2009). Effect of fermentation with Rhizopus oligosporus on some physico-chemical properties of starch extracts from soybean flour. Food Chem. Toxicol. 47, 1401–1405. doi: 10.1016/j.fct.2009.02.023
Olowoyeye, I., and Evbuomwan, B. (2014). Comparative analysis of the effect of size reduction on the drying rate of cassava and plantain chips. Int. J. Geol. Agric. Environ. Sci. 2, 20–27.
Onitilo, M. O., Sanni, L. O., Daniel, I., Maziya-Dixon, B., et al. (2007). Physicochemical and functional properties of native starches from cassava varieties in southwest Nigeria. J. Food Agric. Environ. 5, 108–114.
Otekunrin, O., and Sawicka, B. (2019). Cassava, a 21st century staple crop: how can Nigeria harness its enormous trade potentials? Acta Sci. Agric. 3, 194–202. doi: 10.31080/asag.2019.03.0586
Roca, W. M., and Thro, A. M. (1993). Proceedings of the First International Scientific Meeting of the Cassava Biotechnology Network. Centro Internacional de Agricultura Tropical (CIAT).
Sanni, L. O., Maziya-Dixon, B., Alaya, Y., Okoro, G., Tarawali, J., Mkumbira, M., et al. (2016). Standards for Cassava Products and Guidelines for Export. International Institute of Tropical Agriculture (IITA). Standards Organization of Nigeria.
Sasaki, T., and Matsuki, J. (1998). Effect of wheat starch structure on swelling power. Cereal Chem. 75, 525–529. doi: 10.1094/CCHEM.1998.75.4.525
Silayo, V., Lazaro, E., Yustas, Y., and Laswai, H. (2013). Cassava sun-drying performance on various surfaces and drying bed depths. Tanzania J. Agric. Sci. 12, 31–36.
Simon, P. W. (2014). Progress toward increasing intake of dietary nutrients from vegetables and fruits: the case for a greater role for the horticultural sciences. Hortscience 49, 112–115. doi: 10.21273/hortsci.49.2.112
Tamiru, K. A. (2018). Influence of drying methods on flour quality and cyanide content of cassava root tuber. Int. J. Nutr. Food Sci. 7, 142–147. doi: 10.11648/j.ijnfs.20180704.15
Uchechukwu-Agua, A. D., Caleb, O. J., and Opara, U. L. (2015). Postharvest handling and storage of fresh cassava root and products: a review. Food Bioprocess Technol. 8, 729–748. doi: 10.1007/s11947-015-1478-z
Udomkun, P., Argyropoulos, D., Nagle, M., Mahayothee, B., Alamu, E. O., and Müller, J. (2018). Changes in microstructure and functional properties of papaya as affected by osmotic pre-treatment combined with freeze-drying. J. Food Meas. Charact. 12, 1028–1037. doi: 10.1007/s11694-018-9718-3
Udoro, E. O., Gbadamosi, O. S., and Taiwo, K. (2013). Studies on the production and utilization of dried cassava chips as human food. Int. Union Food Sci. Technol. 1, 1–8. doi: 10.4172/2157-7110.1000293
USDHHS (2019). Dietary Guidelines. U.S. Department of Health and Human Services, U.S. Department of Agriculture. Available online at: http://health.gov/dietaryguidelines/2015/guidelines/
Van Hung, P., and Morita, N. (2005). Effects of granule sizes on physicochemical properties of cross-linked and acetylated wheat starches. Starch 57, 413–420. doi: 10.1002/star.200500417
Keywords: cassava roots, drying methods, functional properties, pasting properties, freeze-drying
Citation: Alamu EO, Manda N, Ntawuruhunga P, Abass A and Maziya-Dixon B (2023) Elite cassava clones (Manihot esculenta) grown in Zambia: effects of drying techniques on their chemical, functional, and pasting properties. Front. Sustain. Food Syst. 7:1129779. doi: 10.3389/fsufs.2023.1129779
Received: 22 December 2022; Accepted: 17 May 2023;
Published: 15 June 2023.
Edited by:
Pankaj B. Pathare, Sultan Qaboos University, OmanReviewed by:
Stefano D'Amico, Austrian Agency for Health and Food Safety (AGES), AustriaEdy Sousa de Brito, Embrapa Alimentos e Territórios, Brazil
Copyright © 2023 Alamu, Manda, Ntawuruhunga, Abass and Maziya-Dixon. This is an open-access article distributed under the terms of the Creative Commons Attribution License (CC BY). The use, distribution or reproduction in other forums is permitted, provided the original author(s) and the copyright owner(s) are credited and that the original publication in this journal is cited, in accordance with accepted academic practice. No use, distribution or reproduction is permitted which does not comply with these terms.
*Correspondence: Emmanuel Oladeji Alamu, o.alamu@cgiar.org