- School of Food Science and Nutrition, University of Leeds, Leeds, United Kingdom
Food and nutrition insecurity are pressing global issues, pertinent to the United Nations’ Sustainable Development Goal 2 (zero hunger). About 1 in 10 people globally are food insecure, with both the COVID-19 pandemic and the Ukraine-Russia crisis exacerbating the problem, partly due to unprecedented shortages in major food commodities, such as wheat, rice, and sunflower oil. Food and nutrition security have been negatively impacted in sub-Saharan African countries like Nigeria and Uganda where, prior to both crises, 25% of the population were already food insecure and children under five and women of reproductive age faced severe undernutrition, micronutrient deficiencies, and their associated health challenges. The recent crises have highlighted the need for a paradigm shift from relying on a few crops to a diversified, sustainable food system that harnesses the potential of neglected and underutilized species (NUS) for food and nutrition insecurity solution, especially in low and middle-income countries. Despite their abundance (>100 edible species) and high nutritional value, various political, socio-cultural, and infrastructural factors have deterred commercialization and utilization of NUS in sub-Saharan Africa. Moreover, there are limited sophisticated studies on many of NUS local to the region. This review aimed to discuss selected NUS, peculiar to Nigeria, namely, African walnut (Tetracarpidium conophorium), African breadfruit (Treculia africana), and Uganda, namely, sesame (Sesamus indicum L.) and pigeon pea (Cajanus cajan L.), and their potential to sustainably contribute towards improved food and nutrition security. The crops are briefly described, and their indigenous uses, nutritional value and medicinal properties highlighted. Issues relating to their production, processing, consumption, and exportation are reviewed considering their contribution towards sustainable food systems.
1. Introduction
The global population is expected to reach approximately 10 billion by 2050, with much of this growth anticipated in sub-Saharan African countries, such as Nigeria, the Democratic Republic of Congo, Ethiopia, Tanzania, and Uganda (United Nations Department of Economic and Social Affairs, Population Division, 2022). Although some progress has been made in recent decades, a significant proportion of people living in sub-Saharan Africa are still hungry, food insecure, and face micronutrient deficiencies, commonly iron deficiency anemia and vitamin A and zinc deficiencies, presenting a huge global challenge (Baldermann et al., 2016; Li et al., 2020; Li and Siddique, 2020; FAO, IFAD, UNICEF, WFP and WHO, 2022). In these countries, malnutrition, and its negative implications on health, and quality of life, are even more pertinent among children under five, women of reproductive age, and pregnant and lactating mothers. Of the United Nations’ 17 Sustainable Development Goals (SDGs), SDG2 (zero hunger) aims to directly address these challenges by 2030, with SDG3 (good health and wellbeing), SDG12 (responsible production and consumption) and SDG15 (life on land), contributing to an enabling environment (Baldermann et al., 2016; Li et al., 2020; Li and Siddique, 2020). Various factors have contributed to global food and nutrition insecurity and malnutrition, including income inequalities, poor soil health, and more recently, climate change and conflict/war (FAO, IFAD, UNICEF, WFP and WHO, 2022). Moreover, the continued reliance globally, on a few (150–200) plant species has negatively impacted nutrition and health outcomes, by contributing to low individual and household dietary diversity, resulting in poor diet quality and inadequate macro and micronutrient intakes. In many sub-Saharan African countries, including some nations where the populations are expected to grow exponentially over the next 30 years, political unrest, the COVID-19 pandemic, and Russia-Ukraine war, negatively impacted global food supply chains and food production, consequently undermining food access, availability, and affordability.
Sustainable agriculture and food system transformation, which capitalize on “neglected and underutilized” species (NUS), are one way of tackling some of these challenges. This is being achieved by broadening the possibilities of what can be eaten as food, outside of the four key commercialized plant species (rice, wheat, maize, and potato) that supply 60% of human calorie and protein intakes (Biodiversity International, 2017; AARINENA, 2021). NUS, sometimes called “orphan crops”, “forgotten foods”, “minor crops” or “under-researched” foods (Gruere et al., 2006) are “wild, domesticated, or semi-domesticated plants, that have been cultivated previously for human and animal food, oil, and medicinal properties, but whose potential to improve livelihoods, food security, justice and sovereignty, has not been maximized by researchers, policy makers and local and global markets, due to their limited competitiveness with c crops commonly traded in mainstream agriculture” (Ulian et al., 2020, p. 422). NUS, although “unconventional”, contribute to incomes and livelihoods of farmers that cultivate them and often are an integral part of the cultural fabric and ethnic identities of people in the places from which they originate (AARINENA, 2021). NUS encompasses a diversity of food groups, including fruit and vegetables, oil seeds, pulses, cereals, roots, and tubers, and although underutilized, are often high in both macro and micronutrients and dietary fibre (Baldermann et al., 2016; AARINENA, 2021; Talabi et al., 2022). For example, moringa (Moringa olifera), quinoa (Chenopodium quinoa), and teff (Eragrostis tef), three NUS, can supply all eight essential amino acids, in addition to energy, minerals, vitamins and bioactives like polyphenols. Moreover, teff has been used in the production of alcoholic beverages and gluten-free baked goods, providing an alternative for people living with coeliac disease (Gebremariam et al., 2014). NUS also have medicinal properties, and the plant parts can yield other products, e.g., fodder, gums, resins, and building materials, which are of high economic value to various industries, e.g., animal industry, construction, pharmaceuticals (Ulian et al., 2020). It is no wonder that NUS have been called “crops of the future” (Baldermann et al., 2016). Moreover, many NUS are not resource-intensive, can grow on marginal lands, are tolerant to environmental stresses like drought, high salinity and can be easily intercropped or rotated with staples and trees (Li and Siddique, 2018, p. 3; AARINENA, 2021). Thus, NUS could contribute to sustainable food systems by enhancing biodiversity and strengthening ecosystem resilience (Padulosi et al., 2013; Shelef et al., 2017; FAO, 2019; Raneri et al., 2019; Ulian et al., 2020). Despite their myriad advantages, NUS are currently only marginally used by rural, smallholder farmers and indigenous communities. NUS have been largely excluded from agricultural and nutrition research, agricultural extension service delivery, and nutrition policy (Li and Siddique, 2018). A few NUS are currently traded in niche markets globally, e.g., quinoa, teff, amaranth, some pulses, partly due to government interventions, investments in research and innovation, and increased technology transfer between national and international partners. In sub-Saharan Africa, NUS utilization are limited due various reasons, including: (1) lack of organized value chains; (2) their perceived low competitiveness and economic potential; (3) limited value-addition know-how stemming from low prioritization in agricultural and food processing and innovation research; (4) ecosystems destruction for development leading to extinction of some species; and (5) the stigma attached to such crops as “food for the poor”, decreasing their popularity, particularly in a context where many sub-Saharan African countries are experiencing a nutrition transition (Akinnifesi et al., 2008; Naluwairo, 2011; Li et al., 2020). Notwithstanding, due to growing interest in healthy and sustainable food systems, NUS have recently gained traction as one of the parts of a multi-pronged approach towards addressing food and nutrition insecurity in emerging economies in Asia and sub-Saharan Africa. In 2018, the Food and Agricultural Organization of the United Nations (FAO) published a document titled “Future Smart Food”. Here, the authors, from an Asian perspective, highlighted examples of NUS as “future-smart foods” that warranted further exploration (FAO, 2019; Li et al., 2020; Li and Siddique, 2020, p. 4). In sub-Saharan Africa, on the other hand, a wider range of NUS remain underexplored (Gebremariam et al., 2014). To push the NUS agenda, the African Orphan Crops Consortium (AOCC), through a participatory exercise with farmers, scientists, development practitioners and producers, recently identified a list of 101 priority African plant species, important for alleviating malnutrition, supporting consumer diets and farmers’ incomes and livelihoods, such as okra, spider plant, Baobab, Mangosteen, finger millet, Locust bean, etc. (Hendre et al., 2019; Talabi et al., 2022). The crops were selected based on three primary criteria: (1) being rich in micro- and macro-nutrient contents; (2) being relevant to Africa; and (3) having a need for developing breeding resources (Hendre et al., 2019). To this end, this review aimed to evaluate the potential of selected NUS species in two sub-Saharan African countries, Nigeria, and Uganda, for improved food and nutrition security and livelihoods improvement, within a sustainability food system. Building on the work by AOCC, we identified four neglected and underutilized species in Nigeria, that is, African walnut (Tetracarpidium conophorium) and African breadfruit (Treculia Africana), and Uganda, that is sesame (Sesamus indicum L.) and pigeon pea (Cajanus cajan L.). The four species fall under three food categories, that is, roots and pulses (pigeon pea), nuts and seeds (sesame, African walnut), and fruit and vegetables (African breadfruit) and were chosen based on five criteria: (1) relevant to Africa; (2) resilient to environmental stress and ability to withstand effects of climate change, e.g., high salinity, drought, heat; (3) high nutritional value (macro and micro-nutrients); (4) potential for increased local and global utilization; and (5) locally available to allow for enhancement of local livelihoods.
2. Methodology
The search period for this review was from August 01, 2022, to November 30, 2022. The review focused primarily on the origins of the plants, the uses of the plant, nutritional composition, and potential for sustainable food systems. As such, search terms encompassing those concepts were combined with the common and/or botanical names of the crops, that is, pigeon pea, African breadfruit, African walnut, and sesame to yield simple search syntax, which were then inputted in two databases (Google Scholar, Scopus) for example: (i) for pigeon peas: ((“pigeon pea*” OR “Cajanas cajan”) AND (origin OR utilisation OR nutrition OR “nutri* value” OR “nutri* composition” OR “nutri* content” OR nutrition OR sustainability)); (ii) for sesame, syntax was: ((sesame) AND (origin OR utilisation OR nutrition OR “nutri* value” OR “nutri* composition” OR “nutri* content” OR nutrition OR sustainability)); (iii) for African walnut: ((“African walnut*” OR “Tetracarpidium conophorium”) AND (origin OR utilisation OR nutrition OR “nutri* value” OR “nutri* composition” OR “nutri* content” OR nutrition OR sustainability)); and (iv) for African breadfruit: ((“African breadfruit*” OR “Treculia africana”) AND (origin OR utilisation OR nutrition OR “nutri* value” OR “nutri* composition” OR “nutri* content” OR nutrition OR sustainability)). There was no restriction on publication year for articles included in this review.
3. Results and discussion
3.1. African walnut (Tetracarpidium conophorium Mull. Arg.)—Nigeria
3.1.1. Origin and utilization
African walnut plant (Tetracarpidium conophorium Mull. Arg.), synonymous with Pleukenetia conophora (Figures 1A–F) was originally classified by Hutchinson and Dalziel (1928a). It is not clear in literature about the specific country where the plant originated from, however, it is widely noted that the plant is indigenous to West Africa, in particular, Nigeria and Benin Republic, as well as Central Africa, mainly, Cameroon, Equatorial Guinea, and Democratic Republic of Congo (Hutchinson and Dalziel, 1928b; Burkill, 1985). Although they are found in Sierra Leone, it is believed that the nuts were transferred from Nigeria through migration during the slave trade era as there are no evidence of the plants in the neighboring countries around Sierra Leone, (Burkill, 1985). The fruit pods at early maturity are light greenish in colour while at late maturity, the colour turns to brownish black (Fasina and Ajibola, 1989; Nkwonta et al., 2016). Agro geography of the nut shows that it is grown in the coastal and lowland humid regions of Nigeria between 4° 15′ and 8° N of the equator (Asaolu, 2009; Nkwonta et al., 2021). The nuts are primarily consumed as snacks after processing by boiling or roasting (Nkwonta et al., 2013). However, the use in ethno botanical medicine such as management of chronic diseases (cancers, diabetes, cardiovascular conditions, hypertension etc.), and other health conditions including sexual dysfunctions, malaria, constipation, and dysentery etc., have been reported in different studies (Malu et al., 2009; Amanze et al., 2011; Onwuli et al., 2014; Akomolafe et al., 2015). Nevertheless, these pharmaceutical and medicinal assertions require more experimental testing and evaluation using sophisticated instruments as well as trial/intervention studies to substantiate the claims.
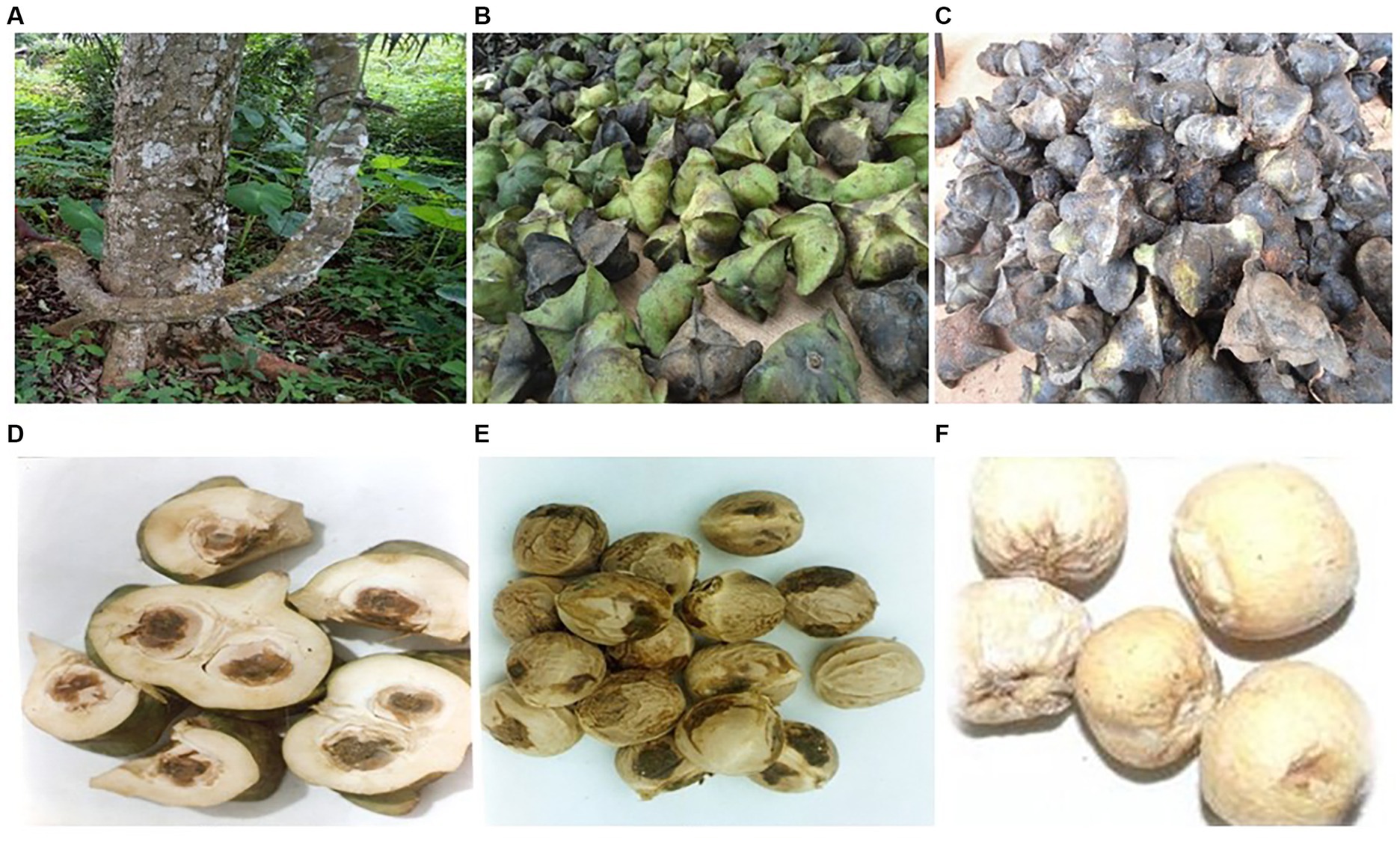
Figure 1. African walnut fruit and kernel. (A) African walnut climber curling around a host tree. (B) Fruit pods at early maturity (green colour). (C) Fruit pods at late maturity (brownish-black). (D) Fruit pods dissection exposing the nuts. (E) Nuts with shell. (F) Exposed edible kernel. Author (A–C) and Nkwonta et al. (2021) (D–F).
3.1.2. Nutritional composition and potential to improve nutrition and food security
African walnut kernels (Figures 1A–F) contains key nutritional characteristics (Table 1) considered essential in terms of tackling protein energy malnutrition, micronutrient deficiencies, maintaining omega-3/omega-6 fatty acid ratio and effecting antioxidation activities in biological systems. The nutritional composition (macro- and micro-nutrients) of the nuts has been reported in different studies. In particular, the protein content of both raw and processed nut samples ranges between 21.7% and 35.2%. It contains key vitamins (B1, B2, B3, B12, C, and E) with ascorbic acid—4.2 mg/100 g and tocopherol—0.123 mg/100 g being the most abundant (Ayoola et al., 2011). The oil content of raw, boiled, and processed samples (38%–49%) contain both linoleic and α-linolenic acids with the latter as the most abundant fatty acid in the oil (Nkwonta et al., 2015). Assessment of the protein content with respect to supporting growth and tissue maintenance highlights its potential use as alternative protein source in infant formulations. The fortification of wheat flour with African walnut flour for baking of various pastries (biscuits) indicated improved essential amino acid composition and lower microbial load of the final product when compared with the control (wheat flour biscuit only), even after storage of up to 8 weeks (Dauda et al., 2020). This is because African walnut flour is one of the oilseeds that contain all the essential amino acids necessary for various biochemical activities in man, particularly in protein synthesis (Tchiegang et al., 2001; Asaolu, 2009). The high concentrations of Vitamins C and E (Ayoola et al., 2011) as well as the presence of phytochemicals, including polyphenols belonging mainly to the classes of flavonoids and phenolic acids (Nkwonta et al., 2021) in the nut, effects antimicrobial and antioxidation on the products and in consumers.
Its potential for food security are embedded in the physicochemical characteristics of the plant and the nuts. African walnut plant is a perennial climber, which currently grows in the wild and utilizes other trees such as the cocoa and kola nut trees as support (Baiyeri and Olajide, 2022). As the plant is perennial, the fruits are produced annually between the months of June and September, thus ensuring the availability of the nuts on a yearly basis. The fruit pods contain two to five nuts with hard-brown shells encasing the edible kernels weighing about 4.50 g/kernel. Although the common method of consumption of the nut is as snacks, the primary food products generated from the kernels are the African walnut oil and the African walnut flour. These are valuable fundamental food forms that can be combined in various proportions with other condiments to produce nutrient-rich cooked or baked products such as pastries—biscuits, cookies, bread, etc. (Ndie et al., 2010; Awofadeju, 2020; Dauda et al., 2020; Awofadeju et al., 2021). Studies have reported favorably on the physicochemical characteristics of the African walnut flour, which has been applied in combination with wheat flour for baking of cookies, buns, and bread (Dauda et al., 2020). Additionally, the flour is used as a thickener in cooking various soups recipes. The large size of the nuts entails that a small harvest will potentially yield large amounts of oil and flour upon processing. This is of huge advantage in terms of ensuring sustainable abundance of the products if local farmers are only able to embark on subsistent farming because of limited availability of farmland. Conversely, the potential high yield of oil and flour from a small amount of the nuts underpins the need to domesticate the various accessions of the nut through germplasm garden and nursery development, study and select variants with higher potentials for high yield and disease resistance, and cultivate them using modern commercial precision farming system and technologies. Additionally, postharvest studies to improve storage time and conditions and ensure availability of the nut all year round are necessary.
3.2. African breadfruit (Treculia Africana)—Nigeria
3.2.1. Origin and utilization
African breadfruit (Figures 2A–C) belongs to the family Moraceae. The leaves are evergreen, and the tree can grow up to heights of 30 m. The tree bears a very large round greenish-yellow spongy-textured seeded fruit (Breadfruit), which can weigh 8.5–10 kg. The origin of the plant is also not clear in the available literature. Although the plant could be found throughout African regions, it is distributed mainly in the West and Central African regions in countries such as Nigeria, Benin Republic, Côte d’Ivoire, Cameroon, Central African Republic, Zambia, Senegal, Gabon, Tanzania, Angola, and Uganda (Ojimelukwe and Ugwuona, 2021; Hassler, 2022). The seeds are the main aspect of the fruit consumed in Nigeria. It is usually made into a porridge and consumed as a meal. Additionally, the seeds are roasted and eaten as snack with coconut or palm kernel seed. This snack is popularly known as “Aki n’Ukwa” in the Igbo-speaking states in Nigeria. Currently, there are four main varieties within the species, i.e., T. Africana Var. Africana, T. Africana Var. inversa, T. Africana Var molis and T Africana var. illcifolia. The differences are based on the size of the fruit head, hairiness of the branchlets and the leaves. The main varieties found in Nigeria are the T. Africana Var. inversa and T. Africana Var molis (Okafor, 1981; Ojimelukwe and Ugwuona, 2021; Hassler, 2022).
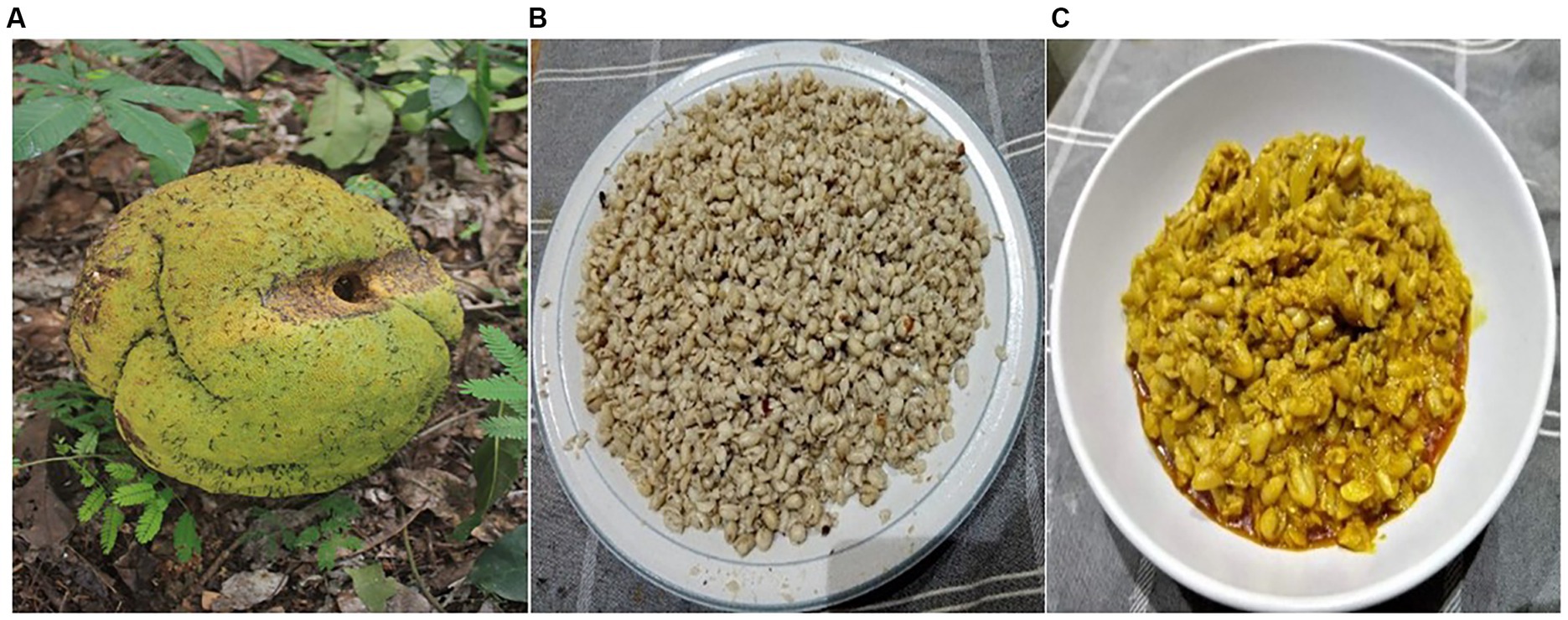
Figure 2. African Breadfruit: (A) Mature fruit. (B) Plane boiled seeds. (C) Porridge. IITA Forest Centre (https://www.flickr.com/photos/iita-media-library/8272252122/in/photostream/) used under CC by-NC license (A) and Author (B,C).
3.2.2. Nutrition composition and potential to improve nutrition and food security
Nutrient analysis of African breadfruit processed using variable methods have shown that the raw and processed seeds are rich in both macro- and micro-nutrients (Table 1). The crude protein content of raw, boiled, and roasted seeds range between 13.00% and 18.32%. Blending of African breadfruit with corn-starch normally utilized as a weanling food for children in Nigeria and other sub-Saharan African countries will help to improve the nutritive value of the food in terms of protein content. The African breadfruit seeds are rich in mineral contents, e.g., sodium, calcium, magnesium, zinc, phosphorus, copper, selenium, potassium (Table 1). It contains about 587 mg per 100 g dry matter serving of potassium (Osabor et al., 2009; Ojimelukwe and Ugwuona, 2021). This is far above the potassium content of bananas, which is about 330.6 mg per 100 g serving (Wall, 2006). Potassium is essential in maintaining blood pressure thus, consumption of African breadfruit meals contributes to towards reduction of the risk of cardiovascular disease. In ethnobotanical medicine, the consumption of the seed and root water extracts are normally recommended for the reduction blood glucose levels. The extracts have also been shown to improve liver functions as they decrease levels of liver function enzymes in test animals (Oyelola et al., 2007; Ojimelukwe and Ugwuona, 2021). The vitamin contents include mainly those in Vitamin B category (B1, B2, B3), Vitamin A and C (Table 1). Studies indicated that milk blend containing Soybeans milk and African breadfruit milk had an improved concentration of Vitamin A with increase in the African breadfruit (Okwunodulu et al., 2021). These vitamins and minerals are necessary for bone and teeth development/maintenance (calcium, potassium, and Vitamin A), tissue repair and antioxidant activities (Vitamin C, selenium). Additionally, the seeds contain certain phytochemicals such as polyphenols, tannins, saponins, and phytates. Some of these phytochemicals serve as antinutrients, which reduce the bioavailability of the micronutrients. However, different studies have reported the reduction of these antinutrients using different processing methods such as fermentation, roasting, cooking/boiling, dehulling, drying, soaking, and sprouting (Ijeh et al., 2010; Olapade and Umeonuorah, 2013; Okwunodulu et al., 2021). Further research is needed to understand the individual polyphenol profile of the seeds, develop adequate postharvest storage treatments to enhance the shelf life of the fruit/seeds while maintaining the nutritional qualities.
Like African walnut, the African Breadfruit is a perennial tree, which takes about 4 years to mature before yielding fruits. However, the productivity lifespan for each tree is above 50 years making it a viable economic tree for continuous long-term food supply. The fruits are produced annually guaranteeing availability of the seed for consumption or conversion to other products. Each fruit, depending on the size, could yield 5–7 kg of seed after processing. Apart from the porridge and roast seeds, other forms of food products can be formulated from the seeds. The seeds are ground into powder and used as flour for baking of different pastries (Giami, 2004). The milk is usually extracted after boiling and blending and can be consumed as plant-based milk or utilized in yoghurt formulation (Ifediba et al., 2018; Okwunodulu et al., 2021) while the oil can be obtained by pressing and are suitable for consumption as well as in soap and shampoo production (Ajiwe et al., 1995). The pulp though not often eaten, is utilized in the formulation of a non-alcoholic beverage (Ejiofor et al., 2012). Despite the diverse food forms obtainable from the seed limited research has been made to upscale the fruit farming, seed production and processing. The production and utilization of the seed is still at subsistence level in Nigeria. There is no food manufacturing company dedicated to exploitation of the potentials of African breadfruit. Currently, the tree is sparsely distributed across the countries where they grow in Africa, particularly in the southern parts of Nigeria. It is among the list of endangered species in southern Nigeria as it is threatened with extinction (Ohajianya and Osuafor, 2017).
3.3. Sustainability issues relating to production and utilization of African walnut and African breadfruit in Nigeria
African walnut plant has broad adaptability potentials. It thrives very well in tropical fertile moist and well-drained loamy soil. It adapts very well in woodlands, fallow fields, and forest areas (Baiyeri and Olajide, 2022). However, studies are yet to be conducted on it potential for growth and survival in temperate climate. Its ability to twine around host trees for support enables it to adapt among competing plants as it reaches the apex of trees to receive sunlight. The twining of the plant helps to join different treetop thereby enhancing forest canopy (Amusa et al., 2014). Additionally, the young plant can be staked until the roots and trunk are strong enough to bear the weight. Subsistence farmers in Nigeria, who use their back gardens or allotments to cultivate the plant, mainly apply this technique. In terms of fertilizer requirements, studies have shown that soil amendments such as poultry manure are sufficient to promote optimum growth and fruit production of African walnut. Application of 10 t ha−1 of poultry manure is sufficient to complement soil nutrients and enable proper development of morphological traits of the African walnut plant at early stages (Baiyeri and Olajide, 2022). This fertilization requirement supports green and sustainable cultivation of the African walnut plant and reduces the risk of inorganic chemical contamination of the soil and disruption of the soil ecosystem. Apart from the nuts kernel, the root, bark, and vegetative parts of the plant are also utilized in ethnobotanical medicine to treat various health conditions. Some of these conditions include malaria, eczema, indigestion, constipation, and diarrhea as well as manage chronic diseases such as diabetes, high blood pressure and cancers (Payal et al., 2015; Suara et al., 2016; Nkwonta et al., 2021). The utilization of the various parts of the African walnut underpins its potential as a high value and economic crop.
The African breadfruit plant grows well in swampy areas in forests, near streams and riverine areas. It also thrives well in medium loamy as well as heavy clay soils with pH being ranging from mildly acidic to neutral and mildly basic (pH 5.0–8.5). The plant is well adapted to tropical and sub-tropical climate however, research is needed to determine its growth and performance in temperate climate (Amujiri et al., 2018). In Nigeria, the tree could be planted in privately owned fields for subsistence, although not close to houses, as the fall of the mature fruit can cause damage to the roof of buildings (Ojimelukwe and Ugwuona, 2021). Apart from consumption of the seed, the plant is utilized in different forms. For example, in agroforestry, the trees serves as a conservation plant because the fall of the leaves serve as compost for the soil around it enabling a thriving ecosystem. Given the huge size of the tree, it serves as a windbreaker against storms and can also be used in flood control (Nuga and Ofodile, 2010; Ojimelukwe and Ugwuona, 2021). The tree’s wood is very suitable for pulp and papermaking as well as timber for other wood-based products. Given that, the lifespan of each tree exceeds 50 years and has a wide range of use in addition to producing fruits all year round which serves as food source, it usefulness as an economic resource for individual of family owners cannot be over-emphasized. Its management is substantially sustainable, as very little care is required to keep the tree productive (Nuga and Ofodile, 2010).
3.4. Factors affecting wider production and utilization of African walnut and African breadfruit in Nigeria
Within the communities where African walnut and African breadfruit are produced in Nigeria, they are well consumed in the most common forms as food when processed by boiling or roasting. They are consumed either as a meal such as the porridge or as snacks. The demands are also very high within these communities. For example, a study on the economics of household demand within an agricultural zone prominent in production of African breadfruit in Nigeria reported the demand rate as very high (63%) with 35% consuming it at least twice a week (Ohajianya and Osuafor, 2017). For example, a study on the economics of household demand within an agricultural zone prominent in production of African breadfruit in Nigeria reported the demand rate (63%) as very high with 35% of the residents consuming it at least twice a week (Ohajianya and Osuafor, 2017). Apart from the nuts kernel, the root, bark, and vegetative parts of African walnut are also utilized in ethnobotanical medicine to treat various health conditions. Some of these conditions include malaria, eczema, indigestion, constipation, and diarrhea as well as manage chronic diseases such as diabetes, high blood pressure and cancers (Payal et al., 2015; Suara et al., 2016; Nkwonta et al., 2021). For African breadfruit, the leaves and the bark are mixed in decoctions to treat various diseases including high blood pressure, diabetes, cough, oral thrush, helminthic infection, and gastro-intestinal complications, e.g., constipation (Oyelola et al., 2007; Kuete et al., 2008; Eleazu et al., 2017, 2018). The utilization of the various parts of the African walnut underpins its potential as a high value and economic crop. Nevertheless, beyond these producing regions, there is minimal demand, availability, and utilization. Several factor such as poor awareness of the general populace on the nutrition and health benefits, lack of government support to indigenous farmers, poor industry interest in exploiting the crops, urbanization, socio-economic transformations leading to shifts in dietary habits, and lack of financial investment/support for sophisticated scientific research all limit the wider production and utilization of these crops. With growth in population, gradual industrialization and need for housing to accommodate influx of employees in urban areas, farmlands are sold or hired out for construction of residential houses. Consequently, deforestation of the areas where these crops are already fully-grown leads to decline in production. Farmers in the rural areas, who cultivate these crops at subsistence level, often retire once they complete the training of their children whom they encourage to move to the urban cities to find jobs that are more lucrative. The continuous decline of existing elderly farmers with no replacement by younger ones inadvertently affects the rate of production of these crops. In terms of dietary shifts resulting from socio-economic and political dynamics, government policies encouraging importation of myriad of highly processed foreign food into the country has enabled acceleration of change in dietary habits of people living in both urban and rural areas in Nigeria. Several families switch to these foreign packaged diets (cereals, noodles, soups, etc.), as they are easy to stock/preserve, save time in preparation and very palatable particularly for the children. Furthermore, consumption of the foreign diets is often associated with upper and middle class families, as such; even the low-income earners strive to belong to the upper class through the switch in their dietary choices. Cumulatively, this preference of imported food over the local diets lowers the demand for production and utilization of local food such as the African breadfruit and African walnut. Finally, in Nigeria and in several other African countries, research findings are not considered as key drivers of development unlike what is obtainable in the western countries and other technologically advanced nations. Hence, the government invests minimally on scientific research activities particularly in the food and agricultural sector. Similarly, food industries invest less in research and development of the underutilized indigenous food crops. Even when independent researchers develop innovative/novel food products, there is lack of motivation and financial support from the government and industry stakeholders to scale-up production the new product through spinout companies. In other words, there is a lack of marketing systems for promoting NUS into large-scaled production. For instance, different scientific publications reporting on development of pastries such as cookies, bread, flour, cooking oil, etc., from African walnut and African breadfruit abound, however, these publications barely go beyond the papers due to lack of finances to move the product from the laboratories to the marketplace. A key challenge is the lack of modern fit-for-purpose infrastructure for industrial scale processing of these crops after harvest. Appropriate husking and nut-cracking machines are needed to facilitate quick large scale processing. Automated machines/systems are often lacking in the areas where these crops are produced as most of the farmers still apply traditional manual processes, thus reducing general production efficiency.
3.5. Recommendations on potential research, stakeholders and policy engagement to increase utilisation of African walnut and African breadfruit in Nigeria, African and globally
To minimize postharvest losses and improve shelf life and general utilization of the African walnut and African breadfruit seeds, comprehensive cold and retail storage experiments with the processed and unprocessed nuts sample with and without shells/bran are necessary. This will inform on best conditions for long and short-term storage necessary for local and export market. Studies on packaging of the nuts as snacks is also necessary given that this is the most popular mode of consumption. As the crops grow on a wide range of soil types, genetic studies following germplasm collections to select accessions that will be more tolerant to challenging climatic conditions will ensure optimum value is obtained from the crops. Reports have shown that these crops are threatened with extinction because of deforestation, socio-cultural shifts in dietary habits and urban migration (Baiyeri and Olajide, 2022). The government and industry stakeholders can therefore develop and promote agricultural land use policies that will favour preservation of farmlands as well as reward farmers efforts in terms of regulating competing imported crops and food products. Food scientists and researchers with viable ideas and intellectual properties for product development using these food sources as raw materials, should be incentivised to roll out spinout companies (micro and small businesses) and exploit further the reasonable utilization of the crops. Only a few studies have reported on the development of flour blends (Ndie et al., 2010; Awofadeju, 2020), bread and cookies (Dauda et al., 2020; Awofadeju et al., 2021) incorporating African walnut flour. More studies are needed to explore utilization of these blends in the culinary and baking industry as well as assess consumer views and acceptability through market analysis. Finally, promotion of African walnut and breadfruit as potential cash crops necessary to ensure food security in the face of challenging poverty and hunger, exacerbated by climate change factors such as flooding and drought, is essential at local, national and international levels. This can be done through funding of research focused on more sophisticated studies on the crop. The publication of the research findings will increase the information database and serve as authentic scientific evident backdrop to educate people.
3.6. Pigeon pea (Cajanus cajan L.)—Uganda
3.6.1. Origin and utilization
Cajanus cajan L. commonly known as pigeon pea, red gram, yellow dhal, no-eyed pea, Angola pea, Cajan pea, Congo pea, gungo pea, and tropical green pea (Raschke and Cheema, 2008; Fuller et al., 2019; Singh et al., 2020; Abebe, 2022), is a legume of the Fabaceae family and the Phaseoleae tribe, which contains many edible bean species, such as, soybean (Glycine max L.), common bean (Phaseolus vulgaris), cowpea (Vigna unguiculata L.) and Bambara nut (Vigna subterranea L.) (Dakora, 2000). Pigeon pea (Figures 3A,B), a perennial diploid shrub, typically grows 1–4 m high and yields a harvest between 6 and 11 months, although some recent varieties can mature in 3–4 months (Odeny, 2007; Kingwell-Banham and Fuller, 2014; Fuller et al., 2019; Namuyiga et al., 2022). Pigeon pea seeds come in various colors (Figure 3B), such as, black, orange, cream, and are bitter or sweet, depending on variety (Upadhyaya et al., 2005). Although growing in popularity recently, pigeon peas remain one of the most underutilized pulses globally, with production in 2021 estimated at nearly 6 million tons, accounting for an estimated 5% of the total global legume production (Hillocks et al., 2000; FAOSTAT, 2022). While India and Myanmar are the largest global producers of pigeon peas to-date, the crop is cultivated by smallholders in a few other countries in the Global South including, Nepal, Kenya, Malawi, and Uganda (Simtowe et al., 2010; FAO, 2019; Abebe, 2022; Namuyiga et al., 2022; Rizvi et al., 2022). The origin of the pigeon pea has been widely debated. Some evidence suggests that Cajanus cajanifolius, the wild progenitor of the domesticated plant, first appeared in parts of Peninsula India (Fuller et al., 2019), from where it was introduced to Africa from India about 4,000 years ago (Simtowe et al., 2010; FAO, 2019). Other scholars have argued the crop to be of African origin, being traced to 2,200–2,400 BC Egypt, from where it was transported to India (Morton et al., 1982). Regardless of origin, what is clear is the versatility of pigeon peas, being eaten as human food and used as medicine, animal fodder, fuel, building and craft-making material, in addition to playing a role in soil enrichment through mulching, nitrogen fixation and soil binding (Upadhyaya et al., 2010; Yang et al., 2020; Abebe, 2022; Namuyiga et al., 2022), highlighting the crop’s immense value for the resource-poor.
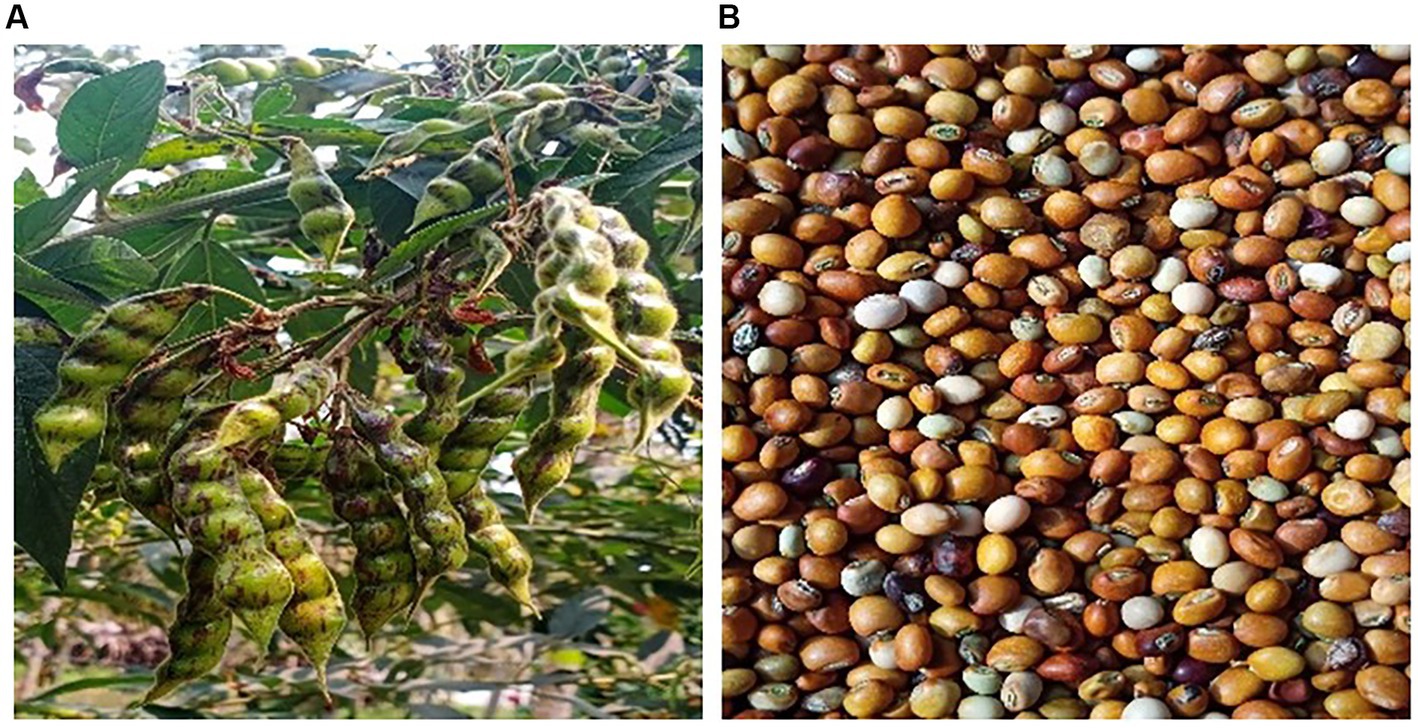
Figure 3. Pigeon pea (A) plant showing pods containing immature seeds, (B) mature dry seeds of varying colours. Harish N at www.pixahive.com (A) and Kundan Kumar at www.pixahive.com. Images used under CCO License (Free to use, Attribution optional).
3.6.2. Nutrition composition and potential to contribute to nutrition and food security
In Uganda, pigeon pea is an important crop which is widely consumed in the diet among smallholders and resource-constrained households, particularly in the Northern and North-Eastern parts of the country, two semi-arid regions that experience only one rainy season annually and show some of the highest rates for food insecurity (Obuo et al., 2004; Manyasa et al., 2009; Namuyiga et al., 2022). Here, pigeon pea seeds are locally known as “lapena” and are eaten when they are fresh or dry and mature, in the form of whole seed sauce or split pea sauce locally called “dek ngor” or “agira”. The green and tender immature seeds and pods, typically harvested 24–30 days after flowering, are sometimes eaten as vegetables, especially during the lean season (Simtowe et al., 2010; Upadhyaya et al., 2010; Kingwell-Banham and Fuller, 2014; FAO, 2019). Pigeon pea is an important food security crop in these areas owing to the that fact that it is not consumed fresh and minimally processed, but can also be dried, stored for long periods, and consumed in the lean season when many other less hardy crops cannot be cultivated. Pigeon pea seeds supply both macro and micronutrients (Table 2) highlighting their relevance for household food and nutrition security, particularly important among low-resource households that might not be able to afford more expensive sources of nutrition, e.g., animal-source foods. Both the immature and mature seeds are low in fat, with palmitic and linoleic acids being the predominant saturated and polyunsaturated fatty acid, respectively (Ade-Omowaye et al., 2015; Abebe, 2022). Pigeon pea seeds also contain moderately high amounts of carbohydrate (Table 2), a significant proportion of which is starch and soluble and insoluble dietary fiber, both of which have been found to play a major role in reducing risk for cardiovascular disease, type 2 diabetes, and some cancers, in addition to reducing body weight, blood pressure, LDL cholesterol and inflammation (Brown et al., 1999; Evans, 2020; Reynolds et al., 2020). Some of this could be due to the carbohydrate in pigeon peas showing a low glycemic index (7.0 ± 1.0 to 54.5 ± 0.6), which attenuates the glycemic response (Singh et al., 2021), or the fact that dietary fibre is a source of microbiologically accessible carbohydrates, which can be fermented by colonic bacteria producing short-chain fatty acids, that confer many health benefits.
Pigeon peas also contains high levels of protein (up to 24%) (Table 2), which are in the form of sulphur and non-sulphur amino acids, that is, lysine, leucine, isoleucine, threonine, histidine, and tryptophan (Fuller et al., 2019; Anitha et al., 2019a), which are limiting amino acids in other legumes, cereals and grains commonly consumed in Uganda, such as, maize, highlighting the role that pigeon pea could play in the complementarity of plant-based diets that are largely consumed in rural Uganda, where the plant is grown (Auma et al., 2019, 2021). Akporhonor et al. (2006) argue that combining pigeon peas with cereals can provide a diet with an essential amino acid profile comparable other crops of high protein value, such as, soybean. In fact, a recent study in Myanmar in which pigeon pea and millet were added to the diets of children 6–23 months, found improved intake of all nine essential amino acids, vitamin C, carotenoids, iron, and magnesium, resulting in significant reductions in proportions of wasted, stunted and underweight children (Anitha et al., 2019b). Moreover, Tapal et al. (2019) demonstrated the high digestibility of protein isolate from pigeon pea milling waste, owing to the high yield of essential amino acids and bioactive peptides, highlighting its potential as an ingredient in the manufacture of functional foods, that can be used to improve health. It’s no wonder that pigeon pea has been called the “poor man’s meat”, owing to the high protein content (Fuller et al., 2019), although amounts will vary depending on seed maturity and variety.
Pigeon pea seeds generally contain good amounts of some B vitamins (Table 2), which are usually deficient in many cereals and grains, characteristic of the Ugandan food basket. The mature seeds are high in minerals that play various roles in the body, including, bone health (calcium, magnesium), blood pressure control (potassium, magnesium) and blood glucose control (magnesium) (Table 2). In their paper, Susmitha et al. (2022) highlighted the potential for pigeon pea seed coat as a material for the manufacture of pharmaceuticals, such as supplements due to high calcium and magnesium content. In addition to macro and micronutrients, pigeon pea also contains many phenolic compounds, such as, flavonoids and stilbenes, that are protective to human health, via their antioxidant and anti-carcinogenic properties and modulating of the gut microbiota (Nix et al., 2015; Talari and Shakappa, 2018; Abebe, 2022). Pigeon peas contain on average about 27 flavonoids, e.g., apigenin, orientin and vitexin (leaves), isoquercitin (pods), and genistein and cajanol (stem and root) (Nix et al., 2015). Pigeon pea leaves show the highest polyphenolic content, compared with pods, stems and roots (Nix et al., 2015), and the white-seeded varieties reportedly contain about a third of the amount in red-seeded varieties (Abebe, 2022). The high phenolic content, in addition to the unique nutritional profile of pigeon pea, could explain why pigeon pea leaf, stem root extracts, as well as seeds, have been found to have cholesterol-lowering and has traditionally been used to manage various conditions, such, diabetes and hepatitis (Kumar et al., 2021; Wei et al., 2022). While pigeon peas are nutrient-dense, the presence of anti-nutritional factors, i.e., phytic acid, tannins, and trypsin inhibitors, can limit protein bioavailability by inhibiting trypsin enzyme, and bioavailability of iron, calcium, zinc, by binding to minerals (Rizvi et al., 2022). Anti-nutritional factors can be reduced or eliminated through mechanisms like soaking, pressure cooking, and germination (Embaby, 2010; Kajihausa et al., 2014), all of which are effective to varying levels. For example, Rizvi et al. (2022) demonstrated that flour from pigeon peas germinated for 72 h, showed an increase in protein content, antioxidant activity, metal chelating activity, and an overall increase in protein digestibility and iron and zinc bioavailability. The authors attributed these improvements to tannins and phytate content decreasing by 60%, and a reduction in the amount of trypsin inhibitor (Rizvi et al., 2022).
3.7. Sesame (Sesamum indicum L.)—Uganda
3.7.1. Origins and utilization
Sesamum indicum L., commonly known as sesame, is an oilseed legume belonging to the order Tubiflorae and the family Pedaliaceae (Bedigian, 1985; Bhat et al., 2014; Wei et al., 2022). Sesame, an herbaceous annual, largely cultivated for its mildly aromatic edible oil that adds a distinct flavor to food, is most likely the first crop cultivated purposely for its oil, having been grown over 6,000 years ago (Namiki, 2007; Bhat et al., 2014; Pathak et al., 2020; Wei et al., 2022). Although “sesame” is the commonly used name, other names used are gingelly, til, benne seed and in Uganda, “simsim”. Sesame (Figures 4A–D) grows on average 60–150 cm tall, with hairy, rectangular, or ovate-shaped leaves that are about 3–10 cm long and 2.5–4 cm wide (Wei et al., 2022). As sesame loves warm climates with little rain, it is mostly produced in tropical and sub-tropical Asia and African countries, including India. In sub-Saharan Africa, Sierra Leone, Sudan, Nigeria, and Uganda have been previously recognized as the leading producers (Namiki, 1995; Idowu et al., 2021), although recent data suggests that Tanzania has now replaced India as the leading global producer (Namiki, 2007; Wei et al., 2022). As of 2020, sesame production stood at approximately 6.8 million tonnes globally, with 146,000 tonnes being produced in Uganda, where in addition to the domesticated, cultivated variety, two wild species exist: Sesamum angolense and Sesamum angustifolium (Wacal et al., 2021; FAOSTAT, 2022). Although sesame seed oil is more economically valuable, production still trails other oil seeds, e.g., soybean, peanuts, rapeseed (Namiki, 2007). Like pigeon peas, sesame seeds come in various sizes and colors, such as, white, black, and brown, however, it is the black and white species are more commonly grown, because the black variety is hardier, being resistant to lodging and water stress and is believed to have higher medicinal value (Namiki, 2007; Wei et al., 2022). The exact origin of sesame is highly debated as the birthplace of Sesamum indicure L., the wild progenitor, has not been established with certainty (Bedigian, 1985). Some authors propose that sesame originated in India, a sub-continent that still has a diverse germplasm for the plant (Bhat et al., 2014; Pathak et al., 2020). However, other authors purport the origin of sesame to be in central Africa, from which it spread to Egypt, the Middle East, China, and beyond (Namiki, 2007). In countries where it is cultivated and consumed, sesame has various applications, including human food, animal feed, cosmetics, and pharmaceuticals.
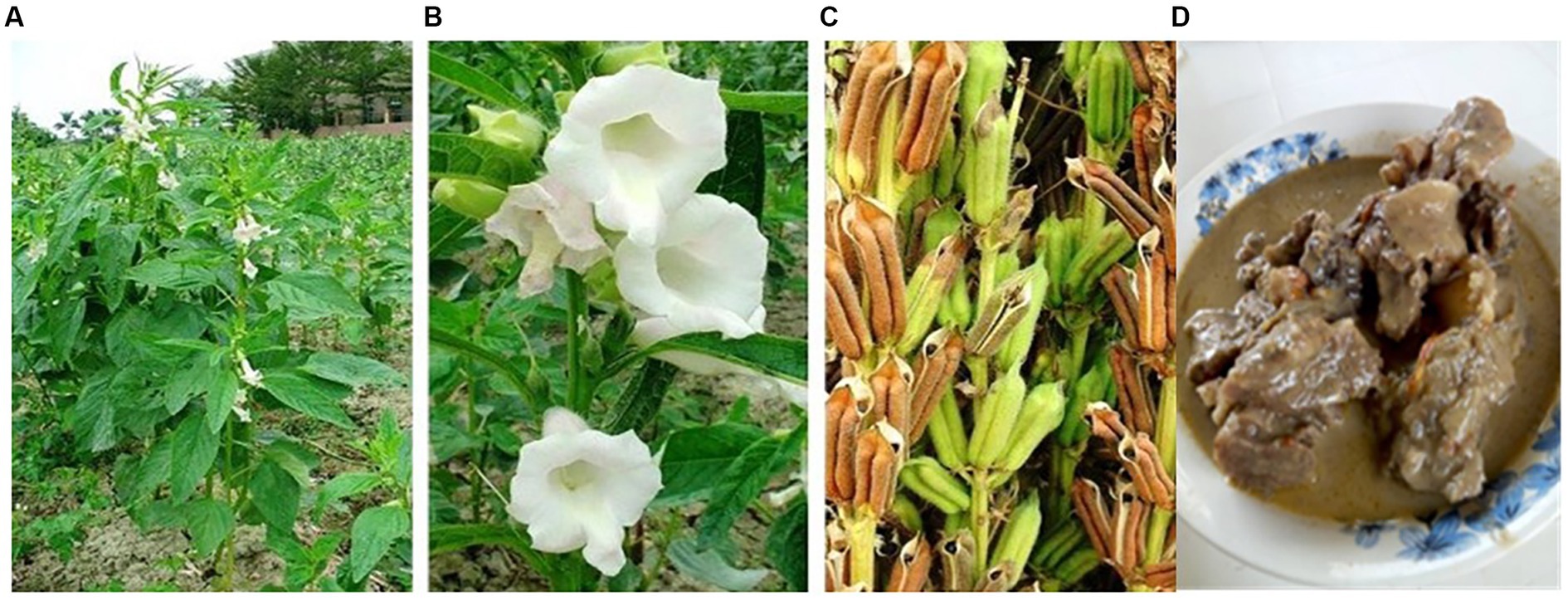
Figure 4. Photo series showing sesame showing: (A) sesame plant at various stages of growth (B) sesame plant flowers in bloom, (C) sesame seeds contained within calyx, and (D) local Ugandan dish prepared from sesame butter and smoked beef. (A–C) Hsu et al. (2011) and author’s own for image (D).
3.7.2. Nutritional composition and potential to contribute to nutrition and food security
In Uganda, sesame is an important food and cash crop, widely used among smallholders and resource-constrained households, particularly in the Northern and Eastern parts of the country (Wacal et al., 2021). Here, sesame seeds are eaten as a snack in the form of roasted seeds, with or without salt added, or “simsim” balls (roasted sesame seeds bound with molten sugar and formed into a rounded shape). Sesame seeds can also be prepared into a butter that is added to various dishes, like fish, chicken, beans, pigeon peas, beef (Figure 4D), sometimes in combination with peanut butter. In some instances, sesame leaves may be eaten as a vegetable (Namiki, 2007). Like pigeon pea, sesame seeds can be dried and stored for long periods of time, making it an important food security crop among these resource-poor households. Sesame seed, sesame cake, and sesame butter are all high in macronutrients and micronutrients. Sesame is a rich source of protein (3%–28%), with considerable amounts of essential amino acids, in addition to carbohydrate and fat (Table 2). The high oil content of sesame seed (Table 2), which constitutes the main source of calories, has earned sesame the name “queen of oil seeds” (Namiki, 2007; Bhat et al., 2014). Sesame seed oil is rich in n-6 and n-3 polyunsaturated fatty acids (PUFA), mainly oleic (≈39.1%) and linoleic (≈40.0%) acids and linolenic (trace amounts), while the saturated fatty acids are found in smaller amounts, that is, palmitic (≈9.4%) and stearic (≈4.76%) (Namiki, 2007). Sesame has an average protein content of 20%, although some varieties can have as high as 23 g/100 g (Table 2). The larger portion of the amino acid content of the protein in sesame is attributed to methionine (36 mg/100 g), cystine (25 mg/100 g), arginine (140 mg/100 g), and leucine (75 mg/100 g), with small amounts of lysine (31 mg/100 g), highlighting the potential for complementarity with other plant protein foods that are higher in lysine, like pigeon pea (see Section 3.6). In addition to the essential amino acids, Namiki (1995) found unroasted sesame seed to contain high amounts of glutamic acid, arginine, aspartic acid, and alanine, the amounts of which reduce with roasting. The carbohydrate content of sesame seed is largely dietary fibre (Table 2), with small amounts of glucose, fructose and some planteose oligosaccharide fractions (Namiki, 2007). The vitamin content of sesame mostly comprises B-vitamins such as, thiamine, niacin (Table 2), which is largely contained in the seed coat, necessitating the use of the whole seed, even as cake or butter to realize the full benefits of these B-vitamins. Although sesame contains no vitamin A, and only small amounts of carotenoids (Table 2), there are considerable amounts of vitamin E, mainly in the form of γ-tocopherols, and only minute amounts of α-tocopherol (Namiki, 2007). The content of many minerals is noteworthy, including potassium, iron, magnesium calcium and selenium (Table 2). Like the B-vitamins, many minerals are contained in the seed coat, and some are bound to anti-nutritional factors, such as, calcium oxalate (Namiki, 2007), presenting some challenges for micronutrient bioavailability.
Sesame has also been found to contain bioactives in the form of isoflavones (daidzein, genistein and glycitein) and campesterols and lignans (sesamin, sesamolin, sesamol and sesaminol glucosides), some of which are not found in other edible oils (Bedigian, 1985; Namiki, 2007; Bhat et al., 2014; Yaseen et al., 2021; U.S. Department of Agriculture, 2022; Wei et al., 2022). Lignans are a special type of dietary fibre that, together with campesterols and isoflavones, have been found to have several health benefits to humans, including lowering amounts of LDL cholesterol and improving the overall blood lipid profile, preventing high blood pressure, as well as having anti-carcinogenic, antimitotic, antivirus, anti-proliferation, pro-apoptosis, anti-inflammatory, anti-metastatic, and antiangiogenic effects (Namiki, 2007; Bhat et al., 2014; Singh et al., 2017; Yaseen et al., 2021; Wei et al., 2022). The anti-inflammatory effects of sesame oil have been attributed to various mechanisms including decrease in lipid oxidation, C-reactive protein, and interleukin-6 (Barbosa et al., 2017). Moreover, the lignans in sesame, as well as γ-tocopherols are strong antioxidants, which enhance the stability and shelf life of sesame oil by delaying or preventing rancidity of the polyunsaturated fatty acid (PUFA) fractions (Fukuda et al., 1985; Bhat et al., 2014). This would be particularly useful for resource-constrained households that might not have adequate storage facilities to maintain the integrity and quality of similar oils with high PUFA content. The nutritional and functional profile of sesame has increased its use in the food industry as health food, as well as seen its applications in the pharmaceutical, cosmetic and other industries as antiseptics, bactericides, disinfectants, antifungal, antibacterial, emollient and laxative, carrier for drugs, solvent for intramuscular injections and healing salve for minor burns, base for creams and lipsticks, natural sunscreen (Bedigian, 1985; Prasad et al., 2012; Bhat et al., 2014), although this is limited in Uganda. However, sesame, like pigeon peas, also has significant amounts of anti-nutritional factors, that is, phytic and oxalic acids, which impact on the bioavailability and digestibility of sesame protein and some micronutrients like calcium. This must be taken into consideration in promoting sesame consumption for food and nutrition security.
3.8. Sustainability issues relating to production and utilization of pigeon pea and sesame in Uganda
Pigeon pea is drought-tolerant and grows well in soils of low fertility. It can thrive in areas with average temperatures of 20–40°C and less than 625 mm of annual rainfall, where other crops like maize would fail (Subbarao et al., 2000; Gichohi-Wainaina et al., 2022). Pigeon pea has high potential because its deep taproots, means the plant can draw water and other nutrients from deeper layers in the soil, allowing it to thrive during dry seasons (Abebe, 2022; Gichohi-Wainaina et al., 2022). Although the pigeon pea can thrive in soils of various pH the crop is sensitive to frost, high salinity, and flooding. Some of these conditions describe what Uganda is already facing due to climate change, with erratic rain patterns and longer-than-usual dry seasons, both without and within the greater North, East, and West Nile regions (Wacal et al., 2021), where pigeon pea is cultivated. While there is scant data on environmental impact of pigeon peas, like other legumes, such as, beans and peas (Clune et al., 2017; Poore and Nemecek, 2018), it can be assumed that pigeon pea cultivation, particularly among smallholders where it is only subject to minimal processing, has a lower water footprint and greenhouse gas emission (GHGE). In studies where pigeon peas have been intercropped with cereals, e.g., maize and millet, the water use efficiency and crop yield of the cereals is greatly enhanced (Musokwa and Mafongoya, 2020; Singh et al., 2020). Moreover, pigeon pea also contributes to maintaining overall soil health, by enhancing fertility and binding soil particles, partly, due to its ability to fix nitrogen in the soil (Musokwa and Mafongoya, 2020; Namuyiga et al., 2022). Some authors like Wei et al. (2022) have suggested that sesame is an easy-to-cultivate, drought-tolerant crop that is suited to intercropping with other cereals and pulses, such as, pigeon pea, making it a suitable crop for areas like Uganda, which is already facing the effects of climate change, particularly in the main sesame-producing areas of the North and East (Demissie et al., 2019; Wacal et al., 2021). Notwithstanding, other authors purport that sesame is sensitive to drought especially during the vegetative stage of growth, which has resulted, in part, to decreased sesame production in Uganda as rains have become less reliable (Wacal et al., 2021). Other factors that have negatively impacted sesame production in Uganda are insect pests and diseases, poor soil fertility as a result of continued poor agronomic practices, poor agronomic practices, e.g., spacing, weeding, etc., low yielding varieties, and limited access to credit by smallholder farmers, who largely engage in the trade (Wacal et al., 2021). While environmental impact at the primary production level may be low for both pigeon pea and sesame, because there is limited research on value-added chains and only small amounts are exported, it is difficult to ascertain the cumulative impact of pigeon pea seeds and processed products from the farm-gate and beyond.
3.9. Factors affecting wider production and utilization of pigeon pea and sesame in Uganda
Anecdotal evidence suggests that pigeon pea was formerly a wild food eaten by wild animals, however, during a period of intense hunger in the 16th and 17th centuries, people in the greater Northern region (Acholi, Lango, and West-Nile) turned to the plant for food (Daily Monitor, 2020). Further evidence indicates that the pigeon pea was part of the pre-colonial and colonial-era diets, constituting an integral part of indigenous food systems of some ethnic groups in Uganda, like the Lango (Obuo et al., 2004; Raschke et al., 2007; Raschke and Cheema, 2008). At the time, and even in present times, pigeon peas, especially in the form of the split-pea sauce “dek-ngor”, had strong cultural connotations, being served at naming ceremonies, and at traditional marriages to the in-laws, during which time it is mixed with shea butter, locally called “moya” (Daily Monitor, 2020). Like pigeon pea, sesame oil was part of traditional diet in Uganda during pre-colonial and colonial times (Raschke and Cheema, 2008). During colonial times, the growth of the cash-crops economy heralded the beginning of a shift from traditional food crop cultivation, which resulted in reduced household and regional availability of traditional, nutrient-dense crops, such as, pigeon peas, sesame, pearl millet (Pennisetum glaucum), finger millet (Eleusine corocana), cowpeas (Vigna unguiculata), Bambara groundnut (Vigna subterranea), all of which can withstand varying levels of water and heat stress (Gura, 1986; Abegaz and Demissew, 1998). Still during colonial times, “modern” foods’, such as, refined sugar, wheat, maize flour, were greatly pushed with little consideration for the nutritional, socio-cultural and economic benefits of the indigenous African foods (Allen, 1955; Latham, 1964; Price, 2000).
Pigeon peas and sesame are generally accepted in Uganda, albeit to a larger degree in the Eastern and Northern regions. However, considering that peanuts and peanut butter are commonly used throughout the country both as a food and spread, and sesame and peanut butter are often used similarly, there is potential for even greater acceptance of sesame throughout the country. On the other hand, pigeon peas could complement other dried beans and peas, which are already used widely (Auma et al., 2021). More recently, Uganda, along with many sub-Saharan African countries, is experiencing a dietary transition—furthering the shift from traditional diets, characterized by legumes, nuts and seeds, fresh roots and tubers, and minimally processed foods, to more Westernized diets, characterized by high intake of refined sugar, refined carbohydrates, animal protein, saturated and trans fats and low dietary fiber (Auma et al., 2019, 2021; Revoredo-Giha et al., 2022). The dietary transition has been driven by globalization and economic growth, which has increased individual and household purchasing power, and marketing and advertising (Popkin, 2001; Popkin et al., 2012). These factors, in addition to wider macro food environment factors, such as, poor markets, little research on improved varieties and value addition, have possibly undermined the importance of pigeon pea and sesame as sustainable, nutritional powerhouses. In this regard, for example, sesame is currently seen more as a cash crop than a food crop, being exported to European and Asian countries, e.g., China, Japan, Turkey, and Vietnam (Wacal et al., 2021), where it is used in many industries. On the other hand, the production and consumption of sunflower oil has increased in Northern Uganda—the same areas in which sesame has traditionally been cultivated, with the resultant effect that more households now use it in place of sesame oil. Nevertheless, given changes in dietary consumption trends, there is scope for processing of both crops into products like noodles, gluten-free baked products, which can appeal to the emerging consumer. For acceptability in urban areas, it is suggested to explore further food processing, such as., pigeon-pea tofu, high-protein nutraceuticals, milks, meat replacers, high-protein, or high-fibre baked goods, which can be consumed by discerning or health-conscious consumers (Astuti et al., 2000; Silky and Tiwari, 2014; Tapal et al., 2019), and used to make baked goods, noodles, among others. The use of pigeon pea flour could act as a preplacement for wheat flour (and gluten-free). Recommendations on potential research, stakeholder, and policy engagement to increase utilization of pigeon pea and sesame in Uganda, Africa, and globally.
In addition to products liked gluten-free baked goods, wing to the nutritional profile and potential for complementarity with cereals and other legumes, food processors can formulate flour blends, comprising germinated or fermented pigeon pea flour as weaning foods to alleviate malnutrition, among children under five and as women of reproductive age. Furthermore, there is potential for the use of both pigeon pea and sesame in the production of local ready-to-use foods (RTUFs), which can be used in the treatment and management of malnutrition in other regions within the country. Here, soybean and peanut butter can be replaced by or used in combination with pigeon pea and sesame, respectively. Furthermore, some authors have identified the potential of pigeon pea and sesame protein isolates and hydrolysates for the manufacture of functional foods (Tapal et al., 2019; Aondona et al., 2021). However, for this to happen, it is critical to protect the germplasm and diversity in existing varieties and conduct research, through National Agricultural Research Laboratories, with support and involvement from national and international research organizations, as well as food and agricultural industry players, policy makers and the public, on improved varieties that are still nutrient-dense but tolerant to environmental stressors, particularly salinity and flooding. Moreover, another starting point is to establish consumers’ knowledge of pigeon pea nutritional and other benefits, as well as understand determinants of pigeon pea consumption or lack thereof. Similarly, there is a need to produce improved sesame varieties that are still high in nutrients, low in anti-nutritional factors, and yet resistant to the effects of climate change, particularly water stress. Farmers need to be educated on good agronomic practices, such as, pest and disease control and soil fertility management, with reliable microcredit facilities extended to them especially at critical times in the crop production cycle, such as, land preparation and harvesting, to prevent post-harvest losses and infestation with microorganisms like Aspergillus. On the other hand, like pigeon pea, consumers need to be educated on the nutritional benefits of sesame oil over alternatives, like sunflower oil and hydrogenated vegetable fat. Also, an exploration of the determinants of sesame seed consumption, or lack thereof, must be established for an understanding of what factors are pertinent to increasing uptake, and consequently, creating local demand. Stakeholders could also explore possible synergies between the two crops by formulating nutrient-enriched products, which harness the strengths of either crop, while at the same time, infrastructure to support the manufacture of these products needs to be put in place among both small and medium-size enterprises and well as large manufacturers. This should not only include adequate transportation to markets and the requisite food processing machinery, but should also consider sources of power, including renewable/sustainable/green energies. Stakeholders might consider taking advantage of the fact that the areas in which both crops are cultivated experience a longer dry season, which could facilitate the implementation of solar energy, for example. Lastly, an exploration of the potential for use of both pigeon pea and sesame in adjunct industries such as an ingredient in pharmaceuticals, and a base in cosmetics, should be explored by reviewing use in case-study countries that make use of them. This might increase among farmers across the country, and ramp up production. However, with any manufacturing processes, environmental impacts in terms of energy, GHGE and water footprint need to be determined, with respect to how that balances out with other aspects of sustainability, that is, economic (smallholder livelihoods enhancement) and sociocultural aspects. In summary, to increase pigeon pea and sesame production and consumption for improved food and nutrition security, a multidisciplinary approach is required between various stakeholders, including academics (plant breeders, nutritionists, and food scientists), local and international third sector organizations and policy makers.
3.10. Summary
Uganda is one of the most ecologically biodiverse countries (>18,000 flora and fauna species) globally (Kimani et al., 2020) and produces more than it consumes. However, a high proportion of children under five are still stunted (28.9%) and anaemic (53%), while high proportions of women of reproductive age suffer from iron deficiency anaemic. Moreover, severe household food insecurity in the country is still high, standing at 23.2% at the national level (FAO, IFAD, UNICEF, WFP and WHO, 2022.) and higher still, on average, in the Greater Northern region (Revoredo-Giha et al., 2022). Similarly, Nigeria, though rich in a variety of crops, with most of them falling within the category of NUS, is facing the same challenge such as severe food and nutrition insecurity, poorly managed land use and westernized diet transition. This is a timely call for more NUS plants to be promoted to be used for food, improve the diet diversity, and therefore, the quality of many household diets and wellbeing.
Raising awareness of the myriad benefits of the sesame, pigeon pea, African breadfruit and African walnut, alongside other NUS, while concurrently establishing value chains, including appropriate processing technologies for novel products, could help increase production and make these crops more desirable to consumers in Uganda, Nigeria, and sub-Saharan Africa generally. Moreover, value-added products could also be economically valuable for export markets. Greater utilization of NUS presents an opportunity to build healthier, more sustainable global, and indigenous food systems. However, this can only be realized by employing evidence-based information on the food and nutrition security potential of NUS and appropriate economically, socially, and environmentally viable processing methods and build a healthy marketing system, to enhance food production and dietary diversity, while contributing to resilience and improved livelihoods of smallholder and rural population.
Author contributions
CN, CA, and YG: conception of idea and revision and proofreading of manuscript. CN and CA: manuscript drafting, contributed equally, and hence, share the first authorship. YG: general supervision. All authors contributed to the article and approved the submitted version.
Funding
YG is supported by UK Biotechnology and Biological Sciences Research Council (BBSRC) Grant number (BB/T008989/1). This publication was supported by the Economic and Social Research Council [grant number ES/T003871/1] under the ARUA-GCRF UKRI Partnership Program as part of the Capacity Building in Food Security (CaBFoodS-Africa) project. The grant holders acknowledge that opinions, findings and conclusions or recommendations expressed in this publication are that of the author(s), and that they accepts no liability whatsoever in this regard.
Conflict of interest
The authors declare that the research was conducted in the absence of any commercial or financial relationships that could be construed as a potential conflict of interest.
Publisher’s note
All claims expressed in this article are solely those of the authors and do not necessarily represent those of their affiliated organizations, or those of the publisher, the editors and the reviewers. Any product that may be evaluated in this article, or claim that may be made by its manufacturer, is not guaranteed or endorsed by the publisher.
References
Abebe, K. B. (2022). The dietary use of pigeon pea for human and animal diets. Sci. World J. 2022, 1–12. doi: 10.1155/2022/4873008
Abegaz, D., and Demissew, S. (1998). Indigenous African useful plants: medicinal plants in Ethiopia, Kenya, Tanzania and Uganda. The United Nations University/the Institute for Natural Resources in Africa (UNU/INRA), survey report presented at the regional workshop for African academics and scientists, 2 to 5 March 1998, Accra, Ghana.
Ade-Omowaye, B. I. O., Tucker, G. A., and Smetanska, I. (2015). Nutritional potential of nine underexploited legumes in South-West Nigeria. Int. Food Res. J. 22, 798–806.
Ajiwe, V. I. E., Okeke, C. A., and Agbo, H. U. (1995). Extraction and utilization of breadfruit seeds oil (Treculia africana). Bioresour. Technol. 53, 183–184. doi: 10.1016/0960-8524(95)00059-N
Akinnifesi, F. K., Sileshi, G., Ajayi, O. C., Chirwa, P. W., Kwesiga, F. R., and Harawa, R. (2008). Contributions of agroforestry Research and Development to livelihood of smallholder farmers in southern Africa: fruit, medicinal, Fuelwood and fodder tree systems. Agric. J. 3, 76–88.
Akomolafe, S. F., Oboh, G., Akindahunsi, A. A., and Afolayan, A. J. (2015). Antiperoxidative activity of Tetracarpidium conophorum leaf extract in reproductive organs of male rats. Evid. Based Complement. Alternat. Med. 2015:798491. doi: 10.1155/2015/798491
Akporhonor, E., Egwaikhide, P., and Eguavoen, I. (2006). Effect of sprouting on in vitro digestibility of some locally consumed leguminous seeds. J. Appl. Sci. Environ. Manag. 10, 55–58.
Amanze, N. J., Agbo, N. J., Eke-Okoro, O. N., and Njoku, D. N. (2011). Selection of yam seeds from open pollination for adoption in yam (Dioscorea rotundata Poir) production zones in Nigeria. J. Plant Breed. Crop Sci. 3, 68–73.
Amarteifio, J. O., Munthali, D. C., Karikari, S. K., and Morake, T. K. (2002). The composition of pigeon peas (Cajanus cajan (L.) Millsp.) grown in Botswana. Plant Foods Hum. Nutr. 57, 173–177. doi: 10.1023/A:1015248326920
Amujiri, A. N., Nwosu, M. O., Nzekwe, U., Osayi, E. E., and Sani, M. B. (2018). Studies on the phenology of African breadfruit (Treculia africana Decne) in south eastern Nigeria. Environ. Ecol. Res. 6, 248–258. doi: 10.13189/eer.2018.060405
Amusa, T. O., Jimoh, S. O., Azeez, I. O., Awodoin, R. O., and Kareem, I. M. (2014). Stock density and fruit yield of African walnut, Plukenetia conophora Mull-Arg (Syn. Tetracarpidium conophorum) in tropical lowland rainforests of Southwest Nigeria. J. Trop. For. Sci. 4, 73–81.
Anitha, S., Govindaraj, M., and Kane-Potaka, J. (2019a). Balanced amino acid and higher micronutrients in millets complements legumes for improved human dietary nutrition. Cereal Chem. 97, 74–84. doi: 10.1002/cche.10227
Anitha, S., Thyn, H., Taku, T., Ashok, J., and Joanna, K. (2019b). Potential for smart food products in rural Myanmar: use of millets and pigeon pea to fill the nutrition gap. J. Sci. Food Agric. 100, 394–400. doi: 10.1002/jsfa.10067
Aondona, M. M., Ikya, J. K., Ukeyima, M. T., Gborigo, T. J. A., Aluko, R. E., and Girgih, A. T. (2021). In vitro antioxidant and antihypertensive properties of sesame seed enzymatic protein hydrolysate and ultrafiltration peptide fractions. J. Food Biochem. 45:e13587. doi: 10.1111/jfbc.13587
Asaolu, S. S. (2009). Amino acid composition of Tetracarpidium conophorum. Orient. J. Chem. 25, 63–66.
Association of Agricultural Research Institutions in the Near East and North Africa (AARINENA); The Asia-Pacific Association of Agricultural Research Institutions (APAARI); Crops For the Future (CFF); The Forum for Agricultural Research in Africa (FARA); The Global Forum for Agricultural Research and Innovation (GFAR); and The Alliance of Biodiversity International and CIAT. (2021). Global manifesto on forgotten foods. Available at: https://aarinena.org/wp-content/uploads/2021/08/Global-Manifesto-on-Forgotten-Foods-Final_0.pdf (Accessed November 1, 2022).
Astuti, M., Meliala, A., Dalais, F. S., and Wahlqvist, M. L. (2000). Tempe, a nutritious and healthy food from Indonesia. Asia Pac. J. Clin. Nutr. 9, 322–325. doi: 10.1046/j.1440-6047.2000.00176.x
Auma, I. C., Pradeilles, R., Blake, M., and Holdsworth, M. (2019). What can dietary patterns tell us about the nutrition transition and environmental sustainability of diets in Uganda? Nutrients 11:342. doi: 10.3390/nu11020342
Auma, I. C., Pradeilles, R., Blake, M., Musoke, D., and Holdsworth, M. (2021). Factors influencing dietary practices in a transitioning food environment: a cross-sectional exploration of four dietary typologies among rural and urban Ugandan women using photovoice. Nutr. J. 19, 1–15. doi: 10.1186/s12937-020-00634-9
Awofadeju, O. F. J. (2020). Evaluation of protein quality in blends prepared from commercial wheat (Triticum aestivum) and yellow maize flours (Zea mays) and African walnut (Tetracarpidium conophorum) protein isolate. Euro. Mod. Stud. J 4, 113–129.
Awofadeju, O. F. J., Awe, A. B., Adewumi, O. J., Amadi, B. J., and Oluwatoke, F. J. (2021). Nutritionally improved cookies from composite flour: African walnut (Tetracarpidium conophorum) with wheat. Niger. Agric. J. 52, 236–240.
Ayoola, P. B., Onawumi, O. O., and Faboya, O. O. P. (2011). Chemical evaluation and nutritive values of Tetracarpidium conophorum (Nigerian walnut) seeds. J. Pharm. Biomed. Anal. 11, 1–5.
Baiyeri, K. P., and Olajide, K. (2022). Chapter lesser known African indigenous tree and fruit plants: recent evidence from literatures and regular cultivation culture. Available at: www.intechopen.com.
Baldermann, S., Blagojević, L., Frede, L., Klopsch, R., Neugart, S., Neumann, A., et al. (2016). Are neglected plants the food for the future? Crit. Rev. Plant Sci. 35, 106–119. doi: 10.1080/07352689.2016.1201399
Barbosa, C. V., Silva, A. S., De Oliveira, C. V. C., Massa, N. M. L., De Sousa, Y. R. F., Da Costa, W. K. A., et al. (2017). Effects of sesame (Sesamum indicum L.) supplementation on Creatine kinase, lactate dehydrogenase, oxidative stress markers, and aerobic capacity in semi-professional soccer players. Front. Physiol. 8, 1–7. doi: 10.3389/fphys.2017.00196
Bedigian, D. (1985). Sesamum indicum L. crop origin, diversity, chemistry and ethnobotany, PhD, University of Illinois, Champaign-Urbana University Microfilms DA8502071 Dissertation Abstracts International 1985 45:3410–B [cited as requested].
Bhat, K. V., Kumari, R., Pathak, N., and Rai, A. K. (2014). Value addition in sesame: a perspective on bioactive components for enhancing utility and profitability. Pharmacogn. Rev. 8, 147–155. doi: 10.4103/0973-7847.134249
Biodiversity International. (2017). Mainstreaming agrobiodiversity in sustainable food systems: scientific foundations for an agrobiodiversity index. Rome: Biodiversity International.
Brown, L., Rosner, B., Willett, W. W., and Sacks, F. M. (1999). Cholesterol-lowering effects of dietary fiber: a meta-analysis. Am. J. Clin. Nutr. 69, 30–42. doi: 10.1093/ajcn/69.1.30
Burkill, H. M. (1985). The useful plants of west tropical Africa, vol. 2. Kew, UK: Royal Botanic Gardens
Clune, S., Crossin, E., and Verghese, K. (2017). Systematic review of greenhouse gas emissions for different fresh food categories. J. Clean. Prod. 140, 766–783. doi: 10.1016/j.jclepro.2016.04.082
Daily Monitor. (2020). Dek-ngor, traditional sauce that also works as food on its own. Available at: https://www.monitor.co.ug/uganda/special-reports/uganda-50/dek-ngor-traditional-sauce-that-also-works-as-food-on-its-own-1515754 (Accessed November 22, 2022).
Dakora, F. D. (2000). Commonality of root nodulation signals and nitrogen assimilation in tropical grain legumes belonging to the tribe Phaseoleae. Funct. Plant Biol. 27:885. doi: 10.1071/PP00015
Dauda, A. O., Abiodun, O. A., Akintayo, O. A., Babayeju, A. A., Salami, K. O., and Oyekanmi, I. A. (2020). Influence of walnut on the nutritional and physicochemical properties of biscuits made from whole wheat. Agrosearch. 20, 45–58. doi: 10.4314/agrosh.v20i1.5s
Demissie, T., Duku, C., Groot, A., Oroma, G., Osumba, J., Budding-Polo, M., et al. (2019). Sesame Uganda: Climate change risks and opportunities. CGIAR Research Program on Climate Change, Agriculture and Food Security (CCAFS); Wageningen Environmental Research, The Netherlands.
Edem, C. A., Dosunmu, M. I., and Bassey, F. I. (2009). Determination of proximate composition, ascorbic acid and heavy metal content of African walnut (Tetracarpidium conophorum). Pak. J. Nutr. 8, 225–226. doi: 10.3923/pjn.2009.225.226
Ejiofor, M. A. N., Obiajulu, O. R., and Okafor, J. C. (2012). Diversifying utilities of African breadfruit as food and feed. Int. Tree Crops J. 5, 125–134. doi: 10.1080/01435698.1988.9752847
Eleazu, C., Ezekwibe, I., Egbe, M., Saidu, S., Eleazu, K., and Egedigwe, C. (2017). Dietary intake of boiled breadfruit (Treculia africana) seeds did not improve hyperglycemia in streptozotocin induced diabetic rats: effect on the oral glucose tolerance of normoglycemic rats. Acta Sci. Pol. Technol. Aliment. 16, 93–99. doi: 10.17306/J.AFS.2017.0460
Eleazu, K., Maduabuchi, P., and Eleazu, C. (2018). Effect of ethanol extract of boiled breadfruit (Treculia Africana) seed on the oral glucose tolerance, lipid profile, and body weight of normoglycemic albino rats. Food Sci. Nutr. 6, 904–911. doi: 10.1002/fsn3.626
Embaby, H. E. (2010). Effect of heat treatments on certain antinutrients and in vitro protein digestibility of peanut and sesame seeds. Food Sci. Technol. Res. 17, 31–38. doi: 10.3136/fstr.17.31
Enujiugha, V. N. (2003). Chemical and functional characteristics of conophor nut. Pak. J. Nutr. 2, 335–338. doi: 10.3923/pjn.2003.335.338
Evans, C. E. L. (2020). Dietary fibre and cardiovascular health: a review of current evidence and policy. Proc. Nutr. Soc. 79, 61–67. doi: 10.1017/S0029665119000673
FAO. (2019). The global economy of pulses. Available at: http://www.fao.org/3/i7108en/i7108en.pdf (Accessed November 20, 2022).
FAO, IFAD, UNICEF, WFP and WHO. (2022). The state of food security and nutrition in the world 2022. Repurposing food and agricultural policies to make healthy diets more affordable. Rome, FAO.
FAOSTAT. (2022). FAO statistical databases (FAOSTAT) Rome, FAO. Available at: https://www.fao.org/faostat/en/#home (Accessed November 20, 2022).
Fasina, O. O., and Ajibola, O. O. (1989). Mechanical expression of oil from conophor nut (Tetracarpidium conophorum). J. Agric. Eng. Res. 44, 275–287. doi: 10.1016/S0021-8634(89)80088-5
Fukuda, Y., Nagata, M., Osawa, T., and Namiki, M. (1985). Chemical aspects of the antioxidative activity of roasted sesame seed oil, and the effect of using the oil for frying. Agric. Biol. Chem. 50, 857–862. doi: 10.1271/bbb1961.50.857
Fuller, D. Q., Murphy, C., Kingwell-Banham, E., Castillo, C. C., and Naik, S. (2019). Cajanus cajan (L.) Millsp. origins and domestication: the south and southeast Asian archaeobotanical evidence. Genet. Resour. Crop. Evol. 66, 1175–1188. doi: 10.1007/s10722-019-00774-w
Gbenga-Fabusiwa, F. J., Oladele, E. P., Oboh, G., Adefegha, S. A., and Oshodi, A. A. (2018). Nutritional properties, sensory qualities and glycemic response of biscuits produced from pigeon pea-wheat composite flour. J. Food Biochem. 42:e12505. doi: 10.1111/jfbc.12505
Gebremariam, M. M., Zarnkow, M., and Thomas Becker, T. (2014). Teff (Eragrostis tef) as a raw material for malting, brewing, and manufacturing of gluten-free foods and beverages: a review. J. Food Sci. Technol. 51, 2881–2895. doi: 10.1007/s13197-012-0745-5
Giami, S. Y. (2004). Comparison of bread making properties of composite flour from kernels of roasted and boiled African bread fruit (Treculia Africana decne) seeds. J. Mater. Res. 1, 16–25.
Gichohi-Wainaina, W. N., Mremi, R., Chande, M., Msuya, J. M., Kumwenda, N. C., Muzanila, Y. C., et al. (2022). Drivers of pigeon pea consumption among school-aged children in Central Tanzania. Front. Sustain. Food Syst. 5:726404. doi: 10.3389/fsufs.2021.726404
Gruere, G., Guiliani, A., and Smale, M. (2006). Marketing underutilized plant species for the benefit of the poor: a conceptual framework, International Food Policy Research Institute, Washington, p. 1.
Gura, S. (1986). A note on traditional food plants in East Africa: their value for nutrition and agriculture. Food Nutr. 12, 18–26.
Hassler, M. (2022). World plants. Synonymic checklist and distribution of the world Flora. Version 14.4; last update December 4th, 2022. Available at: www.worldplants.de (Accessed December 12, 2022).
Hendre, S. P., Muthemba, S., Kariba, R., Muchugi, A., Fu, Y., Change, Y., et al. (2019). African orphan crops consortium (AOCC): status of developing genomic resources for African orphan crops. Planta 250, 989–1003. doi: 10.1007/s00425-019-03156-9
Hillocks, R. J., Minja, E., Nahdy, M. S., and Subrahmanyam, P. (2000). Diseases and pests of pigeon pea in eastern Africa. Int. J. Pest Manag. 46, 7–18. doi: 10.1080/096708700227534
Hsu, D. Z., Chu, P. Y., and Liu, M. Y. (2011). “Extracts of sesame (Sesamum indicum L.) seeds and gastric mucosal cytoprotection” in Nuts and seeds in health and disease prevention. eds. V. R. Preedy, R. R. Watson, and V. B. Patel (Cambridge, MA: Academic Press), 1019–1027.
Hutchinson, J., and Dalziel, J. M. E. (1928a). Flora of West Tropical Africa 1 (2). The London: Crown agents for the colonies, 247–523.
Hutchinson, J., and Dalziel, J. M. (1928b). Tropical African plants: IV. Bull. Misc. Inform. 1928, 296–301. doi: 10.2307/4107088
Idowu, A. O., Alashi, A. M., Nwachukwu, I. D., Fagbemi, T. N., and Aluko, R. E. (2021). Functional properties of sesame (Sesamum indicum Linn) seed protein fractions. Food Prod. Process. Nutr. 3:4. doi: 10.1186/s43014-020-00047-5
Ifediba, D. I., and Nwafor, E. C. (2018). Nutritional and sensory evaluation of African breadfruit-corn yoghurt. Afr. J. Food Sci. 12, 73–79. doi: 10.5897/AJFS2017.1570
Ijeh, I. I., Ejike, C. E., Nkwonta, O. M., and Njoku, B. (2010). Effect of traditional processing techniques on the nutritional and phytochemical composition of African bread-fruit (Treculia africana) seeds. J. Appl. Sci. Environ. 14, 169–173.
Kajihausa, O., Fasasi, R., and Atolagbe, Y. (2014). Effect of different soaking time and boiling on the proximate composition and functional properties of sprouted sesame seed flour. Niger. Food J. 32, 8–15. doi: 10.1016/S0189-7241(15)30112-0
Kimani, A., Mayer, A., and Swiderska, K. (2020). Putting indigenous foods and food systems at the heart of sustainable food and nutrition security in Uganda. Discussion Paper. IIED and Hivos, London and The Hague. Available at: https://www.iied.org/sites/default/files/pdfs/migrate/16663IIED.pdf
Kingwell-Banham, E., and Fuller, D. Q. (2014). “Pigeon pea: origins and development” in Encyclopedia of global archaeology. ed. C. Smith (New York, NY: Springer)
Kuete, V., Metuno, R., Ngameni, B., Mbaveng, A. T., Ngandeu, F., Benzabih, M., et al. (2008). Antimicrobial activity of the methanolic extracts and compounds from Treculia africana and Treculia vacuminata (Moraceae). S. Afr. J. Bot. 75, 111–115.
Kumar, V., Muthu Kumar, S. P., and Tiku, P. K. (2021). Hypocholesterolemic effect of potent peptide and bioactive fraction from pigeon pea by-products in Wistar rats. Int. J. Pept. Res. Ther. 27, 2403–2415. doi: 10.1007/s10989-021-10261-5
Li, X., and Siddique, K. H. M. (2018). Future smart food – rediscovering hidden treasures of neglected and underutilized species for zero hunger in Asia, Bangkok, 242 pp. [cited as requested].
Li, X., and Siddique, K. H. M. (2020). Future smart food: harnessing the potential of neglected and underutilized species for zero hunger. Matern. Child Nutr. 16:e13008. doi: 10.1111/mcn.13008
Li, X., Yadav, R., and Siddique, K. H. M. (2020). Neglected and underutilized crop species: the key to improving dietary diversity and fighting hunger and malnutrition in Asia and the Pacific. Front. Nutr. 7:593711. doi: 10.3389/fnut.2020.593711
Malu, S. P., Obochi, G. O., Edem, C. A., Nyong, B. E., Edem, C. A., Obochi, G. O., et al. (2009). Effect of methods of extraction on phytochemical constituents and antibacterial properties of Tetracarpidium conophorum seeds. Global J. Pure Appl. Sci. 15, 373–376.
Manyasa, E., Silim, S., and Christiansen, J. (2009). Variability patterns in Ugandan pigeon pea landraces. J. SAT Agric. Res. 7, 1–9.
Morton, J. F., Smith, R. E., Lugo-Lopez, M. A., and Abrams, R., (1982). Pigeonpeas (Cajanus cajan Millisp.): a valuable crop of the tropics. Department of Agronomy and Soils, University of Puerto Rico Mayaguez Campus.
Musokwa, M., and Mafongoya, P. (2020). Pigeonpea yield and water use efficiency: a savior under climate change-induced water stress. Agronomy 11:5. doi: 10.3390/agronomy11010005
Naluwairo, R. (2011). Investing in orphan crops to improve food and livelihood security of Uganda’s rural poor: policy gaps, opportunities and recommendations. ACODE Policy Research Series, No. 43. Kampala [cited as requested].
Namiki, M. (1995). The chemistry and physiological functions of sesame. Food Rev. Intl. 11, 281–329. doi: 10.1080/87559129509541043
Namiki, M. (2007). Nutraceutical functions of sesame: a review. Crit. Rev. Food Sci. Nutr. 47, 651–673. doi: 10.1080/10408390600919114
Namuyiga, D. B., Stellmacher, T., Borgemeister, C., and Groot, J. C. J. (2022). A typology and preferences for pigeon pea in smallholder mixed farming systems in Uganda. Agriculture 12:1186. doi: 10.3390/agriculture12081186
Ndie, E. C., Nnamani, C. V., and Oselebe, H. O. (2010). Some physicochemical characteristics of defatted flours derived from African walnut (Tetracarpidium conophorum): an underutilized legume. Pak. J. Nutr. 9, 909–911. doi: 10.3923/pjn.2010.909.911
Nix, A., Paull, C. A., and Colgrave, M. (2015). The flavonoid profile of pigeon pea, Cajanus cajan: a review. Springer Plus 4:125. doi: 10.1186/s40064-015-0906-x
Nkwonta, G. C., Alamar, M. C., Cellek, S., and Terry, L. A. (2021). Overview of potential individual phenolic compounds of African walnut extracts and cytotoxicity assessment in lung cancer cell line (A549). In VIII International Symposium on Human Health Effects of Fruits and Vegetables-FAVHEALTH 2021 1329, 51–64.
Nkwonta, G. C., Carmen, A. M., Landahl, S., and Terry, L. A. (2016). Effects of postharvest storage and processing techniques on the main fatty acids in the profile of oil extracted from African walnut (Tetracarpidium conophorum Mull. Arg.). J. Food Compos. Anal. 45, 87–94. doi: 10.1016/j.jfca.2015.10.001
Nkwonta, G. C., Ezeokonkwo, C. G., Obidoa, O., and Parker, P. E. (2010). Effects of roasted Tetracarpidium conophorum-based died on some haematological parameters, renal and liver function biomarkers in male Wister rats. J. Pharm. Res. 3, 1865–1871.
Nkwonta, G. C., Ezeokonkwo, C. A., and Obizoba, I. C. (2013). Comparative effects of moist and dry heat on nutrient potentials of Tetracarpidium conophorum nut in rats. Pak. J. Nutr. 12, 89–92. doi: 10.3923/pjn.2013.89.92
Nkwonta, G. C., Medina, A., Alamar, M. C., and Terry, L. A. (2015). Impact of postharvest processing on the fungal population contaminating African walnut shells (Tetracarpidium conophorum Mull. Arg) at different maturity stages and potential mycotoxigenic implications. Int. J. Food Microbiol. 194, 15–20. doi: 10.1016/j.ijfoodmicro.2014.10.024
Nuga, O. O., and Ofodile, E. A. U. (2010). Potentials of Treculia africana Decne–an endangered species of southern Nigeria. J. Agri. Soc. Res. 10, 91–99.
Obuo, J. E. P., Omadi, J. R., and Okwang, D. (2004). Pigeon pea seed production and delivery system: experience from the Lango farming system. Uganda J. Agric. Sci. 9, 645–650.
Odeny, D. A. (2007). The potential of pigeonpea (Cajanus cajan (L.) Millsp.) in Africa. Nat. Res. Forum 31, 297–305. doi: 10.1111/j.1477-8947.2007.00157.x
Ohajianya, D., and Osuafor, O. (2017). Economics of household demand for African breadfruit (Treculia Africana) in Owerri agricultural zone of IMO STATE, Nigeria. Int. J. Environ. Agric. Biotech. 2, 2805–2810. doi: 10.22161/ijeab/2.6.5
Ojimelukwe, P. C., and Ugwuona, F. U. (2021). The traditional and medicinal use of African breadfruit (Treculia africana Decne): an underutilized ethnic food of the Ibo tribe of south-east, Nigeria. J. Ethn. Foods 8, 1–13. doi: 10.1186/s42779-021-00097-1
Okafor, J. C. (1981). Delimitation of a new variety of Treculia africana Decaisne subsp. Africana (Moraceae). Bulletin Du Jardin Botanique National de Belgique / Bulletin van de National Plantentuin van België 51, 191–199. doi: 10.2307/3667741
Okwunodulu, I., Chikezie, S., Linus-Chibuezeh, A., and Abasiekong, S. (2021). Evaluation of vegetable milk from milk blends of soybean (Glycine max.) and African breadfruit seeds (Treculia africana). Croatian J. Food Sci. Technol. 13, 78–89. doi: 10.17508/cjfst.2021.13.1.10
Oladiji, A. T., Abodunrin, T. P., and Yakubu, M. T. (2010). Toxicological evaluation of Tetracarpidium conophorum nut oil-based diet in rats. Food Chem. Toxicol. 48, 898–902. doi: 10.1016/j.fct.2009.12.030
Onwuli, D. O., Brown, H., and Ozoani, H. A. (2014). Antihyperglycaemic effect of Tetracarpidium conophorum nuts in Alloxan induced diabetic female albino rats. Int. Sch. Res. Notices 2014, 1–4. doi: 10.1155/2014/124974
Osabor, V. N., Ogar, D. A., Okafor, P. C., and Egbung, G. E. (2009). Profile of the African bread fruit (Treculia africana). Pak. J. Nutr. 8, 1005–1008. doi: 10.3923/pjn.2009.1005.1008
Oyelola, O. O., Moody, J. O., Odeniyi, M. A., and Fakeye, T. O. (2007). Hypoglycemic effect of Treculia africana Decne root bark in normal and alloxan-induced diabetic rats. Afr. J. Tradit. Complement. Altern. Med. 4, 387–391. doi: 10.4314/ajtcam.v4i4.31233
Olapade, A. A., and Umeonuorah, U. C. (2013). Mineral, vitamin and antinutritional content of African breadfruit (Treculia africana) seeds processed with alum and trona. J. Environ. Sci., Toxicol. Food Technol. 5, 71–78.
Padulosi, S., Thompson, J., and Rudebjer, P. (2013). Fighting poverty, hunger, and malnutrition with neglected and underutilized species (NUS): needs, challenges, and the way forward. Biodiversity International, Rome. [cited as requested]
Pathak, N., Verma, N., Singh, A., Bhat, K. V., and Lakhanpaul, S. (2020). Investigations on diverse sesame (S. indicum L.) germplasm and its wild allies reveal wide variation in antioxidant potential. Physiol. Mol. Biol. Plants 26, 697–704. doi: 10.1007/s12298-020-00784-4
Payal, R., Mamshad, A., and Arti, T. (2015). Mint: study on antimicrobial and antioxidant properties of walnut oil. Int. J. Curr. Res. Chem. Pharm. Sci. 1, 51–55.
Poore, J., and Nemecek, T. (2018). Reducing food’s environmental impacts through producers and consumers. Science 360, 987–992. doi: 10.1126/science.aaq0216
Popkin, B. M. (2001). The nutrition transition and obesity in the developing world. J. Nutr. 131, 871S–873S. doi: 10.1093/jn/131.3.871S
Popkin, B. M., Adair, L. S., and Ng, S. W. (2012). Global nutrition transition and the pandemic of obesity in developing countries. Nutr. Rev. 70, 3–21. doi: 10.1111/j.1753-4887.2011.00456.x
Prasad, M. N., Sanjay, K. R., Prasad, D. S., Vijay, N., Kothari, R., and Nanjunda Swamy, S. (2012). A review on nutritional and nutraceutical properties of sesame. J. Nutr. Food Sci. 2, 1–6. doi: 10.4172/2155-9600.1000127
Raneri, J., Padulosi, S., Meldrum, G., and King, O. (2019). Promoting neglected and underutilized species to boost nutrition in LMICs. UNSCN Nutrition 44, 10–25.
Raschke, V., and Cheema, B. (2008). Colonisation, the New World order, and the eradication of traditional food habits in East Africa: historical perspective on the nutrition transition. Public Health Nutr. 11, 662–674. doi: 10.1017/S1368980007001140
Raschke, V., Oltersdorf, U., Elmadfa, I., Wahlqvist, L. M., Cheema, S. B., and Kouris-Blazos, A. (2007). Content of a novel online collection of traditional east African food habits (1930s-1960s): data collected by the max-Planck-nutrition research unit, Bumbuli, Tanzania. Asia Pac. J. Clin. Nutr. 16, 140–151. doi: 10.1017/S1368980007001140
Revoredo-Giha, C., Toma, L., Akaichi, F., and Dawson, I. (2022). Exploring the effects of increasing underutilized crops on consumers’ diets: the case of millet in Uganda. Agric. Food Econ. 10:1. doi: 10.1186/s40100-021-00206-3
Reynolds, A. N., Akerman, A. P., and Mann, J. (2020). Dietary fibre and whole grains in diabetes management: systematic review and meta-analyses. PLoS Med. 17:e1003053. doi: 10.1371/journal.pmed.1003053
Rizvi, H. E. Q., Kumar, K., Ahmed, N., Yadav, N. A., Chauhan, D., Thakur, C., et al. (2022). Influence of soaking and germination treatments on the nutritional, anti-nutritional, and bioactive composition of pigeon pea (Cajanus cajan L.). J. Appl. Biol. Biotechnol. 10, 127–134. doi: 10.7324/JABB.2022.100317
Saxena, K. B., Kumar, R. V., and Rao, P. V. (2002). Pigeonpea nutrition and its improvement. J. Crop. Prod. 5, 227–260. doi: 10.1300/j144v05n01_10
Sekhon, J., Grewal, S. K., Singh, I., and Kaur, J. (2017). Evaluation of nutritional quality and antioxidant potential of pigeon pea genotypes. J. Food Sci. Technol. 54, 3598–3611. doi: 10.1007/s13197-017-2818-y
Shelef, O., Weisberg, P. J., and Provenza, F. D. (2017). The value of native plants and local production in an era of global agriculture. Front. Plant Sci. 8:2069. doi: 10.3389/fpls.2017.02069
Silky, G. M. P., and Tiwari, A. (2014). Development of high protein biscuits using pigeon pea Brokens flour. Int. J. Eng. Innov. Technol. 4, 84–89.
Simtowe, F., Shiferaw, B., Kassie, M., Abate, T., Silim, S., Siambi, M., et al. (2010). Assessment of the current situation and future outlooks for the pigeonpea sub-sector in Malawi. Nairobi: ICRISAT 1–52.
Singh, M., Manickavasagan, A., Shobana, S., and Mohan, V. (2021). Glycaemic index of pulses and pulse-based products: a review. Crit. Rev. Food Sci. Nutr. 61, 1567–1588. doi: 10.1080/10408398.2020.1762162
Singh, D., Mathimaran, N., Boller, T., and Kahmen, A. (2020). Deep-rooted pigeon pea promotes the water relations and survival of shallow-rooted finger millet during drought despite strong competitive interactions at ambient water availability. PLoS One 15:e0228993. doi: 10.1371/journal.pone.0228993
Singh, B., Singh, J. P., Shevkani, K., Singh, N., and Kaur, A. (2017). Bioactive constituents in pulses and their health benefits. J. Food Sci. Technol. 54, 858–870. doi: 10.1007/s13197-016-2391-9
Suara, K. O., Azubuike, C. P., Okubanjo, O. O., and Igwilo, C. (2016). Neutraceuticals and antibacterial properties of methanol extract of Plukenetia conophora (Müll.-Arg.) family Euphorbiaceae leaves and physical properties of its cream formulations. Nigerian journal of pharmaceutical and applied science. Research 5, 91–98.
Subbarao, G. V., Chauhan, Y. S., Johansen, C., and Johansen, C. (2000). Patterns of osmotic adjustment in pigeon pea-its importance as a mechanism of drought resistance. Eur. J. Agron. 12, 239–249. doi: 10.1016/S1161-0301(00)00050-2
Susmitha, D., Kalaimagal, T., Senthil, R., Vetriventhan, M., Anitha, S., Manonmani, S., et al. (2022). Calcium-rich Pigeonpea seed coat: a potential byproduct for food and pharmaceutical industries. Sustainability 14:4918. doi: 10.3390/su14094918
Talabi, A. O., Vikram, P., Thushar, S., Rahman, H., Ahmadzai, H., Nhamo, N., et al. (2022). Orphan crops: a best fit for dietary enrichment and diversification in highly deteriorated marginal environments. Front. Plant Sci. 13:839704. doi: 10.3389/fpls.2022.839704
Talari, A., and Shakappa, D. (2018). Role of pigeon pea (Cajanus cajan L.) in human nutrition and health: a review. Asian J. Diary Food Res. 37, 212–220. doi: 10.18805/ajdfr.DR-1379
Tapal, A., Vegarud, G. E., Sreedhara, A., and Kaul Tiku, P. (2019). Nutraceutical protein isolate from pigeon pea (Cajanus cajan) milling waste by-product: functional aspects and digestibility. Food Funct. 10, 2710–2719. doi: 10.1039/C8FO01933A
Tchiegang, C., Kapseu, C., and Parmentier, M. (2001). Chemical composition of oil from Tetracarpidium conophorum ((Müll. Arg.) Hutch. and Dalz.) nuts. J. Food Lipids 8, 95–102. doi: 10.1111/j.1745-4522.2001.tb00187.x
U.S. Department of Agriculture. (2022). Agricultural research service, food data central. Washington, DC: USDA.
Ulian, T., Diazgranados, M., Pironon, S., Padulosi, S., Liu, U., Davies, L., et al. (2020). Unlocking plant resources to support food security and promote sustainable agriculture. Plants, People, Planet 2, 421–445. doi: 10.1002/ppp3.10145
United Nations Department of Economic and Social Affairs, Population Division. (2022). World Population Prospects 2022: Summary of Results. UN DESA/POP/2022/TR/NO. 3 [cited as requested].
Upadhyaya, H. D., Pundir, R. P. S., Gowda, C. L. L., Reddy, K. N., and Singh, S. (2005). Geographical pattern of diversity for qualitative and quantitative traits in the pigeon pea germplasm collection. Plant Genet. Resour. 3, 332–352. doi: 10.1079/PGR200587
Upadhyaya, H. D., Reddy, K. N., Gowda, C. L. L., and Singh, S. (2010). Identification and evaluation of vegetable type pigeon pea (Cajanus cajan (L.) Millsp.) in the world germplasm collection at ICRISAT genebank. Plant Genet. Resour. 8, 162–170. doi: 10.1017/S1479262110000122
Wacal, C., Basalirwa, D., Okello-Anyanga, W., Murongo, M. F., Namirembe, C., and Malingumu, R. (2021). Analysis of sesame seed production and export trends; challenges and strategies towards increasing production in Uganda. OCL. 28:4. doi: 10.1051/ocl/2020073
Wall, M. M. (2006). Ascorbic acid, vitamin A, and mineral composition of banana (Musa sp.) and papaya (Carica papaya) cultivars grown in Hawaii. J. Food Compost. Anal. 19, 434–445.
Wei, P., Zhao, F., Wang, Z., Wang, Q., Chai, X., Hou, G., et al. (2022). Sesame (Sesamum indicum L.): a comprehensive review of nutritional value, phytochemical composition, health benefits, development of food, and industrial applications. Nutrients 14:4079. doi: 10.3390/nu14194079
Yang, S.-E., Vo, T.-L. T., Chen, C.-L., Yang, N.-C., Chen, C.-I., and Song, T.-Y. (2020). Nutritional composition, bioactive compounds and functional evaluation of various parts of Cajanus cajan (L.) Millsp. Agriculture 10:558. doi: 10.3390/agriculture10110558
Keywords: underutilized species, African walnut, African breadfruit, wild sesame, pigeon pea, food security, nutrition health
Citation: Nkwonta CG, Auma CI and Gong Y (2023) Underutilised food crops for improving food security and nutrition health in Nigeria and Uganda—a review. Front. Sustain. Food Syst. 7:1126020. doi: 10.3389/fsufs.2023.1126020
Edited by:
Beulah Pretorius, University of Pretoria, South AfricaReviewed by:
Tonna Ashim Anyasi, University of Greenwich, United KingdomSamuel Ayofemi Olalekan Adeyeye, Hindustan University, India
Copyright © 2023 Nkwonta, Auma and Gong. This is an open-access article distributed under the terms of the Creative Commons Attribution License (CC BY). The use, distribution or reproduction in other forums is permitted, provided the original author(s) and the copyright owner(s) are credited and that the original publication in this journal is cited, in accordance with accepted academic practice. No use, distribution or reproduction is permitted which does not comply with these terms.
*Correspondence: Chikere G. Nkwonta, c.nkwonta@leeds.ac.uk
†These authors share first authorship