- 1Laboratory of Ecology and Plant Science, School of Biosciences, Mahatma Gandhi University, Kottayam, Kerala, India
- 2School of Biosciences, Mahatma Gandhi University, Kottayam, Kerala, India
The arbuscular mycorrhizal fungi (AMF) are significant fertility-promoting microbes in soils. They enable soil fertility, soil-health and boost crop productivity. There are generalist and specialist groups among AMF in natural soils. Optimized use of specific AMF concerning crops and soils can improve agricultural sustainability. Thus, AMF is becoming an inevitable biological tool for improving crop productivity and soil health. Especially in the context of chemicalized agriculture undermining the sustainability of food security, safety, and human and ecosystem health, alternative agricultural means have become inevitable. Therefore, AMF has become essential in nature-friendly, organic agriculture. Of such farm fields, natural biological activity is enhanced to sustain soil fertility. Crops show increased innate immunity against pests and diseases in many such systems. Moreover, ecosystems remain healthy, and the soil is teeming with life in such farms. The primary goal of the review was a thorough critical analysis of the literature on AMF in organic agriculture to assess its efficiency as an ecotechnological tool in sustainable agricultural productivity. The novelty is that this is the first comprehensive review of literature on AMF concerning all aspects of organic agriculture. A vital systematic approach to the exhaustive literature collected using regular databases on the theme is followed for synthesizing the review. The review revealed the essentiality of utilizing specific mycorrhizal species, individually or in consortia, in diverse environmental settings to ensure sustainable organic crop production. However, for the exact usage of specific AMF in sustainable organic agriculture, extensive exploration of them in traditional pockets of specific crop cultivations of both chemical and organic fields and wild environments is required. Moreover, intensive experimentations are also necessary to assess them individually, in combinations, and associated with diverse beneficial soil bacteria.
1. Introduction
Food safety and security for a growing global population have always been crucial challenges for humans (UN Report, 2022). Chemicalized agriculture is not ensuring agricultural sustainability for the future (Ma et al., 2022) because it causes severe environmental challenges and biodiversity erosion (Bremmer et al., 2021; Lambertini, 2021), impacting soil, human, and ecosystem health. It has been causing soil degradation (FAO and ITPS, 2015; Liu J. et al., 2020; Pahalvi et al., 2021), accumulation of toxic metals (Sahodaran and Ray, 2018), and biocide residues in soils (Silva et al., 2019), affecting its productivity. Continued applications of agrochemicals in the fields cause pest resistance (Phillips, 2020), negatively impacting agricultural production's cost-effectiveness (Durham and Mizik, 2021). Severe water quality deterioration in chemicalized agricultural zones exists (Vera-Herrera et al., 2022). It also contributes to more than 10% of global greenhouse gas emissions (Chai et al., 2019), accelerating global warming. Thus, chemicalized conventional agriculture is challenging food security and safety in the future.
Sustainability in all spheres of human activities, including agriculture, is the focus of the UN's Sustainable Developmental Goals (SDGs) for the world (FAO, 2018; Sridhar et al., 2022). Accordingly, organic farming is emerging as an alternative means of productive and sustainable agriculture (Fitzpatrick et al., 2022; Jaacks et al., 2022). It also enables avoiding environmental impacts and toxicities of chemicalized farming (Amuji, 2022) and improving soil health (Tahat et al., 2020). The scientific world recognizes its potential to meet food security (Morshedi et al., 2017) and food safety (FAO, 2021). Organic agriculture also has the potential to address the crucial challenges of climate change (Cidón et al., 2021) because organic farming can promote excessive sequestration and conservation of carbon in soil systems (Page et al., 2020). Therefore, organic agricultural practice continuously grows globally (IFOAM and FiBL, 2021). Because of the current uprising of a green movement globally and the emergence of new food policies (European Commission Report, 2020) worldwide, the 'green food products' of organic agriculture will be the mainstream food ensuring the health of people (Mie et al., 2017; Ashaolu and Ashaolu, 2020) in the future. Therefore, a paradigm shift is becoming essential for the green organic movement (Runhaar, 2021).
The organic or natural means of soil fertility management include using organic fertilizers and fertility-aiding microbial communities (Yadav et al., 2013; Mohanty et al., 2021; Silveira et al., 2021). The quality and quantity of nutrients released from an organic fertilizer depend on the kind of fertilizers applied (Ye et al., 2020), the physicochemical nature of soils, and the existing biotic community within the same. However, the current low availability and high cost of organic fertilizers limit farmers from supplying sufficient nutrients to crops through organic means (Bergstrand, 2022). Moreover, unfavorable microbial activities in organically enriched soils may cause the leaching of NO3 into groundwaters (Bibi et al., 2016), denitrification, or acidification of soils (Wang J. et al., 2018), causing loss of soil fertility and environmental degradations. Therefore, achieving success in organic agriculture depends on continuous monitoring of the natural microflora and identification of the exact roles of each of them in maintaining soil health and fertility (Reyes-Sánchez et al., 2022). It also depends on our ability to manage the natural microflora in soils scientifically (FAO, 2022; Mrunalini et al., 2022) and use crop-friendly microbes as biofertilizers, which can be an alternative to chemical fertilizers. A judicious additional supply of desirable microorganisms as a biofertilizer (Yang et al., 2021) has become inevitable for the evolution of organic agriculture as a successful alternative mode of agriculture for the sustainability of human civilization (FAO, 2017). Among the microflora in soils, the AMF is the most significant group of organisms utilizable as biofertilizers (Chen et al., 2018; Fall et al., 2022).
The role of AMF in assisting the sustainability of soil fertility and enhancement of crop productivity is one of the most researched and well-known themes in Agronomy (Giovannini et al., 2020). The AMF form a particular category of organisms supporting plants in satisfying their thirst for water and mineral, especially for plants growing in nutrient-deficient systems (Kobae, 2019; Nanjundappa et al., 2019). The AMF positively supports other soil microbiota (Bhale et al., 2018). AMF are more affluent and influential in organic farm soils than chemicalized farms (Mullath et al., 2019; Barceló et al., 2020; Jiang et al., 2021; Huo et al., 2022). Moreover, chemicalized farms usually do not accommodate all the beneficial AMF, especially the sensitive species (Pontes-da-Silva et al., 2018). They are helpful in the upgradation of soil structure, aiding plants in their water relations, and improving heavy metal tolerance in plants (Zhang et al., 2019; Jadrane et al., 2021; Riaz et al., 2021). They aid plants in developing resistance against diseases through microbial-assisted molecular pathway-triggered immunity (Basu et al., 2018). Thus, the AMF is becoming an integral part of the successful functioning of organic farming and the maintenance of crop fields as a sustainable agroecosystem (Kuila and Ghosh, 2022). However, AMF cannot be effective in soils devoid of one or more nutrients. Some species of AMF, especially in specific environmental conditions, can also have adverse effects on host plants. Sometimes they affect soil seed bank viability (Maighal et al., 2016). Therefore, experimental studies have become inevitable in standardizing AMF involving organic agricultural practices (Ray and Valsalakumar, 2010a,b) in specific soils.
In the above contexts, a systematic, thorough critical review of literature has become essential to explore the current status of AMF as an inevitable component in organic agriculture for further intensification of investigations. Accordingly, the scope of AMF as a successful biofertilizer option for all the sustainable farming models, such as organic farming, eco-farming, and natural farming, which differ in certain aspects and concepts, are considered together in the review. Because all such farming options focus on the sustainability of food production, environmental quality, and improvement in public health (EPRS Report, 2016) by avoiding excessive direct use of chemicals in agriculture, and all such models consider natural means of soil fertility management and pest control (Stoleru and Sellitto, 2016; Rempelos et al., 2021) as the major components of agricultural production management. The significant goals included the identification of the gap and scope in AMF concerning organic agriculture in the existing literature. In addition, the review also focused on identifying the tasks ahead in applying specific AMF or their consortia, along with other biological components, as comprehensive alternatives to the chemicalized provision of nutrients for ensuring sustainable productivity in organic agriculture.
A standard critical approach (Smith et al., 2019; Nazari et al., 2020; Tully and McAskill, 2020) to the available exhaustive literature is followed in the preparation of this review. Specific keywords such as “Organic Farming,” “AMF and organic farming,” “AMF and soil structure,” “AMF and water relations,” “AMF and major nutrients,” “AMF and micronutrients,” “AMF and contaminated soil,” “AMF and carbon sequestration,” “AMF and agricultural sustainability,” “role of AMF in improving disease resistance in the crop,” “AMF and adverse effects” and the like are used to identify literature from electronic databases such as Clarivate Web of Sciences, Frontiers, EBSCO, Taylor and Francis, JSTOR, SciFinder, Cambridge University Press, Oxford University Press, Springer and the like for collecting the relevant literature. Since the literature on organic farming is available sufficiently from the 1980s, the literature collection period was fixed as 1989–2022. A similar comprehensive review on the topic (Ryan and Tibbett, 2008) appeared in 2008; however, it discusses the general significance of AMF in agriculture, not emphasizing the need for further research in AMF concerning organic farming. Therefore, the present review is highly imminent and essential.
Overall, the findings reveal that the available literature on AMF in organic farming belongs to various themes such as surveys of AMF in diverse natural and agricultural environments, experimental studies on the role of AMF in various crops under different farming practices, AMF and soil structure in organically managed soils, AMF as an agent of carbon accumulation or carbon cycling in organic farms, AMF as an agent for increasing disease resistance or overcoming stress in crops, AMF as an agent for significant nutrient supply in organic soils, water relations of crops, micronutrient enhancement, heavy metal tolerance, and adverse effects of AMF on organic crops. A critical summary of the details of the findings is discussed below.
2. The literature on AMF concerning organic farming
In general, there has been a continuous growth of literature on AMF concerning organic farming per year (Figure 1) in the past four decades (the 1980s to 2022). The Microsoft Excel-generated data map (Figure 2) shows the region-wide distribution of literature in the world, which reveals that more than 50% of the total publications are from three countries, China (28.37%), the USA (14.01%), and Italy (9.40%). The entire research data on “AMF in Organic Agriculture” collected from the “Web of Science” reveals that it is spread over 32 sub-disciplines. Among the five hundred and eighty-five research publications collected from over the 32 sub-disciplines in journals/books/websites, 248 relevant research articles are critically analyzed to synthesize the current review. Data analysis reveals that a significant share of research publications, 315 (53.84%) of the total 585 publications, belongs to the sub-discipline, Agriculture. This fact emphasizes the significance of AMF in organic agriculture.
3. Utility of AMF in organic agriculture
Ryan and Tibbett (2008) have thoroughly reviewed the significance of AM fungal benefits in organic agriculture. According to them, AMF improves crop growth and supports them in drought avoidance and disease control. They also contribute to long-term ecosystem sustainability by improving and maintaining soil structure, plant community structure, and diversity. Although AMF root colonization in crops is higher in organic farms than in conventional fields, the benefits may vary depending on crops, soils, geographic locations, and seasons. However, they cannot be substituted for fertilizer inputs. Moreover, the authors observe that the AMF flora in conventional chemicalized farm soils can sometimes be “weedy,” less beneficial, or not beneficial to crops. Therefore, the application of AMF in organic agriculture of specific crops, soils, and cultivation practices must be optimized for desirable results. However, researchers have also reported that although AMF in natural soils improves the quality of vegetables, inoculation with non-native mycorrhizal fungi can suppress native AMF in crops and negatively impacts the quality of vegetables (Mukherjee et al., 2020), even in organic agriculture. The same authors also observed that organic farming consistently improves disease tolerance in crops but cannot explain the role of AMF in the same.
Avoiding chemical fertilizers in fields can significantly improve the microbial diversity in soils, including that of AMF. However, the nature and amount of organic matter added to soils decide the abundance and diversity of microbes, including mycorrhiza in soils (Ma et al., 2018, 2022; Naorema et al., 2021). According to Liu L. et al. (2020), decreased availability of readily available phosphorus increases AMF activity in crop fields. Accordingly, while organic fertilizers with less readily available phosphorus trigger the abundance and diversity of AMF in soils, chemical fertilizers with more readily available phosphorus decrease the soil's AMF flora. A comparison of organic and conventional farming suggests that, in the long run, organic farming increases the species diversity of AMF (Kazeeroni and Al-Sadi, 2016; Gazdag et al., 2019) in such fields. In a review of the influence of organic farming systems on soil biodiversity, Leksono (2017) explains that organic agricultural activities cause an increase in AMF spore density and species richness in soils. In general, while organic farming contributes to an increase in the diversity of AMF, intensive soil management practices of conventional agriculture considerably diminish them (Manoharan et al., 2017).
In general, researchers view that AMF enhances NPK uptake by plants (Nadiah et al., 2020; Chandrasekaran, 2022; Shen K. et al., 2022; Shen M. et al., 2022). Thus, AMF assist plants in improving their biomass. AMF also help specific plants in their synthesis of particular metabolites (Trisilawati et al., 2019). Accordingly, AMF are well-known to have a direct positive influence on plant physiology or plant biochemical pathways. Igiehon and Babalola (2017), in their review on the role of biofertilizers in sustainable agriculture, emphasize the significance of AMF in improving the resistance of crops to diseases and tolerance to abiotic environmental stresses. Suja et al. (2017) also show that organically grown plants can ensure stable yield and product quality in crops such as Taro while keeping soil quality intact.
In general, the literature on the utility of AMF in organic agriculture is tremendous. Therefore, the exhaustive literature (46 articles) on various aspects of AMF in organic agriculture is systematically given in Table 1 below for a bird's eye view. The table reveals that the research on AMF in organic farming includes field surveys and field or other experimental studies. It is clear from the table that existing studies endorse AMF as an inevitable component of organic agriculture. It also reveals the significant gap between rigorous experimentations with crop-specific, climate, and soil-specific AMF application trials in the organic farming of diverse crops. Accordingly, exploration of the natural AM flora of specific crop fields in traditional crop belts, both chemicalized and organic, is also essential (Valsalakumar et al., 2007) for identifying the beneficial and weedy AMF for each crop. Then only experimental trials of judicious utilization of AMF in organic agricultural management can be decided.
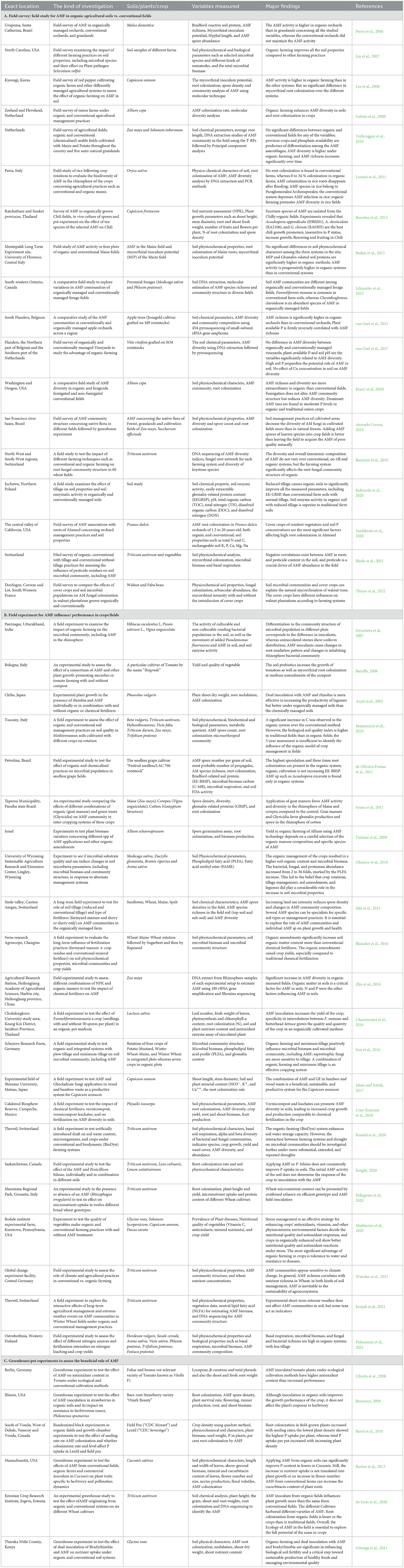
Table 1. Summary of reports on the benefits of organic agriculture, especially the beneficial roles of AMF, as appeared in the literature—a critical analysis.
4. AMF and soil structure
Soil structure is a “master quality” of soil (Ray, 1993; Ray et al., 1993), one of the most significant parameters of soil health. It is the outcome of the sum of biological activities in the soil of any natural environment (Rillig et al., 2017). A good structure with a high percentage of water-stable soil aggregates is a unique marker of organically managed natural fields. Biologically highly organized, structured soils are especially visible in organically enriched and non-chemicalized agricultural fields where the diversity of AMF is also relatively high (Marinho et al., 2019). The AMF directly contributes to good soil structural quality and maintenance in natural soils. Among the diverse factors contributing to soil structure formation, glomalin secretion from extraradical hyphae of AMF is a significant component (Muneer et al., 2020), which helps in soil binding.
Glomalin-related soil proteins (GRSP) are a range of glycoproteins released into soils by the hyphae and spore walls of AMF. It is one of the most extensively studied and thoroughly reviewed topics in AMF concerning soil structure. The hydrophobic and thermo-stable glomalin is released into the soil on AMF spores and degradation of hyphae. In a review of 25 years of research on the role of GRSP in soils, Irving et al. (2021) conclude that it cements and protects soil organic matter and particles as a water-repellent surface material, thus promoting soil aggregation (Figure 3). It is not only GRSP; soil organic matter and organic carbon, in general, also act as a general cementing material to contribute to soil aggregation (Yang et al., 2017). AMF enhances soil aggregate stability by regulating root exudation in plants (Lu et al., 2019).
In a review of the role of glomalin as a potential soil conditioner, Zou et al. (2016) explain the two fractions of GRSP as easily extractable (EE-GRSP) and difficultly extractable (DE-GRSP) kinds. According to them, the total GRSP, the sum of the two fractions, contributes to soil aggregation and influences soil-water relationships. Experiments have shown that the AM-induced production of GRSP and specific soil enzymes are valuable indicators of soil fertility. However, the quantity of such compounds in soils varies with phosphorus levels in soils (Wu et al., 2015).
A review of the significance of glomalin in soil reveals that it influences the permeability of soil surfaces due to their hydrophobicity leading to lesser water loss and better soil moisture conservation (Holátko et al., 2021). In general, AMF enables plants to overcome water stress through enhanced contribution to water-stable soil aggregation (Zhang et al., 2016). An experimental greenhouse study reveals that different AMF influence soil aggregation (Barbosa et al., 2019). However, in two locally adapted tree seedlings, pot experiments with three other AMF species such as Funneliformis mosseae, Glomus versiforme, and Rhizophagus intraradices, show their general influence, especially in causing a significant increase in EE-GRSP and soil aggregation (Zhang et al., 2019). Thus, AMF helps the ecological restoration of many mining-affected sites by contributing to the rise in soil macro aggregates, pore sizes, and an enhanced adhesion of metals in the roots (Zhang et al., 2021).
Singh et al. (2020) observe that it is crucial to keep GRSP production high in soils due to its significance in maintaining soil structural quality in a review. Interaction between plant, soil, and AMF determines the GRSP content in cultivated fields. Therefore, the strategy to improve soil structural quality requires the application of suitable AMF and crops with high root exudates that promote better AMF growth in soils. Accordingly, organic agriculture using appropriate AMF is highly relevant in the reclamation of barren soils, primarily industrially and chemicalized agriculturally degraded soils, to ensure food security and safety for a sustainable human future on the earth. Moreover, experimentation on specific AMF-involved improvement in soil structure has also become highly significant.
5. AMF and carbon sequestration
The role of AMF in carbon sequestration in field soils, especially in Maize-cultivated coal-field soils, is an experimentally proven fact (Wang et al., 2016). The activity of AMF in regulating the delivery and flux of C by variably influencing C sequestration and decomposition, especially by leaving a more significant amount of recalcitrant residual mass of chitinous cell wall, is already thoroughly reviewed (Parihar et al., 2020). According to Agnihotri et al. (2022), GRSP released into the soil by the AMF can prolong the mean residence time of C in soils, which also enables the formation of water-stable soil aggregates that prevent soil degradation, enhance organic matter stabilization, and the like. Plant carbon input into the soil through the shoot, root, and mycorrhizal pathways by C3 and C4 grasses is an experimentally proven fact (Huang et al., 2021). Experimental studies reveal that AMF significantly increases rhizo-carbon stabilization in soil macroaggregates (Jeewani et al., 2021). Zhang et al. (2016) also emphasize the importance of AMF in soil carbon stabilization and sequestration in semiarid steppe ecosystems, especially in the wet seasons. Thus, there is sufficient evidence in the literature to emphasize that AMF is inevitable to C accumulation and C-cycling in cultivated fields, especially in organic farms. However, the role of AMF and involvement in optimum C maintenance in agricultural fields remain open questions for further experimental investigations.
6. AMF and disease resistance in crops
The AMF can support plants in getting easy access to nutrients, even from nutrient-deficient or otherwise environmentally stressed soils (Fard et al., 2020). Naturally, plants with proper AMF associations in the root grow healthier than those without such symbionts in their roots (Dobo, 2022). Thus, even in stressful environments, AMF enables plants to develop better disease resistance. Diagne et al. (2020) state that AM fungi are crucial in building disease resistance and overcoming crop biotic and abiotic stress. An increase in disease resistance is one of the significant positive roles of AMF symbiosis to the plant partner, either by aiding in the uptake of nutrients or helping plants compete for nutrients, thereby enhancing photosynthetic productivity and even antibiosis.
In field experiments, it has been shown that AMF species such as Glomus hoi, Clariodeoglomus clareoideum, and Glomus mossae can provide various defensive benefits to host populations against fungal pathogens such as Powdery mildew (Eck et al., 2022). Similarly, Glomus sp. has been shown to improve resistance to damping-off disease in Cucumber plants caused by Rhizoctonia solani (Aljawasim et al., 2020). Pre-inoculation of F. mosseae can enhance resistance to early blight in Tomato (Song et al., 2015). Root colonization by AMF systematically increases defense-related enzymes and genes in the leaves of tomatoes upon the pathogen challenge. The AMF Rhizophagus irregularis has also been shown to enhance plant resistance against the fungal pathogen Fusarium oxysporum in Tomato plants (Wang et al., 2022). Molecular studies have revealed that AMF proteins such as ATL 31 and SYP 121, and Callose synthase PMR4 are higher in AMF colonized plants, which play a vital role in the priming of Callose accumulation and acting as an induced resistance mechanism in different plant species against fungal plant pathogens such as Botrytis cinerea (Sanmartín et al., 2021).
An experimental study has shown that among the environmental factors, light intensities have a significant influence on mycorrhizal association as well as mycorrhizal-induced disease resistance in plants (De La Hoz et al., 2021). However, when different varieties of Rice are inoculated with AMF, such as Funneliformis mosseae or Rhizophagus irregularis, the plant response to productivity and resistance to blast disease vary between different rice cultivars (Campo et al., 2020), which indicates the need for case by the case study of AMF utilization for disease resistance in Rice.
Mycorrhizae have been shown to add effective disease resistance to avirulent and virulent bacterial and fungal pathogens in tomatoes (Fujita et al., 2022). Moreover, the activation of both Salicylic acid and Jasmonic acid-mediated signaling pathways are enhanced in the primed plant by AMF colonization in the crops. In a review, Kadam et al. (2020) establish that mycorrhizal-induced disease resistance results from a synergistic involvement of AMF-enhanced physiological, phytohormonal and metabolic changes in plants.
Hohmann and Messmer (2017), in a review, have explored the plant genotype-related mycorrhizal responsiveness in crops. They have emphasized the need for an organic breeding program and novel breeding tools, which are essential to use plant-microbe interactions during crop selection efficiently. Similarly, Khrieba and Publishers (2019), in a review of the role of AMF in plant nutrition and protection from pathogens, concludes that AMF root colonization is well-known to improve plant resistance and tolerance to biotic stresses. According to the author, the mechanism underlying the processes are three-fold: (1) providing a physical barrier to pathogens, (2) competing with the pathogen, and (3) producing secondary metabolites, aggressive chemicals and toxins, and the like. The amount and the type of root exudates secreted by the plants also affect the mycorrhizal establishment.
Overall, a critical review of the existing literature establishes that AMF significantly improves crop disease resistance. Therefore, AMF are inevitable to the emergence of sustainable organic agriculture. However, detailed research concerning AMF and disease resistance in specific crops and soils under every climatic zone of organic and chemicalized cultivation regimes against specific pathogens has become essential for enabling the application of AMF as an alternative to toxic chemicalized disease control agents in the successful conduct of organic agriculture.
7. AMF and the macro-nutrients
The importance of AMF for sustainable nutrient availability in organic farming is a well-reviewed topic (Ebbisa, 2022). Mycorrhizal assistance, especially AMF, is essential for sustainable soil availability of nutrients and water to crops in ecological and organic farming (Ortaş, 2018). The success of organic agriculture in the future depends on how intelligently we use highly mycorrhizal host crops in rotations (Njeru, 2018). Moreover, the use of exotic and indigenous mycorrhizae has a decisive role in determining the future of modern agriculture (Bhantana, 2021). According to Ortas and Rafique (2017), the AMF has the unique capacity to extract NPK beyond the rhizosphere of plants and to release the same into host plants using many expressions of plant transporters. However, the amount of nutrient allocation between the host and the AMF symbiont is a fundamental factor facilitating the exchange and allocation process, which should be further explored. The AMF addition augments the capacity of Wheat to have sufficient nutrients from phosphorus-low soils and thus, enhances the crop's yield (Ortas and Bykova, 2018). In general, AM fungi are crucial in increasing the growth and yield of many crops by reducing the need for hazardous pesticides and industrial chemical fertilizers in agriculture (Diagne et al., 2020).
The AMF can aid in organic farming, even in poorly developed soils. The synergistic application of an extensive range of plant growth-promoting bacteria, AM fungi, and the like has become a common practice to enhance nutrient uptake (Ray and Valsalakumar, 2010a) in crops. Even in poor calcareous soils, AMF causes an improvement in the uptake of immobile mineral nutrients present in low concentrations (Lombardo et al., 2020, 2021). A combination of green compost and mycorrhiza improves the uptake of N, P, K, and Ca by Alfalfa crops in overexploited poor Mediterranean soils (Ben-Laouane et al., 2021). Specific studies on different compost systems in organic farming on two species of AMF reveal that AMF activity, especially that of Funneliformis mosseae, is not affected by the other compost systems (Akpinar et al., 2019). According to these authors, AMF species such as Funneliformis mosseae is better than Claroideoglomus etunicatum in the significant nutrient uptake in Allium porum L. In low-input cropping systems of Sesame crops, soil characteristics profoundly influence the composition and species richness of AMF in the fields (Harikumar, 2015). However, further intensive and extensive research on AMF application in diverse environmental settings using different crop varieties is essential in standardizing specific AMF as suitable biofertilizers in organic agriculture. The correlation between AM fungal root colonization and an increase in root density of crops still needs to be adequately correlated under optimal nutrient conditions (Cockerton et al., 2020).
Some of the recent experimental studies reveal some controversial findings also. For example, an experimental field comparison of organic and conventional farming methods shows that compared to traditional farming, organic farming causes more variability in nutrients, antioxidants, and vitamin C contents in diverse crops, and especially, the AMF inoculation results in a reduction in the vitamin C, antioxidants and phytonutrients in vegetables (Mukherjee et al., 2020). According to these authors, there can be potential antagonistic and synergistic interactions between the native and introduced AMF species, which require a period of stabilization. Therefore, long-term studies with more vegetables are essential to establish the exact beneficial role of specific species of AMF in the organic farming of vegetables. In the absence of chemical fertilizers in organic soils, better availability of mineral nutrients, including nitrogen, can be ensured by applying suitable AMF inoculation. In general, the review emphasizes the need for more investigations on soil and crop-specific applications of AMF in specific soil environments under organic farming practices for using the same as an agent for augmenting primary nutrient supply to crops.
8. AMF concerning water relations in soils
The AM fungi generally play a positive role in plant water relations, especially in stressed soil environments, which is also a reviewed topic (Tyagi et al., 2018). According to the authors, although there is significant progress in understanding the role of AMF in helping drought tolerance, ‘omic' data is essential to elucidate the actual mechanisms of AMF-assisted drought tolerance in plants. Experimental studies reveal that AM fungal association can increase net photosynthetic rate in water deficit conditions, which can be related to higher water availability for transpiration (Quiroga et al., 2019). The authors suggest that AMF can enhance the water permeability of root cells to overcome the water deficit.
Field experimental studies reveal that farming practices, especially organic farming, decide the kind of AMF community composition rather than its diversity in crop fields (Kozjek et al., 2021). They reveal that the AMF community can cope with short-term droughts in the field. However, according to Wahdan et al. (2021), the interaction between farming practice and climate decides AMF community composition in the fields. The crops can resist drought using a corresponding change in the AMF community structure in soils, especially in organically managed fields. However, long-term field experiments reveal that experimental drought promotes AMF abundance up to three times higher in organically cultivated fields under biodynamic fertilization than in conventionally managed farms (Kundel et al., 2020). The authors endorse the significance of AMF-assisted biodynamic organic farming for better resistance of crops to overcome the scarcity of water in the soil.
In vegetable crops, AMF supplementation enables overcoming water stress, especially in organic systems. Vegetable crops in a water-stressed organic field environment contain more antioxidants than those under conventional field systems (Mukherjee et al., 2020). The reduced tillage practice followed in organic farming is beneficial in improving the water relations of crops in organic fields (Kobierski et al., 2020). The enhanced water retentions under a continuous crop cover in organically managed soils conserve soil moisture better than in conventionally managed systems, which adds stability to the soil microbiome (Peltoniemi et al., 2021). The authors view that the absence of chemical fertilizers in such an organic system promotes the maintenance of a high diversity of AMF.
The AMF, along with other microbes in an organic environment, form a unique biotic community that contributes to the high quantity of microbial residues such as GRSP in soil, which improves soil aggregate stability to a considerable extent that ultimately can improve the general water regime of the soils (Sun et al., 2016). The AMF-inoculated soils usually have higher soil water potential (Zou et al., 2014). The GRSP secreted into soils by the AMF acts as a drought tolerance mediator that helps produce leaf abscisic acid, indole-acetic acid, and methyl jasmonate, stress mediators (Chi et al., 2018) in plants. The AM inoculation in organic fields enables plants to absorb water and other nutrients from the soil solution through their widespread extraradical hyphae, allowing plants to avoid drought stress (Posta and Hong Duc, 2020). In general, sufficient evidence is available in the literature on the importance of AMF as an inevitable component in organic farming to ensure sustainable management of optimum water utilization of crops in the field. However, intensive experimental studies are essential in optimizing water availability to specific crops in specific soils concerning specific AMF.
9. AMF and micronutrient availability
Mycorrhizal assistance in improving micronutrient uptake in crops is a reviewed topic. The AMF is significant to several plants' absorption of diverse micronutrients (Upadhayay et al., 2019). Nursery inoculation of tomato seedlings with specific AMF can enhance the absorption of nutrients, including the micronutrients such as Mn and Fe, in the crop under salt stress (Balliu et al., 2015). Thus, it enables the crops to overcome stress. According to Coccina et al. (2019), AMF can significantly aid the biofortification of Zinc in barley and Wheat. The authors suggest that AMF assists plants in absorbing micronutrients, even from soils low in the quantity of such micronutrients. Thus, they enable an improvement in the quality of organic foods. In general, experimental studies reveal that mycorrhiza-inoculation can improve yield in Wheat, even in P-deficient soils, by helping the plant to have sufficient P and other micronutrients such as Zinc (Zn), manganese (Mn), copper (Cu), and iron (Fe) from soils (Ortas and Bykova, 2018). The Zinc iron permease protein (ZIP) transporters help acquire Zinc by AM fungi and assist crops in getting sufficient nutrients from soils (Ruytinx et al., 2020). According to the authors, such fungi regulate the expression of some zinc transporters and genes involved in primary metabolism that supply zinc to host plants. The metal transporters are generally located in the extraradical mycelium, especially in Glomus intraradius (Wang, 2017; Wang et al., 2017). In addition to supporting nutrient access from nutrient-deficient soils, the AMF also helps absorb mineral nutrients from an inaccessible organic substrate, mineral particles, and rock surfaces (Teotia et al., 2017). Experimental evidence suggests that specific species of AMF support crops such as grapes in absorbing micronutrients like Zn from soil (Aslanpour et al., 2019).
Overall, a critical analysis of the available literature on the role of AMF in the absorption of micronutrients by plants reiterates that by employing suitable mycorrhiza species for specific crops and soils, micronutrient availability in the organic field can be assured. Thus, applying suitable AMF in organic fields can enhance the productivity and quality of organic produce. However, there is a high scope for experimenting with specific AMF in specific soils concerning the accumulation of particular micronutrients in diverse crops under different climate regimes. Such experiments can optimize environmental conditions for applying specific AMF as an alternative to micronutrient deficiency in organic farming.
10. Heavy metal tolerance and AMF
AMF-induced heavy metal tolerance in organic crops is a highly researched topic, already being reviewed from different perspectives (Begum et al., 2019; Gong and Tian, 2019; Dhalaria et al., 2020; Riaz et al., 2021). Although some heavy metals such as Fe or Cr are helpful to plants in traces, most of the heavy metals in soils act as severe abiotic stress that causes the formation of reactive oxygen species in the cells (Jalmi et al., 2018). According to these authors, plants respond to heavy metals through specific stress-related protein molecules to tackle metal toxicities. Some heavy metals are naturally present in all soils, but toxic heavy metals accumulate in soils through anthropogenic means such as chemicalized agriculture (Sahodaran and Ray, 2018). Chemical fertilizers are notorious for heavy metal contaminants such as Cd, Cr, Ni, Pb, and the like (Alengebawy et al., 2021). Continuous use of such contaminated chemical fertilizers in the field can lead to a gradual accumulation of metals in the soil over years of usage (Adhikari et al., 2021). In general, toxic heavy metals affect soil health by directly influencing the microbial flora of the soil and soil structure (Chu, 2018; Jarosławiecka and Piotrowska-Seget, 2022).
Heavy metals, on absorption into plant cells, modify the biochemical, metabolic, and physical pathways demonstrating reduced growth accompanied by yield and productivity (Srivastava et al., 2017). Therefore, organic agriculture that avoids harmful chemical fertilizers can be an excellent alternative farming method to overcome the crisis of heavy metal accumulation in the fields. Such a benefit of organic farming is reflected in organically grown vegetables, which have significantly lower concentrations of heavy metals than those raised by chemicalized means (Głodowska and Krawczyk, 2017). Janeeshma and Puthur (2020) reveal that the AM-mediated phytoremediation of heavy metals in soils is a well-established theme. The authors conclude that the AMF association enhances plants' primary metal tolerance mechanisms because AMF either directly absorb metals onto the hyphal wall or, through GRSP secretions, binds the heavy metals with the soil and makes them non-available to plants, thus improving plant tolerance to heavy metals in soils.
Riaz et al. (2021) have reviewed AMF-induced heavy metal tolerance in plants. According to them, enzymatic and non-enzymatic mechanisms are active in the heavy metal tolerance in plants. The AMF triggers antioxidant enzymatic protective systems in the host plants on soil exposure to heavy metals. Abdelhameed and Metwally (2019) explain how AMF protects Trigonella sp. against Cd stress, especially by lowering the damaging effects on plant development through enhanced activation of antioxidant enzymes. Thus, the fungus diminishes the detrimental influences of Cd on plant physiology. Gong and Tian (2019) explain in a review the mechanism of AMF action in alleviating heavy metal impact. They reveal the direct contributions of AMF in enhancing metal tolerance by immobilizing heavy metals through direct binding to the cell wall or chelation of the metals in soils by hyphal secretions of glycoproteins. However, according to them, the indirect contributions of AMF in metal tolerance in plants include diverse processes such as inducing the expression of regulatory genes to resist metal stress, regulation, and expression of antioxidant enzymes and the like.
Begum et al. (2019), in a review, explain the role of AMF in plants growing in stressful environments. According to the authors, AMF can be helpful for plants in effectively combatting diverse environmental stresses such as salinity, drought, nutrient stress, alkali stress, cold stress, and extreme temperatures. The authors believe future research should focus on identifying genes and gene products controlling the AMF-mediated growth regulation of plants in stressful environments. Dhalaria et al. (2020), in a review, explain how the AMF acts as a potential agent to avoid metal tolerance in soils. According to them, the AMF is the most promising and effective strategy to overcome heavy metal stress in plants. In certain species of AMF, even the spores accumulate heavy metals such as lead (Salazar et al., 2018) and reduce their availability in soils for plants to absorb.
The AMF also reduces the heavy metal impact in plants such as soybean through a dilution effect on the crop, ensuring the prevention of metal intake (Spagnoletti and Lavado, 2015) or a hyphal exploration of unstressed parts of the soil to avoid metal accumulation in plants (Garg et al., 2017) as a means of avoidance of metals in soils. The study of an orphaned mining site revealed that many Glomeromycota communities survive in these areas and at extreme metal toxicity levels (Sánchez-Castro et al., 2017). Thus, AMF is a handy tool for cultivating crops in heavy metal-contaminated soils, primarily if maintained organically with a high diversity of AMF in soils. However, the AMF cannot degrade the heavy metals in soils. It can only help plants prevent the availability of such metals, and the metal would remain in the system. Moreover, there is evidence in the literature regarding AMF assistance in accumulating heavy metals in plants (Gavrilescu, 2021; Ai et al., 2022; Chen et al., 2022; Szada-Borzyszkowska et al., 2022). Therefore, intensive experimental studies are required to identify and standardize the use of metal-stress-alleviating species of AMF concerning specific crops and soils to overcome plant metal stress in heavy metal-contaminated fields.
11. Adverse effects of AMF in organic agriculture
In general, AMF determines plant community structure, while plant community determines the kind of AMF in a vegetation system (Horn et al., 2017). However, according to the authors, the AMF community in a field or natural system also has independency, which is the outcome of interactions among them in the soil. Previously, researchers (van der Heijden et al., 1998; Lin et al., 2015) viewed interactions in the plant characteristics, including plant functional groups and life histories, as significant predictors of AMF in a plant community. According to them, mycorrhizal dependency determines which plant species dominates vegetation. Moreover, plant-plant interactions in a community are also influenced or decided by the mycorrhizal species in the system (Hoeksema, 2005). In such a situation, unlike monocropping systems, adding a non-native AMF into the soil can have unpredictable outcomes in the community structure, especially in a usual multi-cropping organic farming system.
Experimental studies reveal that in farm fields, in the presence of suitable AMF species, AMF-dependent crops are positively influenced (Kim et al., 2017). In contrast, not all weeds are negatively affected by AMF (Veiga et al., 2011) or vice versa. However, if favorable to weeds, such differential influential roles of AMF on crops and weeds can sometimes negatively affect weed management in agricultural systems, mainly when properly monitored generalized AMF biofertilizers are applied to diverse crop fields.
The harmful effect of AMF on specific species, such as Eucalyptus seedlings, especially those growing in soils deficient in certain minerals such as iron (Janos et al., 2013), is known. The positive influences of AMF on the growth and competence of invasive species such as Rudbeckia lacinata and Solidago gigantea in the natural environment are also well-known (Majewska et al., 2017). Such findings point out that in natural vegetation or cultivated fields, the addition of a new AMF species can have a significant differential influence on species diversity, crop performance, and enhancement in the competitive capacity of invasive species, depending on the specific AMF community in a soil or plant species occupying the area. Thus, introducing an alien species of AMF, even as a biofertilizer, can also harm the community structure of the natural ecosystem, such as an aggressive weed outbreak. Therefore, specific AMF application needs to be judiciously practiced with exact information on their influence on all the species in a natural community or crops and weeds in agricultural systems. Especially in global climate change, the plant-soil biotic interactions can lead to specific unexpected dimensions in crop response to soil factors from ecologically ignorant AMF management in soils (Sendek et al., 2019). Notably, adding an AMF species in organic farming can create community imbalances in agriculture or adjacent natural ecosystems. Currently, organic farms, in general, are multi-cropping systems (Carof et al., 2022; Fernández et al., 2022; Trinchera et al., 2022) that use a high diversity of natural crops and other flora together in the sustainable management of soil fertility and crop productivity.
AMF application can also have adverse impacts on nutrient uptake in plants. Plants in organic farms rich in AM fungi in soils may compete for nitrogen acquisition, and such a contest can reduce the grain yield of maize, especially in N-limiting soils (Wang X. X. et al., 2018). The mycorrhizal dependence of plant species is its constitutive property, and different plant species may react differently to the same mycorrhizal fungus species (Heklau et al., 2021). Plants are regarded as facultatively or obligately mycotrophic. Moreover, the presence of AMF in some plants decreases their tolerance to adaptive traits, such as tolerance to herbivory (Garrido et al., 2010). The above critical review of the literature on the negative influence of AMF on organic agricultural management thus, reveals that while the AMF can be beneficial to organic farming, sometimes the same will have adverse effects on the organic cultivation of specific crops and the stability of natural ecosystems. Therefore, particular studies involving specific plant-fungus species-related experimental works, along with field surveys of AMF in cultivated fields and natural ecosystems, are essential to a better understanding the correct ecological management of AMF in organic farming systems.
12. The future perspectives of AMF concerning organic agriculture
Although chemicalized agriculture affects food security, safety, and sustainability through its negative impact on soil systems (Ouyang and Norton, 2020; Vågsholm et al., 2020; Bisht and Chauhan, 2021), organic farming as a definite alternative to sustainability has not yet been sufficiently rationalized. Experiments reveal that chemical fertilizer usage can be avoided or reduced to a certain extent if specific AMF is used for specific crops in particular soil systems (Begum et al., 2019; Trejo et al., 2021; Dobo, 2022; Kuila and Ghosh, 2022). The future perspective of organic farming as a sustainable agricultural model emphasizes a learned accommodation of the natural microbial biodiversity components, including AMF, in a naturally favorable organic soil environment for ensuring self-sustenance of soil fertility (Tscharntke et al., 2021; Sietz and Neudert, 2022; Uwamungu et al., 2022). Therefore, organic farming as prospective agriculture to rejuvenate the lost natural vigor of our land resources and biodiversity needs further standardization.
Rational and judicious application of crop and soil-specific AMF species is inevitable as biofertilizers in developing future sustainable organic agricultural (Trisilawati et al., 2019) production systems. However, the successful adaptation of mycorrhizal aid in plant growth and ecosystem services requires proper monitoring, knowledge about the effects of agricultural amendments, organizing database tools, good plant breeding, and myco-engineering of the associated microbiota (Rillig et al., 2016; Silva-Flores et al., 2022). In this context, a herculean research task is ahead in utilizing AMF as an ecotechnological tool for agricultural sustainability through organic farming.
The future research prospects include specific surveys of natural mycorrhizal associates of diverse crops in different climatic and soil zones (Pellegrino et al., 2015; Nidheesh et al., 2018) of the world. Along with the traditional spore-based taxonomic methods (Prayudyaningsih et al., 2019), molecular metagenomic approaches to identify the species (Eun Kang et al., 2020; Alrajhei et al., 2022) have become inevitable to fulfill this task in the future.
An extensive and intensive survey of natural mycorrhizal flora needs to be followed by intensive experimental trials at laboratory and field scales (Sahodaran et al., 2019; El Hilali et al., 2022; Ouledali et al., 2022; Wang et al., 2023) to standardize specific strains in specific soils over definite seasons in diverse climatic zones. It is essential to identify specific AMF for particular crops under climate change conditions (Jerbi et al., 2020; Kozjek et al., 2021), primarily to ensure proper application of the same in sustainable organic cultivation systems. In developing AMF as a crop-specific biofertilizer, multiple mycorrhizal combinations that are genetically distant are required (Chen et al., 2017; Crossay et al., 2019), especially for supporting specific crops in specific soil environments and climatic zones.
Identifying the genes and transcription factors of both AMF and host plants that enable the AMF to react to diverse environmental stresses has become an essential task for researchers in this field for the future (Zou et al., 2020; Sun et al., 2022). Such investigations would enable the development of mycorrhizal partnerships with a maximum positive effect on host plants (Guo et al., 2021; Grünfeld et al., 2022). Further studies for identifying generalist and specific species of AMF suitable for diverse organic crop systems concerning different climate and soil systems have become essential. Moreover, an adequate understanding of their crop-supportive processes is inevitable in utilizing mycorrhiza as a definite agent of sustaining soil fertility in organic agricultural systems (Juntahum et al., 2022; Kuila and Ghosh, 2022).
13. Conclusion
The current critical analysis of literature on AMF concerning organic agriculture reveals that there has been a continuous growth in research on the same over the last four decades (1980–2022). Still, more than 50% come from three countries, China, the USA, and Italy. It emphasizes the need for more global research efforts in this regard. However, the existing literature on the application of AMF in organic farming reveals that the AMF has the potential to emerge in organic farming as a successful alternative to chemicalized agriculture for the sustainability of food security and safety in the future. Specific analysis of literature under the different heads reveals the following particular points of view in this regard.
1. Although AMF root colonization is higher in organic crops than in chemicalized farms, the existing research does not recommend AMF as a substitute for chemical fertilizer inputs.
2. The AMF flora in a chemicalized farm can be weedy, whereas their presence in natural organic soils benefits crops in many ways.
3. The exact diversity of AMF flora concerning most crops and natural soils is yet to be explored.
4. Intensive soil management practices of chemicalized agriculture destroy natural AMF flora in soils.
5. The successful application of AMF in organic farms as a crop-beneficial input needs optimization of the application concerning cultivation practices, which demand intensive experimental studies on AMF concerning specific varieties of diverse crops, diversity of soil factors, climate, and other species of plants in the field.
6. AMF application in organic farms enables improvement in soil structure through direct physical binding of soil particles, stimulation of root exudation, and secretion of glomalin-related soil proteins as soil cementing components.
7. The GRSP and soil enzymes can be valuable indicators of soil fertility.
8. Specific AMF applications in barren soils can enable its reclamation.
9. The AMF significantly contributes to carbon sequestration and carbon stabilization in soils.
10. AMF application can improve the quality of plant food through an enhancement in their useful biochemical components, including secondary metabolites.
11. AMF application in organic farming can considerably improve disease resistance in crops.
12. AMF in organic farms improves disease resistance by providing a physical barrier to root pathogens, acting as a competitor, or stimulating the plants to produce defensive secondary metabolites against pests.
13. AMF in organic soils, in conjunction with other PGPRs, can assist plants in accumulating sufficient macro and micronutrients, especially in nutrient-deficient soils.
14. AMF enables plants to thrive in water-deficit soils by promoting increased water accumulation potentials of the host plants.
15. The AMF secreted GRSP act as a mediator for drought tolerance in plants.
16. The AMF in organic soils enable the plants to overcome heavy metal stress by making the metals non-available to plants, either by directly binding the metals on their cell walls or storing them in their spores or indirectly by binding them to soil particle through GRSP.
17. Non-judicious application of the generalist AMF species into fields sometimes becomes more favorable to weeds than the crops.
Overall, the literature analysis reveals that the AMF has yet to become an economically successful alternative to chemical fertilizers in organic farming, which is possible only through the proper acquisition of knowledge on the rational application of specific AMF concerning soils, climate, crops, varieties, and cultivars through further field surveys and experiments. Such rigorous field studies and experimentations with crop-specific and climate and soil-specific species of AMF concerning specific crops under various organic regimes alone can generate sufficient information to transform organic farming into a rational alternate agricultural process to ensure sustainability. Generally, it has become imperative to standardize AMF as a biofertilizer in mono and multi-cropping agricultural organic farming. Therefore, globally focused research on AMF concerning organic agriculture as a precise rational agricultural means has become essential for global food security and safety for the future.
Author contributions
NG collected the literature per the plan and directions of JR. NG and JR analyzed the literature. NG prepared the first draft. JR critically analyzed and revised the same and both finalized the content and format. Both authors contributed to the article and approved the submitted version.
Acknowledgments
The authors express their sincere gratitude to the Mahatma Gandhi University Library online system for an extensive search of the literature and for accessing and downloading sufficient valuable literature for the preparation of this review.
Conflict of interest
The authors declare that the research was conducted in the absence of any commercial or financial relationships that could be construed as a potential conflict of interest.
Publisher's note
All claims expressed in this article are solely those of the authors and do not necessarily represent those of their affiliated organizations, or those of the publisher, the editors and the reviewers. Any product that may be evaluated in this article, or claim that may be made by its manufacturer, is not guaranteed or endorsed by the publisher.
References
Abdelhameed, R. E., and Metwally, R. A. (2019). Alleviation of cadmium stress by arbuscular mycorrhizal symbiosis. Int. J. Phytoremed. 21, 663–671. doi: 10.1080/15226514.2018.1556584
Adhikari, T., Gowda, R. C., Wanjari, R. H., and Singh, M. (2021). Impact of continuous fertilization on heavy metals content in soil and food grains under 25 years of long-term fertilizer experiment. Commun. Soil Sci. Plant Anal. 52, 389–405. doi: 10.1080/00103624.2020.1854290
Agnihotri, R., Sharma, M. P., Prakash, A., Ramesh, A., Bhattacharjya, S., Patra, A. K., et al. (2022). Glycoproteins of arbuscular mycorrhiza for soil carbon sequestration: Review of mechanisms and controls. Sci. Total Environ. 806, 150571. doi: 10.1016/j.scitotenv.2021.150571
Ai, Y. J., Li, F. P., Yang, J. Q., Lu, S., and Gu, H. H. (2022). Research progress and potential functions of AMF and GRSP in the ecological remediation of metal tailings. Sustainability 14, 9611. doi: 10.3390/su14159611
Akpinar, C., Demirbas, A., and Ortas, I. (2019). The effect of different compost compositions on arbuscular mycorrhizal colonization and nutrients concentration of leek (Allium porrum L.) plant. Commun. Soil Sci. Plant Anal. 50, 2309–2320. doi: 10.1080/00103624.2019.1659299
Alengebawy, A., Abdelkhalek, S. T., Qureshi, S. R., and Wang, M. Q. (2021). Heavy metals and pesticides toxicity in agricultural soil and plants: ecological risks and human health implications. Toxics 9, 42. doi: 10.3390/toxics9030042
Aljawasim, B. D. G., Khaeim, H. M., and Manshood, M. A. (2020). “Assessment of biocontrol potential of arbuscular mycorrhizal (Glomus spp.) against damping-off disease (rhizoctonia solani) on cucumber,” in Mycorrhizal Fungi—Utilization in Agriculture and Forestry, ed R. Radhakrishnan (London: Intech Open).
Alrajhei, K., Saleh, I., and Abu-Dieyeh, M. H. (2022). Biodiversity of arbuscular mycorrhizal fungi in plant roots and rhizosphere soil from different arid land environment of Qatar. Plant Direct 6, e369. doi: 10.1002/pld3.369
Amuji, C. F. (2022). Organic farming as a safe alternative to toxic synthetic chemicals usage in tropical crop production. Chem. Proc. 10, 52. doi: 10.3390/IOCAG2022-12190
Aryal, U. K., Xu, H. L., and Fujita, M. (2003). Rhizobia and am fungal inoculation improve bean plants' growth and nutrient uptake under organic fertilisation. J. Sustain. Agric. 21, 27–39. doi: 10.1300/J064v21n03_04
Ashaolu, T. J., and Ashaolu, J. O. (2020). Perspectives on the trends, challenges, and benefits of green, innovative and organic (GSO) foods. Int. J. Gastron. Food Sci. 22, 100273. doi: 10.1016/j.ijgfs.2020.100273
Aslanpour, M., Baneh, H. D., Tehranifar, A., and Shoor, M. (2019). Effect of mycorrhizal fungi on macronutrients and micronutrients in the white seedless grape roots under drought conditions. Int. Trans. J. Eng. Manage. Appl. Sci. Technol. 10, 397–408. doi: 10.14456/ITJEMAST.2019.39
Azevedo Correa, E. J. (2019). Arbuscular mycorrhizal fungi and farm management practices. World J. Agric. Soil Sci. 2, 1–10. doi: 10.33552/WJASS.2019.02.000534
Baird, J. M., Walley, F. L., and Shirtliffe, S. J. (2010). Arbuscular mycorrhizal fungi colonisation and phosphorus nutrition in organic field pea and lentils. Mycorrhiza 20, 541–549. doi: 10.1007/s00572-010-0305-7
Balliu, A., Sallaku, G., and Rewald, B. (2015). AMF inoculation enhances growth and improves the nutrient uptake rates of transplanted, Salt-stressed tomato seedlings. Sustainability 7, 15967–15981. doi: 10.3390/su71215799
Banerjee, S., Walder, F., Büchi, L., Meyer, M., Held, A. Y., Gattinger, A., et al. (2019). Agricultural intensification reduces microbial network complexity and the abundance of keystone taxa in roots. ISME J. 13, 1722–1736. doi: 10.1038/s41396-019-0383-2
Barber, N. A., Kiers, E. T., Theis, N., Hazzard, R. V., and Adler, L. S. (2013). Linking agricultural practices, mycorrhizal fungi, and traits mediating plant-insect interactions. Ecol. Applic. 23, 1519–1530. doi: 10.1890/13-0156.1
Barbosa, M. V., Pedroso, D. D. F., Curi, N., and Carneiro, M. A. C. (2019). Do different arbuscular mycorrhizal fungi affect the formation and stability of soil aggregates? Cienciae Agrotecnol. 43, 1–9. doi: 10.1590/1413-7054201943003519
Barceló, M., van Bodegom, P. M., Tedersoo, L., den Haan, N., Veen, G. F., Ostonen, I., et al. (2020). The abundance of arbuscular mycorrhiza in soils is linked to the total length of roots colonized at ecosystem level. PLoS ONE 15, e0237256. doi: 10.1371/journal.pone.0237256
Baruffa, E. (2008). Cultivating the future based on science: proceedings of the Second Scientific Conference of the International Society of Organic Agriculture Research (ISOFAR), held at the 16th IFOAM Organic World Congress in cooperation with the International Federation of Organic Agriculture Movements (IFOAM) and the ConsorzioModenaBio, 18–20 June 2008 in Modena, Italy. ISOFAR. Available online at: https://orgprints.org/11809/1/Baruffa_11809_ed.doc (accessed May 24, 2022).
Basu, S., Rabara, R. C., and Negi, S. (2018). AMF: The prospect for sustainable agriculture. Physiol. Mol. Plant Pathol. 102, 36–45. doi: 10.1016/j.pmpp.2017.11.007
Bedini, S., Avio, L., Sbrana, C., Turrini, A., Migliorini, P., Vazzana, C., et al. (2013). Mycorrhizal activity and diversity in a long-term organic Mediterranean agroecosystem. Biol. Fertil. Soils 49, 781–790. doi: 10.1007/s00374-012-0770-6
Begum, N., Qin, C., Ahanger, M. A., Raza, S., Khan, M. I., Ashraf, M., et al. (2019). Role of arbuscular mycorrhizal fungi in plant growth regulation: implications in abiotic stress tolerance. Front. Plant Sci. 10, 1068. doi: 10.3389/fpls.2019.01068
Ben-Laouane, R., Ait-El-Mokhtar, M., Anli, M., Boutasknit, A., Ait Rahou, Y., Raklami, A., et al. (2021). Green compost combined with mycorrhizae and rhizobia: a strategy for improving alfalfa growth and yield under field conditions. Gesunde Pflanzen 73, 193–207. doi: 10.1007/s10343-020-00537-z
Bergstrand, K. J. (2022). Organic fertilizers in greenhouse production systems – a review. Sci. Horticult. 295, 110855. doi: 10.1016/j.scienta.2021.110855
Bhale, U. N., Bansode, S. A., and Sing, S. (2018). “Multifactorial role of arbuscular mycorrhizae in agroecosystem,” in Fungi and Their Role in Sustainable Development: Current Perspective, eds P. Gehlot, and J. Singh (Singapore: Springer), 205–220. doi: 10.1007/978-981-13-0393-7_12
Bhantana, P. (2021). Role of mycorrhizal pathways in plant phosphorous and zinc uptake. Biomed. J. Sci. Tech. Res. 36, 28397–28411. doi: 10.26717/BJSTR.2021.36.005834
Bibi, S., Saifullah, N. A., and Dahlaw, S. (2016). Soil Science: Agricultural and Environmental Prospectives. Switzerland: Springer.
Bisht, N., and Chauhan, P. S. (2021). Excessive and Disproportionate Use of Chemicals Causes Soil Contamination and Nutritional Stress. London: Intech Open. doi: 10.5772/intechopen.94593
Blanchet, G., Gavazov, K., Bragazza, L., and Sinaj, S. (2016). Responses of soil properties and crop yields to different inorganic and organic amendments in a Swiss conventional farming system. Agric. Ecosyst. Environ. 230. 116–126. doi: 10.1016/j.agee.2016.05.032
Boonlue, S., Surapat, W., Pukahuta, C., Suwanarit, P., Suwanarit, A., and Morinaga, T. (2012). Diversity and efficiency of arbuscular mycorrhizal fungi in soils from organic chili (Capsicum frutescens) farms. Mycoscience 53, 10–16. doi: 10.1007/s10267-011-0131-6
Borowicz, V. A. (2009). Organic farm soil improves strawberry growth but does not diminish spittlebug damage. J. Sustain. Agric. 33, 177–188. doi: 10.1080/10440040802660087
Bremmer, J., Riemens, M., Reinders, M., and European Parliament European Parliamentary Research Service Scientific Foresight Unit (2021). The Future of Crop Protection in Europe: Study. Available online at: https://www.europarl.europa.eu/RegData/etudes/STUD/2021/656330/EPRS_STU(2021)656330(ANN1)_EN.pdf (accessed May 21, 2022).
Campo, S., Martín-Cardoso, H., Olivé, M., Pla, E., Catala-Forner, M., Martínez-Eixarch, M., et al. (2020). Effect of root colonization by arbuscular mycorrhizal fungi on growth, productivity and blast resistance in rice. Rice 13, 42. doi: 10.1186/s12284-020-00402-7
Carof, M., Godinot, O., and le Cadre, E. (2022). Biodiversity-based cropping systems: a long-term perspective is necessary. Sci. Total Environ. 838, 156022. doi: 10.1016/j.scitotenv.2022.156022
Chai, R., Ye, X., Ma, C., Wang, Q., Tu, R., Zhang, L., et al. (2019). Greenhouse gas emissions from synthetic nitrogen manufacture and fertilisation for main upland crops in China. Carbon Balance Manage. 14, 20. doi: 10.1186/s13021-019-0133-9
Chandrasekaran, M. (2022). Arbuscular mycorrhizal fungi mediated enhanced biomass, root morphological traits and nutrient uptake under drought stress: a Meta-Analysis. J. Fungi 8, 660. doi: 10.3390/jof8070660
Charoonnart, P., Seraypheap, K., Chadchawan, S., and Wangsomboondee, T. (2016). Arbuscular mycorrhizal fungus improves the yield and quality of Lactuca sativa in an organic farming system. ScienceAsia 42, 315. doi: 10.2306/scienceasia1513-1874.2016.42.315
Chen, J., Guo, J., Li, Z., Liang, X., You, Y., Li, M., et al. (2022). Effects of an arbuscular mycorrhizal fungus on the growth of and cadmium uptake in maize grown on polluted wasteland, farmland and slopeland soils in a lead-zinc mining area. Toxics 10, 359. doi: 10.3390/toxics10070359
Chen, M., Arato, M., Borghi, L., Nouri, E., and Reinhardt, D. (2018). Beneficial services of arbuscular mycorrhizal fungi—from ecology to application. Front. Plant Sci. 9, 1270. doi: 10.3389/fpls.2018.01270
Chen, S., Zhao, H., Zou, C., Li, Y., Chen, Y., Wang, Z., et al. (2017). Combined inoculation with multiple arbuscular mycorrhizal fungi improves growth, nutrient uptake and photosynthesis in cucumber seedlings. Front. Microbiol. 8, 2516. doi: 10.3389/fmicb.2017.02516
Chi, G. G., Srivastava, A. K., and Wu, Q. S. (2018). Exogenous, easily extractable glomalin-related soil protein improves drought tolerance of trifoliate orange. Arch. Agron. Soil Sci. 64, 1341–1350. doi: 10.1080/03650340.2018.1432854
Chu, D. (2018). Effects of heavy metals on soil microbial community. IOP Conf. Ser. Earth Environ. Sci. 113, 012009. doi: 10.1088/1755-1315/113/1/012009
Cidón, C. F., Figueiró, P. S., and Schreiber, D. (2021). Benefits of organic agriculture under the perspective of the bioeconomy: a systematic review. Sustainability 13, 6852. doi: 10.3390/su13126852
Coccina, A., Cavagnaro, T. R., Pellegrino, E., Ercoli, L., McLaughlin, M. J., and Watts-Williams, S. J. (2019). The mycorrhizal pathway of zinc uptake contributes to zinc accumulation in barley and wheat grain. BMC Plant Biol. 19, 133. doi: 10.1186/s12870-019-1741-y
Cockerton, H. M., Li, B., Stavridou, E., Johnson, A., Karlström, A., Armitage, A. D., et al. (2020). Genetic and phenotypic associations between root architecture, arbuscular mycorrhizal fungi colonisation and low phosphate tolerance in strawberries (fragaria × ananassa). BMC Plant Biol. 20, 154. doi: 10.1186/s12870-020-02347-x
Crossay, T., Majorel, C., Redecker, D., Gensous, S., Medevielle, V., Durrieu, G., et al. (2019). Is a mixture of arbuscular mycorrhizal fungi better for plant growth than single-species inoculants? Mycorrhiza 29, 325–339. doi: 10.1007/s00572-019-00898-y
Cruz-Koizumi, Y. P., Alayón-Gamboa, J. A., Morón-Ríos, A., Castellanos-Albores, J., Aguilar-Chama, A., and Guevara, R. (2018). Effects of organic and chemical agriculture systems on arbuscular mycorrhizal fungi and green tomato production in Calakmul, Mexico. Agric. Sci. 9, 1145–1167. doi: 10.4236/as.2018.99080
De La Hoz, J. P., Rivero, J., Azcón-Aguilar, C., Urrestarazu, M., and Pozo, M. J. (2021). Mycorrhiza-induced resistance against foliar pathogens is uncoupled of nutritional effects under different light intensities. J. Fungi 7, 402. doi: 10.3390/jof7060402
de Leon, D. G., Vahter, T., Zobel, M., Koppel, M., Edesi, L., Davison, J., et al. (2020). Different wheat cultivars exhibit variable responses to inoculation with arbuscular mycorrhizal fungi from organic and conventional farms. PLoS ONE 15, e0233878. doi: 10.1371/journal.pone.0233878
de Oliveira Freitas, N., Yano-Melo, A. M., Barbosa Da Silva, F. S., de Melo, N. F., and Maia, L. C. (2011). Microbial activity in vineyards Soil biochemistry and microbial activity in vineyards under conventional and organic management in Northeast Brazil. Sci. Agric. 68, 223–229. doi: 10.1590/S0103-90162011000200013
Dhalaria, R., Kumar, D., Kumar, H., Nepovimova, E., Kuca, K., Islam, M. T., et al. (2020). Arbuscular mycorrhizal fungi as potential agents in ameliorating heavy metal stress in plants. Agronomy 10, 815. doi: 10.3390/agronomy10060815
Diagne, N., Ngom, M., Djighaly, P. I., Fall, D., Hocher, V., and Svistoonoff, S. (2020). Roles of arbuscular mycorrhizal fungi on plant growth and performance: importance in biotic and abiotic stressed regulation. Diversity 12, 370. doi: 10.3390/d12100370
Dobo, B. (2022). Effect of arbuscular mycorrhizal fungi (AMF) and rhizobium inoculation on growth and yield of Glycine max L. varieties. Int. J. Agron. 2022, 9520091. doi: 10.1155/2022/9520091
Durham, T. C., and Mizik, T. (2021). Comparative economics of conventional, organic, and alternative agricultural production systems. Economies 9, 64. doi: 10.3390/economies9020064
Ebbisa, A. (2022). “Arbuscular mycorrhizal fungi (AMF) in optimizing nutrient bioavailability and reducing agrochemicals for maintaining sustainable agroecosystems,” in Mycorrhiza—New Insights, ed R. de Sousa (London: Intech Open). doi: 10.5772/intechopen.106995
Eck, J. L., Kytoviita, M. M., and Laine, A. L. (2022). Arbuscular mycorrhizal fungi influence host infection during epidemics in a wild plant pathosystem. New Phytol. 236, 1922–1935. doi: 10.1111/nph.18481
El Hilali, R., Symanczik, S., El kinany, S., Oehl, F., Ouahmane, L., and Bouamri, R. (2022). Cultivation, identification, and application of arbuscular mycorrhizal fungi associated with date palm plants in Drâa-Tafilalet oasis. Rhizosphere 22, 100521. doi: 10.1016/j.rhisph.2022.100521
EPRS Report (2016). Human Health Implications of Organic Food and Organic Agriculture. Available online at: https://www.europarl.europa.eu/RegData/etudes/STUD/2016/581922/EPRS_STU(2016)581922_EN.pdf (accessed May 28, 2022).
Eun Kang, J., Ciampi, A., and Hijri, M. (2020). SeSaMe: metagenome sequence classification of arbuscular mycorrhizal fungi-associated microorganisms. Genomics Proteomics Bioinformatics 18, 601–612. doi: 10.1016/j.gpb.2018.07.010
European Commission Report (2020). “Directorate-general for research and innovation, group of chief scientific advisors,” in Towards a Sustainable Food System: Moving From Food as a Commodity to Food as More of a Common Good: Independent Expert Report. Publications Office. Available online at: https://data.europa.eu/doi/10.2777/282386
Fall, A. F., Nakabonge, G., Ssekandi, J., Founoune-Mboup, H., Apori, S. O., Ndiaye, A., et al. (2022). Roles of arbuscular mycorrhizal fungi on soil fertility: contribution in the improvement of physical, chemical, and biological properties of the soil. Front. Fungal Biol. 3, 723892. doi: 10.3389/ffunb.2022.723892
FAO (2017). “Human health implications of organic food and organic agriculture report,” in FAO Human Health Implications of Organic Food and Organic Agriculture, eds M. Axel, E. Rembiałkowska, H. R. Andersen, P. Grandjean, T. H. Chan, and S. Gunnarsson. Available online at: https://www.europarl.europa.eu/RegData/etudes/STUD/2016/581922/EPRS_STU(2016)581922_EN.pdf (accessed May 28, 2022).
FAO (2018). Transforming Food and Agriculture to Achieve the SDGs 20 Interconnected Actions to Guide Decision-Makers. FAO. https://www.fao.org/3/I9900EN/i9900en.pdf (accessed May 28, 2022).
FAO (2021). Food and Agricultural Organization, Organic foods, Food safety Technical Toolkit for Asia and the Pacific. Available online at: https://www.fao.org/asiapacific/perspectives/one-health/food-safety/food-safety-toolkits/ru/ (accessed May 31, 2022).
FAO ITPS. (2015). “Intergovernmental technical panel on soils. Status of the world's soil resources,” in Intergovernmental Technical Panel on Soils. Available online at: http://www.fao.org/3/a-i5199e.pdf (accessed May 31, 2022).
Fard, S. E., Yarnia, M., Farahvash, F., Behrouzyar, E. K., and Rashidi, V. (2020). Arbuscular mycorrhizas and phosphorus fertilizer affect photosynthetic capacity and antioxidant enzyme activity in peppermint under different water conditions. Acta Agrobotan. 73, 1–13. doi: 10.5586/aa.7345
Fernández, J. A., Ayastuy, M. E., Belladonna, D. P., Comezaña, M. M., Contreras, J., de Maria Mourão, I., et al. (2022). Current trends in organic vegetable crop production: practices and techniques. Horticulturae 8, 893. doi: 10.3390/horticulturae8100893
Fitzpatrick, I. C., Millner, N., and Ginn, F. (2022). Governing the soil: natural farming and bionationalism in India. Agric. Hum. Values 39, 1391–1406. doi: 10.1007/s10460-022-10327-0
Fujita, M., Kusajima, M., Fukagawa, M., Okumura, Y., Nakajima, M., Akiyama, K., et al. (2022). Response of tomatoes primed by mycorrhizal colonisation to virulent and avirulent bacterial pathogens. Sci. Rep. 12, 4686. doi: 10.1038/s41598-022-08395-7
Galván, G. A., Parádi, I., Burger, K., Baar, J., Kuyper, T. W., Scholten, O. E., et al. (2009). Molecular diversity of arbuscular mycorrhizal fungi in onion roots from organic and conventional farming systems in the Netherlands. Mycorrhiza 19, 317–328. doi: 10.1007/s00572-009-0237-2
Garg, N., Singh, S., and Kashyap, L. (2017). “Arbuscular mycorrhizal fungi and heavy metal tolerance in plants: an insight into physiological and molecular mechanisms,” in Mycorrhiza—Nutrient Uptake, Biocontrol, Ecorestoration, eds A. Varma, R. Prasad, and N. Tuteja (Switzerland: Springer), 75–97. doi: 10.1007/978-3-319-68867-1_4
Garrido, E., Bennett, A. E., Fornoni, J., and Strauss, S. Y. (2010). Variation in arbuscular mycorrhizal fungi colonisation modifies the expression of tolerance to above-ground defoliation. J. Ecol. 98, 43–49. doi: 10.1111/j.1365-2745.2009.01586.x
Gavrilescu, M. (2021). Enhancing phytoremediation of soils polluted with heavy metals. Curr. Opin. Biotechnol. 74, 21–31. doi: 10.1016/j.copbio.2021.10.024
Gazdag, O., Kovács, R., Parádi, I., Füzy, A., Ködöböcz, L., Mucsi, M., et al. (2019). Density and diversity of microbial symbionts under organic and conventional agricultural management. Microbes Environ. 34, 234–243. doi: 10.1264/jsme2.ME18138
Ghimire, R., Norton, J., and Norton, U. (2018). Soil organic matter dynamics under irrigated perennial forage-annual crop rotations. Grass Forage Sci. 73, 907–917. doi: 10.1111/gfs.12378
Giovannini, L., Palla, M., Agnolucci, M., Avio, L., Sbrana, C., Turrini, A., et al. (2020). Arbuscular mycorrhizal fungi and associated microbiota as plant biostimulants: research strategies for the selection of the best performing inocula. Agronomy 10, 106. doi: 10.3390/agronomy10010106
Gitonga, N. M., Koskey, G., Njeru, E. M., Maingi, J. M., and Cheruiyot, R. (2021). Dual inoculation of soybean with Rhizophagus irregularis and commercial Bradyrhizobium japonicum increases nitrogen fixation and growth in organic and conventional soils. AIMS Agric. Food 6, 478–495. doi: 10.3934/agrfood.2021028
Głodowska, M., and Krawczyk, J. (2017). Heavy metals concentration in conventionally and organically grown vegetables. Qual. Assur. Saf. Crops Foods 9, 497–503. doi: 10.3920/QAS2017.1089
Gong, X., and Tian, D. Q. (2019). Study the effect mechanism of Arbuscular Mycorrhiza on plants' absorption of heavy metal elements in the soil. Earth Environ. Sci. 267, 052064. doi: 10.1088/1755-1315/267/5/052064
Grünfeld, L., Skias, G., Rillig, M. C., and Veresoglou, S. D. (2022). Arbuscular mycorrhizal root colonisation depends on the spatial distribution of the host plants. Mycorrhiza 32, 387–395. doi: 10.1007/s00572-022-01087-0
Guo, X., Wang, Z., Zhang, J., Wang, P., Li, Y., and Ji, B. (2021). Host-specific effects of arbuscular mycorrhizal fungi on two caragana species in desert grassland. J. Fungi 7, 1077. doi: 10.3390/jof7121077
Harikumar, V. S. (2015). Arbuscular mycorrhizal associations in sesame under low-input cropping systems. Arch. Agron. Soil Sci. 61, 347–359. doi: 10.1080/03650340.2014.932906
Heklau, H., Schindler, N., Buscot, F., Eisenhauer, N., Ferlian, O., Prada Salcedo, L. D., et al. (2021). Mixing tree species associated with arbuscular or ectotrophic mycorrhizae reveals dual mycorrhization and interactive effects on the fungal partners. Ecol. Evol. 11, 5424–5440. doi: 10.1002/ece3.7437
Hoeksema, J. D. (2005). Plant-plant interactions vary with different mycorrhizal fungus species. Biol. Lett. 1, 439–442. doi: 10.1098/rsbl.2005.0381
Hohmann, P., and Messmer, M. M. (2017). Breeding for mycorrhizal symbiosis: focus on disease resistance. Euphytica 213, 113. doi: 10.1007/s10681-017-1900-x
Holátko, J., Brtnický, M., Kučerík, J., Kotianová, M., Elbl, J., Kintl, A., et al. (2021). Glomalin – Truths, myths, and the future of this elusive soil glycoprotein. Soil Biol. Biochem. 153, 108116. doi: 10.1016/j.soilbio.2020.108116
Horn, S., Hempel, S., Verbruggen, E., Rillig, M. C., and Caruso, T. (2017). Linking the community structure of arbuscular mycorrhizal fungi and plants: a story of interdependence? ISME J. 11, 1400–1411. doi: 10.1038/ismej.2017.5
Huang, J., Liu, W., Yang, S., Yang, L., Peng, Z., Deng, M., et al. (2021). Plant carbon inputs through the shoot, root, and mycorrhizal pathways affect soil organic carbon turnover differently. Soil Biol. Biochem. 160, 108322. doi: 10.1016/j.soilbio.2021.108322
Huo, W., Ge Chai, X., Fen Wang, X., He., et al. (2022). Indigenous arbuscular mycorrhizal fungi play a role in phosphorus depletion in organic manure amended high fertility soil. J. Integrat. Agric. 21, 3051–3066. doi: 10.1016/j.jia.2022.07.045
IFOAM and FiBL (2021). “International federation of organic agriculture movements., & for schungs institute für biologischen Landbau (Suisse),” in The World of Organic Agriculture: Statistics & Emerging Trends 2021. FiBL. Available online at: https://www.fibl.org/fileadmin/documents/shop/1150-organic-world-2021.pdf (accessed June 02, 2022).
Igiehon, N. O., and Babalola, O. O. (2017). Biofertilizers and sustainable agriculture: exploring arbuscular mycorrhizal fungi. Appl. Microbiol. Biotechnol. 101, 4871–4881. doi: 10.1007/s00253-017-8344-z
Irving, T. B., Alptekin, B., Kleven, B., and Ané, J. M. (2021). A critical review of 25 years of glomalin research: a better mechanical understanding and robust quantification techniques are required. New Phytol. 232, 1572–1581. doi: 10.1111/nph.17713
Islam, M. Z., and Katoh, S. (2017). The effect of arbuscular mycorrhizal fungi and gliocladium fungi on the yield of small green pepper (Capsicum annuum) grown by sustainable agriculture. Agric. Sci. 8, 1296–1314. doi: 10.4236/as.2017.811094
Jaacks, L. M., Serupally, R., Dabholkar, S., Venkateshmurthy, N. S., Mohan, S., and Roy, A. (2022). Articles Impact of large-scale, the government legislated and funded organic farming training on pesticide use in Andhra Pradesh, India: a cross-sectional study. Lancet Planet. Health 6, e310–e319. doi: 10.1016/S2542-5196(22)00062-6
Jadrane, I., Al feddy, M. N., Dounas, H., Kouisni, L., Aziz, F., and Ouahmane, L. (2021). Inoculation with selected indigenous mycorrhizal complex improves Ceratonia siliqua's growth and response to drought stress. Saudi J. Biol. Sci. 28, 825–832. doi: 10.1016/j.sjbs.2020.11.018
Jalmi, S. K., Bhagat, P. K., Verma, D., Noryang, S., Tayyeba, S., Singh, K., et al. (2018). Traversing the links between heavy metal stress and plant signalling. Front. Plant Sci. 9, 12. doi: 10.3389/fpls.2018.00012
Janeeshma, E., and Puthur, J. T. (2020). The direct and indirect influence of arbuscular mycorrhizae on enhancing metal tolerance of plants. Arch. Microbiol. 202, 1–16. doi: 10.1007/s00203-019-01730-z
Janos, D. P., Scott, J., Aristizábal, C., and Bowman, D. M. J. S. (2013). Arbuscular-mycorrhizal networks inhibit Eucalyptus tetrodonta seedlings in rain forest soil microcosms. PLoS ONE 8, e57716. doi: 10.1371/journal.pone.0057716
Jarosławiecka, A. K., and Piotrowska-Seget, Z. (2022). The effect of heavy metals on microbial communities in industrial soil in the area of PiekarySlaskie and Bukowno (Poland). Microbiol. Res. 13, 626–642. doi: 10.3390/microbiolres13030045
Jeewani, P. H., Luo, Y., Yu, G., Fu, Y., He, X., Van Zwieten, L., et al. (2021). Arbuscular mycorrhizal fungi and goethite promote carbon sequestration via hyphal-aggregate mineral interactions. Soil Biol. Biochem. 162, 108417. doi: 10.1016/j.soilbio.2021.108417
Jerbi, M., Labidi, S., Lounès-Hadj Sahraoui, A., Chaar, H., Jeddi, F., and ben. (2020). Higher temperatures and lower annual rainfall do not restrict, directly or indirectly, the mycorrhizal colonisation of barley (Hordeum vulgare L.) under rainfed conditions. PLoS ONE 15, e0241794. doi: 10.1371/journal.pone.0241794
Jiang, S., An, X., Shao, Y., Kang, Y., Chen, T., Mei, X., et al. (2021). Responses of arbuscular mycorrhizal fungi occurrence to organic fertilizer: a meta-analysis of field studies. Plant Soil 469, 89–105. doi: 10.1007/s11104-021-05153-y
Juntahum, S., Kuyper, T. W., and Boonlue, S. (2022). Distribution of arbuscular mycorrhizal fungi in sugarcane rhizosphere from various agricultural management practices in Northeast Thailand. Curr. Res. Environ. Appl. Mycol. 12, 44–55. doi: 10.5943/cream/12/1/4
Kadam, S. B., Pable, A. A., and Barvkar, V. T. (2020). Mycorrhiza-induced resistance (MIR): a defence developed through the synergistic engagement of phytohormones, metabolites and rhizosphere. Funct. Plant Biol. 47, 880–890. doi: 10.1071/FP20035
Kazeeroni, E. A., and Al-Sadi, A. M. (2016). 454-pyrosequencing reveals variable fungal diversity across farming systems. Front. Plant Sci. 7, 314. doi: 10.3389/fpls.2016.00314
Khrieba, M. I., and Publishers, L. (2019). Mycorrhizae's Role in Plant Nutrition and Protection From Pathogens. Lupine Publishers. Available online at: lupinepublishers.com (accessed May 07, 2022).
Kim, S. J., Eo, J. K., Lee, E. H., Park, H., and Eom, A. H. (2017). Effects of arbuscular mycorrhizal fungi and soil conditions on crop plant growth. Mycobiology 45, 20–24. doi: 10.5941/MYCO.2017.45.1.20
Knerr, A. J., Wheeler, D., Schlatter, D., Sharma-Poudyal, D., du Toit, L. J., and Paulitz, T. C. (2018). Arbuscular mycorrhizal fungal communities in organic and conventional onion crops in the Pacific Northwest United States Columbia Basin. Phytobiomes J. 2, 194–207. doi: 10.1094/PBIOMES-05-18-0022-R
Knight, J. D. (2020). Evaluation of two commercial fungal inoculants for improving phosphorus supply to crops grown in soils with contrasting management histories. Renew. Agric. Food Syst. 36, 278–289. doi: 10.1017/S1742170520000319
Kobae, Y. (2019). Active phosphate uptake in arbuscular mycorrhizal roots under field conditions. Front. Environ. Sci. 6, 159. doi: 10.3389/fenvs.2018.00159
Kobierski, M., Lemanowicz, J., Wojewódzki, P., and Kondratowicz-Maciejewska, K. (2020). The effect of organic and conventional farming systems with different tillage on soil properties and enzymatic activity. Agronomy 10, 1809. doi: 10.3390/agronomy10111809
Kozjek, K., Kundel, D., Kushwaha, S. K., Olsson, P. A., Ahrén, D., Fliessbach, A., et al. (2021). Long-term agricultural management impacts arbuscular mycorrhizal fungi more than short-term experimental drought. Appl. Soil Ecol. 168, 104140. doi: 10.1016/j.apsoil.2021.104140
Kuila, D., and Ghosh, S. (2022). Aspects, problems and utilization of arbuscular mycorrhizal (AM) application as bio-fertilizer in sustainable agriculture. Curr. Res. Microbial Sci. 3, 100107. doi: 10.1016/j.crmicr.2022.100107
Kundel, D., Bodenhausen, N., Jørgensen, H. B., Truu, J., Birkhofer, K., Hedlund, K., et al. (2020). Effects of simulated drought on biological soil quality, microbial diversity and yields under long-term conventional and organic agriculture. FEMS Microbiol. Ecol. 96, fiaa205. doi: 10.1093/femsec/fiaa205
Lambertini, M. (2021). Living Planet Report 2020: Bending the Curve of Biodiversity Loss. Available online at: https://www.zsl.org/sites/default/files/LPR%202020%20Full%20report.pdf (accessed June 01, 2022).
Lee, S.-W., Lee, E.-H., and Eom, A.-H. (2008). Effects of organic farming on communities of arbuscular mycorrhizal fungi. Mycobiology 36, 19. doi: 10.4489/MYCO.2008.36.1.019
Leksono, A. S. (2017). The effect of organic farming systems on species diversity. AIP Conf. Proc. 1908, 030001. doi: 10.1063/1.5012701
Lin, G., Mccormack, M. L., and Guo, D. (2015). Arbuscular mycorrhizal fungal effects on plant competition and community structure. J. Ecol. 103, 1224–1232. doi: 10.1111/1365-2745.12429
Liu, B., Tu, C., Hu, S., Gumpertz, M., and Ristaino, J. B. (2007). Effect of organic, sustainable, and conventional management strategies in grower fields on soil physical, chemical, and biological factors and the incidence of Southern blight. Appl. Soil Ecol. 37, 202–214. doi: 10.1016/j.apsoil.2007.06.007
Liu, J., Zhang, J., Li, D., Xu, C., and Xiang, X. (2020). Differential responses of arbuscular mycorrhizal fungal communities to mineral and organic fertilisation. Microbiol. Open 9, e00920. doi: 10.1002/mbo3.920
Liu, L., Li, C., Zhu, S., Xu, Y., Li, H., Zheng, X., et al. (2020). Combined application of organic and inorganic nitrogen fertilizers affects soil prokaryotic communities compositions. Agronomy 10, 132. doi: 10.3390/agronomy10010132
Lombardo, S., Abbate, C., Pandino, G., Parisi, B., Scavo, A., and Mauromicale, G. (2020). Productive and physiological response of organic potato grown under highly calcareous soils to fertilisation and mycorrhization management. Agronomy 10, 1200. doi: 10.3390/agronomy10081200
Lombardo, S., Scavo, A., Abbate, C., Pandino, G., Parisi, B., and Mauromicale, G. (2021). Mycorrhizal inoculation improves the mineral content of organic potatoes grown under calcareous soil. Agriculture 11, 333. doi: 10.3390/agriculture11040333
Lu, L. H., Zou, Y. N., and Wu, Q. S. (2019). Mycorrhizas mitigate soil replant disease of peach through regulating root exudates, soil microbial population, and soil aggregate stability. Commun. Soil Sci. Plant Anal. 50, 909–921. doi: 10.1080/00103624.2019.1594882
Lumini, E., Vallino, M., Alguacil, M. M., Romani, M., and Bianciotto, V. (2011). Different farming and water regimes in Italian rice fields affect arbuscular mycorrhizal fungal soil communities. Ecol. Applic. 21, 1696–1707. doi: 10.1890/10-1542.1
Ma, K., Wang, Y., Jin, X., Zhao, Y., Yan, H., Zhang, H., et al. (2022). Application of organic fertilizer changes the rhizosphere microbial communities of a gramineous grass on Qinghai–Tibet Plateau. Microorganisms 10, 1148. doi: 10.3390/microorganisms10061148
Ma, M., Jiang, X., Wang, Q., Ongena, M., Wei, D., Ding, J., et al. (2018). Responses of fungal community composition to long—term chemical and organic fertilisation strategies in Chinese Mollisols. Microbiologyopen 7, e00597. doi: 10.1002/mbo3.597
Maighal, M., Salem, M., Kohler, J., and Rillig, M. C. (2016). Arbuscular mycorrhizal fungi negatively affect soil seed bank viability. Ecol. Evol. 6, 7683–7689. doi: 10.1002/ece3.2491
Majewska, M. L., Rola, K., and Zubek, S. (2017). The growth and phosphorus acquisition of invasive plants Rudbeckia laciniata and Solidago gigantea are enhanced by arbuscular mycorrhizal fungi. Mycorrhiza 27, 83–94. doi: 10.1007/s00572-016-0729-9
Manoharan, L., Rosenstock, N. P., Williams, A., and Hedlund, K. (2017). Agricultural management practices influence AMF diversity and community composition with cascading effects on plant productivity. Appl. Soil Ecol. 115, 53–59. doi: 10.1016/j.apsoil.2017.03.012
Marinho, F., Oehl, F., da Silva, I. R., Coyne, D., Veras, J. S., da, N., et al. (2019). High diversity of arbuscular mycorrhizal fungi in natural and anthropized sites of a Brazilian tropical dry forest (Caatinga). Fungal Ecol. 40, 82–91. doi: 10.1016/j.funeco.2018.11.014
Mazzoncini, M., Canali, S., Giovannetti, M., Castagnoli, M., Tittarelli, F., Antichi, D., et al. (2010). Comparison of organic and conventional stockless arable systems: a multidisciplinary approach to soil quality evaluation. Appl. Soil Ecol. 44, 124–132. doi: 10.1016/j.apsoil.2009.11.001
Mie, A., Andersen, H. R., Gunnarsson, S., Kahl, J., Kesse-Guyot, E., Rembialkowska, E., et al. (2017). Human health implications of organic food and organic agriculture: a comprehensive review. Environ. Health. 16, 1–22. doi: 10.1186/s12940-017-0315-4
Mohanty, P., Singh, P. K., Chakraborty, D., Mishra, S., and Pattnaik, R. (2021). Insight into the role of PGPR in sustainable agriculture and environment. Front. Sustain. Food Syst. 5, 667150. doi: 10.3389/fsufs.2021.667150
Morshedi, L., Lashgarara, F., Hosseini, S. J. F., and Najafabadi, M. O. (2017). The role of organic farming in improving food security from the perspective of fars farmers. Sustainability 9, 2086. doi: 10.3390/su9112086
Mrunalini, K., Behera, B., Jayaraman, S., Abhilash, P. C., Dubey, P. K., Swamy, G. N., et al. (2022). Nature-based solutions in soil restoration for improving agricultural productivity. Land Degrad. Dev. 33, 1269–1289. doi: 10.1002/ldr.4207
Mukherjee, A., Omondi, E. C., Hepperly, P. R., Seidel, R., and Heller, W. P. (2020). Impacts of organic and conventional management on the nutritional level of vegetables. Sustainability 12, 8965. doi: 10.3390/su12218965
Mullath, S. K., Błaszkowski, J., Govindan, B. N., Dhaheri, L., Al Symanczik, S., and Al-Yahya'ei, M. N. (2019). Organic farming practices in a desert habitat increased the abundance, richness, and diversity of arbuscular mycorrhizal fungi#1. Emirates J. Food Agric. 31, 969–979. doi: 10.9755/ejfa.2019.v31.i12.2057
Muneer, M. A., Wang, P., Zaib-un-Nisa, L. C., and Ji, B. (2020). Potential role of standard mycorrhizal networks in improving plant growth and soil physicochemical properties under varying nitrogen levels in a grassland ecosystem. Glob. Ecol. Conserv. 24, e01352. doi: 10.1016/j.gecco.2020.e01352
Nadiah, N. S. H., Nursyahidah, R., Jaafar, N. M., Zaharah, S. S., and Muharam, F. M. (2020). Arbuscular mycorrhizal fungi (AMF) and NPK fertilisation rate on the growth of soursop (Annona muricata L.) seedlings. Malaysian J. Soil Sci. 24, 147–159. Available online at: https://www.cabdirect.org/cabdirect/abstract/20203599649 (accessed May, 18, 2022).
Nanjundappa, A., Bagyaraj, D. J., Saxena, A. K., Kumar, M., and Chakdar, H. (2019). “Interaction between arbuscular mycorrhizal fungi and Bacillus spp. In soil enhances the growth of crop plants,” in Fungal Biology and Biotechnology, Vol. 6 (BioMed Central Ltd). doi: 10.1186/s40694-019-0086-5
Naorema, A., Maverick, J., Singh, B. P., and Udayana, S. K. (2021). Microbial Community Structure in Organic Farming and Their Management, 1st Edn. Amsterdam: Elsevier. doi: 10.1016/B978-0-12-822358-1.00004-3
Nazari, M., Riebeling, S., Banfield, C. C., Akale, A., Crosta, M., Mason-Jones, K., et al. (2020). Mucilage polysaccharide composition and exudation in maize from contrasting climatic regions. Front. Plant Sci. 11, 587610. doi: 10.3389/fpls.2020.587610
Nidheesh, K. S., Valsalakumar, N., Potty, V. P., and Ray, J. G. (2018). Ecology of endomycorrhizal association in Musa spp. of south india. Symbiosis 74, 199–214. doi: 10.1007/s13199-017-0508-1
Njeru, E. M. (2018). Exploiting diversity to promote arbuscular mycorrhizal symbiosis and crop productivity in organic farming systems. AIMS Agric. Food 3, 280–294. doi: 10.3934/agrfood.2018.3.280
Ortaş, I. (2018). “Role of microorganisms (mycorrhizae) in organic farming,” in Organic Farming: Global Perspectives and Methods, eds S. Chandran, M. R. Unni and S. Thomas (Amsterdam: Elsevier). doi: 10.1016/B978-0-12-813272-2.00006-9
Ortas, I., and Bykova, A. (2018). The effect of mycorrhiza inoculation and phosphorus application on phosphorus efficiency of wheat plants. Commun. Soil Sci. Plant Anal. 49, 1199–1207. doi: 10.1080/00103624.2018.1455849
Ortas, I., and Rafique, M. (2017). “The mechanisms of nutrient uptake by arbuscular mycorrhiza,” in Mycorrhiza—Nutrient Uptake, Biocontrol, Ecorestoration, 4th Edn, eds A. Varma, R. Prasad, and N. Tuteja (Switzerland: Springer), 1–19. doi: 10.1007/978-3-319-68867-1_1
Ouledali, S., Lumini, E., Bianciotto, V., Khemira, H., and Ennajeh, M. (2022). Diversity of arbuscular mycorrhizal fungi in olive orchard soils in arid regions of southern Tunisia. Arid Land Res. Manage. 36, 411–427. doi: 10.1080/15324982.2022.2037787
Ouyang, Y., and Norton, J. M. (2020). Short-term nitrogen fertilization affects microbial community composition and nitrogen mineralization functions in an agricultural soil. Appl. Environ. Microbiol. 86, e02278–e02219. doi: 10.1128/AEM.02278-19
Page, K. L., Dang, Y. P., and Dalal, R. C. (2020). The ability of conservation agriculture to conserve soil organic carbon and the subsequent impact on soil physical, chemical, and biological properties and yield. Front. Sustain. Food Syst. 4, 31. doi: 10.3389/fsufs.2020.00031
Pahalvi, H. N., Rafiya, L., Rashid, S., Nisar, B., and Kamili, A. N. (2021). Chemical fertilizers and their impact on soil health. Microbiota Biofertil. 2, 1–20. doi: 10.1007/978-3-030-61010-4_1
Parihar, M., Rakshit, A., Singh, V., Vijai, M., Gupta, K., Rana, K., et al. (2020). The potential of arbuscular mycorrhizal fungi in C cycling: a review. Arch. Microbiol. 202, 1581–1596. doi: 10.1007/s00203-020-01915-x
Pellegrino, E., Öpik, M., Bonari, E., and Ercoli, L. (2015). Responses of wheat to arbuscular mycorrhizal fungi: a meta-analysis of field studies from 1975 to 2013. Soil Biol. Biochem. 84, 210–217. doi: 10.1016/j.soilbio.2015.02.020
Pellegrino, E., Piazza, G., Arduini, I., and Ercoli, L. (2020). Field inoculation of bread wheat with Rhizophagus irregularis under organic farming: variability in growth response and nutritional uptake of eleven old genotypes and a modern variety. Agronomy 10, 333. doi: 10.3390/agronomy10030333
Peltoniemi, K., Velmala, S., Fritze, H., Lemola, R., and Pennanen, T. (2021). Long-term impacts of organic and conventional farming on the soil microbiome in boreal arable soil. Euro. J. Soil Biol. 104, 103314. doi: 10.1016/j.ejsobi.2021.103314
Phillips, M. W. A. (2020). Agrochemical industry development, trends in R&D and the impact of regulation. Pest Manage. Sci. 76, 3348–3356. doi: 10.1002/ps.5728
Pontes-da-Silva, E., Magnusson, W. E., Sinervo, B., Caetano, G. H., Miles, D. B., Colli, G. R., et al. (2018). Extinction risks are forced by climatic change and intraspecific variation in the thermal physiology of a tropical lizard. J. Therm. Biol. 73:50–60. doi: 10.1016/j.jtherbio.2018.01.013
Posta, K., and Hong Duc, N. (2020). “Benefits of arbuscular mycorrhizal fungi application to crop production under water scarcity,” in Drought—Detection and Solutions, ed G. Ondrasek (London: IntechOpen). doi: 10.5772/intechopen.86595
Prayudyaningsih, R., Sari, R., and Mangopang, A. D. (2019). Isolation of indigenous arbuscular mycorrhizal fungi (amf) to support revegetation on the nickel post-mining land. IOP Conf. Ser. Earth Environ. Sci. 308, 012038. doi: 10.1088/1755-1315/308/1/012038
Purin, S., Filho, O. K., and Stürmer, S. L. (2006). Mycorrhizae activity and diversity in conventional and organic apple orchards from Brazil. Soil Biol. Biochem. 38, 1831–1839. doi: 10.1016/j.soilbio.2005.12.008
Quiroga, G., Aroca, R., Manuel, J., and Lozano, R. (2019). The arbuscular mycorrhizal symbiosis regulates aquaporins activity and improves root cell water permeability in maize plants subjected to water stress. Plant Cell Environ. 42, 2274–2290. doi: 10.1111/pce.13551
Ray, J. G. (1993). “Role of perennial grasses in controlling degradation of soil systems in steppes,” in Integrated Soil and Sediment Research: A Basis for Proper Protection, Vol 1, eds H. J. P. Eijsackers, and T. Hamers (Dordrecht: Springer). doi: 10.1007/978-94-011-2008-1_78
Ray, J. G., Travleev, A. P., and Belova, N. A. (1993). “The extinction of some perennial grass vegetation and the degradation of chernozem due to anthropo-zoogenial factors,” in Some Steppes of Ukrainian SSR, ed S. Wicherek (Amsterdam: Elsevier), 241–251.
Ray, J. G., and Valsalakumar, N. (2010a). Arbuscular mycorrhizal fungi and Piriformospora indica individually and in combination with Rhizobium on green gram. J. Plant Nutr. 33, 285–298. doi: 10.1080/01904160903435409
Ray, J. G., and Valsalakumar, N. (2010b). Arbuscular mycorrhizal fungi and Rhizobium under varied dozes of NPK on green gram. J. Ecol. Noosphereology, Ukr. 21, 73–84.
Rempelos, L., Baranski, M., Wang, J., Adams, T. N., Adebusuyi, K., Beckman, J. J., et al. (2021). Integrated soil and crop management in organic agriculture: a logical framework to ensure food quality and human health? Agronomy 11, 2494. doi: 10.3390/agronomy11122494
Reyes-Sánchez, L. B., Horn, R., and Costantini, E. A. C. (2022). Sustainable Soil Management as a Key to Preserve Soil Biodiversity and Stop Its Degradation. Available online at: https://www.iuss.org/media/iuss_sustainable_soil_management_as_a_key_to_preserving_soil_biodiversity_and_stopping_its_degradation_book.pdf (accessed June 03, 2022).
Riaz, M., Kamran, M., Fang, Y., Wang, Q., Cao, H., Yang, G., et al. (2021). Arbuscular mycorrhizal fungi-induced mitigation of heavy metal phytotoxicity in metal contaminated soils: A critical review. Journal of Hazardous Materials, 402(March 2020), 123919. doi: 10.1016/j.jhazmat.2020.123919
Riedo, J., Wettstein, F. E., Rosch, A., Herzog, C., Banerjee, S., Buchi, L., et al. (2021). Widespread occurrence of pesticides in the organically managed agricultural soils-the ghost of a conventional agrarian past? Environ. Sci. Technol. 55, 2919–2928. doi: 10.1021/acs.est.0c06405
Rillig, M. C., Muller, L. A. H., and Lehmann, A. (2017). Soil aggregates as massively concurrent evolutionary incubators. ISME J. 11, 1943–1948. doi: 10.1038/ismej.2017.56
Rillig, M. C., Sosa-Hernández, M. A., Roy, J., Aguilar-Trigueros, C. A., Vályi, K., and Lehmann, A. (2016). Towards an integrated mycorrhizal technology: Harnessing mycorrhiza for sustainable intensification in agriculture. Front. Plant Sci. 7, 1625. doi: 10.3389/fpls.2016.01625
Runhaar, H. (2021). Four critical conditions for agroecological transitions in Europe. Int. J. of Agric. Sustain. 19, 227–233. doi: 10.1080/14735903.2021.1906055
Ruytinx, J., Kafle, A., Usman, M., Coninx, L., Zimmermann, S. D., and Garcia, K. (2020). Micronutrient transport in mycorrhizal symbiosis; zinc steals the show. Fungal Biol. Rev. 34, 1–9. doi: 10.1016/j.fbr.2019.09.001
Ryan, M. H., and Tibbett, M. (2008). “The role of arbuscular mycorrhizas in organic farming,” in Organic Crop Production—Ambitions and Limitations, eds H. Kirchmann, and L. Bergström (Switzerland: Springer), 189–229. doi: 10.1007/978-1-4020-9316-6_10
Sahodaran, K. N., Arun, A. K., and Ray, J. G. (2019). Native arbuscular mycorrhizal fungal isolates (Funneliformis mosseae and Glomus microcarpum) improve plant height and nutritional status of banana plants. Exp. Agric. 55, 924–933. doi: 10.1017/S0014479719000036
Sahodaran, N. K., and Ray, J. G. (2018). Heavy metal contamination in “chemicalised' green revolution banana fields in southern India. Environ. Sci. Pollut. Res. 25, 26874–26886. doi: 10.1007/s11356-018-2729-0
Salazar, M. J., Menoyo, E., Faggioli, V., Geml, J., Cabello, M., Rodriguez, J. H., et al. (2018). Pb accumulation in spores of arbuscular mycorrhizal fungi. Sci. Total Environ. 643, 238–246. doi: 10.1016/j.scitotenv.2018.06.199
Säle, V., Aguilera, P., Laczko, E., Mäder, P., Berner, A., Zihlmann, U., et al. (2015). Impact of conservation tillage and organic farming on the diversity of arbuscular mycorrhizal fungi. Soil Biol. Biochem. 84, 38–52. doi: 10.1016/j.soilbio.2015.02.005
Sánchez-Castro, I., Gianinazzi-Pearson, V., Cleyet-Marel, J. C., Baudoin, E., and van Tuinen, D. (2017). Glomeromycota communities survive extreme levels of metal toxicity in an orphan mining site. Sci. Total Environ. 598, 121–128. doi: 10.1016/j.scitotenv.2017.04.084
Sanmartín, N., Pastor, V., Pastor-Fernández, J., Flors, V., Pozo, M. J., and Sánchez-Bel, P. (2021). Role and mechanisms of callose priming in mycorrhiza-induced resistance. J. Exp. Bot. 71, 2769–2781. doi: 10.1093/jxb/eraa030
Schneider, K. D., Lynch, D. H., Dunfield, K., Khosla, K., Jansa, J., and Voroney, R. P. (2015). Farm system management affects the community structure of arbuscular mycorrhizal fungi. Appl. Soil Ecol. 96, 192–200. doi: 10.1016/j.apsoil.2015.07.015
Sendek, A., Karako,ç, C., Wagg, C., Domínguez-Begines, J., do Couto, G. M., van der Heijden, M. G. A., et al. (2019). Drought modulates interactions between arbuscular mycorrhizal fungal diversity and barley genotype diversity. Sci. Rep. 9, 9650. doi: 10.1038/s41598-019-45702-1
Shen, K., He, Y., Xu, X., Umer, M., Liu, X., Xia, T., et al. (2022). Effects of AMF on plant nutrition and growth depend on substrate gravel content and patchiness in the karst species Bidens pilosa L. Front. Plant Sci. 13, 968719. doi: 10.3389/fpls.2022.968719
Shen, M., Zhang, Y., Bo, G., Yang, B., and Wang, P. (2022). Microbial responses to the reduction of chemical fertilizers in the rhizosphere soil of flue-cured tobacco. Front. Bioeng. Biotechnol. 9, 812316. doi: 10.3389/fbioe.2021.812316
Sietz, D., and Neudert, R. (2022). Taking stock of and advancing knowledge on interaction archetypes at the nexus between land, biodiversity, food and climate. Environ. Res. Lett. 17, 113004. doi: 10.1088/1748-9326/ac9a5c
Silva, V., Mol, H. G. J., Zomer, P., Tienstra, M., Ritsema, C. J., and Geissen, V. (2019). Pesticide residues in European agricultural soils—a hidden reality unfolded. Sci. Total Environ. 653, 1532–1545. doi: 10.1016/j.scitotenv.2018.10.441
Silva-Flores, P., Neves, M., Weidlich, E., Fajardo, L., Acuña, L., Aguilera, P., et al. (2022). Mycorrhizas and Ecological Restoration in South America. Switzerland: Springer. doi: 10.1007/978-3-031-12994-0_21
Silveira, R., Mello, T. R. B., Sartori, M. R. S., Alves, G. S. C., Fonseca, F. C. A., Vizzotto, C. S., et al. (2021). Seasonal and long-term effects of nutrient additions and liming on the nifH gene in cerrado soils under native vegetation. iScience 24, 102349. doi: 10.1016/j.isci.2021.102349
Singh, A. K., Zhu, X., Chen, C., Wu, J., Yang, B., Zakari, S., et al. (2020). The role of glomalin in mitigation of multiple soil degradation problems. Crit. Rev. Environ. Sci. Technol. 52, 1604–1638. doi: 10.1080/10643389.2020.1862561
Smith, L. G., Kirk, G. J. D., Jones, P. J., and Williams, A. G. (2019). The greenhouse gas impacts of converting food production in England and Wales to organic methods. Nat. Commun. 10, 4641. doi: 10.1038/s41467-019-12622-7
Song, Y., Chen, D., Lu, K., Sun, Z., and Zeng, R. (2015). Enhanced tomato disease resistance primed by arbuscular mycorrhizal fungus. Front. Plant Sci. 6, 786. doi: 10.3389/fpls.2015.00786
Sousa, C. S., Menezes, R. S. C., Sampaio, E. V. S. B., Oehl, F., Maia, L. C., Garrido, M., et al. (2011). Occurrence of arbuscular mycorrhizal fungi after organic fertilization in maize, cowpea and cotton intercropping systems. Acta Sci. Agron. 34, 149–156. doi: 10.4025/actasciagron.v34i2.13143
Spagnoletti, F., and Lavado, R. (2015). The arbuscular mycorrhiza rhizophagus intraradices reduces the negative effects of arsenic on soybean plants. Agronomy 5, 188–199. doi: 10.3390/agronomy5020188
Sridhar, D., Fernanda, E., Achim, S., María Fore, P., Mlambo-Ngcuka, M., Bachelet, H., et al. (2022). Sustainable Development Goals Contributors Include Transforming Our World. Available onlinre at: https://worldseed.org/wp-~content/uploads/2019/06/SDGs-Transforming-our-world.pdf (accessed November 21, 2021).
Srivastava, R., Roseti, D., and Sharma, A. K. (2007). The evaluation of microbial diversity in a vegetable-based cropping system under organic farming practices. Appl. Soil Ecol. 36, 116–123. doi: 10.1016/j.apsoil.2007.01.008
Srivastava, V., Sarkar, A., Singh, S., Singh, P., de Araujo, A. S. F., and Singh, R. P. (2017). Agroecological responses to heavy metal pollution with special emphasis on soil health and plant performances. Front. Environ. Sci. 5, 64. doi: 10.3389/fenvs.2017.00064
Stoleru, V., and Sellitto, V. M. (2016). “Pest control in organic systems,” in Integrated Pest Management (IPM): Environmentally Sound Pest Management, eds H. Kaur Gill, and G. Goyal (London: InTech). doi: 10.5772/64457
Suja, G., Byju, G., Jyothi, A. N., Veena, S. S., and Sreekumar, J. (2017). Yield, quality and soil health under organic vs conventional farming in taro. Sci. Horticult. 218, 334–343. doi: 10.1016/j.scienta.2017.02.006
Sun, C., Guo, Q., Zeeshan, M., Milham, P., Qin, S., Ma, J., et al. (2022). Dual RNA and 16S ribosomal DNA sequencing reveal arbuscular mycorrhizal fungi-mediated mitigation of selenate stress in Zea mays L. and reshaping of soil microbiota. Ecotoxicol. Environ. Saf. 247, 114217. doi: 10.1016/j.ecoenv.2022.114217
Sun, H., Koal, P., Liu, D., Gerl, G., Schroll, R., Gattinger, A., et al. (2016). Soil microbial community and microbial residues respond positively to minimum tillage under organic farming in Southern Germany. Appl. Soil Ecol. 108, 16–24. doi: 10.1016/j.apsoil.2016.07.014
Szada-Borzyszkowska, A., Krzyzak, J., Rusinowski, S., Starzewska-Sikorska, A., Ratman-Kłosińska, I., and Pogrzeba, M. (2022). The effect of amendments on Lolium perenne roots arbuscular mycorrhizal fungi colonization when cultivated in contaminated soil. Int. J. Environ. Sci. Technol. 19, 9365–9376. doi: 10.1007/s13762-021-03783-4
Tahat, M. M., Alananbeh, K. A., Othman, Y. I., and Leskovar, D. (2020). Soil health and sustainable agriculture. Sustainability 12, 4859. doi: 10.3390/su12124859
Teotia, P., Kumar, M., Prasad, R., Kumar, V., Tuteja, N., and Varma, A. (2017). Mobilization of Micronutrients by Mycorrhizal Fungi. Switzerland: Springer. doi: 10.1007/978-3-319-53064-2_2
Thioye, B., Legras, M., Castel, L., Hirissou, F., Chaftar, N., and Trinsoutrot-Gattin, I. (2022). Understanding arbuscular mycorrhizal colonisation in walnut plantations: the contribution of cover crops and soil microbial communities. Agriculture 12, 1. doi: 10.3390/agriculture12010001
Trejo, D., Sangabriel-Conde, W., Gavito-Pardo, M. E., and Banuelos, J. (2021). Mycorrhizal inoculation and chemical fertilizer interactions in pineapple under field conditions. Agriculture 11, 934. doi: 10.3390/agriculture11100934
Trinchera, A., Migliore, M., Warren Raffa, D., Ommeslag, S., Debode, J., Shanmugam, S., et al. (2022). Can multi-cropping affect soil microbial stoichiometry and functional diversity, decreasing potential soil-borne pathogens? a study on European organic vegetable cropping systems. Front. Plant Sci. 13, 9522910. doi: 10.3389/fpls.2022.952910
Trisilawati, O., Hartoyo, B., Bermawie, N., and Pribadi, E. R. (2019). Application of AMF (arbuscular mycorrhizal fungi) and organic fertilizer to increase the growth, biomass and bioactive content of centella. IOP Conf. Ser. Earth Environ. Sci. 292, 012067. doi: 10.1088/1755-1315/292/1/012067
Tscharntke, T., Grass, I., Wanger, T. C., Westphal, C., and Batáry, P. (2021). Beyond organic farming – harnessing biodiversity-friendly landscapes. Trends Ecol. Evol. 36, 919–930. doi: 10.1016/j.tree.2021.06.010
Tully, K., and McAskill, C. (2020). Promoting soil health in organically managed systems: a review. Organ. Agric. 10, 339–358. doi: 10.1007/s13165-019-00275-1
Tyagi, J., Sultan, E., Mishra, A., Kumari, M., and Pudake, R. N. (2018). “The impact of amf symbiosis in alleviating drought tolerance in field crops,” in Mycorrhiza—Nutrient Uptake, Biocontrol, Ecorestoration, eds A. Varma, R. Prasad, and N. Tuteja (Switzerland: Springer), 533.
Ulrichs, C., Fischer, G., Buttner, C., and Mewis, I. (2008). Comparison of lycopene, β-carotene and phenolic content of tomato using conventional and ecological horticultural practices and arbuscular mycorrhizal fungi (AMF). Agron. Colomb. 26, 40–46. Available online at: https://www.academia.edu/54567020/Comparison_of_lycopene_%CE%B2_carotene_and_phenolic_content_of_tomato_using_conventional_and_ecological_horticultural_practices_and_arbuscular_mycorrhizal_fungi_AMF
UN Report (2022). The Sustainable Development Goals Report, United Nations Publication Issued by the Department of Economic and Social Affairs (DESA). Available online at: https://unstats.un.org/sdgs/report/2022/The-Sustainable-Development-Goals-Report-2022.pdf (accessed June 11, 2021).
Upadhayay, V. K., Singh, J., Khan, A., Lohani, S., and Singh, A. V. (2019). “Mycorrhizal mediated micronutrients transportation in food-based plants: a biofortification strategy,” in Mycorrhizosphere and Pedogenesis, eds A. Varma, and D. K. Choudhary (Singapore: Springer), 1–24. doi: 10.1007/978-981-13-6480-8_1
Üstüner, Ö., Raviv, M., Medina, S., Wininger, S., Gadkar, V., Badani, H., et al. (2009). Evaluation of different compost amendments with is fungal inoculum for optimal growth of chives. Compost Sci. Utiliz. 17, 257–265. doi: 10.1080/1065657X.2009.10702432
Uwamungu, J. Y., Shi, G., Wang, Y., Paliwal, A., Jadhav, R. R., and Wani, A. W. (2022). “Arbuscular mycorrhizal fungi (AMF) for sustainable soil and plant health,” in Microbial and Biotechnological Interventions in Bioremediation and Phytoremediation, ed J. A. Malik (Cham: Springer). doi: 10.1007/978-3-031-08830-8_6
Vågsholm, I., Arzoomand, N. S., and Boqvist, S. (2020). Food security, safety, and sustainability—getting the trade-offs right. Front. Sustain. Food Syst. 4, 16. doi: 10.3389/fsufs.2020.00016
Valsalakumar, N., Ray, J. G., and Potty, V. (2007). Arbuscular mycorrhizal fungi associated with green gram in South India. Agron. J. 99, 1260–1264. doi: 10.2134/agronj2006.0367
van der Heijden, M. G. A., Boller, T., Wiemken, A., and Sanders, I. R. (1998), Different arbuscular mycorrhizal fungal species are potential determinants of plant community structure. Ecology 79:2082–2091. doi: 10.1890/0012-9658(1998)079[2082:DAMFSA]2.0.CO;2
van Geel, M., Ceustermans, A., van Hemelrijck, W., Lievens, B., and Honnay, O. (2015). Decrease in diversity and changes in community composition of arbuscular mycorrhizal fungi in roots of apple trees with increasing orchard management intensity across a regional scale. Mol. Ecol. 24, 941–952. doi: 10.1111/mec.13079
van Geel, M., Verbruggen, E., de Beenhouwer, M., van Rennes, G., Lievens, B., and Honnay, O. (2017). High soil phosphorus levels overrule the potential benefits of organic farming on arbuscular mycorrhizal diversity in northern vineyards. Agric. Ecosyst. Environ. 248, 144–152. doi: 10.1016/j.agee.2017.07.017
Vasilikiotis, C., Li, M., Schmidt, J. E., Azimi, A., Garcia, J., Volder, A., et al. (2020). Orchard management practices affect arbuscular mycorrhizal fungal root colonisation of almonds. Biol. Agric. Hortic. 36, 230–248. doi: 10.1080/01448765.2020.1802777
Veiga, R. S. L., Jansa, J., Frossard, E., and van der Heijden, M. G. A. (2011). Can arbuscular mycorrhizal fungi reduce the growth of agricultural weeds? PLoS ONE 6, e0027825. doi: 10.1371/journal.pone.0027825
Vera-Herrera, L., Romo, S., and Soria, J. (2022). How agriculture, connectivity and water management can affect water quality of a Mediterranean coastal wetland. Agronomy 12, 486. doi: 10.3390/agronomy12020486
Verbruggen, E., Röling, W. F. M., Gamper, H. A., Kowalchuk, G. A., Verhoef, H. A., and van der Heijden, M. G. A. (2010). Positive effects of organic farming on below-ground mutualists: large-scale comparison of mycorrhizal fungal communities in agricultural soils. New Phytol. 186, 968–979. doi: 10.1111/j.1469-8137.2010.03230.x
Wahdan, S. F. M., Reitz, T., Heintz-Buschart, A., Schädler, M., Roscher, C., Breitkreuz, C., et al. (2021). Organic agricultural practice enhances arbuscular mycorrhizal symbiosis in correspondence to soil warming and altered precipitation patterns. Environ. Microbiol. 23, 6163–6176. doi: 10.1111/1462-2920.15492
Wang, F. (2017). Occurrence of arbuscular mycorrhizal fungi in mining-impacted sites and their contribution to ecological restoration: mechanisms and applications. Crit. Rev. Environ. Sci. Technol. 47, 1901–1957. doi: 10.1080/10643389.2017.1400853
Wang, H., Hao, Z., Zhang, X., Xie, W., and Chen, B. (2022). Arbuscular mycorrhizal fungi induced plant resistance against fusarium wilt in jasmonate biosynthesis defective mutant and wild type of tomato. J. Fungi 8, 422. doi: 10.3390/jof8050422
Wang, J., Chadwick, D. R., Cheng, Y., and Yan, X. (2018). Global analysis of agricultural soil denitrification in response to fertiliser nitrogen. Sci. Total Environ. 616–617, 908–917. doi: 10.1016/j.scitotenv.2017.10.229
Wang, L., Zhang, L., George, T. S., and Feng, G. (2023), A core microbiome in the hyphosphere of arbuscular mycorrhizal fungi has functional significance in organic phosphorus mineralization. New Phytolol. doi: 10.1111/nph.18642
Wang, W., Shi, J., Xie, Q., Jiang, Y., Yu, N., and Wang, E. (2017). Nutrient exchange and regulation in arbuscular mycorrhizal symbiosis. Mol. Plant 10, 1147–1158. doi: 10.1016/j.molp.2017.07.012
Wang, X. X., Wang, X., Sun, Y., Cheng, Y., Liu, S., Chen, X., et al. (2018). Arbuscular mycorrhizal fungi negatively affect nitrogen acquisition and grain yield of maize in N deficient soil. Front. Microbiol. 9, 418. doi: 10.3389/fmicb.2018.00418
Wang, Z. G., Bi, Y. L., Jiang, B., Zhakypbek, Y., Peng, S. P., Liu, W. W., et al. (2016). Arbuscular mycorrhizal fungi enhance soil carbon sequestration in the coalfields in northwest China. Sci. Rep. 6, 34336. doi: 10.1038/srep34336
Wu, Q. S., Li, Y., Zou, Y. N., and He, X. H. (2015). Arbuscular mycorrhiza mediates glomalin-related soil protein production and soil enzyme activities in the rhizosphere of trifoliate orange grown under different P levels. Mycorrhiza 25, 121–130. doi: 10.1007/s00572-014-0594-3
Yadav, S. K., Babu, S., Yadav, M. K., Singh, K., Yadav, G. S., and Pal, S. (2013). A review of organic farming for sustainable agriculture in northern India. Int. J. Agron. 2013, 718145. doi: 10.1155/2013/718145
Yang, T., Lupwayi, N., Marc, S. A., Siddique, K. H. M., and Bainard, L. D. (2021). Anthropogenic drivers of soil microbial communities and impacts on soil biological functions in agroecosystems. Glob. Ecol. Conserv. 27, e01521. doi: 10.1016/j.gecco.2021.e01521
Yang, Y., He, C., Huang, L., Ban, Y., and Tang, M. (2017). The effects of arbuscular mycorrhizal fungi on glomalin-related soil protein distribution, aggregate stability and their relationships with soil properties at different soil depths in the lead-zinc contaminated area. PLoS ONE 12, e0182264. doi: 10.1371/journal.pone.0182264
Ye, L., Zhao, X., Bao, E., Li, J., Zou, Z., and Cao, K. (2020). Bio-organic fertilizer with reduced rates of chemical fertilisation improves soil fertility and enhances tomato yield and quality. Sci. Rep. 10, 177. doi: 10.1038/s41598-019-56954-2
Zhang, B., Li, S., Chen, S., Ren, T., Yang, Z., Zhao, H., et al. (2016). Arbuscular mycorrhizal fungi regulate soil respiration and its response to precipitation change in a semiarid steppe. Sci. Rep. 6, 19990. doi: 10.1038/srep19990
Zhang, J., Su, L., Yan, K., Li, M., He, Y., Zu, Y., et al. (2021). An arbuscular mycorrhizal fungus increased the macroaggregate proportion and reduced cadmium leaching from polluted soil. Int. J. Phytoremed. 23, 684–692. doi: 10.1080/15226514.2020.1849014
Zhang, Z., Mallik, A., Zhang, J., Huang, Y., and Zhou, L. (2019). Effects of arbuscular mycorrhizal fungi on inoculated seedling growth and rhizosphere soil aggregates. Soil Tillage Res. 194, 104340. doi: 10.1016/j.still.2019.104340
Zhu, C., Ling, N., Guo, J., Wang, M., Guo, S., and Shen, Q. (2016). The impact of fertilisation regimes on arbuscular mycorrhizal fungal (AMF) community composition was correlated with organic matter composition in maize rhizosphere soil. Front. Microbiol. 7, 1840. doi: 10.3389/fmicb.2016.01840
Zou, Y.-N., Wu, Q.-S., and Kuča, K. (2020). Unravelling the role of arbuscular mycorrhizal fungi in mitigating the oxidative burst of plants under drought stress. Plant Biol. 23, 50–57. doi: 10.1111/plb.13161
Zou, Y. N., Srivastava, A. K., and Wu, Q. S. (2016). Glomalin: a potential soil conditioner for perennial fruits. Int. J. Agric. Biol. 18, 293–297. doi: 10.17957/IJAB/15.0085
Keywords: agricultural sustainability, AMF, biofertilizer, sustainable agriculture, soil degradation, soil fertility
Citation: George NP and Ray JG (2023) The inevitability of arbuscular mycorrhiza for sustainability in organic agriculture—A critical review. Front. Sustain. Food Syst. 7:1124688. doi: 10.3389/fsufs.2023.1124688
Received: 15 December 2022; Accepted: 06 February 2023;
Published: 24 February 2023.
Edited by:
Monika Thakur, Amity University, IndiaReviewed by:
Naleeni Ramawat, Agriculture University, Jodhpur, IndiaMohamed Anli, Cadi Ayyad University, Morocco
Sujata Pandit, Sharda University, India
Copyright © 2023 George and Ray. This is an open-access article distributed under the terms of the Creative Commons Attribution License (CC BY). The use, distribution or reproduction in other forums is permitted, provided the original author(s) and the copyright owner(s) are credited and that the original publication in this journal is cited, in accordance with accepted academic practice. No use, distribution or reproduction is permitted which does not comply with these terms.
*Correspondence: Joseph George Ray, amdyYXlAbWd1LmFjLmlu