- 1Indigenous Cropping Systems Laboratory, Tropical Plant and Soil Sciences Department, University of Hawai‘i at Mānoa, Honolulu, HI, United States
- 2Department of Anthropology, University of Hawai‘i at Mānoa, Honolulu, HI, United States
Most neglected and underutilized crops were developed and utilized within indigenous agroecological cropping systems. While crop suitability must consider the constraints of the environment, the conditions of agroecological systems and the role of crops within those systems should be considered. Such consideration may guide the implementation of appropriate farming systems specific to different ecosystems and microhabitats. Using the Hawaiian archipelago as a model system of socioecological dynamics, we consider the distribution of agroecological systems and their associated crops to explore how agroecological suitability changes across climate, topography, and soils. We conduct spatial modeling of the potential nature and extent of seven agroecological archetypes based on historical records. The seven spatial models of pre-colonial agroecological systems produced extents distributed across much of the Hawaiian archipelago, with clear adaptive patterns within and across the islands. The distribution of cropping system further affects the appropriateness of crop species application. We argue that the consideration of agroecological niches and associated cropping systems is critical for realizing the potential of underutilized crops and improving the efficiency of contemporary agricultural systems.
1. Introduction
Neglected and underutilized species (NUS) have been promoted for their potential to enhance agricultural adaptation to climate shifts, improve agricultural nutrient density, foster biodiversity, and support environmental and cultural ecosystem services (Padulosi et al., 2011; Mayes et al., 2012). Indigenous crops, now nearly synonymous with these underutilized species, were sustainably cultivated for thousands of years prior to being displaced for monocultures of major global commodities. Researching these neglected and underutilized crops and thier cultivation practices can draw out lessons and practices of sustainability valuable to the currently unsustainable global food system. While there is an increased emphasis on the crops themselves, it is important to recognize the full suite of factors influencing species selection and crop outcomes, which include their spatiotemporal compatibility within an enviornment, species compatibility to each cropping system, as well as the sociocultural relationships associated with crop species and cultivars. For long-term sustainability and maximization of ecosystem services associated with agricultural systems, consideration of optimal agroecological systems within various landscapes is critical (Winter et al., 2020).
Environmental conditions are a key consideration for crop suitability, as each individual crop has unique demands from the climate and land. However, the conditions required of agroecological systems may further affect the suitability of a crop. As an example, a forest persisting on weak, infertile soils could be cleared and subsequently cropped with a cereal species, which may persist over time but provide sub-optimal yields. An agroforestry system that mimics a forest's nutrient cycling by filling niches with integrated crops and thereby sustaining soil fertility could be a more productive option, though the cereal crop may not be compatible within the parameters of this agroforestry system (e.g., reduced light levels in the understory). Therefore, although the cereal may exhibit reasonable habitat suitability to an environment, it may not exhibit compatibility with the most suitable cropping system. This predicament highlights the achievement of indigenous agroecological systems, which have developed over thousands of years from cumulative knowledge and thoughtfully mimicked natural ecosystem processes by implementing arrangements of crops that efficiently conform to the land (Winter et al., 2020).
To explore the ecological adaptation of cropping systems, we consider the distribution of cropping systems in the Hawaiian archipelago. Firstly, Native Hawaiian agricultural practices are grounded in a sense of kinship, in which integral linkage between human and environmental health are recognized (Beamer et al., 2022). This cultural mentality, emphasizing the balance between people and the environment, helped propagate the Hawaiian agricultural systems, which employed concepts of ecomimicry that simultaneously sought to produce the food and resources demanded by the society while preserving the function and health of the ecosystems (Lincoln and Vitousek, 2017; Winter et al., 2020; Beamer et al., 2022). Also, island ecosystems are often considered ideal model systems for understanding ecological processes (Vitousek, 1995, 2002) and their interactions with human societies (Kirch, 2007). The Hawaiian archipelago in particular offers relatively unique conditions that represent a remarkable opportunity to explore socioecological dynamics.
Socially, Hawai‘i was settled late by a single cultural group (Polynesian), is highly isolated (~4,000 km from the nearest continental land mass), and developed substantial populations (~400–800,000) with high levels of political complexity (Stannard, 1989; Hommon, 2013). These factors confine Hawai‘i culturally, temporally, and geographically as much as possible for any human settlement. Geologically, the archipelago originates from a single, stationary hotspot, representing a near-perfect age gradient on a relatively stable parent basalt. Hawai‘i's tall, broad-sloping volcanoes (up to ~4,200 m) drive predictable temperature patterns and some of the broadest, yet highly orthogonal, rainfall gradients (~200–10,000 mm/yr) on the planet (Giambelluca et al., 2013). Secondary landscape features, such as topography and soil fertility, evolve as a function of time (geologic age) and rainfall. Within this vast matrix of substrate age and rainfall exists endmembers in both macrotopography and soil fertility. The jagged, crumbling cliffs and deep river valleys of Hawai‘i's older islands represent the eroded extreme in topography while much of the original shield surface is still intact on its younger islands. In the case of soil fertility, Hawai‘i is home to some of the most naturally fertile soils in the world, such as the phosphorus-rich Andisols of leeward Kohala on Hawai‘i Island, but also to highly weathered and leached acidic soils, such as Oxisols one may find on Kaua‘i Island.
These vast climatic and geologic age gradients drive ecological diversity, with the Hawaiian Islands being celebrated as one of the most ecologically diverse and dense locations on the planet (Asner et al., 2005). Less acknowledged is the adaptation of the Native Hawaiian peoples to the highly varied ecologies across the islands, particularly in their agricultural endeavors (Lincoln and Vitousek, 2017; Lincoln et al., 2018). Utilizing a limited number of introduced crop species (Whistler, 2009; Lincoln, 2010), Native Hawaiian cultivators adapted their knowledge and practices to take advantage of the tremendous range of arable habitats that the islands provided, with unique systems often in close proximity. Just as Hawai‘i's ecosystem diversity is exceptional—perhaps paramount—on the planet, so is the Native Hawaiians' adaptive radiation of agroecological systems. This adaptation was powered with a number of indigenous and/or Polynesian introduced crops, such as kalo (taro, Colocasia esculenta), ‘ulu (breadfruit, Artocarpus altilis), and kī (ti leaf, Cordyline fruticosa), with 27 crops composing essentially all Native Hawaiian agricultural endeavors. These crops can all be referred to as underutilized species in the sense that their potential to improve people's livelihoods, as well as food security and sovereignty, are not being fully realized because of their limited competetiveness with commodity crops in mainstream agriculture (Padulosi et al., 2011). This is even more so the case if considered at a local scale, where indigenous crop varieties may have special significance, hyper-local adaptations, and preserve cultural traditions.
Understanding the distribution of Native Hawaiian agroecological systems and their associated crops has the potential to inform more intelligent design and integrate adaptive cultivation systems into contemporary agriculture. Although the agroecological adaptations of Hawai‘i are specific to tropical basalt systems, the conceptual framework of agroecological adaptation is relevant to agricultural systems globally. Considering knowledge of inherent ecological processes and the agricultural opportunities and constraints they offer can support the development of modern systems that are more efficient and resilient, preserve ecosystem services and biodiversity, and introduce more diverse crops and foods (Altieri, 1999, 2002, 2018). Although there is extensive documentation and remnants of the centuries-long standing Hawaiian agroecological systems, vast areas were erased by colonial establishment of monoculture plantations, primarily of sugarcane and pineapple. These plantation systems have subsequently failed in the global economy, with the final plantation in Hawai‘i closing in 2016, further suggesting potential relevance for more adaptive and ecologically efficient cropping systems. Regaining the adaptive knowledge from centuries of Hawaiian cultivators therefore comes from remaining Hawaiian agricultural infrastructure, pockets of preserved cultural practices, historical accounts in texts, and current modeling work, which is where we intend to build.
In this paper, we synthesize seven spatial models representing Native Hawaiian agroecological systems that existed at the end of the 18th century. Using historical records, archaeological databases, and present-day environmental landscape parameters, this study maps the potential suitable areas for these seven agroecological archetypes. Here, we build upon previous spatial models of Hawaiian agroecosystems by mapping less intensive, but just as important, agricultural archetypes and define the environmental landscape parameters confining their ecological niches. We also use literature review to identify seasonal cycles, the crops utilized and their functions within each system, providing specific examples of each system from ethnohistorical and scholarly works. Finally, we discuss what we see as relevant takeaways from these traditional adaptations, thier potential integration into the development of sustainable agricultural systems, and the application of underutilized crops today.
2. Materials and methods
Below, we briefly define each archetype in order of assumed preference for more intensive agricultural forms (i.e., higher agriculture yields per area or unit of labor), along with the predominant crop species and associated environmental drivers used to define the modeling parameters for each system (i.e., rainfall, temperature, slope, substrate age, soil type, and water accessibility).
Through study and an extensive literature review of archaeological, ethnohistorical, and various other studies of diverse Hawaiian agricultural systems (Lincoln, 2010, 2020a,b; Lincoln and Ladefoged, 2014; Lincoln et al., 2014, 2017, 2018, 2021; Lincoln and Vitousek, 2017; Winter et al., 2018, 2020; Kagawa-Viviani et al., 2018b; Quintus et al., 2019), we identified seven forms of agroecology that we define and model: Flooded Pondfield, Intensive Rainfed, Marginal Rainfed, Intensive Colluvial Slope, Marginal Colluvial Slope, Agroforestry, and Novel Forests. We synthesized concepts and parameters defined in numerous previous papers (Kirch, 1977; Schilt, 1984; Allen and McAnany, 1994; Allen, 2001; Vitousek et al., 2004; Kirch et al., 2005; Ladefoged et al., 2009; Kurashima and Kirch, 2011; Lincoln and Ladefoged, 2014; Vitousek et al., 2014; Lincoln et al., 2018; Kagawa-Viviani et al., 2018a; Kurashima et al., 2019; Quintus and Lincoln, 2020; Lincoln, 2020a; Lincoln et al., 2021; Morrison et al., 2021) to identify and parameterize these seven agroecological forms of agriculture. These parameters include rainfall, temperature, slope, substrate age, soil type, and water accessibility (Table 1). Utilizing the defined landscape parameters, spatial models were created in R Studio (RStudio, Inc., Boston, MA) using the “raster” and “rgdal” packages (Hijmans and van Etten, 2011; Bivand et al., 2015; R Team, 2015). Environmental and climatic raster and shapefile data were obtained from the Hawai'i Department of Planning GIS database (http://planning.Hawaii.gov/gis/), the US Geological Survey (USGS) (http://pubs.usgs.gov/ of/2007/1089/), the National Elevational Dataset (NED; https://viewer.nationalmap.gov/datasets/), the US Department of Agriculture's National Resource Conservation Service (NRCS) (http://soildatamart.nrcs.usda.gov/), and the Rainfall (http://rainfall.geography.hawaii.edu/) and Evapotranspiration (http://evapotranspiration.geography.hawaii.edu/) Atlases of Hawai'i (Giambelluca et al., 2013, 2014). The spatial models were created for seven of the eight major Hawaiian islands, with the eighth island (Ni‘ihau) excluded due to a lack of reliable environmental data layers. Since landforms that can support more intensive forms of agriculture (that is, agriculture that yields more in terms of production per area) can typically also support other, less intensive forms, each agricultural model is subtracted from all subsequent models to reflect preference. In the following, we briefly define each agricultural type, keystone crops for each system, and the associated environmental parameters used in model construction. Models are presented in order of assumed preference for more intensive agricultural forms.
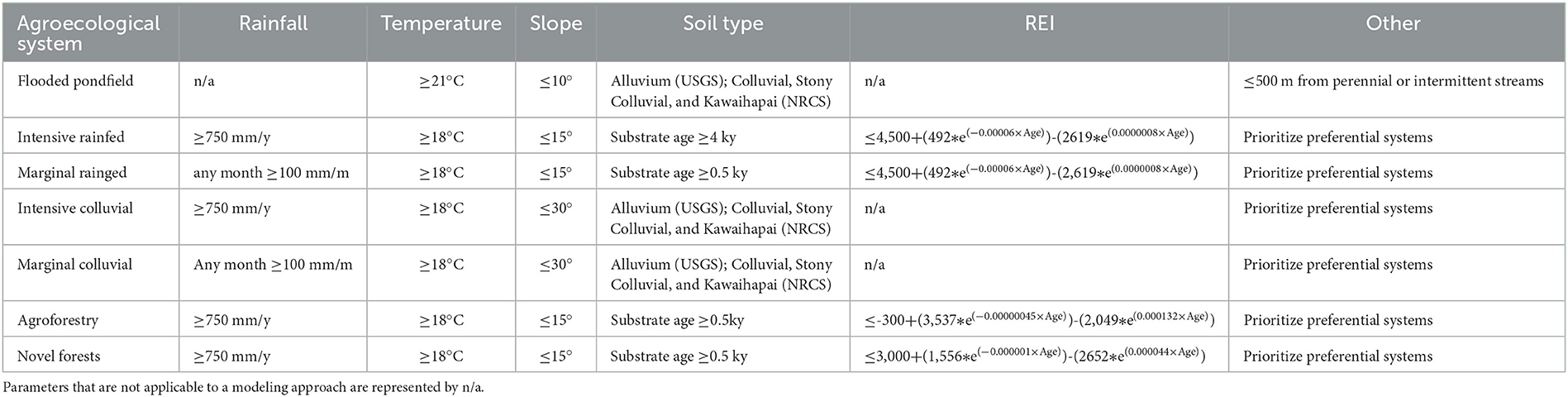
Table 1. Landscape parameters and a brief summary of their characteristics used to model the potential spatial extents of seven pre-colonial Hawaiian agroecological achetypes.
The spatial models are compared to the large body of ethnohistorical and archaeological data describing the extent and form of major traditional agroecological systems in order to generally assess their accuracy. We further utilize a comprehensive ethnohistorical source that describes traditional cultivation for six Hawaiian Islands across 237 ahupua‘a (traditional land division) in order to provide a more systematic and quantitative model validation (Handy, 1940). The literature was further used to qualitatively describe the seasonality, species composition, and functional diversity within the different agroecological systems. The relative species composition for each system archetype was generalized, and qualitative descriptions of the role and micro-niches occupied were noted. This information is used to drive the discussion regarding the application of individual crops within different systems.
2.1. Flooded pondfield
2.1.1. Overview
Pondfields, known as lo‘i in Hawai‘i, were the preferred agricultural features wherever suitable areas of flat land within river valleys or open floodplains were accessible by the gravitational flow of water. Lo‘i occurred in intermittent stream beds, talluvial slopes and alluvial floodplains, and could be fed by water from streams, stream diversions, springs, or the water table (Kirch, 1977). Most commonly, stream systems were diverted through ‘auwai–small canal systems that transferred water to the pondfields and back (Gingerich et al., 2007). Inflow of stream water from these ‘auwai supplied a constant influx of nutrient rich sediments deriving from erosional processes upstream (Palmer et al., 2009). Lo'i systems were dominated by the cultivation of kalo (taro; Colocasia esculenta), the only Polynesian crop introduced to Hawai‘i able to withstand flooded conditions, although other crops were cultivated along the terrace banks and the surrounding areas.
2.1.2. Modeling approach
Modeling of Flooded Pondfield agriculture was restricted to those fed by streams, as reliable environmental spatial data for springs, seepage, or water table depth are not available. Requirements in water supply, temperature, slope, and soil type were constructed independently. Utilizing the shapefile for stream coverage (“darstrams.shp”) from the Hawai‘i Statewide GIS Portal, we selected all streams classified as “perennial” or “intermittent” and applied a 500 m proximity buffer to represent an assumed maximum distribution of stream water. Mean air temperature was used to define a ≥21°C threshold (Onwueme, 1999). The NED at 10 × 10 m resolution was used to define a ≤10° threshold in slope (Earle, 1980; McElroy, 2007). Appropriate substrates were selected from the USGS digital geologic map as “alluvium,” and the NRCS soil survey as “colluvial land,” “stony colluvial land” and “Kawaihapai series” (Cline, 1955; Foote, 1972). The resulting suitable layers for water supply, temperature, slope, and soil type were intersected, with the areas that satisfied all the requirements being used to represent the potential extent of Flooded Pondfield agriculture. This approach closely follows previous modeling efforts (Ladefoged et al., 2009; Kurashima et al., 2019).
2.2. Intensive rainfed
2.2.1. Overview
Intensive rainfed agricultural systems are well-documented in ethnohistory and archaeology, occurring primarily on the leeward slopes of the younger islands where volcanic shield surfaces remain intact (e.g., Newman, 1971, 1972; Allen, 2001; Coil and Kirch, 2005; McElroy, 2007; Ladefoged et al., 2009, 2011). The systems are sometimes referred to as fixed-field systems due to the dominant agricultural infrastructure of kuaiwi–long, linear embankments of earth and/or stone that served both agronomic and social functions (Allen, 2001; Lincoln and Vitousek, 2017; Lincoln et al., 2017). These systems covered vast areas of land, with individual systems ranging up to 15,000 hectares of contiguous agriculture (Ladefoged and Graves, 2008; Ladefoged et al., 2011). Spanning across broad rainfall gradients, these systems are often spatially distinguished as constituting “zones” of planting (Kelly, 1983; Lincoln and Ladefoged, 2014) or exhibit temporal variations with seasonal usage across the system (Lee et al., 2006; Kagawa-Viviani et al., 2018a). Although the crops associated with the vast systems are diverse, the drier regions were clearly dominated by the production of ‘uala (sweet potato; Ipomoea batatas) and ‘uhi (greater yam; Dioscorea alata), while the wetter regions included the cultivation of other staples such as kalo (taro), mai‘a (banana/plantains; Musa spp.), as well as a range of secondary and resource crops such as kī (ti), kō (sugarcane; Saccharum officinarum), ‘awa (kavakava; Piper methysticum), pia (arrowroot; Tacca leontopetaloides), and ‘awapuhi (shampoo ginger; Zingiber zerumbet). Previous work has coupled the occurrence of these high-nutrient demand systems to soil properties that could support the intensification of cropping and adequate rainfall for crop production, resulting in a “sweet spot” of rainfall and pedogenically-derived soil fertility that is dependent on the substrate age (Vitousek et al., 2004, 2014; Ladefoged et al., 2018).
2.2.2. Modeling approach
We follow previous modeling (Ladefoged et al., 2009) in applying a simple Rainfall-Elevation Index (REI) to estimate soil fertility as a function of substrate age, due to the lack of available soil fertility data or high resolution fertility models across the islands. This approach assumes that soil pedogenesis is largely a function of cumulative rainfall (rainfall x time), slightly augmented by temperature (Vitousek et al., 2021, 2022). Previously defined categorical limits (Ladefoged et al., 2009) were fit in a biexponential model to define a continuous REI threshold as a function of substrate age. Raster layers were used to calculate if soil fertility met the threshold conditions at each 10 x 10 m pixel using the following equation:
Geologically young substrates (<4 ky) were excluded due to inadequate soil development (Ladefoged et al., 2009; Lincoln et al., 2014). A minimum annual rainfall necessary for the intensive cultivation of herbaceous crops was defined as 750 mm/yr, a minimum mean air temperature of 18°C, and a maximum slope of 15° (Ngeve et al., 1992; Valenzuela et al., 1994; Ladefoged et al., 2009; Kagawa-Viviani et al., 2018a; Kurashima et al., 2019). The resulting suitability layers for REI, rainfall, temperature, and slope were intersected and areas that satisfied all the requirements were considered the potential extent of intensive rainfed agriculture.
2.3. Intensive colluvial slope
2.3.1. Overview
The lower to middle portions of the valley slopes in Hawai‘i consist of colluvial or talluvial deposits. Active erosion of these slopes transports sediments downwards, which ultimately accumulate on the edge of, and feed into, the alluvial flats. These erosional processes rejuvinated soil fertility and permitted the practice of Hawaiian agriculture on these slopes, with archaeological evidence suggesting that most colluvial slopes were extensively cultivated (Kirch, 1977; Vitousek et al., 2010; Kurashima and Kirch, 2011; Morrison et al., 2021). As evidenced by intensive dryland terracing, stone mounding, and the occasional development of kuaiwi walls, agriculture on colluvial slopes was similar to that of intensive rainfed agriculture. However, the higher environmental heterogeneity of slope, sunlight, and rockiness is reflected in a more ad-hoc layout of infrastructure, with some colluvial areas being more akin to agroforestry suggested by the higher proportion of perennial trees and shrubs. Ethnohistorical sources and botanical surveys indicate Intensive Colluvial systems maintained a greater diversity of crops than Intensive Rainfed systems, that included kalo (taro), ‘ape (swamp taro; Alocasia macrorrhiza), ‘uhi (greater yam), mai'a (banana/plantain), ‘ulu (breadfruit; Artocarpus altilis), pia (arrowroot), kī (ti leaf), olonā (Touchardia latifolia), wauke (paper mulberry; Broussonetia papyrifera) and kukui (candlenut; Aleurites moluccanus).
2.3.2. Modeling approach
While the fertility of volcanic shield surfaces is defined by the amount of weathering those shield surfaces have been subjected to, and is therefore largely depleted and incapable of supporting intensive rainfed agriculture by ~700 ky (Vitousek and Farrington, 1997; Porder and Chadwick, 2009; Chadwick et al., 2021), the active erosion and sediment accumulation associated with colluvial slopes has been shown to adequately regenerate soil fertility to support intensive agricultural production (Vitousek et al., 2010). This relationship is reflected in soil type classifications that were selected for modeling intensive colluvial systems, which included “colluvial land,” “stony colluvial land,” and “Kawaihapai” soil series (Cline, 1955) collected from the NRCS soil survey maps, and “alluvium” and “older alluvium” substrates were selected from the USGS digital geologic map. These two extents were combined to represent appropriate soil substrates rejuvenated through erosional deposits, satisfying the soil fertility constraint of this system of intensive production. Limitations of slope were defined as ≤30°, for which archaeological remnants are clearly documented on slopes up to this intensity (Kurashima and Kirch, 2011; Morrison et al., 2021). We utilize the same rainfall (≥750 mm/yr) and temperature (≥18°C) limitations applied to Intensive Rainfed agriculture. The suitable extents for soil type, slope, rainfall, and temperature were then intersected to define the extent of Intensive Colluvial agriculture.
2.4. Marginal/seasonal rainfed
2.4.1. Overview
Rainfed agriculture was not restricted to the intensive, fixed-field systems described above, but took on a range of other forms in more marginal areas, such as field mounds, micro-sites, modified outcrops or depressions, among others. These less intensive forms of agricultural were spatially more sporadic across the landscape occurring in areas that met some of the environmental requirements for intensive rainfed systems, but not all. Thus, these systems reflect a more opportunistic approach to cultivation, occurring on young, undeveloped soils (< 4 kya) and areas subject to highly variable seasonal rainfall. These developments consisted almost exclusively of ‘uala (sweet potato), the most drought-resistant edible crop cultivated in Hawai'i, although other drought tolerant crops are also associated with them such as ipu (bottle gourd; Lagenaria siceraria). When ‘uala and ipu crops are rooted in pockets of soil or moist microsites their vines can extend out to cover a broader area.
2.4.2. Modeling approach
The modeling approach for marginal/seasonal rainfed agriculture utilized similar parameters as intensive rainfed agriculture, with modifications to the required rainfall and substrate age. With respect to rainfall, we adopt a threshold of any single month receiving ≥100 mm of rainfall as adequate to support at least one crop of sweet potato. This is consistent with other literature that suggests adequate moisture within the first 40 days of planting will result in sweet potato tuberization (Hahn, 1977; Wilson, 1977; Ngeve et al., 1992; Valenzuela et al., 1994; Kagawa-Viviani et al., 2018a). The same REI equation previously outlined for Intensive Rainfed agriculture was used, though substrates 0.5–4ky were included as cultivation of these young flows is well-documented in historical and archaeological literature (Schilt, 1984; Ellis, 2009; Horrocks and Rechtman, 2009).
2.5. Marginal colluvial slope
2.5.1. Overview
As with marginal rainfed systems, sparser agricultural infrastructure alongside perennial plantings have been documented on colluvial slopes in areas too dry or steep to warrant more intensive development. Here, dryland hardwood trees, such as milo (Pacific rosewood; Thespesia populnea), kou (Cordia subcordata), and kamani (Alexandrian laurel; Calophyllum inophyllum), along with the sporadic cultivation of drought-tolerant ‘uala (sweet potato) and ipu (bottle gourd), occurred to take advantage of the fertile, but more arid, soils.
2.5.2. Modeling approach
The modeling of Marginal Colluvial cultivation utilized the same parameters as those outlined for colluvial slope agriculture (i.e., soils, temperature, and slope), differing only in the applied rainfall threshold of ≥100 mm per month—the same as for marginal rainfed agriculture. The suitable extents for soil type, temperature, slope, and rainfall were intersected to define the potential agroecological habitat for Marginal Colluvial agriculture.
2.6. Agroforestry/swidden
2.6.1. Overview
Although modeled as a dicrete agroecological type, agroforestry represents a spectrum of forms and methods that were dominated by trees. Methods of agroforestry were used that incorporated an array of perennial and annual crops, largely in areas that were too rocky or infertile for the cultivation of other crops without the nutrient enrichment and microhabitats created underneath the tree crop canopies (Lincoln and Vitousek, 2017). Among the most well-documented are the pā systems (i.e., pākukui and pāhala), in which trees that were quick to mature and rapidly decompose, such as kukui (candlenut) or hala (screwpine; Pandanus tectorius), were extensively cultivated primarily to support kalo (taro) production (Kalokuokamaile, 1922; Handy, 1940; Handy et al., 1972; Lincoln, 2020a). In pā systems, trees are cultivated as both a resource and as a source of mulch to support nutrient concentration. On young lava substrates, the trees are cut for mulch, and composted in depressions in the lava, while on older substrates, large areas are cleared to dig pits for composting of leaves and small branches (Beckwith, 1932). Vast areas were converted for use in these shifting systems, so that “The broad slopes of the wet windward coast of Hawai'i between the abrupt eroded shore and the high forest...were completely covered by kukui forest. … where were open glades called pākukui in which taro was grown” (Handy et al., 1972, p. 231). Other systems were akin to diversified orchards, in which crop trees such as ‘ulu (breadfruit) and kukui (candlenut) were planted in a well-spaced orientation to support nutrient cycling, aid in water retention, and create micro-habitats suitable to a range of crops such as mai‘a (banana/plantain), wauke (paper mulberry), mamaki (Hawaiian nettle; Pipturus albidus), awapuhi (Shampoo ginger), ‘awa (kavakava) and kalo (taro) (Lincoln and Ladefoged, 2014). In some regions, patches of native forests were preserved within the agricultural mosaic to support the cultivation of specific species, while in contrast there are also documentation of large stands of monocultured tree crops (Lincoln and Vitousek, 2017).
2.6.2. Modeling approach
The establishment of Agroforestry extents relied on many of the same environmental parameters associated with Intensive Rainfed agriculture, including temperature, slope, and rainfall. However, the application of tree crops allowed for persistence in lower fertility soil systems and areas too rocky to support intensive cultivation of tuber crops, effectively allowing for the cultivation of older substrates and wetter environments. We apply previous data demonstrating the delineation of agroforestry systems in pre-contact Hawai‘i (Lincoln, 2020a; Lincoln et al., 2021) to fit a bi-exponential equation delineating suitable REI as follows:
Substrates <0.5 ky were excluded as statewide surveys of core agroforestry trees of kukui (candlenut) (Lincoln et al., 2021) and ‘ulu (breadfruit) (Mausio et al., 2020) did not record trees on substrates of these ages. The suitable extents for REI, rainfall, temperature, and slope were intersected to determine the potential suitable areas for Agroforestry systems.
2.7. Novel forest/arboriculture
2.7.1. Overview
Low intensity forms of arboriculture are extensively documented across Polynesia and are a dominant form of agriculture in terms of land area utilized on most islands (Quintus et al., 2019). Novel forests can take multiple forms, with varying degrees of native forest interference. In some cases, the native forest canopy was retained and shade tolerant crops, such as mai‘a (banana/plantain), ‘uhi (greater yam), hoi (bitter yam, Dioscorea bulbifera), mamaki (Hawaiian nettle), and olonā, were cultivated or, in the very least, encouraged (Handy et al., 1972). Such was the case of the well-documented ‘āpa'a zone on the leeward side of Hawai‘i Island (Lincoln and Ladefoged, 2014; Kelly, 1983). In other cases, the native forests were replaced over time by Polynesian introduced trees, such as ‘ulu (breadfruit), kukui (candlenut), and ‘ohi‘a‘ai (mountain apple; Syzygium malaccense), along with some shade tolerant crops and native species in the understory (Handy et al., 1972; Meilleur et al., 2004; Lincoln, 2020a). Such transitions are often reflected in the archaeological record, depicting a gradual transition from native to introduced trees over time in charcoal, paleobotanical remains, and wooden artifacts. Nonetheless, these less intensively managed tree cropping systems distinguish Novel Forests from Agroforestry.
2.7.2. Modeling approach
Novel forests were applied in areas that were highly infertile, either due to excessive nutrient leaching caused by high rainfall or very old substrates that have been subjected to high amount of cumulative weathering over time. Previous work has demonstrated differing levels of soil fertility as the deciding factor seperating the application of Novel Forests and Agroforestry, and further relates a lower threshold of soil fertility to the development of Novel Forests (Lincoln, 2020a). Using a statewide distribution of kukui (candlenut) as an indicator species of novel forest development (Lincoln et al., 2021), the lower boundary of a REI was derived using the biexponential equation as follows:
Substrates <0.5 ky were excluded as indicator species were not documented on flows these ages. The same limitations of rainfall, temperature, and slope as was indicated for Agroforestry were utilized with the unique REI threshold, with the intersection of all parameter suitability representing the potential area in which Arboriculture could be applied.
3. Results
3.1. Distribution of traditional agroecology
The seven spatial models representing the potential of pre-colonial agroecological systems produced spatial extents distributed across much of the Hawaiian archipelago (Figure 1). The potential extent of total land area for each agroecological system across the state varied from 0.6 to 9.0%, with the sum extent of all the systems equivalent to 27.5% of the archipelago (Table 2). As with many Polynesian islands, arboriculture played a dominant role in the overall agricultural landscape, with both the Agroforestry and Novel Forest categories accounting for a significant portion of the total agricultural potential (32.9 and 25.6%, respectively).
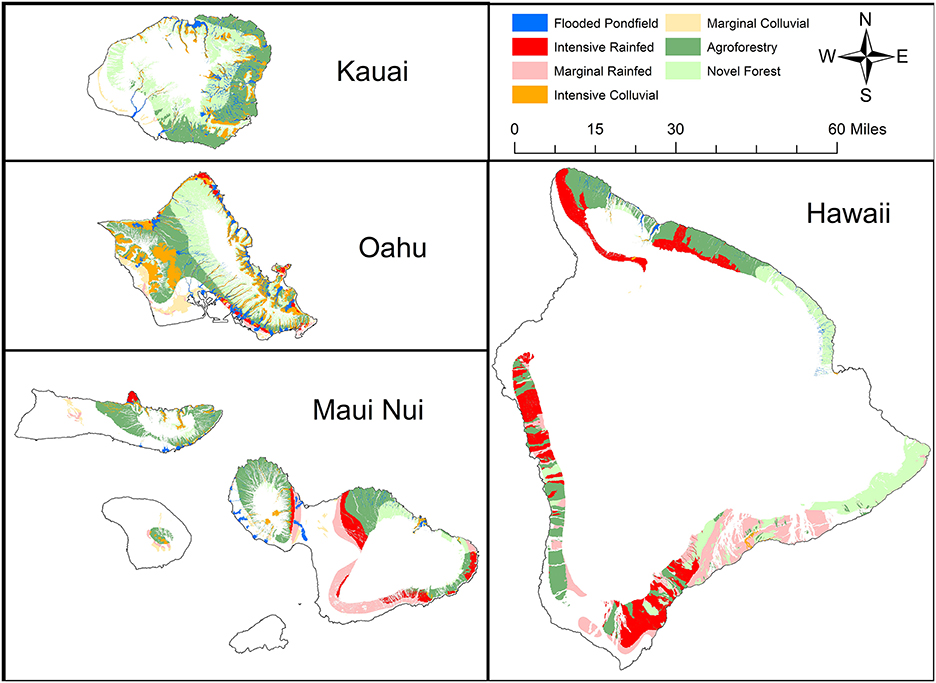
Figure 1. The modeled spatial distribution of the seven agroecological archetypes across the Hawaiian islands.
The portion of lands converted to types of agriculture varied considerably by substrate age and therefore by island, producing patterns in predominant forms of agriculture across the archipelago (Figure 2). The model results indicated some production systems are intrinsically tied together, as seen by similar patterns of extents of Flooded Pondfield and the Colluvial systems. Although modeled using very different approaches, these systems are largely dependent on the formation of river valleys, and therefore follow similar patterns across time and space. Flooded Pondfield systems, along with Intensive and Marginal Colluvial cultivation, have the highest percentage of total agricultural land modeled for the older, more weathered islands of Kaua'i (8, 12, 2%, respectively) and O'ahu (8, 24, and 6%, respectively), while the estimated percentage of these agricultural systems for Maui Nui (1, 0.5, <0.5%, respectively) and Hawai'i Island (<0.5% for all) were considerably less. In contrast, the modeled extents for Intensive and Marginal Rainfed agriculture, were higher for these younger, less eroded islands of Hawai'i (27 and 20%, respectively) and Maui Nui (13 and 18%, respectively) where shield surfaces remain intact, while the modeled extents for these systems combined were minimal for Kaua'i (0%) and O'ahu (5%).
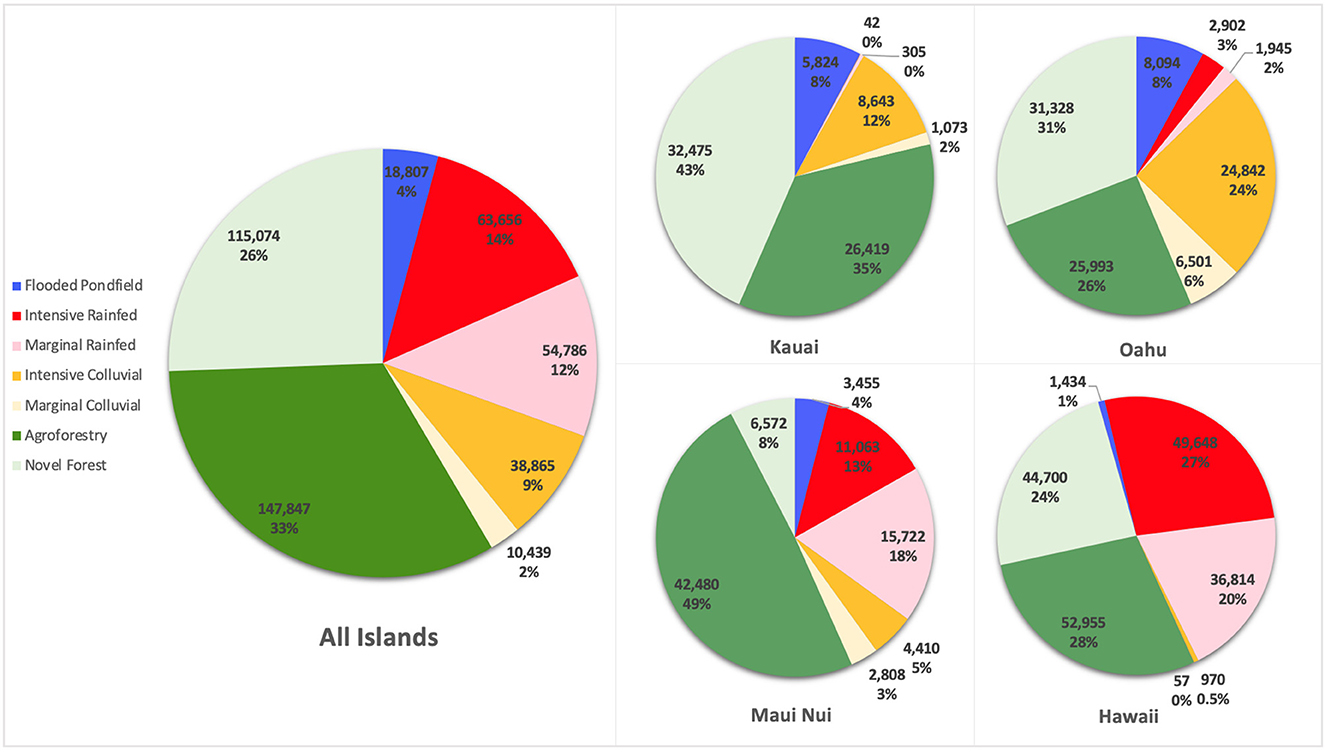
Figure 2. The percent contribution to the agricultural potential for each agroecological archetype by island and for the archipelago as a whole. Maui Nui combines Maui with the three smaller surrounding islands of Moloka'i, Lāna'i, and Kaho'olawe.
The spatial models are consistent with the large body of ethnohistoric and archaeological data describing the form and extent of traditional agroecological systems. More specifically, we find the models align well with the documented extents of the major dryland field systems that have been well studied (Newman, 1972; Allen, 2001; Coil and Kirch, 2005; Kirch et al., 2005; McCoy, 2005; McCoy and Hartshorn, 2007; Ladefoged and Graves, 2008; Ladefoged et al., 2011; Lincoln et al., 2014; Quintus and Lincoln, 2020), capture areas known to have utilized marginal dryland methods (Schilt, 1984; Allen and McAnany, 1994), correspond with the sparse documentation of colluvial slope systems (Kurashima and Kirch, 2011; Morrison et al., 2021), capture the documented extent of agroforestry systems (Lincoln and Ladefoged, 2014; Quintus et al., 2019; Lincoln, 2020a), encompass the extent of remotely-sensed indicators of traditional novel forests (Mausio et al., 2020; Lincoln et al., 2021), and are consistent with previously validated models of wetland agriculture (Ladefoged et al., 2009). Utilizing arguably the most comprehensive and spatially explicit ethnohistorical source (Handy, 1940) to examine positive prediction of agroecological application, the models suggests a high degree of accuracy (Table 3). These numbers closely align with previously conducted validation for Flooded Pondfield, Intensive Dryland, and Intensive Colluvial Slope agriculture (Ladefoged et al., 2009; Kurashima et al., 2019).
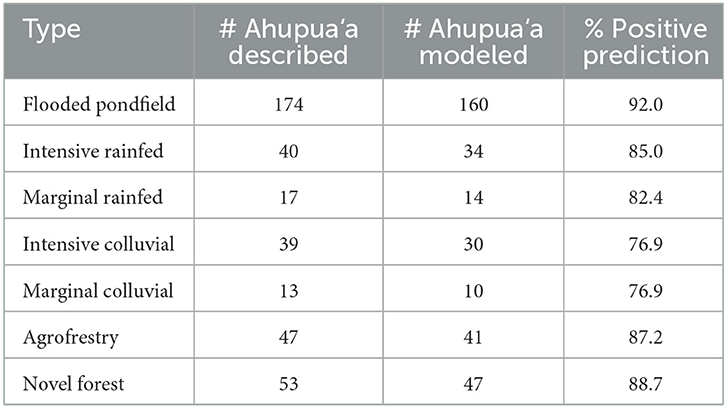
Table 3. Comparison of agroecological distribution described in Handy (1940) and corresponding representation of those forms in the modeled extents.
3.2. Crop associations and patterns
Our review of the literature indicates that each agroecological system supported a distinct suite of crops. Informed by extensive study and literature reviews of various Hawaiian agricultural systems, we compiled a list of 27 crop species and their association with each of the agroecological systems (Table 4). These include traditional Native Hawaiian crops that are commonly cultivated in the global food system, such as ‘uala (sweet potato) and ‘olena (turmeric), along with heavily underutilized crops, such as hala (screwpine) and noni (Indian mulberry). We found that each of the 27 crop species were utilized in at least three different agroecological systems, each of which hosted between 12 and 25 of the 27 crops species. More notably, two crops–kukui (candlenut) and niu (coconut)–were shown to play roles in all seven agroecological systems.
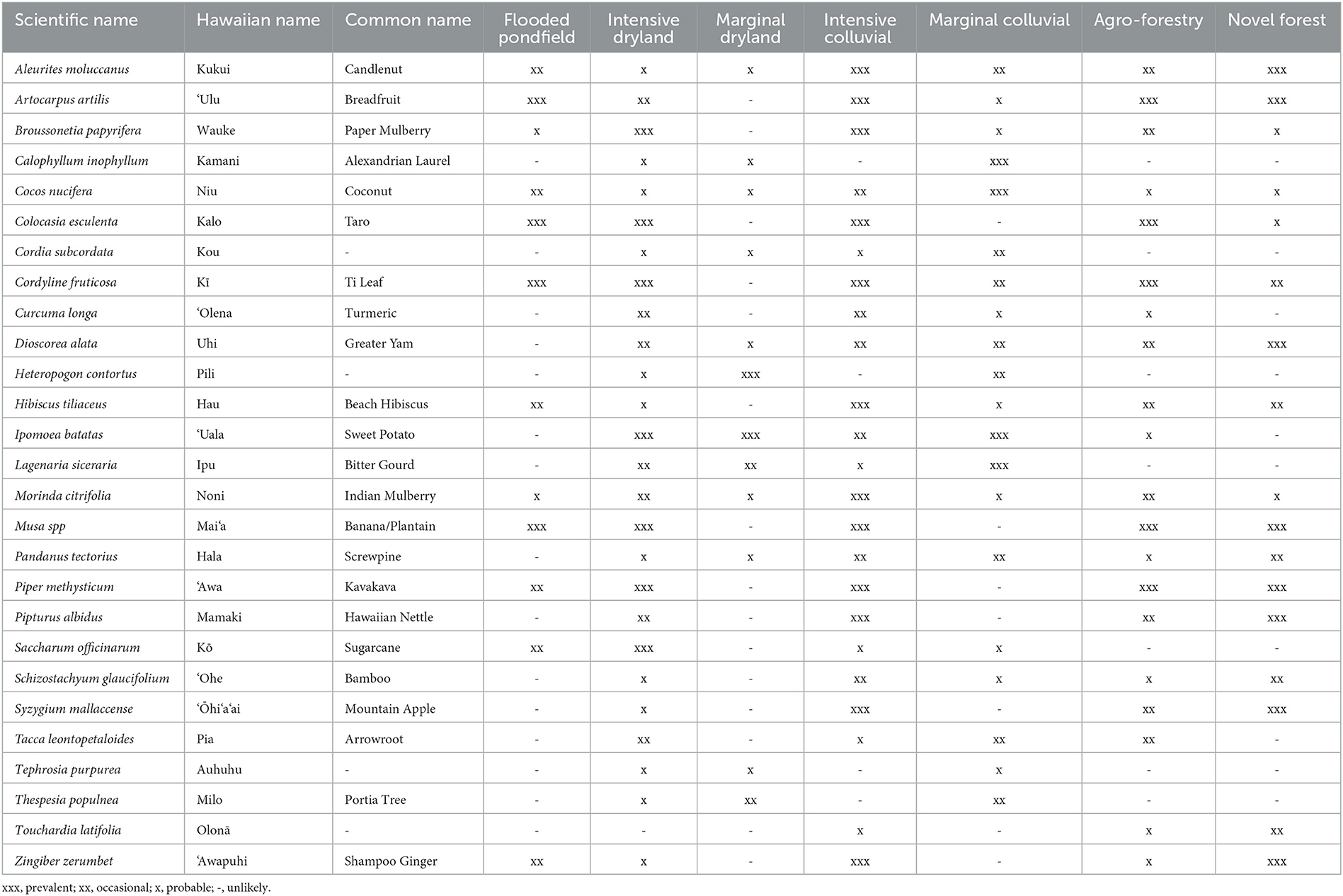
Table 4. Most utilized Hawaiian crop species for food, fiber, medicine, and ceremonial purposes and their association with the seven agroecological types modeled in this paper.
Within the individual systems, crops were cultivated with varying degrees of intensification based on landscape compatibility and environmental parameters, with each crop species purposefully occupying discrete ecological niches within each system. Crops were applied in a multitude of roles, including as primary crops in intensive agricultural systems, as secondary crops in more marginal areas unsuitable for sustaining more intensive cultivation practices, and as a resource to increase cultivable land areas. For example, the dominant staple crop of kalo (taro) was cultivated as a monoculture in the Flooded Wetland systems, as patches of diversified agriculture in Intensive Rainfed systems, and even more sparsely in the light gaps between tree crops of Agroforestry or Novel Forest systems. As the preferred staple crop, we can infer Hawaiian populations would have emphasized kalo cultivation to the maximum extent. The varying intensification of kalo cultivated in each type of system, therefore, is indicative of how much each system could reasonably support. However, the limitations to cultivation can vary and are predominantly restricted by water, nutrients, or light. These three factors can limit productivity of co-crops as well, and may exhibit interplay between crops. Agroforestry systems, for instance, were established in areas where soil fertility was inadequate to support intensive annual cropping. The use of perennial trees, such as ‘ulu (breadfruit), in these areas were two-fold, creating both opportunities and constraints to the agricultural production. In these Agroforestry systems, multi-use tree crops served several functions by providing food, aiding in nutrient cycling and moisture retention, creating suitable micro-environments for other crops, and providing a source of lumber/mulch, but also diminished the quality of light available to herbaceous crops. Due to decreased light inputs, understory crops such as mai‘a (banana/plantain) and wauke (paper mulberry) were paired with tree crops in these Agroforestry systems in order to fill the more shade-tolerant ecological niche. As another example, on young lava flows with limited soil development, trees, particularly kukui (candlenut) and hala (screwpine), were established in deep cracks, and their mulch was then used to accumulate compost in lava depressions, where annual crops could then be cultivated. In drier regions, kalo was planted opportunistically in the dripline of trees such as ‘ulu and kukui to more efficiently concentrate the limited water resources in the area.
The agroecological niche systems, although modeled spatially, also incorporated aspects of temporal management, where planting times were selected to optimize the habitat in terms of temperature or water availability. Marginal Dryland systems were defined to meet the minimal amount of monthly moisture necessary to establish a single crop of ‘uala (sweet potato), ipu (bitter gourd), and other drought tolerant crops. The extent of these marginal systems was not used continuously throughout the year, but shifted cultivation across the landscape throughout the year depending on the season (Kagawa-Viviani et al., 2018a). Other accounts further discuss the seasonal usage of marginal zones, particularly Marginal Dryland zones, in which specified areas were cultivated with rapidly maturing crops during the wet season, and temporarily abandoned during the dry season (Handy et al., 1972). These strategies can be reflected in common sayings, such as “Ua ka ua, i hea ‘oe?” (When it rained, where were you?), suggesting the importance of planting when the rains come (Pukui, 1983). Such adaptations by Native Hawaiian farmers recognized the limited temporal productivity of an ecosystem and capitalized on these opportunities through effective land management practicies.
4. Discussion
The consideration of agroecological niches and associated indigenous cropping systems is, we argue, critical for realizing the potential of underutilized crops in improving the efficiency of modern cropping. The incorporation of geospatially modeled agroecological constraints with an understanding of the associated practices, offers an alternative approach to evaluate site-specific system suitability under low-input agricultural systems, while also providing a more robust comprehension of the varying elements and techniques that should be critically considered. Cropping systems can enhance the maximum capacity of the landscape through innovative practices and ecological adaptations, allowing crop species to thrive. Socially, cropping systems are a product of generational knowledge, concepts, and practices. Unfortunately, colonization and now globalization has led to a decline in such knowledge, contributing to the underutilization of many crops today. While underutilized crops have tremendous potential to play a significant role in addressing issues in global food systems, it is important to remember that these crops were developed and grown in unique cropping systems and with unique practices as a result of site-specific adaptation by traditional cultivators. Direct transfer of underutilized crops into contemporary, Euro-centric concepts of agriculture may not allow for their full potential to be realized.
The agroecological niches modeled in this paper for the Hawaiian Islands demonstrate a strong degree of adaptation by traditional cultivators to various ecosystems. Utilizing a little over two dozen crops, Native Hawaiian cultivators applied a broad range of adaptive agricultural systems that allowed them to exploit the limited amount of suitable land area. These adaptations allowed for the cultivation of crops in over a quarter of the total land area on the islands, across a breadth of diverse and highly contrasting ecological and topographical variation. Systems were constructed and sustained across vast environmental conditions, cultivating flatlands to steeply sloped areas (≤30°), and zones with annual precipitation ranging from 500 to over 5,000 mm/yr, while spanning an age gradient of soil substrates including cultivating the young undeveloped lava flows of Hawai'i Island (>0.5 kya) to highly eroded, nutrient-depleted soils (>5 mya).
The modeled extent of all systems covers approximately one-fourth of the total land area for Hawai‘i, suggesting that Native Hawaiian cultivators had the potential to alter a substantial portion of the landscape. These figures suggest a much larger footprint of land conversion in Hawai‘i than previously postulated, which ranges from ~6.0–9.2% in previous work (Gon et al., 2018; Kurashima et al., 2019) compared to 27.5% in this study. Today, Hawai‘i has ~47% (781,000 ha) of its land zoned for agriculture, however only about 4% of the land base is used for cultivation, including food, forestry, seed, and flowers, while another ~20% is used for pasture (Perroy et al., 2016). In comparison, utilizing a diverse, yet limited number of crop species, Native Hawaiians used adaptive forms of agroecology to maximize the productive capacity of the landscape, while minimizing the impact to ecosystems (Winter et al., 2020). By integrating suitable agricultural systems that preserved the form and function of ecosystem services for any given environment, Native Hawaiians more sustainably cultivated the land than post-European plantation agriculture.
It is important to recognize that our spatial modeling represents the potential for the niche construction of each agroecological system, and is not meant to suggest that the areas were developed in their entirety. Some agroecological systems would have approached 100% conversion of the potential habitat, such as the highly preferred Flooded Pondfield agriculture, where “along the river…on either side...the land had been terraced and walled…all the way…where the last available space was won” (Lydgate, 2021, p. 126). However, the less intensive and more extensive systems such as Novel Forests and Marginal Dryland were developed in patchier, less complete ways. Therefore, while the model represents the total areas that could be developed, the extent and completeness of this development is unclear. Our model validation shows that, at the archipelago level, the extents of the models well cover the distribution of the reported application of each system. However, more thorough incorporation of archaeological documentation and detailed case studies are needed to better understand the relationship between the models and actual development.
The relationship between the cropping system and the crop species is multifaceted. In many cases, it is likely that crop species or varieties developed specific traits through selection in response to the form and function of the cropping systems that they were grown in. In this paper, rather than examine the distribution of individual crops, we explore a range of agroecological cropping systems across the highly diverse ecologies of the Hawaiian archipelago. Each of these cropping systems were utilized to grow a range of crops that, by and large, are considered underutilized crops today. Conversely, each crop was able to be grown across different systems of production, taking on different roles and levels of dominance within the different archetypes. Arguably, the application of different agroecological systems would inherently favor underutilized crops as they have not been the subject of intensive breeding for high-input and monocropped agricultural systems.
A broad range of agroecological systems are described in the literature, ranging from highly intensive systems that approach monocropping, to extremely diverse, low-intensity systems. Although most systems occur along a gradient, the thresholds between some systems were abrupt, accompanied by an equally abrupt shift in the cultivated crop species. The limitations to the gravitational feed of water and the associated shift from Flooded Pondfield to Colluvial Slope agriculture along river valleys is a clear example of this occurrence that is well-documented in the ethnohistorical literature. Similarly, the threshold behavior of soil chemical properties and associated nutrient availability resulted in well-delineated limitations to different rainfed systems. Such shifts in soil properties were demonstrated between Intensive Rainfed and Agroforestry systems, in which soil fertility demands clearly represent a driving constraint between these two systems (Lincoln and Ladefoged, 2014; Lincoln et al., 2014). These abrupt shifts in landscape parameters were sometimes reinforced by sociopolitical boundaries that aligned with such environmental thresholds (Handy et al., 1972; Lincoln and Ladefoged, 2014; Lincoln, 2020a; Lincoln et al., 2022). On the other hand, delineation between intensive and marginal forms of agriculture, both dryland and colluvial, was reliant on rainfall that, while modeled as a threshold relationship, was more likely a gradual shift in practices and crop assemblages across the rainfall gradients. Native Hawaiian peoples who efficiently conformed these now underutilized crops and their respective systems to a vast array of ecological landscapes demonstrated a masterful comprehension of the relationship between crop and environment. This mastery and practice is something that we, as modern day farmers and decision makers, can humbly take away as critical lessons related to preserving the natural ecology of the land by filling its ecological niches with these underutilized crops.
One potential implementation of such concepts would be to apply more textured zoning of agricultural lands, promoting a higher diversity of cropping systems. For instance, some lands could be zoned specifically for agroforestry practices, rather than all agricultural lands being available to all forms of cultivation. Current agricultural zoning does little to restrict or encourage specific applications or forms of agriculture, permitting vulnerable monocultures and inefficient land-uses to encompass all areas, regardless of their ecologies. Using land zoning or other laws to promote ecologically appropriate forms of agricultural production could simultaneously aid in improving resilience and ecosystem services while reducing prohibitive land costs by constraining allowable usage of the land. Such concepts and concerns are raised in discussions of how to improve Hawai‘i‘s contemporary food system and security, in which some 85% of food is imported from outside the state (Leung and Loke, 2008), but have not been seriously presented in terms of legislative action. Even further than considering the utilization of spatial patterns could be the consideration of seasonal land usage, support for multi-species systems, and associated land tenure and access (Blanco et al., 2017; Lincoln et al., 2022).
Data availability statement
The spatial datasets used in the modeling are all freely available with links provided to the location of the spatial layers in their first mention in the text. The model outputs analyzed for this study will be provided by the corresponding author by request.
Author contributions
NL: conceptualization, methodology, formal analysis, resources, data curation, writing—original draft preparation, supervision, project administration, and funding acquisition. NL and TL: software and visualization. TH, TL, and NL: investigation. TH and TL: writing—review and editing. All authors have read and agreed to the published version of the manuscript.
Funding
This work was supported by the National Science Foundation CAREER grant #1941595.
Acknowledgments
We thank Thegn Ladefoged, Natalie Kurashima, and Aurora Kagawa-Viviani and their previous work in modeling Hawaiian agricultural systems.
Conflict of interest
The authors declare that the research was conducted in the absence of any commercial or financial relationships that could be construed as a potential conflict of interest.
Publisher's note
All claims expressed in this article are solely those of the authors and do not necessarily represent those of their affiliated organizations, or those of the publisher, the editors and the reviewers. Any product that may be evaluated in this article, or claim that may be made by its manufacturer, is not guaranteed or endorsed by the publisher.
References
Allen, M. S. (2001). Gardens of Lono: Archaeological Investigations at the Amy BH Greenwell Ethnobotanical Garden, Kealakekua, Hawai‘i. Honolulu, HI: Bishop Museum Press.
Allen, M. S., and McAnany, P. A. (1994). Environmental variability and traditional Hawaiian land use patterns: Manukā's cultural islands in seas of lava. Asian Perspec. 33, 19–55.
Altieri, M. A. (1999). The ecological role of biodiversity in agroecosystems. Agric, Ecosys, Environ. 74, 19–31.
Altieri, M. A. (2002). Agroecology: the science of natural resource management for poor farmers in marginal environments. Agric. Ecosyst. Environ. 93, 1–24. doi: 10.1016/S0167-8809(02)00085-3
Altieri, M. A. (2018). Agroecology: the Science of Sustainable Agriculture. Boulder, CO: Westview Press.
Asner, G. P., Carlson, K. M., and Martin, R. E. (2005). Substrate age and precipitation effects on Hawaiian forest canopies from spaceborne imaging spectroscopy. Remote Sens. Environ. 98, 457–467. doi: 10.1016/j.rse.2005.08.010
Beamer, K., Tau, T. M., and Vitousek, P. M. (2022). Islands and Cultures: How Pacific Islands Provide Paths Toward Sustainability. New Haven, CT: Yale University Press, 243.
Beckwith, M. W. (1932). Kepelino's Traditions of Hawaii. Honolulu, HI: Hawaii The Bernice P Bishop Museum.
Bivand, R., Keitt, T., Rowlingson, B., Pebesma, E., Sumner, M., Hijmans, R., et al. (2015). Package ‘rgdal.’ Bindings for the Geospatial Data Abstraction Library. Available online at: https://cran r-project org/web/packages/rgdal/index html (accessed October 15, 2017).
Blanco, J., Michon, G., and Carrière, S. (2017). Natural ecosystem mimicry in traditional dryland agroecosystems: insights from an empirical and holistic approach. J. Environ. Manage. 204, 111–122. doi: 10.1016/j.jenvman.2017.08.030
Chadwick, O. A., Chorover, J., Chadwick, K. D., Bateman, J. B., Slessarev, E. W., Kramer, M., et al. (2021). “Constraints of climate and age on soil development in Hawai‘i,” in Biogeochemistry of the Critical Zone. Berlin: Springer Nature Press. doi: 10.1007/978-3-030-95921-0_3
Cline, M. G. (1955). Soil Survey of the Territory of Hawaii. United States Department of Agriculture.
Coil, J., and Kirch, P. V. (2005). An Ipomoean landscape: archaeology and the sweet potato in Kahikinui, Maui, Hawaiian Islands. Sweet Potato Pacific Reappr. Oceania Monograph. 56, 71–84.
Earle, T. (1980). Prehistoric irrigation in the Hawaiian Islands: an evaluation of evolutionary significance. Archaeol. Phys. Anthropol. Oceania. 15, 1–28.
Ellis, W. (2009). Journal of William Ellis a Narrative of a Tour Through Hawaii in 1823. Honolulu, HI: Mutual Publising.
Foote, D. E. (1972). Soil Survey of Islands of Kauai, Oahu, Maui, Molokai, and Lanai, State of Hawaii. Washington, DC: Soil Conservation Service, United States Department of Agriculture.
Giambelluca, T. W., Chen, Q., Frazier, A. G., Price, J. P., Chen, Y. L., Chu, P. S., et al. (2013). Online rainfall atlas of Hawai ‘i. Bull. Am. Meteorol. Soc. 94, 313–316. doi: 10.1175/BAMS-D-11-00228.1
Giambelluca, T. W., Shuai, X., Barnes, M. L., Alliss, R. J., Longman, R. J., Miura, T., et al. (2014). Evapotranspiration of Hawai ‘i. Final report submitted to the US Army Corps of Engineers—Honolulu District, and the Commission on Water Resource Management, State of Hawai ‘i.
Gingerich, S., Yeung, C., Ibarra, T. J., and Engott, J. (2007). Water Use in Wetland Kalo Cultivation in Hawai‘i. Report No.: U.S. Geological Survey Open-File Report 2007-1157. Available online at: https://pubs.usgs.gov/of/2007/1157/ (accessed November 27, 2022).
Gon, I., Tom, S. L., and Woodside, U. (2018). ‘Āina Momona, Honua Au Loli—productive lands, changing world: using the hawaiian footprint to inform biocultural restoration and future sustainability in Hawai ‘i. Sustainability 10, 3420. doi: 10.3390/su10103420
Hahn, S. K. (1977). “Sweet potato,” in Ecophysiology of Tropical Crops (New York, NY: Academic Press, Inc.). doi: 10.1016/B978-0-12-055650-2.50013-7
Handy, E. C. (1940). The Hawaiian Planter-Volume I, his plants, methods and areas of cultivation. Bull. Honolulu, HI: Bernice P. Bishop Museum. 161.
Handy, E. S. C., Handy, E. G., and Pukui, M. K. (1972). Native Planters in old Hawaii: Their Life, Lore, and Environment. Vol. 233. Honolulu, HI: The P Bishop Museum Press.
Hijmans, R. J., and van Etten, J. (2011). RASTER Package: Geographic analysis and modeling with raster data. R package version. 2–0. Available online at: https://cran.r-project.org/web/packages/raster/index.html
Hommon, R. J. (2013). The Ancient Hawaiian State: Origins of a Political Society. Oxford: Oxford University Press.
Horrocks, M., and Rechtman, R. B. (2009). Sweet potato (Ipomoea batatas) and banana (Musa sp.) microfossils in deposits from the Kona Field System, Island of Hawaii. J. Archaeol. Sci. 36, 1115–1126. doi: 10.1016/j.jas.2008.12.014
Kagawa-Viviani, A., Levin, P., Johnston, E., Ooka, J., Baker, J., Kantar, M., et al. (2018b). I Ke Ēwe ‘Āina o Ke Kupuna: hawaiian ancestral crops in perspective. Sustainability 10, 4607. doi: 10.3390/su10124607
Kagawa-Viviani, A. K., Lincoln, N. K., Quintus, S., Lucas, M. P., and Giambelluca, T. W. (2018a). Spatial patterns of seasonal crop production suggest coordination within and across dryland agricultural systems of Hawai‘i Island. Ecol. Soc. doi: 10.5751/ES-10369-230320
Kelly, M. (1983). Na Mala o Kona: The Gardens of Kona: A History of Land Use in Kona, Hawai'i. Honolulu, HI: The Bernice P Bishop Museum.
Kirch, P. V. (1977). Valley agricultural systems in prehistoric Hawaii: an archaeological consideration. Asian Perspect. 20, 246–280.
Kirch, P. V. (2007). Hawaii as a model system for human ecodynamics. Am. Anthropol. 109, 8–26. doi: 10.1525/aa.2007.109.1.8
Kirch, P. V., Coil, J., Hartshorn, A. S., Jeraj, M., Vitousek, P. M., and Chadwick, O. A. (2005). Intensive dryland farming on the leeward slopes of Haleakala, Maui, Hawaiian Islands: archaeological, archaeobotanical, and geochemical perspectives. World Archaeol. 37, 240–258. doi: 10.1080/00438240500095074
Kurashima, N., Fortini, L., and Ticktin, T. (2019). The potential of indigenous agricultural food production under climate change in Hawai‘i. Nature Sustain. 2, 191–199. doi: 10.1038/s41893-019-0226-1
Kurashima, N., and Kirch, P. V. (2011). Geospatial modeling of pre-contact Hawaiian production systems on Moloka‘i Island, Hawaiian Islands. J. Archaeol. Sci. 38, 3662–3674. doi: 10.1016/j.jas.2011.08.037
Ladefoged, T. N., and Graves, M. W. (2008). Variable development of dryland agriculture in Hawai‘i: a fine-grained chronology from the Kohala Field System, Hawai‘i Island. Curr. Anthropol. 49, 771–802. doi: 10.1086/591424
Ladefoged, T. N., Kirch, P. V., Gon, I., Chadwick, O. A., Hartshorn, A. S., and Vitousek, P. M. (2009). Opportunities and constraints for intensive agriculture in the Hawaiian archipelago prior to European contact. J. Archaeol. Sci. 36, 2374–2383. doi: 10.1016/j.jas.2009.06.030
Ladefoged, T. N., McCoy, M. D., Asner, G. P., Kirch, P. V., Puleston, C. O., Chadwick, O. A., et al. (2011). Agricultural potential and actualized development in Hawai'i: an airborne LiDAR survey of the leeward Kohala field system (Hawai'i Island). J. Archaeol. Sci. 38, 3605–3619. doi: 10.1016/j.jas.2011.08.031
Ladefoged, T. N., Preston, A., Vitousek, P. M., Chadwick, O. A., Stein, J., Graves, M. W., et al. (2018). Soil nutrients and pre-European contact agriculture in the leeward Kohala field system, Island of Hawai ‘i. Archaeol. Oceania. 53, 28–40. doi: 10.1002/arco.5138
Lee, C. T., Tuljapurkar, S., and Vitousek, P. M. (2006). Risky business: temporal and spatial variation in preindustrial dryland agriculture. Hum. Ecol. 34, 739–763. doi: 10.1007/s10745-006-9037-x
Leung, P., and Loke, M. (2008). Economic impacts of improving Hawaii's food self-sufficiency. Economic Issues, 16, 1–7.
Lincoln, N., Chadwick, O., and Vitousek, P. (2014). Indicators of soil fertility and opportunities for precontact agriculture in Kona, Hawai'i. Ecosphere 5, 1–20. doi: 10.1890/ES13-00328.1
Lincoln, N., Kurashima, N., and Blaich-Vaughan, M. (2022). “Hawai‘i,” in Islands and Cultures: How Pacific Islands Provide Pathways to Sustainability. New Haven, CT: Yale University Press.
Lincoln, N., and Ladefoged, T. (2014). Agroecology of pre-contact Hawaiian dryland farming: the spatial extent, yield and social impact of Hawaiian breadfruit groves in Kona, Hawai'i. J. Archaeol. Sci. 49, 192–202. doi: 10.1016/j.jas.2014.05.008
Lincoln, N., Zhang, Q., and Chen, Q. (2021). State of the state tree: historical and modern ecology of Kukui (Candlenut, Aleurites Moluccanus) in Hawai ‘i. Pac. Sci. 74, 419–434. doi: 10.2984/74.4.9
Lincoln, N. K. (2010). Amy Greenwell Garden Ethnobotanical Guide to Native Hawaiian Plants and Polynesian-Introduced Plants. Honolulu, HI: The Bishop P Museum Press, 135.
Lincoln, N. K. (2020a). Agroforestry form and ecological adaptation in ancient Hawai'i: extent of the pākukui swidden system of Hāmākua, Hawai'i Island. Agric. Syst. 181, 102808. doi: 10.1016/j.agsy.2020.102808
Lincoln, N. K. (2020b). Ko: An Ethnobotanical Guide to Hawaiian Sugarcane Cultivars. Honolulu, HI: University of Hawaii Press.
Lincoln, N. K., Kagawa-Viviani, A., Marshall, K., and Vitousek, P. M. (2017). Observations of Sugarcane and Knowledge Specificity in Traditional Hawaiian Cropping Systems. Hauppauge, NY: Nova Science Publishers. doi: 10.1093/acrefore/9780199389414.013.376
Lincoln, N. K., Rossen, J., Vitousek, P., Kahoonei, J., Shapiro, D., Kalawe, K., et al. (2018). Restoration of ‘āina malo ‘o on Hawai ‘i Island: expanding biocultural relationships. Sustainability. 10, 3985. doi: 10.3390/su10113985
Lincoln, N. K., and Vitousek, P. (2017). Indigenous Polynesian agriculture in Hawai‘i. In: Oxford Research Encyclopedia of Environmental Science.
Mausio, K., Miura, T., and Lincoln, N. K. (2020). Cultivation potential projections of breadfruit (Artocarpus altilis) under climate change scenarios using an empirically validated suitability model calibrated in Hawai'i. PLoS ONE. 15, e0228552. doi: 10.1371/journal.pone.0228552
Mayes, S., Massawe, F. J., Alderson, P. G., Roberts, J. A., Azam-Ali, S. N., and Hermann, M. (2012). The potential for underutilized crops to improve security of food production. J. Exp. Bot. 63, 1075–1079. doi: 10.1093/jxb/err396
McCoy, M. D. (2005). The development of the kalaupapa field system Moloka'I Island Hawai'i. J. Polynesian Soc. 114, 339–358.
McCoy, M. D., and Hartshorn, A. S. (2007). Wind erosion and intensive prehistoric agriculture: a case study from the Kalaupapa Field System, Moloka'i Island, Hawai'i. Geoarchaeology 22, 511–532. doi: 10.1002/gea.20170
McElroy, W. (2007). The Development of Irrigated Agriculture in Wailau Valley, Moloka'i Island, Hawai'i. Unpublished PhD Dissertation, University of Hawai'i, Manoa.
Meilleur, B. A., Jones, R. R., Titchenal, C. A., and Huang, A. S. (2004). Hawaiian Breadfruit: Ethnobotany, Nutrition, and Human Ecology. Honolulu, HI: College of Tropical Agriculture and Human Resources, Univeristy of Hawai'i.
Morrison, A. E., Quintus, S., Rieth, T. M., Filimoehala, C., Duarte, T., Tulchin, J., et al. (2021). Colluvial slope agriculture in context: an extensive agricultural landscape along the slopes of Punalu ‘u Valley, O ‘ahu Island, Hawai ‘i. J. Island Coastal Archaeol. doi: 10.1080/15564894.2021.1998936
Newman, T. S. (1971). Hawaii Island agricultural zones, circa AD 1823: an ethnohistorical study. Ethnohistory 335–51. doi: 10.2307/481073
Newman, T. S. (1972). Two early Hawaiian field systems on Hawaii Island. J. Polynesian Soc. 81, 87–89.
Ngeve, J. M., Hahn, S. K., and Bouwkamp, J. C. (1992). Effects of altitude and environments on sweet potato yield in Cameroon. Tropical Agric. 69.
Onwueme, I. C. (1999). Taro Cultivation in Asia and the Pacific. Food and Agriculture Organization of the United Nations, Regional Office for Asia and the Pacific, Bangkok.
Padulosi, S., Heywood, V., Hunter, D., and Jarvis, A. (2011). Underutilized species and climate change: current status and outlook. Crop Adapt. Climate Change. 26, 507–521. doi: 10.1002/9780470960929.ch35
Palmer, M. A., Graves, M., Ladefoged, T. N., Chadwick, O. A., Duarte, K. T., Porder, S., and Vitousek, P. M. (2009). Sources of nutrients to windward agricultural systems in pre-contact Hawai'i. Ecological Applications, 19, 1444–1453.
Perroy, R. L., Melrose, J., and Cares, S. (2016). The evolving agricultural landscape of post-plantation Hawai ‘i. Appl. Geograp. 76, 154–162. doi: 10.1016/j.apgeog.2016.09.018
Porder, S., and Chadwick, O. A. (2009). Climate and soil-age constraints on nutrient uplift and retention by plants. Ecology 90, 623–636. doi: 10.1890/07-1739.1
Pukui, M. K. (1983). Olelo No‘eau: Hawaiian Proverbs and Poetical Sayings. Honolulu: Bishop Museum Press.
Quintus, S., Huebert, J., Kirch, P. V., Lincoln, N. K., and Maxwell, J. (2019). Qualities and contributions of agroforestry practices and novel forests in pre-European polynesia and the polynesian outliers. Hum. Ecol. 47, 811–825. doi: 10.1007/s10745-019-00110-x
Quintus, S., and Lincoln, N. K. (2020). Integrating local and regional in pre-contact Hawaiian agriculture at Kahuku, Hawai ‘i Island. Environ. Archaeol. 25, 53–68. doi: 10.1080/14614103.2018.1497833
R Team. (2015). RStudio: Integrated Development for R. RStudio, Inc, Boston, MA. Available online at: http://www.rstudio.com (accessed February 19, 2023).
Schilt, R. (1984). Subsistence and Conflict in Kona, Hawaii: An Archaeological Study of the Kuakini Highway Realignment Corridor, ed Bernice P. Honolulu, HI: Bishop Museum.
Stannard, D. E. (1989). Before the Horror: The Population of Hawai'i on the Eve of Western Contact. Honolulu, HI: University of Hawaii Press.
Valenzuela, H., Fukuda, S., and Arakaki, A. (1994). Sweetpotato production guides for Hawai'i. (Honolulu, HI: College of Tropical Agriculture and Human Resources). Available online at: https://www.ctahr.hawaii.edu/oc/freepubs/pdf/RES-146.pdf
Vitousek, P. M. (1995). The Hawaiian Islands as a model system for ecosystem Studies. Pacific Science, 49, 2–16.
Vitousek, P. M. (2002). Oceanic islands as model systems for ecological studies. J. Biogeogr. 29, 573–582. doi: 10.1046/j.1365-2699.2002.00707.x
Vitousek, P. M., Bateman, J. B., and Chadwick, O. A. (2021). A “toy” model of biogeochemical dynamics on climate gradients. Biogeochemistry 154, 183–210. doi: 10.1007/s10533-020-00734-y
Vitousek, P. M., Chadwick, O. A., Hilley, G., Kirch, P. V., and Ladefoged, T. N. (2010). Erosion, geological history, and indigenous agriculture: a tale of two valleys. Ecosystems 13, 782–793. doi: 10.1007/s10021-010-9354-1
Vitousek, P. M., Chadwick, O. A., Hotchkiss, S. C., Ladefoged, T. N., and Stevenson, C. M. (2014). Farming the rock: a biogeochemical perspective on intensive agriculture in Polynesia. J. Pacific Archaeol. 5, 51–61.
Vitousek, P. M., and Farrington, H. (1997). Nutrient limitation and soil development: experimental test of a biogeochemical theory. Biogeochemistry 37, 63–75. doi: 10.1023/A:1005757218475
Vitousek, P. M., Ladefoged, T. N., Kirch, P. V., Hartshorn, A. S., Graves, M. W., Hotchkiss, S. C., et al. (2004). Soils, agriculture, and society in precontact Hawaii. Science 304, 1665–1669. doi: 10.1126/science.1099619
Vitousek, P. M., Treseder, K. K., Howarth, R. W., and Menge, D. N. (2022). A “toy model” analysis of causes of nitrogen limitation in terrestrial ecosystems. Biogeochemistry. 160, 381–394. doi: 10.1007/s10533-022-00959-z
Wilson, L. A. (1977). “Root crops,” in Ecophysiololgy of Tropical Crops (New York, NY: Academic Press). doi: 10.1016/B978-0-12-055650-2.50012-5
Winter, K., Lincoln, N., Berkes, F., Alegado, R., Kurashima, N., Frank, K., et al. (2020). Ecomimicry in Indigenous resource management: optimizing ecosystem services to achieve resource abundance, with examples from Hawai‘i. Ecol. Soc. doi: 10.5751/ES-11539-250226
Keywords: adaptation, agroecology, agroforestry, biocultural, cropping systems, indigenous agriculture, Hawai‘i, sustainable land use
Citation: Lincoln NK, Haensel TP and Lee TM (2023) Modeling Hawaiian Agroecology: Depicting traditional adaptation to the world's most diverse environment. Front. Sustain. Food Syst. 7:1116929. doi: 10.3389/fsufs.2023.1116929
Received: 06 December 2022; Accepted: 13 February 2023;
Published: 16 March 2023.
Edited by:
Tafadzwanashe Mabhaudhi, University of KwaZulu-Natal, South AfricaReviewed by:
Mallika Sardeshpande, University of KwaZulu-Natal, South AfricaSandile Thamsanqa Hadebe, University of Limpopo, South Africa
Copyright © 2023 Lincoln, Haensel and Lee. This is an open-access article distributed under the terms of the Creative Commons Attribution License (CC BY). The use, distribution or reproduction in other forums is permitted, provided the original author(s) and the copyright owner(s) are credited and that the original publication in this journal is cited, in accordance with accepted academic practice. No use, distribution or reproduction is permitted which does not comply with these terms.
*Correspondence: Noa Kekuewa Lincoln, nlincoln@hawaii.edu