- 1CSIR-Crops Research Institute of Ghana, Kumasi, Ghana
- 2Department of Biological Production Science, Tokyo University of Agriculture and Technology, Tokyo, Japan
The global temperature increase of 1.1°C over the past decade and human population growth are already escalating competition for water in locations where water is scarce, adversely affecting agriculture. Water-smart farming was developed to address challenges associated with the availability, access and use of agricultural water. It complements practices and goals related to agricultural water in climate-smart agriculture. It encompasses several climate-responsive food cropping systems, water-saving methods, tools, and technologies to grow food sustainably. Effective implementation of water-smart farming can ameliorate the pressing burden of agricultural water competition. Therefore, the conceptual framework of water-smart farming and its principal elements or ideology are introduced in this review. The major pillars of water-smart cropping, which include conditioning and improving the soil’s capacity to capture and hold moisture, basic infrastructure for water harvesting, storage and use as nature-based cropping solutions were elaborated. Further examples of technologies and best practices in water-smart crop farming, such as breeding for drought-tolerant crop varieties, promoting dryland cropping systems, alternate wetting and drying technology, water drainage and control structures, rainwater harvesting, utilization of irrigation reservoirs, cover cropping and intercropping for enhancing soil moisture conservation were presented. The review also briefly shows the contribution of some of these best practices and adaptive technologies of water-smart cropping toward promoting water-saving methods used for growing rice, maize, legumes, cassava and peri-urban vegetables.
1. Introduction
Agricultural production is critically dependent on water resources, which face escalating threats due to increasing water scarcity. The looming specter of climate change further compounds these challenges by disrupting the delicate balance of the water cycle (Oral et al., 2021). The agricultural sector, in particular, finds itself at a precipice, necessitating a comprehensive management approach to mitigate the impending impacts (Abbass et al., 2022).
In this era of rapid environmental transformation and mounting uncertainties regarding water supplies, it is imperative to guide farmers in transitioning from archaic, high-water-demand agricultural and irrigation techniques to modern, low-water-demand systems and technologies (Moradet, 2005; Feizizadeh et al., 2022). Nature-based Solutions (NbS) have emerged as a pivotal strategy in combatting environmental and agricultural challenges within the context of climate change. NbS encompasses a spectrum of ecosystem-based approaches aimed at addressing both environmental and societal dilemmas (Cohen-Shacham et al., 2019; Sarabi et al., 2019). Prior research on NbS has explored their applications across diverse contexts, including biodiversity conservation, disaster risk reduction, and climate adaptation. These strategies have demonstrated the potential for enhanced ecosystem services, increased community resilience, and cost-effectiveness (Shah et al., 2020; Bayulken et al., 2021; Liu et al., 2021). Nevertheless, challenges related to implementation, scalability, and the imperative for localized adaptation persist (Seddon et al., 2020; Seddon, 2022). Hence, there arises a pressing need to contribute to the existing body of knowledge by directing our focus squarely on water-smart crop farming as an NbS tailored to address climate change’s impact on agriculture.
Effective water resource management stands as a linchpin in ensuring equitable allocation and optimal utilization. At its core, this entails accounting for every drop of water to produce food with minimal losses or, more succinctly, the acclaimed measure of water use efficiency: “the amount of carbon assimilated as biomass or grain produced per unit of water used by the crop” (Hoover et al., 2023). Water-smart cropping solutions encompass a gamut of practices, including dryland rice cultivation, system rice intensification, alternate wetting and drying, promotion of farmer-led reduced irrigation, cover cropping, intercropping of legumes with food crops, and the development of drought-tolerant maize varieties, among others. Widespread adoption of these techniques, coupled with adherence to sound agronomic practices, is essential. Smallholder farmers, who remain dominant in the global agricultural landscape, must be empowered to spearhead climate change adaptation efforts (Mushi et al., 2023; Ndhlovu and Mhlanga, 2023). This empowerment necessitates robust agronomic management encompassing fertilization, irrigation, cropping patterns, and plant protection, in tandem with addressing external factors such as input supply, post-harvest handling facilities, and policy regulations (Islam et al., 2022).
Despite global socioeconomic advancements, the primary impetus behind increased crop output has been the expansion of mainly rainfed cultivated areas (FAOSTAT, 2022; Saito et al., 2023). Consequently, a vast reservoir of untapped potential in water resources beckons, offering ample room for the expansion of irrigation. However, the lion’s share of investment in irrigation schemes hails from the public sector, with limited participation from potential public-private partnerships (Dumani et al., 2023). Numerous studies have underscored the importance of tailored public investments that bolster agricultural productivity and resilience capacity (Otieno et al., 2022; Ankrah et al., 2023; Chen and Hu, 2023). Traditionally, government entities bore the mantle of planning, developing, and constructing irrigation projects. Yet, the landscape is evolving, with the private sector emerging as a significant player and the mantle of irrigation development and management transitioning toward greater user involvement. By empowering farmers to actively engage in participatory irrigation development, collaborative decision-making ensues, facilitating a more effective and inclusive approach (Nalumu et al., 2021).
On a global scale, irrigation accounts for a staggering 70% of freshwater withdrawals and a staggering 90% of freshwater use, exerting profound impacts on terrestrial water and hydrological cycles (Guillaumot et al., 2022; Scanlon et al., 2023). The practical and equitable utilization of groundwater remains a persistent concern in many nations, diminishing the benefits accrued by both individuals and society (Ross et al., 2023; Valipour et al., 2023). Estimates indicate that surface water resources currently support 62% of irrigated land, while groundwater supports the remaining 38% (Ashraf et al., 2021). However, the availability of water within each system at any given moment hinges on various factors, including the presence of lakes, wetlands, artificial reservoir storage capacity, soil types, and rates of soil evaporation. Human activities, such as reservoir construction and wetland drainage, wield significant influence over these determinants. According to the International Water Management Institute (IWMI), small-scale irrigation expansion could potentially add 30 million hectares of irrigated land in sub-Saharan Africa, translating to annual net earnings of US$22 billion. Such an expansion holds the promise of bolstering food security and income for 185 million people, making it a pivotal strategy to address hunger, poverty, and malnutrition in West Africa (Rafael, 2023). To realize this potential, targeted investments and policies aimed at enhancing small-scale irrigation systems must be prioritized (Mango et al., 2018).
This review focuses on elucidating the concept of water-smart crop farming as a nature-based solution to confront the multifaceted challenges posed by climate change. It encompasses a comprehensive examination of the effectiveness and potential of water-smart crop farming practices in mitigating climate change’s impact. This review also delves into defining the framework and essential elements of water-smart crop farming while assessing its contributions to climate change adaptation. Furthermore, the review identifies exemplary nature-based solutions, particularly relevant for smallholder farmers in sub-Saharan Africa. Methodologically, this research incorporates a quality assessment of collected articles and employs a search strategy guided by key concepts related to crop adaptation and sustainable water management.
2. Materials and methods
This review delves into the multifaceted aspects of water-smart crop farming and its potential as a climate change adaptation strategy. The approach adopted in this study draws inspiration from Khan et al. (2003) and is visually depicted in Figure 1. The investigation systematically scoured scientific literature across prominent databases such as Google Scholar, Web of Science, and other relevant sources to identify published research concerning water-smart crop farming as a nature-based solution for climate change adaptation. The search queries were meticulously constructed, predominantly focusing on thematic areas and keywords such as “water-smart farming,” “nature-based solutions,” “climate change,” and “smallholder farming.” The research inquiries that guided this study encompassed:
i. Why do existing frameworks and elements of water-smart climate-smart technologies fall short in delivering benefits to farmers?
ii. What measures are essential to unlock the potential of water-smart agricultural interventions? Specifically,
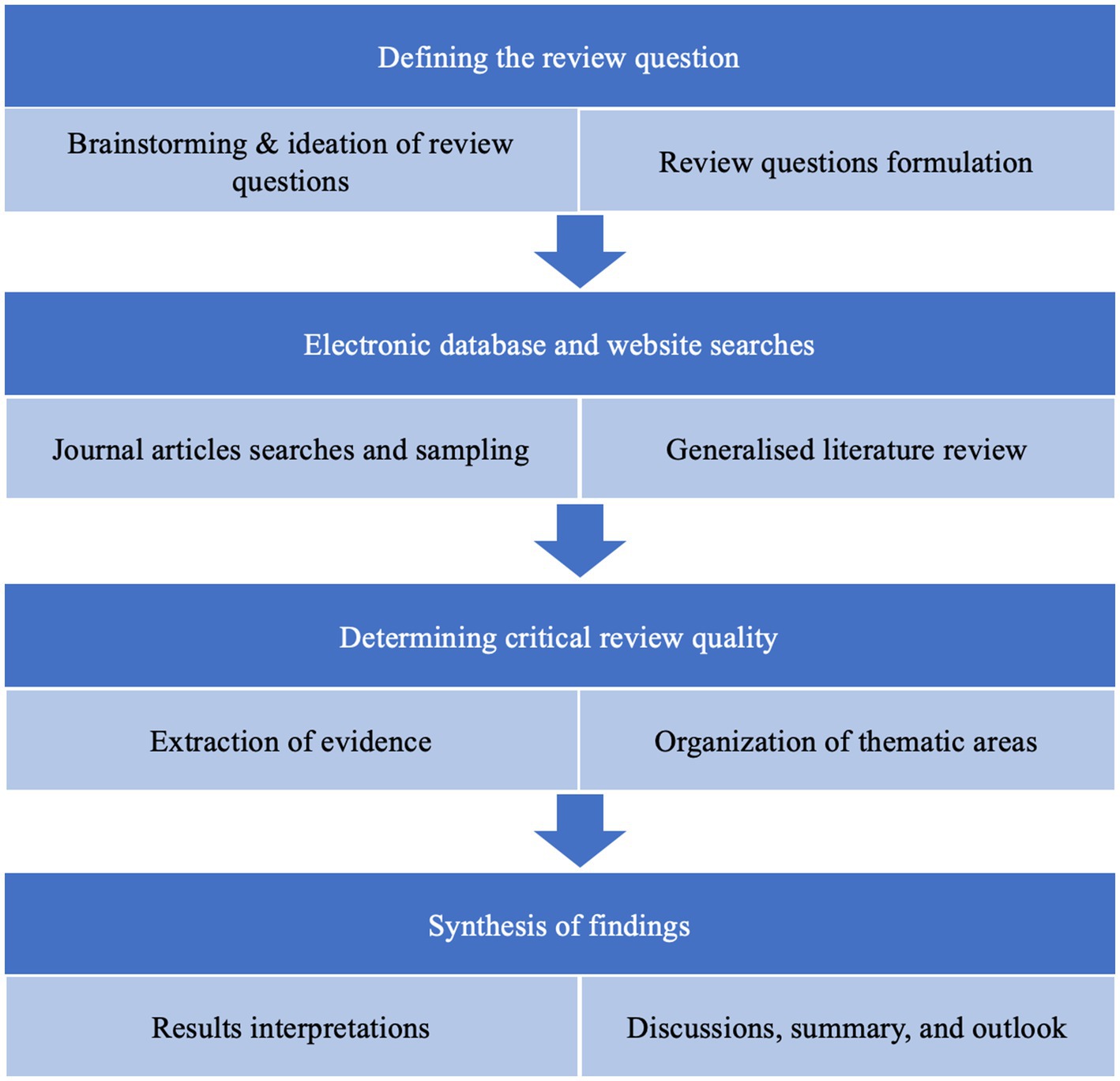
Figure 1. The procedure applied for the peer-reviewed literature searches adapted from Khan et al. (2003).
• To what extent can water-smart crop farming contribute to climate change adaptation?
• What existing water-smart crop farming practices are practical nature-based solutions for smallholder farmers in sub-Saharan Africa?
The initial phase of article identification yielded 520 potential articles for consideration. The process commenced with the removal of duplicate articles, resulting in a refined pool of 380 unique articles eligible for subsequent screening. During the screening phase, articles underwent two sequential filtration steps based on title and abstract. Initially, 213 articles were excluded based on title screening, reducing the count to 115 articles eligible for abstract screening. Following a meticulous review of abstracts, an additional 63 articles were omitted. Exclusion criteria during both title and abstract screening focused on articles that did not reference water-smart farming as a data source. In the final inclusion phase, 52 articles met the eligibility criteria and were selected for further retrieval and in-depth analysis of pertinent information. Table 1 presents a comprehensive summary of key findings derived from the review questions, thematic areas, and keywords employed in the search.
To ensure the reliability and relevance of the gathered literature, a stringent quality assessment was conducted on the searched and retrieved articles, adhering to the criteria established by the Center for Internet Security (CIS). These criteria, inspired by Dixon-Woods et al. (2006), encompassed various factors, including research methodology, data analysis, and the overall credibility of the studies. The study meticulously employed specific key concepts in improving water management and agricultural development to guide the search for relevant articles, revolving around two primary themes: mitigation strategies for crop adaptation to water stress and sustainable water management in the context of climate change. It’s worth noting that references such as Chartzoulakis and Bertaki (2015) and IWMI (2017) played a pivotal role in refining search terms and concepts.
Furthermore, this study incorporated best-practice examples of nature-based solutions gleaned from the author’s extensive research experience at the CSIR-Crops Research Institute. These examples serve as practical illustrations of how water-smart crop farming and climate change adaptation can be effectively implemented in real-world scenarios. Figure 1 outlines the procedure for conducting peer-reviewed literature searches, adapted from Khan et al. (2003). Although the detailed steps of this procedure are not expounded upon in the text, they encompass defining search terms, selecting databases for the search, applying inclusion and exclusion criteria, and retrieving and meticulously reviewing the identified articles.
3. Results and discussion
3.1. Water-smart crop farming, definition, framework and elements
Water-smart crop farming is a vital strategy for smallholder farmers in sub-Saharan Africa and globally. It’s an approach that focuses on optimizing water resource utilization while simultaneously enhancing crop productivity and resilience (Haileslassie et al., 2022; Takeshima and Mano, 2023). Within this framework, several key elements play a crucial role in ensuring the sustainability of agricultural practices among smallholders. Smallholder farmers face unique challenges, often operating in regions with limited water resources and unpredictable rainfall patterns. Climate change exacerbates these issues, making efficient water management essential (Pahl-Wostl, 2007). Water-smart crop farming becomes a lifeline for these farmers by addressing these challenges. One of the primary elements of this approach is the efficient use of water. Smallholders are encouraged to adopt advanced irrigation techniques like drip or sprinkler systems to minimize water wastage (Ali et al., 2020).
Additionally, selecting drought-resistant crop varieties and adjusting planting times based on climate conditions can improve water efficiency while safeguarding crop yields (Khatun et al., 2021). Sustainable water sources are also critical. Rainwater harvesting, utilizing surface water, and managing groundwater resources responsibly are key components. These practices ensure a reliable water supply for farming, reducing the vulnerability of smallholders to water scarcity. Adaptive management is another crucial aspect, allowing smallholders to adjust their farming practices based on changing water availability and climate conditions (Azadi et al., 2021). This flexibility is essential for long-term success. Furthermore, smallholder farmers are encouraged to engage with their local communities and stakeholders. Collaborative efforts can lead to better management of shared water resources, enhancing the sustainability of agricultural practices on a broader scale.
It is estimated that 29% more irrigated areas will be required by 2025 to sustain food production and reduce poverty in Africa and South-East Asia, while freshwater supplies will be diverted from agriculture to meet the increasing demand for domestic use and industry (Chartzoulakis and Bertaki, 2015; IWMI, 2017). To ensure sustainable water management, we propose water harvesting techniques for most smallholder farmers who rely on rainfed agriculture (upland and lowland rice, maize, legumes, cassava, yam, cocoyam, leafy vegetables, eggplants, okra, plantain and bananas etc.). Using climate-smart agriculture principles and promoting related approaches specified per locality, cropping pattern and markets is central to improving agricultural development and sustainable water management (Figure 2). Attention must be given to efficient soil conservation practices, including linkages with upstream land use (Figure 3) and downstream irrigation farming (Figures 2, 4A,B).
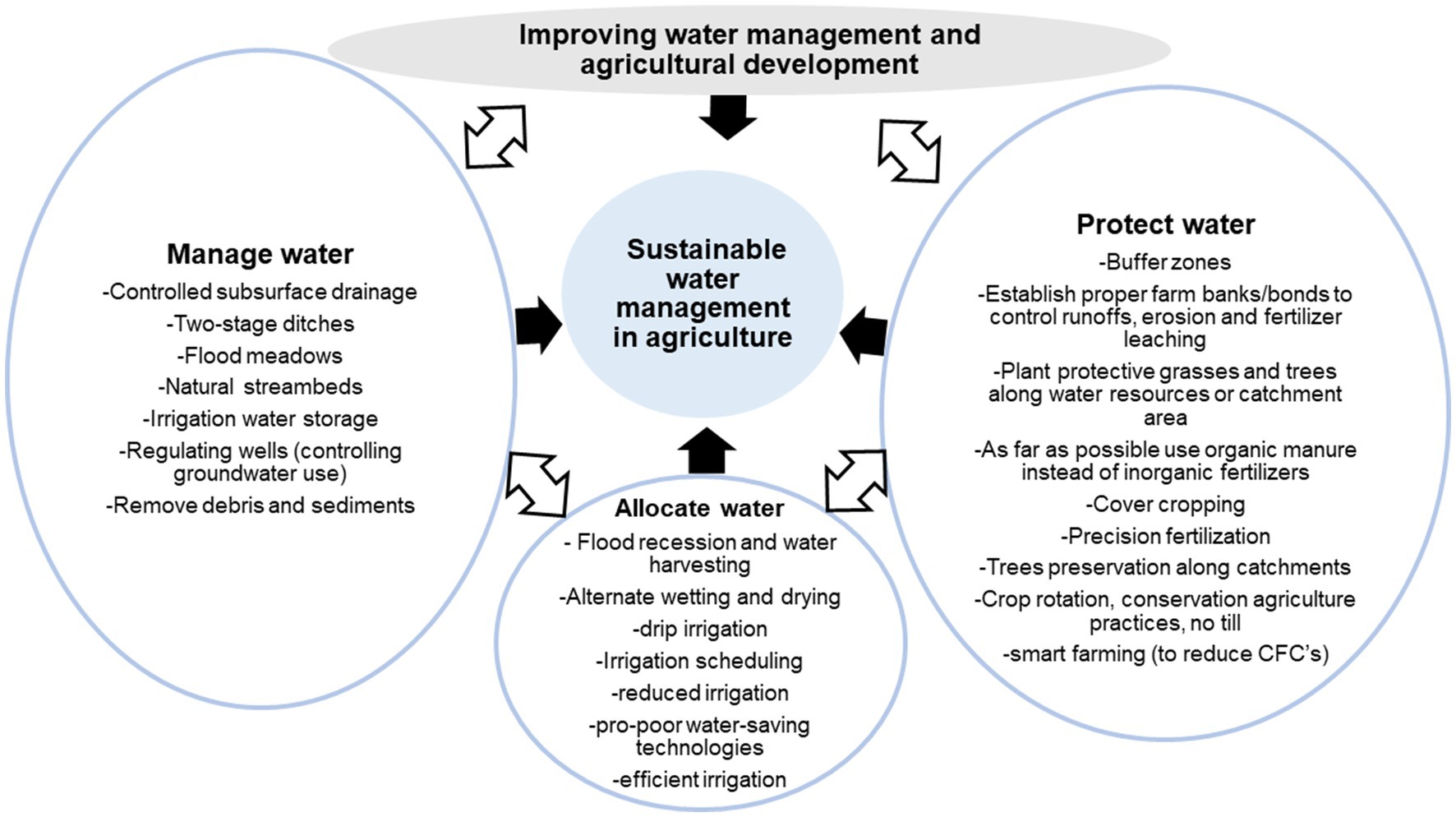
Figure 2. Suggested framework on how to improve water management and agricultural development. Sustainable water management in agriculture may be realized when water is managed, protected and allocated efficiently and effectively. Each circle enlists the various water management features below water protection, management and allocation. CFCs, Chlorofluorocarbons.
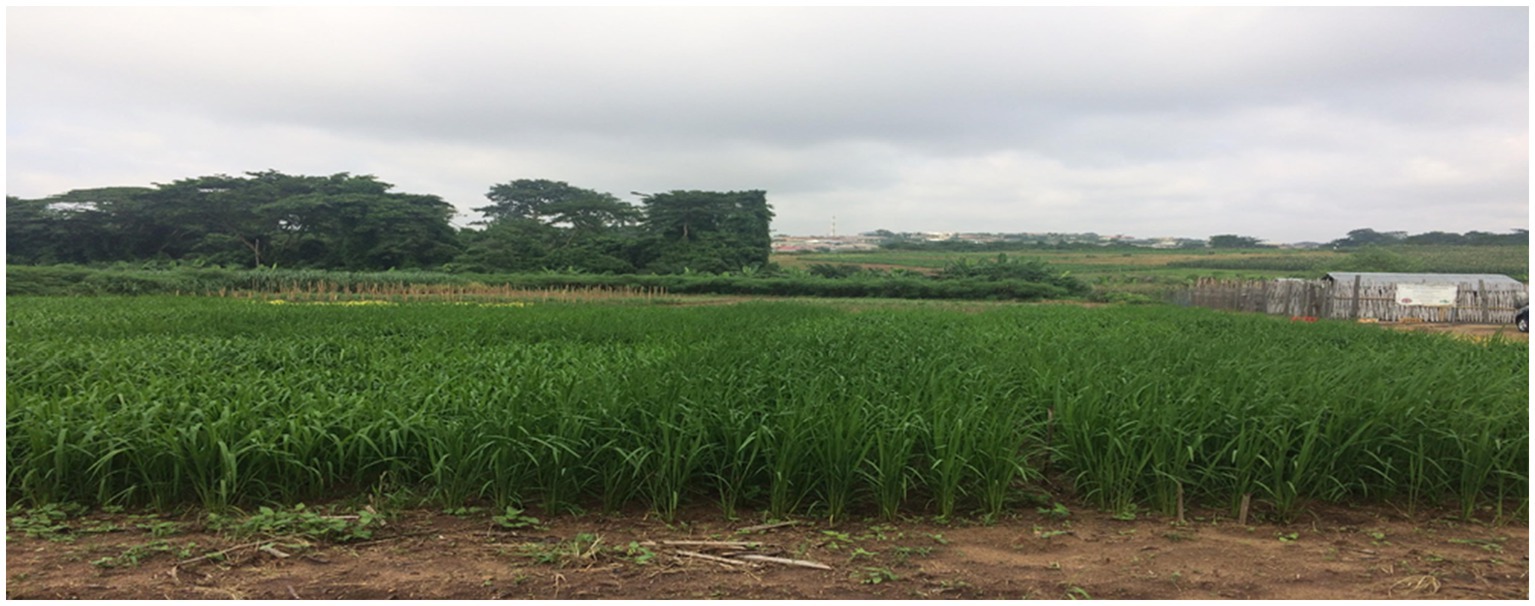
Figure 3. An open field at CSIR-Crops Research Institute in Kumasi, Ghana, showcasing a diverse array of upland or dryland rice varieties on the brink of flowering. Production of upland rice uses 60% less water than what would be required for irrigated lowland rice to flower. However, upland ecology grain yield is usually lower than irrigated or rainfed lowland rice. Picture credit: CSIR-Crops Research Institute, 2017. NB: Lowland rice is cultivated under continuous-flooded transplanted management, whereas upland rice can be grown in rainfed conditions or under sprinkler irrigation (Amante et al., 2012).
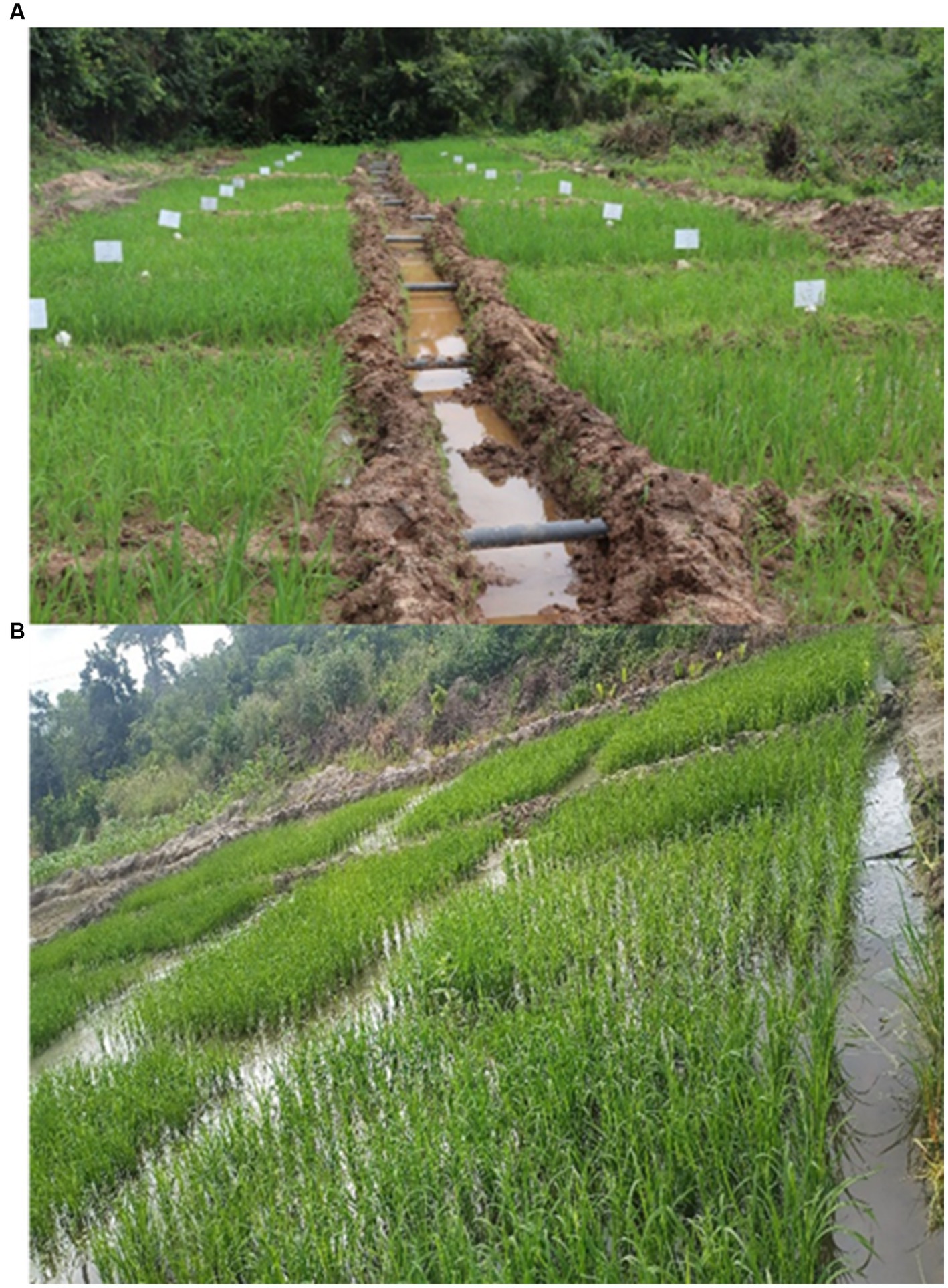
Figure 4. Rice fields showing alternate wetting and drying (A) versus continuous flooding irrigation (B) in the field at CSIR-Crops Research Institute, Kumasi, Ghana (Source: Maxwell Darko Asante, 2021).
Globally, irrigation efficiency is very low since the crops use less than 65% of the applied water. The sustainable use of irrigation water is a priority for agriculture in arid and semi-arid areas (Chartzoulakis and Bertaki, 2015). Therefore, in the face of prevalent water scarcity and climate change, significant efforts should be dedicated to introducing policies geared toward increasing water use efficiency centered on clear-cut goals and targets to achieve more with less water through better management (Chartzoulakis and Bertaki, 2015).
Strategic planning of water resources management and allocation may involve adopting best practices tailored toward (a) setting the sustainable limits of water consumption and (b) making the best use of every drop of water, including non-conventional water sources (FAO, 2022). Four interventions have been proposed and are being piloted in the arid and semi-arid regions; (i) water accounting and auditing system, (ii) framework on water efficiency and water productivity, (iii) analysis of the framework for water-food-energy-climate-ecosystems nexus, (iv) communication and awareness creation (FAO, 2022). These interventions provide an assessment of biophysical and economic water productivity. They also enhance best practices and introduce cheaper technologies based on farmer field schools and decentralized water governance. The interventions must consider rural agro-based industrial value chains, gender dimensions, and incentive frameworks adapted to each local condition. The interventions would ensure higher efficiency/productivity for the 2030 SDGs, and its timeline is attained within safe operational boundaries of water use that define the engagements for water sustainability.
3.2. Facilitating water productivity in small-scale farmers’ fields
To drive on-farm productivity, some simple and easy small-scale irrigation techniques that are budget-friendly may involve hand watering with a hose, buckets and watering cans, and rainwater harvesting (Oteng-Darko et al., 2018). Others may include rainwater storage in drums or earth dugouts (Figure 5), locally made low-cost (PVC pipes-based) sprinklers, misters, and drips connected to the water source (Figures 4A, 3B). An example of a small-scale irrigation approach is indicated in Figure 5. Farmer-led irrigation is cheap, easy to operate and maintain, and easy to move from one plot to the other (IWMI, 2017; World Bank, 2018). The rationale of farmer-led irrigation is that when farmers lead, they own and can drive adoption to neighboring farmers. Barriers to land tenure and water ownership are reduced as farmer-led irrigation schemes are viewed as more secure.
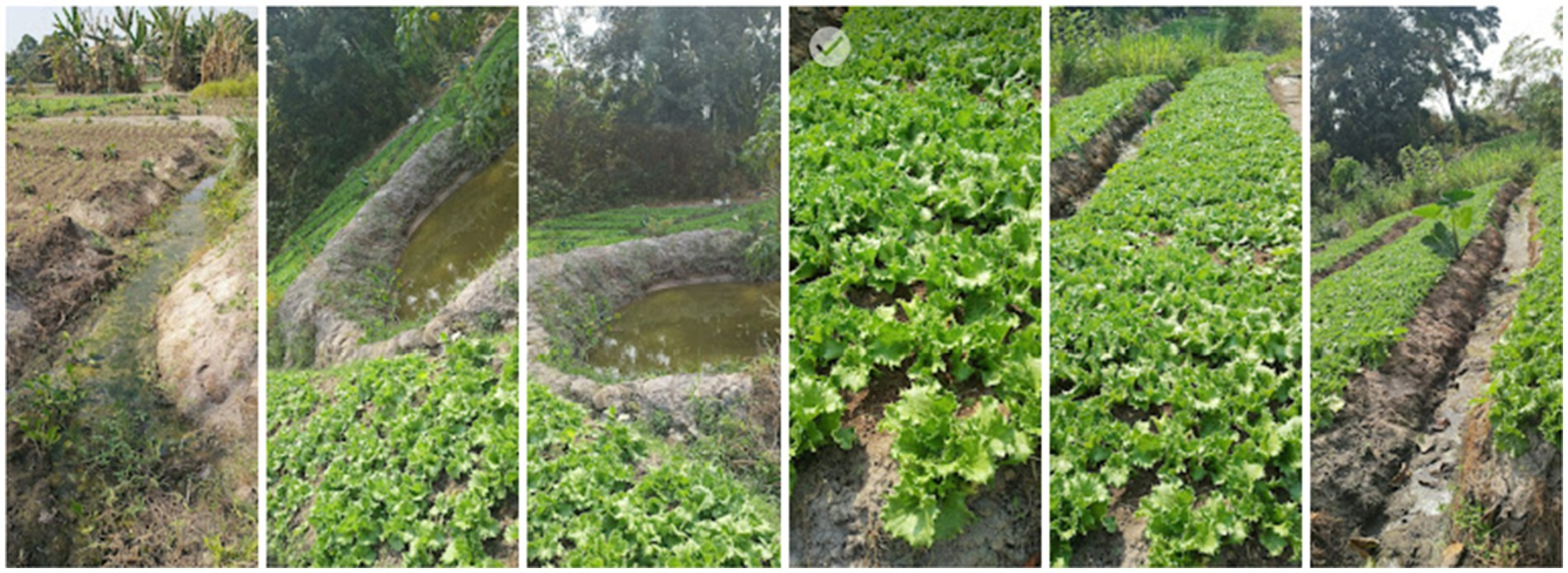
Figure 5. Farmer-led rainwater harvesting dugouts and waterways between beds that are commonly used to irrigate Ghana’s peri-urban smallholder vegetable farms (some common vegetables usually grown include lettuce, cabbage, bell pepper, onion etc.). Picture credit: Felix Frimpong, 2022.
In terms of unconventional water use for irrigation (wastewater use, reuse, and recycling) in small farms, regulatory measures are needed that consciously promote safe water use for different crops. Strong campaigns, public sensitization workshops, research, and knowledge development initiatives may be applied to advance the treatment and use of wastewater for irrigation. For example, freely available water effluents in an aquaponics-based food production system increased maize yields to about 50% over the farmer’s practice and reduced total production costs, thereby improving farmer income and food security (Frimpong et al., 2017). Public and private investments into small-scale irrigation that provide credit access for smallholder farmers could potentially derive significant food and livelihood improvement (Mango et al., 2018).
3.3. Combating climate change using water-smart crop farming–insights, challenges and opportunities
Climate change would rapidly increase water withdrawals, water quality degradation, and competition for water at all levels (FAO, 2013). However, climate-smart water solutions would help mitigate the impact of climate change (dry spells, delayed rainfall, short rain cycles etc.). Most pro-poor irrigation solutions for small-scale farmers may be characterized as climate-smart cropping practices. Some examples of climate-smart water solutions may include alternate wetting and drying (Figures 4A, 3B), cover cropping, intercropping with leguminous crops for soil moisture conservation and water use efficiency for root and tuber crops (Figures 6, 7). For instance, a proper cassava-legume intercropping system allows the arrangement with improved cassava varieties that have erect or semi-erect canopy shape (Figure 7), high yielding 40–60 t/ha, high dry matter content of +30%, resistant to cassava mosaic disease, good for fufu, flour, starch, industrial alcohol and can thrive in several ecologies of coastal, forest, savannah and transition zones (CSIR and MOFA, 2012; Figure 6).
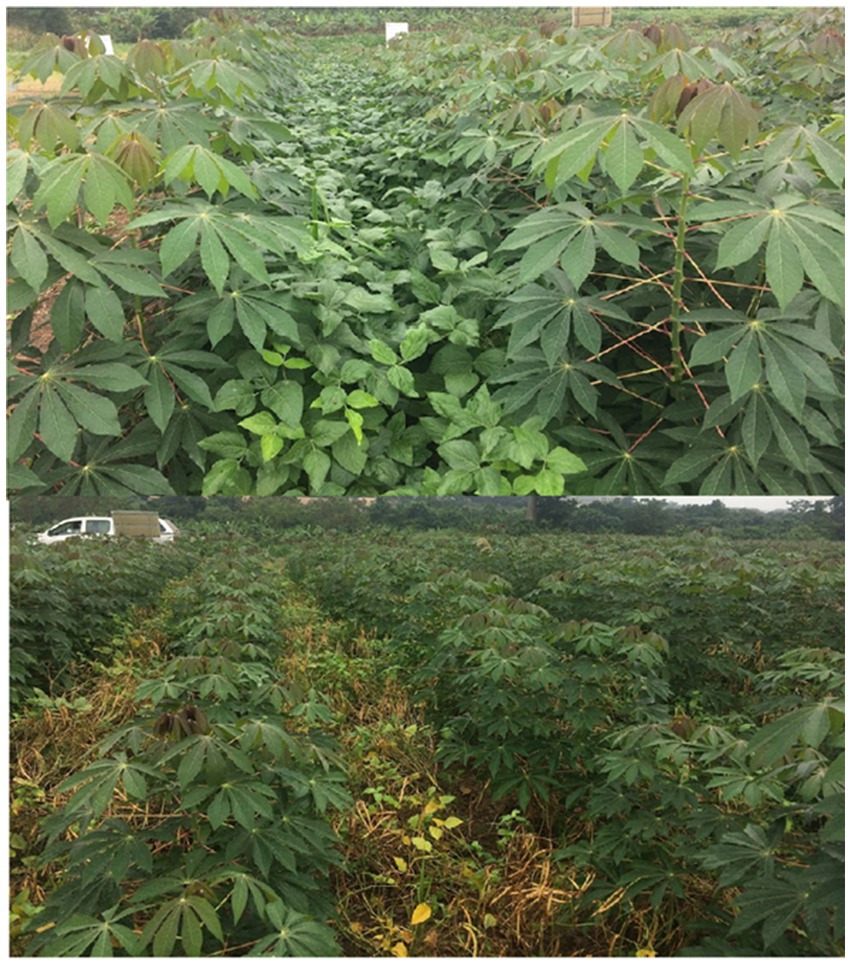
Figure 6. Field demonstration of improved dual-purpose cassava-cowpea integration system at CSIR-Crops Research Institute on-station training site, Fumesua, Ghana. The improved cassava was CRI – Duade Kpakpa spaced at 0.8 m within rows and 1.5 m between rows. Two rows of the cowpea variety, CRI-Anigye (semi-erect type), are planted at 0.5 m × 0.5 m in the middle of two cassava rows. Photo credit: Frimpong Felix, 2016.
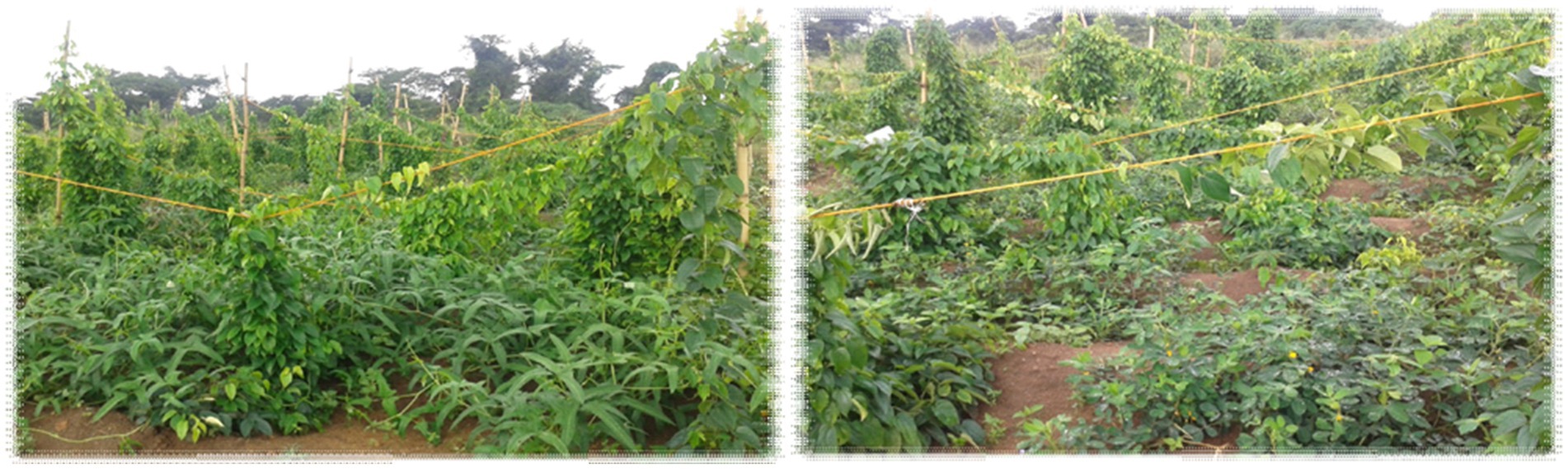
Figure 7. Soil moisture conservation practices to enhance water use efficiency in yam-dual purpose cowpea (A) and yam-groundnut (B) as cover crops in an intercrop. The main crop, the yam, has vines held by the trellis stake (bamboo and ropes) as they climb up to intercept incoming irradiance. The leguminous or secondary crops are sown in available rows/column spaces when the yam is already at the vegetative growth stage (10–12 weeks after planting) to cover up and reduce evapotranspiration to conserve soil moisture.
Another example of water-smart crop farming proven to be able to conserve soil moisture and improve water use efficiency was earlier reported in a yam-pigeon pea intercropping system (Owusu Danquah et al., 2022a). In the example of the yam-pigeon pea intercropping system, the pigeon pea is planted as annual leguminous perennials, while the yam is planted in between alley spaces left where the yam ridges are made. The moderate canopy of the pigeon pea shades the land surface as the yam crops climb onto them as support and intercept sunlight (Owusu Danquah et al., 2022b).
Other innovative water technologies for cereals, horticultural crops and vegetables may include floating agriculture, vertical farming, micro-irrigation, and deficit irrigation for upland rice production (Figure 3). Other water-smart solutions are bunding and leveling to control water drainage in lowland rice (Figures 8A,B), plastic mulching, vermicomposting and organic amelioration, inorganic deep fertilizer placement, and exogenous application of biosynthetic hormones, moderated farming along the banks of reservoirs and rivers (Figure 9). Some climate-smart water solutions may present added advantages. For instance, solar-powered pumps to access groundwater avoid over-pumping by offering small farmers the opportunity to sell excess solar power to the utility (IWMI, 2017). Critical interventions to climate-smart water solutions also include developing and deploying improved drought-tolerant, stress-resilient and high-yielding crops such as maize (STMA, 2020; Figure 10).
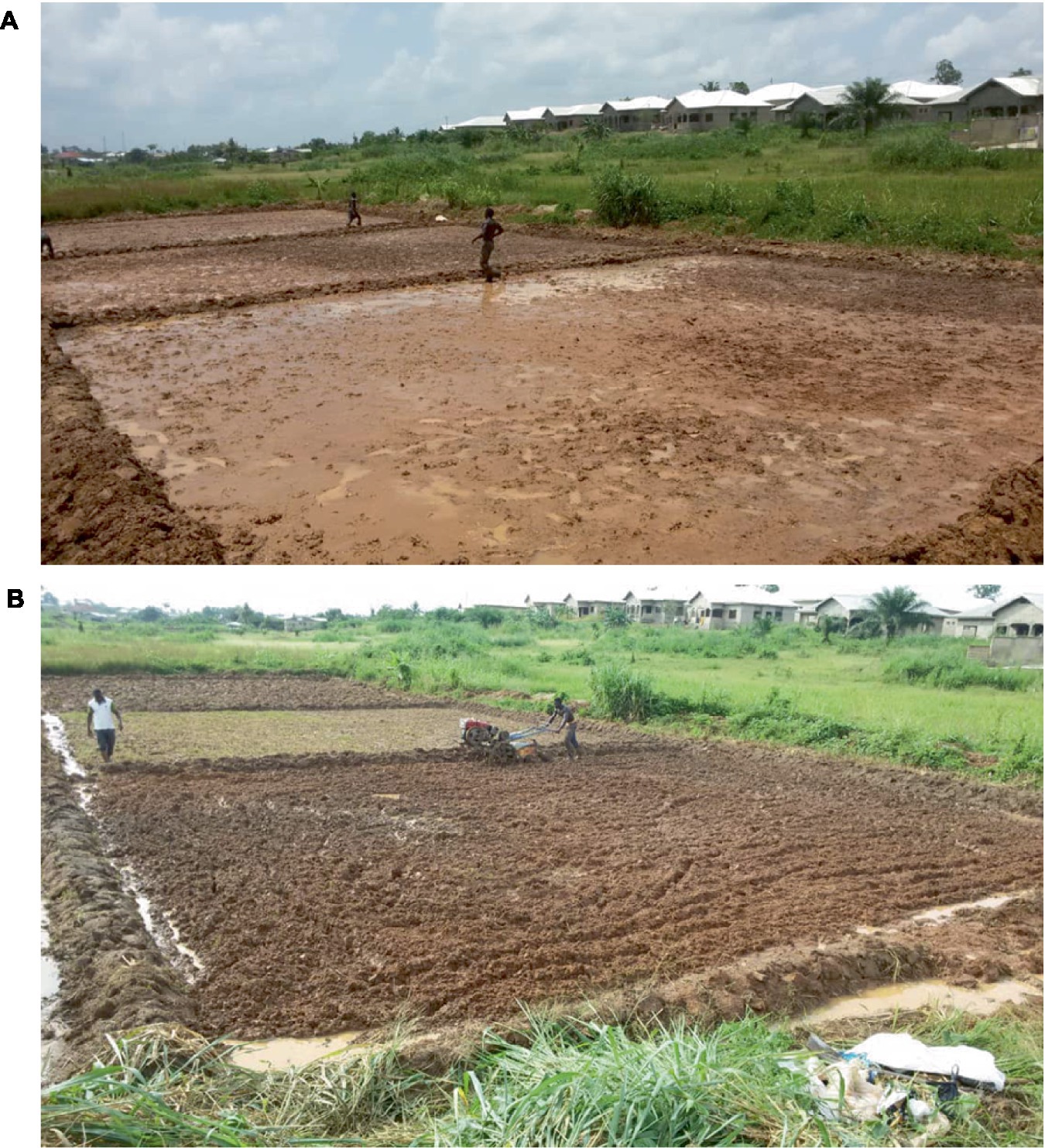
Figure 8. Bunding (A) and leveling (B) to control water drainage for rice breeding activities at an outstation of CSIR-Crops Research Institute, Ghana. Photo credit: Maxwell Darko Asante, 2022.
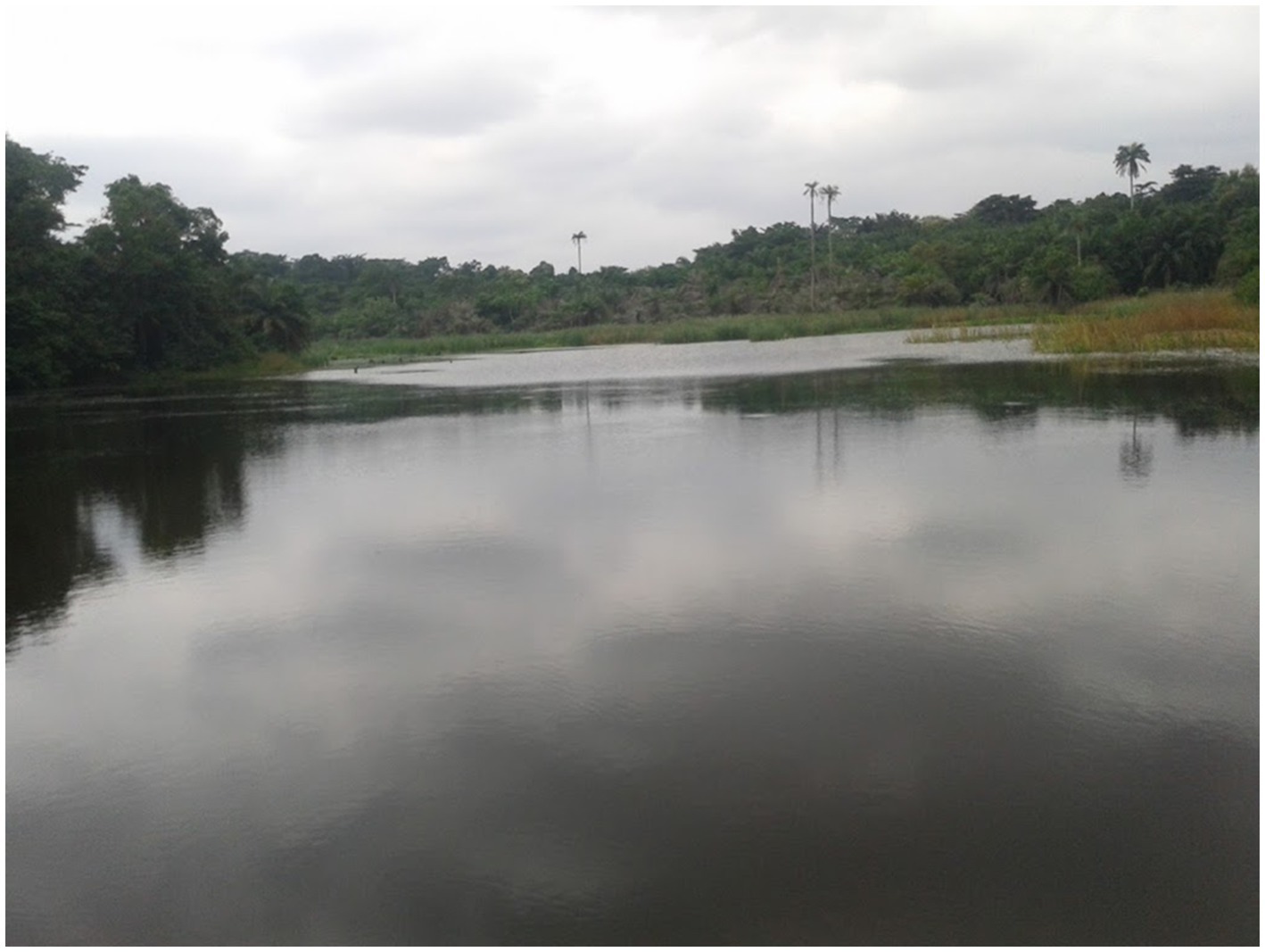
Figure 9. Water reservoir for irrigation, rainwater storage and watershed management of the catchment used for research activities at the CSIR-Crops Research Institute, Fumesua, Ghana. Photo credit: Felix Frimpong, 2016.
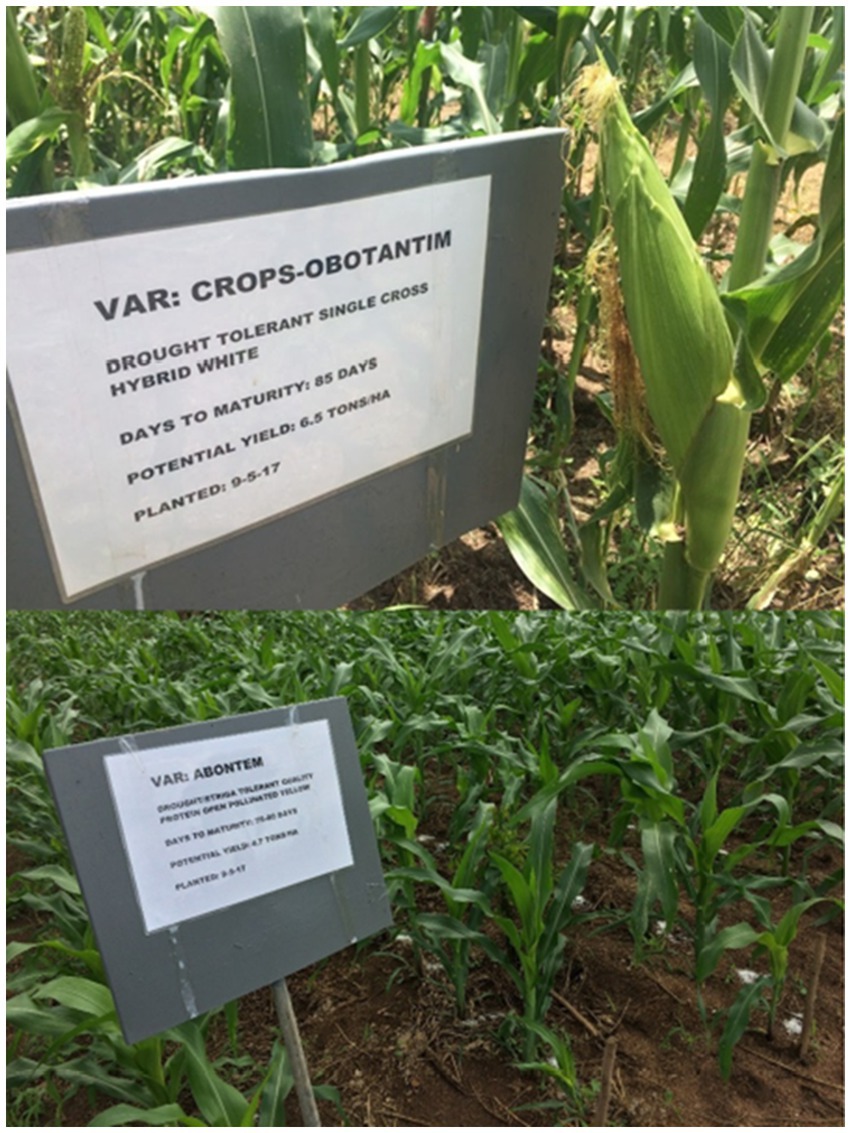
Figure 10. Demonstration of improved drought-tolerant maize varieties (CRI-Obotantim and CRI-Abontem, upper and lower panels, respectively) released by the Stress Tolerant Maize for Africa project at CSIR-CRI, Fumesua on-station. Photo credit: Felix Frimpong, 2016.
In most African countries, including Ghana, governments are key players in the development of the irrigation sectors. For instance, Ghana can boast 23 irrigation schemes from 2016 till date, managed by the Irrigation Development Authority. In Cote d’Ivoire, the Bagri irrigation scheme is a center for vegetables and cereals like tomatoes, onions, green peppers and rice. In Egypt and Niger, the Nile irrigation scheme serves as a major water source for agriculture. Most government-led irrigation schemes adopt participatory-irrigation development with farmers and the private sector. These irrigation schemes have used surface and groundwater resources for effective and efficient development (Peter, 2004).
However, one of the reasons why these climate-smart efforts have not paid off could be attributed to the use of improved varieties and fertilizers without following good agronomic practices (Awio et al., 2022). Good agronomic practices, or “good agronomy, “is an integrated, coherent set of crop, soil, water, weed, disease, and pest management practices crucial to boosting yields alongside fertilizer and improved crop variety use (Awio et al., 2022).
The primary goal of Climate-Smart Agriculture (CSA) is to use modern agricultural technology and advances, such as inorganic fertilizers, pesticides, foodstuffs, food supplements, high-yielding varieties, land management, and irrigation techniques, to achieve high yields and resilient systems (Azadi et al., 2022). Thus, it is vital to meet the food needs of an expanding population while also stimulating the economic growth needed to eliminate poverty (Azadi et al., 2021). However, there is now a growing critique of “climate-smart agriculture” (Alexander, 2019; Eriksen et al., 2019). Ineffective CSA targeting thus lacks the particular livelihood transition capacities of small-scale farming households, which are tied to the opportunities and limits provided by varied agricultural and non-agricultural livelihood routes. Vulnerable-Smart Agriculture (VSA) has been suggested, which begins with small-scale farmers and tries to understand the challenges they confront in improving their livelihoods, followed by the “participatory” design of intervention programs to assist farmers in overcoming these barriers. Vulnerable-Smart Agriculture (VSA) begins on a small scale (Azadi et al., 2021).
3.4. Water-smart crop farming and nature-based solutions synergies
Water-smart crop farming and practical nature-based solutions exhibit substantial correlations, providing valuable insights into sustainable agriculture’s response to climate change challenges. Both approaches prioritize resource optimization, with a particular focus on efficient water utilization and harnessing the potential of natural ecosystems to enhance overall resource efficiency. This convergence becomes especially pertinent in regions grappling with water scarcity and climate-induced disruptions in agricultural systems. Furthermore, both strategies contribute significantly to enhancing climate resilience.
Water-smart crop farming entails the adaptation of crop and irrigation practices to changing climate conditions, ensuring sustained agricultural productivity (Patle et al., 2020). Simultaneously, nature-based solutions bolster ecosystem resilience, indirectly benefiting agriculture by mitigating climate-related challenges. Nature-based solutions often promote biodiversity, facilitating natural pest control and pollination, which ultimately benefit crop health (Cuenca-Cambronero et al., 2023). Notably, water-smart farming practices, such as reduced chemical usage, align with biodiversity-enhancing principles. Nature-based solutions directly leverage these ecosystem services, while water-smart farming practices indirectly reap their rewards.
For instance, consider peri-urban vegetable farming, which employs pro-poor irrigation systems like earth dugouts, bunding, leveling, raised beds, and contours or water drainage structures to efficiently manage water resources while producing food for urban dwellers. Smallholder farmers engaging in peri-urban vegetable production foster biodiversity and promote environmentally friendly practices. These farms offer affordable sources of vegetables and fruits while utilizing previously unproductive land spaces, a crucial aspect for policymaking in sustainable and affordable food production.
Practical examples of nature-based solutions underscore the seamless synergy between these two approaches. Agroforestry, which combines tree planting with agriculture, enhances water retention, provides shade, and improves soil fertility, benefiting both water-smart crop farming and nature-based solutions. Wetland restoration enhances water quality and availability, while cover cropping enhances soil health and resilience, aligning seamlessly with both strategies. Terracing, often associated with water-smart farming, reduces soil erosion and mimics natural landscapes, thus embracing nature-based principles.
Key lessons emerge from these interactions, highlighting the potential for synergistic effects when combining water-smart crop farming with nature-based solutions. However, it underscores the importance of local adaptation, acknowledging the need to tailor solutions to specific regional conditions. Community engagement proves pivotal for successful implementation, and regular monitoring and evaluation remain critical for continuous improvement. Supportive government policies become essential in fostering the adoption of these approaches, with incentives and regulations serving as potent tools to encourage sustainable practices and the preservation of natural ecosystems.
3.5. Farm-level adaptation and mitigation strategies to minimize climate change impacts
The mitigation and adaptation of crop varieties and management, water and soil management, financial schemes, migration, and culture, and finally agriculture and weather services are four themes that can be used to group strategies to reduce the effects of climate change on farms in Africa, possibly Asia, and other parts of the world (Magesa et al., 2023).
Smart-agricultural technologies that depend on improved crop varieties and their management, water and soil management have been substantially addressed and discussed so far in our study. These methods include the use of improved drought-tolerant crop varieties, crop diversification, rainwater harvesting and storage for irrigation as well as watershed management along local farm water banks.
Additionally, changing planting dates and planting early maturing crops. The use of inexpensive dugouts and furrows for vegetable farming in urban and peri-urban dwellers, correct bundling and leveling in rice agriculture, and decreased tillage techniques. Others may include, crop rotations and legumes/tree crops integration, alternate wetting and drying for lowland rice cultivation, yam/cassava legumes intercropping for improved water use efficiency and soil cover.
Other transformational improvements, such as expanding irrigation infrastructure, promoting crop insurance, utilizing better varieties, and expanding options for livelihood diversification have been suggested (Namara and Hilmy, 2014; Magesa et al., 2023). The financial schemes, migration, and culture; and agriculture and weather services aspects of reducing adaptation and mitigation are equally important as those strategies we have elaborated early on for resource-poor smallholder farmers to cope and achieve meaningful crop yield.
4. Conclusion and remarks
In conclusion, this review has elucidated a comprehensive framework for enhancing water management and cultivating sustainable water-smart cropping practices in agriculture. Throughout our analysis, we have underscored several key takeaways. We have emphasized the integral role of Water-Smart Cropping as a nature-based solution for climate change adaptation in agriculture, showcasing its potential to secure water resources for future generations. We have highlighted the diversity of climate-responsive food-cropping systems, water-saving techniques, and technological innovations that constitute the fabric of water-smart farming. These strategies collectively contribute to sustainable food production. Our vision extends to the alignment of water-smart cropping systems with the United Nations’ 2030 sustainable development agenda, focusing on ending poverty, preserving human lives, safeguarding the planet, fostering prosperity, and nurturing partnerships for sustainability. It is imperative to acknowledge that the strong emphasis on water conservation in water-smart cropping may lead to yield penalties or reduced crop yield potential in certain agroecological zones where water is not the primary limiting factor. Moving forward, we propose several avenues for future research that warrant focused attention. Future research should prioritize strategies and interventions aimed at enhancing the widespread adoption of water-smart crop farming practices, especially in resource-limited regions of global south countries. This includes examining effective knowledge transfer mechanisms and adoption incentives. Investigating methods to balance water-saving measures with crop-centric strategies in different agroecological contexts is essential to mitigate potential yield trade-offs. Rigorous assessments of the impact of water-smart cropping on agricultural productivity, livelihoods, and environmental sustainability should be conducted to provide empirical evidence for policymakers and stakeholders. Comparative studies across various regions can help identify best practices and region-specific adaptations of water-smart cropping, contributing to a broader understanding of its applicability. Future research should also consider the role of gender and youth in the adoption and implementation of water-smart cropping practices, with a focus on promoting inclusive and equitable development. In terms of limitations, our study’s predominant focus on water conservation may limit its applicability in agroecological zones where water is not a primary constraint. The findings presented here are based on the West African context, and their generalizability to other regions may vary. In closing, while water-smart crop farming holds promise as a nature-based solution for climate change adaptation in agriculture, its successful implementation demands a nuanced approach that considers regional disparities and seeks to balance water-saving imperatives with crop-centric strategies. The future of sustainable agriculture and food security depends on our ability to bridge these gaps, address limitations, and pursue rigorous research to drive meaningful change in global agricultural practices.
Author contributions
FF, MDA, and PA-Y conceived the research idea. FF and CP wrote the first draft manuscript. FF and MDA shared relevant research photographs for the manuscript. MDA, PA-Y, ED, PFR, AA, KA, MOA, AK, and HB read and edited the manuscript. All authors contributed to the article and approved the submitted version.
Funding
The research was funded with support from the CSIR-Crops Research Institute, Kumasi, Ghana.
Acknowledgments
We would like to acknowledge the Director (M. B. Mochiah) and staff of the CSIR-Crops Research Institute, Ghana, who provided administrative and technical assistance during the desk study and provided valuable resources to this write-up.
Conflict of interest
The authors declare that the research was conducted in the absence of any commercial or financial relationships that could be construed as a potential conflict of interest.
Publisher’s note
All claims expressed in this article are solely those of the authors and do not necessarily represent those of their affiliated organizations, or those of the publisher, the editors and the reviewers. Any product that may be evaluated in this article, or claim that may be made by its manufacturer, is not guaranteed or endorsed by the publisher.
References
Abbass, K., Qasim, M. Z., Song, H., Murshed, M., Mahmood, H., and Younis, I. (2022). A review of the global climate change impacts, adaptation, and sustainable mitigation measures. Environ. Sci. Pollut. Res. 29, 42539–42559. doi: 10.1007/s11356-022-19718-6
Alexander, S. (2019). What climate-smart agriculture means to members of the global Alliance for climate-smart agriculture. Future Food 7, 21–30. doi: 10.17170/kobra-201812207
Ali, A., Xia, C., Jia, C., and Faisal, M. (2020). Investment profitability and economic efficiency of the drip irrigation system: evidence from Egypt*. Irrig. Drain. 69, 1033–1050. doi: 10.1002/ird.2511
Amante, M. M., Vikram, P., Swamy, B. P. M., Maturan, P. C., Dixit, S., and Kumar, A. (2012). Yield potential of rainfed lowland and upland rice in different irrigation ways. Philipp. J. Crop Sci. 2012:11.
Ankrah, D. A., Anum, R., Anaglo, J. N., and Boateng, S. D. (2023). Influence of sustainable livelihood capital on climate variability adaptation strategies. Environ. Sustain. Indic. 18:100233. doi: 10.1016/j.indic.2023.100233
Ashraf, S., Nazemi, A., and AghaKouchak, A. (2021). Anthropogenic drought dominates groundwater depletion in Iran. Sci. Rep. 11:9135. doi: 10.1038/s41598-021-88522-y
Awio, T., Senthilkumar, K., Dimkpa, C. O., Otim-Nape, G. W., Struik, P. C., and Stomph, T. J. (2022). Yields and yield gaps in lowland Rice systems and options to improve smallholder production. Agronomy 12:552. doi: 10.3390/agronomy12030552
Azadi, H., Movahhed Moghaddam, S., Burkart, S., Mahmoudi, H., Van Passel, S., Kurban, A., et al. (2021). Rethinking resilient agriculture: From Climate-Smart Agriculture to Vulnerable-Smart Agriculture. J. Clean. Prod. 319:128602. doi: 10.1016/j.jclepro.2021.128602
Azadi, H., Siamian, N., Burkart, S., Moghaddam, S. M., Goli, I., Dogot, T., et al. (2022). “Climate smart agriculture: mitigation and adaptation strategies at the global scale” in Climate-induced innovation: Mitigation and adaptation to climate change. eds. M. Coromaldi and S. Auci (Berlin: Springer International Publishing), 81–140.
Bayulken, B., Huisingh, D., and Fisher, P. M. J. (2021). How are nature based solutions helping in the greening of cities in the context of crises such as climate change and pandemics? A comprehensive review. J. Clean. Prod. 288:125569. doi: 10.1016/J.JCLEPRO.2020.125569
Bessah, E., Donkor, E., Raji, A. O., Taiwo, O. J., Ololade, O. O., Strapasson, A., et al. (2022). Factors affecting farmers’ decision to harvest rainwater for maize production in Ghana. Front. Water 4:966966. doi: 10.3389/frwa.2022.966966
Chen, D., and Hu, W. (2023). Temporal and spatial effects of heavy metal-contaminated cultivated land treatment on agricultural development resilience. Land 12:945. doi: 10.3390/land12050945
Chartzoulakis, K., and Bertaki, M. (2015). Sustainable water management in agriculture under climate change. Agriculture and Agricultural Science Procedia 4, 88–98. doi: 10.1016/J.AASPRO.2015.03.011
Cohen-Shacham, E., Andrade, A., Dalton, J., Dudley, N., Jones, M., Kumar, C., et al. (2019). Core principles for successfully implementing and upscaling nature-based solutions. Environ. Sci. Pol. 98, 20–29. doi: 10.1016/j.envsci.2019.04.014
CSIR and MOFA. (2012). CSIR crop varieties released and registered in Ghana. Varietal release in Ghana. Available at: https://www.csir.org.gh/images/Doc/NewsLetter/CropVarietiesReleasedandregisteredinGHANA.pdf.
Cuenca-Cambronero, M., Blicharska, M., Perrin, J. A., Davidson, T. A., Oertli, B., Lago, M., et al. (2023, 2023). Challenges and opportunities in the use of ponds and pondscapes as nature-based solutions. Hydrobiologia 850, 3257–3271. doi: 10.1007/s10750-023-05149-y
Dixon-Woods, M., Bonas, S., Booth, A., Jones, D. R., Miller, T., and Sutton, A. J. (2006). How can systematic reviews incorporate qualitative research? Crit. Perspect. Qual. Res. 6, 27–44. doi: 10.1177/1468794106058867
Dumani, A., Mbangcolo, M. M., Mpambani, B., Mpengesi, X., and Titimani, M. (2023). Impact of a multi-stakeholder approach on rural livelihood and socio-economic status of the farming community at Zanyokwe irrigation scheme, Amahlathi local municipality, eastern cape. S. Afr. J. Agric. Ext. 51, 1–12.
Eriksen, S. H., Cramer, L. K., Vetrhus, I., and Thornton, P. (2019). Can climate interventions open up space for transformation? Examining the case of climate-smart agriculture (CSA) in Uganda. Front. Sustain. Food Syst. 3:111. doi: 10.3389/fsufs.2019.00111
FAO. (2013). Sourcebook on climate smart agriculture, Forestry and Fisheries. Rome, Italy: Food and Agricultural Organization of the United Nations. Available at: https://www.fao.org/3/i3325e/i3325e.pdf.
FAO. (2022). Water efficiency, productivity and sustainability in the NENA regions (WEPS-NENA). Food and Agricultural Organization of the United Nations. Available at: https://www.fao.org/in-action/water-efficiency-nena/activities/es/.
FAOSTAT. (2022). Statistical database. Rome: Food and Agriculture Organization of the United Nations. Available at: https://fao.org/faostat/en/#compare.
Fawole, B. E., and Aderinoye-Abdulwahab, S. A. (2021). “Farmers’ Adoption of climate smart practices for increased productivity in Nigeria” in African handbook of climate change adaptation. eds. N. Oguge, D. Ayal, L. Adeleke, and I. Da Silva (Berlin, Cham: Springer International Publishing), 495–508.
Feizizadeh, B., Lakes, T., Omarzadeh, D., Sharifi, A., Blaschke, T., and Karimzadeh, S. (2022). Scenario-based analysis of the impacts of Lake drying on food production in the lake Urmia Basin of northern Iran. Sci. Rep. 12:6237.
Frimpong, F., Amponsah, S., Owusu Danquah, E., Agyeman, K., Oteng-Darko, P., Oppong, C. P., et al. (2017). Assessment of aquaponics-based food production system effluents on the performance of maize. Int. J. Agric. Res. Rev. 5, 615–627.
Guillaumot, L., Smilovic, M., Burek, P., De Bruijn, J., Greve, P., Kahil, T., et al. (2022). Coupling a large-scale hydrological model (CWatM v1. 1) with a high-resolution groundwater flow model (MODFLOW 6) to assess the impact of irrigation at regional scale. Geosci. Model Dev. 15, 7099–7120. doi: 10.5194/gmd-15-7099-2022
Haileslassie, A., Mekuria, W., Uhlenbrook, S., Ludi, E., and Schmitter, P. (2022). Gap analysis and methodological framework to assess and develop water centric sustainable agricultural intensification pathways in sub-Saharan Africa. Front. Water 4:747610. doi: 10.3389/frwa.2022.747610
Hammond, J., Fraval, S., van Etten, J., Suchini, J. G., Mercado, L., Pagella, T., et al. (2017). The rural household multi-indicator survey (RHoMIS) for rapid characterisation of households to inform climate smart agriculture interventions: description and applications in East Africa and Central America. Agric. Syst. 151, 225–233. doi: 10.1016/j.agsy.2016.05.003
Hoover, D. L., Abendroth, L. J., Browning, D. M., Saha, A., Snyder, K., Wagle, P., et al. (2023). Indicators of water use efficiency across diverse agroecosystems and spatiotemporal scales. Sci. Total Environ. 864:160992. doi: 10.1016/j.scitotenv.2022.160992
Islam, M. S., Bell, R. W., Miah, M. M., and Alam, M. J. (2022). Unbalanced fertilizer use in the eastern Gangetic plain: the influence of government recommendations, fertilizer type, farm size and cropping patterns. PLoS One 17:e0272146. doi: 10.1371/journal.pone.0272146
IWMI. (2017). Water solutions for a changing world. IWMI annual report 2016. 32. Colombo, Sri Lanka: International Water Management Institute.
Jin, Q., Raza, S. H., Mahmood, N., Zaman, U., Saeed, I., Yousaf, M., et al. (2022). Exploring influence of communication campaigns in promoting regenerative farming through diminishing farmers’ resistance to innovation: an innovation resistance theory perspective from global south. Front. Psychol. 13:924896. doi: 10.3389/fpsyg.2022.924896
Kangogo, D., Dentoni, D., and Bijman, J. (2021). Adoption of climate-smart agriculture among smallholder farmers: does farmer entrepreneurship matter? Land Use Policy 109:105666. doi: 10.1016/j.landusepol.2021.105666
Khan, K. S., Kunz, R., Kleijnen, J., and Antes, G. (2003). Five steps to conducting a systematic review. J. R. Soc. Med. 96, 118–121. doi: 10.1177/014107680309600304
Khatun, M., Sarkar, S., Era, F. M., Islam, A. K. M., Anwar, M. D. P., Fahad, S., et al. (2021). Drought stress in grain legumes: effects, tolerance mechanisms and management. Agronomy 11:2374. doi: 10.3390/agronomy11122374
Krishnan, S. R., Nallakaruppan, M. K., Chengoden, R., Koppu, S., Iyapparaja, M., Sadhasivam, J., et al. (2022). Smart water resource management using artificial intelligence–a review. Sustainability 14:13384. doi: 10.3390/su142013384
Liu, H.-Y., Jay, M., and Chen, X. (2021). The role of nature-based solutions for improving environmental quality, health and well-being. Sustainability 13:10950. doi: 10.3390/su131910950
Magesa, B. A., Mohan, G., Matsuda, H., Melts, I., Kefi, M., and Fukushi, K. (2023). Understanding the farmers’ choices and adoption of adaptation strategies, and plans to climate change impact in Africa: a systematic review. Clim. Serv. 30:100362. doi: 10.1016/j.cliser.2023.100362
Makate, C. (2019). Effective scaling of climate smart agriculture innovations in African smallholder agriculture: a review of approaches, policy and institutional strategy needs. Environ. Sci. Pol. 96, 37–51. doi: 10.1016/j.envsci.2019.01.014
Mango, N., Clifton, M., Lulseged, T., Powell, M., and Gift, N. (2018). Adoption of small-scale irrigation farming as a climate-smart agriculture practice and its influence on household income in the Chinyanja triangle, Southern Africa. Land 7, 1–19. doi: 10.3390/land7020049
Moradet, S. (2005). And international water management institute. In: Improving Irrigation Project Planning and Implementation Processes in Sub-Saharan Africa: Diagnosis and Recommendations.
Mushi, G. E., Serugendo, G. D. M., and Burgi, P. Y. (2023). Data management system for sustainable agriculture among smallholder farmers in Tanzania: Research-in progress. Inf. Technol. Dev. 2023, 1–24. doi: 10.1080/02681102.2023.2215528
Nalumu, D. J., Mensah, H., and Amponsah, O. (2021). Stakeholder collaboration and irrigation practices in Ghana: issues, challenges, and the way forward. SN Appl. Sci. 3:576. doi: 10.1007/s42452-021-04407-9
Namara, R. E., and Hilmy, S. (2014). Irrigation in West Africa: current status and a view to the future. In: Proceedings of the Workshop Held in Ouagadougou, Burkina Faso, December 1-2, 2010.
Ndhlovu, E., and Mhlanga, D. (2023). “Smart technologies, climate change, and smallholder farmer production in Zimbabwe” in The fourth industrial revolution in Africa: Exploring the development implications of smart Technologies in Africa. eds. D. Mhlanga and E. Ndhlovu (Cham: Springer Nature Switzerland), 293–309.
Ndlovu, N. P., and Borrass, L. (2021). Promises and potentials do not grow trees and crops. A review of institutional and policy research in agroforestry for the southern African region. Land Use Policy 103:105298. doi: 10.1016/j.landusepol.2021.105298
Oral, H. V., Radinja, M., Rizzo, A., Kearney, K., Andersen, T. R., Krzeminski, P., et al. (2021). Management of urban waters with nature-based solutions in circular cities–exemplified through seven urban circularity challenges. Water 13:3334. doi: 10.3390/w13233334
Oteng-Darko, P., Frimpong, F., Sarpong, F., and Leticia, P. A. (2018). Promoting smallholder irrigation for food security-a review. Afr. J. Food Integr. Agric. 2, 1–7. doi: 10.25218/ajfia.2018.01.001.01
Otieno, E. O., Gweyi, J. O., and Oduor, N. O. (2022). “Revolutionizing crops and soil resources’ resilience to climate change: a case for best-fit agronomic practices in low and high input systems” in Agriculture, livestock production and aquaculture: Advances for smallholder farming systems. eds. A. Kumar, P. Kumar, S. S. Singh, B. H. Trisasongko, and M. Rani, vol. 2 (Cham: Springer International Publishing), 15–31.
Owusu Danquah, E., Frimpong, F., Yeboah, S., Narteh, E., Weebadde, C., Ama, S., et al. (2022a). Pigeonpea (Cajanus cajan) and white yam (Dioscorea rotundata) cropping system: improved resource use and productivity in Ghana. Ann. Agric. Sci. 67, 60–71. doi: 10.1016/j.aoas.2022.05.001
Owusu Danquah, E., Danquah, F. O., Frimpong, F., Dankwa, K. O., Weebadde, C. K., Ennin, S. A., et al. (2022b). Sustainable intensification and climate-smart yam production for improved food security in West Africa: a review. Front. Agron. 4:858114. doi: 10.3389/fagro.2022.858114
Pahl-Wostl, C. (2007). Transitions towards adaptive management of water facing climate and global change. Water Resour. Manag. 21, 49–62. doi: 10.1007/S11269-006-9040-4/METRICS
Patle, G. T., Kumar, M., and Khanna, M. (2020). Climate-smart water technologies for sustainable agriculture: a review. J. Water Clim. Change 11, 1455–1466. doi: 10.2166/wcc.2019.257
Peter, J. R. (2004). Participatory irrigation management: a socio-anthropological. Management 303, 297–303.
Rafael, B. M. (2023). The importance of agricultural development projects: a focus on sustenance and employment creation in Kenya, Malawi, Namibia, Rwanda, and Uganda. J. Agric. Chem. Environ. 12, 152–170. doi: 10.4236/jacen.2023.122013
Ross, A., Evans, R., and Nelson, R. (2023). Risks related to groundwater in the Murray Darling basin. Aust. J. Water Resour. 27, 31–46. doi: 10.1080/13241583.2022.2140904
Saito, K., Senthilkumar, K., Dossou-Yovo, E. R., Ali, I., Johnson, J. M., Mujawamariya, G., et al. (2023). Status quo and challenges of rice production in sub-Saharan Africa. Plant Prod. Sci. 26, 320–333. doi: 10.1080/1343943X.2023.2241712
Sarabi, S. E., Han, Q., Romme, A. G. L., De Vries, B., and Wendling, L. (2019, 2019). Key enablers of and barriers to the uptake and implementation of nature-based solutions in urban settings: A review. Resources 8:121. doi: 10.3390/RESOURCES8030121
Scanlon, B. R., Fakhreddine, S., Rateb, A., de Graaf, I., Famiglietti, J., Gleeson, T., et al. (2023). Global water resources and the role of groundwater in a resilient water future. Nat. Rev. Earth Environ 4, 87–101. doi: 10.1038/s43017-022-00378-6
Seddon, N. (2022). Harnessing the potential of nature-based solutions for mitigating and adapting to climate change. Science 1979, 1410–1416. doi: 10.1126/science.abn9668
Seddon, N., Chausson, A., Berry, P., Girardin, C. A. J., Smith, A., and Turner, B. (2020). Understanding the value and limits of nature-based solutions to climate change and other global challenges. Philos. Trans. R. Soc. B 375:120. doi: 10.1098/rstb.2019.0120
Shah, M. A. R., Renaud, F. G., Anderson, C. C., Wild, A., Domeneghetti, A., Polderman, A., et al. (2020). A review of hydro-meteorological hazard, vulnerability, and risk assessment frameworks and indicators in the context of nature-based solutions. Int. J. Disaster Risk Reduc. 50:101728. doi: 10.1016/j.ijdrr.2020.101728
STMA. (2020). Stress tolerant maize builds the resilience of Africa’s smallholder farmers. Stress Tolerant Maize for Africa. STMA Bulletin. Available at: https://stma.cimmyt.org/wp-content/uploads/sites/46/2020/05/STMA-I-FINAL-Bulletin-May-14-2020.pdf.
Takeshima, H., and Mano, Y. (2023). “Intensification of Rice farming: the role of mechanization and irrigation” in Rice Green Revolution in Sub-Saharan Africa. eds. K. Otsuka, Y. Mano, and K. Takahashi (Berlin: Springer Nature), 143–160.
Valipour, E., Ketabchi, H., Safarishali, R., and Morid, S. (2023). Equity, social welfare, and economic benefit efficiency in the optimal allocation of coastal groundwater resources. Water Resour. Manag. 2023, 1–22. doi: 10.1007/s11269-023-03456-6
Wakweya, R. B. (2023). Challenges and prospects of adopting climate-smart agricultural practices and technologies: implications for food security. J. Agric. Food Res. 14:100698. doi: 10.1016/J.JAFR.2023.100698
World Bank. (2018). Farmer-led irrigation development: seeds of opportunity proceedings of the international conference on farmer-led irrigation. January 29-30, 2018. Available at: https://documents1.worldbank.org/curated/en/619301624377224422/Proceedings-of-the-International-Conference-on-Farmer-led-Irrigation.Docx.
Keywords: climate resilience, food production, small-scale irrigation, water-smart cropping, water use efficiency
Citation: Frimpong F, Asante MD, Peprah CO, Amankwaa-Yeboah P, Danquah EO, Ribeiro PF, Aidoo AK, Agyeman K, Asante MOO, Keteku A and Botey HM (2023) Water-smart farming: review of strategies, technologies, and practices for sustainable agricultural water management in a changing climate in West Africa. Front. Sustain. Food Syst. 7:1110179. doi: 10.3389/fsufs.2023.1110179
Edited by:
Anik Bhaduri, Griffith University, AustraliaReviewed by:
David Lopez-Carr, University of California, Santa Barbara, United StatesMuhammad Mubeen, COMSATS University Islamabad, Pakistan
Amani Alfarra, Food and Agriculture Organization of the United Nations, Italy
Copyright © 2023 Frimpong, Asante, Peprah, Amankwaa-Yeboah, Danquah, Ribeiro, Aidoo, Agyeman, Asante, Keteku and Botey. This is an open-access article distributed under the terms of the Creative Commons Attribution License (CC BY). The use, distribution or reproduction in other forums is permitted, provided the original author(s) and the copyright owner(s) are credited and that the original publication in this journal is cited, in accordance with accepted academic practice. No use, distribution or reproduction is permitted which does not comply with these terms.
*Correspondence: Felix Frimpong, ZmVsaXguZnJpbXBvbmdAeWFob28uY29t; Zi5mcmltcG9uZ0Bjcm9wcmVzZWFyY2gub3Jn