- 1State Key Laboratory for Managing Biotic and Chemical Threats to Quality and Safety of Agro-Products, Key Laboratory of Biotechnology in Plant Protection, Ministry of Agriculture and Rural Affairs, Institute of Plant Protection and Microbiology, Zhejiang Academy of Agricultural Sciences, Hangzhou, China
- 2State Key Laboratory of Ecological Pest Control for Fujian and Taiwan Crops, Key Lab of Biopesticide and Chemical Biology, Ministry of Education and Fujian Province Key Laboratory of Insect Ecology, College of Plant Protection, Fujian Agriculture and Forestry University, Fuzhou, Fujian, China
- 3Lishui Academy of Agricultural and Forestry Sciences, Lishui, Zhejiang, China
- 4College of Ecology, Lishui University, Lishui, Zhejiang, China
Introduction: The south American tomato pinworm (Tuta absoluta), an invasive insect pest species, has established itself in more than 33 Chinese prefectures, where it is mainly damaging tomato crops. Immediate efforts have been initiated to find strategies to control this pest. Studies have shown that biochar (BC) amendment to soil can enhance plant growth and resistance to herbivory.
Methods: First, we quantified the morphological performance of tomato plants grown in different coconut bran and bamboo charcoal (v/v) combinations and selected the most beneficial one. Then we checked the T. absoluta survival on the tomato plants grown in the selected bamboo charcoal combination. Finally, we studied the leaf metabolite accumulation and gene expression changes in tomato plants after growing in the selected bamboo charcoal combination.
Results: We found that the 30:1 ratio of bamboo charcoal and coconut bran is the most beneficial to tomato growth as its amendment to soil increased tomato plant height, stem thickness, and chlorophyll content, whereas, the T. absoluta survival decreased. The metabolome profiles of BC tomato leaves showed an increased accumulation of flavonoids, terpenoids, and phenolic acids compared to CK. Transcriptome sequencing resulted in the identification of 244 differentially expressed genes. Most of the upregulated genes were associated with stress-related hub proteins, flavonoid biosynthesis, MAPK and phytohormone signaling, and terpenoid biosynthesis. Additionally, the expression of many genes related to signaling and defense was changed in response to the bamboo charcoal amendment.
Discussion: We conclude that bamboo charcoal induces biosynthesis of flavonoids, terpenoids, and phenolic acids, which improve plant growth and tolerance against T. absoluta, thus reducing the survival of destructive pests.
1. Introduction
Tuta absoluta (South American tomato pinworm) was a newly invaded insect pest species in Ili, Xinjiang, China in 2017, where it caused significant damage to tomato crops (Zhang et al., 2020a,b). This pest originated in Peru, South America and severely damaged tomato crops in the 1950s. Now it has spread to more than 100 countries in South America, Europe, Africa, and Asia (Campos et al., 2017; Biondi et al., 2018; Mansour et al., 2018; Han et al., 2019a). Since China is the largest producer of tomato in the world (Ghimire et al., 2017), the rapid spread of T. absoluta via fruit and seedling is threatening the Chinese tomato industry. Additionally, the fact that it can also feed on other host plants is alarming to local crop production. A recent survey reported that T. absoluta was found in 269 sites in 33 prefectures and the infestation percentage ranged from 78.1 to 100%, which is further alarming and calls for strategies to control this pest.
Among the major control strategies adopted in other countries, chemical control (use of organophosphates, pyrethroids, abamectin, and other broad-range chemicals) remains the principal option to control this invasive insect pest (Desneux et al., 2022). However, this strategy causes severe damage to the environment as well as public health. Furthermore, there are reports on the development of resistance due to short generation time and high biotic potential (Guedes et al., 2019). On the contrary, the biocontrol strategy of using hemipteran mirids has been found to be the most promising due to the ease of their establishment in tomato fields and the high predation rate (Silva et al., 2016). Moreover, nearly 15 arthropod species have been reported to prey on T. absoluta (Zappala et al., 2013). However, such biocontrol strategies require rearing of the bio-control agents on different scales, controlled testing on a domestic scale, and official registration (Yazdanpanah et al., 2021). Among the other strategies, the use of fungi-derived natural products and host plant resistance are suitable from the environment as well as public safety perspectives. Some studies have also reported the adaption of combined approaches such as host plant resistance together with selective insecticides and biocontrol agents (Wangari Nderitu et al., 2020).
One of the non-chemical alternative methods is the use of products derived from agricultural and other organic wastes such as biochar. Biochar amendment to soil has shown pathogen-suppressive effects in a wide range of plant species against plant-pathogenic bacteria, fungi, oomycetes, and nematodes (Poveda et al., 2021). Moreover, the use of biochar amendment can improve plant growth and resistance to herbivory by changing jasmonic acid (JA) levels (Waqas et al., 2018). Studies on tomatoes have shown that biochar application from different feedstocks enhanced plant growth and resistance against Meloidogyne incognita (Arshad et al., 2020, 2021). Biochar application can also reduce the reproductive potential of the English grain aphid by activating the expression of defense-related genes in wheat (Chen et al., 2019). Bamboo charcoal is recognized as a regenerative biochar due to its cost-effectiveness and environment friendliness (Kawakami et al., 2005). It has the potential to improve plant growth by improving soil properties, chlorophyll contents, photosynthesis rate, and biomass (Hua et al., 2012). Moreover, its by-products have shown activities against insect pests such as Tetrenchus cinnabarinus, Emposasca flavescens, and Aphis medicagini (Wang et al., 2015). Thus, these reports indicate that the use of bamboo charcoal can improve plant growth and may provide/enhance tolerance against invading insect pests. Based on these reports, we hypothesize that the addition of bamboo charcoal to the soil (or growing substrate) may improve the growth of tomato plants and induce metabolic responses and gene expression that may increase resistance to T. absoluta infection. Indeed, various abiotic factors could be manipulated to alter plant phenotypic traits, which could in turn trigger bottom-up effects on insect herbivores and even organisms from higher trophic levels (Han et al., 2022). Much evidence has been obtained for T. absoluta management via bottom-up effects (Han et al., 2019b).
The use of transcriptome sequencing and metabolome profiling can help us identify the key metabolites and genes that are accumulated or expressed, respectively, in tomato plants grown in soil amended with bamboo charcoal. A similar strategy has helped researchers identify genes and associated pathways such as protein processing, plant-pathogen interaction, photosynthesis, and signaling in response to different biotic stresses e.g., in pepper against Fusarium wild (Zhu et al., 2021), in oat against oat-stem-rust (Li et al., 2022), and in strawberry fruits against Botrytis cinerea (De Tender et al., 2021). In this study, we used a combined metabolome profiling and transcriptome sequencing approach to understand the key changes in the accumulated metabolites and respective changes in gene expression in leaves of tomato plants grown in coconut bran amended with bamboo charcoal. We also studied if bamboo charcoal amendment effects the survival of T. absoluta on tomato plants. First, we quantified the morphological performance of tomato plants grown in different coconut bran and bamboo charcoal (v/v) combinations and selected the most beneficial one. Then we checked the T. absoluta survival on the tomato plants grown in the selected bamboo charcoal combination. Finally, we studied the leaf metabolite accumulation and gene expression changes in tomato plants after growing in the selected bamboo charcoal combination.
2. Materials and methods
2.1. Plant material and growth in bamboo biochar
The tomato (Solanum lycopersicum L.) variety “Moneymaker” was used as the plant material. The seeds were obtained from Zhejiang Academy of Agricultural Sciences, China. Seeds were surface sterilized and sown in square pots (7 cm wide and 9 cm deep) filled with sterilized coconut bran in an insect-free greenhouse at the experimental station of the Zhejiang Academy of Agricultural Sciences. The day/night temperatures and relative humidity were 24 ± 1/20 ± 1°C and 60 ± 5%. The experimental plants were not exposed to any type of pesticides or herbicides. The experimental plants (treated and CK) were supplemented with water-soluble macro-element fertilizer (OMEX, 18-18-18 NPK) at 2 g per pot to promote plant growth. When the seedlings reached two-leaf stage, they were transferred into different combinations of the sterilized coconut bran and bamboo charcoal v/v mixtures i.e., 10:1 (BC1), 30:1 (BC2), and 50:1 (BC3), whereas, 100% coconut bran was used as control (CK). Each treatment was repeated three times with fifteen plants per repetition (35 plants per treatment). The pots were watered every 2 days. The physiological parameters i.e., plant height and stem diameter were measured on the 10th and 20th day after seedling transplanting. For each trait measurement, eleven plants were randomly selected from each treatment. The means of three replicates were compared by the least significant difference test in Microsoft Excel® 2019 (www.microsoft.com). The most useful mixture (v/v) of coconut bran and bamboo charcoal was selected.
2.2. Insect feeding of BC-grown tomato plants and survivorship of T. absoluta
The South American tomato pinworm (T. absoluta) population was collected from Yili, Xinjiang, China in 2018, and was continuously raised in an artificial climate chamber with temperature, relative humidity, and light/dark period of 25 ± 1°C, 60 ± 5%, and 16/8 h, respectively. Tomato plants reaching five-leaf stage were used for experiments. Ten plants (six repeats for BC and CK each) were put in 50 × 50 × 50 cm3 nylon gauze insect cages and the newly emerged T. absoluta larvae were placed individually on each tomato plant for 12 days. In total, 120 newly emerged T. absoluta larvae were randomly allocated to the two treatments (BC and CK). The individual larvae on the tomato leaf were checked daily for development, and the pupated larvae were recorded as alive. Survival rates were recorded and statistically tested (p < 0.05) by using SPSS (version 26). The probability of survival was determined by using a Log-Rank test and the survival distributions of the T. absoluta on BC and CK plants were compared (GraphPad Prism 9).
2.3. Metabolome analysis
2.3.1. Sample preparation and extraction
Healthy tomato leaf samples (in triplicate) were collected from seedlings transplanted after 20 days. The sampled leaves were washed with distilled water, immediately frozen in liquid nitrogen, and stored in a −80°C freezer. The leaf samples were freeze-dried by vacuum freeze-dryer (Scientz-100F) and crushed using a mixer mill (MM 400, Retsch) with the help of zirconia beads for 1.5 min at 30 Hz. The lyophilized powder (50 mg) was then dissolved in 1.2 mL of 70% methanol solution, followed by vertexing for 30 s. The vertexing was done six times every 30 min. The mixture was then centrifuged at 12,000 rpm for 3 min, the extracts were filtered through a 0.22 μm microfilter (SCAA 104, ANPEL, Shanghai, China), and analyzed in UPLC-MS/MS.
2.3.2. UPLC conditions and ESI-Q TRAP-MS/MS
The extracts were analyzed using a UPLC-ESI-MS/MS system (UPLC, SHIMADZU Nexera X2, https://www.shimadzu.com.cn/; MS, Applied Biosystems 4,500 Q TRAP, Thermofisher, China). The column was Agilent SB-C18 (1.8 μm, 2.1 mm × 100 mm); The mobile phase consisted of solvent A, pure water with 0.1% formic acid, and solvent B, acetonitrile with 0.1% formic acid. For the sample measurements, we used a gradient program with the starting conditions of 95% A, 5% B. Within 9 min, a linear gradient to 5% A, 95% B was programmed, and a composition of 5% A, 95% B was kept for 1 min. Afterwards, a composition of 95% A, 5.0% B was adjusted within 1.1 min and kept for 2.9 min. The flow velocity was set as 0.35 mL per minute; the column oven was set to 40°C; the injection volume was 4 μL. The effluent was alternatively connected to an ESI-triple quadrupole-linear ion trap (QTRAP)-MS.
The ESI source operation parameters were as follows: source temperature 550°C; ion spray voltage (IS) 5,500 V (positive ion mode)/-4,500 V (negative ion mode); ion source gas I (GSI), gas II (GSII), and the curtain gas (CUR) were set at 50, 60, and 25 psi, respectively; the collision-activated dissociation (CAD) was high. Instrument tuning and mass calibration were performed with 10 and 100 μmol/L polypropylene glycol solutions in QQQ and LIT modes, respectively. QQQ scans were ac-quired as MRM experiments with collision gas (nitrogen) set to medium. DP (declustering potential) and CE (collision energy) for individual MRM transitions was done with further DP and CE optimization. A specific set of MRM transitions were monitored for each period according to the metabolites eluted within this period.
2.3.3. Bioinformatics analyses of metabolome data
Principal Component Analysis (PCA) was computed by using the “prcomp” function in R (www.r-project.org). Pearson correlation coefficients (PCC) between samples were calculated by the “cor” function in R and presented as heatmaps.
The metabolites were considered differentially accumulated if the variable importance in projection (VIP) was ≥ 1 and the absolute Log2 FC was (|Log2 FC| ≥ 1.0). The VIP values were extracted from OPLS-DA results, which were generated using the R package MetaboAnalystR. The data was log-transformed and mean centered before OPLS-DA. In order to avoid overfitting, a permutation test (200 permutations) was performed. The identified metabolites were annotated using the KEGG Compound database (http://www.kegg.jp/kegg/compound/), and annotated metabolites were then mapped to the KEGG Pathway database (http://www.kegg.jp/kegg/pathway.html). Pathways to which the differentially accumulated metabolites (DAMs) were mapped were fed into MSEA (metabolite sets enrichment analysis), and their significance was determined by the hypergeometric test's p-values.
2.4. Transcriptome sequencing
2.4.1. RNA extraction, library preparation, and sequencing
Total RNAs were extracted from triplicate leaf samples by using Spin Column Plant total RNA purification Kit (Sangon Biotech, China). RNA integrity and purity were tested by agarose gel electrophoresis and NanoPhotometer, respectively. RNA concentration was measured using spectrophotometer and Qubit 2.0 Fluorometer. PolyA tail enrichment of RNAs was done through Oligo (dT) magnetic beads to obtain mRNAs. The mRNAs were fragmented by using fragmentation buffer, and were used to synthesize cDNA using a kit (QuantiTech Reverse Transcription Kit, Qiagen). The cDNAs were purified by AMPure XP beads, A-tailed, and ligated with sequencing adapters. The fragment size selection was done by using AMPure XP beads, and cDNA libraries were obtained, quantified, and their insert size was detected by 2,100 bioanalyzer (Agilent Technologies, California, United States). q-PCR was used for quantification of an effective library concentration i.e., >2 nM. After finding out the effective library concentration, libraries were pooled, and sequenced on the Illumina HiSeq platform.
2.4.2. Bioinformatic analyses
For analyzing the sequencing data, first, we filtered the data and obtained high-quality reads followed by calculating the sequencing error rate and GC content distribution (Petrussa et al., 2013). Next, the transcriptome sequencing data was compared with the reference genome (Sola-num_lycopersicum.SL3.0) using HISAT2 (Wang et al., 2020). Then, BLAST was used to compare the transcript sequences with KEGG (Mukhtar et al., 2011) database. The transcript expression was computed as Fragments Per Kilobase of transcript per Million fragments mapped (FPKM). Finally, PCC and PCA were computed in R.
Differential gene expression between CK and the treatment group was computed as reported earlier (Liang et al., 2022) by using DESeq2 (Koch et al., 2016). The Benjamini Hochberg method was used to perform multiple hypothesis test corrections on the probability (P-value) to obtain false discovery rate (FDR). The screening criteria for the differentially expressed genes (DEGs) were |log2 Fold Change| ≥ 1 and FDR < 0.05. Venn diagrams were prepared in InteractiVenn (Farhangi-Abriz and Torabian, 2018). Pathway annotation of the DEGs was done in KEGG (https://www.genome.jp/kegg) (Mukhtar et al., 2011). The enrichment of DEGs in different KEGG pathways was done as reported earlier (Petrussa et al., 2013).
To validate the RNA sequencing-based expression, 15 tomato genes were selected for qRT-PCR analysis using the iScript cDNA Synthesis Kit (BIO-RAD, Hercules, CA, USA) and the iTaq Universal SYBR Green Supermix 50 ml (BIO-RAD, Hercules, CA, USA). cDNAs produced during the RNA sequencing stage were used and four biological and three technical replicates were included. The qRT-PCR was based on specific primer pairs (Supplementary Table 1) designed in Primer 3.0 software (http://bioinfo.ut.ee/primer3-0.4.0/) (Ding et al., 2019). TUB gene was used as the reference (Supplementary Table 1).
3. Results
3.1. Morphological performance of tomato seedlings under different bamboo charcoal (v/v) ratios
Plant height of BC1 (coconut bran and bamboo charcoal v/v 10:1), BC2 (coconut bran and bamboo charcoal v/v 30:1), and BC3 (coconut bran and bamboo charcoal v/v 50:1) treatments increased by 11.2, 16.59, and 24.35%, respectively, compared to CK 10 days after the seedlings transplantation. Whereas, after 20 days of transplanting, the plant height of tomato seedlings significantly increased in BC2, but no significant difference was found in BC1 and BC3 compared to CK (Figures 1A, Bi, Supplementary Table 2A). These results suggest that BC2 is suitable combination for tomato seedling growth. After seedlings were transplanted for 10 days, the stem thickness increased in BC3, however, in the case of BC1 and BC2, there was no significant increase as compared to CK. While, stem thickness of the seedlings transplanted after 20 days increased significantly in BC1 and BC2 but the increase was not significant in BC3 (5.06 cm) compared to CK (4.72 cm) (Figure 1Bii, Supplementary Table 2A). These observations further confirm the plant height results that BC2 is the most suitable combination of coconut bran and bamboo charcoal. Finally, the chlorophyll content was significantly different in BC1 as compared to CK in seedlings transplanted after 10 days, whereas the chlorophyll content of BC1 was obviously lower than that of other treatments in seedlings transplanted after 20 days (Figure 1Biii, Supplementary Table 2A). Thus, the plant height, stem thickness, and chlorophyll contents indicate that BC2 is the best mixture that supports tomato seedling growth. Also, these observations indicate that seedlings transplanted after 20 days are a better choice to understand the effect of BC on the growth and chlorophyll content as compared to CK (Figure 1).
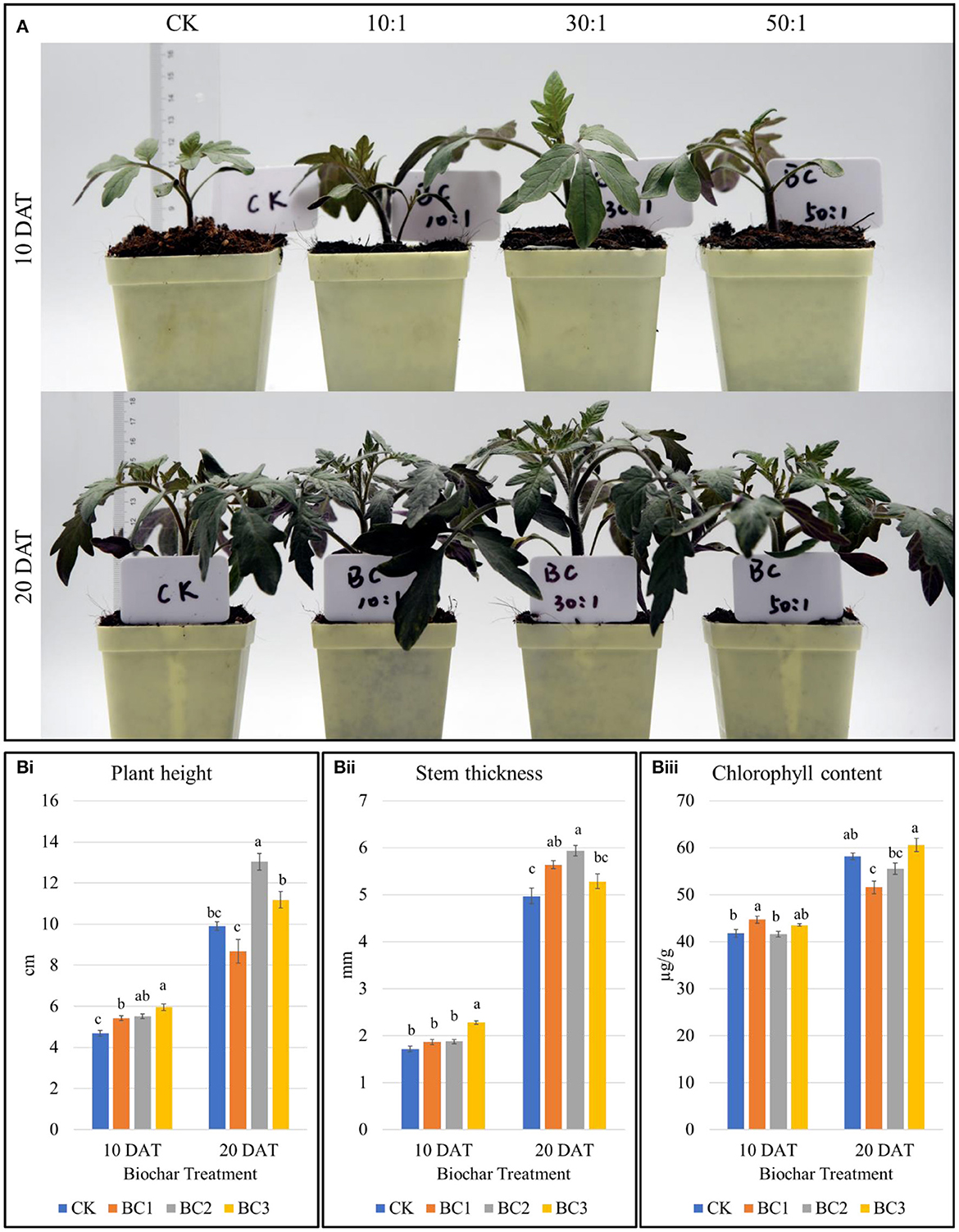
Figure 1. (A) Phenotypes of the tomato plants grown in three different coconut bran and bamboo charcoal combinations. (Bi) Plant height (Bii), stem thickness, and (Biii) chlorophyll content in tomato leaves grown in BC1 (10:1), BC2 (30:1), and BC3 (50:1). The bars show mean ± SEM (n = 11). Different letter in figure b indicates that differences in treatments are significant (p < 0.05).
3.2. Feeding of T. absoluta on tomato seedlings' leaves grown in BC
The T. absoluta were fed on the tomato seedling leaves grown in BC2 (thereafter BC) and CK. The probability of the survival of T. absoluta significantly decreased with increase in days as compared to CK (Figure 2A). Similarly, the survival rate of the T. absoluta was significantly lower in BC as compared to CK (Figure 2B). These observations indicate that BC significantly improves the tolerance of tomato seedlings against T. absoluta.
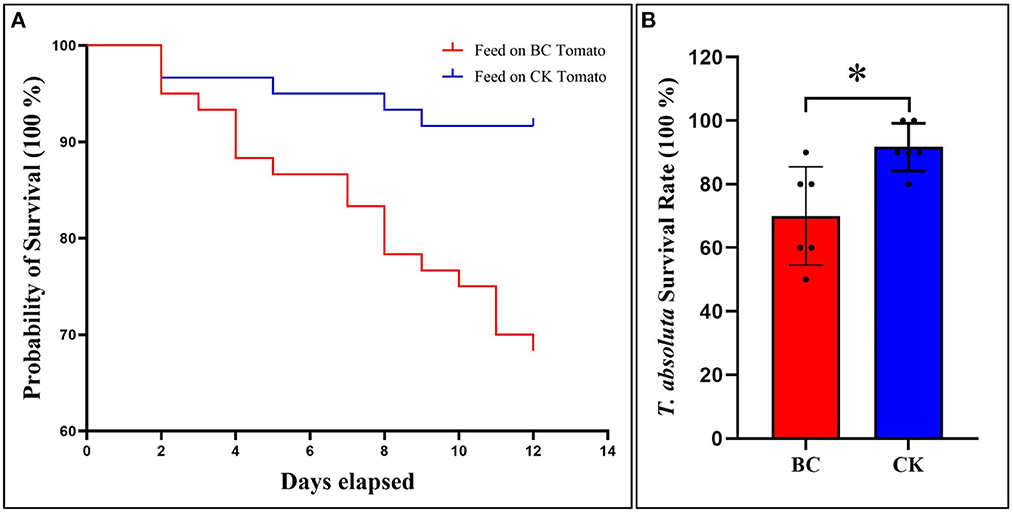
Figure 2. (A) Probability of survival of T. absoluta. (B) T. absoluta survival rate fed on BC and CK. CK (100% coconut bran), BC (30:1 v/v coconut bran and bamboo charcoal). The bars show mean ± SEM (n = 6). *Indicates significant differences between the CK and BC (p < 0.05).
3.3. Metabolomic response of tomato seedlings grown in bamboo charcoal
The metabolome profiles of BC and CK leaves could detect 919 metabolites that were classified as alkaloids, amino acids and derivatives, flavonoids, lignans and coumarins, lipids, nucleotides and derivatives, organic acids, phenolic acids, steroids, and terpenoids (Figure 3A). Principal component analysis showed that BC and CK replicates tended to group together (Figure 3B), whereas PCC was >0.93 (Figure 3C). Both the PCA and PCC analyses indicate that the sampling was reliable. Almost all compound classes were accumulated in higher quantities in BC as compared to CK (Figure 3D).
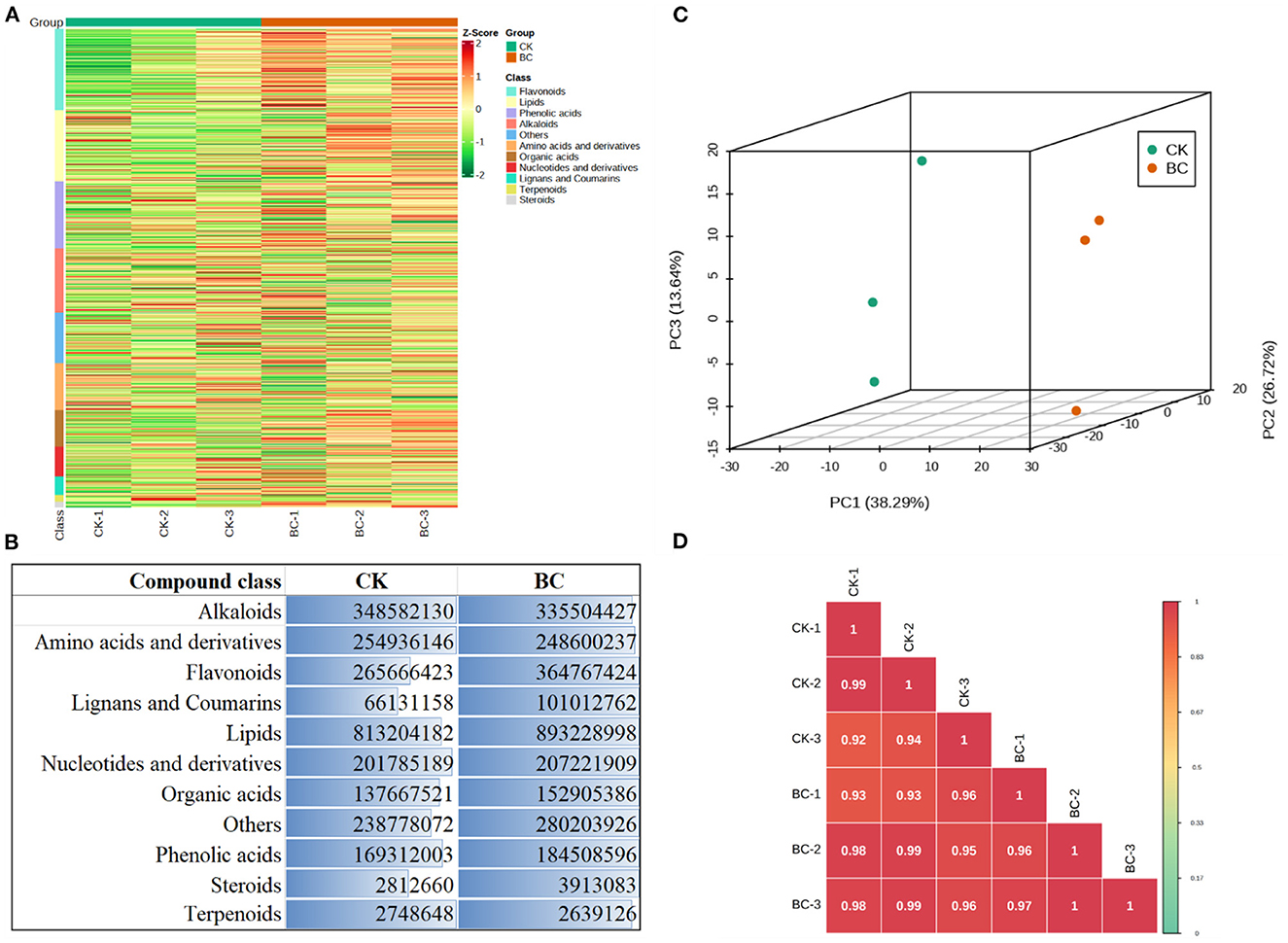
Figure 3. Metabolome analysis of BC (30:1 v/v coconut bran and bamboo charcoal) and CK (100% coconut bran) leaves. (A) Heatmap of the relative intensities of the detected metabolites in CK vs. BC, (B) Sum of accumulated contents of metabolite classes in CK and BC, (C) Principal component analysis, and (D) Pearson's Correlation Coefficient based on the relative metabolite intensities in CK vs. BC.
3.4. Differential metabolome profiles of tomato leaves grown in CK and BC
Based on the screening criteria i.e.., VIP ≥ 1 and |Log2 FC| ≥ 1.0, 39 metabolites were differentially accumulated between BC and CK. These metabolites were classified as flavonoids, amino acids and derivatives, alkaloids, lipids, nucleotides and derivatives, terpenoids, and steroids (Figure 4A). Six metabolites (Dihydrokaempferol-3-O-glucoside, Querce-tin-3-O-glucosyl(1 → 4)rhamnoside-7-O-rutinoside, Querce-tin-3-O-(6"-O-p-coumaroyl)sophoroside-7-O-rhamnoside, p-Coumaroyleuscaphic acid, Dihy-drokaempferol-7-O-glucoside, and Neotigogenin-glucose-glucose-glucose-glucoside) were exclusively detected in BC. In addition to these six compounds, four other compounds belonging to phenolic acid (1) and flavonoids (3) were highly up-accumulated in BC as compared to CK (Figure 4B). Most of the other up-accumulated metabolites in BC were classified as flavonoids, terpenoids, phenolic acids, organic acids, and steroidal saponins (Supplementary Table 3). These observations indicate that BC leaves have higher flavonoid, terpenoid, organic acid, and phenolic acid contents than CK, therefore, growing tomato in 30:1 v/v coconut bran and bamboo charcoal induces their increased biosynthesis.
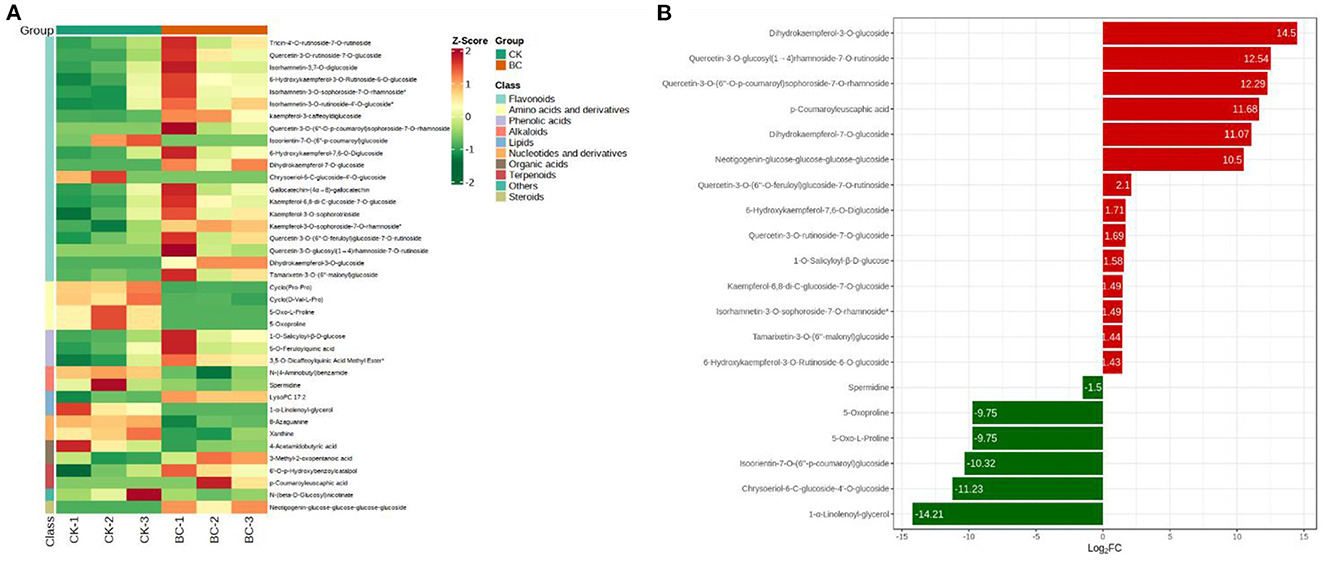
Figure 4. Differential metabolome profile of tomato seedlings grown in CK (100% coconut bran) and BC (30:1 v/v coconut bran and bamboo charcoal). (A) Heatmap of the z-scores and (B) top-10 up- and down accumulated metabolites in CK vs. BC.
On the contrary, the top-10 most accumulated compounds in CK were lipids (1), flavonoids (2), amino acids and derivatives (4), nucleotides and derivatives (2), and alkaloids (1) (Figure 4B). Taken together, higher accumulation of flavonoids, organic acids (3-Methyl-2-oxopentanoic acid), phenolic acids, steroids (Neotigogenin-glucose-glucose-glucose-glucoside), and terpenoids in BC can be related with the decreased survival rate of T. absoluta.
3.5. Transcriptome response of tomato seedlings grown in bamboo charcoal
3.5.1. Summary of transcriptome sequencing
Sequencing of six tomato leaf cDNA libraries resulted in 43.98 to 53.47 million raw reads. After filtering, 42.08–52.15 million clear reads (41.38 Gb clean data) were obtained. The Q20%, Q30%, GC content (%), and error rate (%) were 98.1, 94.227, 42.901, and 0.025%, respectively (Supplementary Table 4). Gene expression was computed as FPKM (Supplementary Figure 1A). The FPKM-based PCA analysis showed that PC1 and PC2 explained 28.41 and 23.98% variation, respectively (Supplementary Figure 1B). The PCC between the replicates of the same treatment and/or control was > 0.95, indicating the reliability of the sampling (Supplementary Figure 1C). A total of 26,046 transcripts were expressed, which could be annotated in GO, KEGG, KOG, NR, Pfam, and SwissProt. The datasets of the transcriptomes from our study were uploaded to NCBI Sequence Read Archive with accession number PRJNA898145.
3.5.2. Differential gene expression profiles of tomato leaves (CK vs. BC)
A total of 224 transcripts were differentially expressed between CK and BC; 144 were downregulated, while 80 were upregulated in BC. These transcripts were enriched in environmental information processing (ABC transporters, MAPK signaling, and plant hormone signal transduction), metabolism, and organismal systems (circadian rhythm and plant-pathogen interaction) (Figures 5A, B). According to GO classification, the highest number of DEGs were related to cellular components (cellular anatomical entity), followed by molecular processes (cellular processes and metabolic processes), and molecular function (catalytic activity and binding) (Figure 5C). The qRT-PCR analysis of 15 randomly selected genes indicated similar transcript levels as of RNA-seq results (Supplementary Figure 2).
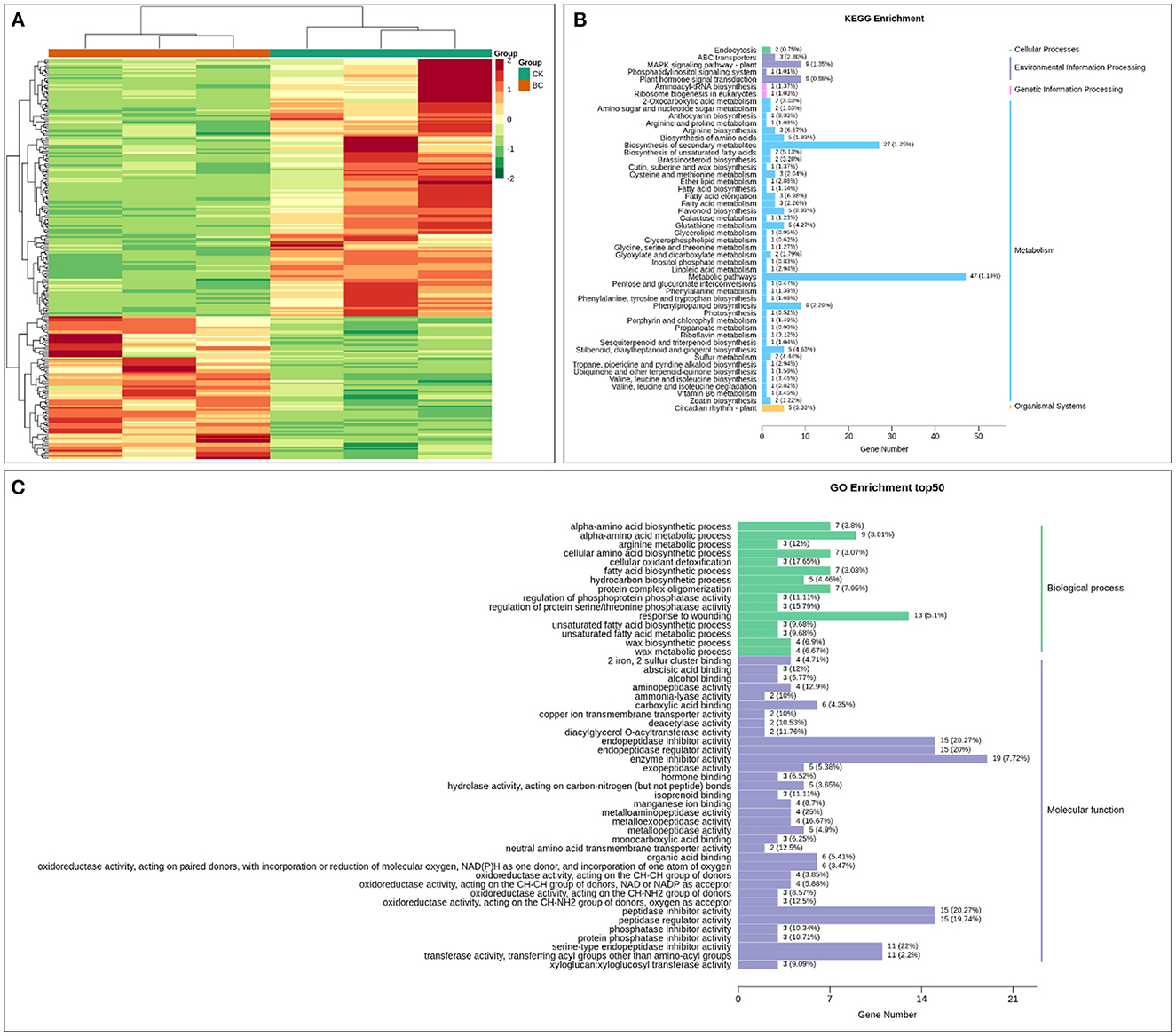
Figure 5. (A) Heatmap of FPKM values of the differentially expressed genes in CK vs. BC. Enrichment of the differentially expressed genes in CK vs. BC in (B) KEGG and (C) GO pathways.
3.5.2.1. Highly up and downregulated genes in BC-grown tomato seedlings
The most upregulated gene in BC was RESPONSE TO LOW SULFUR 2 (LSU2)/EN-HANCED DE-ETIOLATION 6 (Solyc03g096780.1), followed by LSU3 (Solyc03g096770.1), L-ornithine N5-acetyltransferase (Solyc00g272810.1), acyl-lipid Delta6-acetylenase/acyl-lipid (9-3)-desaturase (Solyc08g063090.2), and Probable serine/threonine-protein kinase PBL2 (novel.1843). Whereas, multicystatin (novel.2673), wound-induced proteinase inhibitor (Solyc09g089500.3, Solyc09g089540.3, and Solyc09g083445.1), proteinase inhibitor I-B-like (Solyc09g089530.3), leucyl aminopeptidase (Solyc12g010030.2 and novel.2698), serine protease inhibitor 5-like (Solyc03g098760.2), were downregulated in BC as compared to CK (Supplementary Table 4).
3.5.2.2. Changes in the expressions of MAPK and plant hormone signaling-related genes
Three LRR receptor-like serine/threonine-protein kinase ERECTA (Solyc03g112580.3, Solyc05g015150.3, and Solyc03g007050.3), and abscisic acid receptor PYR/PYL (Solyc06g050500.2) enriched in MAPK signaling pathway—plant were upregulated in BC as compared to CK. Whereas, a protein phosphatase 2C (Solyc03g096670.3), a WRKY22 (Solyc03g007380.2), and a TF MYC2 (Solyc10g009270.3) were downregulated in BC as compared to CK. Other than MAPK signaling, we also observed that phytohormone signaling-related genes were also differentially expressed. Of these, protein brassinosteroid insensitive 1 (BRI1, Solyc00g110870.3) and a DELLA protein (Solyc07g065270.1) were up-regulated in BC as compared to CK, whereas, others including xyloglu-can:xyloglucosyl transferase TCH4 and two gibberellin receptor GID1 were downregulated in BC (Supplementary Table 4). These observations indicate that gibberellin, ABA, brassinosteroid, and salicylic acid signaling play roles in better performance of the BC-grown tomato seedlings as compared to CK.
By removing the log2 foldchange screening criteria, overall, there were 705 genes associated with phytohormone signaling. All these showed variable gene expressions. However, interesting observations were that the brassinosteroid insensitive 1-associated receptor kinase 1 (BAK1) and most of the protein BRI1 were upregulated in BC as compared to CK. Similarly, the MAPKKK17/18 and MAPKK ANP1 transcripts were upregulated in BC as compared to CK (Supplementary Table 5).
3.5.2.3. Changes in the expressions of flavonoid, terpenoid, sugar-related genes
Three genes i.e., nerolidol synthase (Solyc10g005400.3), anthocyanidin 3-O-glucoside 2”'-O-xylosyltransferase (Solyc04g079050.2), and tropinone reductase 1 (Solyc06g083470.3) were upregulated in BC as compared to CK, whereas several other genes enriched in phenylpropanoid biosynthesis, flavonoid biosynthesis, and stilbenoid, diarylheptanoid, and gingerol biosynthesis were downregulated in BC as compared to CK. To further find related genes, we searched the GO terms with annotation as “terpenoids.” This resulted in the identification of five genes; three of which were upregulated in BC as compared to CK including cytochrome P450 family 76 subfamily A (Solyc09g098030.3), solute carrier family 15 (pep-tide/histidine transporter), member 44624 (Solyc03g113250.3), and a typhasterol/6-deoxotyphasterol 2alpha-hydroxylase (Solyc00g170200.1). Whereas, two genes were downregulated in BC as compared to CK; cis-zeatin O-glucosyltransferase (Solyc11g007490.2) and strigolactone esterase (Solyc00g170200.1). The GO terms associated with “polysaccharides” included the basic endochitinase B, beta-amylase, and subtilisin-like protease SBT1.9. These transcripts were downregulated in BC as compared to CK, whereas, xyloglucan:xyloglucosyl transferases, pectinesterase, and a Subtilisin-like protease SBT3 were upregulated in BC as compared to CK (Supplementary Table 4).
We also searched for genes involved in flavonoid biosynthesis regardless of DEG filtering criteria and found 28 transcripts related to flavonoid biosynthesis e.g., chalcone synthase, flavonoid 3′-monooxygenase, shikimate O-hroxycinnamoyltransferase, flavanone 4-reductase, and flavonoid 3′,5′-hydroxylase had higher expressions in BC as compared to CK. These changes are consistent with the observed higher flavonoid accumulation in BC as compared to CK. Moreover, 88 genes related to terpenoid biosynthesis or terpenoid backbone biosynthesis showed higher expressions in BC as compared to CK. Notably, we observed higher expressions of genes annotated as 8-dydroxygeraniol dehydrogenase, (-)-germacrene D synthase, premnaspirodiene oxygenase, (3S,6E)-nerolidol synthase, geranylgeranyl diphosphate synthase, type II, geranylgeranyltransferase type-1 subunit alpha, 4-coumarate-CoA ligase, and squalene monooxygenase in BC as compared to CK. These changes are also consistent with the metabolome profiles of BC and CK. Similarly, 91 genes related to starch and sucrose biosynthesis had higher expressions in BC as compared to CK. Most importantly, we observed the higher expression of starch synthases, trehalose 6-phosphate, endoglucanases, glucan endo-1,3-beta-glucosidases, and trehalose 6-phosphate synthase/phosphatase. These expressions are consistent with the higher accumulation of saccharides in BC as compared to CK (Supplementary Table 5).
Taken together, the transcriptome data confirms that metabolomic profiles are related to flavonoids, terpenoids, and saccharides in BC.
3.5.2.4. Changes in expression of fatty acid and cutin, suberine, and wax-related genes
Twelve DEGs were enriched in fatty acid-related pathways i.e., fatty acid elongation, fatty acid biosynthesis, biosynthesis of unsaturated fatty acids, fatty acid metabolism, and cutin, suberin, and wax biosynthesis. Only four genes associated with these pathways were upregulated. Of these, the 3-ketoacyl-CoA synthase (Solyc05g009270.3), acyl-lipid Delta6-acetylenase/acyl-lipid (9-3)-desaturase (Solyc08g063090.2), solute carrier family 15 (peptide/histidine transporter), member 44624 (Solyc01g096880.3), and the anthocyanin 3-O-glucoside 2-oxylosytransferase were up-regulated in BC as compared to CK (Supplementary Table 4).
Further exploration of genes with fatty acid biosynthesis related annotations showed that 32 transcripts were increasingly expressed in BC as compared to CK. These include 3-oxoacyl-[acyl-carrier protein] reductase, long-chain acyl-CoA synthetase, acetyl-CoA carboxylase, acyl-[acyl-carrier-protein] desaturase, 3-oxoacyl-[acyl-carrier-protein] synthase II, and fat-ty acyl-ACP thioesterase B (Supplementary Table 5).
3.5.2.5. Differential expression of sulfur metabolism, glutathione metabolism, and solute carrier family genes in CK vs. BC
Two genes annotated as adenylyl-sulfate reductase (glutathione) enriched in sulfur metabolism were upregulated. Eight genes annotated as solute carrier family members were differentially ex-pressed. Of these, only solute carrier family 15 members (Solyc03g113250.3 and Solyc01g096880.3) were upregulated in BC, whereas all others including solute carrier family 31, 36, 39, and 50 members were downregulated in BC as compared to CK. Five genes [glutathione S-transferase (GST, Solyc06g069045.1) and four leucyl aminopeptidases (Solyc12g010020.2, Solyc12g010025.1, novel.2698, and Solyc12g010030.2)] were downregulated in BC as compared to CK. However, by considering genes with log2 foldchange lower than 1 and higher than −1, we observed that multiple GST transcripts, L-ascorbate peroxidases, glutathione dehydrogenase/transferases, and glucose-6-phosphate 1-dehydrogenases that were associated with glutathione metabolism had higher FPKM values in BC as compared to CK (Supplementary Tables 4, 5).
Regarding the changes in the expression of transcription factors (TFs), of the 26,046 expressed genes, 1,850 were annotated as TFs, which were classified as 91 TF families. Interestingly, 789 TFs showed upregulation; the most upregulated TFs belonged to AP2/ERF, LOB, bHLH, GNAT, SET, SWI, B3, and WRKY families (Supplementary Table 6).
4. Discussion
Biochar amendment in soil/substrate has shown positive effects on plant defense against different pathogens, however, limited data is available for insect pests (De Tender et al., 2021). The spread of T. absoluta in mainland China (Zhang et al., 2021) calls for the exploration of possible strategies to reduce tomato crop losses. Several studies have also reported positive effects of bamboo charcoal on plant growth and development. Our results that BC (30:1) caused a significant increase in stem thickness and plant height indicate that the usage of bamboo charcoal in a specific v/v ratio could be beneficial for plant growth and development. This observation is consistent with earlier reports that different types and ratios of biochar have shown variations in their effects on plants (Chrysargyris et al., 2020). Our results are also consistent with the earlier reports that incorporation of bamboo charcoal in growth media increased plant height in tea (Gao et al., 2012), stem diameter in Sapium sebiferum (L.) and some temperate broad-leaved trees (Chen et al., 2021), and chlorophyll content in turfgrass (Hua et al., 2012). Thus, we conclude that bamboo charcoal has growth promoting effects on tomato seedlings. In addition, the results that the survival of T. absoluta significantly decreased in BC as compared to CK indicate that its addition increases tomato plants' resistance against T. absoluta. Previously, soil amendment with biochar has shown to have the ability to increase plant's resistance to herbivory (Waqas et al., 2018) and M. incognita infestation (Arshad et al., 2020, 2021). The metabolomic and transcriptomic signatures that are related to such changes in tolerance against T. absoluta are discussed below.
Biochar exerts beneficial effects on plant growth and development. Such effects also cause changes in the biosynthesis of plant primary and secondary metabolites. Earlier studies have shown that the application of biochar causes metabolic shift in different plant organs (Sun et al., 2017). Particularly, biochar application caused significant increase in flavonoid, total sugars, and enzyme activities in basil, and resulted in higher plant height, leaf length, leaf number, and yield (Jabborova et al., 2021). Our data also suggest that the use of bamboo charcoal can result in a higher accumulation of flavonoids, phenolic acids, steroids, and organic acids in tomato leaf (Figure 4). Flavonoids (Lattanzio et al., 2000), organic acids (Morgunov et al., 2017), steroids (Janson et al., 2009) have been implicated in both the plant growth and survival as well as defense against insect pests. Therefore, the higher content of these secondary metabolites is possibly related to the decreased T. absoluata survival rate. One of the inducible chemical defense responses in plants against invading insect pests and herbivores is the synthesis of a wide range of secondary metabolites including flavonoids, terpenoids, and alkaloids (D'Esposito et al., 2021). Our data also indicated a higher accumulation of flavanonols, flavonols, triterpenes, steroidal saponins, phenolic acids, flavones, monoterpenoids, and phenolic acids (Supplementary Table 3). These observations are consistent with the report that biosynthesis of defensive secondary metabolites increased in a partially resistant cherry tomato in response to T. absoluta (D'Esposito et al., 2021). Therefore, from the literature cited and our combined metabolome and transcriptome data, we can conclude that the biosynthesis of these metabolites is a defense response in tomato against T. absoluta and growing in bamboo charcoal can enhance the accumulation of these metabolites in tomatoes.
To further understand the changes in total relative intensities of these metabolite classes in BC, we used the transcriptome sequencing approach. The upregulation of nerolidol synthase transcripts in BC is consistent with earlier report that it is an herbivore-inducible terpene synthase gene in maize (Degenhardt and Gershenzon, 2000). Similar to this report, the higher expression level of the nerolidol synthase transcripts in BC as compared to CK might be a reason for the observed reduced T. absoluta survival rate (Figure 2). Furthermore, the increased expression of other terpene biosynthesis related genes, such as 8-dydroxygeraniol dehydrogenase, (-)-germacrene D synthase, premnaspirodiene oxygenase, (3S,6E)-nerolidol synthase, geranylgeranyl diphosphate synthase, type II, geranylgeranyltransferase type-1 subunit alpha, 4-coumarate-CoA ligase, and squalene monooxygenase, is also consistent with higher terpenoid accumulation in BC (Supplementary Table 3). Thus, it is understandable that bamboo charcoal induces higher expression of terpenoid biosynthesis related genes that lead toward higher terpenoid accumulation and reduced T. absoluta survival on tomato leaves. Similarly, the higher flavonoids content and respective upregulation of flavonoid-biosynthesis genes including chalcone synthase, flavonoid 3'-monooxygenase, shikimate O-hydroxycinnamoyltransferase, flavanone 4-reductase, and flavonoid 3′,5′-hydroxylase (Petrussa et al., 2013), indicate that bamboo charcoal induces higher expression in flavonoids. This higher flavonoid biosynthesis in turn enables tomato plants to tolerate T. absoluta as they play defensive roles in other plant species against insect pests (Lattanzio et al., 2000). Moreover, our data also indicated that bamboo charcoal induces higher expression of starch and sucrose biosynthesis, which is consistent with the earlier reports that biochar amendment can improve the total sugar contents in plants (Sun et al., 2017; Jabborova et al., 2021).
The higher expression of LSU transcripts indicates that bamboo charcoal induces stress-related hub proteins. The LSU proteins have been identified as immune-related hubs and play undefined roles against pathogens (Mukhtar et al., 2011). Furthermore, LSU proteins are involved in various protein-protein interactions with the proteins functioning at different molecular levels (Mukhtar et al., 2011). For example, reduced expressions of LSU genes in Arabidopsis resulted in increased disease (Pseudomonas syringae infection) susceptibility (Garcia-Molina et al., 2017). Therefore, it is possible that LSU proteins perform similar functions in tomato against T. absoluta, and that their expressions can be induced by growing tomato in bamboo charcoal. The upregulation of genes such as L-ornithine N5-acetyltransferase, acyl-lipid delta6-acetylenase, and probable serine/threonine-protein kinase PBL2 in response to bamboo charcoal amendment indicate that tomato plants may resist T. absoluta by involving ornithine metabolism, fatty acid biosynthesis, and PAMP-triggered immunity, respectively.
Phytohormones (ethylene, gibberellin, auxin, brassinosteroid, jasmonates, and salicylic acid) are involved in plant defense responses against insect attack and herbivory (Howe and Jander, 2008). Changes in their contents can activate signal transduction upon insect feeding or plant tissue wounding (Koch et al., 2016). The use of biochar has been shown to increase plant growth-mediated hormones (Farhangi-Abriz and Torabian, 2018). Our results that the expressions of BRI1, BAK1, DELLA, TCH4, MYC2, PYR/PYL, PP2C, and GID1 genes changed in tomato seedlings indicate that bamboo charcoal activates GA, ABA, brassinosteroid, and JA signaling. However, since we did not detect differential changes in phytohormone (or related metabolites), therefore, further research is needed to understand how bamboo charcoal may change the phytohormone profile of the tomato seedlings. Moreover, the increase in expression of genes enriched in MAPK signaling pathway such as ERECTA also suggests that bamboo charcoal can initiate signaling cascades in tomato leaves that help it maintain developmental integrity in biotic stress conditions. This proposition is based on the known role of ERECTA in maintaining plant phenotype and developmental integrity under different stress and microenvironmental scenarios (Douglas et al., 2002). In addition to phytohormone signaling, bamboo charcoal also regulated the expression of oxidative stress related genes such as GST, which plays roles against biotic and abiotic stresses in plants (Estévez and Hernández, 2020). Finally, our results indicate that bamboo charcoal activates the expression of different classes of TFs in tomato leaves to help the plant survive under attack. Earlier studies on different types of biochar have indicated that biochar-mediated plant growth under biotic stress is accompanied by the regulation of the expression of various TFs (Jaiswal et al., 2020). Different classes of TFs have been implicated in resistance against insect-pests e.g., ERF TFs enable tobacco plants to resist Myzus periscae and Spodoptera litura attack (Wu et al., 2020). Similarly, brassinosteroid related TF BIL1/BZR1 have been reported to help plant resist against insect feeding. Therefore, it can be understood that bamboo charcoal induced expression changes in a range of TF families, which in turn enabled tomato plants grow better and resist T. absoluta infestation.
Data availability statement
The datasets presented in this study can be found in online repositories. The names of the repository/repositories and accession number(s) can be found at: https://www.ncbi.nlm.nih.gov/bioproject/PRJNA898145.
Author contributions
LC and XL: conceptualization. QZ and AJ: methodology. TC and JW: software. JZ, JH, and ZZ: validation. MH: formal analysis. SZ: investigation. XR: resources. WD: data curation. YL: writing—original draft preparation. LC and XL: writing—review and editing. LC: visualization. YH: supervision. XL: project administration. XL and YL: funding acquisition. All authors have read and agreed to the published version of the manuscript.
Funding
This research was funded by the Pioneer and Leading Goose R&D Program of Zhejiang (Grant No. 2022C04016); the Joint Funds of the National Natural Science Foundation of China (Grant No. U22A20489); the National Natural Science Foundation of China (Grant No. 32272524); the China Postdoctoral Science Foundation (Grant No. 2021M702906); the Primary Research and Development Plan of Lishui (Grant No. 2020ZDYF02); the Zhejiang Provincial Natural Science Foundation of China under (Grant No. LQ22C140004).
Acknowledgments
We would like to thank Prof. Pengjun Zhang from Ningbo University for sharing the tomato seeds.
Conflict of interest
The authors declare that the research was conducted in the absence of any commercial or financial relationships that could be construed as a potential conflict of interest.
Publisher's note
All claims expressed in this article are solely those of the authors and do not necessarily represent those of their affiliated organizations, or those of the publisher, the editors and the reviewers. Any product that may be evaluated in this article, or claim that may be made by its manufacturer, is not guaranteed or endorsed by the publisher.
Supplementary material
The Supplementary Material for this article can be found online at: https://www.frontiersin.org/articles/10.3389/fsufs.2023.1101151/full#supplementary-material
Supplementary Figure 1. (A) Overall distribution of gene expression, (B) Principal component analysis, and (C) Pearson's correlation coefficient based on FPKM values of the differentially expressed genes be-tween CK and BC tomato leaves.
Supplementary Figure 2. qRT-PCR results of 15 selected genes in tomato. *Indicates significant differences be-tween the CK and BC (*p < 0.05; **p < 0.01; ***p < 0.001).
Supplementary Table 1. List of primers used for qRT-PCR analysis.
Supplementary Table 2. (A) Morphological performance of the tomato plants grown in three different coconut bran and bamboo charcoal combinations. (B) Probability of survival of T. absoluta (raw data).
Supplementary Table 3. List of differentially accumulated metabolites in tomato leaves grown in CK (100% coconut bran) and BC (30:1 v/v coconut bran and bamboo charcoal).
Supplementary Table 4. List of differentially expressed genes in tomato leaves grown in CK (100% coconut bran) and BC (30:1 v/v coconut bran and bamboo charcoal).
Supplementary Table 5. List of genes associated with different KEGG pathways that showed changes in the ex-pression between leaves grown in CK (100% coconut bran) and BC (30:1 v/v coconut bran and bamboo charcoal).
Supplementary Table 6. Expression changes in transcription factors between CK and BC tomato leaves grown in CK (100% coconut bran) and BC (30:1 v/v coconut bran and bamboo charcoal).
References
Arshad, U., Azeem, F., Mustafa, G., Bakhsh, A., Toktay, H., McGiffen, M., et al. (2021). Combined application of biochar and biocontrol agents enhances plant growth and activates resistance against Meloidogyne incognita in tomato. Gesunde Pflanzen 73, 591–601. doi: 10.1007/s10343-021-00580-4
Arshad, U., Naveed, M., Javed, N., Gogi, M. D., and Ali, M. A. (2020). Biochar application from different feedstocks enhances plant growth and resistance against Meloidogyne incognita in tomato. Int. J. Agric. Biol. 24, 961–968. doi: 10.17957/IJAB/15.1522
Biondi, A., Guedes, R. N. C., Wan, F. H., and Desneux, N. (2018). Ecology, worldwide spread, and management of the invasive South American tomato pinworm, Tuta absoluta: past, present, and future. Ann. Rev. Entomol. 63, 239–258. doi: 10.1146/annurev-ento-031616-034933
Campos, M. R., Biondi, A., Adiga, A., Guedes, R. N. C., and Desneux, N. (2017). From the Western Palaearctic region to beyond: Tuta absoluta 10 years after invading Europe. J. Pest Sci. 90, 787–796. doi: 10.1007/s10340-017-0867-7
Chen, H., Chen, C., and Yu, F. Y. (2021). Biochar improves root growth of Sapium sebiferum (L.) Roxb. container seedlings. Agronomy 11, 1242. doi: 10.3390/agronomy11061242
Chen, Y., Li, R., Li, B. P., and Meng, L. (2019). Biochar applications decrease reproductive potential of the english grain aphid Sitobion avenae and upregulate defense-related gene expression. Pest Manage. Sci. 75, 1310–1316. doi: 10.1002/ps.5245
Chrysargyris, A., Prasad, M., Kavanagh, A., and Tzortzakis, N. (2020). Biochar type, ratio, and nutrient levels in growing media affects seedling production and plant performance. Agronomy 10, 1421. doi: 10.3390/agronomy10091421
De Tender, C., Vandecasteele, B., Verstraeten, B., Ommeslag, S., Kyndt, T., and Debode, J. (2021). Biochar-enhanced resistance to Botrytis cinerea in strawberry fruits (but not leaves) is associated with changes in the rhizosphere microbiome. Front. Plant Sci. 12, 700479. doi: 10.3389/fpls.2021.700479
Degenhardt, J., and Gershenzon, J. (2000). Demonstration and characterization of (E)-nerolidol synthase from maize: a herbivore-inducible terpene synthase participating in (3E)-4, 8-dimethyl-1, 3, 7-nonatriene biosynthesis. Planta 210, 815–822. doi: 10.1007/s004250050684
Desneux, N., Han, P., Mansour, R., Arnó, J., Brévault, T., Campos, M. R., et al. (2022). Integrated pest management of Tuta absoluta: practical implementations across different world regions. J. Pest Sci. 95, 17–39. doi: 10.1007/s10340-021-01442-8
D'Esposito, D., Manzo, D., Ricciardi, A., Garonna, A. P., De Natale, A., Frusciante, L., et al. (2021). To-mato transcriptomic response to Tuta absoluta infestation. BMC Plant Biol. 21, 358. doi: 10.1186/s12870-021-03129-9
Ding, T. B., Li, J., Chen, E. H., Niu, J. Z., and Chu, D. (2019). Transcriptome profiling of the whitefly Bemisia tabaci MED in response to single infection of tomato yellow leaf curl virus, tomato chlorosis virus, and their co-infection. Front. Physiol. 10:302. doi: 10.3389/fphys.2019.00302
Douglas, S. J., Chuck, G., Dengler, R. E., Pelecanda, L., and Riggs, C. D. (2002). KNAT1 and ERECTA regulate inflorescence architecture in Arabidopsis. Plant Cell 14, 547–558. doi: 10.1105/tpc.010391
Estévez, I. H., and Hernández, M. R. (2020). Plant glutathione S-transferases: an overview. Plant Gene 23, 100233. doi: 10.1016/j.plgene.2020.100233
Farhangi-Abriz, S., and Torabian, S. (2018). Biochar increased plant growth-promoting hormones and helped to alleviates salt stress in common bean seedlings. J. Plant Growth Regul. 37, 591–601. doi: 10.1007/s00344-017-9756-9
Gao, H. J., Zhang, Z. Z., and Wan, X. C. (2012). Influences of charcoal and bamboo charcoal amendment on soil-fluoride fractions and bioaccumulation of fluoride in tea plants. Environ. Geochem. Health 34, 551–562. doi: 10.1007/s10653-012-9459-x
Garcia-Molina, A., Altmann, M., Alkofer, A., Epple, P. M., Dangl, J. L., and Falter-Braun, P. (2017). LSU network hubs integrate abiotic and biotic stress responses via interaction with the superoxide dismutase FSD2. J. Exp. Bot. 68, 1185–1197. doi: 10.1093/jxb/erw498
Ghimire, N. P., Kandel, M., Aryal, M., and Bhattarai, D. (2017). Assessment of tomato consumption and demand in Nepal. J. Agric. Environ. 18, 83–94. doi: 10.3126/aej.v18i0.19893
Guedes, R., Roditakis, E., Campos, M., Haddi, K., Bielza, P., Siqueira, H., et al. (2019). Insecticide resistance in the tomato pinworm Tuta absoluta: patterns, spread, mechanisms, management and outlook. J. Pest Sci. 92, 1329–1342. doi: 10.1007/s10340-019-01086-9
Han, P., Bayram, Y., Shaltiel-Harpaz, L., Sohrabi, F., Saji, A., Esenali, U. T., et al. (2019a). Tuta absoluta continues to disperse in Asia: damage, ongoing management and future challenges. J. Pest Sci. 92, 1317–1327. doi: 10.1007/s10340-018-1062-1
Han, P., Desneux, N., Becker, C., Larbat, R., Le Bot, J., Zhang, J., et al. (2019b). Bottom-up effects of irrigation, fertilization and plant resistance on Tuta absoluta: implications for integrated pest management. J. Pest Sci. 92, 1359–1370. doi: 10.1007/s10340-018-1066-x
Han, P., Lavoir, A. V., Rodriguez-Saona, C., and Desneux, N. (2022). Bottom-up forces in agroecosystems and their potential impact on arthropod pest management. Ann. Rev. Entomol. 67:239–259. doi: 10.1146/annurev-ento-060121-060505
Howe, G. A., and Jander, G. (2008). Plant immunity to insect herbivores. Ann. Rev. Plant Biol. 59, 41–66. doi: 10.1146/annurev.arplant.59.032607.092825
Hua, L., Chen, Y., and Wu, W. (2012). Impacts upon soil quality and plant growth of bamboo charcoal addition to composted sludge. Environ. Technol. 33, 61–68. doi: 10.1080/09593330.2010.549845
Jabborova, D., Ma, H., Bellingrath-Kimura, S. D., and Wirth, S. (2021). Impacts of biochar on basil (Ocimum basilicum) growth, root morphological traits, plant biochemical and physiological properties and soil enzymatic activities. Sci. Horticult. 290, 110518. doi: 10.1016/j.scienta.2021.110518
Jaiswal, A. K., Alkan, N., Elad, Y., Sela, N., Philosoph, A. M., Graber, E. R., et al. (2020). Molecular insights into biochar-mediated plant growth promotion and systemic resistance in tomato against Fusarium crown and root rot disease. Sci. Rep. 10, 13934. doi: 10.1038/s41598-020-70882-6
Janson, E. M., Grebenok, R. J., Behmer, S. T., and Abbot, P. (2009). Same host-plant, different sterols: variation in sterol metabolism in an insect herbivore community. J. Chem. Ecol. 35, 1309–1319. doi: 10.1007/s10886-009-9713-6
Kawakami, M., Karato, T., Takenaka, T., and Yokoyama, S. (2005). Structure analysis of coke, wood charcoal and bamboo charcoal by Raman spectroscopy and their reaction rate with CO2. ISIJ Int. 45, 1027–1034. doi: 10.2355/isijinternational.45.1027
Koch, K. G., Chapman, K., Louis, J., Heng-Moss, T., and Sarath, G. (2016). Plant tolerance: a unique approach to control hemipteran pests. Fronti. Plant Sci. 7, 1363. doi: 10.3389/fpls.2016.01363
Lattanzio, V., Arpaia, S., Cardinali, A., Di Venere, D., and Linsalata, V. (2000). Role of endogenous flavonoids in resistance mechanism of Vigna to aphids. J. Agric. Food Chem. 48, 5316–5320. doi: 10.1021/jf000229y
Li, Y. H., Lv, P., Mi, J. Z., Zhao, B. P., and Liu, J. H. (2022). Integrative transcriptome and metabolome analyses of the interaction of oat–oat stem rust. Agronomy 12, 2353. doi: 10.3390/agronomy12102353
Liang, Q., Song, K., Lu, M., Dai, T., Yang, J., Wan, J., et al. (2022). Transcriptome and metabolome analyses reveal the involvement of multiple pathways in flowering intensity in mango. Front. Plant Sci. 13, 933923. doi: 10.3389/fpls.2022.933923
Mansour, R., Brévault, T., Chailleux, A., Cherif, A., Grissa-Lebdi, K., Haddi, K., et al. (2018). Occurrence, biology, natural enemies and management of Tuta absoluta in Africa. Entomol. Gen. 38, 83–112. doi: 10.1127/entomologia/2018/0749
Morgunov, I. G., Kamzolova, S. V., Dedyukhina, E. G., Chistyakova, T. I., Lunina, J. N., Mironov, A. A., et al. (2017). Application of organic acids for plant protection against phytopathogens. Appl. Microbiol. Biotechnol. 101, 921–932. doi: 10.1007/s00253-016-8067-6
Mukhtar, M. S., Carvunis, A.-R., Dreze, M., Epple, P., Steinbrenner, J., Moore, J., et al. (2011). Independently evolved virulence effectors converge onto hubs in a plant immune system network. Science 333, 596–601. doi: 10.1126/science.1203659
Petrussa, E., Braidot, E., Zancani, M., Peresson, C., Bertolini, A., Patui, S., et al. (2013). Plant flavonoids—biosynthesis, transport and involvement in stress responses. Int. J. Mol. Sci. 14, 14950–14973. doi: 10.3390/ijms140714950
Poveda, J., Martínez-Gómez, Á., Fenoll, C., and Escobar, C. (2021). The use of biochar for plant pathogen control. Phytopatholog 111, 1490–1499. doi: 10.1094/PHYTO-06-20-0248-RVW
Silva, D. B., Bueno, V. H., Montes, F. C., and van Lenteren, J. C. (2016). Population growth of three mirid predatory bugs feeding on eggs and larvae of Tuta absoluta on tomato. BioControl 61, 545–553. doi: 10.1007/s10526-016-9736-1
Sun, C. X., Chen, X., Cao, M. M., Li, M. Q., and Zhang, Y. L. (2017). Growth and metabolic responses of maize roots to straw biochar application at different rates. Plant Soil 416, 487–502. doi: 10.1007/s11104-017-3229-6
Wang, P., Luo, Y. F., Huang, J. F., Gao, S. H., Zhu, G. P., Dang, Z. G., et al. (2020). The genome evolution and domestication of tropical fruit mango. Genome Biol. 21, 60. doi: 10.1186/s13059-020-01959-8
Wang, P., Maliang, H., Wang, C., and Ma, J. (2015). Bamboo charcoal byproducts as sources of new insecticide and acaricide. Indust. Crops Prod. 77, 575–581. doi: 10.1016/j.indcrop.2015.09.004
Wangari Nderitu, P., Jonsson, M., Arunga, E., Otieno, M., Jamleck Muturi, J., and Wafula, G. O. (2020). Combining host plant resistance, selective insecticides, and biological control agents for integrated management of Tuta absoluta. Adv. Agric. 2020, 623949. doi: 10.1155/2020/6239491
Waqas, M., Shahzad, R., Hamayun, M., Asaf, S., Khan, A. L., Kang, S. M., et al. (2018). Biochar amendment changes jasmonic acid levels in two rice varieties and alters their resistance to herbivory. PLoS ONE. 13, e0191296. doi: 10.1371/journal.pone.0191296
Wu, J., Gao, H., Zhu, X. W., and Li, D. F. (2020). An ERF transcription factor enhances plant resistance to Myzus persicae and Spodoptera litura. Biotechnol. Biotechnol. Equip. 34, 946–954. doi: 10.1080/13102818.2020.1813051
Yazdanpanah, S., Fathipour, Y., Riahi, E., and Zalucki, M. P. (2021). Mass production of Neoseiulus cucumeris (Acari: Phytoseiidae): an assessment of 50 generations reared on almond pollen. J. Econ. Entomol. 114, 2255–2263. doi: 10.1093/jee/toab163
Zappala, L., Biondi, A., Alma, A., Al-Jboory, I. J., Arno, J., Bayram, A., et al. (2013). Natural enemies of the South American moth, Tuta absoluta, in Europe, North Africa and Middle East, and their potential use in pest control strategies. J. Pest Sci. 86, 635–647. doi: 10.1007/s10340-013-0531-9
Zhang, G. F., Wang, Y. S., Gao, Y. H., Liu, W. X., Zhang, R., Fu, W. J., et al. (2020a). First report of the South American tomato leaf miner, Tuta absoluta (Meyrick), in China. J. Integrat. Agric. 19, 1912–1917. doi: 10.1016/S2095-3119(20)63165-3
Zhang, G. F., Xian, X. Q., Zhang, Y. B., Liu, W. X., Liu, H., Feng, X. D., et al. (2021). Outbreak of the South American tomato leaf miner, Tuta absoluta, in the Chinese mainland: Geographic and potential host range expansion. Pest Manage. Sci. 77, 5475–5488. doi: 10.1002/ps.6588
Zhang, G. F., Xian, X. Q., Zhang, Y. B., Zhang, R., Ma, D. Y., Liu, W. X., et al. (2020b). Warning of the dispersal of a newly invaded alien species, tomato leaf miner Tuta absoluta (Meyrick). China Plant Prot. 46, 281–286. doi: 10.16688/j.zwbh.2019061
Keywords: flavonoids, terpenoids, resistance to insect-pest, South American tomato pinworm, phytohormone signaling, LSU hub genes
Citation: Chen L, Li X, Wang J, Chen T, Zhang J, Zhu Q, Huang J, Zhang Z, Hafeez M, Zhou S, Ren X, Dong W, Jin A, Hou Y and Lu Y (2023) Bamboo charcoal mediated plant secondary metabolites biosynthesis in tomato against South American tomato pinworm (Tuta absoluta). Front. Sustain. Food Syst. 7:1101151. doi: 10.3389/fsufs.2023.1101151
Received: 17 November 2022; Accepted: 20 January 2023;
Published: 06 February 2023.
Edited by:
Wen Xie, Insititute of Vegetables and Flowers (CAAS), ChinaReviewed by:
Yibo Zhang, Institute of Plant Protection (CAAS), ChinaYong Liu, Hunan Academy of Agricultural Sciences (CAAS), China
Copyright © 2023 Chen, Li, Wang, Chen, Zhang, Zhu, Huang, Zhang, Hafeez, Zhou, Ren, Dong, Jin, Hou and Lu. This is an open-access article distributed under the terms of the Creative Commons Attribution License (CC BY). The use, distribution or reproduction in other forums is permitted, provided the original author(s) and the copyright owner(s) are credited and that the original publication in this journal is cited, in accordance with accepted academic practice. No use, distribution or reproduction is permitted which does not comply with these terms.
*Correspondence: Yaobin Lu, luybcn@163.com; Youming Hou,
ymhou@fafu.edu.cn
†These authors have contributed equally to this work