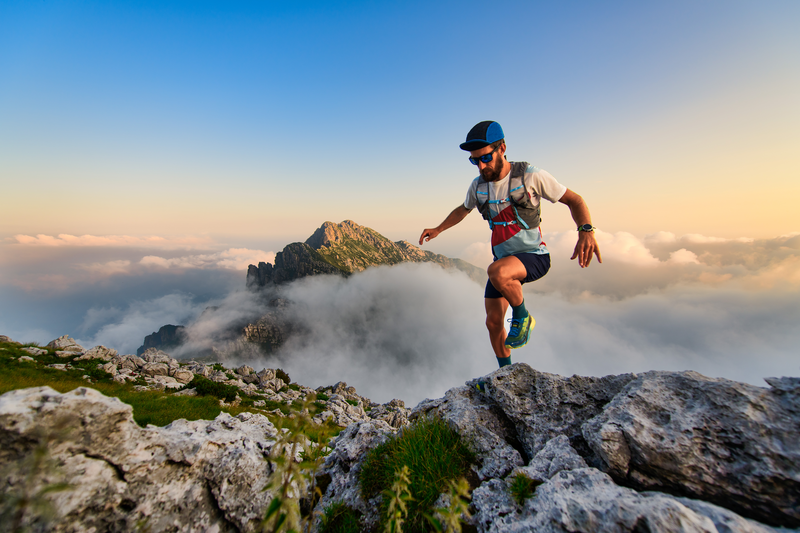
94% of researchers rate our articles as excellent or good
Learn more about the work of our research integrity team to safeguard the quality of each article we publish.
Find out more
ORIGINAL RESEARCH article
Front. Sustain. Food Syst. , 27 January 2023
Sec. Agroecology and Ecosystem Services
Volume 7 - 2023 | https://doi.org/10.3389/fsufs.2023.1097587
This article is part of the Research Topic Nitrogen Use To Improve Sustainable Yields in Agricultural Systems View all 11 articles
Multifunctional plant growth-promoting bioinoculants are used to enhance growth, harvest yields, and add economic value to agricultural crops. In this study, such bioinoculant, BC-II-20 (Pseudomonas sp.), was isolated from the rhizospheric soil of a medicinal plant Bergenia ciliata from the Garhwal Himalayas, Uttarakhand, India. After characterization, supplementation with Pseudomonas sp. was used to study growth stimulation in a commercially important medicinal plant, Andrographis paniculata (Kalmegh), and it depicted enhanced physiological growth parameters under controlled conditions. Bacterial seed priming and also supplementation led to early and increased germination and plants displayed better vegetative growth during the entire growth stages. Early initiation of flowers and the appearance of pods occurred in inoculated plants, ultimately leading to the reduction in the life cycle of the plant. At the time of harvesting, there was an increase in the physiological parameters such as shoot length (38%), root length (14%), fresh weight (57%), dry weight (60%), number of panicles, and root branching. Photosynthetic efficiency was also higher, and ultimately, overall plant growth was improved by bacterial inoculation. The eco-friendly and sustainable use of this bioinoculant will provide an alternative to harmful chemical fertilizers and has become increasingly important. In conclusion, we reported a promising bioinoculant having plant growth-promoting traits, which promotes growth and development in A. paniculata and may be applied to other plants also.
Medicinal plants have microbial communities with a genetically diverse population having multifunctional growth-promoting properties (Premalatha et al., 2021). Plant roots produce a range of organic substances that serve as food for microorganisms, enhancing beneficial microbial activity in the complex environment termed as rhizosphere (Egamberdieva and Teixeira da Silva, 2015). Plants and soil microbes interact, interrelate, and affect each other in the rhizosphere (Mhlongo et al., 2018). These rhizospheric microbes are plant growth-promoting rhizobacteria (PGPR), which stimulate plant growth and development. There are reports that PGPR supplementation leads to an enhancement in phytochemical content in medicinal plants (Egamberdieva and Teixeira da Silva, 2015) and also acts as potential biocontrol agents in many plant diseases (Sharf et al., 2021). These medicinal plants serve as raw materials for traditional herbal medicine. According to the estimates, traditional medical practices such as herbal remedies, indigenous therapies, and others are used in 88% of all countries (WHO, 2019).
B. ciliata belongs to the family Saxifragaceae and can be found at a height of 800–3,000 m in the temperate Himalayan regions. For centuries, it has been used as medicine for several ailments (Ahmad et al., 2018). Various diseases are treated with B. ciliata in the Himalayan region (Kour et al., 2021). Medicinal plants have a distinct microbiome, which produces distinct bioactive secondary metabolites such as flavonoids, terpenoids, glycosides, sterols, and saponins (Ferdosi et al., 2021). Thus, the PGPRs present in the rhizospheric soil associated with B. ciliata may prove beneficial for other medicinal plants also.
A. paniculata belongs to the plant family Acanthaceae and is also known as the “King of Bitters.” The leaves and stems of the plant are used as fresh and dried herbal medicine since ancient times and have active phytochemicals. The most medicinally active phytochemical is andrographolide. The plant has been widely used to treat jaundice, digestive disorders, and hepatoprotection as well as liver tonics, antipyretics, antithrombotics, blood purifiers, and febrifuges (Okhuarobo et al., 2014). The plant is revered as a miraculous remedy in tribal societies and ancient Siddha and Ayurvedic medical systems for a number of medicinal uses. It has been extensively cultivated in India and other South Asian countries (Kumar et al., 2011; Khan et al., 2015). As a powerful immune booster, this plant is in high demand (Premalatha et al., 2021). But the herbage yield of A. paniculata gets affected by indiscriminate harvesting from natural habitats and suffers the risk of drought (Kalariya et al., 2021). Thus, there is a need to increase the cultivation and growth and development of A. paniculata to get more biomass for increased phytometabolite content. Seed dormancy is also a problem during germination in A. paniculata and may be resolved by the application of plant growth-promoting rhizobacteria, as is important to promote vegetative growth where leaves are the important plant part for medicinal purposes (Premalatha et al., 2021).
As a result of intensive cultivation practices, chemical fertilizers are typically used extensively to increase medicinal crop yields and quality. Such agricultural practices may also negatively affect the growth of medicinal plants and their secondary metabolites apart from being expensive and environmentally harmful. Nowadays, environment-friendly, sustainable, and organic approaches are becoming increasingly popular for yield enhancement in medicinal plants (Yilmaz and Karik, 2022; Yuan et al., 2022). All of these are finally directed toward the fulfillment of sustainable development goals (SDGs) adopted by the United Nations.
Therefore, this study was primarily designed to isolate and characterize the rhizobacteria associated with B. ciliata for PGPR traits and to determine the effects of its supplementation. We investigated the effect of bioinoculant, i.e., Pseudomonas sp. on a medicinal plant, A. paniculata, for plant growth promotion under controlled conditions. The beneficial effects of this bioinoculant were observed in root development, shoot development, and early flowering, thereby proving its role as a potential biofertilizer.
Soil sampling was done from the rhizosphere of B. ciliata, a medicinal plant, from district Pauri Garhwal in Uttarakhand, India (30°09′30.5″N 78°51′14.8″E). The intact plant was carefully removed with a 15-cm slab of soil. For bacterial isolation, the soil clumps that were firmly associated with the roots were carefully preserved in sterile polyethylene bags. Bacterial isolation was done using the serial dilution method. Rhizospheric soil sample (1 g) was mixed with autoclaved distilled water (10 ml) and dilution was prepared to range from 10−1 to 10−5. Several bacterial colonies appeared when sterile tryptone soy agar (TSA) plates were subjected to incubation for 24 h (h) at 28 ± 2°C. The distinct colonies were then picked and streaked on nutrient agar plates. The isolates were re-streaked to obtain pure cultures. Later, the selected isolates were characterized for various experiments.
Soil physicochemical properties such as pH, electrical conductivity, organic carbon, and elemental analysis were tested. pH was measured using a glass electrode on a digital pH meter by preparing soil water suspension of ratio (1:2) following the method of Estefan et al. (2017). Electrical conductivity (EC) was measured following the protocol of Bower and Wilcox (1965), in which soil water suspension of ratio (1:2) was measured using a conductivity meter. Organic carbon (OC) was determined by the method of Walkley (1947). Furthermore, the concentrations of macro elements such as phosphorus, potassium, and sulfur and microelements such as zinc, iron, copper, and boron present in soil samples were analyzed after digestion, using atomic absorption spectroscopy (AAS) and standard calibration curves of the above elements.
Pure cultures of rhizobacterial isolates were incubated for 24 h on TSA plates to study their morphological features. As the colonies appeared, the colony morphology, size, shape, and coloration were observed using Bergey's Manual of Determinative Bacteriology (Holt, 1994). Gram staining was done to determine whether the isolate is gram-positive or gram-negative. Biochemical characterization of the bacterial isolate was conducted, which included a catalase test which was performed according to the method of Reiner (2010), an oxidase test by the method of Tarrand and Gröschel (1982), a citrate utilization test by the method of MacWilliams (2009), and Methyl Red (MR), Voges Proskauer (VP) test according to the method of McDevitt (2009). Different carbon sources including sucrose, dextrose, and lactose were used to determine carbohydrate utilization by the bacterial isolate (Reiner, 2010).
Bacterial isolate was tested for the production of IAA by the method described by Ehmann (1977). IAA produced by the selected isolate was detected and quantified using tryptone soy broth (TSB) supplemented with 1 g L−1 tryptophan. The culture was then incubated at 28 ± 2°C for 2–3 days, IAA production was determined by mixing Salkowski reagent in the bacterial culture supernatant, and absorbance was taken at 530 nm using a spectrophotometer.
Screening the bacterial isolate for its ability to fix nitrogen was carried out using Burk's Nitrogen-free medium (HiMedia) with the protocol of Park et al. (2005). Before autoclaving at 121°C for 15 min (min), the medium's pH was adjusted to 7 ± 0.1. Indicator dye bromothymol blue (BTB) was used for the detection of nitrogen-fixing bacteria. After inoculation, the plates were incubated overnight at 28 ± 2°C. The blue-colored zone production around the colony served as a marker for isolates having the nitrogen-fixing ability.
Production of HCN by the bacterial isolate was determined using the method of Bakker and Schippers (1987). Nutrient agar supplemented with glycine was used for the bacterial culture. Whatman filter paper soaked in a solution of 2% (w/v) Na2CO3 and 0.5% (w/v) picric acid was placed on the lid of Petri plates. Plates were then sealed with parafilm and incubated for 48 h at 28 ± 2°C. The presence of volatile HCN was confirmed by a change in color (from yellow to reddish brown) of the soaked Whatman filter paper.
To determine the phosphate-solubilizing ability of the bacterial isolate, bacterial culture was inoculated in Pikovskaya's agar plates containing insoluble tricalcium phosphate, which were then incubated at 28 ± 2°C for 7 days (Pikovskaya, 1948). Potential phosphate solubilizers were the bacterial colonies that produced distinct transparent halos. The phosphate solubilization index (PSI) was calculated using the following formula:
The calcite solubilization was done using DB agar medium in the following composition/L: glucose 5 g, yeast extract 1 g, peptone 1 g, K2HPO4 0.4 g, MgSO4 0.01 g, NaCl 5 g, (NH4)2SO4 0.05 g, CaCO3 5 g, and agar 15 g (Cacchio et al., 2004). The pinpoint inoculation was done on the agar plates and the plates were placed in an incubator at 28 ± 2°C for 5–7 days. Clear zone formation around the colony confirmed calcite solubilization by bacteria (Tamilselvi et al., 2016). By calculating the ratio of the halo zone to colony size, the solubilization index (SI) of the isolate was determined:
Ammonia production by the bacterial isolate was done in peptone water as described by Cappuccino and Sherman (1996). Overnight grown fresh bacterial culture was inoculated in 10 ml of peptone water and incubated for 48 h at 28 ± 2°C. Nessler's reagent (0.5 ml) was added to each tube after incubation and the color change from yellow to brown marked ammonia production in the culture medium.
On skim milk agar or SMA medium (HiMedia), the bacterial isolate was tested for its proteolytic enzyme production (Masi et al., 2021). The appearance of transparent zones around the colonies after 48 h of incubation at 28 ± 2°C indicates protease production.
The selected bacterial isolate was tested for tolerance against drought stress. Drought tolerance was tested on TSB having different concentrations [0, 10, 15, 20, and 25% (w/v)] of polyethylene glycol (PEG) 6000 (Michel and Kaufmann, 1973). A total of 100 μl of overnight grown bacterial culture [107 colony-forming units (CFUs) mL−1] was inoculated in TSB and incubated at 28 ± 2°C followed by visual examination for the growth for 2–3 days. Tolerance to varying concentrations of PEG was examined by streaking the isolate on TSA plates and incubated at 28 ± 2°C. Viable cells in the medium that grew on the TSA plates showed tolerance against drought stress.
A diffusion agar assay was used to assess the antimicrobial resistance of the bacterial isolate (Armalyte et al., 2019). TSB was used to test for resistance to selected antibiotics. In total, six antimicrobial discs (HiMedia, India) were used, including streptomycin, penicillin, chloramphenicol, kanamycin, tetracycline, and erythromycin. After overnight incubation at 30°C, antibiotic susceptibility was determined by the presence of clear zones around the antibiotic discs, which indicated antibiotic susceptibility.
Genomic DNA was isolated from the bacterial cells according to the method of Wright et al. (2017). Bacterial isolate was identified based on the 16S rRNA gene sequence. Briefly, the 16S rRNA gene was amplified using universal bacterial primers 16SF-5′ AGAGTTTGATCCTGGCTCAG 3′ and 16SR-5′ AAGGAGGTGATCCAGCCGCA 3′. The polymerase chain reaction (PCR) was performed with a 50 μl reaction mixture comprising 5 μl of Taq buffer (10 X), 1 μl of dNTPs (12.5 mM), 1 μl of template genomic DNA, 200–250 μmol L−1 of forward and reverse primers (1 μL each), 0.1 μl of Taq DNA polymerase, 1 μl of MgSO4 (50 mM), and the remaining volume was made up with water. DNA amplification was performed in a thermocycler programmed as initial denaturation for 2 min at 98°C, primer annealing for 1 min at 64°C, primer extension for 1 min at 72°C, and a final extension of 10 min at 72°C up to 35 cycles. The 16S rRNA gene amplicons were visualized in 0.8% agarose gel under UV light using a UV transilluminator. The PCR product was purified and subjected to sequencing.
The sequence of the isolate was subjected to bioinformatic analysis for the identification and phylogenetic relationships. Using a standard nucleotide basic local alignment search tool (BLAST) search, the isolate was identified by the comparison of its 16S rRNA gene sequences with nucleotide sequences present in the National Center for Biotechnology Information (NCBI) database. The 16S rDNA sequence of the isolate was aligned with similar sequences retrieved from the NCBI database using the MUSCLE (MEGA 11), and a phylogenetic tree was constructed to determine the evolutionary relationship of the bacterial isolate using the neighbor-joining method of MEGA 11 based on the 500 bootstraps (Tamura et al., 2021).
First, surface sterilization of the seeds of A. paniculata was done with 0.1% HgCl2 solution for 3 min, washed with sterilized distilled for 4–5 times, again sterilized with 70% ethanol for 3 min, and thereafter washed with sterilized distilled water for 2–3 times. Furthermore, the seeds were primed with a bacterial culture that was pelleted down after centrifugation at 1,000 rpm and suspended in sterile water. After bacterization, the seeds were placed on the Petri plates containing layers of moist filter paper. The seeds were then allowed to germinate at 28 ± 2°C. The appearance of a 2–5 mm radicle was considered an initiation of seed germination (Iida and Takemoto, 2018).
A pot experiment was conducted to study the effect of bioinoculant on plant growth parameters of A. paniculata. Bacterized seeds were sown and allowed to germinate in plastic pots containing sterilized artificial soil having no nutrients (cocopeat 60% + vermiculite 20% + perlite 20%). The uninoculated condition was marked as control has only sterilized artificial soil without any addition. Plants were grown in the controlled conditions of light (14-/10-h light/dark cycle), temperature (25 ± 2°C), and relative humidity (~70%). The seedlings supplemented with bioinoculant served as experimental, while the control seedlings had only sterile water. Bioinoculant supplementation was done every 15-day interval till the flowers appeared. The pots were arranged in random order and the experiments were replicated as 10 pots per treatment: one plant per pot and replicated three times. At various growth stages, observations were recorded related to the plant's growth metrics such as vegetative, flowering, and maturation. Then, 70 days after germination, the plants were carefully removed with intact roots from the pots and then washed with distilled water to remove the soil contents. Parameters such as shoot length, root length, leaf number, node number, whole plant fresh, and dry weight were measured, which are treated as standard for plant growth and development. During the above course, only the bacterial inoculum was used as natural fertilizer instead of chemical fertilizers, and pesticides were also avoided.
Chlorophyll and carotenoid contents from the leaves were calculated using acetone extract (Arnon, 1949). A total of 10 ml of 80% acetone was used to grind the fresh leaves (0.1 g). It was then centrifuged for 5 min at 5,000 rpm (Biehler et al., 2010). It was repeated until the residue was colorless after removing the supernatant. The absorbance of the supernatant was measured at 470, 645, and 663 nm against a blank solvent (acetone). The following equations were used to calculate the chlorophyll a, chlorophyll b, total chlorophyll, and carotenoid content in the plant as per the method of Arnon (1949):
Morphophysiological comparison between control and inoculated plants was done carefully after 7, 21, 28, 35, 42, 48, 56, and 60 days after germination. Evaluation of shoot and root morphology, number of tillers (shoots), root length, shoot length, number of branches, and average number of compound leaflets were analyzed and counted in each plant after ~70 days in control and inoculated plants.
The plants were harvested and washed with sterile water after ~70 days of germination. The length of the shoots and roots was measured and recorded. To obtain the total biomass of roots and shoots, the plants were weighed for the fresh weight (FW) and plants were oven-dried for 12 h at 80°C to obtain dry weight (DW).
One-way analysis of variance (ANOVA) was done to confirm the variability of data and the validity of the results. Student's t-test was performed to measure the significance of data at a 95% confidence level.
The soil from which the sampling was done showed a pH of 6.4, optimum organic carbon content, and average distribution of macro- and micro-elements. The rhizobacteria from the rhizospheric soil of the medicinal plant B. ciliata have been isolated. The isolate was rod-shaped and motile when examined under a microscope and was negative for gram reaction. Based on the morphological and biochemical characteristics, the isolate was found to be positive for oxidase, catalase, citrate utilization, and protease production. Isolate does not show any acid production when tested for the utilization of different carbohydrates (sucrose dextrose and lactose) (Figure 1G). It showed tolerance growth on PEG up to 25%. Moreover, the antibiogram profile of the isolate showed its resistance to penicillin, erythromycin, and chloramphenicol whereas its sensitivity to kanamycin, streptomycin, and tetracycline (Figure 2). The morphological and biochemical characteristics of the isolate are summarized in Table 1.
Figure 1. In vitro biochemical and plant growth-promoting traits of bacterial isolate BC-II-20. (A) IAA production, (B) phosphate solubilization, (C) citrate utilization, (D) protease production, (E) calcite solubilization, (F) catalase test, (G) carbohydrate fermentation tests, and (H) ammonia production.
Figure 2. Antibiotic susceptibility test of bacterial isolate BC-II-20 against six different antibiotics (streptomycin, penicillin, chloramphenicol, kanamycin, tetracycline, and erythromycin).
Table 1. Biochemical characteristics, antibiogram profile and drought stress tolerance of the isolate BC-II-20.
The isolate was initially screened for its in vitro plant growth-promoting (PGP) activities such as indole acetic acid (IAA) production, nitrogen fixation, HCN production, ammonia production, phosphate and calcite solubilization, and siderophore production. The isolate exhibited multiple PGP traits and was positive for IAA production (120 μg ml−1 of culture filtrate), solubilized tricalcium phosphate and calcite, produced ammonia and protease, and was negative for chitinase and siderophore production (Figure 1; Table 2).
Molecular identification of the bacterial isolate was done and sequence analysis was performed. After nucleotide BLAST against 16S ribosomal DNA sequence database, a high similarity was observed with the Pseudomonas sp. A phylogenetic tree was generated using the 16S rDNA sequence with 10 different representative sequences from the NCBI database (Figure 3). Then, there were three broad groups: BC-II-20 showed maximum similarity with P. fluorescens, P. proteolytica, and P. brenneri and were grouped together, P. fidesensis, P. meridiana, and P. antarctica were placed in the second group, and the third group comprised P. extremaustralis, P. petroselini, P. gremontii, and P. rodesiae.
Figure 3. Phylogenetic analysis of BC-II-20 based on 16S rDNA sequencing, aligned with MUSCLE and phylogenetic tree, was constructed using the neighbor-joining method in MEGA 11 software (bootstrap value of 500 replicates).
The A. paniculata bioprimed seeds with the bioinoculant (BC-II-20) showed good germination efficiency, and 100% germination of treated seeds was observed, but less germination rate was seen in the control experiment, which was about 90%. The equivalent concentration of bacterial suspension was further chosen for the pot experiments based on the results of the seed germination experiment. In summary, the isolate displayed a stimulating effect on A. paniculata seed germination.
When the isolate was evaluated for its potential for plant growth in pot experiments on A. paniculata, it significantly improved its agronomic performance. The growth of inoculated plants was much better as observed on days 7, 21, and 28 as compared to the control plants, which showed poor growth. The control plant growth was improved from day 35 onward (Figure 4), but inoculated plants displayed a much better response with increasing growth stages. The growth performance of inoculated plants can be summarized as follows: plant height (~34%), the total number of panicles, panicle length, number of pods and flowers and fresh weight (~57%), and total dry matter (~60%) as compared to the un-inoculated control plants (Figure 5; Table 3). As compared to the control plants, the treated plants also significantly enhanced root development, including root length, root surface area, lateral roots, and root volume (Figure 6). Bioinoculant also significantly enhanced leaf chlorophyll a content by ~22%, chlorophyll b content by ~4%, carotenoid content by ~12%, and total chlorophyll content by ~12% (Figure 7; Table 3). For comparative growth analysis, the leaves from control and inoculated plants (60 days) were arranged in acropetal order (older at the bottom and younger at the top). Visibly, the inoculated plants were more green in color and leaf size was also better than the control plants. A very interesting difference was seen in the panicle as the panicle length was more and well-differentiated in the inoculated plants with the presence of flowers and pods (Figure 6B). Figure 6F represents the arrangement of growth stages from immature bud to mature flower and finally the appearance of the pod.
Figure 4. Plant growth and morphology at different growth stages of development in A. paniculata grown in pots under controlled conditions. Growth stages are depicted from days 7 to 60.
Figure 5. (A) Effect of bioinoculant on the shoot and root lengths, (B) shoot fresh and dry weights, and (C) number of panicles in A. paniculata grown for 70 days in pots under the controlled conditions compared to control. The data showing significance are marked with NS, not significant; *very statistically significant; **extremely statistically significant.
Table 3. Effect on different growth parameters of A. paniculata plants treated with bacterial isolate BC-II-20 at the time of harvesting (after ~70 days post germination).
Figure 6. Effect of bacterial isolate BC-II-20 inoculation in the morphology of root, shoot, and leaves in A. paniculata plants (A) control plants, (B) plants inoculated with bioinoculant (BC-II-20), and (C, D) comparison of leaf size in control (A) and inoculated (B) plants in the same plane in acropetal order. The representative construct/representation of a panicle in the inoculated plant (F) compared to the control plant (E), the growth stages of flowers were arranged from top to bottom in an acropetal manner (older flowers at the bottom and younger at the top) in 60 days old plants.
Figure 7. Effects of bioinoculant BC-II-20 on A. paniculata plant chlorophyll content. The data showing significance are marked with NS, not significant; **extremely statistically significant.
The inoculation of the bacteria also positively influenced the onset of early flowering and the overall growth performance of the plants. Flower bud initiation was observed on the 48th day after germination, after a week (54th day—Figure 4 inset), bud initiation fully bloomed flowers of white color were seen, and this observation was absent in uninoculated/control plants. Also, in the inoculated plants, fully mature panicles with a clear distinction from flower bud initiation to pod formation were seen as compared to the control ones, which showed delayed flowering, and overall growth was slow in control plants (Figure 4). The flower appeared after ~60 days in a less prominent panicle in the uninoculated plants.
This study deals with bioinoculant supplementation on A. paniculata plants and its growth-promoting effects. Bioinoculants are naturally occurring soil microbes that reside around the roots to encourage plant growth and development (Paré et al., 2011). Developing healthy plants resilient to abiotic stresses requires an enhancement of soil functions to promote and ensure sustainable crop production. This may be achieved via complex, labor, and cost-intensive genetic engineering approaches, but the use of greener tools such as bioinoculants offers economical end eco-friendly better alternatives. This involves the application of plant growth-promoting bacteria as a sustainable agronomic practice, thereby enhancing plant growth development and yield.
We have isolated and characterized multitrait Pseudomonas sp., which displayed plant growth-promoting attributes such as IAA production, nitrogen-fixing ability, triphosphate solubilization, calcite solubilization, and ammonia production. IAA production is correlated with better root growth, and nitrogen-fixing ability, phosphate and calcite solubilization to provide nitrogen, mineral phosphates, and a source of calcium available to plants. Many microbes have the innate ability to release organic acids, resulting in their phosphate-solubilizing activity. Pseudomonas is one of the most powerful genera having the capability to solubilize phosphate (Kalayu, 2019). As a result, phosphate-solubilizing microbes are widely used in plant growth promotion and maintain soil fertility while reducing the increasing costs of hazardous phosphate fertilizers. Ahmed et al. (2014) also isolated bacteria from the rhizosphere of some medicinal plants and reported their plant growth-promoting attributes. P. brenneri (Hayat et al., 2013) and P. fluorescens (Lally et al., 2017) also displayed tricalcium phosphate solubilization and nitrogen-fixing ability.
Out of six antibiotics, the bacterial isolate seemed to be resistant to only three antibiotics such as penicillin, erythromycin, and chloramphenicol. According to Ferjani et al. (2019), Pseudomonas rhizobacteria expressed a low rate of antibiotic resistance as a result of various pathogens and human activities. Because of low antibiotic resistance, the noxious effects and the evolution of resistance in the environment are limited. Bioinoculant Pseudomonas sp. under study can grow under drought conditions as it showed tolerance at 25% PEG. PGPR application enhanced drought tolerance in potato plants as reported by Batool et al. (2020), thereby proving the role of such beneficial bacteria in abiotic stress management.
Multitrait bacterial isolate BC-II-20 was identified as Pseudomonas sp. based on the sequence of 16S rRNA gene amplification. BC-II-20 Pseudomonas sp. showed a close phylogenetic relationship with P. brenneri and P. fluorescens, which are reported PGPRs. Inoculation of wheat plants with P. brenneri enhanced its shoot, root and overall growth (Hayat et al., 2013) and P. fluorescens acts as efficient PGPRs in many crops (Sah et al., 2021). Pseudomonas is diverse and has been isolated from the rhizosphere of several plants and reported to have plant growth-promoting traits (Qessaoui et al., 2019).
Plants undergo diverse changes that are induced by PGPR, and growth is the result of complex and interrelated pathways (Bharti et al., 2016). Inoculation of A. paniculata with our bacterial isolate significantly increased germination and root branching as compared to the control. The greater root surface that arises from root development can consequently have a favorable impact on the absorption of nutrients and water. According to Premalatha et al. (2021), the availability of nutrients has a major impact on plant productivity and quality. IAA is a phytohormone associated with root development (Ortiz-Castro et al., 2014). The bacterial isolate used in this study is a good IAA producer, thereby promoting root growth of primary roots and subsequent development of secondary roots, thus helping plants to absorb nutrients more effectively as also reported by Patten and Glick (2002). There is some evidence that direct interactions between plants and IAA-producing bacteria can have various outcomes, including phytostimulation, based on the biochemical pathways of bacterial IAA synthesis and regulation (Spaepen et al., 2007).
Photosynthetic ability is increased when growth and nutrition are proper, and this was observed in our study as the inoculated plants had better root development, so more nutrient acquisition and water absorption promoted plant growth and increased plant biomass. Bioinoculant BC-II-20 induced photosynthetic pigments viz. 22% more chlorophyll a content, 12% more carotenoid content, and a 12% increase in total chlorophyll content. Better growth performance after germination was observed in inoculated plants on 7, 21, 28, and 35 days after germination, thereby proving the role of PGPR-mediated growth enhancement. When fully mature plants were compared, we can figure out very prominent panicles in inoculated A. paniculata plants. The presence of more photosynthetic pigments imparted a more greenish color in leaves as observed visually, and the size of leaves was also bigger as compared to the control. Growth and development of many plant species were increased after inoculation with PGPRs, and Cappellari et al. (2013) also reported an increase in plant growth parameters in marigold (Tagetes minuta) after inoculation with P. fluorescens.
In our study, the inoculated plant had early initiation of flowering, and the number of flowers increased, as compared to the uninoculated plant. Early flowering might be due to the indirect effect of PGPR, which can increase the availability of nutrients in the soil and enhance different physiological processes. Redondo-Gómez et al. (2022) reported flower bud induction in strawberry plants after inoculation with PGPRs and colonization by Paenibacillus lentimorbus also resulted in more flowers and seeds in tobacco, as reported by Kumar et al. (2016). It may be hypothesized that early flowering in healthy plants of A. paniculata may reduce its life cycle. These observations were also supported by the results of Poupin et al. (2013), which show that the whole life cycle of the plant may be impacted by Burkholderia phytofirmans, a PGPR which showed accelerated growth and reduced vegetative stages.
Overall enhancement in the growth and physiological parameters of A. paniculata was observed by the application of bioinoculant, BC-II-20 (Pseudomonas sp.) plant growth-promoting traits were displayed by the beneficial microbe in this study, and its direct and indirect effects positively correlated with the increased biomass and better photosynthetic efficiency. Bioinoculant assisted and promoted the growth of the plant in a sustainable manner, and ultimately, its early flowering and maturation led to a reduction in its life cycle, thereby increasing commercial applications. Further experiments are needed to ensure and achieve maximum positive effects using this PGPR, also in other crops. The underlying molecular basis of the observed phenomenon also needs to be undertaken.
The raw data supporting the conclusions of this article will be made available by the authors, without undue reservation.
SY conceived and designed the research study. RT conducted the experiments. RT and SY analyzed the results and wrote the article. SS performed the statistical analyses and editing. All authors reviewed the manuscript. All authors contributed to the article and approved the submitted version.
The authors declare that the research was conducted in the absence of any commercial or financial relationships that could be construed as a potential conflict of interest.
All claims expressed in this article are solely those of the authors and do not necessarily represent those of their affiliated organizations, or those of the publisher, the editors and the reviewers. Any product that may be evaluated in this article, or claim that may be made by its manufacturer, is not guaranteed or endorsed by the publisher.
Ahmad, M., Butt, M. A., Zhang, G., Sultana, S., Tariq, A., and Zafar, M. (2018). Bergenia ciliata: a comprehensive review of its traditional uses, phytochemistry, pharmacology and safety. Biomed. Pharmacother. 97, 708–721. doi: 10.1016/j.biopha.2017.10.141
Ahmed, E. A., Hassan, E. A., Tobgy, K. M. K. E., and Ramadan, E. M. (2014). Evaluation of rhizobacteria of some medicinal plants for plant growth promotion and biological control. Ann. Agric. Sci. 59, 273–280. doi: 10.1016/j.aoas.2014.11.016
Armalyte, J., Skerniškyte, J., Bakiene, E., Krasauskas, R., Šiugždiniene, R., Kareiviene, V., et al. (2019). Microbial diversity and antimicrobial resistance profile in microbiota from soils of conventional and organic farming systems. Front. Microbiol. 10, 892. doi: 10.3389/fmicb.2019.00892
Arnon, D. I. (1949). Copper enzymes in isolated chloroplasts. Polyphenoloxidase in Beta vulgaris. Plant Physiol. 24, 1–15. doi: 10.1104/pp.24.1.1
Bakker, A. W., and Schippers, B. (1987). Microbial cyanide production in the rhizosphere in relation to potato yield reduction and Pseudomonas spp. mediated plant growth-stimulation. Soil Biol. Biochem. 19, 451–457. doi: 10.1016/0038-0717(87)90037-X
Batool, T., Ali, S., Seleiman, M. F., Naveed, N. H., Ali, A., Ahmed, K., et al. (2020). Plant growth promoting rhizobacteria alleviates drought stress in potato in response to suppressive oxidative stress and antioxidant enzymes activities. Sci. Rep. 10, 16975. doi: 10.1038/s41598-020-73489-z
Bharti, N., Pandey, S. S., Barnawal, D., Patel, V. K., and Kalra, A. (2016). Plant growth promoting rhizobacteria Dietzia natronolimnaea modulates the expression of stress responsive genes providing protection of wheat from salinity stress. Sci. Rep. 6, 34768. doi: 10.1038/srep34768
Biehler, E., Mayer, F., Hoffmann, L., Krause, E., and Bohn, T. (2010). Comparison of 3 spectrophotometric methods for carotenoid determination in frequently consumed fruits and vegetables. J. Food Sci. 75, C55–61. doi: 10.1111/j.1750-3841.2009.01417.x
Bower, C. A., and Wilcox, L. V. (1965). “Soluble salts,” in Agronomy Monographs, ed A. G. Norman (Madison, WI: American Society of Agronomy; Soil Science Society of America), 933–951. doi: 10.2134/agronmonogr9.2.c11
Cacchio, P., Contento, R., Ercole, C., Cappuccio, G., Martinez, M. P., and Lepidi, A. (2004). Involvement of microorganisms in the formation of carbonate speleothems in the cervo cave (L'Aquila-Italy). Geomicrobiol. J. 21, 497–509. doi: 10.1080/01490450490888109
Cappellari, L., del, R., Santoro, M. V., Nievas, F., Giordano, W., and Banchio, E. (2013). Increase of secondary metabolite content in marigold by inoculation with plant growth-promoting rhizobacteria. Agric. Ecosyst. Environ. Appl. Soil Ecol. 70, 16–22. doi: 10.1016/j.apsoil.2013.04.001
Cappuccino, J. G., and Sherman, N. (1996). Microbiology: A Laboratory Manual, 4th Edn. New York, NY: Benjamin/Cummings Publishing Company.
Egamberdieva, D., and Teixeira da Silva, J. A. (2015). “Medicinal plants and PGPR: A new frontier for phytochemicals,” in Plant-Growth-Promoting Rhizobacteria (PGPR) and Medicinal Plants, ed D. Egamberdieva, S. Shrivastava, and A. Varma (New York, NY; Cham; Heidelberg: Springer), 287–303. doi: 10.1007/978-3-319-13401-7_14
Ehmann, A. (1977). The van URK-Salkowski reagent - a sensitive and specific chromogenic reagent for silica gel thin-layer chromatographic detection and identification of indole derivatives. J. Chromatogr. A 132, 267–276. doi: 10.1016/S0021-9673(00)89300-0
Estefan, G., Sommer, R., and Ryan, J. (2017). Methods of Soil, Plant, and Water Analysis: A Manual for the West Asia and North Africa Region, 3rd Edn. Available online at: https://repo.mel.cgiar.org/handle/20.500.11766/7512 (accessed December 22, 2022).
Ferdosi, M. F., Khan, I. H., Javaid, A., Hafiz, M. S., Butt, I., and Munir, A. (2021). GC-MS analysis and bioactive components of flowers of Bergenia ciliata, a weed of rock crevices in Pakistan. Pak. J. Weed Sci. Res. 27, 527. doi: 10.28941/pjwsr.v27i4.1012
Ferjani, R., Gharsa, H., Estepa-Pérez, V., Gómez-Sanz, E., Cherni, M., Mahjoubi, M., et al. (2019). Plant growth-promoting Rhizopseudomonas: expanded biotechnological purposes and antimicrobial resistance concern. Ann. Microbiol. 69, 51–59. doi: 10.1007/s13213-018-1389-0
Hayat, R., Sheirdil, R. A., Iftikhar-ul-Hassan, M., and Ahmed, I. (2013). Characterization and identification of compost bacteria based on 16S rRNA gene sequencing. Ann. Microbiol. 63, 905–912. doi: 10.1007/s13213-012-0542-4
Holt, J. G. (1994). Bergey's Manual of Determinative Bacteriology. Philadelphia, PA: Lippincott Williams & Wilkins.
Iida, M., and Takemoto, K. (2018). A network biology-based approach to evaluating the effect of environmental contaminants on human interactome and diseases. Ecotoxicol. Environ. Saf. 160, 316–327. doi: 10.1016/j.ecoenv.2018.05.065
Kalariya, K. A., Gajbhiye, N. A., Meena, R. P., Saran, P. L., Minipara, D., Macwan, S., et al. (2021). Assessing suitability of Andrographis paniculata genotypes for rain-fed conditions in semi-arid climates. Inf. Process. Agric. 8, 359–368. doi: 10.1016/j.inpa.2020.09.003
Kalayu, G. (2019). Phosphate solubilizing microorganisms: promising approach as biofertilizers. Int. J. Agron. 2019, 4917256. doi: 10.1155/2019/4917256
Khan, K., Pankaj, U., Verma, S. K., Gupta, A. K., Singh, R. P., and Verma, R. K. (2015). Bio-inoculants and vermicompost influence on yield, quality of Andrographis paniculata, and soil properties. Ind. Crops Prod. 70, 404–409. doi: 10.1016/j.indcrop.2015.03.066
Kour, H., Raina, R., Verma, P. K., Khan, A. M., Makhmoor Ahmad, B., and Nashiruddullah, N. (2021). Evaluation of the wound healing activity of ethanolic extract of Bergenia ciliata (Haw.) Sternb. Rhizome with excision wound model in Wistar rats. J. Ethnopharmacol. 281, 114527. doi: 10.1016/j.jep.2021.114527
Kumar, B., Verma, S. K., and Singh, H. P. (2011). Effect of temperature on seed germination parameters in Kalmegh (Andrographis paniculata Wall. Ex Nees.). Ind. Crops Prod. 34, 1241–1244. doi: 10.1016/j.indcrop.2011.04.008
Kumar, S., Chauhan, P. S., Agrawal, L., Raj, R., Srivastava, A., Gupta, S., et al. (2016). Paenibacillus lentimorbus inoculation enhances tobacco growth and extenuates the virulence of cucumber mosaic virus. PLoS ONE 11, e0149980. doi: 10.1371/journal.pone.0149980
Lally, R. D., Galbally, P., Moreira, A. S., Spink, J., Ryan, D., Germaine, K. J., et al. (2017). Application of endophytic Pseudomonas fluorescens and a bacterial consortium to brassica napus can increase plant height and biomass under greenhouse and field conditions. Front. Plant Sci. 8, 2193. doi: 10.3389/fpls.2017.02193
MacWilliams, M. P. (2009). Citrate test protocol. American Society for Microbiology Laboratory Protocols. Available online at: https://www.asmscience.org/content/education/protocol/protocol3203 (accessed December 23, 2022).
Masi, C., Gemechu, G., and Tafesse, M. (2021). Isolation, screening, characterization, and identification of alkaline protease-producing bacteria from leather industry effluent. Ann. Microbiol. 71, 24. doi: 10.1186/s13213-021-01631-x
McDevitt, S. (2009). Methyl Red and Voges-Proskauer Test Protocols. Available online at: http://www.asmscience.org/content/education/protocol/protocol.3204 (accessed December 22, 2022).
Mhlongo, M. I., Piater, L. A., Madala, N. E., Labuschagne, N., and Dubery, I. A. (2018). The chemistry of plant–microbe interactions in the rhizosphere and the potential for metabolomics to reveal signaling related to defense priming and induced systemic resistance. Front. Plant Sci. 9, 112. doi: 10.3389/fpls.2018.00112
Michel, B. E., and Kaufmann, M. R. (1973). The osmotic potential of polyethylene glycol 6000. Plant Physiol. 51, 914–916. doi: 10.1104/pp.51.5.914
Okhuarobo, A., Falodun, J. E., Erharuyi, O., Imieje, V., Falodun, A., and Langer, P. (2014). Harnessing the medicinal properties of Andrographis paniculata for diseases and beyond: A review of its phytochemistry and pharmacology. Asian Pac. J. Trop. Dis. 4, 213–222. doi: 10.1016/S2222-1808(14)60509-0
Ortiz-Castro, R., Pelagio-Flores, R., Méndez-Bravo, A., Ruiz-Herrera, L. F., Campos-García, J., and López-Bucio, J. (2014). Pyocyanin, a virulence factor produced by Pseudomonas aeruginosa, alters root development through reactive oxygen species and ethylene signaling in arabidopsis. Mol. Plant Microbe Interact. 27, 364–378. doi: 10.1094/MPMI-08-13-0219-R
Paré, P. W., Zhang, H., Aziz, M., Xie, X., Kim, M.-S., Shen, X., et al. (2011). “Beneficial rhizobacteria induce plant growth: mapping signaling networks in arabidopsis,” in Biocommunication in Soil Microorganisms, Vol. 23, ed G. Witzany (Springer Berlin Heidelberg), 403–412.
Park, M., Kim, C., Yang, J., Lee, H., Shin, W., Kim, S., et al. (2005). Isolation and characterization of diazotrophic growth promoting bacteria from rhizosphere of agricultural crops of Korea. Microbiol. Res. 160, 127–133. doi: 10.1016/j.micres.2004.10.003
Patten, C. L., and Glick, B. R. (2002). Role of Pseudomonas putida indoleacetic acid in development of the host plant root system. Appl. Environ. Microbiol. 68, 3795–3801. doi: 10.1128/AEM.68.8.3795-3801.2002
Pikovskaya, R. I. (1948). Mobilization of phosphorus in soil in connection with vital activity of some microbial species. Mikrobiologiya 17, 362–370.
Poupin, M. J., Timmermann, T., Vega, A., Zuñiga, A., and González, B. (2013). Effects of the plant growth-promoting bacterium Burkholderia phytofirmans PsJN throughout the life cycle of Arabidopsis thaliana. PLoS ONE 8, e69435. doi: 10.1371/journal.pone.0069435
Premalatha, K., Botlagunta, N., Santhosh, D., Hiremath, C., Verma, R. K., Shanker, K., et al. (2021). Enhancement of soil health, germination and crop productivity in Andrographis paniculata (Burm.f.) Nees, an important medicinal crop by using a composite bio inoculant. J. Plant Nutr. 44, 2331–2346. doi: 10.1080/01904167.2021.1899207
Qessaoui, R., Bouharroud, R., Furze, J. N., El Aalaoui, M., Akroud, H., Amarraque, A., et al. (2019). Applications of new rhizobacteria pseudomonas isolates in agroecology via fundamental processes complementing plant growth. Sci. Rep. 9, 12832. doi: 10.1038/s41598-019-49216-8
Redondo-Gómez, S., García-López, J. V., Mesa-Marín, J., Pajuelo, E., Rodriguez-Llorente, I. D., and Mateos-Naranjo, E. (2022). Synergistic effect of plant-growth-promoting rhizobacteria improves strawberry growth and flowering with soil salinization and increased atmospheric CO2 levels and temperature conditions. Agronomy 12, 2082. doi: 10.3390/agronomy12092082
Reiner, K. (2010). Catalase Test Protocol. Available online at: https://asm.org/protocols/catalase-test-protocol (accessed December 22, 2022).
Sah, S., Krishnani, S., and Singh, R. (2021). Pseudomonas mediated nutritional and growth promotional activities for sustainable food security. Curr. Res. Microb. Sci. 2, 100084. doi: 10.1016/j.crmicr.2021.100084
Sharf, W., Javaid, A., Shoaib, A., and Khan, I. H. (2021). Induction of resistance in chili against Sclerotium rolfsii by plant-growth-promoting rhizobacteria and Anagallis arvensis. Egypt. J. Biol. Pest Control 31, 16. doi: 10.1186/s41938-021-00364-y
Spaepen, S., Vanderleyden, J., and Remans, R. (2007). Indole-3-acetic acid in microbial and microorganism-plant signaling. FEMS Microbiol. Rev. 31, 425–448. doi: 10.1111/j.1574-6976.2007.00072.x
Tamilselvi, S. M., Thiyagarajan, C., and Uthandi, S. (2016). Calcite dissolution by brevibacterium sp. SOTI06: a futuristic approach for the reclamation of calcareous sodic soils. Front. Plant Sci. 7, 1828. doi: 10.3389/fpls.2016.01828
Tamura, K., Stecher, G., and Kumar, S. (2021). MEGA11: molecular evolutionary genetics analysis version 11. Mol. Biol. Evol. 38, 3022–3027. doi: 10.1093/molbev/msab120
Tarrand, J. J., and Gröschel, D. H. (1982). Rapid, modified oxidase test for oxidase-variable bacterial isolates. J. Clin. Microbiol. 16, 772–774. doi: 10.1128/jcm.16.4.772-774.1982
Walkley, A. (1947). A critical examination of a rapid method for determining organic carbon in soils-effect of variations in digestion conditions and of inorganic soil constituents. Soil Sci. 63, 251–264. doi: 10.1097/00010694-194704000-00001
WHO (2019). Traditional, Complementary and Integrative Medicine. Available online at: https://www.who.int/teams/integrated-health-services/traditional-complementary-and-integrative-medicine (accessed December 23, 2022).
Wright, M. H., Adelskov, J., and Greene, A. C. (2017). Bacterial DNA extraction using individual enzymes and phenol/chloroform separation. J. Microbiol. Biol. Educ. 18, 18.2.48. doi: 10.1128/jmbe.v18i2.1348
Yilmaz, A., and Karik, Ü. (2022). AMF and PGPR enhance yield and secondary metabolite profile of basil (Ocimum basilicum L.). Ind. Crops. Prod. 176, 114327. doi: 10.1016/j.indcrop.2021.114327
Keywords: Andrographis paniculata, biofertilizer, plant growth promotion, seed biopriming, PGPR, sustainable development goals (SDGs)
Citation: Thakur R, Srivastava S and Yadav S (2023) Multitrait Pseudomonas sp. isolated from the rhizosphere of Bergenia ciliata acts as a growth-promoting bioinoculant for plants. Front. Sustain. Food Syst. 7:1097587. doi: 10.3389/fsufs.2023.1097587
Received: 14 November 2022; Accepted: 02 January 2023;
Published: 27 January 2023.
Edited by:
Arshad Javaid, University of the Punjab, PakistanReviewed by:
Mohamed Anli, Cadi Ayyad University, MoroccoCopyright © 2023 Thakur, Srivastava and Yadav. This is an open-access article distributed under the terms of the Creative Commons Attribution License (CC BY). The use, distribution or reproduction in other forums is permitted, provided the original author(s) and the copyright owner(s) are credited and that the original publication in this journal is cited, in accordance with accepted academic practice. No use, distribution or reproduction is permitted which does not comply with these terms.
*Correspondence: Saurabh Yadav, c2F1cmFiaHlhZGF2NDBAcmVkaWZmbWFpbC5jb20=
Disclaimer: All claims expressed in this article are solely those of the authors and do not necessarily represent those of their affiliated organizations, or those of the publisher, the editors and the reviewers. Any product that may be evaluated in this article or claim that may be made by its manufacturer is not guaranteed or endorsed by the publisher.
Research integrity at Frontiers
Learn more about the work of our research integrity team to safeguard the quality of each article we publish.