- 1College of Development Studies, Addis Ababa University, Addis Ababa, Ethiopia
- 2Ethiopian Institute Policy Studies, Addis Ababa, Ethiopia
Introduction: The objective of this study was to analyze how innovations in Climate-smart agriculture (CSA) contribute to improving the food and nutrition security of smallholder households, thereby promoting sustainable food systems.
Methods: A cross-sectional household survey was conducted among a multi-stage sample of 424 smallholder farmers drawn from five different agroecosystems. To examine households’ food and nutrition security, we used food consumption score (FCS) and modified household dietary diversity score (HDDS) in propensity score matching (PSM) and endogenous switching regression (ESR) estimation models.
Results: PSM results showed that crop residue management, compost, and agroforestry have a significant effect on improving households’ food and nutrition security by 21.3, 13.6, and 16.6%, respectively, whereas Soil and water conservation (SWC) has reduced adopters’ food security by 12.9%. However, the conditional average treatment effect, or ESR result, reveals that households’ food and nutrition security has improved as a result of the adoption of crop residue management, compost, SWC, and agroforestry.
Discussion: Although the impact of crop residue management, compost, and agroforestry is positive, the effect of SWC on household food security has been inconclusive. Hence, it is important to upscale the adoption of multiple CSA innovations to improve smallholder household’s food security in the face of climate change.
1 Introduction
Despite Africa contributing the least to greenhouse gas emissions on a global scale, the continent has experienced significant loss and damage in key development sectors as a result of anthropogenic climate change (Ayugi et al., 2022). Climate projections show that the drought changes over East Africa follow a “dry get drier and wet gets wetter” trend (Haile et al., 2020). Climate change is damaging Africa’s agriculture, forestry, fisheries, and aquaculture (Trisos et al., 2022). Anthropogenic climate change has a significant impact on the livelihoods of smallholder farmers, primarily due to various interconnected factors that include reductions in crop yields, changes in market dynamics, increases in food prices, and the destruction of supply chain infrastructure due to extreme warming, changing precipitation patterns, and the increased frequency of extreme events such as drought and floods (Kotir, 2011; Murray et al., 2016; Harvey et al., 2018; IPCC, 2019). It threatens the food systems of sub-Saharan Africa by affecting all components of food security: food availability, food accessibility, food utilization, and food stability, exacerbating poverty reduction, food security, and achieving the Sustainable Development Goals (SDGs) (Kotir, 2011; Makate, 2019). These adverse effects disrupt agricultural production, making it more challenging for smallholder farmers to sustain their livelihoods exacerbating food insecurity and making food security a major agricultural development concern in Sub-Saharan Africa (FAO, 2020).
Thus, food insecurity has been a major issue in Ethiopia for decades due to a series of production failures that resulted in chronic food insecurity since the 1970s. Since 1950, twelve major drought-induced food security crises have occurred (FDRE, 2019). Due to climate change hazards such as flooding, infrastructures such as roads have been washed away or closed, hindering market access and exacerbating a shortage of available food consumption in the summer lean season (Bewekt, 2012; Melak, 2014). Even in the most productive areas, poorer farmers purchase some of their food, and households depend heavily on markets and in-kind contributions during the agricultural lean seasons (WFP, 2014). Hence, not only food availability but also food access components are critical for food security in Ethiopia.
The adoption of climate-smart agriculture (CSA) is one important step toward improving the well-being of smallholder farming communities in developing countries dealing with climate change and limited land for agricultural expansion (Makate et al., 2019; Mujeyi et al., 2021; Sardar et al., 2021). According to the literature, increased agricultural productivity can improve household welfare by increasing income and improving food security by allowing them to produce their food (Mujeyi et al., 2021; Tesfaye et al., 2021). Hence, farmers decide to adopt CSA innovations depending on several benefits associated with the technologies they adopt, and among these, food security is the most important one (Mujeyi et al., 2021; Teklu et al., 2023b). Adoption of CSA innovations directly affects crop-livestock productivity, non-food agricultural production such as fuel wood lot, and off-farm activities. The agricultural food stack is used for household food consumption, while sales of agricultural products and fuel wood lots, help to access food from the market and pay for health and water sanitation (Teklu et al., 2022). The feedback due to climate change will affect the decision to adopt CSA innovations. Moreover, the increase in food security of the household will increase the welfare of the household and encourage the adoption of CSA innovations that enhance food security (Teklu et al., 2023a). Thus, improving agricultural productivity is a means of enhancing the availability of food, rural income, and other livelihood assets for farm households.
Literature exists on climate change adaptation strategies (Deressa et al., 2011; Tesfaye and Seifu, 2016; Asrat and Simane, 2018; Asmare et al., 2019), yet there is limited literature on the impact of CSA innovations on food security. Although there are few studies, particularly in the Upper Blue Nile Highlands of Ethiopia (Teklewold et al., 2017, 2019), they lack findings on the impact of CSA innovations on food security by taking standard food security measurements. Therefore, the primary goal of this paper is to investigate the impact of CSA innovation adoption on household food and nutrition security.
2 Materials and methods
2.1 Study area
The Choke Mountain watershed is located in Ethiopia’s Blue Nile Highlands. It is located between 9° 38′ 00″ and 10°55′ 24″ North and 37° 07′ 00″ and 38° 17′ 00″ East. The Choke Mountain watershed is part of the Blue Nile highlands which are known to be surplus-producing regions and the water tower of the Blue Nile (Simane et al., 2016). Agriculture is the main economic activity and source of livelihood. The farming system is characterized by a wheat-maize-teff-dominated mixed crop and livestock production system.
2.2 Data source and sampling design
The study employed quantitative data. The quantitative data was generated from household surveys. Household and plot-level survey data gathered consists of information on socio-demographic and economic characteristics; climate-smart agricultural practices and preferences; crop production; livelihood assets; climate change risk and exposure; food consumed and frequency; and climate resilience. In addition, secondary data were also collected from Woreda1 agricultural offices through a desk review that includes information on the agroecosystems, precipitation, temperature, land use, crop production, livestock, population, and other data related to the study objectives. The sample size determination was calculated based on finite population sample size calculation (Cochran, 1977). The sample size is 424 smallholder households. A multi-stage sampling technique was used to randomly select 424 households from the five woredas. Following the selection of households, quantitative data was gathered using a structured household survey questionnaire focused on household and farm characteristics and was collected on a one-to-one interview basis by well-trained and experienced enumerators using Android tablets (Table 1).
2.3 Measuring food security
Several indicators are used to measure food security at the household level, and these commonly include The Household Dietary Diversity Score (HHDS), the Copying Strategy Index (CSI), and the Food Consumption Score (FCS). We used the food consumption score (FCS), which shows the quantity and quality of food consumed by a household in the last 7 days, to measure food security at the household level. FCS encompasses food security’s food availability, access, and utilization aspects (Maxwell et al., 2014; Wekesa et al., 2018).
In the study, a modified Household Dietary Diversity Score (HDDS) was computed for a household based on their consumption of various food items during the preceding 7-day period (FAO, 2010). The food items were divided into eight distinct food groups, and any item consumed by a household within the previous week contributed to the household’s score. The modified HDDS is a continuous score ranging from 0 to 8, representing the household’s diversity of food groups consumed. Cereals, roots and tubers, pulses, vegetables, fruits, meat, eggs, fish, milk and milk products, oils and fats, sugar, and condiments were the food groups considered in the calculation.
2.4 Data analysis
Depending on the nature of the variable and the need for presentation, the descriptive data analysis was conducted to obtain frequencies and cross-tabulations, as well as mean, standard deviation, and percentage. The analysis was done by STATA version 15. T-test and chi-square test were used to determine the statistical significance of variations in CSA innovation adoption on food security. The study examined the impact of CSA innovations on food and nutrition security using Propensity Score Matching (PSM) and Endogenous Switching Regression (ESR). Propensity score matching (PSM) and Endogenous Switching Regression (ESR) are the two latest impact evaluation, methodologies in developing countries. Besides ESR controls the selection bias and the unobserved factor effect of endogeneity in the model. The dependent variables for the ESR are the adoption of CSA innovations such as improved variety, crop residue management, crop rotation, compost, row planting, soil and water conservation, and agroforestry (Shiferaw et al., 2014; Abate et al., 2015; Teshome et al., 2016; Teklewold et al., 2017, 2019; Tesfaye et al., 2017; Mihretie et al., 2022). The independent variables were chosen based on an extensive literature review on CSA innovations in Sub-Saharan Africa and Ethiopia, which was primarily based on previous empirical literature on food security determinants.
The selection of the independent variables for empirical model specification was based on theoretical, and conceptual frameworks, and past empirical adoption of agricultural technologies (Knowler and Bradshaw, 2007; Kassie et al., 2013; Teklewold et al., 2013, 2017, 2019; Agidie et al., 2014; Aryal et al., 2018; Zeng et al., 2018). They include socio-demographic/personal factors such as age, sex, and education level of the household head. Personal factors often influence technology adoption decisions when there are market imperfections and institutional failure (Long et al., 2016; Melisse, 2018). Economic factors such as farm size and the number of plots may influence farmers’ decisions to adopt agricultural technology. Institutional variables such as access to public extension services play a crucial role in enhancing adoption (Chowdhury et al., 2014). Agricultural extension services on climate change and CSA practice-related topics such as conservation agriculture, soil and water conservation, climate change, and agronomic practices also influence the likelihood of the farmers adopting these technologies (Ntshangase et al., 2018). Access to markets, as well as information communication, matters for adoption as farmers get information from multiple sources, including farmer-to-farmer communication, public extension service, and mobile phone and radio (Mittal, 2012; Murage et al., 2012). For the environmental factor, we took soil fertility and plot slope of the farmland as a biophysical factor (Zerihun et al., 2014).
The adoption and impact of CSA innovations on food security were modeled in the setting of a two-stage framework using the Endogenous Switching regression model (di Falco et al., 2011). In the first stage, we use a selection model for CSA innovations adoption where a smallholder farmer chooses to implement CSA innovations if their gain of food security outweighs the loss incurred due to its adoption. Let G* be the latent variable that captures the expected food security gains from its adoption versus not adopting. The latent variable is specified as:
That is, farmer i will adopt CSA innovation (Gi = 1) in response to different socio-demographic, economic, institutional, and environmental factors if G*i > 0 otherwise 0. The vector Z represents variables that maximize the farmer’s food security gains by adopting CSA innovations.
In the second stage, the model estimation assumes that farmers who adopted the CSA technology may have systematically different characteristics from the farmers who did not adopt it. Unobservable characteristics of farmers and their farms may affect both adoption decisions and their outcomes, resulting in inconsistent estimates. By estimating a simultaneous equations model of the adoption of CSA innovation and its impact on food security with an endogenous switching regression (ESR) model of the full information maximum likelihood (FIML) estimation method, the study thus simultaneously controls the effect of factors on adoption decisions and their outcomes (Adego et al., 2019; Table 2).
3 Results and discussion
3.1 Food security and adoption of CSA innovations
The study found that less than one-third, specifically 33%, was classified as food secure based on having an acceptable food consumption score. Whereas, more than two-thirds of the households, specifically 46% were categorized as food insecure as they have a borderline food consumption score, indicating a precarious food security situation, while 21% had a poor food consumption score, indicating significant food insecurity. These findings highlight the high prevalence of food insecurity among the smallholder households surveyed, underscoring the need for interventions to improve their food and nutrition security status (Figure 1).
According to Table 3, the adoption of Climate-smart agriculture (CSA) innovations by smallholder farmers has a significant relationship with their food security status. Specifically, the adoption of crop residue management is positively associated with improved food security, while the adoption of soil and water conservation (SWC) is negatively associated with food security (p < 0.01).
The findings suggest that households that adopt SWC practices have poorer food consumption compared to non-adopters, indicating a higher prevalence of food insecurity among SWC adopters. These households may lack access to daily staple foods, vegetables, as well as oils and pulses, with consumption frequency falling below the recommended levels (four times a week). In contrast, households that adopt crop residue management exhibit more acceptable food consumption patterns, indicating a higher level of food security. These households are likely to have access to daily staple foods, vegetables, oils, and pulses at or above the recommended consumption frequency.
According to the findings, there are variations in the Household Dietary Diversity Score (HDDS) among adopters and non-adopters of specific Climate-smart agriculture (CSA) innovations. Adopters of agroforestry have the highest HDDS (4.43), indicating a greater diversity in their diets. On the other hand, adopters of SWC have the lowest HDDS (3.83), suggesting a lower dietary diversity.
The results indicate that adopters of improved varieties, crop residue management, compost, row planting, and agroforestry have higher HDDS values compared to non-adopters, i.e., CSA adopters consume better-diversified diets.
improved varieties, crop residue management, compost, row planting, and agroforestry positively impact the food utilization component of food security and improve the diversity and quality of the food consumed. Hence, improved variety, crop residue management, compost, and row planting positively affect the food availability and access components of food security. In contrast, agroforestry positively affects the food utilization component. On the other hand, the adoption of SWC negatively affects the food availability component of food security (Figure 2).
According to Table 4, the effect of Climate-smart agriculture (CSA) adoption on the Food Consumption Score (FCS) and food security status of smallholder households has been analyzed. Adopters of improved varieties have an acceptable food security status, with a significantly higher FCS value compared to non-adopters (p < 0.01). This positive effect on food security aligns with previous studies that reported the enhanced food security achieved through the adoption of high-yield improved crop varieties (Shiferaw et al., 2014; Teklewold et al., 2019), which reported that improved varieties have significantly enhanced household food security through the adoption of high-yield-improved wheat varieties. Similarly, Adopters of row planting also have an acceptable food security status, with a significantly higher FCS value compared to non-adopters (p < 0.05). This finding corroborates the notion that row planting practices contribute to improved food security among adopters (Fentie and Beyene, 2019) who reported row planting has enhanced the food security of adopters. Adopters of crop residue management have an acceptable food security status, with a significantly higher FCS value compared to non-adopters (p < 0.01). In contrast, non-adopters of crop residue management have borderline food security. This indicates that crop residue management practices positively impact food security outcomes. Likewise, Adopters of soil and water conservation (SWC) practices have borderline food security, with a significantly lower FCS value compared to non-adopters who have an acceptable food security status (p < 0.01). This negative effect on food security aligns with the finding that the impact of SWC on crop yield is influenced by factors such as rainfall characteristics, crop type, slope, and soil conditions (Teklu et al., 2022). Moreover, the effect of SWC on crop yield was negatively correlated with rainfall for SWC, including level Fanya juu, graded soil bunds, stone bunds, and trash lines, which are the main characteristics of the Choke Mountain watershed (Kato et al., 2011; Wolka et al., 2018).
3.2 Propensity score matching result
The study used propensity score matching, a non-parametric technique, to estimate the average treatment effect of CSA innovation on food security. To estimate the average treatment effect, the estimates of the propensity score for the treated (ATT), matched-treated, and non-treated farming households were calculated using logistic regression. The overlap condition was imposed, and the balancing property was set and satisfied among adopters of CSA innovations. As the selection of CSA innovation discussed in another section here, we only presented the average treatment effect on the treated.
The common support graphing shows that there is substantial overlap between adopters and non-adopters of crop residue management, soil and water conservation, and compost (Figure 3). The imbalance between adopters and non-adopters of these CSA innovations was high in terms of the propensity score before matching. However, the bias was significantly reduced to below 5% after matching. Several variables show significant differences before the matching while after matching they are balanced. Hence, adopters and non-adopters of these CSA innovations have the same distribution in covariates after matching because of low pseudo R2, insignificant value of p of the likelihood ratio test, and the mean standardized bias below 10%. This suggests that there is no systematic difference in the distribution of covariates between both adopters as well as non-adopter groups after matching.
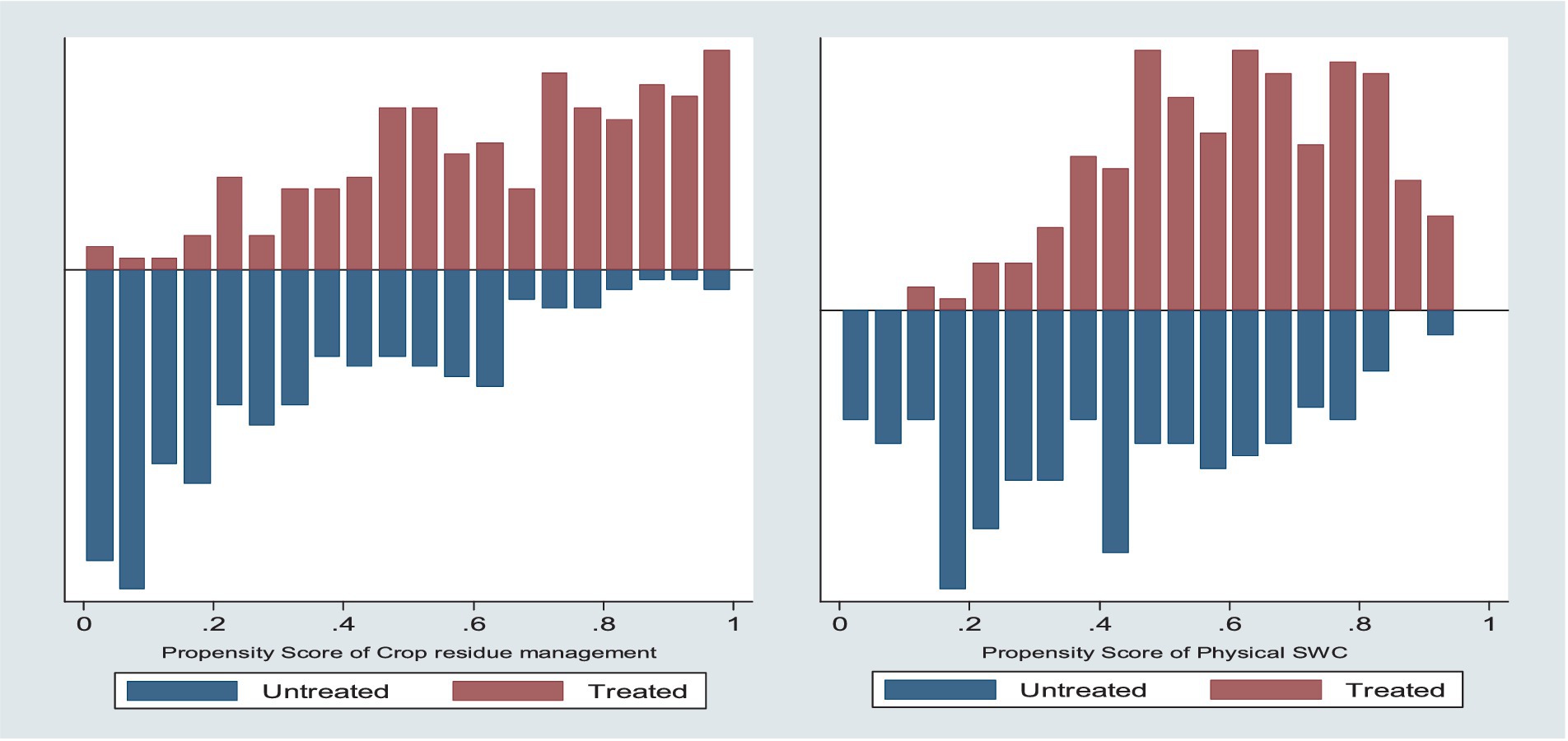
Figure 3. Common support for propensity score estimation of food security for adoption crop residue management and SWC.
Table 5 reports the estimates of the average adoption effects of CSA innovation on the food security of smallholder households estimated by Nearest Neighbor Matching (NNM), Radius matching (RM), Kernel matching (KM), and Stratification matching (SM) methods. The result reveals that households who adopt crop residue management are significantly more food secure (p < 0.01) than non-adopters and adopter household’s food security has increased by 5.62, 8.46, 7.69, and 7.3 points for a matching algorithm of NNM, RM, KM, and SM methods compared to non-adopters. These results are consistent with Mupangwa et al. (2019), who reported that crop residue management enhances food security and agricultural productivity among smallholder farmers in Zambia. On the contrary, households who adopt SWC are significantly less food secure than non-adopters (p < 0.01) as SWC adopter household’s FCS decreased by −6.3, −5.1, −5.44, and −5.9 points for a matching algorithm of NNM, RM, KM, and SM methods compared to non-adopters. However, this result differs from some literature that reports the positive effect of SWC that reported enhanced food security (Zougmoré et al., 2014). It is also consistent with the research (Teshome et al., 2013) which reported that there is significant volatility of profit among adopters of the SWC. In addition, previous research in Ethiopia has also shown that stone bunds are more productive in drier areas than in wetter areas. This result concurs with our finding that our study area receives more than 2000 mm of precipitation annually and is categorized as one of the wetter areas in Ethiopia. Despite all these results, SWC might not be profitable from a private economic point of view but it may be profitable at watershed level (Teshome et al., 2013).
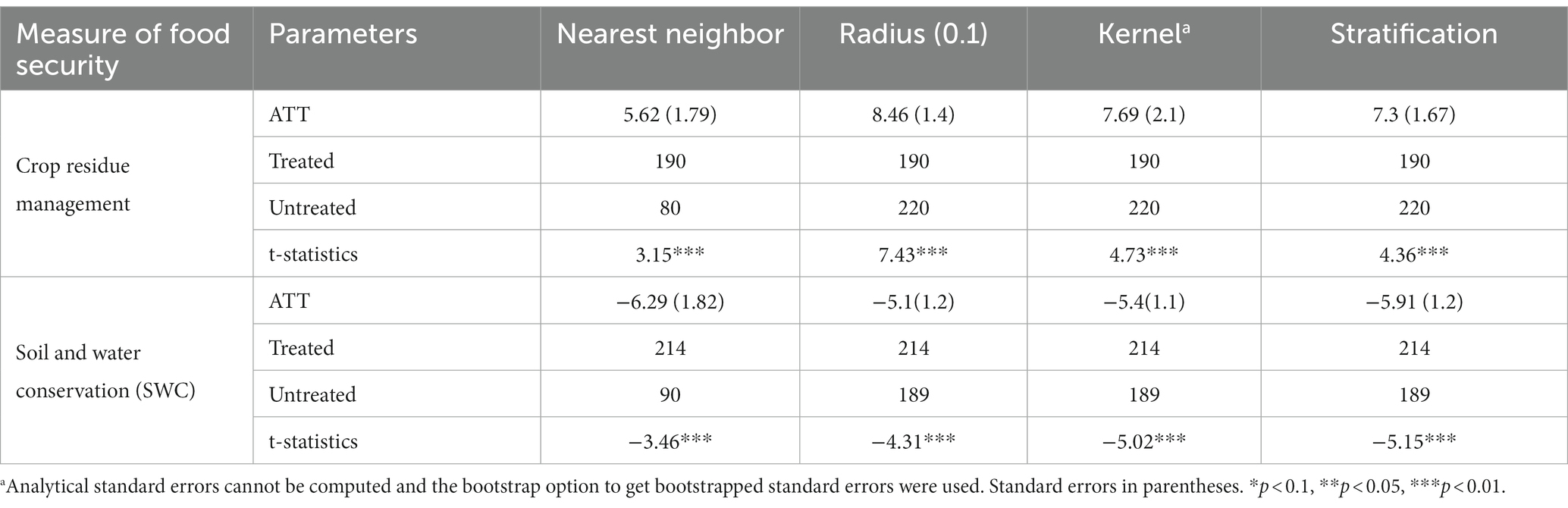
Table 5. Adoption effect of crop residue management and SWC on food security of smallholder households.
Table 6 shows that crop residue management adopters have increased their HDDS by 0.4, 0.276, 0.323, and 0.337 points for the matching algorithm of NNM, RM, KM, and SM methods compared to non-adopters, households who adopt crop residue management are more significantly food secure than non-adopters (p < 0.01). Moreover, the result of HDDS reveals that households who adopt compost and agroforestry are more significantly food secure than non-adopters (p < 0.01). Compost adopter HDDS has increased by 0.462, 0.4, and 0.392 points for the matching algorithm of RM, KM, and SM methods compared to non-adopters while agroforestry adopter HDDS has increased by 0.4, 0.404, and 0.446 points for the matching algorithm of RM, KM, and SM methods compared to non-adopters.
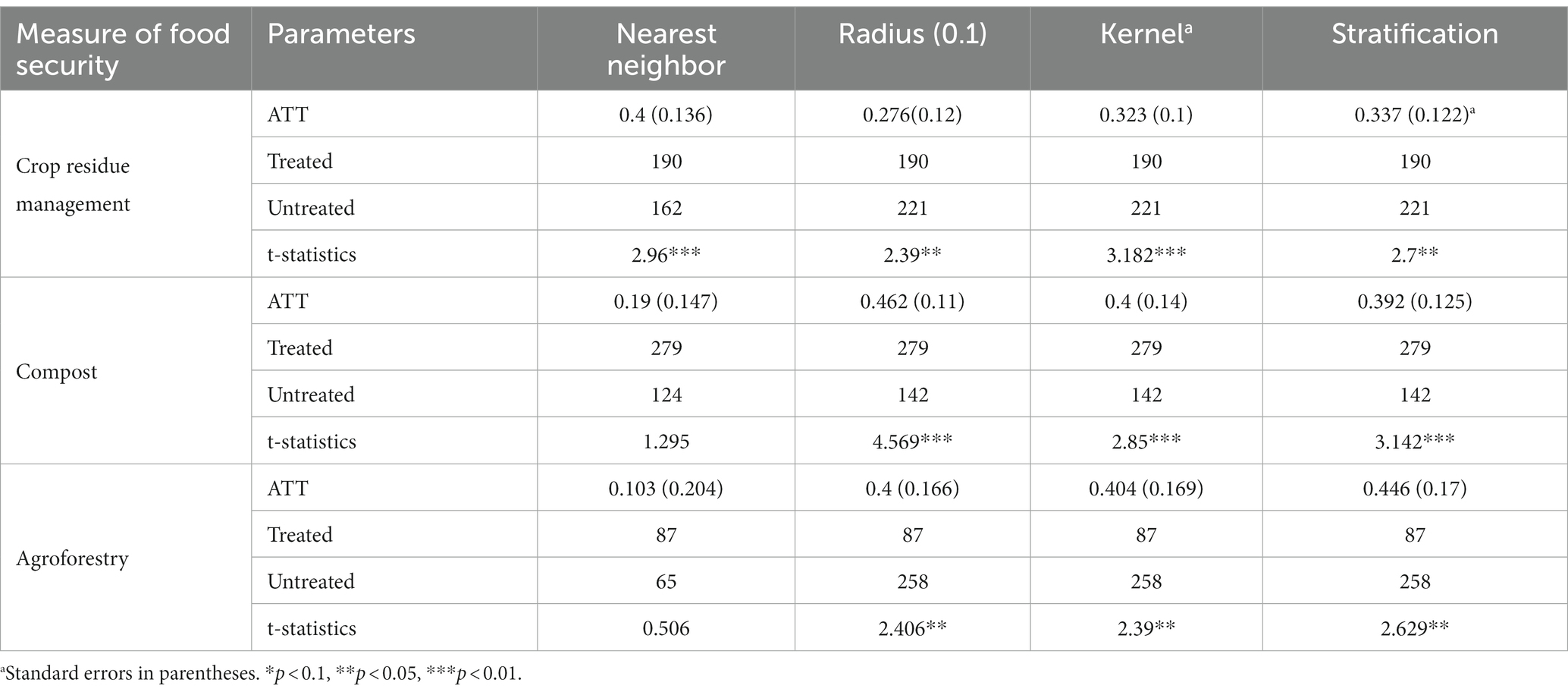
Table 6. Adoption effect of crop residue management, compost, and agroforestry on food security of households.
Since estimating the magnitude of selection bias with non-experimental data is not possible, the study addressed this problem through a method of Rosenbaum bounds sensitivity analysis (Rubin and Rosenbaum, 2006). Hence, the test showed that matching estimators are robust against hidden bias for crop residue management, soil and water conservation, compost, and agroforestry. For interested readers, we will provide the Rosenbaum bounds sensitivity analysis tables.
3.3 Endogenous switching regression results
Table 7 shows the unconditional average adoption effects of CSA innovation on food security (FCS). It indicates that adopters of crop residue management are 21.3% more likely to be food secure than non-adopters whereas adopters of SWC are 12.9% less likely to be food insecure than non-adopters.
Similarly, Table 8 shows that the unconditional average effects on food security (HDDS) indicate that adopters of crop residue management, compost, and agroforestry have 9.5, 13.6, and 16.6% higher household dietary diversity scores than non-adopters which shows crop residue management, compost, and agroforestry adopter households consume more diverse and quality food than non-adopters. However, this naive comparison would drive misleading conclusions because the approach does not consider the difference in the outcome variable caused by observable and unobservable characteristics.
Table 9 presents the true average adoption effects (ATE) of food security (FCS) under actual and counterfactual conditions. In this table, the food consumption score variable of farm households who adopted the crop residue management and SWC were compared with the outcome variables that would have been found if the households had not adopted. To determine the average adoption effects, we compare Columns A and B of Table 9. Column C presents the impacts of the adoption of CSA innovation on food consumption score, computed as the difference between the above columns.
Table 10 shows the true average adoption effects (ATE) of food security (FCS) under actual and counterfactual conditions. The actual adoption of crop residue management, compost, and agroforestry practices has increased the food security of the household.
The current study’s findings on the positive impact of crop residue management, compost, and agroforestry on food security align with existing literature in Mozambique. Similar research conducted in Mozambique has demonstrated that crop residue management, when combined with associated practices, significantly improves food security as measured by the food consumption score (Mango et al., 2017).
Regarding soil and water conservation (SWC) practices in Ethiopia, the literature indicates their effectiveness in reducing surface runoff, nutrient loss, and soil erosion (Bewekt, 2007; Teshome et al., 2013; Simeneh, 2016; Moges and Taye, 2017). However, the impact of SWC practices on crop yield and economic viability is inconsistent and site-specific (Adimassu et al., 2017). For example, the construction of SWC practices like soil and stone bunds initially reduced crop yields, but terraced fields for crops like teff, barley, and maize showed increased crop yields (Kassie and Bluffstone, 2011; Adgo et al., 2013; Adimassu et al., 2014). Additionally, a study conducted in Eastern Ethiopia found that the adoption of SWC not only positively impacted per capita food consumption expenditure and net crop value but also significantly reduced the probability of farmers experiencing food insecurity (Sileshi et al., 2019a,b).
Based on these findings, it is evident that CSA innovations, including crop residue management, compost, and agroforestry, contribute to increased productivity, market access, and food and nutrition security for smallholder farmers. Therefore, policymakers should prioritize nutrition-sensitive CSA initiatives and support interventions that promote diverse and nutritious food production.
4 Conclusion
The study conducted in the Upper Blue Nile Highlands of Ethiopia aimed to examine the impact of Climate-smart agriculture (CSA) innovations on food and nutrition security among smallholder farmers. The researchers used a sample of 424 households from five selected agroecosystems in the region. The food consumption score (FCS) and modified household dietary diversity score (HDDS) were employed as dependent variables in a propensity score matching (PSM) and an endogenous switching regression (ESR) estimation model to assess household food and nutrition security.
The findings of the study indicate that 33% of the households were food secure, 46% were borderline food secure, and 21% were food insecure. The PSM analysis revealed that the adoption of crop residue management, compost, and agroforestry significantly improved food security among smallholder households. Conversely, the adoption of soil and water conservation (SWC) practices reduced the food security status of these households.
Specifically, the adoption of crop residue management, compost, and agroforestry increased food security among adopters by 21.3, 13.6, and 16.6%, respectively. In contrast, the adoption of SWC reduced food security among adopters by 12.9%. However, the ESR analysis, which considers the conditional average effect, demonstrated that the adoption of crop residue management, compost, SWC, and agroforestry improved household food security. Hence, it is important to upscale the adoption of multiple CSA innovations to improve smallholder household’s food security in the face of climate change. Therefore Government and NGOs in a collaborative effort should enhance farmers’ awareness of climate change and climate-smart agriculture innovations through FTC training and Farmer to farmer communication; increase the number of farm demonstrations on the adoption of CSA innovations; enhance the livelihood asset base of smallholder farmers through asset building programs and projects; identify and develop CSA innovations portfolio in terms of synergy and trade-off effect for a particular agroecosystem zone; encourage multiple adoptions of CSA innovations through CSA incentives; provide farmers with vegetable seed, perennials tree seed, and train them to adopt home garden agroforestry systems; and increase the provision of drought resistant and high yield maize and wheat variety.
Data availability statement
The original contributions presented in the study are included in the article/supplementary material, further inquiries can be directed to the corresponding author.
Ethics statement
Ethical review and approval was not required for the study on human participants in accordance with the local legislation and institutional requirements. Written informed consent from the participants was not required to participate in this study in accordance with the national legislation and the institutional requirements.
Author contributions
AT, BS, and MB designed the study and collected and analyzed the research data. All authors contributed to the article and approved the submitted version.
Conflict of interest
The authors declare that the research was conducted in the absence of any commercial or financial relationships that could be construed as a potential conflict of interest.
Publisher’s note
All claims expressed in this article are solely those of the authors and do not necessarily represent those of their affiliated organizations, or those of the publisher, the editors and the reviewers. Any product that may be evaluated in this article, or claim that may be made by its manufacturer, is not guaranteed or endorsed by the publisher.
Footnotes
1. ^Woredas are districts of region.
References
Abate, S., Shiferaw, B., Menkir, A., Wegary, D., Kebede, Y., Tesfaye, K., et al. (2015). Factors that transformed maize productivity in Ethiopia. Food Secur. 7, 965–981. doi: 10.1007/s12571-015-0488-z
Adego, S., Simane, B., and Woldie, G. A. (2019). The impact of adaptation practices on crop productivity in Northwest Ethiopia: an endogenous switching estimation. Dev. Stud. Res. 6, 129–141. doi: 10.1080/21665095.2019.1678186
Adgo, T., Teshome, A., and Mati, B. (2013). Impacts of long-term soil and water conservation on agricultural productivity: the case of Anjenie watershed, Ethiopia. Agric. Water Manag. 117, 55–61. doi: 10.1016/j.agwat.2012.10.026
Adimassu, L., Langan, S., Johnston, R., Mekuria, W., and Amede, T. (2017). Impacts of soil and water conservation practices on crop yield, run-off, soil loss and nutrient loss in Ethiopia: review and synthesis. Environ. Manag. 59, 87–101. doi: 10.1007/s00267-016-0776-1
Adimassu, M., Mekonnen, K., Yirga, C., and Kessler, A. (2014). Effect of soil bunds on runoff, soil, and nutrient losses, and crop yield in the central highlands of Ethiopia. Land Degrad. Dev. 25, 554–564. doi: 10.1002/ldr.2182
Agidie, A., Wassie, H., and Aynekulu, M. (2014). Agroforestry practices and farmers’ perception in koga watershed, upper blue nile basin, Ethiopia. Agric. For. 60, 177–192.
Aryal, J. P., Jat, M. L., Sapkota, T. B., Khatri-Chhetri, A., Kassie, M., Rahut, D. B., et al. (2018). Adoption of multiple climate-smart agricultural practices in the Gangetic plains of Bihar, India. Int. J. Clim. Chang. Strateg. Manag. 10, 407–427. doi: 10.1108/ijccsm-02-2017-0025
Asmare, T., Teklewold, H., and Mekonnen, A. (2019). The effect of climate change adaptation strategy on farm households welfare in the Nile basin of Ethiopia. Int. J. Clim. Chang. Strateg. Manag. 11, 518–535. doi: 10.1108/IJCCSM-10-2017-0192
Asrat, P., and Simane, B. (2018). Farmers’ perception of climate change and adaptation strategies in the Dabus watershed, north-West Ethiopia. Ecol. Process. 7:7. doi: 10.1186/s13717-018-0118-8
Ayugi, E., Eresanya, E. O., Onyango, A. O., Ogou, F. K., Okoro, E. C., Okoye, C. O., et al. (2022). Review of meteorological drought in Africa: historical trends, impacts, mitigation measures, and prospects. Pure Appl. Geophys. 179, 1365–1386. doi: 10.1007/s00024-022-02988-z
Bewekt, W. (2007). Soil and water conservation intervention with conventional technologies in northwestern highlands of Ethiopia: acceptance and adoption by farmers. Land Use Policy 24, 404–416. doi: 10.1016/j.landusepol.2006.05.004
Bewekt, W. (2012). Climate change perceptions and adaptive responses of smallholder farmers in central highlands of Ethiopia. Int. J. Environ. Stud. 69, 507–523. doi: 10.1080/00207233.2012.683328
Chowdhury, H. O., Hambly Odame, H., and Leeuwis, C. (2014). Transforming the roles of a public extension agency to strengthen innovation: lessons from the National Agricultural Extension Project in Bangladesh. J. Agric. Educ. Ext. 20, 7–25. doi: 10.1080/1389224X.2013.803990
Cochran, W. G.Sampling techniques. (1977). Available at: https://scholar.google.com.tr/scholar?q=sampling+techniques&btnG=&hl=en&as_sdt=0,5#0
Deressa, T. T., Hassan, R. M., and Ringler, C. (2011). Assessing household vulnerability to climate change the case of farmers in the Nile Basin of Ethiopia. Int. Food Policy Res. Inst., 1–2.
di Falco, S., Veronesi, M., and Yesuf, M. (2011). Does adaptation to climate change provide food security? A Micro‐perspective from Ethiopia. Am. J. Agric. Econ. 93, 829–846. doi: 10.1093/ajae/aar006
Fentie, A., and Beyene, A. D. (2019). Climate-smart agricultural practices and welfare of rural smallholders in Ethiopia: does planting method matter? Land Use Policy 85, 387–396. doi: 10.1016/j.landusepol.2019.04.020
Haile, T., Tang, Q., Hosseini-Moghari, S.-M., Liu, X., Gebremicael, T. G., Leng, G., et al. (2020). Projected impacts of climate change on drought patterns over East Africa. Earths Future 8, 1–23. doi: 10.1029/2020EF001502
Harvey, S.-R., Saborio-Rodríguez, M., Martinez-Rodríguez, M. R., Viguera, B., Chain-Guadarrama, A., Vignola, R., et al. (2018). Climate change impacts and adaptation among smallholder farmers in Central America. Agric. Food Secur. 7, 1–20. doi: 10.1186/s40066-018-0209-x
IPCC (2019). “Summary for policymakers” in Climate Change and Land: an IPCC special report on climate change, desertification, land degradation, sustainable land management, food security, and greenhouse gas fluxes in terrestrial ecosystems. eds. P. R. Shukla, J. Skea, E. Calvo Buendia, V. Masson-Delmotte, H.-O. Pörtner, and D. C. Roberts, et al. (Cambridge: Cambridge University Press)
Kassie, K., and Bluffstone, H. (2011). Are soil conservation technologies “win-win?” A case study of Anjeni in the north-western Ethiopian highlands. Nat. Resourc. For. 35, 89–99. doi: 10.1111/j.1477-8947.2011.01379.x
Kassie, J., Jaleta, M., Shiferaw, B., Mmbando, F., and Mekuria, M. (2013). Adoption of interrelated sustainable agricultural practices in smallholder systems: evidence from rural Tanzania. Technol. Forecast. Soc. Change. 80, 525–540. doi: 10.1016/j.techfore.2012.08.007
Kato, R., Ringler, C., Yesuf, M., and Bryan, E. (2011). Soil and water conservation technologies: a buffer against production risk in the face of climate change? Insights from the Nile basin in Ethiopia. Agric. Econ. 42, 593–604. doi: 10.1111/j.1574-0862.2011.00539.x
Knowler, B., and Bradshaw, B. (2007). Farmers’ adoption of conservation agriculture: a review and synthesis of recent research. Food Policy 32, 25–48. doi: 10.1016/j.foodpol.2006.01.003
Kotir, J. H. (2011). Climate change and variability in sub-Saharan Africa: a review of current and future trends and impacts on agriculture and food security, environ. Dev. Sustain. 13, 587–605. doi: 10.1007/s10668-010-9278-0
Long, B., Blok, V., and Coninx, I. (2016). Barriers to the adoption and diffusion of technological innovations for climate-smart agriculture in Europe: evidence from the Netherlands, France, Switzerland and Italy. J. Clean. Prod. 112, 9–21. doi: 10.1016/j.jclepro.2015.06.044
Makate, C. (2019). Local institutions and indigenous knowledge in adoption and scaling of climate-smart agricultural innovations among sub-Saharan smallholder farmers. Int. J. Clim. Chang. Strateg. Manag. 12, 270–287. doi: 10.1108/IJCCSM-07-2018-0055
Makate, M., Makate, M., Mutenje, M., Mango, N., and Siziba, S. (2019). Synergistic impacts of agricultural credit and extension on adoption of climate-smart agricultural technologies in southern Africa. Environ. Dev. 32:100458. doi: 10.1016/j.envdev.2019.100458
Mango, S., Siziba, S., and Makate, C. (2017). The impact of adoption of conservation agriculture on smallholder farmers’ food security in semi-arid zones of southern Africa. Agric. Food Secur. 6, 1–8. doi: 10.1186/s40066-017-0109-5
Maxwell, D., Vaitla, B., and Coates, J. (2014). How do indicators of household food insecurity measure up? An empirical comparison from Ethiopia. Food policy 47, 107–116. doi: 10.1016/j.foodpol.2014.04.003
Melak, B. Food insecurity in Ethiopia: population, food production, and market, in 32nd International Conference of the System Dynamics Society, Delft (2014), 1–29.
Melisse, B. (2018). A review on factors affecting adoption of agricultural new technologies in Ethiopia. J. Agric. Sci. Food. Res. 9, 1–6.
Mihretie, M., Misganaw, G. S., and Siyum Muluneh, N. (2022). Adoption status and perception of farmers on improved Tef technology packages: evidence from east Gojjam zone, Ethiopia. Adv Agric 2022:6121071. doi: 10.1155/2022/6121071
Mittal, M. (2012). How do mobile phones contribute to the growth of small farmers? Evidence from India. Q. J. Int. Agric. 51, 227–244.
Moges, D. M., and Taye, A. A. (2017). Determinants of farmers’ perception to invest in soil and water conservation technologies in the North-Western highlands of Ethiopia. Int. Soil Water Conserv. Res. 5, 56–61. doi: 10.1016/j.iswcr.2017.02.003
Mujeyi, M., Mudhara, M., and Mutenje, M. (2021). The impact of climate smart agriculture on household welfare in smallholder integrated crop–livestock farming systems: evidence from Zimbabwe. Agric. Food Secur. 10, 1–15. doi: 10.1186/S40066-020-00277-3
Mupangwa, M., Mutenje, M., Thierfelder, C., Mwila, M., Malumo, H., Mujeyi, A., et al. (2019). Productivity and profitability of manual and mechanized conservation agriculture (CA) systems in eastern Zambia. Renew. Agric. Food Syst. 34, 380–394. doi: 10.1017/S1742170517000606
Murage, A. W., Obare, G., Chianu, J., Amudavi, D. M., and Kha, Z. R. (2012). The effects of dissemination pathways on the speed of push-pull technology adoption in Western Kenya. Q. J. Int. Agric. Int. Agric. 51, 51–71.
Murray, G., Gebremedhin, Z., Brychkova, G., and Spillane, C. (2016). Smallholder farmers and climate smart agriculture: technology and labor-productivity constraints amongst women smallholders in Malawi. Gend. Technol. Dev. 20, 117–148. doi: 10.1177/0971852416640639
Ntshangase, M., Muroyiwa, B., and Sibanda, M. (2018). Farmers’ perceptions and factors influencing the adoption of no-till conservation agriculture by small-scale farmers in Zashuke, KwaZulu-Natal Province. Sustainability 10:555. doi: 10.3390/su10020555
Rubin, D. B., and Rosenbaum, P. R. (2006). The central role of the propensity score in observational studies for causal effects. Match. Sampl. Causal Effect., 170–184. doi: 10.1017/CBO9780511810725.016
Sardar, K., Kiani, A. K., and Kuslu, Y. (2021). Does adoption of climate-smart agriculture (CSA) practices improve farmers’ crop income? Assessing the determinants and its impacts in Punjab province, Pakistan. Environ. Dev. Sustain. 23, 10119–10140. doi: 10.1007/S10668-020-01049-6
Shiferaw, K., Kassie, M., Jaleta, M., and Yirga, C. (2014). Adoption of improved wheat varieties and impacts on household food security in Ethiopia. Food Policy 44, 272–284. doi: 10.1016/j.foodpol.2013.09.012
Sileshi, K., Kadigi, R., Mutabazi, K., and Sieber, S. (2019a). Determinants for adoption of physical soil and water conservation measures by smallholder farmers in Ethiopia. Int. Soil Water Conserv. Res. 7, 354–361. doi: 10.1016/j.iswcr.2019.08.002
Sileshi, K., Kadigi, R., Mutabazi, K., and Sieber, S. (2019b). Impact of soil and water conservation practices on household vulnerability to food insecurity in eastern Ethiopia: endogenous switching regression and propensity score matching approach. Food Secur. 11, 797–815. doi: 10.1007/s12571-019-00943-w
Simane, B. F., Zaitchik, B. F., and Foltz, J. D. (2016). Agroecosystem specific climate vulnerability analysis: application of the livelihood vulnerability index to a tropical highland region. Mitig Adapt Strat. Glob Chang 21, 39–65. doi: 10.1007/s11027-014-9568-1
Simeneh, F. (2016). Perception of farmers toward physical soil and water conservation structures in Wyebla watershed, Northwest Ethiopia, world. J. Agric. Sci. 1, 57–63. doi: 10.5829/idosi.ajps.2015.7.3.12822
Teklewold, M., Kassie, M., and Shiferaw, B. (2013). Adoption of multiple sustainable agricultural practices in rural Ethiopia. J. Agric. Econ. 64, 597–623. doi: 10.1111/1477-9552.12011
Teklewold, H., Mekonnen, A., and Kohlin, G. (2019). Climate change adaptation: a study of multiple climate-smart practices in the Nile Basin of Ethiopia. Clim. Dev. 11, 180–192. doi: 10.1080/17565529.2018.1442801
Teklewold, H., Mekonnen, A., Kohlin, G., and di Falco, S. (2017). Does adoption of multiple climate-SMART practices improve farmers’ climate resilience? Empirical evidence from the Nile basin of Ethiopia. Clim. Chang. Econ. 8:1750001. doi: 10.1142/S2010007817500014
Teklu, S., Simane, B., and Bezabih, M. (2022). Effectiveness of climate-smart agriculture innovations in smallholder agriculture system in Ethiopia. Sustainability 14:16143. doi: 10.3390/su142316143
Teklu, S., Simane, B., and Bezabih, M. (2023a). Effect of climate smart agriculture innovations on climate resilience among smallholder farmers: empirical evidence from the Choke Mountain Watershed of the blue nile highlands of Ethiopia. Sustainability 15:4331. doi: 10.3390/su15054331
Teklu, S., Simane, B., and Bezabih, M. (2023b). Multiple adoption of climate-smart agriculture innovation for agricultural sustainability: empirical evidence from the Upper Blue Nile highlands of Ethiopia. Clim. Risk Manag. 39:100477. doi: 10.1016/j.crm.2023.100477
Tesfaye, B., Blalock, G., and Tirivayi, N. (2021). Climate-smart innovations and rural poverty in Ethiopia: exploring impacts and Pathways. Am. J. Agric. Econ. 103, 878–899. doi: 10.1111/ajae.12161
Tesfaye, K., Kassie, M., Cairns, J. E., Michael, M., Stirling, C., Abate, T., et al. (2017). Potential for scaling up climate smart agricultural practices: examples from sub-Saharan Africa. Clim. Change Adapt. Afr., 185–203. doi: 10.1007/978-3-319-49520-0_12
Tesfaye, S., and Seifu, L. (2016). Climate change perception and choice of adaptation strategies: empirical evidence from smallholder farmers in east Ethiopia. Int. J. Clim. Chang. Strateg. Manag. 8, 253–270. doi: 10.1108/IJCCSM-01-2014-0017
Teshome, A., de Graaff, J., and Kassie, M. (2016). Household-level determinants of soil and water conservation adoption phases: evidence from North-Western Ethiopian highlands. Environ. Manag. 57, 620–636. doi: 10.1007/s00267-015-0635-5
Teshome, A., Rolker, D., and de Graaff, J. (2013). Financial viability of soil and water conservation technologies in northwestern Ethiopian highlands. Appl. Geogr. 37, 139–149. doi: 10.1016/j.apgeog.2012.11.007
Trisos, C., Adelekan, I. O., and Edmond, T. (2022). “IPCC WGII sixth assessment report: Africa” in Climate change
Wekesa, B. M., Ayuya, O. I., and Lagat, J. K. (2018). Effect of climate-smart agricultural practices on household food security in smallholder production systems: micro-level evidence from Kenya. Agric. Food Secur. 7:80. doi: 10.1186/s40066-018-0230-0
Wolka, K., Mulder, J., and Biazin, B. (2018). Effects of soil and water conservation techniques on crop yield, runoff and soil loss in sub-Saharan Africa: a review. Agric. Water Manag. 207, 67–79. doi: 10.1016/j.agwat.2018.05.016
Zeng, D., Alwang, J., Norton, G., Jaleta, M., Shiferaw, B., and Yirga, C. (2018). Land ownership and technology adoption revisited: improved maize varieties in Ethiopia. Land Use Policy 72, 270–279. doi: 10.1016/j.landusepol.2017.12.047
Zerihun, M., Muchie, M., and Worku, Z. (2014). Determinants of agroforestry technology adoption in eastern Cape Province, South Africa. Dev. Stud. Res. 1, 382–394. doi: 10.1080/21665095.2014.977454
Keywords: food and nutrition security, CSA innovations, crop residue management, compost, SWC, agroforestry, endogenous switching regression, Ethiopia
Citation: Teklu A, Simane B and Bezabih M (2024) Climate smart agriculture impact on food and nutrition security in Ethiopia. Front. Sustain. Food Syst. 7:1079426. doi: 10.3389/fsufs.2023.1079426
Edited by:
Eduardo Aguilera, Centro de Estudios e Investigación para la Gestión de Riesgos Agrarios y Medioambientales (CEIGRAM), SpainReviewed by:
Mojisola Olanike Kehinde, Landmark University, NigeriaTony Carr, University of London, United Kingdom
Copyright © 2024 Teklu, Simane and Bezabih. This is an open-access article distributed under the terms of the Creative Commons Attribution License (CC BY). The use, distribution or reproduction in other forums is permitted, provided the original author(s) and the copyright owner(s) are credited and that the original publication in this journal is cited, in accordance with accepted academic practice. No use, distribution or reproduction is permitted which does not comply with these terms.
*Correspondence: Abyiot Teklu, YWJ5aW90LnRla2x1QGFhdS5lZHUuZXQ=