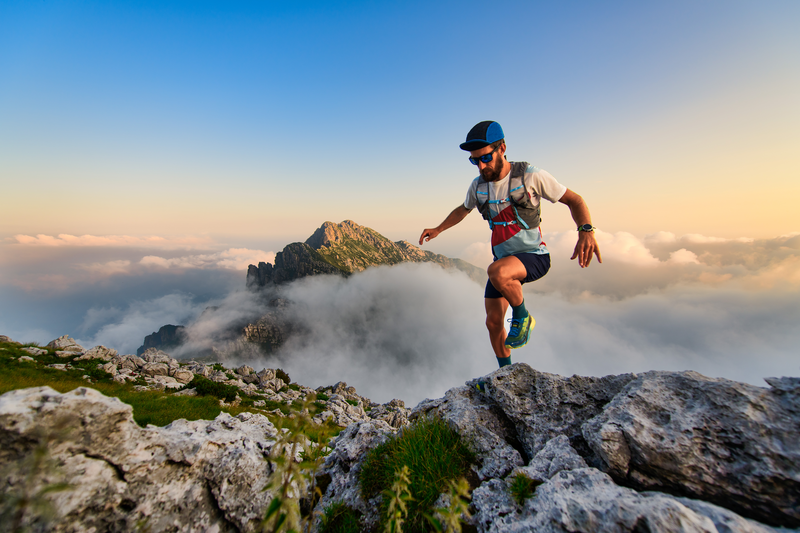
94% of researchers rate our articles as excellent or good
Learn more about the work of our research integrity team to safeguard the quality of each article we publish.
Find out more
REVIEW article
Front. Sustain. Food Syst. , 06 April 2023
Sec. Agroecology and Ecosystem Services
Volume 7 - 2023 | https://doi.org/10.3389/fsufs.2023.1073339
Striga species are obligate parasitic weeds most of which are members of the Orobanchaceae family. They are commonly associated with staple crops and constitute threats to food security, especially in Sub-Saharan Africa. They pose deleterious impacts on staple cereal crops like maize and pearl millet, resulting in 7–10 billion dollars yield losses or, in extreme infestations, entire crop losses. Farmers' limited knowledge about the weed (genetics, ecology, nature of the damage caused, complex life cycle, interactions with its host and associated microbes) and their attitude toward its control have negatively affected its management and sustainability. With the present Striga management such as mechanical, chemicals, cultural and biological measures, it is extremely difficult to achieve its active management due to nature of the association between host plants and parasites, which requires highly selective herbicides. The use of soil microbes has not been well explored in the management of Striga infection in African countries. However, many soil microorganisms have been considered viable biological control techniques for fighting parasitic weeds, due to their vast action and roles they play in the early stage of host-Striga interaction. Their application for pest control is well perceived to be cost-effective and eco-friendly. In this review, we gave a comprehensive overview of major knowledge gaps and challenges of smallholders in Striga management and highlighted major potentials of microbial-based approach with respect to the mechanisms of host-Striga-microbe interactions, and the metagenomics roles on Striga management that include understanding the microbe and microbial systems of Striga-infested soil.
Parasitic weeds in the genus Striga have constituted a major threat to the cultivation of cereal crops such as sorghum, maize, pearl millet, and rice. Striga poses an imminent threat to food security because of up to 90% production loss it causes (Mounde et al., 2020). Striga gesneriodes are classified as holoparasites since they depend solely on the host plants (i.e dicots) for nutrients, water, and carbon since they lack chlorophyll (Irving and Cameron, 2009). However, some other Striga species including Striga hermontica are obligate hemiparasite with the ability to photosynthesize; they fully depend on their host which is mainly cereals crops for nutrients and water, and partially for their carbon requirements. Striga survival depends on its ability to siphon nutrients and water from host crops to aid its own growth and development. The species have 5–7 days to attach to a suitable host for them to be established, or else the radicles will wither if their stored resource in the seeds is depleted (Mwangangi et al., 2021). Striga thus impairs the host plants via penetration and colonization of their root cells, thereby degrading the host function and productive values even before it emerges from the ground (Figure 1). Because of the further resource demand, Striga transitions from an independent to a host-dependent parasitic state. A parasitic association is created once Striga sp. successfully attaches to the host, in which it acts as a sink for the host's metabolites and water. Two separate techniques of resource retrieval are maintained, the first method is its unique capacity to maintain a constant high stomatal conductance, while the second technique is the accumulation of large quantities of osmotically active substances such as mineral ions, sugars, and alcohols such as mannitol (Shen et al., 2006).
Figure 1. Scheme showing the establishment and life cycle of Striga spp. (Striga asiatica (L.) Kuntze).
Striga produce a large number of seeds, which can remain dormant for a long time, for instance, Striga hermontica produces up to 200,000 seeds and S. asiatica about 58,000 (Dafaallah and Babiker, 2016). The seeds are lightweight (~4–7 μg per seed) and are easily dispersed through the wind, agricultural tools and animals, which enrich the seed reserve in the soil. More so, they only germinate after exposure to hot and humid environments, and upon the secretion of strigolactones which is the germination stimulants produced by the host (Jamil et al., 2021). Infestation of Striga spp. is therefore prevalent in places with low soil fertility and rainfall, as well as in agricultural systems that practices intensive cultivation, poor crop management, or shortage of agricultural inputs (Ejeta, 2007).
In Sub-Sahara Africa (SSA), Striga are of food security concerns as they pose a deleterious impact on the production of staple cereal crops like maize and pearl millet. It results in 7–10 billion dollars yield losses, and could lead to entire crop losses in extreme infestations (Kountche et al., 2019). The production of cereal crops in SSA between 2017 and 2019 amounted to approximately 141 metric tons; however, Striga infestation reduced yield to as little as 1,000 kg ha−1, making SSA one of the world's lowest producers (Kanampiu et al., 2018). Up till now, Striga has not been sustainably managed as a result of its peculiar nature which includes the production of large number of seeds, longevity, easy dispersal, variations in their genetic makeup, and the nature of damage caused (Spallek et al., 2013).
Understanding of Striga life cycle is essential in developing a long-term control strategy as this allows selection of most critical stage(s) that could be targeted in the design of novel control approach. Although, farmers' reluctance to embrace approved weed management in most instances has worsened the Striga problem, especially in Africa. One of such situations is the consistent monocropping that results in high infestation and accumulation of Striga seeds in the soil (Midega et al., 2017). The existing control measures of Striga can be employed either as individual or integrated treatments. Some soil microbes have been reported to significantly cause reduction in Striga germination, emergence, and attachment (Hassan et al., 2009; Jamil et al., 2021). Some earlier studies have reported arbuscular mycorrhizal fungi (AMF) for their potential in the management of Striga infested fields (Birhane et al., 2018). They do not only promote growth and control Striga infection, but also participate in the absorption of phosphorus (P), micronutrients, and water from soil through extraradical hyphae (Aggarwal et al., 2011). However, there occur conficting views on the Striga and AMF relationship as another study reported the stimulation of AMF spore germination by strigolactones produced as root exudates of Striga, thereby suggesting the dependency of AMF growth on Striga (Steinkellner et al., 2007).
Researchers, with the advancements in the “omics” technologies have been able to better understand the diversity of soil microbial communities and their functional traits, which are significant ways of defining microbiological parameters (Zhou et al., 2022). Analysis of functional microbial diversity has shown how adaptable microorganisms interact with their environment, identifying trophic linkages, microbes interaction, their participation in biogeochemical cycles, and their responses to environmental changes. The rapid advancement of sequencing technologies, combined with bioinformatic tools enhances the feasibility of large-scale microbial ecology investigations, which also allows a better understanding of the composition and roles of microbes in different ecosystems (Tedersoo et al., 2021).
Striga species often affect various crops, especially cereal crops, and there have been significant efforts made to fully understand their controls. However, no single method of control has been found to be sufficiently effective (Mounde et al., 2020). Conventional approach of weed management is an age-long practice which entails the diversification agricultural operations to reduce dependency on chemical inputs while maintaining buoyant crop outputs and efficient ecosystem services (Rao et al., 2017). Weeding using hand tools are other common conventional method of managing Striga in SSA since majority of the farmers are smallholders. Also, the intercropping of cereal with legumes has been widely engaged to enhance soil fertility in relation to N2 fixation. Other conventional approaches are land fallowing and crop rotation (Kerr et al., 2007). Integrated management of Striga is commonly advocated for and entails the combination of management methods that can work synergistically. Such include the use of Striga-resistant cultivars, arbuscular mycorrhizal fungi (AMF), mycoherbicidal biocontrol, improved tillage, intercropping with legumes, and fertilizer inputs (Babalola and Odhiambo, 2008; Bàrberi, 2019). Cultural methods such as rotation and cultivar selection in weed management are well established in many agricultural systems. However, alternative control measures are necessary, including biological control, which is promising, cost-effective, and safe for the environment.
Mycoherbicides are biological agents that have high host specificity, aggression, and ease of mass manufacturing, long storage life, and genetic diversity that make them effective against Striga (Babalola, 2010b; Zarafi and Dauda, 2019). In recent time, attention have been focused on fungal microorganisms as mycoherbicides against Striga, where Fusarium oxysporum f. f.sp. Strigae (Fos) strain “Foxy-2” has proven to reduce the establishment of Striga spp. by 95% while increasing sorghum yields by approximately 50% (Babalola and Odhiambo, 2008). Bàrberi (2019) confirmed the effectiveness of mycoherbicides developed from Fusarium oxysporum to inhibit Striga which decreases its seed bank in infected soils and its adhesion to cereals. High amounts of the poisonous amino acids L-tyrosin and L-leucine, which are toxic to Striga but not to maize are produced by some F. oxysporum and this interferes with the strictly regulated homeostasis of free amino acids. Also, soil microbes convert methionine secreted by F. oxysporum strains into germination stimulant ethylene which results in Striga seeds germinating suicidally (Babalola et al., 2007; Jamil et al., 2021; David et al., 2022). Watson (2013) proposed that Striga treatment could be accomplished in the field by seed coating with F. oxysporum (FOXY2). It was observed that for easy application and duration, FOXY2 and PSM197 were combined in a granular formulation and Striga emergence was reduced up to 75% in sorghum and maize crops (Beed et al., 2007). However, despite the significance of this findings, F. oxysporum has not been commercially employed by farmers in managing Striga infested field because of its host specificity. The host specificity of most Fusarium species has been recorded at the genus or species level. An instance of this is the efficacy of Isolate Foxy 2 against S. hermonthica and S. asiatica in the screenhouse trials. Also, the high genetic variability of Striga spp. may impact the efficacy of the F. oxysporum-bioherbicide. Therefore, to establish the effectiveness of F. oxysporum f. sp. striga isolates, there is need for its evaluations against multiple Striga populations from different hosts and across different environments, whereas, the vegetative compatibility grouping pattern of the isolates of Fusarium species differs in relation to their host range (Beed et al., 2007). Furthermore, the ineffectiveness of the organism as a single control method could discourage its widespread application, as no single control method has been found successful in managing the Striga menace (Joel, 2000). This thereby showcasee a gap in the knowledge of Striga biology, its host-parasite interaction and the genetic basis of host resistance. Whereas, a combination of methods have proved effective, an instance of such was the combined application of Fusarium oxysporum-based mycoherbicide and host resistance which significantly reduced Striga prevalence and increased crop yield (Mrema et al., 2020).
Several studies have indicated the potential of AMF in alleviating Striga infection. The AMF enhance cereal growth to withstand Striga damage and facilitate host plant's uptake of micronutrients, water, and phosphorus (P) from soil through extraradical fungal hyphae (Olowe et al., 2018). The strigolactones (SLs) exudation by the host in the soil is ultimately reduced by the increased uptake of P via symbiotic interactions by AM fungi, thereby reducing Striga infection (Aquino et al., 2021). Furthermore, rate of Striga infestation is equally lowered by the soil microbes through their release of amino acids such as methionine or by the producing secondary metabolites such as trichothecenes which may interfere with SL perception. Also, there is evidence of fungal degradation by Strigolactone, which may result to decrease in Striga germination and the release of root exudates (Jamil et al., 2021) (Table 1). Soil microbes are potential biological control tools for combating Striga because of their cost-effectiveness and eco-friendliness approach. However, abiotic and biotic factors under field conditions can alter the efficacy of this approach (Berner et al., 2003).
During a screen house trial, four PGPR inhibited Striga infestation in sorghum, and the strain Bacillus subtilis GBO3 resulted in the death of 35%-59% of Striga tubercles emergence and a 23% decrease in Striga attachment (Samejima and Sugimoto, 2018). In another study, the significant decrease of Striga seed germinating activity of sorghum root exudates following treatment with Pseudomonas suspensions, may have resulted from SLs degradation (Jamil et al., 2021). As shown for some isolates of Bacillus, Streptomyces and Rhizobium genera, the production of compounds with antibiotic activity and of extracellular enzymes, such as xylanases, pectinases, and amylases, can directly cause Striga seed decay (Neondo et al., 2017). Soil microbe could also inhibit Striga by releasing amino acids such as methionine or through secondary metabolites production such as β-lactone derivatives that may affect SL perception. SL was found to be degraded by fungi in several investigations, which may have a negative impact on the ability of produced exudates to germinate Striga (Brun et al., 2018). PGPR, AMF and strains of bacteria are considered to be economical, promising and environmentally friendly (Babalola, 2010a; Le Mire et al., 2016). However, the effectiveness of this approach can be affected by a number a of stress factors under field conditions. Thus, the choice of inoculum media, suitable formulations, storage, mass production, shelf life, consistency, compatibility with the host plants, and maintenance of their activity in infected soils are to be taken in to consideration (Vassileva et al., 2021). In-depth study and field-testing under various climatic and environmental circumstances are still necessary for the validation and further development of this microbes-based biocontrol strategy.
The tripartite interaction of the cereals, Striga spp. and rhizospheric microorganisms such as arbuscular mycorrhizal fungi (AMF) and PGPR, has not been sufficiently researched despite the scientific exploration of their bipartite relationship (Mounde et al., 2020). Whereas the in-depth ecological knowledge of this interaction will open up opportunities to create Striga species-specific management strategies. The interaction of AMF, a cereal host crop and Striga spp. is one of the most suitable references of the tripartite interaction researched thus far, and is evident in the reduced germination and emergence of Striga spp. in an AMF treated sorghum field (Bàrberi, 2019; Mwangangi et al., 2021). Strigolactone is a multifunctional plant hormone that is involved in a variety of internal and external functions in plants including the promotion of arbuscular mycorrhizal symbiosis, stimulating the growth of parasitic plants, determining plant architecture and acting as a developmental and environmental signal (Torres-Vera et al., 2014). The Important plant recognition cues for AMF have been reported as the strigolactones present in root exudates and causing hyphal branching in AMF (Basu et al., 2018). The root exudates of mycorrhizal host and nonhost plant species contain at least nine strigolactones, including strigol, sorgolactone, orobanchol, strigyl acetate, 5-deoxystrigol, orobanchyl acetate, epi-orobanchol, sorgomol, and solanacol (Soto-Cruz et al., 2021). The host plant produces more strigolactones to promote the growth of fungi and the establishment of symbioses under the nutrient deficient conditions. More so, some species, in particular root parasitic plants, are able to detect strigolactones as a signal of host presence in the rhizosphere and as a result promotes a parasitic association (López-Ráez et al., 2011). In line with some earlier reports, the effect of strigolactone on the later stage of the AMF—plant interaction is dependent on the plant type involved, as further root colonization by AMF is reduced in mycorrhizal plants compared to the non-mycorrhizal plants. Whereas, the inhibition in the rate of Striga spp. germination has been associated to the reduction in striglactone production caused by the structural and chemical alterations in the roots of AMF-colonized plants that disrupts strigolactone exudation patterns, thereby leading to poor stimulation of Striga spp. seed germination (Steinkellner et al., 2007; Abdelhalim et al., 2019). This can be further affirmed in some earlier reports of the root exudates of the mycorrhizal maize and sorghum plants which induced lower Striga seed germination when compared to the control plants. Similarly, the in vitro branching of AMF was more stimulated by the root exudates from non-mycorrhizal cucumber plants than by exudates from the mycorrhizal cucumber plants (Steinkellner et al., 2007; Fernández-Aparicio et al., 2011).
Certain plant families, such as the Brassicaceae, Chenopodiaceae, and lupins (an exception in the mycorrhizal host family of the Leguminosae), are listed as mycorrhizal nonhost plants, despite the fact that the majority of terrestrial plants are hosts for mycorrhizal fungi. While some data suggest that some mycorrhizal nonhost plants' root exudates include substances that are antagonistic to mycorrhizal fungi, other data suggest that other mycorrhizal nonhost plants' root exudates lack crucial signals for mycorrhizal fungi to colonize their roots (García-Garrido et al., 2009; Wang et al., 2022).
Adequate crop nutrition, particularly phosphate, have been found to inhibit strigolactone exudation, with a minimal impact on the germination and parasitism of Striga spp. (Chesterfield et al., 2020). Additionally, AMF enhances crop phosphorus absorption with favorable response on plant growth and health, thereby partially offsetting the parasitic impacts of Striga spp. The secretion of some detrimental hormones by Striga spp. such as auxins and ABA are mitigated by AMF, hence, it is possible that root parasitic weeds have developed a method to intercept the communication signal and transform it into a germination-inducing signal to react when a suitable host is present (Mounde et al., 2020). This is validated by the study of Mutsvanga et al. (2022) which reported higher chlorophyll contents in plants treated with AMF. Also, upon AMF inoculation, the physiological indicators including stomatal conductivity and photosynthetic rate was reportedly improved, while the biomass of Striga spp. decreased. This was attributed to the host plant that had been colonized by AMF thereby converting strigolactones to mycoradicin, whereas mycoradicin does not support the emergence and germination of Striga spp. (Manjunatha et al., 2018). Conversely, strigolactones production have been reported to stimulate the spore germination of AMF, and thereby aids in AMF growths (Besserer et al., 2006).
Strigolactones have been found in the root exudates of wide range of plants, and they do not only serve as signals for AMF but also for other fungi, especially soilborne fungi.(García-Garrido et al., 2009). According to Ahonsi et al. (2002), Pseudomonas fluorescens and Pseudomonas putida can prevent Striga spp. from emerging when maize hosts are present. This was supported by the report that certain Pseudomonas species, either alone or in combination with N2-fixing Bradyrhizobium japonicum, reduced Striga spp. infestation in cowpea and soybean rhizospheres (Ahonsi et al., 2003). After being treated with Azospirillum brasilense, Striga spp. germination was inhibited, whereas development of the host crop (sorghum) was encouraged (Abbes et al., 2019). Similar results showing the favorable benefits of certain PGPR (such as Pseudomonas putida and Bacillus sp.) against Striga spp. while encouraging the growth of sorghum was reported by Babalola (2010b), more so auxin and auxin-like chemicals generated by PGPR were shown to suppress Striga spp. emergence and haustorium development.
Striga spp. infested crops typically have low IAA levels (Mounde et al., 2020). Hassan et al. (2009) demonstrated that the inoculation of PGPR (such as Azospirillum brasilense, Pseudomonas putida, Bradyrhizobium japonicum and Azospirillum amazonas) delayed and reduced the incidence of Striga spp. for both resistant and susceptible sorghum varieties to counteract such low IAA levels. Bacillus subtilis GBO3 and Bacillus amyloliquefaciens FZB42 were reported to support sorghum growth while preventing the germination of Striga hermonthica (Mounde et al., 2015). The two PGPR's ability to reduce tubercle development as compared to non-inoculated controls provides evidence of their bioherbicidal properties. Some unexplained metabolites, which may compete with the germination stimulants for binding sites, have been postulated to be the origin of seed germination suppression (e.g., strigolactone). Strigolactone is predominantly generated by the host to promote AMF branching and root attachment and to control its above-ground architecture i.e., shoot branching (Jamil et al., 2011). The receptive site DWARF14 hydrolyzes the strigolactone molecule in strigolactone-dependent plants. The repressor DELLA protein interacts with DWARF14 when stigolactone is present (i.e., DWARF53) (Hu et al., 2017). The 26S proteasome breaks down this repressor protein in a combination with strigolactone, DWARF14, DWARF53, and Skp1-Cullin-F-box protein (SCF), which eventually leads to the germination of Striga spp. (Kaniganti et al., 2022). The DWARF14 protein is disrupted by mutation or other methods (such as PGPR-derived inhibitors), which prevents the receptor from transducing the strigolactone signal (Mounde et al., 2020). A new chemical called DL1 that is a powerful inhibitor of the strigolactone-receptive site DWARF14 was identified. Understanding the specific metabolic substances generated by PGPR, which may successfully inhibit DWARF14, would increase the effectiveness of the recommended PGPR metabolic bioherbicidal method in controlling Striga spp. (Mounde et al., 2020) (Table 1).
The genomic assemblages of microbes isolated directly from their environment, without the requirement for preliminary growing under laboratory conditions, are referred to as metagenomics (Handelsman, 2004). Metagenomics provides a generally unbiased assessment of a community's functional (metabolic) capacity as well as its structure (species richness and distribution) (Hugenholtz and Tyson, 2008; Olowe et al., 2023). The DNA extracted from the environment eliminates the necessity for single isolate culturing. In this case, the diversity of microbial strains recovered from the environment increases when DNA is extracted directly from the environment. Metagenomics comprises of parallel sequencing of microbial metagenomes while functional screens can be used to find clones that have genes that code for biosynthesis of natural products and secondary metabolites, which are usually phytotoxic, enzymes and antimicrobial agents that can be used in weed management (Kao-Kniffin et al., 2013).
Metagenomics approaches have been employed by chemical and pharmaceutical industries to screen for novel enzymes and antibiotics. Similar functional screens can be used to find weed-suppressive chemicals that target a wide range of plant stages. The sheer diversity of bacterial and fungal genomes in soil provides a reservoir of genes that code for herbicidal chemical synthesis and resistance to herbicides. As a result, metagenomics techniques can be used to develop two approaches to weed management: (1) the isolation of novel herbicides produced by vector–hosts expressing the biosynthetic gene clusters, and (2) the identification of herbicide resistance genes in vector–hosts exposed to high levels of a herbicide (Kao-Kniffin et al., 2013). The greater possibility to uncover new biosynthetic gene clusters derived from soil microorganisms is one advantage of conducting a metagenomics-based functional screen in weed management (Kao-Kniffin et al., 2013). Structural and functional screens of sequences from the host could be established in order to uncover compounds with new modes of action (Westwood et al., 2018). Then selected clones or strains can be evaluated on a bigger scale in the greenhouse at a later period. The herbicides must be capable of controlling numerous weed targets rather than just one in order to gain widespread acceptability and importance. Their effectiveness should be based on specific stable gene activities or metabolites that can function in the field despite modest changes in environmental temperature and moisture (Westwood et al., 2018).
The discovery of genes and metabolites responsible for the generation of herbicidal chemicals is possible using high-throughput sequencing techniques, advanced computational tools, and metabolomics analysis (Kao-Kniffin et al., 2013; Trognitz et al., 2016). These techniques aimed at improving the screening of microbial generated herbicides have the potential to improve Striga control in a variety of crops and can help improve integrated weed management programs that include bioherbicides and other natural products. Many biosynthetic gene clusters for recognized natural products are arranged into operons with promoters and regulatory sites. While sequence-based screening is useful for analyzing key genes, functional screening based on host expression is best for discovering natural products like new herbicides (Kao-Kniffin et al., 2013). Phylogenetic or functional analyses are commonly analyzed from DNA isolated from environmental sources. Microbial function is investigated using a number of techniques that focus on genes or gene clusters that code for chemicals and proteins. The likelihood of finding a target varies depending on the gene or activity of interest (Kao-Kniffin et al., 2013).
To reduce the population density of the target weed or organism, biological control approaches most usually rely on (micro) organisms or natural chemicals. The traditional biological or inoculative strategy that is widely used entails introducing a natural enemy from its native range to a new location where the weed or pest is a problem. The biocontrol agent is released into the new habitat, and it takes time for the biocontrol organism to build up a population large enough to control the weed or pest. The introduced population is kept alive for a very long time (Trognitz et al., 2016). The research and development of novel herbicides based on natural chemicals, such as microorganisms byproducts or plant extracts is one promising area and herbicidal activity has only been tested on a limited percentage of the world's microbes and plant biodiversity (Westwood et al., 2018). Soil microbes, such as plant growth-promoting bacteria, the application of arbuscular mycorrhizae fungi, and some bacterial strains, significantly reduced Striga germination, adhesion, and emergence (Rocha et al., 2019). For example, strains of Pseudomonas putida and P. fluorescens have been used to suppress the germination of Striga hermonthica seeds (Ahonsi et al., 2002). Approaches to actively use microbial strains in weed management are still in their infancy with most biocontrol agents directed toward the above ground parts of plants (Müller-Stöver et al., 2016). More so, some of the limiting factors in the current application of metagenomics method for microbiological management of Striga include the possibility of the sequencing process to miss low-abundance microbes, inability to completely extract the DNA of the environmental microorganisms, while the sequencing data including species annotation and functional analysis may not be evaluated as a result of the inadequacies in many microbial database. Hence, the urgent need for a sequencing platform with long reads and high accuracy (Zhang et al., 2021). However, some prospective bioherbicidal strains have been successfully used to attack plants at various phases of development (Müller-Stöver et al., 2016). We are currently in the era of “omics” (genomics, proteomics, metagenomics, metabolomics, transcriptomics etc) that may enable new approaches in the management of weeds, including Striga. Computational, biochemical, and molecular approaches, including protein modeling and crystallography, can predict target-site resistance mutations and give early resistance screening prior to the herbicide's commercialization (Shaner and Beckie, 2014).
Today, the repertoire of data generated by completely sequenced genomes and Striga sequencing programs, as well as high-throughput technologies for transcriptome analysis, present a way to supplement more traditional Striga management approaches. This has increased our understanding of microbe-weed interactions, which have impact on decision making, particularly during the microbe selection stage, as well as biocontrol approaches. Such information is crucial in guiding genetic alterations to improve the efficacy and tolerance of bioherbicides (Morin, 2020).
Overcoming the menace caused by Striga is still a major concern to farmers all over the world in ensuring food security. Hence, it is important to source new, affordable, effective, durable, and integrated control strategies. Identifying and exploring potential beneficial microorganisms that affect and destroy (i) germination of Striga seed (ii) formation of the haustorium, and (iii) attachment which often reduces the infection of Striga as a generic, effective, and eco-friendly technique to improve crop production is important. The nature of Striga's life cycle and the mechanism of host infection propels the use of a microbial-based bio-control agent for the depletion of the seed bank. As a result, the isolation of microbes and understanding of seed-microorganism interactions, as well as the isolation and assessment of the tripartite relationships (Striga, plant, and microorganisms), will have significant implications on weed management methods in the future which are aimed at depleting seed banks and increasing the production of crop by peasant farmers.
Furthermore, to create crops with long-lasting resistance, further research into the genetic and molecular underpinnings of host resistance and host-parasite association is required. This knowledge may be translated into resistant crops using genomic resources and current technologies like targeted gene editing and mutant breeding. Intercropping and crop rotation alongside fake hosts are significant and cheaper Striga control techniques. This technique has the potential to be a critical component of present integrated Striga management in sub-Saharan Africa. Furthermore, for toothpick/FOS, push-pull, and seed coating technologies to be effective, farmers' planning, willingness, and commitment, alongside capital, labor, and availability of input in a specific cropping system are critical. Compatibility of soil and climate, farmer knowledge, information distribution, and technology transfer to smallholder farmers are all important variables that need to be further addressed. In addition, the efficacy of new compounds (bio-stimulants, and antagonists) may be influenced by their formulation, application technique, and timing. For rain-fed African agriculture, a proper formulation of effective chemicals, large scale, low-cost synthesis, and their application in the field is critical, most especially for seed bank depletion by biocontrol agents. Furthermore, before their on-farm application, the influence of these compounds on soil flora and fauna, soil structure, persistency, and residual impacts on the environment must be examined. Finally, a smart technological package combining Striga-resistant cultivars with mycoherbicides, fertilizers, herbicide-dependent seed coating, or new chemicals is still needed to accomplish a comprehensive and reliable Striga management. In addition, it is expected that more sequencing data will be accessible for meta-analyses, allowing researchers to delve deeper into more intricate evolutionary concerns and the fundamental mechanisms underlying complex characteristics like metabolic herbicide resistance and control, which would even contribute better to Striga management.
Cereals constitute a major staple food crop across the globe, and is strategic to attaining the Sustainable Development Goal (SDG) of ending hunger by the year 2030. However, Striga constitutes a major setback to cereal productions. This review explores the conventional and the current trends in Striga management. Considering the current deviation from the use of chemical herbicides to an eco-friendly alternative, the contribution of beneficial soil microbes in revitalizing agricultural soils and managing the invading Striga species are promising but yet untapped solutions. Hence, the interaction of the tripartite; host-Striga-microbe was discussed. Furthermore, the application of current biotechnological approaches such as metagenomics are essential tool in exploring weed-suppressive microbe-based chemicals that target a wide range of plant stages. This new approach also uncovers new biosynthetic gene clusters derived from soil microorganisms. Generally, there is need for more scientific investigation aimed at depleting Striga seed banks and increasing the crop production by taking advantage of the genetic and molecular underpinnings of host resistance in designing an appropriate host control measure.
All authors listed have made a substantial, direct, and intellectual contribution to the work and approved it for publication.
OB recognizes National Research Fund (NRF South Africa) for grants (UID123634 and UID132595) that support work in her research group.
The authors declare that the research was conducted in the absence of any commercial or financial relationships that could be construed as a potential conflict of interest.
All claims expressed in this article are solely those of the authors and do not necessarily represent those of their affiliated organizations, or those of the publisher, the editors and the reviewers. Any product that may be evaluated in this article, or claim that may be made by its manufacturer, is not guaranteed or endorsed by the publisher.
Abbes, Z., Trabelsi, I., Kharrat, M., and Amri, M. (2019). Intercropping with fenugreek (Trigonella foenum-graecum) enhanced seed yield and reduced Orobanche foetida infestation in faba bean (Vicia faba). Biol. Agricult. Horticult. 35, 238–247. doi: 10.1080/01448765.2019.1616614
Abdelhalim, T., Jannoura, R., and Joergensen, R. G. (2019). Mycorrhiza response and phosphorus acquisition efficiency of sorghum cultivars differing in strigolactone composition. Plant Soil. 437, 55–63. doi: 10.1007/s11104-019-03960-y
Abdullahi, W. M., Dianda, M., Boukar, O., Dieng, I., Mohammed, G. S., Belko, N., et al. (2022). Integrated management of Striga gesnerioides in cowpea using resistant varieties, improved crop nutrition and rhizobium inoculants. Plant Soil. 473, 1–17. doi: 10.1007/s11104-022-05295-7
Aggarwal, A., Kadian, N., Tanwar, A., Yadav, A., and Gupta, K. (2011). Role of arbuscular mycorrhizal fungi (AMF) in global sustainable development. J. Appl. Nat. Sci. 3, 340–351. doi: 10.31018/jans.v3i2.211
Ahonsi, M. O., Berner, D. K., Emechebe, A. M., and Lagoke, S. T. (2002). Selection of rhizobacterial strains for suppression of germination of Striga hermonthica (Del.) Benth. seeds. Biol. Control. 24, 143–152. doi: 10.1016/S1049-9644(02)00019-1
Ahonsi, M. O., Berner, D. K., Emechebe, A. M., Lagoke, S. T., and Sanginga, N. (2003). Potential of ethylene-producing pseudomonads in combination with effective N2-fixing bradyrhizobial strains as supplements to legume rotation for Striga hermonthica control. Biol. Control. 28, 1–10. doi: 10.1016/S1049-9644(03)00051-3
Anteyi, W. O., and Rasche, F. (2021). Role and in vivo localization of Fusarium oxysporum f. sp. strigae and Bacillus subtilis in an integrated Striga hermonthica biocontrol system. Phyto. Front. 1, 51–61. doi: 10.1094/PHYTOFR-08-20-0011-R
Aquino, B., Bradley, J. M., and Lumba, S. (2021). On the outside looking in: roles of endogenous and exogenous strigolactones. Plant J. 105, 322–334. doi: 10.1111/tpj.15087
Babalola, O., and Akindolire, A. (2011). Identification of native rhizobacteria peculiar to selected food crops in Mmabatho municipality of South Africa. Biol. Agri. Horticult. 27, 294–309. doi: 10.1080/01448765.2011.647798
Babalola, O., Berner, D., and Amusa, N. (2007). Evaluation of some bacterial isolates as germination stimulants of Striga hermonthica. Afr. J. Agric. Res. 2, 27−30.
Babalola, O. O. (2010a). Beneficial bacteria of agricultural importance. Biotechnol. Lett. 32, 1559–1570. doi: 10.1007/s10529-010-0347-0
Babalola, O. O. (2010b). Exogenous cellulase contributes to mycoherbicidal activity of Fusarium arthrosporioides on Orobanche aegyptiaca. Int. J. Agronomy 2010. doi: 10.1155/2010/963259
Babalola, O. O., and Odhiambo, G. D. (2008). Effect of inoculation with Klebsiella oxytoca “10 mkr 7”on striga suicidal germination in Zea mays. World Appl. Sci. J. 3, 57–62. doi: 10.4314/jtmb.v3i2.35455
Bàrberi, P. (2019). Ecological weed management in sub-Saharan Africa: prospects and implications on other agroecosystem services. Adv. Agron. 156, 219–264. doi: 10.1016/bs.agron.2019.01.009
Basu, S., Rabara, R. C., and Negi, S. (2018). AMF: the future prospect for sustainable agriculture. Physiol. Mol. Plant Pathol. 102, 36–45. doi: 10.1016/j.pmpp.2017.11.007
Beed, F. D., Hallett, S. G., Venne, J., and Watson, A. K. (2007). “Biocontrol using Fusarium oxysporum: a critical component of integrated Striga management,” in Integrating New Technologies for Striga Control: Towards Ending the Witch-Hunt. Singapore: World Scientific. p. 283–300. doi: 10.1142/9789812771506_0021
Berner, D. K., Sauerborn, J., Hess, D. E., and Emechebe, A. M. (2003). “The role of biological control in integrated management of Striga species in Africa,” in Biological control in IPM systems in Africa. Wallingford UK: CABI Publishing Wallingford UK. p. 259–276. doi: 10.1079/9780851996394.0259
Berner, D. K., Schaad, N. W., and Völksch, B. (1999). Use of ethylene-producing bacteria for stimulation of Striga spp. seed germination. Biol. Control. 15, 274–282. doi: 10.1006/bcon.1999.0718
Besserer, A., Puech-Pagès, V., Kiefer, P., Gomez-Roldan, V., Jauneau, A., Roy, S., et al. (2006). Strigolactones stimulate arbuscular mycorrhizal fungi by activating mitochondria. PLoS Biol. 4, e226. doi: 10.1371/journal.pbio.0040226
Birhane, E., Gebremeskel, K., Taddesse, T., Hailemariam, M., Hadgu, K. M., Norgrove, L., et al. (2018). Integrating Faidherbia albida trees into a sorghum field reduces striga infestation and improves mycorrhiza spore density and colonization. Agrofor. Syst. 92, 643–653. doi: 10.1007/s10457-016-0027-8
Bouillant, M.-L., Mich,é, L., Ouedraogo, O., Alexandre, G., Jacoud, C., Sall,é, G., et al. (1997). Inhibition of Striga seed germination associated with sorghum growth promotion by soil bacteria. Comptes Rendus de l'Académie des Sciences-Series III-Sciences de la Vie. 320, 159–162. doi: 10.1016/S0764-4469(97)85007-X
Brun, G., Braem, L., Thoiron, S., Gevaert, K., Goormachtig, S., and Delavault, P. (2018). Seed germination in parasitic plants: what insights can we expect from strigolactone research? J. Exp. Bot. 69, 2265–2280. doi: 10.1093/jxb/erx472
Chesterfield, R. J., Whitfield, J. H., Pouvreau, B., Cao, D., Alexandrov, K., Beveridge, C. A., et al. (2020). Rational design of novel fluorescent enzyme biosensors for direct detection of strigolactones. ACS Synthet. Biol. 9, 2107–2118. doi: 10.1021/acssynbio.0c00192
Dafaallah, A. B., and Babiker, A. E. T. (2016). Variability in Striga hermonthica (Del.) Benth. Western Sudan: Populations in Kordofan Area, Western Sudan.
David, O. G., Ayangbenro, A. S., Odhiambo, J. J., and Babalola, O. O. (2022). Striga hermonthica: a highly destructive pathogen in maize production. Environ. Challenges. 8, 100590. doi: 10.1016/j.envc.2022.100590
Ejeta, G. (2007). “The Striga scourge in Africa: a growing pandemic,” in Integrating New Technologies for Striga Control: Towards Ending the Witch-Hunt. Singapore: World Scientific. p. 3–16. doi: 10.1142/9789812771506_0001
Fernández-Aparicio, M., Westwood, J. H., and Rubiales, D. (2011). Agronomic, breeding, and biotechnological approaches to parasitic plant management through manipulation of germination stimulant levels in agricultural soils. Botany. 89, 813–826. doi: 10.1139/b11-075
García-Garrido, J., Lendzemo, V., Castellanos-Morales, V., Steinkellner, S., and Vierheilig, H. (2009). Strigolactones, signals for parasitic plants and arbuscular mycorrhizal fungi. Mycorrhiza. 19, 449–459. doi: 10.1007/s00572-009-0265-y
Handelsman, J. (2004). Metagenomics: application of genomics to uncultured microorganisms. Microbiol. Mol. Biol. Rev. 68, 669–685. doi: 10.1128/MMBR.68.4.669-685.2004
Hassan, M. M., Gani, M., and Babiker, A. (2009). Management of Striga hermonthica in sorghum using soil rhizosphere bacteria and host plant resistance. Int. J. Agric. Biol. 11, 367–373.
Hu, Q., He, Y., Wang, L., Liu, S., Meng, X., Liu, G., et al. (2017). DWARF14, a receptor covalently linked with the active form of strigolactones, undergoes strigolactone-dependent degradation in rice. Front. Plant Sci. 8, 1935. doi: 10.3389/fpls.2017.01935
Irving, L. J., and Cameron, D. D. (2009). You are what you eat: interactions between root parasitic plants and their hosts. Adv. Bot. Res. 50, 87–138. doi: 10.1016/S0065-2296(08)00803-3
Jamil, M., Kountche, B. A., and Al-Babili, S. (2021). Current progress in Striga management. Plant Physiol. 185, 1339–1352. doi: 10.1093/plphys/kiab040
Jamil, M., Rodenburg, J., Charnikhova, T., and Bouwmeester, H. J. (2011). Pre?attachment Striga hermonthica resistance of New Rice for Africa (NERICA) cultivars based on low strigolactone production. New Phytol. 192, 964–975. doi: 10.1111/j.1469-8137.2011.03850.x
Joel, D. M. (2000). The long-term approach to parasitic weeds control: manipulation of specific developmental mechanisms of the parasite. Crop Prot. 19, 753–758. doi: 10.1016/S0261-2194(00)00100-9
Kamble, V. R., and Agre, D. G. (2014). New report on AMF colonization in root parasite Striga gesnerioides and its host Lepidagathis hamiltoniana from high altitude region of Maharashtra. Int. Multidiscip. Res. J. 3, 27−31.
Kanampiu, F., Makumbi, D., Mageto, E., Omanya, G., Waruingi, S., Musyoka, P., et al. (2018). Assessment of management options on Striga infestation and maize grain yield in Kenya. Weed Sci. 66, 516–524. doi: 10.1017/wsc.2018.4
Kaniganti, S., Bhattacharya, J., Prakash, P. B., and Reddy, P. S. (2022). Strigolactone, a neglected plant hormone, with a great potential for crop improvement: A crosstalk with other plant hormones. Environ. Exper. Bot. 204:105072. doi: 10.1016/j.envexpbot.2022.105072
Kao-Kniffin, J., Carver, S. M., and Ditommaso, A. (2013). Advancing weed management strategies using metagenomic techniques. Weed Sci. 61, 171–184. doi: 10.1614/WS-D-12-00114.1
Kerr, R. B., Snapp, S., Chirwa, M., and Shumba, L. (2007). Participatory research on legume diversification with Malawian smallholder farmers for improved human nutrition and soil fertility. Exp. Agricult. 43, 437–453. doi: 10.1017/S0014479707005339
Kountche, B. A., Jamil, M., Yonli, D., Nikiema, M. P., Blanco-Ania, D., Asami, T., et al. (2019). Suicidal germination as a control strategy for Striga hermonthica (Benth.) in smallholder farms of sub-Saharan Africa. Plants, People, Planet. 1, 107–118. doi: 10.1002/ppp3.32
Kushwaha, R. K., Rodrigues, V., Kumar, V., Patel, H., Raina, M., and Kumar, D. (2020). Soil microbes-medicinal plants interactions: ecological diversity and future prospect. Plant Microbe Symbiosis. 263–286. doi: 10.1007/978-3-030-36248-5_14
Le Mire, G., Nguyen, M., Fassotte, B., du Jardin, P., Verheggen, F., Delaplace, P., et al. (2016). Implementing Biostimulants and Biocontrol Strategies in the Agroecological Management of Cultivated Ecosystems. Biotechnologie, Agronomie, Société et Environnement. Gembloux: Presses Agronomiques de Gembloux doi: 10.25518/1780-4507.12717
Lendzemo, V. W., Kuyper, T. W., Matusova, R., Bouwmeester, H. J., and Ast, A. V. (2007). Colonization by arbuscular mycorrhizal fungi of sorghum leads to reduced germination and subsequent attachment and emergence of Striga hermonthica. Plant Signal. Behav. 2, 58–62. doi: 10.4161/psb.2.1.3884
López-Ráez, J. A., Pozo, M. J., and García-Garrido, J. M. (2011). Strigolactones: a cry for help in the rhizosphere. Botany. 89, 513–522. doi: 10.1139/b11-046
Manjunatha, H. P., Nirmalnath, P. J., Chandranath, H. T., Ammanna, S., and Jagadeesh, K. S. (2018). Field evalualtion of native arbuscular mycorrhizal fungi in the management of Striga in sugarcane (Saccharum officinarum L.). J. Pharmacogn. Phytochem. 7, 2496–2500. Available online at: https://www.phytojournal.com/archives/2018/vol7issue2/PartAI/7-2-264-614.pdf
Midega, C. A., Wasonga, C. J., Hooper, A. M., Pickett, J. A., and Khan, Z. R. (2017). Drought-tolerant Desmodium species effectively suppress parasitic striga weed and improve cereal grain yields in western Kenya. Crop Prot. 98, 94–101. doi: 10.1016/j.cropro.2017.03.018
Morin, L. (2020). Progress in biological control of weeds with plant pathogens. Annu. Rev. Phytopathol. 58, 201–223. doi: 10.1146/annurev-phyto-010820-012823
Mounde, L. G., Anteyi, W. O., and Rasche, F. (2020). Tripartite interaction between Striga spp., cereals, and plant root-associated microorganisms: a review. CAB Rev. 15, 1–17. doi: 10.1079/PAVSNNR202015005
Mounde, L. G., Boh, M. Y., Cotter, M., and Rasche, F. (2015). Potential of rhizobacteria for promoting sorghum growth and suppressing Striga hermonthica development. J. Plant Dis. Protect. 122, 100–106. doi: 10.1007/BF03356537
Mrema, E., Shimelis, H., and Laing, M. (2020). Combining ability of yield and yield components among Fusarium oxysporum f. sp. Strigae-compatible and Striga-resistant sorghum genotypes. Acta Agriculturae Scandinavica, Section B—Soil & Plant Science. 70, 95–108. doi: 10.1080/09064710.2019.1674915
Müller-Stöver, D., Nybroe, O., Baraibar, B., Loddo, D., Eizenberg, H., French, K., et al. (2016). Contribution of the seed microbiome to weed management. Weed Res. 56, 335–339. doi: 10.1111/wre.12218
Mutsvanga, S., Gasura, E., Setimela, P. S., Nyakurwa, C. S., and Mabasa, S. (2022). Nutritional management and maize variety combination effectively control Striga asiatica in southern Africa. CABI Agricult. Biosci. 3, 1–14. doi: 10.1186/s43170-022-00108-4
Mwangangi, I. M., Büchi, L., Haefele, S. M., Bastiaans, L., Runo, S., and Rodenburg, J. (2021). Combining host plant defence with targeted nutrition: key to durable control of hemiparasitic Striga in cereals in sub-Saharan Africa? New Phytol. 230, 2164–2178. doi: 10.1111/nph.17271
Neondo, J. O., Alakonya, A. E., and Kasili, R. W. (2017). Screening for potential Striga hermonthica fungal and bacterial biocontrol agents from suppressive soils in Western Kenya. Biocontrol. 62, 705–717. doi: 10.1007/s10526-017-9833-9
Nzioki, H. S., Oyosi, F., Morris, C. E., Kaya, E., Pilgeram, A. L., Baker, C. S., et al. (2016). Striga biocontrol on a toothpick: a readily deployable and inexpensive method for smallholder farmers. Front. Plant Sci. 7, 1121. doi: 10.3389/fpls.2016.01121
Olowe, O. M., Ayangbenro, A. S., Akanmu, A. O., Kutu, F. R., Odhiambo, J. J., and Babalola, O. O. (2023). Comparative insights into the microbial diversity and community structure of striga hermonthica-infested maize rhizosphere. Appl. Sci. 13, 3260. doi: 10.3390/app13053260
Olowe, O. M., Olawuyi, O. J., Sobowale, A. A., and Odebode, A. C. (2018). Role of arbuscular mycorrhizal fungi as biocontrol agents against Fusarium verticillioides causing ear rot of Zea mays L.(Maize). Curr. Plant Biol. 15, 30–37. doi: 10.1016/j.cpb.2018.11.005
Rao, A. N., Brainard, D. C., Kumar, V., Ladha, J. K., and Johnson, D. E. (2017). Preventive weed management in direct-seeded rice: targeting the weed seedbank. Adv. Agron. 144, 45–142. doi: 10.1016/bs.agron.2017.02.002
Rocha, I., Ma, Y., Souza-Alonso, P., Vosátka, M., Freitas, H., and Oliveira, R. S. (2019). Seed coating: a tool for delivering beneficial microbes to agricultural crops. Front. Plant Sci. 10, 1357. doi: 10.3389/fpls.2019.01357
Samejima, H., and Sugimoto, Y. (2018). Recent research progress in combatting root parasitic weeds. Biotechnol. Biotechnol. Equipment. 32, 221–240. doi: 10.1080/13102818.2017.1420427
Shaner, D. L., and Beckie, H. J. (2014). The future for weed control and technology. Pest Manag. Sci. 70, 1329–1339. doi: 10.1002/ps.3706
Shen, H., Ye, W., Hong, L., Huang, H., Wang, Z., Deng, X., et al. (2006). Progress in parasitic plant biology: host selection and nutrient transfer. Plant Biol. 8, 175–185. doi: 10.1055/s-2006-923796
Soto-Cruz, F. J., Zorrilla, J. G., Rial, C., Varela, R. M., Molinillo, J. M., Igartuburu, J. M., et al. (2021). Allelopathic activity of strigolactones on the germination of parasitic plants and arbuscular mycorrhizal fungi growth. Agronomy. 11, 2174. doi: 10.3390/agronomy11112174
Spallek, T., Mutuku, M., and Shirasu, K. (2013). The genus S triga: a witch profile. Mol. Plant Pathol. 14, 861–869. doi: 10.1111/mpp.12058
Steinkellner, S., Lendzemo, V., Langer, I., Schweiger, P., Khaosaad, T., Toussaint, J.-P., et al. (2007). Flavonoids and strigolactones in root exudates as signals in symbiotic and pathogenic plant-fungus interactions. Molecules. 12, 1290–1306. doi: 10.3390/12071290
Tedersoo, L., Albertsen, M., Anslan, S., and Callahan, B. (2021). Perspectives and benefits of high-throughput long-read sequencing in microbial ecology. Appl. Environ. Microbiol. 87, e00626–e00621. doi: 10.1128/AEM.00626-21
Torres-Vera, R., García, J. M., Pozo, M. J., and López-Ráez, J. A. (2014). Do strigolactones contribute to plant defence? Mol. Plant Pathol. 15, 211–216. doi: 10.1111/mpp.12074
Trognitz, F., Hackl, E., Widhalm, S., and Sessitsch, A. (2016). The role of plant–microbiome interactions in weed establishment and control. FEMS Microbiol. Ecol. 92. doi: 10.1093/femsec/fiw138
Tulu, D., Abakemal, D., Keimeso, Z., Kumsa, T., Negera, D., Terefe, W., et al. (2021). International journal of agriculture and biosciences. Int J Agri Biosci. 10, 195–201. doi: 10.47278/journal.ijab/2022.003
Vassileva, M., Malus,à, E., Sas-Paszt, L., Trzcinski, P., Galvez, A., Flor-Peregrin, E., et al. (2021). Fermentation strategies to improve soil bio-inoculant production and quality. Microorganisms. 9, 1254. doi: 10.3390/microorganisms9061254
Wang, Y., He, X., and Yu, F. (2022). Non-host plants: Are they mycorrhizal networks players? Plant Diversity. 44, 127–134. doi: 10.1016/j.pld.2021.06.005
Watson, A. K. (2013). “Biocontrol,” in Parasitic Orobanchaceae. p. 469–497. Berlin/Heidelberg, Germany: Springer. doi: 10.1007/978-3-642-38146-1_26
Westwood, J. H., Charudattan, R., Duke, S. O., Fennimore, S. A., Marrone, P., Slaughter, D. C., et al. (2018). Weed management in 2050: Perspectives on the future of weed science. Weed Sci. 66, 275–285. doi: 10.1017/wsc.2017.78
Yilma, G., and Bekele, M. (2021). The role of soil bacteria in the control of parasitic Striga hermonthica weed. Int J Adv Res Biol Sci 8, 21–29. doi: 10.22192/ijarbs.2021.08.05.002
Zarafi, A., and Dauda, W. (2019). Exploring the importance of fungi in agricultural biotechnology. Int. J. Agric. Sci. Vet. Med. 7, 1–12.
Zhang, L., Chen, F., Zeng, Z., Xu, M., Sun, F., Yang, L., et al. (2021). Advances in metagenomics and its application in environmental microorganisms. Front. Microbiol. 12, 3847. doi: 10.3389/fmicb.2021.766364
Keywords: parasitic weed, soil microbes, metagenomics, biological control, chemical control
Citation: Olowe OM, Akanmu AO, Ayangbenro AS, Fadiji AE, Bitire TD, Odhiambo JJO, Kutu FR and Babalola OO (2023) Trenchant microbiological-based approach for the control of Striga: Current practices and future prospects. Front. Sustain. Food Syst. 7:1073339. doi: 10.3389/fsufs.2023.1073339
Received: 27 October 2022; Accepted: 17 March 2023;
Published: 06 April 2023.
Edited by:
Fei-Hai Yu, Taizhou University, ChinaReviewed by:
Yongge Yuan, Taizhou University, ChinaCopyright © 2023 Olowe, Akanmu, Ayangbenro, Fadiji, Bitire, Odhiambo, Kutu and Babalola. This is an open-access article distributed under the terms of the Creative Commons Attribution License (CC BY). The use, distribution or reproduction in other forums is permitted, provided the original author(s) and the copyright owner(s) are credited and that the original publication in this journal is cited, in accordance with accepted academic practice. No use, distribution or reproduction is permitted which does not comply with these terms.
*Correspondence: Olubukola Oluranti Babalola, b2x1YnVrb2xhLmJhYmFsb2xhQG53dS5hYy56YQ==
Disclaimer: All claims expressed in this article are solely those of the authors and do not necessarily represent those of their affiliated organizations, or those of the publisher, the editors and the reviewers. Any product that may be evaluated in this article or claim that may be made by its manufacturer is not guaranteed or endorsed by the publisher.
Research integrity at Frontiers
Learn more about the work of our research integrity team to safeguard the quality of each article we publish.