- Institute of Advanced Biomedical Engineering and Science, Tokyo Women's Medical University, Tokyo, Japan
Cultured meat produced using cell culture technology can potentially alleviate many of the ethical, environmental, and public health concerns associated with conventional livestock meat production. The industrialization of cultured meat for wide-spread adoption requires new methods to efficiently collect high-quality cells and to preserve their cell quality. Cryopreservation is a widely used technique to enable the long-term storage of cells without causing severe damage. In this study, we focused on the feasibility of cryopreservation to maintain cell quality for storage of bovine myogenic cells harvested from bovine meat based on our unique primary culture method. Primary bovine cells were incubated in a culture dish and then cryopreserved at −80°C for 1 week or 1 year. After thawing, the cells were further cultured for several passages to evaluate the abilities of the cells to proliferate or differentiate into myotubes. Furthermore, the cells were repeatedly cryopreserved for 1 week each time to investigate the impact of the repeated freezing and thawing. Consequently, long-term (within 1 year) or repeated (up to 3 times for 1 week each) cryopreservation at −80°C caused no degradation in the abilities of the cells to proliferate or differentiate, which is important for cultured meat production. We also confirmed that the cryopreservation did not require any unique cell freezing media. Moreover, based on our tissue engineering technique, our cryopreserved bovine myogenic cells had the ability to form sarcomere structures and produce muscle contractions even after they were frozen for 1 year. Although the bovine muscle tissues described here require more mature structures and functions in order to closely mimic native muscle tissue, we believe that the functional maturation of myogenic cells is essential to produce a “tissue-engineered meat” that will have native-like nutrients, texture, and taste that consumers will expect in the future. These results reveal the potential of cryopreserving quality-controlled bovine myogenic cells to contribute to a stable supply of high-quality cells for cultured meat production.
1. Introduction
Cultured meat is produced in vitro by extracting muscle-specific stem cells from livestock meat samples and growing them into muscle tissue for meat such as beef, pork, or chicken (Post, 2012; Post et al., 2020; Takahashi et al., 2022b). Cultured meat production is expected to become a practical alternative to traditional livestock husbandry, and this technology promises to save the world from a future protein crisis, as well as the environmental and ethical concerns caused by current livestock production. Especially, as the global population grows, meat consumption is expected to increase by 73% by 2050, and a new way of producing meat is urgently needed in the world (Tuomisto and Teixeira de Mattos, 2011; Melzener et al., 2021). In response to this growing demand, the development of cultured meat technology has been rapidly progressing. This new strategy for sustainable meat production gained widespread attention after the Mark Post group produced the first cultured meat prototype in 2013 (Post, 2014), and since 2020, the world's first lab-grown chicken has been approved for sale and consumption in Singapore (Chong et al., 2022). Whereas some research groups in life science fields have reported on development of some novel technologies, many challenges remain for industrial scaling-up and cost reduction to ensure a sustainable supply of cultured meat in the future.
For cultured meat production, it is important to efficiently collect high-quality cells and maintain that cell quality throughout the production process (Baquero-Perez et al., 2012). Cryopreservation is a promising technique providing a consistent supply of high-quality cell sources for cultured meat production. This technique is fundamental to the preservation of cultured cells by freezing at very low temperatures for a long time (Jang et al., 2017; Miyamoto et al., 2018), and is required in a wide range of cell culture experiments (Grant et al., 2019; Fu et al., 2020; Erol et al., 2021). Although damage to cells caused by freezing and thawing are of concern, this technology has been well established and various kinds of commercial products such as cell freezing media have been developed. Therefore, most researchers in medicine and biochemistry use cryopreservation on a routine basis. For clinical applications, various kinds of human stem cells such as haematopoietic stem cells can be safely cryopreserved to store the therapeutic cell without any severe damage (Hunt, 2011; Meneghel et al., 2020). When cells are cryopreserved, an important evaluation point is whether they retain not only their proliferative capacity, but also their differentiation capacity (Hunt, 2011). Similarly, for studies of muscle tissue engineering, myogenic cells need to not only proliferate but also maintain their differentiation ability into native-like muscle tissue after they are cryopreserved (Grant et al., 2019).
The number of new studies related to cultured meat has been increasing, but few research groups have achieved any practical applications for cultured meat production (Chriki and Hocquette, 2020; Furuhashi et al., 2021; Kang et al., 2021). Long-term storage of cultured cells, as a cell source for cultured meat, is one technique urgently needed to be addressed. Stig Skrivergaard et al. demonstrated in their previous study that bovine satellite cells were able to maintain their important properties after muscle tissue storage at 4°C for up to 5 days (Skrivergaard et al., 2021). Since cryopreservation is a well-established procedure in other research fields, few studies demonstrated that bovine myogenic cells were frozen in studies of cultured meat production (Ding et al., 2018; Kolkmann et al., 2020; Skrivergaard et al., 2021; Andreassen et al., 2022). However, there is no paper on the impact of cryopreservation on the quality of primary bovine myogenic cells. Ensuring a reliable and consistent source of cells by using cryopreservation will allow us to increase the stable supply of promising cells for cultured meat production. In fact, the need for cryopreserving cells has already been recognized and prompted the creation of a cell bank for cultured meat production in the future (Soice and Johnston, 2021). Establishing a method to cryopreserve myogenic cells from livestock meat samples, including bovine myogenic cells, will allow long-term storage of quality-controlled materials and contribute to the stable supply of raw materials required for cultured meat production.
Our group has established a primary culture method to harvest myogenic cells from bovine meat and successfully expanded them in vitro to be able to produce cultured meat in the future (Takahashi et al., 2022b). Since the primary bovine cells showed high proliferative and differentiation abilities, they have the potential to be used as a high-quality cell source for cultured meat production. On the other hand, we need to frequently harvest the cells through our primary culture procedure every single time. This is problematic since it requires not only effort and time but also a number of sacrifice animals to obtain muscle tissue. In addition, depending on the characteristics of animals from which cells are taken, there is a risk that the harvested cells could have different properties from each primary culture procedure. To improve the first step in cultured meat production, we confirmed in this study the possibility to cryopreserve bovine myogenic cells while maintaining their quality. To evaluate the quality of bovine myogenic cells, we focused on the ability to maintain proliferation and differentiation after freezing and thawing. Furthermore, to ensure the quality of the cells with specific conditions, we demonstrated how long and how many times they were able to be cryopreserved at −80°C with no severe damage. Although cryopreservation techniques have long been discussed in cell culture research, this study uniquely focused on the possibility to preserve not only cell life but also their quality as a cell source for cultured meat production. The aim of this research is to give valuable insight into the potential of cryopreservation for bovine myogenic cells with a hope to establish one cell culture method for cultured meat production.
2. Materials and Methods
2.1. Harvest of primary myogenic cells from bovine meat
Primary cell culture derived from bovine meat was conducted in the same way as our primary culture method (Takahashi et al., 2022b). Bovine myogenic cells were isolated from cheek meat of Japanese Black cattle slaughtered and kindly supplied by Tokyo Shibaura Zoki (Tokyo, Japan). The meat was first immersed in 0.5% chlorhexidine gluconate (5% Hibitane, Sumitomo Pharma, Osaka, Japan) for a few minutes to disinfect its surface, and cut into about 3 × 4 × 0.5 cm of skeletal muscle samples by using a scalpel. The samples were washed with povidone-iodine solution (Meiji Seika Pharma, Tokyo, Japan) for disinfection, followed by rubbing ethanol (Yoshida Pharmaceutical, Saitama, Japan) and Hanks' balanced salt solution (HBSS) (FUJIFILM Wako Pure Chemical, Osaka, Japan). The samples were minced into small pieces about 2 mm by using a scalpel and scissors. Two g of the minced sample was put into a 50 mL conical tube and immersed in 10 mL HBSS containing 1 mg/mL pronase (Pronase from Streptomyces griseus, Sigma-Aldrich, St. Louis, MO, USA), and placed in a water bath for incubation at 37°C while being gently stirred for 1 h. The sample was immersed in 10 mL HBSS with 10% fetal bovine serum (FBS) (NICHIREI BIOSCIENCES INC., Tokyo, Japan) and pipetted with a 25 mL and then a 10 mL measuring pipette each for 10–20 times. The sample was then passed through a 40 μm cell strainer in order to get rid of large segments and the cell suspension was centrifuged at 1,000×g for 10 min. The supernatant was removed and the precipitated pellet was resuspended with 10 mL Dulbecco's Modified Eagle Medium-high glucose (DMEM) (FUJIFILM Wako Pure Chemical) with 10% FBS, 1% penicillin-streptomycin-amphotericin B (FUJIFILM Wako Pure Chemical), and 10 ng/mL basic fibroblast growth factor (bFGF) (KAKEN PHARMACEUTICAL, Tokyo, Japan). The solution was placed in a ϕ10 cm culture dish and incubated for 1 h at 37°C to remove any early adhering cells, such as fibroblasts. After pre-plating, the culture supernatant was transferred to a 5 ~ 6 of ϕ10 cm culture dish coated with iMatrix-511. For preparation, the coated dish was incubated with Easy iMatrix-511 (Nippi, Tokyo, Japan) for 1 h at 37°C. The supernatant was cultured in growth medium for 6–7 days. The medium was changed the day after seeding and every 1 or 2 days thereafter.
2.2. Cryopreservation procedure of bovine myogenic cells
Figure 1 illustrates the experimental timeline with some different conditions. First, primary bovine myogenic cells (passage 0) were harvested from culture dishes by treatment with trypsin and the cell suspension containing 5 × 106 cells was placed in a centrifuge tube. Then, the cell suspension was centrifuged at 300×g for 5 min at 4°C. The supernatant was removed and the cells were resuspended with 1 mL of CELLBANKER 1 (ZENOGEN PHARMA, Fukushima, Japan). The cell suspension (5 × 106 cells/mL) was placed in a cryogenic storage vial. To control freezing rate, the vial was placed in a freeze treatment container, BICELL (NIHON FREEZER, Tokyo, Japan), and then the cells in the container were preserved at −80°C in a deep freezer. After 7 or 8 days (1-week cryopreservation), the frozen cells were thawed in a 37 °C water bath for a few seconds, and the cell suspension was added to 3 mL of DMEM containing 10% FBS in a 15 mL centrifuge tube. The cells were then centrifuged at 300×g for 5 min at 4°C. The supernatant was removed and the cells were resuspended with 10 mL of DMEM containing 10% FBS. Each 1 mL of the solution (5 × 105 cells) was placed in a ϕ10 cm culture dish, and the cells were cultured for several passages. To compare the effects of different cell freezing media, three other different media were also used: CELLBANKER 2 (ZENOGEN PHARMA), LABO Banker 1 (TOSC Japan, Tokyo, Japan), and the mixture of 90% FBS and 10% Dimethyl Sulfoxide (DMSO) (NACALAI TESQUE, INC., Kyoto, Japan). After each cryopreservation, the cells were cultured from passages 1 to 3 (Figure 1A). For experiments of repeated cryopreservation, three samples were made: control samples (0-time cryopreservation), “1 week × 1 time” samples, and “1 week × 3 times” samples (Figure 1B). After the cryopreservation of primary cells (P0), the frozen cells were thawed and passaged nine times (“1 week × 1 time”). To determine the influence of repeated freezing and thawing, the cells were again frozen before passage 4 and then thawed after 1 week (second time). They were then cultured from passages 4 to 6, and frozen again for 1 week (third time). After a total of three times cryopreservation, the cells were cultured to passage 9 (“1 week × 3 times”). All samples were prepared from the bovine cells harvested at the same time (the same meat sample each time). In addition to the 1-week cryopreservation samples, bovine cells were also frozen for 1 year to investigate the influence of longer-term cryopreservation (Figure 1C). After the “1-year cryopreservation”, the cells were thawed and passaged nine times (“1 year × 1 time”).
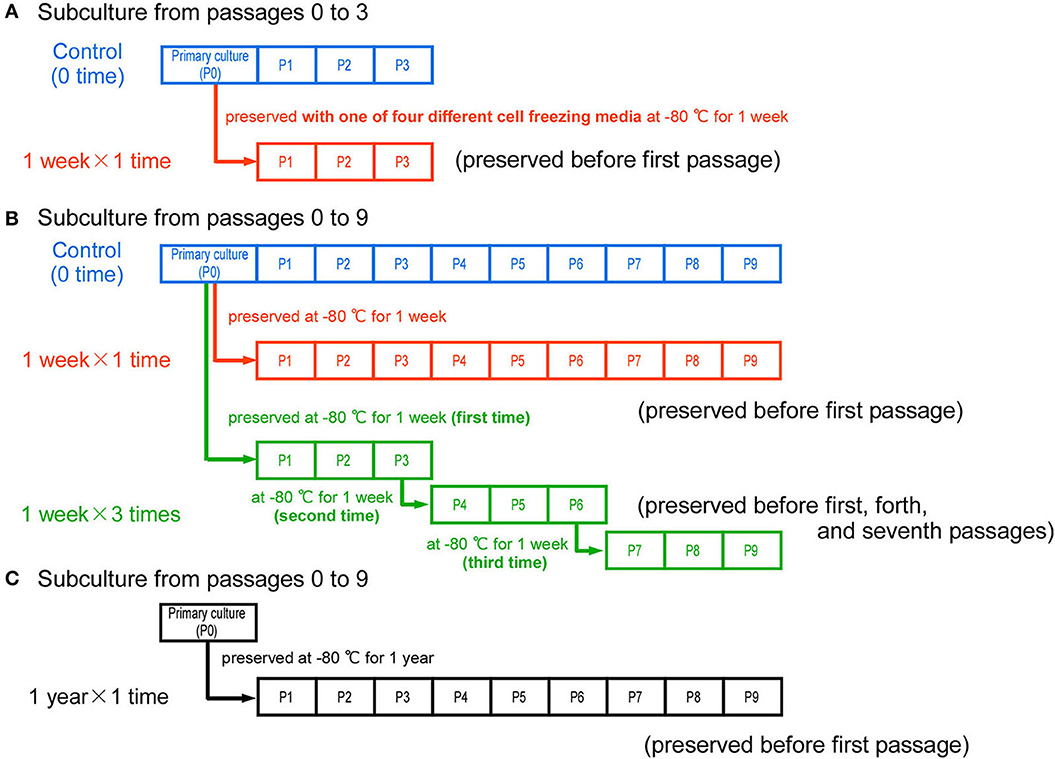
Figure 1. Experimental timeline and the conditions of cryopreservation. (A) As control samples, bovine cells were cultured from passages 0 to 3 without cryopreservation. To compare with the control samples, the cells harvested at the same time were cryopreserved for 1 week with one of four different cell freezing media. After thawing, the cells were cultured from passages 1 to 3. (B) In the control samples, bovine cells were cultured from passages 0 to 9 without cryopreservation. The primary cells (passage 0) were cryopreserved once for 1 week, and cultured to passage 9 after thawing (“1 week × 1 time” samples). To repeatedly freeze and thaw the cells, the cells at passages 3 and 6 were cryopreserved again (“1 week × 3 times” samples). (C) To determine the influence of cryopreservation for 1 year, the primary cells (P0) were cryopreserved for 1 year and then cultured to passage 9 after thawing (“1 year × 1 time” samples).
2.3. Proliferation and differentiation of bovine myogenic cells in repeated passaging
The primary bovine cells were cultured on normal cell culture dishes to increase the population through several passages. For each passage, they were seeded to non-coated dishes and cultured for 2 days in DMEM containing 10% FBS, 10 ng/mL bFGF and 1% penicillin-streptomycin-amphotericin B. Every 2 days, they were harvested by treatment with trypsin and repeatedly seeded at a density of 5 × 105 cells/dish (ϕ10 cm culture dishes) to expand the cell population over 3 passages (Figure 1A), or over 9 passages (Figure 1B). To confirm the differentiation capability, the bovine myogenic cells were seeded at a density of 1 × 106 cells/dish on normal ϕ3.5 cm dishes and cultured for 2–4 days in the growth medium (10% FBS-DMEM). This procedure was conducted at passages 3, 6, and 9. For all the experiments of 1-week cryopreservation (Figures 1A, B), this procedure of repeated passaging was independently performed three times for the primary culture. For the experiments of 1-year cryopreservation (Figure 1C), the procedure was independently performed four times.
2.4. Preparation of micropatterned thermoresponsive substrate
In this study, polymer micropatterning was performed using our previously reported method to prepare a micropatterned thermoresponsive substrate (Takahashi et al., 2013; Takahashi and Okano, 2015). To produce the micropatterns on a hydrophilic polymer, acrylamide (AAm) aqueous solution (50 w/w%) (FUJIFILM Wako Pure Chemical), containing a water-soluble photo-initiator camphorquinone (7,7 dimethyl-2,3-dioxobicyclo[2.2.1] heptane-1-carboxylic acid) (1 w/w%) was poured into an “UpCell” dish (CellSeed, Tokyo, Japan), a commercially available thermoresponsive cell culture dish (Takahashi and Okano, 2019). Then, visible light was irradiated for 20 min onto the culture surface through an appropriate photomask. Stripe-shaped micropatterns (the width of non-irradiation and irradiation regions were 50 μm) were fabricated by grafting polyacrylamide (PAAm).
To confirm the formation of micropatterns on the substrate, fluorescently labeled fibronectins were immobilized on the patterned surface, then the relative immobilized amounts on each of the two different polymer patterns were estimated by the fluorescence intensity (Takahashi et al., 2022b). HyLyteFluor488-labeled bovine fibronectins (Cytoskeleton, Denver, CO, USA) were dissolved in phosphate buffered saline (PBS) (10 μg/mL), and incubated with the micropatterned culture substrate at 37°C for 6 h. After being washed thoroughly with PBS, the difference in the protein immobilization on the micropatterns was observed using a fluorescence microscope (ECLIPSE Ti2-U) (Nikon, Tokyo, Japan).
2.5. Structural and functional maturation of bovine muscle tissue
To produce functionally mature muscle tissue with fibrin-based gel, 1-year cryopreserved bovine myogenic cells were grown to the second passages, and then seeded at a density of 1 × 106 cells/dish onto the micropatterned thermoresponsive culture substrate (irradiation time: 20 min, seeding area: 15 × 15 mm) (Takahashi et al., 2018, 2022a,b). The bovine cells aligned on the patterned substrate were cultured in growth medium for 4 days. Next, to transfer the aligned muscle cells to a fibrin-based gel, a mixture of fibrinogen (from bovine plasma, 20 mg/mL, 2 mL) (Sigma-Aldrich), thrombin (from bovine plasma, 20 U/mL, 500 μL) (Sigma-Aldrich), CaCl2 solution (8 mM, 1 mL), and Matrigel (500 μL) (Corning, Corning, NY, USA) or Basement Membrane Extract (500 μL) (R&D Systems, Inc., Minneapolis, MN, USA), was poured onto the cells (900 μL per a dish). Finally, a square-shaped silicon ring was inserted into the mixture to prevent gel shrinkage and incubated for 30 min at 37°C. After the gel formation was complete, a growth medium [10% FBS-DMEM with anti-fibrinolytic agents, aprotinin (500 KIU/mL, from bovine lung) (FUJIFILM Wako Pure Chemical) and 6-aminocaproic acid (2 mg/mL) (FUJIFILM Wako Pure Chemical)] was added to the culture dish. After 4 days, the medium was replaced with a differentiation medium (DMEM with 2% horse serum (HS; Thermo Fisher Scientific, Waltham, MA, USA) and anti-fibrinolytic agents) and cultured for 7 days. To release the bovine muscle tissue from the culture substrate, the samples were incubated at 20°C for 30 min, and then placed upside down in the growth medium on a 6-well culture plate (Takahashi and Okano, 2015, 2019).
2.6. Immunofluorescence staining
To assess the differentiation ability of cells at passages 3, 6, and 9, the cells were washed three times with PBS, and fixed with 4% paraformaldehyde for 15 min at RT. After washing with PBS, the cells were permeabilized with 0.5% Triton-X solution (Sigma-Aldrich) for 15–30 min at RT. The cells were then washed three times with PBS, incubated in blocking solution [2% bovine serum albumin (BSA) (Sigma-Aldrich)] for 30 min−1 h at RT, and treated with anti-desmin antibody (1:1,000) (MA5-13259, Thermo Fisher Scientific) diluted with 2% BSA at 4°C overnight. After washing with PBS, the cells were treated with fluorescently labeled secondary antibodies (1:1,000) (Thermo Fisher Scientific) for 30–45 min at RT and then incubated with Hoechst 33258 (Dojindo Laboratories, Kumamoto, Japan) for 5 min at RT while being gently stirred. The areas of fluorescent regions were analyzed using ImageJ software (Takahashi et al., 2022b). Muscle tissues with the fibrin-based gel were fixed with 2% paraformaldehyde overnight at 4°C (Takahashi et al., 2018, 2022a,b). After fixation, samples were incubated in blocking solution (5% BSA with 0.2% Triton-X 100) for 12 h at RT. The tissue samples were treated with anti-sarcomeric α-actinin antibody (1:500) (ab9465, Abcam, Cambridge, MA, USA) at 4°C overnight, and then with fluorescently labeled secondary antibodies (1:1,000) for 2 h at RT. For nuclei staining, tissue samples were incubated with Hoechst 33258 for 10 min at RT. Between all steps, samples were washed three times with PBS. Images were acquired using an FV1200 inverted confocal microscope and analyzed using FV10-ASW software.
2.7. Cell viability assay
To determine viability of bovine myogenic cells after cryopreservation for 1 year, trypan blue staining was performed. The frozen cells were thawed using a water bath and the cell suspension was added to 4 mL of DMEM containing 10% FBS. Subsequently, the diluted solution was mixed at 1:1 with 0.2% trypan blue solution, then stained and unstained cells were counted using a cell counting chamber. This cell viability assay was independently performed for each primary culture (n = 3).
2.8. Microscopic observation of bovine muscle tissue contractions
To induce muscle contraction, bovine muscle tissues were electrically stimulated at 28 days after the seeding onto the culture dish. Two carbon electrodes (C-Dish; IonOptix, Milton, MA, USA) were immersed into the medium, then an electrical pulse stimulation (EPS) was applied (voltage: 10 V, frequency: 1 Hz, duration time: 10 msec) using an electrical pulse generator (IonOptix). The EPS-induced muscle contraction was recorded at 30 frames/sec by a CCD camera with phase-contrast microscopy. The resulting tissue contraction distances were calculated using the motion analysis tool ViewPoint (Glenallan Technology Inc., Clinton, NY, USA) (Takahashi et al., 2018, 2022a,b).
2.9. Statistical analysis
Date are expressed as the mean +/− standard error. Multiple comparisons were analyzed by one-way ANOVA (Figures 4C, 5B) or two-way ANOVA (Figures 2B, C, 3A–C, 6D). Statistical significance was considered at *P < 0.05. Statistical processing was performed using R Software.
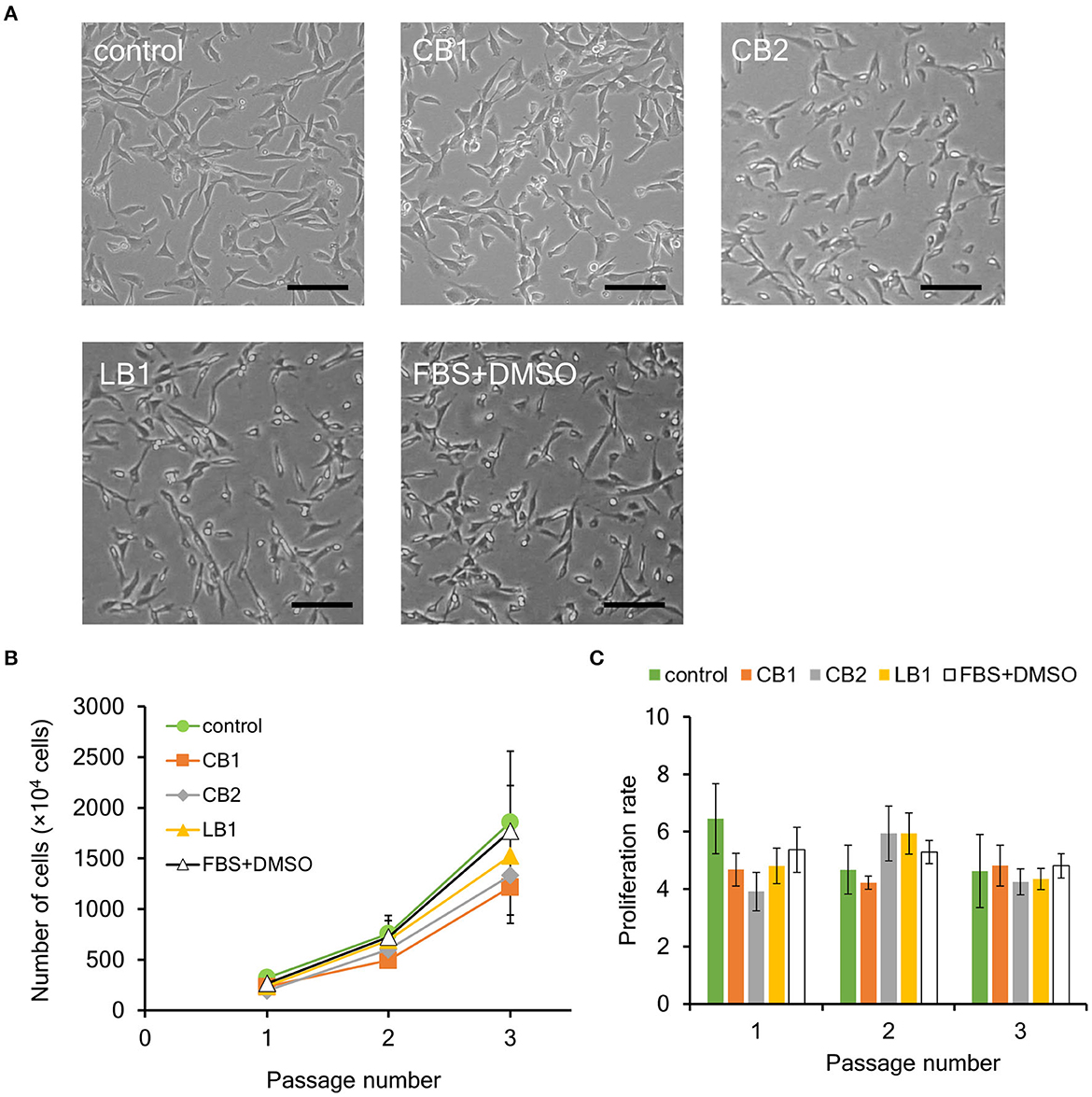
Figure 2. Effect of cryopreservation on proliferation ability of bovine myogenic cells. Primary cells were frozen with different cell freezing media [CELLBANKER 1 (CB1), CELLBANKER 2 (CB2), LABO Banker 1 (LB1), and the mixture of 90% FBS and 10% DMSO (FBS+DMSO)]. (A) Representative microscopic images of myogenic cells at passage 1 with or without cryopreservation (control). The cells were frozen for 1 week and then cultured in a dish after thawing. Scale bar: 100 μm. (B) Proliferation profiles of bovine myogenic cells with or without cryopreservation using CB1, CB2, LB1, and FBS+DMSO from passages 1 to 3 (n = 3). Green circle: control (without cryopreservation), orange square: CB1, gray rhombus: CB2, yellow triangle: LB1, white triangle: FBS+DMSO. (C) Proliferation rate of bovine myogenic cells at each passage with or without cryopreservation (n = 3). Green: control (without cryopreservation), orange: CB1, gray: CB2, yellow: LB1, white: FBS+DMSO.
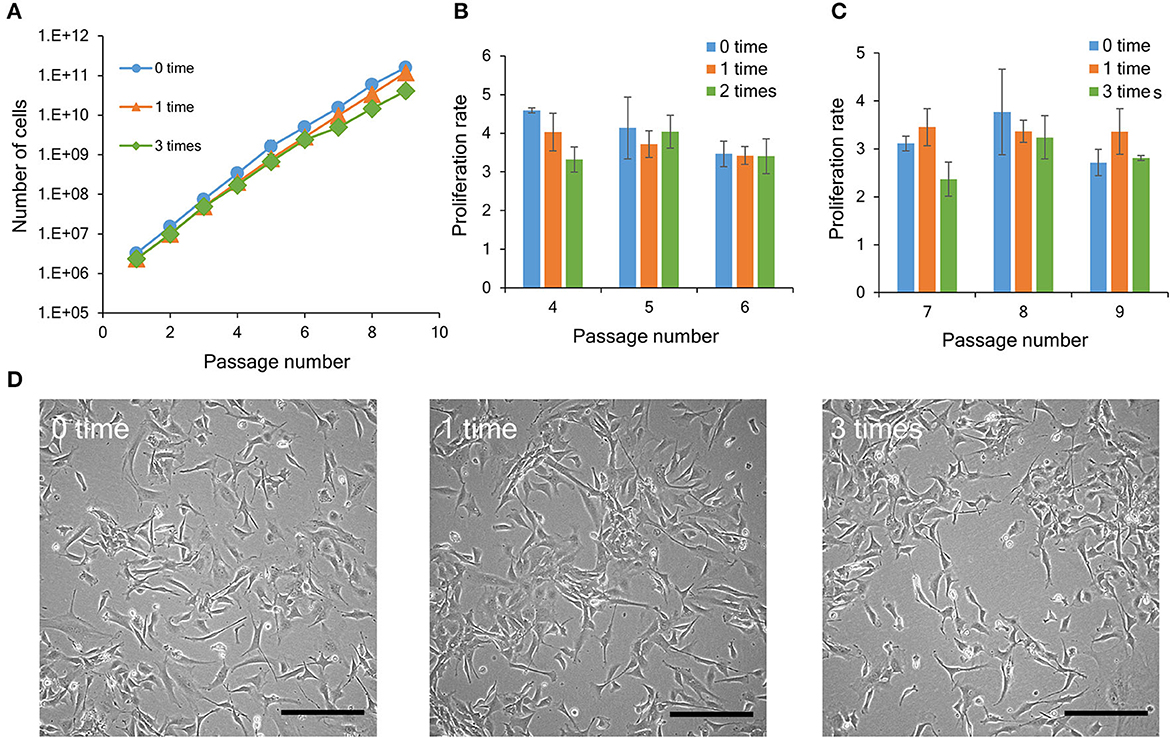
Figure 3. Proliferation ability of bovine myogenic cells with or without repeated cryopreservation. (A) Proliferation profiles of bovine myogenic cells cryopreserved 0, 1, or 3 times from passages 1 to 9 (n = 3). Blue circle: 0 time (without cryopreservation), orange triangle: 1 time, green rhombus: 3 times. (B) Proliferation rate of bovine myogenic cells cryopreserved 0, 1, or 2 times from passages 4 to 6 (n = 3). Blue: 0 time, orange: 1 time, green: 2 times. (C) Proliferation rate of bovine myogenic cells cryopreserved 0, 1, or 3 times from passages 7 to 9 (n = 3). Blue: 0 time, orange: 1 time, green: 3 times. (D) Representative microscopic images of adherent bovine myogenic cells at passage 9 after cryopreservation (0, 1, or 3 times). Scale bar: 200 μm.
3. Results
3.1. Effect of cell freezing media on proliferation ability of bovine myogenic cells
We have previously established a highly efficient primary culture method of bovine myogenic cells for cultured meat production (Takahashi et al., 2022b). In this study, to enhance the value of our bovine myogenic cells as a cell source for cultured meat, we focused on the feasibility to cryopreserve them while maintaining their quality as a cell source. Since proliferation ability is one of the most important properties for a cell source, we first determined the impact of cryopreservation on the proliferation of bovine myogenic cells harvested using our primary culture method.
There are two key factors to consider for cryopreservation: freezing temperature and medium (Whaley et al., 2021). In this study, cryopreservation was performed using a freezer set to −80°C, while there are other procedures enabling freezing cells at even lower temperatures. This is important to increase the versatility of our result by avoiding the use of specialized equipment such as a liquid nitrogen tank (Miyamoto et al., 2018). In this study, three commercially available freezing media (CB1, CB2, and LB1) were used as representative media (Yokonishi et al., 2014; Jang et al., 2017; Balci-Hayta et al., 2018; Naraoka et al., 2021). CB1 and LB1 from two different companies have been widely used to cryopreserve various kinds of mammalian cells, including bovine cells. To determine influence of serum on cryopreservation, CB2 was also used instead of CB1. In addition, the mixture of 90% FBS and 10% DMSO (FBS+DMSO) was also used as a traditional freezing medium (Balci-Hayta et al., 2018). First, bovine myogenic cells were harvested from bovine meat and seeded to culture dishes by using our primary culture method. After 6 or 7 days, the cells were cryopreserved once for 1 week with each of the four different freezing media. In order to evaluate the proliferation ability, the cells were thawed and cultured from passages 1 to 3. Since primary bovine cells were unable to adhere on a normal culture dish, the culture dish was coated with iMatrix-511. On the other hand, the passaged cells adhered on a culture dish without any biomolecule coating. Therefore, in this study, bovine cells were cultured on normal culture dishes to evaluate their proliferation ability. As shown in Figure 2A, bovine cells at passage 1 after the cryopreservation (CB1, CB2, LB1, and FBS+DMSO) similarly adhered on a culture dish as the cells without cryopreservation (control). In addition, all the cells after the 1-week cryopreservation proliferated similarly to the control samples (Figure 2B). Moreover, the proliferation rate of the cells also showed no significant difference among them (Figure 2C). These results indicated that the cryopreservation had no impact on the proliferation ability of the bovine myogenic cells. In addition, each freezing medium could be used to cryopreserve our bovine myogenic cells without any severe damage.
3.2. Influence of repeated cryopreservation on proliferation ability of bovine myogenic cells
To investigate the influence of repeated cryopreservation on bovine myogenic cells, the cells were cryopreserved at passages 0, 3, and 6 (Figure 1B). Consequently, during the passaging procedure (nine passages), the “1 week × 3 times” samples had three kinds of cryopreservation conditions [number of cryopreservation: 1 time (P1–P3), 2 times (P4–P6), and 3 times (P7–P9)]. Since the result in Figure 2 indicated that the four freezing media were equally acceptable for 1-week cryopreservation, this experiment was performed using CB1 as representative freezing medium. The primary cells without cryopreservation (“0-time” group) continued to proliferate during several passages. On the other hand, the bovine cells showed the same proliferation ability after 1-time or 3-time cryopreservation (Figure 3A). This result indicated that bovine myogenic cells were able to be expanded even after they were repeatedly cryopreserved three times. As shown in Figure 1B, in this experiment the 3-time samples actually underwent 1-time and 2-time cryopreserved stages. In order to examine the impact of 2-time cryopreservation, the proliferation rates of the cells at passages 4, 5 and 6 were compared. As a result, the proliferation rates showed no significant difference among the samples (Figure 3B). In addition, when the proliferation rates of the cells at passages 7, 8, and 9 were compared, there was also no significant difference among the samples cryopreserved 0, 1, and 3 times (Figure 3C). Representative microscopic images of adherent cells for the three samples (control, “1 week × 1 time”, and “1 week × 3 times”) at passage 9 show similar numbers and forms of bovine myogenic cells for each sample (Figure 3D). These results suggested that the repeated cryopreservation did not affect the proliferation ability of bovine myogenic cells, at least until three times of freezing and thawing. This consistent proliferation ability is important when considering a cell source for cultured meat production.
3.3. Effect of cell freezing media on differentiation ability of bovine myogenic cells
One of the main characteristics of myogenic cells as a muscle precursor cell is their ability to differentiate into myotubes. As described above, cryopreservation had no severe impact on the proliferation ability of bovine myogenic cells harvested using our primary culture method. However, to be used for cultured meat, muscle precursor cells also need to undergo cell fusion and become muscle fibers in order to more closely mimic the microstructures of native muscle (Furuhashi et al., 2021; Messmer et al., 2022; Takahashi et al., 2022b). Thus, we also evaluated the impact of cryopreservation on the differentiation ability of bovine myogenic cells. In general, myogenic cells are cultured in low serum medium (e.g., 2% horse serum containing medium) to promote differentiation into myotubes (Dennis et al., 2001; Cai et al., 2018). As reported previously, however, our bovine cells quickly formed myotubes and then detached from culture dishes (Takahashi et al., 2022b). Although this result indicates that our cells have a high differentiation ability, the detachment makes it difficult to determine the differentiation efficiency of the myogenic cells. Therefore, to prevent the quick detachment, the cells were cultured in a growth medium (10% FBS containing DMEM) in this study. Even in the growth medium, the bovine myogenic cells showed myotube formation just by culturing the cells at confluence (Figure 4A). This consequence indicated that our bovine cells at passage 3 without cryopreservation had a high differentiation ability. Moreover, the cells after 1-week cryopreservation with CB1 also showed similar myotube formation at passage 3 (Figure 4A). In this study, desmin-positive bovine myotubes were fluorescently stained to evaluate the areas of differentiated myotubes. Figure 4B shows the myotubes formed by the cells after 1-week cryopreservation with CB1 to represent the other cryopreserved samples. These fluorescence images showed similar myotube formations at passage 3 by the cells with and without the cryopreservation. In addition, to quantitatively determine the impacts of cryopreservation, the areas of desmin-positive myotubes formed at passage 3 were analyzed by using ImageJ software. The area of myotubes formed from myogenic cells without cryopreservation was 35.9%, and this was not significantly different from those after cryopreservation with each of the four kinds of cell-freezing media (Figure 4C). Therefore, the cryopreservation with these representative freezing media had no impact on the differentiation ability of bovine myogenic cells, at least until passage 3.
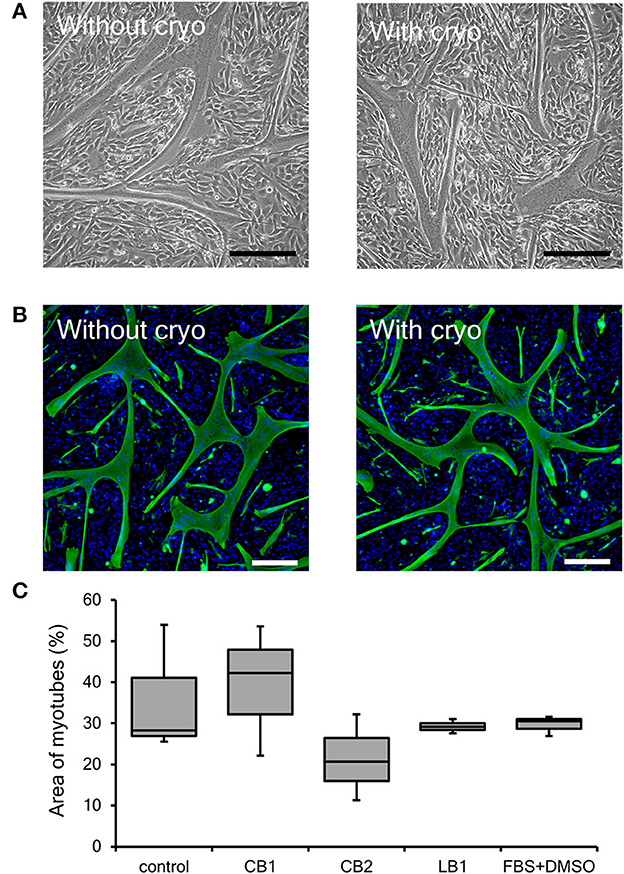
Figure 4. Differentiation ability of bovine myogenic cells frozen with representative cell freezing media. CELLBANKER 1 (CB1), CELLBANKER 2 (CB2), LABO Banker 1 (LB1), and FBS+DMSO were used as cell freezing media. (A) Representative microscopic images of myotubes differentiated from bovine cells with or without 1-week cryopreservation using CB1 at passage 3. Scale bar: 200 μm. (B) Representative fluorescence images of myotubes differentiated from bovine cells with or without 1-week cryopreservation using CB1 at passage 3. Green: desmin, blue: nuclei. Scale bar: 200 μm. (C) Areas of desmin-positive myotubes formed by bovine myogenic cells with or without cryopreservation using each of the four kinds of cell-freezing media (n = 3).
3.4. Influence of repeated cryopreservation on differentiation ability of bovine myogenic cells
In general, the differentiation ability of cultured cells is gradually reduced by repeated passaging (Sabourin et al., 1999; Machida et al., 2004). On the other hand, in our previous study, bovine myogenic cells harvested using our primary culture method showed a consistent differentiation ability even after several passages (Takahashi et al., 2022b). In this study, we confirmed the impact of repeated cryopreservation on differentiation ability of myogenic cells up to passage 9. As shown in Figure 1B, cryopreservation was repeatedly performed three times during nine passages and the cells at P3, P6, and P9 were cultured at 100% confluency to induce myotube differentiation. The impact of cryopreservation on differentiation efficiency was estimated by comparing them to the cells without cryopreservation (0 time), 1-time (1 time), and 3-time cryopreservation (3 times). The representative fluorescence images of differentiated myotubes for the three groups exhibited similar forms of myotubes (Figure 5A). This result implies that the bovine myogenic cells maintained its differentiation ability at passage 9, even after they were cryopreserved three times. In order to quantitatively verify this implication, the areas of myotubes were calculated by using Image J software (Figure 5B). The average areas of myotubes for each condition were 17.0% (0 time), 23.2% (1 time), and 13.1% (3 times). Since there was no significant difference among the three groups, it was confirmed the repeated cryopreservation up to three times of freezing and thawing did not have a negative impact on the differentiation ability of bovine myogenic cells.
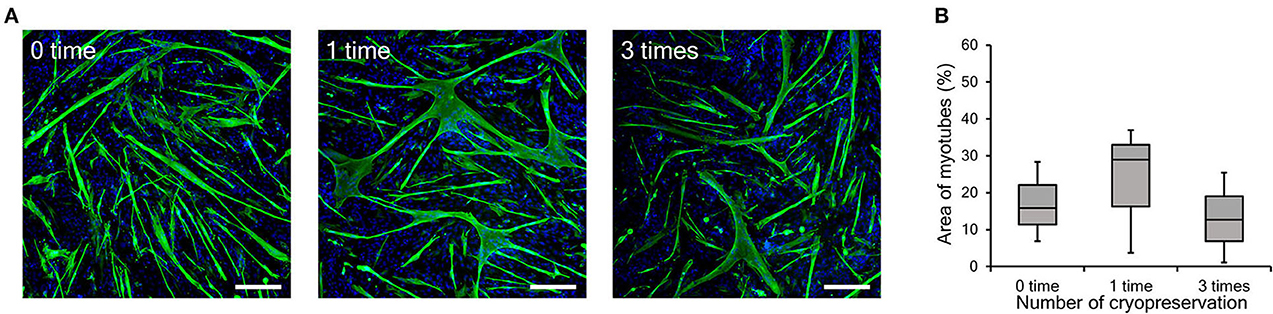
Figure 5. Myotube formation of bovine myogenic cells at passage 9 after cryopreservation (0, 1, or 3 times). (A) Representative fluorescence images of differentiated myotubes from bovine myogenic cells cryopreserved 0, 1, or 3 times. Scale bar: 200 μm. (B) Areas of desmin-positive myotubes formed from bovine myogenic cells cryopreserved 0, 1, or 3 times (n = 3).
3.5. Cryopreservation period of bovine myogenic cells while maintaining cell quality
In this study, to evaluate the impact of repeated cryopreservation using the same primary cells harvested from one piece of meat (the same lot), the bovine myogenic cells were cryopreserved each time for 1 week. On the other hand, the length of the preservation period that maintains cell properties is one of the most important factors for cryopreservation applications (Miyamoto et al., 2018; Taylor et al., 2019). Therefore, in this study, bovine myogenic cells were also cryopreserved with CB1 for 1 year, and then the impact on cell quality was evaluated by the same criteria: proliferation and differentiation abilities. After freezing and thawing, the vitality of the bovine myogenic cells was 97.9 +/– 0.5 %, and the myogenic cells adhered on a culture dish similar to cells without cryopreservation (Figures 2A, 6A). In addition, they continued to proliferate during the nine passages even after cryopreservation for 1 year (Figure 6B). Although the 1-year samples came from different primary cells that were cultured on a different date from those for the 1-week samples, the proliferation profile of “1-year samples” was not significantly different compared to that of the “1-week samples” (Figure 3A). These results suggest that cryopreservation caused no impacts on the proliferation ability of bovine myogenic cells at least until 1-year cryopreservation. In addition, to confirm differentiation ability, the proliferating cells were harvested at P3, P6, and P9, and seeded to culture dishes for myotube formation. Fluorescence images of myotubes differentiated from the 1-year samples at P3, P6, and P9 all showed similar myotube formation (Figure 6C). The average areas of myotubes at P3, P6, and P9 were 31.3, 28.3, and 24.9%, respectively (Figure 6D). Statistically, there was no significant difference between P3, P6, and P9. This result implies that the bovine myogenic cells maintained their differentiation ability during the repeated passages (at least until P9) even after they were frozen for 1 year. Moreover, when the areas of myotube formation were compared with those of 1-week cryopreservation samples, there was no statistically significant difference (Figure 6D). These results lead us to conclude that even when our bovine myogenic cells were frozen for a longer period, cryopreservation caused no severe damage to the proliferation or differentiation ability of bovine myogenic cells for at least 1 year of cryopreservation. Although in this study cryopreservation was trialed for only 1 week and 1 year, Figure 6 indicates that cryopreservation for several weeks or even several months also had no negative impact on the cell abilities.
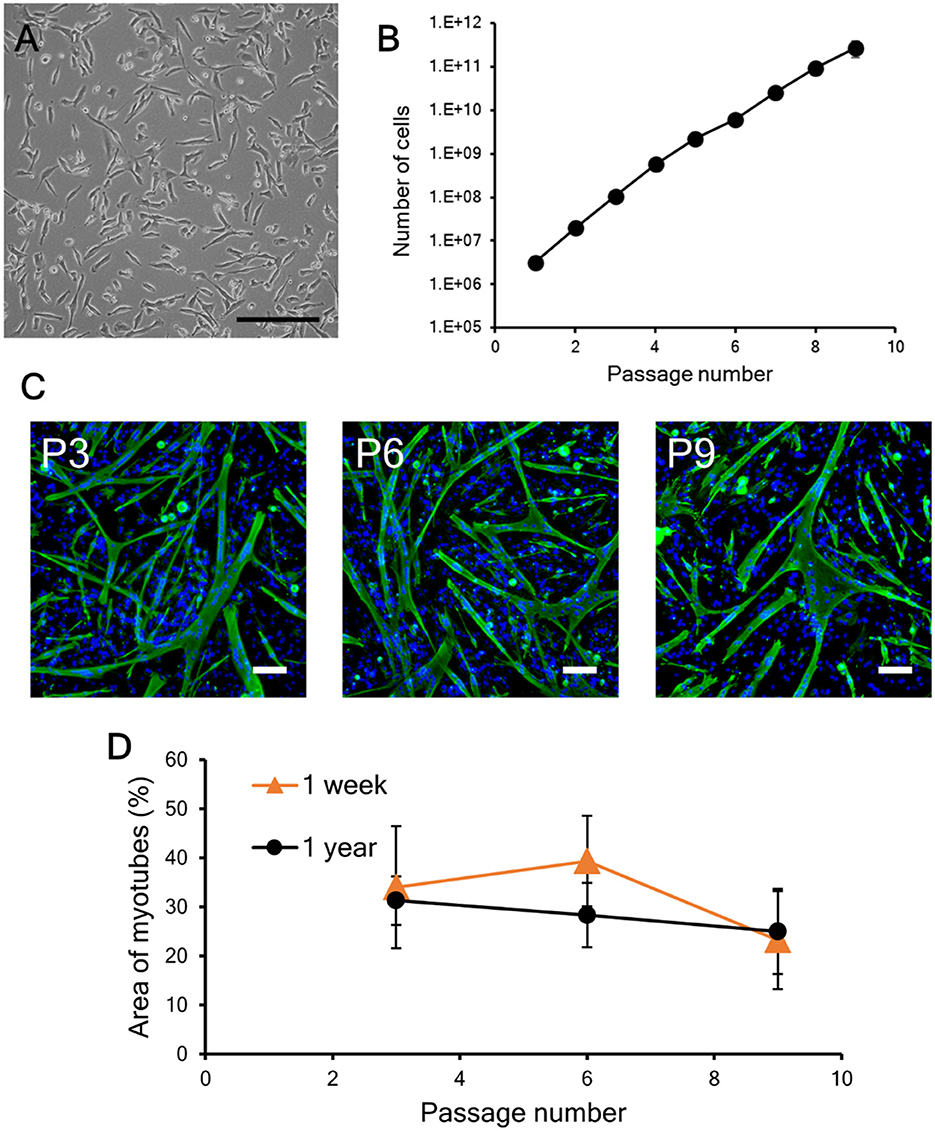
Figure 6. Proliferation and differentiation of bovine myogenic cells cryopreserved for 1 year. (A) Microscopic image of myogenic cells at passage 1 after cryopreservation. The cells were frozen with CB1 for 1 year and then cultured in a dish after thawing. Scale bar: 200 μm. (B) Proliferation profile of 1-year cryopreserved bovine myogenic cells from passages 1 to 9 (n = 4). (C) Representative fluorescence images of myotubes differentiated from myogenic cells at passages 3 (P3), 6 (P6), and 9 (P9) after cryopreservation for 1 year (green: desmin, blue: nuclei). Scale bar: 200 μm. (D) Areas of myotubes formed by bovine myogenic cells cryopreserved for 1 week (n = 3) and for 1 year (n = 4). Orange triangle: 1 week, black circle: 1 year.
3.6. Functional maturation of bovine muscle tissue made from cells cryopreserved for 1 year
Native skeletal muscle has the potential to form sarcomere structures and generate contractile force in its maturation process. Our previous study confirmed that bovine myogenic cells that did not undergo cryopreservation formed sarcomere structures and showed muscle contraction (Takahashi et al., 2022b). These next-step maturations are necessary for bovine cells sourced for cultured meat to mimic native bovine muscles and have the ability to contract. In this study, we further confirmed that after cryopreservation bovine myogenic cells had the ability to functionally mature into contractile muscle fibers. To mimic the aligned structure of native muscle, bovine myogenic cells were first seeded onto a micropatterned substrate. In our previous study, bovine myogenic cells without cryopreservation formed an aligned structure on the substrate (Takahashi et al., 2022b). In this study, the micropatterns were fabricated through the same procedure (Figure 7A). We previously confirmed that the micropatterns were fabricated at a nanoscale thickness (Takahashi et al., 2011). Therefore, the bovine cells were unable to recognize the difference in thickness between the two pattern regions. On the other hand, the fluorescently-labeled protein allowed us to visualize that the two kinds of micropatterns had different affinities for protein immobilization (Figure 7B). The bovine cells recognized the difference in immobilized proteins underneath themselves, and then aligned their orientation on the substrate (Takahashi et al., 2022b). As reported previously, the cells after 1-year cryopreservation also formed an aligned structure on the substrate in the same direction as the micropatterns. After the aligned cells reached to confluence, using our thermally-induced cell detachment technique, the cells were transferred as a tissue sheet onto a fibrin-based gel promoting functional maturation (Figure 7C). The myogenic cells differentiated into myotubes while maintaining the aligned orientation (Figure 7D), and then the aligned myotubes were further cultured on the gel for the next-stage of maturation. Consequently, the aligned myotubes on the gel platform showed striated patterns of sarcomere structures (Figure 7E), which is necessary to produce muscle contraction. In fact, when the cells were electrically stimulated at a frequency of 1 Hz, the bovine tissue contracted according to the electrical stimulation (Supplementary Video 1). Displacements of the twitch contractions were ~15–20 μm (Figure 7F). These results indicate that even after 1 year of cryopreservation, our bovine myogenic cells had the ability to form sarcomere structures and produce muscle contraction. This ability is very important for cryopreserved cell sources to be effectively used for biomimetic bovine muscle tissue engineering.
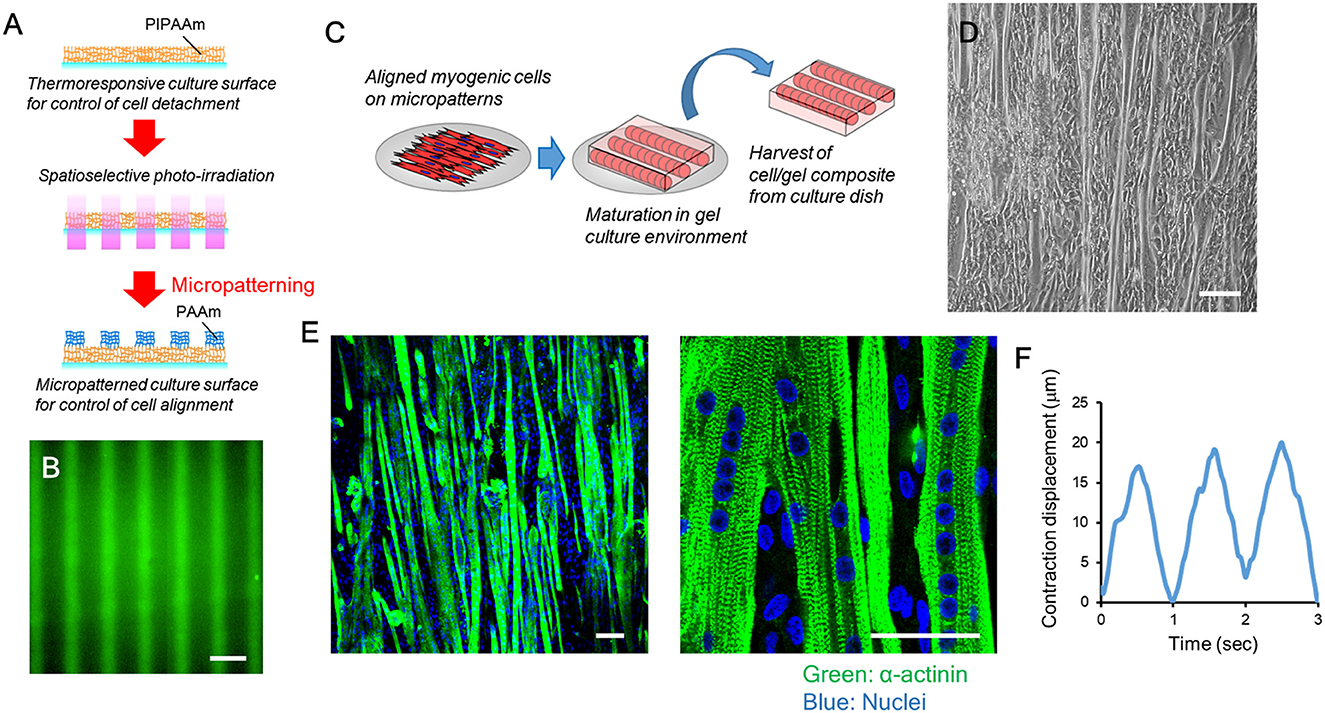
Figure 7. Functional maturation of bovine muscle tissue formed from myogenic cells cryopreserved for 1 year. (A) Schematic illustration of photo-induced micropatterning of PAAm on a thermoresponsive PIPAAm-grafted substrate. (B) Fluorescence image of fluorescently-labeled fibronectin immobilized on the micropatterned substrate. Scale bar: 100 μm. (C) Schematic illustration of the transfer process of aligned myogenic cells from a micropatterned substrate to a fibrin-based gel. The thermoresponsive substrate allows the aligned cells to release from the surface by lowering the culture temperature. After the cells attached to the fibrin-based gel, the cell/gel composite was incubated at 20°C for 30 min and then the construct was placed upside down to a new culture dish. (D) Microscopic image of aligned bovine myotubes on the micropatterned substrate. Scale bar: 100 μm. (E) Representative fluorescence images of functionally mature bovine muscle tissue (formed from cells cryopreserved for 1 year) with sarcomere formation (green: α-actinin, blue: nuclei). Scale bar: 50 μm. (F) Representative contraction displacement profile of a bovine muscle tissue. Electrical stimulation was applied at a frequency of 1 Hz.
4. Discussion
Cultured meat has recently attracted the attention of the world because of the low environmental burden and absence of ethical concerns, compared to the current livestock industry (Tuomisto and Teixeira de Mattos, 2011; Post et al., 2020). The world has just seen the first industrial production of cultured meat, and future proliferation of the technologies for cultured meat production will need to rely on a stable supply of cells such as bovine myogenic cells. We have previously reported on a method to collect primary bovine cells, and also demonstrated that the bovine myogenic cells had a high proliferation ability and showed potential to become a source of high-quality cells to be used in cultured meat production (Takahashi et al., 2022b). Therefore, we believe that our myogenic cells will have an important role in the future of meat technologies. From this background, our study focused on the feasibility to cryopreserve quality-controlled bovine myogenic cells. Cryopreservation has been widely used for various kinds of cell culture experiments in the fields of biochemistry and medical research to store mammalian cells (Wennerholm et al., 2009; Pashaiasl et al., 2013; Miyamoto et al., 2018). Similarly, in studies of cultured meat production, cryopreservation will be a powerful tool for storage of high-quality cells to produce beef, pork, and chicken; however, primary cells immediately harvested from meat have the best quality (Kaur and Dufour, 2012). Therefore, to confidently apply cryopreservation in studies of cultured meat production, we first needed to experimentally confirm that a specific cryopreservation process would maintain the quality of bovine myogenic cells harvested using our primary culture method. This study demonstrated that the fundamental qualities of our bovine myogenic cells were successfully maintained even after repeated or year-long cryopreservation. To the best of our knowledge, this study is the first to clarify the influence of the number of time and the length of cryopreservation on the quality of primary bovine cells to be used in cultured meat production.
There are several commercially available cell freezing media (Yokonishi et al., 2014; Balci-Hayta et al., 2018; Fu et al., 2020; Naraoka et al., 2021). In this study, three representative media were selected to compare the effect of cryopreservation. We found that there was no significant difference between the three kinds of commercial products (CB1, CB2, and LB1). More importantly, the traditional cell freezing medium, a mixture of FBS and DMSO was also similarly suitable for cryopreserving our bovine myogenic cells. That is, any specific freezing medium is not required to preserve the quality of our bovine myogenic cells. On the other hand, DMSO or similar components are typically contained in freezing medium to prevent cells from severe damage, and ideally the use of these components should be avoided in cultured meat production. Therefore, the risks and benefits of cryopreservation need to be further discussed to regulate the use of cultured food production in the future, although we strongly believe that these components must be removed from medium during the culture process. In addition, this study demonstrated that the commonly used freezing method (suspending in a cryovial and cooling in a freezer at −80°C) was suitable for storing the cells, which means that it is not necessary to use liquid nitrogen and a controlled-rate freezer. Although we have not yet confirmed that the cells were able to be cryopreserved under mild conditions such as in a freezer at −20°C without severe damage for 1 year, this study does show that no special equipment for freezing the cells at lower temperatures than −80°C (e.g., liquid nitrogen tank) are required. This application will be advantageous to expand high-quality cell sources for cultured meat production all around the world.
We also investigated the influence of cryopreservation focusing on the two key factors, freezing period and number of repeated freezing. To demonstrate the possibility of long-term cell cryopreservation, it was confirmed that cryopreservation for 1 year caused no negative effects on the proliferation or differentiation abilities of bovine myogenic cells. This result would allow frozen bovine cells to be sent to remote places by commonly used refrigerated transport for commercial cell products or stored for up to 1 year when supplies are unreliable for sustainable production of cultured meat in the near future. Thus, this result is meaningful in terms of delivering a source of high-quality cells for cultured meat anywhere in the world. This will contribute to solving regional food crises in the future due for example to extreme weather. This study confirmed that repeated cryopreservation did not decrease the quality of bovine cells so researchers do not need to avoid multiple freezing and thawing steps in future studies. It is well known that to store protein samples in the long-term repeated freezing and thawing should be avoided since the both processes can cause severe damage to proteins (Cao et al., 2003; Jain et al., 2021). Similarly, researchers generally refrain from repeatedly freezing cells, even if the cells are still alive after the procedures (Jang et al., 2017). Contrary to accepted methods, our study demonstrated that the quality of primary bovine myogenic cells was maintained after cryopreservation for at least three times, which now makes cryopreservation more useful to researchers. Currently, researchers have not established a concrete method for industrial production of cultured meat so it is not always practical to prepare the exact necessary amounts of materials such as cells and media. Now, when there are surplus bovine cells, they can simply be frozen and used again for future studies. This repeated usage makes more efficient use of the materials for cultured meat and prevents wastage, which will eventually lead to sustainable cultured meat production.
We have now confirmed that cryopreservation will allow us to use bovine myogenic cells whenever needed (within 1 year) and as many times (3 times or less) as required by just thawing and refreezing cells, which will make the stock of high-quality bovine cells more useful and stable. Another possibility is that when we find any ideal cells from selected livestock, we will be able to enjoy meat made from these animal cells anytime by cryopreserving the cells beforehand and thawing them when more meat is required. In addition, this study suggested that the frequency of primary culture can be reduced. This is meaningful in terms of reducing not only the time and effort required for primary culture, but also the number of sacrifice animals from which muscle tissues are obtained. Even though we still need to investigate further to understand the expiration period (the limitation of freezing period) of high-quality cell sources, the findings in this study are sufficient for researchers to accelerate their experiments of cultured meat production.
We believe that our native-like tissue production process (growth from muscle progenitor cells to maturated myofibers) can replicate the native-like texture of meat and possibly mimic as-yet recognized native-like nutrients inside cultured meat. Our previous study reported that our tissue engineering method had the potential to produce “tissue-engineered meat”, which is nearly indistinguishable from native muscle tissue (Takahashi et al., 2022b). Based on our tissue engineering technique, this study demonstrated that our cryopreserved bovine myogenic cells had the ability to form sarcomere structures and produce muscle contraction, even after they were frozen for 1 year. However, there is still room for improvement to make muscle tissue indistinguishable from native muscle. In particular, although this study produced cell sheet-based muscle tissue with biomimetic microstructures and function, future cultured meat needs to be scaled up to be acceptable as a popular food item. In our previous study, we demonstrated that 3D bovine muscle tissue was producible by layering multiple muscle sheet tissues (Tanaka et al., 2022). Therefore, this tissue engineering method will be a promising approach for scaling-up of future cultured meat. On the other hand, this technique is time-consuming to prepare a large number of muscle sheet tissues and stack them one-by-one. Therefore, in future work this limitation needs to be solved to produce biomimetic cultured meat on a large scale. In addition, the muscle contractions produced by our bovine muscle tissue need to be improved in order to better mimic the texture of conventional meat. Further research is also needed to identify the nutrients included in our bovine muscle tissue. Lastly, in the future some other quality check approaches, such as the expressions of specific proteins in the bovine cells might be necessary to examine if the nature of cells is affected in some other way by cryopreservation. Although the bovine muscle tissues made here require more mature structures and functions in order to better mimic native muscle tissue more closely; our verification showed the potential to produce biomimetic cultured meat, not just an aggregation of bovine myogenic cells. The functional maturation reported in this study gives us a clue to understand how bovine cells can be frozen while maintaining their important properties.
5. Conclusion
This study examined the feasibility to cryopreserve bovine myogenic cells at −80°C while maintaining the cell quality of the cell source in cultured meat. The four representative cell freezing media (CB1, CB2, LB1, and FBS+DMSO) caused no negative influence on the proliferation and differentiation abilities of bovine myogenic cells up to passage 3. This suggests that no specially prepared cell freezing medium is needed to preserve the cell quality. In addition, the repeated (up to 3 times) cryopreservation did not cause any severe damage in either of the important abilities of bovine myogenic cells. Moreover, long-term (1-year) cryopreservation was able to maintain the quality of bovine myogenic cells. These results not only make the cryopreservation more useful, but also make it possible to deliver the quality-controlled frozen cell source at any time they are needed anywhere in the world. Lastly, our study demonstrated that the 1-year cryopreserved cells had the ability to form sarcomere structures and produce muscle contraction. This verification showed the possibility to produce native-like bovine tissue from cryopreserved cells. While many researchers are investigating innovative technologies for future meat production, fundamental techniques such as the cryopreservation process must be developed first to advance the industry. For instance, in the field of medical and life science research, cell banks are created in order to ensure the quality, safety, and traceability of cryopreserved cells (Cobo et al., 2005; Soice and Johnston, 2021). Our findings, cryopreservation of quality-controlled bovine cells, will support the establishment of cell banks for cultured meat production. The success of repeated and long-term cryopreservation of bovine myogenic cells is an important step to scaling-up the mass production of cultured meat in the near future.
Data availability statement
The datasets presented in this article are not readily available because the datasets generated during and/or analyzed during the current study are available from the corresponding author on reasonable request. Requests to access the datasets should be directed to HT, takahashi.hironobu@twmu.ac.jp.
Author contributions
Conceptualization: AY and HT. Methodology and writing—original draft: RK, AY, and HT. Data analysis: RK and AY. Writing—review and editing: TS. All authors contributed to the article and approved the submitted version.
Funding
This work was partially supported by JST-Mirai Program Grant Number JPMJMI20C1 and JSPS KAKENHI Grant Number 22K18931, Japan.
Acknowledgments
We gratefully acknowledge Dr. Kazuhide Nakajima (Tokyo Meat Market Co., Ltd.) for his kind support. We also thank Mr. Allan Nisbet for his useful comments and editorial assistance.
Conflict of interest
TS is a stakeholder in CellSeed Inc. Tokyo Women's Medical University receives research funds from CellSeed Inc.
The remaining authors declare that the research was conducted in the absence of any commercial or financial relationships that could be construed as a potential conflict of interest.
Publisher's note
All claims expressed in this article are solely those of the authors and do not necessarily represent those of their affiliated organizations, or those of the publisher, the editors and the reviewers. Any product that may be evaluated in this article, or claim that may be made by its manufacturer, is not guaranteed or endorsed by the publisher.
Supplementary material
The Supplementary Material for this article can be found online at: https://www.frontiersin.org/articles/10.3389/fsufs.2023.1023057/full#supplementary-material
Supplementary Video 1. Twitch contraction of a bovine muscle tissue induced by EPS application at a frequency of 1 Hz. The muscle tissue was formed from myogenic cells cryopreserved for 1 year. The video is shown at a field of view of 1,470 × 827 μm.
References
Andreassen, R. C., Rønning, S. B., Solberg, N. T., Grønlien, K. G., Kristoffersen, K. A., Høst, V., et al. (2022). Production of food-grade microcarriers based on by-products from the food industry to facilitate the expansion of bovine skeletal muscle satellite cells for cultured meat production. Biomaterials 286, 121602. doi: 10.1016/j.biomaterials.2022.121602
Balci-Hayta, B., Bekircan-Kurt, C. E., Aksu, E., Dayangac-Erden, D., Tan, E., Erdem-Ozdamar, S., et al. (2018). Establishment of primary myoblast cell cultures from cryopreserved skeletal muscle biopsies to serve as a tool in related research and development studies. J. Neurol. Sci. 393, 100–104. doi: 10.1016/j.jns.2018.08.018
Baquero-Perez, B., Kuchipudi, S. V., Nelli, R. K., and Chang, K.-C.. (2012). A simplified but robust method for the isolation of avian and mammalian muscle satellite cells. BMC Cell Biol. 13, 16. doi: 10.1186/1471-2121-13-16
Cai, A., Hardt, M., Schneider, P., Schmid, R., Lange, C., Dippold, D., et al. (2018). Myogenic differentiation of primary myoblasts and mesenchymal stromal cells under serum-free conditions on PCL-collagen I-nanoscaffolds. BMC Biotechnol. 18, 75. doi: 10.1186/s12896-018-0482-6
Cao, E., Chen, Y., Cui, Z., and Foster, P. R. (2003). Effect of freezing and thawing rates on denaturation of proteins in aqueous solutions. Biotechnol. Bioeng. 82, 684–690. doi: 10.1002/bit.10612
Chong, M., Leung, A. K., and Lua, V. (2022). A cross-country investigation of social image motivation and acceptance of lab-grown meat in Singapore and the United States. Appetite 173, 105990. doi: 10.1016/j.appet.2022.105990
Chriki, S., and Hocquette, J. F. (2020). The Myth of Cultured Meat: A Review. Front. Nutr. 7, 7. doi: 10.3389/fnut.2020.00007
Cobo, F., Stacey, G. N., Hunt, C., Cabrera, C., Nieto, A., Montes, R., et al. (2005). Microbiological control in stem cell banks: approaches to standardisation. Appl. Microbiol. Biotechnol. 68, 456–466. doi: 10.1007/s00253-005-0062-2
Dennis, R. G., Paul, E., Kosnik, I., Gilbert, M. E., and Faulkner, J. A. (2001). Excitability and contractility of skeletal muscle engineered from primary cultures and cell lines. Ame. J. Physiol. Cell Physiol. 280, C288–C295. doi: 10.1152/ajpcell.2001.280.2.C288
Ding, S., Swennen, G. N. M., Messmer, T., Gagliardi, M., Molin, D. G. M., Li, C., et al. (2018). Maintaining bovine satellite cells stemness through p38 pathway. Sci. Rep. 8, 10808. doi: 10.1038/s41598-018-28746-7
Erol, O. D., Pervin, B., Seker, M. E., and Aerts-Kaya, F. (2021). Effects of storage media, supplements and cryopreservation methods on quality of stem cells. World J. Stem Cells 13, 1197–1214. doi: 10.4252/wjsc.v13.i9.1197
Fu, X., Xu, B., Jiang, J., Du, X., Yu, X., Yan, Y., et al. (2020). Effects of cryopreservation and long-term culture on biological characteristics and proteomic profiles of human umbilical cord-derived mesenchymal stem cells. Clin. Proteom. 17, 15. doi: 10.1186/s12014-020-09279-6
Furuhashi, M., Morimoto, Y., Shima, A., Nakamura, F., Ishikawa, H., Takeuchi, S., et al. (2021). Formation of contractile 3D bovine muscle tissue for construction of millimetre-thick cultured steak. NPJ Sci. Food 5, 6. doi: 10.1038/s41538-021-00090-7
Grant, L., Raman, R., Cvetkovic, C., Ferrall-Fairbanks, M. C., Pagan-Diaz, G. J., Hadley, P., et al. (2019). Long-term cryopreservation and revival of tissue-engineered skeletal muscle. Tissue Eng. Part A 25, 1023–1036. doi: 10.1089/ten.tea.2018.0202
Hunt, C. J. (2011). Cryopreservation of human stem cells for clinical application: a review. Transfusion Med. Hemother. 38, 107–123. doi: 10.1159/000326623
Jain, K., Salamat-Miller, N., and Taylor, K. (2021). Freeze–thaw characterization process to minimize aggregation and enable drug product manufacturing of protein based therapeutics. Sci. Rep. 11, 11332. doi: 10.1038/s41598-021-90772-9
Jang, T. H., Park, S. C., Yang, J. H., Kim, J. Y., Seok, J. H., Park, U. S., et al. (2017). Cryopreservation and its clinical applications. Integr. Med. Res. 6, 12–18. doi: 10.1016/j.imr.2016.12.001
Kang, D.-H., Louis, F., Liu, H., Shimoda, H., Nishiyama, Y., et al. (2021). Engineered whole cut meat-like tissue by the assembly of cell fibers using tendon-gel integrated bioprinting. Nat. Commun. 12, 5059. doi: 10.1038/s41467-021-25236-9
Kaur, G., and Dufour, J. M. (2012). Cell lines: valuable tools or useless artifacts. Spermatogenesis 2, 1–5. doi: 10.4161/spmg.19885
Kolkmann, A. M., Post, M. J., Rutjens, M. A. M., van Essen, A. L. M., and Moutsatsou, P. (2020). Serum-free media for the growth of primary bovine myoblasts. Cytotechnology 72, 111–120. doi: 10.1007/s10616-019-00361-y
Machida, S., Spangenburg, E. E., and Booth, F. W. (2004). Primary rat muscle progenitor cells have decreased proliferation and myotube formation during passages. Cell Prolif. 37, 267–277. doi: 10.1111/j.1365-2184.2004.00311.x
Melzener, L., Verzijden, K. E., Buijs, A. J., Post, M. J., and Flack, J. E. (2021). Cultured beef: from small biopsy to substantial quantity. J. Sci. Food Agricult. 101, 7–14. doi: 10.1002/jsfa.10663
Meneghel, J., Kilbride, P., and Morris, G. J. (2020). Cryopreservation as a key element in the successful delivery of cell-based therapies—a review. Front. Med. 7, 592242. doi: 10.3389/fmed.2020.592242
Messmer, T., Klevernic, I., Furquim, C., Ovchinnikova, E., Dogan, A., Cruz, H., et al. (2022). A serum-free media formulation for cultured meat production supports bovine satellite cell differentiation in the absence of serum starvation. Nat. Food 3, 74–85. doi: 10.1038/s43016-021-00419-1
Miyamoto, Y., Ikeuchi, M., Noguchi, H., and Hayashi, S. (2018). Long-term cryopreservation of human and other mammalian cells at −80°C for 8 Years. Cell Med. 10, 2155179017733148. doi: 10.1177/2155179017733148
Naraoka, Y., Mabuchi, Y., Yoneyama, Y., Suto, E. G., Hisamatsu, D., Ikeda, M., et al. (2021). Isolation and characterization of tissue resident CD29-positive progenitor cells in livestock to generate a three-dimensional meat bud. Cells 10, 2499. doi: 10.3390/cells10092499
Pashaiasl, M., Khodadadi, K., Richings, N. M., Holland, M. K., and Verma, P. J. (2013). Cryopreservation and long-term maintenance of bovine embryo-derived cell lines. Reprod. Fert. Dev. 25, 707–718. doi: 10.1071/RD12018
Post, M. J. (2012). Cultured meat from stem cells: challenges and prospects. Meat Sci. 92, 297–301. doi: 10.1016/j.meatsci.2012.04.008
Post, M. J. (2014). An alternative animal protein source: cultured beef. Ann. N. Y. Acad. Sci. 1328, 29–33. doi: 10.1111/nyas.12569
Post, M. J., Levenberg, S., Kaplan, D. L., Genovese, N., Fu, J., Bryant, C. J., et al. (2020). Scientific, sustainability and regulatory challenges of cultured meat. Nat. Food 1, 403–415. doi: 10.1038/s43016-020-0112-z
Sabourin, L. A., Girgis-Gabardo, A., Seale, P., Asakura, A., and Rudnicki, M. A. (1999). Reduced differentiation potential of primary MyoD-/- myogenic cells derived from adult skeletal muscle. J. Cell Biol. 144, 631–643. doi: 10.1083/jcb.144.4.631
Skrivergaard, S., Rasmussen, M. K., Therkildsen, M., and Young, J. F. (2021). Bovine satellite cells isolated after 2 and 5 days of tissue storage maintain the proliferative and myogenic capacity needed for cultured meat production. Int. J. Mol. Sci. 22, 8376. doi: 10.3390/ijms22168376
Soice, E., and Johnston, J. (2021). Immortalizing cells for human consumption. Int. J. Mol. Sci. 22, 11660. doi: 10.3390/ijms222111660
Takahashi, H., Nakayama, M., Itoga, K., Yamato, M., and Okano, T. (2011). Micropatterned thermoresponsive polymer brush surfaces for fabricating cell sheets with well-controlled orientational structures. Biomacromolecules 12, 1414–1418. doi: 10.1021/bm2000956
Takahashi, H., Oikawa, F., Takeda, N., and Shimizu, T. (2022a). Contraction control of aligned myofiber sheet tissue by parallel oriented induced pluripotent stem cell-derived neurons. Tissue Eng. Part A, 28, 661–671. doi: 10.1089/ten.tea.2021.0202
Takahashi, H., and Okano, T. (2015). Cell sheet-based tissue engineering for organizing anisotropic tissue constructs produced using microfabricated thermoresponsive substrates. Adv. Healthcare Mat. 4, 2388–2407. doi: 10.1002/adhm.201500194
Takahashi, H., and Okano, T. (2019). Thermally-triggered fabrication of cell sheets for tissue engineering and regenerative medicine. Adv. Drug Deliv. Rev. 138, 276–292. doi: 10.1016/j.addr.2019.01.004
Takahashi, H., Shimizu, T., Nakayama, M., Yamato, M., and Okano, T. (2013). The use of anisotropic cell sheets to control orientation during the self-organization of 3D muscle tissue. Biomaterials 34, 7372–7380. doi: 10.1016/j.biomaterials.2013.06.033
Takahashi, H., Shimizu, T., and Okano, T. (2018). Engineered human contractile myofiber sheets as a platform for studies of skeletal muscle physiology. Sci. Rep. 8, 13932. doi: 10.1038/s41598-018-32163-1
Takahashi, H., Yoshida, A., Gao, B., Yamanaka, K., and Shimizu, T. (2022b). Harvest of quality-controlled bovine myogenic cells and biomimetic bovine muscle tissue engineering for sustainable meat production. Biomaterials 287, 121649. doi: 10.1016/j.biomaterials.2022.121649
Tanaka, R., Sakaguchi, K., Yoshida, A., Takahashi, H., Haraguchi, Y., Shimizu, T., et al. (2022). Production of scaffold-free cell-based meat using cell sheet technology. NPJ Sci. Food 6, 41. doi: 10.1038/s41538-022-00155-1
Taylor, M. J., Weegman, B. P., Baicu, S. C., and Giwa, S. E. (2019). New approaches to cryopreservation of cells, tissues, and organs. Transfus. Med. Hemother. 46, 197–215. doi: 10.1159/000499453
Tuomisto, H. L., and Teixeira de Mattos, M. J. (2011). Environmental impacts of cultured meat production. Environ. Sci. Technol. 45, 6117–6123. doi: 10.1021/es200130u
Wennerholm, U.-B., Söderström-Anttila, V., Bergh, C., Aittomäki, K., Hazekamp, J., et al. (2009). Children born after cryopreservation of embryos or oocytes: a systematic review of outcome data. Human Reprod. 24, 2158–2172. doi: 10.1093/humrep/dep125
Whaley, D., Damyar, K., Witek, R. P., Mendoza, A., Alexander, M., Lakey, J. R., et al. (2021). Cryopreservation: an overview of principles and cell-specific considerations. Cell Transpl. 30, 0963689721999617. doi: 10.1177/0963689721999617
Keywords: cryopreservation, cellular agriculture, bovine myogenic cells, cultured meat, primary culture, muscle tissue engineering, myotube formation, muscle contraction
Citation: Kakehi R, Yoshida A, Takahashi H and Shimizu T (2023) Repeated and long-term cryopreservation of primary bovine myogenic cells to maintain quality in biomimetic cultured meat. Front. Sustain. Food Syst. 7:1023057. doi: 10.3389/fsufs.2023.1023057
Received: 19 August 2022; Accepted: 31 January 2023;
Published: 24 February 2023.
Edited by:
Arícia Possas, University of Cordoba, SpainReviewed by:
Krishna Pramanik, National Institute of Technology Rourkela, IndiaJulia Beretov, St George Hospital Cancer Care Centre, Australia
Copyright © 2023 Kakehi, Yoshida, Takahashi and Shimizu. This is an open-access article distributed under the terms of the Creative Commons Attribution License (CC BY). The use, distribution or reproduction in other forums is permitted, provided the original author(s) and the copyright owner(s) are credited and that the original publication in this journal is cited, in accordance with accepted academic practice. No use, distribution or reproduction is permitted which does not comply with these terms.
*Correspondence: Hironobu Takahashi, takahashi.hironobu@twmu.ac.jp