- 1Department of Agricultural Entomology, Indian Council of Agricultural Research (ICAR)-Central Institute of Post-Harvest Engineering and Technology, Ludhiana, India
- 2Food Grains and Oilseeds Processing Division, Indian Council of Agricultural Research (ICAR)-Central Institute of Post-Harvest Engineering and Technology, Ludhiana, India
- 3Indian Council of Agricultural Research (ICAR)-Central Institute for Research on Cotton Technology (CIRCOT), Mumbai, India
- 4Indian Council of Agricultural Research (ICAR)-Central Institute of Post-Harvest Engineering and Technology, Ludhiana, India
- 5Department of Agricultural Entomology, Indian Council of Agricultural Research (ICAR)-Vivekananda Parvatiya Krishi Anusandhan Sansthan, Almora, Uttarakhand, India
- 6Faculty of Agriculture, Sri Sri University, Cuttack, Odisha, India
- 7Division of Entomology, Indian Council of Agricultural Research (ICAR)-Indian Agricultural Research Institute, New Delhi, India
- 8Indian Council of Agricultural Research (ICAR)-Research Complex for Eastern Region, Research Center for Makhana, Darbhanga, Bihar, India
- 9Department of Entomology, Krishi Vigyan Kendra, Professor Jayashankar Telangana State Agricultural University, Hyderabad, India
- 10Department of Agricultural Entomology, Indian Council of Agricultural Research (ICAR)-Indian Institute of Oil Palm Research, Pedavegi, Andhra Pradesh, India
Modernization of the agricultural production system led to a significant increase in annual food production intended to meet the ever-growing consumer demand. In many countries, most of the food grains produced is stored for contingency and regular supply. These stored grains, in general, are directly or indirectly infested by insects, resulting in severe grain damages and storage losses, thus, causing a threat to food safety and security. Although a variety of insect management options, such as physical, mechanical, biological, and chemical methods, are available, fumigation has been practiced for decades in storage. However, opportunities for fumigation are narrowing after the phase-out of methyl bromide. Besides, safe food and health concerns paved the path for green chemistry and non-chemical management practices. This review includes the list of stored-grain insects and their detection methods. The different management strategies such as the modern storage structures (hermetic and low-pressure storages), modified or controlled storage atmosphere, application of ozone as fumigant, irradiation, and physical options are presented. Further, the details on sustainable biological options, such as semiochemicals, natural enemies, biopesticides, and entomopathogenic nematodes, are supplemented. The use of inert dusts as grain protectant and in combination with the biological entity is included. Studies on alternative fumigants', novel management options, such as molecular biology tools (RNAi and CRISPR) and nanotechnology in stored grain protection, are also highlighted. This review helps the reader to understand the overall factors affecting grain storage and the different options to manage the insects causing storage losses.
Highlights
This review provides a better understanding of the stored product insects, for example, their life cycle and relationship with the storage temperature, detection methods, storage structures, and insect management/control strategies. This information will be useful in developing protocols for the safe storage of food grains and devising appropriate integrated pest management procedures.
Introduction
Food safety and security is one of the major concerns in the present world. Preservation and safe storage of food grains are essential for their timely delivery to consumers. Different food commodities, such as harvested legumes and cereals, processed plant and animal food products, and semi-perishables need safe storage for their utilization at the domestic or commercial end. Most storage part primarily focuses on grain stored domestically or commercially (Said and Pradhan, 2014). At present, several structures ensure the safe storage of grains, ranging from small metal bins to tall grain elevators/silos. Usually, stored agricultural commodities are prone to contamination and damage by biotic and abiotic factors during the prolonged storage period. Among the biotic agents, insects, mites, rodents, birds, and microorganisms cause an enormous loss in storage. Several insect species substantially damage stored commodities, resulting in about 10–20% of total storage losses (Esther et al., 2014). More than 600 beetle species, 70 moth species, and 355 mite species are listed commonly in insects causing losses of stored products of agricultural and animal origin (Rajendran and Sriranjini, 2008). This enormous pest arena results in a considerable amount of quantitative and qualitative losses in the stored commodity. More often, insect pest causing storage product damage comes from the field and get established at the storage site due to favorable microclimatic conditions. Subsequently, they are retained during various processing and storage channels (Hagstrum and Phillips, 2017). Many insect pests initiate damage during the crop ripening stage and continue to storage operation (Sallam, 1999). However, in storage, the significant sources of infestations are old bags, storage structures, old containers, and cross-over infestations (Perez-Mendoza et al., 2004), harvesters, and other machinery (Sinclair and White, 1980). The initial infestation can be minimized by properly harvesting and drying of grains to a safe storage moisture content that will be provided by grain storage agencies. During storage operations, the movement and spread of stored product insects mainly occur via grain supply from one region to another through the commodity. Sometimes they are also spread by an active flight, as many insects are strong fliers (Ridley et al., 2011).
Storage loss assessment is complex because of several methodological constraints and skilled personnel requirements. Generally, they are conducted at a small experimental scale and extrapolate to bulk storage. Diagnosing insect infestation in a stored commodity is the preliminary step as the damaging symptoms vary between the insects/species. This step is vital for preventing the population buildup and further planning for better storage management strategies. Although several species of insects may be associated with the stored grains, only a few insects potentially cause damage and are well-renowned (Table 1).
Based on the damage severity in a specific region, they can be grouped as major and minor pests. Besides, their feeding preference can be classified as superficial or boring pests. The most basic classification of the storage insects is based on their feeding habit, i.e., “primary pests” and “secondary pests” (Srivastava and Subramanian, 2016). Primary is mainly infest and damage the whole undamaged grains. These can cause severe damage to the grains lot and are difficult to control if unnoticed until their population establishment. Hence, surveillance is needed to prevent their infestation and damage. On the other hand, secondary feeders/pests are generally called “bran bugs” because they establish on the grains that are already damaged either by the primary pests or other miscellaneous damages (Bell, 2014). They survive on broken kernels, debris, or higher moisture weed seeds. The damage caused by secondary feeders can be easily identified as their life stages are visible in the commodity area. Some of these secondary pests are also mold/fungal feeders. They contaminate the grains via their presence and metabolic wastes, resulting in moisture loaded conditions (excretion and condensed heat) and mold development (Magan et al., 2003). Thus, mold development indicates the final state of grain spoilage. Notable mold feeders include foreign grain beetle, rusty grain beetle, hairy fungus beetle, and psocids.
In many cases, multiple insect species are present in the commodity lot at the same time as their feeding niche is different and can sustain simultaneously. The presence of a particular insect also depends on the type of stored grain and region of the warehouse. For instance, a common pest of wheat storage in the northern part of India is khapra beetle, and in the southern region of India is flour beetle (Ramya et al., 2018). The diagnosis of insect infestation in farm-level storage can be generally done by monitoring the insect movement, clumpy grains, and congregated insects near walls or the bottom of storage structures (cold conditions). Precisely, assessment through plastic pitfall traps, screening, sieving, grain weight, color, odor, and nutritional quality of the grains are generally conducted. In addition, determination of carbon dioxide (CO2), headspace analyses of gases, chitin analysis from grain samples, and grain temperature are novel approaches to detect stored insects (Banga et al., 2018).
Insect detection in stored grains
Post-harvest grain management involves mainly scientific handling and safer storage. Several insect pests infest the stored food grains from the field-harvested commodity. Usually, each insect's type of feeding, biology, behavior, ecology, and damaging symptoms are different. Thus, early detection of insects or their damage is crucial for efficient management. The basic of all the detection methods is the visual inspection and encountering stages of insects. Various researchers have devised novel approaches to detect insects infesting stored commodities. For example, using acoustical detection methods, Hagstrum et al. (1996) precisely detected the presence of one infested kernel in 650 g of wheat grains. Using electrical conductance, Pearson et al. (2003) observed hidden internal insect infestations in wheat kernels to 88% for large-sized larvae and 87% for pupae. Brabec et al. (2017) used a modified laboratory roller mill with electrical conductivity and detected the presence of Sitophilus zeamais larvae inside popcorn kernels. Further, the detection accuracy ranged from 75 to 81% of the pupae, 80–91% of the medium larvae, and 43–47% of the tiny larvae based on the roller speed. A trap made of deep learning technique with a vision analysis program was developed by Shen et al. (2018) for detecting Cryptolestes pusillus, Oryzaephilus surinamensis, Tribolium confusum, Sitophilus oryzae, and Rhyzopertha dominica. Red, Green, and Blue (RGB) images of live insect database were established initially and used R-CNN to extract these images and classify the insects. In recent days, stored insect detection is also being done by semiochemical detection and quantification under laboratory conditions using gas chromatography (GC) with detectors, such as flame ionization (FID), electron capture (ECD), photoionization (PID), or mass spectrometry (MS). In most advanced conditions, sensors and biosensors-based detection methods also give better results (Brezolin et al., 2018). The categorization along with the important examples of different detection methods is presented in Figure 1.
Preventive measures
The effective reduction in the initial buildup of insects from field to storage may reduce the burden of insect management in later phases of post-harvest processing and storage. Usually, these measures are traditional or indigenous and are established chiefly based on the experience of our ancestors (Morales, 2002). Since insects are active and passive fliers, preventive management methods are needed to avoid the source of infestation. Several researchers have highlighted the effective preventive management strategies (Hagstrum and Flinn, 2014; Mohapatra et al., 2015; Hagstrum and Phillips, 2017); ample literature is available on how to take care of harvested produce agro-climatic region-wise, in the initial phase. Preventative measures manage the insect buildup in two ways: managing insects immigrating to stored commodity and the insects emerging within the commodities due to pre-infestation (Stejskal, 2015). Most importantly, the drying of food grains to a moisture range of 10–14% (wet basis) before storage is expedient (Shankar and Abrol, 2012). Other preventive practices such as storing new grains in a clean place away from the old lots, plugging all the unnecessary cracks and crevices of storage structures, proper stacking with sufficient interspace and light penetration between stacks, and proper maintenance of warehouses. The details of these practices and their advantages and disadvantages are described in other literature (Shankar and Abrol, 2012; Hagstrum and Phillips, 2017); hence, such information is not included in this article.
Storage structures
Storing food grains is a common practice by farmers and governments for its further utilization. Generally, the grains are held at dwelling places or in huge storage facilities. However, storing grains in different types of structures is widely adopted in various parts of the world (Manandhar et al., 2018). They are generated as tribal knowledge and are exploited with synthetic structures as days pass. The structures may be classified as (a) below-ground and above-ground structures or (b) traditional and improved structures (Hui et al., 2002). During ancient times, men dug pits and stored grains near their dwelling places. Nonetheless, most of the time, they faced damage either by rodents or microbial decay. Gradually, they used to store in bamboo baskets, mud bins above ground, wood elevators, and so on. In the past, comprehensive lists of different storage structures were published by many authors (Deka et al., 2006; Ashok et al., 2018), few of these are antiquated and are minimum visible. Some of the significant ones are given in Figure 2. With the advancement of human knowledge and skills, the shift toward developing and adopting metal bins, automated storage structures, massive warehouses, tall elevators, silos, and other improved structures are observed. In contrast, these structures led to the development of sophisticated storage conditions/microclimates. Eventually, insects also acclimatize with time and spread to various locations through these systems. In the present day, hermetic and low-pressure storage structures are quite popular.
Hermetic storage
The concept of creating a barrier between the commodity and grain has led to the knowledge of hermetic seals. As many conventional structures are internally oxygenated, the idea of de-oxygenation inside the storage structure is utilized in airtight storage to reduce aerobic conditions. This reduction in oxygen (O2) concentration elevates the carbon dioxide (CO2), which negatively affects the metabolic activity of the insects and eventually leads to death. Storing grains in hermetic seal storages is an emerging field of engineering research related to agriculture (Kumar et al., 2017). Storage pest management by an airtight seal or gas-tight seal was advantageous because it increases grain temperature, desiccation, and avoids immigration by enclosing the volatiles inside (Finkelman et al., 2002). Conversely, the gas-tight condition was also detrimental to stored grains. However, in modern management practices, controlled aeration, altering the gas proportion, or including an insect-proof barrier provides additional advantages and success with hermetic storage structures (Navarro et al., 2004). Several scientific studies on designing and developing various configurationally modified hermetic storages are available (Baributsa and Njoroge, 2020). Compared to bags, they are expensive when designed with metals. Still, they are promoted in the world for modern storage facilities. In Asian and African regions, improved underground storage structures for grains, pulses, and oilseeds were still used, as lower O2 concentration reports desired mortality of insects (Somavat et al., 2017). A few of the commonest forms of hermetic structures available are silos (metal, cemented, and plastic), metal drums, hermetic cocoons (from few kilos to tons), hermetic bags, and other polymer bags and containers. These hermetic structures are available in different capacities and sizes (Baributsa and Cristine, 2020).
Many researchers studied the comparative efficacy of developed hermetic bags. More recently, Yewle et al. (2022) compared the efficiency of a few hermetic bags such as PICS®, Grain Pro®, SaveGrain® and Ecotact bags® in comparison with conventional bags such as jute and PP woven. They reported the O2 reduction to 6.4% that results in killing of insects within 2 months of storage. In turn, the grain quality was intact till 6 months. Atta et al. (2020) studied the damage potential of T. castaneum in different storage bags storing wheat. Results showed 100% mortality of T. castaneum at 30 days in an airtight bag. Further, a reduction in insect growth rate (30%), damaged wheat grains (10.16%), and grain weight loss (0.5–1.0%) were also observed. Likewise, Sanon et al. (2011) studied the laboratory and on-farm trials of triple-bagging with heavy-grade polyethylene against C. maculatus. It was found that the insect population was reduced due to the unavailability of O2 (in artificially inoculated treatments), with grains stored for seven months devoid of infestation. Chigoverah and Mvumi (2016) tested the metal silos and hermetic bags under simulated smallholder farmer conditions against stored-maize insect pests and found them effective (100% insect mortality). Similarly, Baoua et al. (2014) tested PICS® bags for maize storage in West Africa; García-Lara et al. (2020) tested hermetic plastic bag (sBagTM), hermetic plastic silo (BioxiloTM) against stored maize insects in Central Mexico. Recently, many researchers have developed bags or structures fabricated with HDPE layers and proved efficient in insect control under smaller trials (De Groote et al., 2013). However, an efficient and economically feasible hermetic storage structure for field/farm level and/or bulk storage is still lacking.
Low-pressure storage
Low-pressure storage implies designing and developing a grain storage structure with reduced pressure inside. This is one of the advancements in the storage structure, which banks on altering the physical environment generally achieved by applying vacuum and creating low-O2 atmospheres. This lower O2 concentration affects physiological processes. In this method, the mortality of insects is caused by hypoxia and dehydration (Navarro et al., 2004). Although the potentiality of its implementation in uniform treatment is limited, the development of flexible and mobile storage structures made of polyvinyl chloride (PVC) liners is lighting up the development in this area. Navarro et al. (2001) demonstrated the relation between low pressures with temperature and moisture in managing T. granarium. They found that quiescent larvae were killed with 25 mm Hg pressure exposed to 172 h at 30°C. Increasing the temperature to 35°C reduced the exposure time to 145 h. Similarly, in field trials, total mortality of E. cautella and P. interpunctella was achieved at 21.3°C in 3 days. Similarly, Mbata and Phillips (2001) observed the relationship between temperature and pressure in modified environmental conditions, proving fatal to the different developmental stages of T. castaneum, P. interpunctella, and R. dominica more effectively than low pressure alone. Further, mortality increased in all life stages with increasing exposure time to low pressure and increasing temperature. In another similar study, Mbata et al. (2005) exposed C. maculatus to different low pressures and temperatures. They reported that the adults were most susceptible, with a mortality of 99% within 0.8 h at 32.5 mm Hg and 30°C. Similarly, Finkelman et al. (2003) used a vacuum approach against T. castaneum, E. cautella, and O. surinamensis and found significant results related to insect mortality. More recently, Kumar et al. (2020) developed a sensor-assisted vacuum hermetic storage for monitoring insect activity and humidity and temperature fluctuations. Although utilizing low pressure alone was a failure in many cases; integration of temperature, humidity, and vacuum (pressure) approach in storage structure development of different capacities is the need of the hour.
Physical methods of protection
Modified storage atmosphere
Insects require O2 for their developmental metabolism. The insects generally intake O2 and exhale CO2. Atmospheric air primarily consists of O2 (21%), CO2 (0.03%), and N2 (78%) (Smit, 2019). The alteration of the required concentration of these gases (in combination with other fumigants-like phosphine) is lethal to stored-grain pests (Constantin et al., 2020). This technique when used to manage the insects in storage is referred to as modified or controlled atmospheric storage. Depletion of O2 (<3%) and elevation of CO2 (>50%) exposure to >24 h significantly distress the insects, which even achieve mortality of residual populations when exposed for prolonged periods (Tutuncu and Emekci, 2019). This phenomenon is efficiently used in developing the storage structures, which are termed as hermetic technology and explained under the different heads. The concept of modified atmosphere storage is based on the modification of storage gas composition, achieved artificially with retention of airtight conditions for a longer time (Wong-Corral et al., 2013). Various gas generators, gas exchange apparatus, and catalytic converters were attached to storage for its application. Several researchers extensively studied the effect of elevated CO2: depleted O2 on stored grain pests (Navarro, 2012). Concerning modified atmosphere storage, the significance of lowering oxygen and anoxia (Conyers and Bell, 2003), higher CO2 and hypercarbia (Riudavets et al., 2009; Wong-Corral et al., 2013), and lower O2 and higher CO2 combinations (Cheng et al., 2012) and high CO2 pressure (Riudavets et al., 2010) on insect management have been documented.
De Carli et al. (2010) studied the effect of modified atmosphere packaging on the mortality of Sitophilus spp. in organic maize grain storage. Modified atmosphere packaging storage compromised 20% O2, or 20, 40, 60, and 80% CO2 (remaining N2) for 30 days at 26°C. The result indicated total insect mortality with 40% CO2 treatment after 5 days. Likewise, modified atmospheres with 50%, 70%, and 90% CO2 were tested at 28°C against bruchids and found total mortality by 9 days (Wong-Corral et al., 2013). Similarly, Iturralde-García et al. (2020) proved that air mixture (with 50% and 70% CO2) is lethal to larval and pupal stages of R. dominica in packaged chickpeas with LT50 ranging from 7 h for larvae to 2 days for pupae. Dose standardization, treatment protocols, and source of CO2 supply are a matter of concern for large-scale treatments. However, based on its effectiveness, CO2 may be integrated with other fumigants in different proportions. Exhaustive studies on field-level research on modified structures with standard doses (a combination of gases) may be prioritized as the alternative to chemical fumigation.
Ozone
Ozone (O3) is a potent oxidizing agent and highly reactive. Generally, O3 is used in water purification, food sanitization, and product deodorizing (White et al., 2010). It can effectively penetrate the grain mass and decompose rapidly to O2 without any residues; these properties create an effective fumigant alternative for stored-grain protection (Pimentel et al., 2009). Artificially, O3 generation is done in gaseous form using air and electricity. In the 1980s, it was initially used in storage to kill insects and other microflora (Jian et al., 2013). The effectiveness of O3 in killing a varied range of stored-grain pests, including internal and external feeders, was established (Isikber and Athanassiou, 2015; Subramanyam et al., 2017). Research on ozone fumigation concentrated on its effect on treating phosphine-resistant populations, empty bin treatment, and disinfestation of grains (Hardin et al., 2010). Sousa et al. (2008) used ozone to manage phosphine-resistant populations of T. castaneum, R. dominica, and O. surinamensis. Ozone was passed @ 150 ppm in a continuous flow of 2 L min−1 and recorded total insect mortality. Hansen et al. (2013) exposed S. granarius and P. interpunctella continuously to ozone @ 33 ppm for 6 d and 131 ppm for 8 d and recorded total mortality of the insects. Amoah and Mahroof (2018) studied the effect of ozone on L. serricorne. Insects were exposed to doses of 100–400 ppm at 50 ppm increments for 1 h and observed the inability of eggs, larvae, and pupae to transform into adults after exposure. Moreover, exposure to higher concentrations killed 90% population within 2 days. A major constraint in the application of ozone as a fumigant is achieving its dosage and the continuous purging through the treatment time. Although O3 proved an effective fumigant, commodity selection for which O3 exposure must be taken care of, as it is a strong oxidant. Studies related to designing and developing compatible storage structures for O3 treatment with closed-loop components, pilot-scale studies, and large-scale applications still need to be established.
Heat or cold temperature (included dielectric heating)
Normal growth and development of each insect require a range of favorable atmospheric conditions specifically temperature, relative humidity, and gas compositions (Figure 3). Deviations from the optimum level negatively affect insect metabolism. In general, a small change in temperature prominently affects the growth and development of insects. Extreme temperatures were lethal to insects that were reported for managing stored-product insects (Bell, 2014). Temperature can be reduced by different aeration strategies (freezing) and grain temperature can be increased by artificial heating techniques, such as dielectric heating, passage of hot air, and others. Either increase or decrease in temperature reaped greater success with insect management. Grain disinfestation using thermal treatments was conventionally practiced by using hot/cool–air/water combinations, in a controlled condition (Macana et al., 2018). Advances in this field include dielectric heating of grains using radio frequency (RF) and microwave (MW) heating (Das et al., 2013). The commonly used frequency for MW heating was 2,450 MHz or 915 MHz, whereas for RF it was 13, 27, or 40 MHz (Wang et al., 2001). These radiations are not only lethal to insects but also cause sterility in the survived populations (Paul et al., 2020). In adults of stored grain insects viz. S. oryzae and T. confusum, the first reports were mentioned on dielectric effects, which were studied by using the 10- and 40-MHz RF dielectric heating (Nelson and Whitney, 1960). Purohit et al. (2013) exposed the Mung bean (12% m.c.) infested with C. maculatus to 200, 300, or 400 W microwave power levels for 14, 28, and 42 s and reported that exposing for 28 s with 400 W power level achieved complete mortality of eggs and reached the grain temperature of 68.1°C. Similarly, Singh et al. (2012) exposed C. chinensis to microwave radiation (2,450 MHz) for 100 s at 700 W and found complete mortality of insects. Thielens et al. (2018) reported the dielectric absorption of radio frequencies by insects after exposure to a range of 2–120 GHz using Micro-CT (computer tomography) imaging. Many recent studies have shown the potentiality of these non-thermal heating techniques for the effective stored-grain pest management, with better grain quality and environmental safety (Paul et al., 2020). Microwave heating is reported to affect the cell structure, cell membrane permeability, and disrupt cell division (Lu et al., 2011). In the adults of T. castaneum, it was also observed that microwave exposure causes cellular changes, such as aggregation of fat body cells, larger cavity appearances in the nucleus, the mitochondria, and golgi apparatus disappearance. These thermal and non-thermal effects led to the death of target insects when exposed to microwave radiation. However, application and exposure safety to humans and other organisms are still need to be established.
Irradiation (ionizing radiations)
Ionizing radiations viz., gamma-rays (emitted from cobalt-60 and cesium-137), x-rays, or electric beams were also used in grain disinfestation (Hallman, 2013). These radiations act at the molecular level can be able to damage the structure of DNA. DNA damage leads to sterilization, mortality, mutations, and abnormalities in insects. The radiations are effectively penetrable, easy operation of irradiators, but require higher initial cost as well as higher dosage that pose risk to human health (Hasan and Khan, 1998). As per reports, irradiation is permitted in at least 33 countries against some stored products; however, 14 countries permit it for all stored products (Hallman, 2013). Chiluwal et al. (2019) exposed C. chinensis adults to gamma radiation (0, 200, 300, 400, 500, and 600 Gy) and found that female fecundity, hatchability, and adult fertility reduced after the dose of 300 Gy. Sileem et al. (2019) studied the effect of gamma-irradiation in combination with food-grade diatomaceous earth (DE) against S. granarius, T. castaneum, and R. dominica. Among their treatments, the combination of 100 Gy gamma-radiation exposure with DE @ 1 g/kg was found significant with complete mortality. Hassan et al. (2019) tested Fast Neutron Irradiation (FNI) @ 0, 64, 128, 192, and 256 Gy against P. interpunctella. The dose 256 Gy proved effective in preventing the development of all immature stages and proved the standard lethal dose. In-depth knowledge of irradiation by the application of gamma rays and x-rays, integration with other compatible methods, and safer handling of these radiations is needed to be studied for field-scale and may be used as an effective alternative against presently practicing methods.
Biological methods
Semiochemicals
Semiochemicals are chemical signals produced by one insect/organism, which evokes behavioral or physiological responses in the receiving insect/organism. They are commonly classified into “pheromones”: intra-specific chemical signals and “allelochemicals”- inter-specific chemical signals (Abd El-Ghany, 2019). In stored-product protection, pheromones are mainly exploited. These are helpful for insects in search of food, mate, egg-laying site, defense, and others (Cox and Collins, 2002). For almost 40 species of stored-product insects, semiochemicals were identified (Phillips and Throne, 2010). They are used in pest management mainly as attracticides, repellents, feeding and oviposition deterrents, mass trapping lures, and mating disruption formulations. Sex (unisex) and aggregation (both sex) pheromones are two main types. Sex pheromones were illustrated in stored-grain insects, such as Pyralid (Phycitinae), Anobiidae, Bruchidae, and Dermestidae. Similarly, aggregation pheromones in Bostrichidae, Curculionidae, Cucujidae, Silvanidae, and Tenebrionidae (Phillips and Throne, 2010). Different types of traps were designed and evaluated for their efficiency in dispensing synthetic lures to attract stored-grain insects (Sambaraju and Phillips, 2008). Though the pheromone components are species-specific some cross-attraction has also been reported (Cox, 2004).
Pheromones are generally used in three ways of pest management viz., monitoring, mass trapping, and mating disruption, like in-field pest management (Trematerra, 2012). Moreover, successful pheromonal studies under laboratory conditions were done in stored-grain moths (Ephestia spp., P. interpunctella, S. cerealella) and stored-grain beetles (L. serricorne, T. granarium, Tribolium spp., S. paniceum). Besides, detailed semiochemistry of bruchids was exhaustively compiled by Rodríguez (2018), hence, they are not discussed in this study. Garcia et al. (2003) sprayed sesquiterpenes (costic-aldehyde, Tessaric acid, eremophilane) isolated from Tessaria absinthioides on larvae of Tenebrio molitor and found that topical application of these sesquiterpenes increased pupal duration and induced morphological deformities. Sambaraju and Phillips (2008) studied the response of P. interpunctella adults to trap with sex lure (Z,E)-9,12-tetradecadienyl acetate (TDA/ZETA) in combination with UV, green, and white light. They found that adult moths were attracted to pheromone traps significantly (71%), with an orientation toward low illuminated surfaces. Losey et al. (2019) tested a range of synthetically available semiochemicals for orientation studies of C. ferrugineus in field and laboratory and reported that the aggregation pheromones namely Cucujolide I and Cucujolide II, deployed when singly or in combination, proved an increased adult attraction. As semiochemicals alter the behavior and biology of both male and female insects, utilization of these chemical constituents in integrated stored-grain pest management is essential. However, it depends on the simultaneous advancements in chemistry, biochemistry, physiology, and genetics. Based on the literature reviewed, it was opined that the use of semiochemicals is the best and sustainable method for stored-grain pest management. However, lack of timely and regional availability along with lack of protocols for application are the constraints.
Natural enemies
Natural enemies (predators and parasitoids) to manage stored pests were studied for a century. However, most of them were focused on population and evolutionary ecology, genetics, toxicology, biology of natural enemies, and others. According to Flinn and Scholler (2012) during the 1942–45 release of Habrobracon hebetor in cacao warehouses of Bahia, Brazil, infested with Cadra cautella was the first recorded mass release of a parasitoid. The use of insect parasitoids and predators against stored-product insect pests was reviewed by several authors time to time (Matthias, 2010; Flinn and Scholler, 2012). After advancement in the integrated pest management approach, biological control becomes one of the most sustained and important components. Problems of insecticide residues in storage and stored-grains, approach toward organic farming, food safety, and others were also boosting up the concentration toward biocontrol (Flinn and Scholler, 2012). The stored-grain ecosystem is incomparable with the field-ecosystem and the storage factors significantly affect the activity of natural enemies (Hagstrum and Subramanyam, 2009). The knowledge of behavior and biology of entomophagous insects attacking stored-grain pests is the prerequisite for biocontrol. Simultaneously, their adaptation in varied conditions in association with the hosts is one of the promising areas of research. Out of the studied 137 references related to biological control in stored products or food industry by Cassi (2017), 51, 21, and 18% were parasitoids, entomopathogens, and predators, respectively. Mites (56%) and heteropterans (24%) were the major predators. The principal composition of parasitoids was pteromalids and braconids (70%) followed by bethylids, ichneumonids, and trichogrammatids (24%) along with chalcidids and eulopids (3%). An exhaustive list of reported and effective natural enemies against stored-product insects is available elsewhere (van Lenteren et al., 2018). Although biological control is one of the effective strategies, appropriately addressing food safety is a concern; the presence of insects (biocontrol agents) also faced sociological issues in the past (Flinn and Scholler, 2012; Belda and Riudavets, 2013; van Lenteren et al., 2018). Cost-effective and field-level inundative applications of predators and parasitoids in the past were very few. Many of the studies are laboratory studies. Integrating biological control strategies with other pest management practices on commercial scale is advancing and getting success slowly.
Microbial-based pesticides
Stored grain protection using biological control is a potential means/technique without harming environmental health (Matthias, 2010). In this study, biopesticides depict mainly pathogenic biological agents, for example, fungus, bacteria, virus, protozoa, and others. Arrays of insect pathogens were tested in laboratory conditions against stored-grain insects (Batta and Kavallieratos, 2018; Kumar et al., 2019). Besides, none of them were seen as commonly used because of their host specificity and narrow climatic adaptations. Synergists were tried in combination, to enhance their efficacy and wide range adaptability especially diatomaceous earth. Some of the commercially available entomopathogenic fungi are Beauveria bassiana, Metarhizium anisopliae, and bacterium—Bacillus thuringiensis (Bt), which were tested majorly against stored-grain pests especially beetles (Batta, 2016). For the stored-grain moths, Bt was tried long back, but the commercial application has limitations because of the higher dose, separation of the carrier after treatment, huge facilities for mass culturing, region-specific strains, and others (Arthurs and Dara, 2019). Bacillus thurengiensis var. kurstaki (Btk) was reported effective on stored grain pests (Shapiro-Ilan et al., 2007) especially P. interpunctella and other moths (Lord et al., 2007). Malaikozhundan and Vinodhini (2018) reported that the Bt (4 × 108 cells/mL) was highly effective against C. maculatus (100% mortality) under laboratory conditions. Although entomopathogens recorded the population reduction as compared to conventional insecticides it is slower and lower in efficacy. The use of entomopathogens mainly fungus was considered as promising in storage conditions. Rumbos and Athanassiou (2017) highlighted the entomopathogenic fungi used in stored pest management. Other pathogens, for example, protozoans and viruses were also tested against stored-grain insect pests. Protozoans, that is, Nosema whitei (Milner, 1973) and Nosema plodiae (Kellen and Lindegren, 1974) were reported long back to infest flour beetles and phycitine moths, respectively. Similarly, viruses (mainly baculoviruses) were also studied majorly in lepidopetan pests, such as P. interpunctella and E. cautella (Vail et al., 1991). Additional research in this field regarding isolation of local strains, screening, and formulation of effective strains, a combination of multiple strains with different host ranges are required for the successful adoption of promising biopesticides.
Entomopathogenic nematodes (EPNs)
As the science of nematodes getting advanced, the use of entomopathogenic nematodes to control insects becomes a novel approach in pest management (Arthurs et al., 2004). Two important genera, namely, Steinernema and Heterhorhabditis proved effective against field crop pests and were also studied and found effective against stored-grain insects (Rumbos and Athanassiou, 2017). Athanassiou et al. (2008) tested the effect of Steinernema feltiae (three strains namely, UK 76, USA/SC, and Hawaii) against T. confusum, and E. kuehniella in wheat under laboratory condition. They observed 100% larval mortality (after 14 d of exposure) with Hawaii strain against T. castaneum, whereas USA/SC strain was found effective against E. kuehniella larvae (69% mortality after 14 d exposure). Laznik et al. (2010) isolated and tested the efficacy of three strains (B30, B49, and 3162) of S. feltiae against S. oryzae adults in a laboratory bioassay. All three strains were equally effective with mortality of 42–72% at 25°C with the lowest LC50 value of 1,165 IJs/adult (after 8-day exposure). Athanassiou et al. (2010) tested the efficacy of three nematode species (H. bacteriophora, S. carpocapsae, and S. feltiae) against four stored-wheat insects (E. kuehniella, R. dominica, S. oryzae, T. confusum) under laboratory conditions. Among the different combinations, only S. feltiae was found effective against E. kuehniella larvae, whereas other strains against other insects did not exceed mortality of 23% with more than 10,000 IJs per ml. De Carvalho Barbosa Negrisoli et al. (2013) tested eight strains of EPNs (H. bacteriophora-5; S. carpocapsae-1; S. rarum-1; S. riobrave-1) against five stored product insect pests (Anagasta kuehniella, Acanthoscelides obtectus, S. oryzae, S. zeamais, and T. molitor). The micro-tube bioassay results showed that all insect species were susceptible to the inoculated strains. Larval stages were more sensitive compared to adults. For a long time, advancement in this field was limited because of their narrowed climatic adaptations and inability to survive in dry conditions. Conversely, isolation of local virulent strains, efficacy evaluation against laboratory hosts, and formulation technologies uplifted the number of success (Rumbos and Athanassiou, 2017). However, a single strain/species of nematode cannot control a wide range of insects as they act species-specific. While formulating a mixture of strains can provide expected stored-grain protection. As all these studies are laboratory-conducted bioassays, field evaluation on bulk scale of such EPNs is lacking. Mass culturing and large-scale field trials may be conducted to boost EPN technology in storage management.
Plant derivatives
Stored product protection with the use of plant derivatives is an age-old practice. Botanicals reported managing the insects in several ways, such as repellents, antifeedants, toxicants, chemosterilants, and growth regulators because of the active ingredients present in them. As the green chemistry of insecticides advanced, the volatiles (essential oils) extracted from plant origin are of main focus (Rajendran and Sriranjini, 2008). The use of botanicals is getting priority globally as they are cost-effective and safe for human kind. The mode of action of plant oils involved mainly neurotoxicity, such as enzyme (Acetyl-choline esterase) inhibition and neuromodulation. Plant families such as meliaceae, myrtaceae, apiaceae, lamiaceae, lauraceae, poaceae, pinaceae, and others are reported to contain insecticidal secondary metabolites/volatiles, such as terpenoides, alkaloids, phenolics, so on and so forth (Talukder, 2006). In the past, studies were conducted on the efficacy of plant products on stored grain pests and many reviews are available regarding essential oils (Mossa, 2016; Singh and Kaur, 2018). Adult insects were generally found susceptible compared to eggs. Rajendran and Sriranjini (2008) exhaustively reviewed the fumigant action of more than 75 plant products tested for their efficacy against stored pests. It was found that tests for fumigant toxicity of plant essential oils mainly focused on T. castaneum, R. dominica, S. oryzae, and S. zeamais. Moth pests such as C. cephalonica and S. cerealella were studied meagerly (Mangang et al., 2020). Although the efficacy of many plants' extracted oils is tested against storage pests, very few of them succeeded in the formulation and registration process for their commercial application. Accordingly, only 1% of the insecticide market constitutes botanicals worldwide (Campolo et al., 2018). As per the health concerns of humans, these plant products may work as alternatives for chemical fumigation. In recent days, studies related to toxicity, screening, efficacy, sorption, formulation, and fumigation studies of botanicals especially essential oils are getting attention.
Chemical management
Majority of the research studies on storage grain protection in the 20th century were based on chemical applications, particularly surface/preventive sprays and fumigation. Fumigation is the way to control the infestations detected, but sprays are regularly taken to avoid the infestation. However, the higher dependency on chemicals has created health and environmental health issues. Nevertheless, the dependency on chemical management was not fully reduced; somehow the other management practices were taken into higher consideration day by day. For long years, dichlorvos was the commonest insecticide used for grain disinfestation, because of its fumigant action. Although resistance to dichlorvos was reported in R. dominica (Zettler and Cuperus, 1990), the chemical served the purpose for long, proved efficient as a surface spray. This effect is due to its chronic toxicity, later this chemical was phased out in many countries. There are several ready references dealt with the chemical protectants used in grains and storage structures (Hertlein et al., 2011). In the present day, insecticides with a novel mode of action, especially the spinosyn group, were extensively studied (Vassilakos et al., 2015). In many post-harvest management steps/conditions such as mills, processing plants application of insecticide played a prominent role in insect management. However, in storage, the chemical application mainly dealt with fumigation.
Fumigation means treatment using the gaseous form. It was a very common method during the 1990s for pest management in bulk storage (Mohapatra et al., 2015). Fumigant is a chemical with higher vapor pressure, toxic to insects, and able to penetrate through the commodity. Unlike chemical sprays, the fumigant requires an airtight condition to achieve full efficacy (Daglish et al., 2018). Relative humidity and temperature in storage, moisture content of seeds, and air tightness were important factors influencing the efficiency of fumigation (Zettler and Arthur, 2000). Out of 16 listed fumigants during the 1980s only a few, especially phosphine and methyl bromide were of use in present-day context. However, after phase-out of methyl bromide due to its ozone-depleting reports (Fields and White, 2002), it is only used for pre-shipment quarantine treatments in some developing countries. The loss of methyl bromide noticeably increased the dependence on phosphine for stored-grain pest management (Rajendran, 2001). Further, resistance to phosphine and reports of repetitive control failures were upraised for the search of alternatives to phosphine and validation of non-chemical practices. Since several reports on the efficacy of phosphine and other fumigants against stored-grain insects were available, they are not discussed in this article. Some of the recent studies are highlighted in Table 2. Recent advances in fumigation included the use of alternative fumigants and formulations. In the future, one should focus on non-chemical approaches for safer and sustainable stored-grain protection.
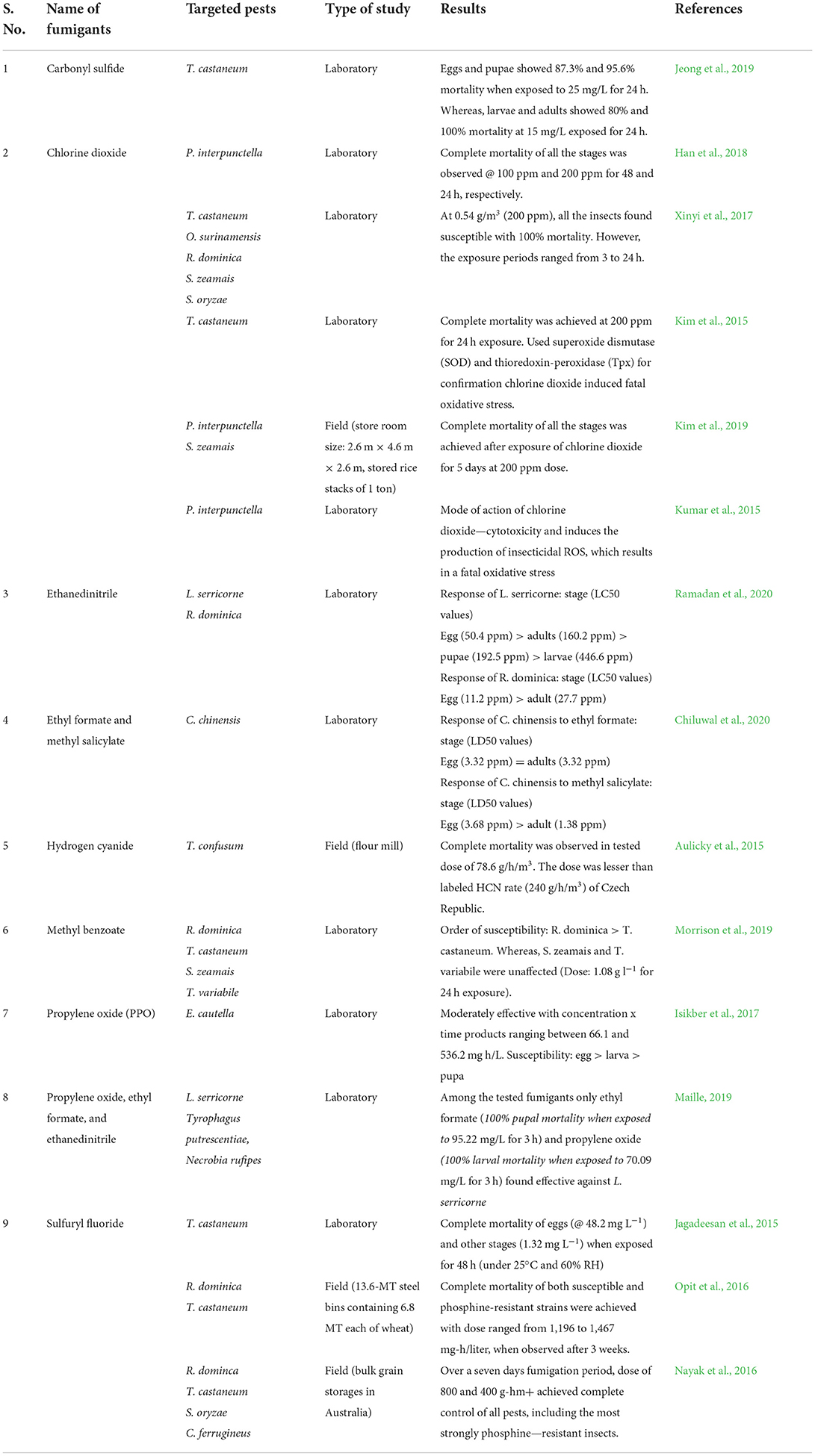
Table 2. Recent studies on efficacy of fumigants (excluding phosphine, methyl bromide and essential oils) against stored-product insects (2015 onwards).
Inert dust
During 1980s, it was reported that inert dust was effective in controlling insect pests. The inert dust is generally classified mainly into four groups (Banks and Fields, 1994) viz., (a) clays, sands, and ashes, (b) minerals, (c) dust that contains synthetic silica (Silicon dioxide—SiO2), and (d) dusts that contain natural silica (Diatomaceous Earth-DE). All these groups were reported effective against stored-grain insect pests. Insect mortality due to inert dust have various mode of action such as blocking of spiracles and digestive tract, absorbs cuticular moisture and epicuticular lipids, abrasion of the cuticle, and others. Though there were more entries, only DE and silica achieved significant results and are studied exclusively by many researchers (Shah and Khan, 2014). Using DE as a sole or mixture with other low-risk control methods was proved a promising alternative to insecticide sprays in storage (Arthurs et al., 2004). Cao et al. (2010) studied the efficacy of five different inert dust (Puliangtai, G-1, G-2, G-3, and G-4) against phosphine-resistant C. ferrugineus. In surface bioassay @ 0.08 g/m2 and 0.1 g/m2 C. ferrugineus recorded susceptible. Johnson et al. (2014) reported that wet application of DE particles increased dust efficacy resulted in 56.0% of insect mortality. Zhang et al. (2014) tested food-grade inert dust against five major insect species of stored grains: R. dominica, S. zeamais, O. surinamensis, T. castaneum, and C. ferrugineus and found that food-grade inert powder uniformly dispersed, and mortality reached 100% on the third day. Advances in the development of newer formulations, integration with other practices, and enhanced applications with lower doses for storage-pest management are increasing. In the future, the contact insecticides used in stored-grain protection may be replaced by food-grade inert dust.
Nanotechnology in stored-grain protection
Nanotechnology experiments are rising in basic and applied scientific fields, including stored-grain protection. The significance of the nanoparticles is hiked because of novel properties, such as changes in electrical conductivity, surface chemistry, and reactivity (Zayed, 2018). Several metallic oxides, namely, zinc, silver, aluminum, silica, and others, were generally formulated as nanoparticles (Stadler et al., 2010). However, zinc oxide nanoparticles were widely used because of their antibacterial, antifungal, UV filtration, higher catalytic, and photochemical activity (Meruvu et al., 2011). Malaikozhundan et al. (2017) synthesized and formulated the Bt-coated zinc oxide nanoparticles (Bt-ZnO NPs) against C. maculatus and reported the reduction of fecundity and hatchability (LC50 - 10.71 μg/mL) with prolonged larval, pupal, and total development period (@25 μg/mL). Debnath et al. (2011) tested entomotoxicity of surface-functionalized silica nanoparticle (SNP) against S. oryzae and found highly effective causing more than 90% mortality. Rahman et al. (2020) tested nickel-oxide nanoparticles (NiO NPs) against C. maculatus infested on the black gram and found decreased fecundity and an increased developmental period over the doses @ 5, 10, 20, and 40 ppm NiO NPs, respectively. Efficacy studies of nanoparticles were also conducted against T. castaneum, S. oryzae, R. dominica, and C. maculatus for their significant effect on adult insect mortality, fecundity, hatchability, and biology under laboratory conditions (El-Saadony et al., 2020). Detailed and field-level studies of nanoparticle applications are required to use this novel approach in stored product protection.
Molecular interventions
The technique of disrupting the normal gene expression of an organism is a novel approach in managing the insects. It involved two molecular tools viz., RNA interference (RNAi) and Clustered Regularly Interspaced Short Palindromic Repeats (CRISPR) (Perkin et al., 2016). The rust-red flour, T. castaneum, was considered as a model insect for these studies, and most of the data available in the published articles was mainly for this insect (Gilles et al., 2015). T. castaneum was preferred host because of easier multiplication in laboratory conditions and its strong RNAi response in all the developmental stages. Typically, RNAi acts at dsRNA and CRISPR deactivates Cas9 (DNA binder) to inhibit further gene expression (Qi et al., 2014). These techniques may be used effectively in stored-grain pest management only after extensive research. Identifying specific genes in insects infesting stored grains is a preliminary step and a concerned area of interest. These novel technologies seem futuristic with other similar techniques, for example, gene editing and gene modification.
Conclusion
Safe handling and protection of stored products are one of the primary areas of research for Post-Harvest Engineers and Entomologists. Presently, food security and safety is the concern of developing and developed countries. Rationalization in preventive management practices, such as proper drying and safer storage, led to the development of several dryers and controlled storage structures that were adopted globally. Among them, hermetic and low-pressure storages are gaining great success; however, the bulk storage applications are limited. Temperature management (heat or cold treatment) inside storage is beneficial as it achieved insect mortality. Heat treatment can also be done using exposure to electromagnetic radiations, such as RF and MW. In addition, the application of CO2 and ozone as fumigants may emerge as alternatives to conventional fumigants. Biological options such as predators, parasitoids, pathogens and EPNs are environmentally safer; however, the formulation, mass production, and field-level adoption are limiting factors for their wide adoption. Chemical ecology and semio-chemistry of stored product insects require in-depth research that erects the lure/trap options to attract and kill. Chemical management of insect pests is preferred as the last resort in field-crop pest management, but in storage, it is used as the first line of protection. Insecticides are mainly utilized for surface sprays and disinfestation. Limited fumigant options are available, wherein the insects have already developed resistance; thus, there is a need for alternatives. Novel scientific areas such as nanotechnology and biotechnology are advancing rapidly and contributing to stored-product protection. Nevertheless, such increasing concern for safe storage and non-chemical pest management has led to advances in several fields. Looking into the dynamic post-harvest systems, modernized and integrated pest management strategies for bulk storage has to be regularly revised. Since grain harvest, drying, handling, packaging, and transport till safe storage, pest management interventions can prevent and reduce grain loss to a significant level.
Author contributions
PG reviewed the information regarding insect detection in stored-grain pests and different techniques of storage pest management. DM wrote about storage structures. AD reviewed physical methods of protection. BG revised the manuscript. AP reviewed biological methods of pest management. IS reviewed about chemical method of pest management. MN reviewed the possibility of use of nano-technology in storage pest management. VP reviewed about molecular interventions for storage pest management. MR compiled and drafted the final manuscript. AS did final correction of the manuscript and language editing. All authors contributed to the article and approved the submitted version.
Funding
The study has been funded by Indian Council of Agriculture Research, New Delhi.
Acknowledgments
The authors appreciate the Director, ICAR-Central Institute of Post-Harvest Engineering and Technology, for providing time and liberty in conducting this review.
Conflict of interest
The authors declare that the research was conducted in the absence of any commercial or financial relationships that could be construed as a potential conflict of interest.
Publisher's note
All claims expressed in this article are solely those of the authors and do not necessarily represent those of their affiliated organizations, or those of the publisher, the editors and the reviewers. Any product that may be evaluated in this article, or claim that may be made by its manufacturer, is not guaranteed or endorsed by the publisher.
References
Abd El-Ghany, N. M. (2019). Semiochemicals for controlling insect pests. J. Plant Protect. Res. 59, 1–11. doi: 10.24425/jppr.2019.126036
Amoah, B. A., and Mahroof, R. M. (2018). Susceptibility of the life stages of cigarette beetle, Lasioderma serricorne (F.) (Coleoptera: Anobiidae) to ozone. J. Stored Prod. Res. 78, 11–17. doi: 10.1016/j.jspr.2018.05.009
Arthurs, S., and Dara, S. K. (2019). Microbial biopesticides for invertebrate pests and their markets in the United States. J. Invertebr. Pathol. 165, 13–21. doi: 10.1016/j.jip.2018.01.008
Arthurs, S., Heinz, K. M., and Prasifka, J. R. (2004). An analysis of using entomopathogenic nematodes against above-ground pests. Bull. Entomol. Res. 94, 297–306. doi: 10.1079/BER2003309
Ashok, Gowda, B., and Shakunthala, N. M. (2018). Different types of grain storage structures for the betterment of livelihood of indian farmers. Int. J. Pure Appl. Biosci. 6, 190–198. doi: 10.18782/2320-7051.6721
Athanassiou, C. G., Kavallieratos, N. G., Menti, H., and Karanastasi, E. (2010). Mortality of four stored product pests in stored wheat when exposed to doses of three entomopathogenic nematodes. J. Econ. Entomol. 103, 977–984. doi: 10.1603/EC09202
Athanassiou, C. G., Palyvos, N. E., and Kakouli-Duarte, T. (2008). Insecticidal effect of Steinernema feltiae (Filipjev) (Nematoda: Steinernematidae) against Tribolium confusum du Val (Coleoptera: Tenebrionidae) and Ephestia kuehniella (Zeller) (Lepidoptera: Pyralidae) in stored wheat. J. Stored Prod. Res. 44, 52–57. doi: 10.1016/j.jspr.2007.04.002
Atta, B., Rizwan, M., Sabir, A. M., Gogi, M. D., and Ali, K. (2020). Damage potential of Tribolium castaneum (Herbst) (Coleoptera: Tenebrionidae) on wheat grains stored in hermetic and non-hermetic storage bags. Int. J. Trop. Insect Sci. 40, 27–37. doi: 10.1007/s42690-019-00047-0
Aulicky, R., Stejskal, V., Dlouhy, M., and Liskova, J. (2015). Validation of hydrogen cyanide fumigation in flourmills to control the confused flour beetle. Czech J. Food Sci. 33, 174–179. Available online at: https://www.agriculturejournals.cz/publicFiles/303_2014-CJFS.pdf
Banga, K. S., Kotwaliwale, N., Mohapatra, D., and Giri, S. K. (2018). Techniques for insect detection in stored food grains: an overview. Food Control 94, 167–176. doi: 10.1016/j.foodcont.2018.07.008
Banks, J., and Fields, P. (1994). “Physical methods for insect control in stored-grain ecosystems,” in Stored-Grain Ecosystems, eds D. S. Jayas, N. D. G. White, and W. E. Muir (New York, NY: Marcel Dekker, Inc.), 353–410.
Baoua, I. B., Amadou, L., Ousmane, B., Baributsa, D., and Murdock, L. L. (2014). PICS bags for post-harvest storage of maize grain in West Africa. J. Stored Prod. Res. 58, 20–28. doi: 10.1016/j.jspr.2014.03.001
Baributsa, D., and Cristine, M. (2020). “Developments in the use of hermetic bags for grain storage,” in Advances in Postharvest Management of Cereals and Grains (Phillipines: Burleigh Dodds Science Publishing), 171–198. doi: 10.19103/AS.2020.0072.06
Baributsa, D., and Njoroge, A. W. (2020). The use and profitability of hermetic technologies for grain storage among smallholder farmers in eastern Kenya. J. Stored Prod. Res. 87, 101618. doi: 10.1016/j.jspr.2020.101618
Batta, Y. A. (2016). Recent advances in formulation and application of entomopathogenic fungi for biocontrol of stored-grain insects. Biocontrol Sci. Technol. 26, 1171–1183. doi: 10.1080/09583157.2016.1201458
Batta, Y. A., and Kavallieratos, N. G. (2018). The use of entomopathogenic fungi for the control of stored-grain insects. Int. J. Pest Manag. 64, 77–87. doi: 10.1080/09670874.2017.1329565
Belda, C., and Riudavets, J. (2013). Natural enemies associated with lepidopteran pests in food and feed processing companies. J. Stored Prod. Res. 53, 54–60. doi: 10.1016/j.jspr.2013.02.006
Bell, C. H. (2014). “Pest control of stored food products: insects and mites,” in Hygiene in Food Processing: Principles and Practice, 2nd Edn., eds H. L. Lelieveld, J. Holah, and D. Napper (York: Woodhead Publishing Series in Food Science, Technology and Nutrition), 494–538. doi: 10.1533/9780857098634.3.494
Brabec, D., Dowell, F., Campbell, J., and West, M. (2017). Detection of internally infested popcorn using electrically conductive roller mills. J. Stored Prod. Res. 70, 37–43. doi: 10.1016/j.jspr.2016.11.002
Brezolin, A. N., Martinazzo, J., Muenchen, D. K., de Cezaro, A. M., Rigo, A. A., Steffens, C., et al. (2018). Tools for detecting insect semiochemicals: a review. Anal. Bioanal. Chem. 410, 4091–4108. doi: 10.1007/s00216-018-1118-3
Campolo, O., Giunti, G., Russo, A., Palmeri, V., and Zappalà, L. (2018). Essential oils in stored product insect pest control. J. Food Qual. 2018, 1–18. doi: 10.1155/2018/6906105
Cao, Y., Li, Y., and Li, G. (2010). Evaluation of inert dusts against phosphine resistant strains of Cryptolestes ferrugineus. Julius Kühn Archiv. 943–946. doi: 10.5073/jka.2010.425.167.191
Cassi, M. S. (2017). Approaches for the Biological Control of Stored Product Pests. Barcelona: Universitat Autònoma de Barcel.
Cheng, W., Lei, J., Ahn, J. E., Liu, T. X., and Zhu-Salzman, K. (2012). Effects of decreased O2 and elevated CO2 on survival, development, and gene expression in cowpea bruchids. J. Insect Physiol. 58, 792–800. doi: 10.1016/j.jinsphys.2012.02.005
Chigoverah, A. A., and Mvumi, B. M. (2016). Efficacy of metal silos and hermetic bags against stored-maize insect pests under simulated smallholder farmer conditions. J. Stored Prod. Res. 69, 179–189. doi: 10.1016/j.jspr.2016.08.004
Chiluwal, K., Kim, J., Bae, S., Do, R.oh, G. H., Park, H. J., and Park, C. G. (2019). Effect of gamma irradiation on fecundity, sterility, and female sex pheromone production of Callosobruchus chinensis (Coleoptera: Bruchidae). J. Econ. Entomol. 112, 156–163. doi: 10.1093/jee/toy317
Chiluwal, K., Lee, B. H., Kwon, T. H., Kim, J., Do Bae, S., Roh, G. H., et al. (2020). Synergistic effect of fumigation with ethyl formate and methyl salicylate on mortality of life stages of adzuki bean beetle, Callosobruchus chinensis (L.). J. Asia-Pacific Entomol. 23, 483–491. doi: 10.1016/j.aspen.2020.03.010
Constantin, M., Jagadeesan, R., Chandra, K., Ebert, P., and Nayak, M. K. (2020). Synergism between phosphine (PH3) and carbon dioxide (CO2): implications for managing PH3 resistance in rusty grain beetle (Laemophloeidae: Coleoptera). J. Econ. Entomol. 113, 1–8. doi: 10.1093/jee/toaa081
Conyers, S. T., and Bell, C. H. (2003). The effect of modified atmospheres on the survival of the eggs of four storage mite species. Exp. Appl. Acarol. 31, 115–130. doi: 10.1023/B:APPA.0000005110.19969.23
Cox, P. D. (2004). Potential for using semiochemicals to protect stored products from insect infestation. J. Stored Prod. Res. 40, 1–25. doi: 10.1016/S0022-474X(02)00078-4
Cox, P. D., and Collins, L. E. (2002). Factors affecting the behaviour of beetle pests in stored grain, with particular reference to the development of lures. J. Stored Prod. Res. 38, 95–115. doi: 10.1016/S0022-474X(01)00010-8
Daglish, G. J., Nayak, M. K., Arthur, F. H., and Athanassiou, C. G. (2018). “Insect pest management in stored grain,” in Recent Advances in Stored Product Protection, eds C. G. Athanassiou and F. H. Arthur (Brisbane, QLD: Springer-Verlag GmbH Germany), 45–63. doi: 10.1007/978-3-662-56125-6_3
Das, I., Kumar, G., and Shah, N. G. (2013). Microwave heating as an alternative quarantine method for disinfestation of stored food grains. Int. J. Food Sci. 2013, 926468. doi: 10.1155/2013/926468
De Carli, M., Bresolin, B., Noreña, C. P. Z., Lorini, I., and Brandelli, A. (2010). Efficacy of modified atmosphere packaging to control Sitophilus spp. in organic maize grain. Braz. Arch. Biol. Technol. 53, 1469–1476. doi: 10.1590/S1516-89132010000600024
De Carvalho Barbosa Negrisoli, C. R., Júnior, A. S. N., Bernardi, D., and Garcia, M. S. (2013). Activity of eight strains of entomopathogenic nematodes (Rhabditida: Steinernematidae, Heterorhabditidae) against five stored product pests. Exp. Parasitol. 134, 384–388. doi: 10.1016/j.exppara.2013.03.008
De Groote, H., Kimenju, S. C., Likhayo, P., Kanampiu, F., Tefera, T., and Hellin, J. (2013). Effectiveness of hermetic systems in controlling maize storage pests in Kenya. J. Stored Prod. Res. 53, 27–36. doi: 10.1016/j.jspr.2013.01.001
Debnath, N., Das, S., Seth, D., Chandra, R., Bhattacharya, S. C., and Goswami, A. (2011). Entomotoxic effect of silica nanoparticles against Sitophilus oryzae (L.). J. Pest Sci. 84, 99–105. doi: 10.1007/s10340-010-0332-3
Deka, M., Bhuyan, M., and Hazarika, L. (2006). Traditional pest management practices of Assam. Indian J. Trad. Knowl. 5, 75–78.
El-Saadony, M. T., Abd El-Hack, M. E., Taha, A. E., Fouda, M. M. G., Ajarem, J. S., Maodaa, S. N., et al. (2020). Ecofriendly synthesis and insecticidal application of copper nanoparticles against the storage pest tribolium castaneum. Nanomaterials 10, 587. doi: 10.3390/nano10030587
Esther, M., Sharon, M., Abirami, C. V. K., and Alagusundaram, K. (2014). Grain storage management in India. J. Postharvest Technol. 2, 12–14. Available online at: http://jpht.in/MenuscriptFile/03b17047-fa10-48ed-b0cb-95fb9222798f.pdf
Fields, P. G., and White, N. D. G. (2002). Alternatives to methyl bromide treatments for stored - product and quarantine insects. Annu. Rev. Entomol. 47, 331–359. doi: 10.1146/annurev.ento.47.091201.145217
Finkelman, S., Navarro, S., Isikber, A., Dias, D., Azrieli, A., Rindner, M., et al. (2002). “Application of vacuum to sealed flexible containers: a viable alternative to disinfestation of durable commodities with methyl bromide,” in Proc. International Conference on Alternatives to Methyl Bromide, 145–149. Available online at: https://www.researchgate.net/profile/Shlomo_Navarro/publication/236047554_APPLICATION_OF_VACUUM_TO_SEALED_FLEXIBLE_CONTAINERS_A_VIABLE_ALTERNATIVE_TO_DISINFESTATION_OF_DURABLE_COMMODITIES_WITH_METHYL_BROMIDE/links/00b49515f0453e78a0000000.pdf (accessed Feburary 03, 2020).
Finkelman, S., Navarro, S., Lotan, Y., Debruin, T., Isikber, A. A., Rindner, M., et al. (2003). “Insect control of cocoa pests using a novel vacuum approach. Advances in Stored Product Protection,” in Proceedings of the 8th International Working Conference on Stored Product Protection (York), 579–582. doi: 10.1079/9780851996912.0579
Flinn, P., and Scholler, M. (2012). “Biological control: Insect pathogens, parasitoids, and predators,” in Stored Product Protection, eds D.W. Hagstrum, T. W. Phillips, and G. Cuperus (Braunschweig: Kansas State University Publications), 203–212.
Garcia, M., Sosa, M. E., Donadel, O. J., Giordano, O. S., and Tonn, C. E. (2003). Effects of some sesquiterpenes on the stored-product insect Tenebrio molitor (Coleoptera: Tenebrionidae). Rev. Soc. Entomol. Argentina. 62, 17–26. Available online at: https://www.biotaxa.org/RSEA/article/view/28353
García-Lara, S., García-Jaimes, E., and Ortíz-Islas, S. (2020). Field effectiveness of improved hermetic storage technologies on maize grain quality in Central Mexico. J. Stored Prod. Res. 87, 101585. doi: 10.1016/j.jspr.2020.101585
Gilles, A. F., Schinko, J. B., and Averof, M. (2015). Efficient CRISPR-mediated gene targeting and transgene replacement in the beetle Tribolium castaneum. Development 142, 2832–2839. doi: 10.1242/dev.125054
Hagstrum, D. W., and Flinn, P. W. (2014). Modern stored-product insect pest management. J. Plant Protect. Res. 54, 205–210. doi: 10.2478/jppr-2014-0031
Hagstrum, D. W., Flinn, P. W., and Shuman, D. (1996). Automated monitoring using acoustical sensors for insects in farm-stored wheat. J. Econ. Entomol. 89, 211–217. doi: 10.1093/jee/89.1.211
Hagstrum, D. W., and Phillips, T. W. (2017). Evolution of stored-product entomology: protecting the world food supply. Annu. Rev. Entomol. 62, 379–397. doi: 10.1146/annurev-ento-031616-035146
Hagstrum, D. W., and Subramanyam, B. (2009). A Review of Stored-Product Entomology Information Sources. Am. Entomol. 5, 174–183. doi: 10.1093/ae/55.3.174
Hallman, G. J. (2013). Control of stored product pests by ionizing radiation. J. Stored Prod. Res. 52, 36–41. doi: 10.1016/j.jspr.2012.10.001
Han, G. D., Jung, Y. H., Kim, B. H., Chun, Y. S., Na, J., and Kim, W. (2018). Response of storage insect species to ClO2 fumigation conditions. J. Stored Prod. Res. 79, 112–115. doi: 10.1016/j.jspr.2018.10.002
Hansen, L. S., Hansen, P., and Vagn Jensen, K. M. (2013). Effect of gaseous ozone for control of stored product pests at low and high temperature. J. Stored Prod. Res. 54, 59–63. doi: 10.1016/j.jspr.2013.05.003
Hardin, J. A., Jones, C. L., Bonjour, E. L., Noyes, R. T., Beeby, R. L., Eltiste, D. A., et al. (2010). Ozone fumigation of stored grain; closed-loop recirculation and the rate of ozone consumption. J. Stored Prod. Res. 46, 149–154. doi: 10.1016/j.jspr.2010.03.002
Hasan, M., and Khan, A. R. (1998). Control of stored-product pests by irradiation. Integrated Pest Manag. Rev. 3, 15–29. doi: 10.1023/A:1009621606024
Hassan, R. S., Mahmoud, E. A., Sileem, T. M., and Sayed, W. A. A. (2019). Evaluation of fast neutron irradiation as a new control method against the Indian meal moth, Plodia interpunctella (Hübner). J. Radiat. Res. Appl. Sci. 12, 24–30. doi: 10.1080/16878507.2019.1594133
Hertlein, M. B., Thompson, G. D., Subramanyam, B., and Athanassiou, C. G. (2011). Spinosad: a new natural product for stored grain protection. J. Stored Prod. Res. 47, 131–146. doi: 10.1016/j.jspr.2011.01.004
Hui, Y. H., Bruinsma, L. B., Gorham, J. R., Nip, W.-K., Tong, P. S., and Ventresca, P. (2002). Food Plant Sanitation. Phillipines: CRC Press. doi: 10.1201/9780203910566
Isikber, A. A., and Athanassiou, C. G. (2015). The use of ozone gas for the control of insects and micro-organisms in stored products. J. Stored Prod. Res. 64, 139–145. doi: 10.1016/j.jspr.2014.06.006
Isikber, A. A., Tunaz, H., Athanassiou, C. G., Bilgili, Y., and Er, M. K. (2017). Toxicity of propylene oxide alone and in combination with low pressure or carbon dioxide against life stages of Ephestia cautella (Walker)(Lepidoptera: Pyralidae) under laboratory conditions. Crop. Protect. 98, 56–60. doi: 10.1016/j.cropro.2017.01.015
Iturralde-García, R. D., Wong-Corral, F. J., Castañé, C., and Riudavets, J. (2020). Susceptibility of Rhyzopertha dominica to high CO2 modified atmospheres in packaged chickpeas. J. Stored Prod. Res. 85, 101537. doi: 10.1016/j.jspr.2019.101537
Jagadeesan, R., Nayak, M. K., Pavic, H., Chandra, K., and Collins, P. J. (2015). Susceptibility to sulfuryl fluoride and lack of cross-resistance to phosphine in developmental stages of the red flour beetle, Tribolium castaneum (Coleoptera: Tenebrionidae). Pest Manag. Sci. 71, 1379–1386. doi: 10.1002/ps.3940
Jeong, G. H., Cho, S. W., Koo, H. N., Kim, H. K., Kim, B., Yang, J., et al. (2019). “Evaluation of fumigation activity of carbonyl sulfide to red flour beetle (Tribolium castaneum),” in 2019 Spring International Conference of KSAE. p. 105–105. Available online at: http://db.koreascholar.com/article.aspx?code=370018
Jian, F., Jayas, D. S., and White, N. D. G. (2013). Can ozone be a new control strategy for pests of stored grain? Agric. Res. 2, 1–8. doi: 10.1007/s40003-012-0046-2
Johnson, L., Kumar, C., Hayles, J., and Losic, D. (2014). “Grain protection with admixed inert dusts (diatomaceous earth): exploring key parameters for improving the efficacy of wet application Johnson, L.*#, Kumar, C., Hayles, J. Losic, D.*,” in 11th International Working Conference on Stored Product Protection Grain (Adelaide, SA), 828–840.
Kellen, W. R., and Lindegren, J. E. (1974). Comparative virulence of Nosema plodiae and Nosema heterosporum in the indian meal moth, Plodia interpunctella. J. Invertebr. Pathol. 23, 242–245. doi: 10.1016/0022-2011(74)90191-8
Kim, B. H., Han, G. D., Kwon, H., Chun, Y. S., Na, J., and Kim, W. (2019). Chlorine dioxide fumigation to control stored product insects in rice stored in a room. J. Stored Prod. Res. 84, 101527. doi: 10.1016/j.jspr.2019.101527
Kim, K. H., Yi, C. G., Ahn, Y. J., Kim, S. I., Lee, S. G., and Kim, J. R. (2015). Fumigant toxicity of basil oil compounds and related compounds to Thrips palmi and Orius strigicollis. Pest Manag. Sci. 71, 1292–1296. doi: 10.1002/ps.3925
Kumar, K. K., Sridhar, J., Murali-Baskaran, R. K., Senthil-Nathan, S., Kaushal, P., Dara, S. K., et al. (2019). Microbial biopesticides for insect pest management in India: current status and future prospects. J. Invertebr. Pathol. 165, 74–81. doi: 10.1016/j.jip.2018.10.008
Kumar, S., Mohapatra, D., Kotwaliwale, N., and Singh, K. K. (2017). Vacuum hermetic fumigation: a review. J. Stored Prod. Res. 71, 47–56. doi: 10.1016/j.jspr.2017.01.002
Kumar, S., Mohapatra, D., Kotwaliwale, N., and Singh, K. K. (2020). Efficacy of sensor assisted vacuum hermetic storage against chemical fumigated wheat. J. Stored Prod. Res. 88, 101640. doi: 10.1016/j.jspr.2020.101640
Kumar, S., Park, J., Kim, E., Na, J., Chun, Y. S., Kwon, H., et al. (2015). Oxidative stress induced by chlorine dioxide as an insecticidal factor to the Indian meal moth, Plodia interpunctella. Pestic. Biochem. Physiol. 124, 48–59. doi: 10.1016/j.pestbp.2015.04.003
Laznik, Ž., Tóth, T., Lakatos, T., Vidrih, M., and Trdan, S. (2010). The activity of three new strains of Steinernema feltiae against adults of Sitophilus oryzae under laboratory conditions. J. Food Agric. Environ. 8, 150–154. Available online at: https://www.researchgate.net/publication/228431732_The_activity_of_three_new_strains_of_Steinernema_feltiae_against_adults_of_Sitophilus_oryzae_under_laboratory_conditions
Lord, J. C., Campbell, J. F., Sedlacek, J. D., and Vail, P. V. (2007). “Application and evaluation of entomopathogens for managing lepidoptera in stored products,” in Field Manual of Techniques in Invertebrate Pathology, eds L. A. Lacey and H. K. Kaya (Frankfort: Springer), 677–693. doi: 10.1007/978-1-4020-5933-9_33
Losey, S. M., Daglish, G. J., and Phillips, T. W. (2019). Orientation of rusty grain beetles, Cryptolestes ferrugineus (Coleoptera: Laemophloeidae), to semiochemicals in field and laboratory experiments. J. Stored Prod. Res. 84, 101513. doi: 10.1016/j.jspr.2019.101513
Lu, P. J., Lin, H. C., Yu, W. T., and Chern, J. M. (2011). Chemical regeneration of activated carbon used for dye adsorption. J. Taiwan Inst. Chem. Eng. 42, 305–311. doi: 10.1016/j.jtice.2010.06.001
Macana, R. J., Moirangthem, T. T., and Baik, O. D. (2018). “50-ohm RF technology based applicator design and fabrication for disinfestation of insect pests in stored grains,” in ASABE 2018 Annual International Meeting Saskatchewan: American Society of Agricultural and Biological Engineers. doi: 10.13031/aim.201801011
Magan, N., Hope, R., Cairns, V., and Aldred, D. (2003). Post-harvest fungal ecology: impact of fungal growth and mycotoxin accumulation in stored grain. Eur. J. Plant Pathol. 109, 723–730. doi: 10.1023/A:1026082425177
Maille, J. M. (2019). The efficacy of propylene oxide, ethyl formate, and ethanedinitrile as fumigants to control Lasioderma serricorne (Fabricius, 1792)(Coleoptera: Anobiidae), Tyrophagus putrescentiae (Schrank, 1781)(Sarcoptiformes: Acaridae), and Necrobia rufipes (De Geer, 1775) (Coleoptera: Cleridae) (Doctoral dissertation). Kansas State University, Lawrence, KS, United States. Available online at: http://hdl.handle.net/2097/40244
Malaikozhundan, B., Vaseeharan, B., Vijayakumar, S., and Thangaraj, M. P. (2017). Bacillus thuringiensis coated zinc oxide nanoparticle and its biopesticidal effects on the pulse beetle, Callosobruchus maculatus. J. Photochem. Photobiol B Biol. 174, 306–314. doi: 10.1016/j.jphotobiol.2017.08.014
Malaikozhundan, B., and Vinodhini, J. (2018). Biological control of the Pulse beetle, Callosobruchus maculatus in stored grains using the entomopathogenic bacteria, Bacillus thuringiensis. Microb. Pathog. 114, 139–146. doi: 10.1016/j.micpath.2017.11.046
Manandhar, A., Milindi, P., and Shah, A. (2018). An overview of the post-harvest grain storage practices of smallholder farmers in developing countries. Agriculture 8, 57. doi: 10.3390/agriculture8040057
Mangang, I. B., Tiwari, A., Rajamani, M., and Manickam, L. (2020). Comparative laboratory efficacy of novel botanical extracts against Tribolium castaneum. J. Sci. Food Agric. 100, 1541–1546. doi: 10.1002/jsfa.10162
Matthias, S. (2010). “Prospects for biological control of stored-product pests,” in International European Symposium on Stored Product Protection “Stress on Chemical Products,” (January) (Germany), 25–31.
Mbata, G. N., Johnson, M., Phillips, T. W., and Payton, M. (2005). Mortality of life stages of cowpea weevil (Coleoptera: Bruchidae) exposed to low pressure at different temperatures. J. Econ. Entomol. 98, 1070–1075. doi: 10.1603/0022-0493-98.3.1070
Mbata, G. N., and Phillips, T. W. (2001). Effects of temperature and exposure time on mortality of stored-product insects exposed to low pressure. J. Econ. Entomol. 94, 1302–1307. doi: 10.1603/0022-0493-94.5.1302
Meruvu, H., Vangalapati, M., Chaitanya Chippada, S., and Rao Bammidi, S. (2011). Synthesis and characterization of zinc oxide nanoparticles and its antimicrobial activity against Bacillus subtilis and Escherichia coli. Rasayan J. Chem. 4, 217–222. Available online at: http://rasayanjournal.co.in/vol-4/issue-1/33.pdf
Milner, R. J. (1973). Nosema whitei, a microsporidan pathogen of some species of Tribolium V. Comparative pathogenicity and host range. Entomophaga 18, 383–390. doi: 10.1007/BF02371014
Mohapatra, D., Kar, A., and Giri, S. K. (2015). Insect pest management in stored pulses: an overview. Food Bioprocess Technol. 8, 239–265. doi: 10.1007/s11947-014-1399-2
Morales, H. (2002). Pest management in traditional tropical agroecosystems: lessons for pest prevention research and extension. Integrated Pest Manag. Rev. 7, 145–163. doi: 10.1023/B:IPMR.0000027502.91079.01
Morrison, W. R., Larson, N. L., Brabec, D., and Zhang, A. (2019). Methyl benzoate as a putative alternative, environmentally friendly fumigant for the control of stored product insects. J. Econom. Entomol. 112, 2458–2468. doi: 10.1093/jee/toz179
Mossa, A. T. H. (2016). Green pesticides: essential oils as biopesticides in insect-pest management. J. Environ. Sci. Technol. 9, 354–378. doi: 10.3923/jest.2016.354.378
Navarro, S. (2012). The use of modified and controlled atmospheres for the disinfestation of stored products. J. Pest Sci. 85, 301–322. doi: 10.1007/s10340-012-0424-3
Navarro, S., Adler, C., Riudavets, J., and Stejskal, V. (2004). “Working group “Integrated Protection of Stored Products.”,” in Proceedings of the Meeting Compte Rendu de la Réunion, IOBC WPRS BULLETIN, Vol. 27 (Czechia).
Navarro, S., Donahaye, J. E., Dias, R., Azrieli, A., Rindner, M. I., Phillips, T., et al. (2001). “Application of vacuum in a transportable system for insect control,” in International Conference on Controlled Atmospheres and Fumigation in Stored Products (Lisbon), 307–315.
Nayak, M. K., Jagadeesan, R., Kaur, R., Daglish, G. J., Reid, R., Pavic, L. W., et al. (2016). Use of sulfuryl fluoride in the management of strongly phosphine-resistant insect pest populations in bulk grain storages in Australia. Indian J. Entomol. 78, 100–107. doi: 10.5958/0974-8172.2016.00030.4
Nelson, S. O., and Whitney, W. K. (1960). Radio-frequency electric fields for stored-grain insect control. Trans. Am. Soc. Agric. Biol. Eng. 3, 133–137. doi: 10.13031/2013.41143
Opit, G. P., Thoms, E., Phillips, T. W., and Payton, M. E. (2016) Effectiveness of sulfuryl fluoride fumigation for the control of phosphine-resistant grain insects infesting stored wheat. J. Econom. Entomol. 109, 930–941. doi: 10.1093/jee/tov395
Paul, A., Radhakrishnan, M., Anandakumar, S., Shanmugasundaram, S., and Anandharamakrishnan, C. (2020). Disinfestation techniques for major cereals: a status report. Compreh. Rev. Food Sci.and Food Saf. 19, 1125–1155. doi: 10.1111/1541-4337.12555
Pearson, T. C., Brabec, D. L., and Schwartz, C. R. (2003). Automated detection of internal insect infestations in whole wheat kernels using a perten SKCS 4100. Appl. Eng. Agric. 19, 727–733. doi: 10.13031/2013.15654
Perez-Mendoza, J., Flinn, P. W., Campbell, J. F., Hagstrum, D. W., and Throne, J. E. (2004). Detection of stored-grain insect infestation in wheat transported in railroad hopper-cars. J. Econ. Entomol. 97, 1474–1483. doi: 10.1093/jee/97.4.1474
Perkin, L. C., Adrianos, S. L., and Oppert, B. (2016). Gene disruption technologies have the potential to transform stored product insect pest control. Insects 7, 46. doi: 10.3390/insects7030046
Phillips, T. W., and Throne, J. E. (2010). Biorational approaches to managing stored-product insects. Annu. Rev. Entomol. 55, 375–397. doi: 10.1146/annurev.ento.54.110807.090451
Pimentel, M. A. G., Faroni, L. R. D. A., Guedes, R. N. C., Sousa, A. H., and Tótola, M. R. (2009). Phosphine resistance in Brazilian populations of Sitophilus zeamais Motschulsky (Coleoptera: Curculionidae). J. Stored Prod. Res. 45, 71–74. doi: 10.1016/j.jspr.2008.09.001
Purohit, P., Jayas, D. S., Yadav, B. K., Chelladurai, V., Fields, P. G., and White, N. D. G. (2013). Microwaves to control Callosobruchus maculatus in stored mung bean (Vigna radiata). J. Stored Prod. Res. 53, 19–22. doi: 10.1016/j.jspr.2013.01.002
Qi, L., Xu, M., Fu, Z., Mira, T., and Zhang, X. (2014). C2SLDS: A WSN-based perishable food shelf-life prediction and LSFO strategy decision support system in cold chain logistics. Food Control 38, 19–29. doi: 10.1016/j.foodcont.2013.09.023
Rahman, M. A., Parvin, A., Khan, M. S. H., Lingaraju, K., Prasad, R., Das, S., et al. (2020). Efficacy of the green synthesized nickel-oxide nanoparticles against pulse beetle, Callosobruchus maculatus (F.) in black gram (Vigna mungo L.). Int. J. Pest Manag. 67, 1–9. doi: 10.1080/09670874.2020.1773572
Rajendran, S. (2001). Alternatives to methyl bromide as fumigants for stored food commodities. Pesticide Outlook 12, 249–253. doi: 10.1039/b110550g
Rajendran, S., and Sriranjini, V. (2008). Plant products as fumigants for stored-product insect control. J. Stored Prod. Res. 44, 126–135. doi: 10.1016/j.jspr.2007.08.003
Ramadan, G. R., Zhu, K. Y., Abdelgaleil, S. A., Shawir, M. S., El-Bakary, A. S., Edde, P. A., et al. (2020). Ethanedinitrile as a fumigant for Lasioderma serricorne (Coleoptera: Anobiidae), and Rhyzopertha dominica (Coleoptera: Bostrichidae): toxicity and mode of action. J. Econom. Entomol. 113, 1519–1527. doi: 10.1093/jee/toz343
Ramya, R., Srivastava, C., and Subramanian, S. (2018). Monitoring of phosphine resistance in Indian populations of Tribolium castaneum (Herbst) from stored wheat. Indian J. Entomol. 80, 19–23. doi: 10.5958/0974-8172.2018.00005.6
Ridley, A. W., Hereward, J. P., Daglish, G. J., Raghu, S., Collins, P. J., and Walter, G. H. (2011). The spatiotemporal dynamics of Tribolium castaneum. (Herbst): adult flight and gene flow. Mol. Ecol. 20, 1635–1646. doi: 10.1111/j.1365-294X.2011.05049.x
Riudavets, J., Castañé, C., Alomar, O., Pons, M. J., and Gabarra, R. (2009). Modified atmosphere packaging (MAP) as an alternative measure for controlling ten pests that attack processed food products. J. Stored Prod. Res. 45, 91–96. doi: 10.1016/j.jspr.2008.10.001
Riudavets, J., Castañé, C., Alomar, O., Pons, M. J., and Gabarra, R. (2010). The use of carbon dioxide at high pressure to control nine stored-product pests. J. Stored Prod. Res. 46, 228–233. doi: 10.1016/j.jspr.2010.05.005
Rodríguez, S. A. (2018). Semiochemistry of the Bruchidae species. J. Stored Prod. Res. 77, 148–155. doi: 10.1016/j.jspr.2018.04.011
Rumbos, C. I., and Athanassiou, C. G. (2017). Use of entomopathogenic fungi for the control of stored-product insects: can fungi protect durable commodities? J. Pest Sci. 90, 839–854. doi: 10.1007/s10340-017-0849-9
Said, P. P., and Pradhan, R. (2014). Food grain storage practices-a review. J. Grain Process. Storage 1, 1–5. Available online at: http://www.jakraya.com/journal/pdf/1-jgpsArticle.pdf
Sallam, M. N. (1999). “Insect damage: post-harvest operations,” in INPhO-Post-Harvest Compendium of FAO (FAO).
Sambaraju, K. R., and Phillips, T. W. (2008). Responses of adult Plodia interpunctella (Hübner) (Lepidoptera: Pyralidae) to light and combinations of attractants and light. J. Insect Behav. 21, 422–439. doi: 10.1007/s10905-008-9140-5
Sanon, A., Dabiré-Binso, L. C., and Ba, N. M. (2011). Triple-bagging of cowpeas within high density polyethylene bags to control the cowpea beetle Callosobruchus maculatus F. (Coleoptera: Bruchidae). J. Stored Prod. Res. 47, 210–215. doi: 10.1016/j.jspr.2011.02.003
Shah, M. A., and Khan, A. A. (2014). Use of diatomaceous earth for the management of stored-product pests. Int. J. Pest Manag. 60, 100–113. doi: 10.1080/09670874.2014.918674
Shankar, U., and Abrol, D. P. (2012). “Integrated pest management in stored grains,” in Integrated Pest Management: Principles and Practice (CABI), 386–407. doi: 10.1079/9781845938086.0386
Shapiro-Ilan, D. I., Lacey, L. A., and Siegel, J. P. (2007). “Microbial control of insect pests of stone fruit and nut crops,” In Field Manual of Techniques in Invertebrate Pathology, eds L. A. Lacey and H. K. Kaya (Springer), 547–565. doi: 10.1007/978-1-4020-5933-9_26
Shen, Y., Zhou, H., Li, J., Jian, F., and Jayas, D. S. (2018). Detection of stored-grain insects using deep learning. Comput. Electron. Agric. 145, 319–325. doi: 10.1016/j.compag.2017.11.039
Sileem, T., Mohamed, S., and Mehany, A. (2019). First Trial to assess gamma-irradiation and diatomaceous earth combination on mortality of some stored product insects. Arab J. Nuclear Sci. Applic. 52, 115–120. doi: 10.21608/ajnsa.2019.6159.1134
Sinclair, E. R., and White, E. R. (1980). Stored products insect pests in combine harvesters on the Darling Downs. Queensland J. Agric. Anim. Sci. 37, 93–99.
Singh, B., and Kaur, A. (2018). Control of insect pests in crop plants and stored food grains using plant saponins: a review. LWT Food Sci. Technol. 87, 93–101. doi: 10.1016/j.lwt.2017.08.077
Singh, R., Singh, K. K., and Kotwaliwale, N. (2012). Study on disinfestation of pulses using microwave technique. J. Food Sci. Technol. 49, 505–509. doi: 10.1007/s13197-011-0296-1
Smit, R. (2019). Postharvest phytosanitary disinfestation strategies using thermal and atmospheric stress : commodity and insect tolerances (Stellenbosch University). Available online at: https://scholar.sun.ac.za/bitstream/handle/10019.1/107254/smit_postharvest_2019.pdf?sequence=1 (accessed July 18, 2021).
Somavat, P., Huang, H., Kumar, S., Garg, M. K., Danao, M. C., Singh, V., et al. (2017). Comparison of hermetic storage of wheat with traditional storage methods in India. Appl. Eng. Agric. 33, 121–130. doi: 10.13031/aea.11792
Sousa, A. H., Faroni, L. R. D. A., Guedes, R. N. C., Tótola, M. R., and Urruchi, W. I. (2008). Ozone as a management alternative against phosphine-resistant insect pests of stored products. J. Stored Prod. Res. 44, 379–385. doi: 10.1016/j.jspr.2008.06.003
Srivastava, C., and Subramanian, S. (2016). Storage insect pests and their damage symptoms: an overview. J. Grain Stor. Res. 78, 53–58. doi: 10.5958/0974-8172.2016.00025.0
Stadler, T., Buteler, M., and Weaver, D. K. (2010). Novel use of nanostructured alumina as an insecticide. Pest Manag. Sci. 66, 577–579. doi: 10.1002/ps.1915
Stejskal, V. (2015). Pest prevention during storage, transportation and handling of stored products. Integrated Protect. Stored Products IOBC WPRS Bull. 111, 171–176. Available online at: https://www.researchgate.net/profile/Vaclav-Stejskal-2/publication/288839823_Pest_prevention_during_storage_transportation_and_handling_of_stored_products/links/5686d33008ae1e63f1f5adf1/Pest-prevention-during-storage-transportation-and-handling-of-stored-products.pdf
Subramanyam, B., Xinyi, E., Savoldelli, S., and Sehgal, B. (2017). Efficacy of ozone against Rhyzopertha dominica adults in wheat. J. Stored Prod. Res. 70, 53–59. doi: 10.1016/j.jspr.2016.12.002
Talukder, F. A. (2006). Plant products as potential stored-product insect management agent - a mini review. Emirates J. Agric. Sci. 18, 17–32. doi: 10.9755/ejfa.v12i1.5221
Thielens, A., Bell, D., Mortimore, D. B., Greco, M. K., Martens, L., and Joseph, W. (2018). Exposure of insects to radio-frequency electromagnetic fields from 2 to 120 GHz. Sci. Rep. 8, 1–10. doi: 10.1038/s41598-018-22271-3
Trematerra, P. (2012). Advances in the use of pheromones for stored-product protection. J. Pest Sci. 85, 285–299. doi: 10.1007/s10340-011-0407-9
Tutuncu, S., and Emekci, M. (2019). Comparative efficacy of modified atmospheres enriched with carbon dioxide against Cadra (=Ephestia) cautella. J. Sci. Food Agric. 99, 5962–5968. doi: 10.1002/jsfa.9871
Vail, P. V., Tebbets, J. S., Cowan, D. C., and Jenner, K. E. (1991). Efficacy and persistence of a granulosis virus against infestations of Plodia interpunctella (Hübner) (Lepidoptera: Pyralidae) on raisins. J. Stored Prod. Res. 27, 103–107. doi: 10.1016/0022-474X(91)90019-9
van Lenteren, J. C., Bolckmans, K., Köhl, J., Ravensberg, W. J., and Urbaneja, A. (2018). Biological control using invertebrates and microorganisms: plenty of new opportunities. Biocontrol 63, 39–59. doi: 10.1007/s10526-017-9801-4
Vassilakos, T. N., Athanassiou, C. G., and Tsiropoulos, N. G. (2015). Persistence and efficacy of spinetoram against three major stored grain beetle on wheat. Crop Prot. 69, 44–51. doi: 10.1016/j.cropro.2014.08.010
Wang, S., Ikediala, J. N., Tang, J., Hansen, J. D., Mitcham, E., Mao, R., et al. (2001). Radio frequency treatments to control codling moth in in-shell walnuts. Postharvest Biol. Technol. 22, 29–38. doi: 10.1016/S0925-5214(00)00187-3
White, S. D., Murphy, P. T., Bern, C. J., and van Leeuwen, J. (Hans). (2010). Controlling deterioration of high-moisture maize with ozone treatment. J. Stored Prod. Res. 46, 7–12. doi: 10.1016/j.jspr.2009.07.002
Wong-Corral, F. J., Castañé, C., and Riudavets, J. (2013). Lethal effects of CO2-modified atmospheres for the control of three Bruchidae species. J. Stored Prod. Res. 55, 62–67. doi: 10.1016/j.jspr.2013.08.005
Xinyi, E., Subramanyam, B., and Li, B. (2017). Responses of phosphine susceptible and resistant strains of five stored-product insect species to chlorine dioxide. J. Stored Prod. Res. 72, 21–27. doi: 10.1016/j.jspr.2017.03.002
Yewle, N., Swain, K. C., Mann, S., and Guru, P. N. (2022). Performance of hermetic bags in green gram [Vigna radiata (L.) R. Wilczek] storage for managing pulse beetle (Callosobruchus chinensis). J. Stored Products Res. 95, 101896. doi: 10.1016/j.jspr.2021.101896
Zayed, G. M. M. (2018). Nano-particles : a recent approach for controlling stored grain insect pests. Acad. J. Agric. Res. 6, 88–94. doi: 10.15413/ajar.2017.IECCNA.14
Zettler, J. L., and Arthur, F. H. (2000). Chemical control of stored product insects with fumigants and residual treatments. Crop Prot. 19, 577–582. doi: 10.1016/S0261-2194(00)00075-2
Zettler, J. L., and Cuperus, G. W. (1990). Pesticide resistance in Tribolium castaneum (Coleoptera: Tenebrionidae) and Rhyzopertha dominica (Coleoptera: Bostrichidae) in wheat. J. Econ. Entomol. 83, 1677–1681. doi: 10.1093/jee/83.5.1677
Zhang, T., Cao, Y., Li, Y. Y., Gao, Y.-S., and Feng, J. H. (2014). “Food-grade inert dust as structural treatment against insect pests,” in 11th International Working Conference on Stored Product Protection, 883–884. doi: 10.14455/DOA.res.2014.155 Available online at: http://spiru.cgahr.ksu.edu/proj/iwcspp/pdf2/11/137.pdf
Keywords: stored grain pests, fumigation, irradiation, biological control, food safety and security, nanotechnology and molecular interventions
Citation: Guru PN, Mridula D, Dukare AS, Ghodki BM, Paschapur AU, Samal I, Nikhil Raj M, Padala VK, Rajashekhar M and Subbanna ARNS (2022) A comprehensive review on advances in storage pest management: Current scenario and future prospects. Front. Sustain. Food Syst. 6:993341. doi: 10.3389/fsufs.2022.993341
Received: 13 July 2022; Accepted: 22 September 2022;
Published: 04 November 2022.
Edited by:
Arun K. Bhunia, Purdue University, United StatesReviewed by:
Muhammad Shakeel, South China Agricultural University, ChinaDebabandya Mohapatra, Central Institute of Agricultural Engineering (ICAR), India
Copyright © 2022 Guru, Mridula, Dukare, Ghodki, Paschapur, Samal, Nikhil Raj, Padala, Rajashekhar and Subbanna. This is an open-access article distributed under the terms of the Creative Commons Attribution License (CC BY). The use, distribution or reproduction in other forums is permitted, provided the original author(s) and the copyright owner(s) are credited and that the original publication in this journal is cited, in accordance with accepted academic practice. No use, distribution or reproduction is permitted which does not comply with these terms.
*Correspondence: Amit Umesh Paschapur, YW1pdHAzOTI5QGdtYWlsLmNvbQ==; P. N. Guru, Z3VydXBuNTAxNkBnbWFpbC5jb20=
†Present address: Ajinath Shridhar Dukare, Chemical and Biochemical Processing Division ICAR-Central Institute for Research On Cotton Technology (CIRCOT), Mumbai, India