- Department of Grassland and Livestock, Norwegian Institute of Bioeconomy Research, NIBIO, Ås, Norway
Digestate, a by-product from anaerobic digestion of organic materials such as animal manure, is considered a suitable plant fertilizer. However, due to its bulkiness and low economic value, it is costly to transport over long distances and store for long periods. Refinement processes to valorize digestate and facilitate its handling as a fertilizer include precipitation of phosphorus-rich mineral compounds, such as struvite and calcium phosphates, membrane filtration methods that concentrate plant nutrients in organic products, and carbonization processes. However, phosphorus retention efficiency in output products from these processes can vary considerably depending on technological settings and characteristics of the digestate feedstock. The effects of phosphorus in plant fertilizers (including those analogous or comparable to refined digestate products) on agronomic productivity have been evaluated in multiple experiments. In this review, we synthesized knowledge about different refinement methods for manure-based digestate as a means to produce phosphorus fertilizers, thereby providing the potential to increase phosphorus retention in the food production chain, by combining information about phosphorus flows in digestate refinement studies and agronomic fertilizer studies. It was also sought to identify the range, uncertainty, and potential retention efficiency by agricultural crops of the original phosphorus amount in manure-based digestate. Refinement chains with solid/wet phase separation followed by struvite or calcium phosphate precipitation or membrane filtration of the wet phase and carbonization treatments of the solid phase were included. Several methods with high potential to extract phosphorus from manure-based wet phase digestate in such a way that it could be used as an efficient plant fertilizer were identified, with struvite precipitation being the most promising method. Synthesis of results from digestate refinement studies and agronomic fertilizer experiments did not support the hypothesis that solid/wet separation followed by struvite precipitation, or any other refinement combination, results in higher phosphorus retention than found for unrefined digestate. Further studies are needed on the use of the phosphorus in the solid phase digestate, primarily on phosphorus-rich soils representative of animal-dense regions, to increase understanding of the role of digestate refinement (particularly struvite precipitation) in phosphorus recycling in agricultural systems.
Introduction
Phosphorus is key to plant growth and agricultural productivity. Rock phosphate, the main source for mineral phosphorus fertilizers, is a finite and dwindling resource (Nesme et al., 2015; Chowdhury et al., 2017). In addition, most of the exploited rock phosphate is used in plant fertilizers and animal feed (Tao et al., 2016). In many agricultural systems, sub-optimal fertilizer management results in large losses of phosphorus from soil, with associated negative environmental effects, particularly eutrophication of water bodies (Schoumans et al., 2014; Kleinman et al., 2015; Chowdhury et al., 2017; Nedelciu et al., 2020). Production of mineral fertilizers from rock phosphate also emits greenhouse gases and generates phosphogypsum, a calcium sulfate hydrate that is weakly radioactive due to its content of uranium and thorium (Nedelciu et al., 2020), as well as other hazardous compounds such as heavy metals (Aydin et al., 2010). Phosphorus losses are a particular problem in intensive animal production regions, where there is a net import surplus of phosphorus through animal feed. In these regions, large amounts of animal manure with a phosphorus content often exceeding plant demand are applied on agricultural land (Nesme et al., 2015). The main reason for local application is the bulkiness and low economic value of manure, which makes it costly to transport (Garbs and Geldermann, 2018).
Manure can be used in anaerobic digesters to produce biogas. The main output is methane, which is used for heating and electricity generation, replacing fossil energy (Yao et al., 2020). The residues from anaerobic digestion, digestate, has a higher concentration of phosphorus and other plant nutrients than the initial input manure (Möller and Müller, 2012; Shi et al., 2018). Despite this nutrient richness, digestate is still bulky and costly to transport over long distances (Herbes et al., 2020). Typically, the dry matter content of manure-based digestate is lower than 13%, the total N content is lower than 10 kg per Mg fresh weight and the total P content lower than 3 kg per Mg fresh weight (Möller and Müller, 2012). In order to overcome the bulkiness of digestate and better exploit its content of phosphorus and other plant nutrients, additional refinement processes for nutrient extraction and concentration into more manageable plant fertilizers have been developed. In a step prior to processing that is specifically designed to extract phosphorus, mechanical solid wet phase separation by screw press or centrifuges can be performed (Guilayn et al., 2019). Precipitation of mineral compounds, such as magnesium ammonium phosphate (commonly named struvite) and calcium phosphates from the wet phase, produces well-documented high-quality fertilizers (Estevez et al., 2014; Tao et al., 2016; Hertzberger et al., 2020; Egene et al., 2021). With membrane filtration of the wet phase, phosphorus is retained in the membrane (Gienau et al., 2018b). Thermal treatments applied to the solid phase include carbonization processes such hydrothermal carbonization (HTC), which is also called wet torrefication (Cao et al., 2019; Aragón-Briceño et al., 2021), and pyrolysis (Tsai et al., 2018). The solid phase can also be composted (Tambone et al., 2015; Torres-Climent et al., 2015).
Struvite and calcium phosphates can also be produced from sources such as municipal or food processing water (Kataki et al., 2016; Zin and Kim, 2019). The agronomic characteristics of these products, particularly phosphorus acquisition and use efficiency in plants, have been evaluated to varying degrees (Kataki et al., 2016; Li et al., 2019). Physical and chemical characteristics of these refined fertilizers are well-known and relatively homogenous across different feedstocks (Rahman et al., 2014; Kataki et al., 2016; Li et al., 2016). Therefore, studies on the agronomic phosphorus use efficiency of struvite and calcium phosphates of non-digestate origin can add useful information regarding the prospects of refining digestate from manure as a means to increase phosphorus retention in the food production chain by recycling phosphorus in animal manure.
Studies on digestate refinement usually evaluate phosphorus retention efficiency as percentage of digestate phosphorus recovered in fertilizer products, such as membrane filtration retentates (Adam et al., 2018) and precipitated struvite (Tao et al., 2016). Previous reviews on the nutrient aspects of digestate refinement have mainly focused on nutrient content of the refined products (Vaneeckhaute et al., 2017; Shi et al., 2018; Vasco-Correa et al., 2018), excluding or only briefly covering their effects on crops and crop productivity. The effects of phosphorus from plant fertilizers on plant productivity have been evaluated in large numbers of agronomic experiments (Schneider et al., 2019). Phosphorus acquisition efficiency can be defined as a plant's ability to take up phosphorus from the soil whereas the phosphorus use efficiency usually means the ability to produce biomass per amount of phosphorus taken up (Veneklaas et al., 2012; Campos et al., 2018). The ratio between plant-absorbed phosphorus and phosphorus applied in the fertilizer is a commonly used metric to assess efficiency of the phosphorus acquisition, whereas the biomass produced per amount of phosphorus supplied, instead of the phosphorus taken up, can be used to assess the phosphorus use efficiency in fertilizer experiments or other agronomic studies (MacDonald et al., 2011). Phosphorus use of a specific product have also be expressed as the amount of phosphorus that is taken up relative to another reference product, such as a commercial mineral phosphorus fertilizer (Vanden Nest et al., 2021).
Some agronomic studies have included effects of unprocessed digestate on soils and plants (Möller and Müller, 2012; Nkoa, 2014; Möller, 2015). However, studies analyzing nutrient uptake after application of refined digestate phosphorus fertilizers or analogous products, did not take percentage nutrient recovery during manufacture of the fertilizer into account. There is thus a lack of understanding on the usefulness and prospect for further development of digestate refinement processes to produce phosphorus fertilizers. Knowledge about the potential of digestate refinement to increase phosphorus retention in the food production chain could be gained by combining information about phosphorus flows obtained in digestate refinement studies and agronomic fertilizer studies. This could help identify processing and use chains with high retention of phosphorus from manure to crop, minimizing losses and uncertainties in phosphorus capture by plants associated with such chains. A high retention of phosphorus in food production chains and reduced losses are, in particular, important to reduce the use of finite rock phosphate resources for mineral phosphorus fertilizer production and the negative environmental consequences that are associated with this production, as well as the resulting from excessive spreading of animal manure, mentioned above.
The main aim of this review was to synthesize knowledge about the usefulness of different refinement methods for manure-based digestate and the efficacy of the refined products as phosphorus fertilizers, hence identifying refinement methods, which can contribute to a high phosphorus retention in the food production chain. An additional aim was to identify the range, uncertainty and potential for improvement of the retention efficiency in agricultural crops of original phosphorus contained in manure-based digestate. To collate information on phosphorus retention in digestate refinement studies and agronomic phosphorus fertilizer studies, a baseline system with non-refined digestate used as a plant fertilizer was compared with systems with solid wet separation followed by struvite and calcium phosphate precipitation, or membrane filtration of the wet phase, and use of the solid fraction as a soil fertilizer directly, or following carbonization (HTC or pyrolysis) or composting. The structure of the overall review is outlined in section Review structure. The results of digestate refinement studies are presented in section Digestate refinement and phosphorus concentration and those on agronomic phosphorus acquisition efficiency in section Refined digestate as phosphorus fertilizer. In section Synthesis and research outlook, the results from sections Digestate refinement and phosphorus concentration and Refined digestate as phosphorus fertilizer are synthesized to identify refinement and use pathways for high retention of phosphorus in the food production system, and areas for future research are identified.
Review structure
Phosphorus flows in the chain from outlet of the anaerobic digester to phosphorus uptake in the plant were considered (Figure 1). Key steps to increase phosphorus retention in food production systems that include anaerobic digestion of manure were identified, i.e., only studies on digestate that fully or partly originated from animal manure were considered. Other digestate feedstocks were not considered. Animal manure-based digestate is an environmental concern in animal-dense regions. It is also characterized by higher phosphorus content than digestate generated from other sources, such as plant residues or municipal and food waste (Wang and Lee, 2021). As regards phosphorus flows in crop production, studies covering manure-based digestate or refined products from manure-based digestate and also analogous or comparable products from other processing chains were considered. In particular, systems with non-refined digestate and systems where wet and solid fractions of separated digestate were refined were compared. The latter systems included wet fraction refinement consisting of a) precipitation of mineral phosphorus-rich compounds such as struvite and calcium phosphates or b) membrane filtration technologies producing retentate with elevated phosphorus concentration, and c) treatments of the solid fraction, including carbonization and composting. The reason for using unprocessed digestate as a baseline, instead of unprocessed manure, was that virtually all phosphorus is retained in the digestate, apart from any leaching losses during anaerobic digestion. This approach allowed a range of phosphorus retention efficiency to be determined and limitations in retention efficiency from specific treatments to be identified.
Digestate refinement and phosphorus concentration
There are various digestate refinement processes which result in phosphorus-rich compounds, including (1) precipitation or crystallization of phosphorus-rich components, specifically struvite and calcium phosphates; (2) membrane filtration technologies, which concentrate phosphorus in organic materials, and (3) carbonization processes, including pyrolysis and HTC, and (4) composting. Processes (1) and (2) are applied to wet phase digestate after solid/wet separation, whereas type (3) and (4) processes are applied to the solid phase digestate (Grigatti et al., 2015; Vaneeckhaute et al., 2017).
Precipitation
Struvite precipitation is normally preceded by solid/wet separation of the digestate, after which struvite is precipitated from the wet phase. In order for struvite to precipitate its constituting ions Mg2+, , and need to exceed the solubility product of struvite (Siciliano et al., 2020). In order decrease the solubility of these ions, the pH of the digestate is increased, usually by adding sodium hydroxide (Vaneeckhaute et al., 2017). The optimal pH which minimizes solubility has varied between around 8 and 10 in experimental studies with digestate (Siciliano et al., 2020) and is also dependent on the digestate origin (Tao et al., 2016). It is also common to add magnesium (in the form of MgO or MgCl2) to enhance the removal of phosphorus (Vaneeckhaute et al., 2017). The precipitation and formation of struvite crystals are also temperature dependent, and optimal ranges typically vary between 25 and 35°C (Siciliano et al., 2020). Moreover, the presence of a number of ions including Ca2+, Na+, K+, , can hinder the formation of struvite crystals (Siciliano et al., 2020) and may also change the morphology of the precipitated struvite crystals (Oliveira et al., 2021). Precipitation of calcium phosphates, such as CaHPO4 × 2H2O or Ca5(PO4)3OH can be an alternative way of extracting phosphorus from digestate (Vaneeckhaute et al., 2017; Barampouti et al., 2020). Also the precipitation of calcium phosphates is highly dependent on the pH (Vanotti and Szogi, 2009). A pH of 9 that is favorable for precipitation is typically reached by adding calcium hydroxide (Vaneeckhaute et al., 2017; Barampouti et al., 2020). The fraction of phosphorus retained in the wet phase after separation governs the fraction of total digestate phosphorus that can be retained in struvite or calcium phosphates. In addition to the conditions during the precipitation process, the precipitated phosphorus fraction varies with the origin and characteristics of the digestate (Table 1). Particle-bound phosphorus comprises a major proportion of total phosphorus in manure-based digestate (Egene et al., 2021). These particles can be solubilized into the wet phase by acidification, e.g., by adding sulfuric acid (Pedizzi et al., 2018) or formic acid (Daumer et al., 2010), or by microwave treatment, thereby increasing the fraction of total digestate phosphorus available for precipitation (Tao et al., 2016).
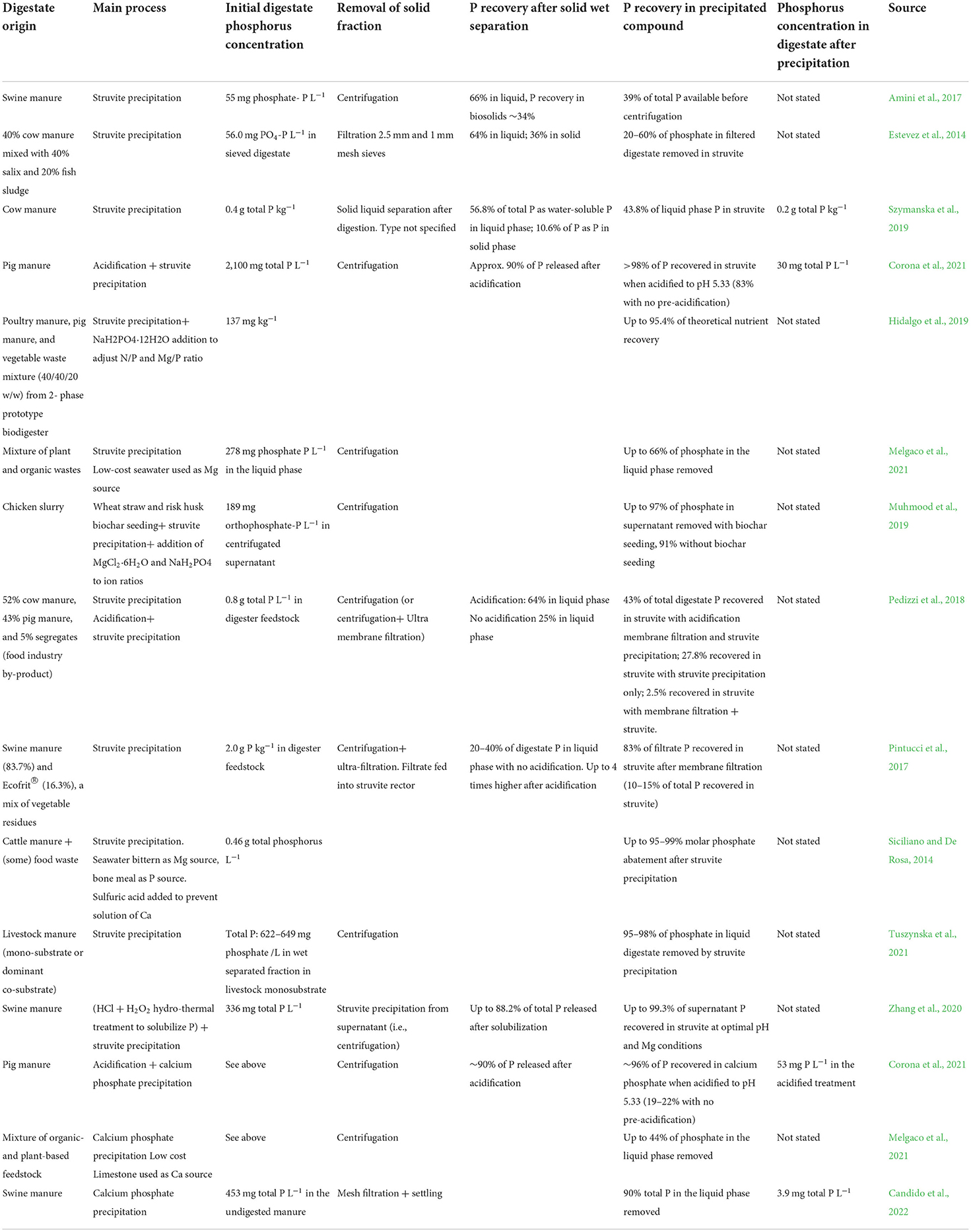
Table 1. Phosphorus (P) retention in experiments on precipitation of struvite and calcium phosphates from manure-based digestate.
Membrane filtration technologies
Different membrane filtration processes, including micro-, ultra-, and nanofiltration, reverse osmosis, forward osmosis, membrane distillation and electrodialysis, have been developed or tested to treat the wet phase of separated digestate (Camilleri-Rumbau et al., 2021). Membranes are often based of polymeric compounds or aluminum or titan oxides. Micro- or ultrafiltration enables the removal of macromolecules, whereas reverse osmosis enables the separation and concentration of phosphates or other soluble compounds (Camilleri-Rumbau et al., 2021). Combining different membranes has been shown to be favorable in removal of both particle-bound and dissolved phosphorus (Vaneeckhaute et al., 2017; Zacharof et al., 2019; Zhan et al., 2020). Hence, micro- ultra- or nanofiltration has often been combined with reverse osmosis in experimental studies resulting in a set of different retentate fractions (Adam et al., 2018; Camilleri-Rumbau et al., 2021). In electrodialytic processes, positive ions are transported across an ion exchange membrane to a cathode and negative ions to an anode (Oliveira et al., 2021). This has been shown an efficient method to separate phosphorus from heavy metals in municipal solid waste and sewage sludge (Oliveira et al., 2020, 2021) and could possibly be applied to digestate from manure as well. Reported retention of phosphorus varies substantially (from 16 to 99%) between membrane filtration studies (Le Corre et al., 2009; Tao et al., 2016; Amini et al., 2017; Yan et al., 2019b; Zubair et al., 2020) (Table 2). Values at the upper end of this range indicate good potential to use membrane filtration as one step in recovering and concentrating phosphorus from digestate. Clogging or fouling of membrane pores have been considered a serious problem (Adam et al., 2018), which can vary between flow conditions, digestate characteristics and membrane types (Camilleri-Rumbau et al., 2021). Even though full-scale filtration facilities have been in operation this treatment method is considered costly (Vaneeckhaute et al., 2017). The phosphorus-rich retentate from these processes still has a low dry matter content and requires further treatment such as drying (Adam et al., 2018) or struvite precipitation (Pedizzi et al., 2018) to be manageable and transportable over long distances. Since soils in animal-dense regions generally already have a high phosphorus content (Kronvang et al., 2009; Senthilkumar et al., 2012), the prospect for using membrane filtration directly, without any further treatment, is limited.
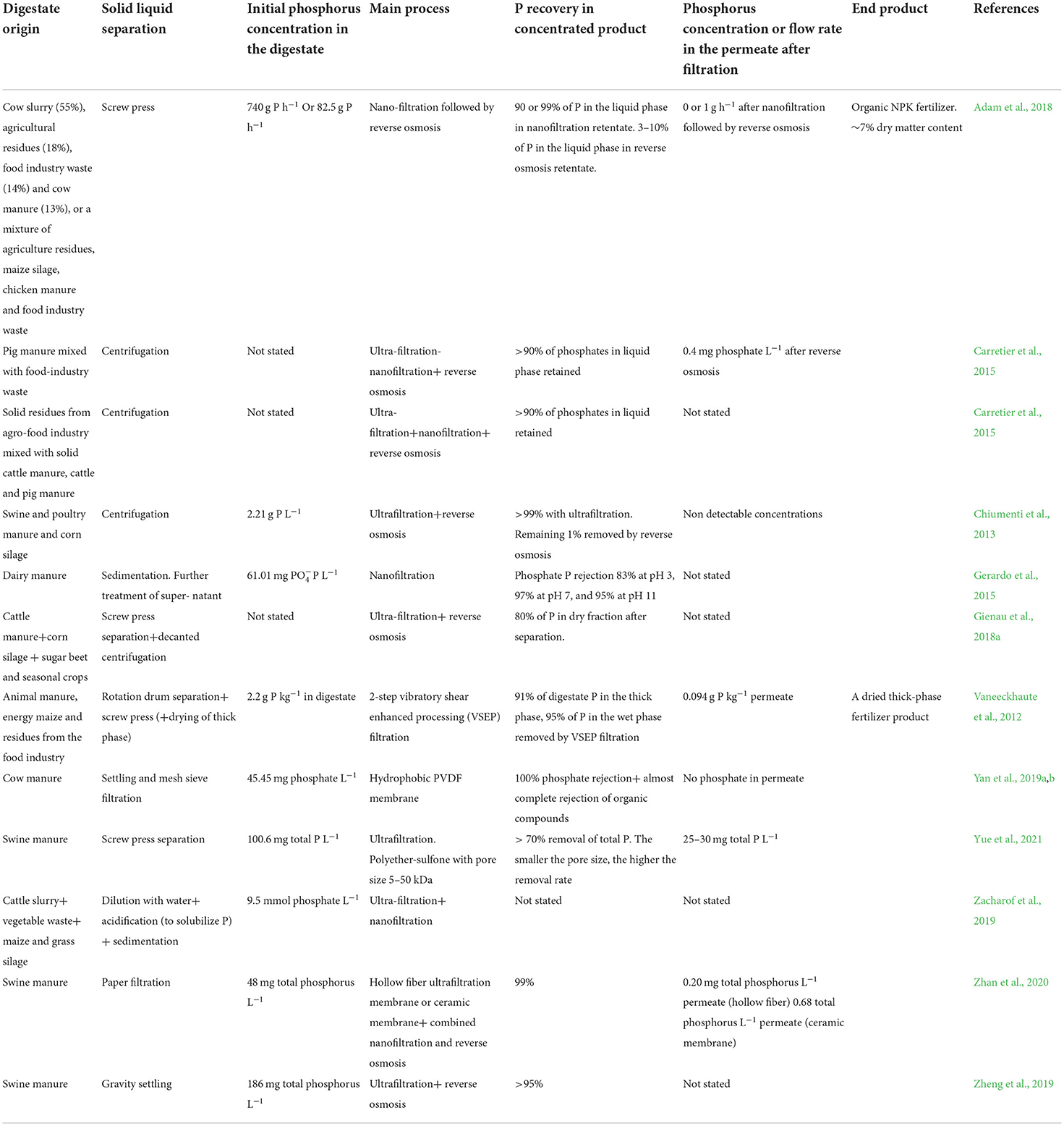
Table 2. Phosphorus (P) retention in experiments with membrane filtration of manure-based digestate.
Treatment of solid phase digestate
After solid/wet separation, solid phase digestate can be used as a plant fertilizer or soil amendment (Vaneeckhaute et al., 2012; Adam et al., 2018). However, this fraction is bulky and further treatment is needed to concentrate its phosphorus content before use in regions far away from its origin where there is a demand for phosphorus fertilizers. In HTC (Cao et al., 2019; Aragón-Briceño et al., 2021), organic compounds are, under contact with hot, pressurized water at a temperature between 180 and 250°C (Selvaraj et al., 2022) in an inert atmosphere, transformed by a series of hydrolysis, decarboxylation and dehydration reactions into charcoal, which mainly consists of the element carbon (Aragón-Briceño et al., 2021). There are also a wet phase and a gas phase output from the HCT process (Jouhara et al., 2018; Zhao et al., 2018; Selvaraj et al., 2022). Hydrochar, the solid phase output from HTC, is easy to handle and transport, especially after being pelleted (Mohammadi, 2021), compared with raw organic materials including digestate (Mohammadi et al., 2020). HTC can be applied to feedstocks with a high water content, preferably 15–25% dry matter (Lanza et al., 2018; Selvaraj et al., 2022). This range of dry matter content matches rather well that of the solid fraction of separated digestate. A few studies have investigated phosphorus retention in hydrocarbonized digestate. In one such study, by Zhao et al. (2018), more than 96.1% of phosphorus from dried and ground, solid phase filtration separated digestate based on a mixture of, 40% maize silage, 30% liquid cattle manure and 30% grass silage was recovered in the solid phase after HTC at 190°C during 3 h. HTC temperatures of 220 and 250°C and same reaction time resulted in a slightly lower phosphorus retention (Zhao et al., 2018). In another study, HTC of dried digestate based on 70% maize silage and 30% cow manure with a solids content of 20% after adding distilled water at 220°C during 185 min resulted in retention of about 90% of the phosphorus in the digestate in the solid phase hydrochar (Funke, 2015). However, phosphorus recovery can vary considerably depending on HTC processing settings, e.g., a maximum recovery of 63% phosphorus in the hydrochar was found at pH 8 and temperature 165°C and a processing time of 500 min in HTC experiments using either agricultural wastes or a mixture of food and agricultural wastes (Stutzenstein et al., 2018). Pyrolysis is also performed in an oxygen limited atmosphere (Bruun et al., 2017; Brassard et al., 2018) but at a higher temperature than HTC, typically between 300 and 1,000°C (Jouhara et al., 2018; Prurapark et al., 2020). Also pyrolysis includes a number degradation processes, which like HTC result in a solid phase, called biochar and mainly consisting of the element carbon, as well as a wet phase, called bio-oil, and gas phase (syngas) (Brassard et al., 2018). It is generally used for materials with a higher dry matter content than HTC (Ro et al., 2010), but it has been evaluated as a way to refine digestate (Cheng et al., 2020). For example, pyrolysis of dried pig manure-based digestate at 800°C has generated biochar with a phosphorus content of 9.27 weight% which meant more than a doubling of the phosphorus concentration in the dried digestate (Tsai et al., 2018). Composting of solid phase manure based digestate has also been tested to improve its characteristics. Ligno-cellulosic bulking agents can be added (Tambone et al., 2015), and the pH and moisture content can be adjusted to improve the composting process (Torres-Climent et al., 2015). Results from composting of non-manure based digestate indicate nature and origin of the digestate can have an impact on the composting effect of the P characteristics of the digestate (Grigatti et al., 2015) but it is difficult to extrapolate these results to manure-based digestate. Moreover, composted solid phase manure-based digestate has a water content within the same range as non-composted solid fraction digestate (Tambone et al., 2015), which makes it unsuitable for long-distance transport.
Refined digestate as phosphorus fertilizer
In this section, the prospects for using the outputs from digestate refinement processes, and analogous products or products that are otherwise comparable as phosphorus fertilizers, are compared with those of non-refined digestate. Together with the information presented in section Digestate refinement and phosphorus concentration, this comparison enabled a full evaluation of phosphorus retention efficiency, including extraction and agronomic performance from anaerobic digester outlet to plant uptake.
Use of refined digestate wet-phase products as plant fertilizer
Struvite is a widely studied phosphorus fertilizer that is easy to handle compared with organic fertilizers and has a nutrient content matching that of many commercial mineral fertilizers (Huygens and Saveyn, 2018). A few studies have compared the efficacy of struvite as a phosphorus fertilizer with that of organic fertilizers, including unprocessed digestate. Vaneeckhaute et al. (2016) observed only non-significant differences in phosphorus uptake and dry weight biomass between maize plants fertilized with 1) unseparated digestate processed from a mixture of maize and energy crops, animal manure, and industrial food waste and 2) struvite in a soil with high and a soil with low phosphorus status. In a greenhouse experiment with Westerwolds ryegrass (Lolium multiflorum L. subsp. Westerwoldicum) on phosphorus-deficient sandy loam soil, Vanden Nest et al. (2021) found that phosphorus exports in biomass of plants fertilized with a group of unseparated, wet, solid, composted solid, and dried digestates, based on animal manure, plant wastes and energy crop material was 86% of, and that the phosphorus exports in plants fertilized with struvite were similar to the phosphorus exports in plants fertilized with triple super phosphate (TSP). In a pot experiment with rye (Secale cereale L.), (Oliveira et al., 2019) found that fertilization with struvite resulted in an even higher uptake than in plants fertilized with single superphosphate, even though the higher phosphorus uptake did not result in a larger shoot biomass. The same type of struvite also resulted in a higher content of Olsen phosphorus than single superphosphate in an incubation experiment (Oliveira et al., 2019). Struvite has also been proven to be as good a fertilizer as TSP in pot experiments with maize at pH 4.6 and 6.6 (Cabeza et al., 2011), and as efficient as monocalcium phosphate for perennial ryegrass (Lolium perenne L.) on a sandy soil (Johnston and Richards, 2003) (Table 3). Incubation studies on agricultural soil found no significant differences in percentage of total phosphorus released as orthophosphate between treatments with manure-based digestates and struvite (Case and Jensen, 2019). Together, the results from these studies indicate equal or better phosphorus acquisition efficiency for struvite than for unprocessed digestate.
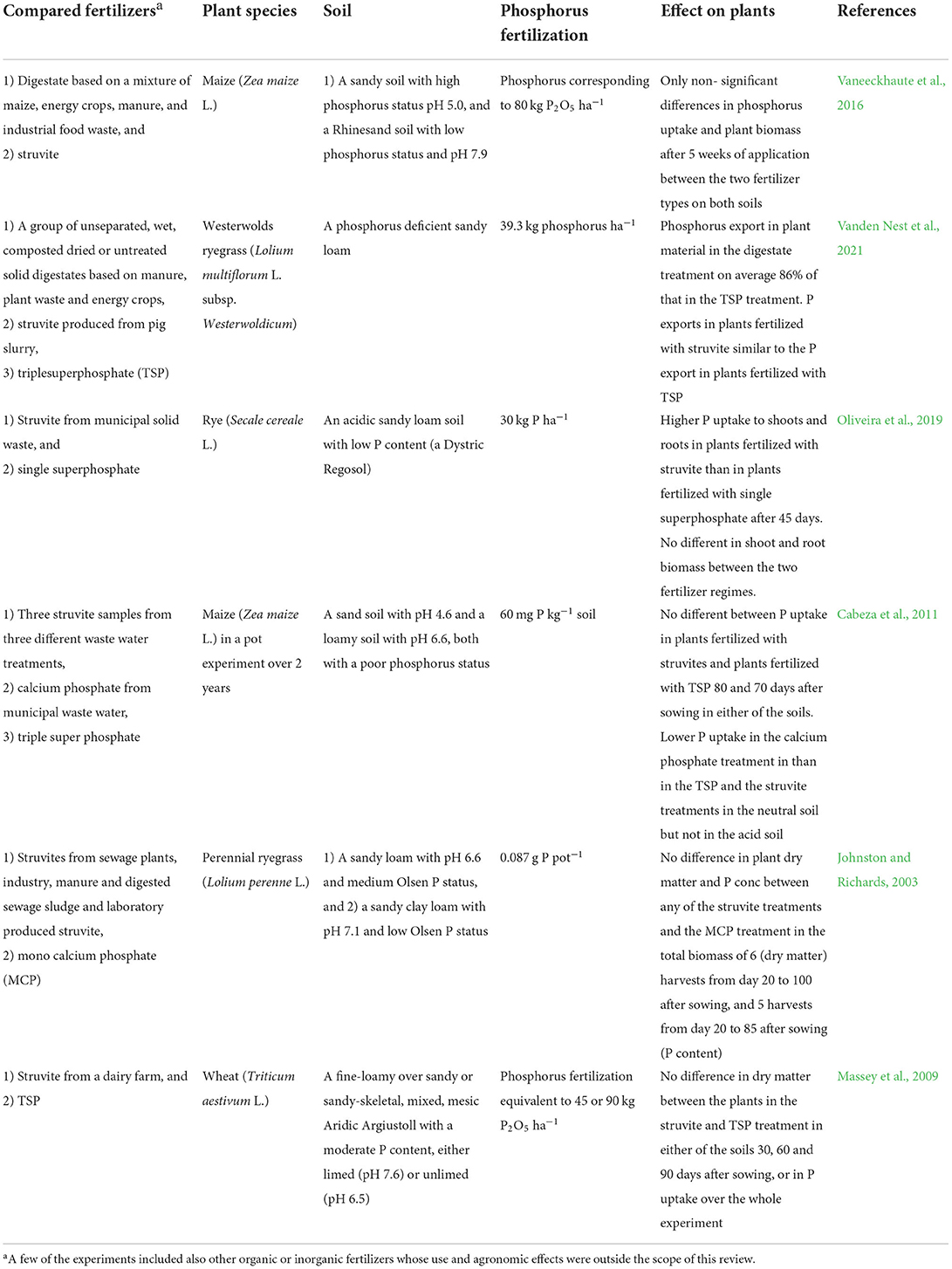
Table 3. Agronomic effects of refined wet phase digestate phosphorus fertilizers on plants compared with those from non-refined digestate and traditional mineral phosphorus fertilizers.
Other studies have shown that struvite and TSP have similar solubility in soils ranging from acid to neutral (Kataki et al., 2016) and that the effects of struvite on phosphorus uptake and crop yield are similar to, or better than, those of TSP and other common mineral phosphorus fertilizers for a broad range of agricultural and horticultural crops (Li et al., 2019). In a meta-analysis, Huygens and Saveyn (2018) found similar phosphorus acquisition efficiency and dry matter yield effects for struvite, other precipitated salts, and mined and synthetic phosphorus fertilizers across conditions representing agricultural production in Europe. However, the efficiency of struvite as a plant fertilizer is reported to be low under high soil pH conditions, due to its low solubility (Kataki et al., 2016), although Massey et al. (2009) found struvite to be an efficient fertilizer in a limed soil with pH as high as 7.6. Moreover, the high magnesium content in struvite sometimes causes magnesium accumulation in soil, affecting plant calcium uptake, soil hydraulic conductivity, and aggregate stability (Kataki et al., 2016).
Calcium phosphate is reported to be an efficient fertilizer only on acid soils and plant phosphorus uptake from this source is often lower than from struvite or synthetic mineral fertilizers (Cabeza et al., 2011; Tarayre et al., 2016), which arguably limits its usefulness as a fertilizer compared with struvite. For raw digestate, more positive effects on organic matter and microbiology have been reported for soil with neutral pH than for alkaline soil (Panuccio et al., 2021), but no studies examining direct effects of soil pH on plant phosphorus uptake after application of unprocessed digestate were found. Sorption experiments show poor soil retention capacity for phosphorus in anaerobically digested slurry and abattoir waste compared with phosphorus in other organic materials, including sewage sludge and compost (Stutter, 2015), indicating a higher risk of phosphorus leaching from digestate.
The physical and chemical characteristics of struvite can affect its usefulness as a plant fertilizer. The crystal size of struvite varies with pH, flow velocity, and supersaturation during precipitation, potentially generating trade-offs between handling efficiency and plant fertilizer uptake. Smaller crystal size favors nutrient release under certain conditions, due to faster dissolution, but requires higher crushing strength, whereas larger crystal size facilitates efficient handling during production, transportation, and application (Kataki et al., 2016). However, crystal size and crushing strength have not been the focus of most studies on struvite precipitation from digestate. The purity of struvite can fluctuate widely, depending on the origin, and the precipitation process can affect usefulness of struvite as a plant fertilizer. A study comparing purity showed a relatively low content of most detectable heavy metals in struvite precipitated from digestate compared with the levels in struvite from other sources (Kataki et al., 2016). However, these results should be interpreted with caution, because only one of the struvite samples was precipitated from digestate and analysis methods may have differed between studies. Moreover, unprocessed digestate based on manure may contain substantial amounts of heavy metals (Li et al., 2018; Tang et al., 2020). Therefore, methods that can separate phosphate ions from metal ions such as electrodialytic processes (Oliveira et al., 2020) could possibly be of particular interest to apply prior to precipitation of struvite from manure-based digestate in order to avoid plant uptake of heavy metals.
Products containing phosphorus, including retentate from filtration methods, can be categorized as organic fertilizers (Adam et al., 2018). The lower nitrogen/phosphorus ratio in filtration retentates in comparison with digestate indicates greater potential for the former as phosphorus fertilizers. However few, if any, experimental studies investigating the use of filtration retentate as phosphorus fertilizer have been published. Although the phosphorus content in retentate is higher than in unprocessed liquid digestate, the total phosphorus content is much lower than in struvite (Kern et al., 2008) and many other mineral phosphorus fertilizers. Therefore, high transportation costs and storage space requirements are likely, meaning that retentate which has not undergone any further treatment is mainly a viable alternative for local use and distribution near the processing facility.
Use of solid phase digestate products as phosphorus-rich plant fertilizer
The phosphorus that ends up in the solid phase after solid wet separation, which typically represents around one-third of total digestate phosphorus (Table 1), is not available for precipitation of struvite or other phosphate salts, or suitable for membrane filtration. To achieve high retention of phosphorus in the food production chain, it is important that this fraction is taken up by crops. In a crop rotation involving forage maize, potatoes (Solanum tuberosum L.), fodder beet (Beta vulgaris L.), and oats (Avena sativa L.), Vanden Nest et al. (2015) found that application of solid phase digestate based on a mixture of organic waste, energy crops, and animal slurry increased soil calcium chloride-extractable phosphorus (P-CaCl2) concentration and phosphorus leaching but not soil ammonium lactate-extractable phosphorus (P-AL) concentration, hot water-extractable phosphorus, or crop yield or quality compared with application of equal, higher, or lower doses of TSP to a soil with initially high phosphorus status. Bach et al. (2022) found similar or higher maize biomass and biomass phosphorus content in treatments that received phosphorus as struvite, calcium phosphate, and magnesium phosphate precipitated from cow manure and maize silage-based digestate in combination with dried solid phase of the same types of digestate, than in treatments that received phosphorus as TSP on two soils with low phosphorus status. Ehmann et al. (2019) found that steam dried solid digestate from pig manure resulted in higher sunflower (Helianthus annuus L.) shoot and flower biomass than a mixture and calcium phosphates and struvite precipitated from the same digestate type. However, Pantelopoulos et al. (2017) found that thermal drying of solid phase digestate decreased phosphorus uptake in ryegrass plants compared with non-dried solid digestate. In another study, acidification of the solid fraction after screw press separation of digestate increased the water-extractable phosphorus content and also plant phosphorus uptake, but not plant biomass, in maize (Zea mays L.) compared with non-acidified solid digestate (Regueiro et al., 2020) (Table 4).
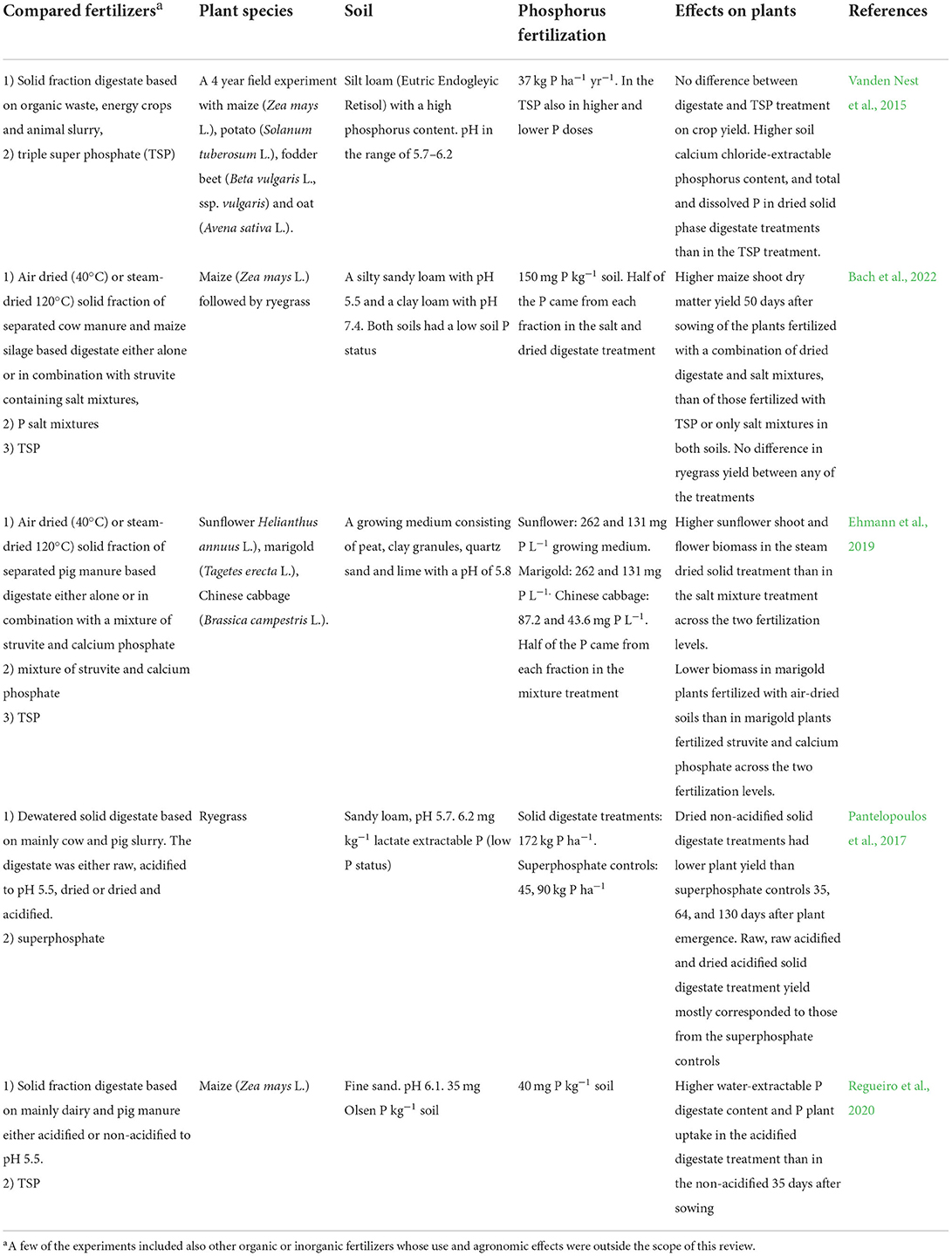
Table 4. Agronomic effects of refined solid phase digestate phosphorus fertilizers on plants compared with those from mineral phosphorus fertilizers.
Another way of increasing phosphorus retention in food production chains could be to use the aqueous phase remaining after HTC of the solid phase of digestate and to perform a second solid wet separation after HTC (Belete et al., 2021). However, the aqueous phase can contain growth-inhibiting substances (Belete et al., 2021). In a hydroponic experiment on maize, the aqueous phase from HTC-processed cow manure digestate was found to be toxic at low dilution rates or to have low fertilizer value at high dilution rates (Celletti et al., 2021). de Jager and Giani (2021) found an increase in soil phosphate after application of hydrochar from digestate based on a mixture of animal manure and plant material to three diverse soils, and observed no negative effects on seed germination of Chinese cabbage (Brassica rapa L. subsp. pekinensis) or positive effects on plant growth. Moreover, it has been shown that high levels of heavy metals in biochars generated by pyrolysis of manure-based digestate can be reduced by citric acid leaching (Zuo et al., 2020).
There is limited information of the effect of composted digestate on plant P availability. For non-manure based digestates, Grigatti et al. (2015) found that the composting effect of phosphorus availability varied with the origin of the digestate. As for composted pig-manure based digestate, there were only limited effects on general digestate characteristics (Tambone et al., 2015) indicating that composting may not be a very useful treatment of manure based digestate.
Solid fractions of separated digestate are widely regarded as soil amendments with potential positive effects on soil organic matter content (Egene et al., 2021) and heavy metal stabilization (Van Poucke et al., 2020), with indirect and long-term positive effects on crop productivity in addition to directly providing plant nutrients. Other benefits include a positive effect of dairy manure-based digestate on plant growth-promoting bacteria with anti-fungal activities and phytohormone production (Qi et al., 2018). However, such effects are difficult to account for in estimates of phosphorus retention capacity in agricultural food production chains. Besides, Coelho et al. (2019) showed low on no direct effect of different liquid digestates, including digestate based partly on pig slurry, on soil microbial gene copy number in a greenhouse experiment with perennial ryegrass (Lolium perenne L.) and white clover (Trifolium repens L.) monocultures and mixtures. Similarly, studies using digestate from non-manure feedstocks show a lack of effect on soil microbial biomass (Andruschkewitsch et al., 2013). Also the potential to spread pathogenic microorganisms through solid phase digestate could be mentioned even though anaerobic digestion generally reduce the pathogen level considerably (Chiapetta et al., 2019). In many cases the solid phase of separated manure-based digestate had a lower concentration of pathogenic bacteria than the unseparated digestate but also increased concentrations of pathogenic bacteria in the solid phase have been reported (Cathcart et al., 2022). Pelleting (Cathcart et al., 2022) or composting (Chiapetta et al., 2019) could reduce the pathogen levels further, at least for some species.
Synthesis and research outlook
Multiple studies report high extraction rate (up to 100%) of phosphorus as struvite or calcium phosphate from separated liquid digestate (Table 1). Membrane filtration studies also report high potential to concentrate phosphorus from manure-based digestate. However, the latter does not provide a solid phosphorus fertilizer that is easily manageable and transportable, and hence is less relevant for systems where the phosphorus needs to be transported out of a production region with high animal densities. Besides, this refinement method is sometimes associated with technical hindrances such as membrane fouling and is also considered costly. Only around 66% of total digestate phosphorus is available for struvite precipitation after solid wet separation of digestate that has not undergone any phosphorus solubilization treatment. Agronomic studies show similar or only slightly better phosphorus uptake and biomass production after struvite fertilization than after fertilization with unprocessed digestate. Agronomic and horticultural experiments do not indicate that the ability to take up phosphorous from soils, and produce plant biomass per amount of phosphorus taken up, is substantially higher after application of from solid phase digestate or products processed from this phase than after application of non-refined digestate. Neither are there any studies reporting higher phosphorus acquisition or use efficiency after application of output products from membrane filtration processes than after application of non-refined digestate. Overall, this means that solid/wet separation followed by precipitation of struvite or calcium phosphates or by membrane filtration does not in itself substantially increase the proportion of digestate phosphorus that can be recycled back into the food production chain through crop uptake. However, even if the phosphorus acquisition and use efficiency of crop fertilized with struvite and calcium phosphates are the same as for crops fertilized with unprocessed digestate the chemical homogeneity of the former products could facilitate the planning of the phosphorus fertilization at least compared to systems where there is a risk of runoff and leaching of phosphorus during the storage of organic fertilizers, or when there is limited access to chemical analyses of organic materials. There are also other reasons why research results to date do not necessarily imply that digestate refinement processes are irrelevant to phosphorus recycling in agricultural systems, but rather reveal uncertainties and research gaps that need further attention. First, far from all studies show a struvite extraction ratio of digestate phosphorus close to 100%. Increasing the proportion of total digestate phosphorus by solubilization of particle-bound phosphorus could increase the total extractable amount considerably. Second, given that struvite is relatively easy to transport over long distances out of animal-dense regions, whereas non-refined digestate is not, comparison of these two types of fertilizers on similar soils is often of limited practical relevance. Third, there are already several commercial facilities, which precipitate struvite from digestate in animal dense regions (Muys et al., 2021), indicating a possibility to further upscale this production.
These uncertainties and limitations indicate a need for more tailored studies on the effects of different phosphorus-rich fertilizers in agriculture. Better knowledge is needed about the phosphorus recycling capacity of systems where digestate phosphorus is exported out of animal-dense regions. This will require determination of the effects of low-phosphorus solid phase digestate on phosphorus acquisition by plants growing on soils with high phosphorus content. High carbon/nitrogen ratio or lignin/nitrogen ratio in organic materials used as soil amendments, including plant residues, is reported to be negatively correlated with soil nitrogen mineralization (Kaleeem Abbasi et al., 2015), suggesting that application of large amounts of phosphorus-poor solid phase digestate, which also has a low nitrogen content, could have similar effects. Therefore, good knowledge of the specific effects of fertilization with low-nutrient solid phase digestate on soils and crops, and of the underlying microbiological mechanisms causing or resulting from these effects, would probably also be beneficial to understanding the prospects of struvite precipitation in manure and phosphorus handling chains. Increased solubilization of phosphorus by acidification or microwave treatment, decreasing the phosphorus content in the solid phase, would further enhance the relevance of such studies. Moreover, processing methods to reduce impurities such as heavy metal contamination, as well as the consequences of large variations in struvite crystal size and chemical purity, and contamination, on phosphorus recycling efficiency and soil accumulation and plant uptake of hazardous compounds need to be investigated further. Thorough agronomic comparisons under conditions that represent regions and soils with high phosphorus demand should be part of such investigations. Feedback from such studies could be used to tailor digestate refinement processes to agronomic demands and to combine the capacity and efficiency of different digestate refinement processes, in particular struvite precipitation, to achieve high phosphorus retention in agronomic production chains. Integrated analyses of digestate refinement and agronomic performance of refined fertilizers would also facilitate further investigations of other aspects of these chains than phosphorus retention, such as life cycle assessments of environmental loads including global warming potential and eutrophication, or economic cost analyses.
Author contributions
Both authors have made a substantial, direct, and intellectual contribution to the work and approved it for publication.
Funding
This study was conducted within the project Manure, fish slurry and digestate: from problem to farmers gold (MAFIGOLD) funded by the Norwegian Research Council (project number 294625).
Conflict of interest
The authors declare that the research was conducted in the absence of any commercial or financial relationships that could be construed as a potential conflict of interest.
Publisher's note
All claims expressed in this article are solely those of the authors and do not necessarily represent those of their affiliated organizations, or those of the publisher, the editors and the reviewers. Any product that may be evaluated in this article, or claim that may be made by its manufacturer, is not guaranteed or endorsed by the publisher.
References
Adam, G., Mottet, A., Lemaigre, S., Tsachidou, B., Trouvé, E., and Delfosse, P. (2018). Fractionation of anaerobic digestates by dynamic nanofiltration and reverse osmosis: an industrial pilot case evaluation for nutrient recovery. J. Environ. Chem. Eng. 6, 6723–6732. doi: 10.1016/j.jece.2018.10.033
Amini, A., Aponte-Morales, V., Wang, M., Dilbeck, M., Lahav, O., Zhang, Q., et al. (2017). Cost-effective treatment of swine wastes through recovery of energy and nutrients. Waste Manag. 69, 508–517. doi: 10.1016/j.wasman.2017.08.041
Andruschkewitsch, M., Wachendorf, C., and Wachendorf, M. (2013). Effects of digestates from different biogas production systems on above and belowground grass growth and the nitrogen status of the plant-soil-system. Grassl. Sci. 59, 183–195. doi: 10.1111/grs.12028
Aragón-Briceño, C. I., Pozarlik, A. K., Bramer, E. A., Niedzwiecki, L., Pawlak-Kruczek, H., and Brem, G. (2021). Hydrothermal carbonization of wet biomass from nitrogen and phosphorus approach: a review. Renew. Energy 171, 401–415. doi: 10.1016/j.renene.2021.02.109
Aydin, I., Aydin, F., Saydut, A., Bakirdere, E. G., and Hamamci, C. (2010). Hazardous metal geochemistry of sedimentary phosphate rock used for fertilizer (Mazidag, SE Anatolia, Turkey). Microchem. J. 96, 247–251. doi: 10.1016/j.microc.2010.03.006
Bach, I. M., Essich, L., Bauerle, A., and Muller, T. (2022). Efficiency of phosphorus fertilizers derived from recycled biogas digestate as applied to maize and ryegrass in soils with different pH. Agriculture 12, 325. doi: 10.3390/agriculture12030325
Barampouti, E., Mai, S., Malamis, D., Moustakas, K., and Loizidou, M. (2020). Exploring technological alternatives of nutrient recovery from digestate as a secondary resource. Renew. Sustain. Energy Rev. 134, 110379. doi: 10.1016/j.rser.2020.110379
Belete, Y. Z., Mau, V., Spitzer, R. Y., Posmanik, R., Jassby, D., Iddya, A., et al. (2021). Hydrothermal carbonization of anaerobic digestate and manure from a dairy farm on energy recovery and the fate of nutrients. Bioresour. Technol. 333, 125164. doi: 10.1016/j.biortech.2021.125164
Brassard, P., Godbout, S., Pelletier, F., Raghavan, V., and Palacios, J. H. (2018). Pyrolysis of switchgrass in an auger reactor for biochar production: a greenhouse gas and energy impacts assessment. Biomass Bioenergy 116, 99–105. doi: 10.1016/j.biombioe.2018.06.007
Bruun, S., Harmer, S. L., Bekiaris, G., Christel, W., Zuin, L., Hu, Y., et al. (2017). The effect of different pyrolysis temperatures on the speciation and availability in soil of P in biochar produced from the solid fraction of manure. Chemosphere 169, 377–386. doi: 10.1016/j.chemosphere.2016.11.058
Cabeza, R., Steingrobe, B., Romer, W., and Claassen, N. (2011). Effectiveness of recycled P products as P fertilizers, as evaluated in pot experiments. Nutr. Cycling Agroecosyst. 91, 173–184. doi: 10.1007/s10705-011-9454-0
Camilleri-Rumbau, M. S., Briceño, K., Fjerbæk Søtoft, L., Christensen, K. V., Roda-Serrat, M. C., Errico, M., et al. (2021). Treatment of manure and digestate liquid fractions using membranes: opportunities and challenges. Int. J. Env. Res. Pub. He. 18, 3107. doi: 10.3390/ijerph18063107
Campos, P., Borie, F., Cornejo, P., López-Ráez, J. A., López-García, Á., and Seguel, A. (2018). Phosphorus acquisition efficiency related to root traits: is mycorrhizal symbiosis a key factor to wheat and barley cropping? Front. Plant Sci. 9, 752. doi: 10.3389/fpls.2018.00752
Candido, D., Bolsan, A. C., Hollas, C. E., Venturin, B., Tapparo, D. C., Bonassa, G., et al. (2022). Integration of swine manure anaerobic digestion and digestate nutrients removal/recovery under a circular economy concept. J. Environ. Manage. 301, 113825. doi: 10.1016/j.jenvman.2021.113825
Cao, Z. B., Jung, D., Olszewski, M. P., Arauzo, P. J., and Kruse, A. (2019). Hydrothermal carbonization of biogas digestate: effect of digestate origin and process conditions. Waste Manag. 100, 138–150. doi: 10.1016/j.wasman.2019.09.009
Carretier, S., Lesage, G., Grasmick, A., and Heran, M. (2015). Water and nutrients recovering from livestock manure by membrane processes. Can. J. Chem. Eng. 93, 225–233. doi: 10.1002/cjce.22125
Case, S. D. C., and Jensen, L. S. (2019). Nitrogen and phosphorus release from organic wastes and suitability as bio-based fertilizers in a circular economy. Environ. Technol. 40, 701–715. doi: 10.1080/09593330.2017.1404136
Cathcart, A., Smyth, B. M., Forbes, C., Lyons, G., Murray, S. T., Rooney, D., et al. (2022). Effect of anaerobic digestate fuel pellet production on Enterobacteriaceae and Salmonella persistence. Glob. Change Biol. Bioenergy 14, 1055–1064. doi: 10.1111/gcbb.12986
Celletti, S., Lanz, M., Bergamo, A., Benedetti, V., Basso, D., Baratieri, M., et al. (2021). Evaluating the aqueous phase from hydrothermal carbonization of cow manure digestate as possible fertilizer solution for plant growth. Front. Plant Sci. 12, 687434. doi: 10.3389/fpls.2021.687434
Cheng, F., Bayat, H., Jena, U., and Brewer, C. E. (2020). Impact of feedstock composition on pyrolysis of low-cost, protein- and lignin-rich biomass: a review. J. Anal. Appl. Pyrolysis 147, 104780. doi: 10.1016/j.jaap.2020.104780
Chiapetta, H., Harrison, J., Gay, J., McClanahan, R., Whitefield, E., Evermann, J., et al. (2019). Reduction of pathogens in bovine manure in three full-scale commercial anaerobic digesters. Water Air Soil Pollut. 230, 111. doi: 10.1007/s11270-019-4163-4
Chiumenti, A., da Borso, F., Teri, F., Chiumenti, R., and Piaia, B. (2013). Full-scale membrane filtration system for the treatment of digestate from a co-digestion plant. Appl. Eng. Agric. 29, 985–990. doi: 10.13031/aea.29.10117
Chowdhury, R. B., Moore, G. A., Weatherley, A. J., and Arora, M. (2017). Key sustainability challenges for the global phosphorus resource, their implications for global food security, and options for mitigation. J. Clean. Prod. 140, 945–963. doi: 10.1016/j.jclepro.2016.07.012
Coelho, J. J., Hennessy, A., Casey, I, Woodcock, T., and Kennedy, N. (2019). Responses of ryegrass, white clover, soil plant primary macronutrients and microbial abundance to application of anaerobic digestates, cattle slurry and inorganic N-fertiliser. Appl. Soil. Ecol. 144, 112–122. doi: 10.1016/j.apsoil.2019.07.011
Corona, F., Hidalgo, D., Martin-Marroquin, J. M., and Meers, E. (2021). Study of pig manure digestate pre-treatment for subsequent valorisation by struvite. Environ. Sci. Pollut. Res. 28, 24731–24743. doi: 10.1007/s11356-020-10918-6
Daumer, M. L., Picard, S., Saint-Cast, P., and Dabert, P. (2010). Technical and economical assessment of formic acid to recycle phosphorus from pig slurry by a combined acidification-precipitation process. J. Hazard. Mater. 180, 361–365. doi: 10.1016/j.jhazmat.2010.04.039
de Jager, M., and Giani, L. (2021). An investigation of the effects of hydrochar application rate on soil amelioration and plant growth in three diverse soils. Biochar 3, 349–365. doi: 10.1007/s42773-021-00089-z
Egene, C. E., Sigurnjak, I., Regelink, I. C., Schoumans, O. F., Adani, F., Michels, E., et al. (2021). Solid fraction of separated digestate as soil improver: implications for soil fertility and carbon sequestration. J. Soil Sediments 21, 678–688. doi: 10.1007/s11368-020-02792-z
Ehmann, A., Bach, I. M., Bilbao, J., Lewandowski, I., and Muller, T. (2019). Phosphates recycled from semi-liquid manure and digestate are suitable alternative fertilizers for ornamentals. Sci. Hortic. 243, 440–450. doi: 10.1016/j.scienta.2018.08.052
Estevez, M., Linjordet, R., Horn, S., and Morken, J. (2014). Improving nutrient fixation and dry matter content of an ammonium-rich anaerobic digestion effluent by struvite formation and clay adsorption. Water. Sci. Technol. 70, 337–344. doi: 10.2166/wst.2014.236
Funke, A. (2015). Fate of plant available nutrients during hydrothermal carbonization of digestate. Chem. Ing. Tech. 87, 1713–1719. doi: 10.1002/cite.201400182
Garbs, M., and Geldermann, J. (2018). Analysis of selected economic and environmental impacts of long distance manure transports to biogas plants. Biomass Bioenergy 109, 71–84. doi: 10.1016/j.biombioe.2017.12.009
Gerardo, M. L., Aljohani, N. H. M., Oatley-Radcliffe, D. L., and Lovitt, R. W. (2015). Moving towards sustainable resources: recovery and fractionation of nutrients from dairy manure digestate using membranes. Water Res. 80, 80–89. doi: 10.1016/j.watres.2015.05.016
Gienau, T., Bruss, U., Kraume, M., and Rosenberger, S. (2018a). Nutrient recovery from anaerobic sludge by membrane filtration: pilot tests at a 2.5 MWe biogas plant. Int. J. Recycl. Org. Waste Agric. 7, 325–334. doi: 10.1007/s40093-018-0218-6
Gienau, T., Bruss, U., Kraume, M., and Rosenberger, S. (2018b). Nutrient recovery from biogas digestate by optimised membrane treatment. Waste Biomass Valori. 9, 2337–2347. doi: 10.1007/s12649-018-0231-z
Grigatti, M., Boanini, E., Cavani, L., Ciavatta, C., and Marzadori, C. (2015). Phosphorus in digestate-based compost: chemical speciation and plant-availability. Waste Biomass Valori. 6, 481–493. doi: 10.1007/s12649-015-9383-2
Guilayn, F., Jimenez, J., Rouez, M., Crest, M., and Patureau, D. (2019). Digestate mechanical separation: efficiency profiles based on anaerobic digestion feedstock and equipment choice. Bioresour. Technol. 274, 180–189. doi: 10.1016/j.biortech.2018.11.090
Herbes, C., Roth, U., Wulf, S., and Dahlin, J. (2020). Economic assessment of different biogas digestate processing technologies: a scenario-based analysis. J. Clean. Prod. 255, 120282. doi: 10.1016/j.jclepro.2020.120282
Hertzberger, A. J., Cusick, R. D., and Margenot, A. J. (2020). A review and meta-analysis of the agricultural potential of struvite as a phosphorus fertilizer. Soil Sci. Soc. Am. J. 84, 653–671. doi: 10.1002/saj2.20065
Hidalgo, D., Martin-Marroquin, J. M., and Corona, F. (2019). A multi-waste management concept as a basis towards a circular economy model. Renew. Sustain. Energy Rev. 111, 481–489. doi: 10.1016/j.rser.2019.05.048
Huygens, D., and Saveyn, H. G. M. (2018). Agronomic efficiency of selected phosphorus fertilisers derived from secondary raw materials for European agriculture. A meta-analysis. Agron. Sustain. Dev. 38, 52. doi: 10.1007/s13593-018-0527-1
Johnston, A., and Richards, I. (2003). Effectiveness of different precipitated phosphates as phosphorus sources for plants. Soil Use Manag. 19, 45–49. doi: 10.1111/j.1475-2743.2003.tb00278.x
Jouhara, H., Ahmad, D., van den Boogaert, I., Katsou, E., Simons, S., and Spencer, N. (2018). Pyrolysis of domestic based feedstock at temperatures up to 300 °C. Therm. Sci. Eng. Prog. 5, 117–143. doi: 10.1016/j.tsep.2017.11.007
Kaleeem Abbasi, M., Mahmood Tahir, M., Sabir, N., and Khurshid, M. (2015). Impact of the addition of different plant residues on nitrogen mineralization–immobilization turnover and carbon content of a soil incubated under laboratory conditions. Solid Earth 6, 197–205. doi: 10.5194/se-6-197-2015, 2015
Kataki, S., West, H., Clarke, M., and Baruah, D. C. (2016). Phosphorus recovery as struvite: recent concerns for use of seed, alternative Mg source, nitrogen conservation and fertilizer potential. Resour. Conserv. Recycl. 107, 142–156. doi: 10.1016/j.resconrec.2015.12.009
Kern, J., Heinzmann, B., Markus, B., Kaufmann, A. C., Soethe, N., and Engels, C. (2008). Recycling and assessment of struvite phosphorus from sewage sludge. Agric. Eng. Int. CIGR J. X CE 12, 1.
Kleinman, P. J. A., Sharpley, A. N., Withers, P. J. A., Bergström, L., Johnson, L. T, and Doody, D. G. (2015). Implementing agricultural phosphorus science and management to combat eutrophication. Ambio 44, 297–310. doi: 10.1007/s13280-015-0631-2
Kronvang, B., Rubæk, G. H., and Heckrath, G. (2009). International phosphorus workshop: diffuse phosphorus loss to surface water bodies–risk assessment, mitigation options, and ecological effects in river basins. J. Environ. Qual. 38, 1924–1929. doi: 10.2134/jeq2009.0051
Lanza, G., Stang, A., Kern, J., Wirth, S., and Gessler, A. (2018). Degradability of raw and post-processed chars in a two-year field experiment. Sci. Total Environ. 628–629, 1600–1608. doi: 10.1016/j.scitotenv.2018.02.164
Le Corre, K. S., Valsami-Jones, E., Hobbs, P., and Parsons, S. A. (2009). Phosphorus recovery from wastewater by struvite crystallization: a review. Crit. Rev. Env. Sci. Tec. 39, 433–477. doi: 10.1080/10643380701640573
Li, B., Boiarkina, I., Young, B., and Yu, W. (2016). Quantification and mitigation of the negative impact of calcium on struvite purity. Adv. Powder Technol. 27, 2354–2362. doi: 10.1016/j.apt.2016.10.003
Li, B., Boiarkina, I., Yu, W., Huang, H. M., Munir, T., Wang, G. Q., et al. (2019). Phosphorous recovery through struvite crystallization: challenges for future design. Sci. Total Environ. 648, 1244–1256. doi: 10.1016/j.scitotenv.2018.07.166
Li, Y., Liu, H., Li, G. X., Luo, W. H., and Sun, Y. (2018). Manure digestate storage under different conditions: chemical characteristics and contaminant residuals. Sci. Total Environ. 639, 19–25. doi: 10.1016/j.scitotenv.2018.05.128
MacDonald, G. K., Bennett, E. M., Potter, P. A., and Ramankutty, N. (2011). Agronomic phosphorus imbalances across the world's croplands. Proc. Natl. Acad. Sci. U.S.A. 108, 3086–3091. doi: 10.1073/pnas.101080810
Massey, M. S., Davis, J. G., Ippolito, J. A., and Sheffield, R. E. (2009). Effectiveness of recovered magnesium phosphates as fertilizers in neutral and slightly alkaline soils. Agron. J. 101, 323–329. doi: 10.2134/agronj2008.0144
Melgaco, L., Robles-Aguilar, A., Meers, E., and Mota, C. (2021). Phosphorus recovery from liquid digestate by chemical precipitation using low-cost ion sources. J. Chem. Technol. Biotechnol. 96, 2891–2900. doi: 10.1002/jctb.6842
Mohammadi, A. (2021). Overview of the benefits and challenges associated with pelletizing biochar. Processes 9, 1591. doi: 10.3390/pr9091591
Mohammadi, A., Venkatesh, G., Sandberg, M., Eskandari, S., Joseph, S., and Granstrom, K. (2020). A comprehensive environmental life cycle assessment of the use of hydrochar pellets in combined heat and power plants. Sustainability 12, 9026. doi: 10.3390/su12219026
Möller, K. (2015). Effects of anaerobic digestion on soil carbon and nitrogen turnover, N emissions, and soil biological activity. A review. Agron. Sustain. Dev. 35, 1021–1041. doi: 10.1007/s13593-015-0284-3
Möller, K., and Müller, T. (2012). Effects of anaerobic digestion on digestate nutrient availability and crop growth: a review. Eng. Life Sci. 12, 242–257. doi: 10.1002/elsc.201100085
Muhmood, A., Lu, J. X., Kadam, R., Dong, R. J., Guo, J. B., and Wu, S. B. (2019). Biochar seeding promotes struvite formation, but accelerates heavy metal accumulation. Sci. Total Environ. 652, 623–632. doi: 10.1016/j.scitotenv.2018.10.302
Muys, M., Phukan, R., Brader, G., Samad, A., Moretti, M., Haiden, B., et al. (2021). A systematic comparison of commercially produced struvite: quantities, qualities and soil-maize phosphorus availability. Sci. Total Environ. 756, 143726. doi: 10.1016/j.scitotenv.2020.143726
Nedelciu, C. E., Ragnarsdottir, K. V., Schlyter, P., and Stjernquist, I. (2020). Global phosphorus supply chain dynamics: assessing regional impact to 2050. Glob. Food Sec. 26, 100426. doi: 10.1016/j.gfs.2020.100426
Nesme, T., Senthilkumar, K., Mollier, A., and Pellerin, S. (2015). Effects of crop and livestock segregation on phosphorus resource use: a systematic, regional analysis. Eur. J. Agron. 71, 88–95. doi: 10.1016/j.eja.2015.08.001
Nkoa, R. (2014). Agricultural benefits and environmental risks of soil fertilization with anaerobic digestates: a review. Agron. Sustain. Dev. 34, 473–492. doi: 10.1007/s13593-013-0196-z
Oliveira, V., Dias-Ferreira, C., Gonzalez-Garcia, I., Labrincha, J., Horta, C., and Garcia-Gonzalez, M. C. (2021). A novel approach for nutrients recovery from municipal waste as biofertilizers by combining electrodialytic and gas permeable membrane technologies. Waste Manage. 125, 293–302. doi: 10.1016/j.wasman.2021.02.055
Oliveira, V., Dias-Ferreira, C., Labrincha, J., Rocha, J. L., and Kirkelund, G. M. (2020). Testing new strategies to improve the recovery of phosphorus from anaerobically digested organic fraction of municipal solid waste. J. Chem. Technol. Biotechnol. 95, 439–449. doi: 10.1002/jctb.6037
Oliveira, V., Horta, C., and Dias-Ferreira, C. (2019). Evaluation of a phosphorus fertiliser produced from anaerobically digested organic fraction of municipal solid waste. J. Clean. Prod. 238, 117911. doi: 10.1016/j.jclepro.2019.117911
Pantelopoulos, A., Magid, J., Jensen, L. S., and Fangueiro, D. (2017). Nutrient uptake efficiency in ryegrass fertilized with dried digestate solids as affected by acidification and drying temperature. Plant Soil 421, 401–416. doi: 10.1007/s11104-017-3463-y
Panuccio, M. R., Romeo, F., Mallamaci, C., and Muscolo, A. (2021). Digestate application on two different soils: agricultural benefit and risk. Waste Biomass Valor. 12, 4341–4353. doi: 10.1007/s12649-020-01318-5
Pedizzi, C., Noyam, I., Sarli, J., Gonzalez-Garcia, S., Lema, J. M., Moreira, M. T., et al. (2018). Environmental assessment of alternative treatment schemes for energy and nutrient recovery from livestock manure. Waste Manag. 77, 276–286. doi: 10.1016/j.wasman.2018.04.007
Pintucci, C., Carballa, M., Varga, S., Sarli, J., Peng, L., Bousek, J., et al. (2017). The ManureEcoMine pilot installation: advanced integration of technologies for the management of organics and nutrients in livestock waste. Water Sci. Technol. 75, 1281–1293. doi: 10.2166/wst.2016.559
Prurapark, R., Owjaraen, K., Saengphrom, B., Limthongtip, I., and Tongam, N. (2020). Effect of temperature on pyrolysis oil using high-density polyethylene and polyethylene terephthalate sources from mobile pyrolysis plant. Front. Energy Res. 8, 541535. doi: 10.3389/fenrg.2020.541535
Qi, G. D., Pan, Z. F., Sugawa, Y., Andriamanohiarisoamanana, F. J., Yamashiro, T., Iwasaki, M., et al. (2018). Comparative fertilizer properties of digestates from mesophilic and thermophilic anaerobic digestion of dairy manure: focusing on plant growth promoting bacteria (PGPB) and environmental risk. J. Mater. Cycles Waste Manag. 20, 1448–1457. doi: 10.1007/s10163-018-0708-7
Rahman, M. M., Salleh, M. A. M., Rashid, U., Ahsan, A., Hossain, M. M., and Ra, C. S. (2014). Production of slow release crystal fertilizer from wastewaters through struvite crystallization – a review. Arab. J. Chem. 7, 139–155. doi: 10.1016/j.arabjc.2013.10.007
Regueiro, I., Siebert, P., Liu, J. N., Muller-Stover, D., and Jensen, L. S. (2020). Acidified animal manure products combined with a nitrification inhibitor can serve as a starter fertilizer for maize. Agronomy 10, 1941. doi: 10.3390/agronomy10121941
Ro, K. S., Cantrell, K. B., and Hunt, P. G. (2010). High-temperature pyrolysis of blended animal manures for producing renewable energy and value-added biochar. Ind. Eng. Chem. Res. 49, 10125–10131. doi: 10.1021/ie101155m
Schneider, K. D., Martens, J. R. T., Zvomuya, F., Reid, D. K., Fraser, T. D., Lynch, D. H., et al. (2019). Options for improved phosphorus cycling and use in agriculture at the field and regional scales. J. Environ. Qual. 48, 1247–1264. doi: 10.2134/jeq2019.02.0070
Schoumans, O., Chardon, W., Bechmann, M., Gascuel-Odoux, C., Hofman, G., Kronvang, B., et al. (2014). Mitigation options to reduce phosphorus losses from the agricultural sector and improve surface water quality: a review. Sci. Total Environ. 468–469, 1255–1266. doi: 10.1016/j.scitotenv.2013.08.061
Selvaraj, P. S., Periasamy, K., Suganya, K., Ramadass, K., Muthusamy, S., Ramesh, P., et al. (2022). Novel resources recovery from anaerobic digestates: current trends and future perspectives. Crit. Rev. Environ. Sci. Technol. 52, 1915–1999. doi: 10.1080/10643389.2020.1864957
Senthilkumar, K., Nesme, T., Mollier, A., and Pellerin, S. (2012). Regional-scale phosphorus flows and budgets within France: the importance of agricultural production systems. Nutr. Cycling Agroecosyst. 92, 145–159. doi: 10.1007/s10705-011-9478-5
Shi, L., Simplicio, W. S., Wu, G., Hu, Z., Hu, H., and Zhan, X. (2018). Nutrient recovery from digestate of anaerobic digestion of livestock manure: a review. Curr. Pollut. Rep. 4, 74–83. doi: 10.1007/s40726-018-0082-z
Siciliano, A., and De Rosa, S. (2014). Recovery of ammonia in digestates of calf manure through a struvite precipitation process using unconventional reagents. Environ. Technol. 35, 841–850. doi: 10.1080/09593330.2013.853088
Siciliano, A., Limonti, C., Curcio, G. M., and Molinari, R. (2020). Advances in struvite precipitation technologies for nutrients removal and recovery from aqueous waste and wastewater. Sustainability 12, 7538. doi: 10.3390/su12187538
Stutter, M. I. (2015). The composition, leaching, and sorption behavior of some alternative sources of phosphorus for soils. Ambio 44, S207–S216. doi: 10.1007/s13280-014-0615-7
Stutzenstein, P., Bacher, M., Rosenau, T., and Pfeifer, C. (2018). Optimization of nutrient and carbon recovery from anaerobic digestate via hydrothermal carbonization and investigation of the influence of the process parameters. Waste Biomass Valor. 9, 1303–1318. doi: 10.1007/s12649-017-9902-4
Szymanska, M., Szara, E., Sosulski, T., Was, A., van Pruissen, G. W. P., Cornelissen, R. L., et al. (2019). A bio-refinery concept for n and p recovery –a chance for biogas plant development. Energies 12, 155. doi: 10.3390/en12010155
Tambone, F., Terruzzi, L., Scaglia, B., and Adani, F. (2015). Composting of the solid fraction of digestate derived from pig slurry: biological processes and compost properties. Waste Manage. 35, 55–61. doi: 10.1016/j.wasman.2014.10.014
Tang, Y., Wang, L., Carswell, A., Misselbrook, T., Shen, J., and Han, J. (2020). Fate and transfer of heavy metals following repeated biogas slurry application in a rice-wheat crop rotation. J. Environ. Manage. 270, 110938. doi: 10.1016/j.jenvman.2020.110938
Tao, W., Fattah, K. P., and Huchzermeier, M. P. (2016). Struvite recovery from anaerobically digested dairy manure: a review of application potential and hindrances. J. Environ. Manage. 169, 46–57. doi: 10.1016/j.jenvman.2015.12.006
Tarayre, C., Clercq, L. D., Charlier, R., Michels, E., Meers, E., Camargo-Valero, M., et al. (2016). New perspectives for the design of sustainable bioprocesses for phosphorus recovery from waste. Bioresour. Technol. 206, 264–274. doi: 10.1016/j.biortech.2016.01.091
Torres-Climent, A., Martin-Mata, J., Marhuenda-Egea, F., Moral, R., Barber, X., Perez-Murcia, M. D., et al. (2015). Composting of the solid phase of digestate from biogas production: optimization of the moisture, C/N ratio, and pH conditions. Commun. Soil Sci. Plant Anal. 46, 197–207. doi: 10.1080/00103624.2014.988591
Tsai, W. T., Fang, Y. Y., Cheng, P. H., and Lin, Y. Q. (2018). Characterization of mesoporous biochar produced from biogas digestate implemented in an anaerobic process of large-scale hog farm. Biomass Convers. Biorefin. 8, 945–951. doi: 10.1007/s13399-018-0344-4
Tuszynska, A., Wilinska, A., and Czerwionka, K. (2021). Phosphorus and nitrogen forms in liquid fraction of digestates from agricultural biogas plants. Environ. Technol. 42, 3942–3954. doi: 10.1080/09593330.2020.1770339
Van Poucke, R., Egene, C. E., Allaert, S., Lebrun, M., Bourgerie, S., Morabito, D., et al. (2020). Application of biochars and solid fraction of digestate to decrease soil solution Cd, Pb and Zn concentrations in contaminated sandy soils. Environ. Geochem. Health 42, 1589–1600. doi: 10.1007/s11356-013-1659-0
Vanden Nest, T., Amery, F., Fryda, L., Boogaerts, C., Bilbao, J., and Vandecasteele, B. (2021). Renewable P sources: P use efficiency of digestate, processed animal manure, compost, biochar and struvite. Sci. Total Environ. 750, 141699. doi: 10.1016/j.scitotenv.2020.141699
Vanden Nest, T. V., Ruysschaert, G., Vandecasteele, B., Cougnon, M., Merckx, R., and Reheul, D. (2015). P availability and P leaching after reducing the mineral P fertilization and the use of digestate products as new organic fertilizers in a 4-year field trial with high P status. Agric. Ecosyst. Environ. 202, 56–67. doi: 10.1016/j.agee.2014.12.012
Vaneeckhaute, C., Janda, J., Vanrolleghem, P. A., Tack, F. M. G., and Meers, E. (2016). Phosphorus use efficiency of bio-based fertilizers: bioavailability and fractionation. Pedosphere 26, 310–325. doi: 10.1016/S1002-0160(15)60045-5
Vaneeckhaute, C., Lebuf, V., Michels, E., Belia, E., Vanrolleghem, P. A., Tack, F. M. G., et al. (2017). Nutrient recovery from digestate: systematic technology review and product classification. Waste Biomass Valori. 8, 21–40. doi: 10.1007/s12649-016-9642-x
Vaneeckhaute, C., Meers, E., Michels, E., Christiaens, P., and Tack, F. M. G. (2012). Fate of macronutrients in water treatment of digestate using vibrating reversed osmosis. Water Air Soil Pollut. 223, 1593–1603. doi: 10.1007/s11270-011-0967-6
Vanotti, M., and Szogi, A. (2009). “Technology for recovery of phosphorus from animal wastewater through calcium phosphate precipitation,” in International Conference on Nutrient Recovery From Wastewater Streams: May (Vancouver, BC), 10–13.
Vasco-Correa, J., Khanal, S., Manandhar, A., and Shah, A. (2018). Anaerobic digestion for bioenergy production: global status, environmental and techno-economic implications, and government policies. Bioresour. Technol. 247, 1015–1026. doi: 10.1016/j.biortech.2017.09.004
Veneklaas, E. J., Lambers, H., Bragg, J., Finnegan, P. M., Lovelock, C. E., Plaxton, W. C., et al. (2012). Opportunities for improving phosphorus-use efficiency in crop plants. New Phytol. 195, 306–320. doi: 10.1111/j.1469-8137.2012.04190.x
Wang, W., and Lee, D. J. (2021). Valorization of anaerobic digestion digestate: a prospect review. Bioresour. Technol. 323, 124626. doi: 10.1016/j.biortech.2020.124626
Yan, Z. S., Liu, K., Yu, H. R., Liang, H., Xie, B. H., Li, G. B., et al. (2019a). Treatment of anaerobic digestion effluent using membrane distillation: effects of feed acidification on pollutant removal, nutrient concentration and membrane fouling. Desalination 449, 6–15. doi: 10.1016/j.desal.2018.10.011
Yan, Z. S., Yang, H. Y., Qu, F. S., Zhang, H., Rong, H. W., Yu, H. R., et al. (2019b). Application of membrane distillation to anaerobic digestion effluent treatment: identifying culprits of membrane fouling and scaling. Sci. Total Environ. 688, 880–889. doi: 10.1016/j.scitotenv.2019.06.307
Yao, Y., Huang, G., An, C., Chen, X., Zhang, P., Xin, X., et al. (2020). Anaerobic digestion of livestock manure in cold regions: technological advancements and global impacts. Renew. Sust. Energ. Rev. 119, 109494. doi: 10.1016/j.rser.2019.109494
Yue, C. D., Dong, H. M., Chen, Y. X., Shang, B., Wang, Y., Wang, S. L., et al. (2021). Direct purification of digestate using ultrafiltration membranes: influence of pore size on filtration behavior and fouling characteristics. Membranes 11, 179. doi: 10.3390/membranes11030179
Zacharof, M. P., Mandale, S. J., Oatley-Radcliffe, D., and Lovitt, R. W. (2019). Nutrient recovery and fractionation of anaerobic digester effluents employing pilot scale membrane technology. J. Water Process. Eng. 31, 100846. doi: 10.1016/j.jwpe.2019.100846
Zhan, Y. H., Yin, F. B., Yue, C. D., Zhu, J., Zhu, Z. P., Zou, M. Y., et al. (2020). Effect of pretreatment on hydraulic performance of the integrated membrane process for concentrating nutrient in biogas digestate from swine manure. Membranes 10, 249. doi: 10.3390/membranes10100249
Zhang, T., He, X. Y., Deng, Y. X., Tsang, D. C. W., Jiang, R. F, Becker, G. C., et al. (2020). Phosphorus recovered from digestate by hydrothermal processes with struvite crystallization and its potential as a fertilizer. Sci. Total Environ. 698, 134240. doi: 10.1016/j.scitotenv.2019.134240
Zhao, X., Becker, G. C., Faweya, N., Correa, C. R., Yang, S., Xie, X., et al. (2018). Fertilizer and activated carbon production by hydrothermal carbonization of digestate. Biomass Convers. Biorefin. 8, 423–436. doi: 10.1007/s13399-017-0291-5
Zheng, T. X., Qiu, Z. L., Dai, Q. Z., and Chen, J. M. (2019). Study of biogas slurry concentrated by reverse osmosis system: characteristics, optimization, and mechanism. Water Environ. Res. 91, 1447–1454. doi: 10.1002/wer.1137
Zin, M. M. T., and Kim, D.-J. (2019). Struvite production from food processing wastewater and incinerated sewage sludge ash as an alternative N and P source: optimization of multiple resources recovery by response surface methodology. Process Saf. Environ. Prot. 126, 242–249. doi: 10.1016/j.psep.2019.04.018
Zubair, M., Wang, S., Zhang, P., Ye, J., Liang, J., Nabi, M., et al. (2020). Biological nutrient removal and recovery from solid and liquid livestock manure: recent advance and perspective. Bioresour. Technol. 301, 122823. doi: 10.1016/j.biortech.2020.122823
Keywords: anaerobic digestion, digestate refinement, fertilizer, membrane filtration, phosphorus acquisition efficiency, phosphorus use efficiency, phosphorus recycling, struvite
Citation: Persson T and Rueda-Ayala V (2022) Phosphorus retention and agronomic efficiency of refined manure-based digestate—A review. Front. Sustain. Food Syst. 6:993043. doi: 10.3389/fsufs.2022.993043
Received: 15 July 2022; Accepted: 20 September 2022;
Published: 11 October 2022.
Edited by:
Paula Alvarenga, University of Lisbon, PortugalReviewed by:
Carmo Horta, Instituto Politécnico de Castelo Branco, PortugalMargarida Quina, University of Coimbra, Portugal
Copyright © 2022 Persson and Rueda-Ayala. This is an open-access article distributed under the terms of the Creative Commons Attribution License (CC BY). The use, distribution or reproduction in other forums is permitted, provided the original author(s) and the copyright owner(s) are credited and that the original publication in this journal is cited, in accordance with accepted academic practice. No use, distribution or reproduction is permitted which does not comply with these terms.
*Correspondence: Tomas Persson, dG9tYXMucGVyc3NvbkBuaWJpby5ubw==
†Present address: Victor Rueda-Ayala, Herbology in Field Crops, Agroscope, Nyon, Switzerland
‡ORCID: Tomas Persson orcid.org/0000-0002-7117-9023
Victor Rueda-Ayala orcid.org/0000-0002-9159-8276