- 1Water Research Commission of South Africa, Lynnwood Manor, Pretoria, South Africa
- 2Agricultural Research Council—Natural Resources and Engineering, Pretoria, South Africa
- 3Risks and Vulnerability Assessment Centre, University of Limpopo, Sovenga, South Africa
- 4Centre for Transformative Agricultural and Food Systems, School of Agricultural, Earth and Environmental Sciences, University of KwaZulu-Natal, Pietermaritzburg, South Africa
- 5International Maize and Wheat Improvement Centre (CIMMYT)-Zimbabwe, Harare, Zimbabwe
- 6Faculty of Science, Engineering and Agriculture, University of Venda, Thohoyandou, South Africa
- 7International Water Management Institute (IWMI), Pretoria, South Africa
Food demand in Africa continues to outstrip local supply, and the continent currently spends over US$35 billion annually on food imports to supplement local deficits. With the advances in agronomy and breeding, commercial crops like maize (Zea mays) and soybean (Glycine max) in the region are under threat from climate change, decreasing rainfall and degraded lands. Unlike commercial crops that are generally adapted from other regions, underutilized indigenous crops are uniquely suited to local environments and are more resilient to climatic variations and tolerant to local pests and diseases. This study, done in Limpopo Province, South Africa, identifies optimal areas for cultivating Bambara groundnuts (Vigna subterannea), an indigenous crop suitable for arid and semi-arid regions. The aim is to promote the production of underutilized indigenous crops at a large scale with fewer resources, while still meeting local demand and reducing the food import budget. Suitability maps are delineated using a multicriteria decision method in a Geographic Information System (GIS). The procedure is important for diversifying farming systems, making them more resilient (to biotic and abiotic stresses and climate change) and more successful at enhancing water, food and nutritional security. With the province's limited water and land resources for agriculture expansion, promoting indigenous underutilized crops is a pathway to reduce water allocated to agriculture, thereby enhancing drought resilience and ensuring water, food and nutritional security. Large tracts of degraded agricultural land deemed unsuitable for adapted crops, and which may require costly land reclamation practices, can be used to cultivate underutilized crops that are adapted to extreme local conditions.
Introduction
Underutilized indigenous crops are locally produced crop species primarily grown in particular native communities but have been losing their popularity as they have not been mainstreamed into the main food system (Mabhaudhi et al., 2019a; Jahanshiri et al., 2020). They cover a limited area that is neglected in terms of research and are consequently underfunded due to their limited importance in the global food market (Chivenge et al., 2015; Akinola et al., 2020). However, they are often characterized by their resilience and adaptation to extreme climatic and edaphic conditions and have local significance (Padulosi et al., 2011; Stamp et al., 2012; Akinola et al., 2020). Indigenous crop varieties are best-suited to local environmental conditions, and farmers' needs in marginal agricultural situations (Mabhaudhi et al., 2016). Their low input requirements give them an economic advantage over commercial crops like maize (Zea mays), soybean (Glycine max), rice (Oryza sativa) and wheat (Triticum aestivum) (Chibarabada et al., 2017). Current changing environments in southern Africa, characterized by extreme droughts and a shortening rain season, favor indigenous crops (Mabhaudhi et al., 2019b; Nhamo et al., 2019). Historically, they have always helped ensure food and nutrition security as part of a balanced diet, when adapted crops fail, or in between harvests (Tadele and Assefa, 2012; Mabhaudhi et al., 2019b). They provide important vitamins, proteins, and micronutrients and contribute to alleviating the challenges of growth stunting in children in developing countries (Chivenge et al., 2015; Akinola et al., 2020). Examples of priority indigenous underutilized crops suitable for southern Africa are shown in Table 1 (Mabhaudhi et al., 2017).
Unlike commercial crops that require costly agronomic practices and a lot of water for their cultivation, underutilized indigenous crops are uniquely suited to local environments, are generally more resistant to certain climatic variations and more tolerant to local pests (Chivenge et al., 2015; Keleman Saxena et al., 2016). Therefore, focusing on the cultivating of indigenous crops is an alternative climate change adaptation strategy (Mabhaudhi et al., 2019b). Furthermore, the consumption of indigenous crops provides nutritional diversity for communities, provides crop rotation options for farmers, creates niche markets in local economies, harnesses and enhances local knowledge (Chivenge et al., 2015; Massawe et al., 2015; Lin Tan et al., 2020). They further provide opportunities to enhance agro-biodiversity at the field level, promote nutritional diversity, disrupt pest and disease cycles (Kahane et al., 2013; Kimani-Murage et al., 2021), and reduce water share allocated to agriculture (Mabhaudhi et al., 2018a). Thus, harnessing and mainstreaming local knowledge and traditional crop species and developing underutilized crop breeds have enormous potential to improve water, food and nutrition security (Padulosi et al., 2013; Adhikari et al., 2017; Gerrano et al., 2021).
Regional and national policies in developing countries aim to increase the area under irrigation as a remedy to meet the food requirements of a growing population and ensure food and water security (CAADP, 2009; NDP, 2011). The increase in the irrigated area mainly targets commercial crops as they require more water than indigenous crops (Shelef et al., 2017; Fernández García et al., 2020). Although such initiatives of promoting commercial crops through the expansion of irrigation sound noble, the main challenges are water scarcity and land unavailability for an expanded irrigated area (Mancosu et al., 2015; Chibarabada et al., 2017). In South Africa, it is estimated that 98% of available water resources are already allocated, with over 60% of available water resources allocated to agriculture (Blignaut and Van Heerden, 2009; Von Bormann and Gulati, 2014). In southern Africa, more than 70% of available freshwater resources are used for agriculture, yet the region is regularly devastated by recurring food insecurity challenges (Nhamo et al., 2018; Ngcamu and Chari, 2020). Under such a changing environment, more emphasis should be on promoting the production of indigenous crops as they are suited to harsh local conditions (Stamp et al., 2012; Chivenge et al., 2015; Mabhaudhi et al., 2016). Indigenous crops are generally acceptable and appealing to local communities as they promote the preservation of culture and improve food selection and preparation concerning local people's needs and cultural values (Baldermann et al., 2016; Mabhaudhi et al., 2019b). Identifying suitable areas for cultivating underutilized crops is the initial step for their promotion, commercialization and mainstreaming into the main food system.
Cropland suitability mapping is an assessment of land performance when used to produce specific crops (Jahanshiri et al., 2020). It is a prerequisite to achieving optimum utilization of the available land resources for sustainable agriculture production (FAO, 1976; Li et al., 2010; Hagos et al., 2022). Adaptation of crop growth to local agro-ecological conditions' capabilities and constraints is a key principle of sustainable land management (Pretty and Bharucha, 2014; Viana et al., 2022). Identifying optimum land for cultivating indigenous crops is critical for the conservation of environmental resources while at the same time achieving maximum yields (Eastman, 1999; Mabhaudhi et al., 2019b). Thus, cropland suitability mapping provides information for growing potential crops and deriving maximum economic benefits with lower production costs (Kihoro et al., 2013). It also facilitates better water and land management. As indigenous underutilized crops are the ‘future food' (Baldermann et al., 2016), this study used relevant agro-ecological factors in a Geographic Information System (GIS) to delineate optimum areas for cultivating Bambara groundnut [Vigna subterannea (L.)] in Limpopo Province, South Africa. The objective is to provide a procedure to delineate optimum areas for cultivating indigenous crops as a first step to promoting and mainstreaming underutilized crops into the main food system, focusing on the Bambara groundnut.
An overview of the Bambara groundnut
Bambara groundnut (Figure 1) is an indeterminate annual crop that grows close to the ground with seeds being produced underground (DALRRD, 2011; Gerrano et al., 2021; Khan et al., 2021). Being a highly adaptable legume, Bambara groundnut is grown in diverse agroecosystems and generally grows well under drought conditions (Mabhaudhi et al., 2013; Khan et al., 2021). Bambara groundnut has a low water requirement (Mabhaudhi and Modi, 2014), and has been identified as exhibiting all three categories of drought adaptation strategies which are escape, avoidance and tolerance (Mayes et al., 2019; Khan et al., 2021). However, the crop has a low tolerance for waterlogged soils and grows best in well-drained soils (Khan et al., 2021). Some varieties of Bambara groundnut exhibit tolerance to salinity (Mayes et al., 2019). Also, the presence of variation in photoperiod and temperature sensitivity among Bambara groundnut genotypes is a good indicator that there is room for improvement in varieties for adaptation in broad agroecological farming zones (Kendabie et al., 2020). Bambara groundnut has been identified as a potential future crop under climate change (Mabhaudhi et al., 2019b; Khan et al., 2021). In South Africa, Bambara groundnut yield and water productivity are projected to increase by ~ 37.5% and 33% under climate change (DALRRD, 2011; Mabhaudhi et al., 2018a).
The Bambara groundnut seeds are rich in carbohydrates, proteins, fiber, ash, fat, and micronutrients, making them a valuable source of nutrition for resource-poor farmers (Lin Tan et al., 2020; Khan et al., 2021). However, limited genetic improvement, poor milling characteristics, long cooking time, and anti-nutritional factors have resulted in the crop being underutilized (Lin Tan et al., 2020; Gerrano et al., 2021). Even though the crop is underutilized, the Bambara groundnut plays a key role in both food, and cultural practices of farmers in Africa and Asia and has been integrated into intercropping systems (Mayes et al., 2019; Lin Tan et al., 2020; Khan et al., 2021).
Materials and methods
Description of the study area
The Limpopo Province of South Africa is the northernmost province, sharing international boundaries with Botswana, Mozambique, and Zimbabwe. The province (Figure 2) covers 125,755 km2, comprising 10.4% of the total national area. It has a population of about 6 million people (StatsSA, 2021). It has a varied topography, ranging from lowlands dominated by bushveld vegetation to imposing mountains rich in native forests and unspoilt savanna wilderness (Cai et al., 2017). The topography is divided into three distinct regions that define the climate and vegetation of the province. These include (a) Lowveld region (arid and semi-arid), (b) Middle-veld region (semi-arid region) and (c) Escarpment region (sub-humid climate with rainfall above 700 mm per annum) (Cai et al., 2017).
Rainfall is received in the summer (October to March), averaging 500 mm per annum, whilst the other three seasons are generally dry. The eastern and northern parts are subtropical, with humid and hot summers. Average summer temperatures are around 27°C. In winter (May to September), the nights are cold and mostly frost-free, with chilly mornings and dry, sunny days (Vincent et al., 2010). However, the Lowveld is very hot with temperatures often exceeding 45°C. The province has abundant fruit and vegetable production as it is endowed with abundant agricultural resources. However, the most limiting resource for agriculture in the province is water, and most of the smallholder farms are under rain-fed agriculture (Oni et al., 2012). Both commercial and smallholder farmers contribute significantly to crop production, although many rural people still practice subsistence farming and are highly vulnerable to climate change.
Background of the land suitability mapping algorithm in South Africa
As knowledge of South African soils grew in the late 1960's and early 1970's, there was also a growing realization that, while the soils in many areas were wellknown and had been comprehensively sampled and studied, there was a real need for a comprehensive reconnaissance survey of the natural resources of the whole country (ARC, 2014). This would include the recording of soils, terrain and macroclimate information so that these three pillars of agricultural production would be addressed simultaneously. This survey, which became known as the Land Type Survey (Land Type Survey Staff 1972–2002), was systematically carried out by the Soil and Irrigation Research Institute (SIRI) of the Department of Agriculture, Land Reform and Rural Development (DALRARD), which in 1992 became the Agricultural Research Council - Institute for Soil, Climate and Water (ARC-ISCW) (ARC, 2014).
The survey's fundamental mapping unit is the land type, which is a unique combination of broad soil pattern, terrain type, and macroclimate. Where any one of these three factors changes, a new land type unit would then be identified (ARC, 2014). The survey was carried out using 1:50,000 scale topo-cadastral maps as background, but it was always intended that the final product would be a series of maps at 1:250,000 scale. After the survey in 2002, a total of 7,071 unique land types had been identified, some of which had more than one occurrence. Therefore, over 15,000 distinct polygons were eventually defined and mapped (ARC, 2014). It is estimated that around 400,000 soil observations (mainly using a soil auger, but also including road cuttings, quarries, riverbanks, etc.) were made, which equates to approximately one observation per 400 ha across the whole country. This is more intense in the more productive areas, such as the Highveld and other eastern zones.
In contrast, the drier areas and the more inaccessible mountainous zones were investigated at a noticeably lower detail level. However, every effort was made to physically cover as much of the landscape as possible (ARC, 2014). Therefore, it is important to note the following:
• The Land Type Survey was carried out across the country, making South Africa probably the only country in Africa, and one of a select few in the world, that has systematic soil information of the whole surface area, backed by comprehensive analytical data.
• The fact that specific principles and guidelines were established and written down (MacVicar et al., 1974; Laker, 2004) means that the survey was carried out to a consistently high, uniform standard throughout the almost 30 years of its completion.
• There was a high degree of staff continuity, with several SIRI/ARC-ISCW employees being involved with the survey for almost the entire 30 years.
The above factors mean that the Land Type Survey can be regarded as a high-quality, comprehensive product that has massively contributed to natural resource characterization, and agricultural productivity in South Africa, and continues to do so. As the only source of continuous natural resource data for South Africa, it is an important national asset.
Digitization of land type data
The knowledge that the land type data, comprising the boundary lines of each mapping unit (vector data) as well as the soil, climate and terrain data contained therein (raster data), could be digitized, and captured on a GIS was the most significant development since the start of the survey. Although this involved a significant amount of trial and error and a great degree of checking and correcting data, it had several advantages. Firstly, the data was available in a digital format, which meant it could be stored, manipulated, and interpreted. Secondly, each land type had a measured area, both in total extent and for the various component terrain types (crest, scarp, mid-slope, foot-slope, valley bottom), one or more of which occurred in varying proportions in every mapping unit. This meant that the estimated occurrence of the soil forms and series (derived from the ground-truthing exercise that comprised the land type field investigation phase), could be empirically linked to each terrain type and consequently to each full land type. This allowed for the determination of soil occurrence within a land type, or combination of land types, to be made.
Algorithm development
Even in the pre-digitisation era, there were various attempts to use land type data for a broad assessment of agricultural potential (Schoeman, 1978; Scheepers, 1984). However, with the advent of GIS and big data management platforms, it has become much easier to store, process and analyse large amounts of data. The first major initiative to use computerized data involved the concept of land suitability. The original concept derived from the USDA (Klingebiel and Montgomery, 1961) considered the allocation of land into one of eight general classes of suitability for rainfed agriculture, depending on the limitations in terms of a combination of soil (depth, structure, rockiness, etc.), climate (rainfall or temperature restrictions) or terrain (slope). The availability of soil data from the Land Type Survey and interpolated climate data from the Agrometeorology database (Laker, 2004) allowed the development of such a classification for South Africa.
The land capability determination was done primarily through an algorithm, a computer-based, step-by-step procedure for calculations, data processing, and automated reasoning. An algorithm's advantage is that instructions can readily be adjusted, then rerun very quickly and efficiently, thus facilitating comparison of previous and new results. In this way, specific algorithms can be created for various crops and adjusted to differing production areas or scenarios (such as winter rainfall in the Western Cape vs. summer rainfall on the Highveld, rain-fed vs. irrigated conditions, and so on) to obtain a set of comparable results.
The algorithm is determined as follows: Using a combination of local knowledge and yield results from a specific area, the various production parameters are determined. These include climatic factors (mainly rainfall and temperature), soil factors (such as soil form, effective depth and texture) and terrain (slope class). The algorithm for Bambara groundnuts, using information derived from all available sources, is shown in Table 2.
Using local expert knowledge from various sources, such as the ARC, the maximum practical, sustainable yield for any crop is established and the type of yield reduction that would make a crop uneconomic and thus not sustainable (DALRRD, 2011). This allows the initial suitability classes to be established. In this case, four suitability classes (S1, S2, S3 and N) are shown (Table 2). Still, it may be logical to determine either a higher or lower number of classes per crop, depending on what is sensible according to the various factors involved. Climatic parameters can then be established, in this case, rainfall over the summer period and different temperature characteristics in the most important part of the growing season. However, other variables, such as evaporation or frost period, may also be used as required (and if available). The assumption is that the lower the rainfall and the more extreme the other parameters, the less suitable the area is for crop production.
The ideal slope class is generally determined as being below 4%, with steeper slopes being both more problematic to cultivate and potentially posing an increased hazard for soil erosion. The various soils occurring within any land type are allocated into a specific soil category (as defined in Table 3). The soils listed in Table 3 comprise all of the soil forms occurring in the Binomial System (MacVicar et al., 1974), which was the system in use at the commencement of the Land Type Survey and was used for the whole project.
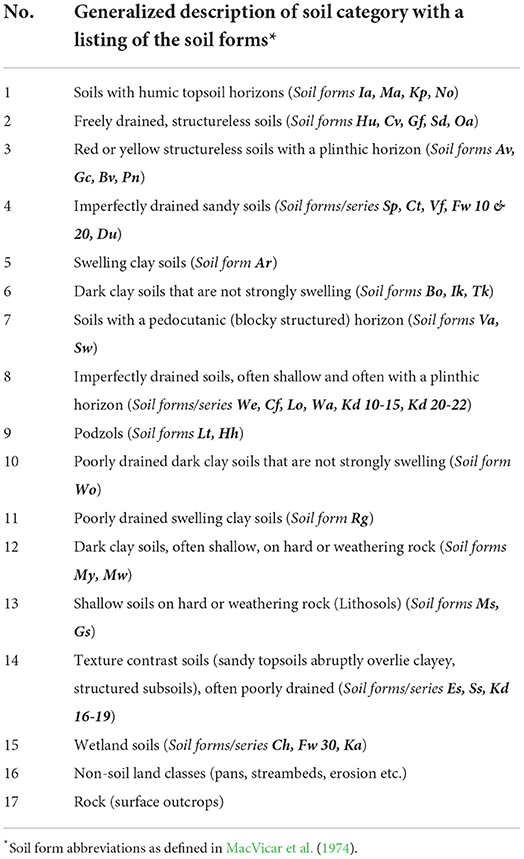
Table 3. A generalized description of the soil categories together with the soil forms (symbols in brackets) used in the crop suitability algorithm.
The soils are logically grouped into seventeen categories, which are more or less sequential in terms of decreasing arable agricultural potential, due to a combination of factors such as texture, structure, drainage, and overall ease of cultivation. Generally, soils in categories 1–4 are those where limitations would be expected to be the least, categories 5–9 are more limiting but can still be used for production if other criteria are met, while categories 10–17 usually pose significant problems and are often not recommended for cultivation.
When this soil category delineation is combined with effective soil depth (defined as the depth from the surface to any layer in the profile that is significantly limiting for root and/or water penetration), soil suitability can then be established. The hypothetical assumption is that the deeper the soil, the higher the suitability class. However, it is wellknown that in certain areas with low to marginal rainfall, coupled with light-textured soils, it is often advantageous to have a limiting layer (such as hard plinthite) at a specific depth range in the profile, to help with water retention in the root zone, especially in times of below-average rainfall. Such a scenario can also be built into the algorithm as needed.
Results and discussion
Algorithm results
For an area to have the highest possible degree of suitability, all the relevant parameters (climate, terrain, and soil) of the algorithm must be met. If one or more of these parameters are not met, the computer uses the algorithm to determine which class the site must be placed. Suppose all parameters are met, except one, then the class will need to be adjusted downwards, and so the principle of the lowest common denominator will apply.
Each land type inventory table is a list of all the soils occurring in that land type and their properties, along with the percentage occurrence of each one. Once the soil from a particular area is assessed in terms of the soil category group and the texture and depth information has been added, the algorithm uses the relevant slope and climate data and combines all these numerical combinations into one assessment per land type to determine relative occurrence percentages. The legend for the various crop suitability maps was designed so that firstly, all the high potential areas are determined and land types with >50%, 30–50% and 10–30% high potential land are identified (shown in shades of green in the map legend). If a land type contains <10% high potential land, the moderate potential areas are then assessed for the same percentages (shown in shades of orange/brown on the map) and so on.
The main benefit of the crop-specific algorithms is that, once the user is satisfied with the results, the respective areas of each suitability class can quickly be calculated (this can also be done for a province, local or district municipality, drainage basin or other study area as desired) and comparisons can be made. This can be used by other specialists, such as agricultural economists, to determine potential profit margins for a specific area.
Identifying areas suitable for growing Bambara groundnut
The suitability algorithm results can then be shown on a map to obtain a graphic representation of the distribution of the various classes. To illustrate this, the results of the dryland (rain-fed) Bambara groundnuts algorithm for the province are shown in Figure 3.
According to the procedure used to develop the map shown in Figure 3, much of Limpopo Province has marginal or no suitability for Bambara groundnut production, mainly due to climatic restrictions. However, if the algorithm is run without the rainfall requirement (using soil and terrain only), the results are significantly different (Figure 4). Under irrigated conditions (Figure 4) there is a much larger area with suitable soils, but where the rainfall is to a greater or lesser degree, insufficient.
As shown in Table 4, only around 22% of the province has moderate or high rainfed suitability and around 37% either has no suitability or falls within a national park. If rainfall is removed as a parameter (so that all moisture requirements need to be supplied by irrigation, the soil suitability situation is very different. In this scenario, around 30% of the province has some or other degree of high potential, with another 29% with moderate potential.
However, the scale of the Land Type Survey (1:250,000) means that the maps show areas of dominance only and that significant variation will exist within many of the map units. Such variation needs to be addressed by more detailed field investigations, as and when required.
Regarding degraded and/or eroded areas, land degradation is a challenging aspect to quantify. It is not easy to do from remote sensing (satellite) data since it takes many forms. Gullies (dongas) may be reasonably easy to delineate, but other aspects also occur, such as loss of topsoil, bush encroachment, soil acidification, and compaction. These problems usually need a detailed field investigation to even start to get reliable results.
The National Land Cover Database, which has gone through at least three versions since the first one in the 1990's, has had various methods to determine degraded land. Due to differences in methodologies between versions 1 and 2, there was a 36% decrease in degraded land in Limpopo (GTI, 2015). In a recent update of the dataset, the category “Degraded Land” has been removed, so there are no up-to-date figures. One source of information is a project carried out by ARC in conjunction with the Department of Agriculture, Forestry and Fisheries (Mararakanye and Le Roux, 2011) where every observable gully in South Africa was physically mapped. When the distribution of gullies in Limpopo (shown in black in Figure 5) is overlaid on the suitability map, it can be seen that there are significant areas in the province where this is a problem for cultivation as well as long-term environmental stability.
There is a need for further research in many areas to quantify the various forms of erosion and soil degradation that will reduce agricultural potential. In this way, some quantification of these areas might be obtained.
Delineating accurate suitability areas for the cultivation of crops is essential under climate change as it improves decisions on crop production and irrigation development to promote food and water security (Magidi et al., 2021b; Hagos et al., 2022). However, the main drawback in achieving accurate statistics on cropped area and the most suitable areas for specific crops has been the lack of reliable input data at reasonably high spatial resolution. There is more that still needs to be done on developing input datasets at acceptable spatial resolution as the current ones are too coarse to derive accurate statistics on spatial distribution and extent of irrigation (Magidi et al., 2021a). As the datasets used in this study may not be readily available in other regions, other crop suitability areas mapping approaches such as the multi-criteria decision method (MCDM) can be used and to achieve reliable results (Jahanshiri et al., 2020; Hagos et al., 2022).
Considerations for climate change adaptation
Our results showed that Limpopo province has some marginal or no unsuitable areas for Bambara groundnut production, mainly due to climatic restrictions. As the climate continues to change, suitable and moderately suitable areas may become marginally or unsuitable for Bambara groundnut production. The main risks associated with climate change include shifts in rainy season and increasing extreme weather conditions (heat stress, drought and floods) (DEEF, 2013) (Table 5). In the shorter term and regardless of land class, farmers, more so those under rainfed systems, are already vulnerable to current weather variability and associated shocks (Kruger and Nxumalo, 2017). Within the delineated production zones, a key element of climate adaptation is building resilience, and this entails redirecting and/or absorbing the disturbance without system collapse. To better represent adaptation, there is a need to expand the research to consider all factors, including social, economic and environmental domains. Also, more systems (agroecosystem, landscape, catchment) and place-based approaches representing local context, knowledge and aspects of food and nutrition security production other than land type, rainfall and temperature may be required (Beveridge et al., 2018).
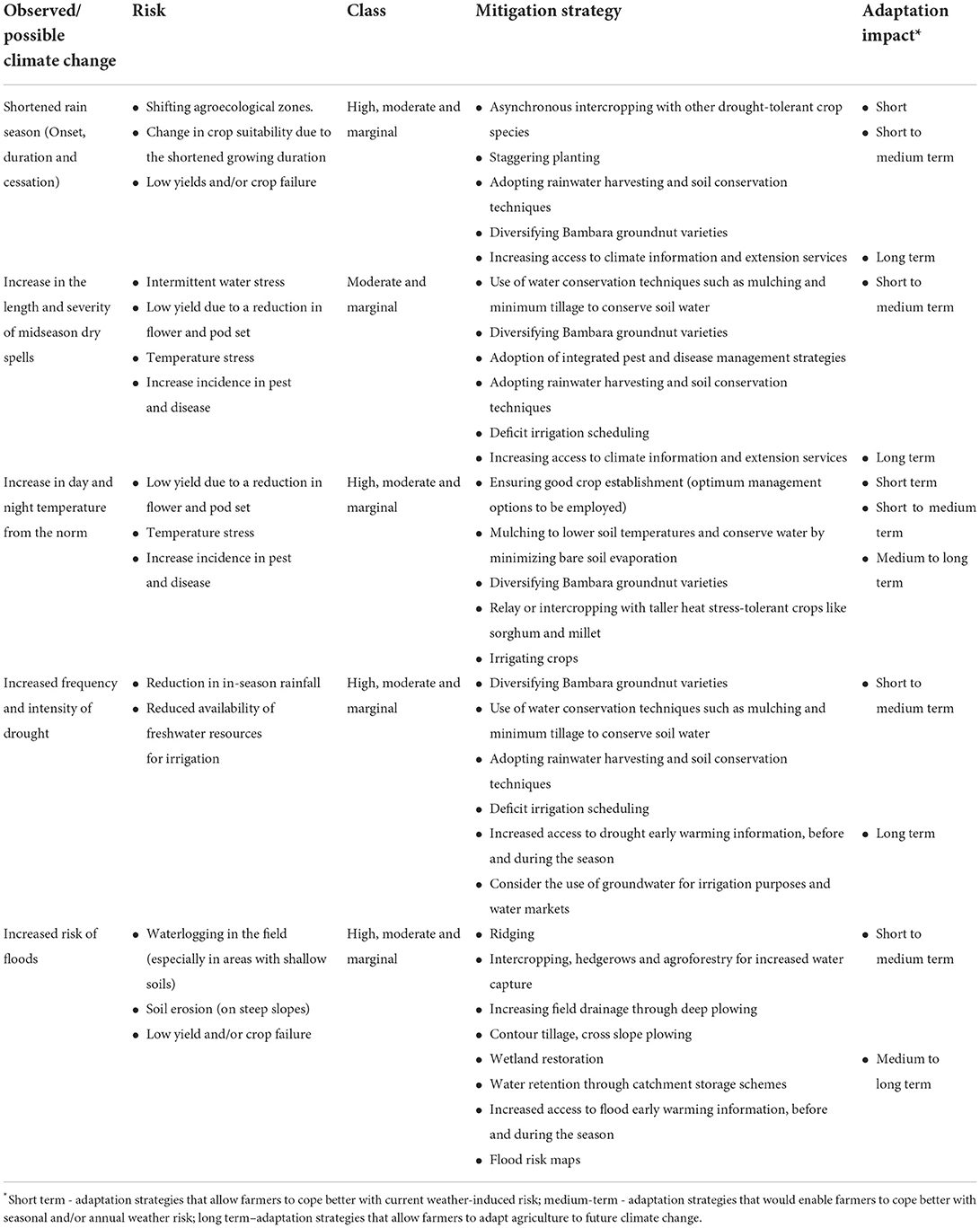
Table 5. Assessing the impacts of climate change for Bambara groundnut suitability [Adapted from Mpandeli et al. (2019) and Chimonyo et al. (2020)].
By comparing the Bambara groundnut crop requirements with the availability of resources, an assessment can be made of the possible levels of investments made in the system. For instance, it would be most favorable to look at areas where water requirements for Bambara groundnut and season rainfall show small differences to invest in an irrigation system. Also, in areas with slopes prone to soil erosion, short- and long-term strategies such as contour plowing and agroforestry, respectively, may be adopted (Table 5). The adoption of multi-cropping coupled with asynchronous or sequential planting can be viewed as a low-cost, short- to medium-term option to improve productivity and resource use efficiency under increasing temperature and water scarcity (Chimonyo et al., 2020) (Table 5). The inclusion of other traditional crops known as drought and heat tolerant into Bambara groundnut cropping systems should also be considered a complementary strategy to increasing medium- and long-term climate resilience in identified marginal areas (Chimonyo et al., 2020).
Estimating the potential land resources suitable for irrigation and evaluating the possible impact of climate change on land suitability are essential for planning a sustainable agricultural system. However, South Africa is already challenged with water scarcity. Groundwater use and water marketing are considered options to alleviate medium- to long-term water scarcity challenges (Matchaya et al., 2019). Still, the extent to which they can bring relief to the stressed water resources is yet unknown (Mabhaudhi et al., 2018b). Tapping into groundwater also requires reliable energy resources, which brings to the fore the need for a water-energy nexus planning to enhance agricultural and water productivity (Magidi et al., 2021b).
Conclusions
By using available natural resource information and matching it to specific crop production requirements, the basic principles of “matching” used in the land evaluation process are followed. The example used here of Bambara groundnuts can be compared with other similar crops, in association with yield estimates and enterprise budgets, to empirically evaluate the suitability for a range of underutilized crops in any specific area. Further to this, and due to the minimum data set required, the method can be replicated across South Africa to assess underutilized crops' suitability. Growing Bambara groundnut with the appropriate management options can be used as an adaptation strategy in areas classified as moderately suitable and marginal. It is imperative to accompany the information regarding land suitability with transformative and autonomous adaptation strategies to mitigate climate risks. In cases where resources are limited, the adoption of multi-cropping and rainwater harvesting, and soil conservation techniques can be used. This is particularly important for resource-poor farmers who reside in many of the marginal areas identified. To smallholder farmers, underutilized crops can address several socio-economic related challenges; therefore, future studies should consider factors such as access to markets, proximity to roads and population density.
Data availability statement
The original contributions presented in the study are included in the article/supplementary material, further inquiries can be directed to the corresponding author/s.
Author contributions
Conceptualization and writing—original draft preparation: LN and TM. Methodology: LN, GP, MW, and TM. Software, formal analysis, and data curation: LN, GP, and MW. Validation: TM, AM, JM, SL, and VC. Resources and project administration: LN, SM, and TM. Visualization: JM, SL, GP, and MW. Supervision: LN and TM. Funding acquisition: AM and TM. Investigation and writing—review and editing: LN, GP, MW, MM, AM, RK, VC, TM, SM, SL, JM, and TM. All authors have read and agreed to the published version of the manuscript.
Funding
The Water Research Commission (WRC) of South Africa was acknowledged for initiating, funding and directing the study through WRC Project No. K5/2717//4 Developing guidelines for estimating green water use of indigenous crops in South Africa and estimating water use using available crop models for selected bio-climatic regions of South Africa. This research was funded, in part, by the Wellcome [Grant number: 205200/Z/16/Z]. For the purpose of Open Access, the author has applied a CC BY public copyright license to any Author Accepted Manuscript version arising from this submission.
Acknowledgments
The authors would like to thank Harold Weepener of the Agricultural Research Council for his role in developing high-resolution maps for the suitability of Bambara groundnuts.
Conflict of interest
The authors declare that the research was conducted in the absence of any commercial or financial relationships that could be construed as a potential conflict of interest.
Publisher's note
All claims expressed in this article are solely those of the authors and do not necessarily represent those of their affiliated organizations, or those of the publisher, the editors and the reviewers. Any product that may be evaluated in this article, or claim that may be made by its manufacturer, is not guaranteed or endorsed by the publisher.
References
Adhikari, L., Hussain, A., and Rasul, G. (2017). Tapping the potential of neglected and underutilized food crops for sustainable nutrition security in the mountains of Pakistan and Nepal. Sustainability 9:291. doi: 10.3390/su9020291
Akinola, R., Pereira, L. M., Mabhaudhi, T., de Bruin, F.-M., and Rusch, L. (2020). A review of indigenous food crops in Africa and the implications for more sustainable and healthy food systems. Sustainability 12:3493. doi: 10.3390/su12083493
ARC (2014). History of ARC-ISCW. Pretoria: Agriculture Research Council - Institute for Soil, Climate and Water (ARC-ISCW).
Baldermann, S., Blagojevi,ć, L., Frede, K., Klopsch, R., Neugart, S., Neumann, A., et al. (2016). Are neglected plants the food for the future? CRC. Crit. Rev. Plant Sci. 35, 106–119. doi: 10.1080/07352689.2016.1201399
Beveridge, L., Whitfield, S., and Challinor, A. (2018). Crop modelling: towards locally relevant and climate-informed adaptation. Clim. Change 147, 475–489. doi: 10.1007/s10584-018-2160-z
Blignaut, J., and Van Heerden, J. (2009). The impact of water scarcity on economic development initiatives. Water SA 35, 415–420. doi: 10.4314/wsa.v35i4.76800
CAADP (2009). Sustainable Land and water management. The CAADP Pillar 1 Framework. ‘Tool' for use by Countries in Mainstreaming and Upscaling of Sustainable Land and Water Management in Africa's Agriculture and Rural Development Agenda. Addis: African Union.
Cai, X., Magidi, J., Nhamo, L., and van Koppen, B. (2017). Mapping Irrigated Areas in the Limpopo Province, South Africa. Colombo: International Water Management Institute (IWMI). doi: 10.5337/2017.205
Chibarabada, T. P., Modi, A. T., and Mabhaudhi, T. (2017). Expounding the value of grain legumes in the semi-and arid tropics. Sustainability 9:60. doi: 10.3390/su9010060
Chimonyo, V. G., Wimalasiri, E. M., Kunz, R., Modi, A. T., and Mabhaudhi, T. (2020). Optimizing traditional cropping systems under climate change: a case of maize landraces and Bambara groundnut. Front. Sustain. Food Syst. 4, 562568. doi: 10.3389/fsufs.2020.562568
Chivenge, P., Mabhaudhi, T., Modi, A. T., and Mafongoya, P. (2015). The potential role of neglected and underutilised crop species as future crops under water scarce conditions in Sub-Saharan Africa. Int. J. Environ. Res. Public Health 12, 5685–5711. doi: 10.3390/ijerph120605685
DALRRD (2011). Production Guideline for Bambara Groundnuts. Pretoria: Directorate Plant Production, Department of Agriculture, Land Reform and Rural Development (DALRRD).
DEEF (2013). Long-Term Adaptation Scenarios Flagship Research Programme (LTAS) for South Africa: Climate Trends and Scenarios for South Africa Department of Environment. Pretoria: Forestry and Fisheries.
Eastman, J. (1999). “Multi-criteria evaluation and GIS,” in: Geographical Information Systems, eds L. Pa, M. Goodchild, D. Maguire, and D. Rhind (New York, NY: Wiley).
FAO (1976). A Framework for Land Evaluation. Rome: Food and Agriculture Organization of the United Nations (FAO).
Fernández García, I., Lecina, S., Ruiz-Sánchez, M. C., Vera, J., Conejero, W., Conesa, M. R., et al. (2020). Trends and challenges in irrigation scheduling in the semi-arid area of Spain. Water 12:785. doi: 10.3390/w12030785
Gerrano, A. S., Eifediyi, E. K., Labuschagne, M., Ogedegbe, F. O., and Hassen, A. I. (2021). “Production practices of bambara groundnut,” in: Food and Potential Industrial Applications of Bambara Groundnut, eds S. A. Oyeyinka and B. I. O Ade-Omowaye (Springer). doi: 10.1007/978-3-030-73920-1_2
GTI (2015). 2013-2014 South African National Land-Cover Dataset: Data User Report and Metadata. Pretoria: GeoTerraImage (GTI), Department of Environmental Affairs (DEA).
Hagos, Y. G., Mengie, M. A., Andualem, T. G., Yibeltal, M., Linh, N. T. T., Tenagashaw, D. Y., et al. (2022). Land suitability assessment for surface irrigation development at Ethiopian highlands using geospatial technology. Appl. Water Sci. 12, 1–11. doi: 10.1007/s13201-022-01618-2
Jahanshiri, E., Mohd Nizar, N. M., Tengku Mohd Suhairi, T. A. S., Gregory, P. J., Mohamed, A. S., Wimalasiri, E. M., et al. (2020). A land evaluation framework for agricultural diversification. Sustainability 12:3110. doi: 10.3390/su12083110
Kahane, R., Hodgkin, T., Jaenicke, H., Hoogendoorn, C., Hermann, M., d'Arros Hughes, J., et al. (2013). Agrobiodiversity for food security, health and income. Agron. Sustain. Dev. 33, 671–693. doi: 10.1007/s13593-013-0147-8
Keleman Saxena, A., Cadima Fuentes, X., Gonzales Herbas, R., and Humphries, D. L. (2016). Indigenous food systems and climate change: impacts of climatic shifts on the production and processing of native and traditional crops in the Bolivian Andes. Front. Public Health 4:20. doi: 10.3389/fpubh.2016.00020
Kendabie, P., Jørgensen, S. T., Massawe, F., Fernandez, J., Azam-Ali, S., and Mayes, S. (2020). Photoperiod control of yield and sink capacity in Bambara groundnut (Vigna subterranea) genotypes. Food Energy Security 9:e240. doi: 10.1002/fes3.240
Khan, M. M. H., Rafii, M. Y., Ramlee, S. I., Jusoh, M., and Al-Mamun, M. (2021). Bambara groundnut (Vigna subterranea L. Verdc): A crop for the new millennium, its genetic diversity, and improvements to mitigate future food and nutritional challenges. Sustainability 13:5530. doi: 10.3390/su13105530
Kihoro, J., Bosco, N. J., and Murage, H. (2013). Suitability analysis for rice growing sites using a multicriteria evaluation and GIS approach in great Mwea region, Kenya. Springerplus 2:265. doi: 10.1186/2193-1801-2-265
Kimani-Murage, E., Gaupp, F., Lal, R., Hansson, H., Tang, T., Chaudhary, A., et al. (2021). An optimal diet for planet and people. One Earth 4, 1189–1192. doi: 10.1016/j.oneear.2021.08.017
Klingebiel, A., and Montgomery, P. (1961). Land-capability classification. Agricultural Handbook. No. 210. Washington, DC: Soil Conservation Service. USDA Gov. Print. Office.
Kruger, A. C., and Nxumalo, M. (2017). Historical rainfall trends in South Africa: 1921–2015. Water SA 43, 285–297. doi: 10.4314/wsa.v43i2.12
Laker, M. (2004). Advances in soil erosion, soil conservation, land suitability evaluation and land use planning research in South Africa, 1978–2003. South Afr. J. Plant Soil 21, 345–368. doi: 10.1080/02571862.2004.10635069
Li, L., Zhao, J., and Yuan, T. (2010). Study on Approaches of Land Suitability Evaluation for Crop Production Using GIS, International Conference on Computer and Computing Technologies in Agriculture. Springer. doi: 10.1007/978-3-642-18336-2_72
Lin Tan, X., Azam-Ali, S., Goh, E. V., Mustafa, M. A., Chai, H. H., Kuan Ho, W., et al. (2020). Bambara groundnut: an underutilized leguminous crop for global food security and nutrition. Front. Nutr. 7:276. doi: 10.3389/fnut.2020.601496
Mabhaudhi, T., Chibarabada, T. P., Chimonyo, V. G. P., and Modi, A. T. (2018a). Modelling climate change impact: A case of bambara groundnut (Vigna subterranea). Phys. Chem. Earth 105, 25–31. doi: 10.1016/j.pce.2018.01.003
Mabhaudhi, T., Chibarabada, T. P., Chimonyo, V. G. P., Murugani, V. G., Pereira, L. M., Sobratee, N., et al. (2019a). Mainstreaming underutilized indigenous and traditional crops into food systems: A South African perspective. Sustainability 11:172. doi: 10.3390/su11010172
Mabhaudhi, T., Chimonyo, V. G., Chibarabada, T. P., and Modi, A. T. (2017). Developing a roadmap for improving neglected and underutilized crops: A case study of South Africa. Front. Plant Sci. 8:2143. doi: 10.3389/fpls.2017.02143
Mabhaudhi, T., Chimonyo, V. G. P., Hlahla, S., Massawe, F., Mayes, S., Nhamo, L., et al. (2019b). Prospects of orphan crops in climate change. Planta 2019, 1–14. doi: 10.1007/s00425-019-03129-y
Mabhaudhi, T., Modi, A., and Beletse, Y. (2013). Growth, phenological and yield responses of a bambara groundnut (Vigna subterranea L. Verdc) landrace to imposed water stress: II. Rain shelter conditions. Water SA 39, 191–198. doi: 10.4314/wsa.v39i2.2
Mabhaudhi, T., and Modi, A. T. (2014). Growth, phenological and yield responses of a bambara groundnut (Vigna subterranea (L.) Verdc.) landrace to imposed water stress under field conditions. South Afr. J. Plant Soil 30, 69–79. doi: 10.1080/02571862.2013.790492
Mabhaudhi, T., Mpandeli, S., Nhamo, L., Chimonyo, V. G., Nhemachena, C., Senzanje, A., et al. (2018b). Prospects for improving irrigated agriculture in southern Africa: Linking water, energy and food. Water 10:1881. doi: 10.3390/w10121881
Mabhaudhi, T., O'Reilly, P., Walker, S., and Mwale, S. (2016). Opportunities for underutilised crops in southern Africa's post−2015 development agenda. Sustainability 8:302. doi: 10.3390/su8040302
MacVicar, C., Scotney, D., Skinner, T., Niehaus, H., and Loubser, J. (1974). A classification of land (climate, terrain form, soil) primarily for rainfed agriculture. South Afr. J. Agricult. Exten. 3, 21–24.
Magidi, J., Nhamo, L., Mpandeli, S., and Mabhaudhi, T. (2021a). Application of the random forest classifier to map irrigated areas using google earth engine. Remote Sensing 13:876. doi: 10.3390/rs13050876
Magidi, J., van Koppen, B., Nhamo, L., Mpandeli, S., Slotow, R., and Mabhaudhi, T. (2021b). Informing equitable water and food policies through accurate spatial information on irrigated areas in smallholder farming systems. Water 13:3627. doi: 10.3390/w13243627
Mancosu, N., Snyder, R. L., Kyriakakis, G., and Spano, D. (2015). Water scarcity and future challenges for food production. Water 7, 975–992. doi: 10.3390/w7030975
Mararakanye, N., and Le Roux, J. (2011). Manual Digitising of Gully Erosion in South Africa Using High Resolution SPOT 5 Satellite Imagery at 1: 10 000 scale. Pretoria: Department of Agriculture, Forestry and Fisheries.
Massawe, F., Mayes, S., Cheng, A., Chai, H., Cleasby, P., Symonds, R., et al. (2015). The potential for underutilised crops to improve food security in the face of climate change. Proc. Environ. Sci. 29, 140–141. doi: 10.1016/j.proenv.2015.07.228
Matchaya, G., Nhamo, L., Nhlengethwa, S., and Nhemachena, C. (2019). An overview of water markets in Southern Africa: an option for water management in times of scarcity. Water 11:1006. doi: 10.3390/w11051006
Mayes, S., Ho, W. K., Chai, H. H., Gao, X., Kundy, A. C., Mateva, K. I., et al. (2019). Bambara groundnut: An exemplar underutilised legume for resilience under climate change. Planta 250, 803–820. doi: 10.1007/s00425-019-03191-6
Mpandeli, S., Nhamo, L., Moeletsi, M., Masupha, T., Magidi, J., Tshikolomo, K., et al. (2019). Assessing climate change and adaptive capacity at local scale using observed and remotely sensed data. Weather Clim. Extremes 26:100240. doi: 10.1016/j.wace.2019.100240
Ngcamu, B. S., and Chari, F. (2020). Drought influences on food insecurity in Africa: A systematic literature review. Int. J. Environ. Res. Public Health 17:5897. doi: 10.3390/ijerph17165897
Nhamo, L., Mabhaudhi, T., and Modi, A. (2018). Preparedness or repeated short-term relief aid? Building drought resilience through early warning in Southern Africa. Water 2018:e09. doi: 10.4314/wsa.v45i1.09
Nhamo, L., Matchaya, G., Mabhaudhi, T., Nhlengethwa, S., Nhemachena, C., and Mpandeli, S. (2019). Cereal production trends under climate change: Impacts and adaptation strategies in southern Africa. Agriculture 9:30. doi: 10.3390/agriculture9020030
Oni, S., Nesamvuni, A., Odhiambo, J., and Dagada, M. (2012). The Study of Agricultural Industry in the Limpopo Province (Executive Summary). Polokwane: Limpopo Province: Department of Agricultural Economics and Extention: Centre for Rural Development.
Padulosi, S., Heywood, V., Hunter, D., and Jarvis, A. (2011). “Underutilized species and climate change: current status and outlook,” in: Crop Adaptation to Climate Change, eds S. Yadav, R. Redden, J. Hatfield, H. Lotze-Campen, and A. Hall (New York, NY: Wiley). doi: 10.1002/9780470960929.ch35
Padulosi, S., Thompson, J., and Rudebjer, P. (2013). Fighting Poverty, Hunger and Malnutrition With Neglected and Underutilized Species: Needs, Challenges and the Way Forward. Bioversity International.
Pretty, J., and Bharucha, Z. P. (2014). Sustainable intensification in agricultural systems. Ann. Bot. 114, 1571–1596. doi: 10.1093/aob/mcu205
Scheepers, J. (1984). An Evaluation of the Agricultural Potential of the Highveld Region in Terms of Dryalnd Cropping and Livestock Production. Pretoria: Division of Agricultural Information.
Schoeman, C. (1978). A method for evaluating and presenting the agricultural potential of land at regional scales. Agrochemophysica 10, 25–37.
Shelef, O., Weisberg, P. J., and Provenza, F. D. (2017). The value of native plants and local production in an era of global agriculture. Front. Plant Sci. 8:2069. doi: 10.3389/fpls.2017.02069
Stamp, P., Messmer, R., and Walter, A. (2012). Competitive underutilized crops will depend on the state funding of breeding programmes: an opinion on the example of Europe. Plant Breed. 131, 461–464. doi: 10.1111/j.1439-0523.2012.01990.x
Tadele, Z., and Assefa, K. (2012). Increasing food production in Africa by boosting the productivity of understudied crops. Agronomy 2, 240–283. doi: 10.3390/agronomy2040240
Viana, C. M., Freire, D., Abrantes, P., Rocha, J., and Pereira, P. (2022). Agricultural land systems importance for supporting food security and sustainable development goals: A systematic review. Sci. Total Environ. 806:150718. doi: 10.1016/j.scitotenv.2021.150718
Vincent, K., Cull, T., and Archer, E. R. (2010). “Gendered vulnerability to climate change in Limpopo province, South Africa,” in: Gender and Climate Change: An Introduction, ed I. Dankelman (London: Earthscan).
Keywords: climate change, resilience, geographic information system, food and water security, dryland agriculture, adaptation
Citation: Nhamo L, Paterson G, van der Walt M, Moeletsi M, Modi A, Kunz R, Chimonyo V, Masupha T, Mpandeli S, Liphadzi S, Molwantwa J and Mabhaudhi T (2022) Optimal production areas of underutilized indigenous crops and their role under climate change: Focus on Bambara groundnut. Front. Sustain. Food Syst. 6:990213. doi: 10.3389/fsufs.2022.990213
Received: 12 July 2022; Accepted: 20 September 2022;
Published: 06 October 2022.
Edited by:
Tapan Kumar Nath, University of Nottingham Malaysia Campus, MalaysiaReviewed by:
Oluwaseyi Samuel Olanrewaju, Centro Internazionale di Ingegneria Genetica e Biotecnologie - ICGEB, ItalyPeter John Gregory, University of Reading, United Kingdom
Copyright © 2022 Nhamo, Paterson, van der Walt, Moeletsi, Modi, Kunz, Chimonyo, Masupha, Mpandeli, Liphadzi, Molwantwa and Mabhaudhi. This is an open-access article distributed under the terms of the Creative Commons Attribution License (CC BY). The use, distribution or reproduction in other forums is permitted, provided the original author(s) and the copyright owner(s) are credited and that the original publication in this journal is cited, in accordance with accepted academic practice. No use, distribution or reproduction is permitted which does not comply with these terms.
*Correspondence: Luxon Nhamo, bHV4b25uJiN4MDAwNDA7d3JjLm9yZy56YQ==