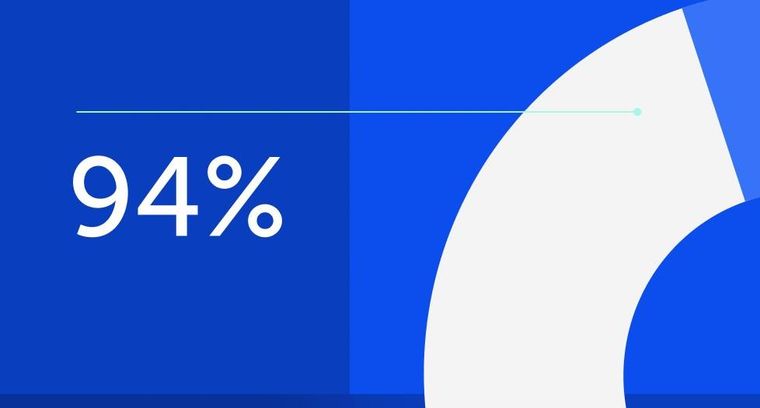
94% of researchers rate our articles as excellent or good
Learn more about the work of our research integrity team to safeguard the quality of each article we publish.
Find out more
ORIGINAL RESEARCH article
Front. Sustain. Food Syst., 10 January 2023
Sec. Agroecology and Ecosystem Services
Volume 6 - 2022 | https://doi.org/10.3389/fsufs.2022.961099
This article is part of the Research TopicContinuous Living Cover: Adaptive Strategies for Putting Regenerative Agriculture into PracticeView all 23 articles
Pennycress (Thlaspi arvense L.) is a new winter annual oilseed crop that can be integrated as a “cash cover crop” in Midwestern USA cropping systems. Relay-cropping pennycress with soybean [Glycine max (L.) Merr.] has been shown as an effective way to produce three crops over 2 years while providing living cover on the agricultural landscape nearly year-round. However, management improvements are needed to optimize pennycress and soybean production in this new system. A 2-year field study was conducted to evaluate three soybean interseeding dates (based on pennycress growth stage) and three soybean cultivars (varied in maturity date) on the overall productivity of this relay system. Interseeding dates were SD1 (rosette stage), SD2 (bolting stage), and SD3 (initial flowering), and soybean cultivars were MG0.2 (early), MG1.1 (standard), and MG1.7 (late). In the second season, relaying soybean reduced pennycress seed yield compared with its monocrop counterpart, but the reduction was lowest (23%) at SD2. Cultivar maturity group impacted soybean seed yields in the relay system, which for MG0.2, MG1.1, and MG1.7 averaged 2,589, 3,196, and 3,445 kg ha−1, respectively. Although there was soybean yield drag associated with relay cropping, the seed yield of the MG1.7 cultivar relay interseeded at SD2 was not significantly different from a monocropped MG1.1 soybean using conventional practice (CP; winter fallow, no pennycress). The results indicate that relay interseeding of longer maturity (MG1.7) soybean for the region at the bolting stage (SD2) of pennycress optimized overall system productivity while keeping the continuous living cover on the agricultural landscape. More research will likely be needed to improve soybean selection and management regionally for this unique relay system.
The agricultural landscape of the Midwest Corn Belt region of the USA is dominated by summer annual cropping systems that rely heavily on corn (Zea mays L.) and soybean production (Sindelar et al., 2017). Because of simplified crop rotations, agricultural diversity has suffered (Aguilar et al., 2015) and, consequently, so has agronomic and environmental sustainability. Intense management (e.g., tillage, fertilizers, and pesticides) of only a few crops to maintain high yields has resulted in unintended negative consequences including reduced water quality (Kladivko et al., 2014), soil erosion (Reicosky, 2015), increase in herbicide-resistant weeds (Mortensen et al., 2012), and declining pollinator diversity and abundance (Eberle et al., 2015; Thom et al., 2018). Furthermore, there is a growing societal trend among consumers of being more concerned about where and how their food is produced, influencing large food and beverage companies to source ingredients from more sustainable systems (Ringquist et al., 2016). A potential strategy to mitigate some of these issues is to employ perennial or annual cropping systems that provide diversity and keep living cover on the agricultural landscape as long as possible throughout the year (Heaton et al., 2013; Ryan et al., 2018). An obvious choice to do this in annual cropping-based systems is the use of cover crops during the fallow season. However, cover crop adoption in the Midwest Corn Belt of the USA has been slow, and farmers often cite the cost of establishing covers and little or no near-term economic return as reasons for this (Myers and Watts, 2015).
Pennycress is a member of the Brassicaceae family and is a new oilseed crop that has gained considerable attention as a potential cash cover crop that can be grown between summer annual commodity crops (Sindelar et al., 2017; Cubins et al., 2019). Several studies, mostly conducted in the upper Midwest USA, have demonstrated that soybean can be successfully double-cropped or relay-cropped with pennycress (Phippen and Phippen, 2012; Johnson et al., 2017; Bishop and Nelson, 2019; Ott et al., 2019; Hoerning et al., 2020). The impact of pennycress on soybean yield, when used in a double-cropping or relay-cropping scenario, has been mixed. For example, in the southern regions of the Corn Belt, pennycress had little or no effect on double-cropped soybean yields as compared with conventional monocrop soybean (Phippen and Phippen, 2012; Bishop and Nelson, 2019). However, in the northern Corn Belt, soybean yield reductions of 18–30% have been reported to be associated with double-cropping and relay-cropping with pennycress as compared with monocrop soybean (Johnson et al., 2017; Ott et al., 2019). Differences between the regions are likely due to a longer growing season in the southern Corn Belt. Regardless of the potential soybean yield drag associated with double-cropping and relay-cropping with pennycress, one thing that remains consistent is that total seed and oil yield per land area (i.e., pennycress + soybean) are generally greater than conventionally producing a single soybean crop (Cubins et al., 2019).
In the Corn Belt region, pennycress is primarily being targeted for integration into corn and soybean systems (Sindelar et al., 2017; Bishop and Nelson, 2019). However, full-season grain corn due to its long growing season presents challenges for establishing pennycress, especially in the northern Corn Belt. Generally, pennycress seed yield and oil content are maximized by planting in early- to mid-September (Dose et al., 2017). Because grain corn is typically harvested in late autumn, there is often little time to directly plant and establish pennycress before the soil freezes. A few studies have evaluated interseeding pennycress into a standing corn crop at various stages of growth (Nolan et al., 2018; Bishop and Nelson, 2019; Mohammed et al., 2020) with mixed results. For instance, Mohammed et al. (2020) demonstrated that good pennycress establishment was achievable by interseeding with a highboy device at the late stages of corn development (R4 to R6), but this did not translate into high seed yields (Patel et al., 2021), likely due to suppressed growth caused by the high amount of corn residue following harvest. More consistent establishment and seed yield results have been achieved by direct planting pennycress in early September following short-season summer crops such as spring wheat (Triticum aestivum L.) (Dose et al., 2017; Ott et al., 2019) and corn harvested for silage (Hoerning et al., 2020) where there is minimal crop residue. In the northern Corn Belt, there is a significant hectarage of small grain cereals such as spring wheat grown followed by fallow soil until the next spring. Therefore, introducing pennycress as a cash cover crop to keep the soil covered in wheat–soybean systems is needed, but the information is limited to optimize pennycress and soybean production.
The relay-cropping system, which involves interseeding soybean into standing pennycress such that their lifecycles overlap during the growing season, effectively keeps living cover on the field year-round. The environmental benefits of using this system are manifold. Weyers et al. (2019) demonstrated that autumn-sown pennycress and winter camelina (Camelina sativa L.) grown in a relay system with soybean reduced nitrate N in soil water by as much as 89% in spring as compared with conventional practices of keeping the soil fallow between summer annual crops. This has a significant implication for water quality given that soils in the Midwest Corn Belt region are most prone to N loss by leaching and runoff in the spring (Strock et al., 2004). Moreover, pennycress can reduce total suspended solids in spring runoff from snow melt and rains by as much as 75% compared with soil left fallow over the winter (Weyers et al., 2021), indicating its ability to prevent soil erosion. Pennycress also suppresses spring and early summer weeds (Johnson et al., 2015) by as much as nearly 100% (Hoerning et al., 2020) when used in a relay system with soybean, resulting in less herbicide use in the subsequent soybean crop. An abundance of pollinating insects visit pennycress when it is flowering (Eberle et al., 2015), and its flowers provide pollen and nectar resources for pollinators (Thom et al., 2016, 2018).
While growing pennycress has many positive environmental effects, pennycress seed oil and meal, like that of rape seed (Brassica napus L.), is presently high in erucic acid (C22:1) and glucosinolates, which are antinutritional and therefore not desirable for food and feed use. However, extensive work is underway to develop commercially viable pennycress genotypes that are low in glucosinolates and possess seed oil profiles conducive to food and feed uses (Chopra et al., 2020). While low glucosinolate pennycress genotypes are being developed, the near-term markets for currently available pennycress seed oil will likely be for biofuels. Pennycress seed oil has been demonstrated to be a good feedstock for making biodiesel (Moser et al., 2009), renewable aviation fuel (Fan et al., 2013), and biolubricants (Cermak et al., 2015).
Although research shows that pennycress can successfully be double-cropped and relay-cropped with soybean and other short-season summer annual crops (Cubins et al., 2019; Moore et al., 2020), little work has focused on improving agronomic management practices for such systems. The present study was designed to address optimizing the timing for relay sowing of soybean into pennycress and explored the effect of soybean maturity. We hypothesized that relaying soybean into pennycress as late as possible during its development (e.g., during bolting or initiation of reproduction) may improve soybean yield by reducing the amount of time the two crops overlap. We also hypothesized that using a longer maturing soybean than normally used for the region might improve productivity by allowing soybean to remain in vegetative growth longer during and after the overlap period. The overall goal of the study was to improve relay-crop soybean yields and minimize yield drag while simultaneously maintaining high pennycress seed yields. The objectives of the study were to determine the effects of soybean interseeding (relay seeding) date and maturity group on pennycress and soybean growth performance, seed yield, and seed qualities.
The study was done over two growing seasons (2015–2016 and 2016–2017) at the USDA-ARS Swan Lake Research Farm near Morris, MN, USA, located at 45°40′N, 95°48′W, and 345 m a.s.l. The soil at the experiment site was predominantly a Barnes loam (fine-loamy, mixed, superactive, and frigid Calcic Hapludolls). The long-term (over the last 30 years) average annual air temperature at the location is 5.7°C, and the long-term average yearly precipitation is 670 mm.
The experimental design was a randomized complete block with a split-plot arrangement. The main plots (9.1 m by 9.1 m) consisted of three soybean sowing dates based on the growth stage of pennycress, which were SD1 (rosette stage), SD2 (bolting or stem elongation stage), and SD3 (initial flowering stage). The subplots, which were 3 m by 9.1 m in size, consisted of three different soybean maturity groups representing early (MG0.2), standard (MG1.1), and late (MG1.7) cultivars for the region. The three soybean cultivars used in both years were from CROPLAN Genetics and were R2T0200 (early), R2C1100 (standard), and R2C1750 (late). The experimental design included three monocrop soybean check treatments that involved no-till sowing of MG0.2, MG1.1, and MG1.7 all at SD2 after winter fallow (no pennycress). Among these check treatments, soybean MG1.1 sown at SD2 was designated as conventional practice (CP), and this is the standard soybean maturity and seeding date for the region considered as normal (i.e., conventional). The CP was used in a planned orthogonal contrast analysis with the pennycress-relayed soybean treatments. The same CP monocrop soybean check treatment was used for contrast analysis in a companion study (Mohammed et al., 2022) for relay-cropping soybean with winter camelina.
Pennycress accession MN106 used for the study originated from a collection made of a natural wild population near Coates, Minnesota, USA. Pennycress was no-till sown with an InterSeeder drill (InterSeeder Technologies, Woodward, PA) into spring wheat stubble (i.e., previous crop) at a seeding rate of 9 kg ha−1 on 19 cm spaced rows leaving every fourth row unseeded (i.e., “skip row”) for relay seeding soybean the following spring. The day before sowing pennycress, 1.12 kg a.i. ha−1 of trifluralin (α, α, α-trifluoro-2,6-dinitro-N,N, -dipropyl-p-toluidine) was applied and lightly incorporated with one pass of a no-till drill for weed control. The pennycress was sown on 10 September 2015 (1st season) and 13 September 2016 (2nd season). The following spring, pennycress plots were broadcast fertilized at a rate of 78–34–34 kg N-P-K ha−1 on 4 April 2016 and 11 April 2017 using urea, diammonium phosphate, and potassium chloride.
Relayed and monocrop soybeans were all sown at a rate of 432,000 seeds ha−1 on 76 cm row spacing using a John Deere MaxEmerge seeder (Model 1730, Moline, IL). The relayed soybean was sown in the skip rows (76 cm row spacing), and all plots contained four rows of soybean. A diagram of the row spacing scheme used is shown by Mohammed et al. (2022). The first soybean sowing date (SD1) was 18 April 2016 and 23 April 2017, SD2 was sown on 5 May in both years, and SD3 was sown on 15 May in both years. No fertilizer was applied to either relayed or monocrop soybean. Weeds were controlled by applying glyphosate [N-(phosphonomethyl)glycine] at 1.3 kg a.i. ha−1 to all plots containing soybean on 28 June 2016 (following the pennycress harvest), and another application at the same rate was made on 1 August. In 2017, the same rate of glyphosate was applied to monocrop soybean on 7 June and applied on 7 July to all relayed soybean for weed control.
At each relay soybean sowing date (SD1, 2, and 3), the height of pennycress was measured from three randomly chosen plants from each plot and averaged. At pennycress harvest, the heights of both pennycress and soybean were measured on six randomly chosen plants in each of the relay and monocrop treatment plots. Pennycress was harvested when at least 90% of its silicles were yellowish-brown in color and seeds were black indicating full maturity (Cubins et al., 2022). Pennycress seeds were harvested with a plot combine (Hege 160, Waldenburg, Germany) on 20 June 2016 and 19 June 2017 from the center of the plot (1.5 m wide), and the plot length was measured to calculate the net plot area. For the relay treatments, this consisted of straddling two rows of soybean to harvest six rows of pennycress, similar to what (Mohammed et al., 2022) have described for winter camelina relay-cropped with soybean. Seeds were dried at 65°C to constant weight before screen cleaning to remove debris. Pennycress seed yields were adjusted to 100 g kg−1 moisture.
At the R7 growth stage prior to full maturity, six soybean plants were randomly sampled from all plots containing soybean (controls and relay treatments) and brought back to the lab where height, node, and pod numbers per plant were measured. At soybean harvest, the number of plants was measured in 1 m of the row from either of the two center rows of each plot to determine plant density. Soybean was combined-harvested (Hege 160, Waldenburg, Germany) for grain at full maturity (R8) by taking the center two rows, and the exact plot length was measured to determine the harvest area for both monocrop and relay-crop treatments. Soybean harvest date varied by year and treatment. In 2016, all (monocrop and relay-crop) MG0.2 soybeans were harvested on 21 September, all MG1.1 soybeans were harvested on 29 September, and all MG1.7 soybeans were harvested on 13 October. In 2017, all monocrop and relay-crop soybeans were harvested on 11 October except for SD3 relayed MG1.7 soybean, which was harvested on 18 October. The grain was dried to constant weight at 65°C and screen cleaned for yield determination. Soybean grain yields were adjusted to 130 g kg−1 moisture.
Weather variables were measured and recorded at an automated weather station located at the experiment site. Daily average air temperature (2-m height) and daily precipitation were used for determining mean monthly temperature and accumulated precipitation (Figure 1). The long-term average (LTA) temperature and precipitation were based on data recorded between 1987 and 2017 (Figure 1).
Figure 1. Average monthly air temperature (A) and monthly cumulative precipitation (B) from 2015 to 2017 including their long-term (30 years) averages (gray bars) at the study site.
The seed oil content of pennycress and soybean was measured by pulsed nuclear magnetic resonance (pNMR) using a Minispec mq10 (Bruker, The Woodlands, TX). Harvested seed from each plot, 5 g for pennycress and 6 g for soybean, was measured by pNMR as previously described (Gesch et al., 2014) after calibrating the instrument independently with pure pennycress and soybean oil, respectively. In brief, clean seed samples were dried for 4 h at 130°C and cooled in a desiccator for 15 min before measuring oil content. After measuring oil, the seed samples were ground to a fine powder in a Wiley Mill, and the total percent N was measured by combustion analysis using a LECO CN828 (LECO Corp., St. Joseph, MI). Crude protein content was estimated by multiplying percent N by 6.25 (Mariotti et al., 2008).
The MIXED procedure of SAS 9.4 (SAS Institute, Cary, NC) was used for data analysis (SAS Institute, 2014). The effect of year on pennycress seed yield was significant; thus, data were analyzed by year using replication as a random effect and sowing date (SD), soybean maturity group (MG), and their interaction (SD × MG) as fixed effects. However, pennycress and soybean plant heights at pennycress harvest did not differ by year and were therefore combined across years with year and replication as random effects and sowing date (SD), soybean maturity group (MG), and their interaction (SD × MG) as fixed effects. Soybean seed yields and plant attributes also did not differ by year, and data were combined across years using year and replication as random effects and sowing date (SD), soybean maturity group (MG), and their interaction (SD x MG) as fixed effects. When ANOVA showed significant treatment effects (P ≤ 0.05), LSD at α = 0.05 was used to differentiate treatment means. Planned orthogonal contrast analysis was performed with SAS to compare the monocrop conventional practice (CP) with the relay treatments, and the results were declared significant when P-values were < 0.05.
From 2015 to 2017, monthly average air temperatures tended to be greater between September and March than the LTA except for February in 2015, which was lower, and November and December of 2017, which were on par with the LTA (Figure 1). Between April and August of all 3 years, air temperatures were generally similar to the LTA. Precipitation distribution varied widely among years with considerably greater amounts of rainfall than normal in May 2015, July 2016, and August 2017 (Figure 1). The total accumulated precipitation in 2016 and 2017, however, was only 12 and 15 mm greater than the LTA (670 mm total), while 2015 was drier, with a total accumulation of 111 mm less than the LTA. It is also important to note that in September and October of 2015, during pennycress sowing and establishment, precipitation was low with greater temperatures than normal, making for quite dry conditions.
Pennycress seed yield across relayed treatments differed considerably between years, and there was a relay sowing date by year interaction (Table 1). Across all relay treatments, pennycress seed yield averaged 352 kg ha−1 in 2016 and 823 kg ha−1 in 2017. In 2017, pennycress seed yield was greater for SD2 than SD3, but this difference was not observed in 2016 (Table 2).
Table 1. Analysis of variance table showing seeding date (SD), soybean maturity group (MG), year, and their interactions on pennycress seed yield and oil content in the relay-cropped treatments, and contrast analysis of monocrop pennycress (MPC) with pennycress relay-sown with soybean (RPC) in 2016 and 2017.
In 2016, pennycress seed yields in the relay treatments were statistically the same as the monocrop control (i.e., no soybean relayed into it) (Table 1). However, in 2017, monocrop pennycress yielded 1,185 kg ha−1, which was generally greater than the seed yield of pennycress from relayed treatments (823 kg ha−1). The only exception in 2017 was for the SD2 MG1.7 relay treatment where the average pennycress yield was 1,071 kg ha−1 and not significantly different than the monocrop control.
Pennycress oil content was quite stable across the years and was not impacted across relayed soybean treatments (Table 1). In 2016, oil content across all relay treatments averaged 337 g kg−1, while in 2017, it was 336 g kg−1. However, in both years of the study, the oil content of monocrop pennycress was slightly less but significantly different than that of plants in the relay treatments (Table 1), averaging 325 g kg−1 in 2016 and 330 g kg−1 in 2017. The average oil content between relayed and monocrop treatments over both years was relatively small (0.9%) and was not practically significant.
Over both years of the study, the height of pennycress plants, at the time soybean was relay-sown, averaged 11 ± 3.0 cm StdDev at SD1, 19 ± 8.6 cm at SD2, and 46 ± 14.8 cm at SD3. At pennycress harvest in the relay treatments, soybean MG but not SD affected pennycress height (P < 0.05), and there was no interaction. Pennycress plants were on average 2.9 cm taller in plots relayed with the MG1.7 soybean than those relay-sown with the MG0.2 cultivar (Table 3). For the relayed soybean plants, height at pennycress harvest was affected by SD (P < 0.05) but not MG. For soybean in SD3, plants were 5.3 cm and 6.1 cm shorter than the soybean in SD1 and SD2, respectively, at the time pennycress was harvested (Table 3). For comparison, at the time of pennycress harvest, the average height of monocrop soybean planted at SD2 was 20.2, 17.6, and 16.7 cm for the MG0.2, MG1.1, and MG1.7 cultivars, respectively. Except for the MG0.2 cultivar, the relayed MG1.1 and 1.7 soybeans were slightly taller (about 3 cm) than their monocrop counterpart at the pennycress harvest.
Table 3. Pennycress and soybean plant heights in the relay treatments of sowing date (SD) and soybean maturity group (MG) at the time of pennycress harvest for data combined over years (2016 and 2017).
The seed yield of relayed soybean was affected by SD and MG, while seed oil and protein content were only influenced by MG (Table 4). Seed yield was lowest for SD1 and did not differ between SD2 and SD3 (Table 5). Both MG1.1 and 1.7 relayed soybeans yielded greater than MG0.2, but MG1.7 and MG1.1 had statistically similar yield. Seed oil content was greater for the MG1.1 and 1.7 soybeans than the MG0.2 cultivar, but the earlier maturing MG0.2 had greater protein content (Table 5).
Table 4. Analysis of variance table showing the effects of sowing date (SD), soybean maturity group (MG), and their interaction (SD × MG) on relayed soybean plant and seed attributes for data combined over years (2016 and 2017).
Table 5. Mean plant and seed attributes for the soybean maturity group (MG) cultivars at different sowing dates (SD) in a relay system with pennycress for data combined over years (2016 and 2017).
Late season relayed soybean plant height (taken at R7) only differed by MG (Table 4), with the MG1.7 being the tallest and the MG0.2 being the shortest cultivar (Table 5). Relayed soybean node, branch, and pod numbers per plant differed by SD and MG (Table 4). All these yield components were greatest in SD1, and branches per plant continued to decline between SD1 and SD3 (Table 5). Similar to height, biomass per plant and the yield components of node, branch, and pod numbers were all lowest for the MG0.2 cultivar (Table 5). Although node, pod numbers, and biomass per plant did not differ between the MG1.1 and 1.7 soybeans, the branch number was slightly greater for the MG1.1 cultivar. Biomass per plant of the relayed MG0.2 soybean was 58% lower than the average of the relayed MG1.1 and 1.7 soybeans (Table 5).
Relay sowing date (SD) and soybean MG affected soybean plant population density (Table 4). Relay sowing at SD3 favored greater plant density (Table 5). Across cultivars, plant density at SD1 was 13% lower than for SD3 at soybean harvest. Both the MG0.2 and 1.1 relayed soybeans gave similar plant densities at harvest, but the MG1.7 cultivar was on average 8% greater.
Compared with the conventional practice (CP) of winter fallow (no pennycress) followed by monocrop MG1.1 soybean sown at an average time of early May (SD2), the relay-cropped soybean yields were generally lower (Table 6). The yield reduction for relayed soybean ranged from as high as 38% (SD1 MG0.2) to as low as 10% (SD2 MG1.7). As shown by the contrast analysis (Table 6), the seed yield of the relayed SD2 MG1.7 was not statistically different from the CP treatment. Seed oil and protein content did not differ between the CP and relayed soybean. However, generally, the oil content was lower and protein content greater for the relayed MG0.2 cultivar than the CP soybean. Soybean plant height and biomass greatly differed between CP and relayed soybean with CP plants always taller and almost always heavier than the relayed soybeans, except for the SD1 MG1.1 treatment (Table 6). Node number per plant differed between CP and relayed soybean, but primarily because node numbers were consistently less for the relayed MG0.2 soybean but were not different from the CP treatment for the relayed MG1.1 and 1.7 cultivars. Branch and pod numbers varied among the treatments but overall were not found to differ between CP and relayed soybean (Table 6).
Table 6. Contrast analysis for CP vs. the different relay treatments and mean soybean agronomic parameters for data combined over years (2016 and 2017).
A planned contrast analysis was also done between the relayed soybean cultivars sown at SD2 and their monocrop counterparts sown on the same date (Table 7). Compared with their monocrop counterparts, relayed soybean generally had lower seed yields, except for the MG1.7, which did not significantly differ from its monocrop control. Seed quality, both oil and protein contents, did not differ between monocrop and relay soybeans (Table 7). Contrast analysis showed plant height was taller and biomass larger for monocrop soybean compared with relayed soybean. Node, branch, and pod numbers were lower for the relayed MG0.2 soybean compared with its monocrop control. However, there was generally no difference in these yield components when comparing the relayed MG1.1 and 1.7 soybeans with their monocrop counterpart (Table 7).
Table 7. Contrast analysis for the monocrop controls at SD2 vs. the relay treatments at SD2 and mean soybean agronomic parameters for data combined over years (2016 and 2017).
The overarching goal of this study was to determine whether relayed soybean yield could be improved by adjusting the sowing date and maturity of soybean without reducing pennycress yield. Regardless of relay or monocrop treatments, pennycress seed yields were much lower in 2016 than in 2017. Although pennycress plant density was not measured, pennycress stands were noticeably less dense (field observation) in 2016. It is likely that the pennycress stand was reduced due to the lack of precipitation and dry conditions during early autumn (September and October) of 2015, followed by a dry spring (March to June) in 2016, which could have resulted in poor emergence and early growth of plants. Pennycress seed germination and emergence are highly dependent on adequate soil moisture (Hazebroek and Metzger, 1990), and low seedling emergence in west central Minnesota of the USA has previously been demonstrated to be linked to low precipitation and dry soil in September and October (Royo-Esnal et al., 2015). Early to mid-September has been shown to be a near optimal time to sow pennycress in the northern Corn Belt of the USA (Dose et al., 2017). Furthermore, when sowing in early autumn, Johnson et al. (2015) have shown that the amount of precipitation during the pennycress growing season is closely associated with seed yield, increasing with increased precipitation.
Pennycress yields were not affected by relay-sowing soybean in 2016, but were in 2017, where generally yields were lower in the relayed treatments as compared with monocrop pennycress. The only exception was the SD2 MG1.7 treatment where seed yield was not different from the monocrop control. The difference was most pronounced in the SD3 treatments where the pennycress plants were initially flowering and averaged 46 cm tall at the time soybean was relay-sown. A similar response was noted in a companion study with winter camelina (Mohammed et al., 2022). However, in that study, when compared with the monocrop check, camelina seed yields were only reduced when relay sowing soybean at the initial flowering of camelina (SD3) and not at the earlier rosette or bolting stages. The decline in pennycress yield associated with relay-cropping was most likely due to damage caused by wheel traffic of equipment used for sowing soybean. In 2017, pennycress plant density was measured in the spring prior to interseeding soybean and was found to average 288 ± 96 StdDev and 261 ± 73 plants m−2 for the monocrop and relay-cropped treatments, respectively. This small difference in plant density was unlikely the reason for yield differences between the two systems. Nevertheless, the yield reduction in 2017 was the least for SD2, which averaged across all three MGs was 23% lower than the monocrop control. Previous research on relay-cropping pennycress and soybean indicated that the less time soybean remained under the pennycress canopy (i.e., lifecycles overlap), the greater its yield (Ott et al., 2019; Hoerning et al., 2020). The results of the present study indicate that soybean can be sown into pennycress at the bolting stage (SD2) to minimize pennycress yield reduction while allowing less time for the two crops to overlap than relaying at the rosette stage (SD1).
In both years of the study, relay-cropping slightly, but consistently, increased pennycress seed oil content by an average of about 9 g kg−1 compared with monocrop pennycress. Although this difference (~1%) was statistically significant, it was not enough to be of practical agronomic significance. A similar response was reported by Mohammed et al. (2022) for winter camelina when it was relayed-cropped with soybean and was most likely due to less available soil N in the relay system caused by competition between the two crops for N uptake during their overlap period. Lower available N for oilseed crops is often associated with greater seed oil synthesis (Gehringer et al., 2006).
A vital aspect of the relay system is being able to harvest pennycress without damaging soybean. When pennycress was harvested, the height difference between pennycress and soybean in the relay treatments was large enough to keep the cutting bar of the combine above the soybean without severing the soybean and causing damage.
Relayed soybean yield was impacted by the sowing date. Across cultivars, soybean relayed at SD1 yielded 13 and 8% less than those sown at SD2 and SD3, respectively. However, the number of nodes, branches, and pods was greater for plants sown at SD1 than at SD2 and SD3. Generally, higher soybean yields are correlated with a greater number of yield components per plant (Akhter and Sneller, 1996). Moreover, an increase in yield components is often associated with lower soybean plant density, which can compensate for yield in lower populations (Carpenter and Board, 1997). However, in the present study, the small but significant increase in nodes, branches, and pods in the SD1 treatment did not compensate in yield for the lower plant density. Although seed size, which was not measured, cannot be ruled out, the most likely reason for the lower yield of SD1 soybean was because of lower plant density at harvest.
Soil moisture availability and light interception are critical factors for the survival and development of interseeded soybean in a relay system (Duncan and Schapaugh, 1997; Gesch and Johnson, 2015; Ott et al., 2019). The reduction in plant density and lower yields of SD1 relayed soybean most likely resulted from extended competition between the two crops (i.e., pennycress and soybean) for soil moisture, nutrients, and light, but especially moisture. In 2016, the precipitation was below normal for May and June (Figure 1) when relayed soybean was emerging and vegetatively developing under the pennycress canopy, which likely intensified competition for available water. Furthermore, it has been demonstrated that the longer soybean remains under a canopy in a relay system, the more intensified competition is for resources, which often leads to reduced soybean plant stands and yields (Wallace et al., 1992; Duncan and Schapaugh, 1997). In the present study, from sowing to pennycress harvest, relayed soybean was under the pennycress canopy for 57 to 63 d in SD1, 45 to 46 d for SD2, and 35 to 36 d for SD3. In a related but independent study where soybean was relayed into pennycress, Hoerning et al. (2020) reported that soybean plant population density and seed yield were greatly reduced compared with monocrop soybean at Morris, Minnesota, in 2016. However, in the same study, plant stands were unaffected by relaying at two other Minnesota sites in the same year (Lamberton and Rosemount). Hoerning et al. (2020) concluded that early season drought was the main cause of the soybean population density and yield reductions. However, in that study, soybean was relayed into broadcast solid seeded pennycress rather than using a direct-drilled skip-row pattern like in the present study. Previous research on relay intercropping of soybean with small grain cereals indicates that skip-row patterns tend to reduce interplant competition and consistently result in greater soybean yields (Duncan et al., 1990; Duncan and Schapaugh, 1997).
Whether relayed or grown as a monocrop, both the MG1.1 and MG1.7 soybeans yielded the MG0.2 cultivar (Tables 5, 7). Regardless of the relay sowing date, the MG0.2 soybean had fewer yield components and plants tended to be shorter and have less biomass than either of the longer maturity soybean cultivars. This result is not surprising given that generally earlier maturing soybean for a region tend to be lower yielding if all other management factors (e.g., plant population and row spacing) are equal (Edwards and Purcell, 2005). However, our study is one of the first to explore the effect of soybean maturity in a relay system with pennycress. In a companion study where the same soybean cultivars were relayed into winter camelina, Mohammed et al. (2022) showed that the longer maturing MG1.7 gave a clear advantage over the commonly used MG1.1 cultivar for the study region. In that study, it was postulated that the advantage of the longer maturity soybean was because of its extended vegetative growth prior to and after removal (i.e., harvest) of the camelina. In the present study, however, there was no clear advantage to relaying the MG1.1 or 1.7 cultivar in pennycress as their yields did not significantly differ when averaged over sowing dates. The difference in soybean cultivar response between the two different winter oilseed relay systems might be related to plant architecture. Ott et al. (2019) demonstrated that when relayed with soybean, pennycress allowed less light penetration to soybean than winter camelina. Therefore, this might have influenced the result of no difference in productivity between the MG1.1 and 1.7 cultivars in the present study with pennycress, whereas Mohammed et al. (2022) reported a difference when relayed into winter camelina. Another potential explanation is that pennycress was harvested about a week earlier than the study of Mohammed et al. (2022). Therefore, the overlap of pennycress and soybean was less than with winter camelina, and the period of soybean vegetative growth during that time may have been less of a factor than with the camelina relay system.
Compared with monocrop soybean, relayed soybean did not differ in seed oil and protein contents, which has important implications given that soybean is the most important vegetable protein source in the world. Soybean yield drag in a relay system is common and mainly due to interplant competition during the growth overlap of the crops (Wallace et al., 1992), which becomes a greater factor the longer they overlap (McBroom et al., 1981). However, relay-cropping, especially in the northern and central regions of the USA, has the advantage over double-cropping in that soybean is seeded earlier at a more normal time, thus allowing it a longer growing season (Nelson et al., 2011). Gesch et al. (2014) demonstrated that in a winter oilseed relay system, earlier planting of soybean greatly reduced soybean yield loss associated with late sowing in double-crop systems.
In the present study, relayed soybean yields were generally lower than that of the conventional practice (CP), but yield loss was considerably less and not significantly different than CP when using the longer maturity soybean (MG1.7) sown in early May (SD2) when pennycress was at the bolting stage. Studies have shown that although there is a yield drag of soybean in the winter oilseed relay system, the combined seed and oil yield of the oilseed and soybean of the relay system are often greater than growing a sole crop of soybean (Gesch et al., 2014; Johnson et al., 2017; Ott et al., 2019; Mohammed et al., 2022). More research is needed to select soybean cultivars with better tolerance to shading that may perform better in the relay system. Nevertheless, there are several ecosystem service benefits to consider when using a continuous cover cropping system such as relay-cropping pennycress and soybean. These services include reducing soil erosion and sequestering soil N (Weyers et al., 2019, 2021), suppressing herbicide-resistant weeds (Hoerning et al., 2020), provisioning pollinators (Eberle et al., 2015), reducing global warming potential (Berti et al., 2017; Cecchin et al., 2021), and greatly increasing agricultural land use efficiency (Mohammed et al., 2022) as compared with conventional corn and soybean systems in the upper Midwest USA. The economics of the pennycress-soybean relay system remain to be addressed and will highly depend on the development of robust markets for pennycress seed oil and meal by-product.
The newly developed system for relay-cropping soybean with pennycress used as a cash cover crop offers a way to keep living cover on the landscape for nearly the entire year. The incorporation of pennycress into agricultural systems will offer new economic opportunities and environmental benefits.
This study demonstrates the importance of managing soybean cultivar selection and sowing date for a given region to optimize the productivity of this unique oilseed relay-cropping system. Our first hypothesis that relay interseeding soybean as late as possible into pennycress to reduce their lifecycle overlap was partially correct in that the best time to relay soybean was around the time pennycress was at its bolting stage. Although relaying soybean reduced pennycress yields 1 out of 2 years during the study, the reduction was least at the bolting stage (SD2). Our second hypothesis that the longer maturity soybean (MG1.7) would be most productive in the relay system was not fully correct. Both the common maturity soybean (MG1.1) and the longer maturing cultivar (MG1.7) across SDs gave similar results in the relay system but were greater yielding than the early soybean (MG0.2). Nevertheless, the seed yield of the MG1.7 soybean relay interseeded at pennycress bolting was not significantly different from the conventional soybean practice (CP). Importantly, seed oil and protein contents did not differ between relayed soybean and their monocrop counterparts. Furthermore, as compared with CP soybean, the relayed MG1.1 and MG1.7 cultivars did not differ in the number of yield components per plant, although relayed soybean tended to be shorter with less biomass. Additional research is needed to further identify soybean genotypes best suited for the system that reduces yield drag. Also, further research is needed to improve other management factors such as row spacing and plant populations (i.e., sowing geometry) of pennycress and soybean in the relay system.
The original contributions presented in the study are included in the article/supplementary material, further inquiries can be directed to the corresponding author.
RG: conceptualization, methodology, investigation, formal analysis, writing original draft, supervision, and project administration. YM: formal analysis and writing-review and editing. HM: methodology, investigation, and writing-review and editing. All authors contributed to the article and approved the submitted version.
This research was fully supported by the U.S. Department of Agriculture.
The authors would like to thank Joe Boots, Chuck Hennen, Scott Larson, and Jay Hanson for their help in field plot management, data collection, sample processing, and testing in the lab. The findings and conclusion in this publication are those of the authors and should not be construed to represent any official USDA or U.S. Government determination or policy. The mention of trade names or commercial products in this publication is solely for the purpose of providing specific information and does not imply recommendation or endorsement by the U.S. Department of Agriculture. The USDA is an equal opportunity provider and employer.
HM was employed by WinField United, Land O'Lakes Inc.
The remaining authors declare that the research was conducted in the absence of any commercial or financial relationships that could be construed as a potential conflict of interest.
All claims expressed in this article are solely those of the authors and do not necessarily represent those of their affiliated organizations, or those of the publisher, the editors and the reviewers. Any product that may be evaluated in this article, or claim that may be made by its manufacturer, is not guaranteed or endorsed by the publisher.
CP, conventional practice; MG, maturity group; SSB, sole soybean; RSB, relayed soybean; SD, seeding date.
Aguilar, J., Gramig, G. G., Hendrickson, J. R., Archer, D. W., Forcella, F., and Liebig, M. A. (2015). Crop species diversity changes in the United States: 1978-2012. PLoS ONE 10, e0136580. doi: 10.1371/journal.pone.0136580
Akhter, M., and Sneller, C. H. (1996). Yield and yield components of early maturing soybean genotypes in the Mid-South. Crop Sci. 36, 877–882. doi: 10.2135/cropsci1996.0011183X0036000400010x
Berti, M. B., Johnson, B. L., Ripplinger, D., Gesch, R. W., and Aponte, A. (2017). Environmental impact assessment of double- and relay-cropping with winter camelina in the northern Great Plains, USA. Agric. Syst. 156, 1–12. doi: 10.1016/j.agsy.2017.05.012
Bishop, L., and Nelson, K. A. (2019). Field pennycress seeding date and corn herbicide management effects on corn, pennycress, and soybean production. Agron. J. 111, 257–263. doi: 10.2134/agronj2018.03.0156
Carpenter, A. C., and Board, J. E. (1997). Branch yield components controlling soybean yield stability across plant populations. Crop Sci. 37, 885–891. doi: 10.2135/cropsci1997.0011183X003700030031x
Cecchin, A., Pourhashem, G., Gesch, R. W., Lenssen, A. W., Mohammed, Y. A., Patel, S., et al. (2021). Environmental trade-offs of relay-cropping winter cover crops with soybean in the maize-soybean rotation. Agric. Syst. 189, 103062. doi: 10.1016/j.agsy.2021.103062
Cermak, S. C., Durham, A. L., Isbell, T. A., Evangelista, R. L., and Murray, R. E. (2015). Synthesis and physical properties of pennycress estolides and esters. Ind. Crops Prod. 67, 179–184. doi: 10.1016/j.indcrop.2015.01.050
Chopra, R., Johnson, E. B., Emenecker, R., Cahoon, E. B., et al. (2020). Identification and stacking of crucial traits required for the domestication of pennycress. Nat. Food 1, 84–91. doi: 10.1038/s43016-019-0007-z
Cubins, J. A., Wells, M. S., Frels, K., Ott, M. A., Forcella, F., Johnson, G. A., et al. (2019). Management of pennycress as a winter annual cash cover crop: A review. Agron. Sustain. Dev. 39, 1–11. doi: 10.1007/s13593-019-0592-0
Cubins, J. A., Wells, S. S., Walia, M. K., Wyse, D. L., Becker, R., Forcella, F., et al. (2022). Harvest attributes and seed quality predict physiological maturity of pennycress. Ind. Crop. Prod. 176, 114355 doi: 10.1016/j.indcrop.2021.114355
Dose, H. L., Eberle, C. A., Forcella, F., and Gesch, R. W. (2017). Early planting dates maximize winter annual field pennycress (Thlaspi arvense L.) yield and oil content. Ind. Crop. Prod. 97, 477–483. doi: 10.1016/j.indcrop.2016.12.039
Duncan, S. R., and Schapaugh, W. T. (1997). Relay-intercropping soybean in different water regimes, planting patterns, and winter wheat cultivars. J. Prod. Agric. 10, 123–129. doi: 10.2134/jpa1997.0123
Duncan, S. R., Schapaugh, W. T., and Shroyer, J. P. (1990). Relay intercropping soybeans into wheat in Kansas. J. Prod. Agric. 3, 576–581. doi: 10.2134/jpa1990.0576
Eberle, C. A., Thom, M. D., Nemec, K. T., Forcella, F., Lundgren, J. G., Gesch, R. W., et al. (2015). Using pennycress, camelina, and canola cash cover crops to provision pollinators. Ind. Crops Prod. 75, 20–25. doi: 10.1016/j.indcrop.2015.06.026
Edwards, J. T., and Purcell, L. C. (2005). Soybean yield and biomass responses to increasing plant population among diverse maturity groups: I. Agronomic characteristics. Crop Sci. 45, 1770–1777. doi: 10.2135/cropsci2004.0564
Fan, J., Shonnard, D. R., Kalnes, T. N., Johnsen, P. B., and Rao, S. (2013). A life cycle assessment of pennycress (Thlaspi arvense L.) -derived jet fuel and diesel. Biomass Bioenerg. 55, 87–100. doi: 10.1016/j.biombioe.2012.12.040
Gehringer, A., Friedt, W., Lühs, W., and Snowdon, R. J. (2006). Genetic mapping of agronomic traits in false flax (Camelina sativa subsp. sativa). Genome 49, 1555–1563. doi: 10.1139/g06-117
Gesch, R. W., Archer, D. W., and Berti, M. T. (2014). Dual cropping winter camelina with soybean in the Northern Corn Belt. Agron. J. 106, 1735–1745. doi: 10.2134/agronj14.0215
Gesch, R. W., and Johnson, J. M.-F. (2015). Water use in camelina-soybean dual cropping systems. Agron. J. 107, 1098–1104. doi: 10.2134/agronj14.0626
Hazebroek, J. P., and Metzger, J. D. (1990). Environmental control of seed germination in Thlaspi arvense (Cruciferae). Amer. J. Bot. 77, 945–953. doi: 10.1002/j.1537-2197.1990.tb15189.x
Heaton, E. A., Schulte, L. A., Berti, M., Langeveld, H., Zegada-Lizarazu, W., Parrish, D., et al. (2013). Managing a second-generation crop portfolio through sustainable intensification: Examples from the USA and the EU. Biofpr. 7, 702–714. doi: 10.1002/bbb.1429
Hoerning, C., Wells, M. S., Gesch, R., Forcella, F., and Wyse, D. (2020). Yield tradeoffs and weed suppression in a winter annual oilseed relay-cropping system. Agron. J. 112, 2485–2495. doi: 10.1002/agj2.20160
Johnson, G. A., Kantar, M. B., Betts, K. J., and Wyse, D. L. (2015). Field pennycress production and weed control in a double crop system with soybean in Minnesota. Agron. J. 107, 532–540. doi: 10.2134/agronj14.0292
Johnson, G. A., Wells, M. S., Anderson, K., Gesch, R. W., Forcella, F., and Wyse, D. L. (2017). Yield tradeoffs and nitrogen between pennycress, camelina, and soybean in relay- and double-crop systems. Agron. J. 109, 2128–2135. doi: 10.2134/agronj2017.02.0065
Kladivko, E. J., Kaspar, T. C, Jaynes, D. B., Malone, R. W., Singer, J., Morin, X.K., et al. (2014). Cover crops in the Upper Midwestern United States: Potential adoption and reduction of nitrate leaching in the Mississippi River Basin. J. Soil Water Conserv. 69, 279–291. doi: 10.2489/jswc.69.4.279
Mariotti, F., Tome, D., and Mirand, P. P. (2008). Converting nitrogen into protein – beyond 6.25 and Jones' factors. Crit. Rev. Food Sci. 48, 177–184. doi: 10.1080/10408390701279749
McBroom, R., Hadley, H., Brown, C., and Johnson, R. (1981). Evaluation of soybean cultivars in monoculture and relay intercropping systems. Crop Sci. 21, 673–676. doi: 10.2135/cropsci1981.0011183X002100050010x
Mohammed, Y. A., Gesch, R. W., Matthees, H. L., and Wells, S. S. (2022). Maturity selection but not sowing date enhances soybean productivity and land use in a winter camelina-soybean relay system. Food Energy Secur. 11, e346. doi: 10.1002/fes3.346
Mohammed, Y. A., Matthees, H. L., Gesch, R. W., Patel, S., Forcella, F., Aasand, K., et al. (2020). Establishing winter annual cover crops by interseeding into maize and soybean. Agron. J. 112, 719–732. doi: 10.1002/agj2.20062
Moore, S. A., Wells, M. S., Gesch, R. W., Becker, R. L., Rosen, C. J., and Wilson, M. L. (2020). Pennycress as a cash cover-crop: Improving the sustainability of sweet corn production systems. Agron. 10, 614. doi: 10.3390/agronomy10050614
Mortensen, D. A., Egan, J. F., Maxwell, B. D., Ryan, M. R., and Smith, R. G. (2012). Navigating a critical juncture for sustainable weed management. BioSci. 62, 75–84. doi: 10.1525/bio.2012.62.1.12
Moser, B. R., Knothe, G., Vaughn, S. F., and Isbell, T. A. (2009). Production and evaluation of biodiesel from Field Pennycress (Thlaspi arvense L.) oil. Energy Fuels 23, 4149–4155. doi: 10.1021/ef900337g
Myers, R., and Watts, C. (2015). Progress and perspectives with cover crops: Interpreting three years of farmer surveys on cover crops. J. Soil Water Conserv. 70, 125A-129A. doi: 10.2489/jswc.70.6.125A
Nelson, K. A., Massey, R. E., and Burdick, B. A. (2011). Harvest aid application timing affects wheat and relay intercropped soybean yield. Agron. J. 103, 851–855. doi: 10.2134/agronj2010.0384
Nolan, R. L., Wells, M. S., Sheaffer, C. C., Baker, J. M., Martinson, K. L., and Coulter, J. A. (2018). Establishment and function of cover crops interseeded into corn. Crop. Sci. 58, 863–873. doi: 10.2135/cropsci2017.06.0375
Ott, M. A., Eberle, C. A., Thom, M. D., Archer, D. W., Forcella, F., Gesch, R. W., et al. (2019). Economics and agronomics of relay-cropping pennycress and camelina with soybean in Minnesota. Agron. J. 111, 1281–1292. doi: 10.2134/agronj2018.04.0277
Patel, S., Lenssen, A. W., Moore, K. J., Mohammed, Y. A., Gesch, R. W., Wells, M. S., et al. (2021). Interseeded pennycress and camelina yield and influence on row crops. Agron. J. 113, 2629–2647. doi: 10.1002/agj2.20655
Phippen, W. B., and Phippen, M. E. (2012). Soybean seed yield and quality as a response to field pennycress residue. Crop Sci. 52, 2767–2773. doi: 10.2135/cropsci2012.03.0192
Reicosky, D. C. (2015). Conservation tillage is not conservation agriculture. J. Soil Water Conserv. 70, 103A−108A. doi: 10.2489/jswc.70.5.103A
Ringquist, J., Phillips, T., Renner, B., Slides, R., Stuart, K., Baum, M., et al. (2016). Capitalizing on the Shifting Consumer Food Value Equation. London, UK: Deloitte Development LLC. 32.
Royo-Esnal, A., Necajeva, J., Torra, J., Recasens, J., and Gesch, R. W. (2015). Emergence of field pennycress (Thlaspi arvense L.): Comparison of two accessions and modelling. Ind. Crops Prod. 66, 161–169. doi: 10.1016/j.indcrop.2014.12.010
Ryan, M. R., Crew, T. E., Culman, S. W., Dehaan, L. R., Hayes, R. C., Jungers, J. M., et al. (2018). Managing for multifunctionality in perennial grain crops. BioSci. 68, 294–304. doi: 10.1093/biosci/biy014
Sindelar, A. J., Schmer, M. R., Gesch, R. W., Forcella, F., Eberle, C. A., Thom, M. D., et al. (2017). Winter oilseed production for biofuel in the US Corn Belt: opportunities and limitations. GCB Bioenergy 9, 508–524. doi: 10.1111/gcbb.12297
Strock, J. S., Porter, P. M., and Russelle, M. P. (2004). Cover cropping to reduce nitrate loss through subsurface drainage in the northern U.S. Corn Belt. J. Environ. Qual. 33, 1010–1016. doi: 10.2134/jeq2004.1010
Thom, M., Eberle, C. A., Forcella, F., Gesch, R., and Weyers, S. (2018). Specialty oilseed crops provide an abundant source of pollen for pollinators and beneficial insects. J. Appl. Entomol. 142, 211–222. doi: 10.1111/jen.12401
Thom, M. D., Eberle, C., Forcella, F., Gesch, R. W., Nemec, K. T., Lundgren, J. G., et al. (2016). Nectar production in oilseeds: food for pollinators in an agricultural landscape. Crop Sci. 56, 727–739. doi: 10.2135/cropsci2015.05.0322
Wallace, S., Whitwell, T., Palmer, J., Hood, C., and Hull, S. (1992). Growth of relay intercropped soybean. Agron. J. 84, 968–973. doi: 10.2134/agronj1992.00021962008400060012x
Weyers, S., Thom, M., Forcella, F., Eberle, C., Matthees, H., Gesch, R., et al. (2019). Reduced potential for nitrogen loss in cover crop-soybean relay systems in a cold climate. J. Environ. Qual. 48, 660–669. doi: 10.2134/jeq2018.09.0350
Keywords: cover crops, pennycress, relay-cropping, soybean, continuous cover, land use productivity
Citation: Gesch RW, Mohammed YA and Matthees HL (2023) Evaluation of soybean selection and sowing date in a continuous cover relay-cropping system with pennycress. Front. Sustain. Food Syst. 6:961099. doi: 10.3389/fsufs.2022.961099
Received: 03 June 2022; Accepted: 15 December 2022;
Published: 10 January 2023.
Edited by:
Carol Williams, University of Wisconsin-Madison, United StatesReviewed by:
Miguel Alfonso, Spanish National Research Council (CSIC), SpainCopyright © 2023 Gesch, Mohammed and Matthees. This is an open-access article distributed under the terms of the Creative Commons Attribution License (CC BY). The use, distribution or reproduction in other forums is permitted, provided the original author(s) and the copyright owner(s) are credited and that the original publication in this journal is cited, in accordance with accepted academic practice. No use, distribution or reproduction is permitted which does not comply with these terms.
*Correspondence: Russ W. Gesch, cnVzcy5nZXNjaEB1c2RhLmdvdg==
Disclaimer: All claims expressed in this article are solely those of the authors and do not necessarily represent those of their affiliated organizations, or those of the publisher, the editors and the reviewers. Any product that may be evaluated in this article or claim that may be made by its manufacturer is not guaranteed or endorsed by the publisher.
Research integrity at Frontiers
Learn more about the work of our research integrity team to safeguard the quality of each article we publish.