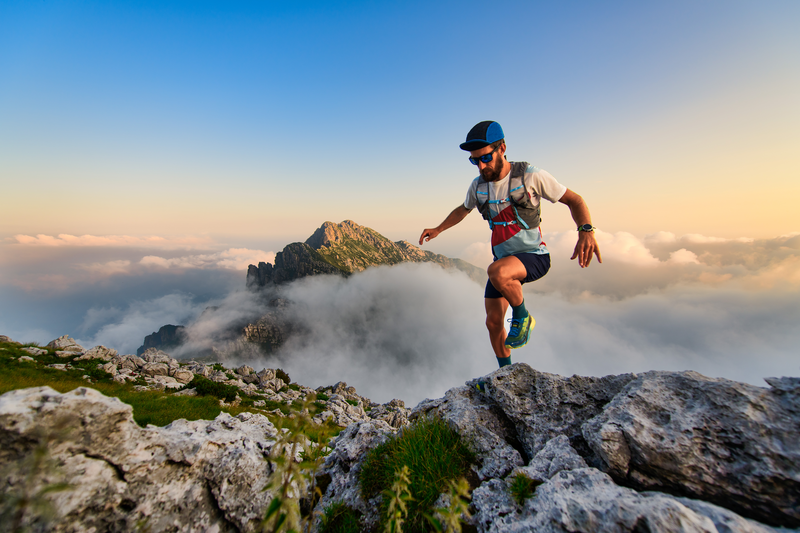
94% of researchers rate our articles as excellent or good
Learn more about the work of our research integrity team to safeguard the quality of each article we publish.
Find out more
ORIGINAL RESEARCH article
Front. Sustain. Food Syst. , 08 July 2022
Sec. Crop Biology and Sustainability
Volume 6 - 2022 | https://doi.org/10.3389/fsufs.2022.955473
This article is part of the Research Topic Plant Growth-Promoting Microorganisms for Sustainable Agricultural Production Volume II View all 5 articles
The biotechnological manipulation of phosphate-solubilizing bacteria (PSB) is gaining prominence to improve the poor phosphorus (P) availability in the soil and maintain crop yields. In this study, we investigated how Rhizobium sp. B02 inoculation influences maize crop development and whether its use reduces phosphate fertilizer rates. We conducted growth promotion assays using P fertilizer doses in two maize genotypes under greenhouse conditions. Morphometric, physiological, and productivity parameters were assessed in three phenological stages: tillering (V5), tassel (VT), and maturity (R6). Maize response was significantly influenced by both inoculation and plant genotype, showing that the plant-promoting effect of inoculation is substantially more prominent in the white endosperm than in the yellow endosperm maize genotype. The development of maize in all phenological stages was promoted by inoculation with Rhizobium sp. B02. The most significant influence of inoculation was observed on shoot dry weight, relative chlorophyll content, shoot P concentration, leaf area, photosynthetic rate, 1,000-grain weight, and grain yield. A 17% gain in grain yield, representing 20 g plant−1, was obtained by inoculation with 50% diammonium phosphate (DAP) compared with the control treatment at the same dose. The complete fertilization control was phenocopied by the white endosperm inoculated at 50% DAP in all productivity parameters. Therefore, half of the P fertilization in white endosperm was replaced by inoculation with Rhizobium sp. B02. Herein, we report the potential of a Rhizobium strain in a non-legume crop to improve P management.
Maize (Zea mays L.) is a crucial agricultural crop in food security (Tanumihardjo et al., 2020). It is one of the cereals with the most substantial production, consumption, export, and rotation worldwide (Poole et al., 2021). This crop occupies the third largest production area in Colombia, after coffee and rice (Fenalce, 2022a). The two most commercialized maize genotypes are the yellow and white endosperm, with 1,017,989 and 586,802 tons in 2020, respectively (Fenalce, 2022a). Nevertheless, the production is not self-sufficient, leading to the importation of 4,829,654 and 296,526 tons of yellow and white endosperms, respectively (Fenalce, 2022b). Other countries are experiencing this critical issue, which is becoming a challenge that crosses borders. In this sense, researchers, decision-makers, and public managers highlight the relevance of changing to sustainable production where farmers can increase maize planting, preserve biodiversity, and optimize fertilization management (Erenstein et al., 2021).
Maize productivity is severely affected by low phosphorus (P) soil availability (Morel et al., 2021). P exists in soils in organic and inorganic forms controlled by geochemical and biological processes. Its deficiency in inceptisol soil, one of the most abundant soils for maize production in Colombia, is mainly due to the strong P adsorption caused by crystalline and amorphous oxides and hydroxides of iron and aluminum (Chen et al., 2015; Vargas et al., 2019). Consequently, more than 80% of the added P in soluble mineral fertilizers becomes unavailable for plant uptake, demanding higher application rates and increasing production costs (Morel et al., 2021). Root morphology is affected, and leaf biomass and P content are reduced by P deficiency, particularly in leaves closer to the cob, limiting maize yield (Zhang et al., 2021). However, P availability is primarily affected by biological activity. Therefore, manipulating soil microorganisms is critical for developing biotechnological solutions.
Microorganisms involved in P solubilization and mineralization of its inorganic (IP) and organic forms (OP) have the potential to reduce P loss from cropping systems. This group of microorganisms includes fungi, actinomycetes, algae, and bacteria, the most predominant and so-called phosphate-solubilizing bacteria (PSB). PSB belongs to a functional subgroup of plant growth-promoting bacteria (PGPB) (Alori et al., 2017). Lowering soil pH is one of the most significant metabolic mechanisms of PSB to solubilize IP through the production of organic acids or the release of protons. The most-reported low molecular weight organic acids are formic, acetic, propionic, lactic, glycolic, fumaric, and succinic acids. Chelation process is another solubilization mechanism that acts through the hydroxy and carboxyl groups of the acids produced by PSB on the cations attached to the insoluble phosphate (Bargaz et al., 2021). At the same time, the synthesis of phosphomonoesterases, phytases, phosphoesterases, phosphodiesterases, and phospholipases mineralize OP by hydrolysis of organic forms of phosphate compounds (Richardson and Simpson, 2011).
Although there is much to explore in the complex PSB–soil–plant system (Raymond et al., 2021), it has been found in recent research that crop plant morphometric, physiological, and productivity parameters are promoted by PSB inoculation. In maize, shoot length, root dry weight, total P uptake, and grain yield have been increased by the inoculation of Pantoea cypripedii (PSB-3) and Pseudomonas plecoglossicida (PSB-5) (Gurdeep and Reddy, 2015). Azospirillum brasilense is another PSB that exerts beneficial effects on maize, representing increases (~27%) in grain yield (Li et al., 2017). Reports of the Rhizobium genus as a PSB in maize are scarce. The combined application of Rhizobium strains and biochar caused significant improvement in the growth and physiological attributes of maize plants (Ahmad et al., 2015). Rhizobium endophytes strains have been isolated from maize roots, which shows the potential role of this genus in rhizospheric metabolic activity and their interaction with non-legume plants (Gao et al., 2017). Surprisingly, most PSB studies in maize have been carried out on a single plant genotype, especially on a few phenological phases, mainly the early and harvest phases. This opens the need to deepen the interaction between PSB inoculation and maize genotype responses.
Rhizobium sp. B02 is a PSB with multiple plant growth-promoting activities, such as symbiotic nitrogen fixation, solubilization of several phosphate sources, indolic compounds synthesis, and siderophore production. This strain has been investigated for its ability to significantly promote growth in cotton crops in low P soil (Romero-Perdomo et al., 2021). Rhizobium sp. B02 has also been studied as an inoculant to increase the biomass of perennial ryegrass and red clover under P-deficient conditions (Santos-Torres et al., 2021). Based on the potential of the B02 strain observed in our previous studies, we conducted this investigation to ascertain whether maize growth of two genotypes under reduced rates of P fertilizer may be promoted by Rhizobium sp. B02 inoculation, assessing the response over three phenological stages. We proposed two hypotheses: first, different plant responses in maize genotypes are triggered by the maize growth promotion of this strain, showing some specificity. Second, the inoculation with Rhizobium sp. B02 allows optimization of the phosphate fertilizer applied.
The PSB strain Rhizobium sp. B02, with the accession number SAMN16969919 of its complete genome in the NCBI database, was used in this study. This microorganism was provided by the Colombian Corporation for Agricultural Research (AGROSAVIA) microorganism collection. The strain was isolated from nodules of Vigna unguiculata (Mendoza and Bonilla, 2014). The strain was selected for its ability, together with the application of rock phosphate, to improve growth, P nutrition, and physiological parameters on several crops in soil with low P availability (Romero-Perdomo et al., 2021; Santos-Torres et al., 2021). For all assays, bacterial cells were obtained from cultures in yeast–mannitol agar plates (Vincent, 1970) and were grown in yeast-mannitol broth for 24 h at 30°C and 150 rpm. After incubation, the bacterial concentration was standardized by spectrophotometry to an optical density of 0.5 at 600 nm (~108 colony-forming units mL−1).
The experiment was carried out under greenhouse conditions at the Nataima research center of AGROSAVIA in Espinal, Tolima, Colombia (4°11′28.39″N latitude and 74°57′38.69″W longitude). The experimental design was a randomized complete block factorial arrangement of 12 treatments, consisting of three factors with their respective levels: hybrid maize genotypes (white endosperm DK-370VTPRO and yellow endosperm 30F35VYHR), the dose of P fertilizer (0, 25, and 50% of recommended P), and PSB inoculation (uninoculated and inoculated with Rhizobium sp. B02). A fertilization dose of 100% was considered positive complete fertilization. The treatments were: (i) 100% DAP without inoculation, (ii) 50% DAP without inoculation, (iii) 25% DAP without inoculation, (iv) 0% DAP without inoculation, (v) 50% DAP with inoculation, (vi) 25% DAP with inoculation, and (vii) 0% DAP with inoculation. The experiment was repeated independently three times using three replicates for each experiment. Thereby, each treatment had nine experimental units. The soil type used was a sieved airdried Inceptisol soil (Soil Science Division Staff, 2017) typical of the region where maize, cotton, and rice crops are cultivated as rotational systems. A total of 24 kg of unsterile soil with low P content (pH: 6.12, organic matter: 1.69 mg kg−1, P: 12.28 mg kg−1, effective cation exchange coefficient: 6.90 cmol kg−1, Ca: 4.79 cmol kg−1, Mg: 1.74 cmol kg−1, K: 0.23 cmol kg−1, Na: <0.14 cmol kg−1) was placed in polyethylene bags of size 35 × 45 cm.
Based on the previous characterization of the soil, the nutrient requirements for maize cultivation, and the rates of P fertilizer per treatment, the fertilization was split: Pre-sowing, 100% phosphorus (P), 18% nitrogen (N), 30% potassium (K), and 100% minor and secondary elements; phenological stage V2 (two true leaves), 30% N, and 30% K; phenological stage V5 (five true leaves), 30% N, and 30% K; phenological stage V8 (true leaves), 22% N, and 10% K. The sources and total concentration of N, P, and K were amide fertilizer (129.8 mg Kg−1 soil), diammonium phosphate (DAP) (157.9 mg Kg−1 soil), and KCl (158.75 mg Kg−1 soil), respectively. These amounts of fertilizers were determined according to García and Correndo (2016). Four maize seeds were sown per bag, and later, thinning was conducted in phenological stage V2, leaving one plant per bag.
The inoculation of Rhizobium sp. B02 was performed 30 min before sowing by immersing the seeds in the bacterial inoculum (~108 CFU mL−1). Subsequently, 10 ml of the bacterial inoculum were added per bag during the phenological stage V5 (Ibrahim and El-Sawah, 2022). For water irrigation, a system was installed with self-compensated drippers discharging 4 L h−1 in each bag, applying an irrigation sheet when the volumetric water content was below the field capacity (~80%). The pests and diseases were managed following the recommendations for conventional maize crops. The environmental conditions during the experiment were 18–38°C and ~55% humidity.
Different plant parameters were measured in three phenological stages: vegetative stage of the tillering (V5) at 12 days after emergence (DAE), vegetative stage of the tassel (VT) at 52 DAE, and reproductive stage of physiological maturity (R6) at 118 DAE. In the V5 stage, we measured shoot length, shoot dry weight, leaf area, relative chlorophyll content, and shoot P content. The relative chlorophyll content was measured using a SPAD-502 chlorophyll meter (Konica Minolta, Tokyo, Japan). In addition to the parameters measured at V5, net photosynthesis rate and transpiration rate were taken at the VT stage. The photosynthetic and transpiration rates were measured by gas exchange measurements between 09:00 and 11:00 with the infrared gas analyzer LI-6400 XT (Lincoln, NE, USA). Finally, we measured the number of lines per cob, number of grains per line, 1,000-grain weight, and grain yield in R6. Three replicates per independent repetition were measured in all parameters for nine measurements, except for relative chlorophyll content and shoot P content. The replicates of each independent experiment were mixed by repetition in this parameter, generating three measurements.
The data obtained were subjected to a multivariate analysis of variance (MANOVA) and a Tukey test to examine whether there were significant differences at a 95% confidence level (p < 0.05) using the SPSS 22.0 program (SPSS Inc., Chicago, IL, USA). The data were also analyzed through a principal component analysis with a biplot using RStudio software version 4.0.2.4 (RStudio Inc., Boston, MA, USA) to determine the correlation between parameters. The correlation between parameters was observed in this model in a more integrated way and was based on both the statistical summary and the angle included between the arrows pointing at two variables as follows: sharp angles indicated positive correlations, squared angles indicated a null correlation, and obtuse angles indicated negative correlations (de Almeida Carvalho-Estrada et al., 2020). Graphics were made using GraphPad Prism 8 software (Graphpad, San Diego, CA, USA).
We conducted a growth promotion assay in two plant genotypes under greenhouse conditions to assess the potential of Rhizobium sp. B02 for maize development during three phenological stages. Overall, we found that inoculation had a significant effect (p < 0.05) on maize and that its influence was dependent on the P fertilization dose (Supplementary Table 1). A significant influence on maize, regardless of the maize genotypes, was exerted by P fertilization. A significant effect on maize growth was not triggered by the interaction between plant genotype and inoculation, showing that the plant growth-promoting potential of inoculation with Rhizobium sp. B02 was strongly influenced by genotype.
The plant parameters gradually increased with the P fertilization rate without inoculation in the white endosperm genotype of maize (Figure 1). Inoculation had a significant influence on the V5 stage (p < 0.05; Supplementary Table 2). We observed that its use slightly increased shoot length, while more marked increases were found in shoot dry weight (Figures 1A,B). Leaf area was also influenced by inoculation when 25 and 50% DAP were applied, but more notably at 0% DAP (Figure 1C). The relative content of chlorophyll was improved by inoculation in all doses, mainly at 0 and 50% DAP (Figure 1D). Regarding shoot P content, the influence of inoculation was subtle (Figure 1E).
Figure 1. Influence of inoculation with Rhizobium sp. B02 on shoot length (A), shoot dry weight (B), leaf area (C), relative chlorophyll content (D), and shoot P content (E) of the white endosperm genotype of maize at the vegetative stage of tillering (V5). Except for shoot P content (three measurements), the means and standard errors resulted from three replicates and three independent repetitions (nine measurements). Different letters indicate significant differences according to Tukey's test (p < 0.05).
Next, we evaluated the influence of inoculation with Rhizobium sp. B02 in the phenological stage of VT. Interestingly, the effect of inoculation was pronounced in the VT stage (Figure 2). Inoculation significantly increased shoot length and shoot dry weight at all DAP doses than the uninoculated controls (Figures 2A,B). The leaf area increased in plants inoculated using the 50% DAP dose (Figure 2C). The relative chlorophyll content was improved in the inoculated treatment independent of the DAP dose by ~12% (Figure 2E). The photosynthetic rate had the same trend as the relative chlorophyll content (Figure 2F). Finally, the transpiration rate increased with the inoculation by ~17 and ~29% at 0 and 50% DAP, respectively (Figure 2G). Therefore, inoculation had a significant effect on maize plants at this stage (p < 0.05; Supplementary Table 3).
Figure 2. Influence of inoculation with Rhizobium sp. B02 on shoot length (A), shoot dry weight (B), leaf area (C), relative chlorophyll content (D), shoot P content (E), photosynthetic rate (F), and transpiration rate (G) of the white endosperm genotype of maize at the vegetative stage of the tassel (VT). Except for relative chlorophyll and shoot P contents (three measurements), means and standard errors result from three replicates and three independent repetitions (nine measurements). Different letters are indicators of significant differences according to Tukey's test (p < 0.05).
Next, we sought to determine whether the productivity of the white endosperm maize was improved by the potential of Rhizobium sp. B02 (Figure 3). The number of lines per cob and the number of grains per line increased by inoculation with 25 and 50% DAP rates, respectively (Figures 3A,B). The 1,000-grain weight parameter increased in the range of 6–10% when inoculation was performed (Figure 3C). Interestingly, inoculation led to increases in grain yield at all reduced DAP doses (Figure 3D). The most significant increase was ~17% at 50% DAP, in which the 100% DAP control was phenocopied. Therefore, a significant influence on maize was triggered by inoculation in the R6 stage, representing physiological maturity (p < 0.05; Supplementary Table 4).
Figure 3. Influence of inoculation with Rhizobium sp. B02 on the number of lines per cob (A), number of grains per line (B), 1,000-grain weight (C), and grain yield (D) of the white endosperm genotype of maize at the reproductive stage of physiological maturity. Means and standard errors result from three replicates and three independent repetitions. Different letters are indicators of significant differences according to Tukey's test (p < 0.05).
We conducted a principal component analysis to address the correlations among the parameters promoted by inoculation over the three phenological stages. Of the 16 plant parameters measured, 14 were promoted by inoculation, as described above, and were included in this analysis. These parameters were shoot dry weight, leaf area, and relative chlorophyll content at V5; shoot length, shoot dry weight, leaf area, shoot P content, photosynthetic rate, relative chlorophyll content, and transpiration rate at VT; and number of lines per cob, number of grains per line, 1,000-grain weight, and grain yield at R6. We noted that 38% of the data variation was explained by the principal component analysis. From the total variance, 25% was accounted for by principal component 1 and 15% by principal component 2 (Figure 4). We also found that the stage with the largest positive correlation between its parameters was V5. Shoot dry weight was the morphometric parameter most closely correlated between vegetative stages V5 and VT. The relative chlorophyll content in V5 showed a positive correlation with the shoot P content in the VT stage. The photosynthetic rate, transpiration rate, and relative chlorophyll content of the VT stage were positively correlated. Likewise, these three parameters have a strong positive correlation with shoot length and a slight positive correlation with shoot P content in the same phenological stage. The 1,000-grain weight was the productivity parameter most positively correlated with the morphometric and physiological parameters from other stages (e.g., shoot dry weight, shoot P content, leaf area, and relative chlorophyll content). The parameters that reflected a strong positive correlation with grain yield were the number of grains per line and the number of lines per cob in the R6 stage and the leaf area of VT. Additionally, the grain yield had less pronounced positive correlations with the shoot dry weight of both stages, the leaf area, and the relative chlorophyll content of V5; the shoot P content of VT; and, finally, the 1,000-grain weight of R6.
Figure 4. Principal component analysis plot correlating morphometric, physiological, and productivity parameters promoted by inoculation with Rhizobium sp. B02 in the white endosperm genotype of maize. SDW, shoot dry weight; LA, leaf area; RCC, relative chlorophyll content; SL, shoot length; P, shoot P content; PH, photosynthetic rate; TR, transpiration rate; NLC, number of lines per cob; NGL, number of grains per line; 1,000 GW, 1,000-grain weight; GY, grain yield; V5, vegetative stage of the tillering; VT, vegetative state of the tassel; R6, reproductive stage of physiological maturity.
As for the yellow endosperm genotype, we found a similar trend in the uninoculated treatments concerning the white endosperm genotype since the plant parameters gradually increased with the DAP fertilization rate (Figure 5). The shoot length did not improve significantly in the inoculated treatments (Figure 5A). Both shoot dry weight and leaf area increased with inoculation at 50% DAP (Figures 5B,C). The relative chlorophyll content slightly improved at 0% DAP with inoculation (Figure 5D). The last parameter measured in this stage was shoot P content. No significant differences were found with inoculation (Figure 5E). Consequently, inoculation had no significant effect on stage V5 (p < 0.05; Supplementary Table 5).
Figure 5. Influence of the inoculation with Rhizobium sp. B02 on shoot length (A), shoot dry weight (B), leaf area (C), relative chlorophyll content (D), and shoot P content (E) of the yellow endosperm genotype of maize at the vegetative stage of tillering (V5). Except for shoot P content (three measurements), the means and standard errors resulted from three replicates and three independent repetitions (nine measurements). Different letters indicate significant differences according to Tukey's test (p < 0.05).
We found that inoculation also had no significant growth-promoting effect on the VT of the yellow endosperm genotype (p < 0.05; Supplementary Table 6). The plant parameters responded differently to the inoculation of Rhizobium sp. B02 concerning the white endosperm (Figure 6). For instance, shoot length was markedly more promoted by inoculation than shoot dry weight (Figures 6A,B). The leaf area showed improvement again with inoculation at 25 and 50% DAP (Figure 6C), while the relative chlorophyll content did not have significant differences in any treatment (Figure 6D). Shoot P content increased slightly in the inoculated treatments when 25 and 50% DAP were applied (Figure 6E). The photosynthetic rate had the same trend as the relative chlorophyll content since there were no significant differences in any treatment (Figure 6F). Finally, the transpiration rate only improved significantly with inoculation at 0% DAP, registering an increase of ~24% (Figure 6G).
Figure 6. Influence of inoculation with Rhizobium sp. B02 on shoot length (A), shoot dry weight (B), leaf area (C), relative chlorophyll content (D), shoot P content (E), photosynthetic rate (F), and transpiration rate (G) of the yellow endosperm genotype of maize at the vegetative stage of the tassel (VT). Except for relative chlorophyll and shoot P contents (three measurements), means and standard errors result from three replicates and three independent repetitions (nine measurements). Different letters are indicators of significant differences according to Tukey's test (p < 0.05).
We noted no significant differences in any treatment in physiological maturity (Figure 7), either in the number of lines per cob or in the number of grains per line (Figures 7A,B). The 1,000-grain weight significantly increased at 25 and 50% DAP in the inoculated treatments (Figure 7C). Finally, grain yield was not significantly increased by inoculation with Rhizobium sp. B02, as the best yield was obtained in 100% DAP treatment (Figure 7D). Therefore, inoculation did not significantly impact maize productivity (p < 0.05; Supplementary Table 7).
Figure 7. Influence of inoculation with Rhizobium sp. B02 on the number of lines per cob (A), number of grains per line (B), 1,000-grain weight (C), and grain yield (D) of the yellow endosperm genotype of maize at the reproductive stage of physiological maturity. Means and standard errors result from three replicates and three independent repetitions. Different letters are indicators of significant differences according to Tukey's test (p < 0.05).
With the same purpose as the previous principal components analysis conducted on the white endosperm, we performed the analysis on the yellow endosperm. Of the 16 plant parameters raised, seven were promoted by inoculation and, therefore, were included in this analysis. These parameters were shoot dry weight, leaf area, relative chlorophyll content at V5, shoot length, leaf area, transpiration rate at VT, and 1,000-grain weight at R6. A total of 60% of the original data variation was explained by principal component analysis, where 33% of the total variance was accounted for by principal component 1 and 27% by principal component 2 (Figure 8). Again, we observed that V5 was the stage with the largest positive correlation with the parameters. The relative chlorophyll content of V5 was positively correlated with the leaf area of VT, which was positively correlated with the shoot length of VT. The transpiration rate of the VT stage was not positively correlated with any parameters. Finally, we also found that the 1,000-grain weight was positively correlated with morphometric and physiological parameters of other stages (e.g., plant length, leaf area, and relative chlorophyll content).
Figure 8. Principal component analysis plot correlating morphometric, physiological, and productivity parameters promoted by inoculation with Rhizobium sp. B02 in the yellow endosperm genotype of maize. SDW, shoot dry weight; LA, leaf area; RCC, relative chlorophyll content; SL, shoot length; TR, transpiration rate; 1,000 GW, 1,000-grain weight; GY, grain yield; V5, vegetative stage of the tillering; VT, vegetative state of the tassel; R6, reproductive stage of physiological maturity.
Maize is one of the crops facing a P crisis (Langhans et al., 2021). In response to this situation, research on microbial inoculants that improve sustainable P management in agriculture has been promoted (Nagpal et al., 2021; Singh and Brar, 2022). Here, we reported the biofertilizing potential of Rhizobium sp. B02 to improve P fertilization in maize crop, addressing whether the potential varies based on the plant genotype.
The development of maize in all phenological stages was improved by inoculation with Rhizobium sp. B02. VT was the phenological stage with the plant parameters most significantly influenced by inoculation. The VT stage has a pivotal role in the growth of maize, given the high concentration of nutrients that the plant accumulates in its tissues before its reproductive stage. This is one of the most sampled phenological stages for optimizing nutrient management (Pavinato et al., 2017). However, the three phenological stages were significantly promoted by inoculation solely in the white endosperm, as well as the morphometric, physiological, and productivity parameters of the maize. Therefore, the growth-promoting effect was markedly more prominent in the white endosperm than in the yellow endosperm maize genotype, corroborating that the inoculation was strongly influenced by the genotype. This finding was an indicator that the potential of Rhizobium sp. B02 was specific to the maize genotype.
Several studies have reported that the plant growth-promoting effect of a strain depends on the plant genotype. For instance, Vanissa et al. (2020) found that maize growth depended on genotype by inoculating Arthrobacter and Bacillus in salinity-affected and P-deficient soils. In studies with Azospirillum brasilense, Burkholderia tropica, and Herbaspirillum frisingense in different wheat, sorghum, and rice genotypes, it has been reported that the growth-promoting effect depends on the plant genotype (Schlemper et al., 2018; Vidotti et al., 2019). However, it is unclear how the potential of promising strains is influenced by the differences between genotypes of the same crop. Unraveling these interactions will lead to the improved effectiveness of the microbial inoculants.
Phosphorus management can be optimized if shoot P concentration, leaf area, and photosynthetic rate are improved to attain high shoot weights and, consequently, high grain yields (Zhang et al., 2018). We found that these five parameters were improved by inoculation in the white endosperm, generating positive correlations between them. We also found that inoculation potential was larger when 50% DAP was applied to white endosperms compared to 25% and 0% DAP. Interestingly, complete fertilization control in all productivity parameters in the white endosperm was phenocopied by the potential of inoculation at a 50% DAP rate. This finding is an indicator that Rhizobium sp. B02 allows P fertilizer application rates to be reduced. In previous studies, PSB has been shown to have some potential, allowing a 33–75% reduction in the rate of P mineral fertilizers in several crops (Sahandi et al., 2019; Rosa et al., 2020; Lopes et al., 2021).
The role of the Rhizobium genus in the P cycle for plant nutrition has been limitedly explored. Some investigations have reported the presence of Rhizobium in the rhizosphere, endosphere, and phyllosphere of non-legume crops (Díez-Méndez and Menéndez, 2021). The Rhizobium genus is also capable of carrying out other plant growth-promoting features, such as solubilizing and mineralizing P, synthesizing indole compounds, and producing siderophores (Iyer and Rajkumar, 2018; Verma et al., 2020). The potential of Rhizobium sp. B02 is likely to be a direct consequence of stimulating plant growth or an indirect result of interacting synergistically with native microbiota (Romero-Perdomo et al., 2019; Amaya-Gómez et al., 2020). Transcriptomic studies with mutant strains are needed to understand in detail which plant genes are involved in the recognition and growth promotion by Rhizobium sp. B02.
Few studies have shown whether the sole application of Rhizobium has a beneficial impact on the development of crops in phosphorus-deficient soils. This was a relevant condition of this study provided by the soil used, low P availability (12.28 mg kg−1), and reduced doses of P fertilizer applied. Abiotic stresses in plants (e.g., nutritional, saline, or drought stress) have been reported in research developments to strongly influence the potential of these microbes to promote growth (Moreno-Galván et al., 2020; Cortés-Patiño et al., 2021; Mendoza-Labrador et al., 2021; Pardo-Diaz et al., 2021).
This study is a step toward optimizing the efficient use of P in maize cultivation through the inoculation of Rhizobium sp. B02. Therefore, field efficacy tests need to be conducted. Although Rhizobium is a bacterial genus mainly associated with the nitrogen nutrition of legume crops, our findings represent insights into the potential of Rhizobium sp. B02 in conditions of nutritional limitation of P.
Inoculation with Rhizobium sp. B02 has a significant effect on growth, physiological traits, and yield of maize, yet its plant-promoting effect is markedly larger on the white endosperm than on the yellow endosperm maize genotype. The development of maize was promoted by inoculation in all phenological stages, wherein the largest increase occurred in the vegetative stage of the tassel. Shoot P concentration, leaf area, photosynthetic rate, shoot dry weight, 1,000-grain weight, and grain yield in white endosperm were promoted by the influence of Rhizobium sp. B02. A plant response similar to 100% DAP was generated by the productivity parameters in the white endosperm with the inoculation at 50% DAP, suggesting an equivalent reduction of ~85 kg of the DAP fertilizer ha−1. Rhizobium sp. B02 represents a promising strain for use as an inoculum in white endosperm maize to reduce rates of P fertilizer application. Examining the use of strains as microbial inoculants in various plant genotypes is necessary to report their potential specifically and, thus, strengthen their implementation as sustainable biotechnology.
The raw data supporting the conclusions of this article will be made available by the authors, without undue reservation.
JB-M, FR-P, and GE-B: conceptualization. JB-M, FR-P, and AS: data curation, formal analysis, and visualization. JB-M, FR-P, LM-C, and GE-B: investigation. JB-M, FR-P, AS, and GE-B: writing—original draft. FR-P and GE-B: writing—review and editing. LM-C and GE-B: project administration. GE-B: funding acquisition and supervision. All authors contributed to the article and approved the submitted version.
This work was funded by AGROSAVIA and the Ministry of Agriculture and Rural Development from Colombia (grant no. 1001359).
The authors declare that the research was conducted in the absence of any commercial or financial relationships that could be construed as a potential conflict of interest.
All claims expressed in this article are solely those of the authors and do not necessarily represent those of their affiliated organizations, or those of the publisher, the editors and the reviewers. Any product that may be evaluated in this article, or claim that may be made by its manufacturer, is not guaranteed or endorsed by the publisher.
All authors are very grateful to the members of the Sustainable Agriculture Systems Group, especially to Mauricio Barón for their support during the greenhouse evaluations.
The Supplementary Material for this article can be found online at: https://www.frontiersin.org/articles/10.3389/fsufs.2022.955473/full#supplementary-material
Ahmad, M. T., Asghar, H. N., Saleem, M., Khan, M. Y., and Zahir, Z. A. (2015). Synergistic effect of rhizobia and biochar on growth and physiology of maize. J. Agron. 107, 2327–2334. doi: 10.2134/agronj15.0212
Alori, E. T., Glick, B. R., and Babalola, O. O. (2017). Microbial phosphorus solubilization and its potential for use in sustainable agriculture. Front. Microbiol. 8, 971. doi: 10.3389/fmicb.2017.00971
Amaya-Gómez, C. V., Porcel, M., Mesa-Garriga, L., and Gómez Álvarez, M. I. (2020). A framework for the selection of plant growth promoting rhizobacteria based on bacterial competence mechanisms. Appl. Environ. Microbiol. 86, e00760. doi: 10.1128/AEM.00760-20
Bargaz, A., Elhaissoufi, W., Khourchi, S., Benmrid, B., Borden, K. A., and Rchiad, Z. (2021). Benefits of phosphate solubilizing bacteria on belowground crop performance for improved crop acquisition of phosphorus. Microbiol. Res. 252, 126842. doi: 10.1016/j.micres.2021.126842
Chen, Z. S., Hseu, Z. Y., and Tsai, C. C. (2015). “Inceptisols,” in The Soils of Taiwan, ed A. E. Hartemink (Dordrecht: Springer), 63–72. doi: 10.1007/978-94-017-9726-9
Cortés-Patiño, S., Vargas, C., Álvarez-Flórez, F., Bonilla, R., and Estrada-Bonilla, G. (2021). Potential of Herbaspirillum and Azospirillum consortium to promote growth of perennial ryegrass under water deficit. Microorganisms 9, 91. doi: 10.3390/microorganisms9010091
de Almeida Carvalho-Estrada, P., Fernandes, J., da Silva, É. B., Tizioto, P., de Fátima Paziani, S., and Duarte, A. P. (2020). Effects of hybrid, kernel maturity, and storage period on the bacterial community in high moisture and rehydrated corn grain silages. Syst. Appl. Microbiol. 43, 126131. doi: 10.1016/j.syapm.2020.126131
Díez-Méndez, A., and Menéndez, E. (2021). “Rhizobium presence and functions in microbiomes of non-leguminous plants,” in Symbiotic Soil Microorganisms, eds N. Shrivastava, S. Mahajan, and A. Varma (Cham: Springer), 241–266. doi: 10.1007/978-3-030-51916-2_16
Erenstein, O., Chamberlin, J., and Sonder, K. (2021). Estimating the global number and distribution of maize and wheat farms. Glob. Food Sec. 30, 100558. doi: 10.1016/j.gfs.2021.100558
Fenalce (2022a). Histórico de área, producción y rendimiento Cereales y Leguminosas. Departamento económico y de apoyo a la comercialización. Available online at: https://app.powerbi.com/view?r=eyJrIjoiOTk3NDZhYTMtZjg5NC00OWIxLWE3NmItOTIzYjdlZmFmNmJhIiwidCI6IjU2MmQ1YjJlLTBmMzEtNDdmOC1iZTk4LThmMjI4Nzc4MDBhOCJ9 (14122021) (accessed December14, 2021).
Fenalce (2022b). Importaciones de cereales y leguminosas. Fondo Nacional Cerealista. Departamento económico y de apoyo a la comercialización. Available online at: https://app.powerbi.com/view?r=eyJrIjoiMTAwM2FmNDAtY2JmOS00ZDM1LWE2MTMtYTM5YTU4NGM2YTg1IiwidCI6IjU2MmQ1YjJlLTBmMzEtNDdmOC1iZTk4LThmMjI4Nzc4MDBhOCJ9 (accessed December 14, 2021).
Gao, J. L., Sun, P., Wang, X. M., Lv, F. Y., Mao, X. J., and Sun, J. G. (2017). Rhizobium wenxiniae sp. nov., an endophytic bacterium isolated from maize root. Int. J. Syst. Evol. Microbiol. 67, 2798–2803. doi: 10.1099/ijsem.0.002025
García, F. O., and Correndo, A. (2016). Cálculo de requerimientos nutricionales. IPNI (International Plant Nutrition Institute). Available online at: http://lacs.ipni.net/article/LACS-1024 (accessed May 30, 2020).
Gurdeep, K. A. U. R., and Reddy, M. S. (2015). Effects of phosphate-solubilizing bacteria, rock phosphate and chemical fertilizers on maize-wheat cropping cycle and economics. Pedosphere 25, 428–437. doi: 10.1016/S1002-0160(15)30010-2
Ibrahim, H. M., and El-Sawah, A. M. (2022). The mode of integration between Azotobacter and Rhizobium affect plant growth, yield, and physiological responses of pea (Pisum sativum L.). Soil Sci. Plant Nutr. 22, 1–14. doi: 10.1007/s42729-021-00727-2
Iyer, B., and Rajkumar, S. (2018). Genome sequence and comparative genomics of Rhizobium sp. Td3, a novel plant growth promoting phosphate solubilizing Cajanus cajan symbiont. Microbiol. Res. 218, 32–40. doi: 10.1016/j.micres.2018.09.007
Langhans, C., Beusen, A. H. W., Mogollón, J. M., and Bouwman, A. F. (2021). Phosphorus for sustainable development goal target of doubling smallholder productivity. Nat. Sustain. 5, 1–7. doi: 10.1038/s41893-021-00794-4
Li, Y., Liu, X., Hao, T., and Chen, S. (2017). Colonization and maize growth promotion induced by phosphate solubilizing bacterial isolates. Int. J. Mol. Sci. 18, 1253. doi: 10.3390/ijms18071253
Lopes, C. M., Silva, A. M. M., Estrada-Bonilla, G. A., Ferraz-Almeida, R., Vieira, J. L. V., Otto, R., et al. (2021). Improving the fertilizer value of sugarcane wastes through phosphate rock amendment and phosphate-solubilizing bacteria inoculation. J. Clean. Prod. 298, 126821. doi: 10.1016/j.jclepro.2021.126821
Mendoza, J. A., and Bonilla, R. (2014). Infectividad y efectividad de rizobios aislados de suelos de la Costa Caribe colombiana en Vigna unguiculata. Rev. Colomb. Biotecnol. 16, 84–89. doi: 10.15446/rev.colomb.biote.v16n2.47246
Mendoza-Labrador, J., Romero-Perdomo, F., Abril, J., Hernández, J. P., Uribe-Vélez, D., and Buitrago, R. B. (2021). Bacillus strains immobilized in alginate macrobeads enhance drought stress adaptation of Guinea grass. Rhizosphere 19, 100385. doi: 10.1016/j.rhisph.2021.100385
Morel, C., Plénet, D., and Mollier, A. (2021). Calibration of maize phosphorus status by plant-available soil P assessed by common and process-based approaches. Is it soil-specific or not? Eur. J. Agron. 122, 126174. doi: 10.1016/j.eja.2020.126174
Moreno-Galván, A., Romero-Perdomo, F. A., Estrada-Bonilla, G., Meneses, C. H. S. G., and Bonilla, R. R. (2020). Dry-caribbean Bacillus spp. strains ameliorate drought stress in maize by a strain-specific antioxidant response modulation. Microorganisms 8, 823. doi: 10.3390/microorganisms8060823
Nagpal, S., Sharma, P., and Kumawat, K. C. (2021). “Microbial bioformulations: Revisiting role in sustainable agriculture,” in Biofertilizers, ed. A. Rakshit, V. Singh-Meena, M. Parihar, H. B. Singh, and A. K. Singh (Sawston: Woodhead Publishing), 329–346. doi: 10.1016/B978-0-12-821667-5.00016-6
Pardo-Diaz, S., Romero-Perdomo, F., Mendoza-Labrador, J., Delgadillo-Duran, D., Castro-Rincon, E., Silva, A. M., et al. (2021). Endophytic PGPB improves plant growth and quality and modulates the bacterial community of an intercropping system. Front. Sustain. Food Syst. 5, 715270. doi: 10.3389/fsufs.2021.715270
Pavinato, P. S., Rodrigues, M., Soltangheisi, A., Sartor, L. R., and Withers, P. J. A. (2017). Effects of cover crops and phosphorus sources on maize yield, phosphorus uptake, and phosphorus use efficiency. Agron. J. 109, 1039–1047. doi: 10.2134/agronj2016.06.0323
Poole, N., Donovan, J., and Erenstein, O. (2021). Agri-nutrition research: revisiting the contribution of maize and wheat to human nutrition and health. Food Policy 100, 101976. doi: 10.1016/j.foodpol.2020.101976
Raymond, N. S., Gómez-Muñoz, B., van der Bom, F. J., Nybroe, O., Jensen, L. S., Müller-Stöver, D. S., et al. (2021). Phosphate-solubilising microorganisms for improved crop productivity: a critical assessment. New Phytol. 229, 1268–1277. doi: 10.1111/nph.16924
Richardson, A. E., and Simpson, R. J. (2011). Soil microorganisms mediating phosphorus availability update on microbial phosphorus. Plant. Physiol. 156, 989–996. doi: 10.1104/pp.111.175448
Romero-Perdomo, F., Ocampo-Gallego, J., Camelo-Rusinque, M., and Bonilla, R. (2019). Plant growth promoting rhizobacteria and their potential as bioinoculants on Pennisetum clandestinum (Poaceae). Rev. Biol. Trop. 67, 825–832. doi: 10.15517/rbt.v67i4.34029
Romero-Perdomo, F. A., Beltrán, J. I., Mendoza-Labrador, J. A., Estrada-Bonilla, G. A., and Bonilla, R. (2021). Phosphorus nutrition and growth of cotton plants inoculated with growth-promoting bacteria under low phosphate availability. Front. Sustain. Food. Syst. 4, 285. doi: 10.3389/fsufs.2020.618425
Rosa, P. A. L., Mortinho, E. S., Jalal, A., Galindo, F. S., Buzetti, S., and Fernandes, G. C. (2020). Inoculation with growth-promoting bacteria associated with the reduction of phosphate fertilization in sugarcane. Front. Environ. Sci. 8, 32. doi: 10.3389/fenvs.2020.00032
Sahandi, M. S., Mehrafarin, A., Badi, H. N., Khalighi-Sigaroodi, F., and Sharifi, M. (2019). Improving growth, phytochemical, and antioxidant characteristics of peppermint by phosphate-solubilizing bacteria along with reducing phosphorus fertilizer use. Ind. Crops Prod. 141, 111777. doi: 10.1016/j.indcrop.2019.111777
Santos-Torres, M., Romero-Perdomo, F., Mendoza-Labrador, J., Gutiérrez, A. Y., Vargas, C., and Castro, E. (2021). Genomic and phenotypic analysis of rock phosphate-solubilizing rhizobacteria. Rhizosphere 17, 100290. doi: 10.1016/j.rhisph.2020.100290
Schlemper, T. R., Dimitrov, M. R., Gutierrez, F. A. S., van Veen, J. A., Silveira, A. P., and Kuramae, E. E. (2018). Effect of Burkholderia tropica and Herbaspirillum frisingense strains on sorghum growth is plant genotype dependent. PeerJ 6, e5346. doi: 10.7717/peerj.5346
Singh, J., and Brar, B. S. (2022). Build-up and utilization of phosphorus with continues fertilization in maize-wheat cropping sequence. Field. Crops. Res. 276, 108389. doi: 10.1016/j.fcr.2021.108389
Soil Science Division Staff (2017). Soil Survey Manual: USDA Handbook No. 18. Washington, DC: Government Printing Office.
Tanumihardjo, S. A., McCulley, L., Roh, R., Lopez-Ridaura, S., Palacios-Rojas, N., and Gunaratna, N. S. (2020). Maize agro-food systems to ensure food and nutrition security in reference to the Sustainable Development Goals. Glob. Food Secur.-Agr. 25, 100327. doi: 10.1016/j.gfs.2019.100327
Vanissa, T. T. G., Berger, B., Patz, S., Becker, M., Turečkov,á, V., Novák, O., et al. (2020). The Response of maize to inoculation with Arthrobacter sp. and Bacillus sp. in phosphorus-deficient, salinity-affected soil. Microorganisms 8, 1005. doi: 10.3390/microorganisms8071005
Vargas, G., León, N., and Hernández, Y. (2019). “Agricultural socio-economic effects in Colombia due to degradation of soils,” in Sustainable Management of Soil and Environment, eds R. Swaroop-Meena, S. Kumar, J. Singh-Bohra, M. Lal-Jat (Singapore: Springer), 289–337. doi: 10.1007/978-981-13-8832-3_9
Verma, M., Singh, A., Dwivedi, D. H., and Arora, N. K. (2020). Zinc and phosphate solubilizing Rhizobium radiobacter (LB2) for enhancing quality and yield of loose leaf lettuce in saline soil. Environ. Sustain. 3, 209–218. doi: 10.1007/s42398-020-00110-4
Vidotti, M. S., Lyra, D. H., Morosini, J. S., Granato, Í. S. C., Quecine, M. C., Azevedo, J. L. D., et al. (2019). Additive and heterozygous (dis) advantage GWAS models reveal candidate genes involved in the genotypic variation of maize hybrids to Azospirillum brasilense. PLoS One 14, e0222788. doi: 10.1371/journal.pone.0222788
Zhang, W., Chen, X. X., Liu, Y. M., Liu, D. Y., Du, Y. F., Chen, X. P., et al. (2018). The role of phosphorus supply in maximizing the leaf area, photosynthetic rate, coordinated to grain yield of summer maize. Field. Crop. Res. 219, 113–119. doi: 10.1016/j.fcr.2018.01.031
Keywords: fertilization, corn, plant growth-promoting bacteria, phosphate-solubilizing bacteria, biofertilizer, phosphorus, Rhizobium
Citation: Beltran-Medina JI, Romero-Perdomo F, Molano-Chavez L, Silva AMM and Estrada-Bonilla GA (2022) Differential Plant Growth Promotion Under Reduced Phosphate Rates in Two Genotypes of Maize by a Rhizobial Phosphate-Solubilizing Strain. Front. Sustain. Food Syst. 6:955473. doi: 10.3389/fsufs.2022.955473
Received: 28 May 2022; Accepted: 13 June 2022;
Published: 08 July 2022.
Edited by:
Everlon Cid Rigobelo, São Paulo State University, BrazilReviewed by:
Hezekiah Korir, Egerton University, KenyaCopyright © 2022 Beltran-Medina, Romero-Perdomo, Molano-Chavez, Silva and Estrada-Bonilla. This is an open-access article distributed under the terms of the Creative Commons Attribution License (CC BY). The use, distribution or reproduction in other forums is permitted, provided the original author(s) and the copyright owner(s) are credited and that the original publication in this journal is cited, in accordance with accepted academic practice. No use, distribution or reproduction is permitted which does not comply with these terms.
*Correspondence: German A. Estrada-Bonilla, Z2Flc3RyYWRhQGFncm9zYXZpYS5jbw==; Z2VybWFuZXN0cmFAZ21haWwuY29t
Disclaimer: All claims expressed in this article are solely those of the authors and do not necessarily represent those of their affiliated organizations, or those of the publisher, the editors and the reviewers. Any product that may be evaluated in this article or claim that may be made by its manufacturer is not guaranteed or endorsed by the publisher.
Research integrity at Frontiers
Learn more about the work of our research integrity team to safeguard the quality of each article we publish.