- 1International Center for Tropical Agriculture (CIAT), Cali, Colombia
- 2Independent Researcher, Yumbo, Colombia
- 3Independent Researcher, Tegucigalpa, Honduras
- 4Colombian Corporation for Agricultural Research (AGROSAVIA), Villavicencio, Colombia
Improving soil organic carbon (SOC) storage enhances soil quality and mitigates climate change. Agricultural and livestock specialists increasingly view tropical grasslands as a potential target for storing more soil carbon while boosting productivity. Earlier research in the 1990s showed the promise of improving SOC storage in the Eastern High Plains of Colombia. But these studies were limited to two experimental stations, without focusing on conditions on farms or under variable management. This research examined whether those early studies did indeed reflect possibilities for improving SOC storage and livestock productivity. We measured SOC stocks at one of the experiment stations from previous research and on farms throughout the study area in Colombia's Eastern High Plains. Complementarily our team sampled other predominant land uses to map SOC storage across the nearly 1 million ha study area. Using that information, we also constructed scenarios suggesting changes in SOC and productivity based on land-use changes. The high SOC accumulation found at experimental sites in the 1990s declined 24 years later. However, SOC storage was over 27 Mg ha−1 yr−1 higher than reference native savanna sites, with an accumulation rate of 0.96 Mg ha−1 yr−1. On farms under variable management, improved pastures stored 10 Mg ha−1 more SOC than degraded pastures or native savanna. For the whole region, we estimate that carbon storage observed across soils and land use of the 1 million ha study area could store 0.08 Gt of carbon down to 1 m depth, with wide variation across the region. While the SOC measured in grasslands in the early 1990s did not persist under inadequate management over the period of two decades, the potential to accumulate SOC of Colombia's Eastern High Plains through appropriate management is high, pointing to a sustainable livestock strategy that boosts productivity and reduces emissions.
Introduction
Global concern for climate change mitigation often focuses on cattle production because it generates between 6 and 13% of world-wide greenhouse gas (GHG) emissions (Crippa et al., 2021; Mason-D'Croz et al., 2022). This would be an incomplete assessment if we consider cattle only as a large source of GHG emissions (Arango et al., 2020). Contribution of cattle to food production and rural economies are just two of the other dimensions that need to be considered (Notenbaert et al., 2021). Sustainable livestock intensification—reducing emissions per unit of milk and meat produced—includes several ways to improve efficiency, use less land, accumulate carbon (C), and avoid deforestation (Rao et al., 2015). Advocates for climate change mitigation encourage these measures to sustain or improve carbon stocks in actual or potential livestock grazing lands of the humid tropics. They also stress the importance and potential of capturing C in the soil, most prominently promoted by the global “4 × 1,000” initiative (Minasny et al., 2017). A growing body of science literature shows that land-use changes and improved grazing practices can build up soil organic carbon (SOC) stocks (Conant et al., 2001, 2017; Guo and Gifford, 2002; Don et al., 2011; Kumar et al., 2018; Xu et al., 2021; Ayarza et al., 2022). Because of their extent and predominant land uses, savanna ecosystems are one major focus of efforts to use grassland management practices to sequester C in the soil.
Previous studies in the grasslands of Colombia's Orinoco region have shown enormous potential for increasing SOC under well-managed pastures in the low-fertility acid soils of the Eastern High Plains savannas (Fisher et al., 1994, 2007; Rao et al., 1994; Rao, 1998; Fisher and Thomas, 2004; Trujillo et al., 2006). Fisher et al. (1994) found 70.4 Mg ha−1 more SOC in grass-legume (Brachiaria humidicola + Arachis pintoi) pastures and 25.9 Mg ha−1 more SOC in grass alone (B. humidicola) pastures compared to native savanna, at one of the two experimental sites in acid soils of Colombia's Eastern High Plains. They estimated an annual rate of SOC accumulation of 7.8 and 4.3 Mg ha−1 yr−1 in grass-legume and grass alone pastures, respectively, emphasizing the importance of nitrogen (N) supply in improving the rate of SOC accumulation. These are high rates of SOC accumulation compared to results from research conducted on other grazing lands (Conant et al., 2001, 2017).
However, researchers conducted these SOC storage studies described above in only a few experimental sites, lacking geographic representation across acid soils of Colombia's Eastern High Plains region. Nor did the earlier studies validate changes in SOC stocks through on-farm evaluations. These limited results from previous studies hinder their utility for guiding agricultural management and policy. Conversely, comparable findings from comprehensive research at multiple sites throughout the Colombian savannas would support a robust strategy for SOC storage in acid soils of the region. Introduced pastures could improve livestock productivity while reducing GHG emissions (Rao et al., 2015; Ayarza et al., 2022; Ramírez-Restrepo et al., 2023). Farmers and livestock keepers could incorporate introduced African grasses, legumes, fertilizer management and rotational grazing as pasture management strategies to increase SOC storage, improving related soil physical, chemical and biological properties as well (Amézquita et al., 2004; Ayarza et al., 2022). These well-managed pastures could mitigate GHG emissions by capturing CO2 from the atmosphere, and by reducing methane (CH4) and nitrous oxide (N2O) emissions, compared to lower quality or degraded pastures (Peters et al., 2012; Rao et al., 2015; Ramírez-Restrepo et al., 2023).
Confirming the high SOC storage results of earlier research would also imply an opportunity for Colombia to strengthen its national emissions reduction strategy (Arango et al., 2020). Only eight countries include SOC sequestration targets in their Intended Nationally Determined Contributions (INDC) toward emissions reduction (CCAFS, 2016). Colombia could join this group by developing strategies to store SOC in Eastern High Plains soils, while also improving agricultural productivity. Recognizing this potential and opportunity, Colombia's Ministry of Agriculture and Rural Development (MADR) commissioned research assessing the potential for SOC sequestration in grazing lands in the Eastern High Plains of the Orinoco region (CIAT Corpoica, 2017).
The International Center for Tropical Agriculture (CIAT) and Colombia's national agricultural research corporation (current Spanish acronym is AGROSAVIA but called Corpoica until 2018) joined efforts in 2011 to investigate SOC storage potential beyond the experimental sites studied in previous research (CIAT Corpoica, 2017).
The CIAT-Corpoica research team pursued four research objectives:
(1) First, our team sought to evaluate the SOC stock values in one experiment station at Colombia's Eastern High Plains. Have the high SOC values found in the mid-1990s persisted 24 years later?
(2) Second, we wanted to determine if SOC stocks on farms under variable management are similar in magnitude to those found on research stations. What are the SOC stock levels in similar sites on farms in the Eastern High Plains region?
(3) Third, we sought to assess the potential for SOC storage at fine geographic resolutions using a digital soil map approach. Given levels of SOC accumulation found at sample sites, what is the potential for improving SOC storage at fine geographic resolution across the Eastern High Plains?
(4) Finally, the team considered scenarios and strategies for sustainable livestock intensification based on the findings. What are possible strategies for responsible and sustainable livestock production in the region?
These four research objectives mentioned above lead to this project's goal of assessing whether livestock intensification for SOC accumulation and storage is a worthwhile sustainable development effort in Colombia's Eastern High Plains.
Materials and methods
Research approach and sampling strategy
This approach allowed us to evaluate changes in SOC accumulation and storage across both time and space (Costa et al., 2013). One of the study sites was the same research plots from earlier work that showed high potential for SOC accumulation and storage (Fisher et al., 1994). Analysis at this site allowed us to evaluate SOC stocks over time (Objective 1). But we also selected commercial farms where we measured SOC stocks in 2011 and again in 2016 (Objective 2). Therefore, we measured SOC stocks over one long period at the experimental site (Carimagua) and over one short period on the farms. Our approach also substituted space for time by measuring SOC stocks on improved and degraded pastures, with a nearby native savanna reference site, as well as at 154 sites across the broad range of land uses (Objective 3).
We conducted this research in the Colombian Eastern High Plains in the municipalities of Puerto López and Puerto Gaitán, in a study area of 970,674 ha (Figure 1). The CIAT-Corpoica research team returned to one of the Fisher et al. (1994) sites to measure SOC stocks in the same plots 24 years later (Objective 1). To improve our understanding of SOC dynamics in pastoral systems beyond this experimental site (Objective 2), our team sampled soils on native savanna, improved but degraded Brachiaria (synonym: Urochloa; Kewscience, 2021) pastures, and on improved Brachiaria pastures under variable management conditions, in a paired study at eight sites in the Eastern High Plains region. Our research team also sampled 154 other sites under different land uses to produce a detailed geographic analysis, allowing us to estimate the overall SOC storage potential (Objective 3). The analysis employed the field data combined with environmental variables to create digital maps of SOC. Finally, we used the field data and secondary sources to produce simple scenarios and analyses suggesting a strategy for SOC storage and sustainable livestock production in the region (Objective 4).
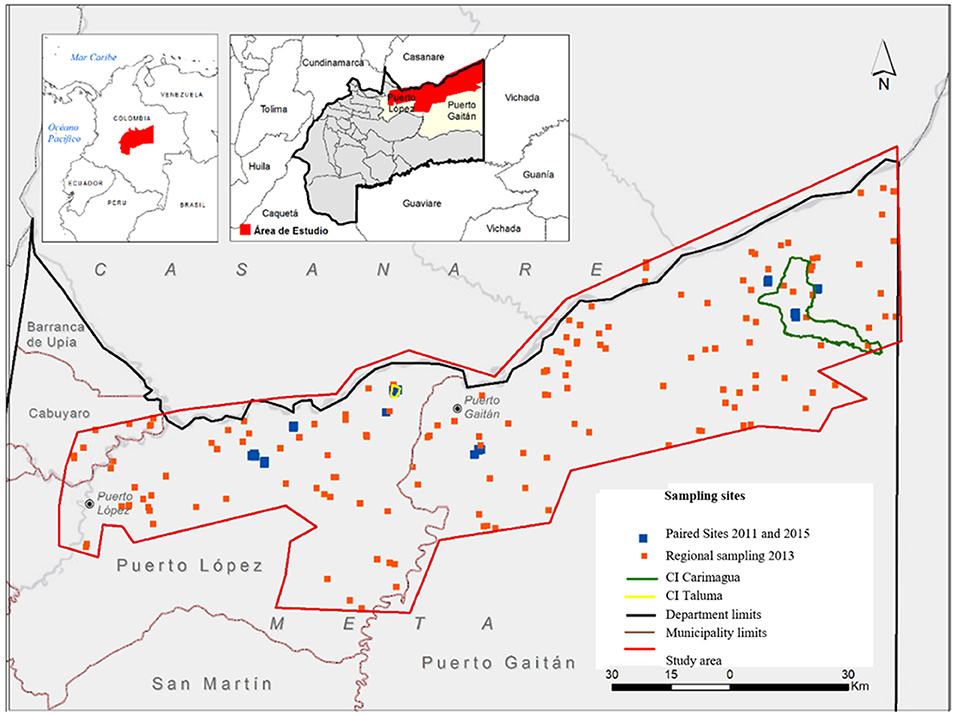
Figure 1. Study area and sampling sites in the Colombian Eastern High Plains, including paired sites to compare improved pastures with native savanna over short time periods and regional soil sampling at 154 sites.
Below, we describe our methods under the categories of (i) soil sampling (objectives 1, 2 and 3), (ii) digital soil mapping (Objective 3), and (iii) land use and agricultural practice scenario analysis (Objectives 4).
During the years 2011, 2013, 2015, and 2016, our research team sampled soils down to 1- or 2-meters depth from native savanna, improved pastures, and degraded pastures at the Carimagua research station and at eight commercial farms, as well as from 154 acid soil sites across the Eastern High Plains under different land uses (Figure 1). Under Objective 1. in the Carimagua research station (Corpoica, C.I. Carimagua) in 2016, the field team sampled to 2 meters soil depth distributed in twelve intervals: 0–5, 5–10, 10–20, 20-40, 40–60, 60–80, 80–100, 100–120, 120–140, 140–160, 160–180, and 180–200 cm, under the following land cover conditions: (1) Brachiaria humidicola, grass alone as the most common improved pasture in the region, (2) B. humidicola + Arachis pintoi, a grass-legume association, recommended in the region (Lascano, 1991) because of its significant impact on increasing cattle live-weight gain (LWG), and (3) a native savanna area, the predominant vegetation cover for cattle grazing in the region, used as the reference treatment. The introduced forage plots sampled (treatments 1 and 2) were the same used by Fisher et al. in 1994 in the first approach for estimating the potential SOC accumulation in the Eastern High Plains region. Fisher et al. (1994) sampled the experimental plots in 1993, having been established in 1987 following recommended tillage and fertilization. Research managers maintained the plots under rotational grazing with a carrying capacity of 3 animal units (AU) ha−1 yr−1 from 1988 to 1993, with annual burning from 1994 to 2004, and no grazing from 2004 to 2016. One animal unit (450 kg) is one animal head per hectare per year as stocking density, reflecting the intensity of grazing by animals. However, it is not known if land managers used fire during the last period. Locals noticed burning during the last period (with unknown frequency).
For Objective 2, paired sites (improved pasture and native savanna) on seven farms and one research station in the municipalities of Puerto López and Puerto Gaitán were sampled down to 1 m depth in 2011 or in 2016 (Table 1). Because of changes in land use between 2011 and 2016, we only report useful data from seven of the eight sites (Table 1). Livestock keepers confirmed that introduced grasses on the seven farms were sown at least 5 years before sampling. Only six of these seven sites are directly comparable, because one farm (La Menina) experienced exceptional pasture management. Farms were selected based on the following two criteria: (1) they had at least two different land uses among either managed and improved pastures, managed but degraded pastures (visually determined), or native savanna, and (2) similar soil texture in the land use areas selected for sampling. Livestock keepers and the research team classified the improved and degraded pastures based on pasture and animal productivity together with visual evaluation. Improved pastures were managed with the application of maintenance fertilizer and rotational grazing where possible. The research team could not always find native savanna reference plots with similar soil texture. We show the results for only six sites because land use changes prevented the use of data from two of the seven farms.
For Objective 3, we sampled soils under different land uses and geomorphology at 154 sites in the year 2013, with three replications at each site (Figure 1). The sampling soil depths were 0–5, 5–10, 10–20, 20–30, 30–40, 40–60, 60–80, 80–100 cm. These 154 points were selected randomly according to their proportion of area in a land-use map (Rodríguez et al., 2013). All 154 points were used for digital soil mapping (DSM). To understand SOC under different land uses, we report below only those sample locations for land use categories where we had four or more sample points for that category, thereby excluding land use categories with few measurements. However, all 154 sample points were used to construct the digital maps of SOC.
Soil sampling and analysis
Within each sampling location, three sites were identified and a 1 m2 frame was placed in each to sample five points: four from the vertices and one in the middle. At each point, a hydraulic sampler was introduced up to 1 or 2 m depth. The five sub-samples were homogenized to get one sample per soil depth needed for analysis. Additionally, a soil pit was excavated from the middle point to get samples for soil bulk density measurements (Figure 2).
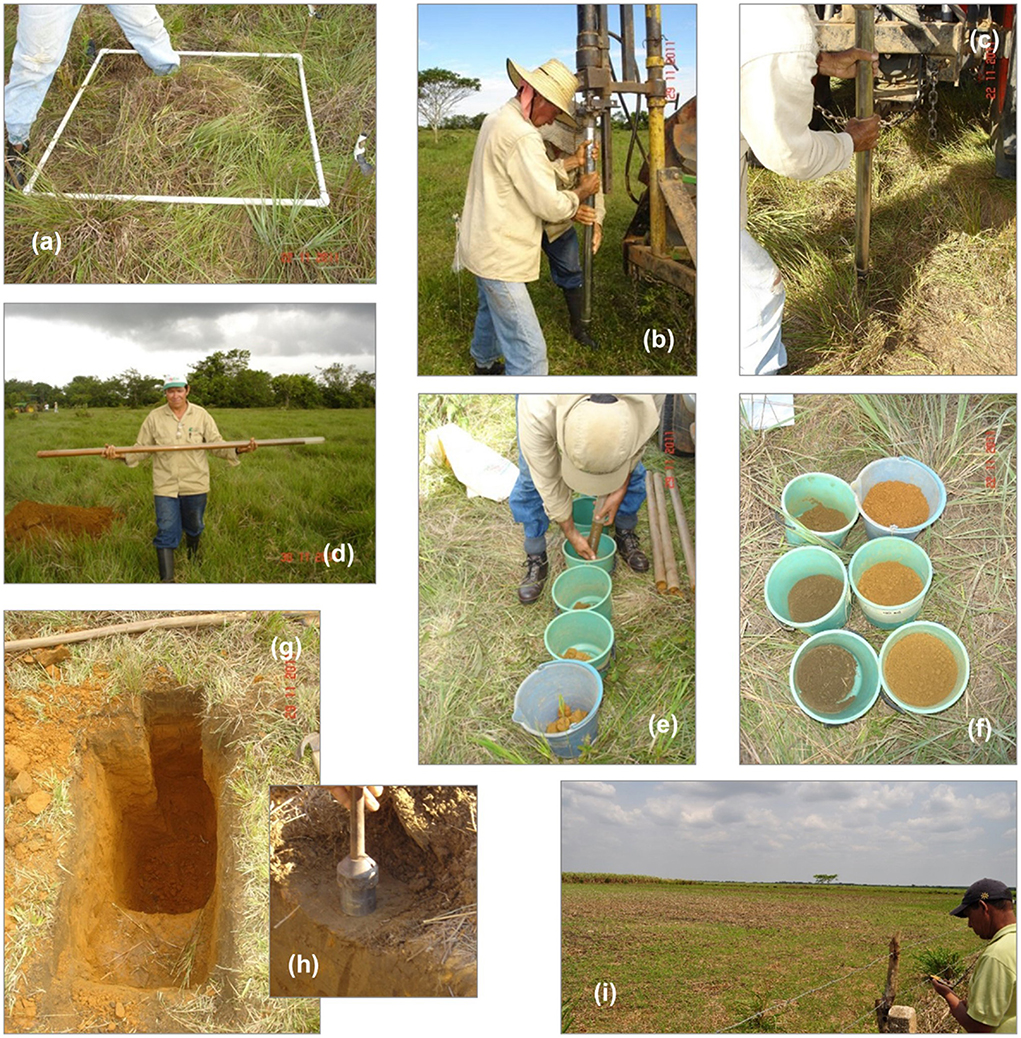
Figure 2. Sampling method to determine carbon storage in the Eastern High Plains region: (a) Frame placement in the sampling site; (b) Hydraulic sampling probe used; (c) Sampling probe introduced into the soil; (d) Sample extraction; (e) Sub-sampling extraction from each sampling probe per depth; (f) Composite sample per sampling depth; (g) Soil pit for bulk density determination; (h) Sample extraction for bulk density determination; (i) Georeferencing of each sampling plot.
Laboratory technicians at CIAT-Palmira homogenized and analyzed the samples by depth interval (dried at 65°C, ground and sieved). Soil organic matter was determined using the Walkley-Black method, modified by concentrated potassium dichromate combined with concentrated sulfuric acid to heat the samples at 120°C for 2 h. This method was used to maintain consistency between our results and those of Fisher et al. (1994) in Carimagua. The results obtained from using this method were validated by measurements made by the stable isotope laboratory at the University of California, Davis, USA. Our approach included bulk density (BD) estimation using the core method and SOC stock calculation by multiplying BD times SOC concentration and soil depth. The values reported on SOC stocks are corrected based on changes in soil BD of different soil layers.
Statistical analysis
The data were analyzed using the statistical analysis software SAS 9.4 (SAS, 2016). Two-way analysis of variance model was used where the land use and depths were the evaluation factors. We separated means using a Tukey test. When the sampling depth was 2 m the SOC was analyzed using 10 depths where the first three depths (0–5, 5–10, and 10–20 cm) were integrated at 0–20 cm.
Digital soil mapping
This study employed a digital soil mapping method to map SOC from point measurements described above to the entire study area. The method helped us to understand the geographic distribution of SOC stocks based on land use, as well as calculate the overall SOC storage potential per unit area and for the study area (Objective 3).
We calibrated and validated a digital soil mapping (DSM) model for the study area in the Eastern High Plains of Meta department, Colombia (Figure 1). The study area corresponds to the Rodríguez et al. (2013) land use map, an area to the south of the Meta River in Puerto López and Puerto Gaitán municipalities.
The digital SOC maps were developed using a DSM approach based on geomorphology and fuzzy logic (Zhu, 1997; Zhu et al., 2001; Ashtekar and Owens, 2013; Ashtekar et al., 2013). We used the Soil Landscape Inference Model (SoLIM) (Zhu et al., 1997, 2001) to perform the fuzzy logic classification. The approach defines rules for each geomorphic unit based on the normal distribution the terrain attributes slope, topographic wetness index, valley depth, and normalized altitude derived from the digital elevation model SRTM 30m (Moore et al., 1993; McBratney et al., 2003; Radula et al., 2018) and of the average annual precipitation, which is based on 1 km resolution data from the Climate Hazards Group InfraRed Precipitation with Stations (CHIRPS) archive (Funk et al., 2014). These data are calibrated with the Colombian Government data (Institute of Hydrology, Meteorology and Environmental Studies, IDEAM) time series precipitation data for 1980–2009.
Because SOC is a dynamic property influenced by land use and management, we defined and compared the geomorphic units in two ways: (1) combining geology (scale 1:500,000 from Gómez et al., 2015) and landscape forms maps, and (2) combining geology, landscape forms, and land use and land cover (scale 1:25,000 from Rodríguez et al., 2013) maps. The Geomorphons add-on in Grass-GIS software generated the landscape forms map, using an algorithm that divides the landscape into nine forms: flat, peak, ridge, shoulder, spur, slope, pit, foot slope, and hollow (Jasiewicz and Stepinski, 2013). The SOC was mapped at 30 m resolution and different soil depths (0–5, 5–10, 10–20, 20–30, 30–40, 40–60, 60–80, 80–100, and 0–100 cm) using the data from the 154 sampled sites (Figure 1) in the equation proposed by Zhu et al. (1997).
The normalized root-mean-square error (RMSE) was used to assess and compare models (with and without land use) in the different soil depths. The RMSE was calculated with 20% of the data not used in model calibration, normalized by field data variation.
Land use and agricultural practice scenarios
Our scenario analysis includes estimates of how land use would change between 2010 and 2042 from baseline conditions and scenarios showing sustainable livestock intensification strategies (Objective 4). The analysis considers potential changes based on improved pastures and removing some land from grazing within the Eastern High Plains region (Figure 1). Understanding the baseline scenario and developing plausible land-use scenarios for the future helps us consider whether the SOC storage potential merits directed land-use changes to realize that potential.
For developing the baseline scenario, land use change rates were combined with calculated areas of land use categories from the Rodríguez et al. (2013) map of the Eastern High Plains. The land-use change rates were developed from Colombian Government data between 2010 and 2015 (MADR, 2016). The rates of change were then applied to different land use categories, projecting values for the period from 2010 to 2042.
To develop plausible livestock development scenarios, we estimated SOC stocks, aboveground carbon stock in secondary forests and changes in livestock stocking rate of improved and well-managed pastures. SOC stock values were based on this study for native savanna and for introduced pastures comprising Brachiaria grass. Previous research in this region provided measures of stocking rate expressed as the number of AU per hectare (Lascano, 1991; CIAT, 2016). We estimated aboveground carbon stock in a secondary forest from one site at the Carimagua research station, using soil sample and vegetation analysis (CIAT, 2017).
The analysis considered three potential scenarios to reduce emissions from the Eastern High Plains region. Our first scenario included conversion from degraded pasture area to improved pasture. The second scenario converts degraded pasture plus an equivalent area of native savanna to improved pasture. The third scenario combined the conversion of all degraded pasture areas to improved pasture, native savanna to improved pasture and native savanna protected from fire and livestock grazing to secondary forest.
Our scenarios assessed effects on improving SOC storage and livestock productivity by estimating annual carbon stock change and accompanying stocking rate change in introduced pastures, both well-managed and degraded pastures. One scenario includes conversion of native savanna to secondary forest, permitted by eliminating anthropogenic fires and cattle grazing. The following assumptions were used to estimate the impact of the three mitigation scenarios: (i) because our map does not distinguish degraded pastures from other introduced pastures, the scenarios assume 50% of pastures are degraded, in line with previous estimates (López-Hernández et al., 2005); (ii) converting degraded pastures or native savannas used in livestock production to improved pasture (introduced) will accumulate SOC up to 1 Mg C ha−1 yr−1 in the soil, based on this study's results at Carimagua research station over 29 years, rounded up from 0.96 Mg C ha−1 yr−1; (iii) native savanna converted to secondary forest will accumulate up to 0.6 Mg C ha−1 yr−1 aboveground, according to our field and laboratory research (CIAT, 2017); (iv) the assumed cattle stocking rate in native savanna, degraded pasture and improved pasture is 0.12, 1 and 2 AU ha−1, respectively (CIAT, 2016); and (v) the proportion of land in native savanna could only be reduced to 25% from its 2010 proportion of 39%, a 14% reduction that is plausible over short to medium time frames.
The baseline condition of land use corresponded to the 2010 values reported by Rodríguez et al. (2013) for 970,674 ha for the Eastern High Plains region. From the total area, about 375,000 ha (39%) corresponds to native savanna, 44,000 ha (5%) to secondary forests and 117,000 ha (12%) to introduced pastures, from which half was considered degraded. The other 434,000 ha (45%) consists of gallery forests, annual crops and tree plantations, and other land uses.
The scenarios included only carbon dioxide (CO2) emissions, excluding methane (CH4) emissions from cattle and nitrous oxide (N2O) emissions from animal residues and soil. The results also were compared with information for the agriculture, forestry, and other land use (AFOLU) sectors that the Colombian government submitted as part of the United Nations Framework Convention on Climate Change (IDEAM, 2016).
Results
Below we summarize our results for SOC stocks from the field and laboratory estimates, digital soil maps showing SOC variability and potential, and our scenario analysis for understanding the potential impacts of possible land-use changes in Colombia's Eastern High Plains.
Soil organic carbon estimates
Soil organic carbon under experimental conditions: Carimagua research station
Research aimed at verifying mid-1990's studies at experimental sites (Objective 1) found that in 2016, two improved pasture arrangements (B. humidicola and B. humidicola + A. pintoi) introduced 29 years before at Carimagua research station had higher SOC content compared to native savanna, particularly at depth of 0–100 cm (Table 2; Figure 3). There were also differences at sampling depths and in treatment interaction by depth (just for SOC concentration). We found the strongest difference at depths of 0–20 cm. Taking as a reference the SOC storage in the native savanna, improved pastures accumulated on average 0.94 Mg ha−1 yr−1 to depths of 2 m, over the 29 years after establishment of pastures. SOC accumulation in the top 100 cm with B.humidicola or B.humidicola + A.pintoi accounted for 94.8% (0.91 Mg ha−1) to 93% (0.84 Mg ha−1) of the SOC increase compared to native savanna, respectively (Table 2). There were no differences between the SOC accumulated by the two options of improved pastures in evaluation at depths of 0–200 cm.
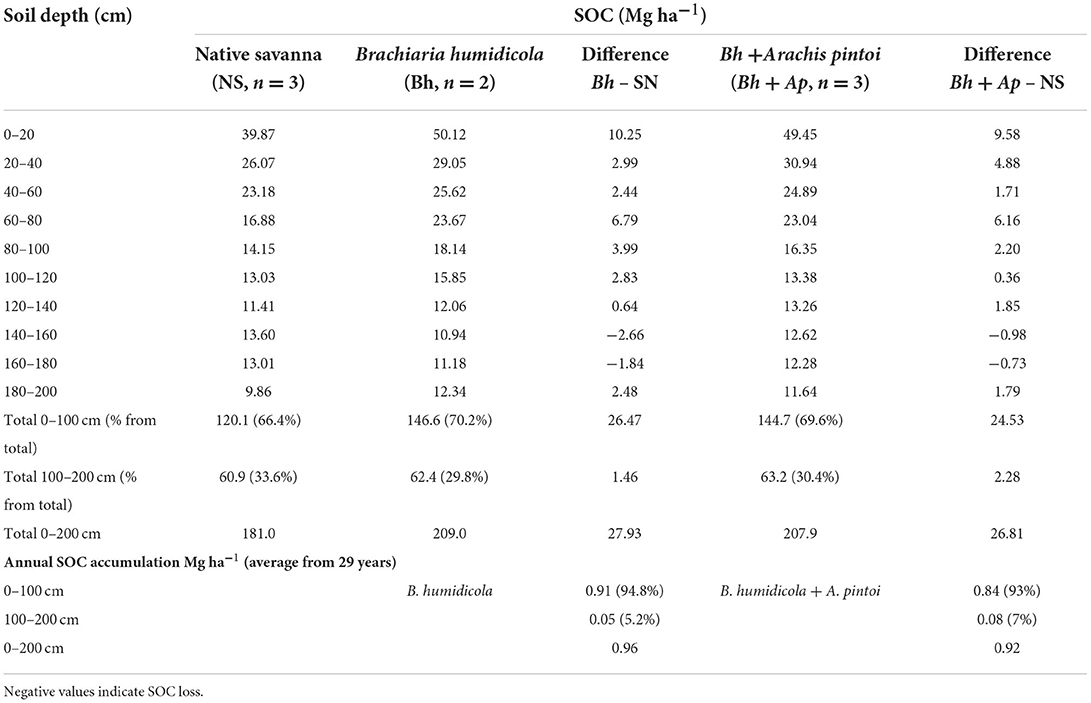
Table 2. Soil organic carbon (SOC) stock (Mg ha−1) in different soil layers up to 2 m depth in pasture sites with Brachiaria humidicola (Bh), B. humidicola + Arachis pintoi (Ap) and native savanna (NS) at the Carimagua research station in 29 year period, sampled in 2016.
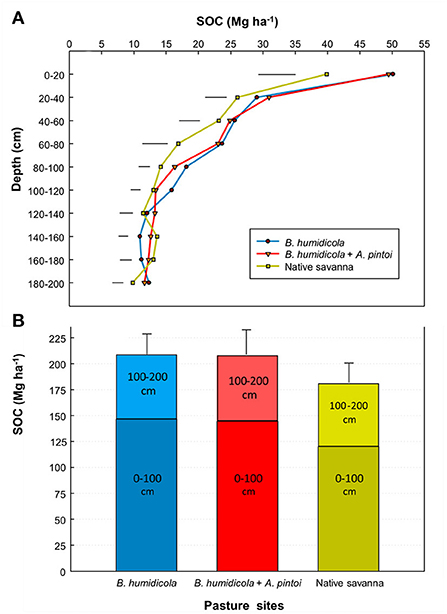
Figure 3. Soil organic carbon (SOC) stock (Mg ha−1) in soil profile up to 2 m depth (A) and the total SOC storage in the pasture sites Brachiaria humidicola, B. humidicola + A. pintoi and native savanna at the Carimagua research station (B).
Over 29 years (1987–2016) SOC storage at Carimagua research station increased by 27.4 Mg ha−1 in B. humidicola pasture and by 26.8 Mg ha−1 in B. humidicola + A.pintoi, compared to native savanna (Table 2), suggesting a potential SOC accumulation of around 0.96 and 0.92 Mg ha−1 yr−1, respectively, even under inadequate management conditions for half of the total period.
Paired site comparison for SOC at six sites in the Eastern High Plains
Research for evaluating SOC stock on farms, beyond experiment stations (Objective 2), found pasture management in the farms was highly variable (Table 1). In five of six sampled farms, SOC storage increased 4 years after the first sampling in the three land-use and management options evaluated (Figure 4A). On farms such as El Porvenir, El Tranquero and Carimagua, the higher amounts of SOC accumulated compared to native savanna between 2011 and 2016 suggest higher SOC storage potential with adequate management of improved pastures. These results, however, were inconsistent with those observed in the other three farms. On average, SOC stocks increased between 2011 and 2016, with improved pastures showing higher values compared to degraded pasture and native savanna (Figure 4B). In well-managed improved pastures, four of the six sites showed SOC accumulation, while two of them lost SOC between 2011 and 2016 (Figure 4C). None of the sites showed a reduced SOC accumulated in native savannas. The best managed farm (La Menina) was not directly compared because its owners employed exceptional management (Figure 4D). For this farm, land managers applied 300 kg ha−1 yr−1 of 18-18-18 (N-P-K) fertilizer to B. humidicola cv. Llanero for seed production, resulting in an annual SOC accumulation of 1.7 Mg ha−1 (Figure 4D). In this farm, higher SOC accumulation at depth of 0–20 cm suggests a positive response to fertilization in terms of net primary productivity in well-managed improved pastures, compared to the same treatment under traditional management.
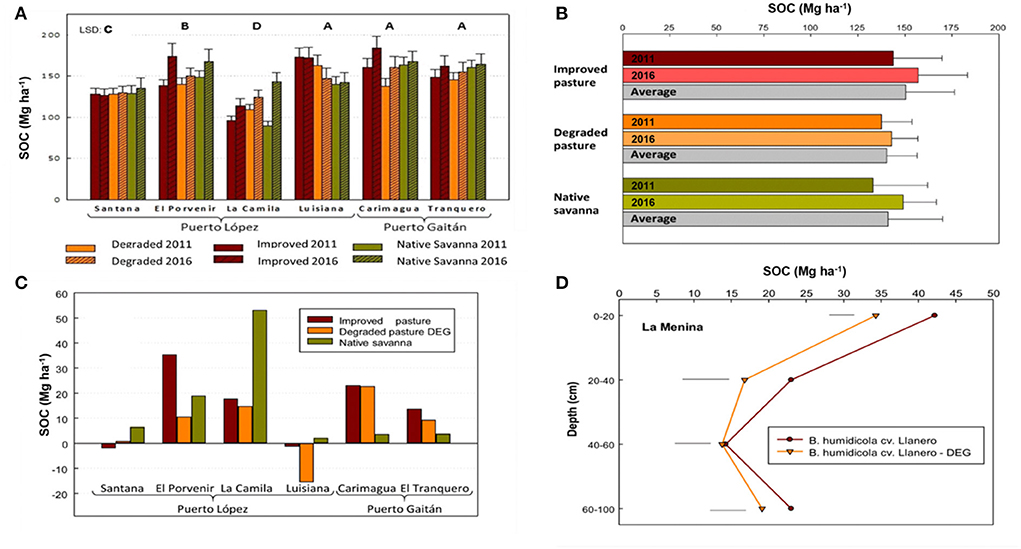
Figure 4. Paired site study results. (A) SOC stock (Mg ha−1) up to 1 m depth in six commercial farms with improved pastures (well-managed pasture), degraded pasture, and native savanna in the Eastern High Plains; (B) averages for these six farms; (C) SOC stock change (Mg ha−1) in 5 years (2011–2016) in the six farms, with positive values indicating SOC accumulation and negative values indicating SOC loss; (D) SOC stock (Mg ha−1) up to 1 m depth in improved (well-managed) and degraded pastures (DEG) located in “La Menina” farm.
Digital soil mapping
Digital soil mapping results aim to portray the fine spatial resolution of SOC stocks from sampling at 154 sites under different land uses and then use those measurements to create interpolated maps of the study area (Objective 3). Below we describe the results from each of the sites, and then from mapping that interpolated values between sites.
Soil organic carbon at sites dispersed across the region
For analyzing SOC stocks by land use, from the 154 sampled points we report here data of 123 points sampled across eight land use categories. The other 31 sampled points are not included since we only report land uses where we made observations for four or more locations in a category (Figure 5). The graph shows the average SOC stock of points for each category, with the minimum and maximum values indicated. Five of the eight categories had fewer than 10 sample points. All these five categories had trees in the landscape. Compared to the average SOC stock in native savanna soil of 83 Mg ha−1, these five categories had between 30 and 60% more SOC. However, several of the categories—oil palm, rubber, gallery forest, forest plantation and pastures with trees—should be treated with caution due to the low number of samples. The two remaining land use categories had 24 or more sample sites. Crop-pasture mosaic (n = 28) and introduced pasture (n = 24) had 30% more SOC than native savanna (n = 42).
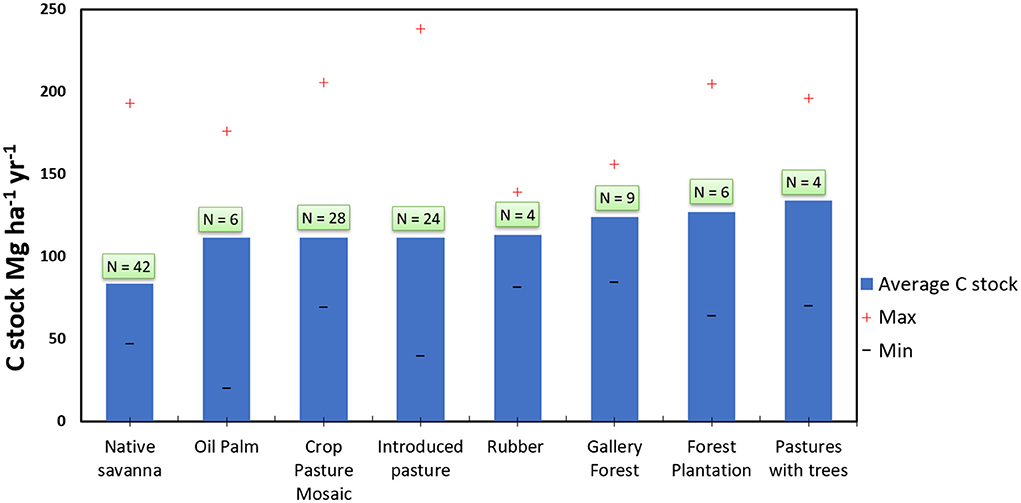
Figure 5. Regional soil sampling results for 123 sites across eight land use categories, showing the average SOC stock (Mg ha−1), with the maximum and minimum values indicated.
Maps of SOC
The DSM demonstrated the importance of land use in SOC storage determinations. Using land use as a variable improved model performance for predicting SOC storage (Table 3). The land use variable had a major effect predicting SOC in the surface layers (< 40 cm), reducing the RMSE between 7 and 20%. The model with and without land use had differences at soil depths between 0–40 cm. Model precision increased by 20% in the surface layers when including land use, allowing estimates of SOC reserves with high precision and resolution.
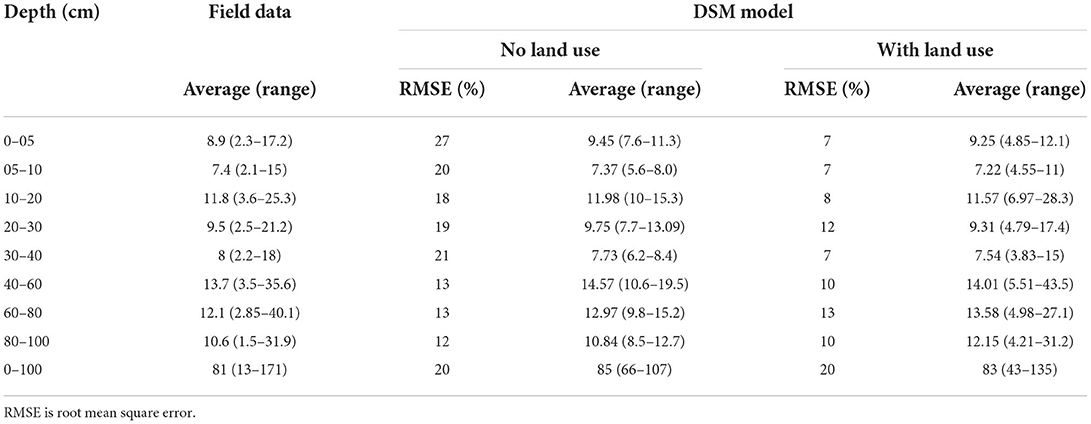
Table 3. Digital soil mapping (DSM) model performance with and without soil use to estimate soil organic carbon (SOC) at different soil depths.
The final model used suggested that by 2016 the study area in the Colombian Eastern High Plains region had an estimated SOC reserve of 0.08 Gt, with the SOC content ranging between 43 and 135 Mg ha−1 up to 1 m depth (Figure 6A). SOC stock also varied with depth (Figures 6B–F) following a decreasing pattern where the higher SOC reserves were found in the surface layers. The soil depths 0–20 and 20–40 cm contributed 62% of the total SOC stock up to 1 m (0.05 Gt) while the deeper layers (>40 cm) store 38% (0.03 Gt) of the total SOC in the soil profile (Figure 7).
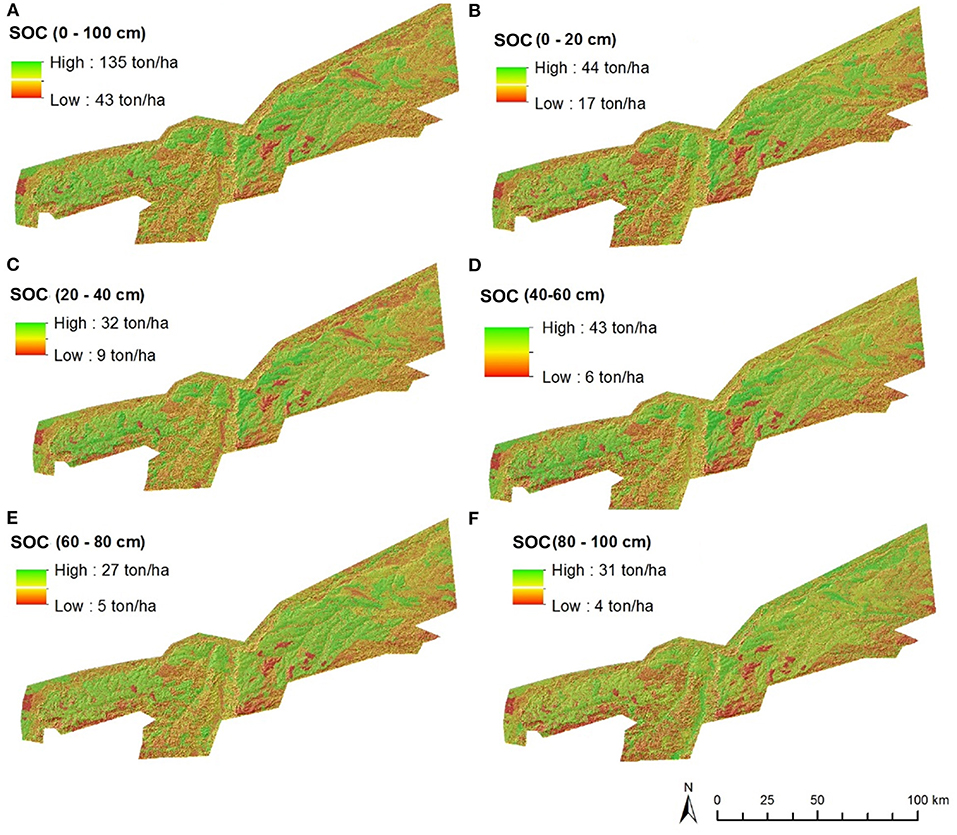
Figure 6. Spatial distribution of soil organic carbon (SOC) storage for depths of 0 - 100 cm (A), 0 - 20 cm (B), 20 - 40 cm (C), 40 - 60 cm (D), 60 - 80 cm (E), and 80 - 100 cm (F) in the Colombian Eastern High Plains.
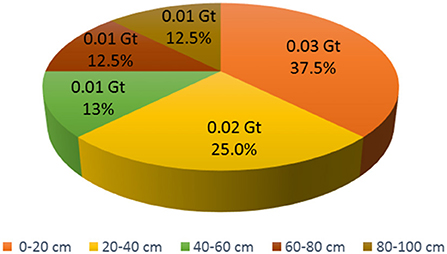
Figure 7. Soil organic carbon (SOC) reserves at different soil depths in the Colombian Eastern High Plains.
The digital soil maps show high variability of SOC stocks to a depth of 1 m across the study area, with values ranging between 43 and 135 Mg ha−1 (Figure 6A). Maps predict higher SOC stock values for about one third of the study area, in relatively homogenous areas as shown in green color in Figure 6. Most of the study area falls into an intermediate class of values, shown as a speckled pattern of green, yellow, and red pixels. Several areas show a clustering of low SOC stock values shown as red pixels.
Strategies and scenarios of land use change and impact on SOC storage
Research results were used to project and speculate on plausible scenarios for sustainable livestock intensification (Objective 4). Projected land use changes (Figure 8) in the High Plains of the Meta department of Colombia suggest land users will decrease native savanna and natural forest area during the three decades following 2010. Forest area could be reduced from 18 to 10% of the total area. The category “mosaic” shows a slight decrease in projected area in the region. These trends project an increase in areas of improved or well-managed pastures, annual crops, and forest plantations. Degraded pasture area is missing from Figure 8 because we do not know the extent of these pastures. However, degraded pasture area will probably increase, implying more GHG emissions. Overall, the land-use change projections show reductions in land uses with lower human interventions (forest and native savanna) and an increase in high human-footprint crops, degraded lands and forestry plantations.
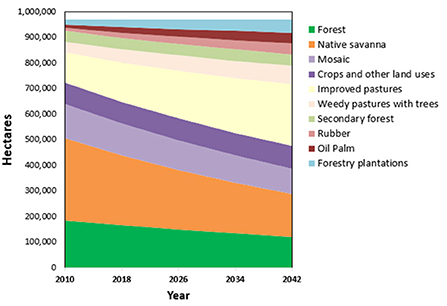
Figure 8. Land use change projection in the Eastern High Plains region from the department of Meta, for years 2010 to 2042, based on Rodríguez et al. (2013) and statistics from Colombian government (MADR, 2016).
The three scenarios proposed showed increases in SOC stocks and herd size (Table 4). Compared to values estimated for the 2010 land use map, the first scenario, which only converts degraded pastures to improved pastures, would increase SOC stocks by over 58,000 Mg. The second scenario, which converts the degraded pastures and some native savanna area to introduced pastures, would increase SOC stocks by more than 117,000 Mg. Finally, the third scenario would increase SOC stocks by more than 138,000 Mg.
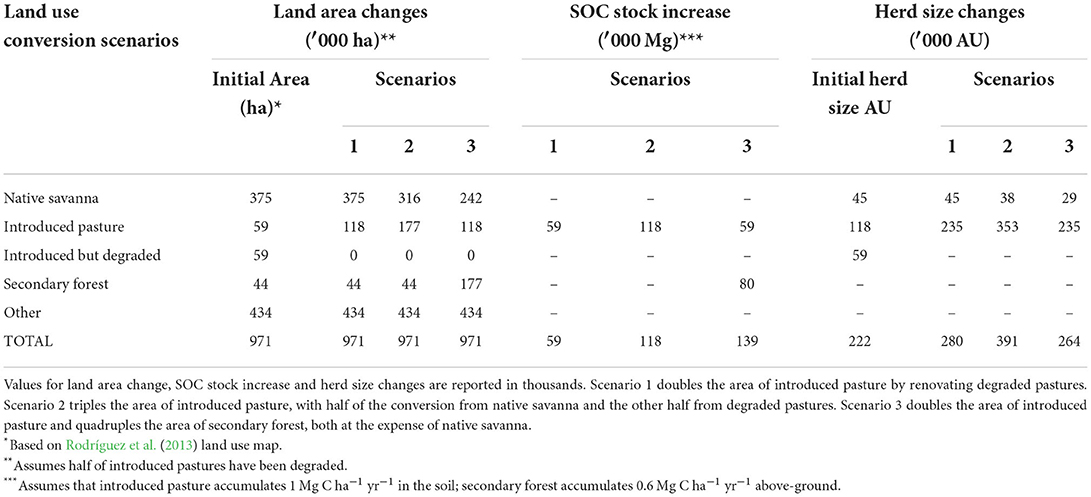
Table 4. Impact of the proposed scenarios on carbon reserves, emissions from the agriculture, forestry, and land use (AFOLU) sector, and herd size.
These estimates of SOC stock increase can be considered in the context of Colombia's national AFOLU emissions and regional herd size changes (Arango et al., 2020). The first scenario had an increase in the carbon stocks of around 0.30% of the national emissions from the AFOLU sector and increase in the regional herd size by 21%. The second scenario increases SOC reserves equivalent to 0.50% of national AFOLU sector emissions, with a regional herd size increase of 76%. In the third scenario, the change in SOC storage was around 0.60% of the emissions from the AFOLU sector, with an increase of nearly 20% in herd size. These projections show the trade-offs between (1) renovation of degraded pastures and protection of native savanna in the first scenario, (2) degraded pasture renovation and conversion of native savanna in the second scenario, and (3) renovation of degraded pastures and conversion of native savanna to secondary forest in the third scenario.
Discussion
Evaluating SOC under experimental conditions at Carimagua research station
SOC at the experimental site accumulated during years when the pasture was well-managed, decreasing subsequently with lack of intervention. Fisher et al. (1994) estimated SOC accumulation rate of 4.3 Mg ha−1 yr −1 down to 0.8 m depth between 1987 and 1993 in grass alone pastures (Table 5). The higher SOC accumulation rate during these years could be attributed to proper management in terms of use of improved forage options and their establishment as pastures, establishment and maintenance fertilizer application, rotational grazing with a stocking rate of 3 AU ha−1 yr−1. Lower SOC storage values and net carbon loss at the same plots in the period after Fisher's study (1994–2016) likely reflect lack of adequate management, in terms of maintenance fertilizer application and proper grazing management that might have affected net primary productivity (above- and belowground), root turnover and nutrient cycling (Ayarza et al., 2022).
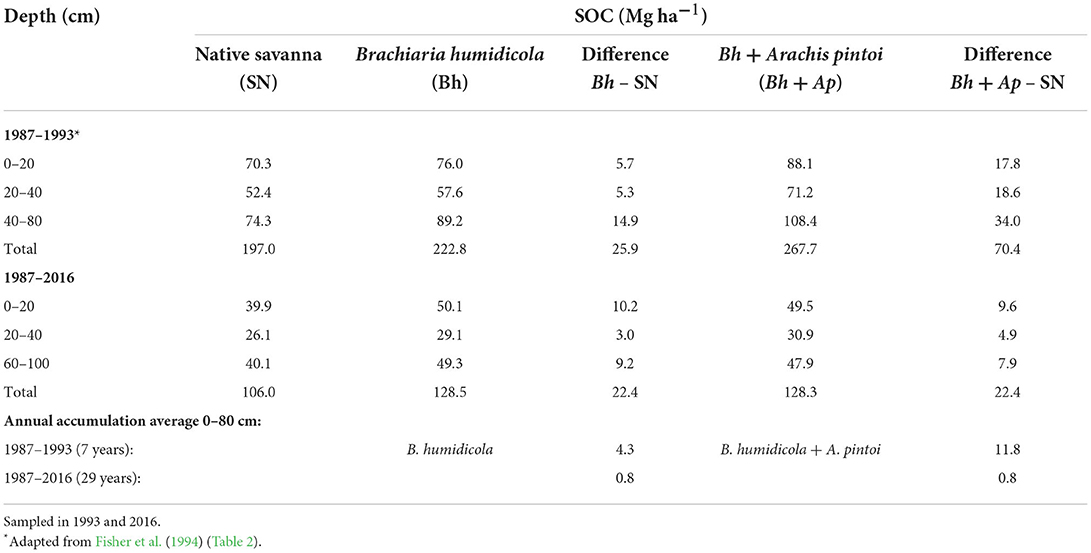
Table 5. Soil organic carbon (SOC) storage up to 0.8 m in pasture sites with Brachiaria humidicola, B. humidicola + A. pintoi and native savanna at Carimagua research station.
Summing up our evaluation of the early promising SOC accumulation at the experimental site (Objective 1), we found that optimal management built up SOC stocks between 1987 and 1993, with inadequate management after 1993 leading to subsequent losses. However, these SOC stocks did not revert to levels found in native savanna, showing the potential for storage over time. While the high levels of SOC stock found in earlier research at Carimagua research station were not maintained, they still yielded almost 1 Mg ha−1 of SOC accumulation over nearly three decades. Damian et al. (2021) used field data collected from three regions with contrasting climatic conditions in Brazil. They used the DayCent model and simulated the effects of converting poorly managed pastures (PMP) to more intensive and diversified systems of pasture management including fertilized pasture (FP). The DayCent model estimated that the conversion of PMP to FP increases the soil C stocks by 0.95 Mg ha−1 yr−1. The model also estimated that the fertilization of the pasture every year (FP) could result into higher SOC stocks. Damian et al. (2021) results taken together with observations made by Fisher et al. (2007) and Ramírez-Restrepo et al. (2023) indicate that the major factors contributing to greater SOC accumulation in improved pastures compared to degraded pastures are greater net primary productivity of both above- and belowground biomass as well as greater root turnover.
Current research considers the potential SOC storage values observed at Carimagua research station under improved pastures as similar to values reported in other tropical savannas (Fisher et al., 1994, 2007; Rondón et al., 2006; Lavelle et al., 2014; Strassburg et al., 2014). The values are higher than those observed in the Brazilian Cerrado in which the soil and climatic conditions are different (Ayarza et al., 2022). Improved pastures could play an important role in increasing net primary productivity and SOC storage potential following a sustainable intensification approach (Rao et al., 2015). The decreased SOC at 0–80 cm depth in the native savanna, B. humidicola and B. humidicola + A. pintoi (54, 58, and 48%) after 1994, when the management was reduced to a minimum, emphasizes the importance of continuous pasture management for increasing and sustaining SOC storage potential. Indeed, other recent research in the Eastern High Plains has confirmed high SOC accumulation under proper pasture management (Costa et al., 2022).
SOC assessment under commercial farms, variable management and non-experimental conditions
SOC storage on commercial farms showed that, on average, SOC stocks were greater on improved pastures compared to native savanna (Figure 4). They were 10 Mg ha−1 higher on improved pastures compared to degraded pasture or native savanna. SOC stocks also increased over time between 2011 and 2016 on improved pastures. But they also increased in native savanna. These mixed results suggest processes and circumstances not captured by the comparisons. For example, on farms such as Santana and Tranquero, native savanna SOC stocks were greater than on improved pastures. The results suggest that introducing improved grasses alone, without improved soil management, does not guarantee the SOC increase seen under experimental conditions. However, livestock keepers rarely used soil amendments and fertilization to increase and/or sustain pasture and animal productivity. The animal carrying capacity in the sampled farms was also variable. On the one farm where fertilizer was applied every year (La Menina; Figure 4D), soils accumulated SOC at a rate of 1.7 Mg ha−1 yr−1, over three times higher than average increases found in a meta-analysis of studies evaluating measures to increase SOC storage (Conant et al., 2017). Therefore, although the results suggest a good potential for SOC storage at the regional level, pasture and grazing management strategies will determine whether SOC accumulates to high levels.
Regional soil sampling and digital soil mapping at fine geographic resolution
SOC under different land uses that are relevant for the region showed general agreement with the Carimagua research station and the paired sites analysis (Figure 5). SOC stocks in improved pastures and the crop pasture mosaics were more than 30% higher than that of native savanna. The other five categories showed SOC stocks also considerably higher than native savanna. Unfortunately, we had fewer than 10 sites for each of the five tree-based categories—oil palm, rubber, gallery forest, forest plantation and pastures with trees. While these data suggest that tree systems have higher SOC stock than native savanna, this result should be considered tentative until further investigation compares these land uses.
SOC measurements from this study agreed with other research in the South American savannas. The differences in SOC stocks between native savanna and Brachiaria pastures found in this study were similar to those found in the Brazilian Cerrado (Braz et al., 2013; de Freitas et al., 2020). Six paired comparisons in this study are also in line with the de Oliveira et al. (2022) analysis of 169 paired sites in Brazil, suggesting that grassland recovery and improvement can accumulate substantial SOC. Overall, the results from this study agreed with Ayarza et al. (2022) for South American acid soil savannas, showing that well-managed pastures with improved tropical forage grasses and legume species can accumulate significant amounts of SOC in the soil.
The DSM modeling showed how land-use is an important variable in predicting changes in SOC stock. When land use was included in the model, the prediction errors were reduced by as much as 20% in the upper layers of the soil. The maps in Figure 6 show a high degree of SOC stock variability throughout the region and at different soil depths. In those areas with lower SOC stock values, there may be better opportunities to increase SOC stocks by changing land use and/or improving strategies of soil management. Over half of the study area is grazing land, with around 80% of that land as native savanna. Any conversion to improved pastures in these areas, particularly with appropriate soil and grazing management, has the potential to increase SOC stocks by establishing land use options with high potential for carbon accumulation in the first 40 cm of soil. About 20% of the grazing land is in improved pastures. One observer estimates that half of all areas in improved pastures have been degraded (López-Hernández et al., 2005). Therefore, these degraded areas represent another opportunity to increase SOC stocks through pasture renovation for regenerative agriculture (Newton et al., 2020) or carbon farming (Jansson et al., 2021).
The maps produced in this study concur with a larger mapping exercise showing low SOC stocks in many parts of the Orinoco region (Rainford et al., 2021). Clearly, Eastern High Plains land users can increase SOC stocks over broad areas by converting native savanna to well-managed introduced pastures. However, native savanna conversion has generated controversy, as these areas are the focus of biodiversity conservation efforts. That's one reason why, in the short term, a better focus may be to improve pasture areas where African grasses have already been introduced but are now degraded. About 10% of the Eastern High Plains study area, where introduced grasses have been degraded, would be a top priority for improving SOC storage potential for effective carbon removal from the atmosphere. In the medium term, as native savannas continue to be converted to other land uses for regional development and/or national food security, incorporation of new grazing areas could consider use of introduced, well-managed pastures to enhance food production and support Colombia's policies on climate change.
The average SOC reserve for the mapped area is 0.08 Gt of C up to 1 meter soil depth and equivalent to 0.29 Gt of CO2 (using the standard conversion factor of 3.67). This is four times the CO2 net emissions reported by Colombia in the AFOLU sector in 2012 (IDEAM, 2015), showing the importance of adequate land use planning and sustainable management to avoid C emissions. This study agrees with long term field trials in the Western Kenya region showing that sustainable management practices such as integrated soil fertility management and conservation agriculture contribute toward mitigating SOC losses (Sommer et al., 2018). This study also aligns with the global analysis of Bossio et al. (2020) showing the importance of protecting existing soil carbon and rebuilding depleted SOC stocks as critical natural climate solutions (NCS) to reduce global warming.
Scenarios and strategies for sustainable livestock intensification
Globally, scientists welcome the role of SOC in NCS. Walker et al. (2022) emphasize C stocks in both soil and vegetation as part of NCS. In line with this study, their C maps show high potential for SOC storage on land in Colombia's Eastern High Plains. Our scenario analysis confirmed their estimates, showing several land-use conversions that could both increase productivity and reduce net carbon emissions (Table 4). The scenario changes to the ~1 million ha study area could reduce between 0.30 and 0.60 percent of national AFOLU emissions. Although based on experimental station results, these plausible changes could also increase herd sizes between 17 and 55 percent, providing a productivity benefit from higher stocking rates on improved pastures. With over 6 million ha of arable land in Colombia (World Bank, 2022), the changes envisioned in the scenarios could be scaled up to other parts of the country.
Our scenarios would only offset the CO2 emissions. Increasing the herd size and fertilized areas not only has an impact on CO2 emissions but also in CH4 and N2O emissions. Costa et al. (2022) showed N2O emission reductions with improved B. humidicola grass in a recent study in silty clay loam soils of the Eastern High Plains. Ramírez-Restrepo et al. (2020) predicted CH4 emissions, animal-environmental metrics and C footprint over the productive lifetime of the tropical beef cattle herds grazed on B. decumbens pastures and reported that under well-managed conditions, these pastures contribute to net C gain through SOC accumulation. While we need more research on in CH4 and N2O, there is clear potential to reduce emissions per unit product (meat and milk) following well-adjusted mitigation options (Peters et al., 2012; Havlík et al., 2014; Lerner et al., 2017; Ramírez-Restrepo et al., 2023; Vera-Infanzón et al., 2023). Improved Brachiaria based pastures could also reduce N2O emissions through biological nitrification inhibition (Byrnes et al., 2017; Costa et al., 2022).
Land use changes to accumulate SOC in the Colombia's Eastern High Plains region could have important effects on emissions reduction while maintaining economic productivity, under appropriate mitigation scenarios. This study found potential SOC storage on well-managed pastures when they are converted from degraded pastures or from native savannas used for livestock production. Protecting native savannas from grazing and fire has resulted in its conversion to secondary forests, positively affecting carbon sequestration (Torrijos et al., 2001).
Conclusion
This research showed large potential for SOC storage in Colombia's Eastern High Plains. Our results found less SOC storage than Fisher et al. (1994) found in the early 90s, suggesting that earlier high levels of SOC accumulation returned to a lower equilibrium value under inadequate management conditions. However, at nearly 1 Mg ha−1 yr−1 of SOC accumulation over the period of 29 years, this value is twice the average of that observed from a meta-analysis of the scientific literature on managed pastures (Conant et al., 2001, 2017). Our comparison of commercial farms showed that introduced and well-managed pastures can accumulate 25 Mg ha−1 more SOC than native savanna or degraded pastures. This potential depends on how well livestock-keepers manage their pastures. Our SOC maps showed plenty of opportunities to improve SOC storage throughout the region, while improving soil productivity. The values of SOC stock vary widely based on soil texture (Ayarza et al., 2022) with high potential to accumulate SOC in many parts of the study area. Renovating degraded pastures is the clearest strategy for re-stocking SOC and regenerating soil health. The scenario analysis showed how well-managed pastures could increase herd size per hectare, freeing up native savanna for forest regeneration and contributing substantially to reducing emissions.
More research is needed to estimate emissions due to land use change. Studies should evaluate those changes through time to fill the gap in knowledge on the acid soils of the Eastern High Plains region (Etter et al., 2010; Romero-Ruiz et al., 2012). This study examined SOC stocks at one site over a 29-year period, and on several farms over a period of several years. But research programs should be working at medium-term timescales with time frames over 10, 15, and 20 years. These studies need careful design and documentation of land use history and management. More research is needed on land-uses representing the full panorama of options for farmers, including forestry plantations, agro-silvopastoral systems and natural forest regeneration.
While some initiatives to sequester soil carbon have begun in the Eastern High Plains, more work is needed to understand how these initiatives can best be implemented. The Colombian government should consider how to improve SOC storage for accounting in its GHG emissions inventory, as well as programs to motivate farmers and ranchers to improve land use and soil conditions in the region. The Colombian government should consider joining the group of countries with dedicated strategies to reduce emissions by increasing SOC stocks.
This research project showed potential for acid soils of Colombia's Eastern High Plains region to replenish carbon in the landscape while improving agricultural productivity. These advances require implementation of highly productive and efficient crop-livestock and agro-silvopastoral systems that allow for SOC accumulation and reduce emission per unit product on agricultural lands while at the same time protecting and increasing tree cover on forest land (Amézquita et al., 2004; Lerner et al., 2017; Arango et al., 2020; Ayarza et al., 2022).
Data availability statement
The raw data supporting the conclusions of this article will be made available by the authors, without undue reservation.
Author contributions
AC led soil carbon analysis and interpretation. MD led analysis and interpretation of digital soil mapping work. GH led scenarios work. OP, JB, MA, AC, MD, GH, and IR contributed to the interpretation, writing and editing of the manuscript. All authors contributed to the article and approved the submitted version.
Funding
Funding and administration to support this research was provided by Colombia's Ministry of Agriculture and Rural Development (MADR) to the Colombian Agricultural Research Corporation, AGROSAVIA and the International Center for Tropical Agriculture (CIAT). We are grateful for the financial support from the Bezos Earth Fund project Using genetic diversity to capture carbon through deep root systems in tropical soils.
Acknowledgments
The authors thank Daniel Vergara for coordinating fieldwork, Sandra Loaiza for analysis of aboveground biomass, Cesar Botero for supervising laboratory analysis, and Silvia Elena Castano for mapping field sites. We are grateful for field support and guidance from Carlos Nagles, Ovidio Rivera, and German Escobar. Finally, the authors are grateful to the late Myles Fisher and others in his team at CIAT whose work on soil carbon in the Colombian Llanos awakened interest on this topic in Colombia and around the world.
Conflict of interest
Authors JB and OP were employed by Colombian Corporation for Agricultural Research (AGROSAVIA).
The remaining authors declare that the research was conducted in the absence of any commercial or financial relationships that could be construed as a potential conflict of interest.
Publisher's note
All claims expressed in this article are solely those of the authors and do not necessarily represent those of their affiliated organizations, or those of the publisher, the editors and the reviewers. Any product that may be evaluated in this article, or claim that may be made by its manufacturer, is not guaranteed or endorsed by the publisher.
Supplementary material
The Supplementary Material for this article can be found online at: https://www.frontiersin.org/articles/10.3389/fsufs.2022.954017/full#supplementary-material
References
Amézquita, E., Thomas, R. J., Rao, I. M., Molina, D. L., and Hoyos, P. (2004). Use of deep-rooted tropical pastures to build-up an arable layer through improved soil properties of an Oxisol in the Eastern Plains (Llanos Orientales) of Colombia. Agric. Ecosyst. Environ. 103, 269–277. doi: 10.1016/j.agee.2003.12.017
Arango, J., Ruden, A., Martinez-Baron, D., Loboguerrero, A. M., Berndt, A., Chacón, M., et al. (2020). Ambition meets reality: achieving GHG emission reduction targets in the livestock sector of Latin America. Front. Sustain. Food Syst. 4, 65. doi: 10.3389/fsufs.2020.00065
Ashtekar, J. M., and Owens, P. R. (2013). Remembering knowledge: an expert knowledge-based approach to digital soil mapping. Soil Horizons 54, 1–6. doi: 10.2136/sh13-01-0007
Ashtekar, J. M., Owens, P. R., Brown, R. A., Winzeler, H. E., Dorantes, M., Libohova, Z., et al. (2013). “Digital mapping of soil properties and associated uncertainties in the Llanos Orientales, South America,” in GlobalSoilMap Conference Proceedings, eds D. Arrouays, and N. McKenzie (Orleans; London. Taylor and Francis Group), 367–372.
Ayarza, M. A., Rao, I., Vilela, L., Lascano, C., and Vera-Infanzón, R. (2022). Soil carbon accumulation in crop-livestock systems in acid soil savannas of South America: a review. Adv. Agron. 173, 163–226. doi: 10.1016/bs.agron.2022.02.003
Bossio, D. A., Cook-Patton, S. C., Ellis, P. W., Fargione, J., Sanderman, J., Smith, P., et al. (2020). The role of soil carbon in natural climate solutions. Nat. Sustain. 3, 391–398. doi: 10.1038/s41893-020-0491-z
Braz, S. P., Urquiaga, S., Alves, B. J., Jantalia, C. P., Guimarães, A. P., dos Santos, C. A., et al. (2013). Soil carbon stocks under productive and degraded Brachiaria pastures in the Brazilian Cerrado. Soil Sci. Soc. Am. J. 77, 914–928. doi: 10.2136/sssaj2012.0269
Byrnes, R. C., Nùñez, J., Arenas, L., Rao, I., Trujillo, C., Alvarez, C., et al. (2017). Biological nitrification inhibition by Brachiaria grasses mitigates soil nitrous oxide emissions from bovine urine patches. Soil Biol. Biochem. 107, 156–163. doi: 10.1016/j.soilbio.2016.12.029
CCAFS (2016). Agriculture's Prominence in the INDCs: Data and Maps. CGIAR Research Program on Climate Change, Agriculture and Food Security. Available from https://go.nature.com/2QoRjSO (accessed August 26, 2021).
CIAT (2016). Annual Reports of the Tropical Forages Program. Available from: http://ciat-library.ciat.cgiar.org/Forrajes_Tropicales/index.htm (accessed August 26, 2021).
CIAT and Corpoica (2017). Carbon Sequestration in the Eastern High Plains of Colombia. Final Report of a Project funded by the Ministry of Agriculture and Rural Development. Bogota, 48.
Conant, R. T., Cerri, C. E., Osborne, B. B., and Paustian, K. (2017). Grassland management impacts on soil carbon stocks: a new synthesis. Ecol. Appl. 27, 662–668. doi: 10.1002/eap.1473
Conant, R. T., Paustian, K., and Elliott, E. T. (2001). Grassland management and conversion into grassland: effects on soil carbon. Ecol. Appl. 11, 343–355. doi: 10.1890/1051-0761(2001)011[0343:GMACIG]2.0.CO;2
Costa, C. Jr., Corbeels, M., Bernoux, M., Piccolo, M. D.C., Neto, M. S., Feigl, B. E., et al. (2013). Assessing soil carbon storage rates under no-tillage: comparing the synchronic and diachronic approaches. Soil Till. Res. 134, 207–212. doi: 10.1016/j.still.2013.08.010
Costa, C. Jr., Villegas, D. M., Bastidas, M., Rubio, N. M., Rao, I., and Arango, J. (2022). Soil carbon stocks and nitrous oxide emissions of pasture systems in Orinoquía region of Colombia: potential for developing land-based greenhouse gas removal projects. Front. Clim. 4, 916068. doi: 10.3389/fclim.2022.916068
Crippa, M., Solazzo, E., Guizzardi, D., Monforti-Ferrario, F., Tubiello, F. N., and Leip, A. J.N. F. (2021). Food systems are responsible for a third of global anthropogenic GHG emissions. Nature Food 2, 198–209. doi: 10.1038/s43016-021-00225-9
Damian, J. M., Matos, E. S., Pedreira, B. C., Carvalho, F. C.F., Premazzi, L. M., Williams, S., et al. (2021). Predicting soil C changes after pasture intensification and diversification in Brazil. Catena 202, 105238. doi: 10.1016/j.catena.2021.105238
de Freitas, I. C., Ribeiro, J. M., Araújo, N. C.A., Santos, M. V., Sampaio, R. A., Fernandes, L. A., et al. (2020). Agrosilvopastoral systems and well-managed pastures increase soil carbon stocks in the Brazilian Cerrado. Rangeland Ecol. Manag. 73, 776–785. doi: 10.1016/j.rama.2020.08.001
de Oliveira, D. C., Maia, S. M.F., Freitas, R. D.C. A., and Cerri, C. E.P. (2022). Changes in soil carbon and soil carbon sequestration potential under different types of pasture management in Brazil. Reg. Environ. Change 22, 1–11. doi: 10.1007/s10113-022-01945-9
Don, A., Schumacher, J., and Freibauer, A. (2011). Impact of tropical land-use change on soil organic carbon stocks–a meta-analysis. Glob. Change Biol. 17, 1658–1670. doi: 10.1111/j.1365-2486.2010.02336.x
Etter, A., Sarmiento, A., and Romero, M. H. (2010). “Land use changes (1970–2020) and carbon emissions in the Colombian Llanos,” in Ecosystem Function in Savannas: Measurement and Modeling at Landscape to Global Scales, eds M. J. Hill and N. P. Hanan (Boca Raton, FL: CRC Press), 383–402.
Fisher, M. J., Braz, S. P., dos Santos, R. S. M., Urquiaga, S., Alves, B. J. R., and Boddey, R. M. (2007). Another dimension to grazing systems: soil carbon. Trop. Grassl. 41, 65–83.
Fisher, M. J., Rao, I., Ayarza, M., Lascano, C., Sanz, I., Thomas, R., et al. (1994). Carbon storage by introduced deep-rooted grasses in the South American savannas. Nature 371, 236–238. doi: 10.1038/371236a0
Fisher, M. J., and Thomas, R. J. (2004). Implications of land use change to introduced pastures on carbon stocks in the central lowlands of tropical South America. Environ. Dev. Sustain. 6, 111–131. doi: 10.1023/B:ENVI.0000003632.82447.4d
Funk, C. C., Peterson, P. J., Landsfeld, M. F., Pedreros, D. H., Verdin, J. P., Rowland, J. D., et al. (2014). A quasi-global precipitation time series for drought monitoring. US Geol. Surv. Data Ser. 832, 1–12. doi: 10.3133/ds832
Gómez, J., Nivia, Á, Montes, N. E., Almanza, M. F., Alcárcel, F. A., and Madrid, C. A. (2015). “Notas explicativas: Mapa Geológico de Colombia,” in Compilando la geología de Colombia: Una visión a, eds J. Gómez and M. F. Almanza (Bogotá: Servicio Geológico Colombiano, Publicaciones Geológicas Especiales), 9-33.
Guo, L. B., and Gifford, R. M. (2002). Soil carbon stocks and land use change: a meta analysis. Glob. Chang. Biol. 8, 345–360. doi: 10.1046/j.1354-1013.2002.00486.x
Havlík, P., Valin, H., Herrero, M., Obersteiner, M., Schmid, E., Rufino, M. C., et al. (2014). Climate change mitigation through livestock system transitions. Proc. Nat. Acad. Sci. U. S. A. 111, 3709–3714. doi: 10.1073/pnas.1308044111
IDEAM PNUD, MADS, DNP, and CANCILLERÍA. (2015). Primer Informe Bienal de Actualización de Colombia. Bogotá.
IDEAM PNUD, MADS, DNP, and CANCILLERÍA. (2016). Inventario nacional y departamental de Gases Efecto Invernadero – Colombia. Tercera Comunicación Nacional de Cambio Climático. Bogotá: IDEAM, PNUD, MADS, DNP, CANCILLERÍA, FMAM.
Jansson, C., Faiola, C., Wingler, A., Zhu, X. G., Kravchenko, A., De Graaff, M. A., et al. (2021). Crops for carbon farming. Front. Plant Sci. 12, 938. doi: 10.3389/fpls.2021.636709
Jasiewicz, J., and Stepinski, T. F. (2013). Geomorphons - a pattern recognition approach to classification and mapping of landforms. Geomorphology 182, 147–156. doi: 10.1016/j.geomorph.2012.11.005
Kewscience (2021). Plants of the World Online. Urochloa. Available form: http://powo.science.kew.org/?q=urochloa (accessed February 11, 2021).
Kumar, S., Meena, R. S., Lal, R., Yadav, G. S., Mitran, T., Meena, B. L., et al. (2018). “Role of legumes in soil carbon sequestration,” in Legumes for Soil Health and Sustainable Management, eds R. S. Meena, A. Das, G. S. Yadav, and R. Lal (Singapore: Springer), 109–138.
Lascano, C. E. (1991). Managing the grazing resource for animal production in savannas of tropical America. Trop. Grasslands 25, 66–72.
Lavelle, P., Rodríguez, N., Arguello, O., Bernal, J., Botero, C., Chaparro, P., et al. (2014). Soil ecosystem services and land use in the rapidly changing Orinoco River Basin of Colombia. Agric. Ecosys. Environ. 185, 106–117. doi: 10.1016/j.agee.2013.12.020
Lerner, A. M., Zuluaga, A. F., Char,á, J., Etter, A., and Searchinger, T. (2017). Sustainable cattle ranching in practice: moving from theory to planning in Colombia's livestock sector. Environ. Manage. 60,176–184. doi: 10.1007/s00267-017-0902-8
López-Hernández, D., Hernández, R., and Brossard, M. (2005). Historia del uso reciente de tierras de Las Sabanas de América del Sur: estudios de casos en Sabanas del Orinoco. Interciencia. 30, 623–630.
MADR (2016). Agronet. Ministerio de Agricultura y Desarrollo Rural. Available from: http://www.agronet.gov.co (accessed January 15, 2022).
Mason-D'Croz, D., Barnhill, A., Bernstein, J., Bogard, J., Dennis, G., Dixon, P., et al. (2022). Ethical and economic implications of the adoption of novel plant-based beef substitutes in the USA: a general equilibrium modelling study. Lancet Planet. Health 6, e658–e669. doi: 10.1016/S2542-5196(22)00169-3
McBratney, A., Mendonça Santos, M., and Minasny, B. (2003). On digital soil mapping. Geoderma 117, 3–5. doi: 10.1016/S0016-7061(03)00223-4
Minasny, B., Malone, B. P., McBratney, A. B., Angers, D. A., Arrouays, D., Chambers, A., et al. (2017). Soil carbon 4 per mille. Geoderma 292, 59–86. doi: 10.1016/j.geoderma.2017.01.002
Moore, I. D., Gessler, P. E., Nielsen, G. A.E., and Peterson, G. A. (1993). Soil attribute prediction using terrain analysis. Soil Sci. Soc. Am. J. 57, 443–452. doi: 10.2136/sssaj1993.03615995005700020026x
Newton, P., Civita, N., Frankel-Goldwater, L., Bartel, K., and Johns, C. (2020). What is regenerative agriculture? A review of scholar and practitioner definitions based on processes and outcomes. Front. Sustain. Food Syst. 4, 194. doi: 10.3389/fsufs.2020.577723
Notenbaert, A. M.O., Douxchamps, S., Villegas, D. M., Arango, J., Paul, B. K., Burkart, S., et al. (2021). Tapping into the environmental co-benefits of improved tropical forages for an agroecological transformation of livestock production systems. Front. Sustain. Food Syst. 5, 742842. doi: 10.3389/fsufs.2021.742842
Peters, M., Rao, I. M., Fisher, M. J., Subbaraoa, G. V., Martens, S., Herrero, G., et al. (2012). “Tropical forage-based systems to mitigate greenhouse gas emissions,” in Eco-efficiency: From Vision to Reality, ed C. H. Hershey (International Center for Tropical Agriculture).
Radula, M. W., Szymura, T. H., and Szymura, M. (2018). Topographic wetness index explains soil moisture better than bioindication with Ellenberg's indicator values. Ecol. Indic. 85, 172–179. doi: 10.1016/j.ecolind.2017.10.011
Rainford, S. K., Martín-López, J. M., and Da Silva, M. (2021). Approximating soil organic carbon stock in the Eastern Plains of Colombia. Front Environ Sci. 9, 685819 doi: 10.3389/fenvs.2021.685819
Ramírez-Restrepo, C. A., Vera-Infanzón, R. R., and Rao, I. M. (2020). Predicting methane emissions, animal-environmental metrics and carbon footprint from Brahman (Bos indicus) breeding herd systems based on long-term research on grazing of neotropical savanna and Brachiaria decumbens pastures. Agric. Syst. 184, 102892. doi: 10.1016/j.agsy.2020.102892
Ramírez-Restrepo, C. A., Vera-Infanzón, R. R., and Rao, I. M. (2023). Carbon footprint of cull beef cows finished on sown pastures in the orinoco savanna of colombia. Arch. Latinoam. Prod. Anim. 31. doilink[10.53588/alpa.310101]10.53588/alpa.310101
Rao, I. M. (1998). “Root distribution and production in native and introduced pastures in the South American savannas,” in Root Demographics and Their Efficiencies in Sustainable Agriculture, Grasslands, and Forest Ecosystems, eds J. E. Box Jr (Dordrecht: Kluwer Academic Publishers), 19–42.
Rao, I. M., Ayarza, M. A., and Thomas, R. J. (1994). The use of carbon isotope ratios to evaluate legume contribution to soil enhancement in tropical pastures. Plant Soil 162, 177–182. doi: 10.1007/BF01347704
Rao, I. M., Peters, M., Castro, A., Schultze-Kraft, R., White, D., Fisher, M., et al. (2015). Livestock plus—the sustainable intensification of forage-based systems to improve livelihoods and ecosystem services in the tropics. Trop. Grassl. Forrajes Trop. 3, 59–82. doi: 10.17138/TGFT(3)59-82
Rodríguez, A., Rubiano, Y., Gutierrez, A., Bernal, J., Rodríguez, H., Stella, N., et al. (2013). Cobertura vegetal y usos del suelo de la Altillanura Plana de los municipios de Puerto López y Puerto Gaitán, Meta: escala 1:25000. Villavicencio: CORPOICA, 32.
Romero-Ruiz, M. H., Flantua, S. G.A., Tansey, K., and Berrio, J. C. (2012). Landscape transformations in savannas of northern South America: Land use/cover changes since 1987 in the Llanos Orientales of Colombia. Appl. Geograp. 32, 766–776. doi: 10.1016/j.apgeog.2011.08.010
Rondón, M., Acevedo, D., Hernández, R. M., Rubiano, Y., Rivera, M., Amézquita, E., et al. (2006). Carbon Sequestration Potential of the Neotropical Savannas (Llanos) of Colombia and Venezuela. Carbon Sequestration in Soils of Latin America. Binghamton, NY: Haworth Press, 213–243.
SAS (2016). SAS Software, Version 9.4. Available online at: http://www.sas.com/ (accessed January 15, 2022).
Sommer, R., Paul, B. K., Mukalama, J., and Kihara, J. (2018). Reducing losses but failing to sequester carbon in soils – the case of conservation agriculture and integrated soil fertility management in the humid tropical agro-ecosystem of Western Kenya. Agric. Ecosyst. Environ. 254, 82–91. doi: 10.1016/j.agee.2017.11.004
Strassburg, B. B., Latawiec, A. E., Barioni, L. G., Nobre, C. A., Da Silva, V. P., Valentim, J. F., et al. (2014). When enough should be enough: Improving the use of current agricultural lands could meet production demands and spare natural habitats in Brazil. Global Environ. Change 28, 84–97. doi: 10.1016/j.gloenvcha.2014.06.001
Torrijos, P., Rangel, J. O., and Rippstein, G. (2001). “Quema y sucesión secundaria de la vegetación en el Centro de Investigación Agropecuaria Carimagua,” in Agroecología y Biodiversidad de las Sabanas en los Llanos Orientales de Colombia, ed G. Rippstein (CIAT).
Trujillo, W., Fisher, M. J., and Lal, R. (2006). Root dynamics of native savanna and introduced pastures in the Eastern Plains of Colombia. Soil Tillage Res. 87, 28–38. doi: 10.1016/j.still.2005.02.038
Vera-Infanzón, R. R., Rao, I. M., Ramírez-Restrepo, C. A., and Hoyos-Garcés, F. (2023). A dynamic simulation model to assess farm level effects of pasture intensification strategies on beef herd outputs and carbon footprints in acid soil savannas of Eastern Colombia. Arch. Latinoam. Prod. Anim.
Walker, W. S., Gorelik, S. R., Cook-Patton, S. C., Baccini, A., Farina, M. K., Solvik, K. K., et al. (2022). The global potential for increased storage of carbon on land. Proc. Nat. Acad. Sci. U. S. A. 119, e2111312119. doi: 10.1073/pnas.2111312119
World Bank (2022). Arable Land (% of Land Area) – Colombia. Available from: https://data.worldbank.org/indicator/AG. LND. ARBL. ZS?locations=CO (accessed February 23, 2022).
Xu, C., Xu, X., Ju, C., Chen, H. Y., Wilsey, B. J., Luo, Y., et al. (2021). Long-term, amplified responses of soil organic carbon to nitrogen addition worldwide. Glob. Change Biol. 27, 1170–1180. doi: 10.1111/gcb.15489
Zhu, A.-X. (1997). A similarity model for representing soil spatial information. Geoderma 77, 217–242. doi: 10.1016/S0016-7061(97)00023-2
Zhu, A.-X., Band, L., Vertessy, R., and Dutton, B. (1997). Derivation of soil properties using a Soil Land Inference Model (SoLIM). Soil Sci. Soc. Am. J. 61, 523–533. doi: 10.2136/sssaj1997.03615995006100020022x
Keywords: soil organic carbon, savanna, livestock, climate change mitigation, sustainable livestock, land use, on-farm evaluation
Citation: Hyman G, Castro A, Da Silva M, Arango M, Bernal J, Pérez O and Rao IM (2022) Soil carbon storage potential of acid soils of Colombia's Eastern High Plains. Front. Sustain. Food Syst. 6:954017. doi: 10.3389/fsufs.2022.954017
Received: 26 May 2022; Accepted: 20 September 2022;
Published: 14 October 2022.
Edited by:
Federico Bert, Instituto Interamericano de Cooperación para la Agricultura, ArgentinaReviewed by:
Lieven Claessens, International Institute of Tropical Agriculture (IITA), KenyaStoécio Maia, Instituto Federal de Alagoas - Campus Marechal Deodoro, Brazil
Copyright © 2022 Hyman, Castro, Da Silva, Arango, Bernal, Pérez and Rao. This is an open-access article distributed under the terms of the Creative Commons Attribution License (CC BY). The use, distribution or reproduction in other forums is permitted, provided the original author(s) and the copyright owner(s) are credited and that the original publication in this journal is cited, in accordance with accepted academic practice. No use, distribution or reproduction is permitted which does not comply with these terms.
*Correspondence: Glenn Hyman, glennhyman@gmail.com