- 1Centre for Agri-Environmental Research, School of Agriculture, Policy and Development, University of Reading, Reading, United Kingdom
- 2School of Biological Sciences, University of Reading, Reading, United Kingdom
- 3UK Centre for Ecology and Hydrology, Wallingford, United Kingdom
Bees provide a vital ecosystem service to agriculture by contributing to the pollination of many leading global crops. Human wellbeing depends not only on the quantity of agricultural yields, but also on the stability and resilience of crop production. Yet a broad understanding of how the diversity and composition of pollinator communities may influence crop pollination service has previously been hindered by a scarcity of standardized data. We used outputs from Bayesian occupancy detection models to examine patterns in the inter-annual occupancy dynamics of the bee pollinator communities of four contrasting crops (apples, field bean, oilseed and strawberries) in Great Britain between 1985 and 2015. We compared how the composition and species richness of different crop pollinator communities may affect the stability of crop pollinator occurrence. Across the four crops, we found that the inter-annual occupancy dynamics of the associated pollinator communities tended to be more similar in smaller communities with closely related pollinator species. Our results indicate that crop pollinator communities composed of a small number of closely related bee species show greater variance in mean occupancy compared to crops with more diverse pollinator communities. Lower variance in the occurrence of crop pollinating bee species may lead to more stable crop pollination services. Finally, whilst our results initially indicated some redundancy within most crop pollinator communities, with no, or little, increase in the variance of overall mean occupancy when species were initially removed, this was followed by a rapid acceleration in the variance of crop pollinator occurrence as each crop's bee pollinator community was increasingly depreciated. High inter-annual variations in pollination services have negative implications for crop production and food security. High bee diversity could ensure more stable and resilient crop pollination services, yet current agri-environment schemes predominantly benefit a limited suite of common species. Management may therefore benefit from targeting a wider diversity of solitary species in order to safeguard crop pollination service in the face of increasing environmental change.
Introduction
Insect pollinators provide vital ecosystem services to agriculture, including most notably pollination of crop plants (Zhang et al., 2007). The majority of leading global crops benefit to some degree from biotic pollination (Klein et al., 2007). Insect pollination increases crop yields, quality and market value (Bommarco et al., 2012; Garratt et al., 2014a; Klatt et al., 2014). Animal-pollinated crops are also disproportionately more important from an economic and nutritional perspective than pollinator-independent staple crops (Vanbergen et al., 2014; Ollerton, 2017). Demand for major insect-pollinated crops has continued to rise in recent decades, leading to global agriculture becoming increasingly dependent upon insect pollinators (Aizen et al., 2019). Documented declines in wild bees (Potts et al., 2010; Powney et al., 2019; Zattara and Aizen, 2021), which provide important pollination services to many crops (Klein et al., 2007), and evidence of pollinator limitation in agricultural crops, have therefore raised concerns about future food security (Reilly et al., 2020).
There is an urgent need to explore the relationship between biodiversity and ecosystem function (Oliver et al., 2015a; Suárez-Castro et al., 2022). Prior evidence indicates that many ecosystem functions are predominantly maintained by a small number of species (Schwartz et al., 2000; Cardinale et al., 2006). This may, however, reflect the small spatio-temporal scales at which most experiments are conducted (Winfree, 2013). For example, Kleijn et al. (2015) conducted a global review of field studies, which reported that 80% of crop flower visits were attributable to just 2% of bee species. However, more recently, Winfree et al. (2018) found that the number of bee species required to provide adequate crop pollination services on a landscape scale is at least one order of magnitude higher than in field experiments, due to species turnover. Calls for cost-effective management to focus on those species that make the greatest contribution to pollination (Kleijn et al., 2015) may not protect crop pollination services due to a failure to encompass species that supplement service provision during times of suboptimal pollination by dominant species (Fijen et al., 2018).
Human wellbeing depends not only on the amount, but also the stability, of agricultural crop production (Garibaldi et al., 2011). Wild bee populations exhibit significant temporal fluctuations (Roubik, 2001; Williams et al., 2001), and around half of yield instability in pollinator-dependent crops is attributable to inter-annual variation in pollination service (Garibaldi et al., 2011). Species-rich pollinator communities have been shown to positively influence the spatial and temporal stability of crop pollination service delivery (Senapathi et al., 2021). Bee crop pollinators can exhibit marked community variation across crop fields and years (Kremen et al., 2002; Klein, 2009; Adamson et al., 2012). Species rich pollinator communities, and lower variability in bee richness, can increase the spatio-temporal stability of crop yields (Martins et al., 2018; Winfree et al., 2018; Hünicken et al., 2021), because less abundant, non-dominant pollinators can potentially replace dominant pollinators if the latter undergo transient declines (Fijen et al., 2018; Winfree et al., 2018).
Pollinator management also needs to consider the resilience of crop pollination services (Senapathi et al., 2015), due to accelerating rates of anthropogenic induced environmental change (Oliver et al., 2015a,b). Bees with different functional traits have been demonstrated to vary in their sensitivity to climate and land use change (Kammerer et al., 2021), and high bee diversity has been shown to buffer crop pollination delivery from changes in weather conditions (Brittain et al., 2013; Rogers et al., 2014). This is in line with what has been termed the ‘insurance hypothesis', whereby biodiversity insures against fluctuations in ecosystem service function, because some species continue to contribute at times when others do not (Yachi and Loreau, 1999). Biodiverse pollinator communities could ensure that crop pollination service is sustained under a range of environmental conditions (Science for Environment Policy, 2020), yet current agri-environment schemes predominantly benefit a limited suite of common species (Vasiliev and Greenwood, 2020).
Whilst species diversity can increase the stability of agricultural production, differences in the traits of both bee species and crop flowers mean that bee species vary in their effectiveness as pollinator of different crops (Garibaldi et al., 2015). Many crops have distinct pollinator communities (Garratt et al., 2014b; Hutchinson et al., 2021). Therefore, the degree to which species richness and functional diversity can stabilize pollination service may vary among crops. However, a broad understanding of how crop pollinator community composition could affect crop pollination service has previously been limited by a dearth of standardized data. Biological records represent a burgeoning tool to address such questions (Powney and Isaac, 2015), with a suite of statistical techniques being developed to overcome the challenges presented by such unstandardized data (Isaac et al., 2014). Employing Hierarchical Bayesian Occupancy Models, distribution records of bee species have been used to develop a pollinator indicator for the UK Biodiversity Indicators that is similar to the abundance data used for other indicator species (Outhwaite and Issac, 2015).
The aim of this study was to explore how the inter-annual occupancy dynamics of different wild bee crop pollinator communities differed, and the potential implications of this for the resilience of crop pollination service. Temporal variability in crop pollinator abundances is directly linked to crop pollination service stability (Senapathi et al., 2021), and given the link between year-to-year changes in the distribution and abundance of pollinating insects (Mason et al., 2018), crop pollination service delivery is also likely to be underpinned by stability of crop pollinator occurrence. Great Britain was used as a case study due to the availability of data on its bee populations (Powney et al., 2019). Four economically important insect-pollinated crops—apple (Malus domestica), field bean (Vicia faba), oilseed rape (Brassica napus) and strawberry (Fragaria x ananassa)—were chosen as focal crops. These crops represent the main orchard, protein, arable, and soft fruit crops, respectively, in Great Britain (Breeze et al., 2021). Previous work has demonstrated that these crops exhibit differences in their bee pollinator community composition. Field bean and strawberry are predominantly visited by bumblebees, whereas apple, and particularly oilseed, can also be visited by a wide diversity of solitary bees (Hutchinson et al., 2021).
Here we combine lists of crop-pollinating bee species for our focal crops (Hutchinson et al., 2021), alongside outputs from occupancy models based upon biological records collected by the Bees, Wasps and Ants Recording Society (BWARS, 2020). Occupancy models are designed to account for variations in the detectability of species, as well as incomplete and biased sampling. The output consists of a mean estimate for the proportion of 1 km grid squares in Great Britain occupied by a given bee species in a particular year, and has previously been used to determine long-term population trends amongst wild bee species (Powney et al., 2019). Long-term temporal trends in such occupancy models are closely related to long term abundance trends (Oliver et al., 2015a), and year-to-year changes in citizen-collected distribution records, have been demonstrated to act as a reasonable surrogate for inter-annual changes in abundance (Mason et al., 2018). We examined patterns in the inter-annual occupancy dynamics of the bee pollinators of these crops to address three main aims: (i) identify groups of crop-pollinating bee species with similar and contrasting inter-annual occupancy dynamics (ii) compare the stability of the occupancy dynamics of different crop pollinator communities, (iii) investigate to what extent pollinator species richness affects the stability of crop pollinator occurrence.
Methods
Crop flower visitors
For each crop a list of bee species was compiled based upon a previous study that used a literature review of crop field surveys, combined with bee ecological trait data, to determine the potential pollinators of each crop in Great Britain (Hutchinson et al., 2021; Supplementary material S1). In this study a total of 229 bee species were initially considered, of which 32% (73) were deemed to be potential pollinators of one or more of our four focal crops. To reflect the fact that not all species make an equal contribution to crop pollination, bee species in this study were grouped into three nested categories as follows:
1. Primary Flower Visitors—Species Identified as “Dominant” Pollinators (Attributed With a Combined Total of 80% of Flower Visits in British Crop Field Studies) in Hutchinson et al. (2021).
2. Core Flower Visitors—Primary Flower Visitors and all Other Species Classed as “Definite” Flowers Visitors (Species Recorded Visiting Crop Flowers in British Field Studies) in Hutchinson et al. (2021).
3. All Flower Visitors—all Above Species, and all Other Species Classified as “Likely” Flower Visitors (Species Recorded in Pan Traps With Crop Flower Visitation Data From Other European Studies to Validate Their Status as Crop Flower Visitors) in Hutchinson et al. (2021).
Further details of how bee species were categorized can be found in Supplementary materials S1, S2.
Inter-annual changes in occupancy
Data from Bayesian occupancy detection models held by the UK Center for Ecology and Hydrology were utilized in all analyses. The occupancy models use occurrence records of bees extracted from the Bees, Wasps and Ants Recording Society (BWARS, 2020) in 1 km grid cells across Great Britain. These observations represent presence-only data, so non-detections from records are inferred from other species within the taxonomic group on the same grid cell and date. The output is derived from two hierarchically coupled sub-models that simultaneously estimate and account for variation in the detectability of different species, while estimating species presence for a given site (1 km grid cell) and year combination. Detectability was included as a covariate in the detection model to account for variation in recorder effort. Further details of the occupancy models used can be found in Outhwaite et al. (2018).
The mean annual occupancy estimates for each bee species that met the “core flower visitor” criteria described were initially used, as these species represent the main known flower visitors of our crops (Hutchinson et al., 2021). Inter-annual changes in occupancy from 1985 to 2015 were calculated for all forty bee species categorized as core flower visitors of one or more of the four crops. Inter-annual changes were calculated by subtracting the occupancy estimate for each year from the year preceding it to establish relative change from one year to the next.
Inter-annual changes in species occupancy were then used to characterize groups of species with comparable occupancy dynamics (i.e., similar directional annual change in the mean proportion of occupied 1 km squares). Using Pearson's correlation coefficient, an occupancy dynamics correlation matrix was created to compare the inter-annual changes in occupancy between each pair of species. The occupancy dynamics matrix was transformed into a distance matrix using the base function “dist” in R (R Core Team, 2020). All values were multiplied by −1 so that pairs of species with the most asynchronous occupancy dynamics had positive values. One was then added to all values because the following analysis cannot include negative values. This resulted in pairs of species with the most asynchronous occupancy dynamics having the highest scores, and species with the most synchronous occupancy dynamics having the lowest scores.
Hierarchical cluster analysis, using Euclidean distances, was then performed with the base function “hclust” in R (R Core Team, 2020), following the methodology described in Greenwell et al. (2019) to identify groups of species with the most similar inter-annual occupancy dynamics. The “hclust” function assigns each species to its own cluster, then proceeds iteratively, joining the two most similar clusters at each stage, until there is just a single cluster. The results were visualized as a dendrogram. To prune the output and identify clusters of species with similar occupancy dynamics, we applied the Kelley–Gardner–Sutcliffe (KGS) penalty function (Kelley et al., 1996) in the “maptree” package (White and Gramacy, 2012). The KGS penalty function uses the species pairwise distance matrix to maximize differences between clusters and similarity within clusters (Carvalho et al., 2011; Soultan et al., 2020). The minimum output value of the KGS function corresponds to the optimal number of clusters.
Inter-annual occupancy variation
To compare the stability of pollinator occurrence over time for each crop's entire suite of known flower visitors a dataset per crop containing the inter-annual changes in occupancy from 1985 to 2015 was created following the methodology described above, for all bee species identified as flower visitors (Supplementary material S2). For field bean, bees were only split into primary and core flower visitors, as no additional likely pollinators were identified for this crop (Hutchinson et al., 2021).
The arithmetic means of occupancy per year for all bee species for each group of flower visitors per crop, as well as the standard deviation, were then calculated. Using standard deviation to assess variance, as used elsewhere (e.g., Karp et al., 2011; Morin et al., 2014; Hautier et al., 2015), rather than the coefficient of variation, was appropriate here because our time series were already centered around a mean of zero. We do not use standard error, which adjusts variance estimates by sample size, because we are interested in absolute deviations in crop pollinator occurrence, which have implications for potential deficits in crop pollination service and crop yields.
Impact of removing bee species on stability of crop pollinator occurrence
Finally, the potential impact of a progressive loss of bee species from crop areas on the stability of crop pollinator occurrence for each of the for focal crops was explored. First the mean occupancy of all fifty bee species identified as crop flower visitors between 2013 and 2015 was calculated. This was done in order to rank species based upon their overall occupancy across Great Britain. The last three years of data were chosen to ensure that recent estimates of occupancy were utilized, but also to account for the fact that a single year of data alone may not reflect the true occupancy of each species, given that bee species exhibit significant inter-annual population fluctuations (Roubik, 2001; Williams et al., 2001). The standard deviation of mean occupancy was then calculated as described above, but this time one bee species at a time was successively removed, to calculate the standard deviation (SD) if one species, then two, then three, and so forth, was removed. Species were removed based upon their mean occupancy between 2013 and 2015, with the species calculated as having the lowest mean occupancy figure removed first, and so on, until only one species, that with the highest mean occupancy, remained.
The above methodology was chosen because those species with the lowest mean occupancy figures will almost certainly correspond to those bee pollinators that are the least likely to occur within each crop's pollinator community. Geographically restricted species are likely to be species that exhibit a greater degree of ecological specialization compared to more geographically widespread species, which in turn are likely to be less abundant in farmland (Wood et al., 2016), as well as at greater risk of population declines (Goulson et al., 2005; Williams, 2005). As such these species are likely to be amongst the first to disappear from crop areas. This hypothesis is supported by increasing evidence that agri-environment schemes developed to increase bee farmland populations predominantly benefit a limited suite of common and generalist bee species (Wood et al., 2015, 2017).
Occupancy uncertainty confidence limits
There is a degree of uncertainty associated with the mean occupancy estimates of all modeled species and this is represented by 95% confidence intervals (Powney et al., 2019). As such all analyses described above were also run using the lower 2.5 and upper 97.5 confidence limit occupancy estimates to check that patterns in occupancy dynamics at the minimum and maximum confidence limits of the occupancy estimates matched those of the mean occupancy estimates.
All analyses were performed using RStudio version 3.4.3 (RStudio Team, 2020).
Results
Crop flower visitors
A total of fifty bee species were identified as flower visitors of one or more of the four crops (Supplementary material S2). Forty species were identified as core flower visitors, ten of which were also identified as primary flower visitors of one or more of the four crops. The primary pollinators comprised six species of Andrena and four species of Bombus (Andrena cineraria, Andrena dorsata, Andrena haemorrhoa, Andrena nigroaenea, Andrena nitida, Andrena scotica, Bombus hortorum, Bombus lapidarius, Bombus pascuorum and Bombus terrestris).
Inter-annual changes in occupancy
The hierarchical clustering analysis using the mean occupancy estimates for the core flower visitors produced a dendrogram that was split into five clusters based upon the KGS penalty function (Figure 1; Supplementary material S3). Flower visitors for each crop were distributed across the dendrogram, with both apple and oilseed rape visitors occurring in five clusters, field bean visitors in four, and strawberry visitors occurring in three clusters.
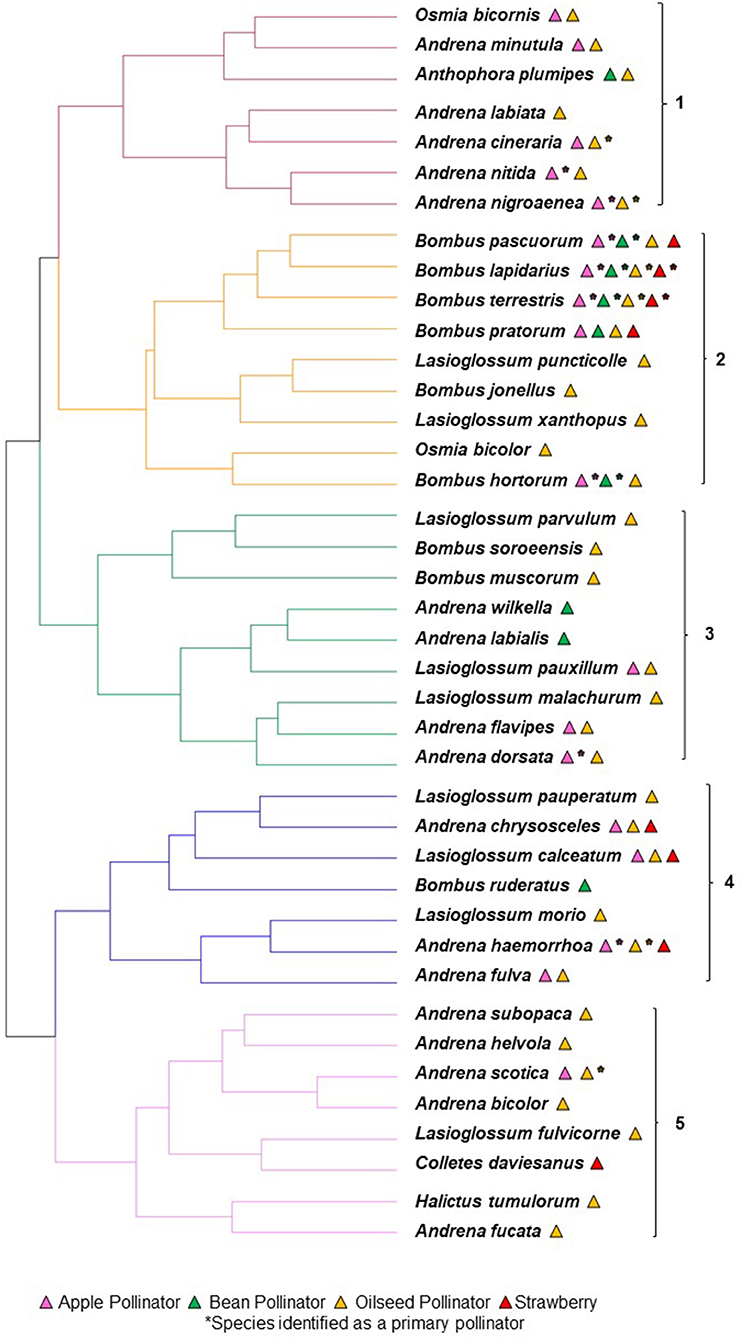
Figure 1. Dendrogram showing the hierarchical relationships amongst five clusters of bee crop pollinators for apple (18 spp.), bean (9 spp.), oilseed (36 spp.) and strawberry (8 spp.) Individual clusters are colored for clarity. Species with more correlated occupancy dynamics join further to the right-hand side of the dendrogram. Triangles with an asterisk to the right-hand side denotes species identified as primary pollinators of that crop.
Amongst the ten species identified as primary pollinators, species from the same genus generally had more synchronous inter-annual occupancy dynamics than species from different genera (Table 1). All species pairs of Andrena (i.e., all possible combinations of two species) were positively correlated, as were all species pairs of Bombus. Of the 24 mixed genus species pairs (i.e., one species of Andrena and one species of Bombus) seventeen were negatively correlated and seven were positively correlated.
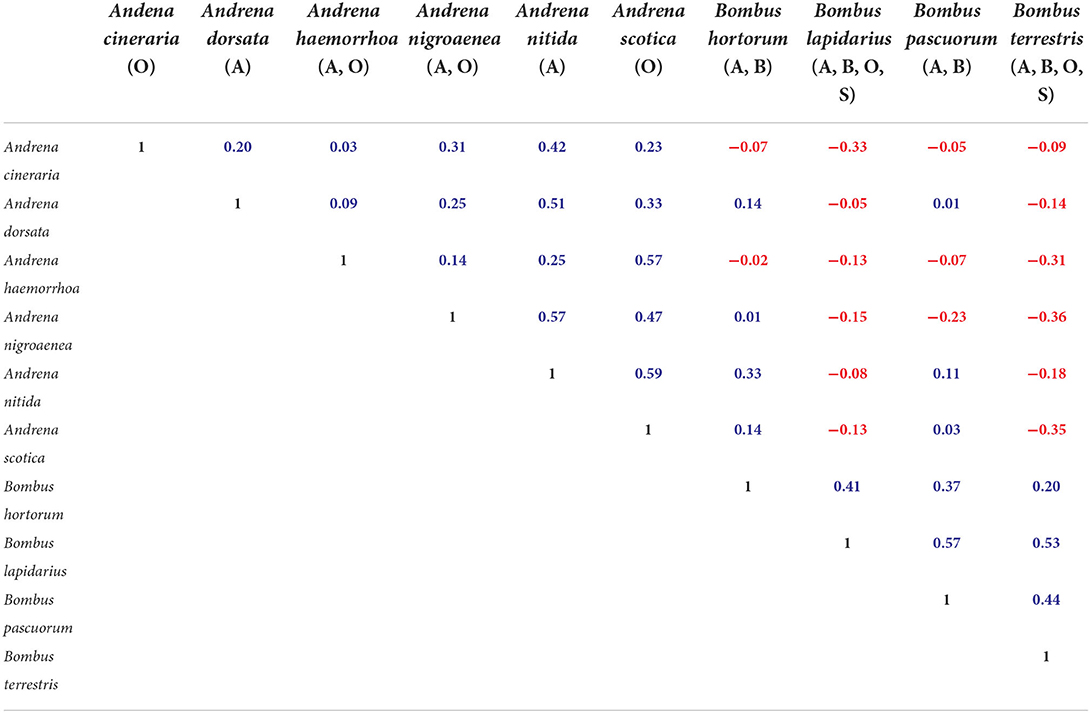
Table 1. Pearson's r correlation scores of inter-annual occupancy estimates for bee species pairs identified as primary pollinators of apple (A), field beans (B), oilseed rape (O) and strawberry (S) crops (positive scores highlighted in blue and negative scores highlighted in red).
The four Bombus species identified as the sole primary flower visitors of bean, two of which are also the sole primary flower visitors of strawberry (Figure 1), were all placed in one cluster. Of those four species the pairs with the most synchronous occupancy dynamics were B. lapidarius and B. pascuorum, and B. lapidarius and B. terrestris (Figure 2A).
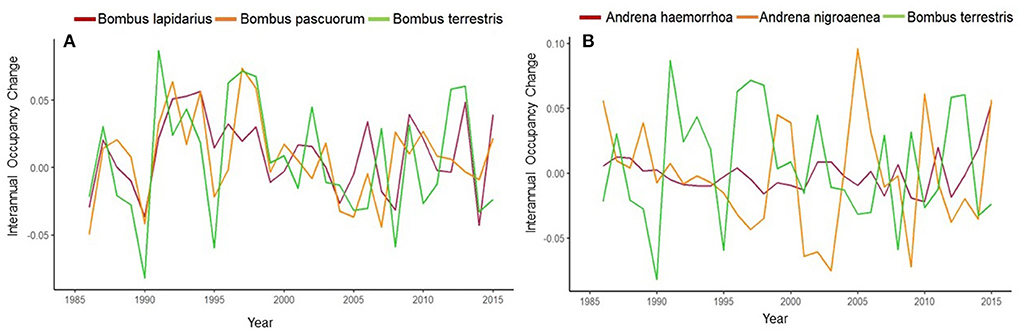
Figure 2. Inter-annual occupancy changes for five bee species. Three Bombus species (A) have positively correlated occupancy dynamics (Bombus lapidarius and Bombus pascuorum (r = 0.57), Bombus lapidarius and Bombus terrestris (r = 0.53), Bombus pascuorum and Bombus terrestris (r = 0.44). Two Andrena species (B) have positively correlated occupancy dynamics (r = 0.47), but have negatively correlated occupancy dynamics with Bombus terrestris (Andrena haemorrhoa and Bombus terrestris (r = −0.31), Andrena nigroaenea and Bombus terrestris (r = −0.36).
The same Bombus species were also identified as primary pollinators of apple and oilseed, alongside six species of Andrena (Figure 1). The primary flower visitors of apple and oilseed rape were split across four clusters. Amongst the shared primary flower visitors to apple and oilseed rape several species pairs had asynchronous occupancy dynamics, including most notably A. nigroaenea and B. terrestris and A. haemorrhoa and B. terrestris (Figure 2B).
Inter-annual occupancy variation
Across all crops, when considering only the primary flower visitors, a greater variation in mean occupancy was found compared to when the core flower visitors or all flower visitors combined were considered. For all crops as the number of species increased across flower visitor categories there was a corresponding decrease in variation in mean occupancy. When only primary pollinators were included, standard deviation ranged between 0.015 and 0.032. The inclusion of all core flower visitors reduced the standard deviation by between 25 and 60%, compared to the primary flower visitors alone. The inclusion of all flower visitors combined reduced the standard deviation by between 40 and 70% compared to the primary flower visitors alone (Table 2; Figures 3A–D).
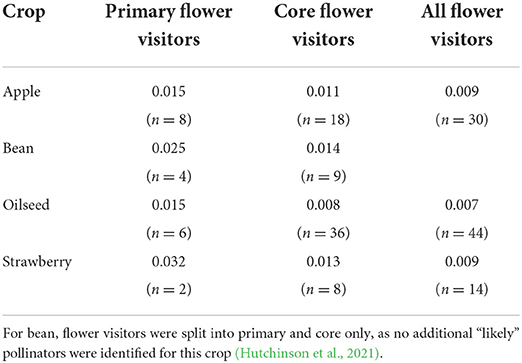
Table 2. Standard deviation of mean occupancy for primary, core and all flower visitors per crop (number of species in brackets).
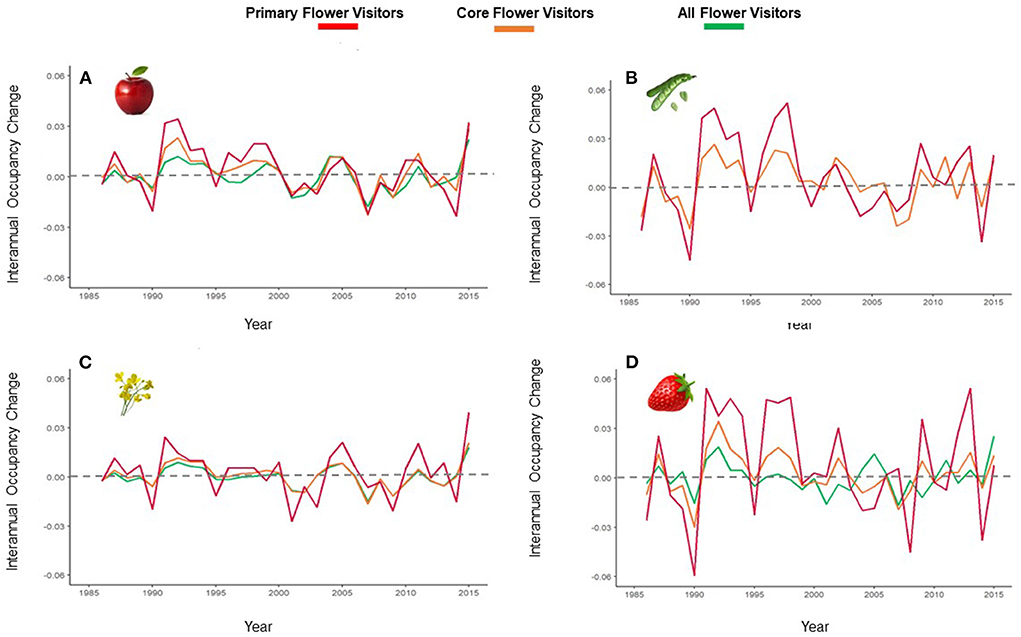
Figure 3. Mean inter-annual occupancy change of primary, core and all visitors for (A) apple, (B) bean (primary and core only), (C) oilseed and (D) strawberry crops. Dashed gray line denotes a mean inter-annual occupancy change of zero.
For the primary and core flower visitor groups, strawberry, which had the fewest bee species in these categories, had the greatest standard deviation. When including all flower visitors, bean, which had the fewest flower visitors overall, had a standard deviation of 0.014, the highest of the four crops. Conversely, when considering all flower-visiting bee species, oilseed, which has the highest number of overall flower visitors, had a standard deviation of 0.007, which was the lowest of all four crops.
Impact of removing bee species on stability of crop pollinator occurrence
As species were removed from each crop's pollinator community, based upon their overall mean occupancy, from low to high, the variation in mean occupancy initially showed no or little increase across all four crops, before beginning to show a marked increase. When approximately a third of each crop's bee pollinators were removed from the dataset set, standard deviation increased by around 25%, which extended to a 50%, 100% and 200% increase when approximately half, two thirds and three quarters of each crop's bee pollinators were removed (Figure 4; Supplementary material S4).
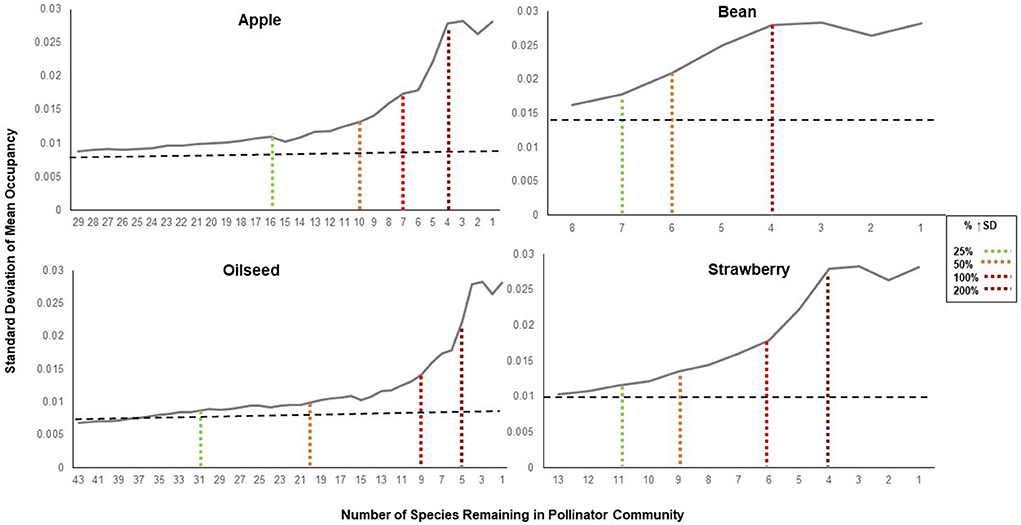
Figure 4. Standard deviation of mean occupancy for bee crop pollinator communities as successive species are removed for apple, bean, oilseed and strawberry crops. Gray dashed line denotes standard deviation of mean occupancy for bee crop pollinator community if all species are present.
Occupancy uncertainty confidence limits
When using the lower 2.5 and upper 97.5 confidence limit occupancy estimates for each species, the same analyses described above generated slightly different numerical results, but followed almost identical patterns to that of the mean occupancy estimates.
For both sets of confidence limit occupancy estimates the hierarchical clustering analysis produced a dendrogram that was split into five clusters based upon the KGS penalty function. The number of clusters to which each crop's flower visitors were assigned was identical to the mean occupancy estimate, except for the upper 97.5 strawberry data, where crop flower visitors were split across four, rather than three, clusters. Whilst some species were placed into slightly different clusters, the same broad patterns remained (Supplementary materials S5a,b). The lower 2.5 and upper 97.5 confidence limit occupancy estimate data again indicated that amongst the ten primary pollinators, species from the same genus generally had more synchronous occupancy dynamics than species from different genera (Supplementary materials S6a,b).
For the data considering the stability of pollinator occurrence both the lower 2.5 and upper 97.5 confidence limit occupancy estimates, whilst generating slightly different SD numbers, followed an identical pattern to that of the mean occupancy estimate data (Supplementary materials S7a,b). Additionally, as species were gradually removed the same patterns emerged, with an initial gradual increase in standard deviation as species were removed, followed by marked increase as each crop's pollinator community was reduced (Supplementary materials S8a,b).
Discussion
Overview
This study is one of the first to explicitly test how bee crop pollinator community composition and species richness might affect the stability of crop pollinator occurrence, and consider the implications of this for pollination services. We found that bee crop pollinator communities composed of a small number of closely related species are likely to exhibit more synchronized inter-annual occupancy dynamics, and show a greater variation in mean occupancy, compared to crop pollinator communities comprised of a more diverse set of bee species. Our results indicate that more species-rich crop pollinator communities comprised of bees from different genera encompass species with asynchronous occupancy dynamics. Asynchrony between species populations is an important mechanism of diversity-stability relationships, and asynchronous fluctuations in pollinator communities could increase the inter-annual stability of the crop pollination services they provide (Senapathi et al., 2021). Our findings support this by showing that crops composed of diverse, species rich pollinator communities are likely to show lower variation in mean occupancy, compared to less species-rich communities. Whilst we focused specifically on crop pollinator occurrence, in the form of inter-annual changes in occupancy, based upon citizen science collected biological records, inter-annual changes in citizen science-based distribution records have been demonstrated to act as proxy for year-to-year changes in species abundances in another group of pollinating insects—butterflies (Mason et al., 2018). It is therefore reasonable to expect that the general patterns we found in bee species occupancy will be reflected in bee species abundances.
Inter-annual changes in occupancy
The first of our analyses indicate that different bee species show variable occupancy dynamics, which may reflect differences in the corresponding life history traits of bee species (Williams et al., 2010), such as body size, nesting type, phenology and reproductive strategy (Michener, 2000). For example, the bee pollinators included in our analyses encompass both cavity and ground-nesting species, as well as a range of dietary breadths, flight periods and sizes. Additionally, these results indicate that crops with dominant pollinators from different genera are more likely to include species with asynchronous occupancy dynamics compared to crops whose dominant pollinators are all from the same genera. For example, synchronous occupancy dynamics were observed within the dominant crop pollinating Andrena species and Bombus species, respectively, which were in contrast to the relatively asynchronous occupancy dynamics observed between the two genera. Previous work in other insects (Lepidoptera) has shown that synchrony in population dynamics is linked to phylogeny (Greenwell et al., 2019) and that traits that mediate response to environmental conditions (cf. “response traits”) have a phylogenetic signal (Melero et al., 2022). The four Bombus species identified as dominant pollinators of the four crops all have relatively similar life histories. They are large species, found throughout Great Britain, nesting in colonies below, or occasionally above, ground in old bird and mammal nests, and fly from March until around October (Else and Edwards, 2018). In contrast the six Andrena species identified as dominant pollinators are relatively less widespread, medium-sized species, found predominantly in the southern half of Great Britain, with single females laying eggs in self-excavated burrows in scattered, or occasionally dense, aggregations in sparsely vegetated soil. In most cases they are spring-flying species, active between March and June (Else and Edwards, 2018). The asynchronous occupancy dynamics exhibited between the two genera indicate that crops pollinated by both Andrena and Bombus species may have pollinator communities with, overall, more stable occupancy dynamics, which could translate to more stable and resilient pollination services. Future work, investigating the phylogenetic relationships, and traits, of bee species could help to explain why particular species exhibit similar, or contrasting, inter-annual changes in occupancy, and inform management to promote more stable occurrence dynamics.
Inter-annual occupancy variation
Our findings support evidence that high bee diversity may stabilize crop pollination service (Klein, 2009; Senapathi et al., 2021) and that a species-rich community of pollinators could help ensure that pollination service is sustained under a range of conditions (Fijen et al., 2018; Science for Environment Policy, 2020). High inter-annual variations in crop pollination service have negative implications for food security and farmer livelihoods (Garibaldi et al., 2011). Thus, whilst crop pollination is often predominantly delivered by a small number of species (Fijen et al., 2018), our results indicate that pollinator management to increase crop yield stability may need to target a more diverse set of bee species than at present. Currently agri-environment schemes primarily benefit common bumblebee species (Wood et al., 2015). However, solitary bee species are more important pollinators of some crops (Hutchinson et al., 2021), and based upon our findings show different occupancy dynamics to bumblebee species, likely driven by differences in ecology that underpin functional trait diversity (Forrest et al., 2015).
Based upon our results the degree to which species richness can reduce variation in mean occupancy, and potentially bee species abundances, may vary, however, among crop types in Britain. Field bean has long tubular flowers, which generally only long-tongued bees, such as Bombus species, can legitimately pollinate (Cook et al., 2003). This crop may be especially susceptible to inter-annual variation in pollination service delivery due to the synchronous occupancy dynamics of its primary pollinator community. Field bean had the lowest total number of bee species identified as flower visitors across the four crops, some of which are short-tongued Andrena species that are unlikely to make a significant contribution to bean pollination (Hutchinson et al., 2021). Long-tongued bees, including bumblebees, are amongst the most severely declining species in the UK, and across Europe (Goulson et al., 2008). As such, management to ensure sustainable pollination service delivery to field bean may be reliant upon encouraging more stable populations of a relatively small core group of bumblebee species. For example, hedgerows have been demonstrated to stabilize bumblebee populations in agricultural fields (Gardner et al., 2021). Additionally, at least one species of long-tongued solitary bee—Anthophora plumipes—has been evidenced as an effective pollinator of field bean (Bond and Kirby, 1999), and based upon our results has less synchronous occupancy dynamics with beans' four primary flower visitors than between them. However, A. plumipes prefers to nest in vertical soil profiles (BWARS, 2021), which are currently not a common feature of agricultural landscapes.
Strawberry, which whilst primarily visited by bumblebees, has flowers with high nectar accessibility (Garibaldi et al., 2015) and can be effectively pollinated by a range of short-tongued solitary species (Bänsch et al., 2021). The lack of current diversity in its known pollinators could reflect the fact that strawberry production is often achieved through the use of plastic-protected tunnel environments (Allen et al., 2015), which may inhibit access by smaller solitary species (Chagnon et al., 1993). Intensive production of crops under plastic may benefit from making flowers more attractive to different pollinators by opening the sides during crop flowering and providing appropriate floral and nesting resources to support short-tongued solitary species.
Oilseed rape and apple had the greatest number of bee species across all three categories of flower visitors and the lowest standard deviation of mean occupancy amongst our four focal crops. Even amongst their primary pollinators, composed of a core group of Andrena and Bombus species, there was evidence of relatively asynchronous occupancy dynamics, possibly related to differences in their solitary vs. social ecology (Michener, 2000). Additionally, both crops are known to be visited by a range of other solitary bee genera (Hutchinson et al., 2021), which our results indicate have variable occupancy dynamics. Stability of pollinator occurrence, and potentially crop pollination service delivery, for both crops could therefore be further promoted through management targeted at a wide diversity of solitary species. For example, current agri-environment measures are heavily biased toward ground-nesting bee species (Image et al., 2022) and rarely incorporate suitable nesting and floral resources for cavity-nesting species (Gresty et al., 2018), which can be important pollinators of both apple and oilseed crops (Hutchinson et al., 2021).
Impact of removing bee species on stability of crop pollinator occurrence
Whilst we found that there was no, or little, increase in the variation of overall mean occupancy as species were initially removed from each crop's pollinator community, this was followed by a rapid acceleration as each crop's pollinator community was increasingly depreciated. Whilst rarer crop pollinators may not contribute significantly to increased stability of pollinator occurrence, management recommendations aimed at supporting a limited subset of dominant pollinating species (Kleijn et al., 2015) could fail to protect crop pollination services under all circumstances (Fijen et al., 2018; Winfree et al., 2018). A diversity of non-dominant crop pollinating species could supplement service provision when dominant ones are performing sub-optimally; for example, after periods of disturbance (Senapathi et al., 2015), such as extreme weather events (Oliver et al., 2015b). Current crop pollinating species that are not dominant now may also ultimately replace dominant species should future environmental conditions force current pollinators outside their climatic niches (Oliver et al., 2015b). Bee species have been demonstrated to show differential responses to a range of factors, including daily weather (Brittain et al., 2013; Rogers et al., 2014) and long-term climate warming (Bartomeus et al., 2013). Conservation measures aimed at promoting greater species richness amongst crop pollinator communities may therefore be vital to protect service provision in light of accelerating rates of anthropogenic-induced environmental changes (Oliver et al., 2015a).
Study limitations
There are a number of caveats to consider when interpretating our results related to the stability of pollinator occurrence across our focal crops. Firstly, whilst the occupancy model estimates utilized in this study have been demonstrated to perform well at dealing with the many of the biases associated with using unstructured biological records (Isaac et al., 2014), there is some degree of uncertainty inherent within the output, which varies among bee species and years (Powney et al., 2019). Additionally, the low sample sizes of some of the categories, i.e., the primary pollinators, could also have resulted in slightly less accurate estimates of variance compared to the other two larger categories of pollinators, in the analyses related to the stability of pollinator occurrence. However, whilst we treated all bee species, years and categories equally, we were largely investigating relative change in mean occupancy estimates, and not making any predictions based upon absolute values. The patterns and observations reported should reflect the true differences among each crop's stability of pollinator occurrence.
Furthermore, whilst there is known to be a positive relationship between species occupancy and abundance (Holt et al., 2002), this may not be the case for all species and all contexts, and may also depend on the scale at which occupancy is considered. We also looked specifically at bee species occupancy, but ecosystem service function depends not only on species occurrence, but also their local abundance (Waldock et al., 2021). We make the assumption here that pollinator occurrence and abundance are closely related, based upon prior evidence that inter-annual changes in citizen science collected distribution records are a reasonable proxy for inter-annual changes in abundance (Mason et al., 2018). However, further work exploring this relationship is urgently required. The positive relationship observed between occupancy and abundance can be noisy and non-linear for some taxa (Schulz et al., 2020). This inconsistent relationship between abundance and occupancy is likely driven by different life histories amongst species, and more research is needed to further understand the relationship between the two measures (Steenweg et al., 2018). Nonetheless given the difficulties in identifying many species, true abundance data to investigate bee trends would be difficult to obtain and our results likely represent the best alternative in its absence. The differing geographical coverage of individual crops may also play an influential role and should also be considered in future research. For example, whilst field bean and oilseed are grown across much of lowland Britain, apple and strawberry production is more concentrated around areas of south-east and western England, where agri-environment participation is generally lower (Image et al., 2022). Consequently, these landscape differences may impact upon the occupancy dynamics of bee species. Finally, whilst bees are the primary pollinators of insect-pollinated crops (Potts et al., 2010), many other insect groups are important pollinators of many crops (Rader et al., 2016). For example, Diptera, notably hoverflies, are known to be abundant and effective pollinators of a wide range of crop types (Rader et al., 2020). A comprehensive review of the impacts of inter-annual occupancy dynamics and community diversity on crop pollination service would also need to consider these, and other, insect groups.
Conclusions
Bee pollinators provide a critical ecosystem service to agriculture by pollinating the majority of leading global crops (Klein et al., 2007). As well as improving the quantity and quality of crop yields (Bommarco et al., 2012; Garratt et al., 2014a; Klatt et al., 2014), increasing evidence indicates that diverse insect pollinator communities can positively influence pollination service stability (Klein, 2009; Garibaldi et al., 2011). Our study indicates that species rich and diverse bee crop pollinator communities exhibit more diverse inter-annual occupancy dynamics and show less variance in mean occupancy than communities composed of small numbers of closely related species. High inter-annual variations in pollination service pose risks to both average crop yields and yield stability, and threaten food security (Senapathi et al., 2021). Given evidence of a positive link between species diversity and the stability of crop pollination services (Garibaldi et al., 2015; Senapathi et al., 2021), which is also supported here, agri-environment schemes aimed at conserving ecosystem-providing organisms (Batáry et al., 2015) may benefit from a greater consideration of solitary bee resource provision. Solitary species are highly speciose (Wood et al., 2016) and are important pollinators of many crops (Woodcock et al., 2013; Martins et al., 2015; Russo et al., 2017; Perrot et al., 2018). Given differences in pollinator community composition, crop-specific management, aimed at specific pollinator taxa, should also help improve future crop pollinator service provision (Garratt et al., 2014b).
Data availability statement
The data analyzed in this study is subject to the following licenses/restrictions: The raw occupancy data, which is owned by the UK Centre for Ecology and Hydrology (CEH), and not the main authorship team, cannot be deposited publicly, but can be accessed via reasonable request to CEH. Requests to access these datasets should be directed to GP - Z2F3bkBjZWguYWMudWs=. Requests to access the data used in the analyses should be directed to the corresponding author.
Author contributions
LH, TB, TO, and MPDG: conceptualization. LH, TB, TO, MPDG, MPG, and GP: research design, writing manuscript, and editing manuscript. GP: data collection. LH and MPG: data analysis. All authors contributed to the article and approved the submitted version.
Funding
LH was supported by NERC QMEE CDT, Grant Number: NE/P012345/1. TB and TO were supported by the Global Food Security Programme Modelling Landscape for Resilient Pollination Services' project, Grant Number: BB/R00580X/1. MPG was supported by the NERC SCENARIO Doctoral Training Partnership.
Acknowledgments
We would like to thank Mauricio Gonzalez-Chang and Costanza Geppert for their constructive comments.
Conflict of interest
The authors declare that the research was conducted in the absence of any commercial or financial relationships that could be construed as a potential conflict of interest.
Publisher's note
All claims expressed in this article are solely those of the authors and do not necessarily represent those of their affiliated organizations, or those of the publisher, the editors and the reviewers. Any product that may be evaluated in this article, or claim that may be made by its manufacturer, is not guaranteed or endorsed by the publisher.
Supplementary material
The Supplementary Material for this article can be found online at: https://www.frontiersin.org/articles/10.3389/fsufs.2022.943309/full#supplementary-material
References
Adamson, N. L., Roulston, T. H., Fell, R. D., and Mullins, D. E. (2012). From april to august-wild bees pollinating crops through the growing season in Virginia, USA. Environ. Entomol. 41, 813–821. doi: 10.1603/EN1207310.1603/EN12073
Aizen, M. A., Aguiar, S., Biesmeijer, J. C., Garibaldi, L. A., Inouye, D. W., Jung, C., et al. (2019). Global agricultural productivity is threatened by increasing pollinator dependence without a parallel increase in crop diversification. Glob. Change Biol. 25, 3516–3527. doi: 10.1111/gcb.14736
Allen, G., Halsall, C. J., Ukpebor, J., Paul, N. D., Ridall, G., and Wargent, J. J. (2015). Increased occurrence of pesticide residues on crops grown in protected environments compared to crops grown in open field conditions. Chemosphere 119, 1428–1435. doi: 10.1016/j.chemosphere.2014.10.066
Bänsch, S., Tscharntke, T., Gabriel, D., and Westphal, C. (2021). Crop pollination services: complementary resource use by social vs solitary bees facing crops with contrasting flower supply. J. Appl. Ecol. 58, 476–485. doi: 10.1111/1365-2664.13777
Bartomeus, I., Park, M. G., Gibbs, J., Danforth, B. N., Lakso, A. N., and Winfree, R. (2013). Biodiversity ensures plant–pollinator phenological synchrony against climate change. Ecol. Lett. 16, 1331–1338. doi: 10.1111/ele.12170
Batáry, P., Dicks, L. V., Kleijn, D., and Sutherland, W. J. (2015). The role of agri-environment schemes in conservation and environmental management. Conservation Biology 29, 1006–1016. doi: 10.1111/cobi.12536
Bommarco, R., Marini, L., and Vaissière, B. E. (2012). Insect pollination enhances seed yield, quality, and market value in oilseed rape. Oecologia 169, 1025–1032. doi: 10.1007/s00442-012-2271-6
Bond, D. A., and Kirby, E. J. M. (1999). Anthophora plumipes (Hymenoptera: Anthophoridae) as a pollinator of broad bean (Vicia faba major). J. Apicult. Res. 38, 199–203.
Breeze, T. D., Bailey, A. P., Balcombe, K. G., Brereton, T., Comont, R., Edwards, M., et al. (2021). Pollinator monitoring more than pays for itself. J. Appl. Ecol. 58, 44–57. doi: 10.1111/1365-2664.13755
Brittain, C., Kremen, C., and Klein, A. M. (2013). Biodiversity buffers pollination from changes in environmental conditions. Global Change Biology 19, 540–547. doi: 10.1111/gcb.12043
BWARS. (2020). Bees, Wasps and Ants Recording Society. Available online at: https://www.bwars.com/home (accessed May 13, 2022).
BWARS. (2021). Anthophora Plumipes. Available online at: https://www.bwars.com/bee/apidae/anthophora-plumipes (accessed May 13, 2022).
Cardinale, B. J., Srivastava, D. S., Duffy, J. E., Wright, J. P., Downing, A. L., Sankaran, M., et al. (2006). Effects of biodiversity on the functioning of trophic groups and ecosystems. Nature 443, 989–992. doi: 10.1038/nature05202
Carvalho, J. C., Cardoso, P., Crespo, L. C., Henriques, S., Carvalho, R., and Gomes, P. (2011). Biogeographic patterns of spiders in coastal dunes along a gradient of mediterraneity. Biodivers. Conserv. 20, 873–894. doi: 10.1007/s10531-011-0001-8
Chagnon, M., Gingras, J., and DeOliveira, D. (1993). Complementary aspects of strawberry pollination by honey and indigenous bees (hymenoptera). J. Econ. Entomol. 86, 416–420.
Cook, S. M., Awmack, C. S., Murray, D. A., and Williams, I. H. (2003). Are honey bees' foraging preferences affected by pollen amino acid composition? Ecol. Entomol. 28, 622–627. doi: 10.1046/j.1365-2311.2003.00548.x
Else, G. R., and Edwards, M. (2018). Handbook of the Bees of the British Isles: Volume 2. London: Ray Society.
Fijen, T. P., Scheper, J. A., Boom, T. M., Janssen, N., Raemakers, I., and Kleijn, D. (2018). Insect pollination is at least as important for marketable crop yield as plant quality in a seed crop. Ecol. Lett. 21, 1704–1713. doi: 10.1111/ele.13150
Forrest, J. R., Thorp, R. W., Kremen, C., and Williams, N. M. (2015). Contrasting patterns in species and functional-trait diversity of bees in an agricultural landscape. J. Appl. Ecol. 52, 706–715. doi: 10.1111/1365-2664.12433
Gardner, E., Breeze, T. D., Clough, Y., Smith, H. G., Baldock, K. C., Campbell, A., et al. (2021). Field boundary features can stabilise bee populations and the pollination of mass-flowering crops in rotational systems. J. Appl. Ecol. 58, 2287–2304. doi: 10.1111/1365-2664.13948
Garibaldi, L. A., Aizen, M. A., Klein, A. M., Cunningham, S. A., and Harder, L. D. (2011). Global growth and stability of agricultural yield decrease with pollinator dependence. Proc. Natl. Acad. Sci. U. S. A. 108, 5909–5914. doi: 10.1073/pnas.1012431108
Garibaldi, L. A., Bartomeus, I., Bommarco, R., Klein, A. M., Cunningham, S. A., Aizen, M. A., et al. (2015). Trait matching of flower visitors and crops predicts fruit set better than trait diversity. J. Appl. Ecol. 52, 1436–1444. doi: 10.1111/1365-2664.12530
Garratt, M. P., Breeze, T. D., Jenner, N., Polce, C., Biesmeijer, J. C., and Potts, S. G. (2014a). Avoiding a bad apple: insect pollination enhances fruit quality and economic value. Agric. Ecosyst. Environ. 184, 34–40. doi: 10.1016/j.agee.2013.10.032
Garratt, M. P., Coston, D. J., Truslove, C. L., Lappage, M. G., Polce, C., Dean, R., et al. (2014b). The identity of crop pollinators helps target conservation for improved ecosystem services. Biol. Conserv. 169, 128–135. doi: 10.1016/j.biocon.2013.11.001
Goulson, D., Hanley, M. E., Darvill, B., Ellis, J. S., and Knight, M. E. (2005). Causes of rarity in bumblebees. Biol. Conserv. 122, 1–8. doi: 10.1016/j.biocon.2004.06.017
Goulson, D., Lye, G. C., and Darvill, B. (2008). Decline and conservation of bumble bees. Ann. Rev. Entomol. 53, 191–208. doi: 10.1146/annurev.ento.53.103106.093454
Greenwell, M. P., Brereton, T., Day, J. C., Roy, D. B., and Oliver, T. H. (2019). Predicting resilience of ecosystem functioning from co-varying species' responses to environmental change. Ecol. Evolut. 9, 11775–11790. doi: 10.1002/ece3.5679
Gresty, C. E., Clare, E., Devey, D. S., Cowan, R. S., Csiba, L., Malakasi, P., et al. (2018). Flower preferences and pollen transport networks for cavity-nesting solitary bees: implications for the design of agri-environment schemes. Ecol. Evolut. 8, 7574–7587. doi: 10.1002/ece3.4234
Hautier, Y., Tilman, D., Isbell, F., Seabloom, E. W., Borer, E. T., and Reich, P. B. (2015). Anthropogenic environmental changes affect ecosystem stability via biodiversity. Science 348, 336–340. doi: 10.1126/science.aaa1788
Holt, A. R., Gaston, K. J., and He, F. (2002). Occupancy-abundance relationships and spatial distribution: a review. Basic Appl. Ecol. 3, 1–13. doi: 10.1078/1439-1791-00083
Hünicken, P. L., Morales, C. L., Aizen, M. A., Anderson, G. K., García, N., and Garibaldi, L. A. (2021). Insect pollination enhances yield stability in two pollinator-dependent crops. Agri. Ecosyst. Environ. 320, 107573. doi: 10.1016/j.agee.2021.107573
Hutchinson, L. A., Oliver, T. H., Breeze, T. D., Bailes, E. J., Brünjes, L., Campbell, A. J., et al. (2021). Using ecological and field survey data to establish a national list of the wild bee pollinators of crops. Agric. Ecosyst. Environ. 315, 107447. doi: 10.1016/j.agee.2021.107447
Image, M., Gardner, E., Clough, Y., Smith, H. G., Baldock, K. C., Campbell, A., et al. (2022). Does agri-environment scheme participation in England increase pollinator populations and crop pollination services? Agric. Ecosyst. Environ. 325, 107755. doi: 10.1016/j.agee.2021.107755
Isaac, N. J., van Strien, A. J., August, T. A., de Zeeuw, M. P., and Roy, D. B. (2014). Statistics for citizen science: extracting signals of change from noisy ecological data. Methods Ecol. Evolut. 5, 1052–1060. doi: 10.1111/2041-210X.12254
Kammerer, M., Goslee, S. C., Douglas, M. R., Tooker, J. F., and Grozinger, C. M. (2021). Wild bees as winners and losers: relative impacts of landscape composition, quality, and climate. Glob. Change Biol. 27, 1250–1265. doi: 10.1111/gcb.15485
Karp, D. S., Ziv, G., Zook, J., Ehrlich, P. R., and Daily, G. C. (2011). Resilience and stability in bird guilds across tropical countryside. Proc. Natl. Acad. Sci. U. S. A. 108, 21134–21139. doi: 10.1073/pnas.1118276108
Kelley, L. A., Gardner, S. P., and Sutcliffe, M. J. (1996). An automated approach for clustering an ensemble of NMR-derived protein structures into conformationally related subfamilies. Protein Eng. Des. Select. 9, 1063–1065.
Klatt, B. K., Holzschuh, A., Westphal, C., Clough, Y., Smit, I., Pawelzik, E., et al. (2014). Bee pollination improves crop quality, shelf life and commercial value. Proc. R. Soc. B Biol. Sci. 281, 20132440. doi: 10.1098/rspb.2013.2440
Kleijn, D., Winfree, R., Bartomeus, I., Carvalheiro, L. G., Henry, M., Isaacs, R., et al. (2015). Delivery of crop pollination services is an insufficient argument for wild pollinator conservation. Nat. Commun. 6, 7414. doi: 10.1038/ncomms8414
Klein, A. M. (2009). Nearby rainforest promotes coffee pollination by increasing spatio-temporal stability in bee species richness. Forest Ecol. Manag. 258, 1838–1845. doi: 10.1016/j.foreco.2009.05.005
Klein, A. M., Vaissiere, B. E., Cane, J. H., Steffan-Dewenter, I., Cunningham, S. A., Kremen, C., et al. (2007). Importance of pollinators in changing landscapes for world crops. Proc. R. Soc. B Biol. Sci. 274, 303–313. doi: 10.1098/rspb.2006.3721
Kremen, C., Williams, N. M., and Thorp, R. W. (2002). Crop pollination from native bees at risk from agricultural intensification. Proc. Nat. Acad. Sci. 99, 16812–16816. doi: 10.1073/pnas.262413599
Martins, K. T., Albert, C. H., Lechowicz, M. J., and Gonzalez, A. (2018). Complementary crops and landscape features sustain wild bee communities. Ecol. Appl. 28, 1093–1105. doi: 10.1002/eap.1713
Martins, K. T., Gonzalez, A., and Lechowicz, M. J. (2015). Pollination services are mediated by bee functional diversity and landscape context. Agric. Ecosyst. Environ. 200, 12–20. doi: 10.1016/j.agee.2014.10.018
Mason, S. C., Hill, J. K., Thomas, C. D., Powney, G. D., Fox, R., Brereton, T., et al. (2018). Population variability in species can be deduced from opportunistic citizen science records: a case study using British butterflies. Insect Conserv. Diversity 11, 131–142. doi: 10.1111/icad.12242
Melero, Y., Evans, L. C., Kuussaari, M., Schmucki, R., Stefanescu, C., Roy, D. B. and Oliver, T. H. (2022). Local adaptation to climate anomalies relates to species phylogeny. Commun. Biol. 5, 1–9. doi: 10.1038/s42003-022-03088-3
Morin, X., Fahse, L., De Mazancourt, C., Scherer-Lorenzen, M., and Bugmann, H. (2014). Temporal stability in forest productivity increases with tree diversity due to asynchrony in species dynamics. Ecol. Lett. 17, 1526–1535. doi: 10.1111/ele.12357
Oliver, T. H., Heard, M. S., Isaac, N. J., Roy, D. B., Procter, D., Eigenbrod, F., et al. (2015a). Biodiversity and resilience of ecosystem functions. Trends Ecol. Evolut. 30, 673–684. doi: 10.1016/j.tree.2015.08.009
Oliver, T. H., Isaac, N. J., August, T. A., Woodcock, B. A., Roy, D. B., and Bullock, J. M. (2015b). Declining resilience of ecosystem functions under biodiversity loss. Nat. Commun. 6, 1–8. doi: 10.1038/ncomms10122
Ollerton, J. (2017). Pollinator diversity: distribution, ecological function, and conservation. Annu. Rev. Ecol. Evolut. Syst. 48, 353–376. doi: 10.1146/annurev-ecolsys-110316-022919
Outhwaite, C., and Issac, N. J. (2015). Building a Better Indicator. Available online at: https://methodsblog.com/2015/12/11/building-a-better-indicator/ (accessed May 13, 2022).
Outhwaite, C. L., Chandler, R. E., Powney, G. D., Collen, B., Gregory, R. D., and Isaac, N. J. (2018). Prior specification in Bayesian occupancy modelling improves analysis of species occurrence data. Ecol. Indicat. 93, 333–343. doi: 10.1016/j.ecolind.2018.05.010
Perrot, T., Gaba, S., Roncoroni, M., Gautier, J. L., and Bretagnolle, V. (2018). Bees increase oilseed rape yield under real field conditions. Agric. Ecosyst. Environ. 266, 39–48. doi: 10.1016/j.agee.2018.07.020
Potts, S. G., Biesmeijer, J. C., Kremen, C., Neumann, P., Schweiger, O., and Kunin, W. E. (2010). Global pollinator declines: trends, impacts and drivers. Trends Ecol. Evolut. 25, 345–353. doi: 10.1016/j.tree.2010.01.007
Powney, G. D., Carvell, C., Edwards, M., Morris, R. K., Roy, H. E., Woodcock, B. A., et al. (2019). Widespread losses of pollinating insects in Britain. Nat. Commun. 10, 1018. doi: 10.1038/s41467-019-08974-9
Powney, G. D., and Isaac, N. J. (2015). Beyond maps: a review of the applications of biological records. Biol. J. Linnean Soc. 115, 532–542. doi: 10.1111/bij.12517
R Core Team. (2020). R: a language and environment for statistical computing. vienna, austria: R foundation for statistical computing. Available online at: https://www.r-project.org/ (accessed May 13, 2022).
Rader, R., Bartomeus, I., Garibaldi, L. A., Garratt, M. P., Howlett, B. G., Winfree, R., et al. (2016). Non-bee insects are important contributors to global crop pollination. Proc. Natl. Acad. Sci. U. S. A. 113, 146–151. doi: 10.1073/pnas.1517092112
Rader, R., Cunningham, S. A., Howlett, B. G., and Inouye, D. W. (2020). Non-bee insects as visitors and pollinators of crops: biology, ecology, and management. Annu. Rev. Entomol. 65, 391–407. doi: 10.1146/annurev-ento-011019-025055
Reilly, J. R., Artz, D. R., Biddinger, D., Bobiwash, K., Boyle, N. K., Brittain, C., et al. (2020). Crop production in the USA is frequently limited by a lack of pollinators. Proc. R. Soc. B 287, 20200922. doi: 10.1098/rspb.2020.0922
Rogers, S. R., Tarpy, D. R., and Burrack, H. J. (2014). Bee species diversity enhances productivity and stability in a perennial crop. PLoS ONE 9, e97307. doi: 10.1371/journal.pone.0097307
Roubik, D. W. (2001). Ups and downs in pollinator populations: when is there a decline? Conserv. Ecol. 5, 2. doi: 10.5751/ES-00255-050102
RStudio Team. (2020). RStudio: Integrated Development for R. RStudio, PBC, Boston, MA. Available online at: http://www.rstudio.com/ (accessed May 13, 2022).
Russo, L., Park, M. G., Blitzer, E. J., and Danforth, B. N. (2017). Flower handling behavior and abundance determine the relative contribution of pollinators to seed set in apple orchards. Agric. Ecosyst. Environ. 246, 102–108. doi: 10.1016/j.agee.2017.05.033
Schulz, T., Vanhatalo, J., and Saastamoinen, M. (2020). Long-term demographic surveys reveal a consistent relationship between average occupancy and abundance within local populations of a butterfly metapopulation. Ecography 43, 306–317. doi: 10.1111/ecog.04799
Schwartz, M. W., Brigham, C. A., Hoeksema, J. D., Lyons, K. G., Mills, M. H., and Van Mantgem, P. J. (2000). Linking biodiversity to ecosystem function: implications for conservation ecology. Oecologia 122, 297–305. doi: 10.1007/s004420050035
Science for Environment Policy. (2020). “Pollinators: importance for nature and human well-being, drivers of decline and the need for monitoring,” in Future Brief 23. Brief Produced for the European Commission DG Environment. Bristol: Science Communication Unit, UWE Bristol.
Senapathi, D., Biesmeijer, J. C., Breeze, T. D., Kleijn, D., Potts, S. G., and Carvalheiro, L. G. (2015). Pollinator conservation—the difference between managing for pollination services and preserving pollinator diversity. Curr. Opin. Insect Sci. 12, 93–101. doi: 10.1016/j.cois.2015.11.002
Senapathi, D., Fründ, J., Albrecht, M., Garratt, M. P., Kleijn, D., Pickles, B. J., et al. (2021). Wild insect diversity increases inter-annual stability in global crop pollinator communities. Proc. R. Soc. B 288, 20210212. doi: 10.1098/rspb.2021.0212
Soultan, A., Wikelski, M., and Safi, K. (2020). Classifying biogeographic realms of the endemic fauna in the Afro-Arabian region. Ecol. Evolut. 10, 8669–8680. doi: 10.1002/ece3.6562
Steenweg, R., Hebblewhite, M., Whittington, J., Lukacs, P., and McKelvey, K. (2018). Sampling scales define occupancy and underlying occupancy–abundance relationships in animals. Ecology 99, 172–183. doi: 10.1002/ecy.2054
Suárez-Castro, A. F., Raymundo, M., Bimler, M., and Mayfield, M. M. (2022). Using multi-scale spatially explicit frameworks to understand the relationship between functional diversity and species richness. Ecography e05844. doi: 10.1111/ecog.05844
Vanbergen, A. J., Heard, M. S., Breeze, T., Potts, S. G., and Hanley, N. (2014). Status and Value of Pollinators and Pollination Services. London: Department for Environment, Food and Rural Affairs, UK, 53.
Vasiliev, D., and Greenwood, S. (2020). Pollinator biodiversity and crop pollination in temperate ecosystems, implications for national pollinator conservation strategies: mini review. Sci. Total Environ. 744, 140880. doi: 10.1016/j.scitotenv.2020.140880
Waldock, C., Stuart-Smith, R. D., Albouy, C., Cheung, W. W., Edgar, G. J., Mouillot, D., et al. (2021). A quantitative review of abundance-based species distribution models. Ecography e05694. doi: 10.1101/2021.05.25.445591
White, D., and Gramacy, R. B. (2012). MAPTREE: Mapping, Pruning, and Graphing Tree Models. R Package Version 1. p. 4–7. Available online at: https://cran.rproject.org/web/packages/maptree/index.html (accessed May 13, 2022).
Williams, N. M., Crone, E. E., T'ai, H. R., Minckley, R. L., Packer, L., and Potts, S. G. (2010). Ecological and life-history traits predict bee species responses to environmental disturbances. Biol. Conserv. 143, 2280–2291. doi: 10.1016/j.biocon.2010.03.024
Williams, N. M., Minckley, R. L., and Silveira, F. A. (2001). Variation in native bee faunas and its implications for detecting community changes. Conserv. Ecol. 5, 1. doi: 10.5751/ES-00259-050107
Williams, P. (2005). Does specialization explain rarity and decline among British bumblebees? A response to Goulson et al. Biol. Conserv. 122, 33–43. doi: 10.1016/j.biocon.2004.06.019
Winfree, R. (2013). Global change, biodiversity, and ecosystem services: what can we learn from studies of pollination? Basic Appl. Ecol. 14, 453–460. doi: 10.1016/j.baae.2013.07.004
Winfree, R., Reilly, J. R., Bartomeus, I., Cariveau, D. P., Williams, N. M., and Gibbs, J. (2018). Species turnover promotes the importance of bee diversity for crop pollination at regional scales. Science 359, 791–793. doi: 10.1126/science.aao2117
Wood, T. J., Holland, J. M., and Goulson, D. (2015). Pollinator-friendly management does not increase the diversity of farmland bees and wasps. Biol. Conserv. 187, 120–126. doi: 10.1016/j.biocon.2015.04.022
Wood, T. J., Holland, J. M., and Goulson, D. (2016). Diet characterisation of solitary bees on farmland: dietary specialisation predicts rarity. Biodiv. Conserv. 25, 2655–2671. doi: 10.1007/s10531-016-1191-x
Wood, T. J., Holland, J. M., and Goulson, D. (2017). Providing foraging resources for solitary bees on farmland: current schemes for pollinators benefit a limited suite of species. J. Appl. Ecol. 54, 323–333. doi: 10.1111/1365-2664.12718
Woodcock, B. A., Edwards, M., Redhead, J., Meek, W. R., Nuttall, P., Falk, S., et al. (2013). Crop flower visitation by honeybees, bumblebees and solitary bees: behavioural differences and diversity responses to landscape. Agric. Ecosyst. Environ. 171, 1–8. doi: 10.1016/j.agee.2013.03.005
Yachi, S., and Loreau, M. (1999). Biodiversity and ecosystem productivity in a fluctuating environment: the insurance hypothesis. Proc. Natl. Acad. Sci. U. S. A. 96, 1463–1468.
Zattara, E. E., and Aizen, M. A. (2021). Worldwide occurrence records suggest a global decline in bee species richness. One Earth 4, 114–123. doi: 10.1016/j.oneear.2020.12.005
Keywords: wild bees, crops, pollination stability, ecosystem service resilience, Bayesian occupancy models
Citation: Hutchinson LA, Oliver TH, Breeze TD, Greenwell MP, Powney GD and Garratt MPD (2022) Stability of crop pollinator occurrence is influenced by bee community composition. Front. Sustain. Food Syst. 6:943309. doi: 10.3389/fsufs.2022.943309
Received: 13 May 2022; Accepted: 07 July 2022;
Published: 02 August 2022.
Edited by:
Matteo Dainese, Eurac Research, ItalyReviewed by:
Mauricio Gonzalez-Chang, Universidad Austral de Chile, ChileCostanza Geppert, University of Padua, Italy
Copyright © 2022 Hutchinson, Oliver, Breeze, Greenwell, Powney and Garratt. This is an open-access article distributed under the terms of the Creative Commons Attribution License (CC BY). The use, distribution or reproduction in other forums is permitted, provided the original author(s) and the copyright owner(s) are credited and that the original publication in this journal is cited, in accordance with accepted academic practice. No use, distribution or reproduction is permitted which does not comply with these terms.
*Correspondence: Louise A. Hutchinson, bC5odXRjaGluc29uQHBnci5yZWFkaW5nLmFjLnVr