- 1Department of Horticulture, Northwestern Washington Research and Extension Center, Washington State University, Mount Vernon, WA, United States
- 2Department of Plant Pathology, Puyallup Research and Extension Center, Washington State University, Puyallup, WA, United States
Double-cropping strawberry (Fragaria × ananassa Duch.) and lettuce (Lactuca sativa L.) could be a sustainable alternative practice for diversified specialty crop growers. Plastic mulch is beneficial for strawberry and lettuce production with soil-biodegradable mulch (BDM) providing opportunities to reduce plastic waste generation and costs of mulch disposal. The objective of this study was to compare non-biodegradable plastic mulches and BDM to bare ground cultivation in a strawberry–lettuce double-cropping system in a Mediterranean climate. A split-plot randomized complete block design experiment with mulch treatment [non-biodegradable polyethylene (PE) film, “weedmat” (woven, PE-based), BDM, and bare ground control] as the main factor and double-cropping rotation scheme (rotation 1 or 2) as the split-plot factor was conducted between 2020 and 2021. Strawberry yield was overall greatest when mulched with BDM, PE, and weedmat compared to the bare ground control. Total soluble solids (TSS), pH, and total titratable acidity (TA) of strawberry differed due to harvest date, but only pH differed due to mulch treatment. For lettuce canopy cover, rotation 2 had greater canopy cover (86%) compared with rotation 1 (66%) 30 days after transplanting. Average head weight (0.4 kg) and head length and diameter (both 20 cm) did not differ due to mulch or rotation. Average soil temperatures under PE and BDM were ~1.5°C higher than under weedmat and 2.5°C higher than the bare ground control. Soil under weedmat had the highest moisture content, whereas the PE, BDM, and bare ground treatments had similar levels. PE, BDM, and weedmat provided equivalent weed suppression, whereas the bare ground control had the most weeds. BDM deterioration estimated as percent soil exposure (PSE) was greatest (59% PSE) by the end of the experiment, whereas PE had 18% PSE and weedmat had no deterioration. Results show that despite high levels of deterioration, BDM performs similarly to PE in terms of soil temperature and moisture modification, weed suppression, and promotion of crop growth and yield in a double-cropped system. Double-cropping strawberry and lettuce is a promising alternative for growers to diversify their crop type and with potential economic benefits by increasing mulch lifespan.
Introduction
The global strawberry industry is expanding due to increased consumer, grocer, and distributor demands for year-around, high-quality fruit (Vanden Heuvel and Lewers, 2008; Samtani et al., 2019; Food and Agriculture Organization of the United States, 2020; Kramer et al., 2021). In the United States (US), the average per capita consumption of fresh strawberries between 2017 and 2019 was about 3.1 kg, more than twice the average consumed during the period between 2000 and 2002 (Kramer et al., 2021). While states like California and Florida lead US strawberry production (Kramer et al., 2021), local production is an important contributor to local and regional food systems.
To remain competitive and to maximize production efficiencies, local specialty crop growers that cultivate strawberry need to develop efficient production practices to remain economically viable while meeting the increasing demands of the fresh market. Double cropping is one strategy that may increase production efficiencies and entails harvesting two crops from the same area, sometimes within the same year for short-cycled crops, but can include multiple years depending on the system and crop. For example, double cropping has been used by corn (Zea mays L.), soybean (Glycine max L.), and wheat (Triticum spp. L.) growers in Brazil due to its high efficiency in land use and resource utilization (Elobeid et al., 2019). In Europe, an empirical analysis showed that increased diversification of land use and management in wheat fields in a Mediterranean climate can increase yield resiliency caused by climate change (Reidsma and Ewert, 2008). Another double-cropping system used to maintain soil nitrogen content is called biomass double cropping and entails planting a warm-season primary crop followed by a cool-season, fall-planted cover crop; this system can reduce nitrate leaching in late spring and fall when compared to a sole- or single-cropping system (Heggenstaller et al., 2008). A tomato (Solanum lycopersicum L.)–lettuce double-cropping system in unheated plastic houses in Spain showed fall-planted lettuce suppressed populations of root-knot nematodes (Meloidogyne javanica L.) in winter, which decreased root-knot nematode pressure on tomato the next year spring (Verdejo-Lucas et al., 2003).
Vegetable crops such as sweet corn, squash (Cucurbita pepo L.), snap bean (Phaseolus vulgaris L.), cucumber (Cucumis sativus L.), and muskmelon (Cucumis melo L.) can be effectively grown in a double-cropping system with strawberry (Albregts and Howard, 1985; Lamont and Poling, 1986; Lamont, 1996), but double-cropping strawberry and lettuce is a relatively new approach and there is little research on this production system. However, production guidelines suggest that day-neutral strawberry and lettuce both grown for fresh market may be well-suited for double cropping together due to their high compatibility for irrigation and nutrient requirements, the potential for short growing cycles, and similar target markets (Whitaker et al., 1962; Galinato and Miles, 2012; Galinato and Walters, 2012; Hoashi-Erhardt and Walters, 2014). Furthermore, lettuce is an economically important leaf vegetable with increasing global production (Mou, 2008; Index Box Platform, 2022), and combined with fresh market strawberry could expand growers' crop diversity.
Double cropping can be more profitable than annual sole cropping in PE-based plasticulture systems because it can maximize the efficiency of PE mulch use by extending the mulches' lifespan, thereby reducing plastic waste generation and labor costs for removal (Waterer et al., 2008; Shah and Wu, 2020). PE mulch is beneficial to produce strawberry and lettuce because it can optimize soil temperature and moisture, suppress weeds, conserve water and fertilizer, and in turn promote total and marketable yields (Lamont, 1993; Garwood, 1998; Fernandez et al., 2001; Freeman and Gnayem, 2005; Miles et al., 2012; Wallace et al., 2012; DeVetter et al., 2017). Weedmat is a black, woven PE or polypropylene ground cover used as an alternative to sawdust mulch in northern highbush blueberry (Vaccinium corymbosum L.) fields due to its long-term durability (Strik and Vance, 2017; Strik and Davis, 2020). The durability of weedmat could be beneficial in double-cropped systems, but its higher initial cost needs to be considered when assessing on-farm viability (Julian et al., 2012).
Soil-biodegradable plastic mulches (BDMs) are an alternative to non-biodegradable PE mulch and have been developed to promote sustainability in agriculture because they can be tilled into soil after harvest to reduce plastic waste generation (DeVetter et al., 2017; Miles et al., 2017). Commercially available BDM is made using polymeric feedstocks derived from a blend of biobased and fossil fuel sources (DeVetter et al., 2021). BDMs are designed to be biodegraded by native soil microorganisms (Khemani and Scholz, 2012). Studies of BDMs in day-neutral strawberry, pie pumpkin (Cucurbita pepo L.), and tomato show BDMs are an effective alternative to PE mulch and maintain yield and crop quality while contributing to weed suppression (Cowan et al., 2014; DeVetter et al., 2017; Ghimire et al., 2018; Zhang et al., 2020a). A meta-analysis of mulch performance also showed yields were equivalent between BDM and PE mulches (Tofanelli and Wortman, 2020). Commercially available BDMs should meet standards that ensure their quality and integrity in agriculture. EN-17033 is one important standard that states a BDM must reach at least 90% degradation in soil within two years (American Society for Testing and Materials, 2018; European Norms, 2018). While growers tend to consider only the higher initial price point of BDMs, BDMs may be more economically beneficial than non-biodegradable plastic mulches given costs for mulch removal and disposal are eliminated. In a double-cropping system, adopting BDMs could further enhance on-farm economics because a single mulch application can be used for producing two crops. However, no published studies show how BDMs perform in a double-cropped system, and their durability and horticultural compatibility need to be determined.
Given the potential of double-cropping and BDMs to support the efficient and sustainable production of strawberry and lettuce, the objective of this study was to compare different mulch types (PE, weedmat, and BDMs) to non-mulched, bare ground cultivation in a day-neutral strawberry–lettuce double-cropping system in a Mediterranean climate. Specific variables of interest were mulch performance and impacts on crop growth, weed suppression, yield, and crop quality. The hypotheses of this experiment were: (1) mulching will positively impact strawberry and lettuce growth, yields, and crop quality compared to the bare ground control; (2) PE, BDM, and weedmat will have equivalent effects on improving yields and crop quality; and (3) weedmat will be the most durable and undergo the least amount of deterioration followed by PE and then BDM.
Materials and methods
Experimental site and design
The experimental field site was located at the Washington State University Northwestern Washington Research and Extension Center (WSU NWREC) in Mount Vernon, Washington (Lat. 48.440226, Long. −122.396019, elevation 6 m). The area has a cool-summer Mediterranean climate (Beck et al., 2018) with average monthly temperatures of 16°C in the summer and 4°C in the winter, with 81% relative humidity in summer and 88% relative humidity in winter (Washington State University, 2020). Soil type was a Skagit silt loam, a fine-silty, mixed, non-acid, mesic Typic Fluvaquent (pH 6.5 and 26 g·kg−1 soil organic matter). The experiment was established as a split-plot randomized complete block design with mulch type as the main-plot factor and double-cropping rotation scheme as the split-plot factor. The main-plot treatments were: (1) PE (FilmTech, LLC., Stanley, WI, US; primary feedstock is PE; 25.4 μm thickness); (2) BDM [Organix Solutions, Bloomington, MN, US; primary feedstock is BASF Ecovio (polybutylene adipate-co-terephthalate, abbreviated as PBAT, and polylactic acid, abbreviated as PLA); 25.4 μm thickness]; (3) weedmat (Extenday, Yakima, WA, US; primary feedstock is PE; 85 g·m−2 density); and (4) a non-mulched, bare ground control. Split-plot treatments were two rotation schemes presented in Table 1. Main- and split-plot lengths were 8 and 3.8 m, respectively. All plots were 80 cm wide, and there were 3 m between row centers for each replicate. Buffer rows were on both sides of the experiment.
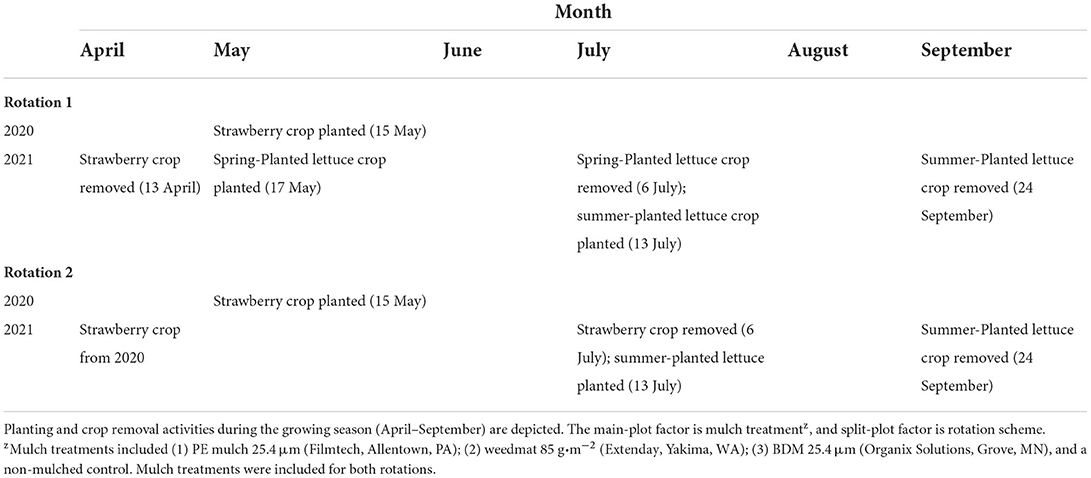
Table 1. Rotation scheme for a strawberry–lettuce double-cropping experiment conducted in a Mediterranean climate (northwestern Washington, USA) from April 2020 to September 2021.
Plot establishment
Pre-plant fertilizer (8N-0P-16K, Wilbur-Ellis Co., Mount Vernon, WA) was applied on 13 May 2020 with a 1.8-m-wide drop spreader over the center of all rows at the rate of 74 kg N·hectare−1. On 26 May 2020, 15–20-cm-high and 80-cm-wide raised beds were formed and mulches were mechanically laid using a dual-bed shaper and mulch layer (Model 2600 Bed Shaper; Rain-Flo Irrigation, East Pearl, PA). Mulches mechanically applied included PE, BDM, and weedmat. Drip irrigation tape (T-Tape, Model #508-08-340, 20 cm emitter spacing, 1.3 LPM per 30.5 m; John Deere, San Diego, CA) was installed underneath the mulch on the center of each raised bed at the same time as mulch laying. Within each raised bed, there were two staggered rows of planting holes made using a custom dibble spaced 30 cm apart within the row and 25 cm apart between rows. Each split plot contained 30 planting holes. On 15 May 2020, day-neutral “Albion” strawberry (sourced from Norcal Nursery, Inc., Anderson, CA) was planted manually in all plots. “Green Star” lettuce (green leaf type; Johnny's Selected Seeds, Fairfield, ME) was sown in 72-cell trays filled with potting mix (Sunshine #3 N and O; Sun Gro Horticulture, Agawam, MA) in the greenhouse at WSU NWREC on 15 April 2021 for spring transplantation (17 May) and 17 June 2021 for summer transplantation (13 July). Additional information on the timing of transplanting, harvesting, and plant removal activities of strawberry and lettuce is shown in Table 1.
Plot maintenance
Runners were clipped from strawberry plants four times in 2020 (29 July, 10 August, 15 September, and 17 October) and once in 2021 (9 June). From May through October 2020 and April through August 2021, drip irrigation was applied to all plots for 60–90 min twice per week or as needed based on soil volumetric moisture levels. Irrigation rate was adjusted based on precipitation. Post-plant liquid fertilizer (20N-8.7P-16.6K, Plant Marvel Laboratories, Inc., Chicago Heights, IL) was dissolved in water and injected into the irrigation system at a rate of 5.68 kg of N·hectare−1 and was followed by 30 min of irrigation. Fertigation was done weekly so that 143 kg of N·hectare−1 was applied annually in addition to the pre-plant fertilizer. This fertilizer regime is also well-suited for lettuce growth (based on Oregon State University, 2010 commercial guidelines). Integrated pest management was implemented for disease and pest control (see Supplementary material for a full description). Handweeding was performed immediately after weed data collection (see below).
Data collection
Strawberry data
Strawberry canopy growth
Strawberry canopy growth was measured in each split plot following weed data collection (see below). A permanent, 1-m-long area that spanned the width of the raised bed in the center of each split plot was marked with flags and used to access canopy cover twice monthly on the first and fifteenth of each month from May 2020 to September 2021. Canopy growth of six strawberry plants was determined after photographing the data collection area and analyzing each photograph using the Canopeo application (Canopeo, Stillwater, OK) developed by the Soil Physics Research Group at Oklahoma State University (Patrignani and Ochsner, 2015). Canopy cover was calculated as the percentage of foliage in the image.
Strawberry fruit yield
The total yield of strawberry fruits was determined from weekly harvests in summer 2020 and 2021. Strawberry harvests occurred six times in 2020, on 19 and 26 August, and 2, 9, 17, and 22 September and five times in 2021, on 31 May, 7 June, 14, 21, and 29 June. In each split plot, mature, uniformly red strawberry fruits were picked by hand. At each harvest, total yield, marketable fruit weight, unmarketable fruit weight, and marketable fruit number were recorded. Fruits were considered unmarketable due to sunburn, presence of mold, small size (<21 mm length), and other damages (e.g., bird or slug damage).
Strawberry fruit quality
Ten marketable strawberry fruits per split plot were randomly selected from each harvest, placed in plastic freezer bags, and stored at −4°C for later fruit quality analyses. Total soluble solids (TSS), pH, and total titratable acidity (using citric acid as the acid equivalent) per volume (g·L−1) were measured in triplicate from juice collected from each split plot for the early, mid, and late harvests. Early, mid, and late harvests corresponded to first and second harvests, third and fourth harvests, and fifth and sixth harvests, respectively. Frozen strawberry samples were thawed at room temperature (21°C) in the lab before juice extraction. Juice was obtained by squeezing samples through one layer of cheesecloth into beakers to obtain a juice free of visible particles. The TSS was measured using a digital refractometer (H19680 Refractometer; Hanna Instruments, Woonsocket, RI). The TA was measured using a digital titrator (HI-84532, Hanna Instruments, Woonsocket, RI) to an endpoint pH of 8.1 using 1 mol/L NaOH. In 2020, juice pH was measured simultaneously using the digital titrator. The methodology was adjusted in 2021 so that pH was measured separately using a digital handheld “pocket” pH meter (4311-E10, Atago® Instruction Manual, Tokyo, Japan). This change was made due to a malfunctioning of the pH meter attached to the titrator in the second year.
Lettuce data
Lettuce canopy cover
Percent canopy cover was similarly measured using digital photographs and the Canopeo application. Photographs were taken every 10 days after transplanting (DAT) until harvest in both rotations. Each photograph was taken 50 cm above the bed surface in the center of the data collection area that consisted of four plants in each subplot. Canopy cover was calculated as the percentage of foliage in the image.
Lettuce yield and quality
Lettuce was harvested in each subplot when 80% of the heads in the subplot reached marketable size (~20 cm diameter). Days to harvest were recorded for each subplot in both rotations. Total head weight and number were recorded, and average weight per head was calculated for each subplot. Ten representative heads were selected randomly from each subplot, total length and diameter were measured, and average head length and diameter were calculated.
Other mulch data
Soil temperature and moisture
Soil temperature and moisture sensors (Sensors ZL6, Meter Group, Inc., Pullman, WA) were installed in the second replicate row on 14 May 2020 in rotation 1 split plots for PE, BDM, and the bare ground control and in rotation 2 split plot for weedmat. Sensors were installed at a 15 cm depth, equally spaced between irrigation drip emitters, and data were recorded every 15 min until 21 September 2021.
Weed dry biomass
Weed dry biomass was measured five times in 2020 (15 June, 14 July, 1 August, 20 September, and 3 December) and 2021 (6 April, 13 May, 11 June, 6 July, and 11 August) in the same 1-m-long area where strawberry canopy growth was assessed. At each sampling date, weeds were collected and then placed into paper bags. Weeds were dried at 60°C for 48 h, and dry biomass was recorded.
Mulch performance
Percent soil exposure (PSE) was used to measure mulch deterioration over time and provides an assessment of mulch performance. PSE was visually estimated within the same 1-m-long area where strawberry canopy growth and weed dry biomass were measured. Ratings occurred twice monthly on the first and fifteenth of each month from May 2020 to September 2021 where a rating of 0% represents a completely intact mulch and 100% represents fully deteriorated mulch with exposed soil. Ratings were made in 1% increments until 20% deterioration, and in 5% increments thereafter (Cowan et al., 2014). Photographs of the PSE area were taken with a digital camera (Sony Cyber-shot DSC-RX0 II, Sony Electronics, San Diego, CA) every 2 weeks during the growing season for digital records.
Statistical analysis
Strawberry and lettuce data were analyzed differently due to horticultural differences between the two crops, number of factors, and resultant structure of the data.
Strawberry data analysis
Strawberry data were checked to ensure model assumptions were met and then subjected to the generalized linear mixed-model procedure using R Studio software (Version 1.4.1106, RStudio PBC, Boston, MA, US). Blocks were treated as a random factor with mulch treatment, year or days after planting (DAP), and rotation as fixed factors. Data were analyzed as a four-way analysis of variance with a least-squares mean option; a post-hoc Tukey–Kramer with an adjustment for multiple comparisons was used for estimates and tests of significance (α = 0.05). For strawberry canopy cover and fruit quality, pairwise comparisons were made using a Bonferroni adjustment. For strawberry fruit quality, P-values for interaction effects between mulch treatment and harvest date were presented. Soil moisture and temperature data are presented by mulch treatment and did not encompass the crop rotation factor. Correlation analysis between weed dry biomass and PSE for mulched treatments was conducted using the Pearson product-moment correlation coefficient method, and the correlation analysis was done by grouping rotation 1, rotation 2, and both of the two rotations separately.
Lettuce data analysis
Lettuce data were checked for assumptions of normality and homogeneity of variance using the Shapiro–Wilk test (α = 0.05) and the Levene's test (α = 0.05), respectively, and then subjected to analysis of variance using a linear mixed-model procedure in R Studio. Blocks were treated as a random factor with mulch treatment, DAT, and rotation as fixed factors. Tukey's honestly significant difference test at a significance level α < 0.05 was used to compare treatment means for significant differences. Additionally, for rotation 1, data were analyzed to assess the difference in canopy cover, yield, and quality due to mulch and lettuce planting time, while in rotation 2, data were analyzed to assess the difference due to mulch given planting time was not an appropriate comparison.
Results
Strawberry results
Strawberry canopy cover
Strawberry plant canopy cover differed due to mulch treatment (P < 0.001) and DAP (P < 0.001) but not due to rotation (P = 0.63; Table 2). Canopy cover differed at 61, 91, 126, 152, and 203 DAP due to mulch treatment. From 61 to 126 DAP (July 2020 to September 2020), strawberry grown in the mulch treatments (PE, BDM, and weedmat) had higher canopy cover than those grown in the bare ground control (P < 0.001). Although PE, BDM, and weedmat were not different from each other, the respective increase in the canopy growth compared to the bare ground control was 120, 100, and 90% by the end of the first year. The strawberry plants senesced during winter, from 126 to 203 DAP. In 2021, mulch treatment did not have any effect on canopy cover after 335 DAP.
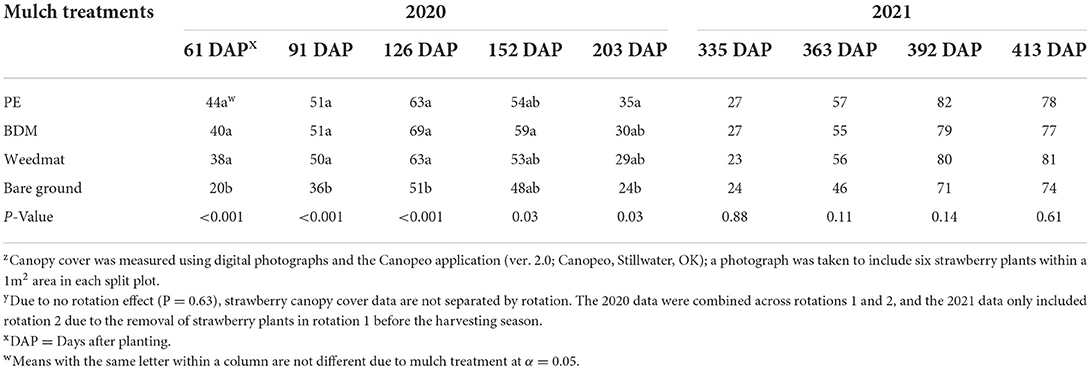
Table 2. Strawberry cv. Albion canopy coverz (%) when grown on raised beds with polyethylene (PE), soil-biodegradable mulch (BDM), weedmat, or bare ground treatments in a Mediterranean climate, May 2020 to July 2021y.
Strawberry fruit yield
Total and marketable yields differed due to mulch treatment (P < 0.001) and harvesting year (P < 0.001) but not due to rotation (P = 0.20). Marketable berry size differed due to year (P < 0.001) but not by mulch treatment (P = 0.24) nor rotation (P = 0.70). There were also no interactions between mulch treatment, harvesting year, and rotation. In 2020, total and marketable yields in both rotations were greatest in BDM (3.03 and 2.22 kg·m−2, respectively) and weedmat (2.83 and 2.18 kg·m−2, respectively) treatments than in the bare ground control (1.54 and 0.98 kg·m−2, respectively) and were intermediate in the PE treatment (2.28 and 1.65 kg·m−2, respectively) (Table 3). In 2021, strawberry grown with PE, BDM, and weedmat produced the highest total yield (6.08, 5.51, and 5.58 kg·m−2, respectively) compared to the bare ground control (4.04 kg·m−2). Marketable yields for strawberry grown with PE and BDM were on average 44% higher than the bare ground control, and weedmat was similar to all other treatments. The average marketable berry size in 2021 in all mulch treatments was smaller than in 2020.
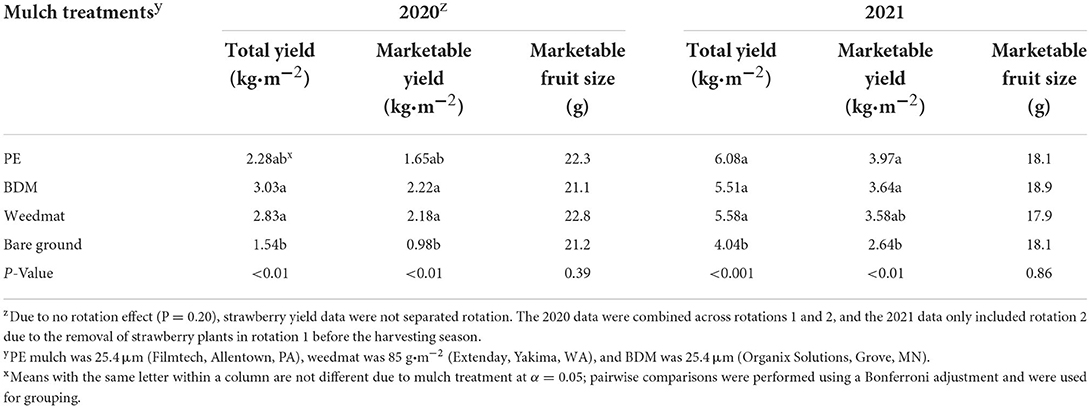
Table 3. Total yield, marketable yield, and marketable fruit size of strawberry cv. Albion grown on raised beds with polyethylene (PE), soil-biodegradable mulch (BDM), weedmat, or bare ground treatments in a Mediterranean climate, 2020 to 2021.
Strawberry fruit quality
The TSS in strawberry juice differed due to harvesting year (P < 0.001) and harvesting date (P < 0.001), but not due to mulch treatment (P = 0.49) or rotation (P = 0.95; Table 4). Fruit juice pH differed due to harvesting year (P < 0.001), mulch treatment (P < 0.001), and harvest date (P < 0.001) but not due to rotation (P = 0.72). The TA in strawberry juice differed due to harvesting year (P < 0.001), harvest date (P < 0.001), and rotation (P < 0.001) but not due to mulch treatment (P = 0.55). Interactions between harvesting year and harvest date (P < 0.001) were observed for TSS, pH, and TA, but there was no interaction between mulch treatment and harvest date. Mulch treatment only influenced pH in 2020 and 2021. Juice pH from plants grown in the bare ground control was on average 3% lower than juice from plants grown in the BDM and weedmat treatments and similar to the PE treatment in 2020. In 2021, juice pH from plants grown in the weedmat was highest, and that in the bare ground control was 2% lower than weedmat but similar to PE and BDM treatments. Overall, harvesting year and harvest date had the most consistent effect on strawberry fruit quality variables. In 2020, TSS and TA were higher than 2021 across all mulch treatments but juice pH was lower. Early harvest had higher TSS and TA than mid-harvest in both 2020 and 2021 growing seasons. Juice pH at early harvest was highest in 2020 but lowest in 2021. Early harvest had higher TSS and pH compared to late harvest in 2020 but were similar in 2021.
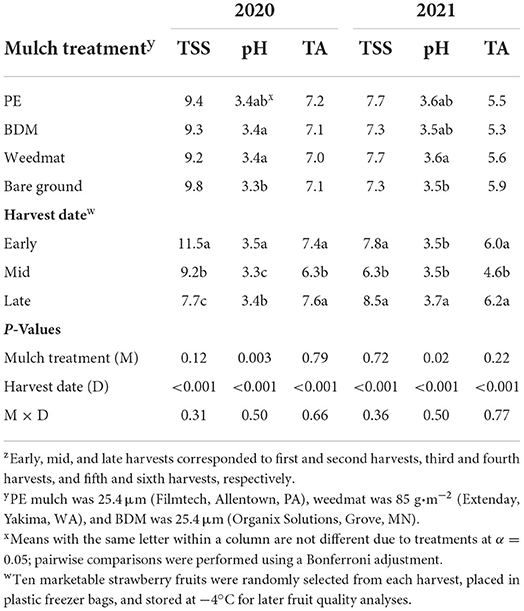
Table 4. Total soluble solids concentration (TSS, %), juice pH, and titratable acidity (TA; citric, g·L−1) of strawberry fruits cv. Albion harvested from plants grown on raised beds with polyethylene (PE), soil-biodegradable mulch (BDM), weedmat, or bare ground treatments in a Mediterranean climate, 2020 to 2021z.
Lettuce results
Lettuce canopy cover
Canopy cover (%) did not differ due to mulch (P = 0.10) but did differ due to rotation (P = 0.004) and increased over the growing season (P < 0.0001). There was no interaction between mulch and rotation (P = 0.89). Overall, rotation 2 had greater canopy cover (34%) compared with rotation 1 (27%) (data not presented). Average canopy cover was 3% at 10 DAT, 13.5% at 20 DAT, and 76% at 30 DAT (P ≥ 0.47; Table 5). At 30 DAT, rotation 2 had greater canopy cover (86%) compared with rotation 1 (66%). In rotation 1, canopy cover at 30 DAT differed due to both mulch and planting time (P = 0.01 and P < 0.0001, respectively), but there was no interaction between mulch and planting time (P = 0.33). For spring-transplanted lettuce in rotation 1, canopy cover was 56% on average for mulched plots at 30 DAT and was greater than the bare ground control (30%) (P = 0.002; Table 6). For summer-transplanted lettuce in rotation 1, the overall average canopy cover was 83% at 30 DAT for all treatments (range 76–88%) and did not differ due to mulch (P = 0.66). Similarly, in rotation 2 at 30 DAT, canopy cover was 86% on average for all treatments (range 83–87%) and did not differ due to mulch (P = 0.76).
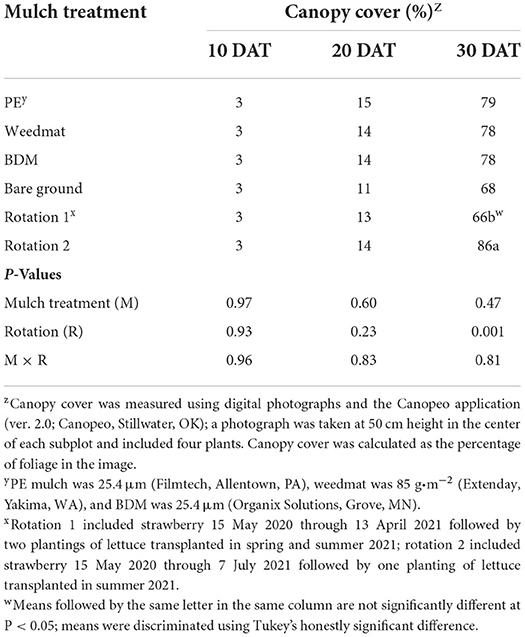
Table 5. Canopy cover (%) of lettuce cv. Green Star grown on raised beds with polyethylene (PE), soil-biodegradable mulch (BDM), weedmat, or bare ground treatments in two different rotation schemes following strawberry in a Mediterranean climate, 2021.
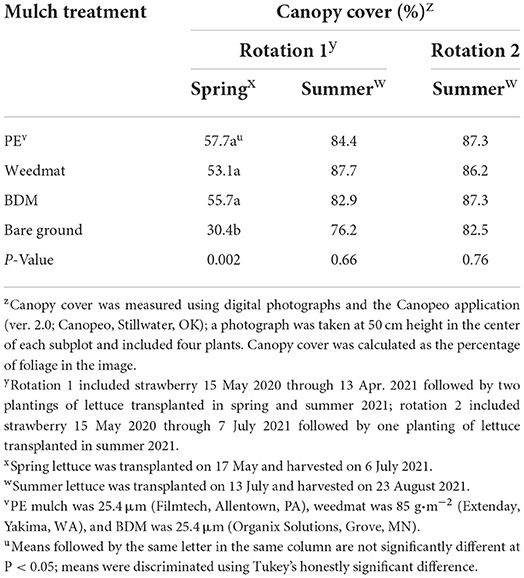
Table 6. Canopy cover (%) at 30 days after transplanting (DAT) of lettuce cv. Green Star grown on a raised bed with polyethylene (PE), weedmat, soil-biodegradable plastic mulch (BDM), or bare ground treatments in two different rotation schemes and two planting times (spring and summer) in a Mediterranean climate, 2021.
Lettuce yield
Days to harvest did not differ due to mulch (P = 0.07) but differed due to rotation (P = 0.005); there was no interaction between mulch and rotation (P = 0.30; Table 7). Average days to harvest was 43 days, but lettuce was harvested earlier in rotation 2 (42 days) compared with rotation 1 (44 days). Average head weight, length, and diameter did not differ due to either mulch (P ≥ 0.15 for all) or rotation (P ≥ 0.27 for all) nor was there an interaction between mulch and rotation (P ≥ 0.16 for all). Overall, average head weight, length, and diameter were 0.4 kg, 21.5 cm, and 19.5 cm, respectively. In rotation 1, days to harvest differed due to both mulch and planting time, and there was an interaction between them (P < 0.0001 for all; Table 8). In summer, all the treatments were harvested at 41 DAT while the bare ground control in spring was harvested at the latest (at 50 DAT). There were no differences in average head weight, head length, and head diameter due to mulch (P ≥ 0.08 for all) or planting time (P ≥ 0.11 for all), nor were there interactions between mulch and planting time (P ≥ 0.15 for all). Average head weight was 0.4 kg, and average head length and diameter were both 20 cm.
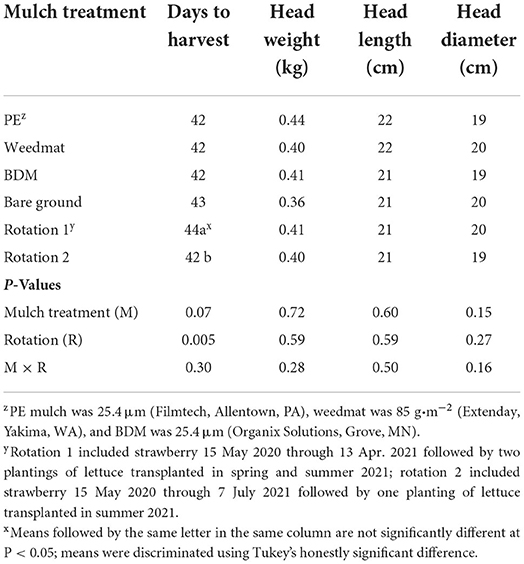
Table 7. Days to harvest, mean head weight (kg), length (cm), and diameter (cm) of lettuce cv. Green Star grown on a raised bed with polyethylene (PE), weedmat, soil-biodegradable plastic mulch (BDM), or bare ground treatments in two different rotation schemes following strawberry in a Mediterranean climate, 2021.
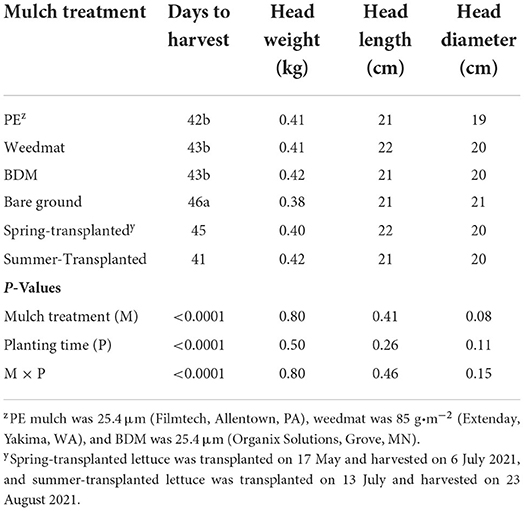
Table 8. Mean head weight (kg), length (cm), and diameter (cm) of lettuce cv. Green Star grown on a raised bed with polyethylene (PE), weedmat, soil-biodegradable plastic mulch (BDM), or bare ground treatments in two lettuce planting time (spring and summer) of rotation 1 following strawberry in a Mediterranean climate, 2021.
Other mulch data results
Soil temperature and moisture
During May to July 2020, average soil temperatures in the PE and BDM treatments were ~1.5°C higher than the weedmat treatment and ~2.5°C higher than bare ground control (see Supplementary material). During the lettuce growing season (May to September 2021), average soil temperatures were similar among the PE, BDM, and bare ground treatments and varied only by 0.1°C, while the weedmat treatment had relatively lower soil temperatures than the other treatments. Soil under weedmat had the highest average soil moisture content across all mulch treatments between May 2020 and Sept. 2021 (averaging 0.35 m3·m−3), while PE, BDM, and bare ground treatments had relatively similar soil moisture contents (range: 0.22–0.35 m3·m−3).
Weed dry biomass
Between May 2020 and September 2021, weed dry biomass differed due to mulch treatment (P < 0.001), rotation (P = 0.01), and DAP (P < 0.001). A rotation effect was only observed at 110 DAP. During the 2-year data collection period, there were minimal weeds across PE, BDM, and weedmat treatments whereas the bare ground control had the greatest weed dry biomass (Figure 1). By the end of summer 2021, cumulative weed dry biomass was greatest in the bare ground control (91 g·m−2 averaged between the rotations) and lowest in PE and BDM treatments (≈1 to 2 g·m−2) and weedmat (5 g·m−2). Although not statistically significant, the weedmat treatment averaged 3.6 g·m−2 greater weed dry biomass than the PE and BDM by the end of summer 2021. During the strawberry growing season in 2020, monthly weed dry biomass differed due to mulch treatment at 60 and 110 DAP. For the summer-lettuce growing season (417–480 DAP), monthly weed dry biomass differed at 60, 110, 417, 453, and 480 DAP due to mulch treatment.
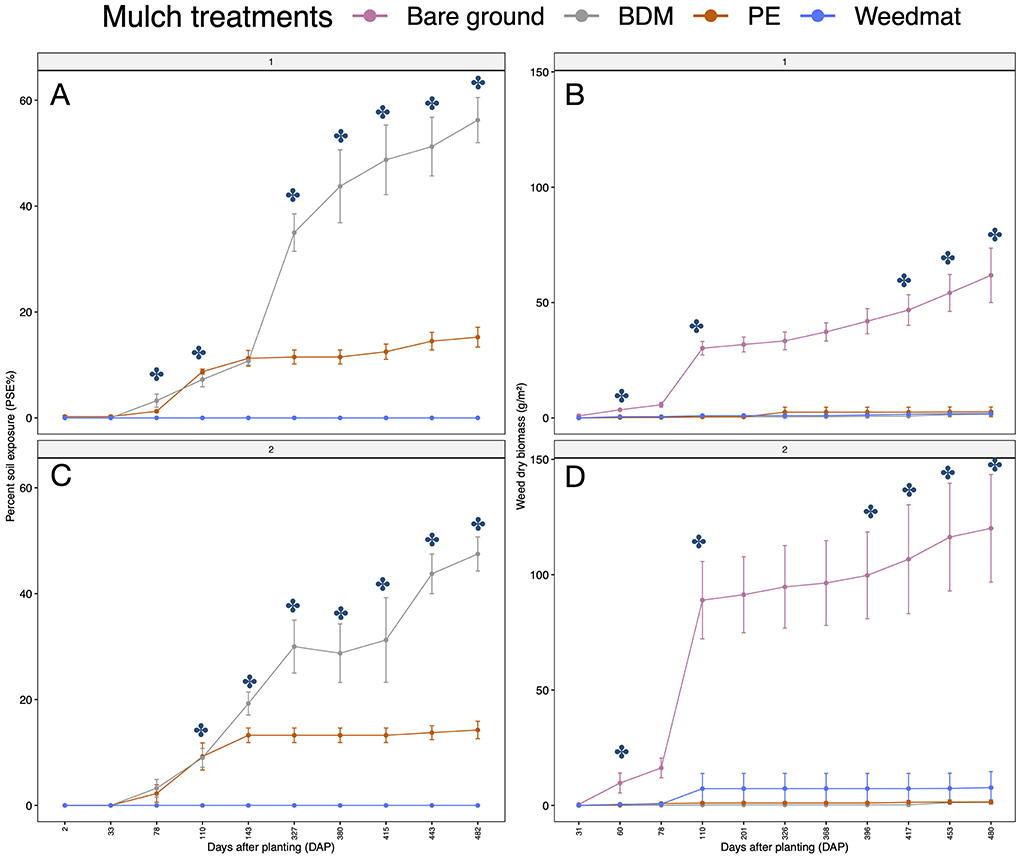
Figure 1. Cumulative weed dry biomass (g·m−2) per m2 and percent soil exposure (PSE, %) in plots with polyethylene (PE), soil-biodegradable mulch (BDM), weedmat, or bare ground treatments in a Mediterranean climate between May 2020 and Sept. 2021. means weed dry biomass or PSE differed due to mulch treatment (P < 0.05). (A,B) represent rotation 1 data, (C,D) rotation 2 data. PSE was not recorded in bare ground plots.
Mulch performance
Between May 2020 and September 2021, PSE differed due to mulch treatment (P < 0.001), rotation (P < 0.001), and DAP (P < 0.001). From 2 to 78 DAP, there was no effect of mulch or rotation nor an interaction of these two factors on PSE (Figure 1). From 93 to 327 DAP, PSE differed at 93, 110, 131, 143, 208, and 327 DAP due to mulch treatment but did not differ due to rotation nor was there an interaction between the two factors. In winter 2020 (110–327 DAP), PSE in the BDM treatment was greater (25% increase) compared to PE (3% increase). During the 2021 spring-lettuce and strawberry double-cropping season (365–415 DAP), PSE differed at 365, 380, 394, and 415 DAP due to mulch treatment and rotation, and there was an interaction between mulch treatment and rotation. In the fall-lettuce growing season (429–496 DAP), PSE differed at 429, 443, 468, 482, and 496 DAP due to mulch treatment, but did not differ due to either rotation nor was there an interaction between these two factors. By the end of summer 2021 (21 September), PSE in the weedmat treatment was 0% (no deterioration observed), whereas PSE was the greatest in BDM plots (59%) and intermediate for PE mulches (18%). Regardless of rotation, weed dry biomass and PSE had no correlation (P = 0.08), but if considering rotation separately, weed dry biomass and PSE were correlated in rotation 2 (P = 0.02) with a correlation coefficient of −0.21. There was no correlation observed in rotation 1 (P = 0.57).
Discussion
Double-cropping strawberry and lettuce with BDM is a promising alternative for growers in a Mediterranean climate to diversify their crop type and with potential economic benefits by increasing mulch lifespan. PE and weedmat mulches provide similar horticultural benefits as BDM, but lack biodegradability and can contribute to plastic waste generation. Due to the increased soil temperature and weed suppression provided by plastic mulches (data not shown), PE, BDM, and weedmat promoted strawberry plant growth compared to the bare ground control. DeVetter et al. (2017) similarly observed plastic mulches promoted day-neutral strawberry plant growth in northwestern Washington as measured by increased crown and leaf biomass compared to the bare ground control. The PE and BDM treatments also had equivalent plant growth effects that were greater than bare ground cultivation in a sweet corn study in northwestern Washington (Ghimire et al., 2020). In our experiment, mulches only increased canopy cover within the first year but not the second. This suggests mulching with PE, BDM, and weedmat promotes growth and establishment of establishing plants and the effects diminish as plants mature.
Total and marketable yields of strawberry were correspondingly greater from plants grown in PE, BDM, and weedmat treated plots and were almost twice as great as the bare ground control after the first cropping season. Although strawberry harvesting only occurred in rotation 2 in the second year of the study, total and marketable yields were nearly double than those in 2020 due to increased crop establishment. In wheat, soybean, and corn systems, double cropping can also increase the yield stability (Grover et al., 2009; Caviglia et al., 2011; Graß et al., 2013). Our results indicate double-cropping strawberry with lettuce in plasticulture also provides yield benefits relative to cultivating strawberry for a single year.
Our findings show TA declined at mid-harvest but increased at late harvest in both years. The TSS and pH showed similar fluctuations, indicating that fruit juice chemistry changes were due to environmental factors. Strawberry fruit development and ripening are a highly regulated process controlled by several quantitative trait loci (QTLs), leading to biochemical and physiological transformations in the nutritional and organoleptic quality traits of ripe fruits (Fait et al., 2008; Zorrilla-Fontanesi et al., 2011; Cervantes et al., 2020). For example, loci sscII-F.1 is strongly influenced by environmental effects, and factors such as temperature, light, and relative humidity can impact strawberry fruit quality (Zorrilla-Fontanesi et al., 2011; Cervantes et al., 2020).
Mulching may impact strawberry fruit quality due to the quantity and quality of light being reflected into the plant canopy (Costa et al., 2014; DeVetter et al., 2017), which may lead to lower TSS in fruits harvested from non-mulched plants (DeVetter et al., 2017). However, this differs from our experiment where TSS did not differ due to mulch treatment. Although weedmat had statistically higher juice pH, the average difference is approximately 0.1 pH unit, which is very slight and may not be commercially meaningful. The PE and BDM treatments had similar TSS, pH, and TA in both years, indicating fruit quality would be unchanged if growers converted from PE to BDM, which is similar to previous observations (Costa et al., 2014; DeVetter et al., 2017).
Mulching increased strawberry canopy growth during the first year relative to the bare ground control, but this effect was lost in the second year. This might be attributed to similar soil temperature conditions in 2021 under PE mulch, BDM, weedmat, and the bare ground control during both spring and summer growing seasons (an explanation for a lack of these differences is provided below). In contrast, Verdial et al. (2001) reported increased soil temperature with black PE mulch (25.1°C) compared to non-mulched bare ground (23.6°C) in “Lucy Brown” iceberg lettuce. Similarly, black PE mulch increased soil temperature by 3.5°C compared to non-mulched bare ground when “Ithaca” crisphead lettuce was grown in Quebec, Canada (Jenni et al., 2004). However, for lettuce, the canopy cover in rotation 2 at 30 DAT was greater by 30% compared to rotation 1. As rotation 1 includes both spring- and summer-transplanted lettuce and rotation 2 includes only summer-transplanted lettuce, the rotation effect is more pronounced due to the planting time of lettuce. Average air temperature was greater by 1.7°C during the summer compared to the spring growing season and likely resulted in greater canopy cover of summer-transplanted lettuce in both rotations.
PE mulch, BDM, and weedmat did not increase soil temperature compared to bare ground control and hence did not result in differences in days to harvest, yield, and quality of lettuce. However, rotation 2 had an earlier harvest as this rotation included only summer-transplanted lettuce. Greater air temperature during summer should have resulted in earlier harvest in both rotations. In rotation 1, mulch treatments expedited the days to harvest by 3 days despite similar soil temperature conditions under the mulch treatments and bare ground control. The lack of differences in lettuce weight, length, and diameter between mulch treatments and the bare ground control was due to different harvest days that allowed the plants to attain their full maturity.
Black plastic mulches can raise soil temperatures by absorbing solar radiation and transferring heat from the mulch to the soil (Lamont, 1993). Similar to this study, a 2°C soil temperature gain was also observed when strawberry was mulched with PE and BDMs compared to bare ground cultivation in northwestern Washington (DeVetter et al., 2017). During winter 2020, mulching did not increase soil temperatures because solar radiation was greatly reduced (55 watt·m−2 in August 2020 whereas 7 watt·m−2 in December 2020; Washington State University, 2020), which demonstrates black plastic mulches have restricted conditions in which they can provide soil warming effects. However, the reduced temperature gain in winter is not likely to be a limitation unless crops are actively growing and being cultivated. Interestingly during the lettuce growing season in 2021, PE and BDM mulches did not raise soil temperatures. This contrasts with other research studies. For example, Nair and Havlovic (2013) found black plastic non-biodegradable mulch (feedstock was not specified) increased soil temperature by 1.5°C at a depth of 10 cm when compared to bare ground cultivation in a high-tunnel lettuce production system in midwestern US, and Lamont (1993) found black PE mulches used in vegetable production can elevate daytime soil temperatures at a depth of 5 cm by 2.8°C compared to bare ground cultivation. Lack of soil temperature gains in the second year may be due to mulch tears and deterioration around the planting holes and/or disturbances to the sensors through the action of crop removal and replanting. Due to its thick but semipermeable structure, weedmat did not elevate soil temperature as much as PE or BDM, which may indicate weedmat could be suitable in climates where high soil temperatures limit crop production.
Plastic mulches can also reduce soil-water evaporation relative to uncovered soil by acting as a physical barrier (Hanlon and Hochmuth, 1989; Snyder et al., 2015). However, PE and BDM treatments did not show increased soil moisture content relative to the bare ground treatment in this experiment. This observation may be because PE and BDMs impeded the percolation of rainwater and/or because irrigation was sufficiently high enough that there were no differences among these treatments. In contrast, higher soil moisture content under weedmat was likely due to its semipermeable structure that allowed rainwater to percolate through and contribute to soil moisture levels (Strik and Davis, 2020). The density of weedmat could also limit soil-water evaporation despite its semipermeable design. Higher soil moisture content likely contributed to reduced soil temperatures under weedmat, as increasing moisture content increases the specific heat of soil (Heitman et al., 2008; Zhang et al., 2021). Other studies in Portugal and Washington found soil under PE and BDM had similar soil moisture levels in pumpkin and strawberry fields, respectively (Costa et al., 2014; Saglam et al., 2017). Interestingly, BDMs were observed to have 100–250 times higher vapor permeability than PE mulches (Bilck et al., 2010; Touchaleaume et al., 2016), so soil moisture content under BDM is likely to be lower than under PE mulch due to greater soil-water evaporation potential. However, results can be conflicting. A similar experiment led by Ghimire et al. (2020) using sweet corn and within 1 km of our experiment found BDM made using PBAT+PLA feedstocks (i.e., the same feedstock as our BDM) generated soil moisture content levels 20% greater than PE and the bare ground treatments in the first year of their study. In contrast, soil moisture under PE was 22% greater than BDM in the second year of their experiment (Ghimire et al., 2020). These results suggest effects on soil temperature are more consistent than soil moisture, but both are subject to year-to-year variation due to precipitation and temperature differences. Irrigation practices may also influence soil moisture dynamics in mulched systems. Soil moisture and temperature can also be subject to variation in sensor calibration and placement in the soil relative to irrigation emitters, but this is unlikely in our experiment given the attention given to installation at the onset of the experiment.
Results showed mulching suppresses weed growth during the growing season. The bare ground treatment had more weeds, while the PE, BDM, and weedmat treatments had equivalent weed suppression. No rotation effect was observed during the strawberry–lettuce double-cropping season (326 to 396 DAP), which indicates weed growth was not impacted by crop type. Although BDM deteriorated over time (Figure 1), the mulch was still effective at weed suppression and performed similarly to non-degradable mulches over the study period. Other studies also observed PE and BDM had equivalent weed suppression although BDM had greater deterioration in pie pumpkin, sweet corn, and day-neutral strawberry production in northwestern Washington (DeVetter et al., 2017; Ghimire et al., 2018, 2020). However, not all BDMs have achieved the same level of weed suppression as PE mulches. Tofanelli and Wortman (2020) reported that BDM color impacts weed management by influencing the quantity and quality of light transmitted through the mulch. Black BDM is often more effective than white or clear BDM at weed suppression depending on climate (Ngouajio et al., 2008; Ghimire et al., 2020).
Although BDMs deteriorate over time, they can still achieve comparable benefits as PE for weed control, soil temperate and moisture optimization, and plant growth and yield promotion (Tofanelli and Wortman, 2020). This assessment of performance usually pertains to annual crop production, so this study is one of the few that looks at BDM performance and deterioration over 2 years of consecutive mulch use. Based on the observations of this study, strawberry and lettuce crop growth had different impacts on BDM deterioration because PSE data only showed a rotation effect and an interaction between mulch treatment and rotation during the 2021 spring-lettuce and strawberry double-cropping season (365 to 415 DAP). However, it is difficult to conclude which crop had a larger impact on deterioration because before the second growing season BDM in each rotation differed by 10%. The BDM deterioration rate can be influenced by mulch formulation and thickness, with thinner and starched-based BDMs deteriorating faster than PBAT + PLA BDMs (Zhang et al., 2020a). Miles et al. (2012) found BDM exposed in open fields deteriorated more than when in a high-tunnel environment regardless of mulch formulation and thickness, demonstrating that environmental conditions influence deterioration rates. Weather circumstances such as strong winds, heavy rains, solar radiation, and winter conditions can enhance the rate of BDM deterioration, and this could be a problem in some overwintering planting systems such as late summer-planted floricane-fruiting raspberry (Rubus idaeus L.) (Zhang et al., 2020b). On-farm activities can also accelerate BDM deterioration due to its lower tensile strength and mechanical resistance compared with non-biodegradable plastic mulches like PE (Zhang et al., 2020b). Weedmat is a woven fabric that does not deteriorate unless it has been cut or pierced. In this 2-year strawberry and lettuce double-cropping system, PE and BDM were deteriorating, which suggests these mulches could be used for up to two seasons for crop production in a Mediterranean climate comparable to where the study was conducted so long as farmers find the aesthetics of deteriorating mulch acceptable (Dentzman and Goldberger, 2020). Weedmat and possibly thicker PE than what was used in this study can be used for more than two growing seasons with no great increase in PSE.
The economic implications of using these alternative mulch technologies still need to be considered. Unfortunately, a detailed cost–benefit analysis that compares BDM, PE, and weedmat is not available. However, it has been estimated that the initial purchase cost of BDM is approximately double the cost of PE mulch (Galinato et al., 2020). Yet, because BDMs do not need to be physically removed and disposed of, the estimated total net change in profit due to BDM adoption compared to PE mulch is $189·hectare−1 (Galinato et al., 2020). Therefore, BDM may be more economical than PE mulch if crop yield and quality are maintained or increased. Regarding weedmat, this mulch may last 5–7 years (Strik and Davis, 2021) and could be reapplied if the material stays intact and a grower has the capability to efficiently remove and redeploy. Weedmat, however, is 10–13 times more expensive than PE mulch based on regional prices, and it would still need to be disposed of after its useful life. In a 3-year raspberry study in Washington, the total cost of weedmat plus handweeding was four times more expensive than PE mulch (DeVetter et al., 2022). Therefore, existing economic information combined with the results in this study suggests double cropping with BDM provides more opportunity to increase profitability compared to PE and weedmat.
In conclusion, our results confirmed the hypotheses that PE, BDM, and weedmat treatments suppress weed growth, modify soil temperature, moderate soil moisture, and promote plant growth and yields compared to a bare ground/non-mulched control in a novel strawberry and lettuce double-cropping system in a Mediterranean climate. These results indicate BDM and weedmat can be alternatives to conventional PE in strawberry and lettuce single- and double-cropped production systems, although cost–benefits of these mulch technologies need to be accounted for. Although BDM deteriorated and averaged 59% PSE by the end of the second year, they produced equivalent or greater crop yields and achieved the same crop quality as PE and weedmat. Soil under weedmat was relatively cooler in temperature but had greater soil moisture, which could be beneficial in warmer climates where water conservation is important. Overall, our results show crop yield and quality can be maintained in a strawberry–lettuce double-cropping system with mulch used for two growing seasons. Furthermore, plasticulture used in a double-cropping system that prolongs mulch use contributes to reduced plastic waste generation, especially when using BDMs that are designed to degrade in soils. Our strawberry yield data show double-cropping strawberry with lettuce is promising and could be an alternative production cycle allowing farmers to harvest two different crops over the course of 2 years.
Data availability statement
The original contributions presented in the study are included in the article/Supplementary material, further inquiries can be directed to the corresponding author.
Author contributions
LD, CM, and LT conceived and designed the experiments. HZ initially established the field trial. XW collected the strawberry, mulch data, and conducted those data analyses. SS collected the lettuce data and conducted lettuce data analysis. XW and SS wrote the original draft. LT, HZ, CM, and LD edited. All authors contributed to the article and approved the submitted version.
Funding
Funding was provided by the Washington State Department of Agriculture (WSDA) Specialty Crop Block Grant Program (project #K2863) and also Hatch projects 1014919 and 1017286.
Acknowledgments
We are thankful to Charlie Gunderson at Norcal Plant Company for the provision of strawberry plants and Emma Rogers, Brenda Madrid, and Rachel Schick for their tremendous help in plot maintenance and crop harvests.
Conflict of interest
The authors declare that the research was conducted in the absence of any commercial or financial relationships that could be construed as a potential conflict of interest.
Publisher's note
All claims expressed in this article are solely those of the authors and do not necessarily represent those of their affiliated organizations, or those of the publisher, the editors and the reviewers. Any product that may be evaluated in this article, or claim that may be made by its manufacturer, is not guaranteed or endorsed by the publisher.
Supplementary material
The Supplementary Material for this article can be found online at: https://www.frontiersin.org/articles/10.3389/fsufs.2022.942645/full#supplementary-material
Abbreviations
BDM, soil-biodegradable plastic mulch; PE, polyethylene; TSS, total soluble solids; TA, titratable acidity; PSE, percent soil exposure; DAP, days after planting (for strawberry data); DAT, days after transplanting (for lettuce data).
References
Albregts, E. E., and Howard, C. M. (1985). Double cropping strawberries with vegetables. Proc. Fla. State Hort. Soc. 98, 299–301.
American Society for Testing and Materials (2018). Standard Test Method for Determining Aerobic Biodegradation of Plastic Materials in Soil. ASTM-D5988-18. West Conshohocken, PA: ASTM International.
Beck, H. E., Zimmermann, N. E., McVicar, T. R., Vergopolan, N., and Berg, A. (2018). Present and future Köppen-Geiger climate classification maps at 1-km resolution. Sci. Data 5, 180214. doi: 10.1038/sdata.2018.214
Bilck, A. P., Grossmann, M. V. E., and Yamashita, F. (2010). Biodegradable mulch films for strawberry production. Polym. Test. 29, 471–476. doi: 10.1016/j.polymertesting.2010.02.007
Caviglia, O. P., Sadras, V. O., and Andrade, F. H. (2011). Yield and quality of wheat and soybean in sole- and double-cropping. Agron. J. 103, 1081–1089. doi: 10.2134/agronj2011.0019
Cervantes, L., Ariza, M. T., Miranda, L., Lozano, D., Medina, J. J., Soria, C., et al. (2020). Stability of fruit quality traits of different strawberry varieties under variable environmental conditions. Agronomy 10, 1242. doi: 10.3390/agronomy10091242
Costa, R., Saraiva, A., Carvalho, L., and Duarte, E. (2014). The use of biodegradable mulch films on strawberry crop in Portugal. Sci. Hortic. 173, 65–70. doi: 10.1016/j.scienta.2014.04.020
Cowan, J. S., Miles, C. A., Andrews, P. K., and Inglis, D. A. (2014). Biodegradable mulch performed comparable to polyethylene in high tunnel tomato (Solanum lycopersicum L.) production. J. Sci. Food Agric. 94, 1854–1864. doi: 10.1002/jsfa.6504
Dentzman, K., and Goldberger, J. R. (2020). Plastic scraps: biodegradable mulch films and the aesthetics of ‘good farming' in US specialty crop production. Agric. Hum. Values 37, 83–96. doi: 10.1007/s10460-019-09970-x
DeVetter, L. W., Shrestha, S., and Hayes, D. (2021). Plastic Mulch Use and Management: What is a Soil-Biodegradable Plastic Mulch Composed of. Washington State University Extension Factsheet. Available online at: https://s3.wp.wsu.edu/uploads/sites/2181/2021/07/What-is-in-a-BDM.pdf (accessed June 9, 2022).
DeVetter, L. W., Zhang, H., Ghimire, S., Watkinson, S., and Miles, C. A. (2017). Plastic biodegradable mulches reduce weeds and promote crop growth in day-neutral strawberry in western Washington. HortScience 52, 1700–1706. doi: 10.21273/HORTSCI12422-17
DeVetter, L. W., Zhang, H., Miles, C., and Benedict, C. (2022). Assessing New Mulch Technologies for Washington Raspberry. Washington State University Extension Whatcom Ag Monthly Newsletter. Available online at: https://extension.wsu.edu/wam/assessing-new-mulch-technologies-for-washington-raspberry/?utm_source=Whatcom+Ag+Monthly&utm_campaign=a1777a1834-Whatcom+AgMonthly+September+2017_COPY_01&utm_medium=email&utm_term=0_4f449891a1-a1777a1834-90618097 (accessed June 9, 2022).
Elobeid, A., Moreira, M. M., de Lima, C. Z., Carriquiry, M., and Harfuch, L. (2019). Implications of biofuel production on direct and indirect land use change: evidence from Brazil. Biofuels Bioenergy Food Secur. 7, 128–130. doi: 10.1016/B978-0-12-803954-0.00007-3
European Norms (2018). Biodegradable Mulch Films for Use in Agriculture and Horticulture – Requirements and Test Methods. EN-17033. Brussels: European Standard, European Committee for Standardization.
Fait, A., Hanhineva, K., Beleggia, R., Dai, N., Rogachev, I., Nikiforova, V. J., et al. (2008). Reconfiguration of the achene and receptacle metabolic networks during strawberry fruit development. Plant Physiol. 148, 730–750. doi: 10.1104/pp.108.120691
Fernandez, G. E., Butler, L. M., and Louws, F. J. (2001). Strawberry growth and development in an annual plasticulture system. HortScience 36, 1219–1223. doi: 10.21273/HORTSCI.36.7.1219
Food Agriculture Organization of the United States (2020). FAOSTAT: Strawberries. Food and Agriculture Organization of the United States. Available online at: http://www.fao.org/faostate/en/#search/strawberries (accessed April 26, 2022).
Freeman, S., and Gnayem, N. (2005). Use of plasticulture for strawberry plant production. Small Fruits Rev. 4, 21–32. doi: 10.1300/J301v04n01_04
Galinato, S. P., and Miles, C. A. (2012). 2011 Cost Estimates of Producing Fresh Market Field-Grown Head Lettuce in Western Washington. Washington State University Extension Publication FS018E. Available online at: http://ses.wsu.edu/enterprise_budgets/ (accessed April 26, 2022).
Galinato, S. P., Velandia, M., and Ghimire, S. (2020). Economic Feasibility of Using Alternative Plastic Mulches: A Pumpkin Case Study in Western WA. Washington State University Extension. TB68E. Available online at: https://pubs.extension.wsu.edu/economic-feasibility-of-using-alternative-plastic-mulches-a-pumpkin-case-study-in-western-washington (accessed May 29, 2022).
Galinato, S. P., and Walters, T. (2012). FS093E Appendix. 2011 Cost Estimates of Producing Fresh Market Field-Grown Strawberry in Washington. Available online at: http://ses.wsu.edu/enterprise_budgets/ (accessed April 26, 2022).
Garwood, T. (1998). An Economic Analysis of Matted Row, Plasticulture, and Greenhouse Production Systems in North Carolina. (Master's thesis), North Carolina State University, Raleigh, NC (United States).
Ghimire, S., Scheenstra, E., and Miles, C. A. (2020). Soil-biodegradable mulches for growth, yield and quality of sweet corn in a Mediterranean-type climate. HortScience 55, 317–325. doi: 10.21273/HORTSCI14667-19
Ghimire, S., Wszelaki, A. L., Moore, J. C., Inglis, D. A., and Miles, C. A. (2018). The use of biodegradable mulches in pie pumpkin crop production in two diverse climates. HortScience 53, 288–294. doi: 10.21273/HORTSCI12630-17
Graß, R., Heuser, F., Stülpnagel, R., Piepho, H., and Wachendorf, M. (2013). Energy crop production in double-cropping systems: Results from an experiment at seven sites. Europ. J. Agron. 51, 120–129. doi: 10.1016/j.eja.2013.08.004
Grover, K.K., Karsten, H. D., and Roth, G. W. (2009). Corn grain yields and yield stability in four long-term cropping systems. Agron. J. 101, 940–946. doi: 10.2134/agronj2008.0221x
Hanlon, E. A., and Hochmuth, G. J. (1989). “Fertilizer recommendations for vegetables grown in polyethylene mulch,” in Proceedings of the National Agricultural Plastics Congress (St. Augustine, FL), 165–171.
Heggenstaller, A. H., Anex, R. P., Liebman, M., Sundberg, D. N., and Gibson, L. R. (2008). Productivity and nutrient dynamics in bioenergy double-cropping systems. Agron. J. 100, 1740–1748. doi: 10.2134/agronj2008.0087
Heitman, J. L., Xiao, X., Horton, R., and Sauer, T. J. (2008). Sensible heat measurements indicating depth and magnitude of subsurface soil water evaporation. Water Resour. Res. 44, W00D05. doi: 10.1029/2008WR006961
Hoashi-Erhardt, W., and Walters, T. (2014). Growing Day-Neutral Strawberries in Western Washington. Washington State University Extension Factsheet FS132E. Available online at: http://pubs.cahnrs.wsu.edu/publications/wp-content/uploads/sites/2/publications/FS132E.pdf (accessed April 26, 2022).
Index Box Platform (2022). World-Lettuce and Chicory-Market Analysis, Forecast, Size, Trends and Insights Update: COVID-19 Impact. Index Box Platform. Available online at: https://www.indexbox.io/store/world-lettuce-and-chicory-market-report-analysis-and-forecast-to-2020/ (accessed April 26, 2022).
Jenni, S., Brault, D., and Stewart, K. A. (2004). Degradable mulch as an alternative for weed control in lettuce produced on organic soils. Acta Hort. 638, 111–118. doi: 10.17660/ActaHortic.2004.638.13
Julian, J. W., Strik, B. C., Larco, H. O., Bryla, D.R., and Sullivan, D. M. (2012). Costs of establishing organic northern highbush blueberry: impacts of planting method, fertilization, and mulch type. HortScience 47, 866–873. doi: 10.21273/HORTSCI.47.7.866
Khemani, K., and Scholz, C. (2012). “Degradable polymers and materials: principles and practice,” in ACS Symposium Series, 1114, 2nd edn (Washington, DC: Oxford University Press).
Kramer, J., Skyler, S., and Linda, C. (2021). Fruit and Tree Nuts Outlook: March 2021. U.S. Department of Agriculture, Economic Research Service. FTS-372. Available online at: https://www.ers.usda.gov/webdocs/outlooks/100827/fts-372.pdf?v=7685.4 (accessed April 26, 2022).
Lamont, W. J. (1993). Plastic mulches for the production of vegetable crops. HortTechnology 3, 35–39. doi: 10.21273/HORTTECH.3.1.35
Lamont, W. J. (1996). What are the components of a plasticulture vegetable system. HortTechnology 6, 150–154. doi: 10.21273/HORTTECH.6.3.150
Lamont, W. J., and Poling, E. B. (1986). A fresh way of looking for profits: double-cropping strawberries, muskmelons. Fruit South 7, 8–11.
Miles, C. A., DeVetter, L., and Ghimire, S. (2017). Suitability of biodegradable plastic mulches for organic and sustainable agricultural production systems. HortScience 52, 10–15. doi: 10.21273/HORTSCI11249-16
Miles, C. A., Wallace, R., Wszelaki, A., Martin, J., Cowan, J., Walters, T., et al. (2012). Deterioration of potentially biodegradable alternatives to black plastic mulch in three tomato production regions. HortScience 47, 1270–1277. doi: 10.21273/HORTSCI.47.9.1270
Mou, B. (2008). “Lettuce,” in Handbook of Plant Breeding. Vegetables I. Asteraceae, Brassicaceae, Chenopodiaceae, and Cucurbitaceae, eds J. Prohens, and F. Nuez (New York, NY: Springer), 75–116. doi: 10.1007/978-0-387-30443-4_3
Nair, A., and Havlovic, B. J. (2013). Mulch and Row Cover Affect Lettuce Production in High Tunnels. Iowa State Research Farm Progress Reports. ISRF 12-12. Available online at: http://lib.dr.iastate.edu/farms_reports/1864 (accessed April 26, 2022).
Ngouajio, M., Auras, R., Fernandez, R. T., Rubino, M., Counts, J. W., and Kijchavengkul, T. (2008). Field performance of aliphatic-aromatic copolyester biodegradable mulch films in a fresh market tomato production system. HortTechnology 18, 605–610. doi: 10.21273/HORTTECH.18.4.605
Oregon State University (2010). Vegetable Production Guides: Lettuce. Oregon State University Extension. Available online at: https://horticulture.oregonstate.edu/oregon-vegetables/lettuce-0 (accessed May 28, 2022).
Patrignani, A., and Ochsner, T. (2015). Canopeo: a powerful new tool for measuring fractional green canopy cover. Agron. J. 107, 2312–2320. doi: 10.2134/agronj15.0150
Reidsma, P., and Ewert, F. (2008). Regional farm diversity can reduce vulnerability of food production to climate change. Ecology 13, 1–16. doi: 10.5751/ES-02476-130138
Saglam, M., Sintim, H. Y., Bary, A., Miles, C., Ghimire, S., Inglis, D., et al. (2017). Modeling the effect of biodegradable paper and plastic mulch on soil moisture dynamics. Agricult. Water Manage. 193, 240–250. doi: 10.1016/j.agwat.2017.08.011
Samtani, J. B., Rom, C. R., Friedrich, H., Fennimore, S. A., Finn, C. E., Petran, A., et al. (2019). The status and future of the strawberry industry in the United States. HortTechnology 29,11–24. doi: 10.21273/HORTTECH04135-18
Shah, F., and Wu, W. (2020). Use of plastic mulch in agriculture and strategies to mitigate the associated environmental concerns. Adv. Agron. 164, 253. doi: 10.1016/bs.agron.2020.06.005
Snyder, K., Grant, A., Murray, C., and Wolff, B. (2015). The effects of plastic mulch systems on soil temperature and moisture in central Ontario. HortTechnology 25, 162–170. doi: 10.21273/HORTTECH.25.2.162
Strik, B. C., and Davis, A. J. (2020). Individual and combined use of sawdust and weed mat mulch in a new planting of northern highbush blueberry I. Impacts on plant growth and soil and canopy temperature. HortScience 55, 1280–1287. doi: 10.21273/HORTSCI15122-20
Strik, B. C., and Davis, A. J. (2021). Individual and combined use of sawdust and weed mat mulch in a new planting of northern highbush blueberry III. Yield, fruit quality and costs. HortScience 56, 363–367. doi: 10.21273/HORTSCI15659-20
Strik, B. C., and Vance, A. J. (2017). Weed management strategies in long-term organic blueberry production systems–impact of mulch type and weed control methods on economics. Acta Hort. 1180, 347–352. doi: 10.17660/ActaHortic.2017.1180.47
Tofanelli, M. B. D., and Wortman, S. E. (2020). Benchmarking the agronomic performance of biodegradable mulches against polyethylene mulch film: a meta-analysis. Agronomy 10, 1618. doi: 10.3390/agronomy10101618
Touchaleaume, F., Martin-Closas, L., Angellier-Coussy, H., Chevillard, A., Cesar, G., Gontard, N., et al. (2016). Performance and environmental impact of biodegradable polymers as agricultural mulching films. Chemosphere 144, 433–439. doi: 10.1016/j.chemosphere.2015.09.006
Vanden Heuvel, J. E., and Lewers, K. (2008). Introduction to workshop: Breeding, growing, and marketing of repeat-fruiting small fruit cultivars for an extended season. HortScience 43, 1634. doi: 10.21273/HORTSCI.43.6.1634
Verdejo-Lucas, S., Sorribas, F. J., Ornat, C., and Galeano, M. (2003). Evaluating Pochonia chlamydosporia in a double-cropping system of lettuce and tomato in plastic houses infested with Meloidogyne javanica. Plant Pathol. 52, 521–528. doi: 10.1046/j.1365-3059.2003.00873.x
Verdial, M. F., de Lima, M. S., Morgor, A. F., and Goto, R. (2001). Production of iceberg lettuce using mulches. Sci. Agric. 58, 737–740. doi: 10.1590/S0103-90162001000400014
Wallace, R. W., Wszelaki, A. L., Miles, C. A., Cowan, J. S., Martin, J., Roozen, J., et al. (2012). Lettuce yield and quality when grown in high tunnel and open-field production systems under three diverse climates. HortTechnology 22, 659–668. doi: 10.21273/HORTTECH.22.5.659
Washington State University (2020). AgWeatherNet. Mount Vernon, WA. Available online at: http://weather.wsu.edu/ (accessed April 26, 2022).
Waterer, D., Hrycan, W., and Simms, T. (2008). Potential to double-crop plastic mulch. Can. J. Plant Sci. 88, 187–193. doi: 10.4141/CJPS07007
Whitaker, T. W., Ryder, E. J., and Hills, O. A. (1962). Lettuce and Its Production, Vol. 221. Washington, DC: United States Department of Agriculture Handbook.
Zhang, H., DeVetter, L. W., Scheenstra, E., and Miles, C. A. (2020a). Weed pressure, yield, and adhesion of soil-biodegradable mulches with pie pumpkin (Cucurbita pepo). HortScience 55, 1014–1021. doi: 10.21273/HORTSCI15017-20
Zhang, H., Miles, C. A., Gerdeman, B., LaHue, D. G., and DeVetter, L. W. (2021). Plastic mulch use in perennial fruit cropping systems—a review. Sci. Hortic. 281, 109975. doi: 10.1016/j.scienta.2021.109975
Zhang, H., Miles, C. A., Ghimire, S., Benedict, C., Zasada, I., Liu, H., et al. (2020b). Plastic mulches improved plant growth and suppressed weeds in late summer-planted floricane-fruiting raspberry. HortScience 55, 565–572. doi: 10.21273/HORTSCI14734-19
Zorrilla-Fontanesi, Y., Cabeza, A., Domínguez, P., Medina, J. J., Valpuesta, V., Denoyes-Rothan, B., et al. (2011). Quantitative trait loci and underlying candidate genes controlling agronomical and fruit quality traits in octoploid strawberry (Fragaria × ananassa). Theor. Appl. Genet. 123, 755–778. doi: 10.1007/s00122-011-1624-6
Keywords: plasticulture, strawberry, lettuce, sustainability, alternative system
Citation: Wang X, Shrestha S, Tymon L, Zhang H, Miles C and DeVetter L (2022) Soil-biodegradable mulch is an alternative to non-biodegradable plastic mulches in a strawberry-lettuce double-cropping system. Front. Sustain. Food Syst. 6:942645. doi: 10.3389/fsufs.2022.942645
Received: 12 May 2022; Accepted: 02 August 2022;
Published: 24 August 2022.
Edited by:
Manosh Kumar Biswas, University of Leicester, United KingdomReviewed by:
Annette Wszelaki, The University of Tennessee, Knoxville, United StatesSaiful Irwan Zubairi, National University of Malaysia, Malaysia
Copyright © 2022 Wang, Shrestha, Tymon, Zhang, Miles and DeVetter. This is an open-access article distributed under the terms of the Creative Commons Attribution License (CC BY). The use, distribution or reproduction in other forums is permitted, provided the original author(s) and the copyright owner(s) are credited and that the original publication in this journal is cited, in accordance with accepted academic practice. No use, distribution or reproduction is permitted which does not comply with these terms.
*Correspondence: Lisa DeVetter, lisa.devetter@wsu.edu