- 1State Key Laboratory of Biology of Plant Diseases and Insect Pests, Institute of Plant Protection, Chinese Academy of Agricultural Sciences, Beijing, China
- 2Tangshan Academy of Agricultural Sciences, Tangshan, China
- 3College of Forestry, Hebei Agricultural University, Baoding, China
Sclerotinia stem rot, caused by Sclerotinia sclerotiorum, is a devastating disease of soybean. Biological control is a potential alternative to chemical fungicides for disease management, and provides broad benefits to the environment, farmers and consumers. Herein, we established a field application technique for biocontrol of Sclerotinia stem rot in soybean using wuyiencin, expanding on a previous study showing biocontrol potential. We used wuyiencin to reduce sclerotia in soybean seed, and disease incidence analysis by seed bioassay revealed an optimal wuyiencin seed soaking concentration of 12.5 μg/mL. We found that different application methods had different effects on soybean plant growth. Soybean pot experiments showed that 100 μg/mL wuyiencin was obtained a significant disease protection effect and promote soybean growth through root irrigation, and the optimal concentration for wuyiencin spraying was 100–200 μg/mL. We tested the efficacy of applying wuyiencin under field conditions, and the protection effect of 200 μg/mL wuyiencin sprayed three times was the best (64.0%), but this was slightly inferior to the protection effect of 200 μg/mL dimethachlon (77.6%).
Introduction
Soybean (Glycine max L. Merr.), as an important economic crop, is not only a source of high quality edible oil for humans, but also a high quality plant protein in animal feed worldwide (Silva et al., 2019). However, plant disease, especially Sclerotinia stem rot (SSR, or white mold), can negatively impact soybean productivity (Pereira et al., 2012). SSR in soybean caused by Sclerotinia sclerotiorum (Lib.) de Bary is a highly destructive and severely yield-limiting disease, especially in temperate climates (Boland and Hall, 1994; Hoffman et al., 1998; Kurle et al., 2001; Danielson et al., 2004). The S. sclerotiorum fungus infects a broad spectrum of host plants (>600), including soybean, inflicting yield losses of up to 70%. The presence of sclerotia mixed in seed may result in lower seed number and weight, and SSR infection within seed pods may lower oil content and reduce seed germination (Mueller et al., 1999; Yang et al., 2022). The distribution of S. sclerotiorum covers 95 countries and almost every continent, including Africa, Asia, Australasia, Europe, North America, and South America (Saharan and Mehta, 2008; Willbur et al., 2019b).
Sclerotia may remain viable in soil for up to 8 years, which plays a major role in the disease cycle (Adams and Ayers, 1979). Sclerotia can germinate via apothecia or mycelia under suitable environmental conditions (Bolton et al., 2006). Once sclerotia conditioning is achieved, apothecia will germinate from sclerotia and produce ascospores, which are ejected and carried via the wind to infect nearby flowers and pods of soybean (Peltier et al., 2012). Sclerotia that germinate via mycelia produce hyphae that directly attack tissues (Bardin and Huang, 2001). Apothecial germination is the primary source of inoculum for infection by S. sclerotiorum (Le Tourneau, 1979). As S. sclerotiorum has a large host range and can infect any plant part, the symptomatology of SSR is highly variable. For soybean, leaves infected with this fungus have water-soaked lesions that expand rapidly into petioles into stems, and lesions develop into necrotic tissues that subsequently display patches of fluffy white mycelia, which are the most obvious sign of disease (Kurle et al., 2001; Bolton et al., 2006). Moreover, as the phytopathogen progresses into the main stem, wilting occurs, and sclerotia form in or on the stem pith, and flowering and bean pods, during high humidity conditions in the harvest period (Purdy, 1979). It is worth noting that SSR is detected more quickly under cool (<28°C) and moist (continuous surface wetness for 40–112 h) conditions (Huang et al., 1998; Willbur et al., 2019a).
Resistant cultivars of S. sclerotiorum are unavailable for most cultivated hosts, making it extremely difficult to control this disease. In the field, integrated management of SSR utilizes a combination of cultural, chemical and biological control strategies (Peltier et al., 2012). Cultural practices include crop rotation using non-host plants, uniform coverage of soil with straw, cleaning implements and of harvesting machines, and modifying row spacing (Garza et al., 2002; Mueller et al., 2002, 2004; de Souza Jaccoud-Filho et al., 2016). The use of chemical fungicides by means of foliar spraying, mainly during the beginning of flowering until the beginning of pod formation, is currently the most effective approach (Chacon-Orozco et al., 2020). However, due to long-term extensive application of chemical fungicides, pathogens have gradually evolved resistance, and pesticide residues can have negative impacts on human health and the environment (Huzar-Novakowiski et al., 2017). Therefore, biocontrol is an alternative to chemical control strategies, including the use of antagonistic bacteria, fungi, actinomycetes and virions, as well as their secondary metabolites and volatile organic compounds (Chacon-Orozco et al., 2020; Ojaghian et al., 2020b). Biological control agents (BCAs) are considered promising alternative management approaches that can address fungicide resistance while minimizing environmental pollution (Abdullah et al., 2008).
Actinomycetes, a numerous and widely distributed group of soil microbes, are Gram-positive bacteria characterized by a genome with a high GC ratio (Olanrewaju and Babalola, 2019). Approximately two thirds of natural antibiotics have been isolated from actinomycetes, ~75% from the Streptomyces genus (Franco-Correa et al., 2010). Streptomyces species are sources of bio-actives, hence they are well-studied, and they are important producers of diverse secondary metabolites such as antifungals, antivirals, insecticides, antibacterials, anti-hypertensives, and many antibiotics and immunosuppressives (Procópio et al., 2012; Viaene et al., 2016). Hence, they are crucial for health, agriculture, and other sectors. In agriculture, many studies have focused on the biocontrol and biofertiliser activities of the Streptomyces genus, and most Streptomyces species have the ability to promote plant growth and inhibit pathogens (Babalola et al., 2009; Jog et al., 2016).
Successful biological control of S. sclerotiorum in vitro has been reported by several researchers using antibiotics (Fernando et al., 2007). Chen et al. (2011) found that Streptomyces spiroverticillatus produces Tautomycin, which strongly inhibits the mycelial growth of S. sclerotiorum, with a median effective concentration (EC50) value of 3.26 × 10−4 μg/mL. In addition, both Rapamycin, a macrolide antibiotic produced by Streptomyces hygroscopicus, and Phencomycin, a secondary metabolite produced by Streptomyces flavofuscus G1, strongly inhibit S. sclerotiorum (Cao et al., 2015; Ojaghian et al., 2020a). However, most previous studies have focused on screening fungal and bacterial antagonists, and the determination of inhibition percentage in vitro, hence we still lack mature and stable biocontrol products, and effective large-scale application of biocontrol technologies in the field for soybean and other vegetable crops.
Wuyiencin is a nucleoside antibiotic produced by Streptomyces albulus var. wuyiensis, a strain first isolated from a natural soil habitat in the Wuyi Mountains of China (Wei et al., 1984; Liu et al., 2018). This highly effective, broad-spectrum, low-toxicity bio-pesticide is mainly used to control a variety of fungal diseases of vegetables, fruits, grains and other crops (Ge et al., 2015; Yang et al., 2021a). In a previous study, we observed that Wuyiencin protects soybean from S. sclerotiorum through antibiosis (inhibition of sclerotia production and germination), but application technologies for controling SSR using Wuyiencin have not been established (Yang et al., 2022).
In the present study, we determined the optimal concentration of Wuyiencin for treating soybean seed. Additionally, the effects of Wuyiencin on soybean seedling growth were investigated following foliar spraying or root irrigation. We subsequently evaluated the effectiveness of Wuyiencin for managing SSR under field conditions, and established an application strategy that could benefit organic soybean production industry and conventional production sectors.
Materials and Methods
Experimental Site and Cultural Conditions
Field trials were conducted at Daoxianghu, Beijing, China (N 40°09.863, E 116°17.043), and Tangshan Academy of Agricultural Sciences, Tianjin, China (N 39°62.495, E 118°23.596). The experimental plots in Beijing and Tianjin were 0.13 ha. All cultural conditions were applied in the same manner for both Beijing and Tianjin trial sites, including cultivars, row spacing, tillage and planting date. Soybean variety Zhonghuang13 was planted at the above two sites on 15 April 2021, and replanted with seedlings to fill gaps on 25 April 2021. Soybean seeds were planted at a row spacing of 20 cm with an average seeding rate of ~45 kg/ha. It was necessary to reduce sclerotia germination by plowing the soil deeply before sowing seeds, and removing weeds. S. sclerotiorum Ss-H (ACCC No. 39616) and Streptomyces albulus var. wuyiensis strain CK-15 (CGMCC NO. 0703) were provided by the Agriculture Culture Collection of China and China General Microbiological Culture Collection Center, respectively. Streptomyces albulus CK-15 was cultured on Mannitol-soybean (MS) agar (20 g soybean flour in distilled water, boiled for 30 min, and filtered into a beaker to obtain 20 g mannitol and 17 g agar/liter) at 28°C in a cultivating box. S. sclerotiorum was cultured on Potato dextrose agar (PDA) (200 g fresh potato tuber, 15 g agar, and 20 g dextrose/liter of distilled water) at 25°C in a cultivating box (Yang et al., 2022).
Testing the Efficacy of Wuyiencin on Soybean Growth When Applied as a Seed Treatment
Wuyiencin preparation was performed as described previously in Yang et al. (2022). Soybean seeds were immersed in 1% NaClO solution for 10 min for surface sterilization, washed three times in sterile distilled water, and blotted on fresh paper towels. Disinfested seeds were soaked for 4 h in different wuyiencin concentrations (12.5, 25, 50, 100 mg/L) or solutions without wuyiencin (negative controls). Treated seeds were placed on sterile paper towels to dry. Thirty seeds for each treatment were randomly selected and placed in petri dishes covered with moist filter paper. Each group included three replicates, and wuyiencin-free controls were included. Petri dishes were placed in an alternating light and dark (L/D = 16 h/8 h) incubator at 25°C with 60% relative humidity. Distilled water was added regularly to keep dishes moist. The germination results for each treatment were recorded for 0–7 days, the length of radicles and embryonic axes was measured after day 7, and germination was classified when the seed radicle length was >0.2 mm. The germination rate for each treatment was calculated as the average percentage of soybean seeds showing germination vs. the total number of soybean seeds.
Analysis of the Effects of Different Wuyiencin Application Methods on Soybean Growth Under Greenhouse Conditions
Soybean seeds were immersed in 1% NaClO solution for 10 min for surface sterilization, washed three times in sterile distilled water, and blotted on fresh paper towels. Three seeds were sown in a 1:1 mixture of soil and sand (v/v) in 20 cm diameter plastic pots and incubated under greenhouse conditions at 25°C with 75% relative humidity and a 16 h light and 8 h dark photoperiod. Soybean seedlings were thinned after the expansion of the first true leaf, leaving a healthy seedling in each pot. Seedlings were irrigated when the topsoil was dry.
When the youngest fully expanded trifoliate leaves of 4-week-old soybean plants had appeared, soybean seedlings with consistent growth were selected to measure the effects of different application methods on the growth traits of soybean seedlings. Six treatments were set up; different wuyiencin concentrations (25, 50, 100 μg/mL) were applied by root irrigation (30 mL in each pot) or foliar spraying (until drops were uniform and no drops were left on leaves). Twenty seedlings were included in each treatment and the experiment was repeated three times. The same amount of water was applied as a negative control. After four applications (7 days per cycle), root length, plant height, dry weight and fresh weight of soybean seedlings in each treatment were measured. Root length was from the junction point of roots and stems to the root tip of soybean plants, and plant height was from the cotyledons to the growing point of soybean plants. Seedlings were placed in an oven at 70°C for 24 h to measure dry weight.
Investigating the Effects of Different Treatments for SSR Disease Management Under Field Conditions
Inoculum Preparation
Artificial inoculation was required because there was no incidence of SSR in either the Beijing or Tianjin locations. One technique for inoculation is sowing sclerotia, hence sclerotia of S. sclerotiorum were collected from the laboratory and used as inoculum. S. sclerotiorum Ss-H (ACCC No. 39616), provided by the Agriculture Culture Collection of China, were used to produce sclerotia on autoclaved potato and carrot medium, and sclerotia were placed in a 4°C refrigerator for 60 days. Following low temperature treatment, sclerotia were spread on 15 May 2021. The sclerotia inoculum was incorporated into the top 10 cm of soil with a cultivator.
Another technique is to inoculate the stems of soybean with S. sclerotiorum mycelium. Sterile toothpicks were immersed in potato dextrose broth (PDB) for 24 h. Mycelial plugs of S. sclerotiorum (6 mm diameter), which were cut from the margin of each colony cultured for 2 days at 25°C, were transferred to PD agar (PDA) plates, and toothpicks were evenly placed around the plugs and cultured at 25°C for 4 days. Toothpicks covered with mycelia were inserted into young stems, the incidence of SSR was observed after 72 h, data were collected by five-point sampling in each plot, and 10 soybean plants were investigated at each point to count diseased soybean plants in each plot. Disease incidence for each treatment was estimated as the average percentage of plants showing visible symptoms of infection vs. the total number of investigated soybean plants.
Treatments and Experimental Design
The experimental design consisted of 12 plots, including positive and negative controls. For the negative control, wuyiencin was not applied, and dimethachlon (CAS No.24096-53-5) served a positive control. Four doses of wuyiencin (12.5, 25, 50, 100 μg/mL) were tested. The above six plots were sprayed twice at sealing ridge and initial flowering stages. For the remaining six plots, negative control, positive control and treatments were sprayed three times at sealing ridge, initial flowering and full bloom stages (Table 1). A randomized complete block design with three replicates for each plot was employed, and protective rows were set around the test field. All plots were sprayed by a backpack sprayer, with separate sprayers used for dimethachlon and wuyiencin. The whole plant was evenly covered using a knapsack manual sprayer, which has a uniform application rate. The amount of water applied to soybean plants per mu was 30 kg. Each application was performed weekly and before 9 a.m. to avoid adverse effects from heat and afternoon winds.
Identification and Scoring of Disease Symptoms
Data were collected by five-point sampling in each plot, and 10 soybean plants were investigated at each point to count diseased soybean plants in each plot. To determine disease severity, each inoculated stem was scored by measuring its spot length, and the stem areas exhibiting white mildew was estimated visually. Each stem was assessed for disease severity on a grading scale of 0–5, where 0 = no symptoms, 1 = spot length <2 cm and light brown color; 2 = spot length 2–4 cm, light brown color, with white mildew; 3 = spots length 4–7 cm, light brown color, with white mildew; 4 = spot length >7 cm, brown color, with white mildew and yellowing of leaf blades; 5 = spot length >7 cm, brown color, white mildew layer, sclerotia formation, and upper branch leaves dead (Figure 1). The disease severity index (DSI) for each treatment was calculated from the above values for each plant using the following formula (Becker et al., 2021):
where n is the number of stems in each severity grade, b is the severity grade, N is the total number of grades used (5), and T is the total number of stems.
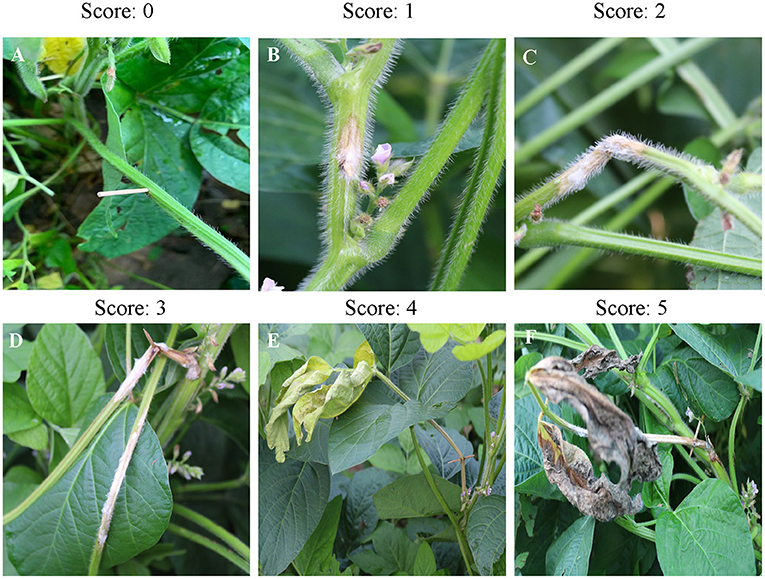
Figure 1. The six-point scale system used to calculate disease severity. Leaves are scored 0 (A), 1 (B), 2 (C), 3 (D), 4 (E), or 5 (F) on the disease severity scale.
The prevention efficacy (CE) of each treatment was calculated according to the following formula:
where c is the disease index of the negative control and t is the disease index of treatments.
Statistical Analysis
Statistical analysis was performed using SPSS 13.0 (SPSS Institute, Chicago, IL, USA) with analysis of variance (ANOVA) tests. Treatments were compared using Fisher's protected least significant difference (LSD) tests to estimate significant differences between means. Differences were considered significant at p < 0.05.
Results
Optimizing the Wuyiencin Concentration for Soaking Soybean Seeds
Seed bioassays showed that wuyiencin had a significant effect on the germination rate and growth of soybean seeds. When treated with a high concentration (>12.5 μg/mL) of wuyiencin, the germination rate and growth of soybean seeds decreased significantly, and the degree of reduction was dose-dependent Figure 2. Compared with controls, 25, 50, and 100 μg/mL wuyiencin inhibited the germination rate of soybean seeds from 87.2 to 82.4, 74.9 and 63.1%, respectively. As shown in Table 2, 50 and 100 μg/mL wuyiencin also significantly inhibited the growth of soybean cotyledon length to 1.89 cm and 1.67 cm, respectively, and both cotyledon length and radicle length of soybean seedlings were significantly decreased following treatment with 25 μg/mL wuyiencin. However, the germination rate and growth of soybean seeds did not change significantly following treatment with 12.5 μg/mL wuyiencin. Therefore, the concentration of wuyiencin should not be above 12.5 μg/mL when soaking soybean seeds.
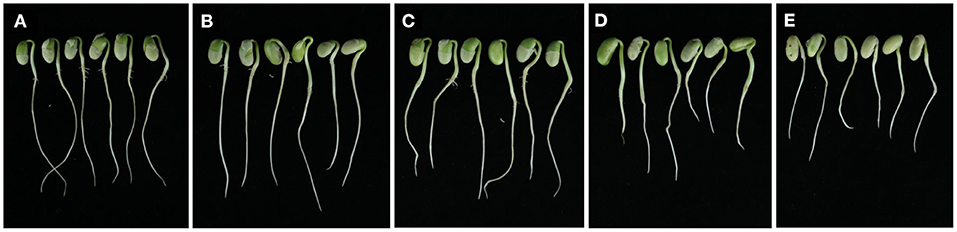
Figure 2. Seed bioassay analysis of the effects of different concentrations wuyiencin on soybean seed growth. From left to right, treatments are 0 (A), 12.5 (B), 25 (C), 50 (D), and 100 (E) μg/mL wuyiencin.
Effects of Wuyiencin on Soybean on Plant Growth Following Root Irrigation or Spraying
In order to efficiently use wuyiencin for controlling SSR in the field, the effects of different application methods on soybean plant growth were explored by greenhouse experiments. Application of wuyiencin at different concentrations via root irrigation had different effects on plant height, root length, fresh weight and dry weight (Table 3). Compared with controls, plant height and root length of soybean were significantly inhibited when treated with 200 μg/mL wuyiencin, and decreased from 55.7 cm and 48.0 cm (SD from controls) to 48.1 cm and 39.2 cm, respectively. Additionally, plant height, fresh weight and dry weight of soybean plants did not change significantly following treatment with 100 μg/mL wuyiencin. Moreover, 50 μg/mL wuyiencin significantly increased plant height and fresh weight of soybean to 60.3 cm and 59.25 g, respectively. Moreover, 50 μg/mL wuyiencin significantly increased plant height and fresh weight of soybean to 60.3 cm and 59.25 g, respectively, but there was no significant difference in plant height and dry weight of soybean plants. Based on these results, it was found that soybean roots were sensitive to wuyiencin, especially high concentrations. Therefore, the optimal concentration of Wuyiencin was 100 μg/mL when applying wuyiencin via root irrigation to obtain a significant disease protection effect and promote soybean growth.
Application of wuyiencin at different concentrations through foliar spraying also had different effects on soybean growth (Table 4). Unlike root irrigation, there was no significant difference in plant height, root length, fresh weight or dry weight of soybean between treatment with 50 μg/mL wuyiencin and controls following spraying. At a wuyiencin concentration of 100 μg/mL, root length of soybean was significantly increased from 47.4 cm (SD from controls) to 52.3 cm. Also, there was no significant on in soybean growth between treating with 200 μg/mL wuyiencin and controls. However, 50 μg/mL wuyiencin significantly increased plant height compared with 200 μg/mL wuyiencin. In conclusion, the optimal concentration or applying wuyiencin by spraying was 100–200 μg/mL.
Control of S. sclerotiorum by Wuyiencin Under Field Conditions
Disease Incidence Evaluation
In the early stages of this study, there was no occurrence of SSR by artificial inoculation through sowing sclerotia in either Tianjin or Beijing. Therefore, we adopted mycelial inoculation in order to determine the field protection effect of wuyiencin against SSR. Wuyiencin was applied at different concentrations for protective spraying before inoculation (at the sealing ridge stage), and the prevention effect of wuyiencin was evaluated according to disease incidence. As shown in Table 5, disease incidence was significantly different between treated and non-treated plots, and different concentrations of wuyiencin had different prevention effects; 25, 50, 100, and 200 μg/mL wuyiencin reduced disease incidence from 80.2% (SD from controls) to 77.1, 58.0, 53.2, and 37.7%, respectively. In addition, the disease incidence was 22.0% following treatment 200 μg/mL dimethachlon, for which the protection effect was significantly better than for 200 μg/mL wuyiencin. Thus, wuyiencin can also reduce disease incidence and has a distinct protection effect against SSR, although the prevention effect of wuyiencin on S. sclerotinia is not as good as that of dimethachlon.
Protection Effect Evaluation
Wuyiencin was sprayed for the first time to prevent infection of S. sclerotiorum at the sealing ridge stage, then for a second time to inhibit the spread of the pathogen at the initial flowering stage. The disease severity index and protection effect of different treatments were calculated. As shown in Table 6, there were significant differences in disease severity between treatments. Compared with negative controls, wuyiencin significantly reduced disease severity Figure 3. After treatment with 200 μg/mL wuyiencin, the disease severity was decreased by 3.24%, and the protection effect was 61.6%, which was not significantly different from that of 100 μg/mL wuyiencin. In addition, disease severity decreased by 3.51% following dimethachlon treatment (as a positive control), which was 72.7% higher than that of 200 μg/mL wuyiencin.
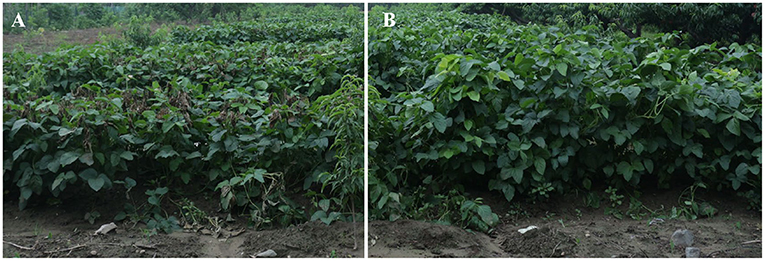
Figure 3. Control efficacy of wuyiencin against Sclerotinia stem rot in soybean. The field study evaluated the effectiveness of treating infected soybean plants with 200 μg/mL wuyiencin. The left-hand side was not treated with any chemical fungicide as a negative control (A) and the right-hand side of the protective rows was treated with wuyiencin (B).
When serious disease occurred, we sprayed wuyiencin for a third time at the full bloom stage to improve the protection effect. The results showed that the protection effect of 200 μg/mL dimethachlon sprayed three times was the best (77.6%), significantly higher than the protection effect of 200 μg/mL wuyiencin sprayed twice under the same conditions (64.0%). Interestingly, there were no significant differences between wuyiencin treatments with different application times, which indicates that it is better to apply wuyiencin before and during the early stages of SSR.
Discussion
In 2019, soybean production in China was 1.81 million tons, compared with only 1.64 million tons in 2021 (Yang et al., 2021b). This decrease has been mainly attributed to yield losses caused by soybean disease, such as SSR, which is caused by S. sclerotiorum, and is severe in many countries (Willbur et al., 2019a). Because breeding programs for disease-resistant soybean have been hampered by limited genetic resources (Lu, 2003), S. sclerotiorum continues to impose serious limitations on soybean production.
Application of fungicides is an important tool for controlling S. sclerotiorum (Ma et al., 2009). Compared with bio-pesticides, chemical fungicides have the advantages of lower cost and higher efficiency for controlling SSR. However, in recent years, excessive and unscientific use of chemical fungicides has led to the emergence of resistant strains. The benzimidazole fungicide carbendazim has been widely and extensively used against S. sclerotiorum for several decades in China (Shi et al., 2000; Zhu and Chen, 2010). High levels of carbendazim resistance and reduced field efficacy have been reported, with the first carbendazim-resistant strain discovered in China in 1995 (Zhang et al., 1998). Widespread control failures due to carbendazim resistance resulted in a suspension of carbendazim application for control of S. sclerotiorum in the 2000s in China (Pan et al., 1997; Shi et al., 2000). In addition, dimethachlon, a dicarboximide fungicide, has been widely used to control SSR in China for many years. In Jiangsu province, dimethachlon has been recommended to supersede carbendazim for the management of SSR since 2002 (Qi et al., 2006; Ma et al., 2009). Wide use of dimethachlon and other dicarboximide fungicides has raised concerns about resistance issues in S. sclerotiorum (Zhou et al., 2014), and these concerns about resistant strains have prompted interest in biological control as an alternative strategy for disease management.
Successful biological control of SSR in soybean and other crops has been achieved by several researchers in greenhouses and field trials using fungal and bacterial antagonists. Trichoderma spp. and Coniothyrium minitans are currently the most widely used biological control agents against SSR (Huang and Hoes, 1976; Harman et al., 2004; Sumida et al., 2018). C. minitans parasitises the hyphae and sclerotia of S. sclerotiorum, and this effective BCA reduced the disease severity index by 68.5% under field conditions, while T. harzianum reduced disease severity index by 38.5% (Zeng et al., 2012b; Willbur et al., 2019a). Bacillus subtilis is a soil-borne microbe that has shown promise for controlling SSR in several crops. Zhang and Xue (2010) reported that the cell suspension of B. subtilis SB24 significantly reduced SSR severity by ~62–90% at 15 days after inoculated with S. sclerotiorum. However, the field protection effect of these microbial fungicides is unstable, especially because microorganisms are sensitive to external environmental factors such as temperature, humidity and light. In addition, microbial fungicide preparations suffer from poor storage stability and short field duration (Eyal et al., 1997). Compared with living biological agents, agricultural antibiotics exert a more stable protection effect and longer product stability. Although previous studies have shown that phencomycin, rapamycin, shenqinmycin and other agricultural antibiotics displayed significant inhibitory effects against S. sclerotiorum, there is a lack of the field efficacy and corresponding application technologies (Cao et al., 2015; Ojaghian et al., 2020a).
Wuyiencin, a secondary metabolite produced by S. albulus CK-15, has a broad activity spectrum and its products is recommended for pollution-free vegetable production and meets national and industry standards for organic product certification (COFFC-R-0903-0070) (Ge et al., 2015). Field application technologies for cucumber powdery mildew, tomato gray mold, tomato leaf mold, and other fungal diseases have achieved great protection effects. Yang et al. (2021a) reported that wuyiencin could significantly reduce powdery mildew disease incidence in cucumber, with a maximum prevention efficacy of 94.38%. In addition, wuyiencin has been applied in many provinces and cities in China, and it has a strong protection effect on S. sclerotiorum in Brassica napus (Zeng and Shi, 2003). Bioassays in vitro showed that wuyiencin had significant inhibition and disease prevention effects against S. sclerotiorum (Yang et al., 2022). However, the field effect of wuyiencin on SSR has not been clarified, and corresponding application technologies are lacking.
In the present study, a field application technique for wuyiencin-mediated control of SSR was established for the first time. Soybean seeds were soaked with 12.5 μg/mL wuyiencin for 4 h before sowing, accompanied by deep plowing. Additionally, 2% wuyiencin (200 μg/mL) was sprayed at the stem (close to the soil surface) of soybean plants at the ridge sealing stage. At the initial flowering stage, 2% wuyiencin (200 μg/mL) was sprayed on soybean leaves, and the protection effect was 61.6%. When serious disease occurred, wuyiencin was sprayed continuously at the full bloom stage, and the protection effect was at 64.0%.
Soaking seeds before sowing is an important part of the application process. Sclerotia are the primary long-term survival structures of S. sclerotiorum, and they can initiate another disease cycle when conditions are suitable (Purdy, 1979; Zeng et al., 2012a). It has reported that wuyiencin could hinder the germination of sclerotia (Yang et al., 2022). Therefore, soybean seeds were immersed in wuyiencin before sowing, so as to inhibit sclerotia present in soybean seeds, and thereby reduce the source of initial infection and disease incidence. In this work, seed bioassays showed that the optimal concentration of wuyiencin for soaking seeds was 12.5 μg/mL. Low concentrations of wuyiencin (< μg/mL) had a poor inhibition effect on sclerotia germination, while high concentrations (>12.5 μg/mL) had a significant inhibition effect on seed germination.
Application technology typically involves spraying. When exploring the effects of different application methods on soybean plant growth, we found that soybean roots were more sensitive to wuyiencin. When 200 μg/mL wuyiencin was applied via root irrigation, the plant height, root length, fresh weight and dry weight of soybean were significantly inhibited, while the agronomic indices of soybean growth showed no significant differences between controls and the treatment group sprayed with 200 μg/mL wuyiencin. Therefore, based on the disease cycle of S. sclerotiorum and the above experimental results, spraying wuyiencin could control SSR under field conditions.
The optimal application time for wuyiencin is based on the characteristics of the infection cycle of S. sclerotiorum. Carpogenic germination leading to ascospore formation also plays a major role in sclerotinia diseases, and sclerotia germinate when exposed to low temperature and high humidity in the first instance (Huzar-Novakowiski et al., 2017; Willbur et al., 2019a). Therefore, the ridge sealing stage is the key stage for wuyiencin for controling SSR, and carpogenic germination can be reduced by spraying with wuyiencin accompanied by deep tillage (Chacon-Orozco et al., 2020). In addition, sclerotia germinating carpogenically produce apothecia, and subsequently ascospores, that infect above-ground portions of soybean, especially flowers (Peltier et al., 2012). Because flowering of crops occurs about the time that canopies close, nutrient sources are available during a time when environmental conditions are more favorable for the growth of S. sclerotiorum (Inglis and Boland, 1990; Turkington et al., 1991). Also, initiation of infection and disease occur in only a few soybeans where mycelia can directly infect other susceptible tissues. Consequently, the best control times for SSR are the initial flowering and full bloom stages of soybean. In the present study, spraying 200 μg/mL wuyiencin at the stems (close to the soil surface) of soybean in the ridge sealing stage could effectively reduce disease incidence by 42.5%, and the protection effect was 64.0% when spraying 200 μg/mL wuyiencin at the ridge sealing, initial flowering, and full bloom stages.
In summary, we established an application strategy for wuyiencin to control SSR in field trials in Tianjin and Beijing, including the optimal concentration for soaking seed, and an effective application method, based on preliminary seed and pot soybean bioassays. However, it is worth noting that the field protection effect of wuyiencin was measured under conditions of artificial inoculation in this study, and both experiment trials at Tianjin and Beijing had no incidence of SSR upon sowing sclerotia. Furthermore, there was a lack of sclerotia germination producing apothecia when mycelia of S. sclerotiorum were directly inoculated into the soybean stem, which ignored the inhibition effect of wuyiencin on sclerotia germination, hence the best protection effect of wuyiencin was only 64.0%. Consequently, we further confirmed the field protection effect of wuyiencin against S. sclerotiorum under natural incidence of SSR. In future studies, wuyiencin could be mixed with, or used instead of, chemical pesticides to improve the protection effect and optimize application technologies.
Data Availability Statement
The original contributions presented in the study are included in the article/supplementary material, further inquiries can be directed to the corresponding author.
Author Contributions
KZ and BG: conceptualization. BG and XH: methodology. MY, JX, and ZL: validation. MY and SZ: formal analysis. MY: data curation and writing-original draft. BG: funding acquisition. XH: investigation. XH and SZ: resources. ZL and BL: visualization. BG and LS: writing-review and editing. All authors contributed to the article and approved the submitted version.
Funding
This work was supported by the National Key Research and Development Program of China (Grant No. 2019YFD1002000) and the Major Research Project of Guangxi for Science and Technology (Grant No. AA18242026).
Conflict of Interest
The authors declare that the research was conducted in the absence of any commercial or financial relationships that could be construed as a potential conflict of interest.
Publisher's Note
All claims expressed in this article are solely those of the authors and do not necessarily represent those of their affiliated organizations, or those of the publisher, the editors and the reviewers. Any product that may be evaluated in this article, or claim that may be made by its manufacturer, is not guaranteed or endorsed by the publisher.
Acknowledgments
The authors would like to thank the Institute of Plant Protection, Chinese Academy of Agricultural Sciences for the experimental platform and Tangshan Academy of Agricultural Sciences for providing the field experiment area.
References
Abdullah, M. T., Ali, N. Y., and Suleman, P. (2008). Biological control of Sclerotinia sclerotiorum (Lib.) de Bary with Trichoderma harzianum and Bacillus amyloliquefaciens. Crop Prot. 27, 1354–1359. doi: 10.1016/j.cropro.2008.05.007
Adams, P., and Ayers, W. (1979). Ecology of sclerotinia species. Phytopathology 69, 896–899. doi: 10.1094/Phyto-69-896
Babalola, O. O., Kirby, B. M., Le Roes-Hill, M., Cook, A. E., Cary, S. C., Burton, S. G., et al. (2009). Phylogenetic analysis of actinobacterial populations associated with Antarctic dry valley mineral soils. Environ. Microbiol. 11, 566–576. doi: 10.1111/j.1462-2920.2008.01809.x
Bardin, S., and Huang, H. (2001). Research on biology and control of sclerotinia diseases in Canada. Can. J. Plant Pathol. 23, 88–98. doi: 10.1080/07060660109506914
Becker, P., Esker, P., and Umaña, G. (2021). Incorporation of microorganisms to reduce chemical fungicide usage in black Sigatoka control programs in costa rica by use of biological fungicides. Crop Prot. 146, 105657. doi: 10.1016/j.cropro.2021.105657
Boland, G., and Hall, R. (1994). Index of plant hosts of Sclerotinia sclerotiorum. Can. J. Plant Pathol. 16, 93–108. doi: 10.1080/07060669409500766
Bolton, M. D., Thomma, B. P., and Nelson, B. D. (2006). Sclerotinia sclerotiorum (Lib.) de Bary: biology and molecular traits of a cosmopolitan pathogen. Mol. Plant Pathol. 7, 1–16. doi: 10.1111/j.1364-3703.2005.00316.x
Cao, Y., Wei, S. P., and Ji, Z. Q. (2015). Investigation on antimicrobial compounds from fermentation broth of Streptomyces flavofuscus G1, an endophyte isolated from Euonymus japonicas. Chin. J. Pestic. Sci. 17, 616–621. doi: 10.3969/j.issn.1008-7303.2015.05.17
Chacon-Orozco, J. G., Bueno, C. Jr., Shapiro-Ilan, D. I., Hazir, S., Leite, L. G., and Harakava, R. (2020). Antifungal activity of Xenorhabdus spp. and Photorhabdus spp. against the soybean pathogenic Sclerotinia sclerotiorum. Sci. Rep. 10, 20649. doi: 10.1038/s41598-020-77472-6
Chen, X., Zhu, X., Ding, Y., and Shen, Y. (2011). Antifungal activity of tautomycin and related compounds against Sclerotinia sclerotiorum. J. Antibiot. 64, 563–569. doi: 10.1038/ja.2011.55
Danielson, G., Nelson, B., and Helms, T. (2004). Effect of Sclerotinia stem rot on yield of soybean inoculated at different growth stages. Plant Dis 88, 297–300. doi: 10.1094/PDIS.2004.88.3.297
de Souza Jaccoud-Filho, D., Sartori, F. F., Manosso-Neto, M., Vrisman, C. M., da Cunha Pierre, M. L., Berger-Neto, A., et al. (2016). Influence of row spacing and plant population density on management of “white mould” in soybean in southern Brazil. Aust. J. Crop Sci. 10, 161–168. doi: 10.11924/j.issn.1000-6850.casb2020-085
Eyal, J., Baker, C., Reeder, J., Devane, W., and Lumsden, R. (1997). Large-scale production of chlamydospores of Gliocladium virens strain GL-21 in submerged culture. J. Ind. Microbiol. Biot. 19, 163–168. doi: 10.1038/sj.jim.2900430
Fernando, W., Nakkeeran, S., Zhang, Y., and Savchuk, S. (2007). Biological control of Sclerotinia sclerotiorum (Lib.) de Bary by Pseudomonas and Bacillus species on canola petals. Crop Prot. 26, 100–107. doi: 10.1016/j.cropro.2006.04.007
Franco-Correa, M., Quintana, A., Duque, C., Suarez, C., Rodríguez, M. X., and Barea, J. M. (2010). Evaluation of actinomycete strains for key traits related with plant growth promotion and mycorrhiza helping activities. Appl. Soil Ecol. 45, 209–217. doi: 10.1016/j.apsoil.2010.04.007
Garza, J. G., Neumann, S., Vyn, T., and Boland, G. (2002). Influence of crop rotation and tillage on production of apothecia by Sclerotinia sclerotiorum. Can. J. Plant Pathol. 24, 137–143. doi: 10.1080/07060660309506988
Ge, B., Cheng, Y., Liu, Y., Liu, B., and Zhang, K. (2015). Biological control of Botrytis cinerea on tomato plants using Streptomyces ahygroscopicus strain CK-15. Lett. Appl. Microbiol. 61, 596–602. doi: 10.1111/lam.12500
Harman, G. E., Howell, C. R., Viterbo, A., Chet, I., and Lorito, M. (2004). Trichoderma species—opportunistic, avirulent plant symbionts. Nat. Rev. Microbiol. 2, 43–56. doi: 10.1038/nrmicro797
Hoffman, D., Hartman, G., Mueller, D., Leitz, R., Nickell, C., and Pedersen, W. (1998). Yield and seed quality of soybean cultivars infected with Sclerotinia sclerotiorum. Plant Dis. 82, 826–829. doi: 10.1094/PDIS.1998.82.7.826
Huang, H., Chang, C., and Kozub, G. (1998). Effect of temperature during sclerotial formation, sclerotial dryness, and relative humidity on myceliogenic germination of sclerotia of Sclerotinia sclerotiorum. Can. J. Bot. 76, 494–499. doi: 10.1139/b98-016
Huang, H., and Hoes, J. (1976). Penetration and infection of Sclerotinia sclerotiorum by Coniothyrium minitans. Can. J. Bot. 54, 406–410. doi: 10.1139/b76-039
Huzar-Novakowiski, J., Paul, P. A., and Dorrance, A. E. (2017). Host resistance and chemical control for management of sclerotinia stem rot of soybean in Ohio. Phytopathology 107, 937–949. doi: 10.1094/PHYTO-01-17-0030-R
Inglis, G. D., and Boland, G. J. (1990). The microflora of bean and rapeseed petals and the influence of the microflora of bean petals on white mold. Can J. Plant Pathol. 2, 129–134. doi: 10.1080/07060669009501015
Jog, R., Nareshkumar, G., and Rajkumar, S. (2016). “Enhancing soil health and plant growth promotion by actinomycetes,” in Plant Growth Promoting Actinobacteria, eds G. Subramaniam, S. Arumugam, and V. Rajendran (Singapore: Springer; CRC Press), 33–45. doi: 10.1007/978-981-10-0707-1_3
Kurle, J. E., Grau, C. R., Oplinger, E. S., and Mengistu, A. (2001). Tillage, crop sequence, and cultivar effects on sclerotinia stem rot incidence and yield in soybean. Agron. J. 93, 973–982. doi: 10.2134/agronj2001.935973x
Le Tourneau, D. (1979). Morphology, cytology, and physiology of sclerotinia species in culture. Phytopathology 69, 887–890. doi: 10.1094/Phyto-69-887
Liu, B., Ge, B., Ma, J., Wei, Q., Khan, A. A., Shi, L., et al. (2018). Identification of wysPII as an activator of morphological development in Streptomyces albulus CK-15. Front. Microbiol. 9, 2550. doi: 10.3389/fmicb.2018.02550
Lu, G. (2003). Engineering Sclerotinia sclerotiorum resistance in oilseed crops. Afr. J. Biotechnol. 2, 509–516. doi: 10.5897/AJB2003.000-1101
Ma, H. X., Feng, X. J., Chen, Y., Chen, C. J., and Zhou, M. G. (2009). Occurrence and characterization of dimethachlon insensitivity in Sclerotinia sclerotiorum in Jiangsu Province of China. Plant Dis. 93, 36–42. doi: 10.1094/PDIS-93-1-0036
Mueller, D., Bradley, C., Grau, C., Gaska, J., Kurle, J., and Pedersen, W. (2004). Application of thiophanate-methyl at different host growth stages for management of sclerotinia stem rot in soybean. Crop Prot. 23, 983–988. doi: 10.1016/j.cropro.2004.02.013
Mueller, D., Dorrance, A., Derksen, R., Ozkan, E., Kurle, J., Grau, C., et al. (2002). Efficacy of fungicides on Sclerotinia sclerotiorum and their potential for control of Sclerotinia stem rot on soybean. Plant Dis. 86, 26–31. doi: 10.1094/PDIS.2002.86.1.26
Mueller, D., Hartman, G., and Pedersen, W. (1999). Development of sclerotia and apothecia of Sclerotinia sclerotiorum from infected soybean seed and its control by fungicide seed treatment. Plant Dis. 83, 1113–1115. doi: 10.1094/PDIS.1999.83.12.1113
Ojaghian, S., Wang, L., and Wang, L. (2020a). Inhibitory efficacy of rapamycin against potato white mold. J Plant Dis. Prot. 127, 483–494. doi: 10.1007/s41348-020-00335-9
Ojaghian, S., Zhang, L., and Wang, L. (2020b). Inhibitory effect of natamycin against carrot white mold caused by Sclerotinia sclerotiorum. Trop. Plant Pathol. 45, 425–433. doi: 10.1007/s40858-020-00369-2
Olanrewaju, O. S., and Babalola, O. O. (2019). Streptomyces: implications and interactions in plant growth promotion. Appl. Microbiol. Biot. 103, 1179–1188. doi: 10.1007/s00253-018-09577-y
Pan, Y. L., Wang, Z. Y., and Wu, H. Z. (1997). Resistance to carbendazim and stability of the resistance in Sclerotinia sclerotiorum. Jiangsu J. Agric. Sci. 13, 32–35.
Peltier, A. J., Bradley, C. A., Chilvers, M. I., Malvick, D. K., Mueller, D. S., Wise, K. A., et al. (2012). Biology, yield loss and control of sclerotinia stem rot of soybean. J. Integr. Pest Manag. 3, B1–B7. doi: 10.1603/IPM11033
Pereira, D. G., Sediyama, T., Reis, M. S., Cruz, C. D., Gomes, J. L. L., and Teixeira, R. D. C. (2012). Avaliação da severidade do oídio [Erisyphe diffusa (U. Braun & S. Takam)] em genótipos de soja, em condições de campo. Rev. Caatinga 25, 25–30.
Procópio, R. E. L., Silva, I. R., Martins, M. K., Azevedo, J. L., and Araújo, J. M. (2012). Antibiotics produced by streptomyces. Braz. J. Infect. Dis. 16, 466–471. doi: 10.1016/j.bjid.2012.08.014
Purdy, L. H. (1979). Sclerotinia sclerotiorum: history, diseases and symptomatology, host range, geographic distribution, and impact. Phytopathology 69, 875–880. doi: 10.1094/Phyto-69-875
Qi, Y. X., Chen, F. X., Su, X. Y., Ding, K. J., Yu, J., and Jiang, M. S. (2006). Monitoring on carbendazim-resistance of Sclerotinia sclerotiorum obtained from the blight stems of rape in Anhui Province. Chin. Agric. Sci. Bull. 9, 371–373.
Saharan, G. S., and Mehta, N. (2008). Sclerotinia diseases of crop plants: biology, ecology and disease management. Springer Science & Business Media. doi: 10.1007/978-1-4020-8408-9
Shi, Z., Zhou, M., Ye, Z., Shi, J., Chen, H., and Wang, Y. (2000). Resistance monitoring of Sclerotinia sclerotiorum to carbendazim. Jiangsu J. Agric. Sci. 16, 226–229.
Silva, F. F. A., Alves, C. C. F., Oliveira, J. G., Vieira, T. M., Crotti, A. E. M., and Miranda, M. L. D. (2019). Chemical constituents of essential oil from Murraya paniculata leaves and its application to in vitro biological control of the fungus Sclerotinia sclerotiorum. Food Sci Tech- Brazil. 39, 413–417. doi: 10.1590/fst.20218
Sumida, C. H., Daniel, J. F., Araujod, A. P. C., Peitl, D. C., Abreu, L. M., Dekker, R. F., et al. (2018). Trichoderma asperelloides antagonism to nine Sclerotinia sclerotiorum strains and biological control of white mold disease in soybean plants. Biocontrol Sci. Techn. 28, 142–156. doi: 10.1080/09583157.2018.1430743
Turkington, T. K., Morrall, R. A. A., and Rude, S. V. (1991). Use of petal infestation to forecast sclerotinia stem rot of canola: the impact of diurnal and weather-related inoculum fluctuations. Can. J. Plant Pathol. 13, 347–355. doi: 10.1080/07060669109500920
Viaene, T., Langendries, S., Beirinckx, S., Maes, M., and Goormachtig, S. (2016). Streptomyces as a plant's best friend? FEMS Microbiol Ecol. 92, fiw119. doi: 10.1093/femsec/fiw119
Wei, R., Lin, D., and Chen, Z. (1984). Identification of the producing strain of agricultural antibiotic Bo-10. Acta. Microbiol. Sin. 24, 401–402.
Willbur, J., McCaghey, M., Kabbage, M., and Smith, D. L. (2019a). An overview of the Sclerotinia sclerotiorum pathosystem in soybean: impact, fungal biology, and current management strategies. Trop. Plant Pathol. 44, 3–11. doi: 10.1007/s40858-018-0250-0
Willbur, J. F., Mitchell, P. D., Fall, M. L., Byrne, A. M., Chapman, S. A., Floyd, C. M., et al. (2019b). Meta-analytic and economic approaches for evaluation of pesticide impact on sclerotinia stem rot control and soybean yield in the North Central United States. Phytopathology 109, 1157–1170. doi: 10.1094/PHYTO-04-18-0124-R
Yang, M., Wei, Q., Shi, L., Wei, Z., Lv, Z., Asim, N., et al. (2021a). Wuyiencin produced by Streptomyces albulus CK-15 displays biocontrol activities against cucumber powdery mildew. J. Appl. Microbiol. 131, 2957–2970. doi: 10.1111/jam.15168
Yang, M., Zhang, W., Lv, Z., Shi, L., and Ge, B. (2022). Evaluation of the inhibitory effects of Wuyiencin, a secondary metabolite of Streptomyces albulus CK-15, against Sclerotinia sclerotiorum in vitro. Plant Dis. 106, 156–164. doi: 10.1094/PDIS-05-21-0987-RE
Yang, M., Zhang, W., Wei, Q., Shi, L., Guo, Y., Zhang, K., et al. (2021b). Sclerotinia stem rot of soybean: a review. Chin. Agric. Sci. Bull. 37, 90–99. doi: 10.11924/j.issn.1000-6850.casb2020-0859
Zeng, H. M., and Shi, Y. P. (2003). Wuyiencin, a new antibiotic for controlling crop fungi disease. Fine Spec. Chem. Z1, 15–16.
Zeng, W., Kirk, W., and Hao, J. (2012a). Field management of sclerotinia stem rot of soybean using biological control agents. Biol. Control 60, 141–147. doi: 10.1016/j.biocontrol.2011.09.012
Zeng, W., Wang, D., Kirk, W., and Hao, J. (2012b). Use of Coniothyrium minitans and other microorganisms for reducing Sclerotinia sclerotiorum. Biol. Control 60, 225–232. doi: 10.1016/j.biocontrol.2011.10.009
Zhang, J., and Xue, A. (2010). Biocontrol of sclerotinia stem rot (Sclerotinia sclerotiorum) of soybean using novel Bacillus subtilis strain SB24 under control conditions. Plant Pathol. 59, 382–391. doi: 10.1111/j.1365-3059.2009.02227.x
Zhang, X. L., Zhang, Z., Zhang, J. M., Zhang, G. F., and Sun, X. M. (1998). The reason and analysis for decrease of carbendazim efficacy against Sclerotinia sclerotiorum. Chin. J. Pestic. Sci. Adm. 19, 15–17.
Keywords: wuyiencin, soybean, Sclerotinia stem rot, field efficacy, biocontrol
Citation: Yang M, Han X, Xie J, Zhang S, Lv Z, Li B, Shi L, Zhang K and Ge B (2022) Field Application of Wuyiencin Against Sclerotinia Stem Rot in Soybean. Front. Sustain. Food Syst. 6:930079. doi: 10.3389/fsufs.2022.930079
Received: 27 April 2022; Accepted: 23 May 2022;
Published: 23 June 2022.
Edited by:
Zhen He, Yangzhou University, ChinaReviewed by:
Yaning Li, Agricultural University of Hebei, ChinaTomislav Cernava, Graz University of Technology, Austria
Xiaoyulong Chen, Guizhou University, China
Copyright © 2022 Yang, Han, Xie, Zhang, Lv, Li, Shi, Zhang and Ge. This is an open-access article distributed under the terms of the Creative Commons Attribution License (CC BY). The use, distribution or reproduction in other forums is permitted, provided the original author(s) and the copyright owner(s) are credited and that the original publication in this journal is cited, in accordance with accepted academic practice. No use, distribution or reproduction is permitted which does not comply with these terms.
*Correspondence: Beibei Ge, gbbcsx@126.com
†These authors have contributed equally to this work and share first authorship