- 1Department of Horticulture, Washington State University, Mount Vernon, WA, United States
- 2Department of Agronomy and Horticulture, University of Nebraska–Lincoln, Lincoln, NE, United States
- 3Department of Biosystems Engineering and Soil Science, University of Tennessee, Knoxville, Knoxville, TN, United States
- 4Department of Crop and Soil Sciences, Washington State University, Puyallup, WA, United States
- 5School of Economic Sciences, Washington State University, Pullman, WA, United States
- 6IMPACT Center, School of Economic Sciences, Washington State University, Pullman, WA, United States
- 7Composite Materials and Engineering Center, Washington State University, Pullman, WA, United States
- 8Gulf Coast Research and Education Center, Institute of Food and Agricultural Sciences, University of Florida, Gainesville, FL, United States
Polyethylene plastic mulches are widely used in specialty cropping systems in the United States due to the horticultural benefits they provide. However, polyethylene mulch is reapplied seasonally, generating large volumes of plastic waste that contribute to plastic pollution concerns. This review synthesizes scientific and industry findings to provide a state of current end-of-life options of polyethylene mulch in the United States and identifies opportunities that can improve plastic waste management with a special emphasis on soil-biodegradable plastic mulches. Major points discussed are: (1) polyethylene mulch use in specialty cropping systems, (2) economic, environmental and waste management impacts of polyethylene mulch use, (3) current common end-of-life pathways of used polyethylene mulch, (4) use of soil-biodegradable plastic mulch as an alternative to reduce the amount of plastic waste in the environment and offset the negative impacts associated with residual non-degradable plastics, (5) socioeconomic factors that reduce the adoption of soil-biodegradable plastic mulch, and (6) limitations of soil-biodegradable mulch. The results of this review conclude that recycling and upcycling of used polyethylene mulch can be a more sustainable disposal option, however cleaning and decontaminating used polyethylene mulch is costly and commercial technology is often not accessible nor economically viable in many regions in the current economic and political situation. To make recycling a viable pathway in the future, research and policy developments are necessary to refine and encourage recycling. Soil-biodegradable plastic mulches can offer an additional opportunity to help address these limitations, but they are not permitted in organic agriculture in the United States. Further studies are necessary to address the current knowledge gaps and gain a better understanding of the factors influencing the degradation of soil-biodegradable mulches under diverse field conditions. Improved end-of-life strategies should continue to be pursued that balance sustainable use of plastic mulch while minimizing environmental risks.
Introduction
The introduction and commercial use of polyethylene plastic mulch films (also known as “plastic mulch” and referred to as “polyethylene mulch” in this manuscript) can be traced back to the early 1960s. Adoption of polyethylene mulch across the years has remained steady and is expected to continue rising. By 2026, the annual estimated growth rate of the global polyethylene mulch film market is 6.5%, reaching a market value of $15.7 billion USD (Markets and Markets, 2021). Polyethylene mulches were first used for commercial vegetable production and have since been the standard material for mulching in agricultural production systems worldwide (Kasirajan and Ngouajio, 2012; Hayes et al., 2019; Serrano et al., 2021). Polyethylene mulch can be manufactured at a relatively low price and has been favored for its ease of application due to its light weight and flexibility as well as its high durability. Well-known immediate benefits to growers of its use include early crop development or fruit ripening, improved crop quality, reduced weed pressure, enhanced plant yields, moderated soil temperature, and more efficient water use (Serrano et al., 2021). For these reasons, many growers rely on polyethylene mulch to grow various specialty crops including but not limited to strawberry (Fragaria × ananassa Duch.), tomato (Solanum lycopersicum L.), pepper (Capsicum annuum L.), and various cucurbit crops such as cucumber (Cucumis sativus L.) and pumpkin (Cucurbita pepo L.) (Figure 1).
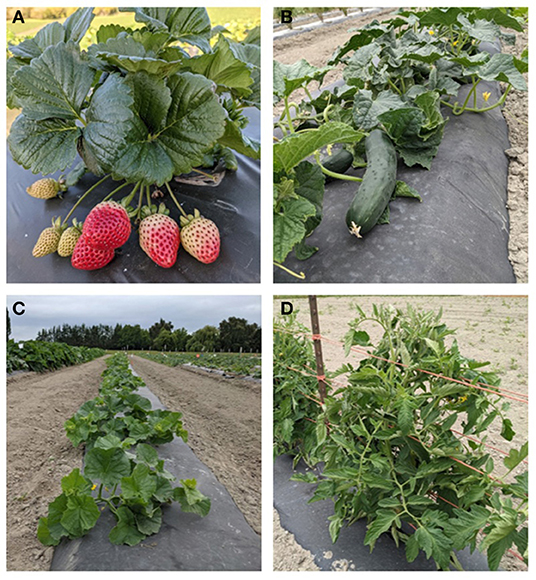
Figure 1. Specialty crop production systems using raised beds covered with polyethylene mulch (A) strawberry, (B) cucumber, (C) pumpkin, and (D) tomato (photos by Brenda Madrid and Lisa W. DeVetter).
Polyethylene Mulch Use in Specialty Cropping Systems
Due to the diversity of crops and cropping systems where mulch is employed, there is no one-size-fits-all style of mulch. Commercially available polyethylene mulches range in color, thickness, permeability, elasticity, and overall durability. Mulch color influences transmission of infrared and photosynthetically active radiation (400–700 nm), which in turn affects weed seed germination, plant growth, and soil temperature (Johnson and Fennimore, 2005). Mulch color can also impact light reflection and absorption, which are important factors that help modify soil and canopy temperatures (Kasirajan and Ngouajio, 2012; Hayes et al., 2019). Reflective mulches with aluminum or silver have been shown to repel aphids and thrips [Thrips tabaci (Lindeman) and Frankliniella sp., respectively] (Adlerz and Everett, 1968; Wolfenbarger and Moore, 1968; Scott et al., 1989; Brown and Brown, 1992). Additives incorporated into silver mulches, such as zinc pyrithione and silver nanoparticles, have also been found to protect crops from bacterial pathogens (Pittol et al., 2017; Hayes et al., 2019). Mulch thickness and formulation influence the permeability of the plastic mulch to water, gases, and germinating weeds (Kader et al., 2017). Elasticity is an important factor for field application and installation purposes. Polyethylene mulch should be able to withstand stretching that occurs during application and be compatible with mechanical hole-punching, transplanting, and/or seeding operations. The optimum type of mulch will also depend upon the time of the year that the mulch is used, crop type(s) grown, the field site's climate, design of the cropping system (i.e., multicropping/relay cropping), and any other on-farm management practices such as soil fumigation (Sanders et al., 1996; Zhang et al., 2021). Durability of polyethylene mulch is dependent upon all these factors and should be considered to ensure that the mulch film lasts throughout the growing season and meets the grower's production goals.
Reduced permeability polyethylene mulch is frequently used in many high-value fruit and vegetable production systems during soil fumigation. In these systems, fumigants are injected into the soil before planting for the management of soilborne pathogens, weeds, and pests including parasitic nematodes that can cause damage to plants and restrict plant growth and crop production (Environmental Protection Agency, 2020). Although the fumigant methyl bromide was phased out through the Montreal Protocol and Clean Air Acts due to its role in depleting stratospheric ozone, limited use is still permitted in the United States through critical-use exemptions and to meet quarantine or pre-shipment criteria. Alternative fumigants to methyl bromide are mostly volatile organic compounds that are directly toxic and can form dangerous air pollutants if emissions are not controlled. Polyethylene mulch is applied during or shortly after soil fumigation to reduce emissions and enhance fumigant retention time in the soil (Figure 2) (Gao et al., 2011). The United States Environmental Protection Agency has strict requirements regarding the type of polyethylene mulch that can be used for soil fumigation. Virtually and totally impermeable mulch, often referred to as tarps, function as low- or zero-permeability films that are effective at reducing fumigant emissions while retaining the fumigant in the soil to improve its efficacy (Gan et al., 1998a,b; Yates et al., 2002). Buffer zones are also required during and after fumigation to provide a safe distance between the application site and bystanders. Because of their high impermeability, many tarps can qualify for buffer zone credits, allowing reductions in buffer zone size at the application site that minimizes the non-cropped area around a field. After a sufficient interval following fumigation, the tarp may be perforated and plants can be directly seeded or transplanted into it, allowing the tarp to function as a mulch.
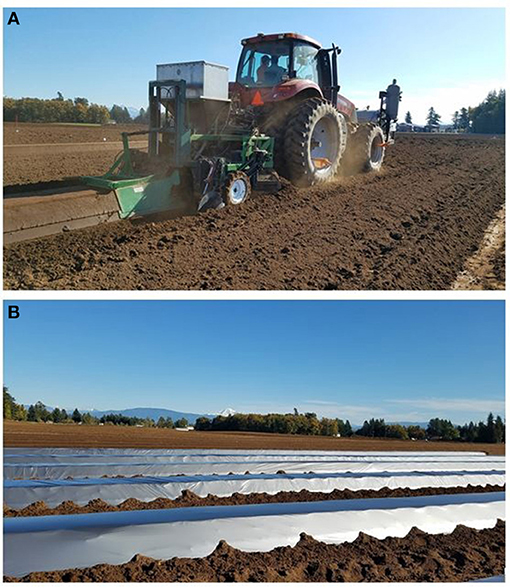
Figure 2. (A) Pre-plant soil fumigation. (B) Tarps applied after soil fumigation to reduce emissions and improve fumigant retention in the soil (photos by Lisa W. DeVetter).
Disadvantages of Polyethylene Mulch
Despite the versatility and various immediate benefits that polyethylene mulch can provide, there are longer term consequences associated with its use that impose negative spillovers onto society and the environment. Polyethylene, the major polymeric component of most plastic mulches, does not degrade readily (Ghatge et al., 2020) and it can take hundreds of years for the polymer structure to undergo physical or chemical changes in the environment (Ohtake et al., 1998). Polyethylene mulch can also adsorb pesticides, concentrating them up to 20 times greater than the soil (Guo et al., 2020), indicating that film fragments could play a role in the distribution, transport, and persistence of pesticides in the environment. Microplastics in the soil may also adhere to plant surfaces (e.g., roots and tubers) (Taylor et al., 2020) or may be taken up by plant roots (Li et al., 2020). These issues raise additional concerns about product quality and health if these plastics are ingested by humans, livestock, and wild animals.
To avoid the accumulation of plastic in soils, polyethylene mulch needs to be removed and disposed of at the end of each growing cycle (Figure 3). However, the mulch removal process can be labor-intensive, expensive, and incomplete. The cost of removal and disposal is dependent upon the amount of plastic that must be removed, the contaminants that adhere to the plastic, as well as how it is removed. Mulch removal may be done mechanically and aided by hand labor or carried out fully by hand. The cost for both scenarios is affected by the availability of labor, wage rate, and how many labor hours are required to complete the task. Operator and manual labor hours related to mulch removal average about 42 h per hectare but vary based on the field's cropping density, type of plastic, and soil type (Velandia et al., 2020a). Additional labor is often required to remove drip tape and polyethylene mulch fragments that may be left in the field. Retrieval of mulch fragments can be difficult and time consuming since the fragments are often small (several square centimeters in size) and at least partially buried in the soil (Goldberger, 2018). Inevitably, not all mulch fragments can be completely removed, and some will remain in the field. If these fragments degrade into micro- and nano-plastics, subsurface transport through soil may be possible (Yu and Flury, 2020).
Transportation of plastic waste to local recycling facilities or a landfill site creates another challenge and additional expense for growers. When calculating the costs of using polyethylene mulch, growers should consider the cost of labor for field removal, cost for transporting the material from a farm to a recycler, and the disposal cost of the polyethylene mulch at a landfill (i.e., gate/tipping fees). Recycling of used polyethylene mulch is not well established in the United States (Moore and Wszelaki, 2016). In addition, the number of recycling facilities that are willing and able to take used polyethylene mulch are limited because the mulch is embossed and subsequently often contaminated with soil particles. Plant debris and pesticide residues are also contaminants found on polyethylene mulches by the end of the season. In many cases, recycling is only an option if the contaminants account for <5% of the total weight of the polyethylene mulch (Clarke, 1996; Steinmetz et al., 2016). However, by the end of the growing season the final weight of most polyethylene mulches can increase by up to 80% due to contamination with soil and plant residues (Ghimire and Miles, 2016). Polyethylene mulches can also contain an array of different resins (beyond polyethylene) that can make downstream recycling processes difficult. Landfill disposal fees are dependent on weight and differ by state or region. A database of municipal solid waste landfills in the United States shows the trends and averages in national and regional tipping fees across six areas. In 2021, it was reported that the average national tipping fee was $54.17 per metric ton, an increase of 0.90% compared to the 2018 tipping fee cost (Environmental Research Education Foundation, 2022).
Fate of Polyethylene Mulch at the End of the Growing Season
Landfilling, stockpiling, and in some cases, in-field burial or burning, are common disposal pathways of used polyethylene mulch in the United States (Figure 4) (Kasirajan and Ngouajio, 2012; Moore and Wszelaki, 2016). Landfilling is the most common disposal method, but the cost and effort to transport polyethylene mulch to a landfill may not be economically viable for all growers. Further, there are negative environmental consequences to placing large amounts of plastic waste into landfills. After burial in a landfill, polyethylene mulch debris, which is frequently embrittled due to exposure to sunlight during its use in the field, will undergo further degradation, producing micro- and nano-plastics that serve as vectors for release of plasticizers and other potential toxicants into the environment. Micro- and nano-plastics can be carried away by wind and water, or taken up by plants (Li et al., 2020; Yu and Flury, 2020). When this occurs, the pollutants can leak into ground and surface water sources (Steinmetz et al., 2016). Stockpiled polyethylene mulch in landfills may also form toxic environmental by-products including aldehydes and ketones (Ohtake et al., 1998; Hakkarainen and Albertsson, 2004).
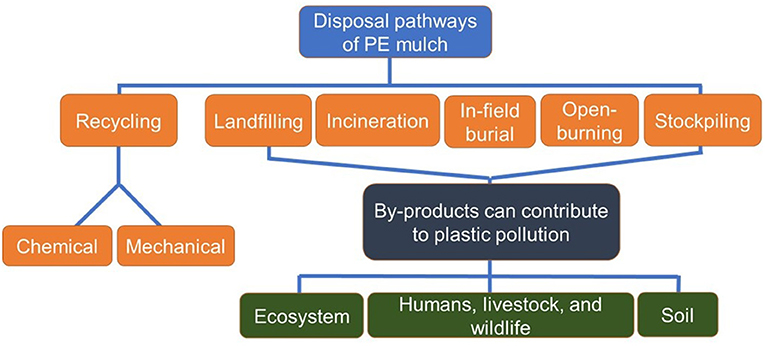
Figure 4. Current end-of-life pathways of used plastic agricultural mulch in the United States (photo image by Brenda Madrid).
On-site stockpiling, burial of polyethylene mulch into field margins, or tillage of the mulch in the field are other disposal methods that growers may practice (Moore and Wszelaki, 2016). These approaches can have undesirable effects on the environment due to the formation of micro- and nano-plastics that can carry adsorbed agrochemicals throughout soils and into adjacent waterways (Kasirajan and Ngouajio, 2012). Research also shows increasing polyethylene mulch residue is associated with increasing concentrations of phthalate acid esters, a potentially carcinogenic and mutagenic compound (Wang et al., 2016). Accumulation of polyethylene mulch and microplastics in soil has been shown to reduce soil health and functioning (Zhang et al., 2020a), including negative effects on earthworms and reductions in soil microbial biomass and functional diversity (Ng et al., 2018). Similar to landfilling, additional concerns arise as polyethylene mulch left in the field can migrate into water resources and become an environmental pollutant in aquatic systems.
Recycling is one sustainable alternative for polyethylene mulch disposal. Mechanical recycling or re-extrusion incorporates closed-loop technologies that can transform used polyethylene mulch by extrusion. In this process the used mulch must first be decontaminated of soil, pesticides, and organic matter residues (Briassoulis et al., 2012). Polyethylene mulches can be mechanically cleaned during field removal with a rotary broom, and mulch removal under relatively dry soil conditions will reduce soil adhesion. However, polyethylene mulch is often embossed with a grid-like pattern to improve the fit of the mulch on soil surfaces under fluctuating temperatures. This in turn can make soil removal very difficult given soil particles adhere more to embossed mulch relative to smooth mulch (G. Jones, Southern Waste Information Exchange, personal communication). Research is needed to refine the best practices for polyethylene mulch cleaning during removal. Once the polyethylene mulch is removed and cleaned, it can be shredded, melted, and re-granulated. The polymer structure of the final product remains the same, and the quality would closely resemble the original product (Horodytska et al., 2018). The recycled material can then be used to make reusable products, such as deck boards (Smith and Wolcott, 2006) and as a partial replacement for asphalt in road resurfacing (Vila-Cortavitarte et al., 2019). Alternatively, polyethylene mulch can be chemically upcycled through processes that include depolymerization, partial oxidation, and pyrolysis, producing valuable chemicals and fuels (Tennakoon et al., 2020; Zhang et al., 2020b). Pyrolysis can provide several benefits over alternative disposal pathways. In pyrolysis, used polyethylene mulch can be degraded at high temperatures between 400 to 1,200°C under anaerobic or low-oxygen conditions (Lee et al., 2021). This facilitates the collection of the individual chemical components that can be used as feedstock to produce new polymers or fuel. Another advantage of this thermochemical process is that it does not require plastic waste to be washed or sorted, which can be costly and laborious (Uzochukwu Eze et al., 2021). Additionally, used polyethylene mulch may be co-pyrolyzed with common organic farm waste to produce biochar, offering a sustainable disposal pathway that may improve the agricultural plastic mulch waste stream (Cisse et al., 2022).
Unfortunately, because collection facilities for agricultural plastics are not widely available, recycling as a disposal method is not accessible nor economically viable for many growers in the United States (Moore and Wszelaki, 2016). Cost to transport polyethylene mulch to a recycling facility in the United States can range from $0.11 to 0.55 per kilogram of plastic (L. Vasquez, Revolution Company, personal communication). Processing contaminated mulch to postconsumer resin adds $0.77–1.21 per kilogram, while transporting the resins from the recycling facility to a manufacturer costs $0.02–0.22 per kilogram. The total postconsumer resin cost is ~$0.90–1.98 per kilogram. In the current market it is not economically viable to recycle polyethylene mulch in the United States, but recycling can be supplemented with subsidies from the government and other entities (L. Vasquez, Revolution Company, personal communication). Another external factor limiting the growth of recycling markets in the United States was the recent Chinese “National Sword” policy enacted in 2018. The National Sword policy banned the importation of plastics for recycling into China, which resulted in reduced demand for agricultural plastic waste in the United States and abroad. Although polyethylene mulch from the United States may not have been directly recycled in China prior to 2018, the National Sword policy indirectly reduced the demand for agricultural plastic waste because recyclers in the United States suddenly experienced a new surplus of less-contaminated plastic waste that is less costly to collect, transport, clean, and recycle. As a result, recycling options for growers are increasingly limited, resulting in the use of less sustainable disposal methods and driving the majority of polyethylene mulch to enter the municipal waste stream through landfill disposal or to be buried or burned on-site. With over 57,152 metric tons of polyethylene mulch used annually in the United States alone (Mancl, 2020), these end-of-life pathways can further contribute to plastic pollution in terrestrial and aquatic systems.
Incineration is another disposal method for used polyethylene mulch and provides a potential opportunity to produce fuel (Moore and Wszelaki, 2016). Plastics contain a comparable fuel value (46,520 kJ/kg) to oil that can be recovered through incineration (Hemphill, 1993; Lamont, 2005; Kasirajan and Ngouajio, 2012). However, similar to recycling, incinerator/power plants that accept polyethylene mulch are limited. Another drawback with incineration is that high-temperature combustion of polyethylene mulch (>1,000°C) can produce carbon monoxide and polycyclic aromatic hydrocarbons (Steinmetz et al., 2016). These emission products are classified as harmful air pollutants that damage the ozone, so they must be contained and then disposed of safely.
Some growers burn polyethylene mulch (and drip tape for irrigation) in open piles. While open burning of polyethylene mulch is illegal in most states, some growers in the Pacific Northwest and Mid-Atlantic report burning their polyethylene mulch waste (Goldberger et al., 2019). Burning plastic at temperatures between 200 and 315°C or less can create carcinogenic polycyclic aromatic hydrocarbons that can be deposited on plants and accumulate in soil (Kasirajan and Ngouajio, 2012; Wortman and Lovell, 2013). Additional toxic substances are emitted in the form of heavy metals, particulates and 1,4-dioxane (Levitan, 2005). On-farm burning leaves behind plastic debris that can cause further environmental problems if the debris enters nearby water channels or is ingested by livestock and wildlife, which can create additional health hazards for humans.
While landfilling is the most common disposal pathway a decrease in available land and their release of hazardous byproducts is a pressing environmental issue (Steinmetz et al., 2016). Incineration may be a viable alternative, however, concerns over harmful emissions from high-temperature combustion render recycling a more sustainable disposal pathway (Steinmetz et al., 2016). Recycling via chemical, mechanical or re-extrusion processes is a desired alternative to managing polyethylene mulch at the end of the growing season. Recycling can improve the agricultural plastic mulch waste stream and would enable the development of reusable products and feedstocks that can be used to create new polymers and fuel. Research to improve and facilitate mulch cleaning prior to and after removal are needed to make recycling more reliable, economically feasible and accessible to growers throughout the United States.
Alternative to Polyethylene Mulch—Soil-Biodegradable Plastic Mulch
Soil-biodegradable plastic mulches may be a sustainable alternative to conventional polyethylene mulch and mitigate environmental health concerns associated with its use and disposal. Soil-biodegradable mulches emerged in the 1990s and provide similar horticultural benefits as plastic mulch made from polyethylene (DeVetter et al., 2017; Ghimire et al., 2018, 2020; Zhang et al., 2020d). However, the widespread adoption of soil-biodegradable mulches has been low due to variable and unpredictable rates of degradation in soils (Kasirajan and Ngouajio, 2012; Sintim and Flury, 2017). The use and mislabeling of photodegradable plastic mulch as biodegradable also negatively impacted the perception of soil-biodegradable mulches at the time, thus limiting its adoption (Kasirajan and Ngouajio, 2012). Recent advances in research and development of biodegradable polymers have enabled the production of new and promising soil-biodegradable mulches. As the global use of plastic mulch continues to rise there is a growing market for soil-biodegradable mulches (Sintim and Flury, 2017). In 2022 the global market for soil-biodegradable mulches was estimated at $62.7 million USD and is projected to continue expanding at a compound annual growth rate of 7.6%, reaching a market of 83.6 million USD by 2026 (Global Industry Analysts, 2022).
Unlike traditional polyethylene mulch they soil-biodegradable mulches are intended to be tilled into the soil at the end of the cropping season (Figure 5) (Hayes et al., 2019; Serrano et al., 2021). After tillage, soil-biodegradable mulch fragments are designed to be biodegraded by microorganisms and converted into microbial cell biomass, carbon dioxide, and water under oxic or aerobic conditions (Kasirajan and Ngouajio, 2012; Hayes et al., 2019). This reduces the amount of plastic waste generated and offsets the negative impacts associated with the traditional disposal pathways (or lack thereof) of polyethylene mulch made from non-biodegradable polymers. It also reduces the labor costs associated with the removal and disposal processes. Therefore, soil-biodegradable mulches have the potential to serve as a more sustainable and economical alternative to polyethylene mulch.
While there are various soil-biodegradable mulches on the market, almost all commercial soil-biodegradable mulches that meet compostability and soil biodegradability standards use one of these feedstocks: (1) ecovio® and ecoflex® [key ingredients: poly(butylene adipate-co-terephthalate) and polylactide] manufactured by BASF (Ludwigshafen, Germany); and (2) Mater-Bi® [key ingredient: poly(butylene adipate-co-terephthalate)] made by Novamont (Novara, Italy). Organix Solutions' Organix A.G. (Edina, Minnesota) and Imaflex Can-Eco (Montreal, Quebec, Canada) are two examples of soil-biodegradable mulches that use ecovio® as the feedstock, and Bio360 (Dubois Agrinovation; Simcoe, Canada) and BioAgri (BioBag Americas; Palm Harbor, Florida) use Mater-Bi®. Product standards have been established to ensure the integrity and performance of soil-biodegradable mulches in cropping systems. The European Standard 17033, issued in 2018, is the first international standard for soil-biodegradable mulches that specifies the requirements and standardized laboratory tests necessary to evaluate composition, biodegradability, and soil ecotoxicity (Hayes and Flury, 2018; Dentzman and Hayes, 2019). The standard also specifies the required dimensional, optical, and mechanical properties for soil-biodegradable mulches. Furthermore, ≥90% biodegradation of the polymeric feedstock must be achieved in aerobic conditions at 20–28°C within 2 years using natural topsoil from an agricultural field or forest site in its standardized laboratory test. American Society for Testing and Materials International (ASTM) has also established the standard American Society for Testing and Materials D6400 that pertains to the biodegradation of soil-biodegradable mulches under industrial composting conditions (Dentzman and Hayes, 2019).
Griffin-LaHue et al. (2022) assessed in-soil degradation of five plastic soil-biodegradable mulches following 4 years of successive application and 2 years after tillage in a Mediterranean climate. Recovered mulch fragments ranged from 4 to 16% by weight of the total mulch mass incorporated in soil demonstrating continuous mulch deterioration. Repeated applications and incorporation did not demonstrate significant accumulation of plastic mulch fragments in soil, however, ≥90% degradation was not achieved within 2 years of tillage as required by EN 17033. Instead modeling field data indicates that thermal time rather than calendar days is more representative of soil-biodegradable mulch degradation under field and laboratory conditions. Given the results, it was predicted that it will take 21–58 months for the soil-biodegradable mulches to attain 90% degradation. This study shows that while laboratory-based assays are necessary, they may not represent the degradability of soil-biodegradable mulches after soil incorporation at a field site (Griffin-LaHue et al., 2022). Additional field test protocols are needed to evaluate soil-biodegradable mulch degradation under diverse soil and environmental conditions to provide site-specific time estimates for complete in-soil degradation.
Socioeconomic Factors Limiting Soil-Biodegradable Plastic Mulch Adoption
The adoption of soil-biodegradable mulches is impacted by socioeconomic factors. Two recent case studies in the United States found that the price of soil-biodegradable mulch relative to polyethylene mulch and labor costs associated with mulch removal had the largest influence on the net profit change for pumpkin growers considering a change from polyethylene mulch to soil-biodegradable mulch (Galinato et al., 2020; Velandia et al., 2020a). Using a 2019 Tennessee fruit and vegetable farmer survey, Velandia et al. (2020b) evaluated farmers' preferences and willingness to pay for soil-biodegradable mulches. Results suggest soil-biodegradable mulch initial price (which may be 2–3 times more expensive than polyethylene mulch), on-farm income, and familiarity with soil-biodegradable mulches were factors influencing farmer adoption. However, results also suggest that producer willingness to pay for soil-biodegradable mulches is below the current soil-biodegradable mulch market prices. Chen et al. (2019) reported that consumers are willing to pay a price premium for products grown with soil-biodegradable mulches, suggesting that it may provide additional revenue opportunities. Specifically, United States consumers were willing to pay 10% above the market price for a 0.5 kg box of strawberries grown with soil-biodegradable mulch. Chen et al. (2020) found that the price premiums for crops grown with soil-biodegradable mulch are more important to farmers than improved soil health or reduced plastic residues in the field, whereas soil health is more of a concern for crop advisors. In addition, growers who were less risk averse and less sensitive to material costs were more willing to adopt soil-biodegradable mulches. Marketable crop yield, which directly affects gross revenues, is another factor to consider when there is a risk of mulch adhesion to the harvested product (Zhang et al., 2020c). Such risks become greater when crops are large and heavy, and the fruit weight rests on top of the mulch (i.e., pumpkin or watermelon).
Jiang et al. (2022) analyzed the application of soil-biodegradable mulches in agricultural production and plastic pollution reduction under both deterministic and stochastic environments. The authors found that soil-biodegradable mulches reduced single-use plastic pollution caused by agricultural production over the long term. However, there was an important tradeoff between soil-biodegradable mulch degradation rates and the functionality as a usable mulch to improve agricultural productivity. Higher crop prices, state imposed minimum wage laws (California Fair Wage Act of 2016, 2015), and landfill tipping fees induced growers to adopt soil-biodegradable mulches. Because higher landfill tipping fees induced substitution to soil-biodegradable mulch, a positive externality arose that reduced plastic waste pollution in farm soils. A corrective tax tended to complement higher landfill tipping fees in achieving greater reduction of plastic waste within the models used in the study (Jiang et al., 2022).
Limitations of Soil-Biodegradable Plastic Mulch
Soil-biodegradable mulches have the potential to be a more sustainable alternative to polyethylene mulch. However, to be a viable alternative, soil-biodegradable mulches should provide similar crop production benefits as polyethylene mulch in terms of durability, weed management, and crop yield and quality enhancement (Miles et al., 2017). Recent work by Tofanelli and Wortman (2020) shows that soil-biodegradable mulches can provide comparable horticultural benefits, but there can be high variability in the function of soil-biodegradable mulches between sites. Complete biodegradation of soil-biodegradable mulch films must also be demonstrated. Current compostability and biodegradation standards employ the use of polymeric feedstocks, which are different from the finished film that has been blended with other ingredients and extruded. The use of isotopic-labeling (13C or 14C) of carbon substrates in soil-biodegradable mulch films can be used to monitor the pathways of carbon in soil and microbial biomass, but its use has been limited to mulch ingredients and not the final film to date (Zumstein et al., 2018; Sander et al., 2019). The degradability of soil-biodegradable mulches in the field is dependent on weathering of the soil-biodegradable mulch, climate, soil type, temperature and moisture, biological activity, tillage practices, and other factors (Chinaglia et al., 2018; Anunciado et al., 2021). As these factors vary widely among sites, there are reports of soil-biodegradable mulch functionality that differs among fields, crop types and years (Li et al., 2014; Sintim, 2018; Sintim et al., 2020; Zhang et al., 2020e).
The application of soil-biodegradable mulches can pose a risk to the environment if mulch fragments do not degrade completely or degrade too slowly in soils. Accumulation of mulch fragments in soils may negatively affect soil health and subsequent crop production (Brodhagen et al., 2017). The fate and residence time of residual micro- and nano-plastic fragments from soil-biodegradable mulch is an additional concern, however, it is difficult to retrieve micro-and nano- particles from soil (Wang et al., 2018). Published literature addressing the effect of micro- and nano-particles is limited. Results from a 4-year study led by Sintim et al. (2021) demonstrate consecutive applications of soil-biodegradable mulch did not have an adverse effect on soil quality compared to polyethylene mulch applications. Further long-term field studies are required to provide a better assessment of the long-term impact of soil biodegradable mulches on soil properties, and quality as well as additional environmental risks. Field studies are also needed to evaluate methods that can be utilized to enhance the degradation rate under soil and environmental conditions that result in slow biodegradation. However, initial research suggests that biostimulants and management practices have limited capacity for accelerating biodegradation of soil-biodegradable mulch in soil (Samuelson, 2019; Thompson et al., 2019).
It is also important to note that soil-biodegradable mulches are not approved for buffer zone reduction credit during pre-plant soil fumigation (DeVetter and Stanghellini, 2020). The United States Environmental Protection Agency (EPA) has a list of approved tarps that have been tested for permeability according to the active ingredients in soil fumigant products. Soil-biodegradable mulches do not meet the qualifications currently, but research is in progress to better understand the permeability and interaction of soil-biodegradable mulches with soil fumigants as well as the potential effect that fumigation may have on soil-biodegradable mulch performance.
Another limitation to the use of soil-biodegradable mulches is that commercially available products cannot be used in certified organic agriculture in the United States, which is different compared to some European countries where it is allowed. This limits the potential for a more environmentally conscience grower demographic to utilize this alternative to conventional polyethylene mulches. Although soil-biodegradable mulches were approved for use in 2014 as an allowed synthetic substance, the mulch must meet other specifications outlined in the United States Department of Agriculture Organic Regulations (7 Code of Federal Regulations, Section 205.601). These criteria specify that a mulch must achieve at least 90% biodegradation within 2 years or less of being incorporated in soil according to one of the following standardized tests: International Organization for Standardization 17556 or ASTM D5988. The soil-biodegradable mulch should also meet the composability specifications outlined by either ASTM D6400, ASTM D6868, European Standards 13432, European Standards 14995, or International Organization for Standardization 17088 (U.S Department of Agriculture, 2015). Furthermore, the mulch must be produced without organisms or feedstock derived from excluded methods and it must be completely biobased [Code of Federal Regulations, Section 205.601 (b)(2)(iii)]. Currently, no commercially available soil-biodegradable plastic mulch meets all the criteria outlined in the organic standard regulation therefore it is recommended to check with an organic certifier before implementing a soil-biodegradable mulch into a certified organic production system.
Conclusion and Future Considerations
Polyethylene mulch is widely used in the United States and worldwide, but current end-of-life disposal pathways are not necessarily sustainable. Current disposal methods can lead to a greater accumulation of non-degradable polyethylene mulch and breakdown products in soil. There is potential for plastic fragments to disperse and pollute nearby ecosystems, imposing direct and indirect costs on society. Recycling via chemical, mechanical or re-extrusion processes can be a more ecologically sound alternative to managing polyethylene mulch at the end of the growing season. Recycling can improve plastic waste management and allow for the development of reusable products and feedstocks that can be used to create new polymers and fuel. The current challenges to recycling polyethylene mulch include reduced demand for recycled product worldwide, economical removal of contaminants adhering to mulch surfaces, and the accessibility and costs of disposal in local areas. Advances in research to improve and facilitate mulch cleaning prior to and after removal are necessary to enable recycling to be more economically feasible. Improving the accessibility of these recycling facilities to growers in all states is also essential. Furthermore, viable policies that promote recycling of conventional plastics should be advanced to provide multiple improved end-of-life pathways for agricultural plastics.
These rising concerns have also renewed interest in the use of soil-biodegradable plastic mulches as an alternative to polyethylene mulch. However, the initial price point of soil-biodegradable mulches is greater than conventional polyethylene mulch and this limits adoption, despite labor-savings during the removal and disposal process and economic studies showing soil-biodegradable mulch use can be economically viable. Additional economic and willingness to pay studies combined with outreach that conveys the potential overall cost savings through reduced labor for mulch removal and disposal should be pursued. Current commercially available soil-biodegradable mulches are also not allowed in organic agriculture in the United States and efforts should be furthered to address the organic standards board's concerns. Degradation of soil-biodegradable mulches in soil is dependent on mulch properties and environmental factors that vary between field sites. Growers are uncertain of the degradation rates of soil-biodegradable mulches at their site, and this further limits their adoption. Future research should investigate the degradability of multiple soil-biodegradable mulches under diverse field conditions, and predictive models for degradation across production scenarios would enable more reliable estimates of degradation in each region. Isotopic labeling is one tool that can aid degradation studies. It is also worthwhile to explore materials that can be applied to soil-biodegradable mulches near or at the end of its field use to enhance the in-soil degradation rate, particularly in environments where biodegradation rates are intrinsically low.
Author Contributions
BM and LD contributed to study conception and design, performed the literature research, and wrote the first draft of the manuscript. All authors contributed to writing—review and editing the draft and read and approved the final manuscript.
Funding
This study was funded by the United States Department of Agriculture National Institute of Food and Agriculture (USDA NIFA) Specialty Crop Research Initiative program (Award Number: 2019-51181-30012) and Washington State Department of Agriculture Specialty Crop Block Grant program (K2525). This work was also supported by the USDA NIFA Hatch projects 1014919, 1017286, and 1014527.
Conflict of Interest
The authors declare that the research was conducted in the absence of any commercial or financial relationships that could be construed as a potential conflict of interest.
Publisher's Note
All claims expressed in this article are solely those of the authors and do not necessarily represent those of their affiliated organizations, or those of the publisher, the editors and the reviewers. Any product that may be evaluated in this article, or claim that may be made by its manufacturer, is not guaranteed or endorsed by the publisher.
Acknowledgments
We would like to acknowledge Brand Karst of BASF and Dan Martens and Sara Guerrini of Novamont for their review. The content of this manuscript appears online as thesis work by BM (Madrid, 2022).
References
Adlerz, W. C., and Everett, P. H. (1968). Aluminum foil and white polyethylene mulches to repel aphids and control watermelon mosaic. J. Econ. Entomol. 61, 1276–1279. doi: 10.1093/jee/61.5.1276
Anunciado, M. B., Hayes, D. G., Astner, A. F., Wadsworth, L. C., Cowan-Banker, C. D., Liquet y Gonzalez, J. E., et al. (2021). Effect of environmental weathering on biodegradation of biodegradable plastic mulch films under ambient soil and composting conditions. J. Polym. Environ. 29, 2916–2931. doi: 10.1007/s10924-021-02088-4
Briassoulis, D., Hiskakis, M., Babou, E., Antiohos, S. K., and Papadi, C. (2012). Experimental investigation of the quality characteristics of agricultural plastic wastes regarding their recycling and energy recovery potential. Waste Mgt. 32, 1075–1090. doi: 10.1016/j.wasman.2012.01.018
Brodhagen, M., Goldberger, J. R., Hayes, D. G., Inglis, D. A., Marsh, T. L., and Miles, C. (2017). Policy considerations for limiting unintended residual plastic in agricultural soils. Environ. Sci. Policy 69, 81–84. doi: 10.1016/j.envsci.2016.12.014
Brown, S. L., and Brown, J. E. (1992). Effect of plastic mulch color and insecticides on thrips populations and damage to tomato. HortTechnology 2, 208–211. doi: 10.21273/HORTTECH.2.2.208
California Fair Wage Act of 2016. (2015). California Attorney General's Office, Initiative 15-0032. Available online at https://www.oag.ca.gov/system/files/initiatives/pdfs/Title%20and%20Summary%20%2815-0032%29.pdf? (accessed June 24, 2022).
Chen, K. J., Galinato, S. P., Marsh, T. L., Tozer, P. R., and Chouinard, H. H. (2020). Willingness to pay for attributes of biodegradable plastic mulches in the agricultural sector. HortTechnology 30, 437–447. doi: 10.21273/HORTTECH04518-20
Chen, K. J., Marsh, T. L., Tozer, P. R., and Galinato, S. P. (2019). Biotechnology to sustainability: consumer preferences for food products grown on biodegradable mulches. Food Res. Intl. 116, 200–210. doi: 10.1016/j.foodres.2018.08.013
Chinaglia, S., Tosin, M., and Degli-Innocenti, F. (2018). Biodegradation rate of biodegradable plastics at molecular level. Polym. Degrad. Stability 147, 237–244. doi: 10.1016/j.polymdegradstab.2017.12.011
Cisse, I., Hernandez-Charpak, Y. D., Diaz, C. A., and Trabold, T. A. (2022). Biochar derived from pyrolysis of common agricultural waste feedstocks and co-pyrolysis with low-density polyethylene mulch film. Waste Biomass Valor. 1–20. doi: 10.1007/s12649-022-01760-7
Clarke, S. P. (1996). Recycling Farm Plastic Films (Original Factsheet). Available online at: http://www.omafra.gov.on.ca/english/engineer/facts/95-019.htm (accessed January 22, 2021).
Dentzman, K., and Hayes, D. (2019). The Role of Standards for Use Biodegradable Plastic Mulches: Truth and Myths. Available online at: https://ag.tennessee.edu/biodegradablemulch/Documents/Standards%20Factsheet%20Formatted%20revised%2015Jan2019.pdf (accessed December 05, 2020).
DeVetter, L. W., and Stanghellini, M. (2020). Soil Fumigation and Biodegradable Plastic Mulch Application. Available online at: https://s3.wp.wsu.edu/uploads/sites/2181/2020/09/Soil-Fumigation-and-Biodegradable-Plastic_Final-for-2020.pdf (accessed December 04, 2020).
DeVetter, L. W., Zhang, H., Ghimire, S., Watkinson, S., and Miles, C. A. (2017). Plastic biodegradable mulches reduce weeds and promote crop growth in day-neutral strawberry in western Washington. HortScience 52, 700–1706. doi: 10.21273/HORTSCI12422-17
Environmental Protection Agency (2020). Tarps. Available online at: https://www.epa.gov/soil-fumigants/tarps/ (accessed December 02, 2020).
Environmental Research Education Foundation (2022). Analysis of MSW Landfill Tipping Fees. Available online at: https://erefdn.org/wp-content/uploads/woocommerce_uploads/2022/02/MSWLF-Tipping-Fees-2021_FINAL-zkhijg.pdf (accessed April 15, 2022).
Galinato, S. P., Velandia, M., and Ghimire, S. (2020). Economic Feasibility of Using Alternative Plastic Mulches: Case Study for Pumpkin in Western WA. Publication TB68E. Available online at: https://pubs.extension.wsu.edu/economic-feasibility-of-using-alternative-plastic-mulches-a-pumpkin-case-study-in-western-washington (accessed February 08, 2021).
Gan, J., Yates, S. R., Becker, J. O., and Wang, D. (1998a). Surface amendment of fertilizer ammonium thiosulfate to reduce methyl bromide emission from soil. Environ. Sci. Technol. 32, 2438–2441. doi: 10.1021/es9707318
Gan, J., Yates, S. R., Wang, D., and Ernst, F. F. (1998b). Effect of application methods on 1,3-D volatilization from soil under controlled conditions. J. Environ. Qual. 27, 432–438. doi: 10.2134/jeq1998.00472425002700020026x
Gao, S., Hanson, B. D., Wang, D., Browne, G. T., Qin, R., Ajwa, H., et al. (2011). Methods evaluated to minimize emissions from preplant soil fumigation. Ca. Agr. 65, 41–46. doi: 10.3733/ca.v065n01p41
Ghatge, S., Yang, Y., Ahn, J. H., and Hur, H. G. (2020). Biodegradation of polyethylene: a brief review. Appl. Biol. Chem. 63, 1–14. doi: 10.1186/s13765-020-00511-3
Ghimire, S., and Miles, C. (2016). Dimensions and Costs of Paper, Polyethylene, and Biodegradable Plastic Mulch. Available online at: http://vegetables.wsu.edu/Dimensions%20%26%20costs%20plastic%20and%20biodegradable%20mulch.pdf/ (accessed March 23, 2021).
Ghimire, S., Scheenstra, E., and Miles, C. A. (2020). Soil-biodegradable mulches for growth, yield, and quality of sweet corn in a mediterranean-type climate. HortScience 55, 317–325. doi: 10.21273/HORTSCI14667-19
Ghimire, S., Wszelaki, A. L., Moore, J. C., Inglis, D. A., and Miles, C. (2018). The use of biodegradable mulches in pie pumpkin crop production in two diverse climates. HortScience 53, 288–294. doi: 10.21273/HORTSCI12630-17
Global Industry Analysts (2022). Global Biodegradable Mulch Film Market to Reach $83.6 Million by 2023. Available online at: https://www.prnewswire.com/news-releases/global-biodegradable-mulch-film-market-to-reach-83-6-million-by-2026–301526421.html (accessed May 06, 2022).
Goldberger, J. R. (2018). AFHVS presidential address: agriculture in the plastic age. Agr. Hum. Val. 35, 899–904. doi: 10.1007/s10460-018-9889-x
Goldberger, J. R., DeVetter, L. W., and Dentzman, K. E. (2019). Polyethylene and biodegradable plastic mulches for strawberry production in the United States: experiences and opinions of growers in three regions. HortTechnology 29, 619–628. doi: 10.21273/HORTTECH04393-19
Griffin-LaHue, D., Ghimire, S., Yu, Y., Scheenstra, E. J., Miles, C. A., and Flury, M. (2022). In-field degradation of soil-biodegradable plastic mulch films in a Mediterranean climate. Sci. Total. Environ. 806, 150238. doi: 10.1016/j.scitotenv.2021.150238
Guo, B., Meng, J., Wang, X., Yin, C., Hao, W., Ma, B., et al. (2020). Quantification of pesticide residues on plastic mulching films in typical farmlands of the North China. Front. Environ. Sci. Eng. 14, 1–10. doi: 10.1007/s11783-019-1181-9
Hakkarainen, M., and Albertsson, A. C. (2004). Environmental degradation of polyethylene. Adv. Polym. Sci.169, 177–200. doi: 10.1007/b13523
Hayes, D. G., Anunciado, M. B., DeBruyn, J. M., Bandopadhyay, S., Schaeffer, S., English, M., et al. (2019). “Biodegradable plastic mulch films for sustainable crop production,” in Polymers for Agri-Food Applications, ed T. Gutiérrez (Cham: Springer Nature Switzerland AG), 183–213.
Hayes, D. G., and Flury, M. (2018). Summary and Assessment of EN 17033: A New Standard for Biodegradable Plastic Mulch Films. Available online at: https://ag.tennessee.edu/biodegradablemulch/Documents/EU%20regs%20factsheet.pdf (accessed March 31, 2021).
Hemphill, D. D. (1993). Agricultural plastics as solid waste: what are the options for disposal? Hort. Tech. 3, 70–73. doi: 10.21273/HORTTECH.3.1.70
Horodytska, O., Valdés, F. J., and Fullana, A. (2018). Plastic flexible films waste management–a state of art review. Waste Mgt. 77, 413–425. doi: 10.1016/j.wasman.2018.04.023
Jiang, J., Marsh, T. L., and Belasco, E. J. (2022). Optimizing Microplastic Pollution in the Soil: A Case of Plastic Mulches (in press).
Johnson, M. S., and Fennimore, S. A. (2005). Weed and crop response to colored plastic mulches in strawberry production. HortScience 40, 1371–1375. doi: 10.21273/HORTSCI.40.5.1371
Kader, M. A., Senge, M., Mojid, M. A., and Ito, K. (2017). Recent advances in mulching materials and methods for modifying soil environment. Soil Tillage Res. 168, 155–166. doi: 10.1016/j.still.2017.01.001
Kasirajan, S., and Ngouajio, M. (2012). Polyethylene and biodegradable mulches for agricultural applications: a review. Agron. Sustain. Dev. 32, 501–529. doi: 10.1007/s13593-011-0068-3
Lamont, W. J. (2005). Plastics: modifying the microclimate for the production of vegetable crops. Hort. Technol. 15, 477–481. doi: 10.21273/HORTTECH.15.3.0477
Lee, N., Joo, J., Lin, K. Y. A., and Lee, J. (2021). Thermochemical conversion of mulching film waste via pyrolysis with the addition of cattle excreta. J. Environ. Chem. Eng. 9, 106362. doi: 10.1016/j.jece.2021.106362
Levitan, L. (2005). Reducing Dioxin Emissions by Recycling Agricultural Plastics: Creating a Viable Alternative to Open Burning. Available online at: https://www.ideals.illinois.edu/bitstream/handle/2142/103280/8-26-0900-Levitan.pdf?sequence=2&isAllowed=y (accessed December 2, 2020).
Li, C., Moore-Kucera, J., Miles, C., Leonas, K., Lee, J., Corbin, A., et al. (2014). Degradation of potentially biodegradable plastic mulch films at three diverse U.S. locations. Agroecol. Sustain. Food Syst. 38, 861–889. doi: 10.1080/21683565.2014.884515
Li, R., Li, L., Zhang, Y., Yang, J., Tu, C., Zhou, Q., et al. (2020). Uptake and accumulation of microplastics in cereal plant wheat. Chinese Sci. Bul. 65, 2120–2127. doi: 10.1360/TB-2020-0030
Madrid, B. (2022). Plastic Mulch Use in Specialty Cropping Systems. (master's thesis). Mount Vernon, WA, Washington State University.
Mancl, K. (2020). Agricultural Plastic in the U.S and China: A Blessing or a Curse? Available online at: https://ocj.com/2020/11/agricultural-plastic-in-the-u-s-and-china-a-blessing-or-a-curse/ (accessed March 15, 2021).
Markets and Markets (2021). Agricultural Films Market by Type (LLDPE, LDPE, Reclaim, EVA, and HDPE), Application ((Greenhouse Film (Alassic Greenhouse, Macro Tunnel), Silage Film (Silage Stretch Wrap), and Mulch Film (Transparent or Clear Mulch)) and Region - Global Forecast to 2026. Available online at: https://www.marketsandmarkets.com/Market-Reports/agricultural-mulch-films-market-741.html/ (accessed June 15, 2021).
Miles, C., DeVetter, L. W., Ghimire, S., and Hayes, D. G. (2017). Suitability of biodegradable plastic mulches for organic and sustainable agricultural production systems. HortScience 52, 10–15. doi: 10.21273/HORTSCI11249-16
Moore, J., and Wszelaki, A. (2016). Plastic Mulch in Fruit and Vegetable Production: Challenges for Disposal. Available online at: https://ag.tennessee.edu/biodegradablemulch/Documents/Plastic%20Mulch%20in%20Fruit%20and%20Vegetable%20Production_12_20factsheet.pdf/ (accessed Jan. 22, 2021).
Ng, E. L., Lwanga, E. H., Eldridge, S. M., Johnston, P., Hu, H. W., Geissen, V., et al. (2018). An overview of microplastic and nanoplastic pollution in agroecosystems. Sci. Total Environ. 627, 1377–1388. doi: 10.1016/j.scitotenv.2018.01.341
Ohtake, Y., Kobayashi, T., Asabe, H., and Murakami, N. (1998). Studies on biodegradation of LDPE-observation of LDPE films scattered in agricultural fields or in garden soil. Polym. Degrad. Stab. 60, 79–84. doi: 10.1016/S0141-3910(97)00032-3
Pittol, M., Tomacheski, D., Simoes, D. N., Ribeiro, V. F., and Santana, R. M. (2017). Antimicrobial performance of thermoplastic elastomers containing zinc pyrithione and silver nanoparticles. Mater. Res. 20, 1266–1273. doi: 10.1590/1980-5373-mr-2017-0137
Samuelson, M. B. (2019). Microbial Response to Biodegradable Mulch: Can Degradation Rate Be Accelerated by Management? (master's thesis). Lincoln, NE, University of Nebraska
Sander, M., Kohler, H. P. E., and McNeill, K. (2019). Assessing the environmental transformation of nanoplastic through 13C–labelled polymers. Nat. Nanotechnol. 14, 301–303. doi: 10.1038/s41565-019-0420-3
Sanders, D., Granberry, D., and Cook, W. P. (1996). Plasticulture for Commercial Vegetables. Available online at: https://content.ces.ncsu.edu/plasticulture-for-commercial vegetables#section_heading_5269/ (accessed February 08, 2021).
Scott, S. J., McLeod, P. J., Montgomery, F. W., and Handler, C. A. (1989). Influence of reflective mulch on incidence of thrips (Thysanoptera: Thripidae: Phlaeothripidae) in staked tomatoes. J. Entomol. Sci. 24, 422–427. doi: 10.18474/0749-8004-24.4.422
Serrano, R. H., Martin-Closas, L., and Pelacho, A. M. (2021). Biodegradable plastic mulches: impact on the agricultural biotic environment. Sci. Total Environ. 750, 141228. doi: 10.1016/j.scitotenv.2020.141228
Sintim, H., Bandopadhyay, S., English, M. E., Bary, A., Liquet y Gonzalez, J. E., DeBruyn, J. M., et al. (2021). Four years of continuous use of soil-biodegradable plastic mulch: impact on soil and groundwater quality. Geoderma 381, 114665. doi: 10.1016/j.geoderma.2020.114665
Sintim, H. Y. (2018). Biodegradable Plastic Mulch: Degradation and Impacts on Soil Health. (PhD dissertation). Mount Vernon, WA, Washington State University.
Sintim, H. Y., Bary, A. I., Hayes, D. G., Wadsworth, L. C., Anunciado, M. B., English, M. E., et al. (2020). In situ degradation of biodegradable plastic mulch films in compost and agricultural soils. Sci. Total Environ. 727, 138668. doi: 10.1016/j.scitotenv.2020.138668
Sintim, H. Y., and Flury, M. (2017). Is biodegradable plastic mulch the solution to agriculture's plastic problem? Environ. Sci. Technol. 51, 1068–1069. doi: 10.1021/acs.est.6b06042
Smith, P. M., and Wolcott, M. P. (2006). Opportunities for wood/natural fiber-plastic composites in residential and industrial applications. For. Prod. J. 56, 4–11.
Steinmetz, Z., Wollmann, C., Schaefer, M., Buchmann, C., David, J., Tröger, J., et al. (2016). Plastic mulching in agriculture. Trading short-term agronomic benefits for long-term soil degradation? Sci. Total Environ. 550:690–705. doi: 10.1016/j.scitotenv.2016.01.153
Taylor, S. E., Pearce, C. I., Sanguinet, K. A., Hu, D., Chrisler, W. B., Kim, Y. M., et al. (2020). Polystyrene nano-and microplastic accumulation at Arabidopsis and wheat root cap cells, but no evidence for uptake into roots. Environ. Sci. Nano. 7, 1942–1953. doi: 10.1039/D0EN00309C
Tennakoon, A., Wu, X., Paterson, A. L., Patnaik, S., Pei, Y., LaPointe, A. M., et al. (2020). Catalytic upcycling of high-density polyethylene via a processive mechanism. Nat. Catal. 3, 893–901. doi: 10.1038/s41929-020-00519-4
Thompson, A. A., Samuelson, M. B., Kadoma, I., Soto-Cantu, E., Drijber, R., and Wortman, S. E. (2019). Degradation rate of bio-based agricultural mulch is influenced by mulch composition and biostimulant application. J. Polym. Environ. 27, 498–509. doi: 10.1007/s10924-019-01371-9
Tofanelli, M. B. D., and Wortman, S. E. (2020). Benchmarking the agronomic performance of biodegradable mulches against polyethylene mulch film: a meta-analysis. Agron. 10, 1618. doi: 10.3390/agronomy10101618
U.S Department of Agriculture (2015). Memorandum to the National Organic Standards Board. Available online at: https://www.ams.usda.gov/sites/default/files/media/NOSB%20Biobased%20Mulch%20Film%20-%20FINAL.pdf (accessed July 01, 2021).
Uzochukwu Eze, W., Umunakwe, R., Obasi, H. C, Ugbaja, M. I., Uche, C. C., and Madufor, I. C. (2021). Plastic waste management: a review of pyrolysis technology. Clean Technol. Recycl. 1, 50–69. doi: 10.3934/ctr.2021003
Velandia, M., Galinato, S. P., and Wszelaki, A. (2020a). Economic evaluation of biodegradable plastic films in Tennessee pumpkin production. Agronomy 10, 51. doi: 10.3390/agronomy10010051
Velandia, M., Jensen, K., DeLong, K. L., Wszelaki, A., and Rihne, A. (2020b). Tennessee fruit and vegetable farmer preferences and willingness to pay for plastic biodegradable mulch. J. Food Distrib. Res. 51, 63–87.
Vila-Cortavitarte, M., Lastra-Gonzales, P., Calzada-Perez, M. A., and Indacoechea-Vega, I. (2019). “The use of recycled plastic as partial replacement of bitumen in asphalt concrete,” in Use of Recycled Plastics in Eco-Efficient Concrete, eds F. Pacheco-Torgal, J. Khatib, and R. Tuladhar (Sawston: Woodhead Publishing), 327–347.
Wang, J., Lv, S., Zhang, M., Chen, G., Zhu, T., Zhang, S., et al. (2016). Effects of plastic film residues on occurrence of phthalates and microbial activity in soils. Chemosphere 151, 171–177. doi: 10.1016/j.chemosphere.2016.02.076
Wang, Z., Taylor, S. E., Sharma, P., and Flury, M. (2018). Poor extraction efficiencies of polystyrene nano- and microplastics from biosolids and soil. PLoS ONE 13, e0208009. doi: 10.1371/journal.pone.0208009
Wolfenbarger, D. O., and Moore, W. D. (1968). Insect abundance on tomatoes and squash mulched with aluminum and plastic sheetings. J. Econ. Entomol. 61, 34–61. doi: 10.1093/jee/61.1.34
Wortman, S., and Lovell, S. T. (2013). Environmental challenges threatening the growth of urban agriculture in the United States. J. Environ. Qual. 42, 1283–1294. doi: 10.2134/jeq2013.01.0031
Yates, S. R., Gan, J., Papiernik, S. K., Dungan, R. S., and Wang, D. (2002). Reducing fumigant emissions after soil application. Phytopathology 92, 1344–1384. doi: 10.1094/PHYTO.2002.92.12.1344
Yu, Y., and Flury, M. (2020). Current understanding of subsurface transport of micro- and nanoplastics in soil. Vadose Zone J. 20, 20108. doi: 10.1002/vzj2.20108
Zhang, D., Ng, E. L., Hu, W., Wang, H., Galaviz, P., Yang, H., et al. (2020a). Plastic pollution in croplands threatens long-term food security. Glob. Chang. Biol. 26, 3356–3367. doi: 10.1111/gcb.15043
Zhang, F., Zeng, M., Yappert, R. D., Sun, J., Lee, Y., LaPointe, A. M., et al. (2020b). Polyethylene upcycling to long-chain alkylaromatics by tandem hydrogenoly-sis/aromatization. Science 370, 437–441. doi: 10.1126/science.abc5441
Zhang, H., DeVetter, L. W., Scheenstra, E., and Miles, C. (2020c). Weed pressure, yield, and adhesion of soil-biodegradable mulches with pie pumpkin (Cucurbita pepo). HortScience 55, 1014–1021. doi: 10.21273/HORTSCI15017-20
Zhang, H., Flury, M., Miles, C., Liu, H., and DeVetter, L. W. (2020e). Soil-biodegradable plastic mulches undergo minimal in-soil degradation in a perennial raspberry system after 18 months. Horticulture 63, 47. doi: 10.3390/horticulturae6030047
Zhang, H., Miles, C., Gerderman, B., LaHue, D. G., and DeVetter, L. W. (2021). Plastic mulch use in perennial fruit cropping systems- a review. Sci. Hort. 109975. doi: 10.1016/j.scienta.2021.109975
Zhang, H., Miles, C., Ghimire, S., Benedict, C., Zasada, I., Liu, H., et al. (2020d). Plastic mulches improved plant growth and suppressed weeds in late summer-planted floricane-fruiting raspberry. HortScience 55, 565–572. doi: 10.21273/HORTSCI14734-19
Keywords: agricultural mulch, biodegradable mulch, plasticulture, microplastic, recycling, plastic waste, plastic pollution, polyethylene
Citation: Madrid B, Wortman S, Hayes DG, DeBruyn JM, Miles C, Flury M, Marsh TL, Galinato SP, Englund K, Agehara S and DeVetter LW (2022) End-of-Life Management Options for Agricultural Mulch Films in the United States—A Review. Front. Sustain. Food Syst. 6:921496. doi: 10.3389/fsufs.2022.921496
Received: 15 April 2022; Accepted: 20 June 2022;
Published: 08 July 2022.
Edited by:
Matias B. Vanotti, United States Department of Agriculture, United StatesReviewed by:
Teresa Gea, Universitat Autònoma de Barcelona, SpainSaowapa Chaiwong, Mae Fah Luang University, Thailand
Copyright © 2022 Madrid, Wortman, Hayes, DeBruyn, Miles, Flury, Marsh, Galinato, Englund, Agehara and DeVetter. This is an open-access article distributed under the terms of the Creative Commons Attribution License (CC BY). The use, distribution or reproduction in other forums is permitted, provided the original author(s) and the copyright owner(s) are credited and that the original publication in this journal is cited, in accordance with accepted academic practice. No use, distribution or reproduction is permitted which does not comply with these terms.
*Correspondence: Lisa Wasko DeVetter, bGlzYS5kZXZldHRlciYjeDAwMDQwO3dzdS5lZHU=
†These authors have contributed equally to this work