- 1Grupo CIDE Competitividad Innovación y Desarrollo Empresarial, Facultad de Ciencias Agropecuarias, Universidad Pedagógica y Tecnológica de Colombia, Tunja, Colombia
- 2Grupo de Química - Física Molecular y Modelamiento Computacional QUIMOL, Facultad de Ciencias, Universidad Pedagógica y Tecnológica de Colombia, Tunja, Colombia
Exploring yield, phenology and their relationship with secondary metabolites in seeds provides a fundamental analysis that expands knowledge on the nutritional quality of seeds and the effect on productive potential. This knowledge is fundamental when improving or selecting nutritionally important crops, including Chenopodium quinoa Willd, which has excellent nutritional properties and contributes to global food security. This species contains saponins, a metabolite that imparts a bitter taste and can be highly toxic to consumers in large quantities. Therefore, the identification and selection of genotypes according to their saponin contents and outstanding agronomic characteristics are fundamental objectives for the genetic improvement programs of these species. Therefore, the objective of this research was to evaluate the characteristics of the grain, the phenology and the saponin content of 30 C. quinoa accessions with an aim to select or relate genotypes according to their yield and grain quality. The accessions were sown using randomized complete blocks (RCB) with nine repetitions for each material. Seven FAO-defined descriptors were evaluated to characterize the grain and physiological maturity. Saponin was extracted using microwave, and the quantification was done with high-performance liquid chromatography (HPLC) which a UV-VIS detector at 277 nm wavelength. The accessions were classified according to their phenology: semi-late (56.7%), late (36.7%), and semi-early (3.3%). The total triterpene saponin content varied from 0.018 to 0.537%. The multivariate and cluster analyses formed groups of accessions with good yields (>62.02 g of seeds per plant) and desirable grain morphological characteristics. The more suitable accessions for the production of saponins are Quinoa semiamarga (0.537%), Quinoa peruana (0.412%) and Amarilla de maranganí (0.305%). Quinoa real and Quinoa primavera are more suitable for food products, which can be used as parents in future quinoa genetic improvement programs in Colombia.
Introduction
The cultivation of quinoa has generated great interest in consumers outside the Andean region, producing significant changes in the global distribution of quinoa, now it is present in more than 120 countries around the world (Alandia et al., 2020). This new scenario has rapidly increased the demand and price of quinoa in the main producing countries. Some challenges for this crop are keeping yields stable, generate technologies that make harvest, post-harvest and transformation processes of quinoa-based by products more efficient, and apply clear rules regarding the commercialization and the benefits of conserving Andean genetic resources. Quinoa represents a good crop for global food security and an opportunity to produce high-quality protein with little impact on the environment (De Bock et al., 2021).
Thus, quinoa (Chenopodium quinoa Willd.) has been recognized internationally for its high nutritional quality because of the ideal balance between lipids (5.5–7.4%), proteins (13.1–16.7%) and carbohydrates (59.9–74.7%) (Villacrés et al., 2022). Thanks to broad genetic variability, it has adapted to different environmental conditions, from sea level to an altitude of more than 4,000 m, and from cold highland climates to subtropical conditions (Fagandini et al., 2021). In addition, quinoa tolerates different types of stress such as salinity, drought, frost, different types of soil pH, and different phytosanitary problems, among others (Naheed et al., 2022; Tovar et al., 2022). This has allowed for the selection, multiplication and adaptation of different cultivars to a wide range of environmental conditions such as acidic or alkaline soils, humid or arid areas, and cold or warm environments (Hussain et al., 2020).
When introducing quinoa genotypes to new regions, the sensitivity to photoperiods and the unalterable phenological cycle must be considered (Granado et al., 2021a), because studies show that high temperatures and long days during the reproductive stage when grain filling occurs, this period can considerably reduce yield, especially when the increase in temperature and changes in the photoperiod occur in the initial stages after pollination (Lu et al., 2016; Fan et al., 2020; Yao et al., 2020).
Quinoa has multiple uses, including human consumption, where the grain is used in the preparation of soups, salads, cereals, alcohol and flour (Alizadeh et al., 2022). It is also used for medicinal purposes (anti-inflammatory, analgesic and disinfectant), in animal feed, and as an insect repellent (Asher et al., 2020; Isam et al., 2021). However, it contains molecules that react with nutrients, thus interfering with their absorption, known as antinutrients, including saponins, phytic acids, oxalates, tannins, and trypsin inhibitors (Pathan and Siddiqui, 2022). Saponins are secondary metabolites found mainly in the outer layer of grains giving them a bitter taste and also generate a hemolytic action on red blood cells (El Hazzam et al., 2020). Quinoa grains contain between 0.00 and 4.4% saponins, which are found in the leaves, shoots and flowers (Lim et al., 2020). The concentration of saponins in quinoa seeds depends on the genotype. Thus, quinoa genotypes have been classified as sweet when they have < 0.11% saponins and as bitter with >0.11% (León et al., 2019). Lin et al. (2019) reported different saponin contents in diverse parts of the quinoa plant, such as leaves (0.97%), grains (1.26%), shoots (1.29%), stems (3.67%), and branches (8.34%). Different factors such as location, abiotic stress like drought and salinity, and variety influence the content of saponins (Granado et al., 2021a). Furthermore, the saponins have been reported to exhibit biological properties such as anticancer, antiobesity, free radical scavenging, and heart disease reduction (El Hazzam et al., 2020).
Quinoa has two saponin types: triterpenic and steroidal, which may vary according to the number and position of the units of sugars attached to the hydrophobic aglycone (Stoleru et al., 2022). The triterpene saponins in quinoa consist of a hydrophilic oligosaccharide bound to a hydrophobic aglycone that is derived from oleanolic acid, hederagenin, phytolaccagenic acid, or serkhanic acid (Ng and Wang, 2021; Villacrés et al., 2022). The study of saponins and quinoa seeds requires the use of advanced methodologies for extraction and quantification. A widely used methodology is microwave-assisted extraction, which is an eco-friendly technology and involves less dangerous chemical reaction, safer chemicals, energy efficiency and the use of renewable raw materials (Colson et al., 2020). Quantification uses high-resolution liquid chromatography (HPLC), which is a versatile and reliable methodology for the quantification of compounds, due to the automation of the equipment, the development of detectors, columns with high sensitivity, and treatment systems to purify samples, which guarantees to determine the saponin content of the quinoa variety (Han et al., 2019). Sweet quinoa is exclusively used as a food product. If it is bitter, it can also be used as food but only with desaponification or for its biological properties of insecticide, antibiotic, antitumor, fungicide, hemolytic and anti-inflammatory in drugs (Rafik et al., 2021; El Hazam et al., 2020).
Therefore, the characterization of saponins will allow the selection of varieties for food and biotechnological use with a view to a better use of the productive potential of quinoa within conservation and genetic improvement programs (Morillo et al., 2020). The objective of this study was to evaluate the characteristics of the grain and the phenology and quantify saponins in thirty accessions of C. quinoa to select outstanding genotypes in the seed collection of the Department of Boyacá-Colombia in terms of the secondary metabolite.
Materials and methods
Plant material
Thirty quinoa (C. quinoa) accessions from the seed collection of the Department of Boyacá (Table 1) were evaluated during the months of May to December 2021. The study was conducted in Tunja-Boyacá Colombia located at an altitude of 2.820 masl, the climatic conditions of the study region were typical of tropical zones; the daily lighting period during the experiment was ~12 h. The minimum temperature during the research ranged between 7.43 and 10.00°C, and the maximum was between 17.80 and 20.00°C, with an average temperature between 13.43 and 14.75°C. The monthly rainfall was < 2 mm, and the average relative humidity was 78%. A Randomized Complete Block Design (RCBD) was used with three replications for each accession, with an experimental unit of nine plants (n = 9). The agronomic management of the trial was in a conventional way as done by farmers. The harvest was done manually at physiological maturity.
Grain characterization and quantification of total triterpene saponins
For the characterization of the grain and the yield of the plants, seven descriptors were used, which were defined by the FAO for quinoa and its wild relatives (Bioversity International, 2013) which have already been evaluated in other studies of morpho-agronomic characterization (Manjarres et al., 2021). Measurements were taken on nine individuals of each accession. Four qualitative variables were evaluated: grain shape (GS) that was lenticular, cylindrical, conical or ellipsoidal, smooth, wavy, or intermediate grain edge (GE), and color of the perigonium and episperm (CP and CE respectively). The quantitative descriptors evaluated were grain diameter (GD), weight of 1,000 seeds (WS) and yield (Y). The days of physiological maturity (from sowing until more than 50% of the plants have mealy grains) and the concentration of saponins (Table 2) were determined.
For the estimation of the concentration of saponins in the grain, microwave-assisted extraction was done with an MDS-6G (SMART) Closed Microwave Digestion/Extraction System of Shanghai SINEO Microwave Chemistry Technology Co. device, where 4.0 g of seeds were placed in 50 mL of 70% ethanol v/v alcohol, at 600 Watts, with 7 irradiation cycles of 16 min at 70°C; finally, the triterpene saponins were purified with crystallization. For the purification of the obtained extracts, an ethanolic solution at 50% of H2O was used; the homogenized mixtures were heated on a plate at 45°C, with continuous agitation for 4 h. Then, they were vacuum filtered through polyamide membrane filters, and each extract was centrifuged at 2,500 rpm for 20 min. The supernatant were cooled to 15°C by adding 1.4 g of CaCl2, the pH was adjusted to 7, and centrifuged again at 3,000 rpm for 30 min. Then, the extract was concentrated in a rotary evaporator at 70 mbar vacuum, 110 rpm and 42°C (Villacis, 2018).
In the final stage of purification, 0.08 mg of each extract was taken and transferred to a 5 mL flask, then mixed with 5 mL of n-butanol in a shaker for 30 min, at the end a volume that varied between 3 and 5 mL of saponin extract. To obtain the organic and clear phase, the samples were centrifuged at 2,000 rpm for 15 min and the organic phase (upper phase) was extracted from the tubes with a syringe.
Quantification by high-resolution liquid chromatography (HPLC) was performed on a SHIMADZU HPLC equipment, model LC-2030, which has a UV-VIS detector, in this case the wavelength used was 277 nm. The equipment has AutoSampler, Waters Spherisorb S5W column (modified silica with hydrophobic functions such as octadecylsilane) (25 cm × 4.6 mm, 5 μm) maintained at 35°C. The mobile phase was in isocratic mode, with 10% water and 90% acetonitrile. Saponin extraction was performed for each accession considering the calibration curve, which was performed with a standard solution of oleanolic acid, correlating the peak area with the concentration. The calibration curve was made with an analytical standard of oleanolic acid ≥98.8% from the commercial company Sigma Aldrich, through eight concentrations analyzed vs. mAu (milli-absorbance units). These concentrations were: 1, 3, 5, 10, 20, 30, 50, 70, and 100 ppm, which were prepared in HPLC grade ethanol and filtered through 0.2 μm syringe filter Whatman™ Puradisc. Subsequently, they were passed in vials to the HPLC equipment with a verified baseline and an established method for quantification, carrying out a pretreatment and purification of the same by the equipment, then the automatic injection of 25 μL per sample was carried out, with a run time of 25 min and a time of analyte retention between 13.54 and 14.01 min for the standard and for the analyte can be seen in the Supplementary Figures 1, 2. This procedure was performed in triplicate to standardize the chromatographic method. To determine the detection limit, solutions with minimum concentrations of the standard (0.05, 0.1, 0.3, 0.5, 0.8, and 0.9 ppm) were used, each injected in triplicate.
With these concentrations, a calibration curve was made and the average areas were extended, and the LOD was evaluated as the lowest content that can be measured with reasonable statistical certainty. Or the lowest analyte content, which was detected and could be identified (Table 3). The detection limit will be extended by six times the lowest concentration of the oleanolic acid standard (0.004 g/L). For its calculation, the critical value was taken with a confidence level of 95%, with a degree of freedom of 2. While the LOQ was evaluated as the lowest concentration of the analyte that can be determined with an acceptable level of precision, repeatability, and trueness. The quantification limit was determined with the standard deviation found in the detection limit multiplied by 10. For repeatability, six runs of the sapogenin standard (oleanolic acid) were carried out, taking the aliquot with the lowest concentration (0.004 g/L), with the same analyst, the same equipment, and during the same day. This means that the minimum analyte concentration that can be detected with the method is 0.23 ppm and the minimum quantifiable amount is 0.84 ppm. The saponin content in each of the samples was estimated based on their dry weight (d.w.).
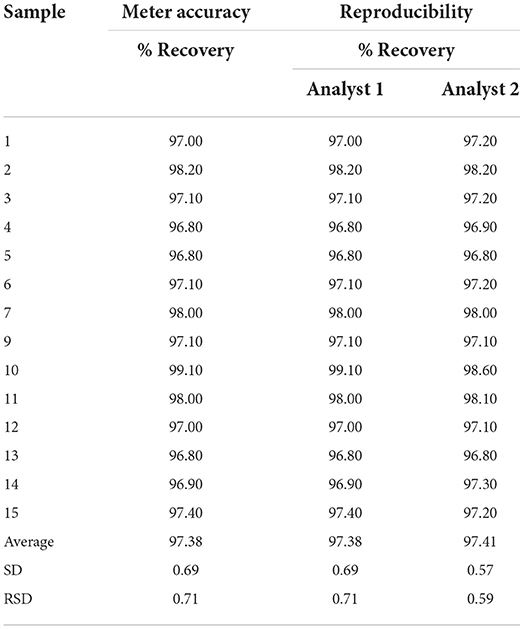
Table 3. Recovery percentage and reproducibility of 15 samples for validation of high-performance liquid chromatography (HPLC).
Statistical analysis
For quantitative variables, a descriptive analysis was carried out. The assumptions for parametric analyses were then verified using R Core Team (2020) and the missMDA package (Josse and Husson, 2016). Spearman's correlation was estimated and plotted using R package “corrplot”: Visualization of a Correlation Matrix (Version 0.84) (Taiyun and Simko, 2017). For the multivariate analysis, a hierarchical grouping in the principal components (HCPC) was carried out with the algorithms in the factoextra package of the R program (Kassambara and Mundt, 2020), which were plotted on a two-dimensional plane using the FactoMineR package (Le et al., 2008).
For qualitative variables, a frequency analysis was performed in the Infostat program (Di Rienzo et al., 2020). The set of qualitative and quantitative variables was used for a factor analysis of mixed data with the factoextra package of the R program. Finally, a dendrogram was made using the Euclidean distance and the hierarchical grouping method with the Ward minimum variance with the FactoMineR package (Le et al., 2008) in the R program (Team, 2020).
Results
The phenological cycle of the 30 accessions was between 144 and 189 days (Table 4), except for Quinoa beteitiva, which did not produce grain. The accessions were classified according to Tapia et al. (2014) as: semi-late (56.7%), with a cycle between 150 and 180 days; late (36.7%); with cycles >180 days; or semi-early (3.3%), with a cycle < 144 days. The material with the shortest cycle was Quinoa real, with 144 days, followed by Quinoa primavera with 157 days; on the contrary, the longest cycle was seen in Quinoa siachoque and Quinoa piartal, with 187 and 189 days, respectively.
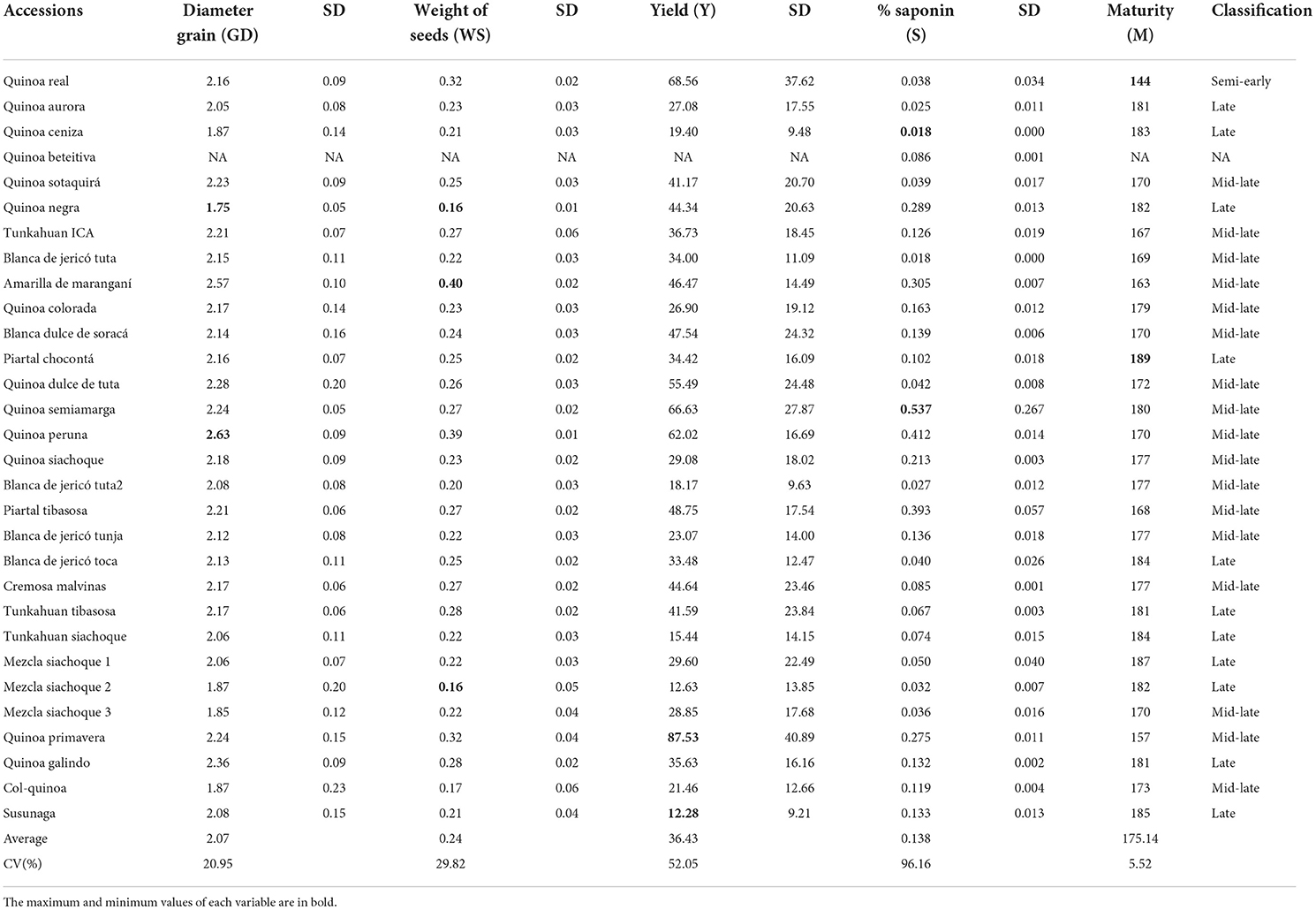
Table 4. Averages, coefficients of variation and standard deviation (SD) for the quantitative variables evaluated in the quinoa accessions.
The saponin content was between 0.018 and 0.537%. The accessions classified as sweet were 76.7%, while 23.3% were bitter, which was the most variable quantitative descriptor with a coefficient of variation of 96.16%. The accession with the highest amount of saponins was Quinoa semiamarga with 0.537%, followed by Quinoa peruana with 0.412%, while the accession with the lowest percentage of saponins was Quinoa ceniza with 0.018%. The diameter of the seeds ranged from 1.75 to 2.63 mm and presented a coefficient of variation of 20.95%.
The material with the highest yield was Quinoa primavera, Y = 87.53 g of seed per plant, while Quinoa susunaga obtained the lowest yield with Y = 12.28 g of seed per plant. Quinoa negra had the lowest value for WS = 0.16 g and GD = 1.75 mm (Table 4). There were statistically significant differences (p < 0.05) (Table 5) in yield, saponin content, weight and diameter of the seeds among the evaluated quinoa accessions.
The seeds of the 30 quinoa accessions had differences in the colorations of the perigonium and episperm. In addition, the accessions had intravarietal variations. The color of the perigonium presented orange, black, brown and beige variations. The color of the episperm was also variable; 69% of the plants had a transparent episperm, 13% were white, 7% were black, and 6% were beige. Four types of grain shapes were observed: cylindrical (83%), ellipsoidal (7%) lenticular (6%), and conical (4%). As for the edge of the grain, 48% of the plants had a wavy edge, 26% were smooth, and 21% were intermediate (Table 6). Spearman's correlation analysis (p ≤ 0.05) showed that the quantitative variables were high and had significant correlations between weight and diameter of the seed (r = 0.88), seed weight and yield (r = 0.80), seed diameter and yield (r = 0.68), and diameter of the seed and saponin content (r = 0.50), along with high and negative correlations between yield and physiological maturity (r = −0.57) (Figure 1).
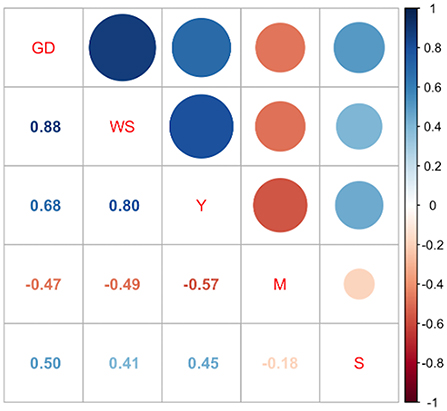
Figure 1. Spearman correlation analysis between quantitative variables. Footnote: GD grain diameter, WS weight of 1,000 seeds, Y yield, M days to physiological maturity, S concentration of triterpene saponins.
The principal component analysis showed that 80. 2% of the total variance was explained by the first two components (CP1 = 62.5% and CP2 = 17.7%); this analysis did not consider Quinoa beteitiva because it did not present grains. The variables with greater contribution to the variation of CP1 included weight of 1,000 grains, yield, seed diameter and saponin concentration. While days to physiological maturity contributed the most to CP2. On the other hand, the variables associated with yield correlated with the saponin concentration (Figure 2). In the graphical representation of the main components, it was observed that the materials were distributed in two groups. Group 1 had the accessions with good yields (46.47 and 87.53 g of seeds per plant) and high concentrations of saponins (0.275 and 0.537%). Group 2 had the sweet accessions since they had concentrations of saponins < 0.11% and semi-late physiological maturity. Finally, Quinoa real was not in these two groups because the intrinsic characteristics of this accession include being very early with physiological maturity at 144 days.
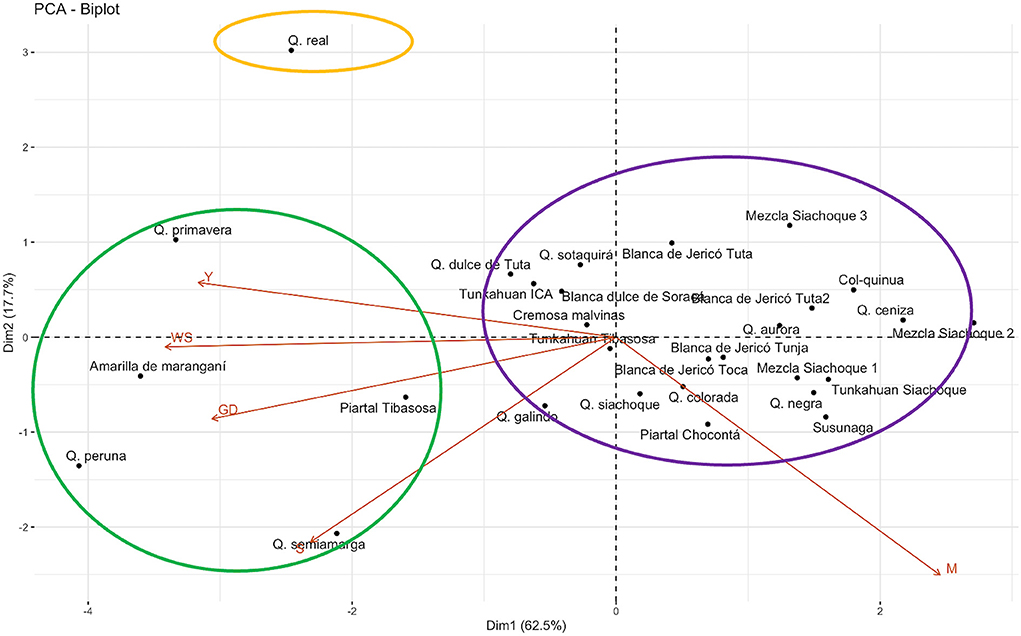
Figure 2. Biplot of the analysis of main components. The variables that contributed to yield were weight and diameter of the seeds. Footnote: GD grain diameter, WS weight of 1,000 seeds, Y yield, M days to physiological maturity, S concentration of triterpene saponins.
The analysis of mixed factors differentiated the accessions mainly by grain characteristics, physiological maturity and saponin content. This analysis showed that the contribution of the variables to the first two components was 44.28%. The variables that contributed positively to the variation of CP1 (27.64%) were physiological maturity (M), weight and diameter of the seeds (WS and GD), and grain edge (GE) (Figure 3). While for CP2 (16.64%), the variables were yield (Y), amount of saponins (S), beige perigonium, transparent episperm and cylindrical grain.
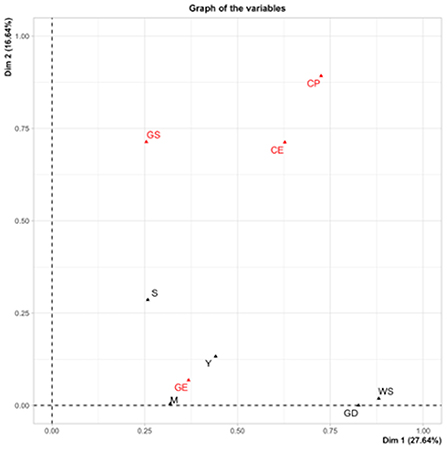
Figure 3. Mixed factor analysis, contribution of the variables that ordered the accessions according to the qualitative and quantitative variables.
The cluster analysis, which considered the qualitative and quantitative variables, formed eight clusters that were not related to the collection area or place of origin of the accessions. The first and second groups had the Quinoa negra and Quinoa ceniza, which had the lowest average grain diameter and weight of 1,000 seeds, 1.81 mm and 0.18 g, respectively. The third group had Amarilla de maranganí and Quinoa peruana, which had a bitter taste with values >0.412% saponins in their seeds, orange grains, cylindrical shape and wavy edge, white episperm, and the greatest weight of 1,000 grains at 0.40 g. Quinoa blanca de jericó from Tunja and Quinoa siachoque formed group four, which had a seed diameter of 2.15 mm, average yield of 26.07 g of seed per plant, cylindrical grain shape, wavy grain edge, and 177 days at physiological maturity. The fifth group had semi-late accessions, sweet quinoa with percentages of saponins < 0.11%, yields between 12.28 and 28.85 g of seed per plant, and average grain weight and diameter of 0.19 g and 1.92 mm, respectively. The sixth group was characterized by accessions that were sweet, except the accession Piartal tibasosa, which had 0.39% saponins, average yield of 42.11 g of seed per plant, intermediate edge and wavy grain, cylindrical grain, and days to physiological maturity between 168 and 184. Group seven had Quinoa real, which was earlier than the other materials with 144 days at physiological maturity, had 68.56 g of seeds per plant and was sweet. Quinoa primavera had the highest yield with 87.53 g of seed per plant, grain diameter and weight of 2.24 mm and 0.32 g, respectively, cylindrical grains, and transparent episperm. Finally, group eight had 13 accessions that presented yields between 15.44 and 36.06 g of seed per plant, average weight of 1,000 grains and seed diameter of 0.24 g and 2.17 mm, respectively, phenologically semi-late material, beige grains, and cylindrical shapes (Figure 4). These results were consistent with the principal component analysis.
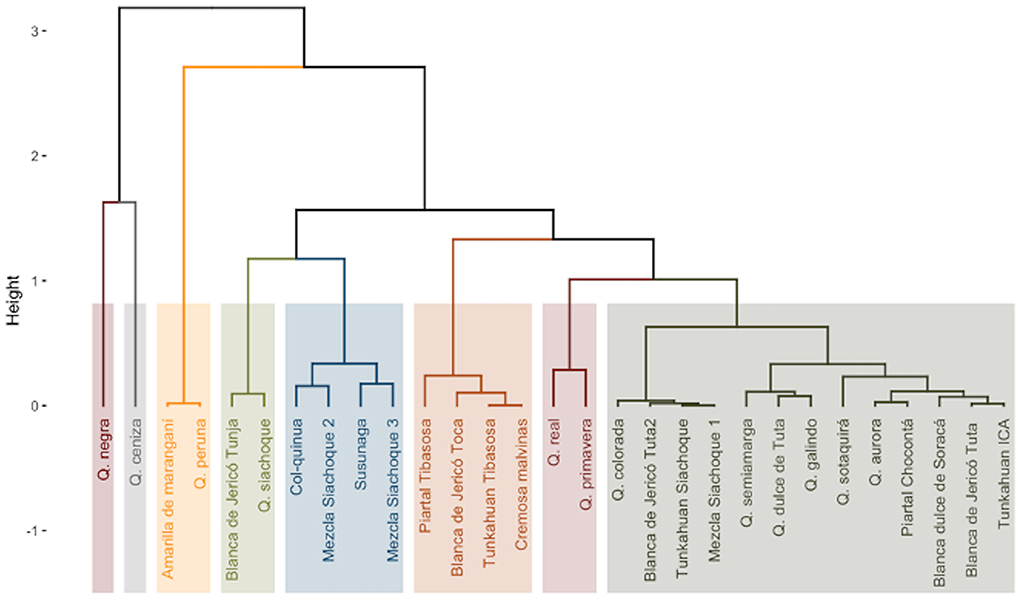
Figure 4. Cluster analysis, eight groups of accessions formed according to the qualitative and quantitative variables evaluated in 30 quinoa accessions. Footnote: GD grain diameter, WS weight of 1,000 seeds, Y yield, M days to physiological maturity, S concentration of triterpene saponins, GS grain shape, GE grain edge, CE grain edge, CP color of the perigonium.
Discussion
Quinoa (C. quinoa) has great adaptability to extreme conditions with alternative metabolic plasticity (Bazihizina et al., 2022). This change is induced by conditions such as temperature, light intensity, nutritional status, relative humidity, and water availability (Morales et al., 2017). In addition, it is capable of undergoing phenological, morphological and physiological changes known as phenotypic plasticity (García et al., 2020). The life cycle of the accessions studied was ~6 months under the conditions evaluated. In cereal crops and pseudocereals such as quinoa, the critical stage for grain yield and quality occurs during the reproductive stage when grain filling occurs (Granado et al., 2021a,b; Matías et al., 2021). Studies have shown that high temperatures and long days during this period can considerably reduce yield, especially when temperatures rise and changes in the photoperiod occur in the initial stages after pollination (Lesjak and Calderini, 2017; Fan et al., 2020; Yao et al., 2020). In the study region, no temperature increases were observed during grain formation. The photoperiod was ~12/12; however, it is important to bear in mind that variable behaviors in the phenology of quinoa accessions depend largely on adaptability and phenotypic plasticity, which influence the productive cycles of each accession (Bonifacio, 2019).
The high nutritional value of quinoa increases its interest worldwide, especially as a crop that could be included in different production systems; however, climate change in particular challenges this and other cultivated species. Currently, there is only limited knowledge on grain yield and other key characteristics that respond, for example, to high temperature conditions (Matías et al., 2021; Tovar et al., 2022). Lesjak and Calderini (2017) evaluated the effect of increased night temperature on yield, number and weight of grains and the processes involved in quinoa growth under nocturnal thermal stress in flowering and grain filling in southern Chile. Aboveground biomass was adversely affected by thermal treatments. The number of grains was significantly affected between treatments and this characteristic correlated positively with grain yield. Little change was found in harvest index, individual grain weight, grain protein content, and water-soluble carbohydrates in response to rising nighttime temperature in this crop.
Saponins are secondary metabolites found in the pericarp of quinoa seeds, which give it a bitter taste when present in substantial amounts. They also have a negative effect on the bioavailability of minerals such as Fe and Zn (Pathan and Siddiqui, 2022). For these two reasons, saponins are considered “antinutrients.” Different breeding programs have focused their efforts on the identification and selection of cultivars with a very low saponin content in the seeds (sweet quinoa cultivars) (Alandia et al., 2020). Koziol (1992) established the limit between sweet seeds and bitter seeds at 0.11% of the weight of the seed, while Mastebroek et al. (2002) considered sweet seeds as having saponin contents between 0.02 and 0.04%, and bitter seeds as having contents between 0.47 and 1.13% d.w. basis. Accordingly, Colombian quinoas were characterized by low saponin contents (< 0.11%) and classified as sweet quinoa, with crops established between 2,600 and 2,800 m above sea level. However, further studies are needed to elucidate how environmental and genotypic effects influence saponin levels in seeds (Alandia et al., 2020). On the other hand, soil conditions, climate and nutrient availability are important factors in the morphoagronomic behavior of this crop. However, physical, chemical and microbiological characteristics are specific to each location and mark the development of plants and the composition, quality and quantity of quinoa grain (Veloza et al., 2016).
The bitter quinoas (>0.11%) in this study were characterized by higher yields than the sweet quinoas (Quinoa peruana and Amarilla de maranganí). Therefore, these accessions have high potential to increase the yield and productivity of quinoa in Colombia, with the added value of having saponins that can be used for other types of industries such as pharmaceutical or cosmetological uses. Agronomically, these accessions are characterized by their high yield, semi-late phenological cycle, large grain and seed weight between 0.3 and 0.4 g. The accessions that presented a high seed productivity and low saponin content, combined with the qualitative grain characteristics, included Quinoa real, an early material with cycles of 144 days and yields of 68.56 g of seeds per plant, and Quinoa primavera with yield of 87.53 g of seed per plant and seed diameter of 2.24 mm. Therefore, these genotypes are recommended as potential parents in breeding programs.
Sweet varieties are usually preferred because the process of removing saponins is avoided. However, some farmers prefer bitter cultivars because saponins can confer resistance to biotic stresses (Colque et al., 2021). Although it has been hypothesized that saponins also confer resistance to downy mildew in quinoa (Khalifa and Thabet, 2018) because they possess antifungal activities (Colque et al., 2021), no correlation has been found between the saponin content of seeds and resistance to mildew in quinoa (Granado et al., 2021a,b). Saponin content is a highly genotype-dependent seed characteristic (De Santis et al., 2018), and no correlation with other seed traits have been found. However, the study by Granado et al. (2021b) found a correlation for the first time between saponin content and other traits related to seed quality, such as germination, protein and flavonoid content. On the other hand, the concentration of saponins is affected by climatic variations (precipitation, temperature and photoperiod) because these factors generate an effect on the synthesis of secondary metabolites as a response to the effects of stress (Delatorre et al., 2015; De Santis et al., 2016; García et al., 2018). Likewise, Koziol (1992) pointed out that altitude influences the final content of saponins. Accessions sown at lower altitudes have a higher content of saponins.
It is important to note that quinoa exhibits strong variability in crop-specific responses to environmental variation. There are reports of different environmental conditions affecting some parameters related to seed quality in quinoa, including seed size and protein or mineral content, depending on the specific genotype (Matías et al., 2021). Thus, different quinoa cultivars have shown substantial differences in nutritional characteristics, which also vary with the environment. However, it is not yet clear whether the parameters that were evaluated are stable among cultivars in different locations or whether constant correlations can be found between nutrition-related parameters (Granado et al., 2021a,b). Recent work by Granado et al. (2021b) showed that some quinoa cultivars, such as Titicaca and Vikinga, exhibit better quality-related characteristics (including higher protein content) despite not being the most productive cultivar when grown in northwestern Spain. Accordingly, the selection of genotypes best suited for a particular growing environment is key in terms of yield potential and tolerance to biotic and abiotic stress, considering different nutritional characteristics (Hussain et al., 2020). Therefore, genetic and environmental factors that determine nutritional characteristics in quinoa must be understood through the use of conventional and molecular tools that unlock the rich biodiversity and crop potency of this crop (Alandia et al., 2020).
Grain processing can play an important role in improving nutritional components, especially bioactive ones, without over-increasing the anti-nutritional factors in grains that reduce nutrient bioavailability. It also helps to improve functional properties that mainly influence the texture and organoleptic properties of the final products (Sharma et al., 2022). With respect to quinoa, few studies have been conducted on the effects of processing on saponins (Han et al., 2019; Mhada et al., 2020), phytates (Castro et al., 2019), bioactive components (such as phenol and flavonoid content), and antioxidant properties, such as 2,2-diphenyl-1-picrylhydrazyl (DPPH) and ferric reducing antioxidant power (FRAP) radical scavenging activity (Han et al., 2019).
Yield is an important but complex characteristic for genetic improvement because it encompasses the cumulative effects of all plant traits and economic value (Reynolds et al., 2020), cannot be easily analyzed, and is usually best analyzed through yield-contributing characteristics, such as harvest index and developmental, photosynthetic and physiological traits (Morton et al., 2019). Grain yield integrates two main components: the number of grains per m2 and the average weight of grains, where yield variations in grain crop species are usually associated with the number of grains (Rehman et al., 2022). The resources of crops are distributed between these two components during grain setting, with compensation and differences in the availability of assimilated per grain, which is a measure of the size of the source for the growing grains (Rehman et al., 2022).
In quinoa, a strong positive relationship has also been found between yield and the number of grains, while grain weight has shown a conservative behavior (Mignone and Bertero, 2007). Bertero and Ruiz (2008) reported that the number of grains in quinoa is highly sensitive to environmental factors from the first anthesis to the end of flowering, followed by the grain filling period. Differences in the number of grains can also be attributed to the partitioning of biomass into reproductive structures during the critical phase (D'Andrea et al., 2006). A better understanding of yield responses to higher temperatures during different phenological phases could reveal important clues about the sensitivity of quinoa grain yield to global warming. García et al. (2019) evaluated the physiological response and proximal composition of the grain to three types of fertilization in the municipality of Oicatá (Boyacá, Colombia), finding that the reproductive stages and the proximal composition of the seeds showed changes attributed to the contribution of organic-mineral fertilizer that improved the production of quinoa grains, while the contribution of NPK showed greater growth and vegetative development but lower yield.
In this study, yield was shown to be correlated with the variables associated with seeds, such as weight and diameter, characteristics that are taken into account for the commercialization of quinoa and are commonly used as material selection criteria for breeding programs (Urdanegui et al., 2021). Likewise, it is necessary to make efficient use of the genetic variability observed in accessions of Colombian quinoa to increase productivity and expand this crop to other regions (Alandia et al., 2020; Manjarres et al., 2021).
This selection of materials contributes to the sustainability of productive activities in Colombia, where good quality in planting material responds to the commercialization needs for grain in the market and in industries.
Conclusion
The results showed that the accessions Quinoa primavera, Quinoa peruana, Quinoa real and Amarilla de maranganí represent the more promising parents for future development of breeding programs that respond to the needs of farmers, producers, consumers and industries in Colombia. With the increase in the demand for quinoa and the potential that Colombia has to produce it, an added value could be given to this crop using saponins as a by-product. Currently, this metabolite is not used; however, the present study found genotypes with morphological characteristics in the grain, yield and saponin content that would allow the entry into new markets taking advantage of the potential use of saponins in the production of medicines, cosmetics, biopesticides, among others. An adequate extraction or saponification would achieve a sustainable use of this resource.
Data availability statement
The original contributions presented in the study are included in the article/Supplementary material, further inquiries can be directed to the corresponding author.
Author contributions
EM: conceptualization, methodology, formal analysis, investigation, resources, writing—original draft, and writing—review and editing. AM: conceptualization, methodology, resources, writing—review and editing, supervision, and project administration. AC: methodology, formal analysis, and conceptualization. CM: methodology, formal analysis, and investigation. All authors contributed to the article and approved the submitted version.
Funding
To the Patrimonio Autónomo Fondo Nacional de Financiamiento para la Ciencia, la Tecnología y la Innovación Francisco José de Caldas-MinCiencias. Cód. 63924.
Acknowledgments
To the CIDE group, Competitividad Innovación y Desarrollo Empresarial of the Faculty of Agricultural Sciences of the UPTC and to the Quimol group of the Faculty of Sciences of the UPTC.
Conflict of interest
The authors declare that the research was conducted in the absence of any commercial or financial relationships that could be construed as a potential conflict of interest.
Publisher's note
All claims expressed in this article are solely those of the authors and do not necessarily represent those of their affiliated organizations, or those of the publisher, the editors and the reviewers. Any product that may be evaluated in this article, or claim that may be made by its manufacturer, is not guaranteed or endorsed by the publisher.
Supplementary material
The Supplementary Material for this article can be found online at: https://www.frontiersin.org/articles/10.3389/fsufs.2022.919885/full#supplementary-material
Supplementary Figure 1. HPLC-UV chromatogram at 210nm of oleanolic acid standard and saponin sample.
Supplementary Figure 2. UV-Vis pattern spectrum of saponins, oleanolic acid, hydrolyzed saponins and microwave saponins with maximum peak at 277 nm.
References
Alandia, G., Rodríguez, J. P., Jacobsen, S. E., Bazile, D., and Condori, B. (2020). Global expansion of quinoa and challenges for the Andean region. Glob. Food Sec. 26, 100429. doi: 10.1016/j.gfs.2020.100429
Alizadeh, G., Lakzadeh, L., Forootanfar, H., and Hamid, A. (2022). Optimization of gluten-free bread production with low aflatoxin level base on quinoa flour containing xanthan gum and laccase enzyme. Int. J. Biol. Macromol. 200, 61–76. doi: 10.1016/j.ijbiomac.2021.12.091
Asher, A., Galili, S., Whitney, T., and Rubinovich, L. (2020). The potential of quinoa (C. quinoa) cultivation in Israel as a dual-purpose crop for grain production and livestock feed. Sci. Hortic. 272, 109534. doi: 10.1016/j.scienta.2020.109534
Bazihizina, N., Vita, F., Balestrini, R., Kiferle, C., Caparrotta, S., Ghignone, S., et al. (2022). Early signalling processes in roots play a crucial role in the differential salt tolerance in contrasting C. quinoa accessions. J. Exp. Bot. 73, 292–306. doi: 10.1093/jxb/erab388
Bertero, H. D., and Ruiz, R. A. (2008). Determination of grain number in sea level quinoa (C. quinoa Willd.) cultivars. Eur. J. Agron. 28, 186–194. doi: 10.1016/j.eja.2007.07.002
Bioversity International (2013). Organización de las Naciones Unidas Para la Agricultura y la Alimentación (FAO), Fundación PROINPA, Instituto Nacional de Innovación Agropecuaria y Forestal (INIAF) and F. I. de D. A. (FIDA). Descriptores Para Quinua y sus Parientes Silvestres. Rome: Bioversity International, 52.
Bonifacio, A. (2019). Improvement of quinoa (C. quinoa willd.) and qañawa (Chenopodium pallidicaule aellen) in the context of climate change in the high Andes. Cienc Investig Agrar. 46, 113–124. doi: 10.7764/rcia.v46i2.2146
Castro, V., Lazarte, C. E., Pérez, D., Sandberg, A. S., Carlsson, N. G., Almgren, A., et al. (2019). Effect of fermentation and dry roasting on the nutritional quality and sensory attributes of quinoa. Food Sci. Nutr. 7, 3902–3911. doi: 10.1002/fsn3.1247
Colque, C., Abondano, M. C., Lund, O. S., Amby, D. B., Piepho, H. P., Andreasen, C., et al. (2021). Genetic variation for tolerance to the downy mildew pathogen Peronospora variabilis in genetic resources of quinoa (C. quinoa). BMC Plant Biol. 21, 41. doi: 10.1186/s12870-020-02804-7
Colson, E., Savarino, P., Claereboudt, E., Cabrera, G., Deleu, M., Lins, L., et al. (2020). Enhancing the membranolytic activity of C. quinoa saponins by fast microwave hydrolysis. Molecules 25, 1731. doi: 10.3390/molecules25071731
D'Andrea, K. E., Otegui, M. E., Cirilo, A. G., and Eyerhabide, G. (2006). Genotypic variability in morphological and physiological traits among maize inbred lines-nitrogen responses. Crop Sci. 46, 1266–1276. doi: 10.2135/cropsci2005.07-0195
De Bock, P., Van Bockstaele, F., Muylle, H., Quataert, P., Vermeir, P., Eeckhout, M., et al. (2021). Yield and nutritional characterization of thirteen quinoa (C. quinoa Willd.) varieties grown in North-West Europe—Part I. Plants 10, 2689. doi: 10.3390/plants10122689
De Santis, G., Maddaluno, C., D'Ambrosio, T., Rascio, A., Rinaldi, M., and Troisi, J. (2016). Characterisation of quinoa (C. quinoa Willd.) accessions for the saponin content in Mediterranean environment. Ital. J. Agron. 11, 277–281. doi: 10.4081/ija.2016.774
De Santis, G., Ronga, D., Caradonia, F., Ambrosio, T. D., Troisi, J., Rascio, A., et al. (2018). Evaluation of two groups of quinoa (C. quinoa Willd.) accessions with different seed colours for adaptation to the Mediterranean environment. Crop Past. Sci. 69, 1264–1275. doi: 10.1071/CP18143
Delatorre, J., Gonzalez, J., and Martinez, E. (2015). Efecto del Fotoperiodo y la Temperatura Sobre la Concentración de Saponinas en tres Variedades de Quinua Provenientes de tres Latitudes. Argentina: V Congreso Mundial de Quinua, 1–10.
Di Rienzo, J. A., Casanoves, F., Balzarini, M. G., Gonzalez, L., Tablada, M., and Robledo, C. W. (2020). InfoStat versión 2020. Centro de Transferencia InfoStat, FCA. Argentina: Universidad Nacional de Córdoba.
El Hazzam, K., Hafsa, J., Sobeh, M., Mhada, M., Taourirte, M., El Kacimi, K., et al. (2020). An insight into saponins from Quinoa (C. quinoa Willd): A review. Molecules. 25, 1059. doi: 10.3390/molecules25051059
Fagandini, F., Bazile, D., Drucker, A. G., Tapia, M., and Chura, E. (2021). Geographical distribution of quinoa crop wild relatives in the Peruvian Andes: a participatory mapping initiative. Environ. Dev. Sustain. 23, 6337–6358. doi: 10.1007/s10668-020-00875-y
Fan, X., Li, Y., Zhu, Y., Wang, J., Zhao, J., Sun, X., et al. (2020). Characterization of physicochemical qualities and starch structures of two indica rice varieties tolerant to high temperature during grain filling. J. Cereal Sci. 93, 102966. doi: 10.1016/j.jcs.2020.102966
García, A., Villanueva, M., Vela, A., and Ronda, F. (2020). Protein and lipid enrichment of quinoa (cv. Titicaca) by dry fractionation. Techno-functional, thermal and rheological properties of milling fractions. Food Hydrocoll. 105, 195770. doi: 10.1016/j.foodhyd.2020.105770
García, M., García, J., and Deaquiz, Y. (2019). Physiological performance of quinoa (C. quinoa Willd.) under agricultural climatic conditions in Boyacá, Colombia. Agron. Colomb. 37, 160–168. doi: 10.15446/agron.colomb.v37n2.76219
García, M. A., Leguizamón, N. Z., Rodríguez, D. C., Torrado, S. K., and Parra, J. (2018). Descripción de las saponinas en quinua (“C. quinoa” willd) en relación con el suelo y el clima: una revisión. Informador Técnico 82, 241–249. doi: 10.23850/22565035.1451
Granado, S., Aparicio, N., Matías, J., Pérez, L. F., Maestro, I., Garcés, I., et al. (2021a). Studying the impact of different field environmental conditions on seed quality of quinoa: the case of three different years changing seed nutritional traits in southern Europe. Front. Plant Sci. 12, 649132. doi: 10.3389/fpls.2021.649132
Granado, S., Vilariño, S., Maestro, I., Matías, J., Rodríguez, M., Calvo, P., et al. (2021b). Genotype-dependent variation of nutritional quality-related traits in quinoa seeds. Plants 10, 2128. doi: 10.3390/plants10102128
Han, Y., Chi, J., Zhang, M., Zhang, R., Fan, S., Dong, L., et al. (2019). Changes in saponins, phenolics and antioxidant activity of quinoa (C. quinoa willd) during milling process. LWT. 114, 108381. doi: 10.1016/j.lwt.2019.108381
Hussain, M. I., Muscolo, A., Ahmed, M., Asghar, M. A., and Al-Dakheel, A. J. (2020). Agro-morphological, yield and quality traits and interrelationship with yield stability in quinoa (C. quinoa Willd.) genotypes under saline marginal environment. Plants. 9, 1763. doi: 10.3390/plants9121763
Isam, M., Fahad, A. L., and Mehmet, M. Ö. (2021). Insights into the nutritional value and bioactive properties of quinoa (C. quinoa): past, present and future prospective. Int. J. Food Sci. 56, 3726–3741. doi: 10.1111/ijfs.15011
Josse, J., and Husson, F. (2016). missMDA: a package for handling missing values in multivariate data analysis. J. Stat. Softw. 70, 1–31. doi: 10.18637/jss.v070.i01
Kassambara, A., and Mundt, F. (2020). Factoextra: Extract and Visualize the Results of Multivariate Data Analyses. (R Package Version 1.0.7).
Khalifa, W., and Thabet, M. (2018). Variation in downy mildew (Peronospora variabilis Gäum) resistance of some quinoa (C. quinoa Willd) cultivars under Egyptian conditions. Middle East J. Agric. Res. 7, 671–682.
Koziol, M. J. (1992). Chemical composition and nutritional evaluation of quinoa (C. quinoa Willd.). J. Food Compos. Anal. 5, 35–68. doi: 10.1016/0889-1575(92)90006-6
Le, S., Josse, J., and Husson, F. (2008). FactoMineR: an R package for multivariate analysis. J. Stat. Softw. 25, 1–18. doi: 10.18637/jss.v025.i01
León, N., Aguilar, S., Quispe, J., Mamani, W., Alfaro, S., and Condezo, L. (2019). A green analytical assay for the quantitation of the total saponins in quinoa (C. quinoa Willd.) based on macro lens-coupled smartphone. Talanta. 204, 576–585. doi: 10.1016/j.talanta.2019.06.014
Lesjak, J., and Calderini, D. (2017). Increase night temperature negatively affects grain yield, biomass and grain number in Chilean quinoa. Front. Plant Sci. 8, 352. doi: 10.3389/fpls.2017.00352
Lim, J., Park, H., and Yoon, K. (2020). Analysis of saponin composition and comparison of the antioxidant activity of various parts of the quinoa plant (C. quinoa Willd.). Food Sci. Nutr. 8, 694–702. doi: 10.1002/fsn3.1358
Lin, M., Han, P., Li, Y., Wang, W., Lai, D., and Zhou, L. (2019). Quinoa secondary metabolites and their biological activities or functions. Molecules. 24, 2512. doi: 10.3390/molecules24132512
Lu, D. L., Huan, Y., Xin, S., and Lu, W. (2016). Effects of high temperature during grain filling on physicochemical properties of waxy maize starch. J. Integr. Agric. 15, 309–316. doi: 10.1016/S2095-3119(15)61095-4
Manjarres, E. H., Arias, D. M., Morillo, A. C., Ojeda, Z. Z., and Cárdenas, A. (2021). Phenotypic characterization of quinoa (C. quinoa Willd.) for the selection of promising materials for breeding programs. Plants 10, 1339. doi: 10.3390/plants10071339
Mastebroek, H., Van Loo, E., and Dolstra, O. (2002). Combining ability for seed yield traits of C. quinoa breeding lines. Euphytica 125, 427–432. doi: 10.1023/A:1016030129541
Matías, J., Rodríguez, M. J., Cruz, V., Calvo, P., and Reguera, M. (2021). Heat stress lowers yields, alters nutrient uptake and changes seed quality in quinoa grown under Mediterranean field conditions. J. Agron. Crop Sci. 207, 481–491. doi: 10.1111/jac.12495
Mhada, M., Metougui, M. L., El Hazzam, K., El Kacimi, K., and Yasri, A. (2020). Variations of saponins, minerals and total phenolic compounds due to processing and cooking of quinoa (C. quinoa Willd.) seeds. Foods 9, 660. doi: 10.3390/foods9050660
Mignone, C. M., and Bertero, H. D. (2007). “Identificación del período crítico de determinación del rendimiento en quínoas de nivel del mar,” in Proceedings of the Congreso Internacional de la Quinua, 23–26 October 2007, Iquique.
Morales, A., Silva, A., Maldonado, J., and Silva, H. (2017). Transcriptional responses of Chilean Quinoa (C. quinoa Willd.) under water deficit conditions uncovers ABA-independent expression patterns. Front. Plant Sci. 8, 216. doi: 10.3389/fpls.2017.00216
Morillo, A. C., Manjarres, E. H., and Morillo, Y. (2020). Evaluación morfoagronómica de 19 materiales de C. quinoa en el Departamento de Boyacá. Biotecnol. Sect. Agropecuario Agroind. 18, 84–96. doi: 10.18684/bsaa.v18n1.1416
Morton, M. J. L., Awlia, M., Al Tamimi, N., Saade, S., Pailles, Y., Negrão, S., et al. (2019). Salt stress under the scalpel–dissecting the genetics of salt tolerance. Plant J. 97, 148–163. doi: 10.1111/tpj.14189
Naheed, N., Abbas, G., Naeem, M. A., Hussain, M. M., Shabbir, R., Alamri, S., et al. (2022). Niquel tolerance and phytoremediation potential of quinoa are modulated under salinity: multivariate comparison of physiological and biochemical attributes. Environ. Geochem. Health. 44, 1409–1424. doi: 10.1007/s10653-021-01165-w
Ng, C. Y., and Wang, M. (2021). The functional ingredients of quinoa (C. quinoa) and physiological effects of consuming quinoa: a review. Food Front. 2, 329–356. doi: 10.1002/fft2.109
Pathan, S., and Siddiqui, R. (2022). Nutritional composition and bioactive components in quinoa (C. quinoa Willd.) greens: a review. Nutrients. 14, 558. doi: 10.3390/nu14030558
Rafik, S., Rahmani, M., Rodríguez, J. P., Andam, S., Ezzariai, A., El Gharous, M., et al. (2021). How does mechanical pearling affect quinoa nutrients and saponin contents?. Plants 10, 1133. doi: 10.3390/plants10061133
Rehman, H., Alharby, H., Al-Zahrani, H., Bamagoos, A., Alsulami, N., Alabsallah, N., et al. (2022). Enriching urea with nitrogen inhibitors improves growth, N uptake and seed yield in quinoa (C. quinoa Willd.) affecting photochemical efficiency and nitrate reductase activity. Plants 11, 371. doi: 10.3390/plants11030371
Reynolds, M., Chapman, S., Herrera, L. A. C., Molero, G., Mondal, S., Pequeño, D. N., et al. (2020). Breeder friendly phenotyping. Plant Sci. 295, 110396. doi: 10.1016/j.plantsci.2019.110396
Sharma, S., Katarina, A., and Singh, B. (2022). Effect of thermal processing in the bioactive compounds, antioxidative, antinutritional and functional characteristics of quinoa (C. quinoa). LWT. 160, 113256. doi: 10.1016/j.lwt.2022.113256
Stoleru, V., Jacobsen, S. E., Vitanescu, M., Jitareanu, G., Butnariu, M., Munteanu, N., et al. (2022). Nutritional and antinutritional compounds in leaves of quinoa. Food Biosci. 45, 101494. doi: 10.1016/j.fbio.2021.101494
Taiyun, W., and Simko, V. (2017). R Package “Corrplot”: Visualization of a Correlation Matrix (Version 0.84).
Tapia, M. E., Canahua, A., and Ignacio, S. (2014). Razas de quinuas del Perú. Lima: ANPE-Perú, CONCYTEC 173.
Team, R. C. (2020). R: A Language and Environment for Statistical Computing. Vienna: R Foundation for Statistical Computing.
Tovar, J., Berry, J., Quillatupa, C., Castillo, E., Acosta, L., Fahlgren, N., et al. (2022). Heat stress changes mineral nutrient concentrations in C. quinoa seed. Plant Direct. 6, e384. doi: 10.1002/pld3.384
Urdanegui, P., Pérez, Á., Estrada, R., Neyra, E., Mujica, Á., and Corredor, F. A. (2021). Rendimiento y evaluación agromorfológica de genotipos de quinua (C. quinoa Willd.) en Huancayo, Perú. Agroind. Sci. 11, 63–71. doi: 10.17268/agroind.sci.2021.01.08
Veloza, C., Romero, G., and Gómez, J. (2016). Respuesta morfoagronómica y calidad en proteína de tres accesiones de quinua (C. quinoa Willd.) en la sabana norte de Bogotá. Rev. UDCA Act. Div. Cient. 19, 325–332. doi: 10.31910/rudca.v19.n2.2016.86
Villacis, C. (2018). Estudio pzara la extracción y purificación de saponinas a partir del mojuelo de quinua como una posible alternativa a la disponibilidad de tensoactivos de origen natural. Sangolqui: Universidad de las Fuerzas Armadas ESPE, 129.
Villacrés, E., Quelal, M., Galarza, S., Iza, D., and Silva, E. (2022). Nutritional value and bioactive compounds of leaves and grains from Quinoa (C. quinoa Willd.). Plants 11, 213. doi: 10.3390/plants11020213
Keywords: oleanolic acid, Chenopodium quinoa, ancestral cultivation, phenology, saponins, grain quality
Citation: Manjarres Hernández EH, Morillo Coronado AC, Cárdenas Chaparro A and Merchán López C (2022) Yield, phenology and triterpene saponins in Colombian quinoa. Front. Sustain. Food Syst. 6:919885. doi: 10.3389/fsufs.2022.919885
Received: 14 April 2022; Accepted: 31 October 2022;
Published: 18 November 2022.
Edited by:
Viji Sarojini, The University of Auckland, New ZealandReviewed by:
Ramnarain Ramakrishna, North Dakota State University, United StatesRebogile Mphahlele, Agricultural Research Council of South Africa (ARC-SA), South Africa
Lochan Singh, National Institute of Food Technology Entrepreneurship and Management, India
Copyright © 2022 Manjarres Hernández, Morillo Coronado, Cárdenas Chaparro and Merchán López. This is an open-access article distributed under the terms of the Creative Commons Attribution License (CC BY). The use, distribution or reproduction in other forums is permitted, provided the original author(s) and the copyright owner(s) are credited and that the original publication in this journal is cited, in accordance with accepted academic practice. No use, distribution or reproduction is permitted which does not comply with these terms.
*Correspondence: Elsa Helena Manjarres Hernández, ZWxzYS5tYW5qYXJyZXNAdXB0Yy5lZHUuY28=