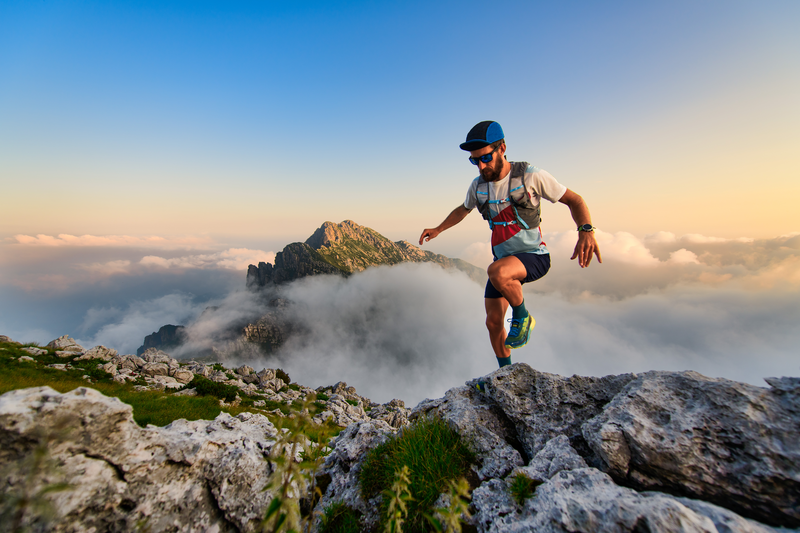
95% of researchers rate our articles as excellent or good
Learn more about the work of our research integrity team to safeguard the quality of each article we publish.
Find out more
ORIGINAL RESEARCH article
Front. Sustain. Food Syst. , 08 July 2022
Sec. Agroecology and Ecosystem Services
Volume 6 - 2022 | https://doi.org/10.3389/fsufs.2022.908231
This article is part of the Research Topic Legumes for Global Food Security, volume II View all 23 articles
Soybean production in sub-Saharan Africa is increasing as farmers open more land areas for cultivation and replace other crops, such as tobacco, in favor of this legume crop. Despite the production is increased in Mozambique, demand for animal feed and oil is not satisfied. As such, farmers explore ways to improve yield per unit area of soybean by using bio-inoculants from various sources and agroecological adaptability. These bio-inoculants are seldom available during planting time, and retail at almost similar prices although yield varied based on the product source, handling, and the rhizobia strain carrier. Mozambique does not produce bio-inoculants, so it obtains the product from neighboring countries or as far as the South American continent. In this study, we evaluated the performance, ecological adaptability, and soybean productivity of seven Bradyrhizobium diazoefficiens strain-based bio-inoculants from several countries with different carrier materials: Biofix, Masterfix, Nitrofix, NitroZam, N-Fixer, Soygro Peat, and Soygro Liquid against a control (non-inoculated) on two soybean varieties Storm and TGx 1904-6F. The trial was conducted in the 2016 and 2017 growing seasons in three agroecologies of Mozambique at Angonia, Nampula, and Ruace. Data on nodulation, plant growth, biomass nitrogen content at beginning of podding (R3) stage, yield, and yield components of soybean were evaluated. Analysis of variance and contrast comparisons were performed on the Statistical Analysis System® 9.4. Nodule weight per plant variedly increased from 7.7 to 167.6 mg with inoculation of both varieties across environments. Plant tissue nitrogen content at the R3 stage was higher in inoculated non-promiscuous variety at 3.9% than the promiscuous counterpart with 3.7%. Storm, a non-promiscuous short-maturity variety of soybean, responded to inoculation and accumulated more N than the medium-to-late maturity promiscuous TGx 1904-6F. Higher N tissue content is an indicator of better nutritive value, as well as high-quality recyclable biomass of inoculated soybean. Both Storm and TGx 1904-6F responded to all inoculants variedly with NitroZam yield of 2,750 kg ha−1 being highest, while Soygro Liquid was lowest with 2,051 kg ha−1 but more than the check with 1,690 kg ha−1 across sites. There were varietal differences in 100-seed weight after inoculation where Storm (15.4 g) had heavier seeds than TGx 1904-6F (13.1 g). The results show that inoculation improved plant growth and development, increased nodulation, and gave higher yields for better economic returns among farmers. Inoculation has the potential of increasing soybean yield, nutritive value, and biomass quality within Mozambique.
Soybean production is becoming an important crop among the farming communities in Mozambique. Efforts by several agricultural development organizations, such as the International Institute of Tropical Agriculture (IITA), have increased the adoption of improved soybean varieties to 89% (AGRA, 2016). For instance, IITA and the Instituto de Investigação Agrária de Moçambique (IIAM) (Agricultural Research Institute of Mozambique) have released and promoted 14 improved soybean varieties. The soybean production system in Mozambique is dominated by smallholder farmers who face a myriad of limitations. These farmers seldom use the necessary inputs, such as quality seeds, fertilizers, and pesticides (Matteo et al., 2016). This situation is occasioned by a lack of access to and unaffordable prices at the time of planting and during the production period. However, the cost of using fertilizer in particular nitrogen could be averted through the use of bio-inoculants. Bio-inoculants have been shown to increase the yield of soybean (Chibeba et al., 2020; Savala et al., 2021) and other legumes, such as cowpea (Kyei-Boahen et al., 2017), in Mozambique. The use of bio-inoculants is also limited by the lack and inaccessibility of the input at the time of planting. Mozambique as a country does not have the capacity to produce bio-inoculants despite studies showing that locally isolated rhizobia perform comparably well to established strains Bradyrhizobium diazoefficiens (USDA110) (Chibeba et al., 2017). Instead, the country relies on the government or selected agricultural development organizations that import bio-inoculants from around the region or as far as the South American continent. The quality of the imported bio-inoculant is often compromised due to a lack of proper transportation and handling facilities. With all these prevailing challenges, soybean production in Mozambique has remained below its production potential, and therefore does not meet the growing soybean grain demand from the local livestock feed industry and for human consumption (Matteo et al., 2016). As such the country spends a lot of foreign exchange on the importation of soybean and its related products in addition to bio-inoculants to meet local demand. Fortunately, most of the available varieties are promiscuous that can freely form a symbiotic association and nodulate with existing native rhizobia strains in the soil (Gyogluu et al., 2016), although the application of bio-inoculants improves nodulation and efficiency of nitrogen fixation.
Not only does Mozambique lack the capacity of producing bio-inoculants but also accompanying policies and information on the handling, trading, and use are absent. This leaves the trade and use of bio-inoculants unregulated resulting in the importation of different bio-inoculant types/grades depending on the accessibility. These bio-inoculants grades (liquid or solid mostly peat based) are often transported like regular cargo from the importing agents to the farmers' fields. No standards exist for the bio-inoculant handlers to follow, hence jeopardizing their effectiveness to improve the biological nitrogen fixation (BNF) process that would have otherwise resulted in increased legume yields. Farmers in Mozambique use seed inoculation of both liquid- and peat-based bio-inoculants plus other good management practices to enhance the efficiency of the BNF process in soybean (Matteo et al., 2016; Savala and Kyei-Boahen, 2020). Although the use of peat-based bio-inoculants is higher than the liquid-based counterparts, their quality varies a lot depending on the source due in part to contamination as most of the bio-inoculants use peat as the carrier material (Woomer et al., 2014). Studies have established that for resource-poor farmers, the use of bio-inoculant alone could go a long way to improve legume yields due to the economic incentive on returns associated with the practice (Kyei-Boahen et al., 2017). Countries that supply Mozambique with bio-inoculants include Argentina, Brazil, Kenya, Malawi, South Africa, Zambia, and Zimbabwe. Past BNF trials conducted in Mozambique have also included bio-inoculants from the United States of America (Savala et al., 2022). The experiments demonstrated the importance of both liquid- and peat-based bio-inoculants applied either on seed or directly to the soil.
The use of bio-inoculants in soybean production tends to be more beneficial when an adequate supply of phosphorus fertilizer plus other necessary agronomic practices are maintained (Singleton et al., 1985, 2001; Tittabutr et al., 2007). Some of the soybean agronomic management practices used by farmers include adjusting the planting time to take advantage of the rainfall condition, variety selection, especially the promiscuous genotypes, and weeds and pest control. However, the use of inorganic fertilizer is limited among Mozambican farmers due to its obnoxiously high price (Zavale et al., 2020). As such non-monetary options like site selection or low-cost investment, such as bio-inoculant use, are often preferred. Soybean is mainly produced in the medium-to-high altitude agroecologies of Mozambique (Walker et al., 2006; AGRA, 2016; Matteo et al., 2016). These ecologies are in Manica, Nampula, Tete, and Zambezia provinces where farmers are increasingly growing promiscuous varieties. Promiscuous varieties can self-nodulate with native rhizobia strains that are present in the soils (Chibeba et al., 2017). Promiscuous soybean varieties can be grown without bio-inoculants, although studies indicate that nodulation and more yield is realized following inoculation. Inoculation of promiscuous soybean varieties yielded more than the non-inoculated ones in many sub-Saharan African countries (Majengo et al., 2011; van Heerwaarden et al., 2018; Chibeba et al., 2020). Although the information on differences in bio-inoculant carriers is lacking, the effectiveness of either peat- or liquid-based bio-inoculants is not documented in the country to guide farmers on selection. Such data could be important in drafting policies on the price, handling, transportation, and use of bio-inoculants in the country. Moreover, this would guide investors to the most suitable bio-inoculant carrier to use if a facility was to be established in Mozambique. Therefore, our study evaluated the performance, ecological adaptability, and soybean productivity of seven Bradyrhizobium diazoefficiens strain-based bio-inoculants acquired from several countries with different carrier materials against a control on nodulation and yield gain of two soybean varieties Storm and TGx 1904-6F. Secondly, we seek to determine the agroecological suitability of bio-inoculants for soybean production in Mozambique.
Soybean field studies were conducted in the 2016 and 2017 growing seasons at three locations in Mozambique: Angonia 14.6273° S, 33.9812° E, 1,115 meters above sea level (m a.s.l.), Ruace 15.2343° S, 36.6883° E, 677 m a.s.l., and Nampula 15.2738° S, 39. 3143° E, 377 m a.s.l.. The sites have monomodal rainfall between December and April averaging at Angonia 900–1,400 mm, Ruace 800–1,400 mm, and Nampula 600–1,100 mm. As such the Angonia and Ruace are characterized by a maize legume cropping system, while Nampula is dominated by a cassava legume cropping system. Soybean is largely produced in these systems as a monoculture for commercial purposes as poultry feed although a little amount is utilized in households to enrich/fortify pastries. In both cropping systems, farmers rarely use fertilizers but mostly rely on the inherent soil fertility with limited residue recycling back to the fields. For each season, new fields previously under maize for two growing periods were used. According to the Soils Atlas of Africa, the predominant soil type at the sites in Angonia and Ruace are Chromic Luvisols, while that in Nampula is Haplic Lixisols (Jones et al., 2013). Ten soil samples were taken from 0 to 30 cm soil layer using a soil auger in a W pattern across the field for the trial before plowing or harrowing. Soils from each site were combined into a composite sample and four subsamples were drawn for chemical and particle-size analysis (Table 1). The pH was determined using a high impedance voltmeter on 1:2 soil–water suspension. Total organic carbon was determined by Walkley–Black method, total N by the Kjeldahl method, P by Olsen's method, and K using ICP-OES after extraction with Mehlich 3. The soil rhizobial population of the experimental field was determined from soil samples taken from all three locations (Table 2) using the most probable number method (Vincent, 1970; Woomer et al., 1990).
Table 2. Soybean rhizobia most probable number (MPN) in soils collected from the experimental site with and without a history of soybean cultivation.
Several Bradyrhizobium diazoefficiens strains USDA110 formerly known as Bradyrhizobium japonicum (Delamuta et al., 2013) bio-inoculant products obtained from the regions across Africa and South America were used for the study (Table 3). The bio-inoculants namely Biofix, Masterfix, Nitrofix, NitroZam, N-Fixer, Soygro Peat, and Soygro Liquid. Two bio-inoculants Biofix and Masterfix were imported from outside the region. Biofix a peat-based bio-inoculant was sourced from a fertilizer-producing company in Kenya, while Masterfix was acquired from Brazil. Three peat-based bio-inoculants used in the study were obtained from the National Agricultural Research Institutes of Malawi (Nitrofix), Zambia (NitroZam), and Zimbabwe (N-Fixer). Also, peat- and liquid-based bio-inoculants by the trade name Soygro were sourced from South Africa. All the peat-based bio-inoculants were applied to the seed using inert gum Arabic solution as the adhesive agent. According to the manufacturer's recommendation, the bio-inoculants were applied to supply ~106 rhizobia cells seed−1.
Table 3. Minimum manufacturer's specifications for colony-forming units per gram (CFU g−1) or milliliter (CFU ml−1) and carrier of the experimental inoculants.
Two soybean varieties, Zamboane (TGx 1904-6F), a promiscuous variety released by IITA and IIAM and Storm (non-promiscuous Seed Co. variety) were used in the experiment. Bio-inoculant products were evaluated against a control. The field experimental design was a randomized complete block with four replications for each of the bio-inoculants. Plots consisted of five rows of 9 m length, 0.50 m row-spacing, and 0.1 m space between plants within rows. Land preparation was accomplished with disc plowing followed by two passes with a disc harrow. Seeds were inoculated in the field under a shade by weighing 1.0 kg of seeds of each cultivar into separate plastic bags for each bio-inoculant then a respective adhesive agent as per the manufacturer's guidelines was added. The seeds were mixed thoroughly to enable coating of each seed with the gum Arabic adhesive agent. Thereafter, respective bio-inoculants were added and further mixed thoroughly until all the seeds were completely covered with bio-inoculant (Table 3) before planting. In both the 2016 and 2017 seasons, the experiments were planted between 18 and 30 December, although scheduling was site-specific depending on the onset of rains in each location. The risk of contamination was avoided by planting the non-inoculated plots first before planting the inoculated ones. Furthermore, the same individuals handled the planting of each bio-inoculant for all four replicates. Planting and weeding (twice) were done manually using a hand-held hoe. To control pests, insecticides were applied using 100 ml of Cypermethrin (200 g active ingredient/l) and 50 ml of Lambda Cyhalothrin (50 g active ingredient/l) in 15 l knapsack sprayers at beginning of flowering.
Data on nodulation, plant growth, biomass nitrogen content at the R3 stage (beginning of podding), yield, and yield components were evaluated. At the R3 growth stage, when pods had reached 10–12 mm long at one of the four uppermost nodes on the main stem, it is regarded as the peak demand for nitrogen in soybean; five soybean plants were randomly selected from each plot and the roots were excavated using a hoe and spade. The soil was carefully washed out from the roots to ensure that all the nodules were recovered as much as possible. The nodules were plucked from the roots by hands, counted, subsequently placed in envelopes, and dried in an oven at 60°C for 48 h. The dried nodules were weighed to determine the dry weight. The remaining plant biomass was dried in an oven at 60°C until a stable weight was attained, and dry weight was determined. Later the biomass was ground to pass through a 2-mm mesh sieve for plant tissue N analysis using inductively coupled plasma optical emission spectrometry (ICP-OES), after extraction with nitric acid and hydrochloric acid. At maturity, 1 m2 area (two middle rows, 1 m long) was randomly selected and harvested to determine above-ground biomass, pod density, and 100-seed weight. The rest of the plots were harvested manually and pods from each plot were threshed manually, and grain yield was determined. The moisture content of grain samples from each plot was measured using the Farmex MT-16 Grain Moisture Tester (AgraTronix LLC, Streetsboro, Ohio, USA) and grain yield in kg/ha was adjusted to 13% moisture content. After threshing the pods, the above-ground plant biomass from the 1 m2 plot area was sun-dried for 10 days to 10% moisture content to determine dry matter yield. Except for land preparation, all field activities were done manually.
Analyses of variance (ANOVAs) were performed on the data collected using PROC GLM in the Statistical Analysis System (SAS)® 9.4 (SAS/STAT®, 2018). A combined analysis across locations and cropping seasons was performed. First, an all locations combined ANOVA was performed to evaluate the effects of the environment, variety, bio-inoculants, and their interactions. As ecological conditions at the locations were different and dominant for all the variables analyzed, effects associated with the growing period were confounded with the weather within seasons (Moore and Dixon, 2015). Thus, the environment was considered a random effect. Environmental effects were significant for all the variables. Consequently, environment and replication were treated as random effects (Moore and Dixon, 2015). A second ANOVA for each environment was performed with a variety and bio-inoculant factors considered as fixed effects. Means were determined for treatments, and comparisons were done using the least significant difference (LSD) at a P ≤ 0.05 significance level (SAS/STAT®, 2018). Correlation analysis of yield components was conducted to determine the effect of the variable on grain yield. Also, contrast analyses by environment and by locations for combined growing seasons were performed on mean grain yield. In this study, we evaluated the performance, ecological adaptability, and soybean productivity of seven Bradyrhizobium diazoefficiens strain-based bio-inoculants with different carrier materials on two soybean varieties.
The soils at the three sites were sandy clay loam with pH ranging from 6.2 to 6.6 but the CEC for the Ruace soil was more than double that for the Angonia and Nampula sites (Table 1). The soil available P, K, and Mg were lower in Nampula than Angonia and Ruace. Total N was relatively lower in Angonia (0.09%) compared to that for Nampula (0.13%) and Ruace (0.15%). The indigenous rhizobial population was relatively high in Angonia and Ruace, whereas the population in Nampula was very limited (Table 2). The low populations of indigenous rhizobia found in Angonia, Nampula, and Ruace soils were not adequate for an effective BNF process necessitating the use of bio-inoculants. Table 3 shows the populations of the colony-forming units/cells per g or ml of the carrier for the inoculant products. NitroZam inoculant from Zambia had the lowest concentration of colony-forming units, whereas Masterfix had the highest concentration.
The main purpose of inoculation is to improve nodulation in legumes through the symbiotic association of the rhizobia strain and the host plant. It is presumed that improved nodulation leads to an increased BNF process that avails more N for plant growth and development. Overall, inoculation resulted in the formation of more nodules on soybean roots (Figures 1, 2). Nodule population per plant was higher for the inoculated soybean than in the control fields. When segregated on a variety basis, TGx 1904-6F formed more nodules than Storm (Figure 1). The number of nodules was also distinct among bio-inoculants at different sites with Masterfix forming the highest nodules per plant in four out of the six environments. Nodule population on soybean roots also differed among sites. For instance, more nodules were formed in Nampula than Ruace in the 2016 growing season (Figure 1). Upon referring to the most probable number of soybean rhizobia strains in the site soils, Nampula seldom had active cells to form the symbiotic relationship necessary for the BNF process (Table 2).
Figure 1. Nodule population (plant−1) following inoculation of soybean at (A) Nampula 2016, (B) Ruace 2016, and (C) Ruace 2017. Small letters on the bars indicate no significance while different letters show significant differences in means.
Figure 2. Nodule dry weight (mg plant−1) following inoculation of soybean at (A) Angonia 2016, (B) Ruace 2016, (C) Angonia 2017, and (D) Ruace 2017. Small letters on the bars indicate no significance while different letters show significant differences in means.
Like the nodule population, nodule dry weight per plant for all the bio-inoculants were significantly higher than that for the control (Figure 2 and Table 4). Inoculation significantly increased nodule dry weight per plant from 11.4 mg (control) to an average of 135 mg (Table 4). The nodule dry weight of a particular treatment at a specific site generally was not related to those with nodule population due to the variation in the nodule sizes. Thus, treatment with the highest number of nodules may not necessarily have the highest nodule dry weight because of variability in nodule sizes. Although significantly more nodules were formed on soybean roots in Nampula (Figure 1), the nodule dry weight (data not presented) did not show any notable trend for the different bio-inoculants and seasons due to the high variability in the data. According to the manufacturer's specifications, Masterfix had the highest units forming colonies per gram (5.0 × 109 CFU g−1) (Table 3). When bio-inoculants were averaged across environments, Masterfix formed heavier nodules per plant (163.0 mg) against the lowest value of 115.1 mg for Soygro liquid (Table 4). In addition, nodulation was distinct by season; nodules formed in 2016 (142.3 mg per plant) were significantly heavier than in the 2017 growing season with 126.7 mg when averaged across the bio-inoculant source although the reverse was observed in Ruace, where 2016 had lighter nodules than 2017.
The results indicated that there were no statistical differences in the plant tissue N content at podding stage across sites. However, nitrogen content in the plant tissue for the varieties in Angonia and Nampula in 2016 were different (Table 5) and there was a significant interaction between variety and bio-inoculant at Nampula in the same season. In the 2016 growing season, Storm plant tissue had a significantly higher percent N than that for TGx 1904-6F for both sites at Angonia and Nampula. The N tissue contents for Ruace 2016, Angonia, and Nampula 2017 were comparable at 4.4, 3.3, and 4.0%, respectively (Table 5). At Nampula in 2016, the plant tissue N content for the varieties varied with the bio-inoculant application. Plant tissue of Storm plants inoculated with Nitrofix had the highest N content (250.3 kg N ha−1), while the control had the lowest (110.8 kg N ha−1), which was not different from that of Soygro liquid bio-inoculant. On the other hand, TGx 1904-6F plants inoculated with N-fixer had the highest tissue N content (214.3 kg N ha−1), whereas the control was the lowest (102.9 kg N ha−1). The Storm had more N in biomass at 165.9 kg N ha−1 than TGx 1904-6F with 147.7 kg N ha−1 (Figure 3). Storm development is faster than the TGx 1904-6F, which is a medium maturity variety. There were more pods initiated on Storm than TGx 1904-6F by the time of sampling hence higher N demand for the variety. In general, the application of bio-inoculants resulted in more plant N uptake at the Nampula site, which had the lowest number of indigenous rhizobia.
Table 5. Varietal differences of mid-season biomass (R3) percent nitrogen content of inoculated soybean.
Figure 3. N uptake by soybean at the beginning of podding (R3) stage in Nampula in 2016. Small letters on the bars indicate no significance while different letters show significant differences in means.
The data showed that site effects were prominent for yield components. Therefore, the components were analyzed for each site and season averaged across varieties. Only Masterfix and NitroZam increased 100-seed weight at Angonia in 2016, whereas in 2017, four bio-inoculants, including Biofix, Masterfix, Nitrofix, and NitroZam, increased the 100-seed weight at this site (Table 6). No significant response to inoculation occurred for 100-seed weight at Nampula and Ruace except for the increase in 100-seed observed for N-Fixer at Ruace in 2017. Across all the sites, soybean seeds were bigger in the 2017 than in the 2016 season. Harvest biomass, on the other hand, was not affected by inoculation in Angonia and Ruace in both seasons. In Angonia, harvest biomass ranged from 5,077 kg ha−1 for the control to 8,899 kg ha−1 for the NitroZam treatment, whereas in Ruace it ranged from 4,533 kg ha−1 for the control to 6,343 kg ha−1 for the Nitrofix treatment (Table 7). However, the harvested biomass of all the bio-inoculant treatments in Nampula was significantly higher than that of the control in both seasons. NitroZam resulted in the highest harvest biomass in 2016, whereas plants inoculated with Nitrofix had the highest harvest biomass in 2017 in Nampula. As observed earlier for 100-seed weight, more harvest biomass was produced in 2017 than in 2016 averaging 7,360 and 5,126 kg ha−1, respectively, across varieties and sites.
Table 6. 100-Seed weight (g) of inoculants during the 2016 and 2017 growing seasons at three sites Angonia, Nampula, and Ruace.
Inoculation did not have any significant effect on the number of pods produced by individual soybean plants nor did it affect the number of seeds per pod. However, variety differences were observed for seed pod−1 and 100-seed weight for most of the environments except for Nampula in 2017 (Table 8). The number of seeds pod−1 was statistically higher for Storm than that for TGx 1904-6F in all environments. Storm had bigger seeds across sites and seasons with an average 100-seed weight of 15.4 g compared to that of TGx 1904-6F, which averaged 13.1 g (Table 8). Our data also showed that variety and bio-inoculant interaction had an inverse relationship between the seeds pod−1 and pods plant−1 at Ruace in the 2017 growing season (Figure 4). As the pods plant−1 increased, the seeds pod−1 declined linearly with an r2 = 0.84. Yield components namely pods per plant, seeds per pod, and seed weight are important parameters that determine yield. These components have a complex relationship that could either be counteractive or complementary in determining yield within different agroecologies.
Figure 4. Variety by inoculant interactions of pods per plant and seeds per pod at Ruace in the 2017 growing season.
Bio-inoculant application increased grain yield in all environments compared to the control, although the yield differences were generally not significant (Figure 5). In Angonia, inoculation with N-fixer gave the highest yield increase of 806 kg ha−1 for Storm, whereas Masterfix resulted in the greatest yield increase of 768 kg ha−1 for TGx 1904-6F in 2016. Similarly, yield increases in Nampula due to inoculation were also higher in 2016 than in 2017 for TGx 1904-6F. NitroZam had the best performance on both Storm and TGx 1904-6F in Nampula; almost tripling Storm yield from 701 kg ha−1 to 2,000 kg ha−1 and TGx 1904-6F by 2.3 times from 1,350 kg ha−1 to almost 3,100 kg ha−1. At Ruace, the highest yield increase of 70% (1,000–1,700 kg ha−1) occurred in 2016 for Storm when inoculated with either Biofix, Masterfix, Nitrofix, or NitroZam. TGx 1904-6F in combination with NitroZam produced the highest yield for that variety at Ruace in 2017. NitroZam doubled the yield of TGx 1904-6F from 1,350 to 2,900 kg ha−1. Generally, grain yields for TGx 1904-6F were higher than that for Storm when average across bio-inoculant treatments, sites, and seasons (Table 8). All the bio-inoculants had statistically higher yields than the control for Storm in Angonia 2016 and Nampula 2016, 2017, and for TGx 1904-6F in Nampula 2016, 2017 and Ruace 2017. Inoculation yield was significantly higher than the control for Storm in Angonia 2016 at 1,550 vs. 966 kg ha−1, Nampula 2016 at 1,583 vs. 701 kg ha−1, and Nampula 2017 at 1,466 vs. 791 kg ha−1. Inoculating TGx 1904-6F, on the other hand, yielded distinctively over the control in Nampula 2017 at 2,341 over 1,449 kg ha−1 and Ruace 2017 with 2,500 against 1,366 kg ha−1 (Figure 5). The interaction between variety and bio-inoculant on yield was diverse within each environment either numerically or statistically different from the control. For instance, in Angonia 2016, N-Fixer and Soygro liquid yields were numerically different from the control but not statistically distinct. In the same site in 2017, the application of bio-inoculants led to a numerically higher yield but not significantly above the control. Inoculation yielded 1,973 kg ha−1 against the control with 1,741 kg ha−1 for Storm, whereas only NitroZam bio-inoculant (2413 kg ha−1) had a significantly higher yield than the control with 1,913 kg ha−1 (Figure 5). At Angonia, yield did not generally respond to the bio-inoculant application in both seasons except for NitroZam in 2017. Our results show that the production of soybean gave yield between 701 and 1,741 kg ha−1 for Storm and between 1,019 and 1,913 kg ha−1 for TGx 1904-6F, while the yield range for inoculating Storm was 1,086 kg ha−1 and for TGx 1904-6F was 1,357–2,941 kg ha−1 (Figure 5). These results show that inoculation leads to an increase in soybean yield.
Figure 5. Inoculated soybean yield in (A) Angonia 2016, (B) Angonia 2017, (C) Nampula 2016, (D) Nampula 2017, (E) Ruace 2016, and (F) Ruace 2017 growing seasons. Small letters on the bars indicate no significance while different letters show significant differences in means.
Contrast analyses confirm the importance of inoculation and the differences in the variety productivity (Figure 6). However, the different yield gains due to the application of bio-inoculant and variety used were more pronounced in Nampula than Angonia and Ruace. At Angonia, bio-inoculant application regardless of grade/carrier led to a yield increase of 425 kg ha−1. When segregated by the carrier, liquid-based bio-inoculant resulted in an average yield increase of 201 kg ha−1, while the peat-based bio-inoculant gave a higher yield increase averaging at 462 kg ha−1 (Figure 6). At the Nampula site, the yield increase with the application of bio-inoculants was 1,103 kg ha−1, which decreased for a liquid-based carrier to 610 kg ha−1, but was higher for peat-based bio-inoculants (1,185 kg ha−1). Similarly, yield increased by 608 kg ha−1 following the use of any bio-inoculant, but the average yield was 331 kg ha−1 for liquid-based bio-inoculant, whereas that for the peat-based bio-inoculants was 653 kg ha−1. In essence, although inoculation led to an increase in yield, peat-based bio-inoculants gave a higher yield than liquid-based bio-inoculant. The variety selected also affected yield gains. At all the sites, changing from using TGx 1904-6F instead of Storm increased yield by 117 kg ha−1, 1,019 kg ha−1, and 790 kg ha−1 in Angonia, Nampula, and Ruace, respectively. More yield gain occurred in Nampula with the use of bio-inoculants, as well as changing from a non-promiscuous variety Storm to a promiscuous TGx 1904-6F.
Figure 6. Yield gain upon application of inoculants in Angonia (A), Nampula (B), and Ruace (C) across two growing seasons in 2016 and 2017.
Soil analysis indicated differences in nutrient content between the sites. Apart from the bio-inoculant application, other soil nutrients are necessary for the effectiveness of the BNF process and fertilizer use. As such, soils in the Nampula site are likely to respond to P, K, and Mg application, while the effect of N application would be prominent in Angonia. Since P availability in the soil enhances the response of bio-inoculant applications, its use in Nampula would likely lead to improved biological N fixation. Soil characteristics indicate that response to the bio-inoculant application on soybean would be observed more in Angonia and Nampula than Ruace. The response to inoculation would also depend on the concentration and viability of the strain contained in the bio-inoculant. The concentration of viable rhizobia strains and their survival in the carrier is an indicator of the effectiveness of a bio-inoculant. In our study, Masterfix bio-inoculant had the highest CFU g−1 (Table 3) and was expected to form more nodules, especially in Nampula where the native rhizobial population was low, but this was demonstrated at Ruace in 2016 (Figure 1) and in terms of the average nodule dry weight (Table 4). Vandeir et al. (2020) reported that Masterfix bio-inoculant with 6.91 × 108 CFU mL−1, which had a higher concentration of Azospirillum cells resulted in more agronomic activity leading to more root, shoot biomass, and yield than Nitro 100 with 4.79 × 108 CFU mL−1 in rice. These results agree with our finding that Masterfix with 5.0 × 109 CFU g−1 led to more nodulation in most of the environments. Perhaps due to the large number of CFU in the bio-inoculant, more cells were able to form associations with the host plant. Inoculation led to the formation of more nodules in Nampula than that at the other two sites (Figure 1), which could be due to the low population of indigenous rhizobia in the soil at Nampula. The presence of a large population of native rhizobia in the soil affects the effectiveness of bio-inoculant strains because of the competition for nodule formation and nodule occupancy on roots (Thies et al., 1991). Perhaps there was less competition from the resident strains in Nampula leading to more nodule formation compared to that in Angonia and Ruace, which had a relatively large indigenous population. Nodulation occurred in the non-inoculated plots, which indicates that the indigenous rhizobia also formed nodules but were not as effective as the bio-inoculant strains. Therefore, there is a need for bio-inoculant application to boost soybean production in Mozambique.
Bio-inoculant carrier influences the effectiveness of inoculation response (Vandeir et al., 2020). Peat-based bio-inoculants are the most popular in Mozambique, but there is a liquid-based bio-inoculant formulation produced in South Africa, which could be available to soybean producers in the country. Hence, the inclusion of the liquid-based bio-inoculant to test the efficacy in relation to the peat-based bio-inoculant. Peat has been shown to protect the rhizobia strains better than liquid carriers (Thao et al., 2002). Inoculating soybean with peat-based bio-inoculant resulted in more nodulation than the liquid-based counterparts in Vietnam (Thao et al., 2002). They reported that the peat-based bio-inoculants formed on average 16 nodules plant−1 with a dry weight of 351 mg plant−1, which was statistically higher than that for the liquid-based bio-inoculants with 12 plant−1 and 280 mg dry plant−1, respectively. These results agree with our findings where the peat-based bio-inoculants led to more nodulation and hence increased yield over the liquid-based bio-inoculant in Angonia 2016, Ruace 2016, and Ruace 2017. Therefore, it is evident that peat-based bio-inoculants hold a higher potential in increasing the nodulation of soybean in Mozambique than the liquid-based bio-inoculants. This could be attributed to the difference in the handling and management of the bio-inoculants before planting and the soil conditions in which the rhizobia strains are introduced. Peat seems to offer better protection to the bio-inoculant strains both during transportation, as well as when in the soil before attaching to the host plant (Vandeir et al., 2020). However, contrasting results were reported in Gauteng and Northwest province of South Africa where more nodules were formed on soybean with liquid than peat bio-inoculant (Gatabazi et al., 2021). Since rhizobia strains contained in the bio-inoculants are live cells, its packaging defines handling and transportation logistics for a country like Mozambique, where all the soybean bio-inoculants are imported from as far as the South American continent. Peat bio-inoculants have a better buffer capacity for the strains against environmental factors like temperature during transportation, storage, or soon after application. Peat-based bio-inoculants have also been shown to outperform liquid bio-inoculants in rice (Vandeir et al., 2020). In this study, peat-based bio-inoculant Nitro 1000 led to better plant development than its liquid counterparts. The low capacity of African agricultural systems to store bio-inoculants in good conditions for a long time has been reported (Woomer et al., 2014). Due to the lack of storage facilities among the smallholder farmers in Mozambique, the likelihood of liquid-based bio-inoculant quality deteriorating before the application is high because of the low buffering capacity offered to the rhizobia strains.
Plant tissue N is translocated to the reproductive parts during growth and development. However, some of the N remains in the nonreproductive plant parts and could be recycled back into the soil to improve soil fertility. Soybean acquires its N both from the soil directly and through the BNF process that takes place effectively when an appropriate rhizobia strain is present to form symbiotic associations with the host plant. The soils in Nampula had a very low population of soybean rhizobia strain (Table 2), because there was no competition from the resident rhizobia leading to more attachment by the introduced cells to the soybean roots. Legumes growing in soils with low populations of indigenous rhizobia increase nodulation when the right strain is applied (Sanginga et al., 2002; Majengo et al., 2011). Our finding corroborates with a study conducted in Eastern Ethiopia where nodulation increased in common beans planted in soils with different populations of indigenous rhizobia (Argaw and Angaw, 2015). In the same study, there were more nodules formed with an increase in the native rhizobia from 82.3 for soils with <100 common bean rhizobia g−1 soil to 186.7 for soils with more than 1,000 common bean rhizobia g−1 soil. As the indigenous rhizobia strains increased, the number of nodules formed on the common bean roots was also higher. Although promiscuous soybean varieties can form nodules freely with indigenous strains, the application of bio-inoculant improved nodulation (Sanginga et al., 2002; Osunde et al., 2003; Gitonga et al., 2011). Our results also showed that nodulation increased with the inoculation of the promiscuous variety TGx 1904-6F more than that for the non-promiscuous Storm.
Although our study did not determine nodule occupancy and activity of the nodules, plant N uptake at the R3 stage was measured. The amount of N accumulated in the plant tissue and yield obtained to serve as pseudo indicators of the BNF process following inoculation. It is presumed that improved nodulation leads to an increased BNF process that avails more N for plant growth and development. The soybean varieties had accumulated different amounts of N in their tissues at the R3 stage. Our results show that inoculated non-promiscuous variety Storm accumulated more N than promiscuous TGx 1904-6F at the R3 stage. Storm is an early maturing soybean variety, hence accumulated N at a faster rate during growth and development than the TGx 1904-6F. Inoculated soybean has been shown to accumulate more than 42% of the total N by the R3/R4 (beginning of podding to beginning of seed) than the inoculated counterparts (Sanginga et al., 1997). Early maturing promiscuous soybean varieties attain maximum N accumulation earlier than medium to late maturity groups. Sanginga et al. (1997) reported that IAC 100 reached maximum N accumulation at R1/R2 stages (beginning of flowering to full boom), while the late maturity promiscuous soybean variety TGx 1519-1D peak was at R3/R4 stage. Our findings where Storm had more tissue N content confirm this study's report (Table 5). Based on the biomass amount at the R3 stage, the total N accumulated in the plants per hectare was determined (Figure 3). Nitrogen accumulated in the plant biomass at the R3 stage increased with inoculation but was distinct with bio-inoculant interaction with the soybean variety. Other studies have reported an increase in N amount accumulated in plant tissue due to inoculation (Gitonga et al., 2011; Savala et al., 2021, 2022; Omari et al., 2022). Storm accumulated more N per ha for the Biofix, Masterfix, Nitrofix, and NitroZam, while TGx 1904-6F had more N when inoculated with N-Fixer and Soygro. These findings contrasted with results from another study in the same locations of Angonia and Ruace where early maturity promiscuous soybean variety TGx 1740-2F accumulated more N by the R3 stage than Safari a non-promiscuous variety (Savala et al., 2021). This signifies that the amount of N tissue at the R3 stage could be variety-related rather than the differences in promiscuity. Not all the amount of biomass N accumulated is translocated to the reproductive parts. Part of the N remains in the biomass and could be recycled back into the soil and added to the residual N for use by the next crop. Inoculation leads to more biomass N accumulation that is important for improving soil N (Omari et al., 2022). Our results confirmed that inoculation leads to more biomass production in all the sites (Table 7). Therefore, we infer that inoculating soybean not only increases the yield but also leads to more biomass production that is important in improving the soil nitrogen through recycling. As such, recycling of inoculated soybean harvest biomass contributes to improving the overall soil health thereby reducing the need for external N sources.
The interaction between the environment, on one hand, and bio-inoculant or variety affected yield components, such as 100-seed weight, seed pod−1, and pods plant−1, differently. This interaction is significant in defining the suitability of bio-inoculants and soybean variety adaptation in specific locations. Although not all applied bio-inoculants resulted in an increase in 100-seed weight, Biofix, Masterfix, Nitrofix, and NitroZam gave higher values than the control in Angonia. The a pplication of bio-inoculants in soybean has been reported to result in increased seed quality and weight that positively increases yield (Gitonga et al., 2011; Tavanti et al., 2020). On the other hand, environment and variety interaction affected 100-seed weight, seeds pod−1, and pods plant−1 (Table 8). Storm had a statistically higher 100-seed weight in all environments than TGx 1904-6F. The 100-seed weight was not affected by the bio-inoculant differences as it is a characteristic controlled more by the genotype's genetic capability. Although the seed weight slightly increased following inoculation, the difference was not statistically significant. Increased numerical values of 100-seed weight were also demonstrated in a study that assessed the productivity of two soybean cultivars upon application of N mineral fertilizers and seed inoculation with Bradyrhizobium diazoefficiens (Ksiezak and Jolanta, 2022). Other yield components that are affected with inoculation are seeds pod−1 and pods plant−1. In our study, we confirmed that seeds pod−1 did not increase with the application of bio-inoculants but rather varied with variety. Storm gave statistically more seeds per pod in four environments, while TGx 1904-6F had many seeds pod−1 in Angonia 2016 only. Like 100-seed weight, seeds pod−1 plant trait is affected more by the genetics of the plant in soybean. However, past studies have reported a greater number of seeds pod−1 following inoculation. Lamptey et al. (2014) and Ksiezak and Jolanta (2022) demonstrated that soybean formed more seeds pod−1 due to inoculation. In these studies, bio-inoculants were combined with other nutrients, such as iron and nitrogen, making it complex to attribute the increase in seeds pod−1 to rhizobia strain.
Improvement in soybean yield due to inoculation has been widely documented. Our study did not fall short of ascertaining the same findings but also showed that variety selection affected soybean yield differently in the specific sites. It was more advantageous growing TGx 1904-6F in Nampula than Storm, whereas both varieties yielded comparable in Angonia. Gitonga et al. (2011) also reported a higher yield between 27.8 and 39.3% of Biofix inoculated TGx 1869 and TGx 1893 promiscuous soybean over the non-promiscuous Gazelle variety at Kaguru, Meru District in Kenya. Similar results have also been documented at Ruace in Mozambique where TGx 1740-2F out-yielded Safari (Savala et al., 2022). In the same study, inoculated Safari gave more yields than TGx 1740-2F at Angonia. Though our yield analysis indicates that promiscuous soybean TGx 1904-6F performed better than Storm, it is profound that there exists a difference in variety suitability for various specific agroecological sites. Therefore, variety selection should consider existing climatical and soil conditions for each site. For instance, Angonia and Ruace have suitable soil conditions and weather for soybean production, hence, both promiscuous and non-promiscuous varieties can be produced. However, the selection of the variety will depend on the weather forecast especially expected season rainfall distribution and amount. Yield also increased with inoculation, but the productivity varied with the site. Bio-inoculant effect on yield was distinct with carrier too. Peat-based bio-inoculants had higher yields than their liquid counterparts. As earlier reported, in the nodulation section, environmental factors could have affected the efficacy of the liquid-based bio-inoculants hence leading to low symbiotic associations for the BNF process. With reduced BNF process in liquid-based bio-inoculant across the sites, the low nitrogen necessary for seed development could have been fixed. This study confirms findings that peat bio-inoculant yielded more than liquid-based bio-inoculants. A study conducted in Vietnam also reported that peat-based bio-inoculants yielded 2.00 and 2.18 t ha−1 against the liquid-based counterparts 1.96 and 2.12 t ha−1 for two rhizobia strains designated as G5 and G6, respectively (Thao et al., 2001). Contrasting studies have also shown that liquid-based bio-inoculant yields more than peat-based bio-inoculants (Hungria et al., 2020; Gatabazi et al., 2021). This justifies that both liquid and peat-based bio-inoculants lead to increased yield in soybean although their effectiveness would depend on handling and the prevailing agroecological conditions. Although bio-inoculants lead to improved productivity in soybean, the key to their effectiveness could lie in the handling and prevailing environmental conditions. The bottom line is that inoculation is necessary for increasing soybean yield. The availability of bio-inoculants holds the potential for better soybean yields for smallholder farmers in Mozambique. This study showed that the use of bio-inoculants increased yield between 201 and 462 kg ha−1 (Figure 6). Considering the cost of bio-inoculants in Mozambique at USD 10 per hectare and the grain selling price of USD 0.90 kg−1, the use of bio-inoculants would result in a monetary profit of USD 170.9–405.8 per hectare.
Application of bio-inoculants has the potential of increasing soybean production in Mozambique regardless of the strain carrier material. Both liquid- and peat-based bio-inoculants led to increased nodulation and yield of soybean over the control. Inoculation improved nodulation and yield at different proportions in soybean production regardless of the strain carrier material depending on the agroecological conditions, such as the population of indigenous rhizobia strain. There was more increase in nodulation in the Nampula site that had the lowest number of indigenous rhizobia strains. However, more nodules and yield were observed with the application of peat-based bio-inoculants than the liquid-based counterparts. The economic gain from yield improvement justifies the use of bio-inoculants for soybean production in Mozambique. This can be further increased if the country produces bio-inoculants locally. Locally producing bio-inoculants has the potential of reducing the cost of the input and will make it accessible and affordable for smallholder farmers in Mozambique. As noted in the study, inoculation improved plant development and production of more biomass with high N content. The magnitude of plant development and biomass production due to the application of bio-inoculants depends on the prevailing agroecological conditions. There was more biomass production in Angonia and Ruace than Nampula site. Angonia and Ruace agroecologies receive relatively more rains and are more suitable for soybean production than Nampula. This biomass could be recycled back to the soil to improve the fertility status, especially N content that would benefit the subsequent crop. In addition to bio-inoculant use, more study is required by the breeders to produce better phosphorus scavenging promiscuous soybean that would effectively form symbiotic associations with indigenous rhizobia strains. Moreover, additional study is required to ascertain nodule occupancy and activity when bio-inoculants are applied in soils with native rhizobia, especially for the promiscuous soybean varieties.
The raw data supporting the conclusions of this article will be made available by the authors, without undue reservation.
CS, SK-B, and DC designed and supervised the experiment. CS participated in field evaluation, data compilation, and oversaw writing up. CS and AW conducted data analysis. All authors contributed to the preparation of the manuscript.
Funding was provided by the USAID through Grant #EEM-G-00-04-000136-00 Platform for Agricultural Research and Technology Innovation and Feed the Future Mozambique Improved Seeds for Better Agriculture (SEMEAR) Grant #AID-BFS-IO-17-00005 projects in Mozambique.
The authors declare that the research was conducted in the absence of any commercial or financial relationships that could be construed as a potential conflict of interest.
All claims expressed in this article are solely those of the authors and do not necessarily represent those of their affiliated organizations, or those of the publisher, the editors and the reviewers. Any product that may be evaluated in this article, or claim that may be made by its manufacturer, is not guaranteed or endorsed by the publisher.
We thank the IITA technical staff Rachad Ussene at Nampula, Irondino Saraiva at Angonia, Carlos Muananamuale, Francisco Domingos, and Mariano Aniceito at Ruace station in Mozambique for managing the trials and assisting in collecting the field related data.
AGRA (2016). Mozambique Early Generation Seed Study. Available online at: https://agrilinks.org/sites/default/files/resource/files/mozambique_early_generation_seed_report.pdf (accessed March 16, 2022).
Argaw, A., and Angaw, T. (2015). Indigenous rhizobia population influences the effectiveness of rhizobium inoculation and need of inorganic N for common bean (Phaseolus Vulgaris L.) production in Eastern Ethiopia. Chemical and Biological Technologies in Agriculture 2, 1–13. doi: 10.1186/s40538-015-0047-z
Chibeba, A. M., Kyei-Boahen, S., Maria, F. G., Marco, A. N., and Mariangela, H. (2017). Isolation, characterization and selection of indigenous bradyrhizobium strains with outstanding symbiotic performance to increase soybean yields in mozambique. Agricult. Ecosyst. Environ. 246, 291–305. doi: 10.1016/j.agee.2017.06.017
Chibeba, A. M., Kyei-Boahen, S., Maria, F. G., Marco, A. N., and Mariangela, H. (2020). Towards sustainable yield improvement: field inoculation of soybean with bradyrhizobium and co-inoculation with azospirillum in mozambique. Arch. Microbiol. 202, 2579–90. doi: 10.1007/s00203-020-01976-y
Delamuta, J. R. M., Renan, A. R., Ernesto, O., Itamar, S. M., Esperanza, M., Mariangela, H., et al. (2013). Polyphasic evidence supporting the reclassification of bradyrhizobium japonicum group ia strains as Bradyrhizobium diazoefficiens Sp. Nov. Int. J. Syst. Evol. Microbiol. 63, 3342–51. doi: 10.1099/ijs.0.049130-0
Gatabazi, A., Barend, J. V., Mireille, A. M., Edgar, M., Robert, M., Ahmed, I. H., et al. (2021). Efficacy of peat and liquid inoculant formulations of bradyrhizobium japonicum strain wb74 on growth, yield and nitrogen concentration of soybean (Glycine max L.). Nitrogen. 2, 332–46. doi: 10.3390/nitrogen2030023
Gitonga, M. N., Gatheri, G. W., Cheruiyot, R., Gitonga, N. M., and Maingi, J. M. (2011). Nodulation and nitrogen fixation in promiscuous and non-promiscuous soybean (Glycine max (L.) Merrill) Varieties in Eastern Kenya. J. Trop. Microbiol. Biotechnology 6. doi: 10.4314/jtmb.v6i1.63744
Gyogluu, C., Kyei-Boahen, S., and Dakora, F. D. (2016). Response of Promiscuous-Nodulating Soybean (Glycine max L. Merr.) genotypes to bradyrhizobium inoculation at three field sites in mozambique. Symbiosis 69, 81–88. doi: 10.1007/s13199-015-0376-5
Hungria, M., Marco, A. N., Campos, L. J. M., Menna, P., Brandi, F., Ramos, Y. G., et al. (2020). Seed pre-inoculation with bradyrhizobium as time-optimizing option for large-scale soybean cropping systems. Agron. J. 112, 5222–36. doi: 10.1002/agj2.20392
Jones, A., Breuning-Madsen, H., Brossard, M., Dampha, A., Deckers, J., Dewitte, O. (eds)., . (2013). Soil Atlas of Africa. Luxembourg: European Commission. ISBN 978-92-79-26715-4.
Ksiezak, J., and Jolanta, B. (2022). The effect of mineral n fertilization and bradyrhizobium japonicum seed inoculation on productivity of soybean (Glycine max (L.) Merrill). Agriculture (Switzerland). 12, agriculture12010110. doi: 10.3390/agriculture12010110
Kyei-Boahen, S., Savala, C. E. N., Chikoye, D., and Abaidoo, R. (2017). Growth and yield responses of cowpea to inoculation and phosphorus fertilization in different. Environments. 8, 1–13. doi: 10.3389/fpls.2017.00646
Lamptey, S., Ahiabor, B., Yeboah, S., and Osei, D. (2014). Effect of rhizobium inoculants and reproductive growth stages on shoot biomass and yield of soybean (Glycine max (L.) Merril). J. Agricult. Sci. 6, v6n5p44. doi: 10.5539/jas.v6n5p44
Majengo, C. O., John Okalebo, R., Lesueur, D., Pypers, P., Ng'etich, W., Musyoki, M., et al. (2011). Interaction between nitrogen and phosphorus microbial inoculants on soybean production in Bungoma, Kenya. Afr. Crop Sci. Conf. Proc. 10, 117–19.
Matteo, F., Otsuki, K., and Schoneveld, G. (2016). “Soya bean expansion in mozambique: exploring the inclusiveness and viability of soya business models as an alternative to the land grab,” in The Public Sphere. 60–86.
Moore, K. J., and Dixon, P. M. (2015). Analysis of Combined Experiments Revisited. Agron. J. 107, 763–71. doi: 10.2134/agronj13.0485
Omari, R. A., Yuan, K., Anh, K. T., Reckling, M., Halwani, M., Egamberdieva, D., et al. (2022). Enhanced soybean productivity by inoculation with indigenous bradyrhizobium strains in agroecological conditions of northeast germany. Front. Plant Sci. 12, 3276. doi: 10.3389/fpls.2021.707080
Osunde, A., Gwam, S., Bala, A., Sanginga, N., and Okogun, J. A. (2003). Responses to Rhizobial Inoculation by Two Promiscuous Soybean Cultivars in Soils of the Southern Guinea Savanna Zone of Nigeria. Biolo. Fert. Soils. 37, 274–79. doi: 10.1007/s00374-003-0609-2
Sanginga, N., Dashiell, K., Okogun, J. A., and Thottappilly, G. (1997). Nitrogen Fixation and N Contribution by Promiscuous Nodulating Soybeans in the Southern Guinea Savanna of Nigeria. Plant and Soil 195, 257–66. doi: 10.1023/A:1004207530131
Sanginga, N., Okogun, J. A., Vanlauwe, B., and Dashiell, K. (2002). The Contribution of Nitrogen by Promiscuous Soybeans to Maize Based Cropping the Moist Savanna of Nigeria. Plant and Soil 241, 223–31. doi: 10.1023/A:1016192514568
SAS/STAT® (2018). 15, 1. User's Guide the GLM Procedure. http://support.sas.com/thirdpartylicenses.
Savala, C. E. N., Chikoye, D., and Kyei-Boahen, S. (2022). Inoculant Formulation and Application Determine Nitrogen Availability and Water Use Efficiency in Soybean Production. In Soybean - Recent Advances in Research and Applications. 102639. doi: 10.5772/intechopen.102639
Savala, C. E. N., and Kyei-Boahen, S. (2020). Potential of Inoculant and Phosphorus Application on Soybean Production in Mozambique. Universal Journal of Agricultural Research 8, 46–57. 2020.080204. doi: 10.13189/ujar.2020.080204
Savala, C. E. N., Wiredu, A. N., Okoth, J. O., and Kyei-Boahen, S. (2021). Inoculant, Nitrogen and Phosphorus Improves Photosynthesis and Water-Use Efficiency in Soybean Production. Journal of Agricultural Science 159, 349–62. doi: 10.1017/S0021859621000617
Singleton, P. W., Abdelmagid, H. M., and Tavares, J. W. (1985). Effect of Phosphorus on the Effectiveness of Strains of Rhizobium Japonicum. Soil Science Society of America Journal 49, 613–16. 03615995004900030016x. doi: 10.2136/sssaj1985.03615995004900030016x
Singleton, P. W., Keyser, H., and Sande, E. (2001). Development and Evaluation of Liquid Inoculants. Inoculants and Nitrogen Fixation of Legumes in Vietnam Edited by D. Herridge ACIAR Proceedings, 109e. https://www.researchgate.net/publication/242193371.
Tavanti, T. R., Renan, F. R., Tavanti, F. S., Galindo, I. S., Larissa, S. D., and Sá, M. E. (2020). Yield and Quality of Soybean Seeds Inoculated with Bacillus Subtilis Strains. Revista Brasileira de Engenharia Agricola e Ambiental 24, 65–71. v24n1p65-71. doi: 10.1590/1807-1929/agriambi.v24n1p65-71
Thao, T. Y., Singleton, P. W., and Herridge, D. (2001). Inoculation Responses of Soybean and Liquid Inoculants as an Alternative to Peat-Based Inoculants. https://www.researchgate.net/publication/240630719.
Thao, T. Y., Singleton, P. W., and Herridge, D. (2002). Inoculants and Nitrogen Fixation of Legumes in Vietnam Edited by D. Herridge ACIAR Proceedings. Vol. 109.
Thies, J. E., Singleton, P. W., and Bohlool, B. (1991). Influence of the Size of Indigenous Rhizobial Populations on Establishment and Symbiotic Performance of Introduced Rhizobia on Field-Grown Legumest. Applied and Environmental Microbiology. Vol. 57. https://journals.asm.org/journal/aem. doi: 10.1128/aem.57.1.19-28.1991
Tittabutr, P., Waraporn, P., Neung, T., Singleton, P. W., and Nantakorn, B. (2007). Growth, Survivaland Field Performance of Bradyrhizobial Liquid Inoculant Formulations with Polymeric Additives. ScienceAsia 33, 69–77. 33, 069. doi: 10.2306/scienceasia1513-1874.2007.33.069
van Heerwaarden, J., Baijukya, F., Kyei-Boahen, S., Adjei-Nsiah, S., Ebanyat, P., Kamai, N., et al. (2018). Soyabean response to rhizobium inoculation across sub-saharan africa: patterns of variation and the role of promiscuity. Agricult. Ecosyst. Environ. 261, 211–18. doi: 10.1016/j.agee.2017.08.016
Vandeir, F. G., Klein, J., Barbosa, F. M., and Kestring, K. D. (2020). Promotion of rice growth and productivity as a result of seed inoculation with Azospirillum brasilense. Afr. J. Agricult. Res. 16, 765–76. doi: 10.5897/AJAR2020.14723
Vincent, J. M.. (1970). A Manual for the Practical Study of the Root-Nodule Bacteria (Oxford and Edinburgh: Blackwell Scientific Publ.),164.
Walker, T., Pitoro, R., Tomo, A., Sitoe, I., Salência, C., Mahanzule, R., et al. (2006). Institute of Agricultural Research of Mozambique Directorate of Training, Documentation, and Technology Transfer Research Report Series Republic of Mozambique Priority Setting for Public-Sector Agricultural Research in Mozambique with the National Agricultural Survey Data.
Woomer, P. L., Bennett, J., and Yost, R. (1990). Overcoming the inflexibility of most-probable-number procedures. Agron. J. 82, 349–53. doi: 10.2134/agronj1990.00021962008200020035x
Woomer, P. L., Mahamadi, D., Wafullah, T. N., Balume, I., Uwizerwa, M., Kyei-Boahen, S., et al. (2014). N2Africa Putting Nitrogen Fixation to Work for Smallholder Farmers in Africa Improving Legume Inoculants and Developing Strategic Alliances for Their Advancement. Available online at: http://www.N2Africa.org (accessed March 15, 2022).
Keywords: biological nitrogen fixation, ecology, nodulation, non-promiscuous, promiscuous, rhizobia, yield
Citation: Savala CEN, Wiredu AN, Chikoye D and Kyei-Boahen S (2022) Prospects and Potential of Bradyrhizobium diazoefficiens Based Bio-Inoculants on Soybean Production in Different Agro-Ecologies of Mozambique. Front. Sustain. Food Syst. 6:908231. doi: 10.3389/fsufs.2022.908231
Received: 30 March 2022; Accepted: 03 June 2022;
Published: 08 July 2022.
Edited by:
Petr Smýkal, Palacký University Olomouc, CzechiaReviewed by:
Tomas Vymyslicky, Agricultural Research, Ltd., CzechiaCopyright © 2022 Savala, Wiredu, Chikoye and Kyei-Boahen. This is an open-access article distributed under the terms of the Creative Commons Attribution License (CC BY). The use, distribution or reproduction in other forums is permitted, provided the original author(s) and the copyright owner(s) are credited and that the original publication in this journal is cited, in accordance with accepted academic practice. No use, distribution or reproduction is permitted which does not comply with these terms.
*Correspondence: Canon Engoke Norris Savala, Y25zYXZhbGFAZ21haWwuY29t
Disclaimer: All claims expressed in this article are solely those of the authors and do not necessarily represent those of their affiliated organizations, or those of the publisher, the editors and the reviewers. Any product that may be evaluated in this article or claim that may be made by its manufacturer is not guaranteed or endorsed by the publisher.
Research integrity at Frontiers
Learn more about the work of our research integrity team to safeguard the quality of each article we publish.