- 1Faculty of Agriculture Udayana University, Denpasar, Indonesia
- 2Laboratory of Biopesticide, Faculty of Agriculture, Udayana University, Denpasar, Indonesia
- 3Laboratory of Soil Science, Faculty of Agriculture, Udayana University, Denpasar, Indonesia
- 4Department of Biology, Faculty of Mathematics and Natural Science, Udayana University, Badung, Indonesia
Corn productivity in Indonesia is still relatively low compared with other countries. Therefore, it is necessary to increase the productivity of corn by using rhizobacteria, which have multiple traits. This study was conducted to obtain indigenous rhizobacteria of Bali that have multiple traits, can produce indoleacetic acid (IAA), fix nitrogen from atmosphere, produce siderophores, colonize roots, increase seed germination, and promote the growth of corn. Isolation of rhizobacteria was carried out from the rhizosphere of plants belonging to the Gramineae family that grows in Bali Island, Indonesia. Six isolates, namely, Sr3, Tb9, Rg1, Rg23, Al27, and Jg8, could produce IAA, fix nitrogen from the atmosphere, produce siderophores, and increased germination rate and vigor index of corn seedling. Among them, three isolates, namely, Rg1, Sr3, and Jg8, significantly (p <0.05) increased the germination rate of corn seeds, increased vigor index, increased root dry weight and shoot dry weight of corn at the age of 7 days, and were able to colonize corn roots. Compared with the control, the rhizobacteria treatment increased the germination of corn seeds ranging from 5.04 to 13.05%. Based on the analysis of the 16S rRNA gene, it was found that these rhizobacteria species were Glutamicibacter nicotianae strain Rg1 (accession number OM349119), Brevibacillus invocatus strain Sr3 (accession number OM327515), and Micrococcus luteus strain Jg8 (accession number OM362349). Under a greenhouse condition, all the three isolates significantly (p <0.05) increased nutrient uptake, the leaf chlorophyll content, net assimilation rate, and crop growth rate of corn when compared with control. These results suggested that these isolates of rhizobacteria obviously promoted the growth of corn and can be developed as biostimulant to promote the growth and increase the corn yield in Bali, Indonesia.
Introduction
Corn (Zea mays L.) is one of the agricultural commodities with high economic value. This commodity is used as raw material for animal feed industry, corn flour, non-cholesterol vegetable oil, and bioethanol, as well as raw materials for processed food such as snacks and cakes (Bantacut et al., 2015). In Indonesia, domestic corn consumption continues to grow faster than the national production, which has forced the government to import corn from the United States, Brazil, and Argentina every year. In 2017, Indonesia imported 517.5 thousand tons of corn, whereas in 2018, Indonesia imported 737.2 thousand tons of corn (Ministry of Agriculture of the Republic of Indonesia., 2019). The Food and Agriculture Organization of the United Nations (FAOSTAT, 2018) reported that the average corn yield per hectare in Indonesia in 2018 was 5.32 tons/ha, lower than Austria at 10.14 tons/ha, Bangladesh at 8.21 tons/ha, Chile at 12.47 tons/ha, New Zealand at 11.06 tons/ha, Qatar at 12.52 tons/ha, and the United Arab Emirates with an average of 28.46 tons/ha in 2018. Based on this reason, efforts to increase domestic corn production in Indonesia are very much needed. One technology to increase corn production is the use of multitraits rhizobacteria that can produce several substances, which function as plant growth promoter. Rhizobacteria are bacteria that live in the rhizospheres or root areas that may produce plant growth hormones and may act as plant growth promoters (biostimulant), fix atmospheric nitrogen (biofertilizer), and produce several substances such as siderophores that can suppress plant pathogen (bioprotectant) to increase crop yields (Mendes et al., 2013).
The mechanisms of rhizobacteria in increasing plant growth directly include nitrogen fixation, phosphate dissolution, phytohormone production (Timmusk, 2003; Kundan et al., 2015; Figueiredo et al., 2016; Goswami et al., 2016), and the production of bacterial volatile compounds (BVCs) (Fincheira et al., 2017; Kanchiswamy et al., 2015; Chung et al., 2016). Nitrogen-fixing rhizobacteria are symbiotic (such as Rhizobium) and nonsymbiotic (such as Azotobacter and Azospirillum) rhizobacteria that fix free nitrogen in the atmosphere into ammonia (NH3) through a deamination process or are first converted into nitrate compounds through nitrification (Mus et al., 2016; Widawati and Suliasih, 2019). Rhizobacteria can also produce phytohormones, namely, a group of non-nutrient organic compounds, both naturally occurring and man-made, which in very low concentration can regulate plant growth and development (Sezgin and Kahya, 2018). Indoleacetic acid (IAA) is one of the phytohormones produced by rhizobacteria belonging to the auxin group. The use of rhizobacteria that fix nitrogen and produce phytohormones and siderophores has been reported by several researchers to increase plant growth and yield (Breedt et al., 2017; Khalimi et al., 2017; Adrianus et al., 2018; Akinrinlola, 2018; Gholami et al., 2018; Youseif, 2018; Wahyudi et al., 2019), but mostly with a single trait. Based on this reason, this study focuses in obtaining rhizobacteria of Bali, which can produce IAA, fix nitrogen, produce siderophores, and obviously promote the growth of corn.
Materials and Methods
Sampling and Isolation
Sampling was done by taking soil and roots from the rhizosphere of plants belonging to the Gramineae family such as Saccharum officinarum, Cymbopogon citratus, Imperata cylindrica, Zea mays, and Pennisetum purpureum. A total of 50 g of soil was taken at random from the rhizosphere of plants growing in Bali. Samples were taken from a layer with a thickness of 1–5 cm from plant roots, put in a plastic bag, and brought to the laboratory using an ice box.
Isolation of rhizobacteria was carried out based on a modified procedure developed by Khambani et al. (2019). A total of 9 ml of saline solution (0.85%) was added to 1 g of soil. The stratified dilution series was carried out with up to 10−7 dilutions. A total of 0.1 ml of suspension from 10−5 to 10−7 dilution was plated on nutrient agar (NA) medium containing 500 mg/l maintained at 28 ± 2°C for 48 h. Colonies that appeared from this medium were then streaked on NA medium to obtain single colony. This colony was maintained in a slant NA medium before being used.
Test for Morphology and Biochemical Properties
The color of bacterial colonies on nutrient agar medium, the shape of the cells, and their reaction to Gram stain were all observed to determine the isolates' morphology. Additionally, we conducted several biochemical tests, including an oxidase test, a catalase test, and a hydrogen sulfide production test.
Test for Indoleacetic Acid-Producing Ability
The screening for the ability to produce IAA was carried out following the method developed by Agustiyani (2016). All the isolates were first grown in a 5-ml tryptic soy broth in a test tube and incubated at 28 ± 2°C in the dark for 48 h. A total of 1 ml Salkowski's solution was put into test tube and the color change was observed. Appearance of a pink color in the suspension indicates that the rhizobacteria isolate are capable of producing IAA.
Test for Ability to Fix Nitrogen
All the isolates that can produce IAA were tested for their ability to fix N2 qualitatively based on the modified method developed by Imamuddin et al. (2015). Bacterial isolates were grown on nitrogen-free bromothymol blue malate medium containing 5 g malic acid, 4 g KOH, 0.5 g K2HPO4, 0.05 g FeSO4.7H2O, 0.01 g MnSO4.7H2O, 0.01 g MgSO4.7H2O, 0.02 g NaCl, 0.01 g CaCl2, 0.002 g Na2MoO4, 0.5% bromothymol blue, and 2% agar with medium pH ranging from 6.6 to 7.0. The cultures were maintained for 48 h at 28 ± 2°C. The color change of the colony to yellow indicated that rhizobacteria were positive for fixing nitrogen.
Test for Ability to Produce Siderophores
Siderophore production was detected using chrome azurol sulfonate (CAS) agar media according to the modified method developed by Khambani et al. (2019). CAS medium consists of 60.5 mg of CAS that was dissolved in 50 ml of distilled water and mixed with 10 ml of iron solution (1 mmol/l of FeCl3.6H2O and 10 mmol/l of HCl) while shaking. This solution was slowly added by 72 mg of hexadecyltrimethylammonium bromide dissolved into 40 ml of water. A total of 10 ml of CAS media was poured into a Petri dish in laminar flow and allowed to solidify. Each rhizobacteria isolate were inscribed on the surface of CAS media and then incubated for 48 h in the dark and at 28 ± 2°C. The color change of the colony to orange indicated that the isolate was positive for producing siderophores.
Test for Ability to Stimulate Seed Germination and Plant Growth
Six isolates of rhizobacteria that showed ability to produce IAA, fix nitrogen, and produce siderophores were tested for their ability to stimulate corn seed germination of corn cultivar Pertiwi that is commonly grown in Bali, Indonesia. Sterilization of corn seeds surface was done by dipping the seeds in 0.02% sodium hypochlorite for 2 min and continued by washing with sterile distilled water. The seeds were then coated with 20% gum arabic and then rolled into a bacterial suspension [106 colony-forming unit (CFU)/ml] with perlite until all the parts of the seeds were coated (Gholami et al., 2009).
Test for germination was done according to the towel paper method (Gholami et al., 2009). This experiment was designed in a completely randomized design with six treatments (five rhizobacteria isolates and one control) and each treatment was replicated four times, so that there were 24 experimental units. Each experimental unit consisted of 100 corn seeds germinated on sterile plastic tray with wet towel paper and maintained in a growth chamber at 28°C for 7 days after which the number of germinated seeds was counted. Root length and shoot length of each seedling were measured to determine the vigor index using formula 1 developed by Abdul-Baki and Anderson (1973).
Formula 1:
VI = (RL + SL) × GR (%)
Where,
VI = Vigor index
RL = Mean root length
SL = Mean shoot length
GR = Germination rate
To determine the dry weight of roots and shoots, 10 plants per experimental unit were taken as samples. Roots and shoots were separated before being dried in an oven at 60°C until the weight was constant.
Observation of Rhizobacterial Colonization on Corn Roots
Three isolates of rhizobacteria that showed the best performance in stimulating seed germination of corn seeds were observed for their ability to colonize corn roots using a scanning electron microscope (SEM). Observations with SEM were focused on the presence of bacterial cells on the roots of corn plants. The preparations for SEM were derived from germinated corn seeds, soaked in rhizobacteria suspension with a density of 106 CFU/ml. Colonization of rhizobacteria was observed with SEM and was carried out based on the method of Souissi et al. (1997). Root cut as a sample was fixed with a 2.5% glutaraldehyde solution in 100 mM phosphate buffer (pH 7.0) at 40°C for 4 h and let to stand at room temperature (25°C) for 1 h. This fixed sample was washed with sodium cacodylate buffer (pH 7.2) and fixed with 1% osmium tetroxide in 0.1 M cacodylate buffer and let to stand at room temperature for 4 h. The sample was washed with distilled water and then subjected to the dehydration process using ethyl alcohol in series (20, 40, 60, 80, 95, and 100%), after which the sample was cut using a freeze cutting device (TF-2, Eiko, Japan). The sample was then dropped with a solution of t-butyl alcohol and placed in a vacuum freeze-drying (ID-2, Eiko, Japan). The dried sample was coated with osmium tetroxide (OPC 60A, Filgen, Japan) and platinum (JUC-5000, JEOL, Japan). The sample was observed under SEM using JEOL JSM-35 (Japan) with an acceleration voltage of 20 kV.
Molecular Identification of Rhizobacteria
Analysis of 16S rRNA gene sequences was applied for rhizobacteria identification. First, DNA of rhizobacteria was extracted and purified using the GeneJET Genomic DNA Purification Kit (Thermo Fisher Scientific). The 16S rRNA gene was amplified by PCR using primer pairs 16S (63F 5′-CAG GCC TAA CAC ATG CAA GTC-3′) and 1387R (5′-GGG CGG WGT GTA CAA GGC-3′) using 2 × Kappa PCR ReadyMix (Kappa Biosystem) at 94°C for 5 min, followed by 30 consecutive cycles at 94°C for 30 s, 55°C for 45 s, and 72°C for 2 min, with the last plus 72°C for 10 min. Nucleotide sequences were determined using the ABI PRISM 3100-Avant Genetic Analyzer. The DNA sequences were then trimmed and assembled using the ChromasPro version 1.5 program. The assembled data is then proceeded to BLAST with data that has been registered in the National Center for Biotechnology Information (NCBI) through the website http://www.ncbi.nlm.nih.gov/BLAST. Several homologous sequence data from BLAST results, which are the closest species, were taken from GenBank data at the NCBI. The data were then analyzed again by aligning the sequences using the MEGA version 6.0 program. Furthermore, the data were analyzed using the PAUP 4.0b program with the maximum parsimony method with 1,000 replicates bootstrap (Calvo et al., 2005). Finally, the phylogenetic tree was designed using TreeGraph 2.0 (Stover and Muller, 2010).
Efficacy of Rhizobacteria to Promote Corn Growth
The efficacy of three isolates of rhizobacteria, namely, Sr3, Rg1, and Jg8, to promote the growth of corn, was tested in a greenhouse. The experiment was designed in a completely randomized block design with four treatments, namely, P0 (control), P1 (treated with isolate Sr3), P2 (treated with isolate Rg1), and P3 (treated with isolate Jg8). Each treatment was repeated six times, so that 24 experimental units were obtained, each consisting of 10 polybags of corn plants. Corn seeds were planted in polybags with a diameter of 30 cm and a height of 40 cm filled with fertile soil and compost with a ratio of 1:3. The treatment of the rhizobacteria suspension was carried out at the time of planting by coating the surface of corn seeds with rhizobacteria suspension with a density of 107 CFU/ml, perlite, and gum arabic. The corn variety used was Pertiwi hybrid corn, which was obtained from the kiosk of agricultural production facilities in Denpasar, Bali. Two seeds were planted per polybag. At 14 days after transplanting, one plant was discarded and another one plant was maintained until the end of the experiment.
The parameters measured were leaf chlorophyll content, leaf wet and dry weight, root wet and dry weight, shoot dry and wet weight, crop growth rate (CGR), and net assimilation rate (NAR). NAR (in mg cm−2 day−1) was measured based on the method of Hasanah and Rahmawati (2014) as presented in formula 2.
Formula 2:
NAR = (W2-W1)/(T2-T1) × (Ln LA2-Ln LA1)/LA2-LA1
Where,
T = Time
W1 = Dry weight of the plant at T1
W2 = Dry weight of the plant at T2
LA1 = Total leaf area at T1
LA2 = Total leaf area at T2
Ln = Natural logarithm
Crop growth rate was measured based on formula 3 used by Tanveer et al. (2014).
Formula 3:
CGR = (W2-W1)/(T2-T1)
T2-T1
Where,
T = Time
W1 = Dry weight of the plant at T1
W2 = Dry weight of the plant at T2
Data Analysis
All the quantitative data were analyzed using ANOVA and continued by significance test using the Duncan's multiple range test at 5%. Statistical analysis was conducted with the help of SPSS software for Windows version 17.0 in 2009.
Results
Six isolates of rhizobacteria of Bali, namely, Sr3 (from rhizosphere of C. citratus), Tb9 (from rhizosphere of S. officinarum), Rg1 and Rg21 (from rhizosphere of P. purpureum), Al27 (from rhizosphere of I. cylindrica), and Jg8 (from rhizosphere of Z. mays), showed capability to produce IAA, fix nitrogen, and produce siderophores. All the other isolates showed positive reaction for Gram reaction test, except the isolate Sr3 that showed negative reaction. On NA, the color of colony of the isolate Sr3 was gray white with rod-shaped cells, isolate Tb9 was yellowish white with coccus-shaped cells, isolate Rg1 was yellowish white with rod-shaped cells, isolate Rg23 was gray white with coccus-shaped cells, Al27 was yellowish white with coccus-shaped cells, and isolate Jg8 was yellow with coccus-shaped cells. All the isolates were oxidase positive and catalase positive and negative for hydrogen sulfide (H2S) production.
Effect of Rhizobacteria on Germination Rate and Seedling Growth Promotion
Treatment with rhizobacteria significantly (p <0.05) increased the germination rate of corn, as given in Table 1. However, three isolates, namely, Tb9, Rg23, and Al2, did not significantly (p > 0.05) affect germination rate when compared with control. There were isolates that significantly (p <0.05) increased germination rate with the increment above 9.0% compared with controls, namely, Rg1 (9.65%), Sr3 (12.61%), and Jg8 (13.05%). Treatment with rhizobacteria significantly (p <0.05) increased the root length of corn plants at the age of 7 days, as given in Table 2.
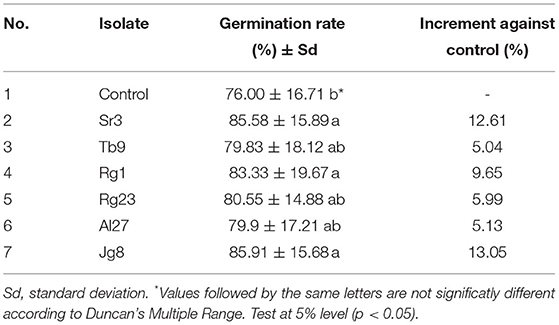
Table 1. Effect of rhizobacteria to the germination rate of corn cultvar Pertiwi at 3 days after treatment.
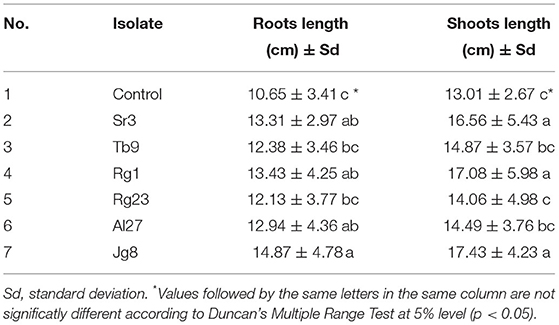
Table 2. Effect of rhizobacteria to the length of roots and shoots of corn cultivar Pertiwi at the age of 7 days.
Treatment with isolate Jg8 showed the highest root length among treatments. Treatment with rhizobacteria also had a significant (p <0.05) effect on corn shoot length at the age of 7 days. Treatment with isolate Jg8 resulted in the highest shoot length of 17.43 cm followed by isolate Rg1 with shoot length of 17.08 cm and Sr3 with shoot length of 16.56 cm. Data in Tables 1, 2 suggested that isolates Rg1, Sr3, and Jg8 are the potent plant growth promoter for corn.
Based on the data of germination rate, root length, and shoot length, the vigor index was determined. Vigor index indicates the ability of seeds to grow normally in field conditions and suboptimum environments (Copeland and McDonald, 2001). Results showed that rhizobacteria treatment significantly (p <0.05) affected the vigor index of corn at the age of 7 days, as shown in Table 3. The increase in vigor index compared with the control varied from 17.43 to 54.73%. The highest increase resulted from treatment with rhizobacteria isolate Jg8 and the lowest increase resulted from treatment with rhizobacteria isolate Rg23.
The dry weight of root and shoot was also affected by the treatment with rhizobacteria. Data in Table 4 showed that treatment with rhizobacteria significantly (p <0.05) increased the dry weight of roots and shoots of corn at the age of 7 days. Isolate Jg8 showed the highest dry weight both for the roots and shoots.
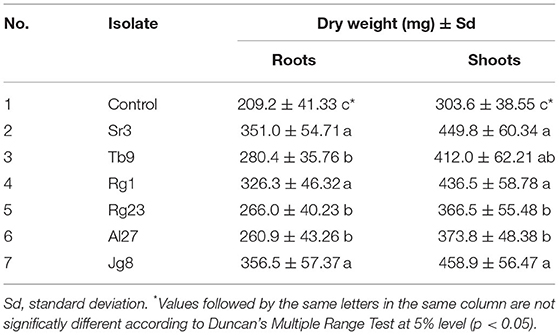
Table 4. Effect of rhizobacteria to the dry weight of roots and shoots of corn cultivar Pertiwi at the age of 7 days.
Colonization of Rhizobacteria on Corn Roots
Rhizobacteria colonization on plant roots is an ideal condition that is expected to provide maximum benefit to plants. The results of this study showed that the three rhizobacteria isolates, namely, Rg1, Sr3, and Jg8, could colonize the roots of corn plants, as shown in Figure 1, whereas no bacteria cell was observed on root of corn of control.
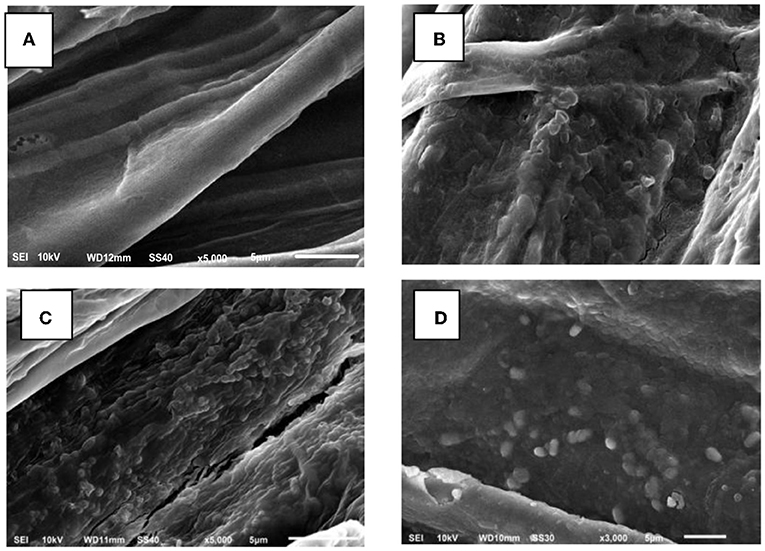
Figure 1. Scanning electrone microscope photographs of corn root cultivar Pertiwi. (A) Root of control. (B) Root colonized by isolate Sr3. (C) Root colonized by isolate Jg8, and (D) Root colonized by isolate Rg1. Bars represent 5μm.
Molecular Identities of Rhizobacteria
Amplification of PCR product of sequence 16S rRNA of isolates Rg1, Sr3, and Jg8 using two primers, viz., 16S (63F 5′-CAG GCC TAA CAC ATG CAA GTC-3′) and 1387R (5′-GGG CGG WGT GTA CAA GGC-3′) resulted in DNA fragments of ±1,500 bp (Figure 2). The DNA fragments were then purified and subjected to sequencing to identify these three isolates based on their similarity with other species of bacteria that previously have been identified and deposited in GenBank.
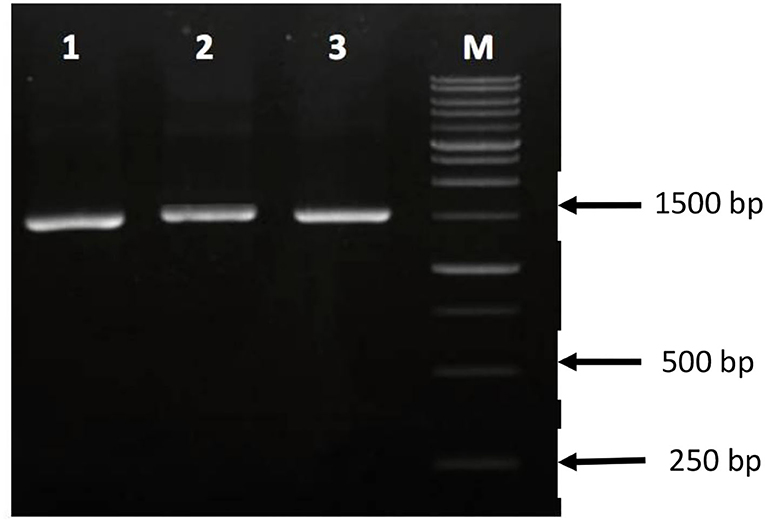
Figure 2. PCR amplification of 16S rRNA genes on agarose gel. 1. Isolate Sr3; 2. Isolate Jg8; 3. Isolate Rg1; and M. 1 kb DNA marker.
Based on sequence comparison with database of GenBank using the BLAST program, isolate Sr3 (accession number OM327515) showed a close relationship with Brevibacillus invocatus (B. invocatus) strain B32, B. invocatus strain B25, B. invocatus strain LMG 18962, B. invocatus strain LMG 18167, B. invocatus strain NCIMB 13772, B. invocatus strain SEPV 7, B. invocatus strain AB14, B. invocatus strain 361, B. invocatus strain C4, B. invocatus strain YNB25, and B. invocatus strain WA111 (Table 5). Based on phylogenetic tree analysis, isolate Sr3 is in the same clade with Brevibacillus invocatus (Figure 3).
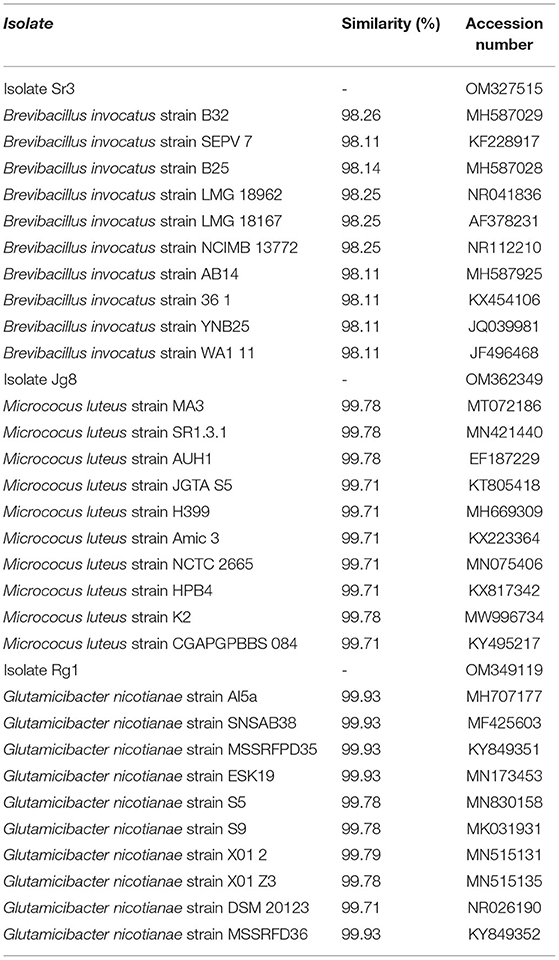
Table 5. Comparison of percentage of similarities of 16S rRNA gene of isolate Rg1, Sr3, and Jg8 with several DNA sequences of GenBank using BLAST program.
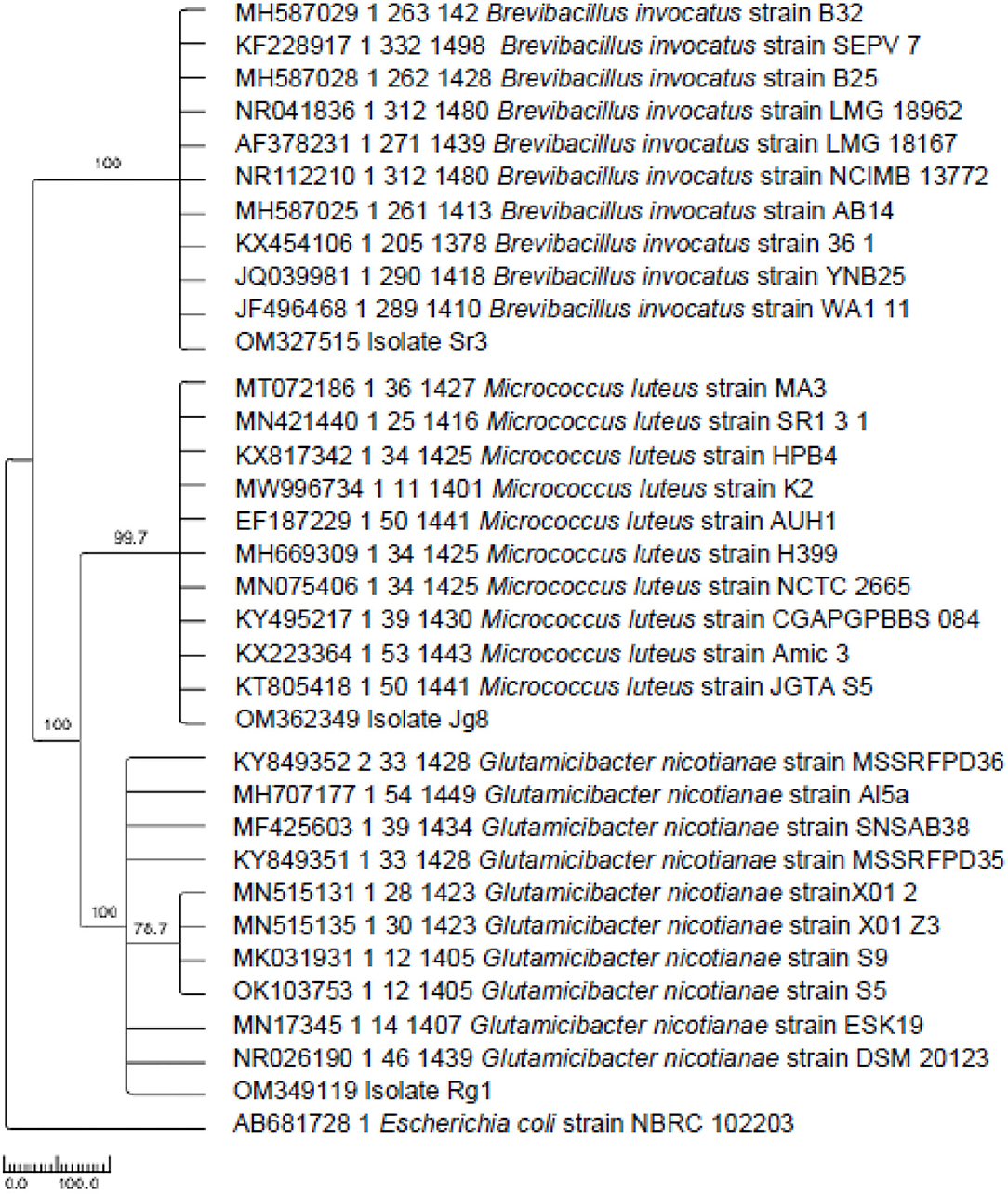
Figure 3. Phylogenetic relationship tree of isolate Rg1, Sr3, and Jg8 with several isolates of bacteria based on 16S rRNA gene using maximum parsimony method.
Sequence of 16S rRNA gene of isolate Jg8 (accession number) OM362349 showed close relationship with Micrococcus luteus (M. luteus) strain MA3, M. luteus strain SR1.3.1, M. luteus strain AUH1, M. luteus strain JGTA S5, M. luteus strain H399, M. luteus strain Amic 3, M. luteus strain NCTC 2665, M. luteus strain HPB4, M. luteus strain K2, and M. luteus strain CGAPGPBBS 084. Based on phylogenetic tree analysis, isolate Jg8 is in the same clade with Micrococcus luteus (Figure 3).
Isolate Rg1 (accession number OM349119) has a close relationship with Glutamicibacter nicotianae (G. nicotianae) strain MSSRFD36, G. nicotianae strain Al5a, G. nicotianae strain SNSAB38, G. nicotianae strain MSSRFPD35, G. nicotianae strain ESK19, G. nicotianae strain S5, G. nicotianae strain S9, G. nicotianae strain X012, G. nicotianae strain X01 Z3, and G. nicotianae strain DSM 20123, as given in Table 5. The result of phylogenetic tree analysis using maximum parsimony method with 1.000 × replicates of bootstrap showed that isolate Rg1 is Glutamicibacter nicotianae because it is in the same clade with the sequence of G. nicotianae (Figure 3).
Growth Promotion Capacity of Rhizobacteria
The content of macronutrients N, P, and K in the leaf of corn treated with three rhizobacteria species, namely, M. luteus Jg8, B. invocatus Sr3, and G. nicotianae, was significantly (p <0.05) higher than that of the control at 28 days after planting (DAP), as shown in Table 6. This study demonstrated that rhizobacteria treatment significantly increases nutrient uptake, particularly N, P, and K.
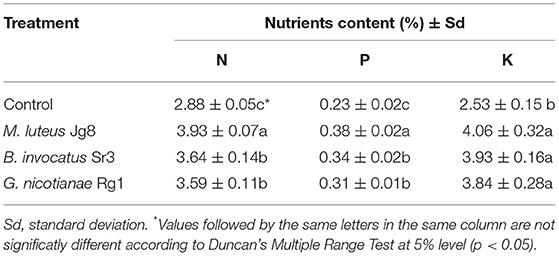
Table 6. Effect of rhizobacteria to the content of N, P, and K in the leaf of corn cultivar Pertiwi at 28 days after planting (DAP).
At 28, 42, and 56 DAP, leaf chlorophyll content was significantly (p <0.05) higher in corn treated with rhizobacteria than in control. The leaf chlorophyll contents of corn plants treated with rhizobacteria did not differ significantly (p > 0.05) between the three species, except at 42 DAP, when leaf chlorophyll contents were significantly higher with M. luteus Jg8 treatment than with B. invocatus Sr3 treatment (Table 7).
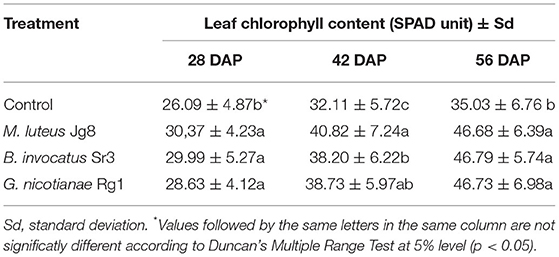
Table 7. Effect of rhizobacteria to the leaf chlorophyll content of corn cultivar Pertiwi at 28, 42, and 56 days after planting (DAP).
In line with the increase in leaf chlorophyll contents, the NAR in corn treated with rhizobacteria was significantly (p <0.05) higher than the control in both the growth period 14–28 and 28–42 DAP, as given in Table 8. The NAR value in the control in the growth period 14–28 DAP was 1.9415 and 2.3829 mg cm−2 day−1 in the growth period 28–42 DAP, whereas the NAR of corn treated with rhizobacteria ranged from 2.4610 to 2.6975 mg cm−2 day−1 and from 3.0563 to 3.1880 mg cm−2 day−1 at growth periods of 14–28 and 28–42 DAP, respectively. The rhizobacteria treatment increased the NAR value by 26.80–38.66% compared with the control in the 14–28 DAP growth period and 29.83–38.15% in the 28–42 DAP growth period.
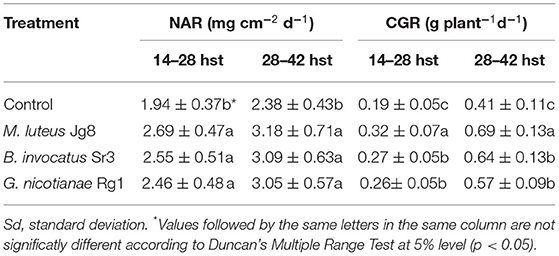
Table 8. Effect of rhizobacteria to the net assimilation rate (NAR) and crop growth rate (CGR) at 14–28 and 28–42 DAP of corn cultivar Pertiwi.
The CGR data showed a similar trend to the NAR data. The growth rate of corn in the treatment with rhizobacteria was significantly (p <0.05) higher than the control, both in the growth period of 14–28 and 28–42 DAP. The plant growth rate (CGR) in control in the growth period 14–28 and 28–42 DAP was 0.1968 and 0.4164 g plant−1 d−1, respectively, whereas the CGR value in plants treated with rhizobacteria in the growth period 14–28 and 28–42 DAP ranged from 0.2591 to 0.3184 g plant−1 d−1 and from 0.5702 to 0.6926 g plant−1 d−1, respectively. Based on this data, it is known that there was an increase in the CGR value compared with the control by 31.66–61.79% in the 14–28 DAP growth period and 39.93–66.33% in the 28–42 DAP growth period.
Discussion
Three species of rhizobacteria were identified in this study: M. luteus Jg8, B. invocatus Sr3, and G. nicotianae Rg1. These three rhizobacteria species exhibit a variety of characteristics, including the ability to produce IAA, fix nitrogen from the atmosphere, and produce siderophores. These three rhizobacteria species can improve corn seed germination, shoot and root length, and colonize corn roots. These three indigenous Balinese rhizobacteria with diverse characteristics are the first to be described. Prior study has concentrated on one of the features, specifically the ability to synthesize IAA (Khalimi et al., 2017) and acetoin (Khalimi et al., 2017; Adrianus et al., 2018). Although prior researchers have reported on rhizobacteria with growth promotion properties, there are no comprehensive data on their diverse characteristics and growth-promoting capabilities on corn.
Micrococcus luteus has been shown to produce antimicrobial compounds against food-borne pathogens such as Salmonella typhimurium, Listeria monocytogenes, Escherichia coli, Klebsiella pneumoniae, and Staphylococcus aureus (Akbar et al., 2014). Dar et al. (2018) reported that the bacteria Micrococcus luteus strains such as WI12, WI41, and WI80 isolated from the rhizosphere of the walnut plant (Juglans regia) can dissolve phosphate and produce IAA, siderophores, and gibberellic acid, so that it has the potential as a plant growth promoter.
Bacteria G. nicotianae strain ZM05 and Acinetobacter tandoii ZM06 in the form of a consortium can decompose dipropyl phthalate (DPrP) and cadmium (Cd), which are often found as environmental contaminants (Wang et al., 2019). The bacteria G. nicotianae strain MSSRFPD35 isolated from the rhizosphere of the Canna indica plant growing in locations contaminated with distillation waste showed the ability to degrade phenol with high efficiency (Purushothaman et al., 2020). These bacteria have potential as bioremediation agents, especially for phenol and its derivatives and heavy metal polluted environments.
Brevibacillus is a genus of Gram-positive bacteria that can live in a variety of habitats and its growth is fast. This genus has the ability to produce various types of enzymes to decompose low-density polyethylene and has potential as a biocontrol agent against plant pathogens (Panda et al., 2014; Ahmed et al., 2018). Treatment with B. invocatus and Ralstonia pickettii significantly increases the plant growth, sugar, flavonoids and phenolics, chlorophyll b, total chlorophyll, carotenoids content, and activities of superoxide dismutase, catalase, and peroxidase in sunflower hybrid Parsun 3 irrigated with water containing high total dissolved solid (Urooj et al., 2021).
Auxins, cytokinins, and gibberellins have been shown to promote plant growth and can alter the root system architecture (Yaxley et al., 2001; Moubayidin et al., 2009; Overvoorde et al., 2010). Additionally, these hormones can increase the surface area of root branches, allowing the plant to access more nutrients from the soil. Indoleacetic acid (IAA) is a well-known compound produced by several rhizobacteria, including Alcaligenes, Azospirillum, Pseudomonas, Pantoea, Rhizobium, and Enterobacter (Spaepen et al., 2007).
Other researchers established that Azospirillum, Agrobacterium, Pseudomonas, and Erwinia produced IAA, which increased the root length, hairs on the root, root branch length, and root surface area of seedlings (Bashan et al., 2004). The three rhizobacteria species identified in this study are free-living bacteria found in the rhizospheres of Gramineae (Poaceae) plants that grow in Bali and are capable of nitrogen fixation from the surrounding environment. This property benefits surrounding plants by increasing the amount of nitrogen available in the soil, which plants can use to grow. These three species have the ability to colonize the roots of corn plants, allowing them to interact very closely with them. As Tabassum et al. (2017) demonstrated, free-living bacteria surrounding plant roots can interact with plants by providing nitrogen to the plants in exchange for root exudates containing amino acids, proteins, enzymes, vitamins, and growth hormones.
Nitrogen is a macroelement that is critical for the growth and development of plants (Gopalakrishnan et al., 2017). Besides being able to produce IAA and fix nitrogen, these three rhizobacteria species can also produce siderophores. Siderophores are compounds with low-molecular weights that can chelate iron (Shaikh and Sayyed, 2015; Mhlongo et al., 2018). On one hand, siderophore-producing rhizobacteria can stimulate plant growth and on the other hand, siderophore-producing rhizobacteria inhibit the growth of plant pathogens by reducing the availability of iron for plant pathogens (Ma et al., 2011). Several bacterial genera have been reported to produce siderophores such as Azospirillum, Dickeya, Klebsiella, Nocardia, Pantoea, Pseudomonas, Azotobacter, Paenibacillus, Bacillus, Serratia, and Streptomyces (dos Santos et al., 2020). There have been no reports regarding the ability of M. luteus, B. invocatus, and G. nicotianae to produce siderophores.
Numerous researchers have documented the role of multitrait rhizobacteria in promoting plant growth and suppressing important plant pathogens (Dinesh et al., 2015; Sharma et al., 2015; Batistaa et al., 2018; Haque et al., 2020; Venieraki et al., 2021). However, the rhizobacteria species with multiple characteristics previously described were not the same as the species described in this study. Venieraki et al. (2021) isolated four rhizobacteria strains that produce the enzyme arylsulfatase [an enzyme that converts organic sulfur to inorganic sulfur, so that it is available to plants, i.e., isolates from the genera Bacillus spp. (strains 1.SG.7 and 5.SG.3) and Pseudomonas spp. (strains 2.SG.20 and 1.C.19)] and all of the rhizobacteria isolates used were multitrait rhizobacteria, which means that they can form biofilms, are salinity tolerant, and are compatible with plants. These four rhizobacteria isolates increased the abundance of lateral roots and shoot biomass, while decreasing salinity stress. Additionally, the study team demonstrated that in-vitro testing of the performance of this mixture of four microbial isolates demonstrated that it could significantly increase the growth of wheat plants compared to single isolates.
Other studies have demonstrated that rhizobacteria treatment can increase the vigor index of seeds, such as Sutariati et al. (2006), who demonstrated that plant growth-promoting rhizobacteria treatment can increase the vigor index of chili seeds. Haque et al. (2020) reported the ability of biofilm-producing bacteria to stimulate the growth of tomato plants. In an experiment in pots under water stress, tomato plant growth was better in the treatment with the rhizobacteria Pseudomonas azotoformans ESR4, P. poae ESR6, P. gessardii ESR9, P. cedrina ESR12, P. chlororaphis ESR15, Stenotrophomonas maltophilia ESR21, and Pseudomonas ESR20 with control (Haque et al., 2020). Two isolates of rhizobacteria, namely, Bacillus spp. RZ2MS9 and Burkholderia ambifaria RZ2MS16, were shown to stimulate the growth of corn and soybean in a greenhouse study (Batistaa et al., 2018) with an increase in dry weight of corn roots at the age of 60 days by 247.8 and 136.9% compared with controls, respectively. Bacillus subtilis strain S25 has muliple traits, i.e., it can dissolve P to produce IAA and produce siderophores to increase germination, root length, and root dry weight of tomato plants grown in greenhouses (Sharma et al., 2015). Treatment with rhizobacteria was able to increase germination and vigor index, as was the case with studies conducted on wheat, where bacteria with multiple traits (producing ammonia, producing siderophores, dissolving phosphate, and as antifungal) were able to increase germination at 4 days of age and vigor index compared with control (Rana et al., 2011). The mode of action by which rhizobacteria in this study increased germination and promoted the growth of corn was not studied; however, IAA production may be related to the increase of seed germination and seedling growth promotion. The germination process starts from the inhibition process, where water enters the seeds with a moisture content of approximately 12%, so that an isotonic balance occurs. The entry of water into the seeds causes the activation of enzymes in the seeds such as amylase, protease, lipase, and nuclease. These enzymes will catalyze the degradation of macromolecules into simple molecules and are ready to be used for germination process. Activation of these catalytic enzymes is carried out by growth hormones such as gibberellins, IAA, kinetin, and cytokinins (Sunarpi et al., 2019). Rhizobacteria M. luteus Jg8, B. invocatus Sr3, and G. nicotianae Rg1 can produce IAA, so that it can stimulate the germination of corn seeds.
It was discovered in this study that three indigenous Balinese rhizobacteria, namely, M. lutesus Jg8, B. invocatus Sr3, and G. nicotianae Rg1, increased nutrient uptake, leaf chlorophyll content, as well as the NAR and CGR of corn plants. This ability is believed to be associated with the isolates' ability to synthesize IAA and fix nitrogen from the environment. Three Bali rhizobacteria species with growth-promoting properties are described for the first time. As a result, these three isolates demonstrate significant potential for use as biostimulants to promote maize growth and production, particularly in Bali. These studies were conducted on a small scale and in a controlled environment, which is one of this study's limitations. To ensure that the identified rhizobacteria perform consistently, field testing in a variety of locations and growth seasons is required. The optimal formulation for rhizobacteria growth and performance in the field is unknown and additional study will be conducted to ensure that farmers, particularly in Bali, can widely use this biological agent.
Conclusion
Three species of rhizobacteria native to Bali were isolated from the rhizospheres of Gramineae plants: M. luteus Jg8, B. invocatus Sr3, and G. nicotianae Rg1. These three rhizobacteria have been shown to increase the germination rate of corn seeds, the length and dry weight of roots and shoots, and the vigor index of the Pertiwi corn cultivar. These three species are capable of producing IAA, fixing nitrogen from the air, producing siderophores, and colonizing corn roots. Under greenhouse conditions, treatment with these rhizobacteria significantly increased nutrient uptake, leaf chlorophyll content, NAR, and CGR, indicating that they have considerable potential for development as biostimulants to promote growth and yield increase in corn, particularly in Bali.
Data Availability Statement
The datasets presented in this study can be found in online repositories. The names of the repository/repositories and accession number(s) can be found below: https://www.ncbi.nlm.nih.gov/, OM349119, https://www.ncbi.nlm.nih.gov/, OM327515, https://www.ncbi.nlm.nih.gov/, OM362349.
Author Contributions
DS contributed mainly in laboratory experiment, data analysis, and manuscript preparation. All authors contributed to the article and approved the submitted version.
Funding
The research grant for this study was provided by Universitas Udayana, Institute of Research and Community Services under scheme Penelitian Invensi Udayana in fiscal year 2021. Contract number: B/96-63/UN14.4.A/PT.01.05/2021.
Conflict of Interest
The authors declare that the research was conducted in the absence of any commercial or financial relationships that could be construed as a potential conflict of interest.
Publisher's Note
All claims expressed in this article are solely those of the authors and do not necessarily represent those of their affiliated organizations, or those of the publisher, the editors and the reviewers. Any product that may be evaluated in this article, or claim that may be made by its manufacturer, is not guaranteed or endorsed by the publisher.
Acknowledgments
We wish to express a high appreciation to the Institute of Research and Community Services, Udayana University, for providing the study grant in the fiscal year 2021 under scheme of Penelitian Invensi Udayana.
References
Abdul-Baki, A. A., and Anderson, J. D. (1973). Vigor determination in soybean seed by multiple criteria. Crop Sci. 13,630–633. doi: 10.2135/cropsci1973.0011183X001300060013x
Adrianus, S. D. N., Adnyana, M., Sudana, M., and Khalimi, K. (2018). Potential of acetoin-producing rhizobacteria from rhizospheres of plants of Graminae grown in Merauke, Indonesia to promote the growth of rice. J. Biol. Agric. Healthcare. 8, 43–49.
Agustiyani, D. (2016). Penapisan dan Karakterisasi Rizobakteria serta Uji Aktivitasnya dalam Mendukung Perkecambahan dan Pertumbuhan Benih Jagung (Zea mays L.). J. Biol. Indonesia. 12, 241–248.
Ahmed, A. I. S., Omer, A. M., Ibrahim, A. I. M., and Agha, M. K. (2018). Brevibacillus spp. in agroecology: the beneficial impacts in biocontrol of plant pathogens and soil bioremediation. Fungal Genom. Biol. 8, 2. doi: 10.4172/2165-8056.1000157
Akbar, A., Sitara, U., Ali, I., Muhammad, N., and Shabir Ahmad Khan, S. A. (2014). Isolation and characterization of biotechnologically potent Micrococcus luteus strain from environment. Pakistan J. Zool. 46, 967–973
Akinrinlola, R. J. (2018). Evaluation of bacillus strains for plant growth promotion potentials on corn (Zea mays), wheat (Triticum aestivum), and soybean (Glycine max). (Thesis). Lincoln: The Faculty of The Graduate College at The University of Nebraskain.
Bantacut, T., Yasser, R. F, and Muammar, T.A. (2015). Pengembangan jagung untuk ketahanan pangan, industri dan ekonomi. J. Pangan. 24, 135–148. Available online at: https://doi.org/10.33964/jp.v24i2.29
Bashan, Y., Holguin, G., and De-Bashan, L. E. (2004) Azospirillum-plant relationships: physiological, molecular, agricultural, and environmental advances (1997–2003). Can. J. Microbiol. 50, 521–577. doi: 10.1139/w04-035
Batistaa, B. D., Lacavab, P. T., Ferraria, A., Teixeira-Silvaa, N. S., Bonatellia, M. L., Tsuia, S., et al. (2018). Screening of tropically derived, multi-trait plant growth- promoting rhizobacteria and evaluation of corn and soybean colonization ability. Microbiol. Res. 206, 33–42. doi: 10.1016/j.micres.2017.09.007
Breedt, G., Labuschagne, N., and Coutinho, T. A. (2017). Seed treatment with selected plant growth-promoting rhizobacteria increases maize yield in the field. Ann. Appl. Biol. 171, 229–236. doi: 10.1111/aab.12366
Calvo, L., Cortey, M., Marin, J. L. G., and Gil, L. J. G. (2005). Polygenic analysis of ammonia-oxidizing bacteria using 16S rDNA, amoA, and amoB genes. Int. Microbiol. 8, 103–110.
Chung, J., Geun, C. S., and Choong, M. R. (2016). Sweet Scents From Good Bacteria: Case Studies on Bacterial Volatile Compounds for Plant Growth and Immunity. Plant Mol. Biol. 90, 677–687. doi: 10.1007/s11103-015-0344-8
Copeland, L. O., and McDonald, M. B. (2001). Principles of Seed Science and Technology, 4th Edn. Boston, MA: Kluwer Academic Publisher. p. 467.
Dar, G. H. S., Sofi, S., Padder, S. A., and Kabli, A. (2018). Molecular characterization of rhizobacteria isolated from walnut (Juglans regia) rhizosphere in Western Himalayas and assessment of their plant growth promoting activities. Biodiversitas. 19, 712–719. doi: 10.13057/biodiv/d190245
Dinesh, R., Anandaraj, M., Kumar, A., Bini, Y. K., Subila, K. P., and Aravind, R. (2015). Isolation, characterization, and evaluation of multi-trait plant growth promoting rhizobacteria for their growth promoting and disease suppressing effects on ginger. Microbiol. Res. 173, 34–43. doi: 10.1016/j.micres.2015.01.014
dos Santos, R. M., Diaz, P. A. E., Lobo, L. L. B., and Rigobelo, E. C. (2020). Use of plant growth-promoting rhizobacteria in maize and sugarcane: characteristics and applications. Front. Sustain. Food Syst. 4, 136. doi: 10.3389/fsufs.2020.00136
Figueiredo, M.D.V.B., Bonifacio, A., Rodrigues, A.C., and Araujo, F.F.D. (2016). “Plant growth promoting rhizobacteria: key mechanisms of action”, in Microbial-Mediated Induced Systemic Resistance in Plants. p. 23–37. doi: 10.1007/978-981-10-0388-2_3
Fincheira, P., Maribel, P., and Andrés, Q. (2017). Volatile organic compounds stimulate plant growing and seed germination of Lactuca sativa. J. Soil Sci. Plant Nutr. 17, 853–867. doi: 10.4067/S0718-95162017000400002
Food Agriculture Organization of the United Nations (2018). Crops. Accessed online at: http://Www.Fao.Org/Faostat/En/?#Data/Qc (accessed July 4, 2020).
Gholami, A., Shahsavani, S., and Nezarat, S. (2009). The effect of plant growth promoting rhizobacteria (PGPR) on germination, seedling growth and yield of maize. Word Acad. Sci. Engi. Tech. 49, 19–24.
Gholami, A., Atena, B., and Somayeh, N. (2018). Effect of seed priming with growth promoting rhizobacteria at different rhizosphere condition on growth parameter of maize. International Meeting on Soil Fertility Land Management and Agroclimatology. Turkey. p. 851–856.
Gopalakrishnan, S., Srinivas, V., and Samineni, S. (2017). Nitrogen fixation, plant growth and yield enhancements by diazotrophic growth-promoting bacteria in two cultivars of chickpea (Cicer arietinum L.). Biocatal. Agric. Biotechnol. 11, 116–123. doi: 10.1016/j.bcab.2017.06.012
Goswami, D., Janki, N. T., and Pinakin, C. D. (2016). Portraying mechanics of plant growth promoting rhizobacteria (PGPR): a review. Cogent Food Agric. 2, 1–19. doi: 10.1080/23311932.2015.1127500
Haque, M. M., Mosharaf, M. K., Khatun, M., Haque, M. A., Biswas, M. S., Islam, M. S., et al. (2020). Biofilm producing rhizobacteria with multiple plant growth-promoting traits promote growth of tomato under water-deficit stress. Front. Microbiol. 11, 542053. doi: 10.3389/fmicb.2020.542053
Hasanah, Y., and Rahmawati, N. (2014). Yield and physiology of soybean under water stress condition with application Bradyrhizobium japonicum induced by Genistein. J. Agon. Indonesia. 42, 110–117.
Imamuddin, H., Dewi, T. K., and Antonius, S. (2015). Isolation and screening of rhizobacteria from soil in Ngawi East Java, as candidates of agent for liquid organic fertilizer production. Nusantara Biosci. 7, 107–111. doi: 10.13057/nusbiosci/n070208
Kanchiswamy, C. N., Mickael, M., and Massimoe, E. M. (2015). Chemical diversity of microbial volatiles and their potential for plant growth and productivity. Front. Plant Sci. 6, 1–23. doi: 10.3389/fpls.2015.00151
Khalimi, K., Suprapta, D. N., Sudana, I. M., and Wirya, I. G. N. A. (2017). Potential of indole acetic acid producing rhizobacteria to promote the growth ang increase the yield of Edamame, a vegetable soybean (Glicine max). Int. J. Pharma. Bio. Sci. 8, 601–608. doi: 10.22376/ijpbs.2017.8.3.b601-608
Khambani, L. S., Ahmed, I. A., and Thierry, R. (2019). Rhizopheric bacteria from pristine grassland have beneficial traits for plant growth promotion in maize (Zea mays L.). Cogent Biol. 5, 1–14. doi: 10.1080/23312025.2019.1630972
Kundan, R., Garima, P., Nitesh, J., and Pavan, K.A. (2015). Plant growth promoting rhizobacteria: mechanism and current prospective. J. Fertil. Pestic. 6, 1–9. doi: 10.4172/2471-2728.1000155
Ma, Y., Prasad, M. N. V., Rajkumar, M., and Freitas, H. (2011). Plant growth promoting rhizobacteria and endophytes accelerate phytoremediation of metalliferous soils. Biotechnol. Adv. 29, 248–258. doi: 10.1016/j.biotechadv.2010.12.001
Mendes, R., Paolina, G., and Jos, M. R. (2013). The rhizosphere microbiome: significance of plant beneficial, plant pathogenic, and human pathogenic microorganisms. FEMS Microbiol. Rev. 37, 634–663. doi: 10.1111/1574-6976.12028
Mhlongo, M. I., Piater, L. A., Madala, N. E., Labuschagne, N., and Dubery, I. A. (2018). The chemistry of plant-microbe interactions in the rhizosphere and the potential for metabolomics to reveal signaling related to defense priming and induced systemic resistance. Front. Plant Sci. 9, 17. doi: 10.3389/fpls.2018.00112
Ministry of Agriculture of the Republic of Indonesia (2019). Total Volume Impor dan Produksi Jagung Nasional. Available online at: https://databoks.katadata.co.id/ datapublish/ 019/08/22/berapa-produksi-dan-volume-impor-jagung-nasional. (accessed Jully 4, 2020).
Moubayidin, L., Di Mambro, R., and Sabatini, S. (2009). Cytokinin-auxin crosstalk. Trends Plant Sci. 14, 557–562. doi: 10.1016/j.tplants.2009.06.010
Mus, F., Matthew, B., Crook, Kevin, G., Amaya, G. C., Barney, A. G., Evangelia, D. K., et al. (2016). Symbiotic nitrogen fixation and the challenges to its extension to nonlegumes. Appl. Environ. Microbiol. 82, 3698–3710. doi: 10.1128/AEM.01055-16
Overvoorde, P., Fukaki, H., and Beeckman, T. (2010). Auxin control of root development. Cold Spring. Harb. Perspect. Biol. 2, a001537. doi: 10.1101/cshperspect.a001537
Panda, A. K., Bisht, S. S., DeMondal, S., Kumar, N. S., Gurusubramanian, G., and Ashok Kumar Panigrahi, A. K. (2014). Brevibacillus as a biological tool: a short review. Antonie Leeuwenhoek. 105, 623–639. doi: 10.1007/s10482-013-0099-7
Purushothaman, D., Jegan, S., Anu, D. A., and Prabavathy, V. R. (2020). Kinetics of phenol biodegradation by heavy metal tolerant rhizobacteria Glutamicibacter nicotianae MSSRFPD35 from distillery effluent contaminated soils. Front. Microbiol. 11, 1573. doi: 10.3389/fmicb.2020.01573
Rana, A., Saharam, B., and Joshi, M. (2011). Identification of multi-trait PGPR isolates and evaluating their potential as inoculant for wheat. Ann. Microbiol. 61, 893–900. doi: 10.1007/s13213-011-0211-z
Sezgin, M., and Kahya, M. (2018). Phytohormones. J. Sci. Technol. 8, 35–39. doi: 10.17678/beuscitech.386726
Shaikh, S. S., and Sayyed, R. Z. (2015). “Role of plant growth promoting rhizobacteria and their formulation in biocontrol of plant diseases”, in N.K. Arora (Ed.). Plant Microbes Symbiosis: Applied Facets. Springer India. doi: 10.1007/978-81-322-2068-8_18
Sharma, R., Walia, A., Chauhan, A., and Shirkot, C. K. (2015). Multi-trait plant growth promoting bactetria from tomato rhizosphere and evaluation of their potential as bioinoculants. Appl. Biol. Res. 17. doi: 10.5958/0974-4517.2015.00019.1
Souissi, T., Kremer, R. J., and White, J. A. (1997). Scanning and transmission electron microscopy of root colonization of leafy spurge (Euphorbia esula L.) seedlings by rhizobacteria. Phytomorphology. 47, 177–193. doi: 10.1007/BF02318983
Spaepen, S., Vanderleyden, J., and Remans, R. (2007). Indole-3-acetic acid in microbial and microorganism-plant signaling. FEMS Microbiol. Rev. 31, 425–448. doi: 10.1111/j.1574-6976.2007.00072.x
Stover, B. C., and Muller, K. F. (2010). TreeGraph 2: combining and visualizing evidence from different phylogenetic analyses. BMC Bioinform. 11, 1–9. doi: 10.1186/1471-2105-11-7
Sunarpi, H., Prasedya, E. S., and Nikmatullah, A. (2019). Makroalga: Sumber biostimulan dan pupuk organik perangsang pertumbuhan tanaman. TrustMedia Publishing, Yogyakarta.
Sutariati, G. A. K., Widodo, S., and Ilyas, S. (2006). Effect of plant growth promoting rhizobacteria on seed germination and seedling growth of hot pepper. Bul. Agron. 34, 46–54. Available online at: https://doi.org/10.24831/jai.v34i1.1275
Tabassum, B., Khan, A., Tariq, M., Ramzan, M., Khan, M. S. I., Shahid, N., et al. (2017). Bottlenecks in commercialisation and future prospects of PGPR. Appl. Soil Ecol. 121, 102–117. doi: 10.1016/j.apsoil.2017.09.030
Tanveer, M., Anjum, S. A., Zahid, H., Rehman, A., and Sajjad, A. (2014). Growth and development of maize (Zea mays L.) in response to different planting methods. J. Agric. Res. 52, 511–522. Available online at: https://apply.jar.punjab.gov.pk/upload/1424962684_115_P5._4270.pdf
Timmusk, S. (2003). “Mechanism of action of the plant growth promoting bacterium Paenibacillus Polymyxa. comprehensive”, in Summaries of Uppsala Dissertations from The Faculty of Science and Technology. p. 908.
Urooj, N., Bano, A., and Asif Riaz, A. (2021). Role of PGPR on the physiology of sunflower irrigated with produced water containing high total dissolved solids (TDS) and its residual effects on soil fertility. Int. J. Phytoremed. 24, 567–579. doi: 10.1080/15226514.2021.1957771
Venieraki, A., Chorianopoulou, S. N., Katinakis, P., and Bouranis, D. L. (2021). Multi-trait wheat rhizobacteria from calcareous soil with biocontrol activity promote plant growth and mitigate salinity stress. Microorganisms. 9, 1588. doi: 10.3390/microorganisms9081588
Wahyudi, A. T., Jepri, A. P., Hanifa, N. F., Hima, D. M., and Abdjad, A. N. (2019). Streptomyces spp. from rhizosphere soil of maize with potential as plant growth promoter. Biodiversitas. 20, 2547–2553. doi: 10.13057/biodiv/d200916
Wang, X., Shen, S., Wu, H., Wang, H., Wang, L., and Lu, Z. (2019). Acinetobacter tandoii ZM06 assists Glutamicibacter nicotianae ZM05 in resisting cadmium pressure to preserve dipropyl phthalate biodegradation. Microorganisms. 9, 1417. doi: 10.3390/microorganisms9071417
Widawati, S., and Suliasih. (2019). Role fo indigenous nitrogen-fixing bacteria in promoting plant growth on post tin mining soil. Makara J. Sci. 23, 28–38. doi: 10.7454/mss.v23i1.10801
Yaxley, J. R., Ross, J. J., Sherriff, L. J., and Reid, J. B. (2001). Gibberellin biosynthesis mutations and root development in pea. Plant Physiol. 125, 627–633. doi: 10.1104/pp.125.2.627
Keywords: rhizobacteria, Gramineae, multiple traits, growth promoter, corn
Citation: Maulina NMI, Suprapta DN, Temaja IGRM, Adnyana IM and Suriani NL (2022) Rhizobacteria of Bali With Obvious Growth-Promoting Properties on Corn (Zea mays L.). Front. Sustain. Food Syst. 6:899736. doi: 10.3389/fsufs.2022.899736
Received: 19 March 2022; Accepted: 07 April 2022;
Published: 23 May 2022.
Edited by:
Everlon Cid Rigobelo, São Paulo State University, BrazilReviewed by:
Becky Nancy Aloo, University of Eldoret, KenyaMd. Motaher Hossain, Bangabandhu Sheikh Mujibur Rahman Agricultural University, Bangladesh
Copyright © 2022 Maulina, Suprapta, Temaja, Adnyana and Suriani. This is an open-access article distributed under the terms of the Creative Commons Attribution License (CC BY). The use, distribution or reproduction in other forums is permitted, provided the original author(s) and the copyright owner(s) are credited and that the original publication in this journal is cited, in accordance with accepted academic practice. No use, distribution or reproduction is permitted which does not comply with these terms.
*Correspondence: Dewa Ngurah Suprapta, bmd1cmFoc3VwcmFwdGEmI3gwMDA0MDt1bnVkLmFjLmlk
†Present address: Ni Made Intan Maulina, Faculty of Agriculture, Dwijendra University, Denpasar, Indonesia