- 1Tropical Forages Program, International Center for Tropical Agriculture (CIAT), Palmira, Colombia
- 2Departamento de Ciencias Agrícolas, Universidad Nacional de Colombia Sede Palmira, Palmira, Colombia
Spittlebug (Hemiptera: Cercopidae) species cause large economic losses on gramineous crops (Poaceae) in tropical and subtropical America. These insects are key pests of sugarcane and forages, crops that experienced a quick expansion in extensive monocultures in Brazil, Colombia, and Mexico. Mobilization toward sustainable crop and livestock systems to supply the growing demand of meat, milk, and sugar in Latin America and the Caribbean region implies developing sustainable and feasible strategies of integrated pest management to control spittlebugs. This review combines information on Cercopidae taxonomy, geographical distribution, insect biology, and control strategies to contribute to the development of integrated pest management in grasses and sugarcane in the Neotropics.
Introduction
Crop and livestock production are expected to grow in Latin America and the Caribbean at least 15% by 2028 (OECD and FAO, 2021). For sugar and meat, projections show an increase particularly in developing countries, being Brazil one of the largest producer and exporter in the world for these two commodities (OECD and FAO, 2021). In this scenario, it is necessary to develop and adopt strategies to move toward sugarcane and livestock sustainable intensive systems, avoiding the expansion of the agricultural frontier in the region (Jusys, 2017). This includes the implementation of efficient integrated pest management (IPM) programs in these traditionally monocultural extensive systems.
Spittlebugs (Hemiptera: Cercopidae) are the main limitation of economically important cash crops in the Neotropical ecozone. Different species are key pests of Brachiaria grasses (Urochloa spp., syn.: Brachiaria spp.; Holmann and Peck, 2002) and sugarcane (Sacharum officinarum L.; Rossato et al., 2019). Also are occasional pests of maize (Zea mays L.) and rice (Oryza sativa L.) (Thompson, 2004; Carvalho and Webb, 2005; Cruz et al., 2009; Heinrichs and Muniappan, 2017). These xylem-feeders have several common names in different countries as follow: spittlebugs (United States of America), froghoppers (Australia and United Kingdom), salivazo or mion (Colombia), salivita (Cuba and Nicaragua), baba de culebra (Central America), candelilla (Venezuela), mosca pinta (Mexico) and cigarrinhas (Brasil). The damage caused by this group in tropical and subtropical America has a large impact on livestock and sugar value chains for the negative effect on production, productivity and the industrial processing of these commodities. Studies to understand the taxonomy, biology, behavior, damage, and control methods of these insects are available. However, this information is scattered. This review summarizes the current studies about Cercopidae taxonomy, geographical distribution and biology, and links it with the IPM strategies available in grasses and sugarcane to provide clear information for spittlebug's control.
Taxonomy
Hemiptera comprises many bugs grouped in three suborders: xylem feeders Auchenorrhyncha (cicadas, spittlebugs, leafhoppers, treehoppers, and planthoppers), phloem feeders Sternorrhyncha (jumping plant lice, whiteflies, aphids, and scale), and true bugs Prosorrhyncha (Heteroptera and Coleorrhyncha) (Dietrich, 2009). The suborder Auchenorrhyncha contains the superfamilies, Cicadoidea (cicadas), Membracoidea (leafhoppers and treehoppers), Fulgoroidea (planthoppers), and the monophyletic superfamily Cercopoidea (spittlebugs), with the families Aphrophoridae, Clastopteridae, Machaerotidae, Epipygidae, and Cercopidae (Paladini et al., 2018). Evidence to support the monophyly of this suborder was provided in molecular analyses (Cryan and Urban, 2012; Misof et al., 2014; Johnson et al., 2018; Skinner et al., 2020) and the presence of a bacterial endosymbiont (Koga and Moran, 2014).
Cercopidae includes the paraphyletic Cercopinae (old world), and the monophyletic Ischnorhininae (new world) subfamilies (Cryan and Svenson, 2010). Fennah (1968) divided the new world species into four tribes: Tomaspidini, Ischnorhinini, Hyboscartini, and Neaenini, and proposed a classification scheme based on morphological characters. From this classification and subsequent updates, the morphological characters describing Cercopoidea superfamily includes head with frontoclypeus inflated; median ocellus absent; ocelli on crown distant from margin; pronotum extended to scutellar suture; body clothed with fine setae; hind coxae conical, tibia without rows of setae but often with one or more conspicuous spines; male subgenital plate present (Carvalho and Webb, 2005; Dietrich, 2005, 2009). For Cercopidae, the descriptive characters are colorful patterns in tegmina, convex postclypeus anterior or laterally compressed, hexagonal pronotum with posterior margin reticulated (Paladini and Cryan, 2012). The main difference with Aphrophoridae family is having the eyes slightly longer than wide and the posterior margin of the pronotum straight (instead of emarginate) (Dietrich, 2009). Machaerotidae and Clastopteridae differ from the other families in having a developed appendix on the forewing, in which Machaerotidae has two or more r-m crossveins in the forewing and lacks an outer fork on the radial vein of the hind wing (Dietrich, 2009).
An illustrated key of new world spittlebugs based on characters as color patterns, styles, plates, and aedeagus of male genitalia was reviewed by Carvalho and Webb (2005). However, the classification of some species is complex for their body similarity, color patterns, and male-genitalia intra-specific variation, the absence of specific accurate descriptors and taxonomic keys, and the lack of diagnostic keys for identification in immature individuals (Cryan and Svenson, 2010; Paladini and Cryan, 2012).
Some inconsistencies in diagnostic characters indicate the lack of reliable evidence for defining major lineages, leading to the rejection of the monophyly of Ischnorhinini and Tomaspidini, and taxonomic instability (Paladini et al., 2015, 2018). For this reason, some authors opted to exclude this tribal-level classification including all the Neotropical cercopids in the Ischnorhininae subfamily, with 62 genera and 438 species (Carvalho and Webb, 2005; Paladini and Cryan, 2012; Paladini et al., 2015; Paladini and Cavichioli, 2017; Castro et al., 2018; Castro et al., 2020).
The main genuses reported attacking gramineous crops in tropical and subtropical America are Aeneolamia Fennah, Deois Fennah, Isozulia Fennah, Kanaima Distant, Mahanarva Distant, Maxantonia Schmidt, Notozulia Fennah, Prosapia Fennah, Sphenorhina Amyot and Serville, Tunaima Fennah, and Zulia Fennah (Table 1).
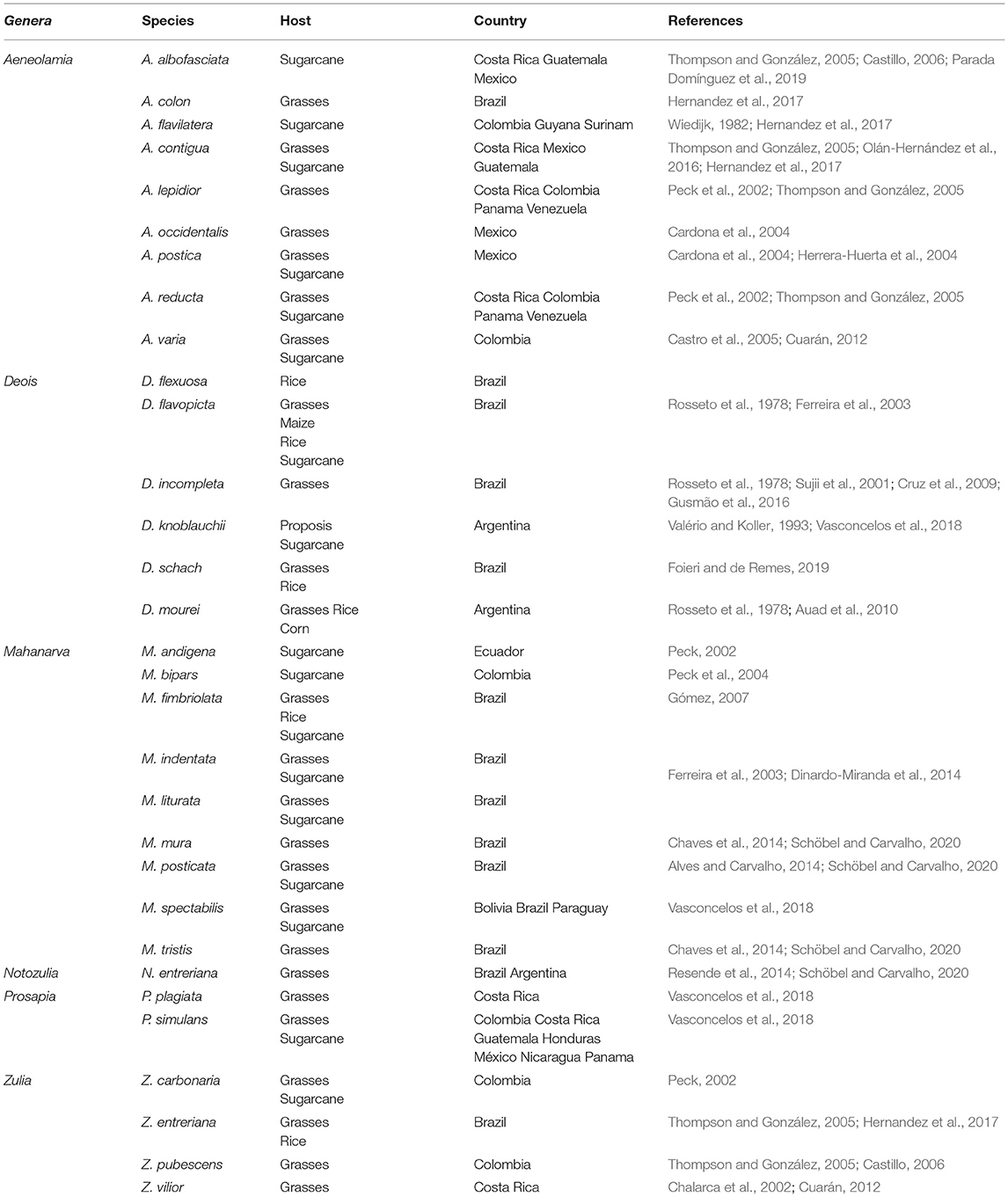
Table 1. Reported economically important cercopid species in gramineous crops in the Neotropical ecozone.
Geographic Distribution
Cercopidae (Hemiptera: Auchenorrhyncha: Cicadomorpha: Cercopoidea) is the most speciose spittlebug family distributed worldwide, being found in most terrestrial ecosystems (Thompson, 2004). Cercopids of gramineous crops occur in the neotropics from the southern United States to northern Argentina (Paladini et al., 2018). The species may vary or coincide in each country (Table 1). For example, the predominant species in Brazil are of the genera Mahanarva, Deois, and Notozulia, while in Colombia the most representative species are from Aeneolamia, Prosapia, and Zulia (Hernandez et al., 2021).
Ecological niche studies modeling the potential distribution under different climate change scenarios, show various current suitable habitats for spittlebugs across Central and South America. Besides, depending on the species, a long-term change in the distribution is estimated particularly due to changes in abiotic factors like precipitations (Schöbel and Carvalho, 2020; Hernandez et al., 2021). These results suggest that they have little ability to quickly adapt to changing environments with a high dependence on climate factors. This tendency was reported in Aphrophoridae, where climate change led to warmer and dryer environments in California, decreasing Philaenus spumarius populations on Erigeron glaucus Ker. (Karban and Huntzinger, 2018).
In the global network database, Global Biodiversity Information Facility (GBIF), 58570 occurrences for Cercopidae are registered. Most of them reported in Costa Rica, the United States, Germany, France, and The Netherlands (GBIF.org., 2022). However, the tropics are the most diverse zones with 70% of the 1,360 described species of this family, considering that approximately 475 are mostly distributed in the American tropics and subtropics (Peck and Thompson, 2008; Dietrich, 2009; Cryan and Svenson, 2010; Hamilton, 2013; Paladini et al., 2018). Thus, more efforts should be made to report cercopid occurrences in biodiversity global networks, prompting research in other fields like phylogenetics, taxonomy, and ecology.
Biology and Behavior
Spittlebugs have hemimetabolous metamorphosis (Figures 1, 2). The duration of each stage varies among species, sites, and climate conditions (Table 2).
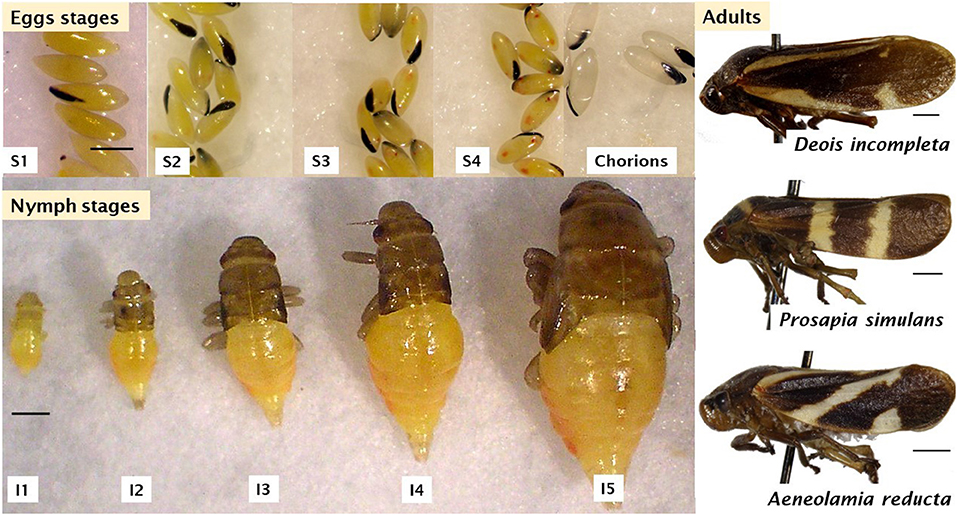
Figure 2. Different stages of spittlebug: Eggs (Aeneolamia varia), nymph (Prosapia simulans), and adults. Scale bar represents a 1 mm. Pictures taken from the archive of the forage entomology laboratory – CIAT.
Eggs
Eggs are laid on soil (1–2 cm of the top of the soil), near to stalks, on litter, and on plant tissues (de la Cruz-Zapata et al., 2016). Northern hemisphere species lay ~35 eggs (Hamilton and Morales, 1992) and tropical species lay between 40 and 100 eggs in 3–10 days (Sotelo and Cardona, 2001). The four egg stages are completed in 2 to 3 weeks (S1–S4; Figure 2) (Peck, 2002; Peck and Thompson, 2008; Dietrich, 2009). Eggs are elongate (1 mm of length and 0.3 mm of width), light-yellow in the first stages, changing to dark-yellow or orange with two reddish spots in the anterior part corresponding to eyes, and other two lighter marks in the posterior part corresponding to the abdomen in the final stage (Figure 2; Valério et al., 2001; Parsa et al., 2011; Peixoto, 2016). In some species, egg diapause is expressed to avoid adverse climate conditions, i.e., dry seasons, taking up to 530 days to hatch (Peck et al., 2002; Peck and Thompson, 2008; Auad et al., 2011). The mechanism that activates diapause is not completely elucidated.
Nymphs
Nymphs have five instars (Figure 2) that may last from 4 to 9 weeks (Table 2) (Hamilton and Morales, 1992; Peck and Thompson, 2008). Nymphs usually feed gregariously on the xylem sap of plant roots, leaves, branches, and crowns (Pires et al., 2000; Sujii et al., 2002; Cid-Muñoz et al., 2020). Young nymphs have weak motility; however, they can move short distances to choose feeding sites (Pires et al., 2000). Hagley and Blackman (1966) and Garcia et al. (2007) found that on roots, nymphs ingest parenchyma cells in the cortex during their first instars, while third, fourth, and fifth instar nymphs reach the xylem vessels and, occasionally, sieve-tube elements of the primary phloem (Figure 3). In this stage, nymphs cover themselves with a foam composed of excreted semi-digested plant fluid, fatty acids (palmitic and stearic acid), carbohydrates, along with mucopolysacccharides and proteins produced by Malpighian tubules (Rakitov, 2002; Tonelli et al., 2018). Each of these components plays a vital role in the stability, viscosity, and elasticity of the foam, functioning as a microhabitat protecting the nymph from desiccation, predation, parasitism, and solar radiation (Martin et al., 2002; Carvalho and Webb, 2005; Chen et al., 2018).
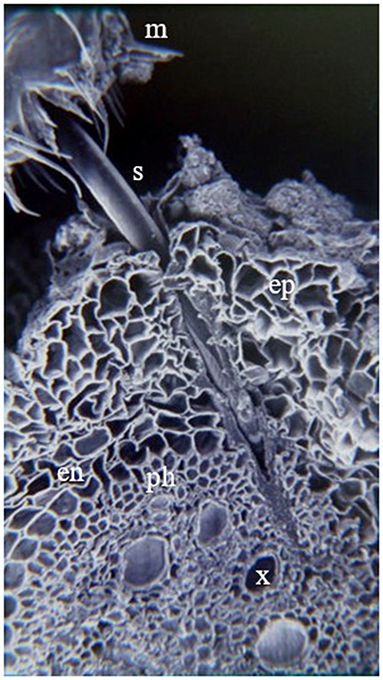
Figure 3. Feeding site of A. varia nymph over U. ruziziensis root. Isect mouth (m), Stylet (s), epidermis (ep), endodermis (en), phloem (ph) and xylem (x). Picture taken from the archive of the forage entomology laboratory – CIAT.
Adults
Longevity ranges between 1 and 3 weeks, exhibiting a plethora of color patterns presenting intraspecific polymorphism (Peck and Thompson, 2008, Figure 2) and have two stout, thorn-like spurs in the hind tibiae, which end in a broadly flared double row of black-tipped spines (Hamilton and Morales, 1992). Males are distinguished from females by genitalia and size, females are larger. Adults have two strategies to avoid possible predators: long jump (115 times their body length; Burrows, 2006; Burrows et al., 2007) and reflex bleeding (Peck, 2000a). The latter consists in emitting odoriferous orange hemolymph from the pretarsal pads as a startle stimulus linked to the jumping ability (Peck, 2000b). As nymphs, adults feed by inserting the stylets through the stomata, passing through the chlorophyll-bearing and parenchyma to reach the metaxylem or vascular bundles (Hagley and Blackman, 1966; Garcia et al., 2007) on the shoots injecting saliva to aid digestion and prevent clogging of the stylet (Crews et al., 1998; Dietrich, 2009).
Mating starts when males emit a call based on vibration through the stems and leaves of the host (Lopez et al., 2001). This call is emitted by tymbals located on the first abdominal segment and is transmitted through the host plant, and it is usually inaudible (Peck and Thompson, 2008; Dietrich, 2009). Copulation occurs during the photophase and, depending on the species, may last from a second to several hours. Females usually mate only once, and males mate several times (Dietrich, 2009).
Feeding Habit
The xylem feeding habit is thought to be a constraint for managing the strong negative pressure of the xylem sap generated by transpiration (Novotny and Wilson, 1997). High rates of this unbalanced food is needed to meet the nutritional requirements since it is composed of diluted amino acids, simple organic acids, and various sugars (Redak et al., 2004). Other diet supplements are provided by intracellular symbiotic microorganisms to synthesize other nutrients (Douglas, 1989). An obligate dependent symbiosis with multiple organisms living in specialized cells, bacteriocytes, or tissues, bacteriomes, vertically transmitted through host generations via ovarial passage was identified in Auchenorrhyncha (Moran et al., 2005; Koga et al., 2012). Depending on the species, spittlebugs contain at least two symbionts that provides essential amino acids: the Candidatus Sulcia muelleri A. (Bacteroidetes) as a common organism in various species usually complemented with the Betaproteobacteria Zinderia insecticola or the Enterobacteriaceae Sodalis glossinidius Dale and Maudlin (1999) (Koga et al., 2013; Koga and Moran, 2014). These paired symbionts show a complementary set of genes related to the 10 essential amino acids required for animals. In Clastoptera arizonana Doering (1929), Sulcia presented the genes involved in the production of leucine, isoleucine, valine, threonine, lysine, arginine, and phenyalanine, Zinderia presented those for tryptophan, methionine, and histidine (McCutcheon and Moran, 2010). Foieri et al. (2022) reported Candidatus sulcia muelleri in the new world Notozulia entreriana (Berg, 1879), Deois mourei (Cavichioli and Sakakibara, 1993), and Deois knoblauchii (Berg, 1879).
Population Dynamics
Abiotic factors play a vital role in population dynamics, characterized by high fluctuations and synchrony (Peck and Thompson, 2008). A peak favoring egg hatching and reducing nymph mortality was observed during the rainy season (Sujii et al., 2002). Thus, in humid zones, the insects achieve up to 6 generations per year (Sotelo and Cardona, 2001). This seasonal dynamic was reported for Zulia carbonaria (Lallemand, 1924) and Aeneolamia reducta (Lallemand, 1924) in colombian pasture systems for bimodal and monomodal rainfall patterns respectively (Peck et al., 2002; Castro et al., 2005), and Aeneolamia contigua (Walker, 1851) in sugarcane crops in Mexico, having a higher abundance of nymphs and adults after the rainy season started (Olán-Hernández et al., 2016). Additionally, Herrera-Huerta et al. (2004) found that high humidity rates increase the egg hatching and survival rate of Aeneolamia postica (Walker, 1858) collected from sugarcane in Mexico in laboratory conditions.
Damage Symptoms and Economic Loss
Plant damage depends on the insect stage and host. For grasses, the first four nymphal stages, the visual damage is usually imperceptible. When nymphs reach the fifth stage, ascendant acropetal chlorosis is observed (Figure 4), and, under a severe attack, the entire aboveground portion of the plant appears dry and dead (Valério et al., 2001). Specifically, for adults the damage is first observed in young leaves, where whitish-chlorotic spots appear around suction points due to parenchyma tissue dilution for the caustic substances present in saliva (Valério et al., 2001, Figure 4). Next, the spots tend to coalesce in chlorotic lesions from the tip to the base of the leaf, and, with high populations, the leaves appear entirely yellow or necrotic (Sotelo and Cardona, 2001; Thompson and González, 2005). Reddish streaks can follow the chlorotic lesions in some genotypes (Lopez et al., 2009). In pasture systems, the quality of the forage is reduced affecting its growth, dry matter production, in-vitro digestibility, protein, phosphorus, magnesium, calcium, and potassium content; palatability, establishment, and persistence (Valério and Nakano, 1988; Peck and Thompson, 2008). Holmann and Peck (2002) discovered that small populations of 10 adults/m2 can cause stunted growth and a decrease in production, as well as 2–8 percent reduction in forage carrying capacity. With larger populations, 25 to 50 adults/m2, the stocking rate may decrease 26–33 percent. Hence, milk and meat production are affected even in low infestations of spittlebugs in forages cultivation. Congio et al. (2020) showed that Mahanarva sp. can decrease beef productivity up to 74% and herbage yield varying from 31 to 43% (depending on level of fertilization and grazing severity of Marandú).
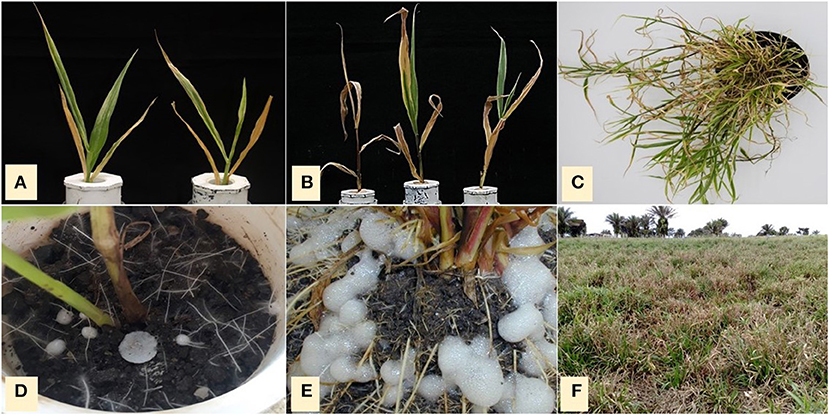
Figure 4. Recognition of spittlebugs. (A–C): damage characteristic caused by adult and nymph respectively; (D,E): nymphs coated by their spittle and; (F): damage caused in fields on grasses. Pictures taken from the archive of the forage entomology laboratory – CIAT.
In sugarcane, Dinardo-Miranda et al. (2008) and Rossato et al. (2019) report that spittlebug attack decreases productivity by reducing the number of healthy stalks, yield, and sugar accumulation resulting in yield losses of 9 ton ha-1 or 8 to 50% for every 1% of spittlebug infestation (de la Cruz-Llanas et al., 2005; Dinardo-Miranda, 2008). The negative impact in industrial processing is evident for the high fiber content and dead or deteriorated stalks that act as contaminants in the milling process making sugar recovery difficult and inhibiting fermentation. High infestation levels increase sugar color, total phenolic compounds, total and volatile juice acidity, and acetaldehyde concentration in distillate. Also reduces apparent sucrose content, Brix, pH, purity, and ethanol content in wine, indicating a lower juice quality for sugar and ethanol production (Madaleno et al., 2008; Mutton et al., 2010; Ravaneli et al., 2011).
In terms of economic losses for sugarcane and introduced pastures in the Neotropics, Holmann and Peck (2002), Thompson (2004), and Auad et al. (2010) reported estimated losses from US$ 840 to 2100 million per year.
Integrated Pest Management (IPM)
Monitoring and Sampling
Spittlebug monitoring and sampling in sugarcane and grasses is based on visualization adults and nymph masses, and detecting chlorotic areas. Techniques for monitoring eggs and nymphs (see: Nilakhe et al., 1984b; Martín-Rivera, 1994) are considered impractical in sugarcane plantations due to high time consumption and difficulty in execution. The most reliable and efficient sampling method for monitoring grasses is to collect adults using a sweep net (Nilakhe et al., 1984a; Morente et al., 2018). The most common method for nymph sampling is to walk through a pre-determined area checking for spittle masses, which are usually on the soil or near host roots (Martín-Rivera, 1994; Pires et al., 2000). The foam can be removed with a soft brush to observe the nymphs feeding. The gregarious generalized behavior of nymphs and their low motility make it easier to locate infestation hot spots in the field for the appearance of wilted or chlorotic areas (Gutiérrez and Gómez, 2009). The most common method to monitor adults in sugarcane is sampling with yellow sticky traps in pre-established plots. Several authors proposed placing yellow traps in crops at various densities and locations (Table 3).
As a result, the majority of economic thresholds are based on the number of adults in a given area. Dinardo-Miranda and Gil (2007) and Dinardo-Miranda et al. (2008) set an economic injury level of 4 spittlebugs per m2 for Mahanarva fimbriolata (Stal, 1854) in sugarcane in Brazil. According to Gómez (2007), the economic threshold of Aeneolamia varia (Fabricius, 1787) for sugarcane in Valle del Cauca, Colombia is 0.2 spittle masses or adults per stalk, or 50 adults per week per trap if yellow sticky traps are used. Resende et al. (2013) found that 8 adults of Mahanarva spectabilis (Distant, 1909) feeding for 6 days influences the physiological function of Urochloa ruziziensis Germ and Evrard in plants with an average of 80 tillers, implying this reference value for spittlebug control in these grasses. Holmann and Peck (2002) defined a low infestation as 10 adults per m2, an intermediate infestation as 25 adults per m2, and a high infestation as 50 adults per m2. A host may exhibit variable levels of resistance or tolerance to different spittlebugs species (Cardona et al., 2004), making establishing an economic threshold difficult because it needs a thorough understanding of the host and its interaction with a specific species.
Cultural Control
Cultural strategies aim to make the ecosystem less suitable for the establishment and proliferation of insect populations (Zaefarian and Rezvani, 2016). In the past, a common practice was control by burning (Beck, 1963; Koller, 1987). However, new strategies aim to implement more sustainable actions based on the knowledge of insect biology. As spittlebugs are highly susceptible to humidity variations, most of the cultural practices are related to avoiding humid and hot microhabitats. These include litter removal from the field and soil mechanical preparation by tillering or discing, to expose the immature stages to desiccation and remove potential secondary hosts (Sáenz et al., 1999; Nachappa, 2004; Dietrich, 2009; Busoli et al., 2014). Lilliston equipment for tillage, identification of focal distribution patterns and removing the straw from the field after harvesting in sugarcane are feasible strategies to reduce nymph infestation for next seasons (Gómez, 2007; de Castro et al., 2019).
In grasses is recommended the diversification of crops, e.g., grasses and leguminous systems or pasture blends mixing tolerant and resistant materials to have a wider diversity in the ecosystem that promotes niches for natural enemies and reduces the feeding sources for spittlebugs (Sotelo and Cardona, 2001). Also, pasture renewal with resistant varieties adapted to local conditions to decrease spittlebug populations, avoiding extensive monocultural meadows and keeping a focal distribution of the insect (Valério and Koller, 1993). These strategies are also important to prevent resistance-breaking biotypes by offering alternative hosts to the insects (Cardona et al., 2004). In highly infested pastures, intensive grazing is also recommended to reduce the biomass through livestock feeding and nymph population for the trampling effect (Valério and Koller, 1993; Sotelo and Cardona, 2001; Thorne et al., 2017).
Other cultural strategies involve the drain management in the field reducing the soil top layer moisture, particularlly in clayey areaswith a higher water retention capacity that favors the nymph and egg survival (Figueredo et al., 2012; de Castro et al., 2019) and fertilization management. The susceptible genotype U. ruziziensis showed a reduced damage and high quality when a recommended dose of fertilizer was applied under Mahanarva spectabilis infestation (45 mg/dm3 of urea, 255 mg/dm3 of superphosphate, and 28 mg/dm3 of potassium chlorate on the planting and 140 mg/dm3 of NPK 20-5-20 on the 30th and the 60th days) (Aguiar et al., 2014). Besides, macronutrient fertilization enhances tolerance traits of grasses by improving the nutritional quality and physiological status of the plants, even if the nymphal survivorship is high (Pires et al., 2000; Valério, 2009; Alvarenga et al., 2019b).
Mechanical Control
The use of traps as a spittlebug control method has received limited attention. Yellow sticky traps, according to Sáenz et al. (1999), may reduce the first generation of adults in sugarcane spittlebugs. In that case, they recommend placing plastic yellow bags covered with a sticky layer on the stalks, depending on the threshold: 25 traps per hectare are required for a population of 0.2 adults per stalk or 0.4 nymphs per stalk; 75 traps are required for a population of 0.3 adults or 0.5 nymphs per stalk; and 100 traps are required for a population of more than 0.8 adults. This tactic should be used only for monitoring because natural enemies may also be trapped (Fernández, 2013).
Microbial Control
Metarhizium Sorokin is the most used entomopathogenic agent to control cercopids in sugar cane and pastures of Brazil, Mexico, Guatemala, Costa Rica, Panama, Venezuela, Ecuador and Colombia (Sotelo and Cardona, 2001; Badilla, 2002; Bustillo et al., 2011; García et al., 2012; Hernández-Domínguez et al., 2016). In Brazil, 1.8 million sugarcane ha are treated to control spittlebugs, being one of the most successful biological control programs in the world (Parra, 2014; Mascarin et al., 2019).
Reasons for the widespread adoption of Metarhizium for pest control is attributed to its worldwide distribution, its broad host range, its ability to inhabit soil or act as an entomopathogen or endophyte, the standardized production and application protocol, and the number of product registrations (Brunner-Mendoza et al., 2019; Sant et al., 2019). Persistence, virulence, and viability depend on the strain and abiotic factors, e.g., temperature, solar radiation, and humidity (Zimmermann, 2007; Ortiz-Urquiza and Keyhani, 2013). These factors influence the quality and efficiency of this agent, so it is needed to increase survivability of the conidia over the time by using registered products and applying under optimal conditions (high relative humidity, avoiding direct solar radiation and extreme temperatures, etc.) (Bustillo et al., 2011).
Despite recommended doses may vary depending on the authors, spittlebug mortality rates above 60% have been found when treated with Metarhizium (Table 4). Within the Metarhizium genus some species can infect many insect species, e.g., Metarhizium robertsii J.F.Bisch., S.A.Rehner & Humber, or can be restricted to certain hosts, e.g., Metarhizium album Petch to Hemiptera (Brunner-Mendoza et al., 2019). The richness and predominance of Metarhizium species vary among ecosystems, and identifying this ecological status allows to understand the interactions with the hosts and crops lead to better use in IPM strategies (Obando et al., 2013; Rezende et al., 2015; Brunner-Mendoza et al., 2017).
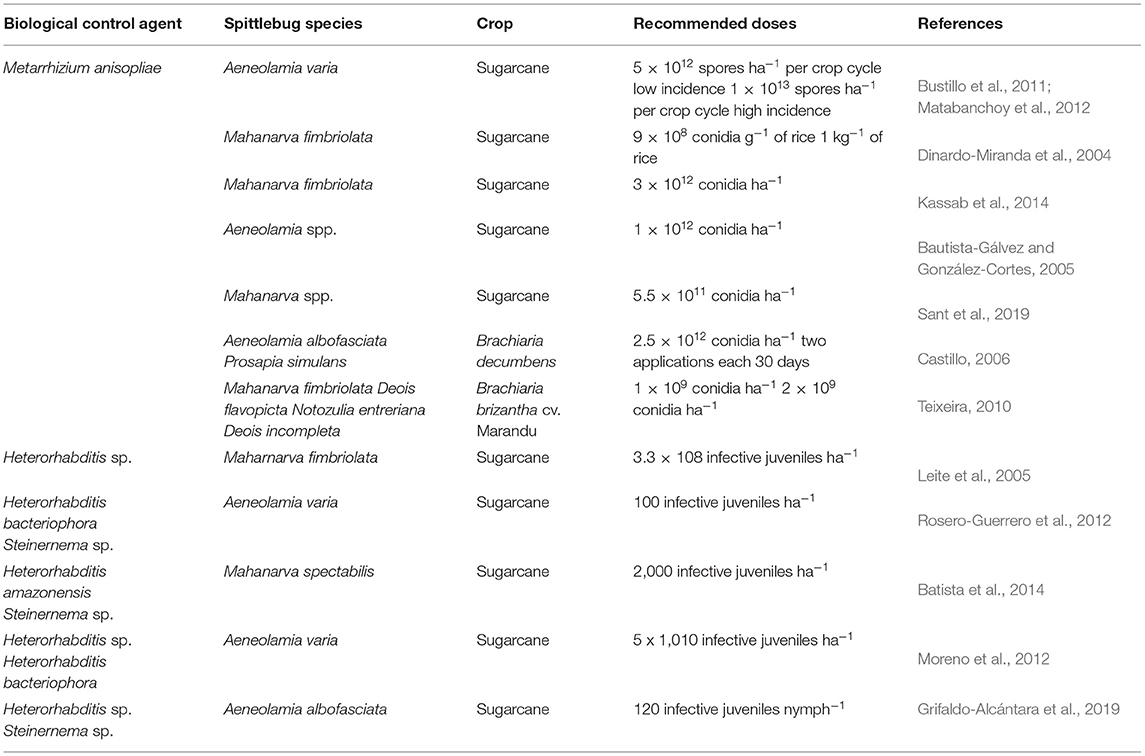
Table 4. Different recommended doses of entomopathogenic fungi Metarrhizium anisopliae and nematodes in sugarcane and grasses to control spittlebugs.
Research on the use of nematodes of the genera Steinernema Travassos and Heterorhabditis Poinar to control spittlebug nymphs increased in the last several years. The interest in these obligate parasites lies in their easy multiplication and application, broad host range, and compatibility with chemical pesticides (Bhat et al., 2020). Previous laboratory and greenhouse studies indicate that native strains of Steinernema and Heterorhabditis cause mortality rates of more than 80% in Mahanarva fimbriolata (Stal, 1854), Mahanarva spectabilis (Distanr, 1909), and Aeneolamia varia (Fabricius, 1787) nymphs in the fifth day after inoculation (Leite et al., 2005; Rosero-Guerrero et al., 2012; Batista et al., 2014). One of the main advantages of these agents is their capacity to move in different environments, e.g., soil or aqueous matter, and locate their hosts by chemotaxis, ambushing or an intermediate strategy between those (Lewis et al., 2006; Lortkipanidze et al., 2016). Consequently, nematodes are effective in controlling spittlebugs as they can penetrate the nymph's foam and can move through the soil to infect other individuals (de Paula Batista and Auad, 2010; Parada Domínguez et al., 2019).
The virulence of entomopathogenic nematodes varies among genera and strains. Batista et al. (2014) found that Steinernema riobrave (Cabanillas et al., 1994) caused greater mortality rates in Mahanarva spectabilis nymphs than other species for its ability to move faster on the soil. While Moreno et al. (2012) and Parada Domínguez et al. (2019) reported that nematodes of the genus Heterorhabditis were more virulent in Aeneolamia varia and Aeneolamia albofasciata (Lallemand, 1939) nymphs, due to the presence of a tooth that makes it easier to penetrate the host through the cuticle.
Biological Control
Predators
The nymphal predator Salpingogaster nigra Schiner, 1868 (Diptera: Syrphidae) is one of the most efficient agents for its high reproduction rate, high fecundity, and short life cycle that allows two or three generations per spittlebug cycle (Sotelo and Cardona, 2001). Despite this potential, this species demonstrated low larval viability in laboratory studies, implying that a mass rearing colony would be impractical (Veríssimo et al., 2018). To maintain and promote S. nigra populations in crops, it is recommended to use conservation biological control techniques, such as providing plants with extrafloral nectaries and available pollen (Bustillo and Castro, 2011; Pérez-Bañón et al., 2013).
Promising Natural Enemies
There are information of the occurrence of other natural enemies such as the egg parasitoids Anagrus urichi Pickles, 1932 and Acmopolynema hervali Gomes, 1948 (Hymenoptera: Mymaridae; Marques and Vilas Boas, 1985; Valério and Oliveira, 2005); the nymph predator Pachycondyla obscuricornis Emery, 1890 (Hymenoptera: Formicidae; Sujii et al., 2004); the adult predators Leptrotrachelus sp. (Coleoptera: Carabidae) and Porasilus barbiellini Curran, 1934 (Diptera: Asilidae; Bueno, 1987). Along with ants and Reduviidae predating immature stages, parasitic mites of adults (Acari: Erythraeidae) and spiders of Salticidae family predating nymphs and adults (Medina, 1995; Sotelo and Cardona, 2001; Castro et al., 2005). However, the use of these insects as biological control agents has not been reported.
Chemical Control
Chemical control is limited only for few situations in which the economic threshold is reached, and the population is increasing. In Colombia, Brazil, and Mexico, several authors recommend using products containing the active ingredient Thiamethoxam to control spittlebug attack (Dinardo-Miranda et al., 2004; Gómez, 2007; Madaleno et al., 2008; Pereira et al., 2010; García-González et al., 2017). Thiamethoxam is a systemic neonicotinoid from the thianicotinyl subclass that works by binding to nicotic acetylcholine receptors, which are involved in signal transmission in the insect central nervous system, causing mobility reduction and inhibiting the feeding reflex (Maienfisch et al., 2001; Thany et al., 2010). Although the doses reported may vary, some authors reported that 250 g of active ingredient per hectare for Aeneolamia varia (Gómez, 2007) and Mahanarva fimbriolata is a viable option because it has a longer residual effect, reducing spittlebug populations faster with higher productivity (Dinardo-Miranda and Gil, 2007; Pereira et al., 2010).
Other active ingredients have been tested, such as fipronil, aldicarb, carbofuran, imidacloprid (Dinardo-Miranda et al., 2004; Nakano et al., 2020). However, the use of chemical control is still a debatable because it has a low efficiency in controlling nymphs and may have an impact on the natural enemies' population and biology, measured survivorship, emergency and parasitism of Trichogramma galloi Zucchi, 1988 in sugarcane (e.g., triflumuron, fipronil, thiamethoxam, lambda-cyhalothrin + thiametoxam and etiprole; de Oliveira et al., 2013). As a result, integration with other IPM common strategies in sugarcane such parasitoid release, is hampered.
For grasses, chemical control is not usually feasible strategy as most of these systems are extensive and pastures are considered of low economic value. Thus, in a large area application would be costly and may lead to intoxication of the livestock, with residuality on meat and milk. Besides, the ecological disequilibrium that would lead to a future rise of spittlebug populations and, to long term development of resistance (Valério and Koller, 1993; Sotelo and Cardona, 2001; Castillo, 2006).
Nakano et al. (2020) reported the use of boric acid in 0.4% (0.4 gr of boric acid/100 ml of water) concentration to control Deois (Acanthodeois) flavopicta (Stal, 1854) and Notozulia entreriana (Berg, 1879) as an alternative to other active ingredients. Results showed the same survival rate as imidacloprid 700WG for both species. The advantages associated to the use of boric acid are economic and environmental as it is low-toxic and relatively harmless to natural enemies, along with the potential uses for mineral nutrition.
Host Plant Resistance
Breeding for resistance to spittlebug attack is the main approach to generate a sustainable and long-term control strategy being easily adopted by producers at a low cost (Valério et al., 2001; Valério, 2013; Grisoto et al., 2018). Antibiosis, tolerance, and antixenosis have been reported for grasses and sugarcane (Miles et al., 1995; Cardona et al., 2004; Dinardo-Miranda et al., 2016). Here we focused on the main advances on Urochloa grasses host-plant resistance to spittlebug and the next challenges in this field.
Urochloa interspecific breeding program at the International Center for Tropical Agriculture (CIAT) with a recurrent selection for specific combining ability (RS-SCA) scheme is a successful case of implementation of host-plant resistance in commercial cultivars, e.g., Mulato II, Cayman, and Cobra (Worthington and Miles, 2015). In America, the founders of these programs came from genetic banks which materials were collected in successive missions to Africa from 1950's to 1980's (Keller-Grein et al., 1996). Most of the economically important cultivars are polyploid apomicts genotypes, being that the case of the first identified unimproved source of resistance to spittlebug attack, U. brizantha cv. Marandu. Introduced from a volcanic region in Africa to Braziland released by EMBRAPA in 1984, it exhibited antibiosis to different American spittlebug species (Nunes et al., 1984). However, recently in central-western Brazil, Almeida et al. (2005) and Valério (2009) reported severe damage caused by nymphs and adults of Mahanarva spp. on cultivar Marandu. This indicates lower levels of antibiosis to this genus compared to Deois spp. or Notozulia spp., causing an increase of Mahanarva spp. populations in the region. Hence, it is needed to identify accessions with antibiosis to immature stages coupled with some tolerance to adult feeding damage, permitting the accumulation of genes for resistance to multiple spittlebug species of economic importance in other Urochloa producing localities like Brazil and Mexico (Cardona et al., 2004; Miles et al., 2006; Pabón et al., 2007).
Reliable standardized methodologies based on no-choice tests were developed to assess nymph and adult damage for obtaining resistant or tolerant materials (Cardona et al., 1999; Lopez et al., 2009; Dinardo-Miranda et al., 2014). Greenhouse screening tests are performed to classify resistant, intermediate, and susceptible genotypes based on visual scoring to measure damage severity and nymph survivorship (Cardona et al., 1999). Some authors also use other measurements such as chlorophyll content, dry mass, weight loss, and biomass weight to compare the response of each genotype (Dinardo-Miranda et al., 2014, 2016, 2018; Peixoto, 2016). More recently, high-throughput phenotyping techniques are being implemented for damage assessing in the CIAT Urochloa breeding program in response to the increase of resistance levels in the recurrent selection cycles to this trait. This methodology is based on the digital images analysis, in which a yellowness index is calculated from the measurement of the chlorotic shoot area in each genotype. Higher accuracy and precision were obtained through this method compared to visual scoring or chlorophyll content (SPAD units) (Hernandez et al., 2020), demonstrating the feasibility of this technique to be incorporated in the program.
In traditional pasture systems, reported antixenosis levels in Urochloa are not sufficiently high to be considered a true resistance mechanism. The polyphagous feeding habit of spittlebugs and the extensive monoculture systems represent lesser chances to find a strong non-preference that lead the insect to not feeding from a host (Cardona and Sotelo, 2005). However, different chemical or physical factors, e.g. volatiles from damage and undamaged plants or root exudates, mediate spittlebug choice of a host (Silva et al., 2017, 2019) determining insect behavior as it can encourage or deter feeding and oviposition (Cosenza, 1982; Bernays and Chapman, 1994). Consequently, this mechanism presents the potential use of plants that exhibit host-plant resistance in diversified or push-pull pasture arrangements, aiming to establish intensive and sustainable livestock systems.
Intra and interspecific genetic independence of resistance was reported, as levels of the main two categories of resistance (i.e., antibiosis and tolerance) vary among Urochloa accessions when are attacked by nymphs or adults of different spittlebug species (Cardona et al., 2004, 2010). Miles et al. (1995, 2006) suggested that the genetic basis for resistance to spittlebug attack is not complex, has more than a single major gene involved, and it improves in response to intense, recurrent selection on reliable phenotypic data obtained through screening tests. Nevertheless, mechanisms of resistance involved in antibiosis and tolerance have not been dilucidated yet. Some studies aimed to evaluate possible resistance factors using synthetic chemical inducers as it stimulates or primes the endogenous immunity of plants to tackle pathogenic attack (Zhou and Wang, 2018). Wetland and terrestrial grasses, including sugarcane, are high to medium silicon accumulators (Alhousari and Greger, 2018). This element is involved in plant regulation of resistance mechanism to pathogen and insect attacks. For sugarcane, using silicic acid in Urochloa grasses, Pennisetum purpureum Schumach, Digitaria sp., and two sugarcane cultivars, RB73-9735 and RB83-5486, did not show a significant effect on Mahanarva spectabilis biological parameters or agronomic characteristics suggesting that is not effective for triggering resistance (Auad and Resende, 2018; Alvarenga et al., 2019a).
Concluding Remarks
Spittlebugs are distributed along tropical and subtropical America, presenting differences in their life cycles, behavior, and distribution. Ecological niche models estimate a variation in current and future suitable habitats under different climate change scenarios depending on the species. Thus, invasion of new species and changes in the population dynamics of these insects may threat sugarcane and pasture productivity for Latin America and the Caribbean in the next years. The extensive nature of these systems makes necessary to use different strategies for spittlebug control, reducing unsustainable practices such as burning and pesticide applications. IPM is not only the integration of strategies but also the collaboration between the research institutes, scientific community, private industry, stakeholders, farmers, and public institutions. Consequently, the development of new cultivars by breeding programs that incorporate host plant resistance is a long term and low-cost strategy that, combined with the above mentioned cultural, mechanical, microbial, and biological control options, constitute preventive and curative measures that producers may adopt to manage this pest with an IPM approach.
Author Contributions
PE, LH, and MM: conceptualization, formal analysis, and resources. PE and LH: writing the original draft and review and editing. MM: supervision. All authors contributed to the article and approved the submitted version.
Funding
This work was funded by the CGIAR Research Program on Livestock. The funders had no role in the design of the study; in the collection, analyses, or interpretation of data; in the writing of the manuscript, or in the decision to publish the results.
Conflict of Interest
The authors declare that the research was conducted in the absence of any commercial or financial relationships that could be construed as a potential conflict of interest.
Publisher's Note
All claims expressed in this article are solely those of the authors and do not necessarily represent those of their affiliated organizations, or those of the publisher, the editors and the reviewers. Any product that may be evaluated in this article, or claim that may be made by its manufacturer, is not guaranteed or endorsed by the publisher.
Acknowledgments
The authors express their deepest gratitude to all researchers who have generated invaluable information which has made it possible to optimize integrated spittlebug management strategies to obtain a sustainable production.
References
Aguiar, M., Auad, A. M., Fonseca, M. G., and Leite, M. V. (2014). Brachiaria ruziziensis responses to different fertilization doses and to the attack of Mahanarva spectabilis (Hemiptera: Cercopidae) nymphs and adults. Sci. World J. 2014, 543813. doi: 10.1155/2014/543813
Alhousari, F., and Greger, M. (2018). Silicon and mechanisms of plant resistance to insect pests. Plants 7, 33. doi: 10.3390/plants7020033
Almeida, P. C., Davila, H., Corsi, M., Goulart, R., and Santos, P. (2005). Nível de infestação de cigarrinhas em áreas com a presença de morte do capim Brachiaria brizantha cv. Marandu. in 42° Reunião Anual da Sociedade Brasileira de Zootecnia, (Goiania: Embrapa), 25–28.
Alvarenga, R., Auad, A. M., Moraes, J. C., and Silva, S. E. B. (2019a). Do silicon and nitric oxide induce resistance to Mahanarva spectabilis (Hemiptera: Cercopidae) in forage grasses? Pest Manag. Sci. 75, 3282–3292. doi: 10.1002/ps.5450
Alvarenga, R., Auad, M., Moraes, J. C., Barbosa da Silva, S. E., and Santos Rodrigues, B. (2019b). Tolerance to nymphs and adults of Mahanarva spectabilis (Hemiptera: Cercopidae) by forage plants in fertilized soils. Pest Manag. Sci. 75, 2242–2250. doi: 10.1002/ps.5361
Alves, R. T., and Carvalho, G. S. (2014). Primeiro registro das espécies de cigarrinhas-da-raiz da cana-de-açúcar Mahanarva spectabilis (Distant) e Mahanarva liturata (Le Peletier & Serville) atacando canaviais na regi?o de Goian?sia (GO), Brasil. Arq. Inst. Biol. 81, 83–85. doi: 10.1590/S1808-16572014000100016
Auad, A., Simões, A., Leite, M., da Silva, S. E., Santos, D., and Monteiro, P. (2011). Seasonal Dynamics of Egg Diapause in Mahanarva Spectabilis (Distant, 1909) (Hemiptera : Cercopidae) on Elephant Grass. Arq. Inst. Biol. (Sao. Paulo). 78, 325–330. doi: 10.1590/1808-1657v78p3252011
Auad, A. M., Domingues, R., Machado, M. A., Souza, L. S., Carvalho, G. S., and Paula-Moraes, S. V. (2010). Genetic variability of Mahanarva sp (Hemiptera: Cercopidae) collected from different sites in Brazil. Genet. Mol. Res. 9, 1005–1010. doi: 10.4238/vol9-2gmr794
Auad, A. M., and Resende, T. T. (2018). Use of chemical inducers as a resistance trigger in Brachiaria grasses and sugarcane. Fla. Entomol. 101, 119–124. doi: 10.1653/024.101.0121
Badilla, F. (2002). Un programa exitoso de control biológico de insectos plaga de la caña de azúcar en Costa Rica. Manejo Integr. Plagas y Agroecol. (Costa Rica) 64, 77–87.
Batista, E. S. P., Auad, A. M., Andal,ó, V., and Monteiro, C. M. de O. (2014). Virulence of entomopathogenic nematodes (Rhabditida: Steinernematidae, Heterorhabditidae) to spittlebug Mahanarva spectabilis (Hemiptera: Cercopidae). Arq. Inst. Biol. (Sao. Paulo). 81, 145–149. doi: 10.1590/1808-1657001152012
Bautista-Gálvez, A., and González-Cortes, N. (2005). Tres dosis de Metarhizium anisopliae sobre la mosca pinta (Aeneolamia spp.) en caña de azúcar en la región de los ríos, estado de Tabasco. Ecos. Rev. Agrop. 21, 37–40. Available online at: https://www.redalyc.org/articulo.oa?id=15404105
Beck, E. W. (1963). Observations on the biology and cultural-insecticidal control of Prosapia bicincta, a Spittlebug, on Coastal Bermudagrass. J. Econ. Entomol. 56, 747–752. doi: 10.1093/jee/56.6.747
Berg, C. (1879). Hemiptera. Argentina. (Continuacion.), Vol. 8. Buenos Aires: Anales de la Sociedad Cientifica Argentina. p. 209–226.
Bernays, E. A., and Chapman, R. E. (1994). “Behavior: The Process of Host-Plant Selection,” in Host-Plant Selection by Phytophagous Insects. Contemporary Topics in Entomology. (Boston, MA: Springer US), 95–165.
Bhat, A. H., Chaubey, A. K., and Askary, T. H. (2020). Global distribution of entomopathogenic nematodes, Steinernema and Heterorhabditis. Egypt. J. Biol. Pest Control 30, 1–15. doi: 10.1186/s41938-020-0212-y
Brunner-Mendoza, C., Moonjely, S., Reyes-Montes, M., Toriello, C., and Bidochka, M. (2017). Physiological and phylogenetic variability of Mexican Metarhizium strains. BioControl 62, 779–791. doi: 10.1007/s10526-017-9839-3
Brunner-Mendoza, C., Reyes-Montes, M., Moonjely, S., Bidochka, M. J., and Toriello, C. (2019). A review on the genus Metarhizium as an entomopathogenic microbial biocontrol agent with emphasis on its use and utility in Mexico. Biocontrol Sci. Technol. 29, 83–102. doi: 10.1080/09583157.2018.1531111
Bueno, V. H. (1987). Aspectos biológicos e ritmo diário das atividades de Porasilus barbiellinii predador da cigarrinha-das-pastagens. Pesqui. Agropecu. Bras. 22, 903–915.
Burrows, M. (2006). Jumping performance of froghopper insects. J. Expt. Biol. 209, 4607–4621. doi: 10.1242/jeb.02539
Burrows, M., Hartung, V., and Hoch, H. (2007). Jumping behaviour in a Gondwanan relict insect (Hemiptera: Coleorrhyncha: Peloridiidae). J. Expt. Biol. 210, 3311–3318. doi: 10.1242/jeb.007914
Busoli, A. C., Netto, J. C., Viana, D., de, L., Aguirre-Gil, O. J., Silva, E. A., et al. (2014). Manejo Integrado de Pragas: Pesquisas, avanços e desafios. Tópicos em Entomol. Agrícola VII 392, 310–329.
Bustillo, P. A. E., and Castro, U. (2011). El salivazo de la caña de azúcar Aeneolamia varia (F.) (Hemiptera: Cercopidae). (Cali, Colombia: Cenicaña Colombia).
Bustillo, P. A. E., Obando, B. J. A, and Castro, U. (2011). Control biológico del salivazo Aeneolamia varia (F.) (Hemiptera: Cercopidae). Uso del hongo Metarhizium anisopliae (Metsch.) Sorokin. Serie divulgativa 12. (Cali, Colombia: Cenicaña Colombia).
Cabanillas, H. E., Poinar Jr, G. O., and Raulston, J. R. (1994). Steinernema riobravis n. sp. (Rhabditida: Steinernematidae) from Texas. Fundam. Appl. Nematol. 17, 123–131. Available online at: https://www.documentation.ird.fr/hor/fdi:39973
Cardona, C., Fory, P., Sotelo, G., Pabon, A., Diaz, G., and Miles, J. W. (2004). Antibiosis and Tolerance to Five Species of Spittlebug (Homoptera: Cercopidae) in Brachiaria spp.: Implications for Breeding for Resistance. J. Econ. Entomol. 97, 635–645. doi: 10.1603/0022-0493-97.2.635
Cardona, C., Miles, J. W., and Sotelo, G. (1999). An Improved Methodology for Massive Screening of Brachiaria spp. Genotypes for Resistance to Aeneolamia varia (Homoptera: Cercopidae). J. Econ. Entomol 92, 490–496. doi: 10.1093/jee/92.2.490
Cardona, C., Miles, J. W., Zuñiga, E., and Sotelo, G. (2010). Independence of Resistance in Brachiaria spp. to Nymphs or to Adult Spittlebugs (Hemiptera: Cercopidae): implications for Breeding for Resistance. J. Econ. Entomol. 103, 1860–1865. doi: 10.1603/EC10004
Cardona, C., and Sotelo, G. (2005). Mecanismos de resistencia a insectos: naturaleza e importancia en la formulación de estrategias de mejoramiento para incorporar resistencia a salivazo en Brachiaria. Pasturas Trop. 27, 1–11. Available online at: https://www.tropicalgrasslands.info/public/journals/4/Elements/DOCUMENTS/2005-vol27-rev1-2-3/Vol_27_rev2_05_pags_2-11.pdf.
Carvalho, G. S., and Webb, M. M. D. (2005). Cercopid Spittle Bugs of the New World (Hemiptera, Auchenorrhyncha, Cercopidae). Bulgaria: Pensoft Publishers.
Castillo, S. (2006). Uso de Metarhizium anisopliae para el control biológico del salivazo (Aeneolamia spp. y Prosapia spp.) en pastizales de Brachiaria decumbens en El Petén, Guatemala (master's thesis). Escuela de posgrado del CATIE, Turrialba, Costa Rica
Castro, U., Carvalho, G., Peck, D., Valdez–Carrasco, J., and Romero–N'apoles, J. (2018). Two new species of the spittlebug genus Ocoaxo Fennah (Hemiptera: Cercopidae) from Mexico, and keys for the groups, group three, and first subgroup. Neotrop. Entomol. 48, 260–268. doi: 10.1007/s13744-018-0629-0
Castro, U., Carvalho, G., Peck, D., Valdez–Carrasco, J., and Romero–N'apoles, J. (2020). Description of two new species of Prosapia (Hemiptera: Cercopidae) from the Nearctic and Neotropics, with a key to species of the P. inferens group. Cana. Entomol. 152, 288–299. doi: 10.4039/tce.2020.20
Castro, U., Morales, A., and Peck, D. C. (2005). Dinámica poblacional y fenología del salivazo de los pastos Zulia carbonaria (Lallemand) (Homoptera: Cercopidae) en el Valle geográfico del Río Cauca, Colombia. Neotrop. Entomol. 34, 459–470. doi: 10.1590/S1519-566X2005000300015
Cavichioli, R. R., and Sakakibara, A. M. (1993). Deois Fennah, Descrição de uma espécie nova e notas taxonômicas (Homoptera, Cercopidae, Tomaspidinae). Rev. Bras. Zool. 10, 747–750. doi: 10.1590/S0101-81751993000400019
Chalarca, J. R., Peck, D., and Canal, N. (2002). Biología comparada de tres especies de salivazo de los pastos del género Zulia (Homoptera: Cercopidae). Rev. Colomb. Entomol. 28, 17–25. Available online at: https://hdl.handle.net/10568/44245
Chaves, V. D. V., Pimentel, G. V., Valverde, A. H. P., Silva, L. A., Barbosa, M. H. P., and Peternelli, L. A. (2014). Biology and preferred oviposition site of the Mahanarva indentata froghopper (Hemiptera: Cercopidae) on sugarcane. Fla. Entomol. 97, 73–79. doi: 10.1653/024.097.0109
Chen, X., Meyer-Rochow, V., Fereres, A., Morente, M., and Liang, A. (2018). The role of biofoam in shielding spittlebug nymphs (Insecta, Hemiptera, Cercopidae) against bright light. Ecol. Entomol. 43, 273–281. doi: 10.1111/een.12496
Cid-Muñoz, R., Cibrián-Tovar, D., Valadez-Moctezuma, E., Estrada-Martínez, E., and Armendáriz-Toledano, F. (2020). Biology and Life Stages of Pine Spittle Bug Ocoaxo assimilis Walker (Hemiptera: Cercopidae). Insects 11, 96. doi: 10.3390/insects11020096
Congio, G. F. S., de Almeida, P. C., Barreto, T. R., Tinazo, V. A., da Silva, T. A. C. C., Costa, D. F. A., et al. (2020). Spittlebug damage on tropical grass and its impact in pasture-based beef production systems. Sci. Rep. 10, 1–12. doi: 10.1038/s41598-020-67490-9
Cosenza, G. W. (1982). Resistência de gramíneas forrageiras a cigarrinha-das- pastagens Deois flavopicta (Stal., 1854), 2nd ed. Planaltina, Brazil: Embrapa Centro de pesquisa agropecuaria dos Cerrados.
Crews, L. J., McCully, M. E., Canny, M. J., Huang, C. X., and Ling, L. E. (1998). Xylem feeding by spittlebug nymphs: some observations by optical and cryo-scanning electron microscopy. Am. J. Bot. 85, 449–460. doi: 10.2307/2446427
Cruz, I., Fernandes da Silva, I., Souza, C., Corrêa, M., Gontijo, M., and Braga da Silva, R. (2009). Damage of the Spittlebug Deois flavopicta (Stal) (Hemiptera: Cercopidae) to maize in intercropping system with Brachiaria grass. Rev. Bras. Milho e Sorgo 8, 117–130. doi: 10.18512/1980-6477/rbms.v8n2p117-130
Cryan, J. R., and Svenson, G. J. (2010). Family-level relationships of the spittlebugs and froghoppers (Hemiptera: Cicadomorpha: Cercopoidea). Syst. Entomol. 35, 393–415. doi: 10.1111/j.1365-3113.2009.00520.x
Cryan, J. R., and Urban, J. M. (2012). Higher-level phylogeny of the insect order Hemiptera: is auchenorrhyncha really paraphyletic? Syst. Entomol. 37, 7–21. doi: 10.1111/j.1365-3113.2011.00611.x
Cuarán, V. L. (2012). Respuesta de variedades de la caña de azúcar (Saccharum spp.) al ataque de las ninfas el salivazo Aeneolamia varia (f.) (Hemiptera: Cercopidae). Tesis Maestría Ciencias Agrarias. Available online at: https://repositorio.unal.edu.co/handle/unal/32750
Dale, C., and Maudlin, I. (1999). Sodalis gen. nov. and Sodalis glossinidius sp. nov., a microaerophilic secondary endosymbiont of the tsetse fly Glossina morsitans morsitans. Int. J. Syst. Evol. Microbiol. 49, 267–275.
de Castro, S. G. Q., Dinardo-Miranda, L. L., Fracasso, J. V., Bordonal, R. O., Menandro, L. M. S., and Franco, H. C. J. (2019). Changes in Soil Pest Populations Caused by Sugarcane Straw Removal in Brazil. Bioenergy Res. 12, 878–887. doi: 10.1007/s12155-019-10019-4
de la Cruz-Llanas, J., Vera-Graziano, J., Lopez-Collado, J., Pinto, V., and Garza-García, R. (2005). Una técnica simple para el desarrollo de ninfas de Aeneolamia postica (Homoptera: Cercopidae). Folia Entomol. Mex. 44, 91–93.
de la Cruz-Zapata, G., García-López, E., Sánchez-Soto, S., Bautista-Martínez, N., Ortiz-Díaz, J. J., and Osorio-Osorio, R. (2016). Identidad de Mosca Pinta (Hemiptera : Cercopidae) y sus Hospederas en Cañaverales en Cárdenas, Tabasco, México. Southwest. Entomol. 41, 145–152. doi: 10.3958/059.041.0116
de Oliveira, H. N., Antigo, M. R., de Carvalho, G. A., Glaeser, D. F., and Pereira, F. F. (2013). Seletividade de inseticidas utilizados na cana-de-açúcar a adultos de Trichogramma galloi Zucchi (Hymenoptera: Trichogrammatidae). Biosci. J. 29, 1266–1273.
de Paula Batista, E., and Auad, A. (2010). Application methods of entomopathogenic nematodes for control of Mahanarva spectabilis (Hemiptera: Cercopidae). Biocontrol Sci. Technol. 20, 1079–1085. doi: 10.1080/09583157.2010.515300
Dietrich, C. H. (2005). Keys To the Families of Cicadomorpha and Subfamilies and Tribes of Cicadellidae (Hemiptera: Auchenorrhyncha). Florida Entomol. 88, 502–517. doi: 10.1653/0015-4040(2005)88502:KTTFOC2.0.CO;2
Dietrich, C. H. (2009). “Auchenorrhyncha: (Cicadas, Spittlebugs, Leafhoppers, Treehoppers, and Planthoppers),” in Encyclopedia of Insects, eds. Resh, V., and Cardé, R. (San Diego, California: Academic Press Inc.), 56–64.
Dinardo-Miranda, L. L. (2008). “Pragas,” in Cana-de-açúcar, eds. Dinardo-Miranda, A. C. M., Vasconcelos, L. L. and Landell, M. G. A. (Campinas, Brazil: Campinas: Instituto Agronômico), 349–404.
Dinardo-Miranda, L. L., Coelho, Á. L., and Ferreira, J. M. G. (2004). Influência da época de aplicação de inseticidas no controle de Mahanarva fimbriolata (Stål) (Hemiptera: Cercopidae), na qualidade e na produtividade da cana-de-açúcar. Neotrop. Entomol. 33, 91–98. doi: 10.1590/S1519-566X2004000100016
Dinardo-Miranda, L. L., da Costa, V. P., Fracasso, J. V., Perecin, D., de Oliveira, M. C., Izeppi, T. S., et al. (2014). Resistance of Sugarcane Cultivars to Mahanarva fimbriolata (Stål) (Hemiptera: Cercopidae). Neotrop. Entomol. 43, 90–95. doi: 10.1007/s13744-013-0182-9
Dinardo-Miranda, L. L., Fracasso, J. V., Perecin, D., Miranda, I. D., Réchia, N. M., and dos Santos Buzzatto, D. F. (2018). Resistance of sugarcane cultivars to Mahanarva fimbriolata. Bragantia 77, 1–12. doi: 10.1590/1678-4499.2017162
Dinardo-Miranda, L. L., Fracasso, J. V., Perecin, D., Oliveira, M. C., de Lopes, D. O. P., and Izeppi, T. S. (2016). Resistance mechanisms of sugarcane cultivars to spittlebug Mahanarva fimbriolata. Sci. Agric. 73, 115–124. doi: 10.1590/0103-9016-2014-0446
Dinardo-Miranda, L. L., and Gil, M. A. (2007). Estimativa do nível de dano econômico de Mahanarva fimbriolata (Stål) (Hemiptera: Cercopidae) em cana-de-açúcar. Bragantia 66, 81–88. doi: 10.1590/S0006-87052007000100010
Dinardo-Miranda, L. L., Pivetta, J. P., and Fracasso, J. V. (2008). Economic injury level for sugarcane caused by the spittlebug Mahanarva fimbriolata (Stål) (Hemiptera : Cercopidae). Sci. Agric. 65, 16–24. doi: 10.1590/S0103-90162008000100003
Doering, K. C. (1929). The Genus Clastoptera in America North of Mexico, Vol.18. Lawrence, KS: Bulletin of the University of Kansas. p. 5–153.
Douglas, A. E. (1989). Mycetocyte simbiosis in insects. Biol. Rev. 64, 409–434. doi: 10.1111/j.1469-185X.1989.tb00682.x
Fabricius, J. C. (1787). “Ryngota,” in Mantissa insectorum sistens species nuper detectas adiectis synonymis, observationibus, descriptionibus, emendationibus, Vol. 2. p. 260–275.
Fagan, E. B., and Kuitert, L. C. (1969). Biology of the two-lined spittlebug, Prosapia bicincta, on Florida pastures (Homoptera: Cercopidae). Fla. Entomol. 52, 199–206. doi: 10.2307/3493854
Fennah, R. G. (1968). Revisionary notes on the New World genera of cercopid froghoppers (Homoptera: Cercopoidea). Bull. Entomol. Res. 58, 165–190. doi: 10.1017/S0007485300055954
Fernández, M. J. (2013). Manejo integrado de chinche salivosa (Aeneolamia postica; Cercopidae) en caña de azúcar en el ingenio Pantaleón. Siquinalá, Escuintla. Sistematizacón de práctica profesional.
Ferreira, E., Barrigossi, J. A., and Castro, E. D. (2003). Homópteros associados ao arroz. Embrapa Gado de Corte.
Figueredo, L., Andrade, O., Cova, J., Mora, O., and Aza, G. (2012). Distribución espacio temporal de ninfas de Aeneolamia varia fabricius (1787) (Hemiptera: Cercopidae) en caña de azúcar a través de un sistema de información geográfica. Entomotropica 27, 7–18.
Foieri, A., de Remes Lenicov, A. M., and Virla, E. G. (2016a). Description of the immature stages and new host plant records of Deois (Deois) mourei (Berg) (Hemiptera: Cercopidae), a species newly recorded from Argentina and Paraguay. Zootaxa. 4161, 419–428. doi: 10.11646/zootaxa.4161.3.9
Foieri, A., de Remes Lenicov, A. M., and Virla, E. G. (2016b). Descriptions of the immature stages and new host plant records of Notozulia entreriana (Berg)(Hemiptera: Cercopidae) pests of grasses in subtropical areas of the Americas. Zootaxa. 4103, 54–62. doi: 10.11646/zootaxa.4103.1.5
Foieri, A., and de Remes, L. A. M. (2019). Cercopoidea types (Hemiptera-Cicadomorpha) housed at the Museo de la Plata entomological collection (Argentina). Pap. Avulsos Zool, 59. doi: 10.11606/1807-0205/2019.59.16
Foieri, A., Decker-Franco, C., Marino de Remes Lenicov, A. M., and Arneodo, J. D. (2022). First identification of bacterial endosymbionts in three South-American spittlebug pests: Notozulia entreriana, Deois mourei and Deois knoblauchii. Bull. Insectol. 75, 1–6.
García, A., Bustillo, P., Castro, U., and Arenas, Y. (2012). Selección de hongos entomopatógenos para controlar salivazos (Hemiptera: Cercopidae) de la caña de azúcar en Colombia. Rev. Colomb. Entomol. 38, 252–259. doi: 10.25100/socolen.v38i2.9001
Garcia, J. F., Grisoto, E., Botelho, P. S. M., Parra, J. R. P., and Appezzato-da-Glória, B. (2007). Feeding site of the spittlebug Mahanarva fimbriolata (Stål) (Hemiptera: Cercopidae) on sugarcane. Sci. Agric. 64, 555–557. doi: 10.1590/S0103-90162007000500014
García-González, J. C., López-Collado, J., Gilberto, C., Villanueva-Jiménez, J. A., and Nava-Tablada, M. A. (2017). Factores bióticos, abióticos y agronómicos que afectan las poblaciones de adultos de mosca pinta (Hemiptera: Cercopidae) en cultivos de caña de azúcar en Veracruz, México. Acta Zoológica Mex. 33, 508–517. doi: 10.21829/azm.2017.3331152
GBIF.org. (2022). Ocurrences Spittlebug. Available online at: https://www.gbif.org/occurrence/map?q=spittlebug (accessed February 2, 2022).
Gómez, L. A. (2007). Manejo del salivazo Aeneolamia varia en cultivos de caña de azúcar en el valle del río Cauca. Valle del Cauca: Cenicaña.
Grifaldo-Alcántara, P. F., Alatorre-Rosas, R., Villanueva-Jiménez, J. A., Hernández-Rosas, F., Stock, S. P., and Ram?rez-Valverde, G. (2019). Evaluación de dos cepas de nematodos entomopatógenos (Steinernematidae, Heterorhabditidae) para el control del salivazo (Hemiptera: Cercopidae) en caña de azúcar. Nematropica. 49, 83–90. Available online at: https://journals.flvc.org/nematropica/article/view/115628
Grisoto, E., Vendramim, J. D., Lourenção, A. L., Usberti Filho, J. A., and Alves de Olinda, R. (2018). Evaluation of forage grass resistance to Mahanarva fimbriolata (Stål). Bragantia 77, 1–9. doi: 10.1590/1678-4499.2016280
Gusmão, M. R., Valério, J. R., Matta, F. P., Souza, F. H., Vigna, B. B., Fávero, A. P., et al. (2016). Warm-season (C4) turfgrass genotypes resistant to spittlebugs (Hemiptera: Cercopidae). J. Econ. Entomol. 109, 1914–1921. doi: 10.1093/jee/tow135
Gutiérrez, Y., and Gómez, L. A. (2009). Algunas experiencias en el manejo del salivazo Aeneolamia varia (Hemiptera: Cercopidae) en el valle del Cauca. in VIII Congreso de la Asociación Colombiana de Técnicos de la Caña de Azúcar, (Cali, Colombia: Asociación Colombiana de Técnicos de la Caña de Azúcar), 160–167.
Hagley, E. A. C., and Blackman, J. A. (1966). Site of feeding of the sugarcane froghopper, Aeneolamia varia saccharina (Homoptera: Cercopidae). Ann. Entomol. Soc. Am. 59, 1289–1291. doi: 10.1093/aesa/59.6.1289
Hamilton, K. G. A. (2013). Revision of Neotropical aphrophorine spittlebugs, part 2: Tribe Orthoraphini (Hemiptera, Cercopoidea). Zootaxa 3710, 201–225. doi: 10.11646/zootaxa.3710.3.1
Hamilton, K. G. A., and Morales, C. (1992). “Cercopidae (Insecta: Homoptera),” in Fauna of New Zealand, eds, Hamilton, K. G. A, (Auckland, New Zealand: DSIR Plant Protection), 40.
Heinrichs, E. A., and Muniappan, R. (2017). IPM for tropical crops: Rice. CAB Rev. 12, 1–31. doi: 10.1079/PAVSNNR201712030
Hernandez, L. M., Espitia, P., Castiblanco, V., and Cardoso, J. A. (2020). Digital imaging outperforms traditional scoring methods of spittlebug tolerance in Urochloa humidicola hybrids. bioRxiv [Preprint]. Available online at: https://www.biorxiv.org/content/10.1101/2020.10.13.338186v1 (accessed February 3, 2022).
Hernandez, L. M., Espitia, P., Florian, D., Castiblanco, V., Cardoso, J. A., and Gómez-Jiménez, M. I. (2021). Geographic Distribution of Colombian Spittlebugs (Hemiptera: Cercopidae) via Ecological Niche Modeling: A Prediction for the Main Tropical Forages' Pest in the Neotropics. Front. Sustain. Food Syst. 5, 1–17. doi: 10.3389/fsufs.2021.725774
Hernandez, L. M., Gomez, M. I., Miles, J. W., and Castiblanco, V. (2017). “Database of cercopidae specimens deposited in ciat arthropod reference collection, mendez, Brasil,” in Program and Abstracts Book: 15th International Auchenorrhyncha Congress and 10th International Workshop on Leafhoppers and Planthoppers of Economic Importance.
Hernández-Domínguez, C., Guzmán-Franco, A. W., Carrillo-Benítez, M. G., Alatorre-Rosas, R., Rodríguez-Leyva, E., and Villanueva-Jiménez, J. A. (2016). Specific Diversity of Metarhizium Isolates Infecting Aeneolamia spp. (Hemiptera: Cercopidae) in Sugarcane Plantations. Neotrop. Entomol. 45, 80–87. doi: 10.1007/s13744-015-0337-y
Herrera-Huerta, R., Lopez-Collado, J., Bravo-Mojica, H., and Domínguez-Valenzuela, J. (2004). Efecto de la temperatura y la precipitación en la eclosión de los huevos de Aeneolamia postica (Walker) (Homoptera: Cercopidae). in XVII Reunión Científica-Tecnológica Forestal y Agropecuaria Veracruz, (Veracruz: Mesa Agrícola), p. 6.
Holmann, F., and Peck, D. C. (2002). Economic Damage Caused by Spittlebugs (Homoptera : Cercopidae) in Colombia : A First Approximation of Impact on Animal Production in Brachiaria decumbens Pastures. Neotrop. Entomol. 31, 275–284. doi: 10.1590/S1519-566X2002000200016
Johnson, K. P., Dietrich, C. H., Friedrich, F., Beutel, R. G., Wipfler, B., and Peters, R. S. (2018). Phylogenomics and the evolution of hemipteroid insects. Proc. Natl. Acad. Sci. U. S. A. 115, 12775–12780. doi: 10.1073/pnas.1815820115
Jusys, T. (2017). A confirmation of the indirect impact of sugarcane on deforestation in the Amazon. J. Land Use Sci. 12, 125–137. doi: 10.1080/1747423X.2017.1291766
Karban, R., and Huntzinger, M. (2018). Decline of meadow spittlebugs, a previously abundant insect, along the California coast. Ecology 99, 2614–2616. doi: 10.1002/ecy.2456
Kassab, S. O., De Souza Loureiro, E., Rossoni, C., Pereira, F. F., Barbosa, R. H., Costa, D. P., et al. (2014). Combinations of Metarhizium anisopliae with chemical insecticides and their effectiveness in Mahanarva fimbriolata (Hemiptera: Cercopidae) control on sugarcane. Fla. Entomol. 97, 146–154. Available online at: https://www.jstor.org/stable/24362448
Keller-Grein, G., Maass, B. L., and Hanson, J. (1996). “Natural variation in Brachiaria and existing germplasm collections,” in Brachiaria: Biology, Agronomy and Improvement, eds. Miles, J., Maass, B. L., and do Valle, C. B,. (Cali, Colombia: CIAT, Embrapa), 16–42.
Koga, R., Bennett, G. M., Cryan, J. R., and Moran, N. A. (2013). Evolutionary replacement of obligate symbionts in an ancient and diverse insect lineage. Environ. Microbiol. 15, 2073–2081. doi: 10.1111/1462-2920.12121
Koga, R., Meng, X., Tsuchida, T., and Fukatsu, T. (2012). Cellular mechanism for selective vertical transmission of an obligate insect symbiont at the bacteriocyte-embryo interface. Proc. Natl. Acad. Sci. U. S. A. 109, 1230–1237. doi: 10.1073/pnas.1119212109
Koga, R., and Moran, N. A. (2014). Swapping symbionts in spittlebugs: evolutionary replacement of a reduced genome symbiont. ISME J. 8, 1237–1246. doi: 10.1038/ismej.2013.235
Koller, W. W. (1987). Tratos culturais em pastagens de Brachiaria decumbens Stapf: efeitos sobre os ovos, ninfas e adultos das cigarrinhas-das-pastagens (Homoptera: Cercopidae). An. Soc. Entomol. Bras. 17, 409–420. doi: 10.37486/0301-8059.v17i2.533
Lallemand, V. (1924). Notes sur les Cercopides de l'Amérique Centrale et Méridionale de la collection de M. C. B. Williams et de la mienne. Ann. Magaz. Nat. Hist. 14, 477–486.
Leite, L. G., Machado, L. A., Goulart, R. M., Tavares, F. M., and Batista Filho, A. (2005). Screening of entomopathogenic nematodes (Nemata: Rhabditida) and the efficiency of Heterorhabditis sp. against the sugarcane root spittlebug Mahanarva fimbriolata (Fabr.) (Hemiptera: Cercopidae). Neotrop. Entomol. 34, 785–790. doi: 10.1590/S1519-566X2005000500010
Lewis, E. E., Campbell, J., Gri, C., Kaya, H., and Peters, A. (2006). Behavioral ecology of entomopathogenic nematodes. Biol. Control 38, 66–79. doi: 10.1016/j.biocontrol.2005.11.007
Lopez, F., Cardona, C., Miles, J. W., Sotelo, G., and Montoya, J. (2009). Screening for Resistance to Adult Spittlebugs (Hemiptera: Cercopidae) in Brachiaria spp.: methods and categories of resistance. J. Econ. Entomol. 102, 1309–1316. doi: 10.1603/029.102.0358
Lopez, F., Peck, D., and Montoya, J. (2001). The importance of Vibrational Communication in the Reproductive Behaviour of Grassland Spittlebugs (Homoptera: Cercopidae). Rev. Colomb. Entomol. 27, 9–15. doi: 10.25100/socolen.v27i1.9657
Lortkipanidze, M. A., Gorgadze, O. A., Kajaia, G. S., Gratiashvili, N. G., and Kuchava, M. A. (2016). Foraging behavior and virulence of some entomopathogenic nematodes. Ann. Agrar. Sci. 14, 99–103. doi: 10.1016/j.aasci.2016.05.009
Madaleno, L. L., Ravaneli, G. C., Presotti, L. E., Mutton, M. Â., Fernandes, O. A., and Mutton, M. J. (2008). Influence of Mahanarva fimbriolata (Stål) (Hemiptera : Cercopidae) Injury on the Quality of Cane Juice. Neotrop. Entomol. 37, 68–73. doi: 10.1590/S1519-566X2008000100010
Maienfisch, P., Angst, M., Brandl, F., Fischer, W., Hofer, D., and Kayser, H. (2001). Chemistry and biology of thiamethoxam: a second generation neonicotinoid. Pest Manag. Sci. 57, 906–913. doi: 10.1002/ps.365
Marques, E., and Vilas Boas, A. (1985). Recuperação do parasito Acmopolynema hervali, de ovos de Mahanarva posticata em Carpina-Pe. Pesqui. Agropecu. Bras. 20, 271–272.
Martin, A. H., Grolle, K., Bos, M. A., Stuart, M. A. C., and van Vliet, T. (2002). Network forming properties of various proteins adsorbed at the air/water interface in relation to foam stability. J. Colloid and Interface Sci. 254, 175–183. doi: 10.1006/jcis.2002.8592
Martín-Rivera, M. (1994). The Effect of Climate and Spittlebug (Aeneolamia albofasciata) on Buffelgrass (Cenchrus cilaris L.) productivity in the Sonoran Desert (dissertation). Utah State University, Logan, UT, United States.
Mascarin, G., Lopes, R., Delalibera, I. J., Fernandes, E. K., Luz, C., and Faria, M. (2019). Current status and perspectives of fungal entomopathogens used for microbial control of arthropod pests in Brazil. J. Invertebr. Pathol. 165, 46–53. doi: 10.1016/j.jip.2018.01.001
Matabanchoy, J., Bustillo, A. E., Castro-Valderrama, U., Mesa, N. C., and Moreno Gil, C. A. (2012). Eficacia de Metarhizium anisopliae para controlar Aeneolamia varia (Hemiptera: Cercopidae), en caña de azúcar. Rev. Colomb. Entomol. 38, 177–181. Available online at: http://ref.scielo.org/ssv34q
McCutcheon, J. P., and Moran, N. A. (2010). Functional convergence in reduced genomes of bacterial symbionts spanning 200 my of evolution. Genome Biol. Evol. 2, 708–718. doi: 10.1093/gbe/evq055
Medina, C. A. (1995). Hormigas depredadoras de huevos de salivazo de los pastos Aeneolamia varia (Hemiptera: Eercopidae) en pasturas de Brachiaria, en los llanos orientales de Colombia. Bol. Mus. Ent. Univ. Valle. 3, 1–13.
Miles, J. W., Cardona, C., and Sotelo, G. (2006). Recurrent selection in a synthetic brachiariagrass population improves resistance to three spittlebug species. Crop Sci. 46, 1088–1093. doi: 10.2135/cropsci2005.06-0101
Miles, J. W., Lapointe, S. L., Escandén, M. L., and Sotelo, G. (1995). Inheritance of Resistance to Spittlebug (Homoptera: Cercopidae) in Interspecific Brachiaria spp. Hybrids. J. Econ. Entomol. 88, 1477–1481. doi: 10.1093/jee/88.5.1477
Misof, B., Liu, S., Meusemann, K., Peters, R. S., Donath, A., and Mayer, C. (2014). Phylogenomics resolves the timing and pattern of insect evolution. Science. 346, 763–767. doi: 10.1126/science.1257570
Moran, N. A., Tran, P., and Gerardo, N. M. (2005). Symbiosis and Insect Diversification: an Ancient Symbiont of Sap-Feeding Insects from the Bacterial Phylum Bacteroidetes. Appl. Environ. Microbiol. 71, 8802–8810. doi: 10.1128/AEM.71.12.8802-8810.2005
Moreno, C. A., Bustillo Pardey, A. E., López Núñez, J. C., Castro Valderrama, U., and Ramírez Sánchez, G. D. (2012). Virulencia de nematodos entomopatógenos para el control del salivazo Aeneolamia varia (Hemiptera: Cercopidae) en caña de azúcar. Rev. Colomb. Entomol. 38, 260–265. doi: 10.25100/socolen.v38i2.9002
Morente, M., Cornara, D., Plaza, M., Durán, J. M., Capiscol, C., and Trillo, R. (2018). Distribution and Relative Abundance of Insect Vectors of Xylella fastidiosa in Olive Groves of the Iberian Peninsula. Insects 9, 175. doi: 10.3390/insects9040175
Mutton, M. J. R., Madaleno, L. L., Ravaneli, G. C., Garcia, D. B., and Mutton, M. A. (2010). Spittlebugs injury on sugarcane increased sugar colour. Proc. Int. Soc. Sugar Cane Technol. 27, 1–5.
Nachappa, P. (2004). TBiology and management of twolined spittlebug, Prosapia bicincta (Say) (Hemiptera:Cercopidae) in turfgrass (master's thesis). University of Georgia, Athens, GA, United States.
Nakano, O., Barros, L. S., and Krol, A. C. A. (2020). Efficiency of boric acid to control pasture spittlebugs Deois flavopicta and Notozulia entreriana (Hemiptera: Cercopidae). Braz. J. Agric. 95, 59–67. doi: 10.37856/bja.v95i1.3978
Nilakhe, S., da Silva, A., and de Souza Filho, J. (1984a). Sampling procedures for spittlebug adults in pastures of Brachiaria decumbens. Pesqui. Agropecu. Bras. 19, 1065–1074. https://bit.ly/3ts4vY4.
Nilakhe, S., Martin, P., Valerio, J., Werner, W., de Souza Filho, J., and Silva, A. (1984b). Sampling plans for spittlebug eggs in pastures of Brachiaria decumbens. Pesqui. Agropecu. Bras 19, 935–941.
Novotny, V., and Wilson, M. (1997). Why are there no small species among xylem-sucking insects? Evol. Ecol. 11, 419–437. doi: 10.1023/A:1018432807165
Nunes, S., Boock, A., Penteado, M. D. O., and Gomes, D. T. (1984). Brachiaria brizantha cv. Marandu, Embrapa Gado de Corte. Documentos 21.
Obando, B. J., Bustillo, P. A. E., Castro, U., and Mesa, N. (2013). Selection of strains of Metarhizium anisopliae to control Aeneolamia varia (Hemiptera: Cercopidae). Rev. Colomb. Entomol. 39, 26–33.
Olán-Hernández, J. F., Sánchez-Soto, S., Bautista-Martínez, N., Zaldivar-Cruz, J. M., and Cortez-Madrigal, H. (2016). Fluctuación poblacional de Aeneolamia contigua (Walker) en el cultivo de caña (Saccharum spp.) en Tabasco, México. Agroproductividad 9, 10–14.
Ortiz-Urquiza, A., and Keyhani, N. O. (2013). Action on the surface: entomopathogenic fungi versus the insect cuticle. Insects 4, 357–374. doi: 10.3390/insects4030357
Pabón, A., Cardona, C., Miles, J. W., and Sotelo, G. (2007). Response of resistant and susceptible Brachiaria spp. genotypes to simultaneous infestation with multiple species of spittlebugs (Hemiptera: Cercopidae). J. Econ. Entomol. 100, 1896–1903. doi: 10.1603/0022-0493(2007)1001896:RORASB2.0.CO;2
Paladini, A., and Cavichioli, R. R. (2017). A new species of Tiodus (Hemipteran, Cercopidae), with a key to species of the genus. Zootaxa. 4281, 242–245. doi: 10.11646/zootaxa.4281.1.24
Paladini, A., and Cryan, J. R. (2012). Nine new species of Neotropical spittlebugs (Hemiptera: Cercopidae: Ischnorhininae). Zootaxa 3519, 53–68. doi: 10.11646/zootaxa.3519.1.3
Paladini, A., Takiya, D. M., Cavichioli, R. R., and Carvalho, G. S. (2015). Phylogeny and biogeography of Neotropical spittlebugs (Hemiptera: Cercopidae: Ischnorhininae): Revised tribal classification based on morphological data. Syst. Entomol. 40, 82–108. doi: 10.1111/syen.12091
Paladini, A., Takiya, D. M., Urban, J. M., and Cryan, J. R. (2018). New World spittlebugs (Hemiptera: Cercopidae: Ischnorhininae): Dated molecular phylogeny, classification, and evolution of aposematic coloration. Mol. Phylogenet. Evol. 120, 321–334. doi: 10.1016/j.ympev.2017.12.020
Parada Domínguez, O., Alatorre Rosas, R., Guzmán Franco, A. W., Hernández Rosas, F., Rojas Avelizapa, L., and Ruíz Vera, V. (2019). Effect of entomopathogenic nematodes on nymphs of Aeneolamia albofasciata and its persistence in sugarcane soils of Veracruz. Rev. Mex. Ciencias Agrícolas 22, 115–127. doi: 10.29312/remexca.v0i22.1863
Parra, J. R. (2014). Biological control in Brazil: an overview. Sci. Agric. 71, 345–355. doi: 10.1590/0103-9016-2014-0167
Parsa, S., Sotelo, G., and Cardona, C. (2011). Characterizing herbivore resistance mechanisms: Spittlebugs on Brachiaria spp. as an example. J. Vis. Exp. 3047. doi: 10.3791/3047
Peck, D., Chalarca, J. R., and Gómez, L. A. (2004). Identity and first record of the spittlebug Mahanarva bipars (Hemiptera: Auchenorrhyncha: Cercopidae) on sugarcane in Colombia. Fla. Entomol. 87, 82–84. Available online at: https://www.jstor.org/stable/3496808
Peck, D. C. (2000a). Reflex bleeding in froghoppers (Homoptera: Cercopidae): variation in behavior and taxonomic distribution. Ann. Entomol. Soc. Am. 93, 1186–1194. doi: 10.1603/0013-8746(2000)0931186:RBIFHC2.0.CO;2
Peck, D. C. (2000b). New Perspectives for Managing Grassland Spittlebugs. Available online at: https://hdl.handle.net/10568/57788 (accessed February 3, 2022).
Peck, D. C. (2002). Distribución y reconocimiento del salivazo de los pastos (Homoptera: Cercopidae) en la Costa Caribe de Colombia. Pasturas Trop. 24, 4–15. Available online at: https://www.tropicalgrasslands.info/public/journals/4/Elements/DOCUMENTS/2002-vol24-rev1-2-3/Vol24_rev1_02_art2.pdf
Peck, D. C., Pérez, A., Medina, J. W., Rojas, J., and Barrios, M. (2002). Fluctuación poblacional y enemigos naturales de Aeneolamia reducta en la Costa Caribe de Colombia. Pasturas Trop. 24, 27–38. Available online at: https://www.tropicalgrasslands.info/public/journals/4/Elements/DOCUMENTS/2002-vol24-rev1-2-3/Vol24_rev1_02_art4.pdf
Peck, D. C., and Thompson, V. (2008). “Spittlebugs (Hemiptera:Cercopoidea),” in Encyclopedia of entomology, ed. J. Capinera, 3512–3515. doi: 10.1016/B978-0-12-374144-8.00203-4
Peixoto, D. (2016). Categorias de resistência de genótipos de Saccharum spp. á cigarrinha (Hemiptera: Cercopidae) da raíz (dissertation). Universidade Estadual Paulista, Jaboticabal, Brazil.
Pereira, J. P., Fernandes, P. M., Veloso, V. D. R. S., and Silva, E. A. D. (2010). Thiamethoxam no controle de Mahanarva fimbriolata, na produtividade e na qualidade tecnológica da cana-de-açúcar. Agrociencia (Uruguay). 14, 26–32.
Pérez-Bañón, C., Arcaya, E., Mengual, X., and Rojo, S. (2013). Preimaginal morphology of the genera Salpingogaster Schiner, 1868 and Eosalpingogaster Hull, 1949 (Diptera: Syrphidae), with its systematic implications. Zootaxa 3599, 361–370. doi: 10.11646/zootaxa.3599.4.4
Pires, C. S., Price, P. W., and de Oliveira, R. C. (2000). Distribution of the Spittlebug Deois flavopicta (Stal, 1854) (Homoptera : Cercopidae) on wild and cultivated host species. An. Soc. Entomol. Bras. 29, 401–412. doi: 10.1590/S0301-80592000000300003
Rakitov, R. A. (2002). Structure and function of the Malpighian tubules, and related behaviors in juvenile cicadas: evidence of homology with spittlebugs (Hemiptera: Cicadoidea and Cercopoidea). Zool. Anz. 241, 117–130. doi: 10.1078/0044-5231-00025
Ravaneli, G. C., Garcia, D. B., Madaleno, L. L., Mutton, M. A., Stupiello, J. P., and Rossini Mutton, M. J. (2011). Spittlebug impacts on sugarcane quality and ethanol production. Pesq. Agropec. Bras. 46, 120–129. doi: 10.1590/S0100-204X2011000200002
Redak, R. A., Purcell, A. H., Lopes, J. R. S., Blua, M. J., Mizell, I. I. I. R. F., et al. (2004). The biology of xylem fluid-feeding insect vectors of Xylella fastidiosa and their relation to disease epidemiology. Annu. Rev. Entomol. 49, 243–270. doi: 10.1146/annurev.ento.49.061802.123403
Resende, T. T., Auad, A. M., Fonseca, M. D. G., Souza Sobrinho, F., Ribeiro dos Santos, D., and da Silva, S. E. B. (2013). The Damage Capacity of Mahanarva spectabilis (Distant, 1909) (Hemiptera: Cercopidae) Adults on Brachiaria ruziziensis Pasture. Sci. World J. 2013, 281295. doi: 10.1155/2013/281295
Resende, T. T., Auad, A. M., and Fonseca, M. G. (2014). How many adults of Mahanarva spectabilis (Hemiptera: Cercopidae) should be used for screening Brachiaria ruziziensis (Poales: Poaceae) resistance? J. Econ. Entomol. 107, 396–402. doi: 10.1603/EC13297
Rezende, J. M., Zanardo, A. B. R., da Silva Lopes, M., Delalibera, I., and Rehner, S. A. (2015). Phylogenetic diversity of Brazilian Metarhizium associated with sugarcane agriculture. BioControl 60, 495–505. doi: 10.1007/s10526-015-9656-5
Rodríguez, J., Castro, U., Morales, A., and Peck, D. (2003). Biología del salivazo Prosapia simulans (Homoptera: Cercopidae), nueva plaga de gramíneas cultivadas en Colombia. Rev. Colomb. Entomol. 29, 149–155. Available online at: https://hdl.handle.net/10568/44246
Rodríguez, J., and Peck, D. (2007). Biología y hábitos de Mahanarva andigena (Hemiptera: Cercopidae) en condiciones de casa de malla. Rev. Colomb. Entomol. 33, 31–35. Available online at: https://hdl.handle.net/10568/44248
Rosero-Guerrero, M., Bustillo-Pardey, A. E., López-Núñez, J. C., Castro-Valderrama, U., and Gómez-López, E. D. (2012). Eficacia de entomonematodos para controlar estados de Aeneolamia varia (Hemiptera: Cercopidae) bajo condiciones de invernadero. Rev. Colomb. Entomol. 38, 266–273. doi: 10.25100/socolen.v38i2.9003
Rossato, J. A. S., Madaleno, L. L., Mutton, M. J. R., Higley, L. G., and Fernandes, O. A. (2019). Photosynthesis, yield and raw material quality of sugarcane injured by multiple pests. PeerJ. 7, e6166. doi: 10.7717/peerj.6166
Rosseto, C. J., Martins, J. F., Schmidt, N. C., and Azzini, L. E. (1978). Danos causados por cigarrinhas de pastagens (Deois flavopicta e D. Schach) em arroz. Bragantia. 37, 35–37. doi: 10.1590/S0006-87051978000100022
Sáenz, C., Salazar, D., Rodriguez, A., Alfaro, D., and Oviedo, R. (1999). “Manejo integrado del salivazo, Aeneolamia sp y Prosapia sp (Hom: Cercopidae) en las regiones cañeras de Costa Rica,” in XI Congreso Nacional Agronómico, V Congreso Nacional de Entomología. p. 155–159.
Sant, N., Iwanicki, A., Alves Pereira, A., Zanardo Botelho, A. B., Rezende, J., and Moral, R. (2019). Monitoring of the field application of Metarhizium anisopliae in Brazil revealed high molecular diversity of Metarhizium spp in insects, soil and sugarcane roots. Sci. Rep. 9, 1–12. doi: 10.1038/s41598-019-38594-8
Schöbel, C., and Carvalho, G. S. (2020). Niche modeling of economically important Mahanarva (Hemiptera, Cercopidae) Species in South and Central America: Are Brazilian spittlebug sugarcane pests potential invaders of south and Central America? J. Econ. Entomol. 113, 115–125. doi: 10.1093/jee/toz252
Silva, S. E. B., Auad, A. M., Moraes, J. C., Alvarenga, R., Claudino, S. S., and Resende, T. T. (2017). Biological performance and preference of Mahanarva spectabilis (Hemiptera: Cercopidae) for feeding on different forage plants. J. Econ. Entomol. 110, 1877–1885. doi: 10.1093/jee/tox180
Silva, S. E. B., Auad, A. M., Moraes, J. C., Alvarenga, R., Fonseca, M. G., and Marques, F. A. (2019). Olfactory response of Mahanarva spectabilis (Hemiptera: Cercopidae) to volatile organic compounds from forage grasses. Sci. Rep. 9, 1–6. doi: 10.1038/s41598-019-46693-9
Skinner, R. K., Dietrich, C. H., Walden, K. K. O., Gordon, E., Sweet, A. D., Podsiadlowski, L., et al. (2020). Phylogenomics of Auchenorrhyncha (Insecta: Hemiptera) using transcriptomes: examining controversial relationships via degeneracy coding and interrogation of gene conflict. Syst. Entomol. 45, 85–113. doi: 10.1111/syen.12381
Sotelo, G., and Cardona, C. (2001). Manejo integrado del salivazo de los pastos con énfasis en resistencia varietal. Centro Internacional de Agricultura Tropical (CIAT), Cali, CO, 140–150.
Sujii, E., Garcia, M., Fontes, E., da Silva, S., and Meyer, J. (2001). Soil temperature and diapause maintenance in eggs of the spittlebug, Deois flavopicta (Hemiptera: Cercopidae). Braz. J. Biol. 61, 605–613. doi: 10.1590/S1519-69842001000400009
Sujii, E. R., Garcia, M. A., Fontes, E. M. G., and O'Neil, R. J. (2004). Pachycondyla obscuricornis as natural enemy of the spittlebug Deois flavopicta. Pesqui. Agropecu. Bras. 39, 607–609. doi: 10.1590/S0100-204X2004000600014
Sujii, E. R., Garcia, M. A., Fontes, E. M. G., Pires, C. S., and O'Neil, R. J. (2002). Effects of meteorological variation on mortality in populations of the spittlebug Deois flavopicta (Homoptera: Cercopidae). Environ. Entomol. 31, 299–305. doi: 10.1603/0046-225X-31.2.299
Teixeira, V. M. (2010). Eficiência de Metarhizium anisopliae (Metsch) Sorok. no controlede cigarrinhas das pastagens (Homoptera: Cercopidae) em capim-marandu (Brachiaria bryzantha) em Corumbiara. Rondônia. Available online at: https://repositorio.ufscar.br/handle/ufscar/96
Thany, S., Tricoire-Leignel, H., and Lapied, B. (2010). “Identification of cholinergic synaptic transmission in the insect nervous system,” in Insect Nicotinic Acetylcholine Receptors. Advances in Experimental Medicine and Biology, ed. S. Thany (New York, USA: Springer) 1–10.
Thompson, V. (2004). Associative nitrogen fixation, C4 photosynthesis, and the evolution of spittlebugs (Hemiptera: Cercopidae) as major pests of neotropical sugarcane and forage grasses. Bull. Entomol. Res. 94, 189–200. doi: 10.1079/BER2004293
Thompson, V., and González, R. L. (2005). La identificación y distribución de los salivazos de la caña de azúcar y los pastos (Homoptera : Cercopidae) en Costa Rica. Manejo Integr. Plagas y Agroecol. 75, 43–51.
Thorne, M. S., Fukumoto, G. K., Curtiss, R. T., and Hamasaki, R. T. (2017). New Spittlebug on Pasture G. https://www.cgaps.org/wp-content/uploads/Spittle-Bug-Alert-March-2017-ver-5.-Final.pdf (accessed February 2, 2022).
Tonelli, M., Gomes, G., Silva, W. D., Magri, N. T., Vieira, D. M., Aguiar, C. L., et al. (2018). Spittlebugs produce foam as a thermoregulatory adaptation. Sci. Rep. 8, 1–6. doi: 10.1038/s41598-018-23031-z
Valério, J., Cardona, C., Peck, D., and Sotelo, G. (2001). “Spittlebugs: bioecology, host plant resistance and advances in IPM,” in Proceedings of the XIX International Grassland Congress, Piracicaba, FEALQ, (Sao Pedro, Piracicaba), 217–221.
Valério, J. R. (2013). “Manejo de Insetos-Praga,” in Forragicultura: ciência, tecnologia e gestão dos recursos forrageiros, eds Reis, R., Bernardes, T., and Siqueira, G, . (Jaboticabal: Multipress), 317–331.
Valério, J. R., and Koller, W. W. (1993). Proposição para o manejo integrado das cigarrinhas-das-pastagens. Pasturas Trop. 15, 10–16.
Valério, J. R., and Nakano, O. (1988). Danos causados pelo adulto da cigarrinha Zulia entreriana na produção equalidade de Brachiaria decumbens. Pesq. Agropec. Bras 23, 447–453.
Valério, J. R., and Oliveira, M. C. M. (2005). Parasitismo de ovos de cigarrinhas-das-pastagens (Homoptera: Cercopidae) pelo microhimenóptero Anagrus urichi Pickles (Hymenoptera: Mymaridae) na região de campo grande, MS. Neotrop. Entomol. 34, 137–138. doi: 10.1590/S1519-566X2005000100021
Valle Ramirez, S., Iparraguirre, M., Puertas, A., Rodríguez, S., Fiallos, A., Hidalgo, L., et al. (2015). Evaluación de dos métodos de monitoreo de Mahanarva andigena Jacobi en el cultivo de la caña de azúcar (Saccharum spp., h?brido) en la provincia de Pastaza, Ecuador. Rev. Prot. Veg. 30, 185–192. Available online at: http://ref.scielo.org/jq2wb5
Vasconcelos, A., Tolotti, A., Santos, R., Azevedo, T. D., Sutil, W., and Filho, W. S. A. (2018). “Novos registros de cigarrinhas (Hemiptera: Cercopidae) associadas ás pastagens no estado do Acre,” in Seminário de Iniciação Científica Da Ufac, Vol. 26 (Rio Branco: Universidade Federal do Acre). Available online at: http://www.alice.cnptia.embrapa.br/alice/handle/doc/1090074
Veríssimo, B. A., Auad, A. M., Silva, S. E. B., and da Silva, G. B. (2018). Biology and Olfactory Response of Salpingogaster nigra Schiner (Diptera: Syrphidae). Fla. Entomol. 101, 702–704. doi: 10.1653/024.101.0412
Walker, F. (1851). List of the Specimens of Homopterous Insects in the Collection of the British Museum. London: Order of the Trustees. p. 637—907.
Wiedijk, F. (1982). Variability in the occurrence of the sugar cane froghopper, Aeneolamia flavilatera (Homoptera: Cercopidae), on sugar estates in Guyana and Surinam. Mededelingen Landbouwhogeschool Wageningen. 7, 1–55.
Worthington, M. L., and Miles, J. W. (2015). “Reciprocal Full-sib Recurrent Selection and Tools for Accelerating Genetic Gain in Apomictic Brachiaria” in Molecular Breeding of Forage and Turf, eds H. Budak, and G. Spangenber (Cham: Springer International Publishing Switzerland 2015), 111–122.
Zaefarian, F., and Rezvani, M. (2016). “Soybean (Glycine max [L.] Merr.) Production Under Organic and Traditional Farming,” in Environmental Stresses in Soybean Production: Soybean Production, (London: Elsevier Inc.), 103–129.
Zhou, M., and Wang, W. (2018). Recent advances in synthetic chemical inducers of plant immunity. Front. Plant Sci. 9, 1613. doi: 10.3389/fpls.2018.01613
Keywords: Brachiaria, sugarcane, Urochloa, integrated pest management, salivazo de los pastos, cigarrinhas das pastagens
Citation: Espitia Buitrago PA, Manzano MR and Hernández LM (2022) Spittlebugs (Hemiptera: Cercopidae): Integrated Pest Management on Gramineous Crops in the Neotropical Ecozone. Front. Sustain. Food Syst. 6:891417. doi: 10.3389/fsufs.2022.891417
Received: 07 March 2022; Accepted: 21 June 2022;
Published: 19 July 2022.
Edited by:
Surendra K. Dara, Oregon State University, United StatesReviewed by:
Jhalendra Rijal, University of California, Davis, United StatesMichael Rethwisch, University of California Agriculture and Natural Resources, United States
Ramandeep Sandhi, FMC Agricultural Solutions, United States
Copyright © 2022 Espitia Buitrago, Manzano and Hernández. This is an open-access article distributed under the terms of the Creative Commons Attribution License (CC BY). The use, distribution or reproduction in other forums is permitted, provided the original author(s) and the copyright owner(s) are credited and that the original publication in this journal is cited, in accordance with accepted academic practice. No use, distribution or reproduction is permitted which does not comply with these terms.
*Correspondence: Luis M. Hernández, l.hernandez@cgiar.org