- 1Indian Council of Agricultural Research (ICAR)-National Bureau of Plant Genetic Resources, New Delhi, India
- 2Division of Plant Physiology, Indian Agricultural Research Institute, New Delhi, India
- 3Jamia Milia Islamia, New Delhi, India
The world's food demand is increasing rapidly due to fast population growth that has posed a challenge to meeting the requirements of nutritionally balanced diets. Pulses could play a major role in the human diet to combat these challenges and provide nutritional and physiological benefits. Pulses such as chickpeas, green gram, peas, horse gram, beans, lentils, black gram, etc., are rich sources of protein (190–260 g kg−1), carbohydrates (600–630 g kg−1), dietary fibers, and bioactive compounds. There are many health benefits of phytochemicals present in pulses, like flavonoids, phenolics, tannins, phytates, saponins, lectins, oxalates, phytosterols peptides, and enzyme inhibitors. Some of them have anti-inflammatory, anti-ulcerative, anti-microbial, and anti-cancer effects. Along with these, pulses are also rich in vitamins and minerals. In this review, we highlight the potential role of pulses in global food systems and diets, their nutritional value, health benefits, and prospects for biofortification of major pulses. The food composition databases with respect to pulses, effect of processing techniques, and approaches for improvement of nutritional profile of pulses are elaborated.
Introduction
Amid continually changing diet patterns, food choices, modernization in food processing technologies, perceptions, food taboos, cultural shifts, and a rise in people's awareness, there is a big challenge to supply nutritious food for an ever-increasing population (Kumar et al., 2014; Changan et al., 2017). The critical question is how to meet the demand for food that provides a healthy and nutritious diet in the coming years without threatening the resources of the earth (Langyan et al., 2022). An overview of the food system and diets across the populations is given in Figure 1. In the past 25 years, the world's population increased by two billion, and it is expected to reach up to 8.5 billion by 2030 and 9.8 billion by 2050 (United Nations World Population Division, 2017). Global efforts toward the Millennium Development Goals of the United Nations helped reduce undernourished individuals from 23.3 to 12.9% of the world population, but more than 800 million individuals were left malnourished throughout the world (UNICEF and World Health Organization, 2017). The Sustainable Development 2030 Agenda has 17 Sustainable Development Goals (SDGs). The second goal is to achieve Zero Hunger which will help end hunger and poverty by 2030. Approximately 2.3 billion people are without food or unable to regularly eat a nutritious, balanced diet. Around 720–811 million people are chronically undernourished. An additional 70–161 million people are estimated to be facing hunger during the recent pandemic. About one-third of women of childbearing age are anemic, while 22% of children younger than 5 years are stunted (UNDP, 2022). Approximately one-third of the world's total population suffers from various types of malnutrition like stunting (short-for-age), undernourishment (the main hunger indicator), micronutrient deficiencies, overweight, and obesity. The global population of middle-class families has doubled to at least 13% of the total population between 2001 and 2011, mainly in South Pacific and Asian countries (Pew, 2017). A majority of people who depend on plant-based foods often fail to meet the recommended daily allowances (RDA) for essential micronutrients. The deficiency of micronutrients may result in severe complications such as intellectual impairment, poor growth, perinatal complications, and a higher risk of mortality and morbidity, further leading to chronic and infectious diseases such as malfunction of thyroid, osteoporosis, colorectal cancer, osteomalacia, and cardiovascular diseases. The deficiency of micronutrients and the undernourishment of pregnant mothers affect about 50% of the global population. It results in increased incidences of intrauterine growth restriction, low birth weights, protein-energy malnutrition, chronic energy deficiencies, and maternal and neonatal mortality (Alae-Carew et al., 2019). A study conducted across 187 countries between 1990 and 2010 underlines a shift toward unhealthy and junk food items such as processed meat, red meat, diets rich in trans-fat, saturated fat, sodium, and free sugar, which may further increase the risk of diet-related non-communicable diseases (Imamura et al., 2015). Therefore, there is a need to diversify the global agriculture and food system to nourish people and address hunger and hidden hunger (Langyan et al., 2021a,b).
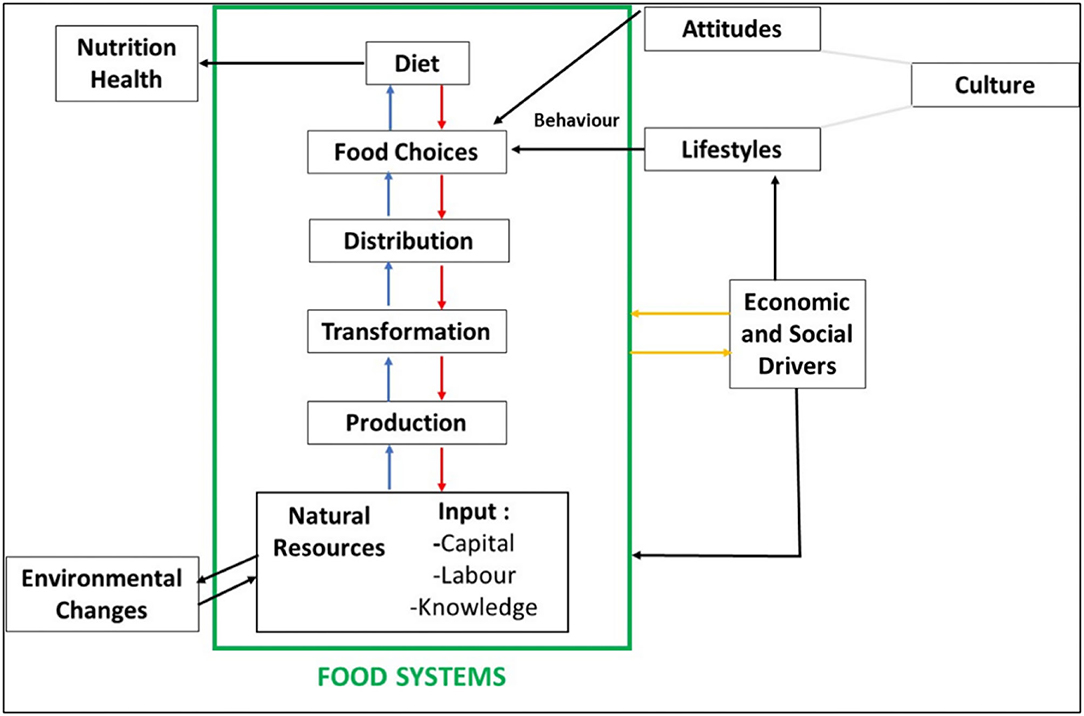
Figure 1. Food systems and diets (Source: Meybeck and Gitz, 2017).
Food production systems also have environmental costs such as deforestation, land degradation, biodiversity and habitat loss, depletion of natural resources, and contamination of soil, air, and water (IPBES, 2018). For instance, food systems emitted approximately one-fourth of anthropogenic greenhouse gas (GHG) and used about 70% of total fresh water in agricultural production (UNICEF and World Health Organization, 2017). In agriculture, pesticides, synthetic fertilizers, and different hormones in animal husbandry led to terrestrial and marine ecosystems pollution and contamination of ecosystems and food products, resulting in severe health complications (Landrigan et al., 2018). Different practices and increasing competition for water, land, and energy affect the ability for sustainable and healthy food production for future generations.
To combat the challenge of providing affordable, nutritious diets at low environmental costs, pulses can play an important role. Pulses and legumes such as soybean, chickpeas, alfalfa, lentils, peanuts, peas, clover, beans, etc., are majorly used for eating purposes as well as animal feed in many areas around the globe. Along with their environmental, nutritional, and agronomic benefits (Oliveira et al., 2019; Singh N. et al., 2021), pulses have been used as plant-based solutions for persisting problems in the food system, which occupy the attention of many researchers, food technologists, and marketers. For instance, legumes can enhance the organic matter of soil and could be used in crop rotations (Considine et al., 2017) and also enhance the production of the crop as well as profitability. For nutritionists, pulses are considered healthy and high nutrient-like protein-rich diet, that mainly decrease the risk of stroke and heart disease (Margier et al., 2018; Mandal et al., 2020). The research in environmental science shows that the quality of nitrogen fixation by most of the pulses can lessen the emissions of greenhouse gases by lowering mineral fertilizers' requirements (Lampkin et al., 2015). The increase in human population and their activities are causing an increase in greenhouse gas concentrations in the atmosphere, which in turn has caused the warming of the atmosphere and surface of the earth (Hall et al., 2017). The increase in warming has increased the frequency and intensity of adverse weather events, leading to long-term climate change. Along with temperature, rainfall, and other extreme climatic conditions, agriculture particularly the production of food may also lead to a direct impact on climate change due to its fragile connection with the environment (Cusworth et al., 2021). The changes in rainfall patterns as well as temperatures affect agricultural output and hence destroy food production systems. Similarly, both droughts and floods are expected to enhance the frequency and intensity of climatic situations that could also lead to decreased production of crops. With respect to climate change, the crop sector is one of the very exposed and sensible areas due to less capacity to adapt, and therefore, assessing the effect of climate change on the production of the crop is mostly important. In the past five decades, the agricultural production in the Global South and the erosion of indigenous and traditional food crops have greatly impacted the global food system (Akinola et al., 2020). When consumed in place of dairy products and meat, pulses have the potential to decrease pressure on resources and reduce agricultural land. More than 50% of the daily requirements of proteins around the world now come from pulses. The increase in diversity of diets linked to the impact on the environment like climate change, and indigenous foods, like local diverse pulses, helps in providing the option of strategic food that improves nutritional quality, enhances the diversity of diets, and adapts to climate change (Pitso and Lebese, 2014). Unpredictable changes in climatic conditions affect the production and security of food globally, especially in developing countries. In intercropping system, pulse crops can adapt to climate change and its effects and hence the best method to enhance climate resilience in farming (Guntukula, 2020).
Pulses are rich in vitamins and minerals and are considered “poor people's meat”. Compared to cereals, pulses such as chickpeas, green gram, peas, horse gram, beans, lentils, and black gram are a rich source of protein, complex carbohydrates, dietary fibers, minerals, folates, and bioactive compounds (Singh U.B. et al., 2021). Moreover, the availability of phytochemicals such as flavonoids, phenolics, tannins, phytates, saponins, lectins, oxalates, phytosterols, peptides, and enzyme inhibitors have anti-inflammatory, anti-ulcerative, anti-microbial, and anti-cancer effects. This review mainly focuses on nutritional value, especially micronutrients, health benefits, and approaches for biofortification of major pulses.
Pulses and Their Production
Pulses or legumes are plant foods from the Fabaceae family, including crops like peas, beans, lentils, and chickpeas. Pulses are annual leguminous crops. Common beans (Phaseolus vulgaris L.), faba beans (Vicia faba L.), chickpeas (Cicer arietinum L.), peas (Pisum sativum L.), mung beans (Vigna radiata L.), cowpeas, and lentils (Lens culinaris Medik.) are among major pulses. In 2020, pulses were grown in a 93.2 million ha area with a total production of 89.8 million t and an average yield of 963.9 kg/ha. There is a wide diversity in pulses with a number of major cultivated species around the world. For enumeration and trade-related purposes, the Food and Agriculture Organization (FAO) classifies the pulses into 11 major groups (Table 1). Each group has different species of pulses that are often grown in diverse geographies, specific agronomic requirements, and nutritional properties. India (23.4 million t), Canada (8.2 million t), China (4.8 million t), Myanmar (4 million t), and Nigeria (2.7 million t) are the top five pulse-producing countries (Faostat, 2020). In several countries, pulses contribute significantly to diet. India (15.4 kg/year per capita), Kenya (17.0 kg/year per capita), and Turkey (13.6 g/year per capita) are top consumers of pulses (Alae-Carew et al., 2019).
Nutritional Profile of Major Pulses
Pulses are an excellent source of key nutrients, including carbohydrates (e.g., fiber and starch), vegetable protein, folate, other vitamins, and minerals like potassium and iron with health-promoting benefits. Pulses are a rich source of essential micronutrients, such as iron, potassium, magnesium, zinc, and B vitamins including folate, thiamin, and niacin (Figure 2). There exists significant variation in general protein and carbohydrate content in common pulses (Table 2). Generally, the approximate nutritional values of pulses are crude protein (21–25%), carbohydrates (60–65%), moisture (10%), and lipids (1–1.5%). Daily mineral requirements can be satisfied by taking 100–200 g pulses (lentil, cowpea, and chickpea), and daily iron requirements can be met by consuming 100 g of most dietary legumes. Additionally, beans are high in several forms of vitamin B such as folic acid, pantothenate, and chickpeas, and beans are high in carotene and vitamin K (Gowda et al., 2015). Chickpeas are high in vitamins, including riboflavin, niacin, thiamin, folate, and the precursor of vitamin A, but at the same time also contain some anti-nutritional factors (Jukanti et al., 2012). Furthermore, chickpeas are high in carbohydrates and protein (Geervani, 1989). Chickpeas have several health benefits when combined with other pulses. Cowpea is a good source of protein, carbohydrate, insoluble and soluble fiber, and essential minerals such as iron, zinc, manganese, phosphorus, and potassium. Despite having a low lipid content, it has a high unsaturated fatty acid profile (Frota et al., 2008). Pigeon pea seeds also include various additional dietary elements like carbohydrates in the cotyledons, protein in the embryo, and fiber in one-third of the seed coat (Saxena et al., 2010). Anti-nutritional elements like oligosaccharides, polyphenols, and enzyme inhibitors can also be found in the pigeon pea seeds.
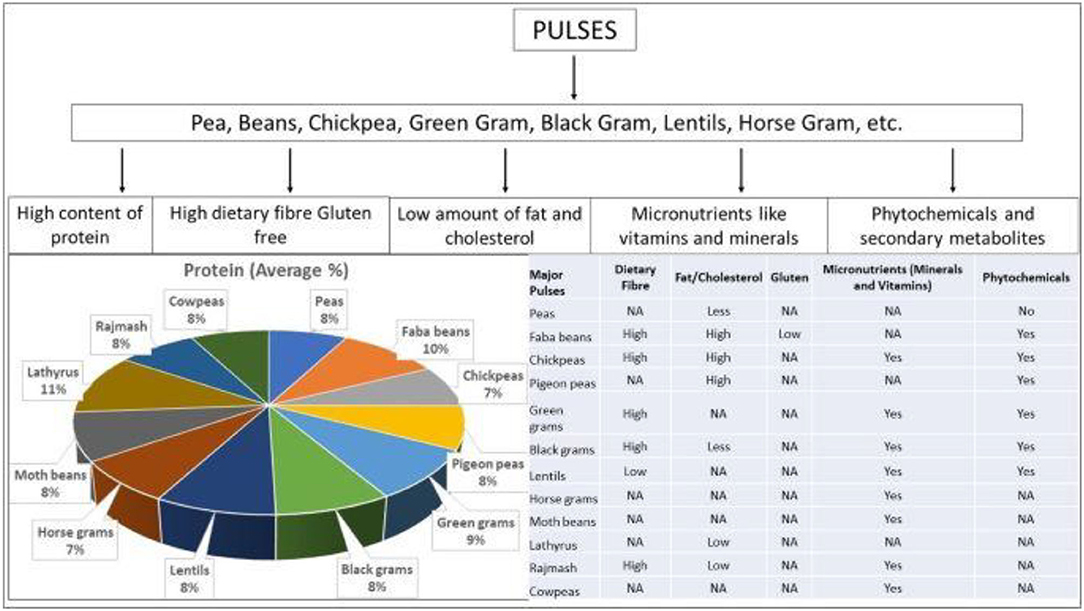
Figure 2. General nutritional profile of different pulses (Source: Venkidasamy et al., 2019). NA, not reported.
Starch and Complex Carbohydrates
Pulses are a good source of carbohydrates (Hoover and Ratnayake, 2002; Tiwari and Shivhare, 2016). These have a lower digestible starch content and a greater amylose concentration. Pulses are a good source of dietary fiber since they contain both soluble (which helps manage body weight, blood sugar levels, and cholesterol) and insoluble (which helps lower cholesterol) fiber. They also have a variety of complex carbohydrates in a large amount, accounting for up to 70% of the seeds. Available and unavailable carbohydrates are categorized based on their nutritional and physiological activities. The increased amount of unavailable carbohydrates such as dietary fibers, resistant starch, and specific oligosaccharides will decrease the pulses' glycemic percentage.
Proteins
Protein deficiency is a widely prevalent problem worldwide with negative consequences on health and the economy. Legumes are considered the primary source of proteins besides providing other essential macro and micronutrients (Khattab and Arntfield, 2009). Pulses are rich in storage proteins such as albumins, globulins, and prolamins and seeds contain several comparatively minor proteins, including protease and amylase inhibitors, lectins, lipoxygenase, and others (Duranti, 2006). The nutritional value of protein-rich foods depends on the quantity and quality of protein. Protein with low content of any essential amino acids is not fully utilized. Instead, it increases the metabolic burden on the body. Protein content varies between pulses: peas contain 20% protein, whereas protein content in lupin is 40% (Vaz Patto et al., 2015). All pulse protein present in cotyledon contains higher quantities of protein since they cover a large portion, the embryonic axis of the seed, and the seed coat. These seed proteins can be classified into structural, storage, and biologically active proteins. The protein quality varies within different pulse species in which lysine and limiting amino acids such as tryptophan, methionine, and cysteine are high. So, with the combination of pulse and cereal protein, an adequate supply of both lysine and sulfur-containing amino acids can be accomplished in human nutrition (Leinonen et al., 2019).
Isoflavones
Pulses generate isoflavones, which are natural plant secondary metabolites. There is a variety of isoflavones, including genistein, glycitein, daidzein, genistin, daidzin, protensin, biochanin A, and formononetin, which protect against diseases such as cardiovascular disease. Isoflavone quantity varies by legume variety; however, soybeans have a lot of them, and they have a lot of estrogenic activity (Oomah and Mazza, 2000). Soy isoflavones are a type of isoflavone that can be purchased commercially. However, the distribution of isoflavone content in pulses has only been explored by a few studies. Bengal gram has the highest isoflavone content of all the major pulses (Chibbar et al., 2010). Green gram and black gram have higher isoflavone content. Pulses' isoflavone content rises after germination (Suneja et al., 2011). The presence of isoflavones in legumes adds to their low cholesterolaemic properties. Soy protein and isoflavones enriched diet has been suggested for reducing blood cholesterol levels (Oomah and Mazza, 2000).
Other Phytochemicals
Pulses contain lectins, enzyme inhibitors, oxalates, oligosaccharides, phytic acids, and phenolic compounds, among other natural bioactive chemicals. Pulses are available in two forms: whole grains and split pulses. Pulses are high in minerals, vitamins, omega-3 fatty acids, carotenoids, anti-nutritional factors (ANFs), and proteins. Legumes and pulses are now considered functional foods due to their favorable nutritional characteristics (Oomah and Mazza, 2000). Plant proteins (beans) have ANFs that affect their digestion. ANFs such as phenolic compounds, trypsin, lectins, chymotrypsin, and amylase inhibitors have a detrimental impact on protein and carbohydrate digestion. In contrast, oxalic acids and phytic acids have a negative impact on mineral absorption. Some ANFs operate as allergens, over-stimulating the immune system, while others interfere with vitamin absorption (Henchion et al., 2017). Most of these thermo-sensitive compounds are eliminated by thermal treatments (cooking), while others are removed by washing. On the other hand, enzyme inhibitors and lectins reduce protein digestion and nutritional absorption. Pulses contain various small components that act as helpful nutrients and ANFs. Phytic acids are found in increasing concentrations in mature legume seeds. Furthermore, the chelating activity of phytic acid can reduce the availability of essential minerals, carbohydrates, and proteins.
Polyphenolic Compounds
The main polyphenolic chemicals in pulses include tannins, flavonoids, and phenolic acids. Phenolic chemicals are abundant in black grains such as black gram, lentils, and red kidney beans. Pulses include antioxidant compounds such as tocopherols, ascorbic acid, and isoflavonoids. Polyphenols may act as “preventive” antioxidants by chelating the catalytic transition metals such as copper and iron, rendering them unavailable for Fenton-type reactions; or they may act as “scavenger” antioxidants, providing an electron to highly reactive free radicals and quenching the radical's reactivity by delocalizing the unpaired electron on the phenolic ring. Dark, highly pigmented kinds of pulses, such as red kidney beans (Phaseolus vulgaris) and black gram (Phaseolus vulgaris), have the highest polyphenolic content (Vigna mungo). The highest phenolic, flavonoid, and condensed tannin content are found in lentils, followed by red kidney and black beans (Singh and Basu, 2012). Chickpea contains many polyphenolic chemicals, including flavonols, flavone glycosides, and oligomeric and polymeric pro-anthocyanidins. Sinapic acid was the most prevalent phenolic acid, and catechin and epicatechin were the most prevalent flavonoids out of all the phenolic compounds found. Until recently, phenolic compounds were thought to be non-nutritive. It was discovered that high levels of polyphenols, particularly tannins, can have negative repercussions by inhibiting iron absorption and blocking digestive enzymes in the gastrointestinal tract (Singh and Basu, 2012).
Food Composition Databases on Pulses
The nutritional composition data of pulses are meager in food composition tables (FCTs) and food composition databases (FCDBs). Despite many FCT/FCDB developed by different countries to a certain extent, detailed and analytical data for vitamins, minerals, and amino acids are lacking in the published literature (FAO, 2016). Moreover, food description is very generic, and it is dominated by major crops which neglect the minor legumes. These factors restricted the ultimate use of data and imprecisions of nutrient intake estimations through pulses consumption. The aim of developing some recent FCDBs was to make available a complete nutrient profile for different species as a tool to accelerate consumption and awareness of pulses for facilitating countries on agriculture projects, programs, and policies.
Pulses FAO/INFOODS User Database for Pulses
The objectives of uPulses suggest the development of a database with representative compositional data on a global, regional, or national level. However, it needs to be recognized that uPulses can only represent available data of a certain quality, rather than “truly representative” compositional states of the foods presented—especially when considering that data availability per species varied significantly for different factors affecting the nutrient composition. The lack of analytical and secondary data is the major limitation of uPulses which did not represent the existing biodiversity of pulses. Only 16 out of the 23 pulse species were considered and investigated in uPulses. The uPulses database contains a total of 16 species of pulses, for which at least one food entry is presented. In total, uPulses holds 177 food entries: 61 food entries for raw pulses and 116 food entries for cooked pulses. Pulses were selected for the data collection according to the FAO classification (1994); however, only those with sufficient data of sound quality were included in uPulses.
Pulses DM FAO/INFOODS Database for Pulses on Dry Matter Basis
The FAO/INFOODS Global Database for Pulses on Dry Matter Basis Version 1.0 (PulsesDM1.0) was developed to provide a complete nutrient profile for different species based on average values presented in the FAO/INFOODS Global Food Composition Database for Pulses version 1.0, also called uPulses (Food and Agriculture Organization of the United Nations, 2017). The PulsesDM database contains a total of 16 species of pulses, for which at least one food entry is presented. PulsesDM holds 177 food entries: 61 food entries for raw pulses and 116 food entries for cooked pulses.
Bio Food Comp
Food biodiversity, defined as the diversity of plants, animals, and other organisms used for food, covering the genetic resources within species, between species, and provided by ecosystems (FAO, 2010), is receiving increasing attention globally (Toledo and Burlingame, 2006; Burlingame et al., 2009; Stadlmayr et al., 2011). It is also recognized that data on food composition and consumption are scarce regarding biodiversity, i.e., foods reported below species level (i.e., variety/cultivar/breed level) or for wild and underutilized foods. Furthermore, these foods are rarely present in food composition tables and databases. To improve the evidence base of the importance of biodiversity to nutrition, Nutritional Indicators for Biodiversity—one on food composition (FAO, 2008) and one on food consumption (FAO, 2010)—were developed. The indicator on food composition counts the number of foods for which at least one value for a nutrient or bioactive component is available, and the food is described sufficiently to identify the genus, species, subspecies, and variety/cultivar/breed. Additionally, as there was no compositional data available on foods counting for biodiversity, FAO, in collaboration with INFOODS has developed the FAO/INFOODS Food Composition Database for Biodiversity (BioFoodComp). The current version 3.0 holds 7,953 entries, including pulses.
AnFood 2.0 FAO/INFOODS Analytical Food Composition Database
The current version of the FAO/INFOODS Analytical Food Composition Database is AnFooD2.0, which is an updated version of AnFooD1.0 and AnFooD1.1 published in 2012 and 2016, respectively. AnFooD2.0 comprises compositional data for 1,940 foods (including pulses) and 364 components collected and compiled mainly by FAO interns and consultants. AnFooD holds data on different edible parts of the same plant/animal food, different maturity stages, raw and processed food (cooked, preserved), and recipes (composite foods). Foods in AnFooD are categorized into one of the 12 food groups, and each food entry has been assigned a unique food code (= food item ID).
TAC Database
The total antioxidant capacity (TAC) database, contains sweets (7), dried fruits (5), pulses (5), nuts (6), cereal products (18), and spices (11), which was examined with the help of three assays. Additionally, it has bound antioxidant compounds that are present in food rich in fibers, such as legumes, nuts, and cereals. Also, among nuts and pulses, lentils, broad beans, and walnuts have TAC elevated levels, while peanuts, pine nuts, and chickpeas have lower antioxidant capacity. This database has been utilized for examining the dietary antioxidant function helpful in disease prevention (Venkidasamy et al., 2019).
Impacts of Processing on the Nutritional Profile
Pulses are generally processed before they are utilized for edible purposes. Hence, different processing techniques such as soaking, dehusking, germination, roasting, and cooking have an effect on nutrition, intake quality, digestibility, and health benefits. For example, the anti-nutritional factors (ANFs) in chickpeas are diminished or removed in different cooking methods. ANFs such as tannins, phytates, proteolytic enzyme inhibitors, and trypsin inhibitor activity are reduced or removed during the processing such as soaking, germination, blanching, and boiling of pulses. It also increases mineral (zinc and iron) bioavailability and protein digestibility. Dry beans contain some oligosaccharides such as stachyose and raffinose that produce flatulence. By cooking (121°C, 15 psi) for 30 min or soaking (0.5% NaHCO3 solution) for 18 h, these oligosaccharides are effectively reduced.
Similarly, soaking of Lathyrus sativus seeds in boiled water, tamarind, or alkaline solutions effectively eliminates neurotoxin β-N-Oxalyl-L-α, β-diaminopropionic Acid (βODAP). However, processing approaches' duration and time need to be standardized (FAO, 2016). Germination is one of the simple methods for enhancing pulses' palatability, digestibility, and nutritional quality. Additionally, germination also increases vitamin (A and C) content, the availability of carbohydrates and protein, and reduces the ANFs in pulses. Water absorption efficiency is also enhanced in black gram, lentil, and chickpea when thermally treated in a pressure cooker. This process declines the absorption of fat and foaming potential. The extrusion cooking approach is used to reduce raffinose (oligosaccharide) content and increase the dietary fiber content (Rachwa-Rosiak et al., 2015).
Moreover, different processing approaches have also been used for enhancing the low glycemic-resistant starch in plant-based foods. The resistant starch (RS) and slow digestible starch (SDS) content in whole pulse flours (black bean, chickpea, broad bean, and lentil) could be increased using different approaches such as annealing hydrothermal treatments and heat moisture treatment (Chávez-Murillo et al., 2018). The fractions of RS and SDS were enhanced with hydrothermal treatments and helped in the improvement of protein degradation in vitro. Therefore, the physical linkage between proteins and starch is enhanced at the molecular level by thermal treatments linked with a more significant number of resistant starch fractions (Magrini et al., 2018). Molecular interaction between starch and protein could be essential for the regulation of digestion of protein and starch. Furthermore, it helps in improving the nutraceutical and functional values.
Health Benefits of Pulses
The availability of a large number of proteins, bioactive compounds as well as dietary fibers in pulses gives various potential health benefits to humans like the prevention of many diseases. For example, chickpeas provide beneficial effects along with other cereals against type 2 diabetes (T2D), cardiovascular diseases (CVD), and many digestive problems. Also, in previous reports, it has been documented that the starch of fermented pulses provided potential anti-carcinogenic activity.
Anti-cancer Activity
Secondary metabolites derived from plants have anti-cancerous activities in different cancer types (Baskar et al., 2016). Previous reports have documented potential anti-carcinogenic activity derived from compounds present in pulses. For instance, the presence of lycopene (carotenoid) in chickpea grain helps in decreasing the risk of prostate cancer. Also, it has been found that a diet rich in carotenoids helps in reducing the risk of many cancers such as lung cancer, due to carotenoids' antioxidant activity (Mittal et al., 2009). In vitro studies reported that the presence of isoflavone biochanin A in chickpea suppresses the growth of cells in stomach cancer and also reduces the growth of tumor in mouse xenograft studies. A diet rich in saponin helps in suppressing azoxymethane-induced preneoplastic lesions in colon cancer.
Anti-diabetic Activity
Throughout the world, diabetes is considered a common metabolic disease caused by insulin deficiency. Various secondary metabolites of plants were shown to have anti-diabetic properties (Baskar et al., 2016). For example, pulses possess elevated levels of amylases and resistant starch (Pittaway et al., 2007). The presence of resistant starch decreases glucose availability by digesting in the small intestine. Dietary foods having low glycemic index (GI) help in increasing the regulation of blood glucose levels, LDL-cholesterol, and risk of thrombosis in patients with diabetes. The foods having low-end digestion rate result in low calories which help in improving bowel health as well as reducing the lipid and post-prandial serum glucose levels (Jenkins et al., 2012). Lower GI is also a critical factor that is needed to reduce the occurrence and severe causes of T2D (Osorio-Diaz et al., 2008). The sensitivity of insulin and glucose tolerance also increases with the uptake of a good amount of resistant starch, which has been involved in the protection of diabetes, cancer, and CVDs.
Protection Against Cardiovascular Disease and Obesity
Pulses are a rich source of dietary fibers, which play an important role in reducing total plasma cholesterol concentration. The fiber-rich diets have hypocholesterolemic activity because of the suppression of the synthesis of fatty acids by butyrate, acetate, and propionate (Crujeiras et al., 2007). Previous studies reported that the inhibition mechanism of propionate on fatty acid and cholesterol biosynthesis leads to suppression of utilization of acetate (acetyl-CoA). Generally, the food rich in dietary fibers helps in maintaining lower body mass index (BMI) as well as serves as an aid to attain faster satiety. Consuming foods with low GI leads to enhancing cholecystokinin (a hunger suppressant and gastrointestinal peptide) production and further enhancing satiety. Most of the pulses are rich sources of dietary fibers as well as have a low GI, which might be helpful in obesity reduction and weight loss. Dietary fiber-rich food enhances bowel health through an increasing number of defecations, softer texture of stool, and ease in defecation. Pulses contain 50–60% of polyunsaturated fatty acids like oleic acid (OA) and linoleic acid (LA). Consumption of LA leads to positive effects on hemostatic factors, the sensitivity of insulin as well as serum lipids that helps in decreasing the risk of coronary heart disease (CHD). The β-carotene (carotenoid) present in pulses has shown to be inversely correlated with CVD. With the increasing uptake of folic acid, the homocysteine concentration present in the serum responsible for CHD is reduced by 13.4–51.7% (Jukanti et al., 2012). β-Sitosterol present in pulses helps in reducing serum cholesterol, thereby decreasing the risk of CHD. Also, the presence of β-sitosterol helps in lowering blood pressure.
Protection Against Other Diseases
Different types of sterols, tocopherols, and tocotrienols are present in pulses which show various activities such as antimicrobial, anti-tumor, anti-ulcerative, and anti-inflammatory along with suppressive effects on cholesterol (Jukanti et al., 2012). Zeaxanthin and lutein present in chickpea play a major role in age-related macular degeneration and also there is an enhancement of natural killer-cell activity by carotenoids. Different growth and developmental processes such as vision, cell division/differentiation, and growth of bones are regulated by vitamin A, a derivative of β-carotene. The carotenoids in pulses also serve as potential compounds utilized in traditional medicine (tonics, stimulants, and aphrodisiacs). Also, they have been utilized for removing parasitic worms from the human body, as a thirst suppressor, appetizers as well as reducing stomach burning sensations (Jukanti et al., 2012).
Antimicrobial Potential of Pulses and Their Derived Products
The resistivity of microbe's selection against traditional antimicrobials needs the production of alternative antimicrobial compounds. For example, phytochemicals have antimicrobial activity against different types of microbes. Natural ingredients in food products help in the protection against pathogens in food (Lindgren et al., 2018; Pina-Pérez and Pérez, 2018). Polyphenols such as quinine, phenolic acids, lignans, tannins, flavonoids, and stilbenes are naturally occurring secondary metabolites of plants that function as antioxidants, anti-cancer, antimicrobial, and anti-inflammatory agents. Polyphenols cause deficiency of essential micronutrients (such as zinc and iron), cell membrane permeabilization, cytoplasmic membrane disruption, and microbial metabolism inhibition that led to the death of microorganisms. Pulses usually contain proteinase inhibitors that are considered antimicrobial components. The hull extract of pigeon peas contains a high amount of flavonoid and phenolic content that slows down high antimicrobial activity against Bacillus cereus (Daglia, 2012). Proteins isolated and purified from chickpea, soybean, and broad bean undergo esterification using methanol, and furthermore, these methylated proteins show antibacterial activity against Staphylococcus aureus, Bacillus subtilis, Pseudomonas aeruginosa, and Escherichia coli (Daglia, 2012). Lectins also show antimicrobial as well as anti-cancer activity with microbial cell wall disruption, and by interaction with components of cell wall like teichoic acids and teichuronic, lipopolysaccharides, and peptidoglycans. The aerial parts, extraction of seeds, and fruit skin of chickpeas show antifungal (Candida albicans) and antibacterial (Klebsiella pneumoniae, E. coli, and Enterococcus faecalis) activity (Al-Snafi, 2016).
Approaches for Improvement of the Nutritional Profile of Pulses Through Biofortification
Although pulses are a rich source of multiple nutrients, the nutritional profile of pulses could be improved further through biofortification, especially with respect to micronutrients. The creation of micronutrient-rich staple food crops could be a cost-effective and long-term way to boost micronutrient consumption and enhance overall human health. The process of enhancing the density of nutrients in a crop is known as biofortification. It is a practical, emerging, and cost-effective technology that employs a variety of strategies, including classical breeding, population mapping, and genetic selection, to produce improved pulse crop lines. In comparison to rice, wheat, and maize, minimal efforts have been made for the biofortification of pulses and legumes. To combat chronic diseases connected to micronutrient deficiencies around the world, biofortification of diverse pulses such as lentils, field pea, and chickpea with highly bioavailable iron, zinc, selenium, and iodine is urgently needed. The modern agricultural system focused mainly on boosting grain yield and crop productivity up until now with secondary consideration to specific issues of human health. Agriculture is now shifting toward growing nutrient-dense food crops in adequate amounts to increase food nutritional quality (Singh et al., 2015; Sellamuthu and Malathi, 2021). Because the edible sections are denser in bioavailable micronutrients, minerals, and vitamins, biofortified pulses have a significant potential to battle hidden hunger and ensure food security. As a result, biofortification will emerge as a less-expensive agricultural-based solution for meeting nutritional needs. Regular use of biofortified staple crops will result in measurable improvements in human health and nutrition. This will facilitate fighting against “hidden hunger” or “micronutrient malnutrition” and provide food security, particularly in poor and developing countries (Garg et al., 2018). There could be several approaches for biofortification and improvement of the nutritional profile of pulses (Figure 3).
Mineral Fertilizer
To boost the mineral concentration in edible tissues, agronomic solutions rely on the administration of mineral fertilizers (White and Broadley, 2009). Mineral fertilizers have been used to promote the health of plants in soil for hundreds of years, but within certain boundaries, the same method can be used to increase mineral accumulation in grains for nutritional purposes (Gomez-Galena et al., 2010). This method is used to increase the amount of Se, I, and Zn which have good mobility in the soil as well as in the plant. Foliar-fertilization strategies may be considered more sustainable and economically viable strategies for micronutrient enrichment of the grains (Cakmak, 2008; Prasad and Narayanan, 2014). Foliar fertilization is the application of fertilizers directly to the leaves. It could be successful when mineral elements are not available immediately in the soil or not readily translocated to edible tissues. Pulse crops have biofortified with micronutrients, Fe, Zn, and Se, through foliar application in various studies that resulted in increased levels of these micronutrients in the harvested grain. Márquez-Quiroz et al. reported an increased concentration of Fe (29–32%) in seeds of cowpeas (Sellamuthu and Malathi, 2021). Ali et al. reported increased Fe concentration (46%) in mungbean upon foliar application of Fe. Similarly, foliar application of Fe and Zn significantly increased the concentration of these minerals along with protein in seeds of cowpeas and chickpeas. Foliar fertilization with urea is reported to improve yield attributes, yield, and chlorophyll content of chickpea (Dhaliwal et al., 2022).
Plant Growth Promoting Microorganisms
Various plant growth-promoting (PGP) soil bacteria, including Enterobacter, Bacillus, and Pseudomonas, can be used to boost micronutrient phytoavailability. These are mostly employed as seed inoculants, and they promote plant growth by producing growth hormones, antibiotics, chitinases, and siderophores, as well as inducing systemic resistance and mineralization. Numerous studies have shown enhanced concentrations of Fe, Se, and Zn using microorganism inoculants and mycorrhizal associations in pulses (Singh U.B. et al., 2021). Furthermore, colonization of Pseudomonas sp., Brevibacterium sp., Bacillus sp., Enterobacter sp., and Acinetobacter sp. in legume roots and nodules has been found to improve nitrogen fixation, plant growth, and grain yield in legumes such as chickpeas, soybeans, and peas (Kushwaha et al., 2021). In comparison to control (uninoculated) plants, inoculation of PGP actinobacteria increased the concentration of seed minerals such as Fe (10–38%) and Zn (13–30%) in chickpea. Similarly, field inoculation with arbuscular mycorrhizal fungi increased the nutritional profile of chickpea grains by boosting Fe and Zn content, as well as yield and protein content. Khalid et al. found that combining PGP rhizobacteria with Fe compound (FeSO4) in soil raised iron concentration in chickpeas by up to 81% when compared to the control, and suggested that microorganisms may play a role in the extra uptake of Fe from the soil when Fe is supplemented.
Plant Breeding
Plant breeding can raise nutritional levels in pulses to the levels needed to improve human nutrition while preserving yield and farmer-favored agronomic features (Dhaliwal et al., 2022). Screening germplasm for accessible genetic diversity, pre-breeding parental genotypes, generating and evaluating micronutrient dense germplasm, conducting genetic studies, and designing molecular markers to reduce costs and speed up the breeding process are all part of the crop development process. Traditional plant breeding methods can help not just big populations, but also people who live in rural areas with limited access to commercially available fortified foods. This method necessitates a one-time investment in plant breeding, but it may be produced and propagated by farmers at essentially no marginal cost over time. Recurring costs are modest, and germplasm can be obtained from anywhere in the world (Shahzad et al., 2021).
Genetic Engineering
In pulse crops, genetic engineering methods could be used to improve various nutritional profiles. When desired trait variation is not naturally available in germplasm, a certain micronutrient is not normally present in crops, and/or alterations cannot be obtained by conventional breeding, biofortification through genetic engineering offers an alternate strategy (Jha and Warkentin, 2020). The recent availability of fully sequenced genomes in a variety of crops bolstered this strategy. Along with boosting the concentration of micronutrients, this strategy can also be used to target the removal of anti-nutrients or the addition of promoters to improve micronutrient bioavailability. Genes involved in diverse metabolic processes in plants, as well as bacteria and other species, could be deployed. Transgenic crop development takes a significant initial investment, but it could be a long-term strategy with the potential to reach vast populations, particularly in underdeveloped nations (Dhaliwal et al., 2022). In pulse crops, the genetic engineering method has been used to improve other nutritional profiles. For example, expression of methionine-rich storage albumin from the Brazil nut increased the concentration of the essential amino acid methionine in transgenic common bean plants by up to 23%, and expression of a sunflower seed albumin gene increased the concentration of methionine in transgenic lupins by up to 94%.
Biofortification of Specific Nutrients in Pulse Crops and Their Status
Iron
Iron (Fe), one of the essential micronutrients for living organisms, plays a major role in various metabolic processes such as deoxyribose nucleic acid (DNA) synthesis and electron transport in the body. Fe is vital for synthesizing oxygen transport proteins (like myoglobin and hemoglobin), and enzymes participate in oxidation/reduction and electron transfer (Abbaspour et al., 2014). In hemoglobin, it acts as an oxygen transporter from the lungs to different body tissues. According to the Food and Nutrition Board of the Institute of Medicine, National Academy of Sciences, the Fe RDA is 18 mg/day (women) and 8 mg/day (men) (https://ods.od.nih.gov/Health_Information/Dietary_Reference_Intakes.aspx). Iron deficiency is considered the most dominant among all deficiencies of micronutrients and majorly leads to anemia, affecting about two billion individuals throughout the world (Benoist et al., 2008). The number of complications such as dizziness, loss of energy, and poor pregnancy results like premature births, babies with lower weight at the time of delivery, delay in development and growth of infants, and poor cognitive skills can be caused by deficiency of iron (Bailey et al., 2015).
In many studies, large variation in iron concentration has been observed in common beans, peas, lentils, chickpeas, and mung beans (Blair et al., 2009, 2011). It has been observed that cultivars of peas (17), lentils (18), chickpeas (8), and common beans (10), grown in many places have an iron concentration of 47.7–58.1, 75.6–100, 48.6–55.6, and 57.7–80.7 mg kg−1, respectively (Ray et al., 2014). According to RDA, the serving 100 g of any of these pulses can provide about 50% iron. Diapari et al. observed various accessions of chickpea having a high concentration of iron (52–60 mg kg−1), which has been used to develop cultivars with more amount of iron concentration (Diapari et al., 2014). In mung bean lines that were commonly found in South Asian regions, there was a significant variation in the concentration of iron (35–87 mg kg−1). Recently, Dissanayaka et al. found significant variation due to environmental conditions in 177 accessions of pea using a genome-wide association study (GWAS) at Rosthern Saskatchewan and Saskatoon (Canada) and Fargo, North Dakota (USA) (Dissanayaka et al., 2020). They also reported an effect of location and genotypes having higher iron concentration at Rosthern in comparison to Saskatoon in peas. The genotype effect was also significant in chickpeas and lentils (Vandemark et al., 2018). The concentration of iron was also showing significant variations by location and variety. For instance, DellaValle et al. (2013) in lentils and Ariza-Nieto et al. (2007) in common beans, found that there was variation in iron concentration in the same place, while Moraghan et al. (2002) found higher iron concentration in seeds grown in acidic soil in comparison to calcareous soil. Diapari et al. (2014) found that genetic factors led to iron concentration variations in peas grown at different places. A number of SNP markers are linked with differences in the iron concentration found in lentils, chickpeas, and peas that are utilized in marker-assisted selection. For example, Diapari et al. (2014, 2015) found a linkage of nine SNPs with an iron concentration in 94 accessions of peas, whereas two SNPs on chromosome 4 and one each on chromosomes 1 and 6 were found in chickpeas.
Zinc
Zinc (Zn) is another important nutrient essential for humans, which is required for different biological functions. It is involved in functions like wound healing improvement, growth and proliferation of cells in membrane signaling systems (MacDonald, 2000), protection from oxidative damage by reactive oxygen species (Prasad et al., 2004), and preventing pancreatic and prostate cancer (Costello and Franklin, 2017). The RDA for zinc is 8 mg/day for women and 11 mg/day for men (https://ods.od.nih.gov/Health_Information/Dietary_Reference_Intakes.aspx). Zinc deficiency may lead to various consequences such as reoccurrence of infections, immune system weakening, mental illness, and retardation of fertility and growth. It also plays a key role in the division of cells and thus significantly affecting women during pregnancy.
Like iron, the concentration of zinc also shows a wide range of variations in chickpeas, lentils, common beans, and peas. It has been observed that significant variation in the concentration of zinc was found in 94 different accessions of chickpea grown in Saskatchewan, Canada (Diapari et al., 2014). In this study, three accessions of kabuli type such as ILC 2555, FLIP85-1C, and CDC Verano (43–48 mg kg−1) and two accessions of desi type FLIP84-48C and FLIP97-677C (41 and 42 mg kg−1) have been identified having the highest concentration of zinc. It has also been observed that there were significant variations in the concentration of zinc in chickpeas (21.1–28.3 mg kg−1), common beans (24.8–33.3 mg kg−1), lentils (36.7–50.6 mg kg−1), and peas (27.4–34 mg kg−1) grown at different locations in Saskatchewan Significant variation for zinc concentration has been found and reported in common beans core collection (>2,400), three populations of common bean, accessions of lentil (>1,600), 20 lines of mung bean, a panel of 94 and 177 pea accessions in two studies and two pea populations, PR-07 and PR-02. A number of SNP markers and QTLs have been identified for the concentration of zinc in chickpeas, peas, lentils, and common beans. For instance, in three populations of common beans, authors identified QTLs for zinc concentration (Blair et al., 2011). Also, in chickpeas, five SNPs were identified for the concentration of zinc, which were found on chromosome numbers 1, 4, and 7 (Diapari et al., 2014). Khazaei et al. (2017) identified 12 SNPs in lentils for zinc concentration in 138 accessions grown at two different places in Saskatchewan, Canada.
Selenium
Selenium (Se) is an important micronutrient majorly involved in the development and protection of the human body from oxidative stress, infections, and cancer progression (Zeng and Combs, 2008). The RDA for selenium is common for both men and women (i.e., 55 μg/day) (https://ods.od.nih.gov/Health_Information/Dietary_Reference_Intakes.aspx). Se deficiency is linked with several diseases such as Keshin-Beck, Keshan, and myxedematous cretinism in humans (Coppinger and Diamond, 2001).
During the harvesting of pulses, the weather conditions and soil quality (like water availability, texture, pH, and aeration) play major roles in the concentration of selenium. Diapari et al. (2015) reported that selenium concentration variations were primarily due to the environment, and less due to the genotype in peas. As compared to Rosthern, the concentration of selenium was more at location Saskatoon, and the difference of Se concentration has not been significant among 94 genotypes. Lentils produced in the Brown and Dark Brown soil of Western Canada have more selenium concentration (425–672 μg kg−1), whereas the selenium concentration is lower in Nepal (180 μg kg−1), Southern Australia (148 μg kg−1), Turkey (47 μg kg−1), Morocco (28 μg kg−1), Northwestern USA (26 μg kg−1), and Syria (22 μg kg−1) compared to soil of Western Canada. In different places of Saskatchewan, large variations in the concentration of selenium were found in lentils (990–1,637 μg kg−1), common beans (381–500 μg kg−1), chickpeas (629–864 μg kg−1), and peas (405–554 μg kg−1). According to RDA, 100 g dry weight of pulses provide 100% of the RDA. Nair et al. reported significant variation in the concentration of selenium, i.e., 210–910 μg kg−1 in lines of mung bean produced in two environments in Hyderabad, India. Also, the evaluation of breeding lines of 80 pea plants obtained from the Czech Republic, United States, Serbia, and Australia had low selenium concentration, whereas when grown in Saskatoon, these peas breading lines have higher selenium concentration (Nair et al., 2015).
Iodine
Iodine (I) is considered an essential constituent of hormones regulating the thyroid, triiodothyronine (T3), and thyroxine (T4), which plays a key role in normal metabolism, development, and growth. RDI for iodine is also common for men and women (i.e., 150 μg/day) (https://ods.od.nih.gov/Health_Information/Dietary_Reference_Intakes.aspx). Iodine deficiency causes goiter, hypothyroidism, developmental delay, cretinism, reduced fertility, and increased infant mortality and prenatal death (Eastman and Zimmermann, 2018). At the time of pregnancy, iodine deficiency led to the offspring's cognitive impairment, which is critical for brain development (Silva de Morais et al., 2020). In human populations, iodine deficiency varied from other micronutrients due to its predominance in developing and developed countries. The reason is the lower concentration of iodine found in agricultural soils and cereal-based crops (Gonzali et al., 2017).
A number of studies have reported different approaches such as foliar fertilization and salt application in irrigation water and soil to biofortified crops using iodine, but there is less information available. The consumption of pulses having lower iodine concentration leads to a deficiency of iodine in human beings (Cakmak et al., 2017). A large number of studies have been focused on the biofortification of vegetables compared to pulse grains due to the easy translocation of most of the iodine to xylem in leafy vegetables (Medrano-Macías et al., 2016).
Carotenoids
Carotenoids, the natural pigments produced by plants and mainly found in foods derived from plants, are not directly synthesized by humans and animals. These act as essential antioxidants in the human body and play a major role in different physiological activities. A large number of carotenoids have been discovered and reported. For example, zeaxanthin and lutein help prevent age-related macular degeneration (Fraser and Bramley, 2004). Also, lutein helps reduce the risk of cataracts and prevent cardiovascular diseases (Alves-Rodrigues and Shao, 2004). Similarly, vitamin A plays a crucial role in normal vision, cell division, and the growth of bones in humans. β-Cryptoxanthin helps stimulate osteoblastic bone formation and inhibits osteoclastic bone resorption, thereby playing a significant role in building bones. Carotenoids also have the properties of fighting against cancer, and cellular organelles are protected from oxidative damage by quenching of reactive oxygen species generated during different metabolic processes. Carotenoids are also considered promoters of iron absorption as these help in the improvement of iron bioavailability in the human body from plant-based foods (Yamaguchi and Uchiyama, 2004).
A large number of studies have reported on the content of carotenoids in pulses (Ashok Kumar et al., 2014, 2015). β-Cryptoxanthin, zeaxanthin, and lutein are found in chickpeas, while lutein, β-carotene, and violaxanthin are found in field peas. One study identified a high concentration of xanthophyll (9–20 mg/100 g), canthoxanthine (21–68 mg/100 g), and beta-carotene (166–431 μg/100 g) in 10 genotypes of chickpea produced in Minot, North Dakota (Thavarajah, 2012). (Ashok Kumar et al., 2014, 2015) evaluated carotenoids content in different cultivars (eight chickpeas and 12 peas) produced in different places in Saskatchewan, Canada, with the help of high-performance liquid chromatography utilizing a diode array detector. It has been found that carotenoids concentration was higher in cotyledons, followed by embryonal axis and pericarp (seed coat), where lutein was majorly found (11.45 μg g−1), followed by violaxanthin (0.52 μg g−1), β-carotene (0.47 μg g−1), and zeaxanthin (0.16 μg g−1). In kabuli type cultivars of chickpea, the concentration of carotenoid was mostly found in cotyledons, followed by embryonal axis and pericarp (seed coat), while in desi type cultivars of chickpea, the highest carotenoid concentration was in seed coat followed by cotyledon and then embryonal axis. Similarly, Ashok Kumar et al. found differences in carotenoid concentration in different cultivars of chickpea (121) and pea (94) grown at different places in Saskatchewan, Canada. Among all carotenoids found, lutein was majorly present in peas and chickpeas (Ashok Kumar et al., 2014, 2015). Furthermore, lutein showed positive correlations with the concentration of zeaxanthin in chickpeas and the concentration of chlorophyll in peas.
Folates
Folates (B9 vitamins) are considered cofactors in many metabolic activities like biosynthesis and metabolism of nucleotides and amino acids in the human body and hence needed for the growth and development of the human body (Scott et al., 2000). In plants, folates are essential in the biosyntheses of alkaloids, chlorophyll, and lignin. Therefore, to fulfill the requirement of folates, humans directly or indirectly depend on plant and/or animal-based food. Folate's deficiency leads to a greater risk of many chronic diseases such as neural tube defects, Alzheimer's disease, impaired cognitive function, cardiovascular diseases, and various types of cancers. Diets rich in folates are highly recommended to women during pregnancy to decrease the risk of neural tube defects in infants. Less intake of folate during pregnancy enhances the risk of pre-term delivery and retarding the growth of the fetus inside the woman's uterus. Hence, folates are essential for the reproductive health of humans.
A large number of approaches such as liquid chromatography (LC) coupled with fluorescence detection (FD) (Sen Gupta et al., 2013), microbiological assays (Fajardo et al., 2017), and mass spectrometry (MS) detection (Jha et al., 2020) have been utilized for quantification of folates from difference pulses. In previous studies, researchers found large variations in pulse folates. For instance, Han and Tyler (2003) used a microbiological assay and identified folates in green cotyledon peas (24.9–64.8 μg/100 g) and yellow cotyledon peas (23.7–55.6 μg/100 g) grown at different places in Saskatchewan, Canada. One study reported the concentration of folate in vegetable peas (59 μg/100 g) consumed in Finland with the help of LC-MS (Vahteristo et al., 1997). Similarly, Rychlik et al. (2007) identified folate concentration variability in different pulses like peas (10–20 μg/100 g), chickpeas (275 μg/100 g), green lentils (110–154 μg/100 g), and white beans (106–164 μg/100 g) by utilizing LC-MS approach. With the help of ultra-performance LC (UPLC), researchers quantified six folate mono-glutamates in four pulses such as chickpeas (351–589 μg/100 g), lentils (136–182 μg/100 g), common beans (165–232 μg/100 g), and pea (23–30 μg/100 g). 5-Methyltetrahydrofolate (5-MTHF), tetrahydrofolate (THF), and 5-formyltetrahydrofolate (5-FTHF) were mostly found in chickpeas, lentils, peas, and common beans (Fernandez-Orozco et al., 2013).
Conclusion and Future Outlook
The rapid growth of the world's population and increasing food demands create challenges in supplying nutrition-rich diets. Food sustainability concerns the production of foods to meet the dietary requirements and future availability, which is one of the sustainable development goals (SDGs). Pulses are considered the dominant and plant-based primary protein sources in human diets that combat these challenges and provide nutritional and physiological benefits. Additionally, pulses play an important role in issues related to the improvement of malnutrition of protein in different underdeveloped and developing countries. Pulses such as chickpeas, green gram, peas, horse gram, beans, lentils, and black gram are the richest protein sources, carbohydrates, dietary fibers, and bioactive compounds. There are many health benefits due to the availability of phytochemicals such as flavonoids, phenolics, tannins, phytates, saponins, lectins, oxalates, phytosterols peptides, and enzyme inhibitors that have anti-inflammatory, anti-ulcerative, anti-microbial, and anti-cancer effects. Pulses are very essential dietary food for managing weight and obesity, help in stabilizing the sugar level of blood, and enhance the level of quality insulin by decreasing spikes and improving insulin resistance.
There are significant research gaps with respect to pulses in all aspects, including genetics to nutritional research. As pulses are mainly grown and consumed in the developing world, there is a need to intensify research efforts on pulses in these countries with global collaborations. One of the glaring aspects is the lack of analytical compositional data with respect to pulse-based foods. This hampers the overall assessment of pulses in the nutritional matrix and designs programs and goals tailored around the incorporation of pulses in the diet. Regular publication of updated versions of food composition databases with diverse pulses is urgently needed. There is a need for coverage of all food groups and inclusion of more data per food group, especially on micronutrients. Sharing of pulses-specific data by researchers and closer collaboration with scientific journals shall help to make FAO/INFOODS Analytical Food Composition Database a vibrant and dynamic database.
The genetic yield potential of pulses is lesser as compared to cereals, making pulse crops less remunerative for farmers and less affordable to consumers. The green revolution was a cereal-based revolution, with minimal impact on the pulses sector. Therefore, there is a need to break the yield barrier in pulses. The green revolution in cereals was based on knowledge of plant developmental biology mutants for plant height traits. Developmental biology research with various pulses as model systems is extremely sparse. Further, photosynthesis research, canopy enhancement for solar radiation capture, source-sink relationship research, etc., is required in greater intensity to address the fundamental problem of low genetic yield potential. Given the diversity of pulse species, there is a need to develop efficient working protocols for pulse transformation, in order to harness the possibility of new breeding techniques, such as genome editing. Greater pulse production and consumption is the need of the hour. This shall provide affordable nutrition to the masses and also make the environment more sustainable.
Author Contributions
SL conceived the idea. SL, FK, RagB, and KT wrote the manuscript. PY edited the whole manuscript, added sections, and improved it to the final level. VB helped in the final formatting. RakB, RG, and AK edited the manuscript. All authors contributed to the article and approved the submitted version.
Conflict of Interest
The authors declare that the research was conducted in the absence of any commercial or financial relationships that could be construed as a potential conflict of interest.
Publisher's Note
All claims expressed in this article are solely those of the authors and do not necessarily represent those of their affiliated organizations, or those of the publisher, the editors and the reviewers. Any product that may be evaluated in this article, or claim that may be made by its manufacturer, is not guaranteed or endorsed by the publisher.
Acknowledgments
The authors are thankful to the Director, ICAR-NBPGR for all the support.
References
Abbaspour, N., Hurrell, R., and Kelishadi, R. (2014). Review on iron and its importance for human health. J. Res. Med. Sci. 19, 164–174.
Akinola, R., Pereira, L. M., Mabhaudhi, T., de Bruin, F. M., and Rusch, L. (2020). A review of indigenous food crops in Africa and the implications for more sustainable and healthy food systems. Sustainability 12, 3493. doi: 10.3390/su12083493
Alae-Carew, C., Bird, F. A., Choudhury, S., Harris, F., Aleksandrowicz, L., Milner, J., et al. (2019). Future diets in India: a systematic review of food consumption projection studies. Global Food Sec. 23, 182–190. doi: 10.1016/j.gfs.2019.05.006
Al-Snafi, A. E. (2016). Antimicrobial effects of medicinal plants (part 3): plant based review. IOSR J. Pharm. 6, 67–92.
Alves-Rodrigues, A., and Shao, A. (2004). The science behind lutein. Toxicol. Lett. 150, 57–83. doi: 10.1016/j.toxlet.2003.10.031
Ariza-Nieto, M., Blair, M. W., Welch, R. M., and Glahn, R. P. (2007). Screening of iron bioavailability patterns in eight bean (Phaseolus vulgaris L.) genotypes using the Caco-2 cell in vitro model. J. Agric. Food Chem. 55, 7950–7956. doi: 10.1021/jf070023y
Ashok Kumar, K., Diapari, M., Jha, A. B., Tar'an, B., Arganosa, G., and Warkentin, T. D. (2015). Genetic diversity of nutritionally important carotenoids in 94 pea and 121 chickpea accessions. J. Food Comp. Anal. 43, 49–60. doi: 10.1016/j.jfca.2015.04.014
Ashok Kumar, K., Tar'an, B., Diapari, M., Arganosa, G., and Warkentin, T. D. (2014). Effect of cultivar and environment on carotenoid profile of pea and chickpea. Crop Sci. 54, 2225–2235. doi: 10.2135/cropsci2013.12.0827
Bailey, R. L., West, K. P. Jr, and Black, R. E. (2015). The epidemiology of global micronutrient deficiencies. Ann. Nutr. Metab. 66(Suppl. 2), 22–33. doi: 10.1159/000371618
Baskar, V., Park, S. W., and Nile, S. H. (2016). An update on potential perspectives of glucosinolates on protection against microbial pathogens and endocrine dysfunctions in humans. Crit. Rev. Food Sci. Nutr. 56, 2231–2249. doi: 10.1080/10408398.2014.910748
Benoist, B., Mc Lean, E., and Cogswell, M. (2008). Worldwide Prevalence of Anaemia 1993-2005: WHO Global Database on Anaemia. Geneva: World Health Organization.
Blair, M. W., Astudillo, C., Grusak, M. A., Graham, R., and Beebe, S. E. (2009). Inheritance of seed iron and zinc concentrations in common bean (Phaseolus vulgaris L.). Mol. Breed. 23, 197–207. doi: 10.1007/s11032-008-9225-z
Blair, M. W., Astudillo, C., Rengifo, J., Beebe, S. E., and Graham, R. (2011). QTL for seed iron and zinc concentrations in a recombinant inbred line population of Andean common beans (Phaseolus vulgaris L). Theor. Appl. Genet. 122, 511–523. doi: 10.1007/s00122-010-1465-8
Burlingame, B., Charrondière, U. R., and Mouille, B. (2009) Food composition is fundamental to the cross-cutting initiative on biodiversity for food and nutrition. J. Food Comp. Anal. 22, 361–365. doi: 10.1016/j.jfca.2009.05.003
Cakmak, I. (2008). Enrichment of cereal grains with zinc: agronomic or genetic biofortification? Plant Soil 302, 1–17. doi: 10.1007/s11104-007-9466-3
Cakmak, I., Prom-U-Thai, C., Guilherme, L. R. G., Rashid, A., Hora, K. H., Yazici, A., et al. (2017). Iodine biofortification of wheat, rice and maize through fertilizer strategy. Plant Soil 418, 319–335. doi: 10.1007/s11104-017-3295-9
Changan, S., Chaudhary, D. P., Kumar, S., Kumar, B., Kaul, J., Guleria, S., et al. (2017). Biochemical characterization of elite maize (Zea mays) germplasm for carotenoids composition. Ind. J. Agric. Sci. 87, 46–50.
Chávez-Murillo, C. E., Veyna-Torres, J. I., Cavazos-Tamez, L. M., de la Rosa-Millán, J., and Serna-Saldívar, S. O. (2018). Physicochemical characteristics, ATR-FTIR molecular interactions and in vitro starch and protein digestion of thermally-treated whole pulse flours. Food Res. Int. 105, 371–383. doi: 10.1016/j.foodres.2017.11.029
Chibbar, R. N., Ambigaipalan, P., and Hoover, R. (2010). Molecular diversity in pulse seed starch and complex carbohydrates and its role in human nutrition and health. Cereal Chem. 87, 342–352. doi: 10.1094/CCHEM-87-4-0342
Considine, M. J., Siddique, K. H., and Foyer, C. H. (2017). Nature's pulse power: legumes, food security and climate change. J. Exp. Bot. 68, 1815–1818. doi: 10.1093/jxb/erx099
Coppinger, R. J., and Diamond, A. M. (2001). “Selenium deficiency and human disease,” in Selenium, eds D. L. Hatfield, U. Schweizer, P. A. Tsuji and V. N. Gladyshev (Boston, MA: Springer), 219–233.
Costello, L. C., and Franklin, R. B. (2017). Decreased zinc in the development and progression of malignancy: an important common relationship and potential for prevention and treatment of carcinomas. Exp. Opin. Ther. Targets 21, 51–66. doi: 10.1080/14728222.2017.1265506
Crujeiras, A. B., Parra, D., Abete, I., and Martínez, J. A. (2007). A hypocaloric diet enriched in legumes specifically mitigates lipid peroxidation in obese subjects. Free Radic. Res. 41, 498–506. doi: 10.1080/10715760601131935
Cusworth, G., Garnett, T., and Lorimer, J. (2021). Legume dreams: the contested futures of sustainable plant-based food systems in Europe. Global Environ. Change 69, 102321. doi: 10.1016/j.gloenvcha.2021.102321
Daglia, M. (2012). Polyphenols as antimicrobial agents. Curr. Opin. Biotechnol. 23, 174–181. doi: 10.1016/j.copbio.2011.08.007
DellaValle, D. M., Vandenberg, A., and Glahn, R. P. (2013). Seed coat removal improves iron bioavailability in cooked lentils: studies using an in vitro digestion/Caco-2 cell culture model. J. Agric. Food Chem. 61, 8084–8089. doi: 10.1021/jf4022916
Dhaliwal, S. S., Sharma, V., Shukla, A. K., Verma, V., Kaur, M., Shivay, Y. S., et al. (2022). Biofortification—A frontier novel approach to enrich micronutrients in field crops to encounter the nutritional security. Molecules 27, 1340. doi: 10.3390/molecules27041340
Dhull, S. B., Kidwai, M. K., Noor, R., Chawla, P., and Rose, P. K. (2021). A review of nutritional profile and processing of faba bean (Vicia faba L.). Legume Sci. 129, 1–13. doi: 10.1002/leg3.129
Diapari, M., Sindhu, A., Bett, K., Deokar, A., Warkentin, T. D., and Tar'an, B. (2014). Genetic diversity and association mapping of iron and zinc concentrations in chickpea (Cicer arietinum L.). Genome 57, 459–468. doi: 10.1139/gen-2014-0108
Diapari, M., Sindhu, A., Warkentin, T. D., Bett, K., and Tar'an, B. (2015). Population structure and marker-trait association studies of iron, zinc and selenium concentrations in seed of field pea (Pisum sativum L.). Mol. Breed. 35, 1–14. doi: 10.1007/s11032-015-0252-2
Dissanayaka, D. N., Gali, K. K., Jha, A. B., Lachagari, V. R., and Warkentin, T. D. (2020). Genome-wide association study to identify single nucleotide polymorphisms associated with Fe, Zn, and Se concentration in field pea. Crop Sci. 60, 2070–2084. doi: 10.1002/csc2.20161
Eastman, C. J., and Zimmermann, M. B. (2018). The Iodine Deficiency Disorders. South Dartmouth, MA: MDText.com, Inc.
Fajardo, V., Alonso-Aperte, E., and Varela-Moreiras, G. (2017). Total folate content in ready-to-eat vegetable meals from the Spanish market. J. Food Compos. Anal. 64, 223–231. doi: 10.1016/j.jfca.2017.10.002
FAO (2008). Expert Consultation on Nutrition Indicators for Biodiversity – 1. Food Composition. Rome: FAO. Available online at: http://www.fao.org/infoods/infoods/foodbiodiversity (accessed December 2021).
FAO (2010). Expert Consultation on Nutrition Indicators for Biodiversity – 2. Food Consumption. Rome: FAO. Available online at: http://www.fao.org/infoods/infoods/food-biodiversity (accessed February 15, 2022).
FAO (2016). FAO/INFOODS Food Composition Database for Biodiversity Version 3.0 – BioFoodComp3.0. Rome: FAO.
Faostat. (2020). Available online at: https://www.fao.org/faostat/en/#home (accessed February 15, 2022).
Faris, M. E., Takruri, H. R., and Issa, A. Y. (2013). Role of lentils (Lens culinaris L.) in human health and nutrition: a review. Med. J. Nutr. Metabol. 6, 3–16. doi: 10.1007/s12349-012-0109-8
Fernandez-Orozco, R., Gallardo-Guerrero, L., and Hornero-Méndez, D. (2013). Carotenoid profiling in tubers of different potato (Solanum sp) cultivars: accumulation of carotenoids mediated by xanthophyll esterification. Food Chem. 141, 2864–2872. doi: 10.1016/j.foodchem.2013.05.016
Food and Agriculture Organization of the United Nations. (2017). FAO/INFOODS Global Database for Pulses. version 1.0 (uPulses1.0). Rome: FAO. Available online at: http://www.fao.org/infoods/infoods/tables-and-databases/faoinfoods-databases/en (accessed February 15, 2022).
Fraser, P. D., and Bramley, P. M. (2004). The biosynthesis and nutritional uses of carotenoids. Prog. Lipid Res. 43, 228–265. doi: 10.1016/j.plipres.2003.10.002
Frota, K. M. G., Soares, R. A. M., and Areas, J. A. G. (2008). Chemical composition of cowpea [Vigna unguiculata (L.) Walp.], RS-Milênio cultivar. Ciênc. Tecnol. Aliment. 28, 470–476. doi: 10.1590/S0101-20612008000200031
Garg, M., Sharma, N., Sharma, S., Kapoor, P., Kumar, A., Chunduri, V., and Arora, P (2018). Biofortified crops generated by breeding, agronomy, and transgenic approaches are improving lives of millions of people around the world. Front. Nutr. 5, 12. doi: 10.3389/fnut.2018.00012
Geervani, P. (1989). Utilization of chickpea in India and scope for novel and alternative uses. Uses Trop. Grain Legum. 27, 47.
Gomez-Galena, S., Rojas, E., Sudhakar, D., Zhu, C., Pelacho, A.M., Capel, T., and Christou, P. (2010). Critical evaluation of strategies for mineral fortification of staple food crops. Transgen. Res. 19:165–180. doi: 10.1007/s11248-009-9311-y
Gonzali, S., Kiferle, C., and Perata, P. (2017). Iodine biofortification of crops: agronomic biofortification, metabolic engineering and iodine bioavailability. Curr. Opin. Biotechnol. 44, 16–26. doi: 10.1016/j.copbio.2016.10.004
Gowda, C. L., Chaturvedi, S. K., Gaur, P. M., Sameer Kumar, C. V., and Jukanti, A. K. (2015). “Pulses research and development strategies for India,” in Pulses Handbook (2015), 17–33. Available online at: www.commodityindia.com (accessed February 15, 2022).
Guntukula, R. (2020). Assessing the impact of climate change on Indian agriculture: evidence from major crop yields. J. Public Aff. 20, e2040. doi: 10.1002/pa.2040
Hall, C., Hillen, C., and Garden Robinson, J. (2017). Composition, nutritional value, and health benefits of pulses. Cereal Chem. 94, 11–31. doi: 10.1094/CCHEM-03-16-0069-FI
Han, J. Y., and Tyler, R. T. (2003). Determination of folate concentrations in pulses by a microbiological method employing trienzyme extraction. J. Agric. Food Chem. 51, 5315–5318. doi: 10.1021/jf0211027
Henchion, M., Hayes, M., Mullen, A. M., Fenelon, M., and Tiwari, B. (2017). Future protein supply and demand: strategies and factors influencing a sustainable equilibrium. Foods 6, 53. doi: 10.3390/foods6070053
Hoover, R., and Ratnayake, W. S. (2002). Starch characteristics of black bean, chick pea, lentil, navy bean and pinto bean cultivars grown in Canada. Food Chem. 78, 489–498. doi: 10.1016/S0308-8146(02)00163-2
Huang, Y. F., Gao, X. L., Nan, Z. B., and Zhang, Z. X. (2017). Potential value of the common vetch (Vicia sativa L.) as an animal feedstuff: a review. J. Anim. Physiol. Anim. Nutr. 101, 807–823. doi: 10.1111/jpn.12617
Imamura, F., Micha, R., Khatibzadeh, S., Fahimi, S., Shi, P., Powles, J., et al. (2015). Dietary quality among men and women in 187 countries in 1990 and 2010: a systematic assessment. Lancet Global Health 3, e132–e142. doi: 10.1016/S2214-109X(14)70381-X
IPBES (2018). “The IPBES assessment report on land degradation and restoration,” in Secretariat of the Intergovernmental Science-Policy Platform on Biodiversity and Ecosystem Services, eds L. Montanarella, R. Scholes and A. Brainich (Bonn), 744. doi: 10.5281/zenodo.3237392
Jenkins, D. J., Kendall, C. W., Augustin, L. S., Mitchell, S., Sahye-Pudaruth, S., Mejia, S. B., et al. (2012). Effect of legumes as part of a low glycemic index diet on glycemic control and cardiovascular risk factors in type 2 diabetes mellitus: a randomized controlled trial. Arch. Intern. Med. 172, 1653–1660. doi: 10.1001/2013.jamainternmed.70
Jha, A. B., Gali, K. K., Zhang, H., Purves, R. W., Tar'an, B., Vandenberg, A., et al. (2020). Folate profile diversity and associated SNPs using genome wide association study in pea. Euphytica 216, 1–16. doi: 10.1007/s10681-020-2553-8
Jha, A. B., and Warkentin, T. D. (2020). Biofortification of pulse crops: status and future perspectives. Plants 9, 73. doi: 10.3390/plants9010073
Jukanti, A. K., Gaur, P. M., Gowda, C. L. L., and Chibbar, R. N. (2012). Nutritional quality and health benefits of chickpea (Cicer arietinum L.): a review. Br. J. Nutr. 108, S11–S26. doi: 10.1017/S0007114512000797
Khattab, R. Y., and Arntfield, S. D. (2009). Nutritional quality of legume seeds as affected by some physical treatments 2. Antinutritional factors. LWT Food Sci. Technol. 42, 1113–1118. doi: 10.1016/j.lwt.2009.02.004
Khazaei, H., Podder, R., Caron, C. T., Kundu, S. S., Diapari, M., Vandenberg, A., et al. (2017). Marker–trait association analysis of iron and zinc concentration in lentil (Lens culinaris Medik.) seeds. Plant Genome 10, plantgenome2017-02. doi: 10.3835/plantgenome2017.02.0007
Kumar, S., and Pandey, G. (2020). Biofortification of pulses and legumes to enhance nutrition. Heliyon 6, e03682. doi: 10.1016/j.heliyon.2020.e03682
Kumar, S., Sangwan, S., Yadav, R., Langyan, S., and Singh, M. (2014). “Maize carotenoid composition and biofortification for provitamin A activity,” in Maize: Nutrition Dynamics and Novel Uses (New Delhi: Springer), 83–91.
Kushwaha, P., Srivastava, R., Pandiyan, K., Singh, A., Chakdar, H., Kashyap, P. L., et al. (2021). Enhancement in plant growth and zinc biofortification of chickpea (Cicer arietinum L.) by Bacillus altitudinis. J. Soil Sci. Plant Nutr. 21, 922–935. doi: 10.1007/s42729-021-00411-5
Lampkin, N., Pearce, B., Leake, A., Creissen, H., Gerrard, C. L., Gerling, R., et al. (2015). The Role of Agroecology in Sustainable Intensification. LUPG agencies, UK.
Landrigan, P. J., Fuller, R., Acosta, N. J., Adeyi, O., Arnold, R., Baldé, A. B., et al. (2018). The Lancet Commission on pollution and health. Lancet 391, 462–512. doi: 10.1016/S0140-6736(17)32345-0
Langyan, S., Bhardwaj, R., Kumari, J., Jacob, S. R., Bisht, I. S., Singh, A., et al. (2021b). Nutritional diversity in native germplasm of maize collected from three different fragile ecosystems of India. Front. Nutr. 9:812599. doi: 10.3389/fnut.2022.812599
Langyan, S., Dar, Z. A., Chaudhary, D. P., Shekhar, J. C., Herlambang, S., El-Enshasy, H., et al. (2021a). Analysis of nutritional quality attributes and their inter-relationship in maize inbred lines for sustainable livelihood. Sustainability 13, 1–12. doi: 10.3390/su131161370
Langyan, S., Yadava, P., Khan, F. N., Dar, Z. A., Singh, R., and Kumar, A. (2022). Sustaining protein nutrition through plant-based foods. Front. Nutr. 8, 772573. doi: 10.3389/fnut.2021.772573
Leinonen, I., Iannetta, P. P. M., Rees, R. M., Russell, W., Watson, C., and Barnes, A. P. (2019). Lysine supply is a critical factor in achieving sustainable global protein economy. Front. Sustain. Food Syst. 3:27. doi: 10.3389/fsufs.2019.00027
Lindgren, E., Harris, F., Dangour, A. D., Gasparatos, A., Hiramatsu, M., Javadi, F., et al. (2018). Sustainable food systems—a health perspective. Sustain. Sci. 13, 1505–1517. doi: 10.1007/s11625-018-0586-x
MacDonald, R. S. (2000). The role of zinc in growth and cell proliferation. J. Nutr. 130, 1500S−1508S. doi: 10.1093/jn/130.5.1500S
Magrini, M. B., Anton, M., Chardigny, J. M., Duc, G., Duru, M., Jeuffroy, M. H., et al. (2018). Pulses for sustainability: breaking agriculture and food sectors out of lock-in. Front. Sustain. Food Syst. 2:64. doi: 10.3389/fsufs.2018.00064
Mandal, S., Mondal, K., Ghoshal, S., Pal, D. B. A., and Acharya, S. K. (2020). Livelihood Implications of pulse in an operating cropping system. Curr. J. Appl. Sci. Technol. 39, 91–99. doi: 10.9734/cjast/2020/v39i2930961
Margier, M., Georgé, S., Hafnaoui, N., Remond, D., Nowicki, M., Du Chaffaut, L., et al. (2018). Nutritional composition and bioactive content of legumes: characterization of pulses frequently consumed in France and effect of the cooking method. Nutrients 10, 1668. doi: 10.3390/nu10111668
Medrano-Macías, J., Leija-Martínez, P., González-Morales, S., Juárez-Maldonado, A., and Benavides-Mendoza, A. (2016). Use of iodine to biofortify and promote growth and stress tolerance in crops. Front. Plant Sci. 7, 1146. doi: 10.3389/fpls.2016.01146
Meybeck, A., and Gitz, V. (2017). Sustainable diets within sustainable food systems. Proc. Nutr. Soc. 76, 1–11. doi: 10.1017/S0029665116000653
Mittal, G., Vadhera, S., Brar, A. P. S., and Soni, G. (2009). Protective role of chickpea seed coat fibre on N-nitrosodiethylamine-induced toxicity in hypercholesterolemic rats. Exp. Toxicol. Pathol. 61, 363–370. doi: 10.1016/j.etp.2008.07.006
Moraghan, J. T., Padilla, J., Etchevers, J. D., Grafton, K., and Acosta-Gallegos, J. A. (2002). Iron accumulation in seed of common bean. Plant Soil 246, 175–183. doi: 10.1023/A:1020616026728
Nair, R. M., Thavarajah, P., Giri, R. R., Ledesma, D., Yang, R. Y., Hanson, P., et al. (2015). Mineral and phenolic concentrations of mungbean [Vigna radiata (L.) R. Wilczek var. radiata] grown in semi-arid tropical India. J. Food Comp. Anal. 39, 23–32. doi: 10.1016/j.jfca.2014.10.009
Olaleye, A. A., Adeyeye, E. I., and Adesina, A. J. (2013). Chemical composition of bambara groundnut (V. subterranea L. Verdc) seed parts. Bang. J. Sci. Indus. Res. 48, 167–178. doi: 10.3329/bjsir.v48i3.17325
Oliveira, B., Moura, A. P. D., and Cunha, L. M. (2019). Increasing pulse consumption to improve human health and food security and to mitigate climate change. Clima. Change Resil. Agric. Agroforest. 21–35. doi: 10.1007/978-3-319-75004-0_2
Oomah, B. D., and Mazza, G. (2000). Bioactive components of flaxseed: occurrence. Phytochem. Phytopharm. 106, 105–112.
Osorio-Diaz, P., Agama-Acevedo, E., Mendoza-Vinalay, M., Tovar, J., and Bello-Perez, L. A. (2008). pasta added with chickpea flour: chemical composition, in vitro starch digestibility and predicted glycemic index pasta adicionada con harina de garbanzo: composición química, digestibilidad in vitro del almidóny predicción del índice glucémico. Cyta J. Food 6, 6–12. doi: 10.1080/11358120809487621
Pew (2017). Pew Research Centre. World population by income. Available online at: http://www.pewglobal.org/interactives/global-population-by-income/ (accessed December 20, 2017).
Pina-Pérez, M. C., and Pérez, M. F. (2018). Antimicrobial potential of legume extracts against foodborne pathogens: a review. Trends Food Sci. Technol. 72, 114–124. doi: 10.1016/j.tifs.2017.12.007
Pitso, F. S., and Lebese, M. R. (2014). Traditional uses of wild edible plants in arid areas of South Africa. J. Hum. Ecol. 48, 23–31. doi: 10.1080/09709274.2014.11906771
Pittaway, J. K., Ahuja, K. D., Robertson, I. K., and Ball, M. J. (2007). Effects of a controlled diet supplemented with chickpeas on serum lipids, glucose tolerance, satiety and bowel function. J. Am. Coll. Nutr. 26, 334–340. doi: 10.1080/07315724.2007.10719620
Prasad, A. S., Bao, B., Beck, F. W., Kucuk, O., and Sarkar, F. H. (2004). Antioxidant effect of zinc in humans. Free Radic. Biol. Med. 37, 1182–1190. doi: 10.1016/j.freeradbiomed.2004.07.007
Prasad, J. S. S., and Narayanan, G. (2014). Minimum switching loss pulse width modulation for reduced power conversion loss in reactive power compensators. IET Power Electron. 7, 545–551. doi: 10.1049/iet-pel.2013.0299
Rachwa-Rosiak, D., Nebesny, E., and Budryn, G. (2015). Chickpeas—Composition, nutritional value, health benefits, application to bread and snacks: a review. Crit. Rev. Food Sci. Nutr. 55, 1137–1145. doi: 10.1080/10408398.2012.687418
Ray, H., Bett, K., Tar'an, B., Vandenberg, A., Thavarajah, D., and Warkentin, T. (2014). Mineral micronutrient content of cultivars of field pea, chickpea, common bean, and lentil grown in Saskatchewan, Canada. Crop Sci. 54, 1698–1708. doi: 10.2135/cropsci2013.08.0568
Rychlik, M., Englert, K., Kapfer, S., and Kirchhoff, E. (2007). Folate contents of legumes determined by optimized enzyme treatment and stable isotope dilution assays. J. Food Comp. Anal. 20, 411–419. doi: 10.1016/j.jfca.2006.10.006
Saxena, K. B., Vijaya Kumar, R., and Sultana, R. (2010). Quality nutrition through pigeonpea—a review. Health 2, 1335–1344. doi: 10.4236/health.2010.211199
Scott, J., Rébeillé, F., and Fletcher, J. (2000). Folic acid and folates: the feasibility for nutritional enhancement in plant foods. J. Sci. Food Agric. 80, 795–824. doi: 10.1002/(SICI)1097-0010(20000515)80:7<795::AID-JSFA599>3.0.CO;2-K
Sellamuthu, K. M., and Malathi, P. (2021). Biofortification of crops to overcome malnutrition in India. Biotica Res. Today 3, 402–405.
Sen Gupta, D., Thavarajah, D., Knutson, P., Thavarajah, P., McGee, R. J., Coyne, C. J., et al. (2013). Lentils (Lens culinaris L.), a rich source of folates. J. Agric. Food Chem. 61, 7794–7799. doi: 10.1021/jf401891p
Shahzad, R., Jamil, S., Ahmad, S., Nisar, A., Khan, S., Amina, Z., et al. (2021). Biofortification of cereals and pulses using new breeding techniques: current and future perspectives. Front. Nutr. 665, 721728. doi: 10.3389/fnut.2021.721728
Silva de Morais, N., Ayres Saraiva, D., Corcino, C., Berbara, T., Schtscherbyna, A., Moreira, K., et al. (2020). Consequences of iodine deficiency and excess in pregnancy and neonatal outcomes: a prospective cohort study in Rio de Janeiro, Brazil. Thyroid 30, 1792–1801. doi: 10.1089/thy.2019.0462
Singh, A. K., Singh, S. S., Prakash, V. E. D., Kumar, S., and Dwivedi, S. K. (2015). Pulses production in india: present status, sent status, bottleneck and way forward. J. AgriSearch 2, 75–83.
Singh, J., and Basu, P. S. (2012). Non-nutritive bioactive compounds in pulses and their impact on human health: an overview. doi: 10.4236/fns.2012.312218
Singh, N., Narula, B., Ujinwal, M., and Langyan, S. (2021). Pigeonpea sterility mosaic virus a green plague-Current status of available drug and new potential targets. Ann. Proteom Bioinform. (2021) 5:008–026. doi: 10.29328/journal.apb.1001013
Singh, U. B., Malviya, D., Singh, S., Singh, P., Ghatak, A., Imran, M., et al. (2021). Salt-tolerant compatible microbial inoculants modulate physio-biochemical responses enhance plant growth, Zn biofortification and yield of wheat grown in saline-sodic soil. Int. J. Environ. Res. Public Health 18, 9936. doi: 10.3390/ijerph18189936
Stadlmayr, B., Nilsson, E., Mouille, B., Medhammar, E., Burlingame, B., and Charrondière, U. R. (2011). Nutrition indicator for biodiversity on food composition – a report on the progress of data availability. J. Food Comp. Anal. 24, 692–698. doi: 10.1016/j.jfca.2010.09.009
Suneja, Y., Grewal, S., Gupta, A., and Kaur, N. (2011). Levels of nutritional constituents and antinutritional factors in black gram (Vigna mungo L. Hepper). Food. Res. 44. 621–628. doi: 10.1016/j.foodres.2010.12.020
Talari, A., and Shakappa, D. (2018). Role of pigeon pea (Cajanus cajan L.) in human nutrition and health: a review. Asian J. Dairy Food Res. 37, 212–220. doi: 10.18805/ajdfr.DR-1379
Thavarajah, P. (2012). Evaluation of chickpea (Cicer arietinum L.) micronutrient composition: biofortification opportunities to combat global micronutrient malnutrition. Food Res. Int. 49, 99–104. doi: 10.1016/j.foodres.2012.08.007
Tiwari, A. K., and Shivhare, A. K. (2016). Pulses in India: Retrospect and Prospects. Director, Govt. of India, Ministry of Agri. and Farmers Welfare (DACand FW), 1–21. Available online at: https://farmer.gov.in/~imagedefault/prospects_2017.pdf (accessed February 15, 2022).
Toledo, Á., and Burlingame, B. (2006). Biodiversity and nutrition: a common path toward global food security and sustainable development. J. Food Comp. Anal. 19, 477–483. doi: 10.1016/j.jfca.2006.05.001
UNDP. (2022). Available online at: https://hdr.undp.org/towards-hdr-2022 (accessed February 15, 2022).
UNICEF and World Health Organization. (2017). The State of Food Security and Nutrition in the World 2017: Building Resilience for Peace and Food Security. Rome.
United Nations World Population Division (2017). Available online at: http://www.un.org/en/development/desa/population/ (accessed December 20, 2017).
Vahteristo, L., Lehikoinen, K., Ollilainen, V., and Varo, P. (1997). Application of an HPLC assay for the determination of folate derivatives in some vegetables, fruits and berries consumed in Finland. Food Chem. 59, 589–597. doi: 10.1016/S0308-8146(96)00318-4
Vandemark, G. J., Grusak, M. A., and McGee, R. J. (2018). Mineral concentrations of chickpea and lentil cultivars and breeding lines grown in the US Pacific Northwest. Crop J. 6, 253–262. doi: 10.1016/j.cj.2017.12.003
Vaz Patto, M. C., Amarowicz, R., Aryee, A. N. A., Boye, J. I., Chung, H. J., MartínCabrejas, M. A., et al. (2015). Achievements and challenges in improving the nutritional quality of food legumes. CRC. Crit. Rev. Plant Sci. 34, 105–143. doi: 10.1080/07352689.2014.897907
Venkidasamy, B., Selvaraj, D., Nile, A. S., Ramalingam, S., Kai, G., and Nile, S. H. (2019). Indian pulses: a review on nutritional, functional and biochemical properties with future perspectives. Trends Food Sci. Technol. 88, 228–242. doi: 10.1016/j.tifs.2019.03.012
Wallace, T. C., Murray, R., and Zelman, K. M. (2016). The nutritional value and health benefits of chickpeas and hummus. Nutrients 8, 766. doi: 10.3390/nu8120766
White, P. J., and Broadley, M. R. (2009). Biofortification of crops with seven mineral elements often lacking in human diets-iron, zinc, copper, calcium, magnesium, selenium and iodine. New Phytol. 182, 49–84. doi: 10.1111/j.1469-8137.2008.02738.x
Yamaguchi, M., and Uchiyama, S. (2004). β-Criptoxanthin stimulates bone formation and inhibits bone resorption in tissue culture in vitro. Mol. Cell. Biochem. 258, 137–144. doi: 10.1023/B:MCBI.0000012848.50541.19
Yao, D. N., Kouassi, K. N., Erba, D., Scazzina, F., Pellegrini, N., and Casiraghi, M. C. (2015). Nutritive evaluation of the Bambara groundnut Ci12 landrace [Vigna subterranea (L.) Verdc. (Fabaceae)] produced in Côte d'Ivoire. Int. J. Mol. Sci. 16, 21428–21441. doi: 10.3390/ijms160921428
Zaheer, M., Ahmed, S., and Hassan, M. M. (2020). Vigna unguiculata (L.) Walp.(Papilionaceae): a review of medicinal uses. J. Pharmacogn. Phytochem. 9, 1349–1352.
Keywords: healthy diet, pulses, sustainable food systems, nutrition, food consumption, sustainable development goals
Citation: Langyan S, Yadava P, Khan FN, Bhardwaj R, Tripathi K, Bhardwaj V, Bhardwaj R, Gautam RK and Kumar A (2022) Nutritional and Food Composition Survey of Major Pulses Toward Healthy, Sustainable, and Biofortified Diets. Front. Sustain. Food Syst. 6:878269. doi: 10.3389/fsufs.2022.878269
Received: 17 February 2022; Accepted: 26 May 2022;
Published: 04 July 2022.
Edited by:
Barbara Burlingame, Massey University, New ZealandReviewed by:
Aejaz Ahmad Dar, Sher-e-Kashmir University of Agricultural Sciences and Technology of Jammu, IndiaPoonam Yadav, Banaras Hindu University, India
Copyright © 2022 Langyan, Yadava, Khan, Bhardwaj, Tripathi, Bhardwaj, Bhardwaj, Gautam and Kumar. This is an open-access article distributed under the terms of the Creative Commons Attribution License (CC BY). The use, distribution or reproduction in other forums is permitted, provided the original author(s) and the copyright owner(s) are credited and that the original publication in this journal is cited, in accordance with accepted academic practice. No use, distribution or reproduction is permitted which does not comply with these terms.
*Correspondence: Sapna Langyan, c2luZ2guc2FwbmEwNkBnbWFpbC5jb20=; Pranjal Yadava, cHJhbmphbC55YWRhdmFAaWNhci5nb3YuaW4=; Rakesh Bhardwaj, cmJfYmlvY2hlbUB5YWhvby5jb20=