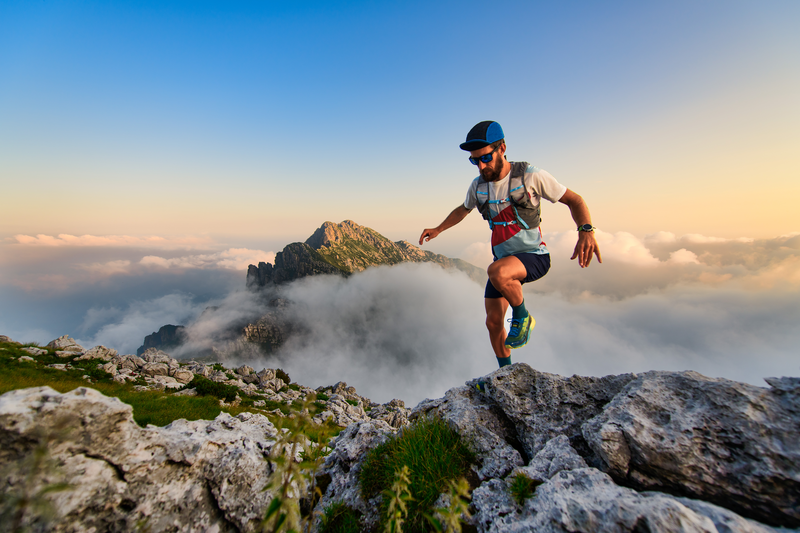
94% of researchers rate our articles as excellent or good
Learn more about the work of our research integrity team to safeguard the quality of each article we publish.
Find out more
ORIGINAL RESEARCH article
Front. Sustain. Food Syst. , 24 June 2022
Sec. Agroecology and Ecosystem Services
Volume 6 - 2022 | https://doi.org/10.3389/fsufs.2022.868870
This article is part of the Research Topic Crop Pest Control and Pollination View all 11 articles
Biophysical evaluations of climate-smart agriculture (CSA) often overlook the potential interactions with and implications for biodiversity and ecosystem services, which are important determinants of food system resilience and sustainability. Drawing on a case study in the East Usambara Mountains, Tanzania, we compare the impacts of CSA with other agricultural management practices on invertebrate pest and natural enemy diversity, and the associated effects on crop damage and crop yield. We found that the most common CSA practices in the region, terracing and trenching with live and compost mulches, provided the best outcomes for crop production, pest suppression and agricultural income. However, greater diversity of pests was observed when neighboring fields planted improved crop varieties, suggesting that the use of improved varieties by farmers creates increased vulnerability to pest damage among neighboring farmers that used local varieties. Also, greater natural enemy diversity was found when neighboring fields were either intercropped or left fallow highlighting spatial flows of ecosystem services between fields. Landcover heterogeneity was positively correlated with pest diversity, whilst landcover richness was positively associated with higher pest volume, highlighting the importance of landscape characteristics in pest and natural enemy dynamics. Finally, we found that crop damage was most severe when pest communities had low species richness, suggesting that a small number of key crop pests contribute to most yield losses. Our findings illustrate that those varied combinations of agricultural management practices lead to heterogeneous biodiversity outcomes and trade-offs, and highlight the importance of local management, neighborhood effects and landscape characteristics. CSA evaluations must therefore look beyond productivity as a measure for success, as trade-offs with invertebrate biodiversity, food production, and environmental sustainability often interact and feedback in complex and unexpected ways.
Climate change poses a significant threat to the future of global food production with implications for agricultural productivity and food system sustainability (Lobell et al., 2011; Dang and Dabalen, 2017; Ray et al., 2019). In addition to climate change, there is widespread global concern about biodiversity loss due to agricultural expansion and intensification (Newbold, 2018; Beckmann et al., 2019). Besides its inherent, intrinsic value, biodiversity underpins vital ecosystem services that support food production (Dainese et al., 2019), such as the biological control of crop pests and diseases by natural enemies (Bommarco et al., 2018). As with food production, biodiversity and biodiversity-based ecosystem services are fundamentally dependent on climate and, hence, vulnerable to climate change (Pecl et al., 2017; Nunez et al., 2019).
Climate smart agriculture (CSA) has been developed as a climate adaptation framework for multiple aspects of agriculture, from field-level practices to food supply chains (Venkatramanan and Shah, 2019). CSA implementation has targeted developing regions such as the sub-Saharan Africa (SSA) (Neufeldt et al., 2013), which are most vulnerable to climate change and have limited capacity to cope, and invest in adaptive institutions and technologies (Fankhauser and McDermott, 2014; Porter et al., 2015; van Wijk et al., 2020). The primary goal of CSA is to achieve triple wins by contributing to three objectives; increasing productivity, increasing the resilience of food production, and mitigating greenhouse gas emissions (Lipper et al., 2018). However, there are gaps in evaluating the success of CSA approaches as studies have predominantly focussed on productivity outcomes (Dinesh et al., 2015; Rosenstock et al., 2019; van Wijk et al., 2020). In a limited number of cases, investigations of the resilience benefits have also been linked to productivity gains, where surplus food minimized the effects of climate impacts and increased the resilience of farmers (Dinesh et al., 2015; van Wijk et al., 2020). With such a narrow agricultural productivity and food security focus, the possible impact of CSA practices on other components that affect agricultural system resilience and environmental sustainability, such as biodiversity and ecosystem service provisioning (Thornton et al., 2018), is a crucial knowledge gap. Evaluation of these broader components would help develop a better understanding of the system-level effectiveness of, and trade-offs associated with the CSA framework. This is critical to enable the identification of agricultural strategies that improve food system multifunctionality necessary for building resilience to climate change (Thornton et al., 2018; van Wijk et al., 2020).
Here, we evaluate the effects of local-scale farm management, including CSA practices, on invertebrate diversity and agricultural yields, focusing on pest prevalence and suppression in the East Usambara Mountains of Tanzania. Specifically, we examine relationships among (i) invertebrate richness, abundance and volume of crop pests and natural enemies, (ii) percentage crop damage and, (iii) annual income from crops (USD ha−1) under varying combinations of CSA and traditional farm management practices. Landcover diversity and configuration around the fields (Duarte et al., 2018; Zhang et al., 2020), and management practices in the neighboring fields affect the invertebrate diversity, pests, and natural enemies through spatial flow and interactions (Redlich et al., 2018). The understanding of the effects of the landscape context and the associated processes on local agrobiodiversity and related ecosystem services is mainly based on studies in large-scale and commercial farming systems in temperate ecosystems (Rusch et al., 2016; Woodcock et al., 2016; Aguilera et al., 2021). Only a few studies have examined the ecosystem services in biodiverse smallholder farming landscapes in SSA (Mkenda et al., 2019). Hence, we also account for the effects of landscape context and the influence of management in the neighboring fields on invertebrate richness, crop damage and income.
Our study provides new insights into the implications of CSA farm management for biodiversity and ecosystem services at local and landscape scales in SSA, which can inform future agricultural transformation strategies in the region and beyond.
The study was conducted in the East Usambara Mountains, Tanzania (Figure 1), where CSA was introduced as part of the ‘Integrated Approaches for Climate Change Adaptation Project' (IACCA), which ran between 2015 and 2019 as part of the European Union-funded Global Climate Change Alliance (GCCA+) programme (Ongawa, 2019). The project established farmer field schools (FFS) and provided training and capacity building of farmer communities in CSA.
Figure 1. Map of the study site and sampling design in Tanzania: East Usambara Mountains in Muheza District of Tanga Region. The traps were placed in the center and margin of 82 focal fields in the study area, with sampling taking place five times between Sept. 2019 and May 2020.
Farming communities in the region predominantly comprise highly diverse but low-input, mixed cropping, livestock (primarily zero-grazing dairy cattle and poultry) and agroforestry smallholder systems. Food crops include maize, beans, yams, banana, and cassava, while commercial crops include spices (cardamom, cinnamon, clove, and black pepper), sugarcane, fruits (jackfruit), and some horticultural produce (tomatoes, onions, leafy vegetables).
We sampled invertebrate diversity and abundance during five sampling occasions at approximately 2-month intervals – September and November 2019, January, March, and May in 2020, in 82 fields across an elevation gradient from 200-400m (lowlands) to 800-1200m (highlands) above sea level. The fields were selected from a dataset of 150 areas containing agronomic details prepared by two local extension officers from the Muheza District Agriculture Office. The selection of the fields was based on the availability and willingness of farmers to participate in this study. We used the agronomic background information to select fields so that 50% of our sampled fields had at least two climate-smart management activities (Table 1) practiced for 2 years or more (as defined by the IAACA project). During each field visit, we recorded information about local management practices in the focal field (i.e., the field we were sampling) and in the nearest neighboring field. The information comprised farm size, the number of crop species in the field, and the presence or absence of the following practices: improved crop variety, mulching, spice plantation, row spacing, trenching, intercrop, agroforestry, and fallow.
As the number, type and combination of management practices varied considerably between fields, we applied the k-means hierarchical clustering algorithm (Qi et al., 2017; Figure 2) to the management practices data to characterize them. This resulted in 5 distinct field categories (Figure 2; Table 1):
Figure 2. PCA Biplot of Farm clusters, where five categories based on the dominant set of management activities emerged. First two axis indicates clear distinction between Monocrop-Trench and CS-Intercrop-Cropdiv. Third axis (not visible) distinguishes between the other three categories.
To account for the influence of landscape context, we computed two landscape-level variables; edge length (density of forest edges) and landcover richness (total number of unique landcovers) within 500m radius of the focal field, using the Copernicus 100m resolution landcover dataset (Buchhorn et al., 2020).
At each field center and margin (2 m inside the field), we sampled invertebrates using three techniques - a combination of two Pitfall traps, a Sticky trap and three Pan Traps. Pitfall traps were installed with 1 m distance between them, sunk in the ground with the rim at the ground level, and filled with water with a drop of liquid soap to break the surface tension. The pan traps comprised a cluster of three pans (UV bright yellow, blue, and white) placed together half-filled with water and a drop of liquid soap. The yellow sticky traps were placed between the pitfall traps, approximately 100 cm above the ground. The traps operated for 72 h, after which the specimens were collected and sorted into morphologically distinct groups (OTU, operational taxa units) by two field assistants (MSc. students from the Sokoine University of Agriculture, Tanzania), and two agricultural extension officers from Muheza District. The specimens were preserved in storage vials containing 90% ethanol to further identify orders, families, and genera. The vials containing the specimens were labeled with unique OTU codes corresponding to taxonomic id, sampling station, field number, and month. The OTUs were further classified into potential pests (predominantly crop herbivores) and potential natural enemies (insect predators and parasitoids). The total number of individuals and length and width (in centimeters) of 2–3 randomly selected individuals were recorded for each OTU. Further, for each sampling occasion (sampling station, technique, and month), we measured abundance (total number of individuals), invertebrate biovolume (volume here onwards, abundance × length × breadth) as a measure of biomass, and richness of OTU (number of unique OTUs), which were used as invertebrate response variables. The invertebrate volume is based on size i.e., length and breadth, as well as the number of individuals and is an indicator of body-size distribution in an invertebrate assemblage.
We also measured crop damage on two randomly selected plants of each crop species (for intercrop fields) at each sampling station by recording the approximate percentage of pest-induced damage observed on leaves, cobs or fruits, and stems. Mean damage observed in all plants and plant parts was used to derive a field-level measure of crop damage.
We collected information about the annual income from the major crops in each field by asking farmers about the quantity they harvested for each crop in the field during our study in 2019–20 (details of each crop yields in supporting information, SI). We converted yields per annum to earnings per hectare per annum (in Tanzanian shillings) by asking the extension officers about the average market price a farmer would get after selling the harvested amount in the local market in Muheza during the period of study. Tanzanian shillings were converted to US dollars using the conversion rate of 1 USD = 2,319.05 TZS as of 30th April 2021. Hereafter, annual yield, or annual income indicates US dollars per hectare earnings during 2019–20.
To investigate the effect of management, neighboring field, and landscape context on invertebrates, we used mixed-effects models with richness as a Generalized Poisson distribution (GLMM) and log-transformed values of abundance and volume as a General Normal distribution (GLM). We grouped the invertebrates into potential pests and natural enemies (hereafter, pests, and natural enemies), and modeled the groups and their richness, abundance, and volume separately.
We further explored management effects on crop damage and yield by modeling crop damage and annual yields as a function of management, neighboring field and landscape context using GLMs.
We also tested the relationship between invertebrates and yields by using GLMs to model crop damage and annual yield as response variables and richness, abundance and volume of pest and natural enemies as predictors.
To account for the effect of seasonality and repeated observations, we included sampling month as a random effect (Table 2). Models were specified using all possible combinations of the explanatory variables and subjected to backward model selection based on the lowest values of Akaike's information criterion (AIC). Estimates and unconditional 95% confidence intervals (CI) were derived from the best fit models (ΔAIC < 2). The effect was considered significant when the 95% CI did not overlap zero. We also standardized all the continuous variables, tested for multicollinearity (with an aim to remove variables responsible for VIF>3) and checked for residual distribution against fitted values to verify model assumptions. All calculations and analyses were undertaken using the statistical software R version 3.4.2 (R Core Team, 2017).
Table 2. Response and explanatory variables included in the mixed-effects models with the month as a random effect.
From September 2019 to May 2020, during the five sampling periods, we collected a total of 93,428 invertebrate individuals with a mean body size of 0.13 mm2 (SD = 0.4 mm2), ranging from 0.0002 to 27 mm2. They were identified to 282 distinct morphospecies (OTUs) belonging to 33 orders, 122 families, and 164 genera (list of species and abundances in Supplementary Tables 1, 2).
The month of sampling had a significant effect explaining the majority of variance in abundance, richness and volume of pests and natural enemies (20–24% random effect; Figure 3). The abundance and volume of invertebrates dropped significantly in November 2019, coinciding with flash floods in the study area that destroyed fields and crops. Following the drop in November, abundance and volume recovered and increased significantly in January before gradually declining until the end of sampling in May.
Figure 3. Effect of sampling month on invertebrate richness (A) abundance (B) and volume (C). November showed significantly reduced abundance and volume, which also correlated with flash floods in the study area.
Farm management clusters showed a significant difference in richness, abundance and volume of pests and natural enemies (Figures 4a–f). Compared to the Monocrop-Trench farms, Mulch-Trench, Spacing-Intercrop and Spice-Intercrop-Cropdiv farms had lower pest diversities (Figures 4a,b). CS-Intercrop fields had a higher pest volume (Figure 4c) but showed no significant difference in pest abundance and richness (Figures 4a,b) compared to monocrop-trench fields. Mulching was associated with lower natural enemy volume (Figure 4f) but did not significantly affect the richness and abundance of natural enemies (Figures 4d,e). Spice-Intercrop fields, however, had a lower richness of natural enemies (Figure 4d), in addition to lower pest richness (Figure 4a), suggesting that Spice-Intercrop fields are associated with reduced overall biodiversity.
Figure 4. The coefficient plot of richness (a,d), abundance (b,e) and volume (c,f) of pests (a–c) and natural enemies (d–f) (3 × 2 models) in response to farm clusters based on management practices, neighbouring field characteristics, and landscape context. The independent variables selected in the best fit model (ΔAIC < 2) are shown. Each panel represents a different model. The positions of the circles represent β-coefficients, horizontal lines on the circles show SE, and colors signify the direction of the effect.
Landscape fragmentation, indicated by edge-length, was associated with lower pest richness (Figure 4a), suggesting no evidence of spill-over of potential pests from non-crop forest habitats. In contrast, landcover diversity, measured by landcover richness, showed a positive effect on pest volume (Figure 4c), suggesting that landscapes with greater heterogeneity support a broader range of pest body sizes. The landscape context variables did not significantly affect natural enemies.
Characteristics of neighboring fields also influenced pest and natural enemy prevalence. Local variety monocrop focal fields, with neighbors practicing intercropping and wider crop row spacing, had fewer pests (Figure 4b) and more natural enemies (Figure 4e). In contrast, local variety focal fields had higher pest richness when the neighboring field had improved crop varieties (Figure 4a). Neighboring fallowed fields also led to a positive association with natural enemies in the focal field, albeit only marginally significant (p = 0.06). Also, at higher altitudes, there were a lower pest and natural enemy abundance (Figures 4b,e) and reduced natural enemy richness (Figure 4d).
In summary, farms with mulching, wider spacing between crop plants, and spice plantations had fewer pests, while management in the neighboring fields, mainly intercrop and fallow, had positive outcomes for natural enemies in the focal fields.
Despite lower pest richness, crop damage in Spacing-Intercrop and Spice-Intercrop fields was significantly greater than CS-Intercrop and Monocrop-Trench fields (Figure 5), suggesting damage was caused by a small number of key crop pests. Crop damage was also positively associated with the number of times the field was cultivated and with neighboring intercrops. Edge length was negatively associated with crop damage, but this was only marginally significant (p = 0.07).
Figure 5. The coefficient plot of crop damage (proportion of crop affected by pests) and crop yield earnings (Annual USD per hectare) in response to farm clusters based on management practices, neighboring field characteristics, and landscape context. The independent variables selected in the best fit model are shown. Each panel represent a different model. The positions of the circles represent β- coefficients, horizontal lines on the circles show SE, and colors signify the direction of the effect.
Annual yields were higher in mulching, spacing, and spice fields compared to Monocrop-trench and CS-intercrop fields. Also, fields at high altitudes had significantly higher earnings than lowland fields. Interestingly, local variety focal fields that had neighbors using improved variety crops showed lower yield earnings.
The models (presented in Figure 6), suggest that pest abundance had a significant positive effect on crop damage (0.12 ± 0.52) and a negative association with annual yields (−5.9 ± 2.7). Furthermore, pest abundance effects on crop damage were influenced by pest richness (-0.03 ± 0.01). At low pest richness (richness <4 OTUs), pest abundance had the strongest positive effect on crop damage and a severe negative relationship with annual yields, indicating that a small number of key pests caused the most damage. On the other hand, neither richness nor abundance of natural enemies showed any significant association with crop damage. Still, they had a significant positive effect on annual yields, which increased with natural enemy abundance (5.2 ± 2.06) and richness (4.3 ± 1.5). However, at very high natural enemy richness (n > 8), natural enemy abundance was negatively associated with annual yields.
Figure 6. Interactive effects of pest (left panels) and natural enemy (right panels) richness and abundance on percentage crop damage (top panels) and annual income from crop yield in USD (bottom panels). Colors represent the different levels of Species Richness – low (red), medium (green), and high (blue). Abundance was log-transformed in the model. The plots indicate back-transformed values for ease of interpretation.
Our study is the first to evaluate the ecological implications of CSA practices deployed in different combinations at field-scale. It helped identify potential synergies and trade-offs among the targeted objectives of CSA practices, annual agricultural yields and income, and outcomes for pest and natural enemy diversities and associated ecosystem service of biocontrol (Table 3). We showed that fields with mulches and Fanya juu trenches had the greatest synergy with the targeted objectives in terms of higher annual agricultural income and lower pest pressures. Surprisingly, the fields with maximum CSA practices did not have significantly different yields or invertebrate diversities, indicating that certain practices (mulches and Fanya Juu trenching) had a significant effect, but lots of practices combined ceased to produce any effect. This may simply be due to different practices having contrasting effects on biodiversity and yields – some positive and others negative, resulting in a no-net effect. Interventions such as greater spacing between crops and spice plantations had lower invertebrate diversities (pests as well as natural enemies), but higher annual returns. This indicates a possible trade-off between biodiversity and agricultural production. Further work in this area is needed to consider trade-offs in agricultural production over time, examining short term losses in the context of long-term goals.
Table 3. Trade-off and synergies among the targeted objectives, biodiversity and annual income returned in the study area.
We found that fields with trenches and mulching, primarily adopted to increase soil quality and moisture conservation in the fields, generally also had improved pest suppression. This was indicated by lower abundance and volume of pests, and higher crop yields and annual agricultural earnings. These fields also had a lower volume of natural enemies, but no impact on natural enemy richness or abundance. The role of mulches in reducing pest densities and diversities have been observed in a number of other studies (Altieri et al., 1985; Hooks et al., 1998; Brown and Tworkoski, 2004; Massaccesi et al., 2020) and is generally attributed to the greater density and diversity of ground-dwelling predators (Carabidae, Staphylinidae, and Arachnids) in the fields with live or compost mulching (Altieri et al., 1985; Schmidt et al., 2004). Past studies have also indicated the positive effects of mulching on predator populations and diversity (Schmidt et al., 2004). Natural enemy abundance and richness in the mulched fields in our study was not significantly different from other field management clusters. However, we observed that natural enemy volumes were significantly lower as most natural enemy individuals caught in the mulching fields were of smaller body sizes (mainly Parasitoids). This may be due to lower habitat diversity in and around the mulching fields, compared to the other fields which had agroforestry, spice plantations and intercropping and supported functionally diverse natural enemy communities comprising greater body-size distribution and diversity.
We did not find any other study evaluating the effect of Fanya juu trenches on invertebrates and our study is potentially the first to suggest that Fanya juu trenches and associated live mulching with grass strips offer significant benefits in terms of pest suppression in addition to the targeted objective of reduced run-off and soil erosion. Live mulches are generally associated with reduced pest infestations and crop damage (Altieri et al., 1985; Johnson and Gurr, 2016; Rehman et al., 2019), as also observed in our study. Our observations are comparable to the effects of push-pull technology where Napier grass has often been used as a “pull” crop, which attracts pests away from the crop and thereby reduces crop damage (Khan et al., 2011). Although it was not planted with the targeted objective of reducing crop pests, planting and live mulching with Napier grass demonstrates that there may be considerable synergies between CSA and conservation and pest management agricultural practices like push-pull. Thus, our study shows that the fields with mulching and trenching had the best outcomes in terms of pest suppression and productivity, and no significant reduction in non-pest or natural enemy diversity.
Among the other field clusters in this study, CS-Intercrop fields, where the maximum number of CSA technologies were deployed, were not significantly different from the monocrop traditional fields in terms of pest or natural enemy richness and abundance, crop damage or yields. Pest volumes, however, were the highest in CS-Intercrop fields. This may be because CSA fields containing improved crop varieties exhibited enhanced plant growth and higher foliar biomass, which attracted a larger functional or body-size diversity of insect herbivores (Real-Santillán et al., 2019). Furthermore, wider crop row spacing, and spice intercrops had lower richness of pest communities, higher crop damage, but greater yields than all other fields. This suggests that despite higher crop damage in food crops (maize and beans), the annual yields in Spice-Intercrop fields were not affected as spice plantations (clove and cardamom) and intercrops (cassava and bananas) mediated the net effect of pest damage.
Our study also showed that farm characteristics such as farm size, number of times it is cultivated, management practices in the neighboring fields and landscape context influenced the pests and natural enemies in the focal field as observed in other studies which are mainly from commercial farming landscapes (Rodriguez-Saona and Thaler, 2005; Madeira et al., 2016; Schulz-Kesting et al., 2021). Hence, this is one of the few studies from biodiverse smallholder farming landscapes in SSA that demonstrates such process are important in a wide range of farming systems. Particularly, farm size was associated with a reduction in abundance and volume of natural enemies. Increasing farm sizes is associated with fragmentation of natural landcover and landscape homogenisation, which may lead to a reduction in biocontrol as natural enemy communities with lower densities and a smaller range of body sizes are known to be less effective in pest control (Letourneau and Goldstein, 2001; Staton et al., 2021). Furthermore, neighbors practicing intercropping was associated with reduced pest abundance and an increase in natural enemy richness in local variety maize monocrops, mainly dominated by Coccinellids. Pest abundance was strongly related with an increase in crop damage and reduction in yields, while natural enemy richness was associated with a reduction in crop damage. This highlights the importance of local heterogeneity created by the difference in management practices between fields, which leads to spatial ecological interactions and flow of ecosystem services between neighboring fields where the characteristics of one field influences pest pressure and biocontrol in the other field (Landis et al., 2000; Sarthou et al., 2014).
Landscape heterogeneity, as indicated by forest edge length, had positive association with pest richness and negative relationship with crop damage. The effect of landscape configuration is typically context-dependent and has often been overlooked in the landscape-pest and biocontrol literature (Karp et al., 2018). An increase in landscape heterogeneity may lead to an increase in pest diversity resulting in aggravated pest pressures and crop damage (Martin et al., 2013; Zhang et al., 2020). In contrast, an increase in pest richness, diversity and evenness can also reduce pest-driven crop damage as some studies indicate (Crowder et al., 2010; Lundgren and Fausti, 2015). Diverse and more evenly distributed pest communities have higher inter-species competition, which reduces the dominance and impact of single and most impactful pest species. In our study, fields with species poor pest communities (2–3 species) and with greater mean abundances (mean 132 ± 74 individuals) were dominated by Diptera, Orthoptera and Lepidoptera groups, and had 4% higher crop damage and 28% lower annual earnings than fields with species-rich pest communities. This supports the possibility that lower pest richness with greater density and dominance of few and more specialized crop herbivores like locusts, fall armyworm, stalk borers and African cotton bollworm may lead to greater crop damage and yield losses. Our findings thereby suggest that diversity decreases herbivory potentially diluting the impact of dominant pests on crop production (Civitello et al., 2015). Therefore, scaling up of CSA must take in to account broader landscape-scale perspective and focus on maintaining heterogeneity in agricultural landscapes through mosaics of monocrop and intercrop fields intermixed with natural and semi-natural landscapes.
Use of improved crop varieties is widely promoted as a climate adaptation (Thierfelder et al., 2016). Our study showed that use of improved crop varieties for drought resistance and shorter growing season in a neighboring field was associated with increased pest richness and reduced yields in the focal fields with local varieties, mainly of maize. This could mean that a shift to improved varieties among one group of farmers may lead to increased vulnerability of another group, thereby highlighting a form of maladaptation. Further studies are needed to explore the spatial flows and interactions of ecosystem services and disservices due to the adoption of new technologies.
We also found a strong effect of crop seasonality as months coinciding with maize plantation (January) and harvest (September), which are themselves timed with respect to expected rains, had the highest levels of pest and natural enemy diversity, abundance, and volume. The effect of sampling season has also been observed across the globe and shows that it is important to take in to account the temporal variability when evaluating ecological responses to agricultural interventions (Habel et al., 2018; Fanelli, 2020; Scheiner and Martin, 2020). Further, one of the sampling occasions, November 2019, coincided with flash floods in the study area during which the invertebrate communities significantly declined in richness, abundance, and volumes. This sudden decline was more likely due to the destructive effect of flash floods on invertebrate habitats as observed in other studies (Hickey and Salas, 1995; Moi et al., 2020). It also reveals the fragility of any farming system, whether they are CSA or not, in the face of increasing climate extremes.
Adoption of CSA may be necessary in the future to reduce agriculture's vulnerability to climate change and ensure food security for an estimated 9 billion people by 2050 (Taylor, 2018). CSA, presently, is focused primarily on technical fixes to increase agricultural productivity and adaptation (Dinesh et al., 2015). However, food production under climate change will also depend largely on sustainably increasing farmer's resilience to climate risks by developing adaptation strategies and managing trade-offs (risks and conflicts) between land use change, biodiversity, and food production (Chandra et al., 2018; Taylor, 2018). Our study highlights the need for agriculture industry to evaluate interactions, synergies, and trade-offs between the different outcomes relating agriculture change from a multi-dimensional perspective. Understanding of risks and challenges farmers face, adaptation strategies they use, and which strategies provide the best outcome in terms of productivity, food security net agricultural income, and environmental stability will be crucial for building more resilient and sustainable food systems of future (MacFall et al., 2015; Aggarwal et al., 2018).
The present study is an evaluation of management practices at local scales. Even though we sampled for multiple months in a year there may be variations and multi-year effects we could not account for. The CSA practices in the study areas were introduced only 3 years before the present study was implemented. Although the three-year time frame may be enough to effect the changes in some invertebrate communities - pests especially, there may still be time lags in responses of indirectly affected trophic groups (e.g., natural enemy communities) causing delayed biodiversity changes (Essl et al., 2015). Overlooking time lags may result in an underestimate of biodiversity impacts and associated trade-offs with food production. Hence, longer-term studies are needed to fully understand the ecological implications and biodiversity impacts of agricultural interventions. Our study, nevertheless, points toward possible trends.
Our study identifies mulching and Fanya juu trenches as CSA practices with potential for maximizing synergies among food production, biodiversity conservation and pest suppression via biocontrol. Furthermore, it also shows that that increasing inter-row spacing of Maize to 70–100 cm and integrating food crops like maize and beans with cash crops, such as spice plantations, improves grain yield and annual income. Broadly, our study highlights the importance of local management and landscape characteristics in maintaining biodiversity. Our study has also uncovered the real risk of negative impacts of CSA intervention and maladaptation on farmer livelihoods. In this study, increased pest richness and abundance, lower yields and income were evident in local-variety fields neighboring those where improved varieties are used, suggesting the use of improved varieties by farmers is creating increased vulnerability among neighboring farmers. The need for further research on assessing biodiversity impacts, and for holistic and long-term evaluation and monitoring of CSA across field and landscape scales is therefore very clear. CSA evaluations must look beyond productivity alone because the trade-offs and synergies among biodiversity, agricultural production and food security, livelihoods and health often interact and feedback on each other. Finally, more local-scale case and long-term studies and syntheses are needed to understand the combined and interactive effects of agriculture intervention and global change processes across different geographies and food production models.
The raw data supporting the conclusions of this article will be made available by the authors, without undue reservation.
HT conceived the idea, designed, and implemented this study with support from SSai, WK, SSal, SMau, and HS. All authors have contributed ideas that inform the structure and narrative of the paper, provided editorial support, agreed to the published version of the manuscript, and contributed to the article and approved the submitted version.
This work was funded by the Biotechnology and Biological Sciences Research Council through UK Research and Innovation, as part of the Global Challenges Research Fund: AFRICAP Programme, Grant Number BB/P027784/1. Research in Tanzania was conducted under COSTECH permit number: 2019-305-NA-2016-101. RD was supported by the University of Leeds MSc in Biodiversity and Conservation programme. Fieldwork in Tanzania was supported by the Economic and Social Research Foundation. The funders had no role in the design of the study, in the collection, analyses, or interpretation of data; in the writing of the manuscript, or in the decision to publish the results.
The authors declare that the research was conducted in the absence of any commercial or financial relationships that could be construed as a potential conflict of interest.
All claims expressed in this article are solely those of the authors and do not necessarily represent those of their affiliated organizations, or those of the publisher, the editors and the reviewers. Any product that may be evaluated in this article, or claim that may be made by its manufacturer, is not guaranteed or endorsed by the publisher.
We are very grateful to the community members and village leaders who gave their valuable time and allowed us to conduct sampling in their fields. Special thanks to Elimeena Baraka, Suzan Donald, Majid Majidi, Mosha Florence, and Manzil Maburuki in Tanzania for their support with data collection.
The Supplementary Material for this article can be found online at: https://www.frontiersin.org/articles/10.3389/fsufs.2022.868870/full#supplementary-material
Aggarwal, P. K., Jarvis, A., Campbell, B. M., Zougmoré, R. B., Khatri-Chhetri, A., Vermeulen, S. J., et al. (2018). The climate-smart village approach: Framework of an integrative strategy for scaling up adaptation options in agriculture. Ecol. Soc. 23, 14. doi: 10.5751/ES-09844-230114
Aguilera, G., Riggi, L., Miller, K., Roslin, T., and Bommarco, R. (2021). Organic fertilisation enhances generalist predators and suppresses aphid growth in the absence of specialist predators. J. Appl. Ecol. 58, 1455–1465. doi: 10.1111/1365-2664.13862
Altieri, M. A., Wilson, R. C., and Schmidt, L. L. (1985). The effects of living mulches and weed cover on the dynamics of foliage- and soil-arthropod communities in three crop systems. Crop. Prot. 4, 201–213. doi: 10.1016/0261-2194(85)90018-3
Beckmann, M., Gerstner, K., Akin-Fajiye, M., Ceausu, S., Kambach, S., Kinlock, N. L., et al. (2019). Conventional land-use intensification reduces species richness and increases production: a global meta-analysis. Glob. Chang Biol. 25, 1941–1956. doi: 10.1111/gcb.14606
Bommarco, R., Vico, G., and Hallin, S. (2018). Exploiting ecosystem services in agriculture for increased food security. Glob. Food Sec. 17, 57–63. doi: 10.1016/j.gfs.2018.04.001
Brown, M. W., and Tworkoski, T. (2004). Pest management benefits of compost mulch in apple orchards. Agric. Ecosyst. Environ. 103, 465–472. doi: 10.1016/j.agee.2003.11.006
Buchhorn, M., Lesiv, M., Tsendbazar, N. -E., Herold, M., Bertels, L., and Smets, B. (2020). Copernicus global land cover layers-collection 2. Remote Sens. 12, 1044. doi: 10.3390/rs12061044
Chandra, A., McNamara, K. E., and Dargusch, P. (2018). Climate-smart agriculture: perspectives and framings. Clim. Policy 18, 526–541. doi: 10.1080/14693062.2017.1316968
Civitello, D. J., Cohen, J., Fatima, H., Halstead, N. T., Liriano, J., McMahon, T. A., et al. (2015). Biodiversity inhibits parasites: Broad evidence for the dilution effect. Proc. Natl. Acad. Sci. U. S. A. 112, 8667–8671. doi: 10.1073/pnas.1506279112
Crowder, D. W., Northfield, T. D., Strand, M. R., and Snyder, W. E. (2010). Organic agriculture promotes evenness and natural pest control. Nature 466, 109–112. doi: 10.1038/nature09183
Dainese, M., Martin, E. A., Aizen, M. A., Albrecht, M., Bartomeus, I., Bommarco, R., et al. (2019). A global synthesis reveals biodiversity-mediated benefits for crop production. Sci. Adv. 5, 1–14. doi: 10.1126/sciadv.aax0121
Dang, H. A. H., and Dabalen, A. L. (2017). Is Poverty in Africa Mostly Chronic Or Transient? Evidence from Synthetic Panel Data. World Bank Gr. 8033, 1–45. doi: 10.1596/1813-9450-8033
Dinesh, D., Frid-Nielsen, S., Norman, J., Mutamba, M., Rodriguez, A. M. L., and Campbell, B. (2015). Climate-smart agriculture effective? A review of selected cases. CGIAR Res. Program Clim. Chang Agric. Food Secur. Work Pap. 129, 89.
Duarte, G. T., Santos, P. M., Cornelissen, T. G., Ribeiro, M. C., and Paglia, A. P. (2018). The effects of landscape patterns on ecosystem services: meta-analyses of landscape services. Landsc. Ecol. 33, 1247–1257. doi: 10.1007/s10980-018-0673-5
Essl, F., Dullinger, S., Rabitsch, W., Hulme, P. E., Pyšek, P., Wilson, J. R. U., et al. (2015). Delayed biodiversity change: no time to waste. Trends Ecol. Evol. 30, 375–378. doi: 10.1016/j.tree.2015.05.002
Fanelli, R. M. (2020). The spatial and temporal variability of the effects of agricultural practices on the environment. Environments 7, 33. doi: 10.3390/environments7040033
Fankhauser, S., and McDermott, T. K. J. (2014). Understanding the adaptation deficit: Why are poor countries more vulnerable to climate events than rich countries? Glob. Environ. Chang 27, 9–18. doi: 10.1016/j.gloenvcha.2014.04.014
Habel, J. C., Seibold, S., Ulrich, W., and Schmitt, T. (2018). Seasonality overrides differences in butterfly species composition between natural and anthropogenic forest habitats. Anim. Conserv. 21, 405–413. doi: 10.1111/acv.12408
Hickey, J. T., and Salas, J. D. (1995). Environmental effects of extreme floods. US- Italy Res Work Hydrometeorol Impacts Manag Extrem Floods 2, 1–23.
Hooks, C. R. R., Valenzuela, H. R., and Defrank, J. (1998). Incidence of pests and arthropod natural enemies in zucchini grown with living mulches. Agric. Ecosyst. Environ. 69, 217–231. doi: 10.1016/S0167-8809(98)00110-8
Johnson, A. C., and Gurr, G. M. (2016). Invertebrate pests and diseases of sweetpotato (Ipomoea batatas): A review and identification of research priorities for smallholder production. Ann. Appl. Biol. 168, 291–320. doi: 10.1111/aab.12265
Karp, D. S., Chaplin-Kramer, R., Meehan, T. D., Martin, E. A., DeClerck, F., Grab, H., et al. (2018). Crop pests and predators exhibit inconsistent responses to surrounding landscape composition. Proc. Natl. Acad. Sci. 115, E7863–E7870. doi: 10.1073/pnas.1800042115
Khan, Z., Midega, C., Pittchar, J., Pickett, J., and Bruce, T. (2011). Push-pull technology: a conservation agriculture approach for integrated management of insect pests, weeds and soil health in Africa. Int. J. Agric. Sustain. 9, 162–170. doi: 10.3763/ijas.2010.0558
Landis, D. A., Wratten, S. D., and Gurr, G. M. (2000). Habitat management to conserve natural enemies of arthropod pests in agriculture. Annu. Rev. Entomol. 45, 175–201. doi: 10.1146/annurev.ento.45.1.175
Letourneau, D. K., and Goldstein, B. (2001). Pest damage and arthropod community structure in organic vs. conventional tomato production in California. J. Appl. Ecol. 38, 557–570. doi: 10.1046/j.1365-2664.2001.00611.x
Lipper, L., McCarthy, N., Zilberman, D., Asfaw, S., and Branca, G. (2018). Climate smart agriculture building resilience to climate change. Springer Nat. 1, 629. doi: 10.1007/978-3-319-61194-5
Lobell, D. B., Schlenker, W., and Costa-Roberts, J. (2011). Climate trends and global crop production since 1980. Science 333, 616–620. doi: 10.1126/science.1204531
Lundgren, J. G., and Fausti, S. W. (2015). Trading biodiversity for pest problems. Sci. Adv. 1, e1500558. doi: 10.1126/sciadv.1500558
MacFall, J., Lelekacs, J. M., LeVasseur, T., Moore, S., and Walker, J. (2015). Toward resilient food systems through increased agricultural diversity and local sourcing in the Carolinas. J. Environ. Stud. Sci. 5, 608–622. doi: 10.1007/s13412-015-0321-1
Madeira, F., Tscharntke, T., Elek, Z., Kormann, U. G., Pons, X., Rösch, V., et al. (2016). Spillover of arthropods from cropland to protected calcareous grassland – the neighbouring habitat matters. Agric. Ecosyst. Environ. 235, 127–133. doi: 10.1016/j.agee.2016.10.012
Martin, E. A., Reineking, B., Seo, B., and Steffan-Dewenter, I. (2013). Natural enemy interactions constrain pest control in complex agricultural landscapes. Proc. Natl. Acad. Sci. U. S. A. 110, 5534–5539. doi: 10.1073/pnas.1215725110
Massaccesi, L., Rondoni, G., Tosti, G., Conti, E., Guiducci, M., and Agnelli, A. (2020). Soil functions are affected by transition from conventional to organic mulch-based cropping system. Appl. Soil Ecol. 153, 1:12. doi: 10.1016/j.apsoil.2020.103639
Mkenda, P. A., Ndakidemi, P. A., Mbega, E., Stevenson, P. C., Arnold, S. E. J., Gurr, G. M., et al. (2019). Multiple ecosystem services from field margin vegetation for ecological sustainability in agriculture: Scientific evidence and knowledge gaps. PeerJ. 2019, 17:49. doi: 10.7717/peerj.8091
Moi, D. A., Ernandes-Silva, J., Baumgartner, M. T., and Mormul, R. P. (2020). The effects of river-level oscillations on the macroinvertebrate community in a river–floodplain system. Limnology 21, 219–232. doi: 10.1007/s10201-019-00605-y
Neufeldt, H., Jahn, M., Campbell, B. M., Beddington, J. R., DeClerck, F., De Pinto, A., et al. (2013). Beyond climate-smart agriculture: toward safe operating spaces for global food systems. Agric. Food Secur. 2, 2:12. doi: 10.1186/2048-7010-2-12
Newbold, T. (2018). Future effects of climate and land-use change on terrestrial vertebrate community diversity under different scenarios. Proc. R.Soc.B Biol. Sci. 285, 20180792. doi: 10.1098/rspb.2018.0792
Nunez, S., Arets, E., Alkemade, R., Verwer, C., and Leemans, R. (2019). Assessing the impacts of climate change on biodiversity: is below 2 C enough? Clim. Change 154, 351–365. doi: 10.1007/s10584-019-02420-x
Ongawa (2019). The integrated approaches for climate change adaptation in the East Usambara Mountains. Glob. Clim. Chang Alliance 1, 50.
Pecl, G. T., Araújo, M. B., Bell, J. D., Blanchard, J., Bonebrake, T. C., Chen, I. C., et al. (2017). Biodiversity redistribution under climate change: Impacts on ecosystems and human well-being. Science 355, 1389:1399. doi: 10.1126/science.aai9214
Porter, J. R., Xie, L., Challinor, A. J., Cochrane, K., Howden, S. M., Iqbal, M. M., et al. (2015). Food security and food production systems. Clim Chang 2014 Impacts, Adapt Vulnerability Part A Glob Sect Asp AR5, 485–534.
Qi, J., Yu, Y., Wang, L., Liu, J., and Wang, Y. (2017). An effective and efficient hierarchical K-means clustering algorithm. Int. J. Distrib. Sens. Netw. 13, 1–17. doi: 10.1177/1550147717728627
R Core Team (2017). R: A Language and Environment for Statistical Computing. R. Found Stat. Comput. 55, 275–286.
Ray, D. K., West, P. C., Clark, M., Gerber, J. S., Prishchepov, A. V., and Chatterjee, S. (2019). Climate change has likely already affected global food production. PLoS ONE 14, 1–18. doi: 10.1371/journal.pone.0217148
Real-Santillán, R. O., del-Val, E., Cruz-Ortega, R., Contreras-Cornejo, H. Á., González-Esquivel, C. E., and Larsen, J. (2019). Increased maize growth and P uptake promoted by arbuscular mycorrhizal fungi coincide with higher foliar herbivory and larval biomass of the Fall Armyworm Spodoptera frugiperda. Mycorrhiza 29, 615–622. doi: 10.1007/s00572-019-00920-3
Redlich, S., Martin, E. A., and Steffan-Dewenter, I. (2018). Landscape-level crop diversity benefits biological pest control. J. Appl. Ecol. 55, 2419–2428. doi: 10.1111/1365-2664.13126
Rehman, M., Liu, J., Johnson, A. C., Dada, T. E., and Gurr, G. M. (2019). Organic mulches reduce crop attack by sweetpotato weevil (Cylas formicarius). Sci. Rep. 9, 1:12. doi: 10.1038/s41598-019-50521-5
Rodriguez-Saona, C., and Thaler, J. S. (2005). Herbivore-induced responses and patch heterogeneity affect abundance of arthropods on plants. Ecol. Entomol. 30, 156–163. doi: 10.1111/j.0307-6946.2005.00682.x
Rosenstock, T. S., Nowak, A., and Girvetz, E. (2019). The climate-smart agriculture papers - investigating the business of a productive, resilient and low emission future. Springer Nat. 1, 197. doi: 10.1007/978-3-319-92798-5
Rusch, A., Chaplin-Kramer, R., Gardiner, M. M., Hawro, V., Holland, J., Landis, D., et al. (2016). Agricultural landscape simplification reduces natural pest control: a quantitative synthesis. Agric. Ecosyst. Environ. 221, 198–204. doi: 10.1016/j.agee.2016.01.039
Sarthou, J. P., Badoz, A., Vaissière, B., Chevallier, A., and Rusch, A. (2014). Local more than landscape parameters structure natural enemy communities during their overwintering in semi-natural habitats. Agric. Ecosyst. Environ. 194, 17–28. doi: 10.1016/j.agee.2014.04.018
Scheiner, C., and Martin, E. A. (2020). Spatiotemporal changes in landscape crop composition differently affect density and seasonal variability of pests, parasitoids and biological pest control in cabbage. Agric. Ecosyst. Environ. 301, 107051. doi: 10.1016/j.agee.2020.107051
Schmidt, M. H., Thewes, U., Thies, C., and Tscharntke, T. (2004). Aphid suppression by natural enemies in mulched cereals. Entomol. Exp. Appl. 113, 87–93. doi: 10.1111/j.0013-8703.2004.00205.x
Schulz-Kesting, K., Thiele, J., Everwand, G., and Dauber, J. (2021). Neighbourhood effect of faba bean (Vicia faba L.) on density of vegetation-dwelling natural biocontrol agents in winter wheat. Biol. Control 160, 104673. doi: 10.1016/j.biocontrol.2021.104673
Staton, T., Walters, R. J., Smith, J., Breeze, T. D., and Girling, R. D. (2021). Evaluating a trait-based approach to compare natural enemy and pest communities in agroforestry vs. arable systems. Ecol. Appl. 31, e02294. doi: 10.1002/eap.2294
Taylor, M. (2018). Climate-smart agriculture: what is it good for? J. Peasant Stud. 45, 89–107. doi: 10.1080/03066150.2017.1312355
Thierfelder, C., Rusinamhodzi, L., Setimela, P., Walker, F., and Eash, N. S. (2016). Conservation agriculture and drought-tolerant germplasm: Reaping the benefits of climate-smart agriculture technologies in central Mozambique. Renew. Agric. Food Syst. 31, 414–428. doi: 10.1017/S1742170515000332
Thornton, P. K., Whitbread, A., Baedeker, T., Cairns, J., Claessens, L., Baethgen, W., et al. (2018). A framework for priority-setting in climate smart agriculture research. Agric. Syst. 167, 161–175. doi: 10.1016/j.agsy.2018.09.009
van Wijk, M. T., Merbold, L., Hammond, J., and Butterbach-Bahl, K. (2020). Improving assessments of the three pillars of climate smart agriculture: current achievements and ideas for the future. Front. Sustain. Food Syst. 4, 558483. doi: 10.3389/fsufs.2020.558483
Venkatramanan, V., and Shah, S. (2019). Climate-smart agriculture technologies for environmental management: The intersection of sustainability, resilience, wellbeing and development. Sustain. Green Technol. Environ. Manag. 1, 29–51. doi: 10.1007/978-981-13-2772-8_2
Woodcock, B. A., Bullock, J. M., McCracken, M., Chapman, R. E., Ball, S. L., Edwards, M. E., et al. (2016). Spill-over of pest control and pollination services into arable crops. Agric. Ecosyst. Environ. 231, 15–23. doi: 10.1016/j.agee.2016.06.023
Keywords: crop pests, natural enemies, food security, neighborhood effects, East Usambara Mountains, Tanzania, Africa
Citation: Tripathi HG, Kunin WE, Smith HE, Sallu SM, Maurice S, Machera SD, Davies R, Florence M, Eze S, Yamdeu JHG and Sait SM (2022) Climate-Smart Agriculture and Trade-Offs With Biodiversity and Crop Yield. Front. Sustain. Food Syst. 6:868870. doi: 10.3389/fsufs.2022.868870
Received: 03 February 2022; Accepted: 16 May 2022;
Published: 24 June 2022.
Edited by:
Wen Xie, Insititute of Vegetables and Flowers (CAAS), ChinaReviewed by:
Fang Ouyang, Institute of Zoology (CAS), ChinaCopyright © 2022 Tripathi, Kunin, Smith, Sallu, Maurice, Machera, Davies, Florence, Eze, Yamdeu and Sait. This is an open-access article distributed under the terms of the Creative Commons Attribution License (CC BY). The use, distribution or reproduction in other forums is permitted, provided the original author(s) and the copyright owner(s) are credited and that the original publication in this journal is cited, in accordance with accepted academic practice. No use, distribution or reproduction is permitted which does not comply with these terms.
*Correspondence: Hemant G. Tripathi, aGd0cmlwYXRoaTA1QGdtYWlsLmNvbQ==
Disclaimer: All claims expressed in this article are solely those of the authors and do not necessarily represent those of their affiliated organizations, or those of the publisher, the editors and the reviewers. Any product that may be evaluated in this article or claim that may be made by its manufacturer is not guaranteed or endorsed by the publisher.
Research integrity at Frontiers
Learn more about the work of our research integrity team to safeguard the quality of each article we publish.