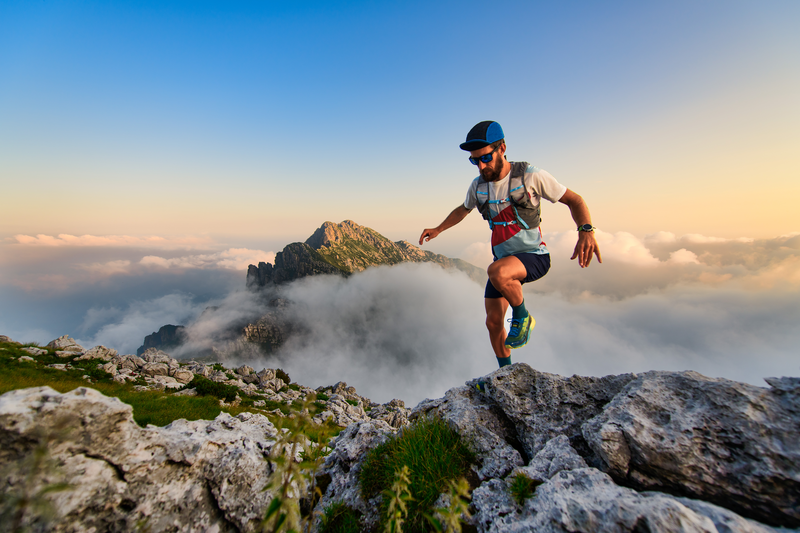
94% of researchers rate our articles as excellent or good
Learn more about the work of our research integrity team to safeguard the quality of each article we publish.
Find out more
REVIEW article
Front. Sustain. Food Syst. , 25 April 2022
Sec. Crop Biology and Sustainability
Volume 6 - 2022 | https://doi.org/10.3389/fsufs.2022.867897
Malnutrition, unhealthy diets, and lifestyle changes are the major risk factors for overweight and obesity-linked chronic diseases in humans adversely impact achieving sustainable development goals. Colored grains are a source of anthocyanins, a group of flavonoids, that contribute positively to human health. This review focuses on genetic variation harnessed through breeding and biotechnology tools for developing anthocyanin-rich grain crops. Agronomic practices, genotype × environment interactions, different stresses, seed development and seed maturity are factors that impact the content and composition of anthocyanins. Significant progress has been made in characterizing genes associated with anthocyanin biosynthesis in cereal and other crops. Breeding has led to the development and release of grain anthocyanin-rich crop cultivars in Europe, America and in some countries in Asia. Notably, genetic engineering utilizing specific transcription factors and gene editing has led to the development of anthocyanin-rich genetic variants without any significant yield penalty. A variety of food products derived from colored grains or flours are now available in grocery stores and supermarkets worldwide. The public perception about anthocyanin-rich food is positive, but availability, affordability, and willingness to pay a higher price than before limit consumption. Together with other seed nutrition traits in breeding programs the inclusion of anthocyanins can ensure the development of cultivars that meet nutrition needs of humans, especially in the developing world.
Overweight and obesity are the major risk factors associated with chronic diseases (for instance, cardiovascular disease, cancer, and diabetes). Cereals are the main source of dietary energy, essential proteins and micronutrients, vitamins, and diverse non-nutrient phytochemicals (bioactive compounds). Consumption of dietary fiber and phytochemicals in whole cereal grains contributes positively to combating chronic diseases. Bioactive compounds in phytochemicals have antioxidant, anticarcinogenic, anti-inflammatory, and anti-microbial properties. Undernutrition and micronutrient deficiencies afflict the developing world where healthy diets are not always affordable. Moreover, obesity and chronic diseases continue to rise worldwide among individuals, households, regions, nations, and continents. Cereal crops pyramided with dense nutrients and phytochemicals hold great promise to meet the growing demand of nutritionally limited populations to achieve sustainable nutrition security (Yu and Tian, 2018).
Food systems include the interactions between agriculture and human health through nutrition. Multi-dietary components of cereals have promise to overcome undernutrition (micronutrient malnutrition, overweight/obesity, chronic diseases) while such a food system research approach from farm to metabolism toward promoting human health and physiological processes is important (Bach Knudsen et al., 2017; Poole et al., 2021a,b). The evidence to date suggests that a cause-effect relationship between regular intake of anthocyanin-rich food and management of overweight and obesity to reduce the risk of chronic diseases seems promising (Araki et al., 2021; Park et al., 2021; Tian et al., 2021; Xu et al., 2021; Zhao et al., 2021; also see Table 1).
Anthocyanins and anthocyanidins are plant pigments. The basic core structure of both the compounds is ionic flavylium (2-phenylchromenylium). Anthocyanin is a water-soluble vacuoles pigment whereas anthocyanidin is the sugar-free counterpart of anthocyanin. Anthocyanins are the largest group of water-soluble pigments found in plant vacuoles that display a range of red, purple, black or blue colors in grains, fruits, and vegetables. Anthocyanins derived from anthocyanidins are the glycoside-form of anthocyanidin, whereas anthocyanidins are aglycones. Anthocyanins esterified with one or more acid groups are referred to as acylated anthocyanins. There characterization as acylated or non-acylated anthocyanins is based on the presence or absence of acyl group. Acylated anthocyanins are further classified as mono-, di-, tri-, and tetra-acylated anthocyanins. The color and stability of anthocyanins depend on pH, light, temperature, and structure. Anthocyanins in acidic condition are red but when pH is increased, they turn blue (Khoo et al., 2017; Vidana Gamage et al., 2022). Differences in pH (1, 3, 5, 7, 9, 11, 13), temperature (5 and 30°C), and exposure to light have been used for measuring stability of anthocyanins (Hu et al., 2014). The acidic conditions favor stability, while as when pH of the solution is increased, anthocyanins became unstable. The acylated anthocyanins are more stable than non-acylated anthocyanins. Anthocyanins with powerful radical scavenging activity display stronger antioxidant capacity. The structures of aglycone and acyl group greatly affect the stability and antioxidant capacity of anthocyanins. Both acylated and non-acylated forms of anthocyanins are present in grains, fruits, and vegetables (Vidana Gamage et al., 2022).
The focus of this review is on variable anthocyanin germplasm, their basis of inheritance, identification of their candidate genes, related single nucleotide polymorphisms (SNPs), and development of anthocyanin-rich cereal grains by crossbreeding including biotech-aided approaches. In addition, the effects of environment and cultivation practices on anthocyanin content and composition, model food for assessment of diet-health complexities, as well as their acceptance by consumers' and affordability as food are highlighted.
Cultivation practices, agroecological differences, abiotic stress, and plant development (particularly the reproductive stage, seed development and maturity) impact in anthocyanin metabolism in plants. Anthocyanin content in the seed of purple waxy maize “Heukjinjuchal” during grain filling increased while the seed color darkened. The relative composition of the anthocyanins varies with the developmental stage. Cyanidin-3-O-glucoside can range from 57 to 409 mg kg−1 in freshly harvested seed and 1,027 mg kg−1 in dry seeds, while pelargonidin-3-β-O-glucoside and malvidin-3-β-O-glucoside are detectable at 21 days after silking (DAS) (Kim et al., 2020). Maize harvested after 26 and 29 DAS of “Heukjinjuchal” had the highest concentration of amino acids and anthocyanins, respectively (Kim et al., 2020). Maximum anthocyanin concentration (51 mg g−1) was detected at 23 to 28 DAS in “super sweet sweetcorn.” The pigment coverage across the pericarp progressively increased from a small spot at the stigma end of the kernel to gradual spread over the entire kernel (Hong et al., 2020). A comparative study involving black seeded rice mutant “9311bk” and its wild type (WT) revealed significantly greater anthocyanin levels in “9311bk” than the WT. In another study, anthocyanin accumulation in “9311bk” increased progressively along with seed development, with the highest level found at seed maturity (Zaidi et al., 2019). On the other hand, early harvest of anthocyanin-rich purple wheat yielded 39% more anthocyanins than at normal harvest (Bustos et al., 2012). Assessing the effect of source-sink ratio and grain position in ear head of purple wheat revealed reduced anthocyanin content in grains at distal positions, while a higher source-sink ratio also increased anthocyanin content, largely due to differences in physiological maturity (Bustos et al., 2012).
Differences in environments due to variation in light intensity, photoperiod, temperature, soil fertility or moisture levels impact anthocyanin content in plants. Genotype, location, and genotype × location were found to significantly affect the content and composition of anthocyanins in wheat (Abdel-Aal et al., 2016). High temperature led to decreased anthocyanin levels in black seeded rice or purple skin- and fleshed-potato by ~12% (Zaidi et al., 2019). Abiotic stresses, including cold, drought, heat, salinity, shading, and light intensity increased anthocyanins to varying levels in colored-grain rice and wheat (Chunthaburee et al., 2015; Li et al., 2018a), while heat stress increased anthocyanins in purple wheat (Hosseinian et al., 2008; Li et al., 2018a). Fertility levels, particularly N, Zn, or Mn application, have been shown to affect anthocyanin content. For example, appropriate dose of N and Zn fertilizers increased grain yield and anthocyanins in purple grain rice (Fongfon et al., 2021). Blue wheat accessions had higher content of anthocyanins across all N levels, while purple wheats accumulated significantly higher anthocyanin content under low N stress (Fan et al., 2020). The application of N fertilizer, however, is beneficial to maximize productivity of modern wheats harboring “Green Revolution” genes. Magnesium fertilization increases anthocyanin content (Bustos et al., 2012) and ameliorates the deleterious effect of elevated temperature on anthocyanins (Guo et al., 2016; Ciscomani-Larios et al., 2021). Likewise, the interaction between anthocyanin biosynthesis genes and abiotic stresses on one hand and variation in daily and seasonal rhythms in response to differences in temperature, light, humidity, and precipitation on the other have profound effects on phytochemical composition in plants (Azuma et al., 2012; Li et al., 2018a; Liebelt et al., 2019; Saad et al., 2021). Thus, understanding how phytochemical composition varies based on time is critical to unlock underlying regulatory connections to improve the quality of phytochemical compounds (Liebelt et al., 2019).
Clearly, environment, genotype × environment interaction, and abiotic stresses affect the content and composition of anthocyanins. Therefore, multi-site testing across diverse agro-ecologies is recommended to identify stable and anthocyanins-rich germplasm for use in crop breeding and to identify sites more favorable for production of anthocyanins-rich crops.
Germplasm is the main source for crop improvement programs because it represents a large pool of genetic diversity. Researchers can use it to draw allelic variation for developing new cultivars with significant nutrient and health benefits.
A variety of grain colors (purple, blue, black, and white) of anthocyanins have been observed in barley, rice, and wheat, with white or brown grains being predominantly used for food purpose. Both maize and sorghum have a variety of seed colors from black, blue, brown, and pink to purple and red. Anthocyanins are localized in outer layers of the grains, for example, anthocyanin in purple grains is localized in pericarp while in blue grains it is localized in aleurone layer. Both aleurone and pericarp layers contain anthocyanins in black or red grains (Abdel-Aal et al., 2012; Francavilla and Joyé, 2020).
Table 2 summarizes various reports that have revealed multi-fold differences for anthocyanin content in grain crop germplasm. Colored-seeded (black, blue, purple) barley germplasm was found to have substantial variation in anthocyanin content (13–1037.8 μg g−1 dry weight, DW), with purple and blue barley containing on average higher anthocyanin content than black barley. Predominant anthocyanin in the purple barley is cyanidin 3-glucoside, whereas in the blue and black barley it is delphinidin 3-glucoside (Kim et al., 2007). Hull-less barley grains (known as “qingke” in Chinese) is a valuable food in Tibet. Tibetan Qingke cultivars (black, blue, white) differ substantially in total phenolics, flavonoids, proanthocyanidins, anthocyanins, and antioxidant capacity. “Black Qingke” cultivar has highest concentrations of these compounds followed by blue and white Qingke cultivars (Lin et al., 2018). In purple qingke (POK) and black qingke (BOK) cultivars, six anthocyanins with most acylated types have been reported. The total anthocyanin content in POK and BOK ranged from 14 to 2,304 μg g−1 and 248.7 to 2,902.9 μg g−1 DW, respectively (Zhang et al., 2021).
Table 2. Natural genetic variation for total anthocyanin content in barley, maize, rice, sorghum, and wheat germplasm pools.
Landraces are dynamic population(s) of a crop that have a historic origin and distinct identity that was locally adapted in association with traditional farming systems. The maize landrace (conical cob) from San Juan Ixtenco, “Tlaxcala,” Mexico represents an important genetic resource rich in bioactive compounds (Mendoza-Mendoza et al., 2017). This type of maize has a high capacity to synthesize and accumulate anthocyanins both in the corncob and the kernel. The pericarp has the highest concentration of anthocyanins (0.2529–2.6452 g 100 g−1 DW) followed by the cob (0.1004–1.1022 g 100 g−1 DW), kernel (0.0398–0.2398 g 100 g−1 DW), and the aleurone layer (0.0044–0.0523 g 100 g−1 DW), respectively (Mendoza-Mendoza et al., 2020). Anthocyanin content in some populations, particularly those with dark color of the kernel and corncob, surpassed the quantity of anthocyanins in the best-known Peruvian purple maize.
Comparative profiling of colored-grain Mexican maize landraces indicated presence of 18 different anthocyanins with cyanidin-based anthocyanins being predominant in dark purple and pelargonidins in red-pink kernels (Peniche-Pavía and Tiessen, 2020). Anthocyanin content was highest in pericarp-pigmented accessions with flavanol–anthocyanin condensed forms, similar to the Andean “Maiz Morado” landraces (Paulsmeyer et al., 2017). Among US southwestern blue maize landraces, five-fold differences averaged across locations 0.43 g kg−1 kernel tissue, with “Santa Clara Blue,” “Yoeme Blue,” “Ohio Blue,” and “Hopi Blue” in the range between 0.52 and 0.58 g kg−1. The predominant anthocyanins were cyanidin 3-glucoside, pelargonidin and peonidin 3-glucoside (Nankar et al., 2016b). “Millo Corvo,” an ancient-colored landrace from Galicia (Spain), is a good source of high amounts of anthocyanins (83.4 mg 100 g−1 flour), particularly predominant in cyanidin (Lago et al., 2015). Traditional farmers in the USA and Mexico borderland region cultivate many types of maize landraces with diverse kernel characteristics reflecting their enculturated preferences. Assessment of 48 diverse accessions of 18 maize races originating from this borderland region indicated a wide range of variation in grain anthocyanin content across and within populations, some with anthocyanin concentration above 50 mg 100g−1 (Ryu et al., 2013). Accession refers to a distinct, uniquely identifiable sample of seeds representing a cultivar, breeding line or a population.
Colored maize grains, preferably blue or purple, are often used to prepare traditional and diverse dishes in Mexico. Assessment of pigmented maize landraces in Mexico revealed that magenta colored maize grains contain anthocyanins in the pericarp and the aleurone layer, while blue and purple maize grains are found in the aleurone layer. The total anthocyanin content in magenta grains was up to 10-fold greater than blue or purple grains with 9–11 anthocyanin derivatives: cyanidin, peonidin and pelargonidin, being the major anthocyanins. The blue and purple maize grains have similar profiles to magenta grains with predominantly mono acylated derivatives of cyanidin (Salinas-Moreno et al., 2013). Among the pigmented native landraces (Olotillo, Olotón, Tehua, Tepecintle, Tuxpeño, Vandeño, Zapalote Grande) of maize in Mexican province of Chiapas, blue-grain types were the most common followed by light red and purple reddish types. Blue- and red-grain type races had highest (213.6–904.0 mg of cyanidin 3–glucoside equiv/kg of sample) and lowest (30.7–188.1 mg of pelargonidin chloride equiv/kg of sample) range variation for anthocyanin content, respectively. Of these, Olotillo could be a better choice for preparation of food products because of its high anthocyanin content together with hard texture (Salinas-Moreno et al., 2012).
Rice germplasm from South and Southeast Asia have multi-fold differences in anthocyanins (Chen et al., 2012; Hosoda et al., 2018). Cyanidin 3-glucoside, peonidin 3-glucoside, cyanidin diglucoside, and malvidin are the predominant anthocyanins in black- and red-seeded rice. Aromatic rice “Chakhao” from the northeastern Indian state Manipur is a speciality glutinous rice. Molecular analysis of “Chakhao” landraces differing in seed color (purple and non-purple) grouped 93 accessions into three subpopulations, with deep purple type forming a distinct cluster. The predominant anthocyanins were cyanidin 3-O-glucoside and peonidin 3-O-glucoside (Bhuvaneswari et al., 2020).
Anthocyanin content among 25 sorghum assessions differing in pericarp pigments varied from 575 to 1,461 g kg−1 seed. The red and brown pericarp accessions PI297139 and PI221723, respectively, had the highest amounts of anthocyanins: 1,461 and 1,376 g kg−1 seed with 3-deoxyanthocyanidins being predominant (Su et al., 2017).
Introgression refers to the transfer of genetic information from one species to another via hybridization between them and repeated backcrossing. Purple- and blue-grain wheat germplasm, the former from a tetraploid wheat from Ethiopia and the latter from the introgression of a diploid wheat with Agropyron elongatum resulted in both purple and blue grains in hexaploid wheat (Zeven, 1991). Comparative assessment of black, blue and purple wheat germplasm from India revealed delphinidin-3-o-galactoside, delphinidin-3-o-glucoside, and cyanindin-3-o-glucoside as major anthocyanins (Sharma et al., 2020a). Significantly higher anthocyanin content was detected in gamma rays-induced mutants (L47, L167, L925) than WT, while K4191, a purple-seeded wheat germplasm, and L925 having highest total anthocyanin content with cyanidin-3-glucoside being the most predominant anthocyanin (Hong et al., 2019). The evaluation of 76 durum and bread wheats for over three seasons revealed that blue-aleurone bread wheat germplasm contains higher anthocyanins than purple- and red-pericarp durum wheats, with 5–8 major anthocyanins in blue-wheat extract and three-anthocyanins in purple and red wheats. Delphinidin 3-O-rutinoside, delphinidin 3-O-glucoside, and malvidin 3-O-glucoside were predominant in blue wheat and cyanidin 3-O-glucoside, peonidin 3-O-galactoside, and malvidin 3-O-glucoside in purple wheat (Ficco et al., 2014). Supplementary Table 1 contains a few germplasm lines (cultivar, breeding lines, genetic stocks, landraces, cultivars) with highest and lowest anthocyanin contents which are useful in breeding and genetics of anthocyanin in barley, maize, rice, sorghum, and wheat. Genetic stocks represent a unique germplasm (cytological stocks, mutants, germplasm sets) of either transient or long-lasting value. Cultivar refers to a cultivated variety; selected and cultivated by humans, while in nature, population of plants within a species differs in some significant way from other members of that species, is known as a variety by botanist. Advanced lines originating from crossbreeding are designated as breeding lines.
Assessing the composition, stability and profiling of colored grains is critical for consumption of anthocyanin-rich foods. Metabolite profiling of hull-less barley cultivars differing in grain colors presented 608 metabolites. Among these ABC transporters, flavonoid biosynthesis and anthocyanin biosynthesis pathways were found to be the predominant metabolic pathways. Colored barley cultivars clustered distinct from white barley (Liang et al., 2021).
Total anthocyanin content in pericarp and aleurone layer among four native maize landraces from “Arrocillo,” “Cónico,” “Peruano,” and “Purepecha” ranged from 54 to 115 mg 100g−1 DW, with similar anthocyanin profiles but differing in relative percentages (Salinas-Moreno et al., 2005). Biochemical attributes of blue and purple maize landraces of southwestern United States revealed that a majority of blue landraces had higher oil and protein contents, while a few were richer in lysine and methionine than the open pollinated variety (OPV) (Nankar et al., 2016a). OPV refers to a type of cultivars originated from open pollination of a set of individuals possessing high genetic diversity, which enable such OPV to adapt to diverse environments. The pericarp-pigmented maize cultivars contain cyanidin-derived anthocyanins that produce blue, red or pink extracts. Maize lines derived from landrace Apache Red (AR) predominantly contained pelargonidin-derived anthocyanins in the pericarp (Chatham et al., 2018). Two new flavanol-anthocyanins, catechin-(4,8)-pelargonidin 3,5-diglucoside and afzelechin-(4,8)-pelargonidin 3,5-diglucoside, in addition to several apigenin-derived C-glycosyl flavones in abundance were also identified. These findings suggest that AR is an important genetic resource for studying flavonoid biosynthesis pathway.
In amber and pigmented (black, red) rice grains about 110 metabolites were found, with black and red rice containing greater levels of flavonoids. Black rice also contained high levels of carotenoids, tocopherols, phytosterols, and monoterpenes, while non-pigmented grains were low in secondary metabolites. The metabolite clusters were based on N and C sources, with black rice rich in C-based secondary metabolites and low in primary metabolites compared with other rice grains. The complex interactions between C and N metabolism of primary and secondary metabolites may contribute to developing new rice cultivars with specific metabolites (Kim et al., 2021).
In advanced breeding lines derived from crosses involving exotic donor lines (blue or purple grains), 22, 23, and 26 different anthocyanins were present in blue, purple and black wheat grains, respectively, with anthocyanin content ranging from 16 to 198 mg kg−1 grains (Garg et al., 2016). The blue (“Skorpion”) and purple (“Jakubz”) wheat cultivars contained 19 and 26 anthocyanins each, with former containing 9.26 mg kg−1 and the latter 13.23 mg kg−1 of total anthocyanins (Bartl et al., 2015). Cyanidin 3-glucoside, cyanidin 3-rutinoside, and peonidin 3-rutinoside were present in both wheats; while delphinidin 3-glucoside and delphinidin 3-rutinoside were present only in blue wheat; and pelargonidin 3-glucoside, pelargonidin 3-rutinoside, and peonidin 3-glucoside present only in purple wheat.
The profiling of anthocyanins in processed bran fraction of purple wheat identified a complex of 5 pigments, including cyanidin 3-glucoside, cyanidin-3-(6-malonyl glucoside), cyanidin 3-rutinoside, peonidin 3-glucoside, and peonidin-3-(6-malonylglucoside), which contributed to 93–96% of the total anthocyanins. Purple wheat bran and its powder both were found to have higher antioxidant activity (Abdel-Aal and Rabalski, 2018).
Assessment of 26 pigmented native maize populations of Mexico revealed that “EO22” and “Pep20” were superior for large grains (44.84 g 100 seeds−1) and high-quality protein (protein: 9.58%; protein quality index: 0.814), tryptophan (0.0765%), and anthocyanins (276.58 mg cyanidin 3-glucoside kg−1 dry matter) (Rojas et al., 2019). The pigmented native landraces from Northeast of Mexico differed in color parameters (L*, C*, h:25.13–63.64, 2.41–33.58, 14.36–359.19, respectively) and richer in carbohydrates (71.30–74.88), protein (9.72–12.57), P (334–720 mg 100 g−1), K (314–397 mg 100 g−1), Mg (116.24–146.75 mg 100 g−1), Zn (2.8–4.5 mg 100 g−1) and Fe (0.77–2.53 mg 100 g−1) (Rodríguez-Salinas et al., 2019).
Flour of purple grain of an Abyssinian wheat landrace from Ethiopia was found to contain 197 mg 100 g−1 anthocyanins together with a distribution of 5.5% ash, 8.5% protein, 3% fat, 7.3% fiber, and 64.8% carbohydrates (Kassegn, 2018). The pigmented and amber (white) wheat had no major differences in nutritional and processing characteristics related to end use quality, but colored wheats had higher protein content and dietary fibers (Kumari et al., 2020), and higher iron and zinc content (Sharma et al., 2018). A wheat-T. boeoticum introgression line Z18-122 that originated from a cross between G52 (T. boeoticum) and Crocus (wheat) had, on an average, a slightly lower (67.24 μg g−1) content of anthocyanins than in G52 (72.31 μg g−1) but significantly higher than that in Crocus (7.81 μ g−1). The essential amino acid content was 48% higher than Crocus (16.16). Zn and Fe contents were 39% (74.5 mg kg−1) and 109% (109.7 mg kg−1) greater than Crocus (Zn, 52.4 and Fe 53.9 mg kg−1) (Liu et al., 2021a). Thus, cultivated gene pools contain usable genetic variation for anthocyanin content and composition and could be explored for developing anthocyanin-dense cereal grains. Gene pool refers to the stock of different genes in an interbreeding population, broadly grouped as primary (members intermate freely within the species), secondary (members closely related with cultivated species, can cross and produce at least some fertile hybrids), and tertiary (distantly related to the members of the primary gene pool; could be intermated with primary gene pool by using in vitro techniques to rescue F1 due to lethality and physiological abnormality of complete sterility or bridging species) gene pool.
Understanding the genetic and molecular basis and identification of genetic tags (DNA markers) associated with trait expression offers great promise to enhance crop breeding efficiency. A candidate gene is a gene identified by its position in a chromosome that relates to trait expression; however, such candidates need to be functionally validated prior to being deployed in crop breeding practices. Functionally characterized genes and those recently discovered “candidate” genes are discussed in this section (Table 3). Transcriptional regulation of anthocyanin biosynthesis involves DNA-binding R2R3 MYB factors, MYC-like helix-loop-helix, and WD40-repeat proteins (Allan et al., 2008; Matus et al., 2008; Hichri, 2010). Specificity of DNA-binding of MYB proteins and, in some instances, bHLH proteins activate subset of genes. WD40-repeat proteins have mostly a general role along the regulatory complex (Hichri, 2010). Additional insights into anthocyanin biosynthesis regulation have also been provided (Jaakola, 2013).
Table 3. Candidate or functionally characterized genes regulating anthocyanin biosynthesis in barley, maize, rice, and wheat.
Anthocyanins in the aleurone layer cause “blue” grain barley, while in the pericarp they account for purple or red barley (Lundqvist et al., 1997). The black pericarp gene (Blp) was mapped between the Indel markers MC_1570156 and MC_162350, with a physical distance of 0.807 Mb and contained 21 annotated genes on chromosome 1H. The sequencing of black and yellow barley led to discovering candidate genes associated with Blp (Long et al., 2019). Five structural (F3′M, HID, UF3GT, UFGT, 5MAT) and one regulatory (Ant1) genes conferred purple seed coat color to hulless barley (Yao et al., 2018). Blue grain barley complementary genes include Blx1, Blx3 and Blx4, which are intricately linked to one another and clustered on chromosome 4HL, with Blx2 and Blx5 on 7HL (Finch and Simpson, 1978). A regulatory complex of MYB–bHLH–WD repeat (MBW) transcription factors (MYB, bHLH/MYC, WD40) and genes HvMyc2 and HvMpc2 on chromosome 4HL and HvWD40 on 6HL regulate anthocyanin synthesis in barley aleurone layer. MBW complex is a highly conserved transcriptional complex model. It regulates WRKY protein control of the flavonoid pigment pathway in plants (Lloyd et al., 2017). The expression of HvMyc2 is confined to aleurone cells but not the non-colored aleurone tissue. Both the genes have been genetically mapped between microsatellite loci XGBS0875-4H (3.4 cM distal) and XGBM1048-4H (3.4 cM proximal) on 4H, where in the Blx-cluster was discovered. The anthocyanin biosynthesis structural gene HvF3′5′H (Strygina et al., 2017) was also mapped at this position. A trigenic cluster MbHF35 including HvMYB4H, HvMYC4H and HvF35H genes in Blx1 locus mapped to ~1.13Mb genetic interval on chromosome 4HL and confers blue color to barley grains (Jia et al., 2020). HvnHID from hull-less purple grain Tibetan barley “Nirumuzha” has also been isolated (Yao et al., 2022). The expression of key genes (HvnF3'H, HvnDRF, HvnANT1, HvnGT) in the anthocyanidin biosynthetic pathway were at higher level while HvnHID expression decreased, thereby it acts as a negative regulator of anthocyanin biosynthesis in “Nirumuzha.”
Comparative transcriptome analysis of two tissues of purple grain maize lines differing in anthocyanin accumulation (Ha0414 with purple pericarp and Ha6130 with purple aleurone layer) identified, respectively, 16 and 14 differentially expressed genes associated with the anthocyanin biosynthesis pathway. Three genes increased in transcript abundance in both the colored pericarp and the aleurone layer, while two other genes increased in transcript abundance in the aleurone layer of Ha6130. Thirty-six transcription factors (TFs)—including bHLH, WRKY and HB—in the pericarp of Hao414 and 79 TFs –including MYB, MYB-related and bHLH TFs in the aleurone layer of Ha6130 with increased transcript abundance suggest tissue-specific accumulation of anthocyanins in pericarp and aleurone layer of maize grains (Li T. et al., 2019).
A C-S-A gene model that controls seed pigmentation was proposed in rice (Sun et al., 2018). C1 encodes a R2R3-MYB transcription factor, S1 encodes a bHLH protein that functions in a tissue-specific manner, and A1 encodes a dihydroflavonol reductase. The C1 and S1 interactions activate expression of A1 to produce purple colored seed containing high accumulation of cyanidin 3-O-glucoside. Loss of function of A1 leads to a red-pigmented seed due to accumulation of hesperetin 5-O-glucoside, rutin, and delphinidin 3-O-rutinoside. Significantly higher expression levels of OsMYB3 were found in pericarps of black rice relative to white rice while knockout of OsMYB3 in black rice caused significant downregulation of 19 anthocyanin metabolites in grains (Zheng et al., 2021). Thus, OsMYB3 is an determinant R2R3-MYB gene for anthocyanin biosynthesis in rice pericarps.
A multidrug and toxic compound extrusion (MATE) gene, OsMATE34, associated with anthocyanin transport in black rice caryopsis has also been identified (Mackon et al., 2021). OsMATE34 has high similarities with other anthocyanin transporter genes, VvAM1, VvAM3, MtMATE2, SlMATE/MTP77, RsMATE8, AtFFT, and AtTT12. Further analysis involving black and white caryopsis at different time intervals after flowering identified 36,079 genes, including 33,157 known and 2,922 new genes. Of these, there were 15,573 differentially expressed genes (DEGs) that were commonly expressed while 1,804 and 1,412 genes were uniquely expressed in black and white caryopsis, respectively. OsMATE34 expression is significantly upregulated in all black caryopsis but not in white caryopsis. OsMATE34 expression is like OsGSTU34, a transporter of anthocyanin in rice leaves. Thus, OsMATE34 (Os08g0562800) is a candidate anthocyanin transporter in rice caryopsis. A novel allele of chromogen (a precursor of a biochemical pigment) gene C, OrC1, associated with anthocyanin biosynthesis in O. rufipogon has also been cloned (Qiao et al., 2021). OrC1 regulates flavonoid biosynthesis pathway and activates a few bHLH and WD40 genes of ternary MYB-bHLH-WD40 complex in indica rice. OrC1 activates the anthocyanin biosynthesis pathway genes, namely, OsCHI, OsF3H and OsANS, and produces six metabolites independently.
TaPpm1 (purple pericarp-MYB 1) and TaPpb1 (purple pericarp-bHLH 1) form a complex to co-regulate purple pericarp color in wheat. TaPpm1 is widely expressed in many tissues including pericarp, while TaPpb1 expression is limited to seeds (Jiang et al., 2018). Gene coexpression network analysis based on weighted gene and involving five stages of seeds development in purple wheat variety ZNM168 identified TraesCS1D02G019200 (TaBZ1 UniGene) as core regulatory gene and TraesCS2A01GS27700 (TaCHS) and TraesCS6B01G006200 (TrANS) as structural genes critical to anthocyanin biosynthesis in wheat (Wang et al., 2021). Blue aleurone 1 (Ba1) confers blue grain color to bread wheat. A comparative assessment of blue and white aleurone layer transcriptomes revealed higher level of transcripts of the bHLH TF ThMYC4E in the blue aleurone phenotype. It forms clusters with genes that regulate anthocyanin biosynthesis. ThMYC4E may be a candidate Ba1 gene controlling the blue aleurone trait in bread wheat with an introgression from its wild relative Th. ponticum (Li et al., 2017). Ba2 confers blue grain trait in T. boeoticum. A comparative transcriptome profiling between blue-grained wheat-T. boeoticum substitution line and its wheat parent identified 41 and 29 unigenes, respectively, related to anthocyanin biosynthesis and transport. TbMYC4A contains three bHLH TF domains (bHLH-MYC_N, HLH, ACT-like), clusters with other blue grain genes from other Triticeae species, and is highly expressed in the blue-grained substitution line. The TbMYC4A-specific marker is also present in T. boeoticum and T. monococcum with blue aleurone but not in other Triticeae species with white aleurone, suggesting TbMYC4A as a candidate gene of Ba2 controlling the blue aleurone trait (Liu et al., 2021b). Transcriptome differences between the triticale lines HM13 (blue aleurone) and HM5 (white aleurone) revealed TsMYC2, a bHLH TF gene that regulates blue aleurone trait in triticale (Secale cereale), and which may be a good gene to be deployed to breed blue-grain triticale (Zong et al., 2019).
Clearly, significant progress has been made in identifying genes associated with anthocyanin biosynthesis in cereal crops, some of which have also been functionally characterized (Table 3). Functionally characterized genes and associated DNA markers may be deployed in crop breeding to select for grain-anthocyanin-rich segregants. Candidate genes can be characterized by either generating random mutants, insertion/deletion mutants (transposons), establishing mutants genetic screen, gain-of-function, or loss-of-function mutants, and microarrays-based gene expression, RNAi knockout lines, or transgene overexpression (Alberts et al., 2002), and targeted gene editing (Kamburova et al., 2017).
Plant breeding has been realigning its agenda to meet end-users' demands of nutrient-rich diets (Patil et al., 2014). Crossbreeding and genetic engineering may significantly increase anthocyanins as evidenced in citrus (Rapisarda et al., 2009), potato (Stobiecki et al., 2003), tomato (Davuluri et al., 2005) and wheat (Gordeeva et al., 2020). Screening of crop germplasm (including breeding populations) often shows a significant and high heritable variation of anthocyanin content, e.g., in maize (Paulsmeyer et al., 2017) or in various fruits and vegetables (Patil et al., 2014 and references therein). However, scanty results about bioavailability are known to show that such increases lead to improving human health (Pascual-Teresa and Sanchez-Ballesta, 2008).
China has defined a daily 50 mg intake of anthocyanins as target (Wallace and Giusti, 2015) but such dietary reference is not available in North America or in Europe (Wallace et al., 2015). Moreover, the dietary intake of anthocyanins may vary according to age, ethnicity, and gender (National Center for Health Statistics, 2015). Therefore, developing a product profile is necessary for targeting anthocyanin-rich crops. The product profile for each crop states target(s) for breeding within a timeframe to develop a new cultivar showing increased bioavailable anthocyanins. The newly bred cultivar aims to replace those in use today to ensure market impacts. In this regard, the use of databases characterizing the intake of anthocyanins (Zamora-Ros et al., 2010, 2013; Sebastian et al., 2015) can assist in identifying main sources and determine their links with dietary quality and related human wellbeing. It is possible that the data may indeed show that a healthy eating index positively correlates with flavonoid intake, but this remains yet to be determined.
Progress achieved to date in the development and release of pigmented (purple, black, blue, or red) cereal grain cultivars is summarized in Table 4. A multi-season trial noted significant differences for agronomic and phytochemical traits among advanced barley cultivars having hull-less pigmented grains. Cluster analysis separated barley cultivars into three groups: with L1997, L3005, L3007, and L3009 being the best for more than four traits including seed yield and antioxidant compounds (Iannucci et al., 2021). Marker-aided backcrossing was employed to develop a set of barley near-isogenic lines (NILs) containing different combinations of Ant1 (bHLH transcription factor) and Ant2 (R2R3-MYB domain transcription factor) alleles (Gordeeva et al., 2019b). NILs carrying dominant alleles of both genes produced purple pericarp. The expression of these genes was upregulated in purple grain barley lines with dominant alleles at both loci. The lines carrying dominant allele either in the Ant1 or the Ant2 locus showed decreased level of expression of the dominant gene but scant activity of the recessive allele. Such an expression was barely detected in uncolored lines with recessive alleles at both loci. Marker-assisted selection approach to develop NIL containing phenolic compounds (anthocyanin and melanin) in the genetic background of barley cultivar “Bowman” was deployed which significantly showed higher total anthocyanin content without any yield-penalty (Glagoleva et al., 2022).
Table 4. Anthocyanins-rich grain crop cultivars or advanced breeding lines and genetic stocks developed through crossbreeding and selection in cereals.
Substantial variation (47–1,289 μg g−1 dry weight) was observed in the anthocyanin seed content among wheat breeding lines (467–1,289 μg g−1 dry weight, mean = 881 μg g−1 in deep purple; 195–679 μg g−1, mean = 496 μg g−1 in blue; 127–438 μg g−1, mean = 221 μg g−1 in purple) and cultivars (117–669 μg g−1, mean = 354 μg g−1 in blue and 47–175 μg g−1, mean 100 μg g−1 in purple). Deep purple lines contained higher concentration of anthocyanin followed by blue and purple lines. The analysis identified 10 target zones, four typically representing blue aleurone types, five purple pericarp types, and one representing both. The peak heights and areas were highly correlated and 7 of 10 target zones contributed to about 90% of the total variation. Multivariate analysis of seven target zones not only separated the lines into purple, blue and deep purple seeded types, but also identified lines with a specific anthocyanin pattern (Böhmdorfer et al., 2018).
A blue-grained substitution line in the genetic background of popular Russian wheat cultivar “Saratovskaya 29” (S29) including the Thinopyrum ponticum chromosome 4 has been developed (Gordeeva et al., 2019a). This substitution line contains 475.7 μg g−1 anthocyanins compared to nil in S 29. Despite carrying the entire chromosome substitution, the 1,000-seed weight, milling, and dough characteristics were comparable or slightly lower than S29. Using genetic markers such as Pp3 and Pp-D1 (which regulate purple pericarp color) and morphological characteristics, the researchers developed a number of advanced bread wheat lines with multiple resistance to diseases and purple-seeded characteristics adapted to the West Siberian region and suitable for functional food production (Gordeeva et al., 2020). Colored grain wheat lines, with black ones having highest total anthocyanin content followed by blue, purple, and white have also been developed (Garg et al., 2016). The yield was similar to those of checks (amber wheat) in regional adaptation trials in India. The National Agri-Food Biotechnology Institute (NABI, Mohali) implemented a pilot project with support from the industry to develop three colored wheat cultivars, namely, “NABIMG-11-Black,” “NABIMG-10-Purple,” and “NABIMG-9-Blue” seed types (Garg, 2018a,b,c; Figures 1, 2). The private sector entrepreneurs are involved through contract farming in the production, popularization, and marketing of processed colored wheat-based products in India. The black wheat cultivar “NABIMG-11-Black” has been gaining popularity among farmers, industry and consumers due to its potential health benefits as well as high-income generation potential. Its comparable yield (3.9 t ha−1) and seed weight (43 g 1,000-seed) with control PBW621 (yield, 4.1 t ha−1; 1000-seed weight, 38 g) after multi-season testing, showed the potential for significantly expanding its cultivation (NABI Annual Report, 2018–2019; https://nabi.res.in/cms?slug=annual-reports). Contract farming refers to agricultural production following an agreement between a buyer and farmer.
Figure 1. Genetic variability for grain color [(A), amber; (B), purple; (C), blue; (D), black] in wheat.
Figure 2. Advanced breeding lines with pigmented grains [(A), amber; (B), black; (C), blue; (D), purple] developed by crossbreeding and selection in wheat.
An anthocyanin-rich (deep purple pericarp) maize cultivar named “Polenta” (R3075) has also been developed (Lago et al., 2014). It ensued from recurrent selection (a method involving reselection generation after generation with interbreeding of selects to reshuffle genes through genetic recombination) in the genetic background of traditional cultivar “Scagliolo” devoid of anthocyanins in northern Italy. “Polenta” contains 109 mg 100 g−1 anthocyanins. The introduction of purple pigmentation in “Scagliolo” genetic background had adversely impacted 100-seed weight (0.198 vs. 0.254 g) and seed germination (84 vs. 92%). This colored “Polenta” cultivar is therefore an important genetic resource that can be recycled in maize breeding to develop lines combining high anthocyanins, acceptable seed size and seed germination to enhance its commercial value. The first anthocyanin-rich maize hybrid cultivar “Saekso 2” was released in South Korea (Choi et al., 2017). The average seed anthocyanin content in relation to “Saeko 1” (0 mg 100 g−1) was 685 mg 100 g−1. FlaNat Research Italia Srl (Italy) reported development of a new purple maize cultivar “Moradyn,” which is adapted to northern Italy and maintains a highly pigmented cob (Ferron et al., 2020).
“Apache Red,” a purple maize landrace, contains a wealth of diversity in anthocyanin composition and content. Using “Apache Red” and population breeding approach new populations were generated with variable flavonoid profiles–flavanol–anthocyanin condensed forms (0–80%), acylated anthocyanins (2–72%), pelargonidin-derived anthocyanins (5–99%), C-glycosyl flavone co-pigments up to 1,904 μg g−1, and with anthocyanin content up to 1,598 μg g−1(Chatham and Juvik, 2021). Thus, it represents to date one of the most anthocyanin diverse pericarp-pigmented source for use in maize breeding and genetics.
India is a home to several pigmented traditional landraces such as “Chak-hao Amubi,” “Chak-hao Poireiton” and “Chak-hao Angangba,” which are black-pigmented rice predominantly grown in Manipur. Pigmented rice cultivars, “Rubin” (red-grain) and “Yuzhnaya Noch” (black-grain), developed by crossbreeding are grown in Russia, while traditional landraces showing pigmented grains were collected in Philippines (Krishnan et al., 2020). Aromatic, black-grained rice lines have been developed through interspecific hybridization between Oryza rufipogon, a red grain donor and source of black rice gene Kala4, with cultivars “Badshabhog” (white grain), “Chenga” (red grain) and “Ranjit” (white grain) (Roy and Shil, 2021). A few nutrient-rich, black-grained rice cultivars namely “Pinhei 1,” “Zhongi 4,” and “Ziyunuo,” which are increasingly popular with consumers, are cultivated in China; while a black rice introgression line in the genetic background of “Koshihikari” in Japan is nutritionally superior to the black-grained rice “Okunomurasaki” (Xia et al., 2021).
Clearly, significant progress has been achieved toward developing advanced breeding lines or cultivars with pigmented (black, purple, blue, or red) grains, with a few already commercialized such as barley, maize, rice, and wheat across continents. The produce from such cultivars may serve as potential sources for production of functional foods.
Genetic engineering of anthocyanin biosynthesis pathway in staple crops has the potential to provide health-promoting food in abundance to minimize the risk of chronic diseases in humans. However, anthocyanin biosynthesis pathway is complex and regulated by complex genetic network involving both structural and regulatory genes (Table 3) and influenced by agronomic practices and environmental factors (Section Factors Affecting the Content and Composition of Anthocyanin). Purple tomato rich in anthocyanins was developed by engineering two snapdragon transcription factors (TFs) and it accumulated anthocyanins in fruits at levels substantially higher than previously reported and at concentrations comparable to the anthocyanin levels in berries (Butelli et al., 2008). The fruits showed intense purple color in both the peel and flesh and antioxidant capacity was increased three-fold. Tomato fruit produces large amounts of primary and secondary metabolites and therefore can serve as an excellent plant source to either increase nutrients for health-promoting biofortified foods or as a production system for high value new compounds (Li et al., 2018b). Also, tobacco cell cultures have been developed as customizable and sustainable alternatives to conventional anthocyanin production platforms by engineering regulatory and enzyme encoding genes, which were transferable to other plant species (Appelhagen et al., 2018).
A few research groups in field crops were successful in enhancing anthocyanins with little or no adverse effect on plant growth, development and reproduction (Table 4). A simple and an efficient multigene expression system based on embryo-specific bidirectional promotor and 2A linker peptides was developed for maize. Eleven genes were introduced into transgenic maize to rebuild the anthocyanin biosynthesis pathway in Hill embryos to develop anthocyanin-rich purple maize germplasm (Liu et al., 2018a,b). A highly efficient vector system for transgene stacking involving a construct containing eight anthocyanin-related genes driven by the endosperm-specific promoters, a selectable marker and a gene for marker excision was successful in generating a novel “purple endosperm” rice germplasm with high anthocyanins (Zhu et al., 2017). It involved expression of the transgenes that enhanced the expression of 13 endogenous anthocyanin biosynthesis genes. Both the approaches provided an efficient and a versatile toolkit for transgene stacking to increase synthesis of anthocyanins.
Genes ZmC1 and ZmR control anthocyanin biosynthesis in maize. Transgenic wheat lines overexpressing ZmC1 and ZmR had highest pigmentation in almost all tissues including seeds, while those overexpressing ZmC1 or ZmR, respectively, had controlled pigmentation in vegetative or reproductive tissues including seeds. The transgenic lines showed greater expression levels of transcripts, conserved homologs, and six wheat structural genes associated with anthocyanin biosynthesis (Riaz et al., 2019). The overexpression of ThMYC4E in transgenic bread wheat showed higher levels of anthocyanins, predominantly cyanidin and delphinidin in seeds, and which had little or no adverse impact on plant growth and development (Zhao et al., 2020). Thus, agronomically valuable anthocyanin-rich genetic resources for crossbreeding are available.
Red seeded rice is a rich source of proanthocyanidins and anthocyanins. Two complementary genes Rc and Rd control red color. A 14-bp frame-shift deletion in the seventh exon of the Rc in cultivated rice resulted in white grains (Sweeney et al., 2007). CRISPR/Cas9 system was deployed to functionally restore rc allele by reverting the 14-bp frame-shift deletion to in-frame mutations, and successfully converted elite white pericarp rice cultivars into red seeded types, which led to a significant increase in proanthocyanidins and anthocyanins agronomically at par with WT (Zhu et al., 2019). CRISPR/Cas9 gene editing system is promising to convert white-seeded into red-seeded rice cultivars. Multinational seed private enterprises are racing to develop CRISPR-edited crops with agriculturally beneficial traits. However, there are many hurdles, including regulation, public acceptance, social and ethical concerns, and whether such crops are going to be categorized as GMOs or non-GMOs. CRISPR-edited crops should be more socially acceptable since such crops are not based on transgenes (Ahmad et al., 2021).
Ascertaining the beneficial effect of anthocyanin-rich food is limited, due to confounding effects by the influence of other metabolites in plant-based food consumed in the diet. Maize is an important food and feed crop with multiple and diverse sources of germplasm with colored grains, and well-researched flavonoid biosynthetic pathway which make it an ideal crop for the development of “near-isogenic” model foods differing only in the content of specific classes of flavonoids, which can be used in animal feeding/human intervention trials to unravel diet-health complexity (Petroni et al., 2014). NILs either lacking both “anthocyanins” and “phlobaphenes,” containing either “phlobaphenes” or “anthocyanins,” and a line that contains both “anthocyanins” and “phlobaphenes” are available. The profiling of these NILs and comparing them to those lacking one or both compounds revealed that “anthocyanin” and “phlobaphene” accumulation contributed significantly (P < 0.05) to antioxidant capacity, while intake of flavonoid-rich maize diets ameliorated experimental colitis in mice. It is a powerful tool to investigate the disease-preventive effects of specific flavonoid compounds in diet (Wu et al., 2021a). Tomato, a widely consumed vegetable worldwide, has abundant genetic and genomic resources. The fruits contain a variety of essential nutrients (minerals, vitamins, proteins, essential amino acids, monounsaturated fatty acids, carotenoids, phytosterols) and antioxidant-rich phytochemicals beneficial to human health (Ali et al., 2021). The variation in color, shape, texture, and volatile compounds make it a unique model organism of Solanaceae family. The growing evidence supports the connection between increased tomato consumption and reduced risk for cardiovascular disease, cancer, neurodegenerative diseases and diabetes, maintenance of a healthy gut microbiome, and improved skin health, fertility, immune response, and exercise recovery (Canene-Adams et al., 2005; Collins et al., 2022). The antioxidant capacity of the genetically engineered tomato fruit containing two snapdragon TFs increased by three-folds and prolonged the life of cancer susceptible mice (Trp53−/−) raised on a diet supplemented with the high-anthocyanin tomatoes (Butelli et al., 2008). Inflammatory bowel diseases (IBD) are chronic inflammatory disorders because of defective immune response toward intestinal bacteria. Intestinal dysbiosis is associated with the onset of IBD that persist even in patients in deep remission. A dietary regime enriched in polyphenols, tomato NILs in the genetic background of Bronze-tomato enriched in flavonols, anthocyanins, and stilbenoids (Scarano et al., 2018), administered to Winnie and control mice showed significant induction of anti-inflammatory genes and may efficiently support IBD remission affecting the intestinal dysbiosis (Liso et al., 2018). Both crossbreeding and biotechnology approaches were used to develop anthocyanins rich germplasm/cultivars (Mattoo et al., 2021), which may be further crossed to develop NILs differing in a particular biochemical compound to investigate its long-term effect on human health. The transcription factors involved in regulating the structural and other genes in the biosynthetic pathway of anthocyanin are depicted in Figure 3 (Courtesy of Frontiers—image_m/fchem-06-00052-g005.jpg).
Figure 3. Regulatory transcription factors in anthocyanin biosynthesis pathway. MYB, bHLH and WD40 modulate the expression of structural genes. (A) Activate regulation of anthocyanin biosynthesis. (B) Repressive regulation of anthocyanin biosynthesis. MYB repressors compete with MYB activators for bHLH JAF13. (C) Repressive regulation of anthocyanin biosynthesis. MYB repressors compete with MYB activators for bHLH AN1. The “ → ” means activation, “—|” means repression and “X” means inactivation. https://www.frontiersin.org/files/Articles/308869/fchem-06-00052-HTML/image_m/fchem-06-00052-g005.jpg.
Insufficient global intake of minerals, vitamins, fibers and phytochemicals, particularly among the poorer communities, is the main cause of malnutrition. HarvestPlus under CGIAR-Research Program on Agriculture for Nutrition and Health (https://a4nh.cgiar.org/) has provided the platform and one time investment to implement cost-effective crop biofortification as key intervention to reduce mineral and vitamin deficiencies in the developing world. Biofortification refers to the development of micronutrient-dense staple crops using traditional breeding practices or biotechnology. Biofortified crops have been released in 40 countries, with more than 48 million people in small holder family benefitting from nutrient dense (Fe, Zn, β-carotene) cultivars (https://www.harvestplus.org). Such crops offer a rural-based intervention by the farmers. Assessment of adoption and consumption of biofortified crops suggest high preference of nutritionally enhanced crops by the target consumers (Dwivedi et al., 2012; Birol et al., 2015). Integrating anthocyanin to biofortification will provide a platform for developing nutrient (Fe, Zn, β-carotene) and anthocyanin-rich staple crop cultivars. The mainstreaming biofortified traits into public and private sector breeding programs, building consumer demand, and providing appropriate policy support is needed to scale up production and consumption of nutrients and/or phytochemicals-rich staple foods together with helping the poorer sectors (Bouis and Saltzman, 2017; Foley et al., 2021).
The demand for anthocyanin-rich food is anticipated to increase with time because of the health benefits. Cultivar development is a time-consuming process, for example, from the time a cross is made to the advancement, testing and release of a new cultivar, it takes 8 to 10 years, and then at least another 5–8 years to upscale production and popularization of new product among all stakeholders. Targeted breeding has led to the development and cultivation of anthocyanin-rich crops (Table 4) though on a limited acreage. Replacing the non-pigmented grains on large acreage to pigmented grains is not easily achievable. An incremental increase in acreage and addressing bottlenecks to production and acceptance of biofortified food-products and policy support by Governments may provide stimulus for large-scale adoption and consumption of biofortified crop produce by agrarian society. A paradigm shift is therefore needed to include nutrition and other health promoting compounds as core breeding issues such as resistance to pest and diseases and productivity. In comparison, transgenics approach on the other hand requires much shorter time frame in which a multi-nutrient product can be developed using a single DNA insertion, as evidenced in rice expressing AtNAS1, PvFERRITIN, CRTI, and ZmPSY genes in polished grains enriched with Fe, Zn, and β-carotene (Singh et al., 2017).
Biofortification may result in altering sensory and flavor attributes, appearance, and other characteristics of food. The anthocyanin-rich food products, including grains and their derived flours, bread, noodles, tortilla, biscuits, and other confectionary products are often seen in grocery stores or supermarkets worldwide (Betran et al., 2001; Syed Jaafar et al., 2013; Urias-Peraldí et al., 2013; Havrlentova et al., 2014). The public perception about acceptance of anthocyanin-rich food will also depend on the availability, affordability, and willingness to pay higher price for nutritionally-enhanced foods vis-à-vis commonly derived products involving white grains and flours.
A significant proportion of wheat is consumed in the form of “chapatti” or flat bread. The research suggests that “chapattis” made from anthocyanin-biofortified wheat flour have soft texture, acceptable sensory quality, good taste, are rich in dietary fibers and protein, but are low in carbohydrates (Kumari et al., 2020). A higher total amino acid content and nutrition index in black and blue wheat flour and chapatti in comparison to other (amber, or purple) flour and chapatti samples has been noted (Sharma et al., 2022). The essential amino acids, however, were similar in flour and chapatti samples. The amino acids on an average were reduced by 11.41, 12.4, 19.0, and 23.8% in black, blue, purple, and white wheat, respectively. The white chapatti samples showed >20% loss in 14 amino acids, while such reduction in black chapatti was noted for only one amino acid. Higher retention of amino acids in colored wheat could be due to the masking and protective effects of anthocyanins on proteins and amino acids from heating and oxidative damage.
A study on bread-making quality and organoleptic properties of bakery products made from anthocyanin-rich wheat showed no difference (rather in some cases higher) than the corresponding properties of products made from colorless wheat grains. A variety of products including bars, crackers, bread, pancake, and porridge made from purple wheat flour substantially differ in nutrition, protein being high in bread, pancake, and porridge and intermediate in bars and crackers. The bran-rich products and whole wheat pancake are rich in dietary fiber. The crackers and bars have higher anthocyanin and dietary fiber with strong antioxidant capacity (Gamel et al., 2020). The anthocyanin-based bakery products increase shelf life and resistance to molding under conditions that are favorable to fungal infection (Khlestkina et al., 2017). Nutritional significance of confectionery products made from flour and bran fraction of anthocyanin-rich (purple) or anthocyanin-poor (white) wheat isogenic lines have been compared (Usenko et al., 2018). The products made from purple-seeded wheat isogenic line had ~2.5-fold higher anthocyanins than in the similar products obtained from white-seeded isogenic line. Thus, use of purple wheat bran as ingredient-enriched confectionery products with a high nutritional value has immense possibilities. However, as noticed with maize in India (Trehan et al., 2018) and wheat in Italy (Pasqualone et al., 2015), people preferred muffins prepared using yellow maize than those made with purple corn, or biscuits from non-pigmented wheat rather than those derived from anthocyanin-rich purple wheat, respectively. It is also known that the different appearance and flavor negatively affect the view on an anthocyanin-derived food product (Hegyi et al., 2016), while the perceived health benefits increase their acceptance (Lampila et al., 2009).
Polvorones, a traditional flour-based confectionery made with whole blue maize flour has higher content of phenolics and anthocyanins as well as antioxidant activity compared with wheat flour-based confectionery. Substitution of wheat flour with whole blue maize flour thus enhanced overall acceptability, as well as the color and flavor of polvorones (Vázquez-Carrillo et al., 2018). There is growing demand for native-traditional foods to improve their intake of both nutrients and health-promoting phytochemicals. A comparative assessment of nutrients and bioactive compounds between industrial and handmade tortillas revealed that handmade tortillas made from blue and white maize were superior in nutritional and bioactive compounds compared to industrial tortillas. The handmade blue maize tortillas showed greater dietary fiber, phenolic acids and anthocyanins and antioxidant capacity than white maize-based industrial tortillas. The ferulic acid in handmade blue tortillas was 4.5-fold greater compared to industrial white maize tortillas. Thus, handmade fresh tortillas are superior in nutritional-nutraceutical properties compared to white maize tortillas (Colín Chávez et al., 2020).
Popcorn is an extremely popular snack globally. No difference was found in popping ability and expansion volume as well as taste perception between popcorn obtained from purple (~66 mg 100 g−1 dry matter, mainly cyanidin) or colorless (yellow kernels) cultivars (Lago et al., 2012). Even though the microwave-assisted popping reduced anthocyanin content by ~46%, the remaining anthocyanin provided a strong antioxidant capacity compared to the colorless grains. The demand for blue maize products in Mexico is booming and consumers are willing to pay a premium price (women, up to 33%; men, up to 19%) for its rich flavor and texture. The desire to go back to natural and to their roots drive consumers to pay higher price for blue maize products (https://www.cimmyt.org/news/blue-maize-is-all-the-rage-but-are-consumers-willing-to-pay/).
Thus, there is a growing awareness among people at large to eat nutritious and health- promoting foods. The availability, affordability and public perception about the unconventional foods that differ in appearance, sensory and flavor attributes limit the intake of such products. However, public perception is gradually changing to favor such foods for health reasons (Talsma et al., 2017; Woods et al., 2020; Rizwan et al., 2021), as also evidenced by the stockpiling of bakery and confectionary products made from colored grains or flours in grocery stores and supermarkets worldwide. The intake of such products is expected to rise many fold once such crops are commercialized for production in the countryside.
Public reluctance about the acceptance of foods derived from grains or flour of transgenic or gene edited crops, relative to foods produced using crossbreeding, is largely due to wrong perception of unknown consequences of adverse effect on human health of the former. Heterogeneity of consumers across cultures of borders, attitude toward science and innovations, and in risk perceptions emerge as key determinants for acceptance and willingness to pay (WTP) for genetically modified food (GMF). Acceptance and WTP are higher when consumers perceive the attributes (nutritional value, taste and flavor or sustainable ecosystems such as reduced water or pesticides) generated by new technology are beneficial. The gene edited-derived foods are systematically less discounted than GMF (Beghin and Gustafson, 2021). Vitamin A deficiency is a public health problem in developing world prompting country-Governments to adopt biofortification of plant-derived foods with pro-vitamin A (β-carotene) enriched foods as vehicle to overcome deficiency. Cassava, maize and sweet potato have been successfully biofortified by exploiting natural genetic variation with elevated levels of provitamin A (Welsch et al., 2010; Nkhata et al., 2020). Rice grains are devoid of β-carotene. The accumulation of β-carotene in the endosperm, popularly coined as “Golden Rice,” was successfully achieved by introducing Zmpsy1 and crtI into a US temperate japonica rice, Kaybonnet (Ye et al., 2000; Paine et al., 2005). This golden trait was subsequently transferred by marker-aided backcross breeding into several locally adapted cultivars (IR64, PSBRc82, BR29) in Asia (Mallikarjuna Swamy et al., 2021). More importantly, no significant differences in seed chemistry, except for β-carotene and related carotenoids, between genetically engineered GR2E (“Golden Rice”) and the near isogenic control were noted. The efficacy trials revealed that milled rice of GR2E could contribute up to 89–113% and 57–99% of the estimated average requirement for vitamin A for preschool children in Bangladesh and the Philippines, respectively (Mallikarjuna Swamy et al., 2019). Both Bangladesh and the Philippines have taken the lead in providing their constituents, respectively, Bt brinjal (eggplant) and GMO maize which have transformed their farmers (Shelton et al., 2018; https://allianceforscience.cornell.edu/blog/2019/01/gmo-corn-transforming-farmers-lives-philippines/). More recently, Department of Agriculture-Philippine Rice Research Institute (DA-PhilRice) in partnership with the International Rice Research Institute (IRRI) has recommended release of provitamin A rich (β-carotene) golden rice cultivar in the Philippine (https://www.irri.org/news-and-events/news/philippines-becomes-first-country-approve-nutrient-enriched-golden-rice).
Furthermore, molecular characterization and biosafety assessment has revealed that biofortified provitamin A rice is safe to eat without any adverse impact on human health (Oliva et al., 2020). “DA-PhilRice” in the genetic background of PSBRc82 has been recently released for commercialization in the Philippines. “DA-PhilRice” has also received food safety approvals from regulators in Australia, New Zealand, Canada, and USA, and is likely to be released soon in Bangladesh. The evidence suggests that consumers have reacted positively to “Golden Rice” and thus can be utilized for food systems to be integrated into consumer diets. In near future, new products enriched with nutrients and bioactive compounds (including anthocyanin), irrespective whether developed by conventional crossbreeding or by use of genetic engineering (including gene editing), are likely to be released for commercialization and will likely be accepted by public in large as staple foods in the developing world to promote human health (Steur et al., 2022).
Secondary metabolism in plants is a source of many potential bioactive compounds some of which have been linked to human health. Anthocyanins belong to a major group of polyphenolic molecules called flavonoids. The biosynthetic pathways leading to synthesis of these bioactive compounds are regulated by a complex of structural and regulatory genes as well as environmental cues. Thus, anthocyanin biofortification is providing new knowledge and advancements in gene stacking through core breeding programs and metabolic engineering advancements to ensure future rich germplasm for nutrition needs of growing human population particularly in the developing world. Both core breeding and genetic engineering of transcription factors have been successfully applied in developing anthocyanin-rich germplasm without significant yield penalty. The evidence suggests that there are changes in public perceptions about the acceptance of biofortified food crops, whether developed by crossbreeding, genetic engineering or gene-editing. The crops include Fe, Zn, or β-carotene dense beans, corn, rice, and wheat developed by crossbreeding; β-carotene enhanced “Golden Rice” by genetic engineering. This certainly is a good avenue for improving the human health worldwide. Thus, including phytochemicals as additional quality parameter for developing nutrients and phytochemicals dense staple crop cultivars and promoting large-scale production and intake of such food products should be the guiding principles to minimize the risk of all forms of malnutrition in human globally. Technological challenges to anthocyanin research include a rapid and cost-effective phenotyping assay capable of analyzing large number of breeding samples, anthocyanin retention and bioavailability, and loss of anthocyanins during cooking/baking or during storage. Confounding effects of food matrix in determining the health benefits associated with phytochemicals is equally challenging to be performed on human. Such issues should be addressed by education and transparency of the methodologies employed to achieve these goals for human health worldwide. Moreover, adopting a science-based regulatory frame uniformly worldwide as well as public awareness is seen as a good knowledge base to promote human consumption of transgenic and gene edited staple crops (Kato-Nitta et al., 2019, 2021; Wu et al., 2021b).
SD and AM: conceptualization, investigation, writing-original draft, and writing-review and editing. MG, SD, and BS: investigation and writing-original draft. RO: conceptualization, project administration, investigation, writing-original draft, and review and editing. All authors contributed to the article and approved the submitted version.
RO acknowledges the grant funding through the PlantePigment and Annatto projects led by Chr.HansenA/S through the projects from Green Development and Demonstration Program (GUDP, Denmark) and Innovationsfonden (Denmark), respectively. AM thanks the support of the United States Department of Agriculture, Agricultural Research Service, Sustainable Agricultural Systems Laboratory, Beltsville, MD 20705-2350, USA. MG acknowledges the funding support of the Department of Biotechnology, New Delhi, India. The funders were not involved in the study design, collection, analysis, interpretation of data, the writing of this article or the decision to submit it for publication.
The authors declare that the research was conducted in the absence of any commercial or financial relationships that could be construed as a potential conflict of interest.
All claims expressed in this article are solely those of the authors and do not necessarily represent those of their affiliated organizations, or those of the publisher, the editors and the reviewers. Any product that may be evaluated in this article, or claim that may be made by its manufacturer, is not guaranteed or endorsed by the publisher.
The Supplementary Material for this article can be found online at: https://www.frontiersin.org/articles/10.3389/fsufs.2022.867897/full#supplementary-material
Abdel-Aal, E.-S. M, Hucl, P., and Rabalski, I. (2018). Compositional and antioxidant properties of anthocyanin-rich products prepared from purple wheat. Food Chem. 254, 13–19. doi: 10.1016/j.foodchem.2018.01.170
Abdel-Aal, E.-S. M., Hucl, P., Shipp, J., and Rabalski, I. (2016). Compositional differences in anthocyanins from blue- and purple-grained spring wheat grown in four environments in Central Saskatchewan. Cereal Chem. 93, 32–38. doi: 10.1094/CCHEM-03-15-0058-R
Abdel-Aal, E. M., Young, J. C., and Rabalski, I. (2006). Anthocyanin composition in black, blue, pink, purple, and red cereal grains. J. Agric. Food Chem. 54, 4696–4704. doi: 10.1021/jf0606609
Abdel-Aal, E. S. M., Choo, T. M., Dhillon, S., and Rabalski, I. (2012). Free and bound phenolic acids and total phenolics in black, blue, and yellow barley and their contribution to free radical scavenging capacity. Cereal Chem. 89, 198–204. doi: 10.1094/CCHEM-10-11-0116
Ahmad, A., Munawar, N., Khan, Z., Qusmani, A. T., Khan, S. H., Jamil, A., et al. (2021). An outlook on global regulatory landscape for genome-edited crops. Int. J. Mol. Sci. 22, 11753. doi: 10.3390/ijms222111753
Alberts, B., Johnson, A., Lewis, J., Raff, M., Roberts, K., and Walter, P. (2002). Molecular Biology of the Cell, 4th Edn. New York, NY: Garland Science; Studying Gene Expression and Function. Available onlie at: https://www.ncbi.nlm.nih.gov/books/NBK26818/
Ali, M. Y., Sina, A. A. I., Khandker, S. S., Neesa, L., Tanvir, E. M., Kabir, A., et al. (2021). Nutritional composition and bioactive compounds in tomatoes and their impact on human health and disease: a review. Foods 10, 45. doi: 10.3390/foods10010045
Allan, A. C., Hellens, R. P., and Laing, W. A. (2008). MYB transcription factors that colour our fruit. Trends Plant Sci. 13, 99–102. doi: 10.1016/j.tplants.2007.11.012
Alnajjar, M., Barik, S. K., Bestwick, C., Campbell, F., Cruickshank, M., Farquharson, F., et al. (2020). Anthocyanin-enriched bilberry extract attenuates glycaemic response in overweight volunteers without changes in insulin. J. Funct. Foods 64, 103597. doi: 10.1016/j.jff.2019.103597
Appelhagen, I., Wulf-Vester, A. M., Wendell, M., Hvoslef-Eide, A.-K., Russell, J., Oertel, A., et al. (2018). Colour bio-factories: towards scale-up production of anthocyanins in plant cell cultures. Metab. Eng. 48, 218–232. doi: 10.1016/j.ymben.2018.06.004
Araki, R., Yada, A., Ueda, H., Tominaga, K., and Isoda, H. (2021). Differences in the effects of anthocyanin supplementation on glucose and lipid metabolism according to the structure of the main anthocyanin: a meta-analysis of randomized controlled trials. Nutrients 13, 2003. doi: 10.3390/nu13062003
Azuma, A., Yakushiji, H., Koshita, Y., and Kobayashi, S. (2012). Flavonoid biosynthesis-related genes in grape skin are differentially regulated by temperature and light conditions. Planta 236, 1067–1080. doi: 10.1007/s00425-012-1650-x
Bach Knudsen, K. E., Nørskov, N. P., Bolvig, A. K., Hedemann, M. S., and Laerke, H. N. (2017). Dietary fibers and associated phytochemicals in cereals. Mol. Nutr. Food Res. 61. doi: 10.1002/mnfr.201600518
Bartl, P., Albreht, A., Skrt, M., Tremlova, B., Oštádalová, M., Šmejkal, K., et al. (2015). Anthocyanins in purple and blue wheat grains and in resulting bread: quantity, composition, and thermal stability. Int. J. Food Sci. Nutr. 66, 514–519. doi: 10.3109/09637486.2015.1056108
Beghin, J. S., and Gustafson, C. R. (2021). Consumers' valuation of and attitudes towards novel foods produced with NEPTs. A review. Economics Working Paper. Department of Economics, Iowa State University, 21012. Available online at: http://lib.dr.istate.edu.econ_workingpapers/134
Betran, F. J., Bockholt, A. J., and Hallauer, A. R. (2001). “Blue corn,” in Specialty Corns, 2nd Edn., ed L. W. Rooney (Boca Raton, FL: CRC Press LLC).
Bhuvaneswari, S., Krishnan, S. G., Bollinedi, H., Saha, S., Ellur, R. K., Vinod, K. K., et al. (2020). Genetic architecture and anthocyanin profiling of aromatic rice from Manipur reveals divergence of Chakhao landraces. Front. Genet. 11, 570731. doi: 10.3389/fgene.2020.570731
Birol, E., Meenakshi, J. V., Oparinde, A., Perez, S., and Tomlins, K. (2015). Developing country consumers' acceptance of biofortified foods: a synthesis. Food Security 7, 555–568. doi: 10.1007/s12571-015-0464-7
Böhmdorfer, S., Oberlerchner, J. T., Fuchs, C., Rosenau, T., and Grausgruber, H. (2018). Profiling and quantification of grain anthocyanins in purple pericarp × blue aleurone wheat crosses by high performance thin layer chromatography and densitometry. Plant Methods 14, 29. doi: 10.1186/s13007-018-0296-5
Bondonno, N. P., Dalgaard, F., Kyrø, C., Murray, K., Bondonno, C. P., Lewis, J. R., et al. (2019). Flavonoid intake is associated with lower mortality in the Danish diet cancer and health Cohort. Nat. Commun. 10, 3651. doi: 10.1038/s41467-019-11622-x
Bouis, H. E., and Saltzman, A. (2017). Improving nutrition through biofortification: a review of evidence from HarvestPlus, 2003 through 2016. Glob. Food Sec. 12, 49–58. doi: 10.1016/j.gfs.2017.01.009
Bustos, D. V., Riegel, R., and Calderini, D. F. (2012). Anthocyanin content of grains in purple wheat is affected by grain position, assimilate availability and agronomic management. J. Cereal Sci. 55, 257–264. doi: 10.1016/j.jcs.2011.12.001
Butelli, E., Titta, L., Giorgio, M., Mock, H.-P., Matros, A., Peterek, S., et al. (2008). Enrichment of tomato fruit with health-promoting anthocyanins by expression of select transcription factors. Nat. Biotechnol. 26, 1301–1308. doi: 10.1038/nbt.1506
Canene-Adams, K., Campbell, J. K., Zaripheh, S., Jeffery, E. H., and Erdman, J. W. Jr. (2005). The tomato as a functional food. J. Nutr. 135, 1226–1230. doi: 10.1093/jn/135.5.1226
Chatham, L. A., and Juvik, J. A. (2021). Linking anthocyanin diversity, hue, and genetics in purple corn. G3 11, jkaa062. doi: 10.1093/g3journal/jkaa062
Chatham, L. A., West, L., Berhow, M. A., Vermillion, K. E., and Juvik, J. A. (2018). Unique flavanol-anthocyanin condensed forms in apache red purple corn. J. Agric. Food Chem. 66, 10844–10854. doi: 10.1021/acs.jafc.8b04723
Chen, K., Wei, X., Zhang, J., Pariyani, R., Jokioja, J., Kortesniemi, M., et al. (2020). Effects of anthocyanin extracts from bilberry (Vaccinium myrtillus L.) and purple potato (Solanum tuberosum L. var. Synkeä Sakarí) on the plasma metabolic profile of Zucker diabetic fatty rats. J. Agric. Food Chem. 68, 9436–9450. doi: 10.1021/acs.jafc.0c04125
Chen, M.-H, McClung, A. M., and Bergman, C. J. (2017). Phenolic content, anthocyanins and antiradical capacity of diverse purple bran rice genotypes as compared to other bran colors. J. Cereal Sci. 77, 110–119. doi: 10.1016/j.jcs.2017.07.010
Chen, X. Q., Nagao, N., Itani, T., and Irifune, K. (2012). Anti-oxidative analysis, and identification and quantification of anthocyanin pigments in different colored rice. Food Chem. 135, 2783–2788. doi: 10.1016/j.foodchem.2012.06.098
Choi, J.-K., Park, J.-Y., Park, K.-J., Kim, H.-Y., Ryu, S.-H., and Seo, Y.-H. (2017). Anthocyanin-rich grain corn hybrid variety 'Saekso 2'. Korean J. Breed. Sci. 49, 289–293. doi: 10.9787/KJBS.2017.49.3.289
Chunthaburee, S., Sanitchon, J., Pattanagul, W., and Theerakulpisut, P. (2015). Effects of salt stress after late booting stage on yield and antioxidant capacity in pigmented rice grains and alleviation of the salt-induced yield reduction by exogenous spermidine. Plant Prod. Sci. 18, 32–42. doi: 10.1626/pps.18.32
Ciscomani-Larios, J. P., Sánchez-Chávez, E., Jacobo-Cuellar, J. L., Sáenz-Hidalgo, H. K., Orduño-Cruz, N., Cruz-Alvarez, O., et al. (2021). Biofortification efficiency with magnesium salts on the increase of bioactive compounds and antioxidant capacity in snap beans. Cienc. Rural 51. doi: 10.1590/0103-8478cr20200442
Colín Chávez, C., Virgen-Ortiz, J. J., Serrano-Rubio, L. E., Martínez-Téllez, M. A., and Astier, M. (2020). Comparison of nutritional properties and bioactive compounds between industrial and artisan fresh tortillas from maize landraces. Curr. Res. Food Sci. 3, 189–194. doi: 10.1016/j.crfs.2020.05.004
Collins, E., Bowyer, C., Tsouza, A., and Chopora, M. (2022). Tomatoes: an extensive review of the associated health impacts of tomatoes and factors that can affect their cultivation. Biology 11, 239. doi: 10.3390/biology11020239
Curtis, P. J., Berends, L., van der Velpen, V., Jennings, A., Haag, L., Chandra, P., et al. (2022). Blueberry anthocyanin intake attenuates the postprandial cardiometabolic effect of an energy-dense food challenge: results from a double blind, randomized controlled trial in metabolic syndrome participants. Clin. Nutr. 41, 165–176. doi: 10.1016/j.clnu.2021.11.030
Damián-Medina, K., Salinas-Moreno, Y., Milenkovic, D., Figueroa-Yáñez, L., Marino-Marmolejo, E., Higuera-Ciapara, I., et al. (2020). In silico analysis of antidiabetic potential of phenolic compounds from blue corn (Zea mays L.) and black bean (Phaseolus vulgaris L.). Heliyon 6, e03632. doi: 10.1016/j.heliyon.2020.e03632
Daneshzad, E., Shab-Bidar, S., Mohammadpour, Z., and Djafarian, K. (2019). Effect of anthocyanin supplementation on cardio-metabolic biomarkers: a systematic review and meta-analysis of randomized controlled trials. Clin. Nutr. 38, 1153–1165. doi: 10.1016/j.clnu.2018.06.979
Davuluri, G. R., Van Tuinen, A., Fraser, P. D., Manfredonia, A., Newman, R., Burgess, D., et al. (2005). Fruit-specific RNAi-mediated suppression of DET1 enhances carotenoid and flavonoid content in tomatoes. Nat. Biotechnol. 23, 890–895. doi: 10.1038/nbt1108
Dwivedi, S. L., Sahrawat, K. L., Rai, K. N., Blair, M. W., Andersson, M. S., and Pfeiffer, W. (2012). Nutritionally enhanced staple food crops. Plant Breed. Rev. 36, 169–291. doi: 10.1002/9781118358566.ch3
Dykes, L., Seitz, L. M., Rooney, W. L., and Rooney, L. W. (2009). Flavonoid composition of red sorghum genotypes. Food Chem. 116, 313–317. doi: 10.1016/j.foodchem.2009.02.052
Eticha, F., Grausgruber, H., Siebenhandl-Ehn, S., and Berghofer, E. (2011). Some agronomic and chemical traits of blue aleurone and purple pericarp wheat (Triticum L.). J. Agric. Sci. Technol. B 1, 48–58.
Fan, X., Xu, Z., Wang, F., Feng, B., Zhou, Q., Cao, J., et al. (2020). Identification of colored wheat genotypes with suitable quality and yield traits in response to low nitrogen input. PLoS ONE 15, e0229535. doi: 10.1371/journal.pone.0229535
Ferron, L., Colombo, R., Mannucci, B., and Papetti, A. (2020). A new Italian purple corn variety (Moradyn) byproduct extract: antiglycative and hypoglycemic in vitro activities and preliminary bioaccessibility studies. Molecules 25, 1958. doi: 10.3390/molecules25081958
Ficco, D. B. M., De Simone, V., Colecchia, S. A., Pecorella, I., Platani, C., Nigro, F., et al. (2014). Genetic variability in anthocyanin composition and nutritional properties of blue, purple, and red bread (Triticum aestivum L.) and durum (Triticum turgidum L. ssp. turgidum convar. durum) wheats. J. Agric. Food Chem. 62, 8686–8695. doi: 10.1021/jf5003683
Finch, R. A., and Simpson, E. (1978). New colours and complementary colour genes in barley. Z Pflanzenzücht 81, 40–53.
Foley, J. K., Michaux, K. D., Mudvahoto, B., Kyazike, L., Cherian, B., Kalejaive, O., et al. (2021). Scaling up delivery of biofortified staple food crops globally: paths to nourishing millions. Food Nutr. Bull. 42, 116–132. doi: 10.1177/0379572120982501
Fongfon, S., Prom-u-thai, C., Pusadee, T., and Jamjod, S. (2021). Responses of purple rice genotypes to nitrogen and zinc fertilizer application on grain yield, nitrogen, zinc, and anthocyanin concentration. Plants 10, 1717. doi: 10.3390/plants10081717
Francavilla, A., and Joyé, I. J. (2020). Anthocyanins in whole grain cereals and their potential effect on health. Nutrients 12, 2922. doi: 10.3390/nu12102922
Gamel, T. H., Wright, A. J., Pickard, M., and Abdel-Aal, E.-S. M. (2020). Characterization of anthocyanin-containing purple wheat prototype products as functional foods with potential health benefits. Cereal Chem. 97, 34–38. doi: 10.1002/cche.10190
Garg, M. (2018a). NABIMG-11-Black (BW/2* PBW621) (IC0620916; INGR17003), a wheat (Triticum aestivum) germplasm with black grain colour (purple pericarp + blue aleurone). Indian J. Plant Genet. Resour. 31, 334–335.
Garg, M. (2018b). NABIMG-10-Purple (BW/2* PBW621) (IC0620915; INGR17002), a wheat (Triticum aestivum) germplasm with purple grain (pericarp) colour. Indian J. Plant Genet. Resour. 31, 333–334.
Garg, M. (2018c). NABIMG-9-Blue; BW/2*/PBW621 (IC0620914; INGR17001), a wheat (Triticum aestivum) germplasm with blue grain (aleurone) colour. Indian J. Plant Genet. Resour. 31, 332–333.
Garg, M., Chawla, M., Chunduri, V., Kumar, R., Sharma, S., Sharma, N. K., et al. (2016). Transfer of grain colors to elite wheat 532 cultivars and their characterization. J. Cereal Sci. 71, 138–144. doi: 10.1016/j.jcs.2016.08.004
Glagoleva, A., Kukoeva, T., Mursalimov, S., Khlestkina, E., and Shoeva, O. (2022). Effect of combining the gene controlling anthocyanin and melanin synthesis in the barley grains on pigment accumulation and plant development. Agronomy 12, 112. doi: 10.3390/agronomy12010112
Gordeeva, E., Badaeva, E., Yudina, R., Shchukina, L., Shoeva, O., and Khlestkina, E. (2019a). Marker-assisted development of a blue-grained substitution line carrying the Thinopyrum ponticum chromosome 4Th(4D) in the spring bread wheat Saratovskaya 29 background. Agronomy 9, 723. doi: 10.3390/agronomy9110723
Gordeeva, E., Shamanin, V., Shoeva, O., Kukoeva, T., Morgounov, A., and Khlestkina, E. (2020). The strategy for marker-assisted breeding of anthocyanin-rich spring bread wheat (Triticum aestivum L.) cultivars in Western Siberia. Agronomy 10, 1603. doi: 10.3390/agronomy10101603
Gordeeva, E. I., Glagoleva, A. Y., Kukoeva, T. V., Khlestkina, E. K., and Shoeva, O. Y. (2019b). Purple-grained barley (Hordeum vulgare L.): marker-assisted development of NILs for investigating peculiarities of the anthocyanin biosynthesis regulatory network. BMC Plant Biol. 19, 52. doi: 10.1186/s12870-019-1638-9
Guo, W., Nazim, H., Liang, Z., and Yang, D. (2016). Magnesium deficiency in plants: an urgent problem. Crop J. 4, 83–91. doi: 10.1016/j.cj.2015.11.003
Harakotr, B., Suriharn, B., Scott, M. P., and Lertrot, K. (2015). Genotypic variability in anthocyanins, total phenolics, and antioxidant activity among diverse waxy corn germplasm. Euphytica 203, 237–248. doi: 10.1007/s10681-014-1240-z
Havrlentova, M., Pšenáková, I., Žofajová, A., Rückschloss, L., and Kraic, J. (2014). Anthocyanins in wheat seed - a mini review. Nova Biotechnol. Chimica 13, 1–12. doi: 10.2478/nbec-2014-0001
Hegyi, A., Kertész, Z., Kuti, T., and Sebok, A. (2016). Consumers' acceptance and attitude towards bioactive enriched foods. Proc. Syst. Dyn. Innovation Food Netw. 2016, 190–208.
Hichri, I., Heppel, S. C., Pillet, J., Léon, C., Czemmel, S., Delrot, S., et al. (2010). The basic helix-loop-helix transcription factor MYC1 is involved in the regulation of the flavonoid biosynthesis pathway in grapevine. Mol. Plant 3, 509–523. doi: 10.1093/mp/ssp118
Hong, H. T., Netzel, M. E., and O'Hare, T. J. (2020). Anthocyanin composition and changes during kernel development in purple-pericarp supersweet sweetcorn. Food Chem. 315, 126284. doi: 10.1016/j.foodchem.2020.126284
Hong, M. J., Kim, D. Y., Nam, B. M., Ahn, J.-W., Kwon, S.-J., Seo, Y. W., et al. (2019). Characterization of novel mutants of hexaploid wheat (Triticum aestivum L.) with various depths of purple grain color and antioxidant capacity. J. Sci. Food Agric. 99, 55–63. doi: 10.1002/jsfa.9141
Hosoda, K., Sasahara, H., Matsushita, K., Tamura, Y., Miyaji, M., and Matsuyama, H. (2018). Anthocyanin and proanthocyanidin contents, antioxidant activity, and in situ degradability of black and red rice grains. Asian Austral. J. Animal Sci. 31, 1213–1220. doi: 10.5713/ajas.17.0655
Hosseinian, F. S., Li, W., and Beta, T. (2008). Measurement of anthocyanins and other phytochemicals in purple wheat. Food Chem. 109, 916–924. doi: 10.1016/j.foodchem.2007.12.083
Hu, N., Zheng, J., Li, W. C., and Suo, Y. R. (2014). Isolation, stability, and antioxidant activity of anthocyanins from Lycium ruthenicum murray and Nitraria tangutorum bobr of Qinghai-Tibetan plateau. Sep. Sci. Technol. 49, 2897–2906. doi: 10.1080/01496395.2014.943770
Huang, B., Wang, Z., Park, J. H., Ryu, O. H., Choi, M. K., Lee, J.-Y., et al. (2015). Anti-diabetic effect of purple corn extract on C57BL/KsJ db/db mice. Nutr. Res. Pract. 9, 22–29. doi: 10.4162/nrp.2015.9.1.22
Iannucci, A., Suriano, S., and Codianni, P. (2021). Genetic diversity for agronomic traits and phytochemical compounds in coloured naked barley lines. Plants 10, 1575. doi: 10.3390/plants10081575
Jaakola, L. (2013). New insights into the regulation of anthocyanin biosynthesis in fruits. Trends Plant Sci. 18, 477–483. doi: 10.1016/j.tplants.2013.06.003
Jayarathne, S., Stull, A. J., Park, O.-H., Kim, J. H., Thompson, L., and Moustaid-Moussa, N. (2019). Protective effects of anthocyanins in obesity-associated inflammation and changes in gut microbiome. Mol. Nutr. Food Res. 2019, 1900149. doi: 10.1002/mnfr.201900149
Jia, Y., Selva, C., Zhang, Y., Li, B., McFawn, L. A., Broughton, S., et al. (2020). Uncovering the evolutionary origin of blue anthocyanins in cereal grains. Plant J. 101, 1057–1074. doi: 10.1111/tpj.14557
Jiang, W., Liu, T., Nan, W., Jeewani, D. C., Niu, Y., Li, C., et al. (2018). Two transcription factors TaPpm1 and TaPpb1 co-regulate anthocyanin biosynthesis in purple pericarps of wheat. J. Exp. Bot. 69, 2555–2567. doi: 10.1093/jxb/ery101
Jokioja, J., Linderborg, K. M., Kortesniemi, M., Nuora, A., Heinonen, J., Sainio, T., et al. (2020). Anthocyanin-rich extract from purple potatoes decreases postprandial glycemic response and affects inflammation markers in healthy men. Food Chem. 310, 125797. doi: 10.1016/j.foodchem.2019.125797
Kamburova, V. S., Nikitina, E. V., Shermatov, S. E., Buriev, Z. T., Kumpatla, S. P., Emani, C., et al. (2017). Genome editing in plants: an overview of tools and applications. Int. J. Agron. 2017, 7315351. doi: 10.1155/2017/7315351
Kassegn, H. H. (2018). Determination of proximate composition and bioactive compounds of the Abyssinian purple wheat. J. Cogent Food Agric. 4, 1421415. doi: 10.1080/23311932.2017.1421415
Kato-Nitta, N., Inagaki, Y., Maeda, T., and Tachikawa, M. (2021). Effects of information on consumer attitudes towards gene-edited foods: a comparison between livestock and vegetables. CABI Agric. Biosci. 2, 14. doi: 10.1186/s43170-021-00029-8
Kato-Nitta, N., Maeda, T., Inagaki, Y., and Tachikawa, M. (2019). Expert and public perceptions of gene-edited crops: attitude changes in relation to scientific knowledge. Palgrave Commun. 5, 137. doi: 10.1057/s41599-019-0328-4
Khlestkina, E. K., Usenko, N. I., Gordeeva, E. I., Stabrovskaya, O. I., Sharfunova, I. B., and Otmakhova, Y. S. (2017). Evaluation of wheat products with high flavonoid content: justification of importance of marker-assisted development and production of flavonoid-rich wheat cultivars. Vavilovskii Zhurnal Genetiki i Selektsii 21, 545–553. doi: 10.18699/VJ17.25-o
Khoo, H. E., Azlan, A., Tang, S. T., and Lin, S. M. (2017). Anthocyanidins and anthocyanins: colored pigments as food, pharmaceutical ingredients, and the potential health benefits. Food Nutr. Res. 61, 1361779. doi: 10.1080/16546628.2017.1361779
Kim, J. T., Yi, G., Chung, I. M., Son, B. Y., Bae, H. H., Go, Y. S., et al. (2020). Timing and pattern of anthocyanin accumulation during grain filling in purple waxy corn (Zea mays L.) suggest optimal harvest dates. ACS Omega 5, 15702–15708. doi: 10.1021/acsomega.0c02099
Kim, M. J., Hyun, J. N., Kim, J. A., Park, J. C., Kim, M. Y., Kim, J. G., et al. (2007). Relationship between phenolic compounds, anthocyanins content and antioxidant activity in colored barley germplasm. J. Agric. Food Chem. 55, 4802–4809. doi: 10.1021/jf0701943
Kim, T. J., Kim, S. Y., Park, Y. J., Lim, S.-H., Ha, S.-H., Park, S. U., et al. (2021). Metabolite profiling reveals distinct modulation of complex metabolic networks in non-pigmented, black, and red rice (Oryza sativa L.) cultivars. Metabolites 11, 367. doi: 10.3390/metabo11060367
Krga, I., and Milenkovic, D. (2019). Anthocyanins: from sources and bioavailability to cardiovascular-health benefits and molecular mechanins of action. J. Agric. Food Chem. 67, 1771–1783. doi: 10.1021/acs.jafc.8b06737
Krishnan, V., Singh, A., Sharma, S., Bollinedi, H., Mabalirajan, U., Kota, S., et al. (2020). Nutrient Dense Pigmented Rice: A Diet for Healthier People. TB-ICN:236/2019. New Delhi: Division of Biochemistry; ICAR-Indian Agricultural Research Institute.
Kumari, A., Sharma, S., Sharma, N., Chunduri, V., Kapoor, P., Kaur, S., et al. (2020). Influence of biofortified colored wheats (purple, blue, black) on physicochemical, antioxidant and sensory characteristics of chapatti (Indian Flatbread). Molecules 25, 5071. doi: 10.3390/molecules25215071
Lago, C., Cassani, E., Zanzi, C., Landoni, M., Trovato, R., and Pilu, R. (2014). Development and study of a maize cultivar rich in anthocyanins: colored polenta, a new functional food. Plant Breed. 133, 210–217. doi: 10.1111/pbr.12153
Lago, C., Landoni, M., Cassani, E., Canatluppi, E., Doria, E., Nielsen, E., et al. (2015). Study and characterization of an ancient European flint white maize rich in anthocyanins: Millo Corvo from Galicia. PLoS ONE 10, e0130110. doi: 10.1371/journal.pone.0130110
Lago, C., Landoni, M., Cassani, E., Doria, E., Nielsen, E., and Pilu, R. (2012). Study and characterization of a novel functional food: purple popcorn. Mol. Breed. 31, 575–585. doi: 10.1007/s11032-012-9816-6
Lampila, P., van Lieshout, M., Gremmen, V., and Lähteenmäki, L. (2009). Consumer attitudes towards enhanced flavonoid content in fruit. Food Res. Intl. 42, 122–129. doi: 10.1016/j.foodres.2008.09.002
Li, N., Li, S., Zhang, K., Chen, W., Zhang, B., Wang, D., et al. (2017). ThMYC4E, candidate Blue aleurone 1 gene controlling the associated trait in Triticum aestivum. PLoS ONE 12, e0181116. doi: 10.1371/journal.pone.0181116
Li, T., Zhang, W., Yang, H., Dong, Q., Ren, J., Fan, H., et al. (2019). Comparative transcriptome analysis reveals differentially expressed genes related to the tissue-specific accumulation of anthocyanins in pericarp and aleurone layer for maize. Sci. Rep. 9, 2485. doi: 10.1038/s41598-018-37697-y
Li, X., Lv, X., Wang, X., Wang, L., Zhang, M., and Ren, M. (2018a). Effects of abiotic stress on anthocyanin accumulation and grain weight in purple wheat. Crop Pasture Sci. 69, 1208–1214. doi: 10.1071/CP18341
Li, Y., Wang, H., Zhang, Y., and Martin, C. (2018b). Can the world's favorite fruit, tomato, provide an effective biosynthetic chassis for high-value metabolites? Plant Cell Rep. 37, 1443–1450. doi: 10.1007/s00299-018-2283-8
Li, M., Li, X., Liqin, Y., Li, H., Liu, J., and Huiying, Z. (2019). Breeding and utilization of black glutinous rice variety Ziyunuo. Acta Agric. Jiangxi 31, 29–32.
Liang, L., Li, W., Tian, M., Pan, J., and Feng, Z. (2021). Metabolomic profiling of five hulless barley with different seed coat colors. Genet. Resourc. Crop Evol. doi: 10.21203/rs.3.rs-839743/v1
Liebelt, D. J., Jordan, J. T., and Doherty, C. J. (2019). Only a matter of time: the impact of daily and seasonal rhythms on phytochemicals. Phytochem. Rev. 18, 1409–1433. doi: 10.1007/s11101-019-09617-z
Liet, V. V., Tuan, P. Q., Trung, N. Q., Anh, N. T. N., and Chang, N. T. (2018). Evaluation selection of purple waxy corn lines for new hybrid variety development. Adv. Plants Agric. Res. 8, 90–97. doi: 10.15406/apar.2018.08.00297
Lim, S., Xu, J., Kim, J., Chen, T.-Y., Su, X., Standard, J., et al. (2013). Role of anthocyanin-enriched purple-fleshed sweetpotato P40 in colorectal cancer prevention. Mol. Food. Nutr. Res. 57, 1908–1917. doi: 10.1002/mnfr.201300040
Lin, S., Guo, H., Gong, J. D. U., Liu, M., Lu, M. Y., Wang, L., et al. (2018). Phenolic profiles, β-glucan contents, and antioxidant capacities of colored Qingke (Tibetan hulless barley) cultivars. J. Cereal Sci. 81, 69–75. doi: 10.1016/j.jcs.2018.04.001
Liso, M., De Santis, S., Scarano, A., Verna, G., Dicarlo, M., Galleggiante, V., et al. (2018). A Bronze-tomato enriched diet affects the intestinal microbiome under homeostatic and inflammatory conditions. Nutrients 10, 1862. doi: 10.3390/nu10121862
Liu, X., Feng, Z., Liang, D., Zhang, M., Liu, X., Hao, M., et al. (2020). Development, identification, and characterization of blue-grained wheat-Triticum boeoticum substitution lines. J. Appl. Genet. 61, 169–177. doi: 10.1007/s13353-020-00553-9
Liu, X., Yang, W., Mu, B., Li, S., Li, Y., Zhou, X., et al. (2018a). Engineering of 'purple embryo maize' with a multigene expression system derived from a bidirectional promoter and self-cleaving 2A peptides. Plant Biotechnol. J. 16, 1107–1109. doi: 10.1111/pbi.12883
Liu, X., Zhang, M., Jiang, X., Li, H., Jia, Z., Hao, M., et al. (2021b). TbMYC4A is a candidate gene controlling the blue aleurone trait in a wheat-Triticum boeoticum substitution line. Front. Plant Sci. 12, 762265. doi: 10.3389/fpls.2021.762265
Liu, X., Zhang, M., Wang, D., Peng, T., Liu, X., Hao, M., et al. (2021a). Anthocyanin composition and nutritional properties of a blue-grained wheat-Triticum boeoticum substitution line. Cereal Res. Commun. doi: 10.1007/s42976-021-00199-1
Liu, Y., Tikunov, Y., Schouten, R. E., Marcelis, L. F. M., Visser, R. G. F., and Bovy, A. (2018b). Anthocyanin biosynthesis and degradation mechanisms in Solanaceous vegetables: a review. Front. Chemistry. Front. Chem. 6, 52. doi: 10.3389/fchem.2018.00052
Lloyd, A., Brockman, A., Aguirre, L., Campbell, A., Bean, A., Centero, A., et al. (2017). Advances in the MYB-bHLH-WD repeat (MBW) pigment regulatory model: addition of a WRKY factor and co-option of an anthocyanin MYB for betalain regulation. Plant Cell Physiol. 58, 1431–1441. doi: 10.1093/pcp/pcx075
Long, Z., Jia, Y., Tan, C., Zhang, X.-Q., Angessa, T., Broughton, S., et al. (2019). Genetic mapping and evolutionary analyses of the black grain trait in barley. Front. Plant Sci. 9, 1921. doi: 10.3389/fpls.2018.01921
Lundqvist, U., Franckowiak, J., and Konishi, T. (1997). New and revised descriptions of barley genes. Barley Genet. Newsl. 26, 22–529.
Mackon, E., Ma, Y., Charlie, G., Dongho, J., Mackon, E., Usman, B., et al. (2021). Computational and transcriptomic analysis unraveled OsMATE34 as a putative anthocyanin transporter in black rice (Oryza sativa L.) caryopsis. Genes 12, 583. doi: 10.3390/genes12040583
Mallikarjuna Swamy, B. P., Marundan, S. Jr., Samia, M., Ordonio, R. L., Rebong, D. B., Ordonio, R. L., et al. (2021). Development and characterization of GR2E golden rice introgression lines. Sci. Rep. 11, 2496. doi: 10.1038/s41598-021-82001-0
Mallikarjuna Swamy, B. P., Samia, M., Boncodin, R., Marundan, S. Jr., Rebong, D. B., et al. (2019). Compositional analysis of genetically engineered GR2E 'Golden rice' in comparison to that of conventional rice. J. Agric. Food Chem. 67, 7986–7994. doi: 10.1021/acs.jafc.9b01524
Mattoo, A. K., Dwivedi, S. L., Dutt, S., Singh, B., Garg, M., and Ortiz, R. (2021). Anthocyanin-rich vegetables for human consumption - focus on potato, sweetpotato and tomato. Int. J. Mol. Sci. 23, 2634. doi: 10.20944/preprints202201.0342.v1
Matus, J. M., Aquea, F., and Arce-Johnson, P. (2008). Analysis of the grape MYB R2R3 subfamily reveals expanded wine quality related clades and conserved gene structure organization across Vitis and Arabidopsis genomes. BMC Plant Biol. 8, 83. doi: 10.1186/1471-2229-8-83
Mehta, A. J., Cassidy, A., Litonjua, A. A., Sparrow, D., Vokonas, P., and Schwartz, J. (2016). Dietary anthocyanin intake and age-related decline in lung function: longitudinal findings from the VA Normative Aging Study. Am. J. Clin. Nutr. 103, 542–550. doi: 10.3945/ajcn.115.121467
Mendoza-Mendoza, C. G., Mendoza-Castillo, M. C., and Delgado-Alvarado, A. (2020). Anthocyanins content in the kernel and corncob of Mexican purple corn populations. Maydica 65, M 21.
Mendoza-Mendoza, C. G., Mendoza-Castillo, M. C., Delgado-Alvarado, A., Castillo-Gonzalez, F., Kato-Yamakake, T. A., and Cruz-Izquierdo, S. (2017). Antocianinas totales y parametros de color en lineas de maiz morado. Rev. Fit. Mex. 40, 471–479. doi: 10.35196/rfm.2017.4.471-479
Nankar, A., Grant, L., Scott, P., and Pratt, R. C. (2016b). Agronomic and kernel compositional traits of blue maize landraces from the Southwestern United States. Crop Sci. 56, 2663–2674. doi: 10.2135/cropsci2015.12.0773
Nankar, A. N., Dungan, B., Paz, N., Sudasinghe, N., Schaub, T., Holguin, F. O., et al. (2016a). Quantitative and qualitative evaluation of kernel anthocyanins from southwestern United States blue corn. J. Sci. Food Agric. 96, 4542–4552. doi: 10.1002/jsfa.7671
National Center for Health Statistics (2015). NHANES 2007-2008 Dietary Data. Centers for Disease Control and Prevention (CDC), Atlanta, GA. Available online at: https://wwwn.cdc.gov/nchs/nhanes/search/datapage.aspx?Component=Dietary&CycleBeginYear=2007 (accessed June 27, 2021).
Nkhata, S. G., Chilungo, S., Memba, A., and Mponela, P. (2020). Biofortification of maize and sweetpotatoes with provitamin A carotenoids and implication on eradicating vitamin A deficiency in developing countries. J. Agric. Food Res. 2, 100068. doi: 10.1016/j.jafr.2020.100068
Oliva, N., Cueto-Reaño, M. F., Trijatmiko, K. R., Samia, M., Welsch, R., Schaub, P., et al. (2020). Molecular characterization and safety assessment of biofortified provitamin A rice. Sci. Rep. 10, 1376. doi: 10.1038/s41598-020-57669-5
Paine, J. A., Shipton, C. A., Chaggar, S., Howells, R. M., Kennedy, M. J., Vernon, G., et al. (2005). Improving the nutritional value of golden rice through increased provitamin A content. Nat. Biotechnol. 23, 482–487. doi: 10.1038/nbt1082
Park, S., Choi, M., and Lee, M. (2021). Effects of anthocyanin supplementation on reduction of obesity criteria: a systematic review and meta-analysis of randomized controlled trials. Nutrients 13, 2121. doi: 10.3390/nu13062121
Pascual-Teresa, S., and Sanchez-Ballesta, M. Y. (2008). Anthocyanins: from plant to health. Phytochem. Rev. 6, 281–299. doi: 10.1007/s11101-007-9074-0
Pasqualone, A., Bianco, A. M., Paradiso, V. M., Summo, C., Gambacorta, G., Caponio, F., et al. (2015). Production and characterization of functional biscuits obtained from purple wheat. Food Chem. 180, 64–70. doi: 10.1016/j.foodchem.2015.02.025
Patil, B. S., Crosby, K., Byrne, D., and Hirschi, K. (2014). The intersection of plant breeding, human health, and nutritional security: lessons learned and future perspectives. HortScience 49, 116–127. doi: 10.21273/HORTSCI.49.2.116
Paulsmeyer, M., Chatham, L., Becker, T., West, M., West, L., and Juvik, J. (2017). Survey of anthocyanin composition and concentration in diverse maize germplasms. J. Agric. Food Chem. 65, 4341–4350. doi: 10.1021/acs.jafc.7b00771
Peniche-Pavía, H. A., and Tiessen, A. (2020). Anthocyanin profiling of maize grains using DIESI-MSQD reveals that cyanidin-based derivatives predominate in purple corn, whereas pelargonidin-based molecules occur in red-pink varieties from Mexico. J. Agric. Food Chem. 68, 5980–5994. doi: 10.1021/acs.jafc.9b06336
Petroni, K., Pilu, R., and Tonelli, C. (2014). Anthocyanins in corn: a wealth of genes for human health. Planta 240, 901–911. doi: 10.1007/s00425-014-2131-1
Poole, N., Donovan, D., and Erenstein, O. (2021a). Continuing cereals research for sustainable health and well-being. Int. J. Agric. Sustain. doi: 10.1080/14735903.2021.1975437
Poole, N., Donovan, J., and Erenstein, O. (2021b). Viewpoint: agri-nutrition research: revisiting the contribution of maize and wheat to human nutrition and health. Food Policy. doi: 10.1016/j.foodpol.2020.101976
Qiao, W., Wang, Y., Xu, R., Yang, Z., Sun, Y., Su, L., et al. (2021). A functional chromogen gene C from wild rice is involved in a different anthocyanin biosynthesis pathway in indica and japonica. Theor. Appl. Genet. 134, 1531–1543. doi: 10.1007/s00122-021-03787-1
Rapisarda, P., Fabroni, S., Peterek, S., Russo, G., and Mock, H.-P. (2009). Juice of new citrus hybrids (Citrus clementina Hort. ex Tan. C. sinensis L. Osbeck) as a source of natural antioxidants. Food Chem. 117, 212–218. doi: 10.1016/j.foodchem.2009.03.101
Riaz, B., Chen, H., Wang, J., Du, L., Wang, K., and Ye, X. (2019). Overexpression of maize ZmC1 and ZmR transcription factors in wheat regulates anthocyanin biosynthesis in a tissue-specific manner. Int. J. Mol. Sci.20, 5806. doi: 10.3390/ijms20225806
Rizwan, M., Zhu, Y., Qing, P., Zhang, D., Ahmed, U. I., Xu, H., et al. (2021). Factors determining consumer acceptance of biofortified food: case of zinc-fortified wheat in Pakistan's Punjab province. Front Nutr. 8, 647823. doi: 10.3389/fnut.2021.647823
Rodríguez-Salinas, P. A., Zavala-Garcia, F., Urías-Orona, V., Muy-Rangel, D., Heredia, J. B., and Niño-Medina, G. (2019). Chromatic, nutritional and nutraceutical properties of pigmented native maize (Zea mays L.) genotypes from the northeast of Mexico. Arab J. Sci. Eng. doi: 10.1007/s13369-019-04086-0
Rojas, E. B., Carrillo, M. G. V., Chulím, N. G. E., Salgado, J. H. H., Valverde, B. R., and Delgado, G. B. (2019). Physicochemical characteristics and quality of the protein of pigmented native maize from Morelos in two years of cultivation. Revista Mexicana Ciencias Agrícolas 10, 683–697. doi: 10.29312/remexca.v10i3.481
Roy, S. C., and Shil, P. (2021). Black rice developed through interspecific hybridization (O. sativa x O. rufipogon): origin of black rice gene from Indian wild rice. bioRXIV. doi: 10.1101/2020.12.25.423663
Ryu, S. H., Werth, L., Nelson, S., Scheerens, J., and Pratt, R. C. (2013). Variation of kernel anthocyanin and carotenoid pigment content in USA/Mexico borderland landraces of maize. Econ. Bot. 67, 98–109. doi: 10.1007/s12231-013-9232-9
Saad, K. R., Kumar, G., Mudliar, S. N., Giridhar, P., and Shetty, N. P. (2021). Salt stress-induced anthocyanin biosynthesis genes and MATE transporter involved in anthocyanin accumulation in Daucus carota cell culture. ACS Omega 38, 24502–24514. doi: 10.1021/acsomega.1c02941
Salehi, B., Sharifi-Rad, J., Cappellini, F., Reiner, Z., Zorzan, D., Imran, M., et al. (2020). The therapeutic potential of anthocyanins: current approaches based on their molecular mechanism of action. Front. Pharmacol. 11, 1300. doi: 10.3389/fphar.2020.01300
Salinas-Moreno, Y., Pérez-Alonso, J. J., Vázquz-Carrillo, G., Aragón-Cuevas, F., and Velázquez-ardelas, G. A. (2012). Anthocyanins and antioxidant activity in maize grains (Zea mays L.) of chalqueño, elotes cónicos and bolita races. Agrociencia 46, 693–706.
Salinas-Moreno, Y., Salinas, C. G., Estrada, C. B., and Martínez, V. A. V. (2013). Content and type variability of anthocyanins in blue/purple-colored grains of Mexican maize populations. Revista fitotecnia mexicana 36(Suppl. 3-A), 285–294. doi: 10.35196/rfm.2013.3-S3-A.285
Salinas-Moreno, Y., Sánchez, G. S., Hernández, D. R., and Lobato, N. R. (2005). Characterization of anthocyanin extracts from maize kernels. J. Chromatogr. Sci. 49, 483–487. doi: 10.1093/chromsci/43.9.483
Sandoval-Ramírez, B. A., Catalán, U., Fernández-Castillejo, S., Rubió, L., Macià, A., and Solà, R. (2018). Anthocyanin tissue bioavailability in animals: possible implications for human health. A systematic review. J. Agric. Food Chem. 66, 11531–11543. doi: 10.1021/acs.jafc.8b04014
Scarano, A., Butelli, E., De Santis, S., Cavalcanti, E., Hill, L., De Angelis, M., et al. (2018). Combined dietary anthocyanins, flavonols, and stilbenoids alleviate inflammatory bowel disease symptoms in mice. Front. Nutr. 4, 75. doi: 10.3389/fnut.2017.00075
Sebastian, R. S., Enns, C. W., Goldman, J. D., Martin, C. L., Steinfeldt, L. C., Murayi, T., et al. (2015). A new database facilitates characterization of flavonoid intake, sources, and positive associations with diet quality among US adults. J. Nutr. 145, 1239–1248. doi: 10.3945/jn.115.213025
Sharma, N., Kaur, R., Mangat, G. S., and Singh, K. (2014). Red pericarp introgression lines derived from interspecific crosses of rice: physicochemical characteristics, antioxidative properties and phenolic content. J. Sci. Food Agric. 94, 2912–2920. doi: 10.1002/jsfa.6632
Sharma, N., Kumari, A., Chundury, V., Kaur, S., Banda, J., Goyal, A., et al. (2022). Anthocyanin biofortified black, blue and purple wheat exhibited lower amino acid cooking losses than white wheat. LWT 154, 112802. doi: 10.1016/j.lwt.2021.112802
Sharma, N., Tiwari, V., Vats, S., Kumari, A., Chunduri, V., Kaur, S., et al. (2020a). Evaluation of anthocyanin content, antioxidant potential and antimicrobial activity of black, purple and blue colored wheat flour and wheat-grass juice against common human pathogens. Molecules 25, 5785. doi: 10.3390/molecules25245785
Sharma, S., Chunduri, V., Kumar, A., Kumar, R., Khare, P., Kondepudi, K. K., et al. (2018). Anthocyanin biofortified colored wheat: nutritional and functional characterization. PLoS ONE 13, e0194367. doi: 10.1371/journal.pone.0194367
Sharma, S., Khare, P., Kumar, A., Chanduri, V., Kumar, A., Kapoor, P., et al. (2020b). Anthocyanin-biofortified colored wheat prevents high fat -diet induced alterations in mice: nutrigenomics studies. Mol. Nutr. Food Res. 64, 1900999. doi: 10.1002/mnfr.201900999
Shelton, A. M., Hossain, M. J., Paranjape, V., Azad, A. K., Rahman, M. L., Khan, A. S. M. M. R, et al. (2018). Bt eggplant project in Bangladesh: history, present status, and future direction. Front. Bioeng. Biotechnol. 6, 106. doi: 10.3389/fbioe.2018.00106
Singh, S. P., Gruissem, W., and Bhullar, N. K. (2017). Single locus genetic improvement of iron, zinc, and beta-carotene content in rice grains. Sci. Rep. 7, 1–11. doi: 10.1038/s41598-017-07198-5
Steur, H. D., Stein, A. J., and Demont, M. (2022). From golden rice to golden diets: how to turn its recent approval into practice. Global Food Security 32, 100596. doi: 10.1016/j.gfs.2021.100596
Stobiecki, M., Matysiak-Kata, I., Franski, R., Skala, J., and Szopa, J. (2003). Monitoring changes in anthocyanin and steroid alkaloid glycoside content in lines of transgenic potato plants using liquid chromatography/mass spectrometry. Phytochem 62, 959–969. doi: 10.1016/S0031-9422(02)00720-3
Strygina, K. V., Böner, A., and Khlestkina, E. K. (2017). Identification and characterization of regulatory network components for anthocyanin synthesis in barley aleurone. BMC Plant Biol. 17, 184. doi: 10.1186/s12870-017-1122-3
Su, X., Rhodes, D., Xu, J., Chen, X., Davis, H., Wang, D., et al. (2017). Phenotypic diversity of anthocyanins in sorghum accessions with various pericarp pigments. J. Nutr. Food Sci. 7, 4. doi: 10.4172/2155-9600.1000610
Sun, X., Zhang, Z., Chen, C., Wu, W., Ren, N., Jiang, C., et al. (2018). The C-S-A gene system regulates hull pigmentation and reveals evolution of anthocyanin biosynthesis pathway in rice. J. Exp. Bot. 69, 1485–1498. doi: 10.1093/jxb/ery001
Sweeney, M. T., Thomson, M. J., Cho, Y. G., Park, Y. J., Williamson, S. H., Bustamante, C. D., et al. (2007). Global dissemination of a single mutation conferring white pericarp in rice. PLoS Genet. 3, e133. doi: 10.1371/journal.pgen.0030133
Syed Jaafar, S. N., Baron, J., Siebenhandl-Ehn, S., Rosenau, T., Böhmdorfer, S., and Grausgruber, H. (2013). Increased anthocyanin content in purple pericarp × blue aleurone wheat crosses. Plant Breed. 132, 546–552. doi: 10.1111/pbr.12090
Talsma, E. F., Melse-Boonstra, A., and Brouwer, I. D. (2017). Acceptance and adoption of biofortified crops in low- and middle-income countries: a systematic review. Nutr. Rev. 75, 798–829. doi: 10.1093/nutrit/nux037
Tian, Z., Li, K., Fan, D., Zhao, Y., Gao, X., Ma, X., et al. (2021). Dose-dependent effects of anthocyanin supplementation on platelet function in subjects with dyslipidemia: a randomized clinical trial. EBioMedicine 70, 103533. doi: 10.1016/j.ebiom.2021.103533
Trehan, S., Singh, N., and Kaur, A. (2018). Characteristics of white, yellow, purple corn accessions: phenolic profile, textural, rheological properties and muffin making potential. J. Food Sci. Technol. 55, 2334–2343. doi: 10.1007/s13197-018-3171-5
Urias-Peraldí, M., Gutiérrez-Uribe, J. A., Preciado-Ortiz, R. E., Cruz-Morales, A. S., Serna-Saldívar, S. O., and García-Lara, S. (2013). Nutraceutical profiles of improved blue maize (Zea mays) hybrids for subtropical regions. Field Crops Res. 141, 69–76. doi: 10.1016/j.fcr.2012.11.008
Usenko, N. I., Khlestkina, E., Asavasanti, S., Gordeeva, E. I., Yudina, R. S., and Otmakhova, Y. S. (2018). Possibilities of enriching food products with anthocyanins by using new forms of cereals. Foods Raw Mater. 6, 128–135. doi: 10.21603/2308-4057-2018-1-128-135
Vázquez-Carrillo, M. G., Aparicio-Eusebio, L. A., Salinas-Moreno, Y., María Ofelia Buendía-Gonzalez, M. O., and Santiago-Ramos, D. (2018). Nutraceutical, physicochemical, andsensory properties of blue corn polvorones, a traditional flour-based confectionery. Plant Foods Human Nutr. 73, 321–327. doi: 10.1007/s11130-018-0692-z
Vidana Gamage, G. C., Lim, Y. Y., and Choo, W. S. (2022). Sources and relative stabilities of acylated and nonacylated anthocyanins in beverage systems. J. Food Sci. Technol. 59, 831–845. doi: 10.1007/s13197-021-05054-z
Wallace, T. C., Blumberg, J. B., Johnson, E. J, and Shao, A. (2015). Dietary bioactives: establishing a scientific framework for recommended intakes. Adv. Nutr. 6, 1–4. doi: 10.3945/an.114.007294
Wallace, T. C., and Giusti, M. M. (2015). Anthocyanins. Adv. Nutr. 6, 620–622. doi: 10.3945/an.115.009233
Wallace, T. C., Slavin, M., and Frankenfeld, C. L. (2016). Systematic review of anthocyanins and markers of cardiovascular disease. Nutrients 8, 32. doi: 10.3390/nu8010032
Wang, F., Ji, G., Xu, Z., Feng, B., Zhou, Q., Fan, X., et al. (2021). Metabolomics and transcriptomics provide insights into anthocyanin biosynthesis in the developing grains of purple wheat (Triticum aestivum L.). J. Agric. Food Chem. 69, 11171–11184. doi: 10.1021/acs.jafc.1c01719
Welsch, R., Arango, J., Bär, C., Salazar, B., Al-Babili, S., Beltrán, J., et al. (2010). Provitamin A accumulation in cassava (Manihot esculenta) roots driven by a single nucleotide polymorphism in a phytoene synthase gene. Plant Cell 22, 3348–3356. doi: 10.1105/tpc.110.077560
Woods, B.-J., Gallego-Castillo, S., Talsma, E. F., and Álvarez, D. (2020). The acceptance of zinc biofortified rice in Latin America: a consumer sensory study and grain quality characterization. PLoS ONE 15, e0242202. doi: 10.1371/journal.pone.0242202
Wu, B., Chang, H., Marini, R., Chopra, S., and Reddivari, L. (2021a). Characterization of maize near-isogenic lines with enhanced flavonoid expression to be used as tools in diet-health complexity. Front. Plant Sci. 11, 619598. doi: 10.3389/fpls.2020.619598
Wu, F., Wesseler, J., Zilberman, D., Russell, R. M., Chen, C., and Duboc, A. C. (2021b). Opinion: allow golden rice to save lives. Proc. Natl. Acad. Sci. U.S.A. 118, e2120901118. doi: 10.1073/pnas.2120901118
Xia, D., Zhou, H., Wang, Y., Li, P., Fu, P., Wu, B., et al. (2021). How rice organs are colored: the genetic basis of anthocyanin biosynthesis in rice. Crop J. 9, 598–608. doi: 10.1016/j.cj.2021.03.013
Xu, Z., Xie, J., Zhang, H., Pang, J., Li, Q., Wang, X., et al. (2021). Anthocyanin supplementation at different doses improves cholesterol efflux capacity in subjects with dyslipidemia- a randomized controlled trial. Eur. J. Clin. Nutr. 75, 345–354. doi: 10.1038/s41430-020-0609-4
Yang, X., Wang, J., Xia, X., Zhang, Z., He, J., Nong, B., et al. (2021). OsTTG1, a WD40 repeat gene, regulates anthocyanin biosynthesis in rice. Plant J. 107, 198–214. doi: 10.1111/tpj.15285
Yao, X., Su, L., Yao, Y., An, L., Bai, Y., Li, X., et al. (2022). Isolation, sequencing of the HvnHID gene and its role in the purple grain colour development in Tibetan barley. Czech J. Genet. Plant Breed. 58, 1–9. doi: 10.17221/34/2021-CJGPB
Yao, X., Wu, K., Yao, Y., Bai, Y., Ye, J., and Chi, D. (2018). Construction of a high-density genetic map: genotyping by sequencing (GBS) to map purple seed coat color (Psc) in hulless barley. Hereditas 155, 37. doi: 10.1186/s41065-018-0072-6
Ye, X., Al-Babili, S., Klöti, A., Zhang, J., Lucca, P., Beyer, P., et al. (2000). Engineering the provitamin A (beta-carotene) biosynthetic pathway into (carotenoid-free) rice endosperm. Science 287, 303–305. doi: 10.1126/science.287.5451.303
Yu, S., and Tian, L. (2018). Breeding major cereal grains through the lens of nutrition sensitivity. Mol. Plant 11, P23–P30. doi: 10.1016/j.molp.2017.08.006
Zaidi, S., Zakari, S. A., Zhao, Q., Khan, A. R., Shah, J. M., and Cheng, F. (2019). Anthocyanin accumulation in black kernel mutant rice and its contribution to ROS detoxification in response to high temperature at the filling stage. Antioxidants 8, 510. doi: 10.3390/antiox8110510
Zamora-Ros, R., Andres-Lacueva, C., Lamuela-Ravent, R. M., Berenguer, T., Jakszyn, P., Barricarte, A., et al. (2010). Estimation of dietary sources and flavonoid intake in a Spanish adult population (EPIC-Spain). J. Am. Dietetic Assoc. 110, 390–398. doi: 10.1016/j.jada.2009.11.024
Zamora-Ros, R., Knaze, V., Lujan-Barroso, L., Romieu, I., Scalbert, A., Slimani, N., et al. (2013). Differences in dietary intakes, food sources and determinants of total flavonoids between Mediterranean and non-Mediterranean countries participating in the European prospectiveiInvestigation into cancer and nutrition (EPIC) study. Brit. J. Nut. 109, 1498–1507. doi: 10.1017/S0007114512003273
Zeven, A. C. (1991). Wheats with purple and blue grains: a review. Euphytica 56, 243–258. doi: 10.1007/BF00042371
Zhang, H., Shao, Y., Bao, J., and Beta, T. (2015). Phenolic compounds and antioxidant properties of breeding lines between the white and black rice. Food Chem. 172, 630–639. doi: 10.1016/j.foodchem.2014.09.118
Zhang, T., Ma, J., Wu, X., Hao, Z., Dun, C., and Chen, C. (2021). Qualitative and semi-quantitative assessment of anthocyanins in Tibetan hulless barley from different geographical locations by UPLC-QTOF-MS and their antioxidant capacities. Open Chem. 19, 1–8. doi: 10.1515/chem-2020-0186
Zhao, S., Xi, X., Zong, Y., Li, S., Li, Y., Cao, D., et al. (2020). Overexpression of ThMYC4E enhances anthocyanin biosynthesis in common wheat. Int. J. Mol. Sci. 21, 137. doi: 10.3390/ijms21010137
Zhao, Y., Xu, H., Tian, Z., Wang, X., Xu, L., Li, K., et al. (2021). Dose-dependent reductions in plasma ceramides after anthocyanin supplementation are associated with improvements in plasma lipids and cholesterol efflux capacity in dyslipidemia: a randomized controlled trial. Clinical Nutr. 40, P1871–1878. doi: 10.1016/j.clnu.2020.10.014
Zheng, J., Wu, H., Zhao, M., Yang, Z., Zhou, Z., Guo, Y., et al. (2021). OsMYB3 is a R2R3-MYB gene responsible for anthocyanin biosynthesis in black rice. Mol. Breed. 41, 51. doi: 10.1007/s11032-021-01244-x
Zhou, C., Zeng, Z., Suo, J., Li, X., Bian, H., Wang, J., et al. (2021). Manipulating a single transcription factor, Ant1, promotes anthocyanin accumulation in barley grains. J. Agric. Food Chem. 69, 5306–5317. doi: 10.1021/acs.jafc.0c08147
Zhu, Q., Yu, S., Zeng, D., Liu, H., Wang, H., Yang, Z., et al. (2017). Development of ”purple endosperm rice” by engineering anthocyanin biosynthesis in the endosperm with a high-efficiency transgene stacking system. Mol. Plant. 10, 918–929. doi: 10.1016/j.molp.2017.05.008
Zhu, Y., Lin, Y., Chen, S., Liu, H., Chen, Z., Fan, M., et al. (2019). CRISPR/Cas9-mediated functional recovery of the recessive rc allele to develop red rice. Plant Biotechnol. J. 17, 2096–2105. doi: 10.1111/pbi.13125
Keywords: food bioactives, malnutrition, human health, biotechnology, cereal crops
Citation: Dwivedi SL, Mattoo AK, Garg M, Dutt S, Singh B and Ortiz R (2022) Developing Germplasm and Promoting Consumption of Anthocyanin-Rich Grains for Health Benefits. Front. Sustain. Food Syst. 6:867897. doi: 10.3389/fsufs.2022.867897
Received: 01 February 2022; Accepted: 14 March 2022;
Published: 25 April 2022.
Edited by:
Giuseppina Rea, National Research Council (CNR), ItalyReviewed by:
Roberto Pilu, University of Milan, ItalyCopyright © 2022 Dwivedi, Mattoo, Garg, Dutt, Singh and Ortiz. This is an open-access article distributed under the terms of the Creative Commons Attribution License (CC BY). The use, distribution or reproduction in other forums is permitted, provided the original author(s) and the copyright owner(s) are credited and that the original publication in this journal is cited, in accordance with accepted academic practice. No use, distribution or reproduction is permitted which does not comply with these terms.
*Correspondence: Autar K. Mattoo, YXV0YXIubWF0dG9vQHVzZGEuZ292
Disclaimer: All claims expressed in this article are solely those of the authors and do not necessarily represent those of their affiliated organizations, or those of the publisher, the editors and the reviewers. Any product that may be evaluated in this article or claim that may be made by its manufacturer is not guaranteed or endorsed by the publisher.
Research integrity at Frontiers
Learn more about the work of our research integrity team to safeguard the quality of each article we publish.