- 1Technology Transfer Centre, Edmund Mach Foundation, San Michele all'Adige, Italy
- 2Department of Agronomy, Food, Natural Resources, Animals and Environment, University of Padova, Legnaro, Italy
- 3Research and Innovation Centre, Edmund Mach Foundation, San Michele all'Adige, Italy
The western honey bee, Apis mellifera, is the most important and widespread managed pollinator species. Honey bee diet is based on nectar and pollen, and pollen diversity and composition, in particular, affect colony health and fitness. As landscape composition is strongly linked to floral resource heterogeneity, it could influence the resource intake of honey bees. This work aimed to explore how the composition of pollen collected by honey bees was modulated by seasonality and landscape composition heterogeneity in a mountainous cultivated area of Northern Italy. We selected 13 locations, and at each location, we placed two honey bee colonies from which we collected pollen samples every month during the whole flowering season for two consecutive years. We then analyzed pollen samples in the laboratory and determined the Shannon diversity index of each pollen sample and the temporal pollen taxon replacement. We extracted the cover of the main habitat types at three spatial scales and tested the effect of landscape diversity and composition using Principal Component Analysis. Honey bees foraged on a high number of floral resources, however, they mostly collected pollen from a small number of taxa, with pollen type composition changing throughout the flowering season. In early spring and late summer, most pollen grains were collected from a few plant species, while from May to August the number of collected pollen types was significantly higher. Landscape composition affected pollen diversity only at the end of the flowering season. While honey bees were able to collect highly diverse pollen throughout spring and summer regardless of landscape composition, in late summer, when pollen collected is fundamental for the overwintering of the colony and its development in the following season, semi-natural areas became crucial for honey bee foraging activities, with pollen diversity increasing with increasing percentages of semi-natural areas. Our research highlighted the importance for honey bees of certain seasonal resources and of semi-natural habitats at the end of the flowering season, which ensure the subsistence of their colonies throughout the year.
Introduction
In recent years, pollinator abundance and diversity faced a strong decline due to multiple anthropogenic pressures (Potts et al., 2010). One of the main causes of this decline is the loss and fragmentation of natural areas, which led to a decrease in plant diversity, potentially determining insufficient nutrition for pollinators (Goulson et al., 2008). In particular, the honey bee, Apis mellifera Linnaeus, is the most widespread managed pollinator species and its presence is crucial not only for ensuring the reproduction of plant species in natural habitats but also for crop production, which is positively impacted by honey bee pollination both in terms of quantity and quality (Hung et al., 2018; Rollin and Garibaldi, 2019).
The honey bee is a eusocial species whose colonies can host more than 50,000 individuals (Von Frisch, 1954; Fontana, 2019). Its diet is based on nectar and pollen. Nectar is a source of energy and, after being transformed into honey, it constitutes the food stocks through which the colony survives during winter. Pollen is a source of protein and lipids, and in addition to direct feeding of larvae and adult workers, it is necessary for the secretion of two substances essential for the life of the colony, i.e., the royal jelly, which is the food for all the larvae in the first 3 days and for queens during their whole life (Winston, 1991; Fontana, 2019), and the wax, of which honeycombs are made (Hepburn, 1986; Tautz, 2008). Pollen availability not only influences the development and reproduction of the colony in the short term, but as for honey, it is also fundamental for the overwintering of the colony and its development in the following season (Alaux et al., 2017).
The quality of pollen in terms of nutrient content varies from one plant species to another (Roulston and Cane, 2000), and for this reason, honey bees must have access to diverse pollen sources in order to assure colony health. Only landscapes with a certain degree of floristic diversity can therefore guarantee adequate resources for honey bees (Di Pasquale et al., 2016). Recent studies showed that the proportion of semi-natural habitat within the landscape is positively related to honey bee pollen diversity and protein content (Donkersley et al., 2014; Cannizzaro et al., 2022) and to the probability of winter survival of the colonies (Rutschmann et al., 2022), while habitat fragmentation negatively affects the abundance of pollen collected by honey bees (Ochungo et al., 2021). Moreover, heterogeneous landscapes have been shown to support honey bees also by reducing their foraging distances, therefore allowing them to consume fewer resources to obtain food (Danner et al., 2017).
In this work, we aimed at understanding the effect of landscape composition and seasonality on the diversity of pollen collected by honey bees in a mountainous cultivated area in Northern Italy. We selected 13 locations, from which we collected pollen samples monthly from two honey bee colonies during spring and summer of 2019 and 2020. After analyzing pollen samples in the laboratory, we determined the Shannon diversity index for each pollen sample and temporal beta diversity of pollen at each location. To assess landscape heterogeneity, we calculated the cover of the main habitat types at 1, 3, and 5 km radius buffers around the sampling locations, and analyzed landscape composition through Principal Component Analysis and Shannon diversity index. We hypothesize that pollen composition would change throughout the flowering season, following plant phenology at least in early spring and late summer, when floral resources are relatively scarce. Moreover, we expect that landscape composition would strongly affect pollen composition, with high-diverse landscapes supporting honey bee colonies by offering a wider range of pollen types in comparison to homogeneous landscapes.
Materials and Methods
Study Area
The study was carried out in the Trentino province, an area in Northern Italy covering about 6,214 km2. The area is generally mountainous, but it is characterized by a considerable landscape heterogeneity, with about 80% semi-natural areas, 15% agricultural areas, and 5% urban areas. As a result, the climate is highly variable. The mean annual temperature is about 9°C, and the mean annual precipitation at 200 m a.s.l. is 1,200 mm.
We selected 13 sampling locations, which were characterized by great variability in landscape composition (Supplementary Table 1; Figure 1). Three sites were close to apple orchards, three were close to vineyards, three were in an urban setting, and three were far from agricultural areas. The average elevation of the sampling locations was 533 m a.s.l. (min = 93 m a.s.l., max = 1,481 m a.s.l.). The mean air temperature at the sampling sites during the sampling periods, i.e., from April to September of 2019 and 2020, was 17°C.
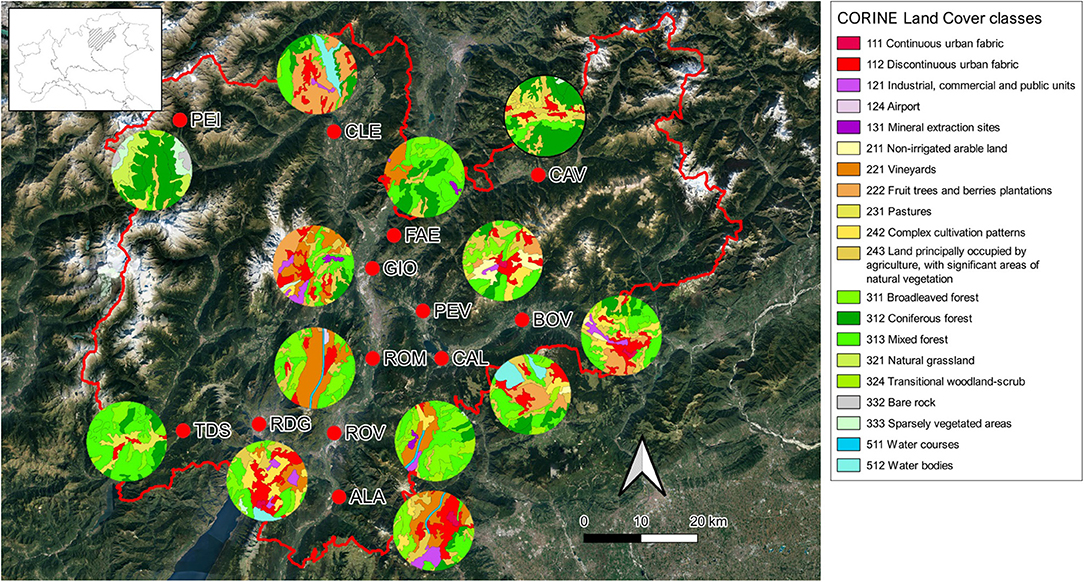
Figure 1. Map of the 13 sampling locations, also showing the landscape composition at 3 km radius buffers around the sampling locations using CORINE Land Cover classes. Location acronyms are ALA (Ala), BOV (Borgo Valsugana), CAL (Caldonazzo), CAV (Cavalese), CLE (Cles), FAE (Faedo), GIO (Giovo), PEI (Peio), PEV (Pergine Valsugana), RDG (Riva del Garda), ROM (Romagnano), ROV (Rovereto), and TDS (Tiarno di Sopra). See Supplementary Table 1 for additional information on sampling locations.
Experimental Design
In 2019 and 2020, we placed one small apiary consisting of two honey bee colonies at each sampling location. All colonies were originated from the livestock managed by Edmund Mach Foundation and had sister queens of A. m. carnica x A. m. ligustica. The colonies were managed directly by Edmund Mach Foundation personnel according to the local beekeeping practice. From April to September, we carried out pollen samplings every month, for a total of six pollen samples collected per colony per year. At some locations, however, the number of pollen samples was lower due to adverse climatic conditions. In particular, in 2019, at one location only three samples could be collected, and only five at other two. In 2020, only five samples were collected at three locations. Pollen samples were collected activating pollen traps at the hive entrance for 48 h, Pollen samples were then stored at −20°C.
Landscape Composition
For each sampling location, we extracted the cover of the main habitat types using the CORINE Land Cover (CLC) database (© European Union, Copernicus Land Monitoring Service 2018, European Environment Agency) at three spatial scales, i.e., the local foraging scale of honey bees (1 km radius buffer around the sampling locations) and two landscape foraging scales of honeybees (3 and 5 km radius buffers around the sampling locations). Following the CLC classification, we considered a total of 24 land-use classes (Supplementary Table 2). Landscape composition was heterogeneous across sites. In 3 km radius buffers around the sampling locations, an average of 33% of the land was covered by agricultural areas (min = 7%, max = 52%), 51% by semi-natural areas (min = 26%, max = 92%), 13% by urban areas and other artificial surfaces (min = 0, max = 30%), and 2% by other areas (min = 0, max = 13%) (Supplementary Table 1).
As most of these classes were highly correlated, we performed a Principal Component Analysis (PCA) to extract the landscape composition at each of the three spatial scales. We extracted the first two eigenvalues, PC1 and PC2, which explained 38, 48, and 45% of the total landscape variability at 1, 3, and 5 km radius buffers around the sampling locations (Supplementary Table 3). PC1 was positively related to semi-natural areas, in particular coniferous forests, natural grasslands, and areas with sparse vegetation, and negatively related to intensive areas, in particular urban areas and vineyards (Supplementary Table 3). Therefore, high values of PC1 can be interpreted as a high proportion of semi-natural areas within the landscapes.
Moreover, we calculated the Shannon diversity index for landscape composition using the 24 land-use classes at each of the three spatial scales. Shannon diversity index quantifies the heterogeneity of landscapes, taking into account both richness and evenness of land-use classes, with low values of the index indicating a low landscape heterogeneity. Shannon diversity index was calculated using the R package vegan (Oksanen et al., 2020). All statistical analyses were performed using the R software version 3.6.1 (R Core Team, 2019).
Pollen Analysis
From each pollen sample, we extracted two grams of pollen pellets, which were dissolved in distilled water and mixed using an advanced vortex mixer (VELP Scientifica, ZX3). We took 20 μl of the obtained suspension and placed it on a microscopic slide. Once the suspension of water and pollen was dry, we placed a drop of glycerin jelly on top of the sediment, and we covered it with a slide. Pollen was then observed under the optical microscope (Optika, B500PPH). For each sample, we counted about 500 pollen grains applying the “transect” method (Tamic et al., 2011). Pollen grains were identified at the lowest possible taxonomic level according to available literature (Ricciardelli d'Albore, 1998; Bucher, 2004; El-Labban, 2020) and palynological databases (PalDat, 2000; PollenAtlas). The identified pollens were classified following the “pollen types” nomenclature proposed by Persano Oddo and Ricciardelli d'Albore (1989).
For each pollen sample, we calculated the Shannon diversity index. As for landscape composition, the Shannon index for pollen reflects both richness and evenness of pollen samples, with lower values indicating a lower diversity in pollen sample composition. Moreover, to understand how pollen composition changed throughout the flowering season, we calculated the mean beta richness and replacement at each location over the six sampling months, based on presence/absence data. All pollen indices were calculated using the R package vegan (Oksanen et al., 2020).
Statistical Analysis
First, to determine the effect of landscape composition and seasonality on pollen diversity, we built two linear mixed-effect models for each spatial scale using the R package nlme (Pinheiro et al., 2019). In all models, the response variable was pollen Shannon index. Selected explanatory variables were collection month, year, landscape Shannon index, and the interaction between month and landscape Shannon index for the first model, and collection month, year, PC1, PC2, and the interactions between month and PC1 and between month and PC2 for the second model. We also included the sampling location as random factor in all models. Starting from each full model, we used a backward deletion procedure, removing one-by-one the interactions with p-value > 0.05, and re-ran the model to correctly interpret model main effects.
Second, to explore the effect of landscape composition on temporal beta diversity of pollen, we built four linear models for each spatial scale. We selected beta richness and replacement of pollen as response variables, PC1 and PC2 as explanatory variables for the first model, and landscape Shannon index as explanatory variable for the second model.
Results
We analyzed a total of 116,979 pollen grains in 224 samples collected during 2 years. We identified 122 plant taxa, most of them (n = 93) at the genus level (Supplementary Table 4). We observed 48 pollen types in April, 80 in May, 77 in June, 67 in July, 69 in August, and 50 in September. The most abundant types were Hedera spp. (n = 16,896 pollen grains), Plantaginaceae (n = 10,303 pollen grains), and Malus/Pyrus spp. (n = 7,826 pollen grains). On the other hand, the most prevalent taxa were Compositae T-form, which includes the genera Taraxacum and Cichorium (found in 149 pollen samples), Compositae H-form, which includes the genera Helianthus, Petasites, and Senecio (found in 117 pollen samples), and Trifolium repens group (found in 110 pollen samples).
The monthly pollen samples were dominated by a handful of taxa, and there was a strong temporal turnover in the composition of pollen samples (Figure 2). In spring, honey bees mostly collected pollen on Malus/Pyrus spp. (21% of total pollen grains), Salix spp. (18%), and Compositae T-form (12%), with only three species making up half of the collected pollen grains (Figures 2A,B). In June, Castanea sativa became the prevalent pollen type (29%), together with T. repens group (8%), Filipendula spp. (7%), and Vitis spp. (6%) (Figure 2C). In July, the most visited taxa were Plantaginaceae (39%), T. repens group (9%), Clematis spp. (7%), and Parthenocissus spp. (7%) (Figure 2D). Plantaginaceae were also found in August (16%), but pollen was mostly collected on Artemisia spp. (22%) and, to a lesser extent, on Compositae H-form (9%) and Thalictrum spp. (8%) (Figure 2E). In September, almost all pollen was collected on Hedera spp. (79%) (Figure 2F).
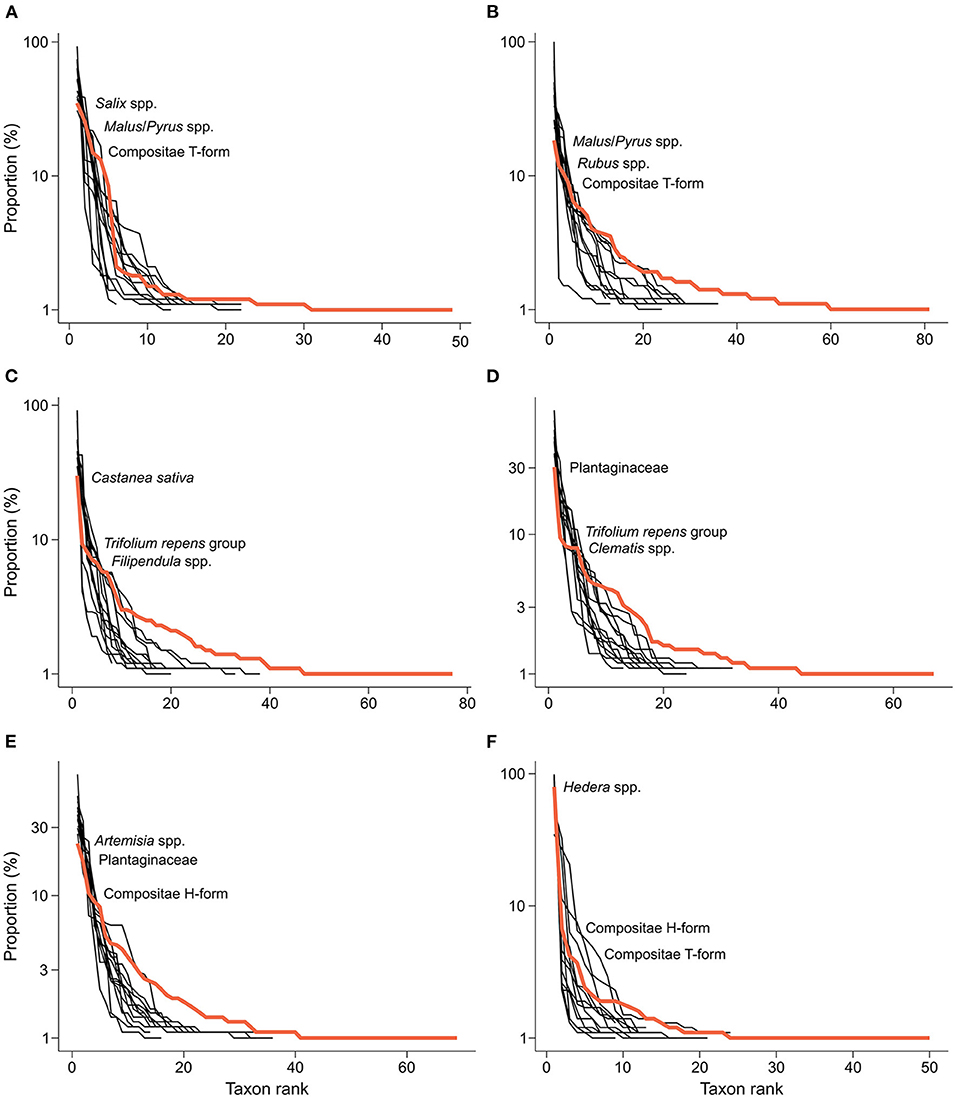
Figure 2. Rank abundance plots showing the relative proportion of the taxa found in pollen samples collected in (A) April, (B) May, (C) June, (D) July, (E) August, and (F) September of 2019 and 2020. Black lines represent single sampling locations, while colored lines represent the average of all locations for each sampling month. Species are ranked on the x-axis from left to right from the most to least abundant. Y-axis is log-transformed to improve clarity.
Pollen Shannon index was strongly influenced by the collection month (Table 1). Pollen diversity was higher in May, July, and August, while pollen samples of April and particularly September were more homogeneous (Figure 3A). Moreover, pollen Shannon index responded to the interaction between month and landscape PC1 at 3 and 5 km. Landscape composition had no effect on pollen diversity from April to August, however, in September the diversity of collected pollen increased with increasing landscape PC1, suggesting a positive effect of semi-natural habitat on pollen collection in late summer (Figures 3B,C).
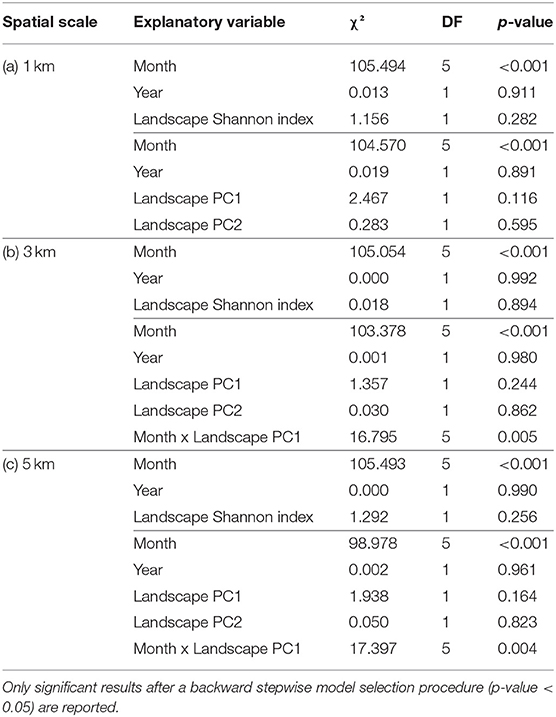
Table 1. Results of the linear mixed-effect models testing the response of pollen Shannon index at the three spatial scales, i.e., (a) 1 km radius buffer, (b) 3 km radius buffer, and (c) 5 km radius buffer around the sampling locations, to month, year, landscape Shannon index, and the interaction between month and landscape Shannon index; and month, year, landscape PC1 and PC2, and the interactions between month and landscape PC1 and month and landscape PC2.
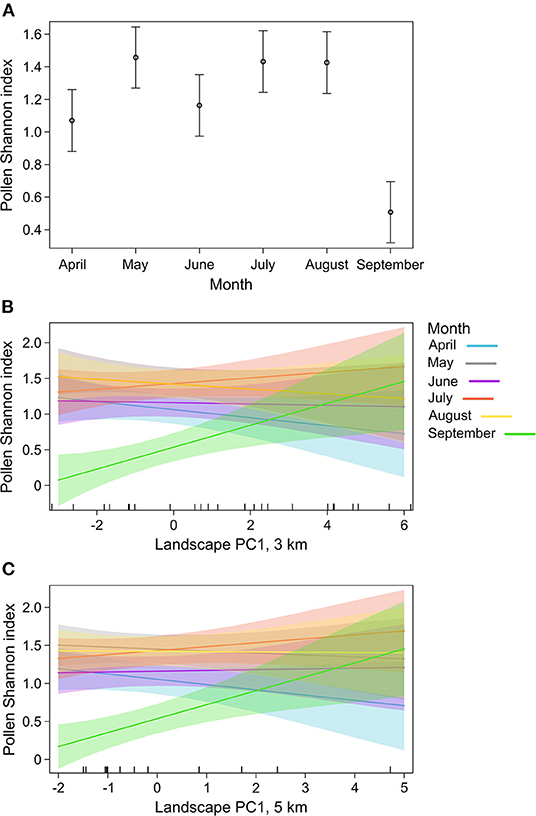
Figure 3. Plot showing the effect on pollen Shannon index of (A) collection month, (B) the interaction between month and landscape PC1 at 3 km radius buffer around the sampling locations, and (C) the interaction between month and landscape PC1 at 5 km radius buffer around the sampling locations. Plots include model estimates (points and lines) and 95% confidence intervals (bars and shaded areas).
Temporal beta richness and replacement did not change in response to landscape composition at any of the selected spatial scales (Table 2). In general, beta richness values were high at all locations (min = 0.783, max = 0.880), while beta replacement values were particularly low (min = 0.235, max = 0.342).
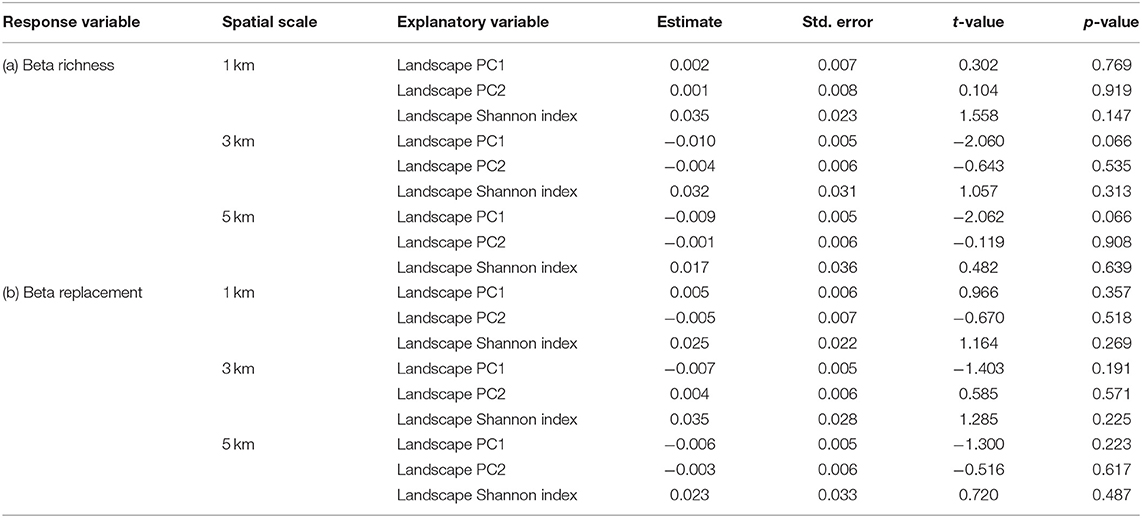
Table 2. Results of the linear models testing the response of (a) mean beta richness and (b) mean beta replacement of pollen samples to PC1 and PC2 at the three spatial scales, i.e., 1 km radius buffer, 3 km radius buffer, and 5 km radius buffer around the sampling locations.
Discussion
The survival, prosperity, and reproduction of honey bee colonies depend on the ability of honey bees to collect and store honey and pollen (Brodschneider and Crailsheim, 2010). In this study, by observing almost 117,000 pollen grains, we were able to collect information on honey bee foraging behavior from early spring to late summer. Moreover, we highlighted how pollen diversity was strongly shaped by seasonality, while landscape composition affected pollen diversity only at the end of the flowering season.
Effect of Seasonality on Honey Bee Foraging Preferences and Pollen Diversity
Honey bees, despite being extraordinarily polylectic, usually select a limited number of flowering plant species to forage on (Lau et al., 2019). Here, we observed a strong temporal turnover in the composition of pollen collected by honey bees, which partly reflects honey bee foraging preferences, and partly reflects plant phenology and pollen availability in the study area, at least in the early and late flowering season. In particular, trees were revealed to be a key resource for honey bees in spring. Salix spp. in April, Malus/Pyrus spp. in May, and Castanea sativa in June were the main pollen taxa collected by honey bees. The importance of trees for honey bees is well-known (Donkersley, 2019), as they are often among the early-flowering species. We showed that Compositae such as Taraxacum spp., Helianthus spp., and Senecio spp., and the legume Trifolium repens strongly supported honey bees throughout spring and summer. In August, Artemisia spp. pollen was highly represented, as this taxon is common in fallows and urban areas. In September, almost all pollen was collected on Hedera spp., which was the most abundant flowering plant species in late summer in the study area (Prosser et al., 2019).
Pollen diversity was also shaped by seasonality. We observed that the start and end of the flowering season, i.e., April and September, were characterized by a dearth of floral resources, while we observed a peak of pollen diversity in May. Interestingly, despite the high percentage of agricultural areas in certain landscapes, we did not highlight a strong effect of mass flowering crops such as apple, which flowers in April and May, which can potentially reduce the diversity of pollen collected by honey bees, as bees tend to focus on these resources. The diversity of pollen collected in August was surprisingly high, given that resources are usually relatively scarce at the end of summer (Garbuzov et al., 2015; Requier et al., 2015; Danner et al., 2017; Sponsler et al., 2020). The high diversity found in August could be explained by the mid-elevation of sampling sites, which causes a shift in pollen decline from August to September.
Since many of the pollen taxa that we collected were grouped at the family level, and almost all the remaining ones were identified at the genus level, pollen diversity could have been even higher in some seasons, if we had been able to achieve species-level identifications of pollen.
Interactive Effect of Seasonality and Landscape Composition on Pollen Diversity
Our results highlighted that diversity of pollen collected by honey bees was influenced by the interaction between collection month and landscape composition, i.e., the proportion of semi-natural areas.
Pollen diversity was independent of the proportion of semi-natural areas from April to August. Several studies found that pollen composition was not affected by landscape composition (Danner et al., 2017; Guzman et al., 2019; Simanonok et al., 2020; Jones et al., 2021). This can be explained by both the structure of selected landscapes and the foraging behavior of the honey bee. Even if some landscapes were strongly modified by anthropogenic activities, they always included a certain proportion of semi-natural habitats, ranging from 25 to 83%, which comprised both open habitats and forests. Moreover, many agricultural areas, which ranged from 7 to 52%, were intermixed with semi-natural habitats. In all landscapes, honey bees were therefore able to collect pollen in areas that offered a high amount of resources, at least until mid-summer. Moreover, honey bees can travel more than 10 km from their hive, although they usually forage <1 km away from the hive (Von Frisch, 1967; Visscher and Seeley, 1982; Tautz, 2008; Seeley, 2019). Several studies highlight that landscape composition affects the distance to which honey bees forage, and in particular that their foraging distance increases in simplified landscapes (Steffan-Dewenter and Kuhn, 2003; Abou-Shaara, 2014; Danner et al., 2017). A study from the UK reports that in landscapes dominated by the common heather (Calluna vulgare), the average distance of foraging honey bees strongly changed during the season: while in May it was about 1 km, in August, during the flowering period of the common heather, the average foraging distance increased up to 5.5 km (Beekman and Ratnieks, 2000). In our study, however, we did not collect data on how far honey bees traveled to collect pollen. In some areas, honey bees may need to travel much further to obtain food resources, with potentially negative consequences for colony fitness.
On the other hand, in September, pollen diversity increased with increasing proportion of semi-natural habitats at 3 and 5 km radius buffers. While honey bees are able to collect heterogeneous pollen independently of landscape composition from spring to mid-summer, the scarcity of floral resources in late summer may turn semi-natural areas into key habitats. In this part of the season, when nectar sources are decreasing (Tew et al., 2022), honey bees search for the most diverse pollen sources in order to breed winter individuals that must develop adequate fat bodies (Frias et al., 2016). Semi-natural areas in the landscape can promote late-season pollen protein and winter survival of honey bee colonies (Kuchling et al., 2018; Simanonok et al., 2020; Rutschmann et al., 2022). Therefore, the higher number of resources offered by these habitats can be crucial for honey bees at such a critical stage of the colony cycle.
Conclusion
Our work highlighted that diversity of pollen was shaped by seasonality, as we observed a strong temporal turnover in the diversity of pollen collected by honey bees. Landscape composition only affected pollen diversity at the end of the flowering season. In spring and summer, honey bees were able to efficiently forage in all landscapes, probably even due to the presence of a few key plant species such as Trifolium repens that could strongly support colonies (Filipiak et al., 2017). In late summer, when resources were generally scarce, semi-natural areas became fundamental for honey bees, as they offered a wider range of floral resources. However, more research on this topic is needed, as landscape composition could also affect other aspects of honey bee ecology. For example, complementing this study with observations on foraging flight distances and colony fitness could help elucidate the potential effect of landscape simplification on honey bees. Moreover, another aspect that should be taken into account is the potential contamination of food sources for bees, as the presence of intensively cultivated areas can affect pollen quality due to pesticide presence (Zioga et al., 2020).
Data Availability Statement
The raw data supporting the conclusions of this article will be made available by the authors, without undue reservation.
Author Contributions
VM, PF, GA, and CI designed the research. VM, LZ, and PF performed the fieldwork. VM performed the palynological analysis. AC, LM, and RZ analyzed the data. VM, AC, LM, and PF wrote the article. All authors have read and approved the final version of the manuscript.
Conflict of Interest
The authors declare that the research was conducted in the absence of any commercial or financial relationships that could be construed as a potential conflict of interest.
Publisher's Note
All claims expressed in this article are solely those of the authors and do not necessarily represent those of their affiliated organizations, or those of the publisher, the editors and the reviewers. Any product that may be evaluated in this article, or claim that may be made by its manufacturer, is not guaranteed or endorsed by the publisher.
Acknowledgments
We thank C. Martinello, M. Vettori, G. Deimichei, G. Cellana, G. Migazzi, and G. Mora for their help during the fieldwork.
Supplementary Material
The Supplementary Material for this article can be found online at: https://www.frontiersin.org/articles/10.3389/fsufs.2022.865368/full#supplementary-material
References
Abou-Shaara, H. F.. (2014). The foraging behaviour of honey bees, Apis mellifera: a review. Vet. Med. Czech. 59, 1–10. doi: 10.17221/7240-VETMED
Alaux, C., Allier, F., Decourtye, A., Odoux, J. F., Tamic, T., Chabirand, M., et al. (2017). A ‘Landscape physiology' approach for assessing bee health highlights the benefits of floral landscape enrichment and semi-natural habitats. Sci. Rep. 7, 40568. doi: 10.1038/srep40568
Beekman, M., and Ratnieks, F. L. W. (2000). Long-range foraging by the honey-bee, Apis mellifera L. Funct. Ecol. 14, 490–496. doi: 10.1046/j.1365-2435.2000.00443.x
Brodschneider, R., and Crailsheim, K. (2010). Nutrition and health in honey bees. Apidologie 41, 278–294. doi: 10.1051/apido/2010012
Bucher, E.. (2004). Lo Spettro Pollinico dei Mieli dell'Alto Adige. Bolzano: Laboratorio biologico dell'Agenzia per la protezione dell'ambiente e la tutela del lavoro. p. 676.
Cannizzaro, C., Keller, A., Wilson, R. S., Elliott, B., Newis, R., Ovah, R., et al. (2022). Forest landscapes increase diversity of honey bee diets in the tropics. For. Ecol. Manage. 504, 119869. doi: 10.1016/j.foreco.2021.119869
Danner, N., Keller, A., Härtel, S., and Steffan-Dewenter, I. (2017). Honey bee foraging ecology : season but not landscape diversity shapes the amount and diversity of collected pollen. PLoS ONE 12, 9–12. doi: 10.1371/journal.pone.0183716
Di Pasquale, G., Alaux, C., Le Conte, Y., Odoux, J.-F., Pioz, M., Vaissière, B. E., et al. (2016). Variations in the availability of pollen resources affect honey bee health. PLoS ONE 11, e0162818. doi: 10.1371/journal.pone.0162818
Donkersley, P.. (2019). Trees for bees. Agric. Ecosyst. Environ. 270–271, 79–83. doi: 10.1016/j.agee.2018.10.024
Donkersley, P., Rhodes, G., Pickup, R. W., Jones, K. C., and Wilson, K. (2014). Honeybee nutrition is linked to landscape composition. Ecol. Evol. 4, 4195–4206. doi: 10.1002/ece3.1293
El-Labban, M.. (2020). Beekeepers' Guide for Pollen Identification of Honey. Jayh: Mohammad El-Labban.
Filipiak, M., Kuszewska, K., Asselman, M., Denisow, B., Stawiarz, E., Woyciechowski, M., et al. (2017). Ecological stoichiometry of the honeybee: pollen diversity and adequate species composition are needed to mitigate limitations imposed on the growth and development of bees by pollen quality. PLoS ONE 12, 1–31. doi: 10.1371/journal.pone.0183236
Fontana, P.. (2019). The Joy of bees, Bees as a Model of Sustainability and Beekeeping as an Experience of Nature and Human History. Verona: WBA Project.
Frias, B. E. D., Barbosa, C. D., and Lourenço, A. P. (2016). Pollen nutrition in honey bees (Apis mellifera): impact on adult health. Apidologie 47, 15–25. doi: 10.1007/s13592-015-0373-y
Garbuzov, M., Schurch, R., and Ratnieks, F. L. W. (2015). Eating locally: dance decoding demonstrates that urban honey bees in Brighton, UK, forage mainly in the surrounding urban area. Urban Ecosyst. 18, 411–418. doi: 10.1007/s11252-014-0403-y
Goulson, D., Lye, G. C., and Darvill, B. (2008). Decline and conservation of bumble bees. Annu. Rev. Entomol. 53, 191–208. doi: 10.1146/annurev.ento.53.103106.093454
Guzman, A., Gaines-Day, H. R., Lois, A. N., Steffan, S. A., Brunet, J., Zalapa, J., et al. (2019). Surrounding landscape and spatial arrangement of honey bee hives affect pollen foraging and yield in cranberry. Agric. Ecosyst. Environ. 286, 106624. doi: 10.1016/j.agee.2019.106624
Hepburn, H. R.. (1986). Honey Bees and Wax: An Experimental Natural History. Berlin: Springer Verlag, 72–125.
Hung, K.-L. J., Kingston, J. M., Albrecht, M., Holway, D. A., and Kohn, J. R. (2018). The worldwide importance of honey bees as pollinators in natural habitats. Proc. R. Soc. B. Biol. Sci. 285, 20172140. doi: 10.1098/rspb.2017.2140
Jones, L., Brennan, G. L., Lowe, A., Creer, S., Ford, C. R., and de Vere, N. (2021). Shifts in honey bee foraging reveal historical changes in floral resources. Commun. Biol. 4, 1–10. doi: 10.1038/s42003-020-01562-4
Kuchling, S., Kopacka, I., Kalcher-Sommersguter, E., Schwarz, M., Crailsheim, K., and Brodschneider, R. (2018). Investigating the role of landscape composition on honey bee colony winter mortality: a long-term analysis. Sci. Rep. 8, 12263. doi: 10.1038/s41598-018-30891-y
Lau, P., Bryant, V., Ellis, J. D., Huang, Z. Y., Sullivan, J., Schmehl, D. R., et al. (2019). Seasonal variation of pollen collected by honey bees (Apis mellifera) in developed areas across four regions in the United States. PLoS ONE 14, e0217294. doi: 10.1371/journal.pone.0217294
Ochungo, P., Veldtman, R., Kinyanjui, R., Abdel-Rahman, E. M., Muli, E., Muturi, M. N. K., et al. (2021). Pollen diversity and protein content in differentially degraded semi-arid landscapes in Kenya. J. Apic. Res. 60, 828–841. doi: 10.1080/00218839.2021.1899656
Oksanen, F. J., Blanchet, G., Friendly, M., Kindt, R., Legendre, P., and McGlinn, D.. (2020). Vegan: Community Ecology Package. R Package Version 2.5-7. Available online at: https://CRAN.Rproject.org/package=vegan (accessed February 24, 2022).
PalDat (2000). PallDat: A Palynological Database. Available online at: www.paldat.org (accessed December 15, 2021).
Persano Oddo, L., and Ricciardelli d'Albore, G. (1989). Nomenclatura melissopalinologica. Apicoltura 5, 63–72.
Pinheiro, J., Bates, D., DebRoy, S., and Sarkar, D. R Core Team. (2019). nlme: Linear and Nonlinear Mixed Effects Models. R Package Version 3.1.
PollenAtlas. Available online at: www.pollenatlas.net (accessed December 15, 2021)..
Potts, S. G., Biesmeijer, J. C., Kremen, C., Neumann, P., Schweiger, O., and Kunin, W. E. (2010). Global pollinator declines: trends, impacts and drivers. Trends Ecol. Evol. 25, 345–353. doi: 10.1016/j.tree.2010.01.007
R Core Team (2019). R: A Language and Environment for Statistical Computing. Vienna: R Foundation for Statistical Computing. Available online at: http://www.R-project.org (accessed January 20, 2022).
Requier, F., Odoux, J.-F., Tamic, T., Moreau, N., Henry, M., Decourtye, A., et al. (2015). Honey bee diet in intensive farmland habitats reveals an unexpectedly high flower richness and a major role of weeds. Ecol. Appl. 25, 881–890. doi: 10.1890/14-1011.1
Ricciardelli d'Albore, G.. (1998). Mediterranean Melissopalynology. Perugia: Università degli Studi di Perugia.
Rollin, O., and Garibaldi, L. A. (2019). Impacts of honeybee density on crop yield: a meta-analysis. J. Appl. Ecol. 56, 1152–1163. doi: 10.1111/1365-2664.13355
Roulston, T. H., and Cane, J. H. (2000). Pollen nutritional content and digestibility for animals. Plant Syst. Evol. 222, 187–209. doi: 10.1007/BF00984102
Rutschmann, B., Kohl, P. L., Machado, A., and Steffan-Dewenter, I. (2022). Semi-natural habitats promote winter survival of wild-living honey bees in an agricultural landscape. Biol. Conserv. 266, 109450. doi: 10.1016/j.biocon.2022.109450
Seeley, T. D.. (2019). The Lives of Bees: The Untold Story of the Honey Bee in the Wild. Princeton, NJ: Princeton University Press.
Simanonok, M. P., Otto, C. R. V., and Smart, M. D. (2020). Do the quality and quantity of honey bee-collected pollen vary across an agricultural land-use gradient? Environ. Entomol. 49, 189–196. doi: 10.1093/ee/nvz139
Sponsler, D. B., Shump, D., Richardson, R. T., and Grozinger, C. M. (2020). Characterizing the floral resources of a North American metropolis using a honey bee foraging assay. Ecosphere 11, e03102. doi: 10.1002/ecs2.3102
Steffan-Dewenter, I., and Kuhn, A. (2003). Honeybee foraging in differentially structured landscapes. Proc. Biol. Sci. 270, 569–575. doi: 10.1098/rspb.2002.2292
Tamic, T., Aupinel, P., Odoux, J. F., Loublier, Y., and Chabirand, M. (2011). Optimisation d'une méthode de dénombrement de grains de pollen adaptée a l'étude de l'alimentationde l'abeille domestique. Polen 21, 51–55.
Tew, N. E., Baldock, K. C. R., Vaughan, I. P., Bird, S., and Memmott, J. (2022). Turnover in floral composition explains species diversity and temporal stability in the nectar supply of urban residential gardens. J. Appl. Ecol. 00, 1–11. doi: 10.1111/1365-2664.14094
Visscher, P. K., and Seeley, T. D. (1982). Foraging strategy of honey bee colonies in a temperate deciduous forest. Ecology 63, 1790–1801. doi: 10.2307/1940121
Von Frisch, K.. (1954). The Dancing Bees, An Account of the Life and Senses of the Honey Bee. London: Methuen and Co. LTD.
Keywords: foraging behavior, landscape heterogeneity, mountains, PCA, pollinators, resource seasonality, Shannon diversity index
Citation: Malagnini V, Cappellari A, Marini L, Zanotelli L, Zorer R, Angeli G, Ioriatti C and Fontana P (2022) Seasonality and Landscape Composition Drive the Diversity of Pollen Collected by Managed Honey Bees. Front. Sustain. Food Syst. 6:865368. doi: 10.3389/fsufs.2022.865368
Received: 29 January 2022; Accepted: 09 March 2022;
Published: 31 March 2022.
Edited by:
Kimberly Ann Stoner, Connecticut Agricultural Experiment Station, United StatesReviewed by:
Patrick Laurenz Kohl, Julius Maximilian University of Würzburg, GermanyBożena Denisow, University of Life Sciences of Lublin, Poland
Copyright © 2022 Malagnini, Cappellari, Marini, Zanotelli, Zorer, Angeli, Ioriatti and Fontana. This is an open-access article distributed under the terms of the Creative Commons Attribution License (CC BY). The use, distribution or reproduction in other forums is permitted, provided the original author(s) and the copyright owner(s) are credited and that the original publication in this journal is cited, in accordance with accepted academic practice. No use, distribution or reproduction is permitted which does not comply with these terms.
*Correspondence: Valeria Malagnini, dmFsZXJpYS5tYWxhZ25pbmkmI3gwMDA0MDtmbWFjaC5pdA==; Andree Cappellari, YW5kcmVlLmNhcHBlbGxhcmkmI3gwMDA0MDtwaGQudW5pcGQuaXQ=
†ORCID: Valeria Malagnini orcid.org/0000-0001-9686-0299
Andree Cappellari orcid.org/0000-0002-6726-1323
Lorenzo Marini orcid.org/0000-0001-7429-7685
Livia Zanotelli orcid.org/0000-0002-4431-8585
Roberto Zorer orcid.org/0000-0003-3832-6033
Claudio Ioriatti orcid.org/0000-0002-8919-9217
Paolo Fontana orcid.org/0000-0001-9710-759X
‡These authors have contributed equally to this work and share first authorship