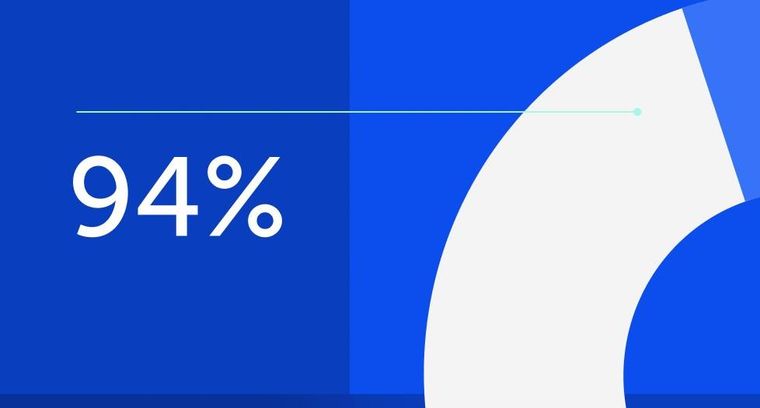
94% of researchers rate our articles as excellent or good
Learn more about the work of our research integrity team to safeguard the quality of each article we publish.
Find out more
ORIGINAL RESEARCH article
Front. Sustain. Food Syst., 19 May 2022
Sec. Nutrition and Sustainable Diets
Volume 6 - 2022 | https://doi.org/10.3389/fsufs.2022.863936
This article is part of the Research TopicAdvanced Strategies for the Plant Protection/Nutrition and Functional Food ProductionView all 5 articles
The public has recommended the use of botanicals with insecticidal properties because of the environmental and human health hazards associated with the utilization of synthetic chemicals. Farmers and other stakeholders need to have optimal knowledge of the chemical treatment of grain. Also understanding how synthetic chemicals and botanicals impact the environment, and the cost involved in treating maize grain is important. Although some research had been done on Actellic Super and azadirachtin in other parts of the world based on different research goals, this study rather focused on peculiar assumptions, scope, goals, and system boundary. The objectives of this study were to evaluate the environmental impacts of the active ingredients in Actellic Super (pirimiphos-methyl and permethrin) and NeemAzal (azadirachtin), and also to perform cost analysis on the usage of Actellic Super and azadirachtin for the treatment of maize grain. Data from Sustainable Minds database and TRACI database were used to assess CO2 emissions and impacts of pirimiphos-methyl and permethrin during manufacturing and transport. Also, the ecotoxicity and health impacts of azadirachtin, pirimiphos-methyl, and permethrin were assessed. A unit of Ghana cedi per kilogram of grain was used for the cost analysis. Pirimiphos-methyl manufacturing recorded the lowest CO2eq. kg/kg emissions and impacts (mPts/kg) compared with permethrin. Air transport of pirimiphos-methyl and permethrin resulted in higher CO2eq. kg/kg emissions and impacts unlike sea transport. Pirimiphos-methyl recorded the highest ecotoxicity while the least was permethrin. Importantly, azadirachtin had no human health impacts compared with pirimiphos-methyl. Actellic Super costs 224% less compared with azadirachtin. However, the costs of both insecticides depended on foreign exchange rate. The treatment cost increased with grain capacity irrespective of the insecticide used. Although treatment cost increased with grain capacity, the economies of scale favored the largest grain capacity.
The damage caused by Sitophilus zeamais (Motschoulsky, 1855; order—Coleoptera, family—Curculionidae) to stored grains in Sub-Saharan Africa (SSA) is enormous and has been a threat to food security for decades. Although many techniques have been used to control S. zeamais infestations in stored maize grains, the most predominant treatment method is the use of synthetic chemicals. However, the excessive use of these chemicals has many environmental and health detrimental impacts (Farage, 1989; Markowitz, 1992; Gupta et al., 2001). One of the most recently used synthetic insecticides in SSA including Ghana is Actellic Super. This is a broad spectrum insecticidal dust used to control many cereal weevils. Actellic Super is composed of two compounds which are Pirimiphos-methyl (80 g) and Permethrin (15 g). Actellic Super Dust can persist on grains and inert surfaces for several months (TWIGA Chemical Industries Limited, 2015). The manufacturing of synthetic fertilizers and pesticides contributes more than 480 × 106 tons of greenhouse gas (GHG) emissions to the atmosphere each year. Carbon emissions associated with the manufacturing and transport and storage of insecticides were estimated to be between 1.2 and 11.9 kg CO2eq. kg−1 (Pimentel, 1980; Lal, 2004).
The public has recommended the use of botanicals with insecticidal properties because of the health hazards and ecological challenges linked to synthetic insecticides usage. These botanicals are biodegradable, locally available, and inexpensive in controlling insects in stored products (Owusu, 2001; Cherry et al., 2005; Isman, 2006; Matta, 2010; Danga et al., 2015). Azadirachtin is the predominant compound with insecticidal ability found in Azadirachta indica (Schmutterer, 1990). Azadirachtin is primarily an antifeedant, and also physiologically disrupts in normal insect growth/molting, repels larvae and adults, sterilizes adults, and deters egg laying (Mordue and Nisbet, 2000). Azadirachtin pesticide (botanical) is environmentally friendly, so selective, persists shortly, pest specific, not harmful to organisms of no interest, and has a low effect on the ecosystem (Koul et al., 1990; Ascher, 1993; Barrek et al., 2004). There is a worldwide increase in the utilization of azadirachtin insecticide to control insects due to its potency (Mordue and Blackwell, 1993).
Grain producers treat grains at a cost. The insecticides used in treating grains are bought from agrochemical agents, and prices of insecticides change with time, and this puts financial constraints on farmers. Farmers and other stakeholders need to have optimal knowledge of the chemical treatment of the grain. Also understanding how synthetic chemicals and botanicals impact the environment, and the cost of maize grain treatment is important. Although some research has been done on Actellic Super, azadirachtin, and other chemicals in other parts of the world based on different research goals (Guinee et al., 2010; Walker et al., 2011), this study focused on specific assumptions, scope, goals, and system boundaries. Therefore, the objectives of this study were to evaluate the environmental impacts of the active ingredients in Actellic Super (pirimiphos-methyl and permethrin) NeemAzal (azadirachtin) and to perform cost analysis on the usage of Actellic Super and azadirachtin for maize grain treatment. The assumptions made were based on existing factors in Ghana.
The environmental impact assessments of the active ingredients in Actellic Super (pirimiphos-methyl and permethrin) during manufacturing and transport, and human health and ecotoxicity impacts assessment associated with the usage of pirimiphos-methyl, permethrin, and azadirachtin were performed. Sustainable Minds database (Version 3.0, 2013) was used to obtain graphical output for impacts assessment associated with the manufacturing and transport of pirimiphos-methyl and permethrin, and Tool for the Reduction and Assessment of Chemical and other Environmental Impacts (TRACI) database (Version 2.1, 2012) was used to obtain graphical output for human health and ecotoxicity impacts assessment associated with the usage of pirimiphos-methyl, permethrin, and azadirachtin. This was not a full life cycle assessment (LCA).
The goal of this study was to evaluate the environmental, human health, and ecotoxicity impacts of pirimiphos-methyl, permethrin, and azadirachtin. This was to help farmers and decision makers decide on the appropriate insecticide (synthetic or botanical) to use.
The focus of this study was on the active ingredients; pirimiphos-methyl, permethrin, and azadirachtin used to treat maize grain. Therefore, a functional unit of 1 kg of pirimiphos-methyl and permethrin was used in the manufacturing and transport analysis. The functional unit of the ecotoxicity and human health impacts was based on the quantity (kg) of pirimiphos-methyl, permethrin, and azadirachtin required to treat 1 kg of maize grain.
Figure 1 shows the system boundary for the manufacturing and transport of pirimiphos-methyl and permethrin, and the usage of pirimiphos-methyl, permethrin, and azadirachtin.
Figure 1. System boundary for the environmental assessment: sustainable minds was used for the manufacturing and transport of insecticides, and TRACI was used for insecticides usage (Generated using microsoft vision).
The impacts categories for this study were global warming potential (CO2 equivalent emissions and impacts), human health impacts (cancer or non-cancer), and ecotoxicity impacts.
Since Actellic Super is most commonly imported from Kenya, freight from Kenya to Ghana was assumed to be 2,739 mi (air), and 5,862.071 mi (Sea: Via Cape of Good Hope, 10 Knots). Actellic Super contains pirimiphos-methyl (16 g/kg), and permethrin (3 g/kg), and the application rate used was 5.0 × 10−2 kg per 90 kg of maize (according to users' protocol). Regarding the 5.6 × 10−4 kg per 1 kg of maize grain, 4.7 × 10−4 kg of pirimiphos-methyl, and 8.8 × 10−5 kg of permethrin were required to treat 1 kg of grain. According to Nukenine et al. (2011), 0.012 kg of azadirachtin is required to treat 1 kg of maize to achieve 99% mortality within 14 days. Therefore, 1.2 × 10−2 kg of azadirachtin was applied to 1 kg of grain in the analysis.
The cost analysis of maize grain treatment using Actellic Super and azadirachtin was performed. This can help farmers select the most cost-effective insecticide (natural or synthetic) to use.
The unit cost of treatment used was Ghana cedi per 1 kg of grain (GH/kg). Thus, the cost of treating 1 kg of grain using azadirachtin and Actellic Super.
Domestic transport fares, costs of other handling implements (such as gloves, nose, and mask), and the cost of the woven polypropylene storage bags were excluded from the analysis. The costs are similar in both case scenarios. Farming inputs and structures and storage materials (bins or bags) were not considered in the system boundary.
The grain storage period was assumed to be 6 months. The average baseline costs of treating 1 kg of maize grain were GH0.0112 and GH
0.005 for azadirachtin and Actellic Super, respectively (based on agrochemical shops in Ghana). Dollar-to-cedi exchange rate and an inflation rate of minus 50%, plus 50%, and 100% were used. Based on the baseline costs, the cost analysis was performed using 1, 500, 1,000, 1,500, 2,000, and 2,500 kg grain capacities. A percent rebate was applied to purchasing a larger quantity of insecticides based on the price list used by FREB Pharmaceuticals Ltd (agrochemicals producer/agent in Kenya on 29 January 2015). The rebates used were 15, 30, 45, 60, and 75%, and were applied at 500 kg grain capacity intervals. However, insecticides' price rebate of 75% was applied to 2,500 kg (and above) grain capacity. The data were graphed, and regression analysis was then used.
The CO2eq. emissions impacts measured were ecological, resource depletion, and human health damage (Figure 2). Generally, pirimiphos-methyl manufacturing had the lowest CO2eq. emissions and impacts (9.6 CO2eq. kg/kg and 0.71 mPts/kg). Permethrin manufacturing was higher concerning CO2eq. emissions and impacts (25 CO2eq. kg/kg and 1.7 mPts/kg). Permethrin manufacturing emitted 15.4 CO2eq. kg/kg higher than pirimiphos-methyl manufacturing. Pirimiphos-methyl manufacturing and transport (sea and air) was even better than permethrin manufacturing regarding CO2 eq. emissions and impacts. The emissions (CO2eq. kg/kg) and impacts (mPts/kg) were higher when pirimiphos-methyl and permethrin were shipped by air from Kenya to Ghana, although that of permethrin was very high. For example, air transport of permethrin emitted 11.0 kg CO2eq. more than permethrin manufacturing. Air transport of pirimiphos-methyl emitted 10.4 CO2eq. kg/kg more than pirimiphos-methyl manufacturing. However, CO2eq. kg/kg emissions and impacts (mPts/kg) of the sea transport of pirimiphos-methyl and permethrin were similar to their manufacturing.
Figure 2. CO2 equivalent emissions and impacts of pirimiphos-methyl and permethrin during manufacturing and transport (air and sea freight) per kg of insecticide. Impact per FU (mPts) = ecological, resource depletion, and human health damage (generated using Sustainable Minds database, version 3.0, 2013).
Carbon emissions associated with the manufacturing and transport and storage of insecticides were estimated to be between 1.2 and 8.1 kgCO2eq./kg (Pimentel, 1980). West and Marland (2002) estimated 4.6 kg CO2eq./kg emissions in the manufacturing, packaging, and transport of insecticides. Approximately 1.2–11.9 kg CO2eq./kg emitted from insecticides manufacturing was reported by Lal (2004), after recalculating carbon equivalent from earlier reports. The pirimiphos-methyl manufacturing emissions (CO2eq. kg/kg) for this study was within the CO2eq. kg/kg range reported by Lal (2004). But the emissions (CO2eq. kg/kg) from permethrin manufacturing were above that range. Thus, permethrin manufacturing contributes largely to GHG emissions and global warming, hence its manufacturing must be stopped. To ensure permethrin and pirimiphos-methyl are not manufactured, farmers could be entreated to desist from the purchase and use of Actellic Super. According to PAN-Germany (2010), permethrin (pyrethroid) is highly toxic to bees. The EPA (US) listed permethrin (pyrethroid) as a possible human carcinogen, highly bioaccumulative, and persistent in water. Therefore, the use of permethrin affects food security, the health of humans, and the environment, and causes global warming. Importantly, pirimiphos-methyl (organophosphate) is not classified among the most dangerous pesticide (PAN-Germany, 2010). Pirimiphos-methyl manufacturing had low emissions (CO2eq. kg/kg) and impacts (mPts/kg), but its composition in Actellic Super is five times more than permethrin. Therefore, GHG and global warming effects of pirimiphos-methyl might be similar to permethrin. Hence, the manufacturing and transport of pirimiphos-methyl and permethrin can be discouraged. However, if the manufacturing and transport could not be stopped, then sea transport of Actellic Super could be encouraged. This could substantially reduce CO2eq.kg−1 emissions and impacts (mPts/kg) associated with the transport of Actellic Super to Ghana.
The ecotoxicity impacts associated with using azadirachtin, pirimiphos-methyl, and permethrin were determined (Figure 3). All three chemicals seriously impacted water, but negligibly impacted air and soil. Their easy solubility in water might have caused a heavy impact on water. However, the limited moisture in air and soil might have resulted in low solubility and ecotoxicity impacts. Similar impacts on water were recorded using pirimiphos-methyl (128.0 CTUeco/kg), azadirachtin (115.0 CTUeco/kg), and permethrin (104.0 CTUeco/kg). Azadirachtin had the highest ecotoxicity impacts on air, followed by permethrin, and pirimiphos-methyl. Azadirachtin had the highest ecotoxicity impacts on soil, followed by pirimiphos-methyl, but permethrin had a very low impact on soil. When air, water, and soil were combined, the total ecotoxicity impact was pirimiphos-methyl (134.0 CTUeco/kg), azadirachtin (124.0 CTUeco/kg), and permethrin (106.0 CTUeco/kg).
Figure 3. Ecotoxicity impacts of azadirachtin, pirimiphos-methyl, and permethrin usage on air, water, and soil per 1 kg of maize grain (generated using TRACI database, version 2.1, 2012).
Figure 4 shows the health effects associated with using azadirachtin, pirimiphos-methyl, and permethrin. Azadirachtin did not record any human health impacts (0 CTUnon-cancer/kg). However, human health impacts using pirimiphos-methyl were 1.63 × 10−8 CTUnon-cancer/kg, and negligible using permethrin (2.58 × 10−10 CTUnon-cancer/kg). Human health impacts due to insecticide-contaminated water were high compared to soil and air. Thus, 1.31 × 10−08 CTUnon-cancer/kg (pirimiphos-methyl) and 1.51 × 10−10 CTUnon-cancer/kg (permethrin). The high water ecotoxicity impact resulted in high human health impacts (non-cancer) due to water.
Figure 4. Human health impacts of azadirachtin, pirimiphos-methyl, and permethrin usage on air, water, and soil per 1 kg of maize grain (generated using TRACI database, version 2.1, 2012).
To have effective insect mortality, pirimiphos-methyl and azadirachtin are applied in large masses. Approximately 1.2 × 10−2, 4.7 × 10−4, and 8.8 × 10−5 kg of azadirachtin, pirimiphos-methyl, and permethrin, respectively, were used to treat 1 kg of grain. Therefore, the relatively large mass might have resulted in high ecotoxicity impacts of azadirachtin and pirimiphos-methyl. The low ecotoxicity and human health impacts of permethrin might be due to the smaller mass used. Despite the smaller mass of permethrin (88 mg/kg) used, when the ecotoxicity and human health impacts are compared to azadirachtin and pirimiphos-methyl, permethrin usage cannot be considered friendly environmentally and healthwise. If permethrin had been used in a larger mass as azadirachtin (12,000 mg/kg), ecotoxicity and human health impacts would have been overwhelming. According to PAN-Germany (2010), permethrin is more toxic compared to azadirachtin and pirimiphos-methyl. The low composition of permethrin in Actellic Super might be due to its ecotoxicity and human health impacts potency. Generally, the human health impacts of azadirachtin and permethrin were better, and the ecotoxicity impacts of permethrin were better.
The Penn State Extension (2006) categorized insecticides containing azadirachtin, pirimiphos-methyl, and permethrin into “Toxicity Category III” (thus, to be used with caution). Worth noting, azadirachtin was designated to be of general use, and pirimiphos-methyl and permethrin were designated to be of restricted use. In their report, azadirachtin toxicity effects (LD50) required massive concentrations (5,000 mg/kg or more in oral use and 2,000 mg/kg or more in dermal use). Although the mass of azadirachtin used in this study was 12,000 mg/kg, higher than the 5,000 mg/kg, there were no human health impacts. Hence, this study affirms the assertion that azadirachtin is specific to the target organism. Therefore, in a worst-case scenario, farmers can use azadirachtin to treat grains without any health concerns. Azadirachtin is preferred to pirimiphos-methyl and permethrin because it is usually safer for non-target organisms, and the environment (Buss and Park-Brown, 2002; Charleston et al., 2006). Reports indicate that azadirachtin insecticide is environmentally friendly, so selective, transient, pests-specific, harmless to other organisms of no interest, and has a low effect on the ecosystem (Ascher, 1993; Mordue and Blackwell, 1993; Barrek et al., 2004). Encouraging the global use of botanical insecticides such as azadirachtin would overcome many ecological and environmental challenges.
Pirimiphos-methyl is required in a smaller mass to cause toxicity effects (LD50) (tech. 5–20 mg/kg, oral; 220 mg/kg, dermal). Pirimiphos-methyl has a broader toxicity effect including oral, dermal, inhalation, accident, wildlife, fish, and aquatic species. The high ecotoxicity and human health impacts of pirimiphos-methyl could be attributed to its broader toxicity effect. Actellic Super must be handled with caution as its major compositions (pirimiphos-methyl and permethrin) have broad-spectrum toxicity effects. Permethrin is a broad-spectrum and restricted insecticide, and highly dangerous for bees, fish, and aquatic species. Unlike pirimiphos-methyl, permethrin is required in a higher mass to cause toxicity effects (tech. >4,000 mg/kg, oral; >4,000 mg/kg, dermal), and is generally harmless to humans (Penn State Extension, 2006). Importantly, unlike pirimiphos-methyl which highly bioaccumulates and persists, permethrin decomposes in the soil within a few days (Toynton et al., 2009).
The cost of treating 1 kg of maize grain was higher using azadirachtin compared to Actellic Super. The price of azadirachtin was ~224% higher than the Actellic Super (Figure 5). The increase in treatment costs using Actellic Super or azadirachtin corresponded with the increased inflation rate. When grain capacity was increased, treatment costs also increased non-linearly. However, treatment cost using azadirachtin was higher than Actellic Super (Figure 6). Change in treatment cost using Actellic Super or azadirachtin increased proportionally with grain capacity (Figure 7). Significant cost differences occurred between 1, 500, 1,500, and 2,000 kg capacities, but negligible cost difference occurred between 2,000 and 2,500 kg. The graph shows that treatment costs increased as grain capacity increased. Infinite application of price rebate (%) on Actellic Super or azadirachtin could have decreased the treatment costs to negative values. The unit treatment cost (economies of scale) decreased as grain capacity increased (Figure 8).
Figure 5. The costs of azadirachtin and actellic super per treatment of 1 kg of maize at the present price, and dollar-to-cedi exchange rate and an inflation rate of minus 50%, plus 50%, and plus 100% (generated using Microsoft Excel).
Figure 6. Costs of treating 1, 500, 1,000, 1,500, 2,000, and 2,500 kg capacities of maize grains by using azadirachtin and actellic super (generated using Microsoft Excel).
Figure 7. Change in the costs of treatment by using azadirachtin and actellic super at different capacities of grains (kg) (generated using Microsoft Excel).
Figure 8. The unit cost of treating maize grain in Ghana (economies of scale) (generated using Microsoft Excel).
Since the inflation rate affected the price of insecticides, when the inflation rate was low the treatment cost decreased. Azadirachtin and Actellic Super are imported into Ghana, hence their prices depend on the exchange rate. When the exchange rate increases farmers in Ghana would have to purchase insecticides at a high price. Therefore, an increase in inflation and exchange rates can make treatment costs very expensive to farmers. The non-linearity of treatment cost with increasing capacity due to the applied rebates (%) was essential. There was value for money handling larger grain capacities. Treatment cost of 2,500 kg capacity decreased as price rebate (%) on insecticides was highest, and beyond that capacity price rebate (%) remained constant. The polynomial regression equation could better predict (R2 = 1) treatment costs, and differences in costs when Actellic Super or azadirachtin is to be used. Similarly, the polynomial regression equation could better predict the unit cost of treatment (R2 = 1). So, a substantially lower treatment cost can be achieved when farmers treat large grain quantities. Thus, farmers can enjoy a high rebate (%) on the price of insecticides. Smallholder farmers in Ghana and other SSA do not enjoy significant rebates (%) on the prices of insecticides. Because they harvest small quantities of grain, and hence experience expensive treatment costs. Post-harvest loss (PHL) is massive among farmers financially constrained to purchase insecticides.
At all levels of grain capacity, treatment costs were high when azadirachtin was used. The high treatment cost was due to the large quantity (mass) of azadirachtin required to treat grain. Prices of botanical insecticides are high compared to synthetic insecticides (Shabozoi et al., 2011; Amoabeng et al., 2014; Ngbede et al., 2014). The large quantity of azadirachtin required coupled with a high market price limits its usage among farmers. Although azadirachtin or any other botanical insecticide is friendly environmentally and healthwise, it is very expensive to use. Farmers that used botanical insecticides experienced fewer economic benefits (Leatemia, 2003). Farmers may then opt for synthetic insecticides which are detrimental to their health, consumers, and the environment. Mostly, botanical insecticides are scarce in markets, and therefore farmers in SSA who can even afford to buy them do not have access to them.
Essentially, to avoid treatment costs associated with using botanical or synthetic insecticides, farmers in Ghana (SSA) can apply plant materials (leaves, seeds, branches, powders) from neem, pepper, basil, and other (Belmain and Stevenson, 2001) plants. Smallholder farmers can have temporary financial relief if the grain is shortly stored. According to Amoabeng et al. (2014), local plant materials are inexpensive to use compared to synthetic insecticides.
Pirimiphos-methyl manufacturing and transport (sea and air) recorded lower CO2eq. kg/kg emissions and impacts (mPts/kg) compared with manufacturing only permethrin. It is advisable to transport both pirimiphos-methyl and permethrin by sea instead of air. Hence, to reduce GHG emissions and global warming, the manufacturing and air transport of pirimiphos-methyl and permethrin can be discouraged. On the other hand, ecotoxicity impacts of permethrin were lower followed by azadirachtin, and then pirimiphos-methyl. Although azadirachtin recorded no human health impacts (cancer and non-cancer) that of pirimiphos-methyl was substantial. This study, therefore, affirms the assertion that azadirachtin is specific to targeted organisms, and can preferably be recommended for grain treatment, unlike pirimiphos-methyl and permethrin.
Azadirachtin costs 224% higher than Actellic Super, though the prices of azadirachtin and Actellic Super depend on foreign exchange rates. Invariably, the cost of treatment is dependent on the price of insecticides. However, farmers can benefit from a percentage rebate for buying larger quantities of insecticides. Regrettably, peasant or smallholder farmers do not benefit significantly from the percentage rebate since they do not buy larger quantities of insecticides, hence, these farmers experience a higher cost of treatment and higher PHL. Although treatment cost increased with grain capacity, the economies of scale favored the largest grain capacity. Scarcity of azadirachtin coupled with the required larger quantity (mass), treatment costs are normally very high. This, therefore, compels farmers to opt for synthetic insecticides despite the associated health and environmental issues.
The raw data supporting the conclusions of this article will be made available by the authors, without undue reservation.
KR designed the experiment and edited and paper. BD conducted the experiment and drafted the paper. Both authors contributed to the article and approved the submitted version.
The authors declare that the research was conducted in the absence of any commercial or financial relationships that could be construed as a potential conflict of interest.
All claims expressed in this article are solely those of the authors and do not necessarily represent those of their affiliated organizations, or those of the publisher, the editors and the reviewers. Any product that may be evaluated in this article, or claim that may be made by its manufacturer, is not guaranteed or endorsed by the publisher.
Amoabeng, B. W., Gurr, G. M., Gitau, C. W., and Stevenson, P. C. (2014). Cost-Benefit analysis of botanical insecticide use in cabbage: implications for smallholder farmers in developing countries. Crop Prot. 57, 71–76. doi: 10.1016/j.cropro.2013.11.019
Ascher, K. R. S. (1993). Non-conventional insecticidal effects of pesticides available from the Neem tree, Azadirachta indica. Arch. Insect Biochem. Physiol. 22, 433–449. doi: 10.1002/arch.940220311
Barrek, S., Olivier, P., and Grenier-Loustalot, M. F. (2004). Analysis of neem oils by LC-MS and degradation kinetics of Azadirachtin-A in a controlled environment: characterization of degradation products by HPLC–MS-MS. Anal. Bioanal. Chem. 378, 53–63. doi: 10.1007/s00216-003-2377-0
Belmain, S., and Stevenson, P. (2001). Ethnobotanicals in Ghana: reviving and modernizing age-old farmer practice. Pesticides Outlook 12, 233–238. doi: 10.1039/b110542f
Buss, E. A., and Park-Brown, S. G. (2002). Natural Products for Insect Pest Management. UF/IFAS Publication ENY-350. Available online at: http://edisifasufledu/IN197 (accessed March 10, 2016).
Charleston, D. S., Kfir, R., Dicke, M., and Vet, L. E. (2006). Impact of botanical extracts derived from Melia azedarach and Azadirachta indica on populations of Plutella xylostella and its natural enemies: a field test of laboratory findings. Biol. Control 39, 105–114. doi: 10.1016/j.biocontrol.2006.05.012
Cherry, A. J., Bantino, A., Djegui, D., and Lomers, C. (2005). Suppression of the stem borer Sesamia calamistis (Lepidoptera: Noctuidae) in maize following seed dressing, topical application and stem injection with African isolates of Beauveria bassiana. Int. J. Pest Manage. 50, 67–73. doi: 10.1080/09670870310001637426
Danga, S. P. Y., Nukenine, E. N., Fotso, G. T., and Adler, C. (2015). Use of NeemPro®, a neem product to control maize weevil Sitophilus zeamais (Motsch.) (Coleoptera: Curculionidae) on three maize varieties in Cameroon. Agric. and Food Secur. 4, 1–7. doi: 10.1186/s40066-015-0039-z
Farage, E. M. (1989). Enzyme and behavioral changes in young chicks as a result of carbaryl treatment. J. Toxicol. Environ. Health 26, 119–132. doi: 10.1080/15287398909531237
Guinee, J. B., Reinout, H., Gjalt, H., Alessandra, Z., Paolo, M., Roberto, B., et al. (2010). Life cycle assessment: the past, present, and future. Environ. Sci. Technol. 45, 90–96. doi: 10.1021/es101316v
Gupta, A., Upadhyay, R. K., and Saxena, P. N. (2001). Toxicity evaluation of certain blood biochemical parameters in Passer domesticus (Linn.). J. Sci. Ind. Res. India 60, 668–674.
Isman, M. B. (2006). Botanical insecticides, deterrents, and repellents in modern agriculture and an increasingly regulated world. Annu. Rev. Entomol. 51, 45–66. doi: 10.1146/annurev.ento.51.110104.151146
Koul, O., Isman, M. B., and Ketkar, C. M. (1990). Properties and uses of Neem, Azadirachta indica. Can. J. Bot. 68, 1–11. doi: 10.1139/b90-001
Lal, R. (2004). Carbon emission from farm operations. Environ. Int. 30, 981–990. doi: 10.1016/j.envint.2004.03.005
Leatemia, J. A. (2003). Development of a botanical insecticide from Ambon and surrounding areas (Indonesia) for local use. Ph.D. diss., Vancouver, Canada: the University of British Columbia, Department of Plant Science, Faculty of Agricultural Sciences.
Markowitz, S. B. (1992). Poisoning of an urban family due to misapplication of household organophosphate and carbamate pesticides. Clin. Toxicol. 32, 295–300. doi: 10.3109/15563659209038640
Matta, F. B. (2010). Essential oils from six herbal plants for biocontrol of the maize weevil. Hortscience 45, 592–598. doi: 10.21273/HORTSCI.45.4.592
Mordue, A. J., and Blackwell, A. (1993). Azadirachtin: an update. J. Insect Physiol. 39, 903–924. doi: 10.1016/0022-1910(93)90001-8
Mordue, A. J., and Nisbet, A. J. (2000). Azadirachtin from the Neem Tree azadirachta indica: its action against insects. An. Soc. Entomol. Brasil 29, 615–632. doi: 10.1590/S0301-80592000000400001
Motschoulsky, V. (1855). Voyages: Lettre de M. de Motschulsky a M. Menetries. Etudes Entomoloqiques. 4, 1–84.
Ngbede, S. O., Nwanguma, E. I., Ibekwe, H. N., Onyegbule, U. N., Okpara, S. C., and Uwalaka, O. A. (2014). Cost: benefits analysis of botanical insecticide use in watermelon production in Okigwe, Southeastern Nigeria. Int. J. Scientific Technol. Res. 3, 16–20.
Nukenine, E. N., Tofel, H. K., and Adler, C. (2011). Comparative efficacy of NeemAzal and local botanicals derived from Azadirachta indica and Plectranthus glandulosus against Sitophilus zeamais on maize. J. Pest Sci. 84, 479–486. doi: 10.1007/s10340-011-0371-4
Owusu, E. O. (2001). Effect of some Ghanaian plant components on control of two stored-product insect pests of cereals. J. Stored Prod. Res. 37, 85–91. doi: 10.1016/S.0022-474X.(00)00010-2
PAN-Germany (2010). Environmental strategies to replace DDT and control malaria. Pestizid Aktions-Netzwerk (PAN) e. V (PAN-Germany).
Penn State Extension (2006). Pesticide Education: Toxicity of Pesticides. University Park, PA: Pennsylvania State University, College of Agricultural Sciences.
Pimentel, D. (1980). “Energy inputs for the production, formulation, packaging, and transport of various pesticides,” in Handbook of Energy Utilization in Agriculture, eds Pimentel, D., (Florida: CRC Press), pp. 45–55.
Schmutterer, H. (1990). Properties and potential of natural pesticides from the neem tree, Azadirachta indica. Annu. Rev. Entomol. 35, 271–297. doi: 10.1146/annurev.en.35.010190.001415
Shabozoi, N. U. K., Abro, G. H., Syed, T. S., and Awan, M. S. (2011). Economic appraisal of pest management options in Okra. Pak. J. Zool. 43, 869–878.
Toynton, K., Luukinen, B., Buhl, K., and Stone, D. (2009). Permethrin General Fact Sheet. Oregon: National Pesticide Information Center, Oregon State University Ext. Services.
TWIGA Chemical Industries Limited (2015). Actellic Super 25 EC. Nairobi, Kenya. www.twigachemicals.com
Walker, M. B., Faber, A., Pudełko, R., Kozyra, J., Syp, A., and Borek, R. (2011). Life cycle assessment (LCA) of crops for energy production. J. Food Agric. Environ. 9, 698–700.
Keywords: treatment of maize grains, azadirachtin, Actellic Super, environmental assessment, cost analysis
Citation: Darfour B and Rosentrater KA (2022) Environmental Assessment and Cost Analysis of the Manufacturing, Transport, and Use of Actellic Super and Azadirachtin Insecticides for the Treatment of Maize Grains. Front. Sustain. Food Syst. 6:863936. doi: 10.3389/fsufs.2022.863936
Received: 10 February 2022; Accepted: 15 April 2022;
Published: 19 May 2022.
Edited by:
Darija Lemić, University of Zagreb, CroatiaReviewed by:
Petar Kljajić, Institute of Pesticides and Environmental Protection, SerbiaCopyright © 2022 Darfour and Rosentrater. This is an open-access article distributed under the terms of the Creative Commons Attribution License (CC BY). The use, distribution or reproduction in other forums is permitted, provided the original author(s) and the copyright owner(s) are credited and that the original publication in this journal is cited, in accordance with accepted academic practice. No use, distribution or reproduction is permitted which does not comply with these terms.
*Correspondence: Kurt A. Rosentrater, a2Fyb3NlbnRAaWFzdGF0ZS5lZHU=
Disclaimer: All claims expressed in this article are solely those of the authors and do not necessarily represent those of their affiliated organizations, or those of the publisher, the editors and the reviewers. Any product that may be evaluated in this article or claim that may be made by its manufacturer is not guaranteed or endorsed by the publisher.
Research integrity at Frontiers
Learn more about the work of our research integrity team to safeguard the quality of each article we publish.