- Environment and Life Sciences Research Center, Kuwait Institute for Scientific Research, Kuwait City, Kuwait
This work describes the optimization of the pressure–time combination for the inactivation of Listeria monocytogenes in fish medium using a wide range of pressure (225–525 MPa) and holding time (5–30 min). Thereafter, the yellowfin seabream (Acanthopagrus arabicus) filets (100 g each) were subjected to high-pressure (HP) treatment at the optimum pressure/time combination, and the impact of HP on the amino acid profile, fatty acid profiles, color, and texture was assessed. Glycine, glutamic acid, and alanine were recorded as the major amino acids, which did not change significantly after pressurization. Conversely, alanine—the leading free amino acid—dropped significantly after treatment. The fatty acid analysis indicated that oleic acid and palmitic acid accounted for 29.88 and 25.59% of the total fatty acids, respectively. Pressurization did not influence the fatty acid profiles, nutritional quality indices, and hardness of yellowfin seabream fish. The color pigments of filets, measured as a* and b*, changed significantly after the treatment. Overall, this work indicates that HP treatment can be utilized to maintain the nutritional quality of seabream filets; however, further research is needed to maintain the visual color of the fish.
Introduction
The yellowfin seabream (Acanthopagrus arabicus sp. nov), Sparidae, is a typical euryhaline estuarine fish. The fish possesses some interesting features, including good economic value, good meat quality, resistance to diseases, and decent adaptability to various environments (Zhu et al., 2021). The fish is distributed on a few continents, starting from Australia to Asia to the Middle East to Southeastern Africa (Hayashi, 1993). The yellowfin seabream is very popular in Middle Eastern countries because of its excellent protein and lipid quality, resulting in significant commercial exploitation of the fish in the state of Kuwait (locally known as sheim). The shelf-life of the fish in chilled storage is limited due to enzymatic and microbial degradation. Therefore, a suitable preservation method could ensure the availability of the fish to fish lovers throughout the year. A lot of information is available on osmotic stress, genomic studies, and diets of the aquaculture of yellowfin seabream (Lin et al., 2020; Zhu et al., 2021); however, limited information is available on the processing or preservation of the fish for its commercial success.
Fish is not only a major source of protein but also rich in fatty acids (unsaturated), vitamins, and various macro- or micro-elements. Fish protein is easy to digest and rich in amino acids, in particular, lysine, alanine, cysteine, methionine, glutamic acid, threonine, and tryptophan. Furthermore, amino acid profiling of fish might provide a guideline for establishing the nutritional database, which can be further explored to assess changes in quality during preparation, processing, and storage (Gomez-Limia et al., 2021). The nutritional quality of fish has also been related to the content of long-chain polyunsaturated fatty acids (LC-PUFA) and its superior profiling (Usydus et al., 2011). The PUFA with proven health benefits are docosahexaenoic acid (DHA, 22:6n-3), arachidonic acid (ARA, 20:4n-6), and eicosapentaenoic acid (EPA, 20:5n-3). The beneficial effects of PUFA n-3 acids in the prevention of cardiovascular diseases and some types of cancer have been established (Monroig et al., 2018).
Refrigeration is the best practice to preserve fish for a short period before selling to the customers. However, psychrotrophic foodborne pathogens, including Listeria monocytogenes, can grow in a refrigerated condition, and many times they can reappear after processing or even after following a strict sanitary practice (Nordvi et al., 2007). With prolonged storage in frozen condition, the fish undergoes denaturation of protein and/or oxidation of lipids that could lead to the deterioration of the quality of fish (e.g., loss of flavor and odor, weight, or texture) (Karlsdottir et al., 2014). To avoid such undesirable quality changes, non-thermal processing technologies are currently exploited, including high-pressure processing, dielectric heating, ultrasound, UV-C light, pressure shift freezing, or hyperbaric storage (Aubourg et al., 2013; Monteiro et al., 2017; Alba et al., 2019; Siewe et al., 2020; Ahmed et al., 2021a,b; Wang et al., 2021). Among these techniques, high-pressure (HP) processing has a successful presence in food industries for a wide range of products. In the HP system, an enormous pressure (300–900 MPa) is transmitted uniformly to the pre-packed food products for a few minutes to inactivate pathogens (i.e., pasteurization) or even inactivate heat-resistant bacteria by employing pressure-assisted thermal sterilization (PATS), which employs a combination of high pressure with elevated temperature. PATS has additional advantages over HP treatment to inactivate bacterial spores in low-acid food and produce shelf-stable food (Al-Ghamdi et al., 2020). The Food and Drug Administration has approved the process for the commercial pasteurization of shelf-stable foods.
HP treatment has been demonstrated to inactivate a wide range of food pathogens and endogenous enzymes that cause spoilage and to extend the shelf-life with a minimum impact on sensory and other quality parameters (Considine et al., 2008; Reyes et al., 2015). Furthermore, it has been reported that pressures higher than 400 MPa were adequate to inactivate the spoilage microbes of the abalone muscle, and the physico-chemical and sensory characteristics remained unaffected at below 300 MPa (Jo et al., 2014). While studying the effect of HP on the rigor status of abalone fish, Hughes et al. (2015) observed that a pressure/time combination of 300 MPa/5 min caused significant toughening of pre-rigor meat; however, the texture of post-rigor processed abalone was not changed significantly. These results demonstrated that fish quality depends upon the intensity of the pressure applied during the processing, followed by the holding time. Literature pertaining to the HP treatment of fish and muscle protein has been limited (Cropotova et al., 2020; Ahmed et al., 2021a,b). HP treatment induces structural changes in fish proteins that change the conformation and activity of proteins. It has been reported that the intermolecular β-sheet structure of abalone fish was disrupted at 200 MPa and that the β-sheet structure can fold on itself from the interactions that stabilize the protein structure between 300 and 400 MPa (Cepero-Betancourta et al., 2020). Conversely, pressurization at 400 and 600 MPa flowed by storage at 0 and 15 days did not alter the fatty acid profiles of oysters (Liu et al., 2022). Individual fish species and their constituents differed significantly in nutritional profiles (e.g., proteins and lipids) and therefore in their quality indices. So, individual attention is required for each fish species and their constituents when these undergo a new processing technology. To our knowledge, no systematic studies have been performed to date on the amino acid and fatty acid profiles of yellowfin seabream subjected to high-pressure treatment. Therefore, the objectives of this work were twofold: (i) to optimize the pressure/time combination by inactivating the test organism L. monocytogenes and thereafter (ii) to study the influence of optimum HP condition (pressure/time) on the amino acid and fatty acid profiles of yellowfin seabream fish. It would lead to the acceptability of the technique in the fish industry.
Materials and Methods
Preparation of the Sample
Fresh yellowfin seabream, which is locally known as “sheim” (Acanthopagrus latus), was procured from a local fish market (deceased form; caught in Kuwait Bay) in the state of Kuwait (May 2018). The fish samples (average weight of 1.35 kg and height of 35.6 cm, total of 10 samples) were transported to the laboratory in chilled condition inside an ice box. The samples were washed with chilled water, cleaned thoroughly, fileted aseptically using sterilized equipment, and packed immediately. The fish samples were stored in a refrigerator (4°C) for a maximum of an hour until further processing.
Test Organism and Microbial Media
Remel Europe Ltd. (Dartford, UK) delivered the cultiloops of L. monocytogenes (ATCC 19114) strain. Plate count agar, peptone water buffer, polymyxin–acriflavin–lithium chloride–ceftazidime–aesculin–mannitol (PALCAM) agar base, and PALCAM selective adjunct were obtained from Oxoid (Basingstoke, UK). TM Media (Bhiwadi, India) supplied the tryptic soy broth.
Slurry Preparation for Test Organism Inoculation
Fish slurry was made by mixing yellowfin seabream fish (100 g) and deionized water (900 ml) in a stomacher bag. The mixture was homogenized in a Stomacher (Lab Blender 400, Spain) for a minute, and the pH was adjusted to 6.94, followed by centrifugation (3,000 rpm/10 min; MIKRO 220R; Andreas Hettich GmbH and Co., Germany). The supernatant was vacuum-filtered, and the slurry was dispensed into HP-resistant low-density polyethylene (LDPE) bags (Whirl-Pak®, USA). A stock culture [1 × 106−1 × 107 colony-forming units (CFU)/ml] of L. monocytogenes was inoculated into 9 ml of slurry. The inoculations were prepared in duplicate for each dilution.
Fish Sample Preparation and the High-Pressure Treatment
The yellowfin seabream filets were divided into 80-g portions, filled into LDPE bags (Whirl-Pak®, USA), and vacuum-sealed. High-pressure treatment was conducted in a 2-L vessel of the high-pressure system (Avure Technologies WA, USA) at 26 ± 1.5°C. About 6–8 samples containing bags were treated with high pressure in each run to minimize sample variance. Water was used as the pressure-transmitting medium. The pressurization and depressurization rates of the HP system were 5 and 26 MPa/s, respectively.
Preliminary Study With L. monocytogenes-Contaminated Slurry
The preliminary study was carried out in a wide range of pressure starting from 225 to 525 MPa and holding time of 5–30 min using different pressure/time combinations to inactivate L. monocytogenes so that the process condition (pressure/time) would be optimized for the preservation of yellowfin seabream filets. The medium's temperature gradually increased from 26 to 35°C when the pressure increased from atmospheric (0.101 MPa) to 525 MPa because of adiabatic compression.
The microbe-inoculated sample without HP treatment was considered the positive control sample.
Pressure Treatment of Yellowfin Seabream Filets
Based on the complete inactivation of the test organism L. monocytogenes in a fish medium, the pressure/time combination of 375 MPa/20 min was employed for the processing of yellowfin seabream filet. All the pressure treatment runs were performed twice. The post-process samples were placed in a refrigerator (4 ± 0.6°C) before analysis. Three different batches of samples were taken for analysis.
Microbial Enumeration
All samples (without pressure treatment, without inoculation, and pressure-treated) were enumerated by spread plating using appropriate dilution onto PALCAM Listeria-specific agar following the method described earlier for the HP-treated pomfret fish (Ahmed et al., 2021a). Plate count agar was employed for the negative control samples.
Analysis of Total Amino Acids and Free Amino Acids
The total amino acid (TAA) and free amino acid (FAA) compositions of the yellowfin seabream filets were analyzed according to the methods described earlier for pomfret filets (Ahmed et al., 2021a). Briefly, the acid-hydrolyzed specimen was placed in a glass ampoule at 110°C for 20 h under a nitrogen atmosphere, and the TAA composition was analyzed. A number of preparation steps (e.g., filtration, concentration, and dissolution) were checked before injection to the ultra-high-performance liquid chromatography (UHPLC) system. For the free fatty acid analysis, absolute ethyl alcohol was used for the extraction, followed by centrifugation of the blends at 2,800 rpm/10 min. The supernatant was evaporated; the residue was redissolved in 0.1 N HCl and injected into UHPLC. Samples were extracted twice, and 2 injections per vial were made.
Each amino acid was identified and quantified using a UHPLC system (1290 Infinity LC System, Agilent Technologies, Germany), hooked up with a fluorescence detector (Agilent G1321B) in a ZORBAX Eclipse plus C18 column (150 mm × 4.6 mm) with a 3.5-μm packing material. The details of the specific injection programs and calculations are available elsewhere (Ahmed et al., 2021a,b).
Fatty Acid Profile
The methanol/chloroform mixture (1:1, v/v) was used for the extraction of lipid contents (Bligh and Dyer, 1959). The fatty acids were trans-esterified to methyl esters (FAMEs) by base-catalyzed transesterification and thereafter by boron trifluoride-catalyzed esterification (AOCS, 1998).
For the GC parameters, 1 μl of FAMEs was injected into a gas chromatograph (7890B, Agilent, Santa Clara, CA, USA), which was equipped with an autosampler and mass spectrometer (MS 59778B). The separation was performed in a DB-23 capillary column (60 m × 250 μm × 0.15 μm) (Agilent Technologies, CA, USA) at a constant pressure using helium as the carrier gas and with a flow rate of 25 ml/min. The split ratio was 5:1. The temperature was set in a few steps: the starting temperature was 50°C, increased to 130°C at a heating rate of 10°C/min and held for 1 min, increased to 170°C at 10°C/min, further increased to 215°C at 2.75°C/min and held for 12 min, and finally increased to 230°C at 40°C/min, with holding for 3 min. Two injections were prepared from each vial.
In terms of MS conditions, the injector and source temperatures were 250°C, and the transfer line temperature was 290°C. The ionization energy was fixed at 70 eV in the electron ionization mode, and the detector was set in the mass range from 40 to 550 at 1.56 scans per second. Each fatty acid methyl ester was identified and quantified by MS library searches (Wiley 10th with NIST 2014, Agilent Technologies, Palo Alto, CA, USA), followed by comparing the retention time and spectra with MS runs of external standards (37 component standard mix from Supelco, Bellefonte, PA, USA). The chromatograph was attached to the equipment software (Mass Hunter Workstation, Agilent Technologies). The fatty acid compositions were calculated in terms of total fatty acid percentage.
Nutritional Quality Indices
The atherogenicity index (AI), thrombogenicity index (TI), and hypocholesterolemic to hypercholesterolemic ratio (H/H) were calculated using the following equations (Ulbricht and Southgate, 1991; Monteiro et al., 2017):
Color Measurement
The tristimulus color values of yellowfin seabream filets were recorded using a LabScan XE colorimeter (Hunter Associates Laboratory, Reston, VA, USA). The instrument was standardized with a standard black and white tile prior to the measurement. The filet was placed in a glass cell and placed above the light source, and the tristimulus color values, namely, L* (lightness), a* (redness/greenness), and b* (yellowness/blueness) were recorded.
Textural Measurement
The textural profile analysis (TPA) was performed in a TA-XT Plus Texture Analyzer (Stable Micro-Systems, Surrey, UK) at 25°C. The test was used to obtain the TPA parameters in two cycles using a P-6 probe. The filet sample (average thickness of 40 ± 2 mm) was compressed to 50% of its original thickness, with a test speed of 10 mm/s. The selected texture parameters, namely, hardness, adhesiveness, and cohesiveness, were calculated from the force–time plot using the Exponent 32 software (Stable Micro Systems Ltd, Surrey, UK). The maximum resistance to the penetration of the cylinder at 20-mm depth is considered the hardness, and the value was presented as the maximum force (N). Cohesiveness measures the ratio between the positive area during the second compression to the area during the first compression. Adhesiveness calculates the negative area (N sec) for the first compression.
Statistical Analysis
The measurements were performed in triplicate. A t-test was used to determine the difference between the control and high-pressure treated samples using JMP 15 software (SAS Institute Inc., NC, USA). The test was conducted to determine significant differences, with a confidence interval of 95%.
Results and Discussion
High-Pressure Condition Optimization by Inactivating L. monocytogenes
A wide range of pressure (225–525 MPa) and holding time (5–30 min) application showed that the number of surviving L. monocytogenes in yellowfin seabream slurry was reduced significantly (P < 0.05) with increasing pressure and holding time (Figure 1). An applied pressure level of 225 MPa and a maximum holding time of 30 min merely accomplished a log reduction of 1.01 of the surviving L. monocytogenes against the sample without pressurization (6.96 log CFU/ml). Conversely, a pressure/time combination of 300 MPa/30 min reduced the number of surviving L. monocytogenes by 3 to 4 log cycles from the original counts. The numbers of survivors was reduced below the detection limit at a pressure/time combination of 375 MPa/20 min or 450 MPa/10 min or 525 MPa/5 min. Hence, a pressure/time combination of 375 MPa/20 min was selected as the optimum processing condition for yellowfin seabream filets. These results are consistent with published data. It has been reported that complete inactivation of L. monocytogenes could not be accomplished up to 300 MPa (Das et al., 2016). The survival of L. monocytogenes in prawn dropped by 3.3 log cycles at 400 MPa and a holding time of 12 min, while 5 log reduction was recorded in frankfurters at 700 MPa/9 min (Lucore et al., 2000). It can be concluded that the inactivation of L. monocytogenes depends on several factors, including pressure intensity, holding time, medium, and temperature. The destruction of microorganisms followed after the pressure treatment due to damage in their cell membrane, and the applied pressure also disrupted the structure of protein and its functionality (Yousef and Courtney, 2003).
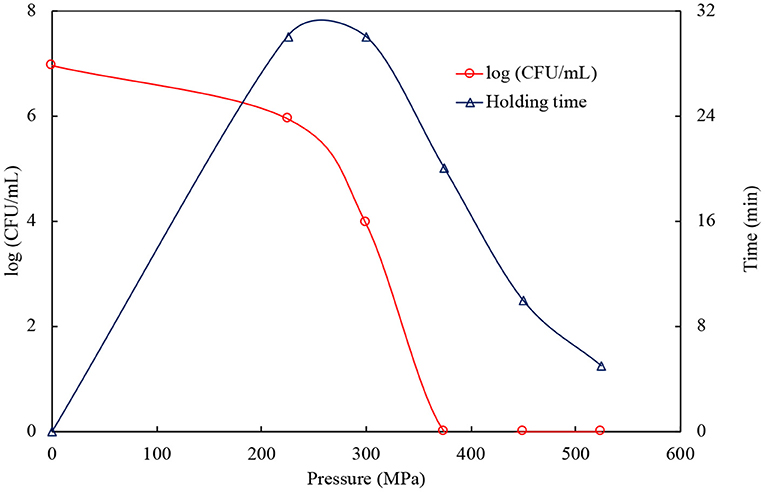
Figure 1. Effect of high pressure and holding time on the inactivation of Listeria monocytogenes in yellowfin seabream slurry.
Total and Free Amino Acid Composition
The changes in the TAA and FAA profile of yellowfin seabream after the HP treatment are shown in Table 1. A total of 17 TAA and 19 FAA were identified and quantified in yellowfin seabream filets. The yellowfin seabream protein contained a significant amount of leucine (0.76 mmol/g), lysine (0.71 mmol/g), threonine (0.48 mmol/g), valine (0.44 mmol/g), and isoleucine (0.34 mmol/g), while histidine had the least amount (0.22 mmol/g) of total amino acids. The supplementation of lysine could improve the nutritional profile of cereal-based products. Among non-essential amino acids, the dominating components were glycine (1.48 mmol/g), glutamic acid (1.37 mmol/g), alanine (1.27 mmol/g), and aspartic acid (0.95 mmol/g). The tyrosine content was the least in amount in the filet (0.22 mmol/g). A similar TAA profile has been observed for a local grouper (e.g., hamour) fish (Ahmed et al., 2021a). The amino acid profile for a series of freshwater fish showed that the major amino acids are glutamic acid, aspartic acids, arginine, lysine, leucine, and valine (Mai et al., 1980). The quality index of the protein was calculated from the ratio between essential amino acids (EAA) and nonessential amino acids (NEAA) (Swendseid et al., 1963), and the calculated value was 0.49 for the filet. These values demonstrate that the EAA were in lower concentrations than the NEAA in the filet samples. The ratio was significantly lower than those reported for the spotted grouper (0.79) (ElShehawy et al., 2016), indicating a lower protein quality in the studied fish. Such variations could be attributed to many factors, including season, geography of catchment, feed, and so on.
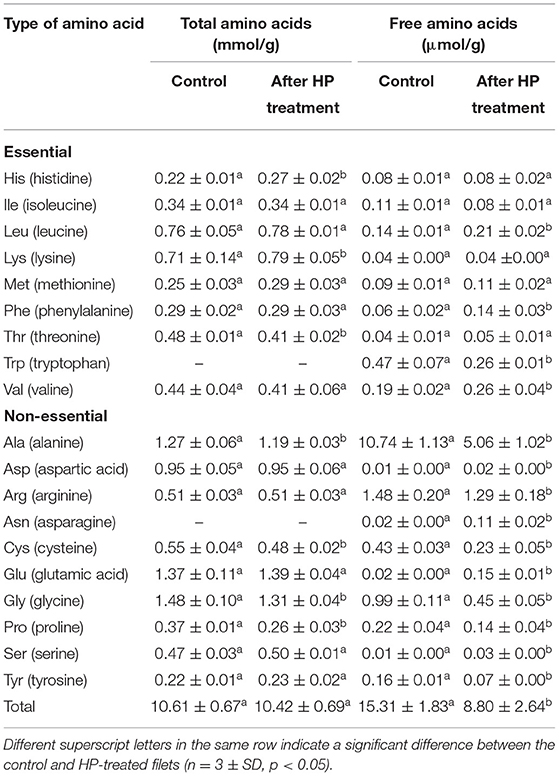
Table 1. Influence of high-pressure (HP) treatment on total and free amino acids of yellowfin seabream fish.
The amino acid analysis showed a change in the individual total amino acid concentration following the high-pressure treatment at 375 MPa for 20 min (Table 1). A few amino acids showed a significant decrease in concentration after the pressure treatment, particularly proline, where a 30% decrease was recorded, followed by threonine (15%), cysteine (13%), and glycine (11.5%). A similar decrease in threonine was detected earlier in the HP-treated pomfret (Ahmed et al., 2021a). Conversely, a significant increase was registered for histidine (23%), followed by lysine (11%). Similar results were reported for HP-treated water-soaked soybeans (Ueno et al., 2010). They observed retention of free amino acid contents at 200 MPa; however, the increase in isoleucine (0.24 to 0.37 mmol/g) was significant (P < 0.05) after pressurization. Additionally, the concentration of glycine dropped significantly from 1.85 to 1.44 mmol/g (P < 0.05). It can be inferred that the high pressure might have affected a specific amino acid in fish.
Among essential free amino acid (EFAA) contents in yellowfin seabream filet, trypsin (Trp) was the highest (0.47 μmol/g), followed by valine (0.19 μmol/g), leucine (0.14 μmol/g), and isoleucine (0.11 μmol/g) (Table 1). On the other hand, the presence of a significant amount of alanine (10.74 μmol/g) was recorded among NEAA, which was followed by arginine (1.48 μmol/g) and glycine (0.99 μmol/g). A higher range of alanine (17.96 mg/100 g) and arginine (3.86 mg/100 g) concentrations has been reported for the yellow croakers (Kim et al., 2020). After the HP treatment, the total FAA values were reduced significantly (P < 0.05) from 15.31 to 8.80 μmol/g. Pressurization had a significant (P < 0.05) impact on the individual free amino acid, and the reduction was significant for tyrosine, alanine, and tryptophan, which were reduced by 56, 53, and 45%, respectively, of their original concentrations. Conversely, nine of the free amino acids, including glutamic acid, asparagine, serine, and aspartic acid, showed a significant increase in their concentration after the HP treatment. The decrease in basic amino acid concentration demonstrates that their solubilization from muscle proteins was slower than their transformation into biogenic amines through decarboxylation (Ciampa et al., 2012). While working with the HP treatment of seedlings of Brussel sprouts with a pressure range of 800 MPa, Barba et al. (2017) reported that pressurization had a significant effect in 10 of the FAA, and seven other FAA remained unaffected by the treatment. It can be postulated that the change in individual free amino acid is fish specific and consistent with earlier reports which demonstrated that the stabilization of free amino acids in loins occurred after the HP treatment (300 to 400 MPa for 10 min at 20°C) due to the inactivation of amino peptidase. Histidine and lysine remained unchanged, and tyrosine dropped after pressurization, which are key factors for food safety since they are the main precursors of biogenic amines.
The quality index (EAA/NEAA) for free amino acids provides a good approximation to calculate the production of FAA after any treatment. An equal production of EAA and NEEA yields a value of unity. The quality index of the yellowfin seabream filets increased from 0.09 to 0.16 after the HP treatment, which comes below the range reported for sea bream (0.18 to 0.27) (Calanche et al., 2019). The summation of the EAA remained almost constant after pressurization, whereas the NEAA dropped to half of its original value (Table 1). The change in the quality index could be happening because of chemical and enzymatic reactions, where they act as substrates leading to the formation of secondary products (Ruiz et al., 1999).
Impact of Pressurization on Free Fatty Acid Profile
The fatty acid composition of yellowfin seabream filets before and after pressure treatment is reported in Table 2. The filets without pressurization had a marginally higher percentage of saturated fatty acids (SFAs; 39.79%) over the monounsaturated fatty acids (MUFAs; 38.17%) and the polyunsaturated fatty acids (PUFAs; 17.13%). The obtained values of SFAs, MUFAs, and PUFAs differed significantly when compared with the values reported for the yellow seabream muscle (Mohammad, 2011). The SFA, MUFA, and PUFA values of the liver and muscles of wild and cultured male and female A. latus were 19.45 to 24.17, 16.86 to 21.40, and 57.10 to 64.24%, respectively. Such a large difference in these values could be attributed to fish species, diet, location, sex, and other factors. Palmitic acid (C16:0) was the predominating SFA, accounting for 25.59% of the total fatty acids, followed by stearic acid (C18:0; 8.37%) and myristic acid (C14:0; 3.17%). The observed C16:0 was significantly higher than the reported values for yellowfin seabream (8.49 to 10.54%), while the C18:0 and C14:0 values were comparable (Mohammad, 2011). Conversely, C16:0 and C18:0 for the pomfret muscle were found to be relatively higher, while C14:0 was lower than the observed values (Zhao et al., 2010). Among the MUFAs, oleic acid (C18:1n9c; 29.88%) was the most abundant monounsaturated fatty acid. The n-6 PUFAs had a significantly higher value (13.97%) than that of the n-3 PUFAs (7.23%), and the ratio of n-3/n-6 was found to be 0.52. DHA (C22:6n3, 4.46%) was the dominating PUFA in the filets, followed by arachidonic acid (AA, C20:4n6, 2.66%) and EPA (C20:5n3, 1.26%) (Table 2). A similar observation was reported for yellowfin seabream, where C18:1n9c and DHA were the dominant fatty acids (Mohammad, 2011).
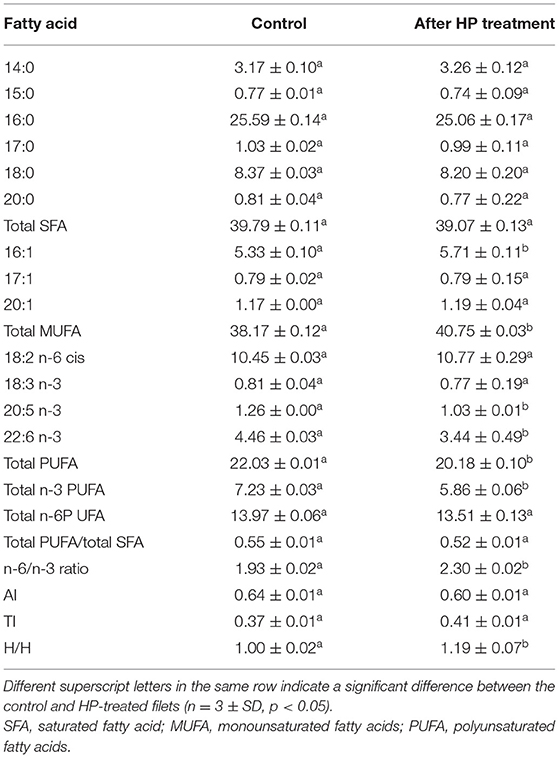
Table 2. Influence of high-pressure (HP) treatment on the fatty acid profile of yellowfin seabream fish.
Pressurization (375 MPa/20 min) significantly influenced the MUFA and PUFA of yellowfin seabream filet (P < 0.05), while the decrease in total SFA was insignificant (P > 0.05) (Table 2). After the HP treatment, each individual SFA, except C14:0, was marginally decreased, leading to a drop in the overall SFA value from 39.79 to 39.07% (P > 0.05). The drop in SFA value was mainly attributed to the decrease in palmitic acid (C16:0, >2%) after the HP treatment. However, the reason is not clear. The changes in SFA content with pressurization are consistent with the results reported earlier for salmon filet (Yagiz et al., 2009), where no changes in the composition of C14:0, C16:0, and C18:0 were observed after the HP treatment (150 MPa/300 MPa for 15 min at 20°C). Conversely, Marques et al. (2019) found an increase in the SFA contents of differently sized ragworms (Hediste diversicolor) after the HP treatment. The total and individual MUFA contents increased insignificantly after the pressure treatment (Table 2). A similar increasing trend of MUFA has been reported for ragworms after the treatment (Marques et al., 2019). All three major PUFAs, namely, AA, EPA, and DHA, were decreased significantly from 2.66 to 2.01, 1.26 to 1.03, and 4.46 to 3.44%, respectively, after the HP treatment.
The significant decrease in individual and total PUFA of yellowfin seabream after the pressure treatment was supported by previous studies for different fish species. A similar significant decrease in PUFAs after the HP treatment was reported for Atlantic salmon (300 MPa/15 min), mackerel filets (100 MPa/5 min, 300 MPa/2 min/5 min, and 500 MPa/2 min/5 min), and pork (>350 MPa) (Yagiz et al., 2009; He et al., 2012; Alba et al., 2019). The decrease in PUFA has been postulated as due to the damage of the cell membrane imparted by the treatment, which releases the lipases and free metal ions (Orlien et al., 2000). Unsaturated fatty acids including DHA are prone to oxidation because of the presence of double bonds (Barba et al., 2015). Additionally, the cell membranes are rich in PUFA, so an increase in the rate of the hydrolysis of PUFAs (e.g., DHA from disorganized cell membranes) and succeeding oxidation or direct oxidation of PUFA without previous hydrolysis due to cell damage are expected (Xu et al., 2008).
The high dietary (n-6)/(n-3) PUFA ratio has a significant role in preventing many non-communicable diseases, including cancer and cardiovascular disease (Nindrea et al., 2019). An increased dietary intake enriched with (n-3) PUFA, EPA, DHA, and linolenic acid decreases the desaturation of linoleic acid by producing AA and improves human health (Rose and Connolly, 1999). A PUFA-to-SFA ratio of not less than 0.1 coupled with n6/n3 ratio of below 4 is recommended for the prevention of non-communicable diseases in humans (HMSO, 1994). The HP treatment did not affect the PUFA/SFA significantly (P > 0.05), whereas the n6/n3 ratio increased significantly from 1.93 to 2.30 (P < 0.05), although the values remained within the recommended value of <4.
Lipid Quality Indices
The quality of the lipid fraction in the yellowfin seabream filet can be assessed by measuring the nutritional quality indices from the fatty acid composition data. The quality indices AI, TI, and hypocholesterolemic fatty acids/hypercholesterolemic fatty acids (H/H) are reported in Table 2. Since AI and TI are correlated to the risk of atherosclerosis and platelet aggregation, a minimum value of AI and TI, respectively, is desired for protection against coronary heart disease (Turan et al., 2007). The AI of yellowfin seabream filets decreased marginally from 0.64 to 0.60 after the pressure treatment (P > 0.05). This observation is contradictory with the value reported for mackerel, where the AI value has been reported from 0.71 to 0.90 after a pressure treatment of 300 MPa (Alba et al., 2019). An increase in TI from 0.37 to 0.41 after the HP treatment is well supported by the work of Alba et al. (2019) for pressurized mackerel. Fernandes et al. (2014) reported the AI and TI values for sardines and mackerel as 0.60 and 0.48 and as 0.20 and 0.24, respectively, and they opined that the optimum AI and TI values should be up to 1 and 0.5 to get maximum health benefits from the fish. The H/H ratio is linked to cholesterol metabolism (Testi et al., 2006). The H/H value for seabream filet increased insignificantly from 1 to 1.19 after the treatment (P > 0.05). A higher H/H value of 1.63 to 1.93 has been reported for tilapia filets (Monteiro et al., 2017).
Effect of HP on Color and Texture Properties
The tristimulus color values, namely, L*, a*, and b* values, of untreated yellowfin seabream fish filets were 57.39 ± 0.14, 2.32 ± 0.11, and 11.54 ± 0.52, respectively. After the HP treatment, the lightness (L*) value did not significantly decrease (56.74 ± 0.44; P > 0.05), indicating the maintenance of the whiteness of the filets. The redness (a*) and yellowness (b*) values, which are mainly attributed to pigments that are present (mostly myoglobin) in their flesh, increased significantly (P < 0.05) to 5.97 ± 0.14 and 13.46 ± 0.12 under the same conditions. It indicates that pressure significantly affected the pigments present in the filets. A similar increase in b* values has been reported for pressure-treated haddock and mackerel minces compared to untreated samples (Arnaud et al., 2018), and those authors advocated that protein and lipid oxidation reactions might be responsible for the increase in color after the HP treatment. Furthermore, the increase in yellow pigmentation with high pressure might be because of the accumulation of secondary lipid oxidation products between the aldehyde groups generated during the oxidation of lipids and free amino groups of phospholipids and proteins (Tokur and Korkmaz, 2007).
The TPA of yellowfin seabream filets indicated that the hardness (5.92 ± 0.58 N) and compressibility (24.51 ± 3.14 N) did not change significantly with the applied pressure; those values equaled 6.15 ± 1.66 N and 24.21 ± 4.22 N, respectively. However, the adhesiveness and cohesiveness of the HP-treated sample changed significantly from−1.49 to−0.31 N mm and−0.25 to−0.05, respectively. It has been reported that the textural properties of fish, including hardness, are dependent on pressure intensity and holding time, resulting in the highest values of hardness at 450 MPa/5 min for Atlantic mackerel (Aubourg et al., 2013). A significant change in the cohesiveness and adhesiveness in yellowfin seabream was well supported by Aubourg et al. (2013). Overall, there is no consensus to support a distinct effect of HP on the hardness of fish with increasing pressure; increases have been reported for a selected fish (e.g., cod treated at 200 or 500 MPa for 2 min), while others (e.g., mackerel or salmon) do not follow the trend when treated at 200 and 500 MPa for 2 min (Christensen et al., 2017). During high-pressure treatment, the fish protein undergoes denaturation, aggregation, changes in actin–myosin interaction, α-actinin release, and tissue compression; these changes play an active role in contributing to the textural properties of fish. A wide variation in texture parameters after HP treatment among fish species has been attributed to the intensity and duration of pressure, storage conditions, and methodology adopted.
Conclusions
The findings of this study demonstrated that the locally available yellowfin seabream is a good source of amino acids and free fatty acids. High-pressure processing (375 MPa/20 min) could have potential to produce safe fish filets as tested against L. monocytogenes, a common food pathogen. Pressurization affects the total amino acids insignificantly (P > 0.05), whereas the changes in free amino acids are found to be significant (P < 0.05). Pressure treatment had a little effect (P > 0.05) on SFA and MUFA. HP treatment significantly (P < 0.05) reduced the PUFA content, in particular, AA, EPA, and DHA. The nutritional quality index values calculated from the fatty acid profile confirmed the retention of AI, TI, and H/H after the HP treatment. The color pigments of the filets change significantly as evidenced through color a* and b* values. HP may have some negative impacts on PUFA, and so more studies are required to investigate those aspects so that the process could be useful in relation to the seafood industry.
Data Availability Statement
The raw data supporting the conclusions of this article will be made available by the authors, without undue reservation.
Author Contributions
JA contributed to writing—original draft and funding acquisition. SH, SA, MM, and LT contributed to formal analysis. All authors contributed to the article and approved the submitted version.
Funding
This work was supported by the Kuwait Institute for Scientific Research (Grant Number FB 117K).
Conflict of Interest
The authors declare that the research was conducted in the absence of any commercial or financial relationships that could be construed as a potential conflict of interest.
Publisher's Note
All claims expressed in this article are solely those of the authors and do not necessarily represent those of their affiliated organizations, or those of the publisher, the editors and the reviewers. Any product that may be evaluated in this article, or claim that may be made by its manufacturer, is not guaranteed or endorsed by the publisher.
References
Ahmed, J., Habeebullah, S. F. K., Alagarsamy, S., Thomas, L., Hussain, J., and Jacob, H. (2021a). High-pressure treatment of silver pomfret (Pampus argenteus): inactivation of Listeria monocytogenes, impact on amino acid profile, and changes during storage. J. Food Process. Preserv. 45, e15296. doi: 10.1111/jfpp.15296
Ahmed, J., Habeebullah, S. F. K., Thomas, L., Jacob, H., Mulla, M. Z., Jacob, H., et al. (2021b). Effect of high-pressure treatment and refrigerated storage on the amino acid profile, color, and texture of hammour (Epinephelus coioides) fillets. J. Food Process. Preserv. 45, e15977. doi: 10.1111/jfpp.15977
Alba, M., Pérez-Andrés, J. M., Harrison, S. M., Brunton, N. P., Burgess, C. M., and Tiwari, B. K. (2019). High pressure processing on microbial inactivation, quality parameters and nutritional quality indices of mackerel fillets. Innov. Food Sci. Emerg. Technol. 55, 80–87. doi: 10.1016/j.ifset.2019.05.010
Al-Ghamdi, S., Sonar, C. R., Patel, J., Albahr, Z., and Sablani, S. S. (2020). High pressure-assisted thermal sterilization of low-acid fruit and vegetable purees: microbial safety, nutrient, quality, and packaging evaluation. Food Control 114, 107233. doi: 10.1016/j.foodcont.2020.107233
AOCS. (1998). Official Methods and Recommended Practices of the American Oil Chemists' Society. Champaign, IL: American Oil Chemists' Society.
Arnaud, C., Lamballerie, M., and Pottier, L. (2018). Effect of high pressure processing on the preservation of frozen and re-thawed sliced cod (Gadus morhua) and salmon (Salmo salar) fillets. High Press. Res. 38, 62–79. doi: 10.1080/08957959.2017.1399372
Aubourg, S. P., Torres, J. A., Saraiva, J. A., Guerra-Rodríguez, E., and Vázquez, M. (2013). Effect of high-pressure treatments applied before freezing and frozen storage on the functional and sensory properties of Atlantic mackerel (Scomber scombrus). LWT Food Sci. Technol. 53, 100–106. doi: 10.1016/j.lwt.2013.01.028
Barba, F. J., Poojary, M. M., Wang, J., Olsena, K., and Orlien, V. (2017). Effect of high pressure processing and storage on the free amino acids in seedlings of Brussels sprouts. Innov. Food Sci. Emerg. Technol. 41, 188–192. doi: 10.1016/j.ifset.2017.03.004
Barba, F. J., Terefe, N. S., Buckow, R., Knorr, D., and Orlien, V. (2015). New opportunities and perspectives of high-pressure treatment to improve health and safety attributes of foods. A review. Food Res. Int. 77, 725–742. doi: 10.1016/j.foodres.2015.05.015
Bligh, E. G., and Dyer, W. J. (1959). A rapid method of total lipid extraction and purification. Can. J. Biochem. Physiol. 37, 911–917. doi: 10.1139/y59-099
Calanche, J., Tomas, A., Martinez, S., Jover, M., Alonso, V., Roncalés, P., et al. (2019). Relation of quality and sensory perception with changes in free amino acids of thawed seabream (Sparus aurata). Food Res. Int. 119, 126–134. doi: 10.1016/j.foodres.2019.01.050
Cepero-Betancourta, Y., Opazo-Navarrete, M., Janssen, A. E. M., Tabilo-Munizaga, G., and Pérez-Won, M. (2020). Effects of high hydrostatic pressure (HHP) on protein structure and digestibility of red abalone (Haliotis rufescens) muscle. Innov. Food Sci. Emerg. Technol. 60, 102282. doi: 10.1016/j.ifset.2019.102282
Christensen, L. B., Hovda, M. B., and Rode, T. M. (2017). Quality changes in high pressure processed cod, salmon and mackerel during storage. Food Control 72, 90–96. doi: 10.1016/j.foodcont.2016.07.037
Ciampa, A., Picone, G., Laghi, L., Nikzad, H., and Capozzi, F. (2012). Changes in the amino acid composition of Bogue (Boops boops) fish during storage at different temperatures by 1H-NMR spectroscopy. Nutrients. 4, 542–553. doi: 10.3390/nu4060542
Considine, K. M., Kelly, A. L., Fitzgerald, G. F., Hill, C., and Sleator, R. D. (2008). High-pressure processing-effects on microbial food safety and food quality. FEMS Microbiol. Lett. 281, 1–9. doi: 10.1111/j.1574-6968.2008.01084.x
Cropotova, J., Mozuraityte, R., Standal, I. B., Ojha, S., Rustad, T., and Tiwari, B. (2020). Influence of high-pressure processing on quality attributes of haddock and mackerel minces during frozen storage, and fishcakes prepared thereof. Innov. Food Sci. Emerg. Technol. 59, 102236. doi: 10.1016/j.ifset.2019.102236
Das, S. K. V., Joseph, L. G., Kamalakanth, C. K., and Bindu, J. (2016). High pressure destruction kinetics along with combined effect of potassium sorbate and high pressure against Listeria monocytogenes in Indian white prawn muscle. Ann. Microbiol. 66, 245–251. doi: 10.1007/s13213-015-1100-7
ElShehawy, S. M., Gab-Alla, A. A., and Mutwally, H. M. A. (2016). Amino acids pattern and fatty acids composition of the most important fish species of Saudi Arabia. Int. J. Food Sci. Nutr. Eng. 6, 32–41. doi: 10.5923/j.food.20160602.02
Fernandes, C. E., Vasconcelos, M. A. S., Ribeiro, M. A., Sarubbo, L. A., Andrade, S. A. C., and Filho, A. B. M. (2014). Nutritional and lipid profiles in marine fish species from Brazil. Food Chem. 160, 67–71. doi: 10.1016/j.foodchem.2014.03.055
Gomez-Limia, L., Franco, I., and Martínez-Su'arez, S. (2021). Effects of processing step, filling medium and storage on amino acid profiles and protein quality in canned European eels. J. Food Compos. Anal. 96, 103710. doi: 10.1016/j.jfca.2020.103710
Hayashi, M. (1993). “Sparidae,” in Fishes of Japan With Pictorial Key to the Species, ed T. Nakabo. 1st Edn. Tokyo: Tokai University Press. p. 746–749.
He, Z., Huang, Y., Li, H., Qin, G., Wang, T., and Yang, J. (2012). Effect of high-pressure treatment on the fatty acid composition of intramuscular lipid in pork. Meat Sci. 90, 170–175. doi: 10.1016/j.meatsci.2011.06.022
HMSO. (1994). Nutritional Aspects of Cardiovascular Disease: Report on Health and Social Subjects. London: HMSO Department of Health.
Hughes, B. H., Greenberg, N. J., Yang, T. C., and Skonberg, D. I. (2015). Effects of rigor status during high-pressure processing on the physical qualities of farm-raised abalone (Haliotis rufescens). J. Food Sci. 80, C40–C48. doi: 10.1111/1750-3841.12717
Jo, Y. J., Jung, K. H., Lee, M. Y., Choi, M. J., Min, S. G., and Hong, G. P. (2014). Effect of high-pressure short-time processing on the physicochemical properties of abalone (Haliotis discus hannai) during refrigerated storage. Innov. Food Sci. Emerg. Technol. 23, 33–38. doi: 10.1016/j.ifset.2014.02.011
Karlsdottir, M. G., Sveinsdottir, K., Kristinsson, H. G., Villotd, D., Craft, B. D., and Arason, S. (2014). Effects of temperature during frozen storage on lipid deterioration of saithe (Pollachius virens) and hoki (Macruronus novaezelandiae). Food Chem. 156, 234–242 doi: 10.1016/j.foodchem.2014.01.113
Kim, B.-S, Oh, B.-J, Lee, J.-H, Yoon, Y. S, and Lee, H.-I. (2020). Effects of various drying methods on physicochemical characteristics and textural features of yellow croaker (Larimichthys polyactis). Foods 9, 196; doi: 10.3390/foods9020196
Lin, G., Zheng, M., Li, S., Xi, J., Fang, W., Gao, D., et al. (2020). Response of gut microbiota and immune function to hypoosmotic stress in the yellowfin seabream (Acanthopagrus latus). Sci. Total Environ. 745, 140976. doi: 10.1016/j.scitotenv.2020.140976
Liu, C., Gu, Z., Lin, X., Wang, Y., Wang, A, Sun, Y., et al. (2022). Effects of high hydrostatic pressure (HHP) and storage temperature on bacterial counts, color change, fatty acids and non-volatile taste active compounds of oysters (Crassostrea ariakensis). Food Chem. 372, 15131247. doi: 10.1016/j.foodchem.2021.131247
Lucore, L. A., Shellhammer, T. H., and Yousef, A. E. (2000). Inactivation of Listeria monocytogenes Scott A on artificially contaminated frankfurters by high-pressure processing. J. Food Protect. 63, 662–664. doi: 10.4315/0362-028X-63.5.662
Mai, J., Shetty, J. K., Kan, T. M., and Kinsella, J. E. (1980). Protein and amino acid composition of select freshwater fish. J. Agric. Food Chem. 28, 884–885. doi: 10.1021/jf60230a034
Marques, B., Lillebo, A. I., Domingues, M., Saraiva, J. A., and Calado, R. (2019). Effect of high-pressure processing (HPP) on the fatty acid profile of different sized ragworms (Hediste diversicolor) cultured in an integrated multi-trophic aquaculture (IMTA) system. Molecules 24, 4503. doi: 10.3390/molecules24244503
Mohammad, Z. (2011). Variations in lipid content and fatty acids compositionin wild and cultured yellowfin seabream, Acanthopagrus latus in the Persian Gulf. J. Persian Gulf Mar. Sci. 2, 53–61. Available online at: https://www.sid.ir/en/Journal/ViewPaper.aspx?ID=263832
Monroig, O., Tocher, D. R., and Castro, L. F. (2018). “Polyunsaturated fatty acid biosynthesis and metabolism in fish,” in Polyunsaturated Fatty Acid Metabolism, ed. G. C. Burdge (New York, NY: Academic Press and AOCS), 31–60.
Monteiro, M. L. G., M'arsico, E. T., Canto, A. C. V. S., Costa-Lima, B. R. C., Costa, M. P., Viana, F. M., et al. (2017). Impact of UV-C light on the fatty acid profile and oxidative stability of Nile tilapia (Oreochromis niloticus) fillets. J. Food Sci. 82, 1028–1035. doi: 10.1111/1750-3841.13685
Nindrea, R. D., Aryandono, T., Lazuardi, L., and Dwiprahasto, I. (2019). Association of dietary intake ratio of n-3/n-6 polyunsaturated fatty acids with breast cancer risk in Western and Asian countries: a meta-analysis. Asian Pac. J. Cancer Prevent. APJCP 20, 1321. doi: 10.31557/APJCP.2019.20.5.1321
Nordvi, B., Egelandsdal, B., Langsrud, O., Ofstad, R., and Slinde, E. (2007). Development of a novel, fermented and dried saithe and salmon product. Innov. Food Sci. Emerg. Technol. 8, 163–171. doi: 10.1016/j.ifset.2006.09.002
Orlien, V., Hansen, E., and Skibsted, L. H. (2000). Lipid oxidation in high-pressure processed chicken breast muscle during chill storage: critical working pressure in relation to oxidation mechanism. Eur. Food Res. Technol. 211, 99–104. doi: 10.1007/s002179900118
Reyes, J. E., Tabilo-Munizaga, G., Pérez-Won, M., Maluenda, D., and Rocob, T. (2015). Effect of high hydrostatic pressure (HHP) treatments on microbiological shelf-life of chilled Chilean jack mackerel (Trachurus murphyi). Innov. Food Sci. Emerg. Technol. 29 107–112. doi: 10.1016/j.ifset.2015.01.010
Rose, D. P., and Connolly, J. M. (1999). Omega-3 fatty acids as cancer chemopreventive agents. Pharmacol. Ther. 83, 217–244. doi: 10.1016/S0163-7258(99)00026-1
Ruiz, J., Garcia, C., Diaz, M. C., Cava, R., Tejeda, F. J., and Ventanas, J. (1999). Dry cured Iberian ham non-volatile components as affected by the length of the curing process. Food Res. Int. 32, 643–651. doi: 10.1016/S0963-9969(99)00142-8
Siewe, F. B., Kudre, T. G, Bettadaiah, B. K., and Narayan, B. (2020). Effects of ultrasound-assisted heating on aroma profile, peptide structure, peptide molecular weight, antioxidant activities and sensory characteristics of natural fish flavouring. Ultrason. Sonochem. 65, 105055. doi: 10.1016/j.ultsonch.2020.105055
Swendseid, M. E., Villalobos, J., and Friedrich, B. (1963). Ratios of essential-to-nonessential amino acids in plasma from rats fed different kinds and amounts of proteins and amino acids. J. Nutr. 80, 99–102.
Testi, S., Bonaldo, A., Gatta, P. P., and Badiani, A. (2006). Nutritional traits of dorsal and ventral fillets from three farmed fish species. Food Chem. 98, 104–111. doi: 10.1016/j.foodchem.2005.05.053
Tokur, B., and Korkmaz, K. (2007). The effects of an iron-catalyzed oxidation system on lipids and proteins of dark muscle fish. Food Chem. 104, 754–760. doi: 10.1016/j.foodchem.2006.12.033
Turan, H., Sonmez, G., and Kaya, Y. (2007). Fatty acid profile and proximate composition of the thornback ray (Raja clavata, L. 1758) from the Sinop coast in the Black Sea. J. Fish. Sci. 1, 97–103. doi: 10.3153/jfscom.2007012
Ueno, S., Shigematsu, T., Watanabe, T., Nakajima, K., Murakami, M., Hayashi, M., et al. (2010). Generation of free amino acids and γ- aminobutyric acid in water-soaked soybean by high-hydrostatic pressure processing. J. Agric. Food Chem. 58, 1208–1213. doi: 10.1021/jf903102t
Ulbricht, T. L. V., and Southgate, D. A. T. (1991). Coronary heart disease: seven dietary factors. Lancet 338, 985–992. doi: 10.1016/0140-6736(91)91846-M
Usydus, Z., Szlinder-Richert, J., Adamczyk, M., and Szatkowska, U. (2011). Marine and farmed fish in the Polish market: comparison of the nutritional value. Food Chem. 126, 78–84. doi: 10.1016/j.foodchem.2010.10.080
Wang, X., Wang, X., Feng, T., Shen, Y., and Xia, S. (2021). Saltiness perception enhancement of fish meat treated by microwave: The significance of conformational characteristics, water and sodium mobility. Food Chem. 347, 129033. doi: 10.1016/j.foodchem.2021.129033
Xu, W., Xu, X., Zhou, G., Wang, D., and Li, C. (2008). Changes of intramuscular phospholipids and free fatty acids during the processing of Nanjing dry-cured duck. Food Chem. 110, 279–284. doi: 10.1016/j.foodchem.2007.11.044
Yagiz, Y., Kristinsson, H. G., Balaban, M. O., Welt, B. A., Ralat, M., and Marshall, M. R. (2009). Effect of high pressure processing and cooking treatment on the quality of Atlantic salmon. Food Chem. 116, 828–835. doi: 10.1016/j.foodchem.2009.03.029
Yousef, A. E., and Courtney, P. D. (2003). “Basics of stress adaptation and implications in new-generation foods,” in Microbial Stress Adaptation and Food Safety, 1st Edition Edn., eds. Yousef, A. E. and Juneja, V. K. Boca Raton: CRC Press.
Zhao, F., Zhuang, P., Song, C., Shi, Z., and Zhang, L. (2010). Amino acid and fatty acid compositions and nutritional quality of muscle in the pomfret, Pampus punctatissimus. Food Chem. 118, 2, 224–227. doi: 10.1016/j.foodchem.2009.04.110
Keywords: high-pressure treatment, fatty acids profile, amino acids, atherogenicity index (AI), Listeria inactivation
Citation: Ahmed J, Habeebullah SFK, Alagarsamy S, Mulla MZ and Thomas L (2022) Impact of High-Pressure Treatment on Amino Acid Profile, Fatty Acid Compositions, and Texture of Yellowfin Seabream (Acanthopagrus arabicus) Filets. Front. Sustain. Food Syst. 6:857072. doi: 10.3389/fsufs.2022.857072
Received: 18 January 2022; Accepted: 21 February 2022;
Published: 07 April 2022.
Edited by:
Mahendran Radhakrishnan, Indian Institute of Food Processing Technology, IndiaReviewed by:
Liliana Gonçalves Fidalgo, Instituto Politécnico de Beja, PortugalRuta Galoburda, Latvia University of Life Sciences and Technologies, Latvia
Mansour Torfi Mozanzadeh, South Iran Aquaculture Research Center, Iran
Copyright © 2022 Ahmed, Habeebullah, Alagarsamy, Mulla and Thomas. This is an open-access article distributed under the terms of the Creative Commons Attribution License (CC BY). The use, distribution or reproduction in other forums is permitted, provided the original author(s) and the copyright owner(s) are credited and that the original publication in this journal is cited, in accordance with accepted academic practice. No use, distribution or reproduction is permitted which does not comply with these terms.
*Correspondence: Jasim Ahmed, amFobWVkMmtAeWFob28uY29t; amFhaG1lZEBraXNyLmVkdS5rdw==