- 1Department of Plant Agriculture, University of Guelph, Guelph, ON, Canada
- 2Harrow Research and Development Centre, Agriculture and Agri-Food Canada, Harrow, ON, Canada
World-wide, sustainable crop production is increasingly dependent on the protection of crops from adverse local climate conditions by using controlled environment agriculture (CEA) facilities. Today's greenhouses and plant factories are becoming very technologically advanced. Important breakthroughs in our understanding of the deployment of affordable artificial lighting systems that can supplement and even replace solar radiation is the subject of this perspective article. The key to improving sustainable CEA is to synchronize those environmental cues that best entrain the natural circadian rhythm of the crop. Patterns of circadian rhythms reflect the balance of daily metabolic cycles and phenological stages of development that integrate and anticipate environmental changes for all complex organisms. Within the last decade, our understanding of the use of light-emitting diodes (LEDs) as spectrally tunable tools for stimulating plant responses has expanded rapidly. This perspective proposes that extending the photoperiod in CEA is an economically sustainable goal to for year-round productivity of tomato, using dynamic LED shifts that entrain the circadian rhythm. When the photoperiod is extended too far, tomato experiences injury. To avoid yield reduction, we look to nature for clues, and how circadian rhythms evolved in general to long-photoperiods during the summer in high-latitudes. It follows that circadian rhythm traits are good targets for breeders to select new tomato cultivars suitable for CEA. Circadian rhythm entrainment, using dynamic LED cues, can be tailored to any latitude-of-origin crop, and thus expands the strategies ensuring sustainable food security including healthy diets locally in any region of the world.
Introduction
The strategy of using circadian rhythm (CR) entrainment principles to maximize yield sustainably is innovative and deserves application. In nature, plants evolved to predictable environmental cues that ensured fitness during different seasons (i.e., light quality, intensity, and duration). Controlled environment agriculture (CEA) facilities are sustainable production systems that can mimic nature's cues. This brief perspective article focuses on CR entrainment when designing lighting recipes, because light energy drives crop productivity.
To maximize CEA yields, implementing continuous lighting (CL) strategies is economically viable (Lanoue et al., 2019, 2021a,b; Hao et al., 2020a,b). Low intensity, long-photoperiod lighting can achieve the same daily light integral (DLI) as a short-photoperiod reliant on high light. DLI is understood to be a limiting factor of yield in CEA. Capital cost expenditure is reduced under CL strategies, since fewer fixtures are required. Furthermore, lighting during the nighttime greatly reduces the electricity costs as many countries have off-peak pricing during the night. Utilizing off-peak electricity lessens the demand on power grids. In plant factories, where air-conditioning consumes 25% of total electricity, low-intensity light at night imposes a lower thermal load, reducing costs (Kozai and Niu, 2016a,b). One possible problem of CL in greenhouses is a nuisance issue in urban areas because of light pollution. New policies are being developed to implement different strategies for deploying nighttime light-abatement curtains. Regardless, strategies using CL can sustainably reduce capital and electrical costs.
Crops respond differently to CL. While lettuce (Ohtake et al., 2018) and cucumber (Lanoue et al., 2021a) are relatively CL-tolerant, tomato (Hillman, 1956) and peppers (Demers and Gosselin, 2002) show photoperiodic injury (PI) characterized by interveinal chlorosis and reduced yield. Although, CL-tolerant tomato genotypes have been identified, this adaptation still needs acclimation. To effectively retain vegetative-generative balance and avoid decreasing leaf area, CL-tolerant transplants require 7-weeks of acclimation by incrementally increasing the photoperiod from 16 to 24 h (van Ieperen, 2016; Hao et al., 2018). PI has been a historical problem in trying to grow tomato under CL ever since artificial lighting became widespread (Garner and Allard, 1927).
A successful CL acclimation strategy, showing PI-tolerance, involved alternating red LEDs during daytime and low intensity blue LEDs during the night (12 h-R/12 h-LB) (Lanoue et al., 2019). During low natural light periods, late-November to early-December, tomato developed larger leaves, taller stems, and had higher yields at first harvest periods in January-February. During the later harvest periods in March-May, there was no significant difference in yield between alternating 12 h-R/12 h-LB and matched DLI treatment of non-alternating 12 h-R+LB/12 h-dark (Lanoue et al., 2019). Importantly, reversed 12 h-LB day/12 h-R night and 24 h-R+LB/0 h-dark induced the worst PI and yield reduction. Extending to 16 h-R/8 h-LB proved to be the best treatment in reducing light intensity by 12% while sustaining yield (Hao et al., 2020b).
Light quality plays a key role in PI. Lanoue et al. (2021b) showed that tomatoes grown under supplemental monochromatic red light has less PI and recovered faster when compared to broad spectrum lighting. Velez-Ramirez et al. (2019) showed improved PI-tolerance with supplemental far-red (FR) 24 h-HPS+FR/0 h-dark. FR has positive morphological effects on canopy architecture (Hao et al., 2016, 2017a; Kalaitzoglou et al., 2019; Zhang et al., 2019). Furthermore, FR increases dry matter partitioning to fruit through upregulation of sugar metabolism and transport, especially at anthesis, increasing sink strength (Ji et al., 2020).
It is well-known that a disrupted CR causes PI (Highkin and Hanson, 1954; Hillman, 1956; Velez-Ramirez et al., 2017a). But solving this problem requires applying CR entrainment principles. The CR phenotype, termed chronotype, governs crop responses to environmental cues, such as light and temperature shifts. Knowing the chronotype informs the grower how best to apply dynamic LED entrainment strategies that improve acclimation. We suggest that alternating lighting using LEDs can be considered as a CR entrainment strategy.
Tomato is our case study as it displays CR desynchrony symptoms as PI, has recent literature describing CR latitudinal-cline, and has demonstrated successful CL commercial-scale trials previously mentioned. The sections below will briefly walk-through CR entrainment principles and present the perspective that high-latitude chronotypes would be the best fit for CL in CEA. Finally, preliminary evidence using LEDs discusses whole-plant gas exchange patterns displaying entrained rhythmicity that leads to enhanced growth rate and no PI under CL, presents hypotheses and predictions on the nature of CR disruption in PI, comments on CR inputs other than light, and briefly points out methods for CR phenotyping.
Circadian Rhythm Entrainment
The CR can be viewed as an endogenous network of transcription factors, metabolites, protein products, and post-translational states often in negative feedback loops organized into a morning complex (MC), core complex (CC), and evening complex (EC) that oscillates with a period of ~24 h under constant free-running conditions (see reviews by McClung, 2006, 2019). The endogenous free-running CR period length under constant conditions (τ) should resonate with the environment period (T) for optimal fitness. For example, Arabidopsis and Cyanobacteria mutants with 20 h τ (non-24 h τ) grown in resonating 20 h T outperform mutants whose τ≠T, by comparing vegetative growth and competitive cultures, respectively (Dodd et al., 2005; Ma et al., 2013). Improved fitness was dependent on the τ-chronotype, regardless of which CR gene mutation that caused it (Ma et al., 2013). However, τ is not necessarily 24 h, unlike earth's rotation (T = 24 h), and the discrepancy can provide entrainment clues by investigating phase responses (ψ) (see “the art of entrainment” Roenneberg et al., 2003). Thus, an entrainment program refers to the daily phase-shifting cues that bring τ in resonance with T.
The endogenous phase at which an environmental input (i.e., light pulse) (zeitgeber, “time giver”) is given and the differential endogenous phase sensitivity to the zeitgeber will determine the ψ relationship (phase of entrainment) (Roenneberg et al., 2003). This differential sensitivity of a zeitgeber to phase of entrainment is also known as gating. Light sensitivity is generally gated at dawn and dusk enabling anticipation of the next day's length (McWatters and Devlin, 2011). Gated inputs may activate or repress regulatory components in a signal transduction pathway, interact directly in the cascade, or both (Hotta et al., 2007). A zeitgeber's influence on ψ may also be masked by its direct action (Roenneberg et al., 2003). The reader is referred to a few foundational studies on CR input photoreceptors, namely phytochromes (Phys) and cryptochromes (Crys), that describe light quality, light intensity, period shortening or lengthening, and their interactions on overall chronotype behaviour (Lin et al., 1998; Cantón and Quail, 1999; Tóth et al., 2001; Yanovsky et al., 2001; Millar, 2003; Hughes et al., 2012).
CR can be entrained parametrically, ψ being a continuous function of the input (i.e., gradual changes across light intensity), or non-parametrically, ψ being discretely shifted by an input (i.e., abrupt light pulses), with a consensus that both are involved (Aschoff, 1981; Pittendrigh, 1981; Daan, 2000). Webb et al. (2019) proposed the CR has dynamic plasticity; timing between CR components can change and accommodates both entrainment modes by considering oscillation velocity being accelerated or decelerated by an input (parametric) or by an abrupt phase-shift from a stimulation with no effect on velocity (non-parametric).
Understanding the “Latitudinal Rule” Proposed by Pittendrigh and Takamura
A central theme to this perspective is the latitudinal rule. Evolution seems to have adapted similar latitudinal gradients in chronotype across various organisms such as insects, fish, birds, mammals, and plants, however, it was best described in insect systems (Yerushalmi and Green, 2009; Hut et al., 2013). High-latitude chronotypes require different entrainment regimes than lower-latitude chronotypes and knowing these differences will help in developing compatible long-photoperiod dynamic LED recipes. Pittendrigh and Takamura (1989) found high-latitude Drosophila strains had longer τ than lower-latitude strains. High-latitude strains also had lower sensitivity to light intensity, making phase-shifts from a 15-min pulse for lower-latitude strains equivalent to a 120-min pulse for high-latitude strains. In phase response curve terminology, lower-latitude strains have strong resetting type-0 responses and high-latitude strains have weak resetting type-1 responses (Pittendrigh and Takamura, 1989).
Aschoff's rule (diurnal animal and plant τ lengthens under CL) was applied to build a model that incorporates latitudinal strain relationships (Pittendrigh and Takamura, 1989). The zeitgeber strength of light input, to achieve the necessary ψ for τ = T resonance, is conditionally compensated between intensity and duration. The compensation is conditional since high-latitudes have longer days and weaker intensity than lower-latitudes, a parametric trade-off, but there is also non- parametric trade-off in daily photoperiod changes. High-latitude adaptation uses a τ > T, creating a larger –ψ to keep up with greater daily photoperiod changes. Lower-latitudes donot need a longer τ, and subsequently do not require a larger –ψ, thus they rely on greater component sensitivity for fine-tuned ψ. In formulating a latitudinal rule by Pittendrigh and Takamura (1989), it seems τ and type-0/1 sensitivity are the main traits used for adaptation to seasonal variations in photoperiod across latitudes. Therefore, high-latitude strains have a long-τ and lower sensitivity (type-1). This conditionality on latitude-of-origin chronotype is crucial in understanding why permutations in DLI (via photoperiod or light intensity) do not impact all cultivars/ species similarly.
Part of the latitudinal rule was shown in plants while screening 150 Arabidopsis accessions that revealed a correlation between long-τ and long-photoperiod adaptation (Michael et al., 2003). Greenham et al. (2017) confirmed long-τ chronotypes in high-latitudes by observing its natural selection in wild Mimulus guttatus populations across a latitudinal gradient and unintentional breeding for it in soybean maturity groups. CR sensitivity, the other part of latitudinal rule, was shown by Gigantea expression, a CR component, being less sensitive to lengthening of photoperiod in high-latitude-of-origin Arabidopsis accessions (de Montaigu and Coupland, 2017).
Importantly, there is a link between the latitude-of-origin chronotype and phenotypic plasticity in response to photoperiod changes. Gigantea is pleiotropic, it interacts in the EC and has effects on the phenotype of stem growth rate. Counter-gradient variation was used to explain how all accessions retained a similar phenotype although they each grow in different latitude-of-origins, yet if one accession is grown in the other's latitude it would express a different phenotype (Conover and Schultz, 1995; de Montaigu and Coupland, 2017).
Applying the Latitudinal Rule to a Case Study of Tomato in CEA
Tomato has an altered phase and lengthened τ associated with domestication and northern migration to Europe (Müller et al., 2016, 2018), reinforcing the latitudinal rule. However, more studies are needed to verify the positive correlation between northern-latitude bred tomato cultivars and PI-tolerance under CL. Velez-Ramirez et al. (2014) noted the tomato cultivar “Sub-Arctic Plenty” had PI-tolerance independent of chlorophyl-a-b-binding-protein-13 (CAB-13) inferred PI-tolerance and proposed it was related to its northern-latitude breeding program (northern Alberta, Canada). As for sensitivity, PhyA and PhyB1 CR signaling pathways are altered (Müller et al., 2016, 2018). Velez-Ramirez et al. (2019) also identified PhyA and PhyB1 transduced PI-tolerance in a light quality-dependent manner. PhyB1 expression is upregulated more under CL in PI-tolerant genotypes (Velez-Ramirez et al., 2019). Furthermore, PhyB1 overexpression and light intensity-dependent signaling results in a longer τ (Somers et al., 1998; Salomé et al., 2002; Müller et al., 2018). These results suggest PI-tolerance may be obtained by long-τ chronotypes and altering PhyB1-/PhyA-dependent sensitivity.
A Simple Model of Solar Spectral Circadian Rhythm Inputs Driving the Latitudinal Rule
Natural CR entrainment patterns have evolved to the solar patterns prevalent at a geolocation as described previously. Although natural T = 24 h, the photoperiod within 24 h changes depending on season and latitude. For optimal fitness in the environment, τ = T, ψ must perfectly match the progression of seasonal photoperiod changes at a given latitude. Seasonal and latitudinal parametric entrainment cues (amplitude and acceleration) and non-parametric (daily dawn/dusk phase shifts in photoperiod) are proposed as key inputs that supply the CR with geotemporal information (Figure 1A).
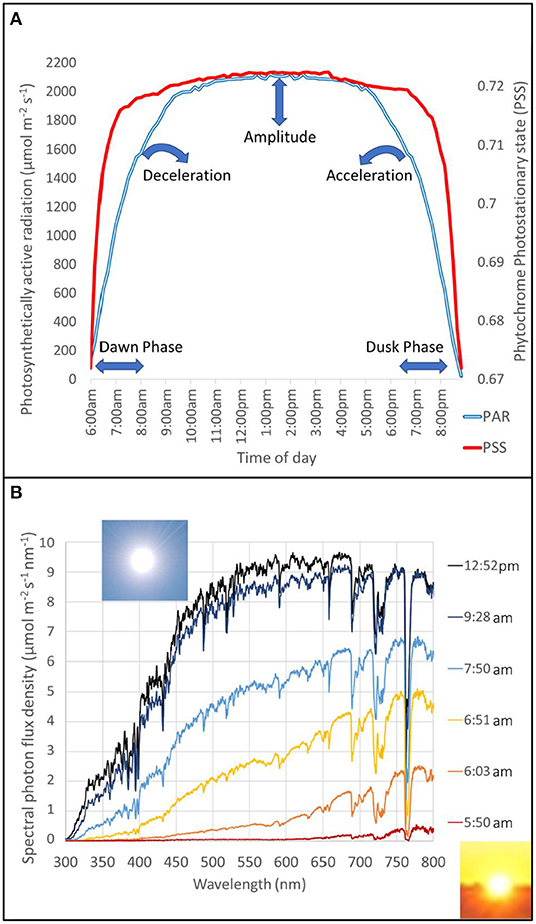
Figure 1. A simple model of seasonal and latitudinal zeitgeber patterns for plants and daily solar spectral shifts. Gating circadian light inputs during dawn and dusk is logical when considering the natural solar spectral shifts. There is a dramatic change in light quality and intensity during the first 2 h of the photoperiod whereas there is a minor change during the hours approaching solar noon (A,B). Spectral change during time-of-day (dawn to solar noon) with a few timepoints highlighted, and others omitted for clarity, is emphasized to note the difference between morning and solar noon (B). Measurements were taken every 10 min using Flame-S-XR1 (Ocean Optics Inc, Florida, USA) on Oceanview software with a 10 μm slit grating, 100 ms integration time, and a cosine corrector (CC-3-UV-S) pointed directly at the sun at every timepoint during the 2017 summer solstice in Guelph, Ontario, Canada (43.5°N, 80.2°W). Using this day-long dataset and datasets from different seasons (data not shown), timepoints were transformed to solar elevations using sun path maps (Oregon, 2007) to model the seasonal, and latitudinal trends (A). Phytochrome photostationary state (PSS) (Sager et al., 1988), was calculated to represent plant perceived light quality shifts, and photosynthetically active radiation (PAR) was calculated to represent plant perceived light intensity shifts (A). The daily pattern changes only in phase and amplitude over the seasons. It changes in phase, amplitude, and deceleration/acceleration across latitudes. Acceleration refers to differences in non-linear slopes of PAR and PSS. Acceleration of spectral change relates to Webb et al. (2019) parametric entrainment mode and Müller et al. (2016, 2018) describing the deceleration of the circadian clock in tomato's northward domestication. The proposed model of spectral changes can be used in tests on counter-gradient variation of phenotypes (for example leaf area and stem elongation) across latitude-of-origin chronotypes. The model shows a high-latitude will have a lower acceleration, in PSS and PAR, than a lower-latitude. We can set up two controlled environments, one matching the high-latitude and the other matching the lower-latitude PSS and PAR acceleration, and both set to a 12 h photoperiod to mimic the fall/spring equinox where both latitudes would experience the same photoperiod. Then, grow two different latitude-of-origin ecotypes/cultivars, one originating from a high-latitude and another from a lower-latitude, in each controlled environment. It is predicted that the high-latitude ecotype would have a relatively normal phenotype in the high-latitude environment whereas the lower-latitude ecotype would have an exaggerated phenotypic response in the high-latitude environment (larger leaf area and stem elongation). The opposite trend would be observed in the lower-latitude environment. This simplified experiment would support the notion that counter-gradient variation could normalize the phenotypic response if we took chronotypes into consideration and designed an LED recipe that altered the acceleration accordingly (or phase shifted a “critical PSS/PAR” value).
Assuming clear days, daily trends of light intensity and spectral quality are dependent on solar elevation. As the sun transits the sky, atmospheric thickness can be over 10-times greater near the horizon than at solar noon on a summer day (NOAA, 2021), which dramatically increases the path length that filters light quality non-uniformly through Rayleigh scattering and decreases light intensity uniformly through Mie scattering. Light quality shifts to its ~90% maximum much sooner (~1 h) than light intensity (~3–4 h) and then changes relatively little in late-morning to late-afternoon (Figures 1A,B). If the spectra are normalized to solar elevation, sun path maps (Oregon, 2007) can be used to predict patterns of daily, seasonal, and latitudinal changes. However, this method is limited as there are seasonal changes in atmospheric parameters (pressure, temperature, and humidity) and latitudinal differences in air mass. These differences could be accounted for in future models, nonetheless, a simplified model that used acquired solar spectral data and sun path maps is presented (Figure 1A). This model supports why light quality is a key gated CR input (through Phy pathways) and why this pathway is modified according to the latitudinal rule.
Discussion
Rhythmic Whole-Plant Gas Exchange Patterns Under Continuous Light
A preliminary CL dynamic LED recipe that produced a healthy tomato plant in a closed environment is presented that combines alternating spectra and FR (Figure 2). The diurnal net carbon exchange rate (NCER) and evapotranspiration (ET) data show rhythmic patterns (Figures 2B,C). Future work will extend the subjective day, compare bright pulses at different phases during morning to afternoon, and introduce FR pulses at different phases from end-of-day (EOD) to end-of-night (EON).
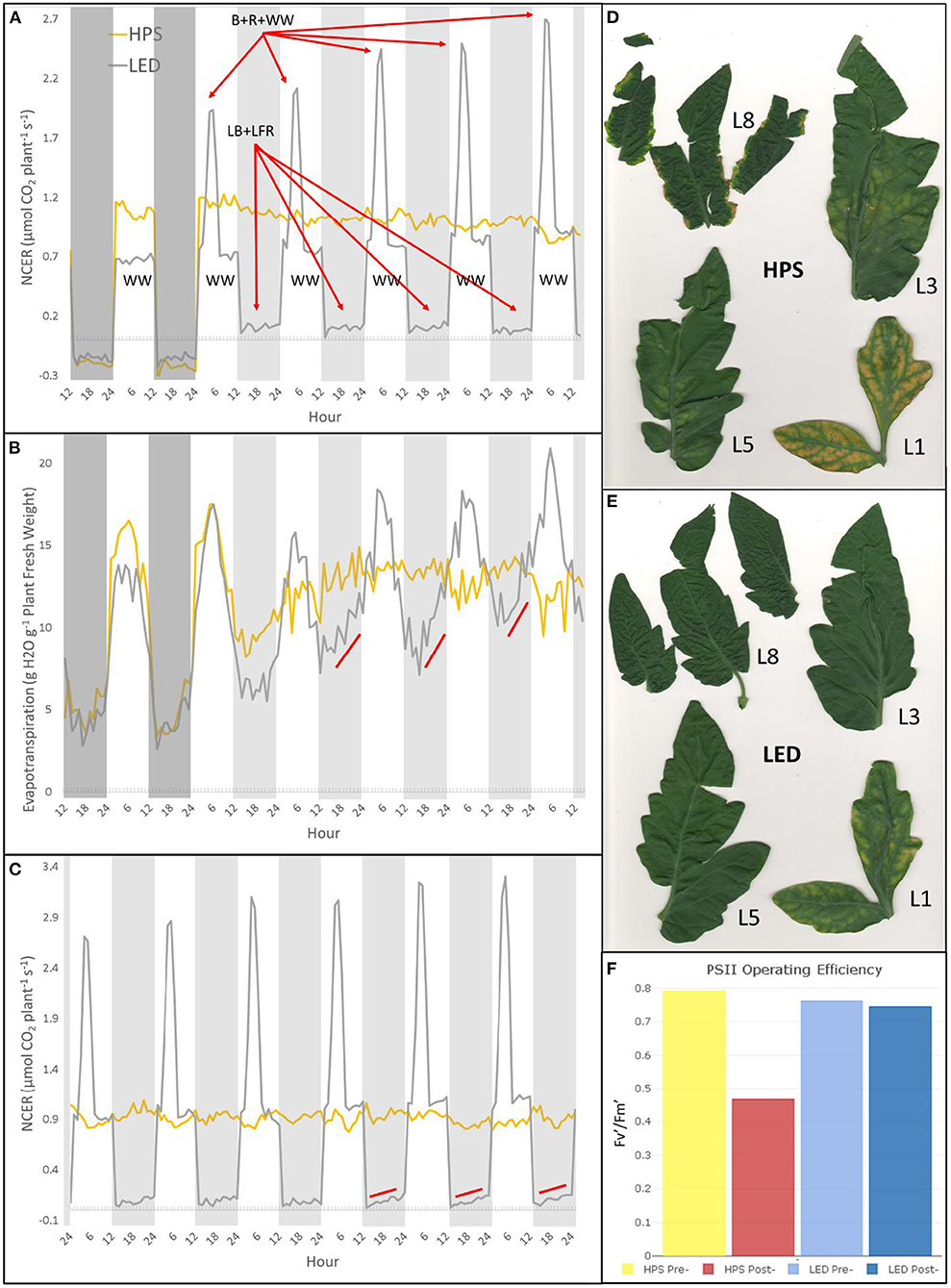
Figure 2. An example of circadian rhythm entrainment using a dynamic LED recipe in a whole-plant gas exchange system. The dynamic LED recipe, which combines Lanoue et al. (2019) and Velez-Ramirez et al. (2019) treatments, grows a healthy and rhythmic 5week-old tomato “Basket Vee” plant under continuous light without photoperiodic injury (LED, black line). A originally larger (evident by its higher whole-plant Net Carbon Exchange Rate) 5 week-old sister tomato “Basket Vee” plant, seeded and grown under same pre-treatment conditions in a growth chamber (12 h light/12 h dark), was treated at the same time, lost rhythmicity, and developed severe photoperiodic injury under continuous high-pressure sodium lights (HPS, yellow line). The data was collected by a controlled environment whole-plant system previously used to study light quality effects on net carbon exchange rate (NCER) and evapotranspiration (ET) (Lanoue et al., 2017, 2018; Leonardos et al., 2019). (A,B) are the first 6 days since the plants were placed in the whole-plant system, whereas (C) is the last 6 days showing NCER only. The total experiment spanned 12 subjective days and 14 subjective nights, with NCER measurements every 9 min (averaged every hour) and ET based on weigh scale logged every 30sec and summed every hour. Both plants were pre-entrained to 200 μmol m−2 s−1 of their respective light qualities, warm white (LED), and high-pressure sodium (HPS), under 12 h light/12 h dark. Then, for the dynamic recipe, the photoperiod was still 12 h subjective day/12 h subjective night, the photoperiod light is warm-white LED (3000K) at 200 μmol m−2 s−1, but in addition there was a 3.5 h pulse of red (R), blue (B), and warm-white (WW) LED of 400 μmol m−2 s−1 at zeitgeber time 2.5 (8 a.m. subjective day ON, 10:30 a.m. light pulse given), and the subjective night was 50 μmol m−2 s−1 low blue (LB) and 50 μmol m−2 s−1 low far-red (LFR) (12 h-WW + 3.5 h@ZT2.5 R+B+WW/12 h-LB+LFR). This recipe was repeated every 24 h. The bright morning pulse was meant to mimic what would happen in a greenhouse, where a section of canopy at some point of the morning would have direct sun exposure and then be shaded as the solar elevation changes. The dynamic LED recipe is compared to a sister plant grown under HPS at the same DLI with constant 200 μmol m−2 s−1 24 h day/0 h night (LED DLI calculation includes LFR, otherwise it's slightly lower than HPS DLI). HPS started off as a larger plant, but by 5 days its NCER decreased slightly, whereas LED NCER increased (A). Note, dynamic LED NCER is above the whole-plant compensation point during subjective night (A,C). The dynamic LED recipe also induced ET rhythms, with increases preceding subjective day (showing the increase is not a “masking” error) (B, slopped lines). HPS clearly shows a retention of ET rhythm on the first subjective night (notice ET decreases even though it was under continuous light), however HPS lost the detectable rhythm the following subjective night (B). HPS NCER and ET patterns do not show rhythms after the first subjective day/night of free-running conditions, unlike Arabidopsis in Dodd et al. (2004) that displayed clear rhythms, which could be due to tomato's weak rhythms. Also, on the 9th, 10th, and 11th day of treatment, after longer-term entrainment cycles, the dynamic LED recipe has clear NCER increases in anticipation of subjective day indicated with red sloped lines (C). The increase can be a rhythmic change in whole-plant radiation capture and/or a decrease in respiration and/or an increase photosynthetic performance. Regardless, this shows it takes time to entrain at the whole-plant level and there were observed rhythms in NCER that were not due to masking error. The LED plant clearly had healthy leaves, indicated by leaf number (D). Whereas HPS had accelerated senescence of L1, interveinal chlorosis in L3/L5, marginal chlorosis in L8, and more brittle leaves (E). Furthermore, photosystem II operating efficiency was measured as another proxy of leaf health on L3 before the whole-plant system run (Pre-) and after the run (Post-) (F). It remained high in the LED treatment, despite the ~2 weeks of leaf maturity difference, whereas it dropped nearly 50% in the HPS treatment (F).
Does Continuous Light Lock the Circadian Rhythm in Its Evening Complex?
Müller et al. (2016, see Section Applying the Latitudinal Rule to a Case Study of Tomato in CEA) showed TIMING-OF-CAB-EXPRESSION-1 expression patterns under CL, that suggest CR is “locked” in EC. Although the wild accession is transcriptionally rhythmic, its amplitude is relatively reduced by not being sufficiently repressed in its anti-peaks, supporting the need for entrainment cycles regardless of genotype. Being locked in EC can have repercussions on photosynthesis and other metabolic processes that are upregulated at dawn by the MC. It could provide a network-wide explanation as to why fitness associated with carbon metabolism declines under CL (i.e., photosynthetic genes are downregulated, senescence genes are upregulated, and starch degradation is inhibited) (Velez-Ramirez et al., 2017b, 2019; dos Anjos et al., 2018; Webb et al., 2019; Lee et al., 2021). It's possible that LB night treatment is essential in transitioning the CR out of EC. If so, temporal transcriptomics and carbon pools would reveal correlations dependent on LB night treatment between (a) the full anti-peak of EC CR transcripts by dawn and (b) the subsequent upregulation of MC CR transcripts at dawn. Starch pools are rhythmic under the LB night treatment, providing downstream support of the hypothesis (Lanoue et al., 2019).
Entraining With Other Environmental Inputs
All environmental inputs should be considered for their CR entrainment effects in addition to their traditional physical/physiological effects. For example, temperature shifts are very important inputs to CRs and can induce ψ for a robust entrainment program. This is distinguished from “temperature compensation,” as the latter refers to the retention of τ under a broad range of physiological temperatures once stabilized after >24 h (Pittendrigh, 1954). PI-tolerance has been found by providing temperature differentials (Haque et al., 2015, 2017) and temperature drops (Ikkonen et al., 2015; Hao et al., 2017b). Temperature drops have been successfully integrated into CL commercial production trials that resulted in reduced PI and increased yield (Hao et al., 2017b). Combining temperature differentials and drops with light quality and intensity shifts seems like an interesting area of study considering PhyB has been shown to integrate light and thermal signals (Legris et al., 2016).
Furthermore, PI-tolerance has been observed to depend on rootstock choice and the lighting environment effects on the rootstock (Lanoue et al., 2021b). A possible explanation, in context of this perspective, is that CR oscillators display organ specificity, with τ shown to be longer in roots than shoots and the roots are independently light sensitive (Bordage et al., 2016). Thus, long-distance rootstock-derived CR outputs may be inputs for scion CR entrainment. Also, rootzone entrainment, via irrigation timing, creates temperature, nutrient availability, and water potential rhythms.
Measuring and Testing Entrainment
Classifying the chronotype is an untapped technique that can resolve some apparently disparate cultivar responses to light quality and gives more breeding traits. Chronotyping could be accomplished via the TRiP screening protocol developed by Greenham et al. (2015) and laborious phase response curves may be simplified via the “singularity response” method given by Masuda et al. (2021). The end-goal is a cumulative combination (light quality, intensity, duration, and temperature shift induced phase responses) creating a net –ψ that makes τ resonate with T under CL. The latitudinal rule, natural seasonal/latitudinal spectral shift trends, and counter-gradient variation allow the initial prediction of phase responses. This can help guide dosage and phase of FR cues. It has been shown that FR-PhyA dependent hypocotyl elongation is a gated response to EOD and not EON (Seaton et al., 2018). If the hypothesis is correct, EOD-EON could be an adjustable timeframe to maintain a desired morphological phenotype consistently between cultivars with differing τ and type-0–1 sensitivity.
Conclusion
Our perspective is to apply CL in CEA with dynamic LED shifts that entrain the circadian rhythm of crops. It follows that traits such as long-τ chronotype and enhanced sensitivity are good targets for breeders designing new CEA crops (see McClung, 2021 for additional CR breeding considerations). However, it may be possible to accommodate chronotypes from all latitudes to CL by adjusting CR entrainment according to their phase responses. Ideally, a universal framework for CEA lighting will require a dynamic LED recipe for greenhouses, and another for closed systems lacking natural light. We propose a standardized day-spectrum, night-spectrum, and timed FR cues can predictably keep tomato entrained under CL (with future work on other CEA crops). The discussed dynamic entrainment ideas for CEA offer strategies for growers in any region of the world to contribute to nutritious sustainable food production (Marcone et al., 2020).
Data Availability Statement
The original contributions presented in the study are included in the article/supplementary material, further inquiries can be directed to the corresponding author/s.
Author Contributions
TM integrated the circadian concepts, curated solar spectral and plant data, analysed data, drafted, and revised the manuscript. EL curated the plant data and revised the manuscript. JL and XH informed commercial trials section and revised the manuscript. BM contributed to and funded solar spectra work in Figure 1 and revised the manuscript. BG helped fund, direct research, and revised the manuscript. All authors contributed to the article and approved the submitted version.
Funding
This study was supported grants from OMAFRA Alliance and OVGV (UG-T1-2021-100933) to BG. The Foundational Science Program of Agriculture and Agri-Food Canada (AAFC) (J-002228.001.04) to XH. Project #298244, Highly Qualified Personnel (HQP) Graduate Scholarship.
Conflict of Interest
The authors declare that the research was conducted in the absence of any commercial or financial relationships that could be construed as a potential conflict of interest.
Publisher's Note
All claims expressed in this article are solely those of the authors and do not necessarily represent those of their affiliated organizations, or those of the publisher, the editors and the reviewers. Any product that may be evaluated in this article, or claim that may be made by its manufacturer, is not guaranteed or endorsed by the publisher.
Acknowledgments
OMAFRA and University of Guelph partnership who appointed the Ontario greenhouse sector's off-season production goal as a research priority, accepting our proposal, and funding the acquisition and interpretation of solar data along with the initial circadian concepts. Jasmine Mah and Dr. David Llewellyn for their collaborations on calibrations, calculations, brainstorming, and friendly company while collecting solar spectra on several occasions. Drs. Kathleen Greenham and C. Robertson McClung whose enthusiasm, encouragement, and further correspondence after our lively discussion at the 2018 Plant Biology Conference in Montreal, QC, Canada (July 14–18th) inspired the latitudinal-cline concepts.
References
Aschoff, J. (1981). “Chapter 6: Freerunning and entrained circadian rhythms,” in Biological Rhythms, ed. J. Aschoff (Boston, MA: Springer), 81–93.
Bordage, S., Sullivan, S., Laird, J., Millar, A. J., and Nimmo, H. G. (2016). Organ specificity in the plant circadian system is explained by different light inputs to the shoot and root clocks. New Phytol. 212, 136–149. doi: 10.1111/nph.14024
Cantón, F. R., and Quail, P. H. (1999). Both phyA and phyB mediate light-imposed repression of PHYA gene expression in Arabidopsis. Plant Physiol. 121, 1207–1216. doi: 10.1104/pp.121.4.1207
Conover, D. O., and Schultz, E. T. (1995). Phenotypic similarity and the evolutionary significance of counter-gradient variation. Trends Ecol. Evol. 10, 248–252. doi: 10.1016/S0169-5347(00)89081-3
Daan, S. (2000). Colin Pittendrigh, Jürgen Aschoff, and the Natural Entrainment of Circadian Systems. At: The Colin S. Pittendrigh Lecture Presented at the Sixth Meeting of the Society for Research on Biological Rhythms, Amelia Island, May 9, 1998. J. Biol. Rhythms 15, 195–207. doi: 10.1177/074873040001500301
de Montaigu, A., and Coupland, G. (2017). The timing of GIGANTEA expression during day/night cycles varies with the geographical origin of Arabidopsis accessions. Plant Signal. Behav. 12, 1–7. doi: 10.1080/15592324.2017.1342026
Demers, D. A., and Gosselin, A. (2002). Growing greenhouse tomato and sweet pepper under supplemental lighting: optimal photoperiod, negative effects of long photoperiod and their causes. Acta. Hortic. 580, 83–88. doi: 10.17660/ActaHortic.2002.580.9
Dodd, A. N., Parkinson, K., and Webb, A. A. R. (2004). Independent circadian regulation of assimilation and stomatal conductance in the ztl-1 mutant of Arabidopsis. New Phytol. 162, 63–70. doi: 10.1111/j.1469-8137.2004.01005.x
Dodd, A. N., Salathia, N., Hall, A., Kévei, E., Tóth, R., Nagy, F., et al. (2005). Plant circadian clocks increase photosynthesis, growth, survival, and competitive advantage. Science 309, 630–633. doi: 10.1126/science.1115581
dos Anjos, L., Pandey, P. K., Moraes, T. A., Feil, R., Lunn, J. E., and Stitt, M. (2018). Feedback regulation by trehalose 6-phosphate slows down starch mobilization below the rate that would exhaust starch reserves at dawn in Arabidopsis leaves. Plant Direct. 2:e00078. doi: 10.1002/pld3.78
Garner, W. W., and Allard, H. A. (1927). Effect of short alternating periods of light and darkness on plant growth. Science 66, 40–42. doi: 10.1126/science.66.1697.40
Greenham, K., Lou, P., Puzey, J. R., Kumar, G., Arnevik, C., Farid, H., et al. (2017). Geographic variation of plant circadian clock function in natural and agricultural settings. J. Biol. Rhythms 32, 26–34. doi: 10.1177/0748730416679307
Greenham, K., Lou, P., Remsen, S. E., Farid, H., and McClung, C. R. (2015). TRiP: Tracking Rhythms in Plants, an automated leaf movement analysis program for circadian period estimation. Plant Methods 11, 1–11. doi: 10.1186/s13007-015-0075-5
Hao, X., Guo, X., Lanoue, J., Zhang, Y., Cao, R., Zheng, J., et al. (2018). A review on smart application of supplemental lighting in greenhouse fruiting vegetable production. Acta Hortic. 1227, 499–506. doi: 10.17660/ActaHortic.2018.1227.63
Hao, X., Guo, X., Zheng, J., Celeste, L., Khosla, S., and Chen, X. (2017a). Response of greenhouse tomato to different vertical spectra of LED lighting under overhead high pressure sodium and plasma lighting. Acta Hortic. 1170, 1003–1110. doi: 10.17660/ActaHortic.2017.1170.129
Hao, X., Lanoue, J., and Zheng, J. (2020a). Continuous LED lighting can significantly reduce light fixture costs without compromising fruit yield and quality in greenhouse cucumber production. Hortscience 55:S363. doi: 10.3390/plants10020378
Hao, X., Lanoue, J., Zheng, J., and Khosta, S. (2020b). Daytime red and nighttime blue led lighting allows injury-free production in greenhouse tomatoes grown with continuous (24 h) lighting. Hortscience 55, S31–S32. doi: 10.3389/fpls.2019.01114
Hao, X., Little, C., Zheng, M. J., and Cao, R. (2016). Far-red LEDs improve fruit production in greenhouse tomato grown under high-pressure sodium lighting. Acta Hortic. 1134, 95–102. doi: 10.17660/ActaHortic.2016.1134.13
Hao, X., Zhang, Y., Guo, X., Little, C., Zheng, J. M., and Khosla, S. (2017b). Temperature drop improved responses of greenhouse fruit vegetables to long photoperiod of supplemental lighting. Acta Hortic. 1182, 185–192. doi: 10.17660/ActaHortic.2017.1182.22
Haque, S. M., de Sousa, A., Soares, C., Kjaer, H. K., Fidalgo, F., Rosenqvist, E., et al. (2017). Temperature variation under continuous light restores tomato leaf photosynthesis and maintains the diurnal pattern in stomatal conductance. Front. Plant Sci. 8:1602. doi: 10.3389/fpls.2017.01602
Haque, S. M., Kjaer, H. K., Rosenqvist, E., and Ottosen, C.-O. (2015). Continuous light increases growth, daily carbon gain, antioxidants, and alters carbohydrate metabolism in a cultivated and a wild tomato species. Front. Plant Sci. 6:522. doi: 10.3389/fpls.2015.00522
Highkin, R. H., and Hanson, B. J. (1954). Possible interaction between light-dark cycles and endogenous daily rhythms on the growth of tomato plants. Plant Physiol. 29, 301–302. doi: 10.1104/pp.29.3.301
Hillman, S. W. (1956). Injury of tomato plants by continuous light and unfavorable photoperiodic cycles. Am. J. Bot. 43, 89–96. doi: 10.1002/j.1537-2197.1956.tb10469.x
Hotta, T. C., Gardner, J. M., Hubbard, E. K., Baek, J. S., Dalchau, N., Suhita, D., et al. (2007). Modulation of environmental responses of plants by circadian clocks. Plant Cell Environ. 30, 333–349. doi: 10.1111/j.1365-3040.2006.01627.x
Hughes, M. R., Vrana, D. J., Song, J., and Tucker, L. C. (2012). Light-dependent, dark-promoted interaction between Arabidopsis cryptochrome 1 and phytochrome B proteins. J. Biol. Chem. 287, 22165–22172. doi: 10.1074/jbc.M112.360545
Hut, R. A., Paolucci, S., Dor, R., Kyriacou, C. P., and Daan, S. (2013). Latitudinal clines: an evolutionary view on biological rhythms. Proc. R. Soc. B. 280, 1–9. doi: 10.1098/rspb.2013.0433
Ikkonen, E. N., Shibaeva, T. G., Rosenqvist, E., and Ottosen, C.-O. (2015). Daily temperature drop prevents inhibition of photosynthesis in tomato plants under continuous light. Photosynthetica 53, 389–394. doi: 10.1007/s11099-015-0115-4
Ji, Y., Ocaña, D. N., Choe, D., Larsen, D. H., Marcelis, L. F. M., and Heuvelink, E. (2020). Far-red radiation stimulates dry mass partitioning to fruits by increasing fruit sink strength in tomato. New Phytol. 228, 1914–1925. doi: 10.1111/nph.16805
Kalaitzoglou, P., van Ieperen, W., Harbinson, J., van der Meer, M., Martinakos, S., Weerheim, K., et al. (2019). Effects of continuous or end-of-day far-red light on tomato plant growth, morphology, light absorption, and fruit production. Front. Plant Sci. 10:322. doi: 10.3389/fpls.2019.00322
Kozai, T., and Niu, G. (2016a). “Chapter 2: Role of the plant factory with artificial lighting (PFAL) in urban areas. plant production system,” in Plant Factory, eds. T. Kozai, G. Niu, and M. Takagaki (London: Academic Press), 7–33.
Kozai, T., and Niu, G. (2016b). “Chapter 4: Plant factory as a resource-efficient closed plant production system,” in Plant Factory, eds. T. Kozai, G. Niu, and M. Takagaki (London: Academic Press), 69–90.
Lanoue, J., Leonardos, E. D., Khosla, S., Hao, X., and Grodzinski, B. (2018). Effect of elevated CO2 and spectral quality on whole plant gas exchange patterns in tomatoes. PLoS One 13:e0205861. doi: 10.1371/journal.pone.0205861
Lanoue, J., Leonardos, E. D., Ma, X., and Grodzinski, B. (2017). The effect of spectral quality on daily patterns of gas exchange, biomass gain, and water-use-efficiency in tomatoes and Lisianthus: an assessment of whole plant measurements. Front. Plant Sci. 8:1076. doi: 10.3389/fpls.2017.01076
Lanoue, J., Thibodeau, A., Little, C., Zheng, J., Grodzinski, B., and Hao, X. (2021b). Light spectra and root stocks affect response of greenhouse tomatoes to long photoperiod of supplemental lighting. Plants 10, 1–23. doi: 10.3390/plants10081674
Lanoue, J., Zheng, J., Little, C., Grodzinski, B., and Hao, X. (2021a). Continuous light does not compromise growth and yield in mini-cucumber greenhouse production with supplemental LED light. Plants 10, 1–18.
Lanoue, J., Zheng, J., Little, C., Thibodeau, A., Grodzinski, B., and Hao, X. (2019). Alternating red and blue light-emitting diodes allows for injury-free tomato production with continuous lighting. Front. Plant Sci. 10:1114.
Lee, J., Kang, M. H., Kim, J. Y., and Lim, P. O. (2021). The role of light and circadian clock in regulation of leaf senescence. Front. Plant Sci. 12:170. doi: 10.3389/fpls.2021.669170
Legris, M., Klose, C., Burgie, E. S., Rojas, C. C., Neme, M., Hiltbrunner, A., et al. (2016). Phytochrome B integrates light and temperature signals in Arabidopsis. Science. 354, 897–900. doi: 10.1126/science.aaf5656
Leonardos, E. D., Ma, X., Lanoue, J., and Grodzinski, B. (2019). Leaf and whole plant gas exchange and water-use-efficiency of chrysanthemums under HPS and LEDs during vegetative and flower induction stages. Can. J. Plant Sci. 99, 639–653. doi: 10.1139/cjps-2018-0245
Lin, C., Yang, H., Guo, H., Mockler, T., Chen, J., and Cashmore, R. A. (1998). Enhancement of blue-light sensitivity of Arabidopsis seedlings by a blue light receptor cryptochrome 2. Proc. Natl. Acad. Sci. 95, 2686–2690. doi: 10.1073/pnas.95.5.2686
Ma, P., Woelfle, M. A., and Johnson, C. H. (2013). An evolutionary fitness enhancement conferred by the circadian system in Cyanobacteria. Chaos Solit. Fractals 50, 65–74. doi: 10.1016/j.chaos.2012.11.006
Marcone, M. F., Madan, P., and Grodzinski, B. (2020). An overview of the sociological and environmental factors influencing eating food behavior in Canada. Front. Nutr. 7:77. doi: 10.3389/fnut.2020.00077
Masuda, K., Tokuda, I. T., Nakamichi, N., and Fukuda, H. (2021). The singularity response reveals entrainment properties of the plant circadian clock. Nat. Commun. 12, 1–7. doi: 10.1038/s41467-021-21167-7
McClung, C. R. (2006). Plant circadian rhythms. Plant Cell. 18, 792–803. doi: 10.1105/tpc.106.040980
McClung, C. R. (2021). Circadian clock components offer targets for crop domestication and improvement. Genes 12:374. doi: 10.3390/genes12030374
McWatters, G. H., and Devlin, F. P. (2011). Timing in plants - a rhythmic arrangement. FEBS Lett. 585, 1474–1484. doi: 10.1016/j.febslet.2011.03.051
Michael, T. P., Salome, P. A., Yu, H. J., Spencer, T. R., Sharp, E. L., and McPeek, M. A. (2003). Enhanced fitness conferred by naturally occurring variation in the circadian clock. Science 302, 1049–1053. doi: 10.1126/science.1082971
Millar, J. A. (2003). A suite of photoreceptors entrains the plant circadian clock. J. Biol. Rhythms 18, 217–226. doi: 10.1177/0748730403018003004
Müller, N. A., Wijnen, C. L., Srinivasan, A., Ryngajllo, M., Ofner, I., Lin, T., et al. (2016). Domestication selected for deceleration of the circadian clock in cultivated tomato. Nat. Genet. 48, 89–93. doi: 10.1038/ng.3447
Müller, N. A., Zhang, L., Koornneef, M., and Jiménez-Gómeza, J. M. (2018). Mutations in EID1 and LNK2 caused light-conditional clock deceleration during tomato domestication. Proc. Natl. Acad. Sci. 115, 7135–7140. doi: 10.1073/pnas.1801862115
NOAA (2021). Earth System Research Laboratory Global Monitoring Division, Solar Calculation Details. Available online at: https://www.esrl.noaa.gov/gmd/grad/solcalc/calcdetails.html (accessed January 13, 2022).
Ohtake, N., Ishikura, M., Suzuki, H., Yamori, W., and Goto, E. (2018). Continuous irradiation with alternating red and blue light enhances plant growth while keeping nutritional quality in lettuce. Hortscience 53, 1804–1809. doi: 10.21273/HORTSCI13469-18
Oregon. (2007). University of Oregon Solar Radiation Monitoring Laboratory. Sun Path Chart Program. Available online at: http://solardat.uoregon.edu/SunChartProgram.html (accessed January 13, 2022).
Pittendrigh, S. C. (1954). On temperature independence in the clock system controlling emergence time in Drosophila. Zoology 40, 1018–1029. doi: 10.1073/pnas.40.10.1018
Pittendrigh, S. C. (1981). “Chapter 7: Circadian systems: entrainment,” in Biological Rhythms, ed. J. Aschoff (Boston, MA: Springer), 95–124.
Pittendrigh, S. C., and Takamura, T. (1989). Latitudinal clines in the properties of a circadian pacemaker. J. Biol. Rhythms 4, 217–235. doi: 10.1177/074873048900400209
Roenneberg, T., Daan, S., and Merrow, M. (2003). The art of entrainment. J. Biol. Rhythms 18, 183–194. doi: 10.1177/0748730403018003001
Sager, C. J., Smith, O. W., Edwards, L. J., and Cyr, L. K. (1988). Photosynthetic efficiency and phytochrome photoequilibria determination using spectral data. Trans. ASABE 31, 1883–1889. doi: 10.13031/2013.30952
Salomé, A. P., Michael, P. T., Kearns, V. E., Fett-Neto, G. A., Sharrock, A. R., and McClung, C. R. (2002). The out of phase 1 mutant defines a role for PHYB in Circadian phase control in Arabidopsis. Plant Physiol. 129, 1674–1685. doi: 10.1104/pp.003418
Seaton, D. D., Toledo-Ortiz, G., Ganpudi, A., Kubota, A., Imaizumi, T., and Halliday, K. J. (2018). Dawn and photoperiod sensing by phytochrome A. Proc. Natl. Acad. Sci. 115, 10523–10528. doi: 10.1073/pnas.1803398115
Somers, D. E., Devlin, P. F., and Steve, K. A. (1998). Phytochromes and cryptochromes in the entrainment of the Arabidopsis circadian clock. Science 282, 1488–1490. doi: 10.1126/science.282.5393.1488
Tóth, R., Kevei, E., Hall, A., Millar, A. J., Nagy, F., and Kozma-Bognar, L. (2001). Circadian clock-regulated expression of phytochrome and cryptochrome genes in Arabidopsis. Plant Physiol. 127, 1607–1616. doi: 10.1104/pp.010467
van Ieperen, W. (2016). Plant growth control by light spectrum: fact or fiction? Acta Hortic. 1134, 19–24. doi: 10.17660/ActaHortic.2016.1134.3
Velez-Ramirez, A. I., Carreño-Quintero, N., Vreugdenhil, D., Millenaar, F. F., and van Ieperen, W. (2017b). Sucrose and starch content negatively correlates with PSII maximum quantum efficiency in tomato (Solanum lycopersicum) exposed to abnormal light/dark cycles and continuous light. Plant Cell Physiol. 58, 1339–1349. doi: 10.1093/pcp/pcx068
Velez-Ramirez, A. I., Dünner-Planella, G., Vreugdenhil, D., Millenaar, F. F., and van Ieperen, W. (2017a). On the induction of injury in tomato under continuous light: circadian asynchrony as the main triggering factor. Funct. Plant Biol. 44, 597–611. doi: 10.1071/FP16285
Velez-Ramirez, A. I., van Ieperen, W., Vreugdenhil, D., van Poppel, P. M. J. A., Heuvelink, E., and Millenaar, F. F. (2014). A single locus confers tolerance to continuous light and allows substantial yield increase in tomato. Nat. Commun. 5, 1–13. doi: 10.1038/ncomms5549
Velez-Ramirez, A. I., Vreugdenhil, D., Millenaar, F. F., and van Ieperen, W. (2019). Phytochrome A protects tomato plants from injuries induced by continuous light. Front. Plant Sci. 10:19. doi: 10.3389/fpls.2019.00019
Webb, A. A. R., Seki, M., Satake, A., and Caldana, C. (2019). Continuous dynamic adjustment of the plant circadian oscillator. Nat. Commun. 10, 1–9. doi: 10.1038/s41467-019-08398-5
Yanovsky, J. M., Mazzella, M. A., Whitelam, C. G., and Casal, J. J. (2001). Resetting of the circadian clock by phytochromes and cryptochromes in Arabidopsis. J. Biol. Rhythms 16, 523–530. doi: 10.1177/074873001129002213
Yerushalmi, S., and Green, R. M. (2009). Evidence for the adaptive significance of circadian rhythms. Ecol. Lett. 12, 970–981. doi: 10.1111/j.1461-0248.2009.01343.x
Keywords: circadian rhythm, LEDs, controlled environment agriculture (CEA), photoperiod, continuous light, tomato, photoperiodic injury
Citation: Marie TRJG, Leonardos ED, Lanoue J, Hao X, Micallef BJ and Grodzinski B (2022) A Perspective Emphasizing Circadian Rhythm Entrainment to Ensure Sustainable Crop Production in Controlled Environment Agriculture: Dynamic Use of LED Cues. Front. Sustain. Food Syst. 6:856162. doi: 10.3389/fsufs.2022.856162
Received: 16 January 2022; Accepted: 14 March 2022;
Published: 06 April 2022.
Edited by:
Autar Krishen Mattoo, Agricultural Research Service (USDA), United StatesReviewed by:
Srinidhi V. Holalu, University of California, Berkeley, United StatesJude E. Maul, United States Department of Agriculture, United States
Copyright © 2022 Marie, Leonardos, Lanoue, Hao, Micallef and Grodzinski. This is an open-access article distributed under the terms of the Creative Commons Attribution License (CC BY). The use, distribution or reproduction in other forums is permitted, provided the original author(s) and the copyright owner(s) are credited and that the original publication in this journal is cited, in accordance with accepted academic practice. No use, distribution or reproduction is permitted which does not comply with these terms.
*Correspondence: Telesphore R. J. G. Marie, TWFyaWV0JiN4MDAwNDA7dW9ndWVscGguY2E=