- 1Department of Food Science and Human Nutrition, Michigan State University, East Lansing, MI, United States
- 2Department of Animal Science, Michigan State University, East Lansing, MI, United States
As the global population increases, so does meat consumption. This trend is accompanied by concerns regarding the meat industry, and consumers are demanding transparency on the environmental and health effects of the products they are purchasing. Many leading health organizations recommend reducing red meat consumption. Nevertheless, no differentiation is made among red meats and beef. The beef production system is generally ignored despite nutritional differences between grain- and grass-fed beef. Compared to grain-fed beef, grass-fed beef contains a healthier fatty acid profile, including more omega-3 polyunsaturated fatty acids and conjugated linoleic acid, and increased concentrations of phytochemicals desired by health-conscious customers. However, there is a lack of consistency among grass-fed beef in the United States regarding clear product labeling and cattle dietary components. Grass-fed beef labeling confusion has emerged, including misunderstandings between grass-fed and grass-finished beef. Along with this, previous studies observed significant nutritional variation among grass-finished beef from different producers across the country. Cattle diet has the strongest influence on the nutritional composition of beef. Therefore, understanding differences in feeding practices is key to understanding differing nutritional quality of grass-fed beef. Feeding cattle diverse pastures composed of multiple plant species including grasses and legumes managed in a rotational grazing fashion results in higher omega-3 polyunsaturated fatty acids and phytochemical levels in beef compared to feedlots and monocultures. Seasonal differences including changes in temperature, rainfall, grazing practices, and plant growth cycles affect the nutritional composition of feeds and ultimately meat. Additional feeds utilized in grass-fed beef production systems such as conserved forages may reduce or increase health-promoting nutrients in grass-fed beef, while supplements such as grape byproducts and flaxseed may improve its nutritional profile. Further research should measure the effects of individual feedstuff and the finishing period on the nutritional profile on grass-fed beef. A better understanding of these details will be a step toward the standardization of pasture-raised ruminant products, strengthening the relationship between grass-fed beef consumption and human health.
Introduction
Globally, meat consumption continues to increase along with population and per capita income (Godfray et al., 2018). However, there are legitimate concerns regarding the sustainability of meat; greater consumption requires increased production and consequently, greater global warming, pollution, and water waste (Ritchie et al., 2018). Meat production is a source of methane emissions, accounting for ~15% of all anthropogenic emissions (Gerber et al., 2013). Further, it accounts for a third of all agricultural water use (Godfray et al., 2018). Consumers are becoming more cognizant of what they are purchasing and how it was produced and are willing to pay a premium price for local, healthy, and environmentally friendly products (Asioli et al., 2017). Some consumers are moving toward alternatives like plant-based products and are reducing their meat consumption (Hodson and Earle, 2018; Delon, 2019).
Meat is important in many cultures and humans have consumed meat for centuries because of its nutritional qualities as well as its taste (Pighin et al., 2016; Melendrez-Ruiz et al., 2019). Beef is highly nutrient-dense, providing energy, protein, fat, and other micronutrients like zinc, iron, selenium, and B vitamins (Omaye and Omaye, 2019). Beef is a significant source of desirable omega-3 (n−3) polyunsaturated fatty acids (PUFAs), including α-linolenic acid (ALA), eicosapentaenoic acid (EPA), docosapentaenoic acid (DPA), and docosahexaenoic acid (DHA) as well as ruminal trans fatty acids (FAs) such as conjugated linoleic acid (CLA) and trans vaccenic acid (TVA). These ruminal trans FAs are purported to have health-promoting benefits, including protection against the development of coronary heart disease (Scollan et al., 2014). However, the health benefits of ruminal trans FAs still require investigation. Some studies exploring the health effects of ruminal vs. industrial trans FAs found possible negative impacts (Gebauer et al., 2015; Verneque et al., 2020).
Grass-fed beef (GFB) meets the demands of consumers who are concerned about nutrition and the environment (Xue et al., 2010). Compared to grain-fed beef, grass-fed and grass-finished beef contains less total fat, less cholesterol, and less myristic and palmitic acids—saturated fatty acids (SFAs) found to be more deleterious for cholesterol levels (Ponnampalam et al., 2006; Alfaia et al., 2009). GFB contains twice as much CLA and up to 25% more PUFAs compared to conventional beef (Van Elswyk and Mcneill, 2014; Berthelot and Gruffat, 2018; Lenighan et al., 2019; Prache et al., 2020; Butler et al., 2021). Grass-fed production increases n−3 PUFAs without increasing omega-6 (n−6) PUFAs, reducing the n−6:n−3 ratio (Daley et al., 2010). Health-promoting phytochemicals including phenolics, terpenoids, and carotenoids are observed to be higher in GFB (Van Vliet et al., 2021b). Further, properly managed grass-fed systems encourage plants to sequester more carbon, promote plant diversity, and improve the quality of fresh-water systems (Godfray et al., 2018; Van Vliet et al., 2021b). Grass-fed systems utilize forage as a sustainable and available source of long-chain n−3 PUFAs as an alternative to marine sources (Scollan et al., 2014).
Grass-fed products usually command premiums in retail markets, but the definitions of what consumers are buying are not always clear (Bronkema et al., 2019). “Grass-fed” and “grass-finished” are often used interchangeably, but they do not necessarily refer to the same type of production. According to the USDA, beef can be labeled as “grass-fed” if cattle have been fed exclusively forages throughout their lifetime excluding milk from their mother and have continuous access to pasture throughout the growing season (Food Safety Inspection Service, 2019). While no grain or grain byproducts are permissible, additional forage sources such as hay, silage, or baleage may be provided to “grass-fed” cattle. Mathews and Johnson (2013) suggested some silages may consist of large amounts of grain. Definitions for “grass-finished” are less clear, though this typically refers to cattle that were fattened only on forages prior to slaughter (Mathews and Johnson, 2013).
Variations in “grass-fed” and “grass-finished” cattle diets, finishing date, and the addition of supplemental feeds can result in significant nutritional variation among beef products (Dewhurst et al., 2001; Revello-Chion et al., 2011; Bronkema et al., 2019; Jain et al., 2020). For instance, a nutritional survey of grass-finished beef found that the n−6:n−3 ratio varied from as low as 1.8:1 to as high as 28.3:1. Mineral and antioxidant content of grass-finished beef also varied significantly by producer (Bronkema et al., 2019). Further, cattle finished in the spring had greater n−3 and n−6 PUFAs compared to cattle finished in the fall (Jain et al., 2020). The surprising variations highlight the need to determine how various factors can influence the nutritional composition of grass-finished beef. The goal of this review is to analyze the influence of cattle diet, seasonal variations, and supplementation on the nutritional quality of grass-fed and grass-finished beef and discuss how these differences can impact human health.
How Grass-Fed and Grass-Finished Beef Align With Human Health
Health Rationale
Non-communicable diseases account for 41 million deaths each year. The two leading non-communicable diseases are cardiovascular diseases (CVDs) and cancer. Chronic disease and inflammation are influenced by environmental factors, with diet playing a significant role (Fritsche, 2015; World Health Organization, 2018; Sanchez-Rodriguez et al., 2019). Based on epidemiological studies, red meat consumption is often associated with increased risks of diabetes, CVDs, and cancer (Wolk, 2017). These claims led health organizations, such as the American Heart Association (AHA), to make public health recommendations to reduce red meat consumption (Arnett et al., 2019). However, epidemiological studies do not differentiate between production systems and types of red meat which are important factors affecting the nutritional profile (Provenza et al., 2019). Beef from grass-fed production systems is more consistent with nutritional recommendations, especially regarding n−3 PUFAs and phytochemicals (Vannice and Rasmussen, 2014; Omaye and Omaye, 2019; Van Vliet et al., 2021b). Omega-3 FAs are important compounds in foods that are linked to health benefits regarding reducing inflammation, blood triacylglycerols, and the risk of CVDs, depression, and arthritis (Calder, 2015; Saini and Keum, 2018). Further, phytochemicals including phenolic compounds also have multiple cardiovascular health benefits including protection against oxidative stress and modulation of blood pressure (Medina-Remón et al., 2015; Omaye and Omaye, 2019). Though public health recommendations suggest a decrease in red meat consumption to prevent chronic diseases, GFB addresses some of the nutritional concerns.
Fatty Acids, Phytochemicals, and Human Health
The typical Western diet is usually high in SFAs and n−6 PUFAs and deficient in n−3 PUFAs, related to an increased risk of developing CVDs, diabetes, obesity, and cancer (Simopoulos, 2002). However, FAs need to be considered individually to assess their effects on human health (Calder, 2015; Bloomfield et al., 2016). Saturated FAs as a whole are thought to promote inflammation and increase total low-density lipoprotein (LDL) cholesterol and insulin resistance. This is significant because LDL cholesterol is linked with incidence of coronary heart diseases (Billingsley et al., 2018). Therefore, SFAs increase the risk of CVDs, type 2 diabetes, and inflammation (Fritsche, 2015; Billingsley et al., 2018). However, not all SFAs have the same effects. Stearic acid, for example, has a neutral effect on LDL cholesterol, while myristic acid and palmitic acid have a total cholesterol-raising effect (FAO, 2010). Reduction of SFA consumption is usually linked to a replacement with other nutrients. When SFAs are replaced with refined carbohydrates, total serum cholesterol increases, along with the risk of developing CVDs (Dinicolantonio et al., 2016). Dietary intake of monounsaturated fatty acids (MUFAs) is thought to be beneficial for human health, especially when the increase of MUFAs is coupled with a decreased intake of SFAs. Oleic acid intake is associated with a lower risk of CVD and CVD mortality, while palmitoleic acid may increase insulin sensitivity and improve the blood lipid profile (Calder, 2015). Two important PUFAs are linoleic acid (LA) and ALA. The human body cannot synthesize these essential FAs, but they are important to human health as they are precursors for other long-chain PUFAs of interest including arachidonic acid, EPA, DPA, and DHA (Saini and Keum, 2018). Omega-3 PUFAs have anti-inflammatory effects while n−6 PUFAs do not (Simopoulos, 2006). The n−3 PUFAs DHA and EPA are linked to healthier cardiovascular function and can be synthesized from the precursor ALA (Parolini, 2019; Mendivil, 2021). However, the conversion from ALA to long-chain n−3 PUFAs remains low and is influenced by sex and LA concentrations (Harnack et al., 2009; Welch et al., 2010; Zhou et al., 2019). The n−6:n−3 ratio in the Western diet is estimated to be between 15:1 and 20:1 compared to 1:1 in wild animals or traditional human diets (Simopoulos, 2002, 2006). A lower n−6:n−3 ratio is considered important to prevent chronic diseases (Simopoulos, 2006; Husted and Bouzinova, 2016). Overall, because each FA has a different effect, and the relative proportions of each FA can change health outcomes, it is important to analyze the FA profile of beef to understand its effects on human health.
Unsaturated trans FAs are an important topic in the connection between FAs and human health. Unsaturated trans FAs have their double bonds in the trans configuration. With the usual configuration of unsaturated FAs being cis; trans FAs are formed either naturally via metabolic processes like microbial activity in ruminant animals or industrially by hydrogenation (Markiewicz-Keszycka et al., 2013; Calder, 2015). Each cis unsaturated FA can give multiple trans isomers, but the major ones include elaidic acid (trans C18:1 n−9), TVA (trans C18:1 n−11), and CLA (c9 t11 C18:2 and c12 t10 C18:2) (Calder, 2015). The c9 t11 CLA isomer is mainly found in bovine milk and meat, while the t10 c12 form is mainly found in processed oils (Lindmark Månsson, 2008; Calder, 2015; Alothman et al., 2019). Unsaturated trans FAs have different biological properties compared to the cis configuration, and their functions differ based on how they were produced. Trans FAs produced by industrial hydrogenation of plant oils are related to higher risks of CVDs compared to other FA classes (Calder, 2015; Del Razo Olvera et al., 2017; Qiu et al., 2018). On the other hand, trans FAs created by biohydrogenation in ruminants (TVA and CLA) are not associated with heart disease (Kalač, 2011). CLA, especially the c9 t11 isomer, and its precursor TVA, are purported to have health benefits, including managing insulin resistance and blood pressure as well as improving lipid metabolism, in moderate doses (Field et al., 2009; Menaa et al., 2013; Da Silva et al., 2015). It is important to note the differences between industrial trans FAs, which should be avoided, and ruminant trans FAs, which confer some health benefits, since the two are structurally similar but have different effects. Despite this distinction, the health benefits of ruminal trans FAs still require investigation. Recent studies exploring ruminal trans FAs found potential negative health effects including increasing cardiometabolic risk factors such as the lipid profile similarly to that of industrial trans FAs (Gebauer et al., 2015; Verneque et al., 2020).
Other than FAs, phytochemicals such as phenolic compounds in foods have numerous beneficial health effects (Serra et al., 2021). Phenolic compounds are secondary metabolites derived from plants, and their chemical structure is characterized by having at least one phenolic group. They can be divided into two categories: non-flavonoids, also called phenolic acids, and flavonoids which include flavonols, flavanones, flavones, flavanols, isoflavones, anthocyanidins, and chalcones. Although they are not essential for major biological mechanisms, they do have important ecological functions and possess antioxidant properties (Cianciosi et al., 2018; Pogorzelska-Nowicka et al., 2018). Phenolic compounds stabilize free radicals by giving up one hydrogen from their hydroxyl group; thus, the degree of antioxidant activity of each compound depends on the number of hydroxyl groups (Kumar et al., 2015; Cianciosi et al., 2018). Carotenoids, including β-carotene and lutein, are another class of phytochemicals found in plentiful amounts in plants. These compounds can act as precursors to vitamin A in humans, have antioxidative effects, and reduce the risk of metabolic diseases (Van Vliet et al., 2021b). Because of the potential of phytochemicals to reduce oxidative stress and inflammation, consumers are looking for foods containing these compounds (Provenza et al., 2019).
Fatty Acids and Phytochemicals in Grass-Fed or Grass-Finished Beef
Fatty acid profiles in meat vary from species to species and from animal to animal. Poultry is usually leaner and therefore contains less fat, while red meat usually contains more fat (Biesalski, 2005). Because cattle diet has the biggest impact on the nutritional profile of beef, the FA profile differs based on the production system (Berthelot and Gruffat, 2018; Lenighan et al., 2019; Prache et al., 2020). Regardless of feeding regime, SFAs are abundant in beef, with stearic acid accounting for approximately one-third of total SFAs. Previous studies mainly agree that grass-feeding or finishing results in higher levels of SFAs (around 45% total FA) compared to grain-finishing (43%) (Duckett et al., 2009; Daley et al., 2010; Van Elswyk and Mcneill, 2014). Nevertheless, it is important to note that grass-finished beef products are leaner than grain-finished products (Alfaia et al., 2009). Grass-finished beef has 1.4 g less SFAs than grain-finished beef per 100 g (Van Elswyk and Mcneill, 2014). Furthermore, grass-finished beef contains around 3% more stearic acid (C18:0) compared to grain-finished beef; stearic acid is considered neutral in regard to effects on plasma LDL cholesterol (Leheska et al., 2008; Alfaia et al., 2009; Daley et al., 2010; Van Elswyk and Mcneill, 2014). Concentrations of individual SFA were reported in the literature; unfortunately, not all articles report values using the same units, so it is difficult to compare them directly. Many sources report higher concentrations of myristic acid (C14:0) and palmitic acid (C16:0), considered to be detrimental to serum cholesterol levels, in grain-finished beef (Duckett et al., 2009, 2013; Daley et al., 2010; Van Elswyk and Mcneill, 2014). Overall, grass-finished beef has a more favorable SFA profile (Daley et al., 2010).
Monounsaturated fatty acids make up nearly half of beef fat, with oleic acid (cis C18:1 n-9) being the most abundant (Leheska et al., 2008). Oleic acid is the most prevalent cis MUFA in the human diet, and it is widely available in plant and animal products. Its effects on human health include lower LDL cholesterol levels and blood pressure, as well as improved insulin sensitivity. These effects are improved when oleic acid is used as a replacement of SFAs (Calder, 2015). It has been reported that GFB has between 30 and 70% less MUFAs compared to grain-finished beef. More specifically, grain-finished beef has up to 1.8 g more MUFAs per 100 g tissue (2.61 vs. 0.79 g per 100 g meat) (Duckett et al., 2013; Van Elswyk and Mcneill, 2014). These findings are interesting from a human-health standpoint since consumption of high-oleic acid beef was linked to increased plasma high-density lipoprotein (HDL) cholesterol (Gilmore et al., 2011; Van Elswyk and Mcneill, 2014).
The key FAs of interest in GFB are the PUFAs, especially n−3 and n−6 PUFAs. Significant differences in n−6 concentrations have been reported in the literature with grass-finished beef containing less n−6 PUFAs compared to grain-finished beef (Davis et al., 2022; Klopatek et al., 2022). Typically, grass-raised products have higher levels of n−3 PUFAs, leading to a more favorable n−6:n−3 ratio. The n−6:n−3 ratio in GFB is around 1.53:1 while the ratio in grain-fed beef is about 7.65:1 (Daley et al., 2010; Pighin et al., 2016). It has been reported that when the amount of grain in the feed is increased, the concentration of n−3 PUFAs decreases and the concentration of n−6 PUFAs increases. The length of time on feed also influences the PUFA content of meat. Klopatek et al. (2022) found that cattle grazing for 20 months and finished for 45 days on a high concentrate diet displayed a n−6:n−3 ratio of 2.5:1 compared to animals kept on pasture for 20 or 25 months without any concentrate displaying a n−6:n−3 ratio of 1.5:1. This was mainly due to a decrease in n−3 PUFA concentrations and it was confirmed by a n−6:n−3 ratio of 5.5:1 in animals that were fed a concentrate diet for 128 days in a feedlot. Analyzing the effects of various feedstuff on the n−6:n−3 ratio in GFB will help consumers to understand these vast differences and select the healthiest GFB products. Beef from cattle fed diets rich in grass and other forages also have about 2–3 times higher concentrations of c9 t11 CLA and TVA than grain-fed cattle (Leheska et al., 2008; Alfaia et al., 2009). This is mainly due to a more favorable rumen pH which allows for more efficient microbial biohydrogenation (French et al., 2000; Kraft et al., 2008).
Phytochemicals are also variables of interest that differ based on the production system. Grass-finished beef contains higher amounts of common antioxidants including 3 times more α-tocopherol (vitamin E) and 1.5 to 10 times more β-carotene than grain-finished beef (Duckett et al., 2009; Pighin et al., 2016; Bronkema et al., 2019; Logan et al., 2020). Although intrinsic biological factors such as breed and age can affect carcass fat color, grass-fed beef usually has a yellower fat, mainly due to carotenoids found in the lush green forages they are grazing on (Dunne et al., 2009). Yellow carcass fat is generally related to healthier FA profiles and higher antioxidant content (Daley et al., 2010). Even though direct comparison of phenolic compounds in grass-finished and grain-finished beef has not yet been reported in the literature, differences in phenolics were observed in milk based on grass or concentrate diets (Besle et al., 2010; Prache et al., 2020). Furthermore, some findings suggest that cattle finished on forages might showcase higher phenolic content and diversity in their meat (Provenza et al., 2019; Van Vliet et al., 2021a,b). When cattle graze on phytochemically diverse mixture of plants, the sensory and biochemical characteristics of their carcasses are modified (Alothman et al., 2019; Provenza et al., 2019; Van Vliet et al., 2021b). For instance, a study comparing inflammatory responses of subjects after consuming kangaroo meat (eating a mixture of phytochemically diverse plants) or beef meat (fed a high-grain diet) showed that people who consumed the kangaroo meat had lower inflammatory responses (Arya et al., 2010). However, it is important to note that the generic term “grass-fed” or “grass-finished” does not reflect phytochemical diversity of the feed. There are many variations that exist among grass-fed and grass-finished diets, and these differences can greatly influence the nutritional properties of beef (Figure 1).
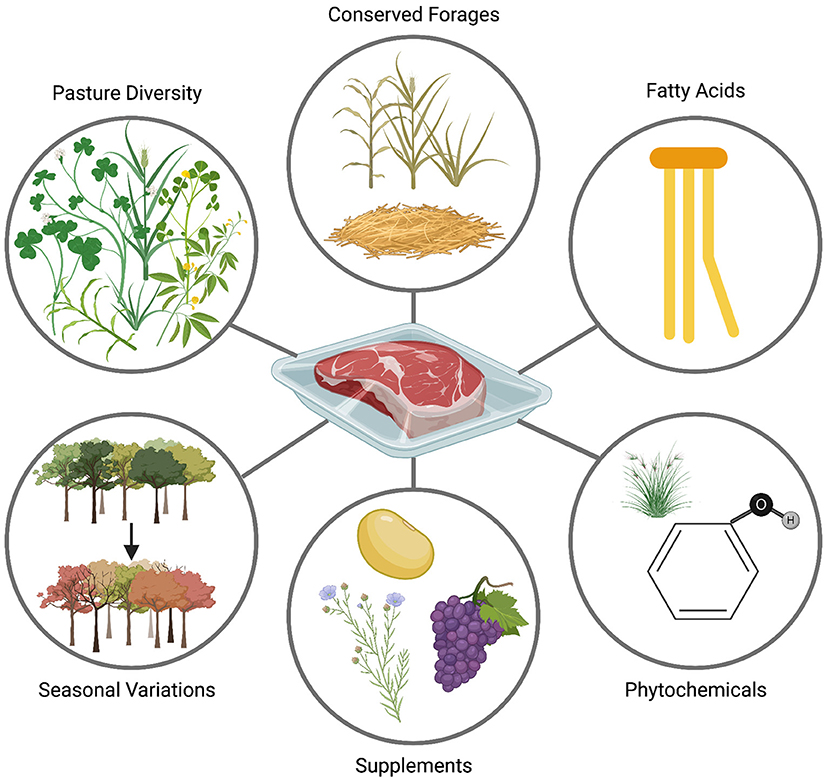
Figure 1. The effects of pasture diversity, seasonal variations, feed conservation, and supplementation on phytochemicals and fatty acids of beef. Cattle finished in different seasons exhibit different fatty acid profiles and phytochemical content. Supplementing cattle diets with flaxseed, algae, or other conserved forages can also affect the nutritional quality of beef. Created with BioRender.com.
How Variations In Cattle Diet Influence Meat Nutritional Quality
Regenerative Agriculture and Pasture Diversity
Increasing demand for GFB and the use of regenerative agriculture practices go hand in hand (Spratt et al., 2021; Van Vliet et al., 2021b). Regenerative agriculture can be defined as a practice that links soil health and livestock management to farm profitability, human, animal, and ecosystem health, as well as food system sustainability (Spratt et al., 2021). Regenerative agriculture might also be referred to as ecological agriculture, conservation agriculture, permaculture, or holistic management and focuses on restoring holistic and regenerative systems supported by ecosystems that allow healthy soils. Farmers and ranchers with livestock typically use a holistic grazing method with the purpose of increasing soil health, moisture retention, and fertility while continuously moving animals between habitats to allow optimal forage conditions (Gosnell et al., 2019). More specifically, regenerative grazing involves rest-rotation cycles: grazing periods followed by forage rest periods to allow plant recovery (Spratt et al., 2021). Regenerative agriculture is known to improve biodiversity and to enhance ecological function (Provenza et al., 2019). Grazing systems used in regenerative agriculture imitate natural ecosystems and improve plant diversity (Van Vliet et al., 2021b). When compared to feedlots or monocultures, soil, animal, and human health are favored when herbivores, including cattle, graze on phytochemically diverse mixtures of grasses and trees (Provenza et al., 2019). However, diverse plant species and grazing systems have varying effects on the nutritional profile of beef (Table 1).
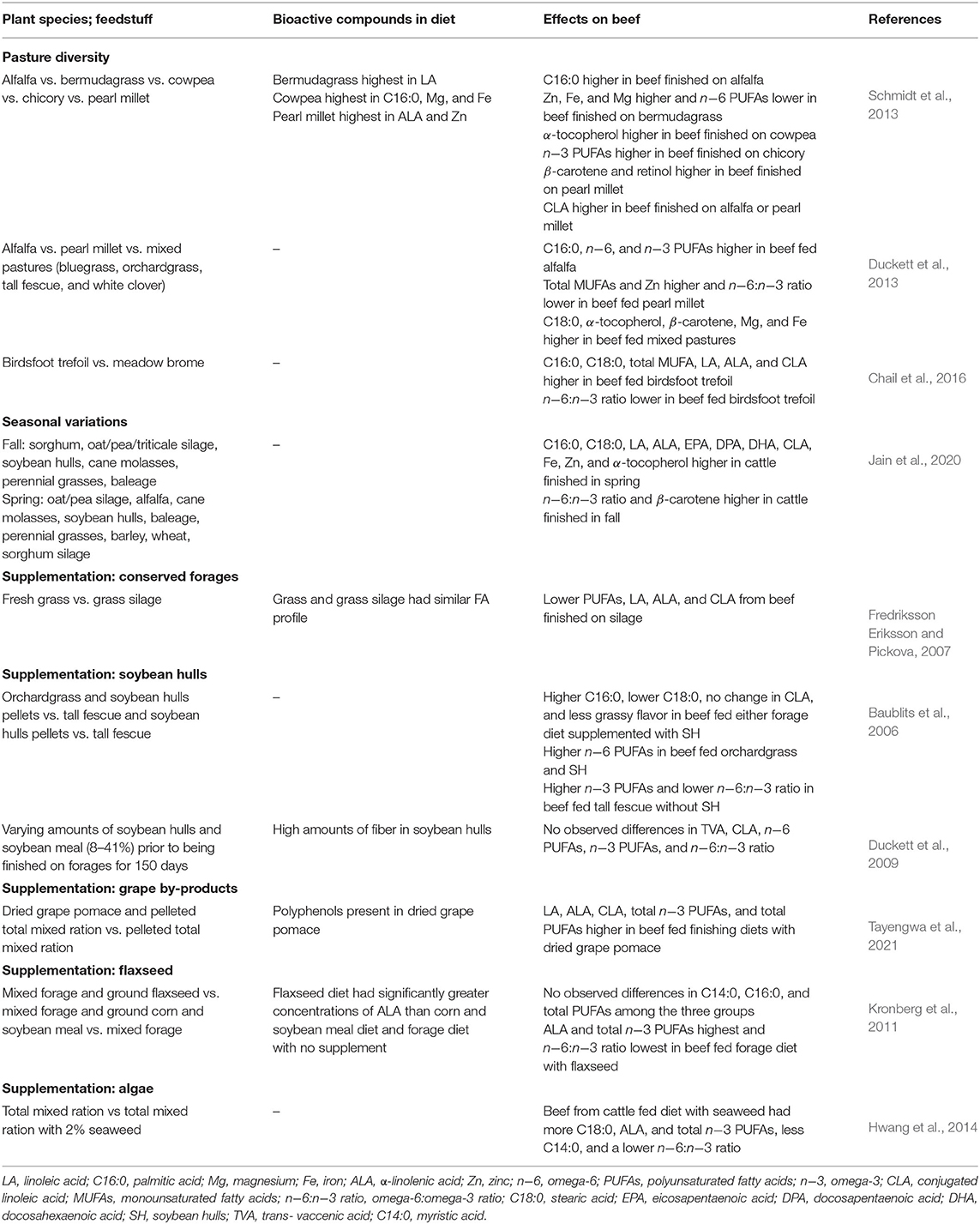
Table 1. Effects of various feedstuff and their bioactive compounds on the nutritional profile of beef.
The “grass-fed” label does not always reflect the phytochemical diversity of the diet (Provenza et al., 2019). Large variations are observed among grass-finished beef (Bronkema et al., 2019). Not all GFB graze on the same type of pastures. Forages including grass and clover contain high concentrations of ALA (50–75% of total FAs), which is the essential FA that can be synthesized into beneficial EPA and DHA (Scollan et al., 2014; Bronkema et al., 2019). Chloroplasts contain high levels of PUFAs, explaining why green plants have high concentrations of ALA (Elgersma et al., 2013). Orchard grass, tall fescue, and perennial ryegrass have 2, 4, and 7 more mg of ALA per g of dry matter compared to alfalfa, respectively (Dierking et al., 2010). Schmidt et al. (2013) reported concentrations of main FAs in alfalfa, bermudagrass, chicory, pearl millet, and cowpea. Bermudagrass contained 7% more ALA than cowpea and pearl millet, while pearl millet and chicory contained 8% more LA than alfalfa and bermudagrass. Other forage mixtures including pearl millet, bluegrass, and clovers increased the n−3 content of beef by more than 2% compared to beef fed a concentrate diet (Duckett et al., 2013). Adding different varieties of plants like red clover can help increase levels of ALA and LA (Scollan et al., 2006). Nutritional profiles of plants differ based on the leaf-to-stem ratio, with leaves containing more n−3 PUFAs than stems as Elgersma et al. (2005) found a positive relation between proportion of leaf blades and C18:3. In general, an increase in n−3 PUFAs is observed in diverse pastures compared to perennial ryegrass and lowland pastures.
There is an increasing interest in botanically diverse pastures as cattle feed. However, the information available in the literature remains scarce (Scollan et al., 2014). Different plant species have varying effects on the nutritional quality of beef products. The subcutaneous fat n−6:n−3 ratio of cattle grazing on cicer milkvetch was greater compared to cattle grazing on meadow bromegrass or treated in feedlot. The lower subcutaneous fat n−6:n−3 ratio was found in animals grazing on birdsfoot trefoil (Allen, 2021). It is important to note that unsaturated FAs are toxic to rumen bacteria and therefore undergo extensive rumen biohydrogenation. LA and ALA are hydrogenated to the extent of 70–95 and 85–100%, respectively (Lock et al., 2006). Based on this, increasing PUFA concentrations in the diet could lead to more biohydrogenation and formation of stearic acid, but these rates also depend on other factors such as rumen pH, plant secondary metabolites, and the impact of plant cell walls on the availability of free FAs for biohydrogenation (Lock et al., 2006; Fredriksson Eriksson and Pickova, 2007; Jenkins et al., 2008; Lee et al., 2018). These findings emphasize the importance of defining plant species in pasture and how they affect rumen biohydrogenation and beef nutrient profiles.
Herbivores and plants work synergistically, leading plants to produce a wide array of phytochemicals. These phytochemicals accumulate in meat and milk when animals graze on these diverse pastures, but these metabolites remain underdiscussed when assessing the nutritional quality of meat (Van Vliet et al., 2021b). Plant diversity and grazing are important elements of regenerative agriculture; they play major roles in soil and environmental health, as well as contributing health-enhancing phytonutrients for animals and humans (Provenza et al., 2015). However, factors other than pasture diversity contribute to the nutritional properties of cattle feeds.
Seasonal Variations
The diversity of production systems reflects differences in nutritional profiles of beef (Table 1). Significant nutritional differences are observed among grass-finished beef. However, there are more factors affecting the quality of beef including season, geography, and climate (Mathews and Johnson, 2013; Bronkema et al., 2019; Jain et al., 2020). These variations seen in beef are due to variations in feeds. Factors such as plant maturity and development, cutting date, soil, weather, and light exposure play major roles in the nutritional composition of feeds (Dewhurst et al., 2001; Khan et al., 2009; Garcia et al., 2016).
Generally, grasses decline in FA quality faster than legumes or grains, highlighting the importance of seasonal variations (Kilcher, 1981; Glasser et al., 2013). Nutritional quality of feeds varies with plant growth and maturity, as well as the leaf-to-stem ratio (Boufaïed et al., 2003; Glasser et al., 2013). Different growth periods have been identified in fresh grass between May and September in temperate Northern Hemisphere areas. These growth periods are further subdivided into the primary growth, and the first, second, and third regrowth. Based on these cycles, it was found that total FAs and ALA are higher during the primary growth before strongly declining during the second regrowth, which is a stemmy regrowth period, and increasing again during the last regrowth cycle, which is a leafy regrowth period. The opposite trend was true for LA (Bauchart et al., 1984). These growth and regrowth periods emphasize the importance of plant growth and the leaf-to-stem ratio when assessing the nutritional quality of forages since forage lipids are mainly of leaf origin (Boufaïed et al., 2003). Total fat in grasses is usually higher in early spring before gradually declining, while concentrations of the SFA palmitic acid gradually increase throughout the season (Mir et al., 2006). Concentrations of LA, as well as MUFAs such as C16:1 and C18:1 usually follow the same pattern as palmitic acid, while the beneficial n−3 PUFA ALA decreases over time (Garcia et al., 2016). Following the gradual decrease of ALA, increasing concentrations are seen in the late season because of regrowth vegetation cycles (Glasser et al., 2013). Throughout the season, forages have a more beneficial FA profile compared to grains since seeds are higher in n−6 PUFAs while leaves are higher in n−3 PUFAs (Butler, 2014). Forages are also the largest natural source of vitamins for ruminants, but concentrations vary based on species and maturity. A study comparing α-tocopherol and β-carotene in grasses and legumes found that the highest levels of vitamins were found in the fall and were based on regrowth cycles (Danielsson et al., 2008).
Temperature and weather affect the quality of forages. Higher temperatures during the summer months negatively affect the quality of feeds by increasing plant maturation and cell wall lignification (Revello-Chion et al., 2011). Regarding precipitation, rainfall promotes grass quality and productivity (Mir et al., 2006; Revello-Chion et al., 2011). On the other hand, water deficit decreases forage quality by reducing the proportion of leaves. This is because nutrients migrate to the roots, decreasing the important leaf-to-stem ratio (Revello-Chion et al., 2011). Furthermore, precipitation directly affects the FA biosynthesis in forages; lipid biosynthesis is decreased or even inhibited under water stress (Gigon et al., 2004).
Seasonal differences in feeds ultimately affect the nutritional composition of beef. Jain et al. (2020) reported that cattle finished in the spring exhibit higher levels of n−3 (including ALA, EPA, DPA, DHA) and n−6 PUFAs, stearic acid, and oleic acid. The n−6:n−3 ratio is also significantly lower in the spring compared to the fall. The higher levels of n−3 PUFAs in the spring are most likely due to higher n−3 levels in spring forages but also higher levels of antioxidants protecting n−3 PUFAs from oxidation and biohydrogenation. Sodium, phosphorus, and β-carotene were reported to be significantly higher in the fall, while magnesium, potassium, iron, zinc, selenium, and α-tocopherol were higher in the spring. Even if feed composition is usually self-reported by producers, it has been found that mineral levels in forages are higher in the spring rather than summer and fall (Jain et al., 2020).
Fatty acid content in beef is also affected by the region and the climate. For instance, Southern regions experience hot weather most of the year with some short periods of weather changes, while regions of the Midwest for example experience drastic weather changes based on seasons that last a few months each. Heat stress might be linked to differences in FA profiles (Steiner et al., 2015; Jain et al., 2020).
Seasonal variations are important to consider in the production of GFB. A well-managed grazing system taking into consideration feed nutritional differences based on season and weather conditions is crucial to determine optimal finishing phases to yield the healthiest nutrient profile in beef.
Supplementation: Effects of Different Supplementary Feeds in U.S. GFB
Providing only fresh forage to grass-fed cattle can become difficult for producers, especially since fresh grass is not always readily available in some regions and seasons. During the winter for instance, producers rely on hay or haylage, as well as non-starchy feeds like alfalfa rations, wheat, or oat straws (Gwin, 2009). According to the USDA, “hay, haylage, baleage, silage, crop residue without grain, and other roughage sources” may be added to grass-fed cattle diets, but these feeds are nutritionally different from fresh forage (Food Safety Inspection Service, 2019). Further, in some cases, additional supplementary feeds such as soybean hulls (SH) or grapeseed extracts may be added to forage-fed beef to improve beef quality and utilize byproducts of other industries (Kiesling, 2013; Muñoz-González et al., 2019). It is important to note that the addition of feed supplements described in this section varies by labeling organizations and does not necessarily reflect what is permitted in “grass-fed” labels outside of the U.S. such as certification by A Greener World in the United Kingdom or by the Pasture-fed Cattle Assurance System in Australia (A Greener World; PCAS). Thus, grass-fed cattle diets in the U.S. can be composed of an array of feeds which may lead to differences in nutritional profiles, particularly FA and phytochemical content, of GFB (Table 1).
Conserved Forages
Grasses and legumes can be conserved by drying or fermentation. Hay is prepared by cutting and quickly drying grasses or legumes until they reach <20% moisture (Allen et al., 2011). Forages are spread into a field and raked until dry before storing (Tripathi et al., 1995). Silage, haylage, and baleage are preserved by fermentation in an oxygen-free environment in which bacteria convert sugar from forages into organic acids such as lactic acid; this lowers the pH and prevents spoilage (Tripathi et al., 1995). Silage refers to forages that are fermented at a high-moisture content (roughly >50% moisture) in an air-tight environment such as a silo. Haylage refers to a low-moisture silage (roughly 35–55% moisture) that is made after forages are cut and wilted (Allen et al., 2011; U.S. Department of Agriculture, 2021). Lastly, baleage, or round bale silage, refers to forage that is cut, wilted, and fermented in tightly wrapped bales (American Grassfed Association, 2020). These preservation methods have implications for the nutritional quality of the feed and thus may impact ruminant products.
Conserved forages often have reduced nutritional quality compared to fresh forages. Drying or fermenting forages decreases antioxidant and phenolic concentrations (Owens et al., 1997; Butler, 2014). In the process of making hay or silage, 80% of the carotenoid content is lost (Pickworth et al., 2012). Further, the wilting of forages for drying or ensiling results in oxidation of PUFAs, particularly ALA. In this process, lipolysis is catalyzed by plant lipases, releasing PUFAs from plant membranes. These free PUFAs are then oxidized with exposure to air by lipoxygenases, and some products of this process may be lost as components of volatile organic compounds, thus reducing PUFA content of plant tissues (Kalač and Samková, 2010). This loss of PUFAs is often accompanied by an increase in the relative amount of palmitic acid, given that SFAs are less susceptible to oxidation (Van Ranst et al., 2009; Kalač and Samková, 2010). Fresh grass contains higher concentrations of ALA, LA, and oleic acid compared to hay (Daley et al., 2010; Butler, 2014; Jain et al., 2020). Moreover, in a review of fresh and conserved forages, fresh perennial ryegrass contained 71.8% ALA, 8.8% LA, and 11.4% palmitic acid compared to perennial ryegrass hay with 55.9% ALA, 14.0% LA, and 15.8% palmitic acid, and perennial ryegrass silage with 52.2% ALA, 13.4% LA, and 21.2% palmitic acid (Kalač and Samková, 2010). Changes in FA profiles of feeds can alter FA metabolism in the rumen and therefore the FA content of beef products (Buccioni et al., 2012; Glasser et al., 2013).
Regardless, the magnitude of the change in the nutritional quality of forages is dependent on the method and quality of preservation (Glasser et al., 2013). Tripathi et al. (1995) noted that the process of haymaking is particularly susceptible to shattering and dropping of leaves, the most nutritious part of the plant. In comparison, silage, haylage, and baleage making are much less susceptible to leaf loss (Tripathi et al., 1995). Further, compared to ensiled forages, haymaking is more susceptible to nutrient loss due to sunlight and inclement weather (Tripathi et al., 1995; Coblentz and Akins, 2018). A meta-analysis of reported FA profiles of forages assessed the relationship between preservation methods and changes in the FA profile (Glasser et al., 2013). Turning fresh forage into hay did not impact the LA content, but it caused a decrease in total fat, total FAs, and ALA. At most, ALA decreased by 17%, and it was observed that this decrease was greater under poor haymaking conditions. Haymaking, especially under poor conditions such as wet weather, was the second most deleterious factor affecting the ALA content of forages following the cutting date when compared to other preservation, vegetation stage, and fertilization factors (Glasser et al., 2013).
Ensiled forages have many advantages compared to dry hay. In general, ensiling does not greatly impact the FA profile, but instead the extensive lipolysis involved in ensiling leads to an increase in the free FA content (Kalač and Samková, 2010; Glasser et al., 2013). This increase in free FA content may impact biohydrogenation in the rumen of cattle given that lipolysis must occur prior to biohydrogenation (Van Ranst et al., 2009). The impact of turning fresh forages and legumes into silage differs among unwilted silages, wilted silages, and haylages. In a meta-analysis of reported forage FA profiles, total fat content was increased in unwilted and wilted silages, while total FAs were only increased in unwilted silages. Wilted silages and haylages had 5% lower ALA content, while ensiling without wilting did not impact the ALA content compared to their fresh counterparts (Glasser et al., 2013). Though ensiling forages protects FAs from oxidation, aeration of ensiled forages prior to feeding exposes the free FAs to oxygen, inducing oxidation (Kalač, 2011). Exposing grass silages to air for 24 h lowered the PUFA and total FA content and increased the proportion of palmitic acid (Khan et al., 2009). However, the oxidation of FAs is generally still greater in hay which has a longer exposure to air (Kalač, 2011). These results indicate that ensiled forages, compared to hay, may be a more desirable supplementary feed for grass-fed production systems.
However, it is important to note that the composition of the feed itself may influence beef nutrient profile to a greater extent than the feed's preservation method. Butler (2014) highlighted the importance of feed composition by noting differences in beef nutrient profile among types of silages provided: grass silages led to enhanced beef CLA content, while clover and legume silages led to enhanced n−3 content. Maize silages, not permitted in GFB, led to increased beef n−6 content (Butler, 2014). On the other hand, Glasser et al. (2013) found that forage vegetation stage and conservation method had a greater impact on nutrient profile compared to differences among forage species. Some studies reviewed by Glasser et al. (2013) noted an increase in n−3 content with a greater proportion of grasses and a decrease in n−3 content with a greater proportion of legumes, but these differences were not as large as those observed due to preservation method (Lourenço et al., 2007b; Steinshamn and Thuen, 2008). Further, while grass species can influence the FA content of grass silages, plant maturity at harvest caused the most variation, predominantly in n−3 content (Khan et al., 2012). Based on this, there are important differences in feed composition, plant maturity, and preservation method to take note of when considering incorporating conserved forages into GFB systems.
There is limited evidence demonstrating how feeding conserved forages impacts the nutritional quality of GFB. A review of studies comparing various fresh pasture and silage diets concluded that the FA profile of beef finished on fresh grass was more favorable, including greater n−3 PUFAs and CLA, compared to beef finished on grass silage; however, many of the studies included in this review compared diets containing both forages and concentrates (Kalač, 2011). A study conducted by Fredriksson Eriksson and Pickova (2007) compared the FA and α-tocopherol content of exclusively grass-fed cattle finished on fresh grass in September compared to exclusively grass-fed cattle finished on grass silage in February. Though they found that the grass and grass silage diets had a similar FA profile, beef finished on silage had lower PUFA and significantly lower LA, ALA, and CLA. The authors suggested that the higher PUFA content in beef from the fresh grass group may be because the cell wall limits the biohydrogenation of FAs in fresh grass as compared to the free FAs in grass silage. Further, the authors suggested that the higher plant secondary metabolite content in fresh grass compared to grass silage in the alpine region included in the study may limit biohydrogenation (Fredriksson Eriksson and Pickova, 2007). Similarly, red clover silages are found to increase PUFA content in meat compared to grass silages (Lourenço et al., 2007a; Lee et al., 2009; Van Ranst et al., 2009). Red clover silages reduce lipolysis leading to less free FAs available for biohydrogenation due to lipase-inhibiting compounds like polyphenol oxidase (PPO), though evidence suggests the reduction in lipolysis and PUFA biohydrogenation may occur independently of PPO activity (Van Ranst et al., 2009; Lee et al., 2018). More research comparing finishing cattle on fresh grass compared to conserved forages or fresh grass diets supplemented with conserved forage is needed to better understand how conserved forages alter the nutritional profile of beef.
Soybean Hulls
Soybean hulls are another supplement to GFB used by some U.S. producers during the finishing phase (Bronkema et al., 2019). Soybean hulls refer to the seed coats of soybeans that are removed in the process of soybean crushing (Poore et al., 2002). Soybean hulls are mostly composed of fiber with low amounts of lignin and are known to have high potential digestibility for ruminants without lowering ruminal pH (Poore et al., 2002; Pugh, 2003). There are mixed results in the current literature about the effects of SH supplementation on the nutritional profile of GFB. In one study, there were no observed differences in CLA, TVA, n−3 PUFAs, n−6 PUFAs, and the n−6:n−3 ratio among cattle fed varying amounts of SH prior to forage finishing for 150 days (Duckett et al., 2009; Bronkema et al., 2019). However, in another study, cattle fed fescue or orchardgrass supplemented with SH had greater total fat, lower n−3 PUFAs, and a greater n−6:n−3 ratio compared to cattle fed only fescue. It is important to note that the n−6:n−3 ratio was still below four, CLA did not decrease, and the intensity of grassy flavor decreased in the beef supplemented with SH (Baublits et al., 2006). According to sensory studies conducted in Chicago and San Francisco, only about 23% of consumers preferred the taste of GFB as opposed to grain-fed beef (Gwin, 2009). Thus, a reduction in the intensity of grassy flavor by SH supplementation may increase palatability of GFB to consumers. In another study, CLA concentrations and n−3 PUFAs were greater and the n−6:n−3 ratio was lower in cattle fed a SH supplement compared to cattle fed a corn supplement (Kiesling, 2013). CLA and n−3 PUFAs were also increased and the n−6:n−3 ratio decreased in lambs when SH were included as a replacement for corn (Costa et al., 2012). There was not a comparison group to cattle or lambs fed purely forages in the aforementioned studies, but the results indicate that SH could be a better supplement than corn. It is hypothesized that since SH contain significant amounts of fiber and maintain ruminal pH at optimal levels, more biohydrogenation can occur, leading to greater amounts of CLA and TVA (Kiesling, 2013). Further studies need to be done to clearly elucidate the effects of SH supplementation and the mechanisms for these effects. However, SH are not permitted by some organizations providing grass-fed labels, including the American Grassfed Association, so producers need to keep this in mind when considering supplements for grass-fed cattle (American Grassfed Association, 2020).
Grape Pomace and Grape Seed Extract
Increased levels of UFAs found in GFB might render meat more subject to biohydrogenation and oxidation. To avoid this, cattle feeds can be supplemented with waste or byproducts from the food industry that possess antioxidative capabilities. The winemaking industry, for example, generates large amounts of waste and byproducts including grape pomace and grape seed extracts (Brenes et al., 2008). The valorization of these byproducts would reduce the environmental impact of winemaking and would add functional nutrients to meat (Muñoz-González et al., 2019). These byproducts contain significant amounts of bioactive compounds such as antioxidants, phenolic compounds, and fiber. Grape pomace and grape seed extracts contain high levels of polyphenols including anthocyanins, proanthocyanins, and flavanols (Brenes et al., 2008; Arola-Arnal et al., 2013; Muñoz-González et al., 2019). Adding these functional ingredients to feeds instead of adding them during the processing stages allows these compounds to remain bioavailable and to be metabolized by the animal (Antonini et al., 2020). Natural antioxidants like grape pomace or grape seed extracts can exhibit better antioxidative properties than conventional antioxidants like butylated hydroxyanisole (BHA) or butylated hydroxytoluene (BHT) (Kumar et al., 2015). Thus, adding grape pomace or grape seed extracts to cattle feeds could be beneficial.
In rats, grape seed extract feeding led to a dose-dependent increase in muscle polyphenol content (Serra et al., 2013). In addition, rats fed grape seed polyphenols had significantly greater adipose tissue accumulation of flavanols and their metabolites (Margalef et al., 2015). When these byproducts were added to monogastric animal feeds, the meat had higher levels of α-tocopherol, PUFAs, and less lipid peroxidation (Muñoz-González et al., 2019). A study on the effects of grape pomace concentrate in chickens concluded that the polyphenols found in grape pomace concentrate were absorbed in high enough amounts to modulate antioxidant activity in chicken muscle tissue (Brenes et al., 2008). Other studies evaluating the effects of grape seed extracts in birds suggested that grape polyphenols and their metabolites might be absorbed and remain in active tissues (Muñoz-González et al., 2019). These findings suggest that supplementing cattle feed with grape pomace or grape seed extracts could help improve the shelf-life of beef products and help to maintain higher levels of PUFAs in beef (Serra et al., 2013).
Several studies have investigated the effect of adding grape seed extract to ground beef on lipid oxidation. Oxidative stability is commonly measured by the thiobarbituric acid-reactive substances (TBARS) value. In one study, beef patties supplemented with grape seed extract had mean TBARS values of approximately 0.59 MDA/kg compared to 2.94 MDA/kg for beef patties without grape seed extract. The upper limit of rancidity acceptable to consumers is around 2 MDA/kg (Gomez et al., 2014). Adding grape seed extract to the meat also kept TBARS values relatively steady. Beef samples without grape seed extract had increasing levels of TBARS over time, from 0.57 mg MDA/kg to about 3.24 mg MDA/kg. However, beef samples with grape seed extract stayed relatively constant around 0.53 MDA/kg (Gomez et al., 2015). It is important to note that in these studies, grape seed extract was added directly to ground beef samples.
The effect of grape pomace or grape seed extract on the FA profile of beef is not well-known. One recent study observed that adding dried grape pomace to the finishing diet of beef cattle significantly increased CLA, n−3 PUFAs, and total PUFAs compared to the control. Supplementing dried grape pomace also decreased aldehydes, ketones, and alcohols in beef as well without significant changes in sensory properties albeit a modest reduction in tenderness compared to controls (Tayengwa et al., 2021). These results are promising but limited. Future research on the effects of grape pomace and grape seed extract on the nutritional profile and sensory attributes of beef is needed.
Similar to grape seed extract, cherry has also been investigated for its impact on lipid stability in beef. Britt et al. (1998) found that, like grape seed extract, adding cherry to ground beef patties decreased rates of oxidation and kept TBARS values under the upper limit of rancidity (Britt et al., 1998). Since adding these products to beef directly produced positive results, future studies should investigate the effects of adding grape seed extract or cherry tissue to cattle feed on the lipid stability of the beef produced.
Flaxseed
Flaxseed is another supplement used by some U.S. GFB producers. Flaxseed oil is a significant source of ALA (45–52% of total FAs) and antioxidants including α-tocopherol and phenolic compounds (Pouzo et al., 2016). Because of these natural properties, flaxseed supplementation is a potential way to increase concentrations of n−3 PUFAs and improve the oxidative stability of beef. There have been several studies investigating the effects of flaxseed supplementation on the FA profile of beef fed fresh forages, conserved forages, and concentrate. Mapiye et al. (2013) found that beef from cattle fed red clover silage with flaxseed had about double the proportions of ALA (1.59 vs. 0.68%) and total n−3 PUFAs (2.04 vs. 1.10%), and about 5 times more TVA (6.37 vs. 1.11%) in intramuscular fat compared to beef from cattle that were fed the control diet without flaxseed. Beef from cattle fed the flaxseed diet also contained less myristic acid and palmitic acid (Mapiye et al., 2013). Another study also found that beef from cattle fed grass hay or barley silage supplemented with flaxseed had greater ALA, total n−3 PUFAs, and TVA as well as less palmitic acid than cattle fed just grass hay or barley silage (Nassu et al., 2011). Kronberg et al. (2011) reported that beef from cattle fed forage diets with flaxseed had a significantly lower n−6:n−3 ratio compared to beef from cattle fed forage diets with corn and soybean meal and beef from cattle fed forage diets with no supplements (2.34:1 vs. 3.63:1 vs. 3.41:1). However, they did not observe differences in myristic acid and palmitic acid (Kronberg et al., 2011). While there are variations in the extent of differences, especially regarding SFAs, it is widely agreed that flaxseed supplementation increases ALA concentrations and total n−3 PUFAs in beef.
Regarding oxidative stability, a study conducted by Pouzo et al. (2016) found that adding low amounts of flaxseed to pasture diets improved lipid stability of beef. Interestingly, adding high amounts of flaxseed had deleterious effects on lipid stability. It is hypothesized that the low amount of flaxseed provided enough antioxidants to offset the increase in lipid peroxidation caused by elevated n−3 PUFA levels, leading to greater oxidative stability (Pouzo et al., 2016). This is an avenue that has not been extensively studied, so further research is needed to better understand the effects of varying amounts of flaxseed supplementation on the oxidative stability of beef.
Algae
Consumption of fish high in long-chain n−3 PUFAs is low in the American diet. Therefore, there has been an interest in supplementing cattle with marine ingredients such as algae to increase the n−3 content of beef (Glover et al., 2012; Morais et al., 2020). Seaweed, a macroalgae, are a supplement of interest because of their high concentrations of phenolic compounds, pigments, carotenoids, PUFAs, and minerals such as calcium, potassium, and iodine (Schmid et al., 2018; Morais et al., 2020). Algae can synthesize ALA and LA as well as the long-chain n−3 PUFAs, EPA and DHA, and generally have an n−6:n−3 ratio around 1:1 (Schmid et al., 2018). Seaweed are fast growing, have a high biomass yield, and do not compete with other crops for arable land or fresh water. However, there is wide variation in nutritional composition among different seaweeds, and they are susceptible to heavy metal bioaccumulation (Morais et al., 2020). Despite this, seaweed has beneficial effects when it is added to cattle feed.
For instance, feeding seaweed to cattle may address the challenge of increasing the n−3 content of beef caused by biohydrogenation (Stamey et al., 2012). Generally, 85–100% of ALA is hydrogenated in the rumen if unprotected (Glover et al., 2012). While there is a lack of evidence demonstrating whether seaweed supplementation improves the FA profile of GFB, it was found that grain-fed cattle supplemented with seaweed produced beef with more ALA, total n−3 PUFAs, and stearic acid, less myristic acid, and a lower n−6:n−3 ratio compared to the control diet (Hwang et al., 2014). Smith (2017) demonstrated that supplementing grass-fed cattle with algae resulted in higher n−3 PUFA concentrations compared to grain-fed cattle supplemented with algae. Further, animals fed only grass can consume more algae, resulting in an increased intake of n−3 PUFAs, and meat with more EPA and DHA per serving (Smith, 2017). It is important to note that the efficacy of feeding marine ingredients high in n−3 PUFAs depends on the strength of the algal cell wall and the acidity of the rumen. A lower ruminal pH results in greater breakdown of algal cell walls and thus greater loss of n−3 PUFAs to biohydrogenation (Smith, 2017). Due to their high antioxidant content, seaweed may act to prevent oxidation in beef products, similar to grape byproducts and flaxseed (Morais et al., 2020). Overall, there is limited evidence demonstrating the efficacy of seaweed as a cattle feed supplement including its impact on the nutritional composition of GFB (Morais et al., 2020; Costa et al., 2021). Additional research should focus on the potential of these marine organisms as grass-fed cattle feed supplements.
Challenges
While GFB products have many advantages, there are some challenges to consider. GFB is usually produced on a much smaller scale than conventional products. These products are mostly sold in local farms and farmers markets, which makes it harder for producers to reach customers despite growing purchasing interest (Gwin, 2009; Mathews and Johnson, 2013). This limitation partially explains why conventional production systems are used on a larger scale. Production systems based on forage diets take longer to finish cattle than conventional systems due to a less energy-concentrated diet (Gwin, 2009; Mathews and Johnson, 2013; Hayek and Garrett, 2018). Finding efficient genotypes for grass-finishing is another challenge that producers need to consider. Doyle et al. (2021) pointed out that early maturing genotypes might be more suitable for grass-finishing due to their higher potential for fat deposition at a younger age whereas late maturing genotypes might be more suitable for a grass and concentrate system. U.S. customers are accustomed to having affordable and year-round-available beef in supermarkets. Grass-fed products are usually more expensive, not widely available in single-serving packs in supermarkets, and not available on a year-round basis (Gwin, 2009; Gwin et al., 2012). Along with convenience and affordability, U.S. customers prefer the tenderness, juiciness, marbling, and milder flavor of conventional beef compared to GFB (Gwin, 2009; Mathews and Johnson, 2013).
Producers who wish to finish their cattle on grass face challenges including having insufficient grass and land to grow pastures (Hayek and Garrett, 2018). Depending on the region, fresh grass may not be available all year long for grazing (Duckett et al., 2009; Jain et al., 2020). Therefore, producers must adapt and find ways to feed their cattle during seasons when fresh pastures are not available while still respecting the labeling definitions for grass-fed or finished beef. For this reason, the supplement options that we mentioned in this review might be helpful to overcome the lack of fresh grass.
Increasing n−3 PUFAs in beef is an important way to improve the nutrient profile to favor human health, but this comes with a set of challenges. Fatty acids are subject to oxidation which limits the shelf-life of meat and can result in undesirable, rancid flavors (Kumar et al., 2015). Increased levels of PUFAs in meat can lead to increased lipid peroxidation if not accompanied by adequate antioxidant content (Pighin et al., 2016; Pogorzelska-Nowicka et al., 2018; Saini and Keum, 2018). Grazing on antioxidant-rich, diverse pastures might provide adequate antioxidant levels (Van Vliet et al., 2021b). Grape seed extract and flaxseed supplementation, both important sources of antioxidants, are promising ways to increase n−3 PUFAs and improve the oxidative stability of GFB, but further research is needed in order to comprehensively evaluate the effects of these supplements.
Recommendations
To produce beef that has the greatest potential to benefit consumer health, nutrition recommendations indicate the importance of increasing n−3 PUFA content, reducing n−6 PUFA content, and increasing CLA content (Woods and Fearon, 2009; Butler, 2014; Vannice and Rasmussen, 2014). Farmers and ranchers need thorough information on feeding practices and awareness of variations based on season and feed ingredients used (Table 1). If permitted by the relevant grass-fed standards, cattle fed a botanically diverse pasture mixture managed in a rotational grazing manner, supplemented with phytochemically-rich ingredients such as grape byproducts, flaxseed, or algae would produce beef products high in health-enhancing nutrients such as phenolic compounds, n−3 PUFAs, and CLA. Season and weather should also be considered to assess plant's growth and re-growth cycles and leaf-to-stem ratios. In temperate climates, finishing cattle in the spring compared to the fall produces beef with higher beneficial bioactive compounds. Feeds are of higher nutritional quality either during the early or late grazing season. Grazing management should be adapted to give pastures adequate recovery, and the symbiotic relationship between ruminants and pastures should be supported. When fresh forages cannot be fed, conserved ingredients with the highest nutritional potential should be used. High quality ensiled forages such as silage or baleage are typically preferred to hay because of reduced leaf loss. While not always permitted in GFB, SH supplementation can decrease the intensity of the “grassy” flavor of GFB while having neutral or positive effect on the nutrient profile. Testing of FA and antioxidant content of feeds is also encouraged to ensure the highest nutritional quality. Early maturing steers might have an advantage over late maturing genotypes due to their potential for greater fat deposition at a younger age which may reduce the finishing period before slaughter (Doyle et al., 2021). These recommendations based on the information provided in this review would lead to healthier beef products not only for human health, but also for soil, animal, and environmental health.
Conclusion
World-leading organizations recommend reducing red meat consumption However, differences exist among red meats when comparing grass-fed and grass-finished beef. The human health recommendations often neglect the beef production system employed. Grass-finished beef nutrient profile is typically more consistent with nutritional recommendations as it is higher in beneficial n−3 PUFAs and phytochemicals. Variations in nutritional profiles exist among pasture-raised beef, resulting in unequal pasture-raised products and misleading labels. This suggests a need for a “truth in label” based on validation tests and labeling of the FA content of GFB.
This review highlighted the benefits of producing and consuming GFB, but also emphasized the need for standardization. Rotational grazing systems carried out on botanically-rich pastures reinforce the symbiotic relationship between ruminants and landscapes, leading to healthier animals, environment, and humans. Nevertheless, it is critical to determine the effects of different ingredients allowed in GFB on meat nutritional quality. Seasonal differences and supplementation affect the healthfulness of GFB and need to be reported to give consumers a representative idea of the nutritional profile of the products they are consuming.
Future research should focus on assessing and comparing the nutritional profiles of commonly used feeds allowed in GFB production. New efforts should be directed toward developing metabolomic methods to better identify and quantify bioactive compounds that are not well-reported in the literature yet (e.g., FA isomers in ruminants and phytochemicals such as phenolic compounds). The effects of phenolic-rich waste and byproducts from the food industry on meat should be assessed. We also propose that a standardized grass-fed label is implemented, mentioning the production system utilized including the diet. Addressing these research and production gaps will lead to improved grass-fed cattle management and production, with the hope of improving human health.
Author Contributions
LK and JF provided substantial contributions to the conception and design of the work. LK, SS, VJ, JR, and JF were involved in drafting the work or revising it critically for important intellectual content. JR and JF provided approval for publication of the content. JF agrees to be accountable for all aspects of the work in ensuring that questions related to the accuracy or integrity of any part of the work are appropriately investigated and resolved. All authors contributed to the article and approved the submitted version.
Funding
JF and JR report a grant from the Greenacres Foundation to study the effects of supplemental feeds on the nutritional profile of grass-finished beef.
Conflict of Interest
The authors declare that the research was conducted in the absence of any commercial or financial relationships that could be construed as a potential conflict of interest.
Publisher's Note
All claims expressed in this article are solely those of the authors and do not necessarily represent those of their affiliated organizations, or those of the publisher, the editors and the reviewers. Any product that may be evaluated in this article, or claim that may be made by its manufacturer, is not guaranteed or endorsed by the publisher.
References
A Greener World. Certified Grassfed by AGW Standards. Available online at: https://agreenerworld.org.uk/certifications/certified-grass-fed/standards/ (accessed April 16 2022)
Alfaia, C. P. M., Alves, S. P., Martins, S. I. V., Costa, A. S. H., Fontes, C. M. G. A., Lemos, J. P. C., et al. (2009). Effect of the feeding system on intramuscular fatty acids and conjugated linoleic acid isomers of beef cattle, with emphasis on their nutritional value and discriminatory ability. Food Chem. 114, 939–946. doi: 10.1016/j.foodchem.2008.10.041
Allen, B. G. (2021). Fatty Acid Composition of Forages and Their Effect on the Fatty Acid Composition in Beef Cattle. (Master's thesis), Utah State University, Logan, UT (United States).
Allen, V. G., Batello, C., Berretta, E. J., Hodgson, J., Kothmann, M., Li, X., et al. (2011). An international terminology for grazing lands and grazing animals. Grass Forage Sci. 66, 2–28. doi: 10.1111/j.1365-2494.2010.00780.x
Alothman, M., Hogan, S. A., Hennessy, D., Dillon, P., Kilcawley, K. N., O'donovan, M., et al. (2019). The “grass-fed” milk story: understanding the impact of pasture feeding on the composition and quality of bovine milk. Foods 8, 350. doi: 10.3390/foods8080350
American Grassfed Association (2020). American Grassfed Association Grassfed Ruminant Standards–July 2020. Available online at: https://www.americangrassfed.org/wp-content/uploads/2021/03/AGA-Grassfed-Ruminant-Standards-2020-2.pdf (accessed July 15, 2021).
Antonini, E., Torri, L., Piochi, M., Cabrino, G., Meli, M. A., and De Bellis, R. (2020). Nutritional, antioxidant and sensory properties of functional beef burgers formulated with chia seeds and goji puree, before and after in vitro digestion. Meat Sci. 161, 108021. doi: 10.1016/j.meatsci.2019.108021
Arnett, D. K., Blumenthal, R. S., Albert, M. A., Buroker, A. B., Goldberger, Z. D., Hahn, E. J., et al. (2019). 2019 ACC/AHA guideline on the primary prevention of cardiovascular disease: a report of the American college of cardiology/American heart association task force on clinical practice guidelines. Circulation 140, e596–e646. doi: 10.1161/CIR.0000000000000678
Arola-Arnal, A., Oms-Oliu, G., Crescenti, A., Del Bas, J. M., Ras, M. R., Arola, L., et al. (2013). Distribution of grape seed flavanols and their metabolites in pregnant rats and their fetuses. Mol. Nutr. Food Res. 57, 1741–1752. doi: 10.1002/mnfr.201300032
Arya, F., Egger, S., Colquhoun, D., Sullivan, D., Pal, S., and Egger, G. (2010). Differences in postprandial inflammatory responses to a modern v. traditional meat meal: a preliminary study. Br. J. Nutr. 104, 724–728. doi: 10.1017/S0007114510001042
Asioli, D., Aschemann-Witzel, J., Caputo, V., Vecchio, R., Annunziata, A., Næs, T., et al. (2017). Making sense of the “clean label” trends: a review of consumer food choice behavior and discussion of industry implications. Food Res. Int. 99, 58–71. doi: 10.1016/j.foodres.2017.07.022
Baublits, R. T., Brown, A. H. Jr., Pohlman, F. W., Rule, D. C., Johnson, Z. B., Onks, D. O., et al. (2006). Fatty acid and sensory characteristics of beef from three biological types of cattle grazing cool-season forages supplemented with soyhulls. Meat Sci. 72, 100–107. doi: 10.1016/j.meatsci.2005.06.007
Bauchart, D., Verite, R., and Remond, B. (1984). Long-chain fatty acid digestion in lactating cows fed fresh grass from spring to autumn. Can. J. Anim. Sci. 64, 330–331. doi: 10.4141/cjas84-285
Berthelot, V., and Gruffat, D. (2018). “Fatty acid composition of muscles,” in INRA Feeding System for Ruminants (Wageningen, NL: Wageningen Academic Publishers).
Besle, J. M., Viala, D., Martin, B., Pradel, P., Meunier, B., Berdague, J. L., et al. (2010). Ultraviolet-absorbing compounds in milk are related to forage polyphenols. J. Dairy Sci. 93, 2846–2856. doi: 10.3168/jds.2009-2939
Biesalski, H. K. (2005). Meat as a component of a healthy diet - Are there any risks or benefits if meat is avoided in the diet? Meat Sci. 70, 509–524. doi: 10.1016/j.meatsci.2004.07.017
Billingsley, H. E., Carbone, S., and Lavie, C. J. (2018). Dietary fats and chronic noncommunicable diseases. Nutrients 10, 1385. doi: 10.3390/nu10101385
Bloomfield, H. E., Koeller, E., Greer, N., Macdonald, R., Kane, R., and Wilt, T. J. (2016). Effects on health outcomes of a mediterranean diet with no restriction on fat intake: a systematic review and meta-analysis. Ann. Intern. Med. 165, 491–500. doi: 10.7326/M16-0361
Boufaïed, H., Chouinard, P. Y., Tremblay, G. F., Petit, H. V., Michaud, R., and Bélanger, G. (2003). Fatty acids in forages. I. Factors affecting concentrations. Can. J. Anim. Sci. 83, 501–511. doi: 10.4141/A02-098
Brenes, A., Viveros, A., Goñi, I., Centeno, C., Sáyago-Ayerdy, S. G., Arija, I., et al. (2008). Effect of grape pomace concentrate and vitamin E on digestibility of polyphenols and antioxidant activity in chickens. Poult. Sci. 87, 307–316. doi: 10.3382/ps.2007-00297
Britt, C., Gomaa, E. A., Gray, J. I., and Booren, A. M. (1998). Influence of cherry tissue on lipid oxidation and heterocyclic aromatic amine formation in ground beef patties. J. Agric. Food Chem. 46, 4891–4897. doi: 10.1021/jf980233y
Bronkema, S. M., Rowntree, J. E., Jain, R., Schweihofer, J. P., Bitler, C. A., and Fenton, J. I. (2019). A nutritional survey of commercially available grass-finished beef. Meat Muscle Biol. 3, 116–126. doi: 10.22175/mmb2018.10.0034
Buccioni, A., Decandia, M., Minieri, S., Molle, G., and Cabiddu, A. (2012). Lipid metabolism in the rumen: new insights on lipolysis and biohydrogenation with an emphasis on the role of endogenous plant factors. Anim. Feed Sci. Technol. 174, 1–25. doi: 10.1016/j.anifeedsci.2012.02.009
Butler, G. (2014). Manipulating dietary PUFA in animal feed: implications for human health. Proc. Nutr. Soc. 73, 87–95. doi: 10.1017/S0029665113003790
Butler, G., Ali, A. M., Oladokun, S., Wang, J., and Davis, H. (2021). Forage-fed cattle point the way forward for beef? Future Foods 3, 100012. doi: 10.1016/j.fufo.2021.100012
Calder, P. C. (2015). Functional roles of fatty acids and their effects on human health. J. Parenter. Enteral Nutr. 39, 18S−32S. doi: 10.1177/0148607115595980
Chail, A., Legako, J. F., Pitcher, L. R., Griggs, T. C., Ward, R. E., Martini, S., et al. (2016). Legume finishing provides beef with positive human dietary fatty acid ratios and consumer preference comparable with grain-finished beef. J. Anim. Sci. 94, 2184–2197. doi: 10.2527/jas.2015-0241
Cianciosi, D., Forbes-Hernández, T. Y., Afrin, S., Gasparrini, M., Reboredo-Rodriguez, P., Manna, P. P., et al. (2018). Phenolic compounds in honey and their associated health benefits: a review. Molecules 23, 2322. doi: 10.3390/molecules23092322
Coblentz, W. K., and Akins, M. S. (2018). Silage review: recent advances and future technologies for baled silages. J. Dairy Sci. 101, 4075–4092. doi: 10.3168/jds.2017-13708
Costa, L. S., Silva, R. R., Da Silva, F. F., De Carvalho, G. G. P., Simionato, J. I., Marques, J. D. A., et al. (2012). Centesimal composition and fatty acids of meat from lambs fed diets containing soybean hulls. Rev. Bras. de Zootec. 41, 1720–1726. doi: 10.1590/S1516-35982012000700023
Costa, M., Cardoso, C., Afonso, C., Bandarra, N. M., and Prates, J. M. (2021). Current knowledge and future perspectives of the use of seaweeds for livestock production and meat quality: a systematic review. J. Anim. Physiol. Anim. Nutr. 105, 1075–1102. doi: 10.1111/jpn.13509
Da Silva, M. S., Julien, P., Perusse, L., Vohl, M. C., and Rudkowska, I. (2015). Natural rumen-derived trans fatty acids are associated with metabolic markers of cardiac health. Lipids 50, 873–882. doi: 10.1007/s11745-015-4055-3
Daley, C. A., Abbott, A., Doyle, P. S., Nader, G. A., and Larson, S. (2010). A review of fatty acid profiles and antioxidant content in grass-fed and grain-fed beef. Nutr. J. 9, 10. doi: 10.1186/1475-2891-9-10
Danielsson, H., Nadeau, E., Gustavsson, A.-M., Jensen, S., Søegaard, K., and Nilsdotter-Linde, N. (2008). Contents of α-tocopherol and β-carotene in grasses and legumes harvested at different maturities. Grassl. Sci. Eur. 13, 432–434.
Davis, H., Magistrali, A., Butler, G., and Stergiadis, S. (2022). Nutritional benefits from fatty acids in organic and grass-fed beef. Foods 11, 646. doi: 10.3390/foods11050646
Del Razo Olvera, F. M., Melgarejo Hernández, M. A., Mehta, R., and Aguilar Salinas, C. A. (2017). Setting the lipid component of the diet: a work in process. Adv. Nutr. 8, 165S−172S. doi: 10.3945/an.116.013672
Delon, N. (2019). Scarlet Letters: Meat, Normality and the Power of Shaming. Available online at: https://booksandideas.net/Scarlet-Letters.html?fbclid=IwAR3-vnkuYstePQdwpnf8qX3aUyI6lcczOZb48IbldfdYNKYd9zkPvUAtEm4 (accessed June 15, 2021).
Dewhurst, R. J., Scollan, N. D., Youell, S. J., Tweed, J. K. S., and Humphreys, M. O. (2001). Influence of species, cutting date and cutting interval on the fatty acid composition of grasses. Grass Forage Sci. 56, 68–74. doi: 10.1046/j.1365-2494.2001.00247.x
Dierking, R. M., Kallenbach, R. L., and Roberts, C. A. (2010). Fatty acid profiles of orchardgrass, tall fescue, perennial ryegrass, and alfalfa. Crop Sci. 50, 391–402. doi: 10.2135/cropsci2008.12.0741
Dinicolantonio, J. J., Lucan, S. C., and O'keefe, J. H. (2016). The evidence for saturated fat and for sugar related to coronary heart disease. Prog. Cardiovasc. Dis. 58, 464–472. doi: 10.1016/j.pcad.2015.11.006
Doyle, P. R., Mcgee, M., Moloney, A. P., Kelly, A. K., and O'riordan, E. G. (2021). Effect of post-grazing sward height, sire genotype and indoor finishing diet on steer intake, growth and production in grass-based suckler weanling-to-beef systems. Animals 11, 2623. doi: 10.3390/ani11092623
Duckett, S. K., Neel, J. P. S., Fontenot, J. P., and Clapham, W. M. (2009). Effects of winter stocker growth rate and finishing system on: III. Tissue proximate, fatty acid, vitamin, and cholesterol content. J. Anim. Sci. 87, 2961–2970. doi: 10.2527/jas.2009-1850
Duckett, S. K., Neel, J. P. S., Lewis, R. M., Fontenot, J. P., and Clapham, W. M. (2013). Effects of forage species or concentrate finishing on animal performance, carcass and meat quality. J. Anim. Sci. 91, 1454–1467. doi: 10.2527/jas.2012-5914
Dunne, P. G., Monahan, F. J., O'mara, F. P., and Moloney, A. P. (2009). Colour of bovine subcutaneous adipose tissue: a review of contributory factors, associations with carcass and meat quality and its potential utility in authentication of dietary history. Meat Sci. 81, 28–45. doi: 10.1016/j.meatsci.2008.06.013
Elgersma, A., Maudet, P., Witkowska, I. M., and Wever, A. C. (2005). Effects of nitrogen fertilisation and regrowth period on fatty acid concentrations in perennial ryegrass (Lolium perenne L.). Ann. Appl. Biol. 147, 145–152. doi: 10.1111/j.1744-7348.2005.00020.x
Elgersma, A., Søegaard, K., and Jensen, S. K. (2013). Fatty acids, α-tocopherol, β-carotene, and lutein contents in forage legumes, forbs, and a grass-clover mixture. J. Agric. Food Chem. 61, 11913–11920. doi: 10.1021/jf403195v
FAO (2010). Fats and fatty acids in human nutrition: report of an expert consultation. Food Nutr. Pap. 91, 1–166. Available online at: https://www.fao.org/3/i1953e/I1953E.pdf
Field, C. J., Blewett, H. H., Proctor, S., and Vine, D. (2009). Human health benefits of vaccenic acid. Appl. Physiol. Nutr. Metab. 34, 979–991. doi: 10.1139/H09-079
Food Safety Inspection Service (2019). Food Safety and Inspection Service Labeling Guideline on Documentation Needed to Substantiate Animal Raising Claims for Label Submissions. Available online at: https://www.fsis.usda.gov/sites/default/files/media_file/2021-02/RaisingClaims.pdf (accessed June 12, 2021).
Fredriksson Eriksson, S., and Pickova, J. (2007). Fatty acids and tocopherol levels in M. longissimus dorsi of beef cattle in Sweden - a comparison between seasonal diets. Meat Sci. 76, 746–754. doi: 10.1016/j.meatsci.2007.02.021
French, P., Stanton, C., Lawless, F., O'riordan, E. G., Monahan, F. J., Caffrey, P. J., et al. (2000). Fatty acid composition, including conjugated linoleic acid, of intramuscular fat from steers offered grazed grass, grass silage, or concentrate-based diets. J. Anim. Sci. 78, 2849–2855. doi: 10.2527/2000.78112849x
Fritsche, K. L. (2015). The science of fatty acids and inflammation. Adv. Nutr. 6, 293S−301S. doi: 10.3945/an.114.006940
Garcia, P. T., Pordomingo, A., Perez, C. D., Rios, M. D., Sancho, A. M., Volpi Lagreca, G., et al. (2016). Influence of cultivar and cutting date on the fatty acid composition of forage crops for grazing beef production in Argentina. Grass Forage Sci. 71, 235–244. doi: 10.1111/gfs.12167
Gebauer, S. K., Destaillats, F., Dionisi, F., Krauss, R. M., and Baer, D. J. (2015). Vaccenic acid and trans fatty acid isomers from partially hydrogenated oil both adversely affect LDL cholesterol: a double-blind, randomized controlled trial. Am. J. Clin. Nutr. 102, 1339–1346. doi: 10.3945/ajcn.115.116129
Gerber, P. J., Steinfeld, H., Henderson, B., Mottet, A., Opio, C., Dijkman, J., et al. (2013). Tackling Climate Change Through Livestock – A Global Assessment of Emissions and Mitigation Opportunities. Rome: Food and Agriculture Organization of the United Nations (FAO).
Gigon, A., Matos, A. R., Laffray, D., Zuily-Fodil, Y., and Pham-Thi, A. T. (2004). Effect of drought stress on lipid metabolism in the leaves of Arabidopsis thaliana (ecotype Columbia). Ann. Bot. 94, 345–351. doi: 10.1093/aob/mch150
Gilmore, L. A., Walzem, R. L., Crouse, S. F., Smith, D. R., Adams, T. H., Vaidyanathan, V., et al. (2011). Consumption of high-oleic acid ground beef increases HDL-cholesterol concentration but both high- and low-oleic acid ground beef decrease HDL particle diameter in normocholesterolemic men. J. Nutr. 141, 1188–1194. doi: 10.3945/jn.110.136085
Glasser, F., Doreau, M., Maxin, G., and Baumont, R. (2013). Fat and fatty acid content and composition of forages: a meta-analysis. Anim. Feed Sci. Technol. 185, 19–34. doi: 10.1016/j.anifeedsci.2013.06.010
Glover, K. E., Budge, S., Rose, M., Rupasinghe, H. P., Maclaren, L., Green-Johnson, J., et al. (2012). Effect of feeding fresh forage and marine algae on the fatty acid composition and oxidation of milk and butter. J. Dairy Sci. 95, 2797–2809. doi: 10.3168/jds.2011-4736
Godfray, H. C. J., Aveyard, P., Garnett, T., Hall, J. W., Key, T. J., Lorimer, J., et al. (2018). Meat consumption, health, and the environment. Science 361, eaam5324. doi: 10.1126/science.aam5324
Gomez, I., Beriain, M. J., Mendizabal, J. A., Realini, C., and Purroy, A. (2015). Shelf life of ground beef enriched with omega-3 and/or conjugated linoleic acid and use of grape seed extract to inhibit lipid oxidation. Food Sci. Nutr. 4, 67–79. doi: 10.1002/fsn3.251
Gomez, I., Beriain, M. J., Sarries, M. V., Insausti, K., and Mendizabal, J. A. (2014). Low-fat beef patties with augmented omega-3 fatty acid and CLA levels and influence of grape seed extract. J. Food Sci. 79, S2368–2376. doi: 10.1111/1750-3841.12682
Gosnell, H., Gill, N., and Voyer, M. (2019). Transformational adaptation on the farm: processes of change and persistence in transitions to ‘climate-smart' regenerative agriculture. Glob. Environ. Change 59, 101965. doi: 10.1016/j.gloenvcha.2019.101965
Gwin, L. (2009). Scaling-up sustainable livestock production: innovation and challenges for grass-fed beef in the U.S. J. Sustain. Agric. 33, 189–209. doi: 10.1080/10440040802660095
Gwin, L., Durham, C. A., Miller, J. D., and Colonna, A. (2012). Understanding markets for grass-fed beef: taste, price, and purchase preferences. J. Food Distrib. Res. 43, 91–111. doi: 10.22004/ag.econ.145331
Harnack, K., Andersen, G., and Somoza, V. (2009). Quantitation of alpha-linolenic acid elongation to eicosapentaenoic and docosahexaenoic acid as affected by the ratio of n6/n3 fatty acids. Nutr. Metab. 6, 8. doi: 10.1186/1743-7075-6-8
Hayek, M. N., and Garrett, R. D. (2018). Nationwide shift to grass-fed beef requires larger cattle population. Environ. Res. Lett. 13, 084005. doi: 10.1088/1748-9326/aad401
Hodson, G., and Earle, M. (2018). Conservatism predicts lapses from vegetarian/vegan diets to meat consumption (through lower social justice concerns and social support). Appetite 120, 75–81. doi: 10.1016/j.appet.2017.08.027
Husted, K. S., and Bouzinova, E. V. (2016). The importance of n-6/n-3 fatty acids ratio in the major depressive disorder. Medicina 52, 139–147. doi: 10.1016/j.medici.2016.05.003
Hwang, J. A., Islam, M. M., Ahmed, S. T., Mun, H. S., Kim, G. M., Kim, Y. J., et al. (2014). Seamustard (Undaria pinnatifida) improves growth, immunity, fatty acid profile and reduces cholesterol in hanwoo steers. Asian Austral. J. Anim. Sci. 27, 1114–1123. doi: 10.5713/ajas.2014.14072
Jain, R., Bronkema, S. M., Yakah, W., Rowntree, J. E., Bitler, C. A., and Fenton, J. I. (2020). Seasonal differences exist in the polyunsaturated fatty acid, mineral and antioxidant content of U.S. grass-finished beef. PLoS ONE 15, e0229340. doi: 10.1371/journal.pone.0229340
Jenkins, T. C., Wallace, R. J., Moate, P. J., and Mosley, E. E. (2008). Board-invited review: recent advances in biohydrogenation of unsaturated fatty acids within the rumen microbial ecosystem. J. Anim. Sci. 86, 397–412. doi: 10.2527/jas.2007-0588
Kalač, P. (2011). The effects of feeding fresh forage and silage on some nutritional attributes of beef: an overview. J. Agrobiol. 28, 1–13. doi: 10.2478/v10146-011-0001-z
Kalač, P., and Samková, E. (2010). The effects of feeding various forages on fatty acid composition of bovine milk fat: a review. Czech J. Anim. Sci. 55, 521–537. doi: 10.17221/2485-CJAS
Khan, N. A., Cone, J. W., Fievez, V., and Hendriks, W. H. (2012). Causes of variation in fatty acid content and composition in grass and maize silages. Anim. Feed Sci. Technol. 174, 36–45. doi: 10.1016/j.anifeedsci.2012.02.006
Khan, N. A., Cone, J. W., and Hendriks, W. H. (2009). Stability of fatty acids in grass and maize silages after exposure to air during the feed out period. Anim. Feed Sci. Technol. 154, 183–192. doi: 10.1016/j.anifeedsci.2009.09.005
Kiesling, D. D. (2013). Performance, Carcass Traits and Fatty Acid Profiles of Yearling Beef Cattle Supplemented With Self-Fed Byproducts on Pasture. (Master's Thesis), Iowa State University, Ames, IA (United States).
Kilcher, M. R. (1981). Plant development, stage of maturity and nutrient composition. J. Range Manag. 34, 363. doi: 10.2307/3897904
Klopatek, S. C., Xu, Y., Yang, X., Oltjen, J. W., and Vahmani, P. (2022). Effects of multiple grass- and grain-fed production systems on beef fatty acid contents and their consumer health implications. Food Sci. Technol. 2, 712–721. doi: 10.1021/acsfoodscitech.2c00021
Kraft, J., Kramer, J. K. G., Schoene, F., Chambers, J. R., and Jahreis, G. (2008). Extensive analysis of long-chain polyunsaturated fatty acids, CLA, trans-18-1 isomers, and plasmalogenic lipids in different retail beef types. J. Agric. Food Chem. 56, 4775–4782. doi: 10.1021/jf8001813
Kronberg, S. L., Scholljegerdes, E. J., Lepper, A. N., and Berg, E. P. (2011). The effect of flaxseed supplementation on growth, carcass characteristics, fatty acid profile, retail shelf life, and sensory characteristics of beef from steers finished on grasslands of the northern Great Plains. J. Anim. Sci. 89, 2892–2903. doi: 10.2527/jas.2011-4058
Kumar, Y., Yadav, D. N., Ahmad, T., and Narsaiah, K. (2015). Recent trends in the use of natural antioxidants for meat and meat products. Compr. Rev. Food Sci. Food Saf. 14, 796–812. doi: 10.1111/1541-4337.12156
Lee, M. R., Evans, P. R., Nute, G. R., Richardson, R. I., and Scollan, N. D. (2009). A comparison between red clover silage and grass silage feeding on fatty acid composition, meat stability and sensory quality of the M. longissimus muscle of dairy cull cows. Meat Sci. 81, 738–744. doi: 10.1016/j.meatsci.2008.11.016
Lee, M. R. F., Fychan, R., Tweed, J. K. S., Gordon, N., Theobald, V., Yadav, R., et al. (2018). Nitrogen and fatty acid rumen metabolism in cattle offered high or low polyphenol oxidase red clover silage. Animal 13, 1–12. doi: 10.1017/S1751731118003294
Leheska, J. M., Thompson, L. D., Howe, J. C., Hentges, E., Boyce, J., Brooks, J. C., et al. (2008). Effects of conventional and grass-feeding systems on the nutrient composition of beef. J. Anim. Sci. 86, 3575–3585. doi: 10.2527/jas.2007-0565
Lenighan, Y. M., Nugent, A. P., Moloney, A. P., Monahan, F. J., Walton, J., Flynn, A., et al. (2019). A modelling approach to investigate the impact of consumption of three different beef compositions on human dietary fat intakes. Public Health Nutr. 23, 2373–2383. doi: 10.1017/S1368980019003471
Lindmark Månsson, H. (2008). Fatty acids in bovine milk fat. Food Nutr. Res. 52, 5–8. doi: 10.3402/fnr.v52i0.1821
Lock, A. L., Harvatine, K. J., Drackley, J. K., and Bauman, D. E. (2006). “Concepts in fat and fatty acid digestion in ruminants,” in Proceeding Intermountain Nutrition Conference, (Logan, UT: Utah State University) 85–100.
Logan, B. G., Hopkins, D. L., Schmidtke, L., Morris, S., and Fowler, S. M. (2020). Preliminary investigation into the use of Raman spectroscopy for the verification of Australian grass and grain fed beef. Meat Sci. 160, 107970. doi: 10.1016/j.meatsci.2019.107970
Lourenço, M., De Smet, S., Raes, K., and Fievez, V. (2007a). Effect of botanical composition of silages on rumen fatty acid metabolism and fatty acid composition in longissimus muscle and subcutaneous fat of lambs. Animal 1, 911–921. doi: 10.1017/S1751731107000316
Lourenço, M., Van Ranst, G., De Smet, S., Raes, K., and Fievez, V. (2007b). Effect of grazing pastures with different botanical composition by lambs on rumen fatty acid metabolism and fatty acid pattern of longissimus muscle and subcutaneous fat. Animal 1, 537–545. doi: 10.1017/S1751731107703531
Mapiye, C., Turner, T. D., Rolland, D. C., Basarab, J. A., Baron, V. S., Mcallister, T. A., et al. (2013). Adipose tissue and muscle fatty acid profiles of steers fed red clover silage with and without flaxseed. Livest. Sci. 151, 11–20. doi: 10.1016/j.livsci.2012.10.021
Margalef, M., Pons, Z., Bravo, F. I., Muguerza, B., and Arola-Arnal, A. (2015). Plasma kinetics and microbial biotransformation of grape seed flavanols in rats. J. Funct. Foods 12, 478–488. doi: 10.1016/j.jff.2014.12.007
Markiewicz-Keszycka, M., Czyzak-Runowska, G., Lipinska, P., and Wójtowski, J. (2013). Fatty acid profile of milk - a review. Bull. Vet. Inst. Pulawy 57, 135–139. doi: 10.2478/bvip-2013-0026
Mathews, K. H., and Johnson, R. J. (2013). Alternative Beef Production Systems: Issues and Implications. Economic Research Services LDPM-128-01. Available online at: https://www.ers.usda.gov/webdocs/outlooks/37473/36491_ldpm-218-01.pdf?v=7815.8 (accessed May 23, 2021).
Medina-Remón, A., Tresserra-Rimbau, A., Pons, A., Tur, J. A., Martorell, M., Ros, E., et al. (2015). Effects of total dietary polyphenols on plasma nitric oxide and blood pressure in a high cardiovascular risk cohort. The PREDIMED randomized trial. Nutr. Metab. Cardiovasc. Dis. 25, 60–67. doi: 10.1016/j.numecd.2014.09.001
Melendrez-Ruiz, J., Chambaron, S., Buatois, Q., Monnery-Patris, S., and Arvisenet, G. (2019). A central place for meat, but what about pulses? Studying French consumers' representations of main dish structure, using an indirect approach. Food Res. Int. 123, 790–800. doi: 10.1016/j.foodres.2019.06.004
Menaa, F., Menaa, A., Menaa, B., and Treton, J. (2013). Trans-fatty acids, dangerous bonds for health? A background review paper of their use, consumption, health implications and regulation in France. Eur. J. Nutr. 52, 1289–1302. doi: 10.1007/s00394-012-0484-4
Mendivil, C. O. (2021). Dietary fish, fish nutrients, and immune function: a review. Front. Nutr. 7, 617652. doi: 10.3389/fnut.2020.617652
Mir, P. S., Bittman, S., Hunt, D., Entz, T., and Yip, B. (2006). Lipid content and fatty acid composition of grasses sampled on different dates through the early part of the growing season. Can. J. Anim. Sci. 86, 279–290. doi: 10.4141/A05-050
Morais, T., Inácio, A., Coutinho, T., Ministro, M., Cotas, J., Pereira, L., et al. (2020). Seaweed potential in the animal feed: a review. J. Mar. Sci. Eng. 8, 559. doi: 10.3390/jmse8080559
Muñoz-González, I., Chamorro, S., Pérez-Jiménez, J., López-Andrés, P., Álvarez-Acero, I., Herrero, A. M., et al. (2019). Phenolic metabolites in plasma and thigh meat of chickens supplemented with grape byproducts. J. Agric. Food Chem. 67, 4463–4471. doi: 10.1021/acs.jafc.9b00222
Nassu, R. T., Dugan, M. E., He, M. L., Mcallister, T. A., Aalhus, J. L., Aldai, N., et al. (2011). The effects of feeding flaxseed to beef cows given forage based diets on fatty acids of longissimus thoracis muscle and backfat. Meat Sci. 89, 469–477. doi: 10.1016/j.meatsci.2011.05.016
Omaye, A. T., and Omaye, S. T. (2019). Caveats for the good and bad of dietary red meat. Antioxidants 8, 1–10. doi: 10.3390/antiox8110544
Owens, F. N., Secrist, D. S., Hill, W. J., and Gill, D. R. (1997). The effect of grain source and grain processing on performance of feedlot cattle: a review. J. Anim. Sci. 75, 868–879. doi: 10.2527/1997.753868x
Parolini, C. (2019). Effects of fish n-3 PUFAs on intestinal microbiota and immune system. Mar. Drugs 17, 374. doi: 10.3390/md17060374
PCAS. Certified Pasturefed Approved Supplements List. Available online at: https://pcaspasturefed.com.au/approved-supplements-list (accessed April 16, 2022)..
Pickworth, C. L., Loerch, S. C., Kopec, R. E., Schwartz, S. J., and Fluharty, F. L. (2012). Concentration of pro-vitamin A carotenoids in common beef cattle feedstuffs. J. Anim. Sci. 90, 1553–1561. doi: 10.2527/jas.2011-4217
Pighin, D., Pazos, A., Chamorro, V., Paschetta, F., Cunzolo, S., Godoy, F., et al. (2016). A contribution of beef to human health: a review of the role of the animal production systems. Sci. World J. 2016, 8681491. doi: 10.1155/2016/8681491
Pogorzelska-Nowicka, E., Atanasov, A. G., Horbańczuk, J., and Wierzbicka, A. (2018). Bioactive compounds in functional meat products. Molecules 23, 1–19. doi: 10.3390/molecules23020307
Ponnampalam, E. N., Mann, N. J., and Sinclair, A. J. (2006). Effect of feeding systems on omega-3 fatty acids, conjugated linoleic acid and trans fatty acids in Australian beef cuts: potential impact on human health. Asia Pac. J. Clin. Nutr. 15, 21–29.
Poore, M. H., Johns, J. T., and Burris, W. R. (2002). Soybean hulls, wheat middlings, and corn gluten feed as supplements for cattle on forage-based diets. Vet. Clin. North Am. Food Anim. Pract. 18, 213–231. doi: 10.1016/S0749-0720(02)00021-X
Pouzo, L. B., Descalzo, A. M., Zaritzky, N. E., Rossetti, L., and Pavan, E. (2016). Antioxidant status, lipid and color stability of aged beef from grazing steers supplemented with corn grain and increasing levels of flaxseed. Meat Sci. 111, 1–8. doi: 10.1016/j.meatsci.2015.07.026
Prache, S., Martin, B., and Coppa, M. (2020). Review: authentication of grass-fed meat and dairy products from cattle and sheep. Animal 14, 854–863. doi: 10.1017/S1751731119002568
Provenza, F. D., Kronberg, S. L., and Gregorini, P. (2019). Is grassfed meat and dairy better for human and environmental health? Front. Nutr. 6, 26. doi: 10.3389/fnut.2019.00026
Provenza, F. D., Meuret, M., and Gregorini, P. (2015). Our landscapes, our livestock, ourselves: restoring broken linkages among plants, herbivores, and humans with diets that nourish and satiate. Appetite 95, 500–519. doi: 10.1016/j.appet.2015.08.004
Pugh, R. B. (2003). Soybean Hull Supplementation to Pasture-Based Beef Cattle Finishing Diets. (Master's Thesis), University of Tennessee, Knoxville, TN (United States).
Qiu, B., Wang, Q., Liu, W., Xu, T. C., Liu, L. N., Zong, A. Z., et al. (2018). Biological effects of trans fatty acids and their possible roles in the lipid rafts in apoptosis regulation. Cell Biol. Int. 42, 904–912. doi: 10.1002/cbin.10958
Revello-Chion, A., Tabacco, E., Peiretti, P. G., and Borreani, G. (2011). Variation in the fatty acid composition of alpine grassland during spring and summer. Agron. J. 103, 1072–1080. doi: 10.2134/agronj2010.0509
Ritchie, H., Reay, D. S., and Higgins, P. (2018). Potential of meat substitutes for climate change mitigation and improved human health in high-income markets. Front. Sustain. Food Syst. 2, 16. doi: 10.3389/fsufs.2018.00016
Saini, R. K., and Keum, Y. S. (2018). Omega-3 and omega-6 polyunsaturated fatty acids: dietary sources, metabolism, and significance — a review. Life Sci. 203, 255–267. doi: 10.1016/j.lfs.2018.04.049
Sanchez-Rodriguez, E., Biel-Glesson, S., Fernandez-Navarro, J. R., Calleja, M. A., Espejo-Calvo, J. A., Gil-Extremera, B., et al. (2019). Effects of virgin olive oils differing in their bioactive compound contents on biomarkers of oxidative stress and inflammation in healthy adults: a randomized double-blind controlled trial. Nutrients 11, 561. doi: 10.3390/nu11030561
Schmid, M., Kraft, L. G. K., Van Der Loos, L. M., Kraft, G. T., Virtue, P., Nichols, P. D., et al. (2018). Southern Australian seaweeds: a promising resource for omega-3 fatty acids. Food Chem. 265, 70–77. doi: 10.1016/j.foodchem.2018.05.060
Schmidt, J. R., Miller, M. C., Andrae, J. G., Ellis, S. E., and Duckett, S. K. (2013). Effect of summer forage species grazed during finishing on animal performance, carcass quality, and meat quality. J. Anim. Sci. 91, 4451–4461. doi: 10.2527/jas.2012-5405
Scollan, N. D., Dannenberger, D., Nuernberg, K., Richardson, I., Mackintosh, S., Hocquette, J. F., et al. (2014). Enhancing the nutritional and health value of beef lipids and their relationship with meat quality. Meat Sci. 97, 384–394. doi: 10.1016/j.meatsci.2014.02.015
Scollan, N. D., Hocquette, J. F., Nuernberg, K., Dannenberger, D., Richardson, I., and Moloney, A. (2006). Innovations in beef production systems that enhance the nutritional and health value of beef lipids and their relationship with meat quality. Meat Sci. 74, 17–33. doi: 10.1016/j.meatsci.2006.05.002
Serra, A., Blade, C., Arola, L., Macia, A., and Motilva, M. J. (2013). Flavanol metabolites distribute in visceral adipose depots after a long-term intake of grape seed proanthocyanidin extract in rats. Br. J. Nutr. 110, 1411–1420. doi: 10.1017/S0007114513000706
Serra, V., Salvatori, G., and Pastorelli, G. (2021). Dietary polyphenol supplementation in food producing animals: effects on the quality of derived products. Animals 11, 401. doi: 10.3390/ani11020401
Simopoulos, A. P. (2002). The importance of the ratio of omega-6/omega-3 essential fatty acids. Biomed. Pharmacother. 56, 365–379. doi: 10.1016/S0753-3322(02)00253-6
Simopoulos, A. P. (2006). Evolutionary aspects of diet, the omega-6/omega-3 ratio and genetic variation: nutritional implications for chronic diseases. Biomed. Pharmacother. 60, 502–507. doi: 10.1016/j.biopha.2006.07.080
Smith, D. M. (2017). Method of Increasing Levels of Omega-3 Fatty Acids in Beef Products by Administeration of a Grass and Algae Diet. U.S. patent application US 2017/0354166 A1. Aspen, CO.
Spratt, E., Jordan, J., Winsten, J., Huff, P., Van Schaik, C., Jewett, J. G., et al. (2021). Accelerating regenerative grazing to tackle farm, environmental, and societal challenges in the upper Midwest. J. Soil Water Conserv. 76, 15A−23A. doi: 10.2489/jswc.2021.1209A
Stamey, J. A., Shepherd, D. M., De Veth, M. J., and Corl, B. A. (2012). Use of algae or algal oil rich in n-3 fatty acids as a feed supplement for dairy cattle. J. Dairy Sci. 95, 5269–5275. doi: 10.3168/jds.2012-5412
Steiner, J. L., Schneider, J. M., Pope, C., Pope, S., Ford, P., and Steele, R. F. (2015). Southern plains assessment of vulnerability and preliminary adaptation and mitigation strategies for farmers, ranchers and forest land owners. Available online at: https://www.fs.usda.gov/treesearch/pubs/50256 (accessed May 03, 2022).
Steinshamn, H., and Thuen, E. (2008). White or red clover-grass silage in organic dairy milk production: grassland productivity and milk production responses with different levels of concentrate. Livest. Sci. 119, 202–215. doi: 10.1016/j.livsci.2008.04.004
Tayengwa, T., Chikwanha, O. C., Neethling, J., Dugan, M. E. R., Mutsvangwa, T., and Mapiye, C. (2021). Polyunsaturated fatty acid, volatile and sensory profiles of beef from steers fed citrus pulp or grape pomace. Food Res. Int. 139, 109923. doi: 10.1016/j.foodres.2020.109923
Tripathi, H. P., Singh, A. P., Upadhyay, V. S., Kessels, H. P. P., Harika, A. S., Singh, S., et al. (1995). ”Forage conservation, storage and feeding,“ in Handbook for Straw Feeding System, eds K. Singh and J. B. Schiere (New Delhi: ICAR), 303–323.
U.S. Department of Agriculture (2021). Silage. Available online at: https://agclass.nal.usda.gov/mtwdk.exe?k=glossary&l=60&w=13896&s=5&t=2 (accessed August 10, 2021).
Van Elswyk, M. E., and Mcneill, S. H. (2014). Impact of grass/forage feeding versus grain finishing on beef nutrients and sensory quality: the U.S. experience. Meat Sci. 96, 535–540. doi: 10.1016/j.meatsci.2013.08.010
Van Ranst, G., Fievez, V., Vandewalle, M., De Riek, J., and Van Bockstaele, E. (2009). Influence of herbage species, cultivar and cutting date on fatty acid composition of herbage and lipid metabolism during ensiling. Grass Forage Sci. 64, 196–207. doi: 10.1111/j.1365-2494.2009.00686.x
Van Vliet, S., Bain, J. R., Muehlbauer, M. J., Provenza, F. D., Kronberg, S. L., Pieper, C. F., et al. (2021a). A metabolomics comparison of plant-based meat and grass-fed meat indicates large nutritional differences despite comparable Nutrition Facts panels. Sci. Rep. 11, 13828. doi: 10.1038/s41598-021-93100-3
Van Vliet, S., Provenza, F. D., and Kronberg, S. L. (2021b). Health-promoting phytonutrients are higher in grass-fed meat and milk. Front. Sustain. Food Syst. 4, 555426. doi: 10.3389/fsufs.2020.555426
Vannice, G., and Rasmussen, H. (2014). Position of the academy of nutrition and dietetics: dietary fatty acids for healthy adults. J. Acad. Nutr. Diet. 114, 136–153. doi: 10.1016/j.jand.2013.11.001
Verneque, B. J. F., Machado, A. M., De Abreu Silva, L., Lopes, A. C. S., and Duarte, C. K. (2020). Ruminant and industrial trans-fatty acids consumption and cardiometabolic risk markers: a systematic review. Crit. Rev. Food Sci. Nutr. 62, 2050–2060. doi: 10.1080/10408398.2020.1836471
Welch, A. A., Shakya-Shrestha, S., Lentjes, M. A., Wareham, N. J., and Khaw, K. T. (2010). Dietary intake and status of n-3 polyunsaturated fatty acids in a population of fish-eating and non-fish-eating meat-eaters, vegetarians, and vegans and the product-precursor ratio [corrected] of alpha-linolenic acid to long-chain n-3 polyunsaturated fatty acids: results from the EPIC-Norfolk cohort. Am. J. Clin. Nutr. 92, 1040–1051. doi: 10.3945/ajcn.2010.29457
Wolk, A. (2017). Potential health hazards of eating red meat. J. Intern. Med. 281, 106–122. doi: 10.1111/joim.12543
Woods, V. B., and Fearon, A. M. (2009). Dietary sources of unsaturated fatty acids for animals and their transfer into meat, milk and eggs: a review. Livest. Sci. 126, 1–20. doi: 10.1016/j.livsci.2009.07.002
World Health Organization (2018). Non-Communicable Diseases. WHO. Available online at: https://www.who.int/news-room/fact-sheets/detail/noncommunicable-diseases (accessed July 2, 2021).
Xue, H., Mainville, D., You, W., and Nayga, R. M. (2010). Consumer preferences and willingness to pay for grass-fed beef: empirical evidence from in-store experiments. Food Qual. Prefer. 21, 857–866. doi: 10.1016/j.foodqual.2010.05.004
Keywords: finishing system, beef, fatty acids, phytochemicals, sustainability, pasture
Citation: Krusinski L, Sergin S, Jambunathan V, Rowntree JE and Fenton JI (2022) Attention to the Details: How Variations in U.S. Grass-Fed Cattle-Feed Supplementation and Finishing Date Influence Human Health. Front. Sustain. Food Syst. 6:851494. doi: 10.3389/fsufs.2022.851494
Received: 10 January 2022; Accepted: 27 April 2022;
Published: 19 May 2022.
Edited by:
Kurt A. Rosentrater, Iowa State University, United StatesReviewed by:
Gillian Butler, Newcastle University, United KingdomSusan Kay Duckett, Clemson University, United States
Copyright © 2022 Krusinski, Sergin, Jambunathan, Rowntree and Fenton. This is an open-access article distributed under the terms of the Creative Commons Attribution License (CC BY). The use, distribution or reproduction in other forums is permitted, provided the original author(s) and the copyright owner(s) are credited and that the original publication in this journal is cited, in accordance with accepted academic practice. No use, distribution or reproduction is permitted which does not comply with these terms.
*Correspondence: Jenifer I. Fenton, imigjeni@msu.edu