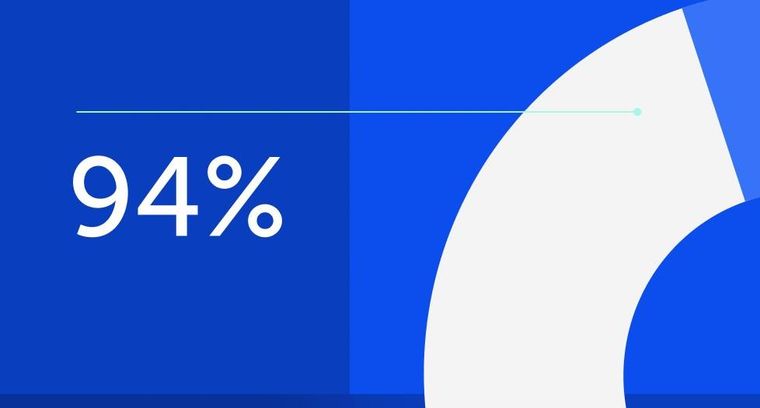
94% of researchers rate our articles as excellent or good
Learn more about the work of our research integrity team to safeguard the quality of each article we publish.
Find out more
ORIGINAL RESEARCH article
Front. Sustain. Food Syst., 04 April 2022
Sec. Crop Biology and Sustainability
Volume 6 - 2022 | https://doi.org/10.3389/fsufs.2022.845512
This article is part of the Research TopicHeavy Metal(loid) Stress-alleviating and Phytostimulating Microorganisms: Dual-performing Warhorses in Soil-bioremediationView all 10 articles
Fungicides play an immense role in quenching the infection of Panama wilt in banana. However, the use of fungicides and the monoculture of banana cultivars have resulted in the development of new races like race 4 which challenges scientists across the globe to identify new candidates for biological suppression of Fusarium oxysporum f.sp. cubense (Foc). Hence, attempts were made to dissect the endophytes from resistant banana cultivar YKM5 (Yengambi–AAA) to suppress Foc KP (race 4) infecting cv. Karpooravalli (ABB; Pisang Awak). Among the various endophytes, Bacillus velezensis YEBBR6 inhibited the mycelial growth up to 63% over control and hyper-parasitized the mycelium of Foc KP. Scanning electron microscope analysis revealed the ramification by B. velezensis over the hyphae of Foc KP leading to lysis. Analysis of VOCs/NVOCs compounds from the zone of inhibition, confirmed the presence of unique biomolecules including linoelaedic acid, nonanol, acetylvaleryl, 5–hydroxyl methyl furfural, clindamycin, allobarbital, 3-thiazolidine carboxamide, azulene, aminomorpholine, procyclidine, campholic acid, 3 amino-4 hydroxy phenyl sulfone, 3–deoxy mannoic lactone, hexadecanoic acid, oleic acid, and dihydroacridine of an antifungal and antimicrobial nature. Considering the diverse antimicrobial property, biohardening of micropropagated banana cv. Karpooravalli (ABB) with a liquid formulation of B. velezensis YEBBR6 (8 × 108 cfu/ml) and challenge inoculation with Foc KP promoted plant growth compared to uninoculated control. Besides, incidence of Fusarium wilt was reduced by 100% over inoculated control in greenhouse conditions. Furthermore, the expression of transcription factors and defense genes WRKY, MAPK, CERK 1, LOX, and PAL increased by several folds compared to inoculated and healthy control and thus suppressed Fusarium wilt of banana cv. Karpooravalli (ABB). Also, cytoscape analysis of defense genes indicated the coordinated expression of various other genes associated with it. Hence, our study confirmed the scope for exploring B. velezensis on a commercial scale for the management of Fusarium wilt race 4 causing wilt across genomes of banana.
Graphical Abstract. Induction of innate immune response and growth promotion in banana by B. velezensis against Foc.
Globally, banana is commercially cultivated in several tropical and subtropical regions. Monoculture of banana has resulted in the outbreak of Fusarium wilt. Panama wilt of banana caused by Fusarium oxysporum f.sp. cubense (Foc) is a dreadful disease affecting the livelihood of the farming community and the sustainability of banana-based industries. Pathogens survive as chlamydospores in soil for decades. Furthermore, owing to the proliferation of pathogens, outbreak of race 4 has emerged as a most destructive pathogen worldwide (Butler, 2013). Race 4 was observed in 90% of banana plantations in South China (Cheng et al., 2012). Recently in India, Foc TR4 has been reported in Gujarat and Bihar, indicating the potential spread and threat in the near future (Nakkeeran et al., 2021). Hence, to date there are no effective fungicides to curb the spread and infection of banana by race 4. This lacuna warrants the development of a novel management strategy to quench Fusarium wilt of banana. Besides, one school of thought also emphasizes that continuous application of chemical fertilizers acidifies the soil and accentuates the multiplication of Foc resulting in severe outbreaks of Fusarium wilt (Zhao et al., 2018). Considering the serious threat imposed by Foc, an effective alternate method has to be developed for the management of Fusarium wilt of banana. To reach sustainability and profitability in food production, plant growth-promoting rhizobacteria (PGPR) play a key role and also aid in increasing the productivity of plants both under biotic and abiotic stress. Furthermore, PGPR suppress plant pathogens through competition, antibiosis, lysis, and through induction of systemic resistance (Anupama et al., 2014; Narasimha Murthy et al., 2021). In this juncture, biological control will pave the way for the sustainable management of wilt caused by different races of Foc. Amidst the various biocontrol agents, bacterial endophytes can be well explored for the same, rather than fungicides (Nel et al., 2006). Several endophytic Bacillus spp. have been explored for the management of Fusarium wilt of banana as they are bestowed with beneficial attributes including plant growth promotion, induction of immune response, and suppression of Foc (Nakkeeran et al., 2021; Saravanan et al., 2021a). In the recent past, Bacillus spp. have been well explored for the management of soil-borne and foliar diseases. The antagonistic Bacillus spp. quench plant pathogens through the production of antifungal biomolecules, antimicrobial peptides, and through the induction of an immune response (Nakkeeran et al., 2019). However, the versatile Bacillus spp. genome of B. velezensis comprises genes coding for different antimicrobial peptides, hydrolytic enzymes, growth hormones, and induction of immune response contributing to antiviral and antifungal action (Saravanan et al., 2021b). Moreover, B. velezensis has broad-spectrum action against several fungal pathogens (Meng and Hao, 2017). However, based on the perusal of literature there are no reports describing the exploration of immune response mediated by B. velezensis to manage Foc infection in banana. In the current investigation, B. velezensis YEBBR6 isolated from the bract of resistant genotype YKM 5 was evaluated for antifungal activity, growth promotion, secondary metabolite production, and reprogramming of immune response for the management of Fusarium wilt of banana through biohardening of micropropagated cv. Karpooravalli (Pisang Awak–ABB) plantlets.
The wilt affected banana corms from different banana-cultivating provinces of Tamil Nadu, India pertaining to Lakshmipuram village of Theni province (10° 11′ 16.62″ N77° 47′ 34.4112″E/ latitude 10.187950/ longitude 77.792892), Chinnamanur village of Theni province (9° 50′ 22.776″N77° 22′ 58.0692″E/ latitude 9.839660/ longitude 77.382797), Thondamuthur village of Coimbatore province (11°00′35″N 76°49′41″E/ latitude 10.9905/ longitude 10.9905), Sirumugai village of Coimbatore province (11° 19′ 16.9″N, 77° 0′ 18.8″E/ latitude 11.3183/ longitude 77.0066), Ammapalayam village of Salem province (11° 40.7123′ N 78° 7.4319′ E/ latitude 11.678539/ longitude 78.123865), Athani village of Erode province (11.5232° N, 77.5120° E/ latitude 11.515219/ longitude 77.452367), Andhiyur village of Erode province (11° 34′ 37.4628″N 77° 35′ 15.8280″E/ latitude 11.577073/ longitude 77.587730), and Gobichettipalayam village of Erode province (11.4548° N, 77.4365° E/ latitude 11.450410/ longitude 77.430036) were collected. Pathogenic Fusarium spp. associated with banana cultivars susceptible to Panama wilt of banana were isolated as per the protocol described by Nelson et al. (1983). Genomic DNA of Fusarium was extracted from the mycelium of pure culture through the CTAB method (Griffith and Shaw, 1998). Genomic DNA was used as a template for PCR amplification of Foc isolates using ITS1 (5′-TCCGTAGGTGAACCTGCGG-3′) and ITS4 (5′-TCCTCCGCTTATTGATATGC-3′) primers (White et al., 1990). Further to confirm the Foc races, secreted in Xylem (SIX) Six13c 343 F (5′CAGCCTCCTAGCGTCGAAAA 3′) and Six13c R (5′CCGTGATGGGGTACGTTGTA 3′) were used (Czislowski et al., 2018). The program cycle comprised of initial denaturation (95°C) for 2 min, followed by 40 cycles of denaturation (95°C) for 1 min, annealing at 58°C for 1 min, extension for 1 min at 72°C, and with a final extension at 72°C. Gel electrophoresis and staining was done by loading 10 μl of PCR product on 1% agarose gel in TAE buffer at 80 V for 50 min at 25°C. A 1 kb DNA ladder was used to determine the size of amplified genomic products. PCR products were photographed using a gel documentation system. The amplified genomic product was sequenced by Eurofins Genomics Biotech Pvt. Ltd., Bangalore, India. Gene homology searches were performed using NCBI BLAST. Sequences were compared with different Foc isolates retrieved from the GenBank database. Newly obtained sequences were submitted to the GenBank database (New York, USA) and accession numbers were obtained. Phylogenetic analysis was performed with MEGA7 software (Tamura et al., 2007).
An antagonistic bacterial endophyte was isolated from 11-month-old Foc-resistant banana cv. Yengambi KM5 (AAA) maintained at the Banana Field Gene Bank, Tamil Nadu Agricultural University, Coimbatore (latitude: 11° 07′ 3.36″ N, longitude: 76° 59′ 39.91″ E), Tamil Nadu, India. The identity of antagonistic bacterial endophytes against Foc KP was confirmed using a 1.5 kb full-length 16S rRNA gene: 8 F (5′AGAGTTTGATCCTGGCTCAG-3′), 1492 R (5′GGGTTACCTTGTTACGACTT-3′). Antagonistic isolates YEBBR6, YEBN2, YEBRH5, YEBRT4, YEBFR1, and YEBFL6 that inhibited Foc KP were confirmed as Bacillus velezensis (MT372157), Bacillus albus (MT120179), Achromobacter xylosoxidans (MK258170), Beijerinckia fluminensis (MK263670), Acinetobacter refrigeratoris (MT326234), and Bacillus endophyticus (MT326238), respectively (Saravanan et al., 2021a).
The efficacy of bacterial endophyte B. velezensis YEBBR6, isolated from resistant banana cv. Yengambi KM5 (AAA) was tested in vitro against F. oxysporum f. sp. cubense Foc KP (NCBI accession no. MW 436477). The ability of the antagonist B. velezensis to suppress Foc KP was assessed through a dual culture technique. A 9 mm mycelial disc was excised using a sterile cork borer from a 7-day-old culture of Foc KP. It was placed on one side of a Petri plate containing PDA medium, 10 mm away from the periphery. The bacterial endophyte (24 h old) was streaked on the medium 10 mm away from the periphery, exactly opposite the mycelial disc of Foc KP. The plates were incubated at 28 ± 2°C for 7 days. The VOCs/NVOCs produced by B. velezensis YEBBR6 in PDA medium from the zone of inhibition were extracted by excising the agar from the zone of inhibition using a sterile scalpel. Excised agar with VOCs/NVOCs was blended with HPLC-grade acetonitrile in 1:4 ratios (5 g agar in 20 ml of HPLC grade acetonitrile). The mixture was sonicated twice for 30 s at 30% of the power of the sonicator for homogenization. After homogenization, samples were centrifuged and filtered to remove solid particles. The samples were dried in a vacuum flash evaporator (Rotrva Equitron Make). After removing the eluent, the final product was dissolved in 1 ml of HPLC-grade methanol (Cawoy et al., 2015). The difference in VOCs/NVOCs profile produced during the interaction of B. velezensis YEBBR6 with Foc KP was characterized with PDA control, pathogen-inoculated control and bacterial antagonist-inoculated control through GC/MS (GC Clarus 500 Perkin Elmer Analysis) using the NIST version 2005 MS data library.
Liquid formulation was developed by inoculating single colonies of B. velezensis YEBBR6, B. albus YEBN2, Achromobacter xylosoxidans YEBRH5, Beijerinckia fluminensis YEBRT4, Acinetobacter refrigeratoris YEBFR1, and Bacillus endophyticus YEBFL6 in Luria Bertani (LB) broth. Inoculated LB broth was incubated at 28 ± 2°C for 72 h in an orbital shaker at 150 rpm. The concentration of bacterial cells in the culture broth was assessed by measuring the absorbance at 600 nm in a bio-spectrophotometer (Eppendorf Make) at an optical density (OD) of 1.0 ABS. Later, bacterial suspension in LB broth pertaining to six different bacterial antagonists was blended with 10 ml of glycerol (1%), 10 ml of tween 20 (1%), and 1% poly vinylpyrrolidone (PVP) supplied from Sigma–Aldrich. The resultant mixture of individual bacterial antagonists was mixed by incubation in an orbital shaker at 150 rpm for 10 min. The formulation of individual bacterial antagonists was adjusted to 8 × 108 cfu/ml (Vinodkumar et al., 2017).
Micropropagated banana cv. Karpooravalli (ABB) plantlets were biohardened with a liquid formulation of antagonistic bacterial endophytes (8 × 108 cfu/ml). Micropropagated plantlets maintained in the protrays were biohardened on days 15 and 30 by drenching the root zone with 1% bacterial formulation. Biohardened plantlets were planted in polybags filled with EC (< 0.6 mS / cm) and pH (7)-stabilized sterile Cocopeat and incubated in the mist chamber. After 1 month of primary hardening, the micropropagated plantlets were subjected to secondary hardening. During secondary hardening, banana plantlets were transplanted in polybags (10 cm × 15 cm) containing one part of sterile red soil, one part of sterile sand, and one part of sterile farmyard manure mixture. The plantlets were hardened twice at 15-day intervals with 1% liquid formulation of antagonistic bacterial endophytes (8 × 108 cfu/ml) in the root zone until saturation and maintained in a greenhouse for further studies. Micropropagated banana cv. Karpooravalli (ABB) plantlets drenched with water served as untreated control.
The photosynthetic rate (μmol CO2 m−2 s−1), transpiration rate (mmol H2O m−2 s−1), stomatal conductance (m mol m−2s), chlorophyll stability index, and relative water content (%) were recorded on the fully expanded leaves of banana plantlets using the LI-COR (LI-6400XT) Portable Photosynthesis System (PPS). The leaf chamber was the open type and measurements were taken at 10:00 h (IST). While taking measurements, photosynthetically active radiation of 1,500 μmol m−2 s−1 was maintained with an inbuilt light source. A temperature of 25 ± 5°C, relative humidity of 65 ± 5%, and reference carbon dioxide at a concentration of 380 mol CO2 mol air−1 were also maintained. Observations were recorded after the plant reached steady-state photosynthesis (15 days after transferring to the greenhouse).
The experiment consisted of six treatments, viz., B. velezensis YEBBR6, B. albus YEBN2, Achromobacter xylosoxidans YEBRH5, Beijerinckia fluminensis YEBRT4, Acinetobacter refrigeratoris YEBFR1, and Bacillus endophyticus YEBFL6, replicated three times with 10 plantlets per replication. Inoculum of Foc KP was multiplied in quarter-strength Potato Dextrose Broth (PDB) incubated for 7 days at 28 ± 2°C. Conidial load in the culture broth was assessed using a hemocytometer. Concentration of conidia was adjusted to 1 × 106 spores per ml (Catambacan and Cumagun, 2021). Biohardened micropropagated banana cv. Karpooravalli (ABB; Pisang Awak) plantlets with different bacterial endophytes were challenged with conidial suspension of Foc KP. Simultaneously, untreated control was also maintained. Banana plantlets biohardened with different bacterial endophytes challenged with Foc KP were watered regularly until saturation of soil moisture. Eight weeks after inoculation of Foc KP, the degree of disease severity was assessed. It was assessed based on leaf yellowing using a modified disease rating scale (Dita et al., 2014). The intensity of rhizome discoloration was scored by examining the longitudinal section of the pseudostem to the rhizome using a modified rating scale (Carlier et al., 2003).
Percent disease severity was calculated as:
One centimeter of banana root bits were fixed in 2.5% glutaraldehyde and 2.5% formaldehyde prepared in 0.1 M phosphate buffer of pH 7.2 (Liu et al., 2014) for 24 h at 4°C. Samples were exposed to a mild vacuum so as to ensure rapid infiltration of the fixative into the specimens. The fixed specimens were washed three times with 0.1 M phosphate buffer (pH 7.2) by incubation at 23°C for 30 min each time. The specimens were infiltrated overnight with 30% glycerol in water as a cryoprotectant. Later, specimens were frozen in liquid nitrogen, then cross-sectioned with a sterile scalpel. Later, specimens were post-fixed in 1% OsO4 in 0.1 M phosphate buffer (pH 7.2) for 1 h. Subsequently, samples were washed three times in deionized water and incubated for 30 min for each wash. Next, specimens were dehydrated in 100% ethanol and air-dried to remove the ethanol residues. The dried specimens were mounted on aluminum stubs and sputter-coated with gold. SEM photographs pertaining to colonization of the rhizoplane by B. velezensis YEBBR6 and Foc KP and hyperparasitic interaction of B. velezensis YEBBR6 on Foc KP were documented with Hitachi S-3500N 143 SEM at 15 kV.
Total RNA was extracted from the roots of banana biohardened with B. velezensis YEBBR6 challenged with Foc KP using Trizol (Sigma Aldrich) at 0 h, 24 h, 48 h, and 72 h after Foc KP inoculation (Chomczynski and Sacchi, 1987). Similarly, RNA was extracted from untreated healthy control, B. velezensis YEBBR6 alone, and Foc KP-inoculated control. RNA extracted from different treatments was made up to 3,000 ng/l using Nanodrop (Eppendorf Make, Germany). According to the manufacturer's protocol, respective RNA from different treatments was digested using DNase I (Sigma Aldrich, USA). Further, the quality of RNA was determined by measuring the absorbance value at an A260/A280 ratio. RNA was converted to cDNA with the ThermoFischer Scientific-RevertAid First Strand cDNA Synthesis Kit (cat. # K1622). A ratio of 1.8 + 0.2 indicated the best quality of nucleic acid. The cDNA was diluted 10-fold and used for qRT-PCR analysis. It was performed in a BIO-RAD CFX manager system. The reaction mixture for qRT-PCR comprised 3 μl of cDNA template, 10 μl of SYBR Green master mix (KAPA SYBR@FAST for LightCycler 480, Cat-KK4610), 0.8 μl of 10 μM forward primer, and 0.8 μl of 10 μM reverse primer. The final volume was made up to 20 μl using nuclease free water. The PCR program included denaturation at 95°C for 10 min, amplification for 40 cycles at 95°C for 30 s, 60°C for 30 s, and 72°C for 30 s. It was followed by standard melting temperature analysis. The major defense gene transcripts assessed for the induction of resistance response against Foc KP infection were WRKY33, mitogen-activated protein kinase (MAPK), chitin elicitor receptor kinase (CERK 1), lipoxygenase (LOX), and phenylalanine ammonia lyase (PAL). For each defense gene expression study, three biological replicates and two technical replicates were maintained throughout the study. The fold changes in gene expression were calculated using the formula ΔΔCt = Δ Ct sample– Δ Ct reference. The relative fold changes in the transcript level were represented graphically by converting the ΔΔCT value to 2−ΔΔCt (Livak and Schmittgen, 2001). Statistical analysis for relative fold change was performed using TIBCO Spotfire Analyst version 7.11.1.
Defense-related proteins in banana cv. Karpooravalli (ABB) plantlets that had a predominant role against Foc KP infection were further investigated for their interacting partners using the STRING database (Szklarczyk et al., 2021). Protein sequences of WRKY33, MAPK, CERK1, PAL, and LOX were used as a query against the double haploid Pahang (Musa acuminata) banana genome to understand the interacting protein partners. Information regarding interacting partners was obtained based on text mining, experiments, databases, co-expression, neighborhood, gene fusion, and co-occurrence results. Query protein and their interacting protein domain information in addition to the number of interacting partners belonging to a particular domain in the network were analyzed. A degree-sorted network was constructed using transcription factor, defense related genes, and their interacting proteins. Protein domains of all the interacting partners were analyzed to understand the functional relevance and their association with query protein. A medium confidence level of 0.40 and minimum of 10 interactors were used as parameters for the construction of the network.
Analysis of protein-protein interaction network between WRKY33, MAPK, CERK1, PAL, and LOX proteins was obtained using the STRING database. It was exported to Cytoscape 3.9 version for analysis. Using STRING and enrichment map applications in Cytoscape, STRING enrichment was performed and a map was constructed. Functional enrichment was performed by merging all the five proteins with their interacting partners.
Amplification of internal transcribed spacers of eight different isolates of Foc for ITS 1 and ITS 4 regions with specific primers yielded an expected amplicon size of 560 bp. Nucleotide sequences of amplicons pertaining to eight different isolates confirmed the identity of Foc. The sequences subjected to multiple alignments were submitted to GenBank and were provided with accession numbers, viz., Foc KP MW436477, Foc KP MW 436476, Foc NP1 MW436482, Foc NP2 MW436483, Foc KP2 MW436485, Foc RS2 MW436581, Foc RS3 MW436484, and Foc RS1 MW43658. Phylogenetic analysis of Foc isolates revealed the presence of three major clusters. Cluster 1 comprised Foc KP MW 436476, Foc KP MW436477, and Foc NP1 MW436482. Cluster 2 had five isolates including Foc NP2 MW436483, Foc KP2 MW436485, Foc RS2 MW436581, Foc RS3 MW436484, and Foc RS1 MW43658. Cluster 3 comprised Fusarium oxysporum f.sp. lycopersici (Fol) as an out group (Supplementary Figure S1). Based on the pathogenicity study, Foc KP was most virulent compared to other isolates. Sequencing of Six13c for the isolate Foc KP confirmed the presence of race 4 bearing the accession number MW323435. It had similarity with Foc isolate BRIP62892 bearing the accession number KX435021 and also matched with VCG group 0122 pertaining to the Cavendish group (AAA) of Philippines origin. Hence, Foc KP was used throughout the study.
Bacillus velezensis YEBBR6 inhibited the mycelial growth of Foc KP up to 63% compared to untreated control (Supplementary Figure S2). Other bacterial endophytes, B. albus YEBN2, A. xylosoxidans YEBRH2, Beijerinckia fluminensis YEBRT4, Acinetobacter refrigeratoris YEBFR1, and B. endophyticus YEBFL6 were not equally effective as B. velezensis YEBBR6 in the suppression of mycelial growth of Foc KP in vitro.
Fifteen bioactive metabolites were characterized through GC/MS from the zone of inhibition during the ditrophic interaction between B. velezenzis YEBBR6 and Foc KP. They included dihydro acridine, nonanol, hexadecanoic acid, oleic acid, clindamycin, 5-hydroxymethylfurfural, azulene, 3 amino-4 hydroxy phenyl sulfone, campholic acid, procyclidine, linoelaedic acid, acetylvaleryl, allobarbital, aminomorpholine, and 3-thiazolidine carboxamide (Supplementary Figure S3 and Supplementary Table S1). Six bioactive metabolites were produced by B. velezensis YEBBR6 in the absence of Foc KP. They were identified as 1,3-propanediol, clindamycin, 4H-pyran, pentanoic acid, acetaldehyde, and hexadecanoic acid (Supplementary Figure S4 and Supplementary Table S2). Bioactive metabolites produced by Foc KP were identified as 2-propen-1-ol, 3H-pyrazol-3-one, hexanoic acid, cyclohexan, 1H-azonine, trioxsalen, and butanamide (Supplementary Figure S5 and Supplementary Table S3). The Venn diagram of differentially expressed bioactive metabolites during the interaction of bacterial endophyte B. velezensis YEBBR6, either with Foc KP or without Foc KP, and Foc KP alone revealed that B. velezensis YEBBR6 produced six bioactive metabolites. But, during the interaction of B. velezensis YEBBR6 with Foc KP, 15 bioactive metabolites were produced. Comparison of bioactive metabolites between B. velezensis YEBBR6 alone and B. velezensis YEBBR6 with Foc KP revealed the production of two bioactive metabolites in common, viz., hexadecanoic acid and clindamycin (Figure 1).
Figure 1. Comparative distribution of VOCs/NVOCs produced by B. velezensis YEBBR6, B. velezensis YEBBR6 + Foc KP, and + Foc KP alone.
Micropropagated banana plantlets treated with B. velezensis YEBBR6, B. albus YEBN2, A. xylosoxidans YEBRH2, Beijerinckia fluminensis YEBRT4, Acinetobacter refrigeratoris YEBFR1, and B. endophyticus YEBFL6 increased plant growth parameters (Figure 2). Among the bacterial endophytes, B. velezensis YEBBR6 significantly increased pseudostem height (38.63 cm), pseudostem width (5.9 cm), leaf area (1.290 m2), root length (54.75 cm), and leaf emergence rate (Phyllochron) (6.0), compared to 11.21 cm, 3.2 cm, 0.09 m2, 36.1 cm, and 12.0 in control plants, respectively (Figure 3A and Supplementary Figures S6–S10). B. velezensis YEBBR6-treated banana plants also enhanced the photosynthetic rate to 24.61 mol m−2 s−1 against 13.09 mol m−2 s−1 in non-bacterized control plants. Further, transpiration rate and stomatal conductance in B. velezensis-treated banana plants was 3.95 mol.cm−2 s−1, 1.01 mmol m−2 s, while it was 2.64 mol.cm−2 s−1, 0.06 mmol m−2 s in non-bacterized control plants. Biohardened banana plants had higher levels of total chlorophyll (1.29 mg/g1), chlorophyll stability index (88.6%), and relative water content (83.3%) than non-bacterized control plants (Supplementary Table S4).
Figure 2. Phenotypic response and plant growth promotion of micropropagated banana plantlets biohardened with bacterial endophytes.
Figure 3. (A) Plant growth promotion of micropropagated banana plantlets biohardened with Bacillus velezensis YEBBR6. (B) Effect of Fusarium wilt on biohardened micropropagated Karpooravalli banana plantlets with Bacillus velezensis YEBBR6 against Foc in pot culture.
Banana plantlets biohardened with B. velezensis YEBBR6, B. albus YEBN2, Achromobacter xylosoxidans YEBRH2, Beijerinckia fluminensis YEBRT4, Acinetobacter refrigeratoris YEBFR1, and B. endophyticus YEBFL6 against Foc KP indicated that banana plantlets biohardened with B. velezensis at 10 ml/plant had zero incidence of wilt. But, the banana plantlets biohardened with B. albus and Acinetobacter refrigeratoris had 10 % wilt against 30 % in banana plants biohardened with B. endophyticus. However, 100 % wilt incidence was observed in inoculated control (Figure 3B and Supplementary Table S5). As B. velezensis YEBBR6 was effective in the suppression of Fusarium wilt, it was subsequently used for further studies.
Analysis of the roots of banana plantlets biohardened with B. velezensis YEBBR6 using scanning electron microscopy confirmed the colonization of bacterial cells on the root surface. Agglomerates of bacterial cells resulted in the formation of biofilm on the root surface (Figure 4A). Besides, the roots of banana plantlets biohardened with B. velezensis YEBBR6 followed by challenge inoculation with Foc KP witnessed hyperparasitism of Foc KP mycelium and microconidia by the bacterial cells of B. velezensis (Figures 4B,C). However, in pathogen-inoculated control, proliferation of microconidia was noticed along the root zone of banana plantlets (Figure 4D).
Figure 4. (A) Colonization of Bacillus velezensis YEBBR6 in the root zone of biohardened banana plantlets. (B,C) Colonization of Bacillus velezensis YEBBR6 in the mycelium and microconidia of Foc KP on biohardened banana plantlets after challenge inoculation with Foc KP. (D) Colonization of micro and macroconidia in infected plantlets after challenge inoculation with Foc KP.
Biohardening of banana plantlets with B. velezensis YEBBR6 challenged with or without Foc KP altered the expression of the WRKY transcription factor, MAPK, chitin elicitor receptor kinase, lipoxygense, and PAL genes responsible for plant defense. Irrespective of different treatments, the WRKY 33 transcript was downregulated in all the treatments at 0 h. The transcription rate of the WRKY 33 gene was affected immediately after inoculation with Foc KP and in biohardened plants. The level of the transcript in Foc KP-inoculated control increased after 24 h and declined after 48 and 72 h. However, the transcript of WRKY 33 was upregulated in banana plantlets biohardened with B. velezensis at 24, 48, and 72 h after treatment. Interestingly, a 1.87-fold increase of the WRKY 33 gene transcript was observed in banana plantlets biohardened with B. velezensis YEBBR6 challenged with Foc KP. But, in untreated healthy control, upregulation of WRKY 33 (0.33-fold) was noticed only at 72 h (Figure 5A).
Figure 5. (A) Expression pattern of WRKY 33 transcription factor in biohardened banana cv. Karpooravalli (ABB) under mono, di, and tritrophic interactions at different time periods. (B) Expression pattern of MAPK in biohardened banana cv. Karpooravalli (ABB) under mono, di, and tritrophic interactions at different time periods. (C) Expression pattern of CERK 1 in biohardened banana cv. Karpooravalli (ABB) under mono, di, and tritrophic interactions at different time periods. (D) Expression pattern of LOX in biohardened banana cv. Karpooravalli (ABB) under mono, di, and tritrophic interactions at different time periods. (E) Expression pattern of PAL in biohardened banana cv. Karpooravalli (ABB) banana under mono, di, and tritrophic interactions at different time periods.
The expression level of MAPK transcripts varied between different treatments. Banana plantlets biohardened with B. velezensis YEBBR6 challenged with Foc KP increased the expression of MAPK transcripts up to 2.32-fold after 72 h in comparison with biohardened plants that were not challenged with Foc KP. Besides, only a 0.69-fold change of the WRKY 33 transcript was noticed in pathogen-inoculated control after 48 h and it decreased further after 72 h of inoculation (Figure 5B).
Induction of the chitin elicitor receptor kinase (CERK 1) transcript associated with innate immune response was initiated after 24 h of inoculation with pathogen Foc KP. However, upregulation was more pronounced after 72 h in plantlets biohardened with B. velezensis YEBBR6 coupled with challenge inoculation of Foc KP. The expression of the CERK1 transcript was 2.2-fold higher than in biohardened plants that had not been inoculated with Foc KP. Furthermore, the expression level of CERK1 transcripts was reduced in untreated healthy control compared to pathogen-inoculated and biohardened plantlets challenged with Foc KP (Figure 5C).
Lipoxygenase (LOX) is a key enzyme involved in the induction of the immune response in plants, therefore attempts were made to understand the ability of B. velezensis YEBBR6 to modulate immune response against Foc KP. Biohardened plants challenged with Foc KP increased the transcript level of fatty acid dioxygenase LOX up to 2.6-fold after 72 h of inoculation. The expression of the LOX transcript was 1.6-fold greater in B. velezensis YEBBR6 and was downregulated after 72 h. Comparison on the expression of the LOX transcript in inoculated control reflected a 1.7-fold change after 72 h, while in healthy control, there was no increase in the LOX gene transcripts (Figure 5D).
Assessing the expression of phenylalanine ammonia lyase (PAL) revealed a significant increase of PAL transcripts in B. velezensis YEBBR6 biohardened banana plantlets challenged with Foc KP. After 48 h of challenge inoculation with Foc KP, the level of induction of the PAL transcript was 1.9 times higher than other treatments. After 48 h, the PAL transcript in Foc KP-inoculated control was observed only up to 0.6-fold, and decreased after 72 h. However, the activity of PAL was downregulated in healthy control (Figure 5E).
STRING analysis was performed to understand the functional association of defense-related genes with other proteins and their conserved domains. From our analysis, most of the interacting proteins for each query protein had similar conserved domains and were clustered together in the network. Protein sequences of defense-related genes were used as input to retrieve the interacting partners based on the double haploid Pahang (Musa acuminata) banana genome. Details on the query protein and their interacting protein domain and number of interacting partners in the network are listed in Supplementary Table S6. The degree-sorted network constructed using transcription factor and defense-related genes and their interacting proteins are illustrated in Figure 6.
Protein-protein interaction of WRKY transcription factor indicated the involvement of a large protein family with diverse functions. They were expressed in response to pathogens, elicitors, and defense-related phytohormones such as salicylic acid (SA) or jasmonic acid (JA). Protein-protein interaction of MAPK revealed that it belongs to the kinase family and interacted with G protein domains and protein tyrosine phosphatase domains similar to chitin elicitor receptor kinase 1-like protein. Besides, functional domains of five out of ten interacting proteins were uncharacterized in the given network. CERK 1 protein pertaining to the protein kinase family protected plants at multiple layers against invading pathogens by conferring PAMP-triggered immunity (PTI) or effector-triggered immunity (ETI). Regardless, CERK proteins interacted with the LysM domain and guanine nucleotide-binding domain (Supplementary Table S6). Protein interaction of LOX indicated that it interacted with proteins containing LOX, phospholipase, and PLAT/LH2 conserved domains. These proteins were associated with membrane or lipid-associated proteins. Similarly, protein-protein interaction of PAL reflected that chalcone synthase (CHS) was essential for the formation of 4,2′, 4′,6′-tetrahydroxychalcone, responsible for the biosynthesis of anthocyanin pigments, anti-microbial phytoalexins, and flavonoid inducers of Rhizobium nodulation genes. Nonetheless, stilbene synthases (STSs) occurred in a limited number of unrelated plants and synthesized the backbone of stilbene phytoalexins that have antifungal properties and contribute to pathogen defense. Despite the other genes, PAL also induced defense against pathogens by triggering other enzymes linked with different pathways.
Merging of WRKY33, MAPK, CERK1, PAL, and LOX resulted in the formation of an enlarged network comprising of 52 nodes. Three isoforms of guanine nucleotide-binding proteins interacted commonly with CERK and MAPK protein targets. Apart from this, there was no common interacting proteins between the five protein targets. Functional enrichment analysis resulted in the formation of more than five clusters. A heat cluster map was generated based on the functional relevance of each of the proteins. In the enrichment figure, each cluster was linked with the query protein to indicate the commonality and the difference observed with the five query proteins and their interaction partners. Based on the enrichment map, domain, and pathway information of five proteins, CERK1 was linked with the LysM domain and guanine nucleotide-binding protein domain. LOX was linked with linoleic acid, alpha-linolenic acid, arachidonic acid metabolism, ether lipid metabolism, glycerophospholipid metabolism, lipoxygenase, phospholipase, and PLAT/LH2 conserved domains. The MAPK gene was associated with G protein domains and protein tyrosine phosphatase domains. While, PAL was coordinated with AMP binding, chalcone synthase, polyketide synthase, and thiolase-like domains. The WRKY transcription factor was associated with the VQ5 domain and WD40 repeat containing domain (Figure 7).
Figure 7. Functional enrichment analysis of defense genes in biohardened Karpooravalli (ABB) banana plantlets.
Fusarium wilt of banana, caused by Fusarium oxysporum f. sp. cubense (Foc), is one of the most devastating diseases hindering the banana industry. A recent outbreak of Foc tropical race 4 (Foc TR4) can infect and destroy almost all banana cultivars. Hence, the present study, using molecular approaches to understand the versatile nature and distribution of Foc races infecting banana in Tamil Nadu province of South India through ITS 1 and 4 and Six 13c, revealed the presence of race 4 (Foc KP–MW323435). Likewise, ITS region has been used as a molecular marker to confirm the identity of Foc-infecting Musa spp. (ABB) in Southern Mexico (Leong et al., 2009; Maldonado-Bonilla et al., 2019). Further, in the present study, sequencing of Six 13c-343 for the isolate Foc KP confirmed the presence of race 4 bearing the accession number MW323435. Sequences of the isolate Foc KP had the similarity with Foc isolate BRIP62892 bearing the accession number KX435021. Further, it also matched with VCG group 0122 pertaining to the Cavendish (Musa AAA) group of banana with Philippines origin (Czislowski et al., 2018; Carvalhais et al., 2019). Similarly, Wong et al. (2019) confirmed the presence of Foc TR4 in Peninsular Malaysia based on transcription elongation factor (TEF-1) and reported the shift in genetic variability among Foc isolates. Despite the existence of different races, management of race 4 remains a challenging task. Though fungicides are effective, usages of fungicides are discouraged due to environmental concern and resistance development among plant pathogens (Raza et al., 2017). Moreover, none of the fungicides confer 100 % protection against Foc. Due to the detrimental effect of chemical fungicides on the environment, human health, and other living organisms, researchers have focused on discovering potential biocontrol candidates as a viable alternative for plant disease management (Syed Ab Rahman et al., 2018).
Considering this critical situation, structuring the rhizosphere and endosphere with potential bacterial antagonists in the susceptible banana cultivars will pave the way for the management of Foc. Among all bacterial endophytes, the Bacillus species were exploited largely for suppression of soil-borne pathogens around the globe due to rapid growth and the ability to synthesize a large number of secondary metabolites which play a key role in antibiosis against deleterious microorganisms (Radhakrishnan et al., 2017; Fira et al., 2018; Aloo et al., 2019). Besides, in the recent past, biological control of plant diseases has gained popularity as an eco-friendly disease management strategy.
Based on the significance in the management of Fusarium wilt of banana, our finding has furthered the introduction of a potential endophyte B. velezensis YEBBR6 with multifaceted attributes contributing to the suppression of Foc KP. Similarly, the versatile nature of antifungal secondary metabolites from endophytic Brachybacterium paraconglomeratum isolated from the resistant cultivar YKM5 was also responsible for the suppression of Foc under in vitro conditions (Saravanan et al., 2021a). The VOCs and NVOCs are also referred to as small signaling molecules (SSMs), which are involved in cellular crosstalk. They play a significant role in competition, synergistic interaction, and communication (Adnani et al., 2017). SSMs from the antagonistic microflora also promote the growth of symbionts and inhibit plant pathogens (Khalid and Keller, 2021). Like the cellular crosstalk mediated by small signaling molecules between the beneficial microbiome and inimical microbes dwelling in the rhizosphere, small signaling molecules were also induced during the interaction between the fungal pathogen and bacterial antagonist in vitro. Likewise, co-culturing of B. velezensis YRBBR6 along with Foc KP also induced the secretion of secondary metabolites, viz., dihydroacridine, nonanol, hexadecanoic acid, oleic acid, clindamycin, 5-hydroxymethylfurfural, azulene, 3 amino-4 hydroxy phenyl sulfone, campholic acid, procyclidine, linoelaedic acid, acetylvaleryl, allobarbital, aminomorpholine, and 3-thiazolidine carboxamide. Gatasheh et al. (2017) reported that dihydroacridine disrupted DNA synthesis and served as a DNA intercalating agent in several microorganisms leading to broad-spectrum antimicrobial activity. Mohamad et al. (2018) explained that B. atrophaeus strain XEGI50 inhibited the mycelial growth of V. dahliae by producing 13 putative compounds, including 1,2-benzenedicarboxylic acid, bis (2-methylpropyl) ester 9,12-octadecadienoic acid (Z, Z)-, methyl ester 9-octadecenoic acid, methyl ester decanedioic acid, bis(2-ethylhexyl) ester, and hexadecanoic acid. Walters et al. (2004) reported that fatty acids, viz., linolenic acid, linoleic acid, erucic acid, and oleic acid promoted plant growth and possessed antifungal action against Rhizoctonia solani, Pythium ultimum, and Pyrenophora avenae. The antifungal activity of nonanol produced by endophytic B. velezensis ZSY-1 was also reported by Gao et al. (2017) against A. solani and B. cinerea. Zhang et al. (2017) reported the broad spectrum antimicrobial activity of a-phellandrene and nonanal against Penicillium cyclopium. Further, Guay (2007) reported the antimicrobial action of clindamycin against different microbes. Abd Alhameed et al. (2020) reported the antimicrobial activity of thiazolidine-2,4-dione carboxamide against bacteria and fungus. To our surprise, B. velezensis YRBBR6 also produced dihydroacridine, clindamycin, and nonanol bestowed with antifungal action against Foc KP. Thus, the bacterial endophyte B. velezensis YRBBR6 is unique in the sense that it produced novel biomolecules responsible for the suppression of mycelial growth of Foc KP, which has not been reported earlier by other researchers for the management of Foc. Hence, it is hypothesized that an array of secondary metabolites produced by B. velezensis YRBBR6 might be responsible for the suppression of Foc KP.
Endophytic B. velezensis YRBBR6 not only possessed antifungal property but also promoted plant growth. This was also endorsed by the research findings of Compant et al. (2016) who reported that the bacterial endophytes improved plant health and growth through a variety of mechanisms, including phytohormone synthesis, nitrogen fixation, phosphate solubilization, stimulation of defense responses, and reduction of abiotic stress by lowering ethylene levels. Yuan et al. (2013) reported that B. amyloliquefaciens strain NJN-6 served as a biofertilizer and promoted the growth of micropropagated banana plants treated with B. amyloliquefaciens NJN-6. Biohardening of banana cv. Karpooravalli (ABB) plants with B. velezensis (YEBR6) promoted plant growth through the increase in pseudostem height, width, leaf area, root length, and emergence of new leaves. The research finding of Gamez and his associates emphasized that the banana plants inoculated with rhizobacteria increased plant height, leaf number, leaf area, pseudostem thickness, root and shoot fresh weight, and root and shoot dry weight (Gamez et al., 2019). Micropropagated banana plantlets treated with Bacillus and Pseudomonas promoted plant growth by increasing pseudostem height, width, number of leaves, leaf area, and yield parameters (Kavino et al., 2011). Kavino et al. (2014) also observed a significant difference in phyllochrons in biohardened banana plants compared to untreated control. Further, Ajit kumar et al. (2020) also reported that bacterial endophytes improved pseudostem height, pseudostem width (diameter), number of roots, and total number of leaves in banana plants. Rajamanickam et al. (2018) recorded increased growth parameters in biohardened banana plants compared to untreated control. Thus, the phytobiome plays a key role in improving plant health. Colonization of the endosphere and rhizosphere by bacterial endophytes enhanced plant growth by mobilizing nitrogen, production of phytohormones, acquisition of nutrients, and also conferred resistance to biotic and abiotic stresses (Kandel et al., 2017). Thus, to harness the potential benefits mediated through endophytes, colonization of the rhizosphere and endosphere is very crucial. Successful colonization of the rhizoplane by bacterial antagonists is a prerequisite for biocontrol and plant growth promotion (Gao et al., 2016; Kang et al., 2019). Considering the significance of colonization by endophytes, the present investigation confirmed the colonization of endophytic B. velezensis YEBBR6 on the rhizosphere. Similarly, scanning electron microscopy analysis of a cucumber root surface applied with B. amyloliquefaciens UCMB5113 revealed the colonization of bacterial cells and formation of biofilm leading to plant growth promotion (Palmqvist et al., 2015). In corroboration with our findings, micropropagated banana plantlets inoculated with B. velezensis RFP-N67 colonized roots xylem cells in a successful manner. On the other hand, it also entered inside the root at a higher population density of bacterial cells. Likewise, we could observe the colonization of the banana pseudostem by endophytic isolate YEBBR6 through SEM. It was also speculated that B. velezensis RFP-N67 proliferated normally in banana to impose their biocontrol functions, and in particular during the presence of Foc, antagonistic bacterial isolate RFP-N67 can quickly inhibit the growth of the pathogen (He et al., 2021). Similar responses were also induced by the antagonistic bacterial endophyte in the presence of Foc KP responsible for quenching the infection of the wilt pathogen. It was also witnessed through the hyperparasitic behavior of the antagonistic bacteria B. velezensis YEBBR6 in the micropropagated banana cv. Karpooravalli (ABB) plantlets through scanning electron micrographs.
As B. velezensis YEBBR6 promoted plant growth, we further focused our studies to assess the impact of the bacterial antagonist on physiological attributes of banana plantlets. Physiological attributes contribute toward plant defense and serve as the medium of interaction with the environment and climatic conditions. These attributes are linked with the physiological development associated with cellular processes including transpiration, photosynthesis, stomatal conductance, relative water content, and regulation of plant hormones (Shah et al., 2020). In our study, micropropagated banana plants treated with B. velezensis YEBBR6 increased major physiological parameters including photosynthetic rate, stomatal conductance, relative water content, and chlorophyll stability index compared to untreated control. Similarly, Shamsuddin et al. (2000) reported that inoculation of banana plantlets with PGPR had the highest photosynthetic rate and stomatal conductance. Besides, it also increased stomatal conductance and lowered proline concentration in leaves of banana seedling grown under abiotic stress (Shamsuddin et al., 1999). Similarly, biohardened banana plants with B. velezensis YEBBR6 also increased stomatal conductance.
Soil application of B. amyloliquefaciens NJN6 to banana plantlets increased the population density of beneficial rhizomicrobiome and thus aided in the management of Fusarium wilt by decreasing colonization of Foc in the banana rhizosphere (Xue et al., 2015). In vitro bacterization of tissue-cultured banana plantlets with endophytic B. subtilis EPB56 and EPB10 reduced Fusarium wilt by 78 % compared to pathogen-inoculated control (Kavino and Manoranjitham, 2018). B. velezensis isolates (Y6 and F7) enhanced the antagonistic activity against banana Fusarium wilt (Cao et al., 2018). Combined application of B. velezensis H-6 with acid soil ameliorant (ASA) suppressed the incidence of Foc race 4 up to 63.3% to 66.7%. Besides, it also enhanced growth promotion in banana plants (Huang et al., 2019). Furthermore, Wang et al. (2013) reported that B. amyloliquefaciens W19 acted in synergy with organic fertilizer to reduce the incidence of Fusarium wilt other than plant growth promotion. Hitherto, biohardening of banana cv. Karpooravalli (ABB) with endophytic B. velezensis YEBBR6 completely protected the plantlets from the establishment of the host pathogen relationship of Foc KP compared to the other bacterial endophytes investigated in the present study. Bacterial endophyte B. velezensis YEBBR6 not only suppressed the host pathogen relationship of Foc KP, but also reduced mycelial proliferation in vitro and promoted plant growth. Hence, attempts were also made to understand the regulation of transcription factors and defense genes expressed during mono, di, and tritrophic interactions with B. velezensis YEBBR6 and Foc KP in biohardened banana plantlets. Bacterial endophytes can induce immune response in plants by its macromolecules and MAMP molecules via host signals (Wei et al., 1991). Profiling the expression in biohardened micropropagated banana cv. Karpooravalli (ABB) with B. velezensis YEBBR6 had a clear upregulation pattern of WRKY 33 transcription factor, PAL, LOX, MAPK, and CERK 1 in biohardened plants challenged with Foc KP compared to inoculated control and untreated control plants. Transcription parameters regulate a broad range of signal transduction pathways with various tasks, and thus play a key role in the induction of plant defense. WRKY, being a group of transcription regulators in plants, can bind to box in promoters of target genes to regulate transcription (Eulgem et al., 2000). WRKY transcription factors also coordinate a variety of signaling pathways and have a crucial regulatory role in plant defense responses (Zhang et al., 2019). They also regulate pathogen-associated molecular pattern-triggered immunity (PTI) and effector-triggered immunity (Chen et al., 2019).
WRKYs also interact with mitogen-activated protein kinase (Rushton et al., 2010; Mao et al., 2011), MAP kinase kinase kinase (MEKK) (Guan et al., 2014), calmodulin (Rushton et al., 2010), and histone deacetylases (HDAs). Zhang et al. (2019) recorded the expression of seven different WRKY genes, including WRKY4 (Ma10_g03630), WRKY22 (Ma10_g06870), WRKY25 (Ma06_g34370), and WRKY26 (Ma03_g09270, Ma06_g01150, Ma08_g01650, and Ma11_g18140) during Foc infection and suggested that expression of these WRKY genes might be responsible for the constitutive defense mechanism. Thus, the multiple fold increase of WRKY 33 transcription factor in bacterized banana cv. Karpooravalli (ABB) plants by B. velezensis YEBBR6 might have triggered the constitutive defense response against Foc KP. In agreement with our finding, Vanthana et al. (2019) reported that MAMP molecules of B. velezensis VB7 increased the expression of the WRKY gene in tomato plants compared to control against GBNV. Next to the WRKY transcription factor, phosphorylation of appropriate protein substrates is highly essential to catalyze the expression of defense genes and regulation of cell functions in the midst of biotic and abiotic stress through the association of MAPKs, one of the largest group of transferases (Onyilo et al., 2017; Xu et al., 2017; Jagodzik et al., 2018; Vanthana et al., 2019). In addition, MAPK cascades play an important role in signal transduction and regulate crosstalk between important hormonal pathways including auxin (AUX), abscisic acid (ABA), jasmonic acid (JA), salicylic acid (SA), ethylene (ET), brassinosteroids (BR), and gibberellins (GA) (Mishra et al., 2006; Rodriguez et al., 2010; Lu et al., 2015). Furthermore, cascades of MAPK are also involved in regulation of signaling related to multiple defense responses, defense hormones, reactive oxygen species (ROS) generation, stomatal closure, defense gene activation, phytoalexin biosynthesis, cell wall strengthening, and hypersensitive response (HR) cell death (Meng and Zhang, 2013). Considering the significant impact of MAPK, our focus on understanding the regulation of MAPK in B. velezensis YEBBR6-treated banana plants challenged with Foc KP confirmed a 2.42-fold increased expression of the MAPK gene. McNeece et al. (2019) also reported the co-expression of five different types of defense genes related to MAPK influencing PTI and ETI against plant pathogenic Fusarium species.
Despite the induction of WRKY 33 and MAPK defense genes, PAL, LOX, and CERK 1 transcripts were also increased in B. velezensis-treated banana cv. Karpooravalli (ABB) plantlets compared to in untreated control. PAL, being the first enzyme involved in the phenylpropanoid pathway during biotic and abiotic stress, catalyzes the first step in the phenylpropanoid pathway and regulates defense signaling (Lyne et al., 1976). It is also involved in the conversion of phenylalanine to trans-cinnamic acid which is the entry step for channeling carbon from primary metabolism into phenylpropanoid secondary metabolism in plants (Campbell and Ellis, 1992; Ritter and Schulz, 2004). All these pathways bestowed the metabolites with antifungal activity. Apart from the induction of metabolites with antifungal activity, PAL interacts with chalcone synthase, polyketide synthase, and stilbene synthases which are responsible for antifungal action (Schanz et al., 1992; Okada et al., 2004; Zhu et al., 2004). Induction of PAL in banana plants suppressed Foc infection (Wang et al., 2016). Application of bacterial endophytes against Foc accumulated defense-related enzymes such as PO, PPO, and PAL (Ajit kumar et al., 2020). Thus, based on protein-protein interaction, increase in the expression of PAL transcripts in banana plantlets biohardened with B. velezensis YEBBR6 against Foc KP might have simultaneously induced various pathways and proteins responsible for the suppression of Fusarium wilt via the induction of SAR. Interestingly, banana plantlets of banana cv. Karpooravalli (ABB) biohardened with B. velezensis YEBBR6 against Foc KP also increased the transcript levels of CERK 1 and LOX genes. CERK 1, being a cell surface receptor, plays a pivotal role in the induction of innate immunity against biotic and abiotic stresses (Shinya et al., 2014). Expression of CERK 1 also can co-express LysM domain-based defense genes contributing to the immune response against Foc KP. Besides, CERK 1 protein and its interacting partners recognize pathogen entry and mediate signaling events leading to suppression of the pathogen. Induction of LOX in biohardened banana cv. Karpooravalli (ABB) plantlets by B. velezensis and Foc KP might have promoted plant growth and plant defense. Hydroperoxidation products of the LOX pathway are responsible for seed germination, plant growth, development, plant senescence, and plant defense against insect and disease attacks.
Ultimately, the present investigation emphasized that biohardening of micropropagated banana cv. Karpooravalli (ABB) with B. velezensis YEBBR6 promoted plant growth and suppressed the infection of Fusarium wilt through the induction of WRKY 33 transcription factor, MAPK, and defense genes including PAL, LOX, and CERK 1. Further protein-protein interaction also confirmed the co-expression of different domains involved in innate immunity and growth promotion. Subsequently, B. velezensis YEBBR6 also produced antifungal metabolites clindamycin and nonanol which are responsible for inhibiting the mycelial growth of Foc KP. Thus, biohardening with multifaceted B. velezensis YRBBR6 can be explored for the management of Fusarium wilt of banana.
Investigation on biohardening of the micropropagated susceptible cultivar cv. Karpooravalli (Pisang Awak ABB) with bacterial endophyte B. velezensis YEBBR6 derived from the resistant genotype YKM5 on reprogramming of innate immunity against Foc KP revealed the versatile production of metabolically active biomolecules contributing to the suppression of Foc KP. On the other hand, biohardening enhanced the growth promotion of banana plantlets by increasing the plant height and production of number of leaves in comparison with untreated control. Scanning electron micrographs also confirmed the colonization of the rhizoplane by B. velezensis YEBBR6, followed by hyperparasitism of Foc KP in the rhizosphere. Challenge inoculation of biohardened cv. Karpooravalli (Pisang Awak ABB) with Foc KP enhanced the transcript level of WRKY transcription factor, MAPK, and other defense genes including CERK 1, LOX, and PAL. Functional enrichment of different defense genes and transcription factors was linked with different domains responsible for growth promotion, induction of systemic resistance, and systemic-acquired resistance. As a result, the current study has opened up the scope for exploring the immense potential of B. velezensis YEBBR6 to bioharden micropropagated banana plantlets on a commercial scale to create preimmunized seedlings for the management of Foc KP (race 4).
The original contributions presented in the study are included in the article/Supplementary Material, further inquiries can be directed to the corresponding author.
SN conceptualized the research and was associated with technically guiding and executing the research. RS performed lab experiments. NS and VR carried out the bioinformatics analysis. MK and SV coordinated the experiments associated with defense gene expression. MR, AK, SH, and VM edited the manuscript. All authors read and approved the final manuscript.
The authors declare that the research was conducted in the absence of any commercial or financial relationships that could be construed as a potential conflict of interest.
All claims expressed in this article are solely those of the authors and do not necessarily represent those of their affiliated organizations, or those of the publisher, the editors and the reviewers. Any product that may be evaluated in this article, or claim that may be made by its manufacturer, is not guaranteed or endorsed by the publisher.
The authors extend their sincere appreciation to the Department of Plant Pathology, Department of Plant Biotechnology, DBT-BTIS facility at Department of Plant Molecular Biology and Bioinformatics, Department of Fruit science, Department of Nanoscience and Technology, Tamil Nadu Agricultural University, Coimbatore, Tamil Nadu, India for providing facilities.
The Supplementary Material for this article can be found online at: https://www.frontiersin.org/articles/10.3389/fsufs.2022.845512/full#supplementary-material
Abd Alhameed, R., Almarhoon, Z., Bukhari, S. I., El-Faham, A., de la Torre, B. G., and Albericio, F. (2020). Synthesis and antimicrobial activity of a new series of thiazolidine-2, 4-diones carboxamide and amino acid derivatives. Molecules 25, 105. doi: 10.3390/molecules25010105
Adnani, N., Chevrette, M. G., Adibhatla, S. N., Zhang, F., Yu, Q., Braun, D. R., et al. (2017). Coculture of marine invertebrate-associated bacteria and interdisciplinary technologies enable biosynthesis and discovery of a new antibiotic, keyicin. ACS Chem. Biol. 12, 3093–3102. doi: 10.1021/acschembio.7b00688
Ajit kumar, S., Bhattacharyya, A., and Baruah, A. (2020). Endophyte mediated activation of defense enzymes in banana plants pre-immunized with covert endophytes. Indian Phytopathol. 73, 433–441. doi: 10.1007/s42360-020-00245-8
Aloo, B. N., Makumba, B. A., and Mbega, E. R. (2019). The potential of Bacilli rhizobacteria for sustainable crop production and environmental sustainability. Microbiol. Res. 219, 26–39. doi: 10.1016/j.micres.2018.10.011
Anupama, N., Murali, M., Jogaiah, S, and Amruthesh, K. N. (2014). Crude oligosacchraides from Alternaria solani with Bacillus subtilis enhance defense activity and induce resistance against early blight disease of tomato. Asian J. Sci. Technol. 5, 412–416. Available online at: https://www.fao.org/fileadmin/templates/banana/documents/Docs_Resources_2015/TR4/13ManualFusarium.pdf
Campbell, M. M., and Ellis, B. E. (1992). Fungal elicitor-mediated responses in pine cell cultures: III. Purification and characterization of phenylalanine ammonia-lyase. Plant Physiol. 98, 62–70. doi: 10.1104/pp.98.1.62
Cao, Y., Pi, H., Chandrangsu, P., Li, Y., Wang, Y., Zhou, H., et al. (2018). Antagonism of two plant-growth promoting Bacillus velezensis isolates against Ralstonia solanacearum and Fusarium oxysporum. Sci. Rep. 8, 1–14. doi: 10.1038/s41598-018-22782-z
Carlier, J., De Waele, D., and Escalant, J. V. (2003). “Global evaluation of musa germplasm for resistance to fusarium wilt, Mycosphaerella leaf spot diseases and nematodes: in-dept evaluation,” in INIBAP Technical Guidelines 7, eds A. V'ezina and C. Picq (Montpellier: The International Network for the Improvement of Banana and Plantain Arceaux 49 Press), 12.
Carvalhais, L. C., Henderson, J., Rincon-Florez, V. A., O'Dwyer, C., Czislowski, E., Aitken, E. A., et al. (2019). Molecular diagnostics of banana Fusarium Wilt targeting secreted-in-xylem genes. Front. Plant Sci. 10, 547. doi: 10.3389/fpls.2019.00547
Catambacan, D. G., and Cumagun, C. J. R. (2021). Weed-associated fungal endophytes as biocontrol agents of Fusarium oxysporum f. sp. cubense TR4 in cavendish banana. J. Fungi. 7, 224. doi: 10.3390/jof7030224
Cawoy, H., Debois, D., Franzil, L., De Pauw, E., Thonart, P., and Ongena, M. (2015). Lipopeptides as main ingredients for inhibition of fungal phytopathogens by Bacillus subtilis/amyloliquefaciens. Microb. Biotechnol. 8, 281–295. doi: 10.1111/1751-7915.12238
Chen, X., Li, C., Wang, H., and Guo, Z. (2019). WRKY transcription factors: evolution, binding, and action. Phytopathol Res. 1, 1–15. doi: 10.1186/s42483-019-0022-x
Cheng, Y., Zhou, Y., Yang, Y., Chi, Y. J., Zhou, J., Chen, J. Y., et al. (2012). Structural and functional analysis of VQ motif-containing proteins in Arabidopsis as interacting proteins of WRKY transcription factors. Plant Physiol. 159, 810–825. doi: 10.1104/pp.112.196816
Chomczynski, P., and Sacchi, N. (1987). Single-step method of RNA isolation by acid guanidinium thiocyanate-phenol-chloroform extraction. Anal. Biochem. 16, 156–159. doi: 10.1016/0003-2697(87)90021-2
Compant, S., Saikkonen, K., Mitter, B., Campisano, A., and Mercado-Blanco, J. (2016). Editorial special issue: soil, plants and endophytes. Plant Soil. 405, 1–11. doi: 10.1007/s11104-016-2927-9
Czislowski, E., Fraser-Smith, S., Zander, M., O'Neill, W. T., Meldrum, R. A., Tran-Nguyen, L. T., et al. (2018). Investigation of the diversity of effector genes in the banana pathogen, Fusarium oxysporum f. sp. cubense, reveals evidence of horizontal gene transfer. Mol. Plant Pathol. 19, 1155–1171. doi: 10.1111/mpp.12594
Dita, M. A., Pérez-Vicente, L., and Martínez, E. (2014). Inoculation of Fusarium oxysporum f. sp. cubense causal agent of fusarium wilt in banana. Technical manual prevention and diagnostic of Fusarium wilt (Panama disease) of banana caused by Fusarium oxysporum f. sp. cubense. Tropical Race. 4, 55–58.
Eulgem, T., Rushton, P. J., Robatzek, S., and Somssich, I. E. (2000). The WRKY superfamily of plant transcription factors. Trends Plant Sci. 5, 199–206. doi: 10.1016/S1360-1385(00)01600-9
Fira, D., Dimki,ć, I., Beri,ć, T., Lozo, J., and Stankovi,ć, S. (2018). Biological control of plant pathogens by Bacillus species. J. Biotechnol. 285, 44–55. doi: 10.1016/j.jbiotec.2018.07.044
Gamez, R., Cardinale, M., Montes, M., Ramirez, S., Schnell, S., and Rodriguez, F. (2019). Screening, plant growth promotion and root colonization pattern of two rhizobacteria (Pseudomonas fluorescens Ps006 and Bacillus amyloliquefaciens Bs006) on banana cv. Williams (Musa acuminata Colla). Microbiol. Res. 220, 12–20. doi: 10.1016/j.micres.2018.11.006
Gao, S., Wu, H., Yu, X., Qian, L., and Gao, X. (2016). Swarming motility plays the major role in migration during tomato root colonization by Bacillus subtilis SWR01. Biol. Control. 98, 11–17. doi: 10.1016/j.biocontrol.2016.03.011
Gao, Z., Zhang, B., Liu, H., Han, J., and Zhang, Y. (2017). Identification of endophytic Bacillus velezensis ZSY-1 strain and antifungal activity of its volatile compounds against Alternaria solani and Botrytis cinerea. Biol.Control 105, 27–39. doi: 10.1016/j.biocontrol.2016.11.007
Gatasheh, M. K., Kannan, S., Hemalatha, K., and Imrana, N. (2017). Proflavine an acridine DNA intercalating agent and strong antimicrobial possessing potential properties of carcinogen. Karbala Int. J. Mod. Sci. 3, 272–278. doi: 10.1016/j.kijoms.2017.07.003
Griffith, G. W., and Shaw, D. S. (1998), Polymorphisms in Phytophthora infestans: four mitochondrial haplotypes are detected after PCR amplification of DNA from pure cultures or from host lesions. Appl.Environ. Microbiol. 64, 4007–4014. doi: 10.1128/AEM.64.10.4007-4014.1998
Guan, Y., Lu, J., Xu, J., McClure, B., and Zhang, S. (2014). Two mitogen-activated protein kinases, MPK3 and MPK6, are required for funicular guidance of pollen tubes in Arabidopsis. Plant Physiol. 165, 528–533. doi: 10.1104/pp.113.231274
Guay, D. (2007). Update on clindamycin in the management of bacterial, fungal and protozoal infections. Expert Opin. Pharmacother. 8, 2401–2444. doi: 10.1517/14656566.8.14.2401
He, P., Li, S., Xu, S., Fan, H., Wang, Y., Zhou, W., et al. (2021). Monitoring Tritrophic Biocontrol Interactions Between Bacillus spp., Fusarium oxysporum f. sp. cubense, tropical race 4, and banana plants in vivo based on fluorescent transformation system. Front. Microbiol. 3089. doi: 10.3389/fmicb.2021.754918
Huang, J., Pang, Y., Zhang, F., Huang, Q., Zhang, M., Tang, S., et al. (2019). Suppression of Fusarium wilt of banana by combining acid soil ameliorant with biofertilizer made from Bacillus velezensis H-6. Eur. J. Plant Pathol. 154, 585–596. doi: 10.1007/s10658-019-01683-5
Jagodzik, P., Tajdel-Zielinska, M., Ciesla, A., Marczak, M., and Ludwikow, A. (2018). Mitogen-activated protein kinase cascades in plant hormone signaling. Front. Plant Sci. 9, 1387. doi: 10.3389/fpls.2018.01387
Kandel, S. L., Joubert, P. M., and Doty, S. L. (2017). Bacterial endophyte colonization and distribution within plants. Microorganisms 5, 77. doi: 10.3390/microorganisms5040077
Kang, X., Guo, Y., Leng, S., Xiao, L., Wang, L., Xue, Y., et al. (2019). Comparative Transcriptome Profiling of Gaeumannomyces graminis var. tritici in Wheat Roots in the Absence and Presence of Biocontrol Bacillus velezensis CC09. Front. Microbiol. 10, 1474. doi: 10.3389/fmicb.2019.01474
Kavino, M., and Manoranjitham, S. K. (2018). In vitro bacterization of banana (Musa spp.) with native endophytic and rhizospheric bacterial isolates: novel ways to combat Fusarium wilt. Eur. J. Plant Pathol. 151, 371–387. doi: 10.1007/s10658-017-1379-2
Kavino, M., Manoranjitham, S. K., Balamohan, T. N., Kumar, N., Karthiba, L., and Samiyappan, R. (2011), December. Enhancement of growth Panama wilt resistance in banana by in vitro co-culturing of banana plantlets with PGPR endophytes. Int. Symp. Trop. Subtrop. Fruits 1024, 277–282.
Kavino, M., Manoranjitham, S. K., Balamohan, T. N., Kumar, N., Karthiba, L., and Samiyappan, R. (2014). Enhancement of growth and panama wilt resistance in banana by in vitro co-culturing of banana plantlets with pgpr and endophytes. Acta Hortic. 1024, 277–282. doi: 10.17660/ActaHortic.2014.1024.37
Khalid, S., and Keller, N. P. (2021). Chemical signals driving bacterial–fungal interactions. Environ. Microbiol. 23, 1334–1347. doi: 10.1111/1462-2920.15410
Leong, S. K., Latiffah, Z., and Baharuddin, S. (2009). Molecular characterization of Fusarium oxysporum f. sp. cubense of banana. Am. J. Appl. Sci. 6, 1301–1307. doi: 10.3844/ajassp.2009.1301.1307
Liu, Y., Zhang, N., Qiu, M., Feng, H., Vivanco, J. M., Shen, Q., et al. (2014). Enhanced rhizosphere colonization of beneficial Bacillus amyloliquefaciens SQR9 by pathogen infection. FEMS Microbiol. Lett. 353, 49–56. doi: 10.1111/1574-6968.12406
Livak, K. J., and Schmittgen, T. D. (2001). Analysis of relative gene expression data using real-time quantitative PCR and the 2(-Delta Delta C(T)) Method. Methods 25, 402–408. doi: 10.1006/meth.2001.1262.
Lu, K., Guo, W., Lu, J., Yu, H., Qu, C., Tang, Z., et al. (2015). Genome-wide survey and expression profile analysis of the mitogen-activated protein kinase (MAPK) gene family in Brassica rapa. PLoS ONE 10, 0132051. doi: 10.1371/journal.pone.0132051
Lyne, R. L., Mulheirn, L. J., and Leworthy, D. P. (1976). New pterocarpinoid phytoalexins of soybean. J. Chem. Soc. Chem. Comm. 13, 497–498. doi: 10.1039/c39760000497
Maldonado-Bonilla, L. D., Calderón-Oropeza, M. A., Villarruel-Ordaz, J. L., and Sánchez-Espinosa, A. C. (2019). Identification of novel potential causal agents of Fusarium wilt of Musa sp. AAB in southern Mexico. Plant Pathol. Microbiol. 10, 10–24105. doi: 10.35248/2157-7471.10.479
Mao, G., Meng, X., Liu, Y., Zheng, Z., Chen, Z., and Zhang, S. (2011). Phosphorylation of a WRKY transcription factor by two pathogen-responsive MAPKs drives phytoalexin biosynthesis in Arabidopsis. Plant Cell. 23, 1639–1653. doi: 10.1105/tpc.111.084996
McNeece, B. T., Sharma, K., Lawrence, G. W., Lawrence, K. S., and Klink, V. P. (2019). The mitogen activated protein kinase (MAPK) gene family functions as a cohort during the Glycine max defense response to Heterodera glycines. Plant Physiol. Biochem. 137, 25–41. doi: 10.1016/j.plaphy.2019.01.018
Meng, Q., and Hao, J. J. (2017). Optimizing the application of Bacillus velezensis BAC03 in controlling the disease caused by Streptomyces scabies. Biol. Control. 62, 535–544. doi: 10.1007/s10526-017-9799-7
Meng, X., and Zhang, S. (2013). MAPK cascades in plant disease resistance signaling. Annu. Rev. Phytopathol. 51, 245–266. doi: 10.1146/annurev-phyto-082712-102314
Mishra, N. S., Tuteja, R., and Tuteja, N. (2006). Signaling through MAP kinase networks in plants. Arch. Biochem. Biophys. 452, 55–68. doi: 10.1016/j.abb.2006.05.001
Mohamad, O. A., Li, L., Ma, J. B., Hatab, S., Xu, L., Guo, J. W., et al. (2018). Evaluation of the antimicrobial activity of endophytic bacterial populations from Chinese traditional medicinal plant licorice and characterization of the bioactive secondary metabolites produced by Bacillus atrophaeus against Verticillium dahliae. Front. Microbiol. 9, 924. doi: 10.3389/fmicb.2018.00924
Nakkeeran, S., Rajamanickam, S., Saravanan, R., Vanthana, M., and Soorianathasundaram, K. (2021). Bacterial endophytome-mediated resistance in banana for the management of Fusarium wilt. 3 Biotech. 11, 1–13. doi: 10.1007/s13205-021-02833-5
Nakkeeran, S, Vinodkumar, S, Renukadevi, P., Rajamanicam, S., and Jogaiah, S. (2019). “Bioactive molecule from Bacillus spp., an effective tool for plant stress management,” in Bioactive Molecules in Plant Defense. 1–23. Available online at: https://www.springerprofessional.de/bioactive-molecules-from-bacillus-spp-an-effective-tool-for-plan/17190160.
Narasimha Murthy, K., Soumya, K., Udayashankar, A. C., Srinivas, C., and Jogaiah, (2021). “Biocontrol potential of plant growth promoting rhizobacteria (PGPR) against Ralstonia solanacearum: Current and future prospects,” in Biocontrol Agents and Secondary Metabolites. Karnatak University, Dharwad, Karnataka, India, 153–180.
Nel, B., Steinberg, C., Labuschagne, N., and Viljoen, A. (2006). The potential of nonpathogenic Fusarium oxysporum and other biological control organisms for suppressing Fusarium wilt of banana. Plant Pathol. 55, 217–223. doi: 10.1111/j.1365-3059.2006.01344.x
Nelson, P. E., Toussoun, T. A., and Marasas, W. F. (1983). Fusarium species: An Illustrated Manual for Identification. University Park, PA: Pennsylvania State University Press.
Okada, Y., Sano, Y., Kaneko, T., Abe, I., Noguchi, H., and Ito, K. (2004). Enzymatic reactions by five chalcone synthase homologs from hop (Humulus lupulus L.). Biosci. Biotechnol. Biochem. 68, 1142–1145. doi: 10.1271/bbb.68.1142
Onyilo, F., Tusiime, G., Chen, L. H., Falk, B., Stergiopoulos, I., Tripathi, J. N., et al. (2017). Agrobacterium tumefaciens-mediated transformation of Pseudocercospora fijiensis to determine the role of PfHog1 in osmotic stress regulation and virulence modulation. Front. Microbiol. 8, 830. doi: 10.3389/fmicb.2017.00830
Palmqvist, N. G. M., Bejai, S., Meijer, J., Seisenbaeva, G. A., and Kessler, V. G. (2015). Nano titania aided clustering and adhesion of beneficial bacteria to plant roots to enhance crop growth and stress management. Sci. Rep. 5, 1–12. doi: 10.1038/srep10146
Radhakrishnan, R., Hashem, A., and Abd_Allah, E. F. (2017). Bacillus: a biological tool for crop improvement through bio-molecular changes in adverse environments. Front. Physiol. 8, 667. doi: 10.3389/fphys.2017.00667
Rajamanickam, S., Karthikeyan, G., Kavino, M., and Manoranjitham, S. K. (2018). Biohardening of micropropagated banana using endophytic bacteria to induce plant growth promotion and restrain rhizome rot disease caused by Pectobacterium carotovorum subsp. carotovorum. Sci. Hortic. 231, 179–187. doi: 10.1016/j.scienta.2017.12.037
Raza, W., Ling, N., Zhang, R., Huang, Q., Xu, Y., and Shen, Q. (2017). Success evaluation of the biological control of Fusarium wilts of cucumber, banana, and tomato since 2000 and future research strategies. Crit. Rev. Biotechnol. 37, 202–212. doi: 10.3109/07388551.2015.1130683
Ritter, H., and Schulz, G. E. (2004). Structural basis for the entrance into the phenylpropanoid metabolism catalyzed by phenylalanine ammonia-lyase. Plant Cell. 16, 3426–3436. doi: 10.1105/tpc.104.025288
Rodriguez, M. C., Petersen, M., and Mundy, J. (2010). Mitogen-activated protein kinase signaling in plants. Annu. Rev. Plant Biol. 61, 621–649. doi: 10.1146/annurev-arplant-042809-112252
Rushton, P. J., Somssich, I. E., Ringler, P., and Shen, Q. J. (2010). WRKY transcription factors. Trends Plant Sci. 15, 247–258. doi: 10.1016/j.tplants.2010.02.006
Saravanan, R., Nakkeeran, S., Saranya, N., Haripriya, S., Kavino, M., Anandham, R., et al. (2021a). Differential bacterial endophytome in Foc-resistant banana cultivar displays enhanced antagonistic activity against Fusarium oxysporum f . sp. cubense (Foc). Environ. Microbiol.
Saravanan, R., Nakkeeran, S., Saranya, N., Senthilraja, C., Renukadevi, P., Krishnamoorthy, A. S., et al. (2021b). Mining the Genome of Bacillus velezensis VB7 (CP047587) for MAMP genes and non-ribosomal peptide synthetase gene clusters conferring antiviral and antifungal activity. Microorganisms 9, 2511. doi: 10.3390/microorganisms9122511
Schanz, S., Schröder, G., and Schröder, J. (1992). Stilbene synthase from Scots pine (Pinus sylvestris). FEBS Lett. 313, 71–74. doi: 10.1016/0014-5793(92)81187-Q
Shah, A. A., Khan, W. U., Yasin, N. A., Akram, W., Ahmad, A., Abbas, M., et al. (2020). Butanolide alleviated cadmium stress by improving plant growth, photosynthetic parameters and antioxidant defense system of Brassica oleracea. Chemosphere 261, 127728. doi: 10.1016/j.chemosphere.2020.127728
Shamsuddin, Z.H., Mia, M.A.B., Wahab, and Marziah, M. (2000). “Growth and physiological attributes of hydroponically grown bananas inoculated with plant growth promoting rhizobacteria,” in Tropical Plant Biology Research in Malaysia: Fruit and Vegetables: Joint Proceedings of the 11th Malaysian Society of Plant Physiology Conference and 2nd National Banana Seminar (Malaysian Society of Plant Physiology), 324–327.
Shamsuddin, Z. H., Amr, H. G., Mia, M. A. B., Halimi, M. S., Zakaria, W., and Marziah, M. (1999). Symbiotic and associative N2 fixation with vegetable soybean, oil palm and bananas. Biotechnol. Sustain. Utilizat. Biol. Resour. Trop. 14. 102–118.
Shinya, T., Yamaguchi, K., Desaki, Y., Yamada, K., Narisawa, T., Kobayashi, Y., et al. (2014). Selective regulation of the chitin-induced defense response by the Arabidopsis receptor-like cytoplasmic kinase PBL 27. Plant J. 79, 56–66. doi: 10.1111/tpj.12535
Syed Ab Rahman, S. F., Singh, E., Pieterse, C. M. J., and Schenk, P. M. (2018). Emerging microbial biocontrol strategies for plant pathogens. Plant Sci. 267, 102–111. doi: 10.1016/j.plantsci.2017.11.012
Szklarczyk, D., Gable, A. L., Nastou, K. C., Lyon, D., Kirsch, R., Pyysalo, S., et al. (2021). The STRING database in 2021: customizable protein–protein networks, and functional characterization of user-uploaded gene/measurement sets. Nucleic Acids Res. 49, D605–D612. doi: 10.1093/nar/gkab835
Tamura, K., Dudley, J., Nei, M., and Kumar, S. (2007). MEGA4: molecular evolutionary genetics analysis (MEGA) software version 4.0. Mol. Biol. Evol. 24, 1596–1599. doi: 10.1093/molbev/msm092
Vanthana, M., Nakkeeran, S., Malathi, V. G., Renukadevi, P., and Vinodkumar, S. (2019). Induction of in planta resistance by flagellin (Flg) and elongation factor-TU (EF-Tu) of Bacillus amyloliquefaciens (VB7) against groundnut bud necrosis virus in tomato. Microb. Pathog. 137, 103757. doi: 10.1016/j.micpath.2019.103757
Vinodkumar, S., Nakkeeran, S., Renukadevi, P., and Malathi, V. G. (2017). Biocontrol potentials of antimicrobial peptide producing Bacillus species: multifaceted antagonists for the management of stem rot of carnation caused by Sclerotinia sclerotiorum. Front. Microbiol. 8, 446. doi: 10.3389/fmicb.2017.00446
Walters, D., Raynor, L., Mitchell, A., Walker, R., and Walker, K. (2004). Antifungal activities of four fatty acids against plant pathogenic fungi. Mycopathologia 157, 87–90. doi: 10.1023/B:MYCO.0000012222.68156.2c
Wang, B., Yuan, J., Zhang, J., Shen, Z., Zhang, M., Li, R., et al. (2013). Effects of novel bioorganic fertilizer produced by Bacillus amyloliquefaciens W19 on antagonism of Fusarium wilt of banana. Biol. Fertil. Soils. 49, 435–446. doi: 10.1007/s00374-012-0739-5
Wang, Z., Li, J. Y., Jia, C. H., Li, J. P., Xu, B. Y., and Jin, Z. Q. (2016). Molecular cloning and expression of four phenylalanine ammonia lyase genes from banana interacting with Fusarium oxysporum. Biol. Plant. 60, 459–468. doi: 10.1007/s10535-016-0619-1
Wei, G., Kloepper, J. W., and Tuzun, S. (1991). Induction of systemic resistance of cucumber to Colletotrichum orbiculare by select strains of plant growth-promoting rhizobacteria. Phytopathology 81, 1508–1512. doi: 10.1094/Phyto-81-1508
White, T. J., Bruns, T., Lee, S. J., and Taylor, J. (1990). Amplification and direct sequencing of fungal ribosomal RNA genes for phylogenetics. PCR Protocols 18, 315–322. doi: 10.1016/B978-0-12-372180-8.50042-1
Wong, C. K. F., Zulperi, D., Vadamalai, G., Saidi, N. B., and Teh, C. Y. (2019). Phylogenetic Analysis of Fusarium oxysporum f. sp. cubense Associated with Fusarium Wilt of Bananas from Peninsular Malaysia. Sains Malaysiana 48, 1593–1600. doi: 10.17576/jsm-2019-4808-04
Xu, C., Liu, R., Zhang, Q., Chen, X., Qian, Y., and Fang, W. (2017). The diversification of evolutionarily conserved MAPK cascades correlates with the evolution of fungal species and development of lifestyles. Genome Biol. Evol. 9, 311–322. doi: 10.1093/gbe/evw051
Xue, C., Penton, C. R., Shen, Z., Zhang, R., Huang, Q., Li, R., et al. (2015). Manipulating the banana rhizosphere microbiome for biological control of Panama disease. Sci. Rep. 5, 1–11. doi: 10.1038/srep11124
Yuan, J., Ruan, Y., Wang, B., Zhang, J., Waseem, R., Huang, Q., et al. (2013). Plant growth-promoting rhizobacteria strain Bacillus amyloliquefaciens NJN-6-enriched bio-organic fertilizer suppressed Fusarium wilt and promoted the growth of banana plants. J. Agric. Food Chem. 61, 3774–3780. doi: 10.1021/jf400038z
Zhang, J. H., Sun, H. L., Chen, S. Y., Zeng, L., and Wang, T. T. (2017). Anti-fungal activity, mechanism studies on α-Phellandrene and Nonanal against Penicillium cyclopium. Bot. Stud. 58, 1–9. doi: 10.1186/s40529-017-0168-8
Zhang, L., Cenci, A., Rouard, M., Zhang, D., Wang, Y., Tang, W., et al. (2019). Transcriptomic analysis of resistant and susceptible banana corms in response to infection by Fusarium oxysporum f. sp. cubense tropical race 4. Sci. Rep. 9, 1–14. doi: 10.1038/s41598-019-44637-x
Zhao, M., Yuan, J., Zhang, R., Dong, M., Deng, X., Zhu, C., et al. (2018). Microflora that harbor the NRPS gene are responsible for Fusarium wilt disease-suppressive soil. Agric,. Ecosyst. Environ., Appl. Soil Ecol. 132, 83–90. doi: 10.1016/j.apsoil.2018.08.022
Keywords: banana, Fusarium wilt, Bacillus velezensis, biohardening, VOCs/NVOCs compounds, defense genes expression
Citation: Saravanan R, Nakkeeran S, Saranya N, Kavino M, Ragapriya V, Varanavasiappan S, Raveendran M, Krishnamoorthy AS, Malathy VG and Haripriya S (2022) Biohardening of Banana cv. Karpooravalli (ABB; Pisang Awak) With Bacillus velezensis YEBBR6 Promotes Plant Growth and Reprograms the Innate Immune Response Against Fusarium oxysporum f.sp. cubense. Front. Sustain. Food Syst. 6:845512. doi: 10.3389/fsufs.2022.845512
Received: 29 December 2021; Accepted: 18 February 2022;
Published: 04 April 2022.
Edited by:
Narayan Chandra Mandal, Visva-Bharati University, IndiaReviewed by:
Sudisha Jogaiah, Karnatak University, IndiaCopyright © 2022 Saravanan, Nakkeeran, Saranya, Kavino, Ragapriya, Varanavasiappan, Raveendran, Krishnamoorthy, Malathy and Haripriya. This is an open-access article distributed under the terms of the Creative Commons Attribution License (CC BY). The use, distribution or reproduction in other forums is permitted, provided the original author(s) and the copyright owner(s) are credited and that the original publication in this journal is cited, in accordance with accepted academic practice. No use, distribution or reproduction is permitted which does not comply with these terms.
*Correspondence: S. Nakkeeran, bmFra2VlcmFuYXl5YUB0bmF1LmFjLmlu
Disclaimer: All claims expressed in this article are solely those of the authors and do not necessarily represent those of their affiliated organizations, or those of the publisher, the editors and the reviewers. Any product that may be evaluated in this article or claim that may be made by its manufacturer is not guaranteed or endorsed by the publisher.
Research integrity at Frontiers
Learn more about the work of our research integrity team to safeguard the quality of each article we publish.