- 1Theoretical Biology, Department of Physics, Chemistry and Biology, Linköping University, Linköping, Sweden
- 2Environmental Technology and Management, Department of Management and Engineering, Linköping University, Linköping, Sweden
Urban agriculture has a high potential to contribute to local circular economies, for instance by using nitrogen, phosphorus, and potassium in city organic waste streams as fertilizer inputs. However, inefficient use of waste-derived fertilizers could contribute to local water quality impairment related to nitrogen and phosphorus losses. Organic waste derived fertilizers are particularly challenging from a nutrient stoichiometry perspective, making over- and under-application of a particular nutrient likely. Where, and under what conditions, urban agriculture acts as a net positive for a circular nutrient economy vs. a nutrient water quality risk remains unclear. Here we review empirical peer-reviewed studies (2000–2021) on soil- and ground-based urban agriculture with a stated concern for nutrient losses to water. Of the 20 publications retained and reviewed (out of 241 screened), only seven measured losses to waters. There were four experimental studies, of which three measured nutrient leachate losses under different garden management practices. Of the 16 studies done in real-world conditions, only four quantified losses to water as leachate; average losses spanned 0.005 to 6.5 kg ha−1 for phosphorus, and 0.05 to 140 kg ha−1 for nitrogen. 13 of the 16 non-experimental studies provided data on nutrient inputs and harvested crop outputs, which could be used to calculate garden nutrient balances—an indicator of nutrient use efficiency. Although the value ranges were large, most studied gardens showed nutrient surpluses (inputs > crop harvest) for nitrogen and phosphorus (but not potassium); these surpluses were identified as a risk for losses to water. Contextual factors such as different access to fertilizers and knowledge, along with regulations and environmental factors can help explain the wide range of balance values and nutrient losses observed. Although a large surplus of inputs was often linked to increased leachate losses, it was not always the case in the limited number of studies we identified. Our review suggests that more field studies that measure losses to waters, and document contextual factors, are needed to determine how urban agriculture may contribute to a sustainable circular economy for all three nutrients without nutrient-related water quality impairment.
Introduction
There are widely expressed concerns about the unsustainability of current food systems, in particular the use of resources in production (Viljoen and Wiskerke, 2012; Restrepo and Morales-Pinzón, 2018). Cities, as hubs of consumption, can play an important role in changing the way natural resources are used for the production of food and in the transition toward a more sustainable and circular economy (Restrepo and Morales-Pinzón, 2018). In a “linear” urban economy, cities are seen as the place where large amounts of food are consumed and thus waste is created, and this is made possible by exploiting natural (and human) resources in a city's rural hinterlands (Wiskerke, 2015). In contrast, a circular economy approach aims to close material and substance loops, thereby reducing resource consumption, waste generation, and environmental impact, as well as increasing reuse of nutrients, energy, and water (Jurgilevich et al., 2016; Deksissa et al., 2021). A circular urban food system closes resource loops by using unavoidable organic waste streams from cities to safely support agricultural production. Although linking urban and rural food supply systems, is, and will continue to be, central to feeding cities sustainably (Kloppenburg et al., 2000; Viljoen and Wiskerke, 2012; Forssell and Lankoski, 2015), there are also opportunities for within city food production and circular resource use.
Organic waste is often bulky and heavy, which makes reuse between urban waste producers and rural farms challenging, e.g., the costs and fuel consumption associated with the transport (Westerman and Bicudo, 2005). Urban agriculture (UA) is co-located with waste production and thus provides an opportunity to facilitate a circular nutrient economy with low transport needs. The FAO defines UA as “small areas (e.g., vacant plots, gardens, verges, balconies, containers) within the city for growing crops […] [UA] is perceived as agriculture practices within and around cities which compete for resources (land, water, energy, labor) that could also serve other purposes to satisfy the requirements of the urban population” (FAO, 2007, p. 1). At the same time, UA is viewed as a tool to fight food insecurity and the poverty that urbanization is causing (Tank, 2016) and increasingly for its potential to contribute to more circular urban food systems (Jurgilevich et al., 2016; Deksissa et al., 2021). Circular UA would mean that the inputs for growing crops are derived from reused or recycled urban waste streams. This can include heat, space, water, or one of the largest inputs that UA requires: nutrients. Nutrients are abundant in organic urban waste streams. Food and landscaping waste, as well as animal and human excreta, can be treated to create valuable fertilizers that contain essential plant nutrients like nitrogen (N), phosphorus (P), and potassium (K) for reuse in UA.
There is a high potential for nutrient reuse in UA, but this can only be achieved sustainably if nutrients are used efficiently. UA can involve the inefficient use of resources in a particular city (Goldstein et al., 2016; Small et al., 2019a), which can in turn contribute to excessive resource extraction and pollution. Nutrient losses caused by ineffective N and P management in rural agriculture have been identified as main contributors to water quality impairment (Schröder et al., 2004). Such losses can be, for example, caused by fast-release soluble fertilizer applications, but also by manure supply combined with precipitation events after application (Smith et al., 2007). Nitrate originating from agricultural activities is seen as one of the most important groundwater quality problems worldwide (Li et al., 2014) and surface water eutrophication is driven by losses from non-point sources (Schaffner et al., 2009). As UA practices have been expanding at a fast pace worldwide (Kavitha et al., 2015; Houessou et al., 2020) it is important to assess the risks for similar problems (Stewart et al., 2013; Aubry and Manouchehri, 2019). UA often involves growing vegetables and fruits, and studies have shown that commercial scale horticulture is nutrient intensive and often nutrient inefficient (Breś, 2009; Shukla et al., 2010). Moreover, due to the current lack of rules and regulations on nutrient management in UA, losses might be more difficult to mitigate than in large scale agriculture. Therefore, potential environmental and health risks of UA through excessive application of fertilizers cannot be overlooked. Also, inefficient adoption of circular practices in UA could actually add to the risk for nutrient losses because organic inputs have variable concentrations and forms of nutrients, and are therefore harder to manage efficiently to avoid losses (Allen et al., 2006). Moving toward a circular economy approach in UA without negatively contributing to other sustainability priorities, such as water quality, requires an improved understanding of nutrient losses in current UA. A review of the current knowledge about the topic is an important first step in this direction.
There are multiple types of UA, each with their own cultivation techniques, and they likely present different risks with regards to nutrient losses (Goldstein et al., 2016; Whittinghill et al., 2016; Small et al., 2018). For instance, hydroponic cultivation practices, which are often used to grow crops in buildings (e.g., in vertical farming), can be connected to an urban sewage system (Zareba et al., 2021). Nutrients not taken-up by crops may thus find their way to a wastewater treatment plant instead of directly to the surrounding environment. Some hydroponic systems may even be designed as closed-loop systems where losses of water, and thus nutrients, are minimized if not eliminated (Rufí-Salís et al., 2020). Rooftop farming on the other hand might lead to increased nutrients in a city's stormwater system if leachate from the roof is channeled toward the street in the same way rainwater would be (Harada et al., 2018). Hence, different nutrient and water management systems in UA can result in different nutrient losses. In soil- and ground-based UA, where cultivation occurs directly in in-situ soil, excess nutrients, not taken up by the crops, could be stored in the soil and be used by crops in subsequent years. However, it is also possible that nutrients leave the plot through leaching to groundwater or through runoff and erosion. As in rural agriculture, the relative importance of those loss pathways in UA can be affected by irrigation practices, but also by other aspects of urban hydrology such as stormwater drains, imperious surfaces, and altered soil infiltration rates (Lintern et al., 2020). Soil- and ground-based UA is commonly practiced worldwide (Orsini et al., 2013; Drechsel, 2015). Given its ubiquity, and its high potential for unintended nutrient losses, it is important to determine how much, and under what conditions, this type of UA contributes to nutrient loading of urban waters. Such information is essential to make management recommendations which support circularity and clean water.
In this paper, we wanted to address the role that soil- and ground-based urban agriculture might play for nutrient loading to ground- and surface water. We present the results from a review of peer-reviewed literature (years 2000–2021) to understand the state of knowledge regarding this subject in different cities around the world. Our aim was to find out if current published work was sufficient to assess if certain UA practices are contributing to nutrient-related water quality impairment, and we asked the following questions:
1) How many studies measured nutrient losses to water, what loss pathways did they quantify, and was UA deemed a significant source of nutrients to receiving waters in those cases?
2) Is there evidence of excessive N, P, and K application in soil- and ground-based UA, thus leading to risks for losses to waters?
3) What factors have studies identified as drivers of nutrient management practices that can influence the potential losses to waters?
Methodology
To better understand if and how UA might be contributing to nutrient losses to waters, and what affects those potential losses, we conducted a literature review (see Figure 2). Here we explain how we found and selected the studies, and how they were analyzed. In rural agriculture, the term “farm-gate balance” is defined as the difference between nutrient inputs and intentional outputs at farm level, and is often used as a tool to evaluate nutrient flows and as a proxy for risk of nutrient losses to waters including through runoff, erosion, and leaching (Van Beek et al., 2003; Fangueiro et al., 2008). In order to compare studies which were concerned with losses to waters, but did not always measure them, we compiled or calculated garden-gate balances for N, P and K when possible.
Search Strategy
We searched for relevant peer-reviewed studies by using a combination of a primary keyword (urban agriculture, and its synonyms) with secondary keywords (water, nutrient, runoff, leaching) in Web of Science (Table 1). We searched for literature from the year 2000 until March 2021 and included worldwide studies. We only included English language studies, which means our synthesis will miss findings published in other languages and in reports by municipalities and non-academic stakeholder groups. This systematic search resulted in a total of 233 titles, of which 12 studies were retained after screening the title and abstract (Table 1; Figure 1). An additional 8 relevant studies were found with a snowball-type method (looking at cited studies in the studies retained in the systematic search and also searching for studies that cited the 12 retained studies) using Google Scholar and Google.
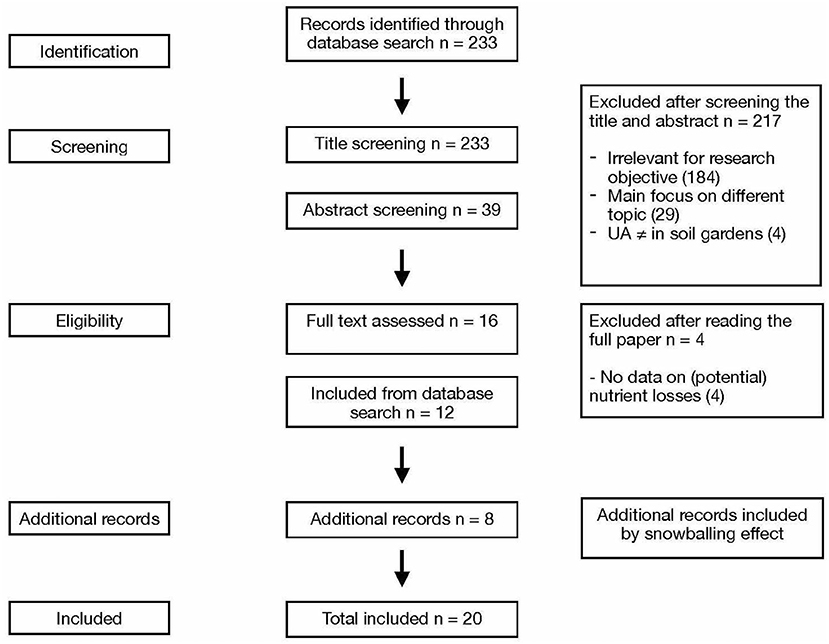
Figure 1. Number of studies captured in searches and removed at different stages of the selection process.
In this review, we focused on UA practices that were soil- and ground-based. Studies were rejected at the screening and eligibility steps (Figure 2) if the studies were not focused on soil- and ground-based UA and on N, P, or K flows in these systems. We excluded studies that only took into account the impacts of urban and agricultural land use separately as this is not UA. We also excluded soilless, vertical and rooftop systems as these type of UA can implement different technologies that affect potential nutrient leaching in different ways than soil- and ground-based UA [as per Goldstein et al. (2016) comparing ground-based-non-conditioned systems to three other types]. Excluding these other types of UA does not mean that they cannot contribute to sustainable urban food production (or water quality risks) and we will come back to some of these considerations in our discussion.
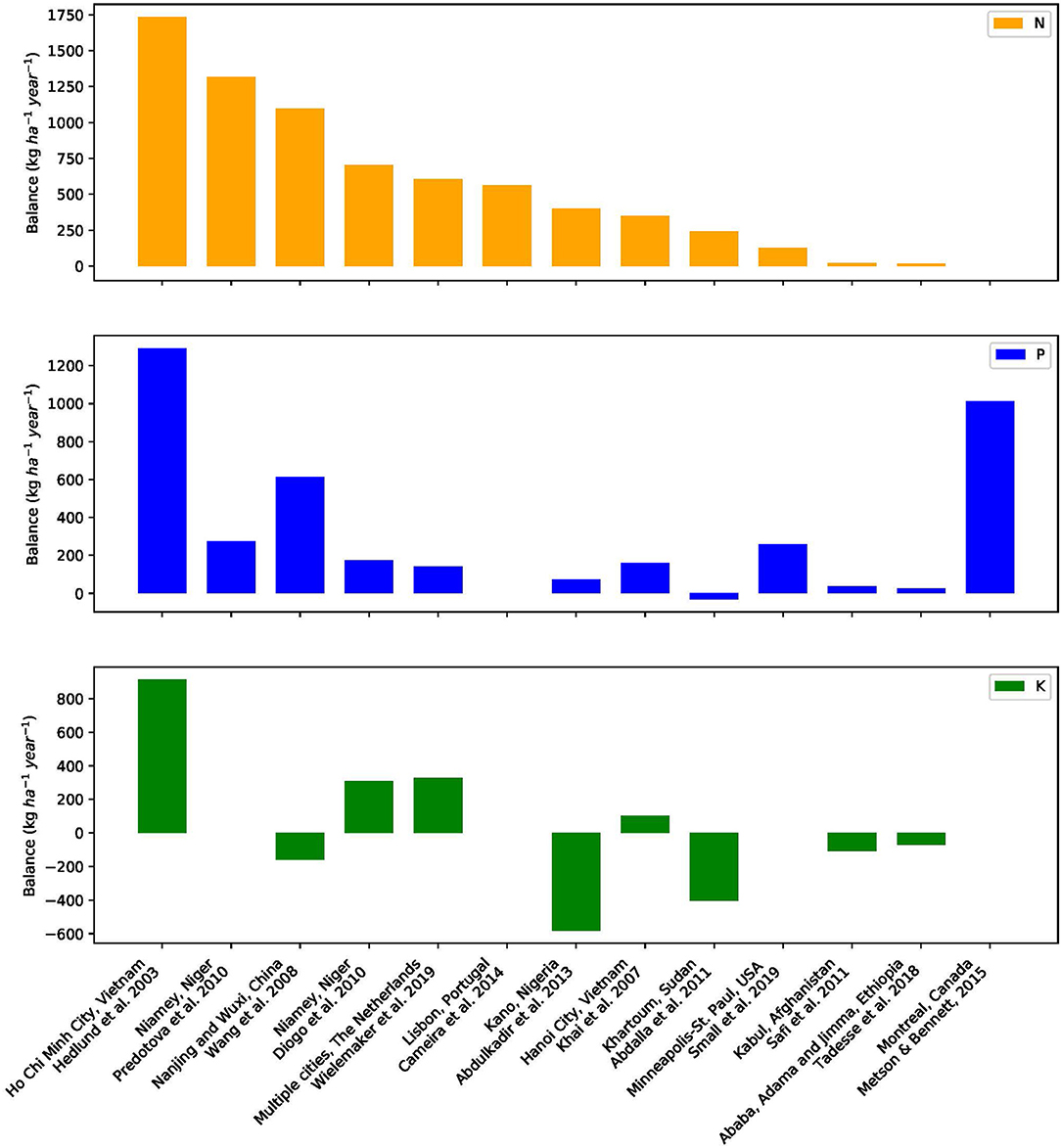
Figure 2. Average garden-gate nutrient balances for the studies that quantified inputs and outputs for each nutrient. Note that there is no nitrogen (N) value for Montreal and no phosphorus (P) value for Lisbon. Potassium (K) values are absent for Montreal, Niamey, Lisbon, and Minneapolis-St. Paul. The order of the cities is kept constant across all three panels, moving from the highest N balance (left) to the lowest (right).
Calculation of Garden-Gate Balances
To be able to compare the potential for nutrient losses to waters in different studies, we used available data about relevant nutrient inputs and outputs. Quantified inputs of farm-gate balances are usually feed concentrates, mineral fertilizers, bought animals, irrigation water, forage and by-products bought, and atmospheric deposition (Nevens et al., 2006). Outputs can include exported milk, exported animals, exported crops, and exported manure (Van Beek et al., 2003; Bassanino et al., 2007). The focus of farm-gate balances is usually not on the balance entries (a specific input or output), but whether there is an overall surplus or deficiency at the farm. Including more balance entries (e.g., nitrogen fixation), results in a more accurate balance and thus a more accurate environmental risk indicator. However, including more balance entries also increases complexity and uncertainty (Van Beek et al., 2003).
Here we adapt the concept of farm-gate balance to UA, where a garden is considered the equivalent of a farm. We will refer to the garden-gate balance, or simply “nutrient balance” or “balance” calculated as:
where B is the garden-gate balance expressed as kilograms per hectare per year (kg ha−1 year−1) and i is a nutrient (N, P, or K). If B is positive the inputs were bigger than outputs which equates to a surplus of a nutrient, whereas if B is negative the outputs were larger than inputs which represents an annual deficit. Thirteen studies included data about the nutrient inputs and outputs of multiple non-experimental urban gardens, which we used to calculate a mean garden-gate balance for UA in that setting. Some studies also showed data on the range of balance values, which we also present in our results. As the different studies used different data collection methods, the mean nutrient surplus or deficiency for a certain city can't be used to draw firm conclusions about differences between cities. Still, the calculated balances give a clear indication of how large the variability may be and thus helps answer our second research question.
The sum of inputs include:
Where Fert. is inorganic (mineral) fertilizer; Org. is organic fertilizer (e.g., fresh or composted manure, purchased plant or animal derived fertilizers such as bone meal, home- or community-made plant and food waste composts); Amend. stands for soil amendments containing nutrients (e.g., potting mix); Irr. is irrigation water and Sed. is flood-related sediment deposition. We aimed to include the same type of inputs and outputs for each nutrient (N, P, or K) balance to make comparisons across studies possible.
When possible we used balances that were presented by the authors themselves but if balances needed to be calculated we only used information that was presented in the studies (Supplementary Tables S1, S2). For inputs, we aimed to include purposely applied fertilizers and irrigation water. In one study (Abdalla et al., 2012), the gardens profited from river flooding, which we also included as a fertilizer input. Only a few studies included atmospheric N deposition as an input (Wang et al., 2008; Diogo et al., 2010; Abdulkadir et al., 2013), which we excluded from two of the three datasets to better match the inputs considered across studies. We were not able to calculate the N balance without atmospheric deposition with the data in Wang et al. (2008); but this should not affect our comparisons among cities because across the other studies atmospheric N deposition was lower than 1% of total inputs. For better cross-study comparison, we also excluded inputs and outputs that would have had minor impact on the overall garden-gate balance, such as root exudates and seedlings, which were present in two studies (Khai et al., 2007; Safi et al., 2011). In a few studies, irrigation water contributed substantially to the overall inputs and was therefore included in the balance calculation (Supplementary Table S1). If irrigation water was not mentioned as an input (as in most studies) we assumed it was not a large source of nutrients (e.g., drinking water).
The sum of outputs refers to:
Where Crop is the crop harvest or the crop uptake. We used crop harvest or crop uptake depending on the data available in each article. Crop uptake is always larger than crop harvest as it also includes the crop residues, such as the roots, whereas the crop harvest only includes the plant parts that are harvested from the field. One article included, in addition to the crop harvest, leachate as an output for 1 out of 4 gardens and it was not possible to subtract it from the numbers given in the paper; the amount of nutrient leachate compared to the average crop harvest reported was negligible (Supplementary Table S1).
Not all studies specified or included all inputs and outputs (see Supplementary Table S1 for what was included and Supplementary Table S2 for calculation details). The majority of the reviewed studies focused on vegetable gardening and as such is the basis on which we compared garden-gate balances. There were however three studies (Abdalla et al., 2012; Abdulkadir et al., 2013; Metson and Bennett, 2015) where we could not separate data for vegetable cultivation from field and livestock systems. For these three cities we thus present nutrient balances based on the mixed farming systems they had quantified.
Analysis
To answer our first research question, we looked at how many studies included research on nutrient losses to waters in UA and how they measured and quantified those losses. To create an overview of the different studies included in this review, we extracted information from each study regarding their research method and sample size, and what each study's authors highlighted as their most important findings (Table 2). In Tables 3, 4 we present the findings on measured nutrient losses in experimental and real-world UA respectively. To answer the second research question, we used nutrient balances (as per section Calculation of Garden-Gate Balances) to indicate whether gardens in a particular city exhibited N, P, or K nutrient surpluses or deficiencies (Table 5; Figure 2).
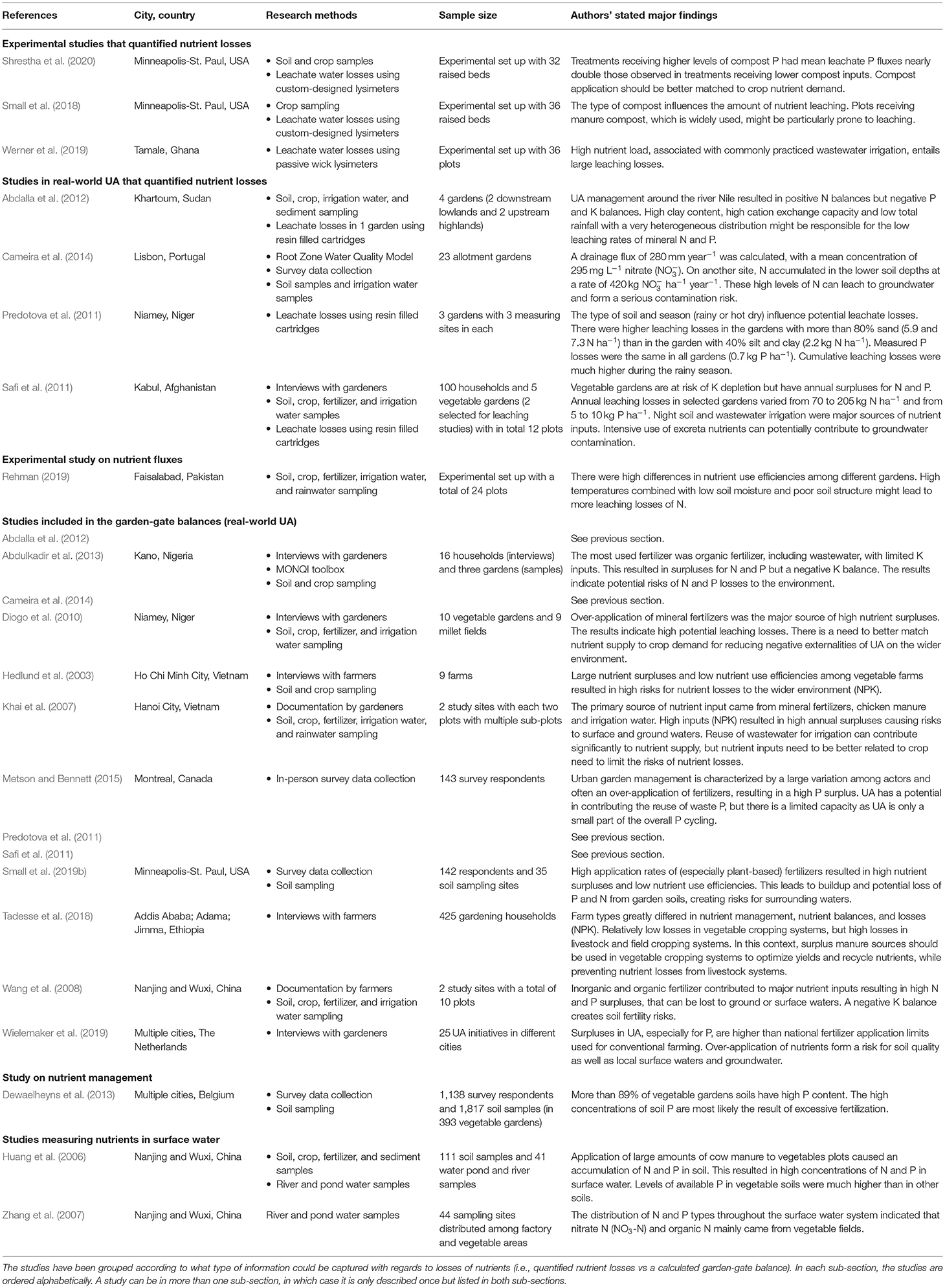
Table 2. Summary of the 20 peer-reviewed studies (2000–2021, focused on soil- and ground-based UA and losses to waters) retained through the review process.
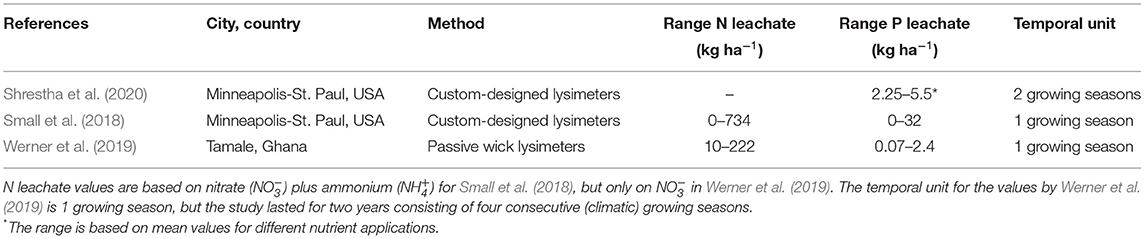
Table 3. Ranges of nitrogen (N) and phosphorus (P) leachate found in studies that had an experimental design to quantify leachate under different management conditions.
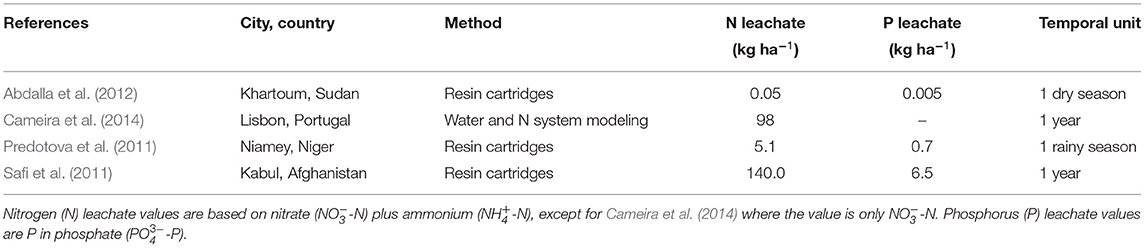
Table 4. Average nutrient losses found in studies that quantified leachate in real-world UA (non-controlled) settings.
Research question three required a more qualitative review of the retained studies. Previous framework papers on urban nutrient flows and resource use in UA highlight the need to consider the unique constellation of social, economic, technological, and ecological factors to identify drivers of observed patterns and intervention points (Metson et al., 2015a,b; Small et al., 2019a). These papers provided broad categories of contextual factors to consider. Previous work on rural agriculture also gave us a reference point for the type of biophysical and management practice factors which affect losses to waterway. With this framing, we carefully reviewed the result and discussion sections of the different studies to extract what the authors though were the factors that drove (influenced, or mediated) the leachate and/or balance values in their case study. A first level of factors was related to identifying garden-level management practices (e.g., type of fertilizer used, or type of crops grown) and plot-specific characteristics (e.g., soil type). A second level of factors related to reasons for adopting certain practices and the surrounding context (e.g., precipitation). Factors were not grouped according to an existing framework, but rather emerged from identifying commonalities among studies and their contexts, in this case Global North and Global South.
We answer the three research questions in our Results section. For our Discussion section, we explore if other factors, such as the data collection methods, might affect the values and their variability. Moreover, we explain how UA could fit in the perspective of a circular economy and formulate future study directions.
Results
Few Studies Measure Losses of Nutrients to Waters
We found 20 relevant studies that quantified nutrient flows in soil- and ground-based UA gardens in 20 years of peer-reviewed literature. The majority of the 20 studies suggest that UA can increase the risk for nutrient losses to surface and ground waters, in particular leaching (Table 2). Importantly, only a few studies contained data on measured losses. Leachate was measured in three experimental studies and four in-situ studies.
Three studies designed experiments to test how different management practices affected nutrient losses from UA to ground- and surface waters. Management practices included different types and amounts of inputs applied to plots as well as different crops grown. The studies then measured losses with lysimeters (Table 3). These studies show that higher fertilizer application rates resulted in higher nutrient leachate losses in Minneapolis Saint-Paul, USA, and Tamale, Ghana. The type of input used also had a large impact on leachate N and P concentrations. Manure compost, which is commonly used across cities included in this review, was particularly prone to leaching in Minneapolis Saint-Paul (Small et al., 2018; Shrestha et al., 2020). In Tamale, irrigating the gardens with wastewater resulted in significantly higher losses than irrigating with tap water (Werner et al., 2019). The leaching rates measured in Minneapolis Saint-Paul were higher than in Tamale (Table 3). Our review also identified an experimental study which tested different input treatments but did not measure losses. They put forward that poor soil structure and high temperatures, combined with low soil moisture, can potentially enhance nutrient losses (Rehman, 2019). In summary, nutrients from manure and wastewater are important for closing nutrient loops, but high inputs, combined with poor soil characteristics and high temperatures, can contribute to increased nutrient leaching.
Out of the 16 non-experimental studies, only four measured losses to waters (Table 4). Three used resin filled cartridges to measure nutrient losses through leaching (Predotova et al., 2011; Safi et al., 2011; Abdalla et al., 2012). One study did soil sampling to use the Root Zone Water Quality model and compute nutrient leachate losses (Ahuja et al., 2000; Cameira et al., 2014). These few studies in real-world contexts show very different average leachate losses. The nutrient losses found in Lisbon, Portugal, and Kabul, Afghanistan, were much higher than the losses found in the studies from Khartoum, Sudan and Niamey, Niger. Higher leachate rates may pose a risk for high nutrient loadings to waters. However, the actual amount of nutrients reaching surrounding waters were not measured in these studies.
Two studies did measure N and P in surface water close to UA. N and P concentrations in rivers and ponds in areas dominated by UA vegetable production were compared to those in areas dominated by factories in Nanjing and Wuxi, China (Huang et al., 2006; Zhang et al., 2007). The areas dominated by vegetable gardens had high concentrations of N and P in surface water. High fertilizer applications cause an accumulation of N and P in the soil and the authors state that runoff and leaching from these soils can explain those high nutrient concentrations. One should note, however, that this is an inferred result by comparing areas, and not getting at the mechanisms of loss from UA to waters.
The ten remaining non-experimental studies did not include water sampling or water loss models; instead, they inferred that there may be a high potential for losses based on soil nutrient status or nutrient balance calculations where only crop harvest or uptake was considered an output.
Large Input Surpluses Are Common for N and P
Among the 13 studies with reported nutrient inputs and outputs, almost all show surpluses (i.e., positive values after calculating garden-gate nutrient balances, Figure 2, Supplementary Table S3). These surpluses indicate that the input of fertilizers and/or irrigation water is higher than the output as crop uptake/harvest, suggesting a risk for nutrient losses. There is high variability in balance values among studies, with the highest average surpluses of N, P and K found in a study from Ho Chi Minh City, Vietnam. Only nine of the studies included K, and few of them showed a surplus for this element, suggesting that many UA cultivation systems depend on the K pool in the soil.
There is not only a range of garden balance values between cities, but also within cities (Table 5). As one might expect, nutrient management practices can vary substantially from one gardener to the next, even in the same allotment garden area. Therefore, balance values will vary within a city. Moreover, soil type and structure can differ within cities, which influences the capacity of the soil to act as a nutrient buffer, leading to more variety in nutrient management practices, and in turn potential losses.
Multiple Factors Influence the Potential for Losses
We identified very few studies that met our selection criteria, and they vary in geographical context, and therefore give only an indication on (potential) nutrient losses to waters. The authors of the studies we compiled, point out multiple reasons for variations in nutrient balances (and leachate losses). Some of these factors focus on the reasons behind specific management practices (i.e., why people use the inputs they do), but other contextual factors, biophysical and social, are also mentioned. In the following subsections we synthesize these factors for the Global North and South separately, as they seem to differ.
Input Preferences and (Lack of) Knowledge and Regulations in the Global North
In the Global North, where high nutrient surpluses are reported, UA gardeners often have access to various types of inputs from shops and their surroundings, and would like to contribute to circularity. The studies we examined showed that practitioners favored “natural” inputs (i.e., recycled organic matter). In Minneapolis-St. Paul, almost all of the 142 survey respondents used only plant-based or manure composts; only four respondents mentioned the use of mineral fertilizers (Small et al., 2019b). Also in the studies from the Netherlands and Portugal, the main inputs were compost and manure (Cameira et al., 2014; Wielemaker et al., 2019). In the Netherlands, gardeners preferred fertilizers derived from organic sources because they did not want to use mineral fertilizers (Wielemaker et al., 2019). This was also the case in Montreal, where UA gardeners favored homemade composts (Metson and Bennett, 2015). In Flanders, Belgium, 70% of the used compost in UA was also homemade (Dewaelheyns et al., 2013).
Even if there are preferences for recycled inputs, there is often a lack of knowledge on the composition of these organic inputs, making it difficult to target crop demand, which in turn, make it easy to over-apply inputs. In Belgium, gardeners had limited knowledge on the composition of their compost (Dewaelheyns et al., 2013). Similarly, urban gardeners in Lisbon did not consider non-synthetic fertilizer N sources in their fertilization planning, and often used inputs with inadequate nutrient ratios (Cameira et al., 2014). Although more than half of the surveyed gardeners in the Netherlands indicated that their use of fertilizer was based on a mix of experience and knowledge, the UA gardeners used excessive amounts of fertilizer leading to high nutrient surpluses (Wielemaker et al., 2019). This indicates that UA practitioners' source of knowledge and type of experiences are important. Lack of standard knowledge and experience about UA can result in overconfidence and unintentionally lead to inefficient nutrient use and associated risks of nutrient losses.
Dependency on Local Nutrient Resources and Income From UA in the Global South
In the Global South, UA practitioners are often more dependent on local, and free, inputs than in the global North. However, this can also lead to nutrient imbalances. For instance, in UA gardens in China, Vietnam, Nigeria and Afghanistan, wastewater was an important contributor to nutrient inputs, especially for N (Khai et al., 2007; Wang et al., 2008; Predotova et al., 2011; Safi et al., 2011; Abdulkadir et al., 2013). Almost all of the UA gardens in these cities had surpluses of N and P (Figure 2). However, for studies that showed water as an important nutrient input, K was deficient (Table 5). Also, imbalanced inputs can decrease crop yields, at times leading to further losses. UA gardeners in China reported that reduced yields were affecting their decision to apply more N and P, while the study results suggest that the yield reduction was a result of a negative K balance (Wang et al., 2008).
Besides wastewater, UA gardeners in the Global South studies we reviewed often used manure to fertilize their garden plots. Like in the Global North, the application of manure seems to have led to an over-application of nutrients in many cities. The amount of manure that was used in Ethiopia was related to whether the urban farmers raised livestock, in addition to the vegetables, to gain more income (Tadesse et al., 2018). However, due to scarcity of land, storing the manure was not possible. The lack of land led to manure accumulation on the farm, which contributed to higher surpluses of N, P and K (453, 85, 264 kg ha−1 year−1 respectively) than in gardens growing only vegetables (Figure 2). Similarly, the study from Lisbon, Portugal (Global North) reported that the highest amount of manure applied in gardens (60 tons ha−1 year−1) was a result of a farmer that produced poultry and did not have an efficient means to manage the generated manure (Cameira et al., 2014).
The capacity to make an income from different UA practices also affected nutrient management in studies from the Global South. Like in rural agriculture, possible profits depend on the type of crops that are cultivated and the type and amount of nutrient inputs used (which both vary in price). In Nanjing and Wuxi, China, UA gardeners whose income was fully dependent on selling products from their garden had higher nutrient use efficiencies (less surpluses), than gardeners who also had other sources of income (Wang et al., 2008). When the gardeners' income was not only dependent on vegetable farming, they cultivated crops with longer growing cycles. Those crops required less management but higher amounts of cow manure, leading to higher surpluses and increased risk for losses. The price of nutrient inputs is another economic aspect that can affect nutrient management. For example, in Sudan, manure is scarce and as such is an important source of income for some farmers. This scarcity seems to have led to more efficient nutrient use and lower surpluses in urban gardens (Abdalla et al., 2012).
Discussion
UA as Part of a Circular Nutrient Economy
This review shows that soil- and ground-based UA can contribute to a circular economy by reusing nutrients in organic waste streams. All of the reviewed studies indicated that the majority of UA practitioners incorporate urban waste in their vegetable cultivation practices. These recycled inputs include composts and manure, as well as wastewater. However, an inefficient use of nutrients (expressed as a surplus based on the garden-gate balance calculations) was wide-spread; most authors highlighted the risks for losses of nutrients to urban waters given these N and P surpluses. To claim that UA can play an important part of a sustainable circular economy, these recycled nutrient inputs need to be managed effectively and efficiently.
Reducing and Recycling Nutrient Inputs
The large number of studies showing a nutrient surplus demonstrates that inputs can likely be reduced to minimize unintentional losses. Although approaching annual garden-gate equilibrium (no surplus or deficit) can reduce the risk of losses to water, it is important to remember that over many years it can increase the risk of nutrient related yield limits; soil testing and active management may be required to balance such risks and benefits. However, many of the garden-gate balances show such a large surplus that, at present, input reductions are not likely to cause deficiencies. For example, the nutrient inputs in UA farms in the Netherlands exceeded mean crop demand by 450% for N, 600% for P, and 250% for K (Wielemaker et al., 2019).
Still, reducing inputs, while targeting crop demand, to minimize nutrient losses can be challenging with organic waste derived inputs. Manure composts are particularly prone to leaching because of their low bulk density and the high mineralization rates associated with their labile organic matter content (Small et al., 2018). Generally, when composts (or soil) have a low bulk density, there is a higher porosity and water permeability, which results in more leaching (Ghorbani et al., 2019). In addition, nutrient imbalances in organic fertilizers may increase losses as gardeners often dose fertilizers based on a specific element, often N. For example, if manure compost (low N:P ratio) is primarily used to meet crop N demand, it can lead to a P build-up in the soil and increased risk for P losses (Small et al., 2018, 2019b). Better formulated recycled fertilizers to match the crop needs would benefit UA. Adding materials with high C:N ratios, such as woodchips or straw, to manure composts can help to increase microbial immobilization of nutrients and form a slow-release organic fertilizer that also helps reduce nutrient leaching (Small et al., 2018).
Alternative sanitation systems could also help create safer nutrient products for reuse in UA. Irrigation with wastewater that is contaminated with pathogens can lead to the contamination of vegetables (Diogo et al., 2010). It is therefore important to consider more than only nutrient content to decide on how to best reuse waste-streams for a circular economy. The potential of urine deviated vacuum toilets, anaerobic digesters, and struvite precipitation systems to collect and treat human waste for reuse in UA was studied in Rotterdam, the Netherlands (Wielemaker et al., 2018). With an efficient use of those recycled nutrients (avoiding over-application), there is potential to achieve city self-sufficiency for P. Assuming a marketable yield of 45,000 kg ha−1, using recycled nutrients for cultivation on only 1,010 hectares (around one-third of the cities' potential space for UA) could supply enough vegetables for all residents. However, a better understanding of the potential spread of pathogens, micro-pollutants, and pharmaceuticals associated with products derived from such new sanitation systems is still needed (de Wilt et al., 2016; Wielemaker et al., 2018).
Improving Water Management
Taking into account precipitation patterns and irrigation practices can also help to reduce nutrient losses without negatively affecting nutrient circularity. For instance, when water fluxes are high, leaching can be the major output pathway from garden plots (Werner et al., 2019). Therefore, combining high nutrient applications with irrigation can lead to high nutrient losses. In Hanoi City, Vietnam, where there were high inputs of wastewater, a lack of optimal timing of fertilizer application with crop demand possibly resulted in the low nutrient use efficiencies that were found (Khai et al., 2007). When using wastewater, irrigation and fertilization are combined by default and can lead to losses as leaching occurs when soil water content exceeds field capacity (Lehmann and Schroth, 2003). Therefore, high nutrient losses can occur when irrigating with wastewater, even if net garden-gate balances are relatively low. For example, in Kabul, Afghanistan, where there were only small surpluses of N and P, irrigation with wastewater still probably contributed to the observed high leachate losses (Safi et al., 2011, Table 4). Over-application of wastewater could be a result of aiming to meet crop nutrient demand, while not paying enough attention to the soil water content. Studies have shown that wastewater irrigation can be an efficient way to provide nutrients to crops in rural agriculture, but that it is important to match it to crop nutrient demand (Jaramillo and Restrepo, 2017). As suggested for rural agriculture, instead of applying large inputs of wastewater (or manure) at once, applying smaller amounts distributed throughout the growing season could help to lower the risks of losses and improve crop yields (Burton et al., 2008; Velasco et al., 2012). In addition, in places where there are heavy precipitation events, using a greenhouse to cover the soil from high water fluxes would help to limit leaching losses (Wang et al., 2018). Rainwater runoff from the greenhouse could then be collected (i.e., by using rainwater harvesting systems) to be used later for irrigation (Hofman and Paalman, 2014). Moreover, intelligent irrigation systems with soil moisture sensors could help to manage water inputs more efficiently and therefore help reduce nutrient leaching (Kumar et al., 2013). Such solutions for water management are used in rural agriculture. Implementing greenhouse structures or smart technologies in UA might pose different challenges than in rural farms, in part because of smaller plot sizes. Gardener interests might be limited given these solutions require financial and knowledge investments, while gardeners rarely bare the direct cost of eutrophication.
In summary, there are different strategies to lower the risk of nutrient losses from soil- and ground-based UA. Composts and wastewater are frequently used inputs in UA and can potentially contribute to a circular economy as they are both from reused sources. However, such recycled organic wastes often have an imbalanced nutrient ratio and to meet crop demand these sources should be better managed. Using new (and old) technologies that can transform these organic waste-streams into products that are safe and have a known N:P:K ratio, and matching inputs to different types of UA, can help to make UA part of a circular biobased economy.
Future Directions in Studying Nutrient Losses in UA
Nutrient losses in agriculture, including UA, are driven by a complex set of processes. Types and amounts of nutrient inputs must be accounted for, but other factors such as soil type, irrigation patterns, age of establishment of the cultivation plot, slope of an area, and climate are also important (Velthof et al., 2009; Sharpley et al., 2015). Based on the findings of this review, we suggest future directions for research on nutrient losses in UA.
The Urban Setting
Although there are similarities between potential nutrient loss pathways in UA and rural agriculture, some risks (and thus solutions) differ. The two systems may have different management practices and the land-use and infrastructure systems that surround food production fields/areas are also in stark contrast. The distance between a field (or pasture) and surface waters plays a role in the potential for nutrient losses (Yuan et al., 2009; Evans et al., 2014). In rural agriculture, riparian buffer strips are commonly used as a method to protect surface waters from quality degradation caused by erosion and runoff from adjacent agricultural lands (Stutter et al., 2012; Cole et al., 2020). In contrast, urban gardens are positioned within, or close to, the built environment, including roads or housing blocks. This may leave no space for such buffer strips. At the same time, the hydrology of the urban environment is heavily modified; stormwater drains and large amounts of impervious systems cause rapid runoff after precipitation events (Lintern et al., 2020). If UA gardens are drained through these systems, they could disproportionally contribute to high nutrient loads to urban waters. On the other hand, by being a pervious land-use in a “sea of concrete,” UA can help to reduce stormwater runoff such as has been shown in urban vacant lots (Kelleher et al., 2020). In addition, the high infiltration and evapotranspiration potential of UA highlights the benefits gardens can have to the cities' hydrological ecosystem (Chapman et al., 2022). Although the above examples demonstrate that the unique context of cities is likely to mediate nutrient losses from UA, the studies in this review did not explicitly account for how the urban setting of gardens may impact losses to waters.
Variability in UA Management
The high variability in nutrient balances, both among and within cities, suggests that more studies are needed to understand gardeners nutrient management practices. The widest ranges were found for gardens in Montreal and Minneapolis-St. Paul. Both of these studies used the same survey method with over 140 respondents in each city. In other studies, a lower number of interviewees or survey respondents were often used which might result in a lower variability among gardens. However, in the study from Ethiopia the variability among garden-balances is low even though the study is based on a relatively large number of survey respondents. This may be related to the low average balance, with almost no N and P surpluses, and a negative K balance; most gardeners had very low or no inputs. A lack of access to fertilizers makes all gardeners more similar to one another. This indicates that, in cities where there are various options for garden fertilization, it is important to include multiple gardens in one study to cover a wide range of nutrient management practices and thus the associated variety of potential losses.
Measuring Nutrient Losses
Even though nutrient balances are a useful tool to estimate the possible losses, it should be combined with water quality measurements. For instance, the reviewed studies show that surpluses do not always translate to high leaching and vice versa. Even when garden-gate nutrient balances values are relatively low, nutrient leachate values can still be very high (Safi et al., 2011). The study in Niamey, Niger showed much higher nutrient surpluses than the study in Kabul, Afghanistan, while the leachate values in the former were much lower (Table 4; Figure 2). Although three of the four studies which measured leachate in real UA settings show that losses can be high, understanding under what conditions this happens is essential to make management recommendations. The large variability in measured losses, and garden-gate balance values across nutrients and cities, support our assertion that measuring losses while accounting for urban contextual factors is necessary when moving forward.
Increased measurements are also needed to explore the risk of N and P losses via other pathways than leaching. Although we identified two studies that used water pond and river samples to indicate that water pollution related to UA can occur via leaching and runoff (Huang et al., 2006; Zhang et al., 2007), most of the studies we reviewed focus on only the leaching potential of UA. Some of the study authors suggest that erosion and runoff in UA might be unlikely or minimal because cultivation areas are usually flat (Hedlund et al., 2003; Wang et al., 2008), but this is likely context specific. Future studies looking at nutrient losses to waters should preferably include multiple gardens in the same city, because nutrient balances (and leaching) can be highly variable among gardens in one city (Table 5).
Year-Round Measurements
The experimental studies we reviewed highlighted the possibility of seasonal influence on losses (Rehman, 2019; Werner et al., 2019). Irrigation and precipitation affect the movement of nutrients and they are seasonally variable. There seems to be a higher risk of nutrient losses during wet seasons (Werner et al., 2019). In the real-world garden study in Khartoum, Sudan, low rainfall during the sampling period most likely contributed to the low nutrient leachate losses measured (Abdalla et al., 2012). Although nutrient loss patterns are highly seasonal in rural agriculture (Amery and Vandecasteele, 2015; Schoumans, 2015), UA may have less (or more) seasonality. It might be easier to cover a small UA plot in the non-growing season to avoid losses (lower seasonality). In contrast, if the growing season is extended in some cities because of the urban heat island effect, perhaps periods of fertilization and high precipitation may co-occur in novel ways (maybe higher seasonality). It is important to perform more studies with year-round measurements to fill this knowledge gap.
Contextual Research
Nutrient related water quality measurements should be combined with more contextual research on nutrient use and recycling in UA. This review suggests that management practices differ between the Global North and South, but also among cities in the same country. Contextual information collected along-side water measurement data will help to:
a) understand why people manage their nutrients the way they do,
b) what stimulates or limits the use of recycled fertilizer products,
c) to what extent gardeners' behavior vs. city characteristics influences the risk for nutrient losses.
Such contextual research can also help identify how potential rules and regulations may encourage sustainable nutrient use through UA. Based on the studies included in this review, there seems to be a lack of rules and guidelines regarding nutrient use in UA in many cities, which might contribute to inefficient nutrient management (i.e., large annual nutrient surpluses). In Lisbon, some of the gardens are regulated by municipal regulations, but these regulations almost exclusively refer to the size and location of gardens and not to cultivation practices (Cameira et al., 2014). In the Netherlands, UA is not governed by the same application limits as rural agriculture; surveyed gardeners would on average exceed the N limit for rural agriculture, and 84% of them the P application limit of 22 kg ha−1 year−1 (Wielemaker et al., 2019). The expansion of UA across the Global North as part of green infrastructure or urban food strategies without regulations or training could pose a risk to waters given that excess inputs is the rule rather than the exception.
Based on our review, we put forward that combining research on year-round nutrient losses and UA context will allow for better recommendations to optimize nutrient recycling, limit losses, and realize the full potential of UA to contribute to circular economies. In addition, given the development of (and interest in) new technologies, and urban space limitations, other types of UA (e.g., rooftops and building-integrated greenhouses) should also be monitored and evaluated for nutrient loss risks. Studying all forms of UA is necessary to fully understand the possible risks and possibilities regarding sustainable nutrient cycling in urban food production and make locally-appropriate choices for investments among options.
Conclusion
In summary, only a few peer-reviewed studies have measured nutrient losses from soil- and ground-based UA, and it is currently not possible to conclude if certain UA practices lead to high nutrient losses to urban waters. Multiple studies included in this review did however quantify nutrient inputs and crop outputs without measuring losses to water. Most of these studies quantified values for N, P and K. Garden-gate balances with large surpluses of N and P were common across cities in the Global North as well as the Global South. The authors of studies that measured losses, and those that presented high nutrient surpluses, tend to highlight that the risk of loss to waters is high. Still, there was a large variability in garden-balance values among and within cities. Differences in types and amounts of inputs, as well as crop choice, were related to contextual factors. Some large surpluses were linked to a lack of knowledge about nutrient management in the Global North. In the Global South, a dependency on wastewater contributed to high risks of N and P losses, but in some cases also to risks of limited crop growth due to K deficiencies. Even when nutrients are in a deficit, or surpluses are relatively low, the use of wastewater contributed to high nutrient leachate losses. Although garden-gate balances are a valuable tool, they are not enough to predict nutrient leachate loss. The loss of nutrients from soils is a result of complex processes which are affected by multiple factors that operate at diverse temporal and spatial scales. A combination of field studies that measure losses to waters, and research on contextual factors, are needed to better understand the potential role of UA for sustainable nutrient cycling. Based on the limited studies available, our review can only indicate a risk for nutrient losses from soil- and ground-based UA to surrounding waters, and stress the need for further empirical work globally.
Author Contributions
PV performed the literature review and garden-gate balance calculations. GM, KT, and PV conceived the study and wrote and edited the manuscript. GM and KT acquired funding and supervised. All authors contributed to the article and approved the submitted version.
Funding
This research was funded by the Swedish Council for Sustainable Development (Formas 2019-01890).
Conflict of Interest
The authors declare that the research was conducted in the absence of any commercial or financial relationships that could be construed as a potential conflict of interest.
Publisher's Note
All claims expressed in this article are solely those of the authors and do not necessarily represent those of their affiliated organizations, or those of the publisher, the editors and the reviewers. Any product that may be evaluated in this article, or claim that may be made by its manufacturer, is not guaranteed or endorsed by the publisher.
Supplementary Material
The Supplementary Material for this article can be found online at: https://www.frontiersin.org/articles/10.3389/fsufs.2022.842930/full#supplementary-material
References
Abdalla, S. B., Predotova, M., Gebauer, J., and Buerkert, A. (2012). Horizontal nutrient flows and balances in irrigated urban gardens of Khartoum, Sudan. Nutr. Cycl. Agroecosys. 92, 119–132. doi: 10.1007/s10705-011-9476-7
Abdulkadir, A., Leffelaar, P. A., Agbenin, J. O., and Giller, K. E. (2013). Nutrient flows and balances in urban and peri-urban agroecosystems of Kano, Nigeria. Nutr. Cycl. Agroecosys. 95, 231–254. doi: 10.1007/s10705-013-9560-2
Ahuja, L., Rojas, K., and Hanson, J. D. (2000). Root Zone Water Quality Model: Modelling Management Effects on Water Quality and Crop Production. Colorado: Water Resources Publication.
Allen, S. C., Nair, V. D., Graetz, D. A., Jose, S., and Nair, P. R. (2006). Phosphorus loss from organic versus inorganic fertilizers used in alleycropping on a Florida Ultisol. Agric. Ecosyst. Environ. 117, 290–298. doi: 10.1016/j.agee.2006.04.010
Amery, F., and Vandecasteele, B. (2015). Wat weten we over fosfor en landbouw? Merelbeke: Instituut voor Landbouw-en Visserijonderzoek.
Aubry, C., and Manouchehri, N. (2019). Urban agriculture and health: assessing risks and overseeing practices. Field Actions Sci. Rep. 20, 108–111.
Bassanino, M., Grignani, C., Sacco, D., and Allisiardi, E. (2007). Nitrogen balances at the crop and farm-gate scale in livestock farms in Italy. Agric. Ecosyst. Environ. 122, 282–294. doi: 10.1016/j.agee.2007.01.023
Breś, W. (2009). Estimation of nutrient losses from open fertigation systems to soil during horticultural plant cultivation. Polish J. Environ. Stud. 18, 341–345.
Burton, D., Zebarth, B., Gillam, K., and MacLeod, J. (2008). Effect of split application of fertilizer nitrogen on N2O emissions from potatoes. Can. J. Soil Sci. 88, 229–239. doi: 10.4141/CJSS06007
Cameira, M. R., Tedesco, S., and Leitao, T. E. (2014). Water and nitrogen budgets under different production systems in Lisbon urban farming. Biosyst. Eng. 125, 65–79. doi: 10.1016/j.biosystemseng.2014.06.020
Chapman, E. J., Small, G. E., and Shrestha, P. (2022). Investigating potential hydrological ecosystem services in urban gardens through soil amendment experiments and hydrologic models. Urban Ecosystems 2022, 1–12. doi: 10.1007/s11252-021-01191-7
Cole, L. J., Stockan, J., and Helliwell, R. (2020). Managing riparian buffer strips to optimise ecosystem services: a review. Agric. Ecosyst. Environ. 296, 106891. doi: 10.1016/j.agee.2020.106891
de Wilt, A., Butkovskyi, A., Tuantet, K., Leal, L. H., Fernandes, T. V., Langenhoff, A., et al. (2016). Micropollutant removal in an algal treatment system fed with source separated wastewater streams. J. Haz. Mater. 304, 84–92. doi: 10.1016/j.jhazmat.2015.10.033
Deksissa, T., Trobman, H., Zendehdel, K., and Azam, H. (2021). Integrating urban agriculture and stormwater management in a circular economy to enhance ecosystem services: connecting the dots. Sustainability 13, 8293. doi: 10.3390/su13158293
Dewaelheyns, V., Elsen, A., Vandendriessche, H., and Gulinck, H. (2013). Garden management and soil fertility in Flemish domestic gardens. Landsc. Urban Plan. 116, 25–35. doi: 10.1016/j.landurbplan.2013.03.010
Diogo, R. V., Buerkert, A., and Schlecht, E. (2010). Horizontal nutrient fluxes and food safety in urban and peri-urban vegetable and millet cultivation of Niamey, Niger. Nutr. Cycl. Agroecosyst. 87, 81–102. doi: 10.1007/s10705-009-9315-2
Drechsel, P. (2015). Cities and Agriculture: Developing Resilient Urban Food Systems. London: Routledge.
Evans, K. O., Burger, L. W. Jr, Riffell, S., and Smith, M. D. (2014). Assessing multiregion avian benefits from strategically targeted agricultural buffers. Conserv. Biol. 28, 892–901. doi: 10.1111/cobi.12311
Fangueiro, D., Pereira, J., Coutinho, J., Moreira, N., and Trindade, H. (2008). NPK farm-gate nutrient balances in dairy farms from Northwest Portugal. Eur. J. Agron. 28, 625–634. doi: 10.1016/j.eja.2008.01.007
FAO (2007). Urban and Peri-Urban Agriculture. FAO. Available online at: http://www.fao.org/unfao/bodies/coag/coag15/x0076e.htm (accessed March 02, 2021).
Forssell, S., and Lankoski, L. (2015). The sustainability promise of alternative food networks: an examination through alternative characteristics. Agric. Human Values 32, 63–75. doi: 10.1007/s10460-014-9516-4
Ghorbani, M., Asadi, H., and Abrishamkesh, S. (2019). Effects of rice husk biochar on selected soil properties and nitrate leaching in loamy sand and clay soil. Int. Soil Water Conserv. Res. 7, 258–265. doi: 10.1016/j.iswcr.2019.05.005
Goldstein, B., Hauschild, M., Fernández, J., and Birkved, M. (2016). Testing the environmental performance of urban agriculture as a food supply in northern climates. J. Cleaner Prod. 135, 984–994. doi: 10.1016/j.jclepro.2016.07.004
Harada, Y., Whitlow, T. H., Templer, P. H., Howarth, R. W., Walter, M. T., Bassuk, N. L., et al. (2018). Nitrogen biogeochemistry of an urban rooftop farm. Front. Ecol. Evol. 6, 153. doi: 10.3389/fevo.2018.00153
Hedlund, A., Witter, E., and An, B. X. (2003). Assessment of N, P and K management by nutrient balances and flows on peri-urban smallholder farms in southern Vietnam. Eur. J. Agron. 20, 71–87. doi: 10.1016/S1161-0301(03)00076-5
Hofman, J., and Paalman, M. (2014). Rainwater harvesting, a sustainable solution for urban climate adaptation? Nieuwegein: KWR Watercycle Research Institute.
Houessou, M. D., van de Louw, M., and Sonneveld, B. G. (2020). What constraints the expansion of urban agriculture in Benin? Sustainability 12, 5774. doi: 10.3390/su12145774
Huang, B., Shi, X., Yu, D., Öborn, I., Blombäck, K., Pagella, T. F., et al. (2006). Environmental assessment of small-scale vegetable farming systems in peri-urban areas of the Yangtze River Delta Region, China. Agric. Ecosyst. Environ. 112, 391–402. doi: 10.1016/j.agee.2005.08.037
Jaramillo, M. F., and Restrepo, I. (2017). Wastewater reuse in agriculture: A review about its limitations and benefits. Sustainability 9, 1734. doi: 10.3390/su9101734
Jurgilevich, A., Birge, T., Kentala-Lehtonen, J., Korhonen-Kurki, K., Pietikäinen, J., Saikku, L., et al. (2016). Transition towards circular economy in the food system. Sustainability 8, 69. doi: 10.3390/su8010069
Kavitha, A., Somashekar, R., and Nagaraja, B. (2015). Urban expansion and loss of Agriculture land-A case of Bengaluru city. Int. J. Geom. Geosci. 5, 492–498.
Kelleher, C., Golden, H. E., Burkholder, S., and Shuster, W. (2020). Urban vacant lands impart hydrological benefits across city landscapes. Nat. Commun. 11, 1–11. doi: 10.1038/s41467-020-15376-9
Khai, N. M., Ha, P. Q., and Öborn, I. (2007). Nutrient flows in small-scale peri-urban vegetable farming systems in Southeast Asia—a case study in Hanoi. Agric. Ecosyst. Environ. 122, 192–202. doi: 10.1016/j.agee.2007.01.003
Kloppenburg, J., Jack, L., ezberg, S., De Master, K., Stevenson, G., and Hendrickson, J. (2000). Tasting food, tasting sustainability: defining the attributes of an alternative food system with competent, ordinary people. Human Organ. 59, 177–186. doi: 10.17730/humo.59.2.8681677127123543
Kumar, N. D., Pramod, S., and Sravani, C. (2013). Intelligent irrigation system. Int. J. Agric. Sci. Res. 3, 23–30.
Lehmann, J., and Schroth, G. (2003). Nutrient Leaching. Trees Crops and Soil Fertility. Wallingford: CABI Publishing. 151–166.
Li, P., Wu, J., Qian, H., Lyu, X., and Liu, H. (2014). Origin and assessment of groundwater pollution and associated health risk: a case study in an industrial park, northwest China. Environ. Geochem. Health 36, 693–712. doi: 10.1007/s10653-013-9590-3
Lintern, A., McPhillips, L., Winfrey, B., Duncan, J., and Grady, C. (2020). Best management practices for diffuse nutrient pollution: wicked problems across urban and agricultural watersheds. Environ. Sci. Technol. 54, 9159–9174. doi: 10.1021/acs.est.9b07511
Metson, G. S., and Bennett, E. M. (2015). Phosphorus cycling in Montreal's food and urban agriculture systems. PLoS ONE 10, e0120726. doi: 10.1371/journal.pone.0120726
Metson, G. S., Bennett, E. M., Kapuscinski, A. R., and Salvador, R. J. (2015a). Facilitators and barriers to organic waste and phosphorus re-use in MontrealFacilitators and barriers to organic waste and phosphorus re-use in Montreal. Elem. Sci. Anthrop. 3:000070. doi: 10.12952/journal.elementa.000070
Metson, G. S., Iwaniec, D. M., Baker, L. A., Bennett, E. M., Childers, D. L., Cordell, D., et al. (2015b). Urban phosphorus sustainability: systemically incorporating social, ecological, and technological factors into phosphorus flow analysis. Environ. Sci. Policy 47, 1–11. doi: 10.1016/j.envsci.2014.10.005
Nevens, F., Verbruggen, I., Reheul, D., and Hofman, G. (2006). Farm gate nitrogen surpluses and nitrogen use efficiency of specialized dairy farms in Flanders: evolution and future goals. Agric. Syst. 88, 142–155. doi: 10.1016/j.agsy.2005.03.005
Orsini, F., Kahane, R., Nono-Womdim, R., and Gianquinto, G. (2013). Urban agriculture in the developing world: a review. Agron. Sustain. Dev. 33, 695–720. doi: 10.1007/s13593-013-0143-z
Predotova, M., Bischoff, W. A., and Buerkert, A. (2011). Mineral-nitrogen and phosphorus leaching from vegetable gardens in Niamey, Niger. J. Plant Nutr. Soil Sci. 174, 47–55. doi: 10.1002/jpln.200900255
Rehman, S. U. (2019). Major nutrient fluxes and water use efficiency of winter vegetables under peri-urban farming of north-western punjab (Pakistan). Pak. J. Bot. 51, 1753–1759. doi: 10.30848/PJB2019-5(18)
Restrepo, J. D. C., and Morales-Pinzón, T. (2018). Urban metabolism and sustainability: precedents, genesis and research perspectives. Resourc. Conserv. Recycl. 131, 216–224. doi: 10.1016/j.resconrec.2017.12.023
Rufí-Salís, M., Petit-Boix, A., Villalba, G., Sanjuan-Delmás, D., Parada, F., Ercilla-Montserrat, M., et al. (2020). Recirculating water and nutrients in urban agriculture: an opportunity towards environmental sustainability and water use efficiency? J. Clean. Prod. 261, 121213. doi: 10.1016/j.jclepro.2020.121213
Safi, Z., Predotova, M., Schlecht, E., and Buerkert, A. (2011). Horizontal matter fluxes and leaching losses in urban and peri-urban agriculture of Kabul, Afghanistan. J. Plant Nutr. Soil Sci. 174, 942–951. doi: 10.1002/jpln.201000385
Schaffner, M., Bader, H.-P., and Scheidegger, R. (2009). Modeling the contribution of point sources and non-point sources to Thachin River water pollution. Sci. Total Environ. 407, 4902–4915. doi: 10.1016/j.scitotenv.2009.05.007
Schoumans, O. F. (2015). Phosphorus Leaching From Soils: Process Description, Risk Assessment and Mitigation. Alterra: Wageningen-UR.
Schröder, J., Scholefield, D., Cabral, F., and Hofman, G. (2004). The effects of nutrient losses from agriculture on ground and surface water quality: the position of science in developing indicators for regulation. Environ. Sci. Policy 7, 15–23. doi: 10.1016/j.envsci.2003.10.006
Sharpley, A. N., Bergström, L., Aronsson, H., Bechmann, M., Bolster, C. H., Börling, K., et al. (2015). Future agriculture with minimized phosphorus losses to waters: Research needs and direction. AMBIO 44, 163–179. doi: 10.1007/s13280-014-0612-x
Shrestha, P., Small, G. E., and Kay, A. (2020). Quantifying nutrient recovery efficiency and loss from compost-based urban agriculture. PloS ONE 15, e0230996. doi: 10.1371/journal.pone.0230996
Shukla, S., Boman, B., Ebel, R., Roberts, P., and Hanlon, E. (2010). Reducing unavoidable nutrient losses from Florida's horticultural crops. HortTechnology 20, 52–66. doi: 10.21273/HORTTECH.20.1.52
Small, G., Mcdougall, R., and Metson, G. S. (2019a). Would a sustainable city be self-sufficient in food production? Int. J. Des. Res. Nat. Ecodyn. 14, 178–194. doi: 10.2495/DNE-V14-N3-178-194
Small, G., Shrestha, P., and Kay, A. (2018). The fate of compost-derived phosphorus in urban gardens. Int. J. Des. Res. Nat. Ecodyn. 13, 415–422. doi: 10.2495/DNE-V13-N4-415-422
Small, G., Shrestha, P., Metson, G. S., Polsky, K., Jimenez, I., and Kay, A. (2019b). Excess phosphorus from compost applications in urban gardens creates potential pollution hotspots. Environ. Res. Commun. 1, 091007. doi: 10.1088/2515-7620/ab3b8c
Smith, D., Owens, P., Leytem, A., and Warnemuende, E. (2007). Nutrient losses from manure and fertilizer applications as impacted by time to first runoff event. Environ. Pollut. 147, 131–137. doi: 10.1016/j.envpol.2006.08.021
Stewart, R., Korth, M., Langer, L., Rafferty, S., Da Silva, N. R., and van Rooyen, C. (2013). What are the impacts of urban agriculture programs on food security in low and middle-income countries? Environ. Evid. 2, 1–13. doi: 10.1186/2047-2382-2-7
Stutter, M. I., Chardon, W. J., and Kronvang, B. (2012). Riparian buffer strips as a multifunctional management tool in agricultural landscapes: introduction. J. Environ. Qual. 41, 297–303. doi: 10.2134/jeq2011.0439
Tadesse, S. T., Oenema, O., van Beek, C., and Ocho, F. L. (2018). Diversity and nutrient balances of urban and peri-urban farms in Ethiopia. Nutr. Cycl. Agroecosyst. 111, 1–18. doi: 10.1007/s10705-018-9911-0
Tank, F. (2016). Urban Agriculture. FAO. Available online at: http://www.fao.org/family-farming/detail/en/c/461898/ (accessed December 01, 2021).
Van Beek, C., Brouwer, L., and Oenema, O. (2003). The use of farmgate balances and soil surface balances as estimator for nitrogen leaching to surface water. Nutr. Cycl. Agroecosyst. 67, 233–244. doi: 10.1023/B:FRES.0000003619.50198.55
Velasco, J. L., Rozas, H. S., Echeverría, H. E., and Barbieri, P. A. (2012). Optimizing fertilizer nitrogen use efficiency by intensively managed spring wheat in humid regions: effect of split application. Can. J. Plant Sci. 92, 847–856. doi: 10.4141/cjps2011-146
Velthof, G. L., Oudendag, D., Witzke, H. P., Asman, W. A. H., Klimont, Z., and Oenema, O. (2009). Integrated assessment of nitrogen losses from agriculture in EU-27 using MITERRA-EUROPE. J. Environ. Qual. 38, 402–417. doi: 10.2134/jeq2008.0108
Viljoen, A., and Wiskerke, J. S. (2012). Sustainable Food Planning: Evolving Theory and Practice. Wageningen, Netherlands: Wageningen. doi: 10.3920/978-90-8686-187-3
Wang, H.-J., Huang, B., Shi, X.-Z., Darilek, J. L., Yu, D.-S., Sun, W.-X., et al. (2008). Major nutrient balances in small-scale vegetable farming systems in peri-urban areas in China. Nutr. Cycl. Agroecosyst. 81, 203–218. doi: 10.1007/s10705-007-9157-8
Wang, X., Zou, C., Gao, X., Guan, X., Zhang, Y., Shi, X., et al. (2018). Nitrate leaching from open-field and greenhouse vegetable systems in China: a meta-analysis. Environ. Sci. Pollut. Res. 25, 31007–31016. doi: 10.1007/s11356-018-3082-z
Werner, S., Akoto-Danso, E. K., Manka'abusi, D., Steiner, C., Haering, V., Nyarko, G., et al. (2019). Nutrient balances with wastewater irrigation and biochar application in urban agriculture of Northern Ghana. Nutr. Cycl. Agroecosyst. 115, 249–262. doi: 10.1007/s10705-019-09989-w
Westerman, P., and Bicudo, J. (2005). Management considerations for organic waste use in agriculture. Bioresour. Technol. 96, 215–221. doi: 10.1016/j.biortech.2004.05.011
Whittinghill, L. J., Hsueh, D., Culligan, P., and Plunz, R. (2016). Stormwater performance of a full scale rooftop farm: Runoff water quality. Ecol. Eng. 91, 195–206. doi: 10.1016/j.ecoleng.2016.01.047
Wielemaker, R., Oenema, O., Zeeman, G., and Weijma, J. (2019). Fertile cities: nutrient management practices in urban agriculture. Sci. Total Environ. 668, 1277–1288. doi: 10.1016/j.scitotenv.2019.02.424
Wielemaker, R. C., Weijma, J., and Zeeman, G. (2018). Harvest to harvest: recovering nutrients with New Sanitation systems for reuse in Urban Agriculture. Resour. Conserv. Recycl. 128:426–437. doi: 10.1016/j.resconrec.2016.09.015
Yuan, Y., Bingner, R. L., and Locke, M. A. (2009). A review of effectiveness of vegetative buffers on sediment trapping in agricultural areas. Ecohydrol. Ecosyst. Land Water Process Interact. Ecohydrogeomorphol. 2, 321–336. doi: 10.1002/eco.82
Zareba, A., Krzemińska, A., and Kozik, R. (2021). Urban vertical farming as an example of nature-based solutions supporting a healthy society living in the urban environment. Resources 10, 109. doi: 10.3390/resources10110109
Keywords: water quality, nutrient balance, nutrient cycling, circular economy, nutrient losses
Citation: van de Vlasakker PCH, Tonderski K and Metson GS (2022) A Review of Nutrient Losses to Waters From Soil- and Ground-Based Urban Agriculture—More Nutrient Balances Than Measurements. Front. Sustain. Food Syst. 6:842930. doi: 10.3389/fsufs.2022.842930
Received: 24 December 2021; Accepted: 21 February 2022;
Published: 25 March 2022.
Edited by:
Michael Martin, Swedish Environmental Research Institute (IVL), SwedenReviewed by:
Martí Rufí-Salís, Institut de Ciència i Tecnologia Ambientals (ICTA), SpainHenrik Haller, Mid Sweden University, Sweden
Copyright © 2022 van de Vlasakker, Tonderski and Metson. This is an open-access article distributed under the terms of the Creative Commons Attribution License (CC BY). The use, distribution or reproduction in other forums is permitted, provided the original author(s) and the copyright owner(s) are credited and that the original publication in this journal is cited, in accordance with accepted academic practice. No use, distribution or reproduction is permitted which does not comply with these terms.
*Correspondence: Paulien C. H. van de Vlasakker, cGF1bGllbi52YW4uZGUudmxhc2Fra2VyJiN4MDAwNDA7bGl1LnNl