- 1Department of Biotechnology, Graduate School of Engineering, Osaka University, Suita, Japan
- 2School of Life Sciences and Technology, Institut Teknologi Bandung, Bandung, Indonesia
- 3Center of Remote Sensing, Institut Teknologi Bandung, Bandung, Indonesia
- 4Industrial Biotechnology Division, Institute for Open and Transdisciplinary Research Initiatives, Osaka University, Suita, Japan
- 5Osaka University-Shimadzu Omics Innovation Research Laboratories, Osaka University, Suita, Japan
Banana (Musa acuminata) is one of the most important crop plants consumed in many countries. However, the commercial value decreases during storage and transportation. To maintain fruit quality, postharvest technologies have been developed. Storage at low temperature is a common method to prolong the shelf life of food products, especially during transportation and distribution. Another emerging approach is the use of chitosan biopolymer as an edible coating, which can extend the shelf life of fruit by preventing moisture and aroma loss, and inhibiting oxygen penetration into the plant tissue. Gas chromatography-mass spectrometry metabolite profiling of the banana ripening process was performed to clarify the global metabolism changes in banana after chitosan coating or storage at low temperature. Both postharvest treatments were effective in delaying banana ripening. Interestingly, principal component analysis and orthogonal projection to latent structure regression analysis revealed significant differences of both treatments in the metabolite changes, indicating that the mechanism of prolonging the banana shelf life may be different. Chitosan (1.25% w/v) treatment stored for 11 days resulted in a distinct accumulation of 1-aminocyclopropane-1-carboxylic acid metabolite, an important precursor of ethylene that is responsible for the climacteric fruit ripening process. Low temperature (LT, 14 ± 1°C) treatment stored for 9 days resulted in higher levels of putrescine, a polyamine that responds to plant stress, at the end of ripening days. The findings clarify how chitosan delays fruit ripening and provides a deeper understanding of how storage at low temperature affects banana metabolism. The results may aid in more effective development of banana postharvest strategies.
Introduction
Fruit ripening is a complex process influenced by numerous biotic and abiotic factors, including light, hormones, temperature, and genotype. Fruit comprises two different groups according to its behavior upon ripening: climacteric and non-climacteric. Climacteric fruits, such as banana (Musa acuminata), mango, and mangosteen, are characterized by a burst of ethylene production and cell respiration. Ripening-associated events in climacteric fruit lead to biochemical and physiological changes that ultimately affect color, texture, flavor, and aroma changes (Silva et al., 2009; Lerslerwong et al., 2013; Asif et al., 2014; White et al., 2016). Better understanding of the fruit ripening process may help to evaluate the optimal maturity and fruit quality, and reduce postharvest fruit losses.
Banana is consumed worldwide thus considered as an important fruit in terms of its potential as a functional and nutraceutical food. As a climacteric fruit, the physiochemical changes that occur during banana ripening occur rapidly, resulting in color changes and notably perishable fruit after storage at room temperature. Banana peel color is a major criterion of the maturity and the grade of fruit maturity. In the fresh fruit market, bananas are usually harvested when the peel color is deep green or recognizable as a fully green mature ripe grade. Later, the peel color changes to yellow, which is preferable by consumers. Because bananas have a relatively short shelf life, postharvest packaging, and handling strategies are necessary to achieve optimal fruit maturity and to maintain quality (Lustriane et al., 2018).
Postharvest technology development is one of the most important processes affecting food quality. Several postharvest technologies, which include low temperature and modified atmosphere packaging, have been extensively developed to achieve the most favorable conditions for quality improvement or shelf life improvement in several climacteric fruits, such as bananas, plums, and peaches (Facundo et al., 2012; Valero et al., 2013; Yinzhe, 2013; García et al., 2014; Kumar Raghav et al., 2016; Brizzolara et al., 2018). However, these technologies are expensive and energy intensive. Edible coating is an alternative method that can extend the shelf life of fruit owing to its ability to prevent moisture loss, aroma loss, and inhibit oxygen penetration into the plant tissue. Refined methods of edible coatings have received much attention recently because they are less expensive, simple, and biodegradable, for example tragacanth gum, carboxymethyl cellulose, and corn zein, that has been applied on apricots, mangoes, nectarines and apples, respectively (Ali et al., 2020, 2021; Mendes-Oliveira et al., 2022). Chitosan is a promising biopolymer for edible coating. It is a linear polysaccharide consisting of β-(1→4)-linked 2-amino-2-deoxy-D-glucose residues, which is a deacetylated derivative of chitin, the second most abundant polysaccharide in nature after cellulose, and whose many sources include exoskeletons of crustaceans, insects, mollusks, and fungi. Chitosan has many benefits for agriculture, food, and medicine because of its many functional properties that include antioxidant, antimicrobial activities, and ability to form a film. It can reduce the respiration rate of fruit by forming a coating on the fruit and adjusting the permeability of carbon dioxide and oxygen (Jianglian, 2013; Luo and Wang, 2013). Although the effect of chitosan on fruit storage has been confirmed through chemical and physical analyses, only a few studies on its mechanism to delay fruit ripening have been performed. Existing studies on banana postharvest technologies have focused on physiochemical parameters or targeting only a few processes related to color changes, texture, and enzymatic assays in the peel and pulp parts.
In recent years, metabolomics has been widely used as an important tool to support postharvest fruit development and ripening studies, including sapodilla, litchi, and peach (Das and De, 2015; Bustamante et al., 2016; Yun et al., 2016; Brizzolara et al., 2018). To better elucidate the complex mechanism of metabolic response during postharvest technologies in banana, we performed gas chromatography-mass spectrometry (GC-MS) metabolite profiling of the banana ripening process. We also compared the effects of different postharvest treatments on the banana ripening process. The treatments used were room temperature (RT, 27 ± 3.4°C) as a control, chitosan treatment (C), and low temperature (LT, 14 ± 1°C). Multivariate analysis was performed to correlate specific metabolites with color changes that occurred during banana postharvest treatment. This is the first report of a metabolomics approach to understand the effects of postharvest treatment in banana.
Materials and Methods
Plant Materials
A batch of mature green bananas (M. acuminata, AAA group, Cavendish subgroup) from Indonesia with maturity indices stage 2 based on United States Department of Agriculture (https://www.ams.usda.gov/sites/default/files/media/Bananas_Visual_Aid%5B1%5D.pdf) was used in this study. Banana samples were visually inspected for uniformity in size, color, and absence of physical damage and fungal infection before they were selected as the samples used in this experiment.
Temperature Treatment
The aforementioned LT and control (RT) storage conditions were examined. This postharvest LT treatment was previously reported as an effective treatment to prolong shelf life (Piriyavinit et al., 2011; Mustafa et al., 2018). For temperature treatment, the fruits were stored at RT or LT for 10 days and were randomly collected on days 0, 1, 3, 5, 7, and 9 for examination. Three fruits were collected at each day of sampling and quickly quenched in liquid nitrogen and stored at −20°C prior to extraction and homogenization.
Chitosan Coating
Food grade chitosan (high molecular weight, 85–89% deacetylated) purchased from Biotech Surindo (Cirebon, Indonesia) was used as an edible banana coating. A 1.25% (w/v) chitosan solution was prepared in acetic acid by adding the appropriate amount of chitosan and stirring. The pH was adjusted to 5.5 with 1 M NaOH. Bananas were coated with chitosan by immersion in the solution for 2 min, air-dried at RT, and stored at RT (27 ± 3.4°C). Three bananas were inspected and collected at days 0, 1, 3, 5, 7, 9, and 11. The changes in peel color were documented by taking photographs at each observation time.
Total Soluble Solids Measurement, Metabolite Extraction, and Derivatization
The total soluble solids (TSS) content of banana fruit was determined by using a refractometer (ATAGOⓇ N-1α, Tokyo, Japan) and performed as described before by Pratiwi (Sa Pratiwi et al., 2015). Results were expressed as degree Brix (°Brix). Briefly, 15 g banana fruit pulp in each treatment was homogenized using a blender with 45 mL of distilled water. The mixture was centrifuged at 14,000 rpm for 5 min. A few drops of the filtrate were then placed on the prism of the refractometer before reading. The refractometer was calibrated with distilled water to give a 0 °Brix reading at each measurement. The extraction and derivatization procedures were performed as previously described with slight modifications (Ikram et al., 2020). Briefly, the middle part (~2 cm) of banana was cut, separated into pulp and peel, and quenched with liquid nitrogen on days 0, 1, 3, 5, 7, 9, and 11. The cut bananas were ground into powder using a mortar or multi-bead shocker (Yasui Kikai, Osaka, Japan). Ten milligrams of powdered banana was mixed with 1 mL of the extraction solvent, which was methanol (Wako Chemical, Osaka, Japan), chloroform (Kishida Chemical Co., Ltd., Osaka, Japan), or ultrapure water (Wako Chemical) at a ratio of 2.5/1/1 (v/v/v) together with an internal standard (Ribitol 20, mg/mL) by vortexing. The samples were incubated at 37°C and 1,200 rpm for 30 min. Centrifugation was performed at 4°C and 10,000 rpm for 3 min, and 600 μL of the supernatant was transferred into a 1.5 mL microtube. Ultrapure water (300 μL) was added and vortexed to increase the separation between the polar and non-polar phases. The combined supernatant of all samples was centrifuged for 3 min at 4°C and 10,000 rpm. The supernatant was transferred and evaporated in a spin dryer (Taitec, Aichi, Japan) for 1.5 h at RT. The evaporated samples were lyophilized overnight in a freeze dryer (Taitec). Methoxyamine hydrochloride (100 μL, 20 mg/ml in pyridine) was added to each sample and incubated at 30°C and 1,200 rpm for 90 min. Subsequently, 50 μL N-methyl-N-trimethylsilyl—trifluoroacetamide (MSTFA; GL Science, Tokyo, Japan) was added and incubated at 37°C and 1,200 rpm for 30 min before GC/MS analysis.
GC-MS Analysis
An Ultra QP-2010 gas chromatography coupled with a mass spectrometer and an AOC-20i/s auto-injector was used for analysis (Shimadzu, Kyoto, Japan). The mass spectrometer was tuned and calibrated prior to the analysis. One microliter of derivatized sample was injected in the split mode, with a ratio of 11/1 (v/v) and an injection temperature of 230°C. The flow rate of the helium carrier gas was 1.12 mL/min with a linear velocity of 39.0 cm/s. The column temperature was held at 80°C for 2 min, raised to 330°C at a rate of 15°C/min, and then maintained at 330°C for 6 min. The transfer line and ion-source temperatures were 250 and 200°C, respectively. Ions were generated via electron ionization at 70 eV. Spectra were recorded using at 20 scans/s over a mass range of 85–500 m/z. The detection voltage was automatically set after auto-tuning for each analysis batch. A standard alkane mixture (C8-C40) was injected at the beginning of the analysis for tentative identification.
Data Analyses
The raw GC-MS data were processed using the GC-MS solution software package (Shimadzu, Kyoto, Japan). Peak alignment was executed using MetAlign (Wagenigen; download for free at http://www.wageningenur.nl/). The pre-processed data were then subjected to peak annotation using AIOutput and our laboratory's in-house library made with authentic standards. In addition to the in-house library, 1-aminocyclopropane-1-carboxylic acid (ACC) peak was confirmed by co-injection with an authentic standard (Sigma-Aldrich Japan Ltd., Tokyo, Japan). Multivariate analysis was performed using SIMCA-P+ version 13 (Umetricts, Umea, Sweden) for principal component analysis (PCA) and orthogonal projections to latent structures (OPLS) without data transformation to observe data patterns based on their differences and similarities. The data were auto-scaled to reduce the mask effect from the abundant metabolites. PCA was used as a non-supervised multivariate analysis of banana ripening stages, which can clarify the understanding of the relationship between the samples and the metabolite features. OPLS analysis is a supervised multivariate analysis that is commonly used to predict significant metabolites using observed values, such as color changes. In the present study, OPLS was used to describe several variables of PCA and was projected to regression or discriminant using the explanatory variable. From OPLS analysis, variable important in projection (VIP) score can be calculated based on the root of the value taken after the sum over all model dimensions of contribution (variable influence) is divided by the total explained sum of squares X and sum of square Y by the OPLS model and is multiplied by the number of terms in the model (Galindo-Prieto et al., 2014).
Result
Ripening Process of Banana
Metabolic changes during the banana ripening process were investigated using GC-MS analysis. Three fruits per observation day were used. The analysis was performed separately for the peel and pulp portions of banana. We tentatively identified 99 metabolites from the pulp and 96 metabolites from the peel using the National Institute of Standards and Technology and our in-house library (Supplementary Table 1). Two PCA models of the GCMS-derived dataset were used. The first was based on the fruit pulp samples and the second was based on the peel samples. The first two principal components (PCs) within each model provided a total explained variance of 39.2 and 41%, respectively (Figure 1). The PCA was designed to provide an overview of all observations or samples in a dataset (Fujimura et al., 2011). In PCA analysis, the data were rearranged into a new axis (coordinate) termed PC. PC should be created to maximize the variance among samples. The greatest variance would be explained by PC1, the second largest variance by PC2, and so on. The results showed that the trend of PC1 described the metabolite change trends during banana ripening from mature green or raw stages to overripe stages with senescence spots appearing during observation.
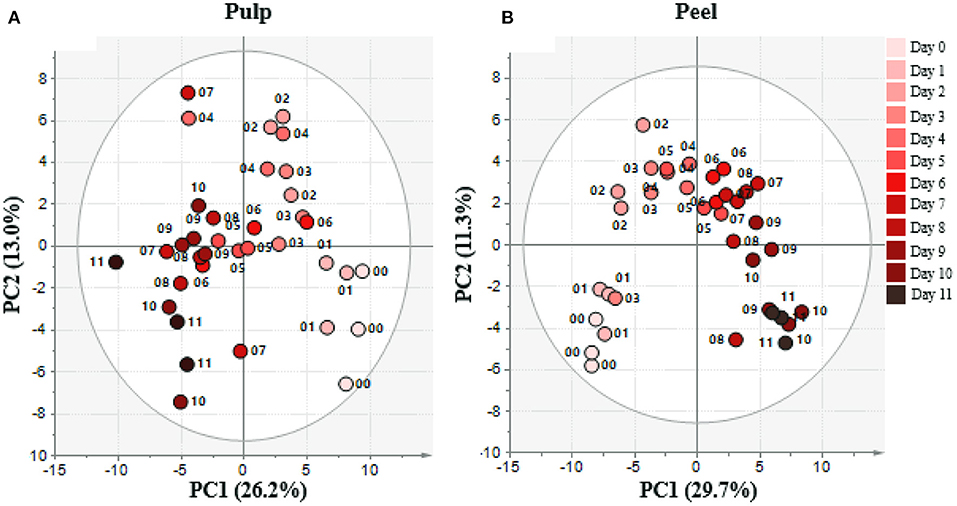
Figure 1. PCA plot of day 0–11 samples of (A) pulp and (B) peel from metabolome data sets during banana ripening. The data were analyzed using SIMCA-P+ version 13. Metabolome data was obtained by GC/MS analysis and processed and annotated using MetAlign and AIOutput (Scaling: auto-scale, transformation: none). Numbers represent day of sampling. Colors from light-red to dark-red represents the ripening stage.
OPLS analysis was used to regress PCA variables (metabolite intensity) on the number of days. This allowed us to verify whether the number of days could accurately predict metabolite-based ripening stages. OPLS analysis was conducted using the number of days as the response variable (y-variable) and metabolite peak intensity values as the predictor variables (x-variables) with the number of latent variables used in this study being two. Representative samples of one batch of ripening processes were selected as a training set. Representative samples from different batches were used as a dataset to validate the prediction model. OPLS analysis was performed in both the pulp and peel ripening processes to confirm the robustness of the prediction model. The constructed models displayed a linear coefficient (R2) and prediction ability (Q2) > 0.5 (Figure 2). High R2 and Q2 values indicate an excellent predictive model. To verify our results, we added the test set into the model in which they fit perfectly in the predicted regression line. Moreover, the root mean square error (RMSE) was calculated to determine how well the observed ripening stages matched the actual ripening stages of banana. The RMSE of estimation (RMSEE) was 1.17 in pulp and 0.55 in peel. The values did not deviate distinctly from the RMSE of prediction (RMSEP) value of 1.09 in pulp and 0.59 in peel. The findings indicated the validity of the regression model. The collective results indicated the successful development of a prediction model for the banana ripening process based on metabolomic data. Contributing metabolites were selected based on the five highest variable importance in projection (VIP) scores obtained from the PLS model. Among the 99 annotated metabolites, 10 were important in our prediction model the pulp and peel of banana. The metabolites that changed during the banana ripening process in the pulp were xylonic acid, propylene glycol, 2-aminoethanol, galactose+glucose, and 2-isopropylmalic acid. Those that changed in the peel part were valine, cinnamyl alcohol, acetoacetic acid, galacturonic acid, and meso erythritol.
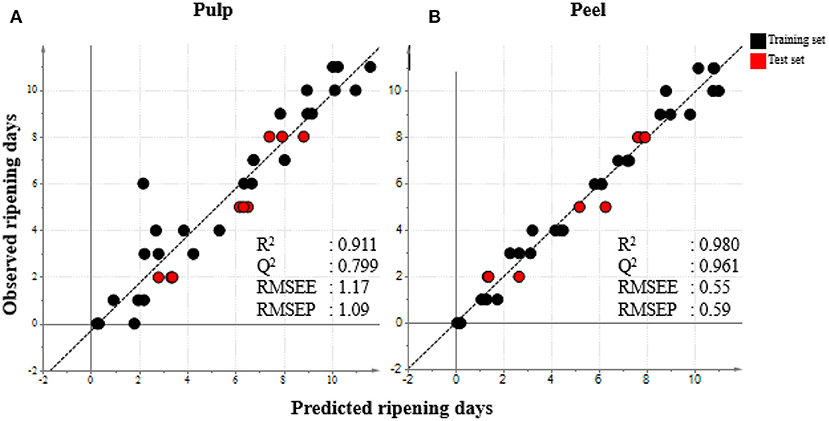
Figure 2. PLS regression plot of day 0–11 samples from metabolome data sets of (A) pulp and (B) peel during the banana ripening process. The data were analyzed using SIMCA-P+ version 13. Metabolome data was obtained by GC/MS analysis and processed and annotated using MetAlign and AIOutput. The explanatory and response variable was metabolites and number of days, respectively. Numbers represents days of sampling. Black color represents samples used to construct the model (training set). Red color represents test set samples.
Effect of LT Treatment on Banana
The LT and RT treatments were evaluated during 9 days of storage (Figure 3). Differences in these treatments were evaluated using a metabolomics approach to confirm the metabolite changes. A total of 70 and 66 metabolites were annotated in the pulp and peel of banana, respectively (Supplementary Table 2). After GC-MS analysis, the data sets of RT and LT treatments were subjected to multivariate statistical analysis. PCA revealed two PCs explaining 43.3 and 37.4% of metabolite variation in pulp and peel, respectively, between the two temperature treatments (Figure 4A and Supplementary Figure 1). PC1 describes the difference in metabolite change trends between RT and LT treatments from the raw to ripening stages in the pulp part (Figure 4A). As can be seen in the PCA score plot of pulp, LT treatment produced markedly different metabolite changes from RT. This indicates that LT treatment was effective in prolonging banana shelf life by halting the increase in ripening-associated metabolites. Thus, LT prevented metabolic changes. This trend was also confirmed in the OPLS regression analysis, which showed that LT treatment halted metabolites related to the ripening process. The predicted ripening days were up until day 5 or 6 at LT and until day 9 at RT (Figure 4B). Similar to pulp, peel displayed the same trend of metabolic changes during treatment.
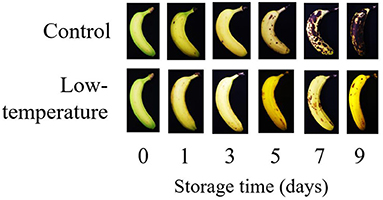
Figure 3. Picture of banana ripening process under control condition (room temperature 27 ± 3.4°C) and low temperature condition (LT, 14 ± 1°C) during nine days of storage time.
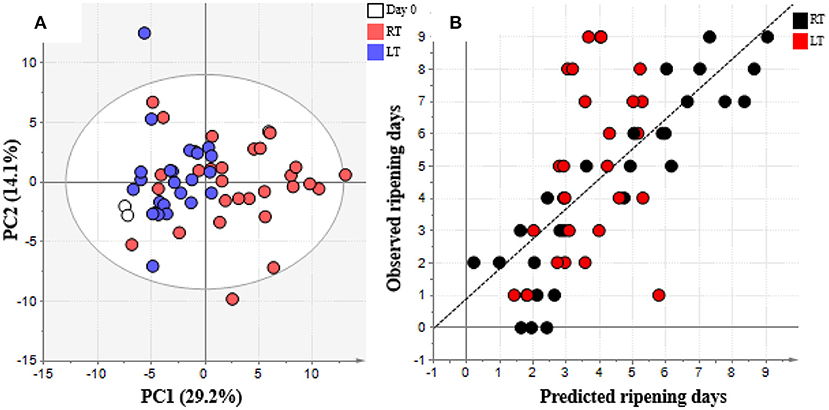
Figure 4. (A) PCA plot and (B) OPLS regression model of day 0–9 samples from pulp of banana treated in LT metabolome data sets analyzed using SIMCA-P+ version 13. Metabolome data was obtained by GC/MS analysis and processed and annotated using MetAlign and AIoutput (Scaling: auto-scale, transformation: none). In (A), white represents day 0, red represents room temperature (RT), blue represents low temperature (LT). In (B), black represents RT and red represents LT.
Effect of Chitosan Coating on Banana
Banana ripening process after coated with chitosan compared to control stored for 11 days were showed in Figure 5. The processed GC/MS data set of bananas treated with chitosan with 100 annotated metabolites from pulp and 101 annotated metabolites from peel were subjected to multivariate data analysis (Supplementary Table 3). The PCA score plot of chitosan treated banana data sets on pulp and peel parts are shown in Figure 6A and Supplementary Figure 2, respectively. The PC1 and PC2 vectors varied by 34.5 and 13.6%, respectively. Along with the PC1 vector, both uncoated and chitosan-coated banana samples were aligned in PC1, which describes the ripening process. This result indicates that the ripening process was the major factor for the data variance. Chitosan-coated samples were plotted on the negative side more than the uncoated samples in PC1, compared to samples from the same maturation stage. This finding suggests that metabolite changes during the ripening process were successfully observed, and that these changes were delayed in chitosan-coated banana. The delayed ripening process of chitosan treated bananas was confirmed by total soluble solids (TSS) changes (Supplementary Figure 3) and OPLS regression (Figure 6). TSS values showed to be increasing along the ripening process. Compared to control, chitosan-coated sample showed lower TSS values that indicate slower ripening process. For OPLS regression analysis, using chitosan treated banana as the test set and uncoated banana as the training set to create the model, we observed that the chitosan treated banana ripening process was shifted to the left. The finding means that the predicted ripening days were delayed compared to the uncoated condition (Figure 6B). On the other hand, PC2 of the PCA plot (Figure 6A) implied differences between the uncoated and chitosan-coated groups. This result suggests that the ripening of chitosan-coated banana was different from that of the normal (uncoated) banana. Since the samples on day 11 from chitosan-coated banana were especially clustered away from uncoated samples, it is important to understand which metabolites contributed to this separation. A similar trend in PCA and OPLS regression was also observed in the peel of chitosan treated banana.
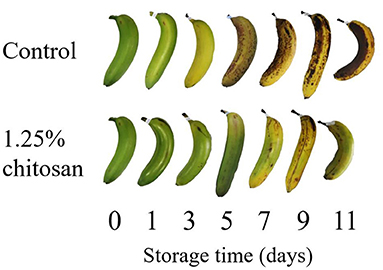
Figure 5. Picture of banana ripening process under control condition (untreated) and chitosan-treated condition (1.25% chitosan) during 11 days of storage time.
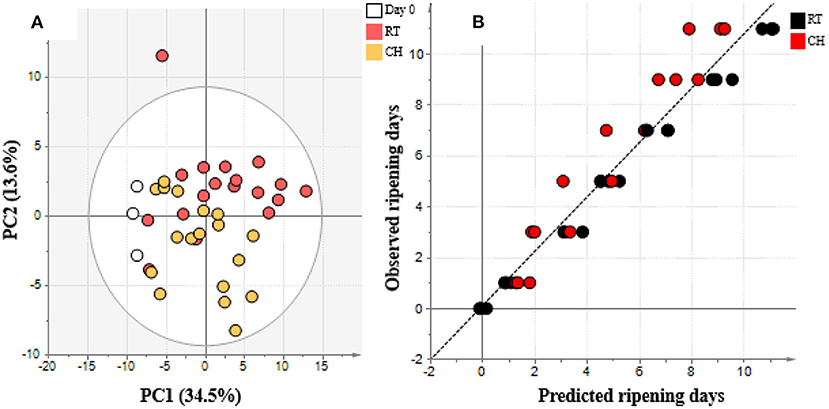
Figure 6. (A) PCA plot and (B) OPLS regression model of day 0–11 samples from pulp of banana treated by chitosan metabolome data sets analyze using SIMCA-P+ version 13. Metabolome data was obtained by GC/MS analysis and processed and annotated using MetAlign and AIoutput (Scaling: auto-scale, transformation: none). In (A) the white color represents day 0, the red color represents room temperature (RT), and yellow color represents chitosan treatment (CH). In (B), black represents RT and red represents CH.
To evaluate the differences between the last day of observations in both LT and CH treated banana, especially the pulp portion that is consumed, OPLS-discriminant analysis (OPLS-DA) was performed. The explanatory variables used were metabolites obtained from GC/MS. The response variable was set to “0” for control or untreated banana and “1” for LT or CH treated banana. Figure 7 is the plot of OPLS-DA for control and untreated pulp with LT treatment (Figure 7A) and CH treatment (Figure 7B). Both OPLS-DA plots showed that these treatments were significantly different from the control. The metabolites that were affected by the treatment are shown in Table 1. LT treatment affected putrescine, β-alanine, and malic acid. CH treatment affected 1-aminocyclopropane-1-carboxylic acid (ACC), glycine, and α-ketoglutaric acid. The VIP score indicates the degree of importance for the metabolites during the model construction, and the coefficient indicating its positive (similarly) or negative (differently) correlated compared to the control treatment. The relative abundance of these VIP metabolites in the control untreated, LT treated, and CH treated banana are shown in Figure 8.
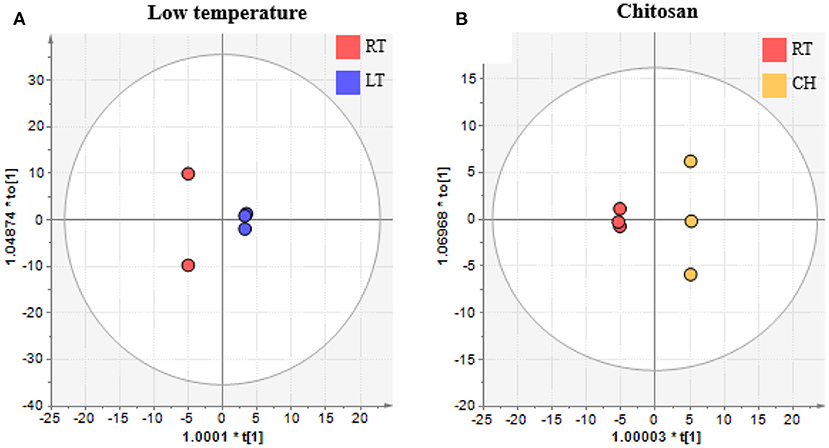
Figure 7. OPLS-DA score plot from metabolome data sets of banana pulp treated with (A) low temperature and (B) chitosan. Data were analyzed using SIMCA-P+ version 13. Metabolome data was obtained by GC/MS analysis and processed and annotated using MetAlign and AIoutput. Red color represents room temperature (RT), blue color represents low temperature (LT), and yellow represents chitosan treatment (CH).

Table 1. Top three variable important in projection metabolites from OPLS-DA of banana pulp treated by low temperature and chitosan.
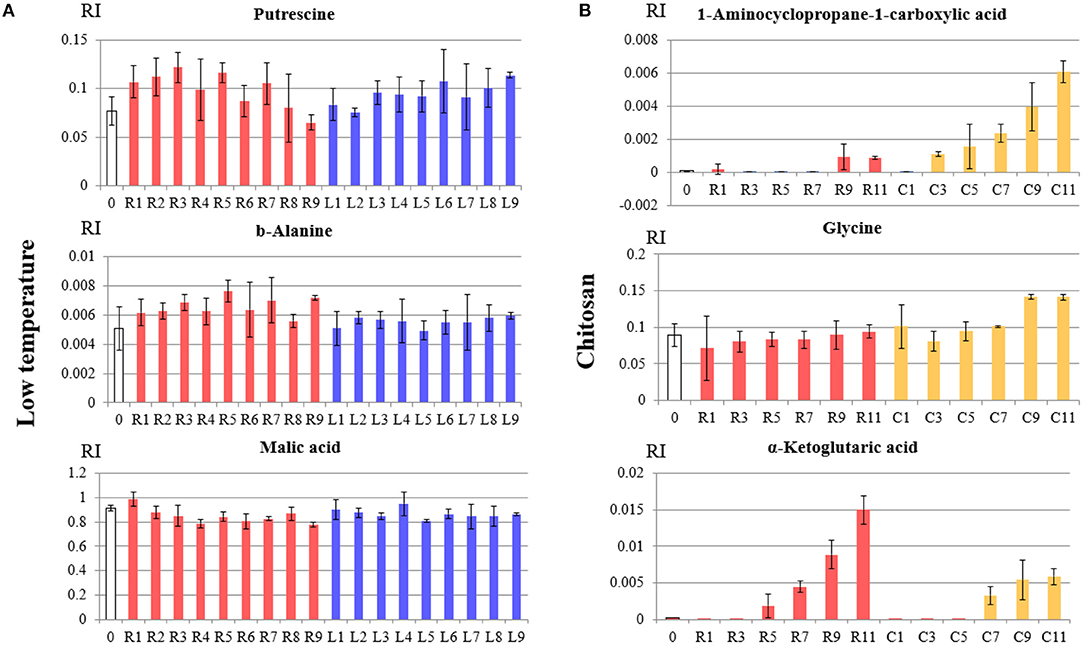
Figure 8. Bar graph of variable important in projection metabolites between (A) room temperature vs. low temperature treatment, and (B) untreated vs. chitosan treated banana pulp. The vertical axis represents the relative intensity of metabolites from GC/MS. The horizontal axis represents the treatment and number of days. White (0) represents day 0, red color (R) represents room temperature and untreated banana, blue (L) represents low temperature treatment, and yellow color (Y) represents chitosan treatment.
Discussion
Banana is a climacteric fruit that continues to ripen after harvest (Dwivany et al., 2020). In this study, the banana ripening process was evaluated in banana pulp and peel. The metabolites that affected the pulp during the banana ripening process were xylonic acid, propylene glycol, 2-aminoethanol, galactose+glucose, and 2-isopropylmalic acid. Effects on peel were evident for valine, cinnamyl alcohol, acetoacetic acid, galacturonic acid, and meso erythritol. Xylonic acid is associated with the plant cell walls. Xylonic acid is a constituent of hemicellulose in pomegranate; thus, its involvement in the banana ripening process might indicate cell wall modification during ripening (Deng et al., 2021). The other accumulated metabolite during banana ripening was propylene glycol. This metabolite is a well-known gas used to accelerate the ripening process of fruit. These results can be used as a basis to indicate the ripening process of banana and can provide useful feedback for postharvest technology studies.
Ethanolamine, also known as 2-aminoethanol, is an important plant metabolite that is related to the production of choline and membrane lipids, such as phosphatidylethanolamine and phosphatidylcholine (Kwon et al., 2012). The importance of ethanolamine also confirms previous results in mangosteen (Parijadi, 2018). Moreover, galacturonic acid and galactose reportedly to contribute to cell wall degradation, which affects fruit softening during the ripening process (Burana-osot et al., 2010). 2-Isopropylmalic acid is present in tropical fruits, such as melon, banana, and pear (Vázquez-Manjarrez et al., 2020). The ripening process is related to a senescence process that correlates with hormones that are influenced by biotic or abiotic stresses, such as ethylene and melatonin (Zhao et al., 2021). The observed accumulation of 2-isopropylmalic acid during ripening confirms previous results of an increase in the intensity of 2-isopropylmalic acid in sugar beet during salt-stressed conditions (Liu et al., 2020).
Other than galacturonic acid, which has been described previously, another important metabolite during the ripening process in peel is valine. Valine is a proposed precursor of 2-methylpropyl, a major volatile compound in banana. Valine might be responsible for the aroma development during banana ripening (Alsmairat et al., 2018). Similar to valine, cinnamyl alcohol is the substrate for acyl transferase in banana, an enzyme responsible for ester formation. Cinnamyl alcohol might also contribute to aroma development in banana (Beekwilder et al., 2004). While these two metabolites are related to the production of aroma in banana, acetoacetic acid might indicate the oxidation of flavoring substances during banana ripening (Saito et al., 2017). On the other hand, meso erythritol might not be related to the aroma compound in banana, but rather may be a protective or growth accelerating agent. Previous studies have reported the effectiveness of erythritol in killing certain insects, such as Drosophila melanogaster (Scanga et al., 2018). In addition, erythritol shortens the germination time in some plants, including white radish, garlic, and Arabidopsis (Kuroda et al., 2008). Thus, erythritol might serve as a sweetener and also to regulate plant development and protect against stress.
In the present study, to more comprehensively understand the mechanism to delay banana ripening through postharvest treatment, we compared the current standard LT treatment with the emerging method of chitosan coating. The two treatments displayed similar effects and similarly delayed ripening of banana peel and pulp. Both treatments were able to reduce the rate of banana ripening process as shown in the peel color changes of banana for LT treatment (Figure 3) and chitosan treatment (Figure 5). For LT treatment, day 3 banana showed similar level of ripening stage with control (stage 5 yellow with green tips). However, the deterioration was significantly different as shown in day 9 (Figure 3). For chitosan treatment, the uncoated banana on day 3 was on stage 5 (yellow with green tips), while the coated banana was on stage 2 (light green) (Figure 5). While on day 9, the uncoated banana had already started to decay while the banana treated with chitosan was still on stage 4 (more yellow than green) (Figure 5). Different days of observation were used (day 9 for LT, day 11 for chitosan treatment) based on the browning level of control banana (Figures 3, 5). Despite the difference in storage days, these treatments were able to affect the metabolite intensity in the banana, thus shortening the predicted ripening days compared to control and untreated banana, or rendering banana less ripe based on its metabolite profile (Figure 4B).
OPLS-DA was performed to determine the process behind the LT and chitosan delay of banana ripening. Metabolites that were affected by the LT compared to the control condition were putrescine, β-alanine, and malic acid. Metabolites that were affected by chitosan treatment were ACC, glycine, and α-ketoglutaric acid. The different metabolites that were affected by these treatments indicates different regulatory mechanisms between the LT and chitosan delay of ripening. Of the metabolites affected by LT, putrescine can regulate plant senescence and response to stress (Takeda et al., 1997). Putrescine has also been correlated with the ripening process of banana; exogenously provided putrescine was able to maintain the shelf life of banana (Takeda et al., 1997; Archana and Suresh, 2019). Moreover, β-alanine was reported to be inhibited in mangosteen during the ripening process under LT conditions (Parijadi, 2018). This inhibition of amino acid biosynthesis, specifically β-alanine, is presumably caused by hypoxic conditions during LT treatment (Parijadi, 2018). The involvement of malic acid in LT treatment also indicates the role of malic acid in the banana ripening process due to its correlation with the sweet acid taste of banana (Parijadi, 2018). The accelerated accumulation of malic acid in banana is evidence of the positive correlation between malic acid and the ripening process (Maduwanthi and Marapana, 2019). In the present study, the difference in accumulation of all metabolites was small (Figure 8A). Nonetheless, this small difference was sufficient to regulate the ripening process and delay ripening.
In contrast to the LT treatment, the accumulation of important metabolites differed between untreated and chitosan treated bananas. The first characteristic metabolite in chitosan treated samples was ACC, a precursor of ethylene in the biosynthesis pathway (Yang and Hoffman, 1984). ACC abundantly accumulated and increased gradually during the ripening process in chitosan-coated banana, and compared to the accumulation of ACC in untreated banana, the accumulation was close to zero up until day 11 (Figure 8B). This might indicate that chitosan treatment regulates the delay of the ripening process by inhibiting ethylene production. In general, amino acids are biostimulants that may induce positive effects on plant growth and mitigate abiotic stress in plants (Zargar Shooshtari et al., 2020). Glycine is a small amino acid that can act as a signaling molecule to increase the activity of antioxidant enzymes (Zargar Shooshtari et al., 2020). A prior study described that the exogenous application of glycine to coriander increased antioxidant activity and other growth parameters, such as plant dry weight and stem diameter (Mohammadipour and Souri, 2019). Another possibility for the role of glycine might be related to Gly betaine, given the prior report of its effectiveness in protecting against abiotic stress (Chen and Murata, 2008). The higher accumulation of α-ketoglutaric acid in untreated banana compared to chitosan treated banana was consistent with previous results that indicated the accumulation of α-ketoglutaric acid during banana ripening process (Yun et al., 2019). The accumulation of α-ketoglutaric acid (also known as 2-ketoglutaric acid and 2-oxoglutarate) might be related to the energy-metabolism pathway in the tricarboxylic acid cycle or the production of gibberellic acid hormone, which participates in fruit ripening, suggesting that chitosan suppressed this effect (Araújo et al., 2014; Xiong et al., 2018). The difference in accumulation of these metabolites was also supported by the changes of TSS values of chitosan-coated banana during the ripening process. TSS values indicated the changes of starch to soluble sugars in ripening process, and lower values of TSS in chitosan-coated banana might indicate slower ripening process compared to control (Dadzie and Orchard, 1997). This result also confirms a previous study that showed slower increase of TSS values during the chitosan-coated banana ripening process (Maqbool et al., 2011).
Finally, the present study is the first to use a GC-MS metabolomics approach to evaluate the effect of LT and chitosan treatments in banana. Both treatments delayed banana ripening by delaying metabolite changes in banana fruit during the ripening process. The treated fruit was less ripe compared to the control condition. However, the treatments might have different mechanisms. LT treatment affects putrescine, β-alanine, and malic acid, which may be an indicator of hypoxia or regulation of plant senescence through polyamines. Chitosan treatment affects ACC, glycine, and α-ketoglutaric acid, which affect hormone-related processes and antioxidant processes. Even though the ripening model was constructed in this study, further improvements can be made by incorporating physicochemical parameters, such as color changes and texture analysis, as response variables. Hypotheses of the role of metabolites in this study also need further confirmation to strengthen the knowledge of the postharvest effect in the banana ripening process. Nonetheless, the results from this study provide a better understanding of LT and chitosan postharvest strategies for banana. The data will inform further research to control the ripening process in banana.
Data Availability Statement
The original contributions presented in the study are included in the article/Supplementary Material, further inquiries can be directed to the corresponding author/s.
Author Contributions
AP, KY, FD, and SP designed the experiments. AP and KY performed the experiments, analyzed the data, and wrote the manuscript. MI and SP analyzed the data and wrote the manuscript. FD, KW, SP, and EF conceived the study and participated in its design and coordination. All authors have read and agreed to the published version of the manuscript.
Funding
This work was supported by the International Research Fund (No: 693/IT1.B07.1/TA.00/2021) with the title of Studi Pasca Panen Pisang Berbeda Genom dengan Pendekatan Multidisiplin Menggunakan Teknologi Remote Sensing dan Omics.
Conflict of Interest
The authors declare that the research was conducted in the absence of any commercial or financial relationships that could be construed as a potential conflict of interest.
Publisher's Note
All claims expressed in this article are solely those of the authors and do not necessarily represent those of their affiliated organizations, or those of the publisher, the editors and the reviewers. Any product that may be evaluated in this article, or claim that may be made by its manufacturer, is not guaranteed or endorsed by the publisher.
Acknowledgments
The authors thank Mr. Akira Kato from Great Giant Foods Japan Co., Ltd., for providing banana samples for this study.
Supplementary Material
The Supplementary Material for this article can be found online at: https://www.frontiersin.org/articles/10.3389/fsufs.2022.835978/full#supplementary-material
References
Ali, S., Anjum, M. A., Nawaz, A., Naz, S., Ejaz, S., Sardar, H., et al. (2020). Tragacanth gum coating modulates oxidative stress and maintains quality of harvested apricot fruits. Int. J. Biol. Macromol. 163, 2439–2447. doi: 10.1016/j.ijbiomac.2020.09.179
Ali, S., Anjum, M. K., Khan, A. S., Nawaz, A., Ejaz, S., Khaliq, G., et al. (2021). Carboxymethyl cellulose coating delays ripening of harvested mango fruits by regulating softening enzymes activities. Food Chem. 131804. doi: 10.1016/j.foodchem.2021.131804
Alsmairat, N., Engelgau, P., and Beaudry, R. (2018). Changes in free amino acid content in the flesh and peel of ‘Cavendish' banana fruit as related to branched-chain ester production, ripening, and senescence. J. Am. Soc. Horticult. Sci. 143, 370–380. doi: 10.21273/JASHS04476-18
Araújo, W. L., Martins, A. O., Fernie, A. R., and Tohge, T. (2014). 2-oxoglutarate: linking TCA cycle function with amino acid, glucosinolate, flavonoid, alkaloid, and gibberellin biosynthesis. Front. Plant Sci. 5:552. doi: 10.3389/fpls.2014.00552
Archana, T. J., and Suresh, G. J. (2019). Putrescine and spermine affects the postharvest storage potential of banana Cv. grand naine. Int. J. Curr. Microbiol. Appl. Sci. 8, 3127–3137. doi: 10.20546/ijcmas.2019.801.334
Asif, M. H., Lakhwani, D., Pathak, S., Gupta, P., Bag, S. K., Nath, P., et al. (2014). Transcriptome analysis of ripe and unripe fruit tissue of banana identifies major metabolic networks involved in fruit ripening process. BMC Plant Biol. 14:316. doi: 10.1186/s12870-014-0316-1
Beekwilder, J., Alvarez-Huerta, M., Neef, E., Verstappen, F. W. A., Bouwmeester, H. J., and Aharoni, A. (2004). Functional characterization of enzymes forming volatile esters from strawberry and banana. Plant Physiol. 135, 1865–1878. doi: 10.1104/pp.104.042580
Brizzolara, S., Hertog, M., Tosetti, R., Nicolai, B., and Tonutti, P. (2018). Metabolic responses to low temperature of three peach fruit cultivars differently sensitive to cold storage. Front. Plant Sci. 9:706. doi: 10.3389/fpls.2018.00706
Burana-osot, J., Soonthornchareonnon, N., Chaidedgumjorn, A., Hosoyama, S., and Toida, T. (2010). Determination of galacturonic acid from pomelo pectin in term of galactose by HPAEC with fluorescence detection. Carbohydr. Polym. 81, 461–465. doi: 10.1016/j.carbpol.2010.03.001
Bustamante, C. A., Monti, L. L., Gabilondo, J., Scossa, F., Valentini, G., Budde, C. O., et al. (2016). Differential metabolic rearrangements after cold storage are correlated with chilling injury resistance of peach fruits. Front. Plant Sci. 7:1478. doi: 10.3389/fpls.2016.01478
Chen, T. H. H., and Murata, N. (2008). Glycinebetaine: an effective protectant against abiotic stress in plants. Trends Plant Sci. 13, 499–505. doi: 10.1016/j.tplants.2008.06.007
Dadzie, B. K., and Orchard, J. E. (1997). Routine Post Harvest Screening of Banana/Plantain Hybrids:Criteria and Methods. Rome: International Plant Genetic Resources Institute.
Das, S., and De, B. (2015). Analyzing changes in metabolite profile during postharvest ripening in achras sapota fruits: GC-MS based metabolomics approach. Int. Food Res. J. 22, 2288–2293. Available online at: http://www.ifrj.upm.edu.my/22%20(06)%202015/(16).pdf
Deng, G., Bi, F., Liu, J., He, W., Li, C., Dong, T., et al. (2021). Transcriptome and metabolome profiling provide insights into molecular mechanism of pseudostem elongation in banana. BMC Plant Biol. 21:125. doi: 10.1186/s12870-021-02899-6
Dwivany, F. M., Aprilyandi, A. N., Suendo, V., and Sukriandi, N. (2020). Carrageenan edible coating application prolongs cavendish banana shelf life. Int. J. Food Sci. 2020. doi: 10.1155/2020/8861610. Available online at: https://downloads.hindawi.com/journals/ijfs/2020/8861610.pdf
Facundo, H. V. V., Garruti, D. S., dos Santos Dias, C. T., Cordenunsi, B. R., and Lajolo, F. M. (2012). Influence of different banana cultivars on volatile compounds during ripening in cold storage. Food Res. Int. 49, 626–633. doi: 10.1016/j.foodres.2012.08.013
Fujimura, Y., Kurihara, K., Ida, M., Kosaka, R., Miura, D., Wariishi, H., et al. (2011). Metabolomics-driven nutraceutical evaluation of diverse green tea cultivars. PLoS One 6:e23426. doi: 10.1371/journal.pone.0023426
Galindo-Prieto, B., Eriksson, L., and Trygg, J. (2014). Variable influence on projection (VIP) for orthogonal projections to latent structures (OPLS). J. Chemometr. 28, 623–632. doi: 10.1002/cem.2627
García, M., Casariego, A., Díaz, R., and Roblejo, L. (2014). Effect of edible chitosan/zeolite coating on tomatoes quality during refrigerated storage. Emirates J. Food Agric. 26, 238–246. doi: 10.9755/ejfa.v26i3.16620
Ikram, M. M. M., Ridwani, S., Putri, S. P., and Fukusaki, E. (2020). GC-MS based metabolite profiling to monitor ripening-specific metabolites in pineapple (Ananas comosus). Metabolites 10, 1–15. doi: 10.3390/metabo10040134
Jianglian, D. (2013). Application of chitosan based coating in fruit and vegetable preservation: a review. J. Food Process Technol. 04, 5–8. doi: 10.4172/2157-7110.1000227
Kumar Raghav, P., Agarwal, N., and Saini, M. (2016). Edible coating of fruits and vegetables: a review. Int. J. Sci. Res. Mod. Ed. I. 188–204. Available online at: http://ijsrme.rdmodernresearch.com/wp-content/uploads/2016/04/24.pdf
Kuroda, K., Hirakawa, S., Suzuki, M., Shinji, K., Ogasa, K., Uraji, T., et al. (2008). Growth acceleration of plants and mushroom by erythritol. Plant Biotechnol. 25, 489–492. doi: 10.5511/plantbiotechnology.25.489
Kwon, Y., Yu, S. I., Lee, H., Yim, J. H., Zhu, J. K., and Lee, B. H. (2012). Arabidopsis serine decarboxylase mutants implicate the roles of ethanolamine in plant growth and development. Int. J. Mol. Sci. 13, 3176–3188. doi: 10.3390/ijms13033176
Lerslerwong, L., Rugkong, A., Imsabai, W., and Ketsa, S. (2013). The harvest period of mangosteen fruit can be extended by chemical control of ripening-a proof of concept study. Sci. Horticult. 157, 13–18. doi: 10.1016/j.scienta.2013.03.027
Liu, L., Wang, B., Liu, D., Zou, C., Wu, P., Wang, Z., et al. (2020). Transcriptomic and metabolomic analyses reveal mechanisms of adaptation to salinity in which carbon and nitrogen metabolism is altered in sugar beet roots. BMC Plant Biol. 20:138. doi: 10.1186/s12870-020-02349-9
Luo, Y., and Wang, Q. (2013). Recent advances of chitosan and its derivatives for novel applications in food science beverages. J. Food Process Beverages 1, 1–13. Available online at: https://www.avensonline.org/wp-content/uploads/JFPB-2332-4104-01-0002.pdf
Lustriane, C., Dwivany, F. M., Suendo, V., and Reza, M. (2018). Effect of chitosan and chitosan-nanoparticles on post harvest quality of banana fruits. J. Plant Biotechnol. 45, 36–44. doi: 10.5010/JPB.2018.45.1.036
Maduwanthi, S. D. T., and Marapana, R. A. U. J. (2019). Comparative study on aroma volatiles, organic acids, and sugars of ambul banana (Musa acuminata, AAB) treated with induced ripening agents. J. Food Qual. 2019:7653154. doi: 10.1155/2019/7653154
Maqbool, M., Ali, A., Alderson, P. G., Zahid, N., and Siddiqui, Y. (2011). Effect of a novel edible composite coating based on gum arabic and chitosan on biochemical and physiological responses of banana fruits during cold storage. J. Agric. Food Chem. 59, 5474–5482. doi: 10.1021/jf200623m
Mendes-Oliveira, G., Gu, G., Luo, Y., Zografos, A., Minas, I., and Nou, X. (2022). Edible and water-soluble corn zein coating impregnated with nisin for listeria monocytogenes reduction on nectarines and apples. Postharvest Biol. Technol. 185:111811. doi: 10.1016/j.postharvbio.2021.111811
Mohammadipour, N., and Souri, M. K. (2019). Effects of different levels of glycine in the nutrient solution on the growth, nutrient composition, and antioxidant activity of coriander (Coriandrum sativum L.). Acta Agrobot. 72, 13–16. doi: 10.5586/aa.1759
Mustafa, M. A., Ali, A., Seymour, G., and Tucker, G. (2018). Delayed pericarp hardening of cold stored mangosteen (Garcinia mangostana L.) upon pre-treatment with the stress hormones methyl jasmonate and salicylic acid. Sci Horticult. 230, 107–116. doi: 10.1016/j.scienta.2017.11.017
Parijadi, A. A. R. (2018). Metabolic Profiling of Garcinia mangostana (Mangosteen): A New Insight into Quality after Postharvest Treatment. Osaka University.
Piriyavinit, P., Ketsa, S., and van Doorn, W. G. (2011). 1-MCP extends the storage and shelf life of mangosteen (Garcinia mangostana L.) fruit. Postharvest Biol. Technol. 61, 15–20. doi: 10.1016/j.postharvbio.2011.02.007
Sa Pratiwi, A., Dwivany, F. M., Larasati, D., Islamia, H. C., and Martien, R. (2015). Effect of chitosan coating and bamboo FSC (fruit storage chamber) to expand banana shelf life. AIP Conf. Proc. 1677:100005. doi: 10.1063/1.4930763
Saito, K., Hasegawa-Baba, Y., Sekiya, F., Hayashi, S. M., Mirokuji, Y., Okamura, H., et al. (2017). Japan Flavour and Fragrance Materials Association's (JFFMA) safety assessment of food-flavouring substances uniquely used in Japan that belong to the class of aliphatic primary alcohols, aldehydes, carboxylic acids, acetals and esters containing additiona. Food Addit. Contam. A Chem. Anal. Control Expo Risk Assess. 34, 1474–1484. doi: 10.1080/19440049.2017.1333160
Scanga, S. E., Hasanspahič, B, Zvorničanin, E., KoŽenjić, J. S, Rahme, A. K., and Shinn-Thomas, J. H. (2018). Erythritol, at insecticidal doses, has harmful effects on two common agricultural crop plants. PLoS One 13:e0192749. doi: 10.1371/journal.pone.0192749
Silva, D. F. P., Salomão, L. C. C., de Siqueira, D. L., Cecon, P. R., and Rocha, A. (2009). Potassium permanganate effects in postharvest conservation of the papaya cultivar sunrise golden. Pesquisa Agropecuária Brasileira 44, 669–675. doi: 10.1590/S0100-204X2009000700003
Takeda, Y., Yoza, K. I., Nogata, Y., Kusumoto, K. I., Voragen, A. G. J., and Ohta, H. (1997). Putrescine accumulation in banana fruit with ripening during storage. Phytochemistry 46, 57–60. doi: 10.1016/S0031-9422(97)00211-2
Valero, D., Díaz-Mula, H. M., Zapata, P. J., Guillén, F., Martínez-Romero, D., Castillo, S., et al. (2013). Effects of alginate edible coating on preserving fruit quality in four plum cultivars during postharvest storage. Postharvest Biol. Technol. 77, 1–6. doi: 10.1016/j.postharvbio.2012.10.011
Vázquez-Manjarrez, N., Vázquez-Manjarrez, N., Vázquez-Manjarrez, N., Ulaszewska, M., Garcia-Aloy, M., Garcia-Aloy, M., et al. (2020). Biomarkers of intake for tropical fruits. Genes Nutr. 15:11. doi: 10.1186/s12263-020-00670-4
White, I. R., Blake, R. S., Taylor, A. J., and Monks, P. S. (2016). Metabolite profiling of the ripening of mangoes Mangifera indica L. Cv. ‘Tommy Atkins' by real-time measurement of volatile organic compounds. Metabolomics 12, 1–11. doi: 10.1007/s11306-016-0973-1
Xiong, R., Tang, H., Xu, M., Zeng, C. B., Peng, Y., He, R., et al. (2018). Transcriptomic analysis of banana in response to phosphorus starvation stress. Agronomy 8:141. doi: 10.3390/agronomy8080141
Yang, S. F., and Hoffman, N. E. (1984). Ethylene biosynthesis and its regulation in higher plants. Annu. Rev. Plant Physiol. 35, 155–189. doi: 10.1146/annurev.pp.35.060184.001103
Yinzhe, Y. (2013). Effect of chitosan coating on preserving character of post-harvest fruit and vegetable: a review. J Food Process Technol. 4, 1–4. doi: 10.4172/2157-7110.1000254
Yun, Z., Li, T., Gao, H., Zhu, H., Gupta, V. K., Jiang, Y., et al. (2019). Integrated transcriptomic, proteomic, and metabolomics analysis reveals peel ripening of harvested banana under natural condition. Biomolecules 9:167. doi: 10.3390/biom9050167
Yun, Z., Qu, H., Wang, H., Zhu, F., Zhang, Z., Duan, Z., et al. (2016). Comparative transcriptome and metabolome provides new insights into the regulatory mechanisms of accelerated senescence in litchi fruit after cold storage. Sci. Rep. 6, 1–16. doi: 10.1038/srep19356
Zargar Shooshtari, F., Souri, M. K., Hasandokht, M. R., and Jari, S. K. (2020). Glycine mitigates fertilizer requirements of agricultural crops: case study with cucumber as a high fertilizer demanding crop. Chem. Biol. Technol. Agric. 7:19. doi: 10.1186/s40538-020-00185-5
Keywords: metabolomics, transcriptomics, chitosan, banana, ripening
Citation: Parijadi AAR, Yamamoto K, Ikram MMM, Dwivany FM, Wikantika K, Putri SP and Fukusaki E (2022) Metabolome Analysis of Banana (Musa acuminata) Treated With Chitosan Coating and Low Temperature Reveals Different Mechanisms Modulating Delayed Ripening. Front. Sustain. Food Syst. 6:835978. doi: 10.3389/fsufs.2022.835978
Received: 15 December 2021; Accepted: 24 January 2022;
Published: 03 March 2022.
Edited by:
Danar Praseptiangga, Sebelas Maret University, IndonesiaReviewed by:
Sajid Ali, Bahauddin Zakariya University, PakistanDebabandya Mohapatra, Central Institute of Agricultural Engineering (ICAR), India
Copyright © 2022 Parijadi, Yamamoto, Ikram, Dwivany, Wikantika, Putri and Fukusaki. This is an open-access article distributed under the terms of the Creative Commons Attribution License (CC BY). The use, distribution or reproduction in other forums is permitted, provided the original author(s) and the copyright owner(s) are credited and that the original publication in this journal is cited, in accordance with accepted academic practice. No use, distribution or reproduction is permitted which does not comply with these terms.
*Correspondence: Sastia Prama Putri, sastia_putri@bio.eng.osaka-u.ac.jp
†These authors have contributed equally to this work