- Food Security and Safety Focus Area, Faculty of Natural and Agricultural Sciences, North-West University, Mmabatho, South Africa
Sustainable intensification is a means that proffer a solution to the increasing demand for food without degrading agricultural land. Maize is one of the most important crops in the industrial revolution era, there is a need for its sustainable intensification. This review discusses the role of maize in the industrial revolution, progress toward sustainable production, and the potential of nitrifying bacteria and archaea to achieve sustainable intensification. The era of the industrial revolution (IR) uses biotechnology which has proven to be the most environmentally friendly choice to improve crop yield and nutrients. Scientific research and the global economy have benefited from maize and maize products which are vast. Research on plant growth-promoting microorganisms is on the increase. One of the ways they carry out their function is by assisting in the cycling of geochemical, thus making nutrients available for plant growth. Nitrifying bacteria and archaea are the engineers of the nitrification process that produce nitrogen in forms accessible to plants. They have been identified in the rhizosphere of many crops, including maize, and have been used as biofertilizers. This study's findings could help in the development of microbial inoculum, which could be used to replace synthetic fertilizer and achieve sustainable intensification of maize production during the industrial revolution.
Introduction
An agroecosystem where yields are increased without an adverse effect on the environment and a need for additional non-agricultural land is referred to as sustainable intensification (SI) (Pretty and Bharucha, 2014). The focus on agricultural intensification to increase yield for the growing population has escalated environmental degradation (Armstrong Mckay et al., 2019). Furthermore, many agriculturists have yet to adopt environmental sustainability because the problem of low yields has not been addressed (Figure 1). Sustainable intensification can concurrently address environmental security and food security. This is because as agricultural production would be increased, environmental degradation would be reduced simultaneously without acquiring more land for farm use (Hunt et al., 2019). The components of SI (Figure 1) protect the process of an ecosystem and biological diversity while achieving an increase in food production. However, to achieve this aim, the development of suitable techniques for estimating both the sustainability and intensification of agriculture is needed (Hunt et al., 2019). Therefore, studying the interaction of microorganisms and the ecosystem would help maximize their services to ensure a better ecosystem.
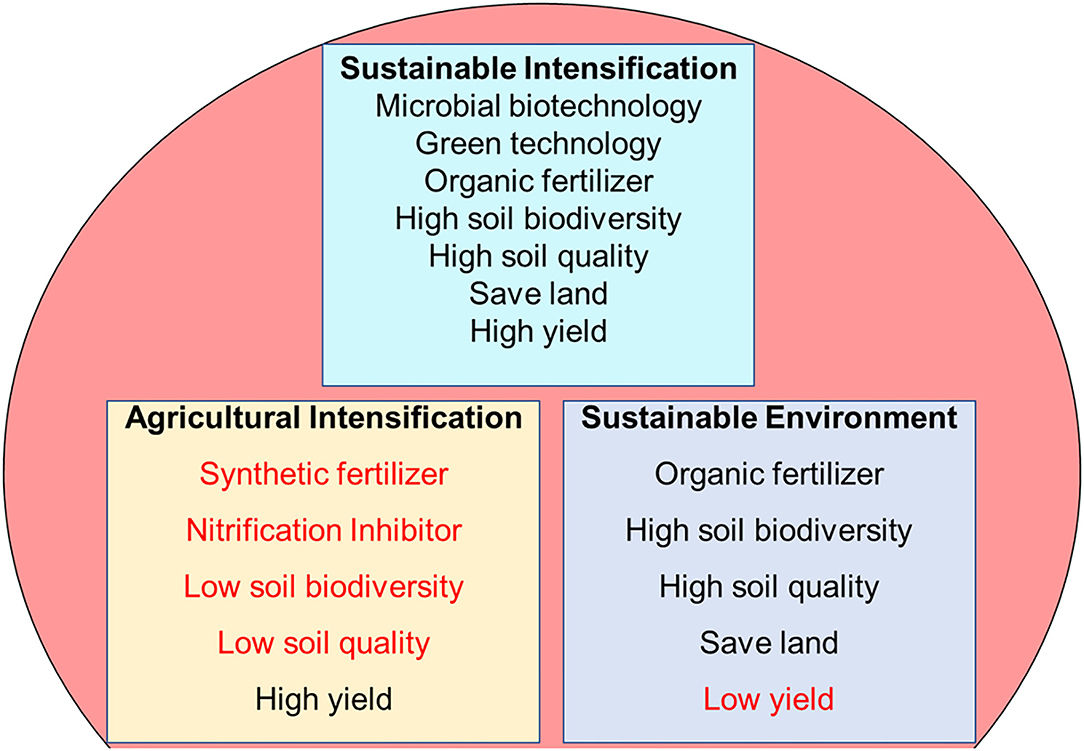
Figure 1. Components of sustainable intensification, agricultural intensification, and sustainable environment.
Industrial revolution (IR) connotes industrialization that began way back in the seventeenth century (More and More, 2002). The industrial revolution brought about the expansion of farm crop yield and goods produced from them. Over the years IR has improved drastically as a result of mechanical production, electricity, electronics, telecommunication, computers, cyber-physical systems, genetic engineering, green revolution, and the internet (Prisecaru, 2016; Vu and Le, 2019). This has affected the agricultural sector also because the innovation of technology is crucial to the renovation and cultivation of food. Food security is part of the challenges the industrial revolution intends to resolve (Prisecaru, 2016). Expectations have been raised regarding using new technologies to conserve resources and improve food nutrients. People are malnourished because nutrient requirements are not being met. Therefore, there is a need for further global green revolution if the world needs to be fed. The industrial revolution could contribute to the security of food by improving crops by artificially adjusting important microbes associated with crops.
Maize is an important staple crop in the industrial revolution and is still in high demand worldwide, considering its importance as food, additives in industrial products, scientific research, and economy. The necessity to intensify its production sustainably is of paramount importance. Modifications in the nitrogen cycle have acutely disturbed the structuring and functioning of the natural ecosystem. The suitable range of nitrogen levels has been altered within the ecospheres and has posed a challenge to the issue of nitrogen maintenance (Xu et al., 2016). The increasing nitrogen level is partly caused by the input of nitrogen-based synthetic fertilizers. Consequently, to avert the challenge with the use of synthetic fertilizers, the inoculation of plant growth-promoting microbes wholly or together with manures would be critical in improving maize productivity for industrial revolution. Nitrifying bacteria with traits that promote plant growth have the potential of achieving sustainable intensification. This review discusses the role of maize in the industrial revolution, progress toward sustainable production, and the potential of nitrifying bacteria and archaea to achieve sustainable intensification.
Significance of Maize in the Industrial Revolution
Maize accounts for a significant amount of daily food in most developing regions. It is referred to as yellow gold because of its usefulness as food, animal feeds, and manufacturing processed food and non-food materials. Several studies have been carried out on maize because of its economic importance. Maize is one of the few crops that have attracted the attention of researchers in the area of genetic enhancement (Badu-Apraku and Fakorede, 2017). Aside from its importance, researchers choose to work with maize because it is suitable to cultivate and easy to collect data from Chen et al. (2015). Considering the foresight of industrial revolution, it is necessary to elaborate its role and point out how it can be cultivated in an environmentally friendly way.
Maize Products
The IR has caused an increase in agricultural products, both raw materials and industrialized. The processing of food rapidly has created sufficient time for human liberation, labor market participation and children care (Reardon et al., 2019). This has resulted in a reduced death rate and increased birth rate, causing a sharp increase in population, and placing high demand on resources. According to Dowswell et al. (2019), 20 million tons of maize is used for starch, 10 million tons are used for ethanol fuel production, 3 million for cereal and baked products, 0.7 million for cereal and hybrid seed sales. As a result of its reduced price as compared to other crops, maize has been used as feed formulae in animal rearing.
Maize is of high nutritional value and has been considered raw material for many industrial productions (Adiaha et al., 2016). This includes biomethane production, bioplastic, paper making, packaging and many additives. The agricultural sector substantially contributes to job creation and international marketing (Rekha and Singh, 2018). The effect of any technology in agriculture should be weighed against product output, profits, health, and environmental effect (Reardon et al., 2019). Over processed food has led to obesity, diabetes, and several health problems, hence the need to ensure the fortification of foods with sufficient nutrients (Reardon et al., 2019).
Economic Importance of Maize
Since the transcend of IR, global economic growth has been increasing. The production of maize ranks first in Latin America and Africa, while in Asia it is ranked third after rice and wheat (Dowswell et al., 2019). The demand and supply for maize globally for food and non-food products are usually on the increase. Yearly, 15 million metric tons (MMT) are used for animal feed, 4.25 MMT for industrial use, 1.36 MMT is used as food (Yadav et al., 2016). Considering its value for domestic, industrial and economic use (Adiaha et al., 2016), investing in the increase in maize production is an opportunity for any country. Maize is grown in 170 countries using 184 M ha of land with a production of about 1016 MMT (Food Agricultural Organization of the United Nations, 2017)). Various countries have benefited from the exportation and importation of maize.
In India maize has an annual production of 24.26 million metric tons (MMT) (Yadav et al., 2016). There was a rapid increase in the production of maize from 1950 to 1980, while 1983 marked a sharp decrease in maize production (Dowswell et al., 2019). It generates income for the government as it is used by countries as a commercialized product (Adiaha et al., 2016). Companies and individual entrepreneurs are collaborating with large-scale farmers to produce high-quality maize seeds. This helps mitigate their high demand and insufficient supply (Jonga et al., 2018). Seed quality determines crop yield and productivity. Jonga et al. (2018) advised that a quality management system should be put in place by the companies and entrepreneurs to ensure better products continuously.
Scientific Research on Maize
The role of maize in scientific research for the industrial revolution cannot be overemphasized (Table 1). Some upcoming scientists wonder why there is intensive research on maize when compared to other cereals. Aside from its importance as food and uses in industrial products, maize is easy to cultivate and manage, thus the results are observed easily and juxtaposed occasionally to other plants. Notable of its use in genetic studies, Jiao et al. (2017) referred to it as a model species for agricultural and genetic research. Maize plant has been used to check the quality of soil (Adiaha et al., 2016). The cob is useful in the treatment of waste. Okoya et al. (2015) reported the efficiency of maize cob in the removal of lead and chromium from waste.
Food Security
The quantity and quality of food have been threatened by unfavorable environmental conditions. To meet the needs of the high population, the quantity of food must be increased without jeopardizing the quality. In search of a solution, maize has been a choice crop by researchers (Adiaha et al., 2016; Otsuka and Muraoka, 2017). According to Abate et al. (2015), after considering factors that can be used to combat food security, maize was chosen as the best cereal to be cultivated in Ethiopia. He further explained that in terms of calorie intake, maize is the most important staple food. Otsuka and Muraoka (2017) acknowledged maize to be the most important cereal, considering its production and consumption. The development of the agricultural sector is necessary to reduce poverty and secure food. The need to secure food should be reinforced with green revolution that would drastically increase the yield of crops in a sustainable way. Therefore, maize which is easily cultivated and possess lots of nutrient has the potential to combat food insecurity globally. Maize cultivation has dropped the rate of poverty and improved the lives of local farmers, especially in developing countries Adiaha et al. (2016).
Industrial Revolution of Maize
The agricultural sector, in general, has benefitted from industrial revolution using green and microbial biotechnology. Presently, green revolution has been anchored on genetically modified food and agrochemicals alongside several other inventions and technology (Llewellyn, 2018). Otsuka and Muraoka (2017) stated that the green revolution has helped resolve food crisis however, some countries are yet to meet the global standard of maize yield and attributed this to low soil quality. Also, food insecurity is rising, crop yields are lower than expected when compared to farmers' input, many crop plants are susceptible to disease and the environment is being depleted. An improvement in the present green revolution is necessary, this could be achieved by scientific and biotechnological research toward agricultural production.
The focus is now on sustainably feeding the growing population. Increasing land productivity is a crucial requirement in meeting the growing demand for food in every region. Implementing technology in agriculture can cause a global transformation. Brill (1981) suggested the possibility of getting a hybrid plant with foreign genetic material that would make it possible for the plant to efficiently use atmospheric nitrogen. The possibility of using recombinant DNA techniques in microbial breeding for agriculture is still at a primitive stage, while engineering of beneficial soil microorganisms associated with the specific crop is ongoing. The inoculation of bacteria into soil has been seen to have a positive effect on plant growth (Ndeddy Aka and Babalola, 2016). The beneficial microorganisms can be cultured, grown in fermentation tanks and isolated for use. This can be taken practically to revolutionize industrial maize production.
Achieving Sustainable Intensification
In-depth knowledge of the dynamism of nitrogen would require research on the distribution, function, structure, and contribution of Bacteria and Archaea associated with its cycling process (He et al., 2012). Inoculation of microorganisms is a biotechnological environmentally safe alternative to increase crop production (Alori et al., 2017; Olanrewaju et al., 2017). The microorganism with the highest benefit could be useful for biotechnology breeding (Walters et al., 2018). Integration of microbes with organic material can also be considered Enebe and Babalola (2018). This would reduce the need for synthetic fertilizer and achieve SI. A new system incorporating different components that can boost maize production can be put in place (Figure 2).
Plant Growth Promoting Microorganisms
Unavailability of nutrients, pest infestation, and drought are some of the challenges to plant growth. Some microorganisms referred to as plant growth promoters have been observed to have traits that could help combat these challenges. One of the ways to address these challenges is to assist in the cycling of geochemical making nutrients available for plant growth (Etesami and Adl, 2020). Inoculation of microorganisms is a biotechnological alternative to increase crop productivity, increase the availability of nutrients, reduce the use of synthetic fertilizer, and achieve SI (Table 2). Bacillus subtilis was reported by Zheng et al. (2018) to be able to influence the physical, chemical and hydrological characteristics of the rhizosphere, thus improving drought tolerance of plants in the long run. They ascribed this attribute of Bacillus subtilis to their production of extracellular polymeric substances. Using genomic information, Wang et al. (2018) ascertained the usefulness of Streptomyces albireticul and Streptomyces alboflavus as a biocontrol agent.
Nitrifying Bacteria and Archaea
Surprisingly, nitrifying bacteria and archaea (Table 3) have not been focused on as plant growth-promoting bacteria. Considering their importance in nitrate production and oxidizing ammonia in soils and substrates, this calls for attention in scientific research. Aside from their major function of nitrification, they could have other plant growth-promoting traits. They can be classified into three distinct groups depending on the key enzymes possessed. The first group is the ammonia-oxidizing bacteria and archaea, second is the nitrite-oxidizing bacteria (Table 3) and the third is comammox bacteria (oxidation of ammonia to nitrate) (Stein and Klotz, 2016). Key enzymes used by these organisms are ammonia monooxygenase (AmoA), hydroxylamine oxidoreductase (HAO), and nitrite oxidoreductase (NXR) (Kuypers et al., 2018).
Based on nutrition, the nitrifying bacteria and archaea could be divided into heterotrophs and autotrophs (Liu et al., 2015). The heterotrophs depend on other organisms or dead organic matter for food while the autotrophs can synthesize their food. The autotrophs could further be divided into photoautotrophs (possess bacteriochlorophyll and use solar energy to produce food) and chemoautotrophs (using the oxidation of certain chemicals to produce food). Cellular respiration of nitrifying bacteria and archaea could either be aerobic (with oxygen) or anaerobic (without oxygen) (Muck et al., 2019). The group of organisms involved in anaerobic ammonium nitrification is known as anammox, they carry out nitrification in oxygen-depleted zones (Rich et al., 2018).
Nitrifying microbes include chemolithotrophic members, members of Betaproteobacteria, Gammaproteobacteria, and members of the Thaumarchaeota (Stein, 2019). The reactions occur under varying soil characteristics with some abiotic components contributing to it (Heil et al., 2016). Also, there are heterotrophic and methanotrophic bacteria that oxidize ammonium to nitrite efficiently (Stein and Klotz, 2016). High temperature changes soil nitrifying communities as a result of an increase in the rate of chemical production (Nguyen et al., 2019). pH between 7 and 9 is best for the activity of ammonia oxidizing bacteria and nitrite-oxidizing bacteria, as higher than that disrupts their activity (Heil et al., 2016). Environmental factors determine the group of nitrifying microorganisms that would be prevalent in a habitat or substrate.
Nitrifying bacteria are widely used in aquaculture management (Ruiz et al., 2020; Ajijah et al., 2021) and waste management (Sepehri et al., 2020; Zhao et al., 2020). It is rarely used in cropping. Nitrobacter, on the other hand, has been used as a biofertilizer both alone (Doost et al., 2019) and in groups of micro-consortiums (Vatandoost et al., 2019). Doost et al. (2019) discovered that the protein content of Canola improved when compared to the control. Beyond aquaculture and waste management, there is still a need to expand the use of nitrifying bacteria in cropping systems. Nitrifire 5x, MicrobeLift Nite-out II, Scape bac up, Nitrobacter multi-probiotic, Nbc1, and Nbc2 are some of the commercially available application-based nitrifying bacteria. Although these products were intended for use in aquaculture, their novel application in crop management can be investigated.
Excess ammonia in the soil as a result of synthetic ammonium-based fertilizer affects the environment negatively (Lehtovirta-Morley, 2018). The presence of nitrifying bacteria in the soil reduces ammonia. This makes the soil less acidic and, as such, other beneficial microorganisms can proliferate, thus promoting soil quality. Also, nitrate, which is eventually produced from the nitrification process, elongates lateral roots (Mantelin and Touraine, 2004), mediates signaling pathways of phytohormones, expands leaves, and induces flowers in plants (Hachiya and Sakakibara, 2016). Furthermore, plants' yields and growth are increased and there is little or no dependence on synthetic fertilizer and other agrochemicals that degrade the soil.
Electron Transport Chain
The enzymatic process of nitrification can be divided into three pathways: NH3 oxidation pathway, NH2OH oxidation pathway, and NO2 oxidation pathway. The enzymatic process is carried out by an electron transport chain and the reaction is exergonic (a biochemical reaction that releases energy) (Wendeborn, 2019). Ammonia monooxygenase (AMO) turns ammonia into NH2OH with the gain of two electrons (Daims et al., 2015). The electron is obtained from subsequent oxidation of hydroxylamine, and the energy liberated is obtained from the linked reaction of oxygen reduced to water (Wendeborn, 2019). AMO exists in an integral membrane protein and is a member of the copper membrane monooxygenase (CuMMO) family. The mechanism by which CuMMO carries out its oxidation could help in the development of monitored synthetic oxidation (Lancaster et al., 2018).
Four electrons are used by hydroxylamine oxidoreductase (HAO), a multiheme enzyme used to oxidize NH2OH to NO2-. Two of the electrons used in oxidizing hydroxylamine return to AMO while the remaining two enter the respiratory electron transport chain, terminating the electron acceptor using O2 (Daims et al., 2015). This reaction is also exergonic and the energy produced is higher if coupled with a reduction of water (Wendeborn, 2019). According to Lancaster et al. (2018), oxygen is not required for HAO activity, and NO is the product of NH2OH oxidation and not NO2. He further explained that NO is a reactive molecule, as its transformation to other forms of nitrous oxide could be a non-enzymatic reaction. This might be true, however (Wendeborn, 2019), reported some organisms that can oxidize NO2 to NO3.
Nitrite oxidoreductase possessed by some NOB oxidizes nitrite to nitrate with the use of electrons donated from oxygen. However, it can also be produced when nitrite donates electrons to reduce CO2 to glucose by some photosynthetic bacteria (Wendeborn, 2019).
Comammox (complete ammonia oxidizer) was predicted by Costa et al. (2006) and discovered in Nitrospira by Daims et al. (2015) and Van Kessel et al. (2015). They can utilize eight electrons to oxidize NH3 to NO (Lancaster et al., 2018). Broda (1977) predicted two chemolithotrophic organisms that can carry out anammox (Anaerobic ammonia oxidation). One of the bacteria responsible for anammox was identified as Planctomycetales in 1999 (Strous et al., 1999). Anammox microorganisms in an environment where oxygen is depleted can make use of nitrite instead of oxygen as the electron acceptor producing dinitrogen (Wendeborn, 2019). Considering the complex metabolic pathway in the nitrification process, there might be more discoveries to be made to manage the process efficiently.
Availability of Ammonia in the Soil and Organic Waste
Ammonia-based substance is the substrate used by AOA and AOB. They can obtain it from ammonia-based organic waste or soil organic matter. Organic waste improves the quality of soil because it positively affects the growth of soil microorganisms. The natural process of nitrification does not provide sufficient nitrate. Therefore, to strike a balance between the modern process and the natural process, it would be good to provide a technology that would mimic the natural process. Organic fertilizers have been made from composting of organic waste and vermicomposting (Caceres et al., 2018). Plant growth-promoting microorganisms can be used along with these organic materials (Domenico, 2020). One of the biological approaches suggested for SI is to increase biological diversity in the agricultural systems (Petersen and Snapp, 2015). Nitrate has been successfully produced from ammonium contained in vegetable waste using Nitrosomonas sp. and Nitrobacter sp. by Naghdi et al. (2018). Synthetic fertilizer is the cause of excessive amounts of nitrate because it speeds up the rate of nitrification. The gradual and systematic production of nitrate is considered safe for the ecosystem and a better alternative to synthetic fertilizer.
Identification and Isolation of Nitrifying Bacteria and Archaea
Microorganisms are ubiquitous, however, their composition varies in different habitats as a result of varying environmental factors. In time past isolation and identifying of microorganisms are usually carried out after culturing. Recently, metagenomics survey has enabled the easy identification of microorganisms. Known and unknown nitrifying microorganism strain has been identified from different habitats via metagenomics analysis (Clark et al., 2021). The establishment of the presence of nitrifying bacteria and archaea provides a guide on what type is to be isolated and cultured. Although nitrifying bacteria and archaea have been difficult to culture, however, some researchers have been successful in that regard (Könneke et al., 2005; Mellbye et al., 2017). Könneke et al. (2005), isolated nitrifying archaea using serial dilution and incubated them with a medium enriched with ammonia at 21–23°C. The use of mineral salt media with varying formulations has been used by Mellbye et al. (2017). Furthermore, Fujitani et al. (2015), explained the possibility of isolating them from nitrifying granules in a wastewater plant and cultivating them in a liquid culture rich in ammonia. Molecular characterization of the nitrifying microorganisms can also be carried out using 16S rRNA gene sequencing after serial dilution, DNA extraction and PCR amplification (Hastuti et al., 2019). Cultivating nitrifier community unique to maize plant can be carried out and used to increase their population in maize rhizosphere. This would increase the bioavailability of nitrogen in the soil, thereby replacing nitrogen-based fertilizers.
Conclusion and Perspective
Sustainable intensification proffers the solution to the conflicts of meeting the increasing demand for food and ensuring a sustainable environment. Industrial revolution merges trends in intelligent automation with artificial intelligence, this results in remarkable improvement in technology, growth in economy and unimaginable progress. Maize accounts for a significant amount of daily food in most developing regions and it is important to scientific and industrial use. Considering the need to increase maize production, microorganisms with growth-promoting properties can help achieve proper management, sustainable agriculture and sustainable environments. Agriculture has used large amounts of land globally, with major implications for reactive nitrogen from synthetic fertilizers and the use of nitrifying inhibitors to inefficiently manage the system. Nitrifying bacteria and archaea can transform ammonia locked up in soil organic matter and organic waste matter. They can be inoculated wholly or together with ammonium-based organic waste into the rhizosphere of maize. Although the biotechnological formulation and use are still in their primitive stage. Identifying and isolating nitrifying microorganism communities and structures associated with maize is a step toward achieving SI.
Author Contributions
OEA reviewed and wrote the first draft of the paper, while OOB critiqued, proofread, and contributed substantially to the structure of the manuscript. Both authors contributed to the article and approved the submitted version.
Funding
The study was funded by the National Research Foundation of South Africa through OOB from the grants (UID 123634 and UID 132595).
Conflict of Interest
The authors declare that the research was conducted in the absence of any commercial or financial relationships that could be construed as a potential conflict of interest.
Publisher's Note
All claims expressed in this article are solely those of the authors and do not necessarily represent those of their affiliated organizations, or those of the publisher, the editors and the reviewers. Any product that may be evaluated in this article, or claim that may be made by its manufacturer, is not guaranteed or endorsed by the publisher.
Acknowledgments
OOB appreciates the National Research Foundation of South Africa for the grant (UID123634, UID132595) that has supported research in her lab. OEA would like to thank the North-West University for its doctoral bursary and research support.
References
Abate, T., Shiferaw, B., Menkir, A., Wegary, D., Kebede, Y., Tesfaye, K., et al. (2015). Factors that transformed maize productivity in Ethiopia. Food Security 7, 965–981. doi: 10.1007/s12571-015-0488-z
Adiaha, M., Agba, O., Attoe, E., Ojikpong, T., Kekong, M., Obio, A., et al. (2016). Effect of maize (Zea mays L.) on human development and the future of man-maize survival: a review. World Sci. News 59, 52–62.
Ajijah, N., Apriyana, A. Y., Sriwuryandari, L., Priantoro, E. A., Janetasari, S. A., Pertiwi, T. Y. R., et al. (2021). Beneficiary of nitrifying bacteria for enhancing lettuce (Lactuca sativa) and vetiver grass (Chrysopogon zizanioides L.) growths align with carp (Cyprinus carpio) cultivation in an aquaponic system. Environ. Sci. Pollut. Res. 28, 880–889. doi: 10.1007/s11356-020-10468-x
Alori, E. T., Glick, B. R., and Babalola, O. O. (2017). Microbial phosphorus solubilization and its potential for use in sustainable agriculture. Front. Microbiol. 8, 971. doi: 10.3389/fmicb.2017.00971
Armstrong Mckay, D. I., Dearing, J. A., Dyke, J. G., Poppy, G. M., and Firbank, L. G. (2019). To what extent has sustainable intensification in England been achieved? Sci. Total Environ. 648, 1560–1569. doi: 10.1016/j.scitotenv.2018.08.207
Badu-Apraku, B., and Fakorede, M. (2017). Maize in Sub-Saharan Africa: importance and production constraints, in Advances in Genetic Enhancement of Early and Extra-Early Maize for Sub-Saharan Africa, eds Badu-Apraku, B., and Fakorede, M., (Cham: Springer), 3–10.
Bezrutczyk, M., Zöllner, N. R., Kruse, C. P., Hartwig, T., Lautwein, T., Köhrer, K., et al. (2021). Evidence for phloem loading via the abaxial bundle sheath cells in maize leaves. Plant Cell 33, 531–547. doi: 10.1093/plcell/koaa055
Brenner, D. J., Krieg, N. R., Staley, J. T., and Garrity, G. M. (2005). Bergey's manual® of Systematic Bacteriology: Volume Two: The Proteobacteria, Part A Introductory Essays. Springer. Available online at: https://link.springer.com/book/10.1007/0-387-29298-5
Brill, W. J. (1981). Agricultural microbiology. Sci. Am. 245, 198–215. doi: 10.1038/scientificamerican0981-198
Broda, E. (1977). Two kinds of lithotrophs missing in nature. Zeitschrift Allgemeine Mikrobiologie 17, 491–493. doi: 10.1002/jobm.19770170611
Caceres, R., Malińska, K., and Marfa, O. (2018). Nitrification within composting: a review. Waste Manage. 72, 119–137. doi: 10.1016/j.wasman.2017.10.049
Calugar, R. E., Has, V. V., Varga, A., Vana, C. D., Copandean, A., and Has, I. (2018). The role of cytoplasmatic diversification on some productivity traits of maize. Euphytica 214, 90–100. doi: 10.1007/s10681-018-2171-x
Cardozo, P., Di Palma, A., Martin, S., Cerliani, C., Esposito, G., Reinoso, H., et al. (2021). Improvement of maize yield by foliar application of Azospirillum brasilense Az39. J. Plant Growth Regul. 1–9. doi: 10.1007/s00344-021-10356-9
Chen, D., Lan, Z., Hu, S., and Bai, Y. (2015). Effects of nitrogen enrichment on belowground communities in grassland: Relative role of soil nitrogen availability vs. soil acidification. Soil Biol. Biochem. 89, 99–108. doi: 10.1016/j.soilbio.2015.06.028
Chukwuneme, C. F., Babalola, O. O., Kutu, F. R., and Ojuederie, O. B. (2020). Characterization of actinomycetes isolates for plant growth promoting traits and their effects on drought tolerance in maize. J. Plant Interact. 15, 93–105. doi: 10.1080/17429145.2020.1752833
Clark, I. M., Hughes, D. J., Fu, Q., Abadie, M., and Hirsch, P. R. (2021). Metagenomic approaches reveal differences in genetic diversity and relative abundance of nitrifying bacteria and archaea in contrasting soils. Sci. Rep. 11, 1–9. doi: 10.1038/s41598-021-95100-9
Contreras-Cornejo, H. A., Viveros-Bremauntz, F., Del-Val, E., Macías-Rodríguez, L., López-Carmona, D. A., Alarcón, A., et al. (2021). Alterations of foliar arthropod communities in a maize agroecosystem induced by the root-associated fungus Trichoderma harzianum. J. Pest Sci. 94, 363–374. doi: 10.1007/s10340-020-01261-3
Costa, E., Pérez, J., and Kreft, J.-U. (2006). Why is metabolic labour divided in nitrification? Trends Microbiol. 14, 213–219. doi: 10.1016/j.tim.2006.03.006
Daims, H., Lebedeva, E. V., Pjevac, P., Han, P., Herbold, C., Albertsen, M., et al. (2015). Complete nitrification by nitrospira bacteria. Nature 528, 504–509. doi: 10.1038/nature16461
De Carvalho Nascimento, R., Cavalcanti, M. I. P., De Jesus Correia, A., Escobar, I. E. C., De Freitas, A. D. S., Nóbrega, R. S. A., et al. (2021). Maize-associated bacteria from the Brazilian semiarid region boost plant growth and grain yield. Symbiosis 83, 347–359. doi: 10.1007/s13199-021-00755-7
Deba, F. A., Isma'il, S., Sahal, M. R., and Okpanachi, I. Y. (2019). Evaluation of economic importance of locally produced manure over inorganic fertilizer for maize production: vegetative performance and cost implication. Am. J. Mol. Biol. 9, 64–74. doi: 10.4236/ajmb.2019.92006
Domenico, P. (2020). Optimised fertilisation with zeolitites containing Plant Growth Promoting Rhizobacteria (PGPR) in Ranunculus asiaticus. Biol. Pharm. Sci. 10, 096–102. doi: 10.30574/gscbps.2020.10.1.0011
Doost, H. V., Sharifi, R. S., Farzaneh, S., and Panah, D. H. (2019). Effects of bio-and chemical-organic fertilizers on yield, some physiological traits and fatty acids composition of canola. Bangladesh J. Bot. 48, 113–122. doi: 10.3329/bjb.v48i1.47422
Dowswell, C. R., Paliwal, R. L., and Ronald, P. C. (2019). Maize in the Third World. Boca Raton, FL: CRC Press.
Enebe, M. C., and Babalola, O. O. (2018). The influence of plant growth-promoting rhizobacteria in plant tolerance to abiotic stress: a survival strategy. Appl. Microbiol. Biotechnol. 102, 7821–7835. doi: 10.1007/s00253-018-9214-z
Etesami, H., and Adl, S. M. (2020). Plant Growth-Promoting Rhizobacteria (PGPR) and their action mechanisms in availability of nutrients to plants, in Phyto-Microbiome in Stress Regulation, eds Kumar, M., Kumar, V., and Prasad, R., (Singapore: Springer), 147–203.
Fasusi, O. A., Amoo, A. E., and Babalola, O. O. (2021). Propagation and characterization of viable arbuscular mycorrhizal fungal spores within maize plant (Zea mays L.). J. Sci. Food Agric. 101, 5834–5841. doi: 10.1002/jsfa.11235
Food Agricultural Organization of the United Nations (2017). Production Quantity of Maize, Green by Country. Available online at: http://www.fao.org/faostat/en/#data/QC/visualize (accessed Febuary 7, 2022).
Fujitani, H., Kumagai, A., Ushiki, N., Momiuchi, K., and Tsuneda, S. (2015). Selective isolation of ammonia-oxidizing bacteria from autotrophic nitrifying granules by applying cell-sorting and sub-culturing of microcolonies. Front. Microbiol. 6, 1159. doi: 10.3389/fmicb.2015.01159
Gavilanes, F. Z., Andrade, D. S., Zucareli, C., Horácio, E. H., Yunes, J. S., Barbosa, A. P., et al. (2020). Co-inoculation of Anabaena cylindrica with Azospirillum brasilense increases grain yield of maize hybrids. Rhizosphere 15, 100224. doi: 10.1016/j.rhisph.2020.100224
Gerardi, M. H. (2003). Nitrification and Denitrification in the Activated Sludge Process. Newyork: John Wiley & Sons.
Ghazy, N., and El-Nahrawy, S. (2021). Siderophore production by Bacillus subtilis MF497446 and Pseudomonas koreensis MG209738 and their efficacy in controlling Cephalosporium maydis in maize plant. Arch. Microbiol. 203, 1195–1209. doi: 10.1007/s00203-020-02113-5
Gil-Ortiz, R., Naranjo, M. Á., Ruiz-Navarro, A., Caballero-Molada, M., Atares, S., García, C., et al. (2021). Agronomic assessment of a controlled-release polymer-coated urea-based fertilizer in maize. Plants 10, 594. doi: 10.3390/plants10030594
Hachiya, T., and Sakakibara, H. (2016). Interactions between nitrate and ammonium in their uptake, allocation, assimilation, and signaling in plants. J. Exp. Bot. 68, 2501–2512. doi: 10.1093/jxb/erw449
Hao, L., Zhang, Z., Hao, B., Diao, F., Zhang, J., Bao, Z., et al. (2021). Arbuscular mycorrhizal fungi alter microbiome structure of rhizosphere soil to enhance maize tolerance to La. Ecotoxicol. Environ. Saf. 212, 111996. doi: 10.1016/j.ecoenv.2021.111996
Hastuti, Y. P., Rusmana, I., Nirmala, K., Affandi, R., and Tridesianti, S. (2019). Identification and characterization of nitrifying bacteria in mud crab (Scylla serrata) recirculation aquaculture system by 16S rRNA sequencing. Biodiversitas J. Biol. Divers. 20, 1339–1343. doi: 10.13057/biodiv/d200524
He, J.-Z., Shen, J.-P., Zhang, L.-M., and Di, H. J. (2012). A review of ammonia-oxidizing bacteria and archaea in Chinese soils. Front. Microbiol. 3, 296–303. doi: 10.3389/fmicb.2012.00296
Heil, J., Vereecken, H., and Brüggemann, N. (2016). A review of chemical reactions of nitrification intermediates and their role in nitrogen cycling and nitrogen trace gas formation in soil. Eur. J. Soil Sci. 67, 23–39. doi: 10.1111/ejss.12306
Hou, P., Liu, Y., Liu, W., Liu, G., Xie, R., Wang, K., et al. (2020). How to increase maize production without extra nitrogen input. Resourc. Conserv. Recycl. 160, 104913. doi: 10.1016/j.resconrec.2020.104913
Hunt, M. L., Blackburn, G. A., and Rowland, C. S. (2019). Monitoring the sustainable intensification of arable agriculture: the potential role of earth observation. Int. J. Applied Earth Observ. Geoinformation 81, 125–136. doi: 10.1016/j.jag.2019.05.013
Jiao, Y., Peluso, P., Shi, J., Liang, T., Stitzer, M. C., Wang, B., et al. (2017). Improved maize reference genome with single-molecule technologies. Nature 546, 524–527. doi: 10.1038/nature22971
Jonga, M., Waiganjo, E., and Njeru, A. (2018). Influence of product quality on organizational performance of seed maize companies in Kenya. J. Agric. Sci. 10, 109–116. doi: 10.5539/jas.v10n5p109
Kennett, D. J., Prufer, K. M., Culleton, B. J., George, R. J., Robinson, M., Trask, W. R., et al. (2020). Early isotopic evidence for maize as a staple grain in the Americas. Sci. Adv. 6, 3245. doi: 10.1126/sciadv.aba3245
Kong, J., Jin, J., Dong, Q., Qiu, J., Li, Y., Yang, Y., et al. (2019). Maize factors ZmUBP15, ZmUBP16 and ZmUBP19 play important roles for plants to tolerance the cadmium stress and salt stress. Plant Sci. 280, 77–89. doi: 10.1016/j.plantsci.2018.11.014
Könneke, M., Bernhard, A. E., José, R., Walker, C. B., Waterbury, J. B., and Stahl, D. A. (2005). Isolation of an autotrophic ammonia-oxidizing marine archaeon. Nature 437, 543–546. doi: 10.1038/nature03911
Koops, H., and Stehr, G. (1991). Classification of eight new species of ammonia-oxidizing bacteria: Nitrosomonas communis sp. nov., Nitrosomonas ureae sp. nov., Nitrosomonas aestuarii sp. nov., Nitrosomonas marina sp. nov., Nitrosomonas nitrosa sp. nov., Nitrosomonas eutropha sp. nov., Nitrosomonas oligotropha sp. nov. and Nitrosomonas halophila sp. nov. Microbiology 137, 1689–1699. doi: 10.1099/00221287-137-7-1689
Kuypers, M. M., Marchant, H. K., and Kartal, B. (2018). The microbial nitrogen-cycling network. Nat. Rev. Microbiol. 16, 263–276. doi: 10.1038/nrmicro.2018.9
Lancaster, K. M., Caranto, J. D., Majer, S. H., and Smith, M. A. (2018). Alternative bioenergy: updates to and challenges in nitrification metalloenzymology. Joule 2, 421–441. doi: 10.1016/j.joule.2018.01.018
Lehtovirta-Morley, L. E. (2018). Ammonia oxidation: ecology, physiology, biochemistry and why they must all come together. Feder. Euro. Microbiol. Soc. Microbiol. Lett. 365, 1–9. doi: 10.1093/femsle/fny058
Li, H., Peng, T., Wang, Q., Wu, Y., Chang, J., Zhang, M., et al. (2017). Development of incompletely fused carpels in maize ovary revealed by miRNA, target gene and phytohormone analysis. Front. Plant Sci. 8, 463. doi: 10.3389/fpls.2017.00463
Liu, R., Suter, H., He, J., Hayden, H., and Chen, D. (2015). Influence of temperature and moisture on the relative contributions of heterotrophic and autotrophic nitrification to gross nitrification in an acid cropping soil. J. Soils Sediments 15, 2304–2309. doi: 10.1007/s11368-015-1170-y
Llewellyn, D. (2018). Does global agriculture need another green revolution? Engineering 4, 449–451. doi: 10.1016/j.eng.2018.07.017
Mantelin, S., and Touraine, B. (2004). Plant growth-promoting bacteria and nitrate availability: impacts on root development and nitrate uptake. J. Exp. Bot. 55, 27–34. doi: 10.1093/jxb/erh010
Mcloughlin, F., Augustine, R. C., Marshall, R. S., Li, F., Kirkpatrick, L. D., Otegui, M. S., et al. (2018). Maize multi-omics reveal roles for autophagic recycling in proteome remodelling and lipid turnover. Nat. Plants 4, 1056–1070. doi: 10.1038/s41477-018-0299-2
Mellbye, B. L., Spieck, E., Bottomley, P. J., and Sayavedra-Soto, L. A. (2017). Acyl-homoserine lactone production in nitrifying bacteria of the genera Nitrosospira, Nitrobacter, and Nitrospira identified via a survey of putative quorum-sensing genes. Appl. Environ. Microbiol. 83, 01540–01557. doi: 10.1128/AEM.01540-17
Moreno, A. D. L., Kusdra, J. F., and Picazevicz, A. A. (2021). Rhizobacteria inoculation in maize associated with nitrogen and zinc fertilization at sowing. Revista Brasil. Engenharia Agrícola e Ambiental 25, 96–100. doi: 10.1590/1807-1929/agriambi.v25n2p96-100
Mpanga, I. K., Nkebiwe, P. M., Kuhlmann, M., Cozzolino, V., Piccolo, A., Geistlinger, J., et al. (2019). The form of N supply determines plant growth promotion by P-solubilizing microorganisms in maize. Microorganisms 7, 38–56. doi: 10.3390/microorganisms7020038
Muck, S., De Corte, D., Clifford, E. L., Bayer, B., Herndl, G. J., and Sintes, E. (2019). Niche differentiation of aerobic and anaerobic ammonia oxidizers in a high latitude deep oxygen minimum zone. Front. Microbiol. 10, 2141. doi: 10.3389/fmicb.2019.02141
Naeem, U., Ul Haq, I., Afzaal, M., Qazi, A., Yasar, A., Bari Tabinda, A., et al. (2021). Investigating the effect of Aspergillus niger inoculated press mud (biofertilizer) on the potential of enhancing maize (Zea mays. L) yield, potassium use efficiency and potassium agronomic efficiency. Cereal Res. Commun. 1–14. doi: 10.1007/s12517-021-07025-2
Naghdi, M., Cledon, M., Brar, S. K., and Ramirez, A. A. (2018). Nitrification of vegetable waste using nitrifying bacteria. Ecol. Eng. 121, 83–88. doi: 10.1016/j.ecoleng.2017.07.003
Ndeddy Aka, R. J., and Babalola, O. O. (2016). Effect of bacterial inoculation of strains of Pseudomonas aeruginosa, Alcaligenes feacalis and Bacillus subtilis on germination, growth and heavy metal (Cd, Cr, and Ni) uptake of Brassica juncea. Int. J. Phytoremediation 18, 200–209. doi: 10.1080/15226514.2015.1073671
Nguyen, L. T. T., Broughton, K., Osanai, Y., Anderson, I. C., Bange, M. P., Tissue, D. T., et al. (2019). Effects of elevated temperature and elevated CO2 on soil nitrification and ammonia-oxidizing microbial communities in field-grown crop. Sci. Total Environ. 675, 81–89. doi: 10.1016/j.scitotenv.2019.04.181
Okoya, A., Akinyele, A., Amuda, O., and Ofoezie, I. (2015). Chitosan grafted modified maize cob for removal of lead and chromium from wastewater. Ethiopian J. Environ. Stud. Manage. 8, 881–892. doi: 10.4314/ejesm.v8i2.3S
Olanrewaju, O. S., Glick, B. R., and Babalola, O. O. (2017). Mechanisms of action of plant growth promoting bacteria. World J. Microbiol. Biotechnol. Genetic Eng. Rev. 33, 197. doi: 10.1007/s11274-017-2364-9
Otsuka, K., and Muraoka, R. (2017). A green revolution for sub-Saharan Africa: past failures and future prospects. J. Afr. Econ. 26, 73–98. doi: 10.1093/jae/ejx010
Perdomo, J. A., Capó-Bauçà, S., Carmo-Silva, E., and Galmés, J. (2017). Rubisco and rubisco activase play an important role in the biochemical limitations of photosynthesis in rice, wheat, and maize under high temperature and water deficit. Front. Plant Sci. 8, 490–505. doi: 10.3389/fpls.2017.00490
Petersen, B., and Snapp, S. (2015). What is sustainable intensification? Views from experts. Land Use Policy 46, 1–10. doi: 10.1016/j.landusepol.2015.02.002
Pretty, J., and Bharucha, Z. P. (2014). Sustainable intensification in agricultural systems. Ann. Bot. 114, 1571–1596. doi: 10.1093/aob/mcu205
Prisecaru, P. (2016). Challenges of the fourth industrial revolution. Knowledge horizons. Economics 8, 57–63.
Qin, W., Heal, K. R., Ramdasi, R., Kobelt, J. N., Martens-Habbena, W., Bertagnolli, A. D., et al. (2017). Nitrosopumilus maritimus gen. nov., sp. nov., Nitrosopumilus cobalaminigenes sp. nov., Nitrosopumilus oxyclinae sp. nov., and Nitrosopumilus ureiphilus sp. nov., four marine ammonia-oxidizing archaea of the phylum Thaumarchaeota. Int. J. Syst. Evolut. Microbiol. 67, 5067–5079. doi: 10.1099/ijsem.0.002416
Reardon, T., Echeverria, R., Berdegué, J., Minten, B., Liverpool-Tasie, S., Tschirley, D., et al. (2019). Rapid transformation of food systems in developing regions: highlighting the role of agricultural research & innovations. Agric. Syst. 172, 47–59. doi: 10.1016/j.agsy.2018.01.022
Rekha, R., and Singh, P. (2018). Futures trading of maize in India: a tool for price discovery and risk management. Int. Res. J. Agric. Econ. Stat. 9, 113–119. doi: 10.15740/HAS/IRJAES/9.1/113-119
Rich, J. J., Arevalo, P., Chang, B. X., Devol, A. H., and Ward, B. B. (2018). Anaerobic ammonium oxidation (anammox) and denitrification in Peru margin sediments. J. Marine Syst. 207, 1–13. doi: 10.1016/j.jmarsys.2018.09.007
Ruiz, P., Vidal, J. M., Sepúlveda, D., Torres, C., Villouta, G., Carrasco, C., et al. (2020). Overview and future perspectives of nitrifying bacteria on biofilters for recirculating aquaculture systems. Rev. Aquacult. 12, 1478–1494. doi: 10.1111/raq.12392
Schaechter, M. (2009). Encyclopedia of Microbiology. San Diego, CA: San Diego, State University, Academic Press.
Sepehri, A., Sarrafzadeh, M.-H., and Avateffazeli, M. (2020). Interaction between Chlorella vulgaris and nitrifying-enriched activated sludge in the treatment of wastewater with low C/N ratio. J. Clean. Prod. 247, 119164. doi: 10.1016/j.jclepro.2019.119164
Sharma, V., Prasanna, R., Hossain, F., Muthusamy, V., Nain, L., Shivay, Y. S., et al. (2021). Cyanobacterial inoculation as resource conserving options for improving the soil nutrient availability and growth of maize genotypes. Arch. Microbiol. 203, 2393–2409. doi: 10.1007/s00203-021-02223-8
Silva, I. T. D. O. (2021). Selection of Metarhizium spp. for the management of Spodoptera frugiperda (Lepidoptera: Noctuidae) through inoculation in maize seeds and production of conidia and indole-3-acetic acid (Masters Dissertation), Universidade de São Paulo, São Paulo, Brazil.
Song, Y., Li, Z., Liu, J., Zou, Y., Lv, C., and Chen, F. (2021). Evaluating the impacts of Azotobacter chroococcum inoculation on soil stability and plant property of maize crop. J. Soil Sci. Plant Nutr. 21, 824–831. doi: 10.1007/s42729-020-00404-w
Stein, L. Y. (2019). Insights into the physiology of ammonia-oxidizing microorganisms. Curr. Opin. Chem. Biol. 49, 9–15. doi: 10.1016/j.cbpa.2018.09.003
Stein, L. Y., and Klotz, M. G. (2016). The nitrogen cycle. Curr. Biol. 26, 94–98. doi: 10.1016/j.cub.2015.12.021
Strous, M., Fuerst, J. A., Kramer, E. H., Logemann, S., Muyzer, G., Van De Pas-Schoonen, K. T., et al. (1999). Missing lithotroph identified as new planctomycete. Nature 400, 446–449. doi: 10.1038/22749
Tourna, M., Stieglmeier, M., Spang, A., Könneke, M., Schintlmeister, A., Urich, T., et al. (2011). Nitrososphaera viennensis, an ammonia oxidizing archaeon from soil. Proc. Nat. Acad. Sci. U.S.A. 108, 8420–8425. doi: 10.1073/pnas.1013488108
Upadhyay, H., Gangola, S., Sharma, A., Singh, A., Maithani, D., and Joshi, S. (2021). Contribution of zinc solubilizing bacterial isolates on enhanced zinc uptake and growth promotion of maize (Zea mays L.). Folia Microbiol. 56, 543–553. doi: 10.1007/s12223-021-00863-3
Van Kessel, M. A., Speth, D. R., Albertsen, M., Nielsen, P. H., Den Camp, H. J. O., Kartal, B., et al. (2015). Complete nitrification by a single microorganism. Nature 528, 555–559. doi: 10.1038/nature16459
Vatandoost, H., Seyed, S. R., and Kheirizadeh, A. Y. (2019). Effect of irrigation levels and plant growth promoting rhizobacteria on yield, some physiological and biochemical indices of rapeseed (Brassica napus L.). J. Crop Prod. Process. 9, 99–111. doi: 10.29252/jcpp.9.2.99
Vu, A., and Le, T. Q. (2019). Development orientation for higher education training programme of mechanical engineering in industrial revolution 4.0: a perspective in Vietnam. J. Mech. Eng. Res. Dev. 42, 71–73. doi: 10.26480/jmerd.01.2019.71.73
Walters, W. A., Jin, Z., Youngblut, N., Wallace, J. G., Sutter, J., Zhang, W., et al. (2018). Large-scale replicated field study of maize rhizosphere identifies heritable microbes. Proc. Natl. Acad. Sci. U.S.A. 115, 7368–7373. doi: 10.1073/pnas.1800918115
Wang, C., Wang, Y., Ma, J., Hou, Q., Liu, K., Ding, Y., et al. (2018). Screening and whole-genome sequencing of two streptomyces species from the rhizosphere soil of peony reveal their characteristics as plant growth-promoting rhizobacteria. Biomed Res. Int. 2018, 1–11. doi: 10.1155/2018/2419686
Wang, J.-L., Li, T., Liu, G.-Y., Smith, J. M., and Zhao, Z.-W. (2016). Unraveling the role of dark septate endophyte (DSE) colonizing maize (Zea mays) under cadmium stress: physiological, cytological and genic aspects. Sci. Rep. 6, 1–12. doi: 10.1038/srep22028
Webber, H., Ewert, F., Olesen, J. E., Müller, C., Fronzek, S., Ruane, A. C., et al. (2018). Diverging importance of drought stress for maize and winter wheat in Europe. Nat. Commun. 9, 1–10. doi: 10.1038/s41467-018-06525-2
Wendeborn, S. (2019). The chemistry, biology and modulation of ammonium nitrification in soil. Angew. Chemie Int. Ed. 58, 2–23. doi: 10.1002/anie.201903014
Xu, Z., Jiang, Y., and Zhou, G. (2016). Nitrogen cycles in terrestrial ecosystems: climate change impacts and mitigation. Environ. Rev. 24, 132–143. doi: 10.1139/er-2015-0066
Yadav, O., Prasanna, B., Yadava, P., Jat, S., Kumar, D., Dhillon, B., et al. (2016). Doubling maize (Zea mays) production of India by 2025-challenges and opportunities. Indian J. Agric. Sci. 86, 427–434.
Zhao, Y., Li, W., Chen, L., Meng, L., and Zheng, Z. (2020). Effect of enriched thermotolerant nitrifying bacteria inoculation on reducing nitrogen loss during sewage sludge composting. Bioresour. Technol. 311, 123461–123469. doi: 10.1016/j.biortech.2020.123461
Keywords: biotechnology, food security, bacteria, archaea, sustainable agriculture
Citation: Ayiti OE and Babalola OO (2022) Sustainable Intensification of Maize in the Industrial Revolution: Potential of Nitrifying Bacteria and Archaea. Front. Sustain. Food Syst. 6:827477. doi: 10.3389/fsufs.2022.827477
Received: 02 December 2021; Accepted: 23 February 2022;
Published: 21 March 2022.
Edited by:
Sanjay Singh Rathore, Indian Agricultural Research Institute (ICAR), IndiaReviewed by:
Durgesh K. Jaiswal, Savitribai Phule Pune University, IndiaAjar Nath Yadav, Eternal University, India
Javid A. Parray, University of Kashmir, India
Copyright © 2022 Ayiti and Babalola. This is an open-access article distributed under the terms of the Creative Commons Attribution License (CC BY). The use, distribution or reproduction in other forums is permitted, provided the original author(s) and the copyright owner(s) are credited and that the original publication in this journal is cited, in accordance with accepted academic practice. No use, distribution or reproduction is permitted which does not comply with these terms.
*Correspondence: Olubukola Oluranti Babalola, olubukola.babalola@nwu.ac.za
†ORCID: Olubukola Oluranti Babalola orcid.org/0000-0003-4344-1909
Oluwatobi Esther Ayiti orcid.org/0000-0002-6164-8965