- 1Earth and Life Institute—Agronomy, Université Catholique de Louvain, Ottignies-Louvain-la-Neuve, Belgium
- 2Division of Bio-Economics, Katholieke Universiteit Leuven, Leuven, Belgium
- 3World Wildlife Fund, Brussels, Belgium
In Europe, cattle production is confronted with major challenges across all dimensions of sustainability, urging the need to promote environmentally friendly but also economically viable livestock systems. In addition, animal protein consumption greatly exceeds the dietary guidelines in most European countries. The protein transition, defined as the rebalancing between animal and alternative proteins in diets, is presented as a solution to mitigate the harmful effects of cattle production on the environment, but also as an opportunity to induce healthier diets. Yet, the implications of such a transition on current livestock farmers are still unclear. In this article, we investigate different factors associated with a protein transition (e.g., reduction of herd size, increased concentrate autonomy and increased share of pastures) and assess their implications for the economic performance of dairy and beef farmers in Wallonia, Belgium. In the dairy sector, we find that a reduction in herd size, a higher share of pastures and an increased concentrate autonomy are correlated with lower operating costs, resulting in higher margins. Therefore, a switch to more extensive grazing systems that rely on on-farm fodder production can entail economic benefits for farmers. In the beef sector, on the other hand, farm characteristics are uncorrelated with most economic indicators, but highly associated with subsidies. This suggests that changes in this sector will rather be induced by policy choices than by economic parameters.
Introduction
The impact of livestock production, and specifically cattle systems, on the environment and human health is heavily debated. On the one hand, cattle systems and diets that rely heavily on processed and red meat products are associated with strong negative externalities (Pais et al., 2020). Human health externalities include obesity and non-communicable diseases, including increased risk of type 2 diabetes, cardiovascular diseases and some types of cancers (Willett et al., 2019). Environmental externalities include greenhouse gas emissions, especially methane and nitrous oxide, water and air pollution through nutrients leakage, air pollution by ammonia and nitrogen oxide, and depletion of water resources (Gerber et al., 2013). Indirect effects arise from pasture management and the production of feed crops for animals. Soy feed is increasingly imported as a high-quality feed from tropical countries and incorporated in concentrates. The production of other frequently used feed crops, such as maize and molasses supplements, is often associated with environmental externalities as well, particularly due to the high use of pesticides (Antier et al., 2020). These effects are especially pronounced in zero-grazing and more intensive cattle systems, which are increasingly common in European countries (Meul et al., 2012). On the other hand, if managed in well balanced crop and livestock systems, cattle production can also provide key ecosystem services, contributing to soil fertility (Watson et al., 2002), converting non-edible biomass into nutrient dense food for humans (Karlsson and Röös, 2019), helping to mitigate climate change through carbon storage in pastures (Stanley et al., 2018), and preserving biodiversity in grasslands (Teague and Kreuter, 2020).
The protein transition, defined as a rebalancing of protein consumption between animal and alternative proteins,1 is increasingly presented as a solution to mitigate the harmful effects of livestock production on the environment (Machovina et al., 2015; Prag and Henriksen, 2020) and on animal welfare (de Boer and Aiking, 2011), but also as an opportunity to restore healthier diets (Friel et al., 2009; de Boer and Aiking, 2017). The need for a protein transition is especially high in European countries, as protein intake is too high compared to the national dietary guidelines (Resare Sahlin et al., 2020) and the proportion of protein consumed is predominantly of animal origin, especially from processed and red meat that are characterized by a high ratio of saturated fat content (de Boer and Aiking, 2019)2. In addition, consumers are increasingly aware of these issues and demand more sustainable and healthier livestock production (de Boer and Aiking, 2018). While the protein transition focuses on the consumer side, such a transition implies a profound transformation of food systems, including livestock production systems (Manners et al., 2020). Factors associated with a protein transition could include reduction in herd size as a consequence of a decrease in meat and dairy consumption, but also a shift to animal-based protein with a lower environmental impact (Houzer and Scoones, 2021). This may entail a shift from more intensive systems highly dependent on the use of forage maize and concentrates to more extensive systems that are more feed self-sufficient (Van Zanten et al., 2016).
Despite the potential positive effects a protein transition might entail in European countries, it is not clear how this will affect the economic performance of current European cattle farmers. An extensive literature focuses on the effects of intensification and feeding practices on profitability and eco-efficiency, but there are some shortcomings. While both dairy and beef sectors are the subject of studies investigating externalities of cattle production, economic studies in Europe rarely focus on beef farms and mostly investigate dairy farms (Alvarez et al., 2008; Ma et al., 2018). The economic implications might be highly different for the two sectors because of different cost structures and levels of subsidy (Smil, 2002). Within the existing studies on the impact of intensification in the dairy sector, there is mixed evidence on the economic performance (Alvarez et al., 2008; Ma et al., 2018) and eco-efficiency (Basset-Mens et al., 2009). Studies evaluating the impact of grazing on profitability find mixed evidence as well (Foltz and Lang, 2005; Gillespie et al., 2009; Hanson et al., 2013; Dutreuil et al., 2014). Specific attention has been paid recently to feed self-sufficiency, with evidence that some European consumers prefer livestock products raised with local feed (Escribano, 2016). In addition, the demand for regionally produced feed is expected to increase to allow for the production of organically produced livestock (Escribano, 2018). Mixed evidence of increased feed self-sufficiency on economic performance are found at the EU and farm level (Lebacq et al., 2015; Deppermann et al., 2018). Nor do these studies investigate the role of subsidies in famers' revenues (Vrolijk et al., 2010). Yet, the European cattle sector, and especially the beef sector, is strongly supported by subsidies from the Common Agricultural Policy (CAP). In 2020, the annual monetary allocation for voluntary coupled support was €4.24 billion, of which 40% is dedicated to the beef sector and 21% to the dairy sector (European Commission, 2020).
In this article, we contribute to these research gaps by providing micro-economic evidence on the economic implications of a protein transition in Wallonia, Belgium. We investigate different factors associated with a protein transition, such as a reduction of herd size and a shift in feeding practices and assess their implications for the economic performance of Walloon dairy and beef farmers. We use panel data from the Farm Accountancy Data Network (FADN) database over the period 2014–2017 and estimate random effects models to control for a wide set of time-variant and -constant characteristics. We investigate different economic indicators (e.g., productivity, price, gross revenues, operating costs, operating profits and operating profit margins) to disentangle cost-reducing and/or revenue-enhancing effects. In addition, we analyse to what extent these factors are associated with an increase (or reduction) in subsidy dependence.
Studying the Walloon dairy and beef sectors is relevant for three reasons. First, the agricultural sector in Wallonia is strongly dominated by livestock farming and more precisely by cattle breeding (SPW, 2020). In 2017, Wallonia counted 4,596 dairy farms and 6,452 beef farms with a total number of 1.1 million cattle (Statbel, 2017). Yet, the two sectors are in economic difficulty, with farmers' incomes often unprofitable and highly dependent on subsidies. In addition, demand for animal-based products is decreasing in Wallonia, as in the rest of Europe (Hocquette and Chatellier, 2011). As a consequence, the average age of Walloon breeders is increasing with fewer candidates to take over the farm. There is an urgent need to develop farming systems that are economically profitable to farmers but also environmentally sustainable. Second, the dairy sector and to a lesser extent the beef sector rely on imported soy converted into concentrates to feed the cows, as in many other Western European countries (Jouan et al., 2020). Soy consumption in Wallonia is estimated at 179,000 tons/year for the dairy sector and 65,000 tons/year for the beef sector of which 54,000 tons/year are for the fattening of bulls and cows (Riera et al., 2020). Livestock production systems that are self-sufficient based on the farm feeding capacity of grass and forage crops have been recently promoted by the EU to counteract the dependence on soy imports (European Commission, 2018). Whether or not this entails economic benefits to farmers has not been studied. Third, both sectors exhibit a large diversity, with systems differing in herd and land size, and grazing and feeding practices (Riera et al., 2020). This heterogeneity allows us to assess the linkages with farms' economic performance.
Literature Review
Cattle systems have strongly intensified over the past decades in the EU and other high-income regions, resulting in higher productivity but also higher use of concentrates and higher livestock density (Peyraud et al., 2014). Whether or not this intensification has been economically profitable is highly debated. On the one hand, Ma et al. (2018) show that moving from low-input systems to high-input systems in the dairy sector in New Zealand is associated with higher operating expenses but not with higher operating profits, leading to lower operating profit margins. Comparing high-input to low-input dairy farms in Europe, Bijttebier et al. (2017) find that even though low-input farms are smaller and less productive, they are not necessarily less profitable and competitive than high-input farms. On the other hand, Alvarez et al. (2008) find a positive correlation between intensification (i.e., higher number of dairy cows per hectare of land, genetically improved dairy cattle, higher concentrate use in diets) and economic efficiency of dairy farms in Spain. This is mainly due to lower average total costs for high-input farms, although this critically depends on input prices.
Another strand in the literature has looked at implications of intensification for eco-efficiency, which is usually measured by combining a life cycle assessment with a data envelopment analysis. A higher eco-efficiency score indicates a lower environmental pressure for a given level of economic activity. Basset-Mens et al. (2009) show that intensification of New Zealand farms is detrimental to their eco-efficiency while the findings of Soteriades et al. (2016) in France suggest that the impact of intensive dairy farming on the environment depends on particular farming systems and circumstances. Bava et al. (2014) show that intensification of dairy farms in Italy has no negative environmental impact when results are expressed per milk unit but that impacts expressed per hectare are positively associated with the intensification level. Some articles support that there is no trade-off between the economic profitability and the environmental impact of a farm in the dairy sector (Thomassen et al., 2009; Jan et al., 2012). However, these results highly depend on the choice of units and on the environmental impact considered (Thomassen et al., 2009; Lebacq et al., 2013). Large differences in resource efficiency and environmental impact per kg of product can be observed between different species and production systems within species, e.g., grass-fed vs. concentrates-fed beef and dairy (Capper, 2012).
Some studies have looked specifically at different feeding strategies and grazing systems. The potential of low-cost fully grazing strategies with a low share of supplementary feeding is of growing interest (Dillon et al., 2008; Reinsch et al., 2021), as pasture-based production systems can contribute both to more resilient farming systems and reduce environmental impacts (Schils et al., 2007). Soder and Rotz (2001) investigate the economic and environmental impact of four levels of concentrate supplementation in grazing dairy herds in Pennsylvania, USA. They show that higher levels of concentrates in the diets are associated with a greater profitability, but also with higher nitrogen leaching losses and thus a negative environmental impact. Dutreuil et al. (2014) compare the impact of three feeding strategies on production costs and net returns in Wisconsin, USA of organic and conventional farms. They show that organic farms have higher net returns because of reduced costs, while conventional farms have lower net returns because the revenues from the high-producing cows are insufficient to compensate for the high feed cost, including high soybean purchases. In addition, they find that grazing systems have the lowest feed cost, but the lower milk productivity leads to lowest net returns. Foltz and Lang (2005) find no impact of grazing systems on profitability when controlling for structural factors. These results are, however, contrasted with other studies finding positive effects of managed grazing on profitability. Meul et al. (2012) compare profitability of grazing and zero-grazing dairy systems in Flanders (Belgium) showing a higher profitability for grazing systems because of reduced costs of concentrates and by-products. Hanson et al. (2013) and Gillespie et al. (2009) report that increased reliance on grazing is associated with higher profits. These two studies focus on US dairy farms though. Economic evidence for the beef sector in Europe is lacking.
Another way farmers may reduce financial costs linked to feeding is to increase feed self-sufficiency. However, feed self-sufficiency, and in particular protein self-sufficiency, is very low in Europe, which is probably due to the high opportunity costs linked to feed self-sufficiency. In 2017, the EU demand for plant proteins amounted to 27 million tons of crude protein, of which 17 million were imported and 13 million were soybean based (European Commission, 2018). It is estimated that 98% of these imports are used for livestock production as feed (Hiel et al., 2018). Gaudino et al. (2018) find that complete feed self-sufficiency is not an optimal strategy from an economic perspective for dairies in Italy as it is not possible to fulfill the potential energy and protein intake of a large number of cows with on-farm production. Similarly, Deppermann et al. (2018) emphasize the potential increased production costs linked to a higher feed self-sufficiency. These results are in contrast with those of Lebacq et al. (2015) who investigate the relationship between input self-sufficiency (including feed self-sufficiency) and the environmental and economic sustainability of Belgian dairy farms. They show that more autonomous farms use less inputs for the same output level, thereby minimizing the trade-off between environmental and economic performance. Econometric analyses to assess the impact of feed self-sufficiency on different economic parameters are still lacking.
Within the EU, it is important to assess the role of subsidies provided by the CAP, given the high reliance of the dairy and particularly the beef sector on subsidies (Vrolijk et al., 2010). Latruffe et al. (2016) show that higher subsidies in the Belgian dairy sector are associated with lower managerial effort and technical efficiency. Zhu et al. (2012) also show that a higher share of subsidy in the income of three EU countries negatively affects farm technical efficiency and farmers' motivation. However, subsidies can also affect the attitude toward risk (Serra et al., 2008), with some authors arguing that financially constrained farms are potentially less risk averse if they are subsidized (Hennessy, 1998). Despite its economic importance and high reliance on subsidies to complement farm incomes, the beef sector has been overlooked in most empirical studies analyzing the impact of CAP support on technical efficiency (Cillero et al., 2014).
Research Background
The Walloon dairy and beef sectors have strongly intensified over the last few decades (Figure 1), with a decrease in the total number of animals, an increase in productivity per cow and a restructuring of farms (i.e. a decrease in the number of farmers but an increase in the per farm herd size) (SPW, 2020). Figure 2 shows that milk production increased slightly in Belgium and remained stable in Wallonia between 2002 and 2018 while meat production decreased in Belgium and Wallonia from 2015. Activities in the beef sector are strongly differentiated, with beef farms either specialized in rearing and maintaining suckler cows,3 or in fattening bulls and cows. Closed farms performing both activities occur too but are largely in the minority (Tessier et al., 2020). Beef farms in Wallonia are mainly dedicated to breeding activities. The region is highly dependent on other territories such as Flanders to fatten and slaughter the different types of cattle.
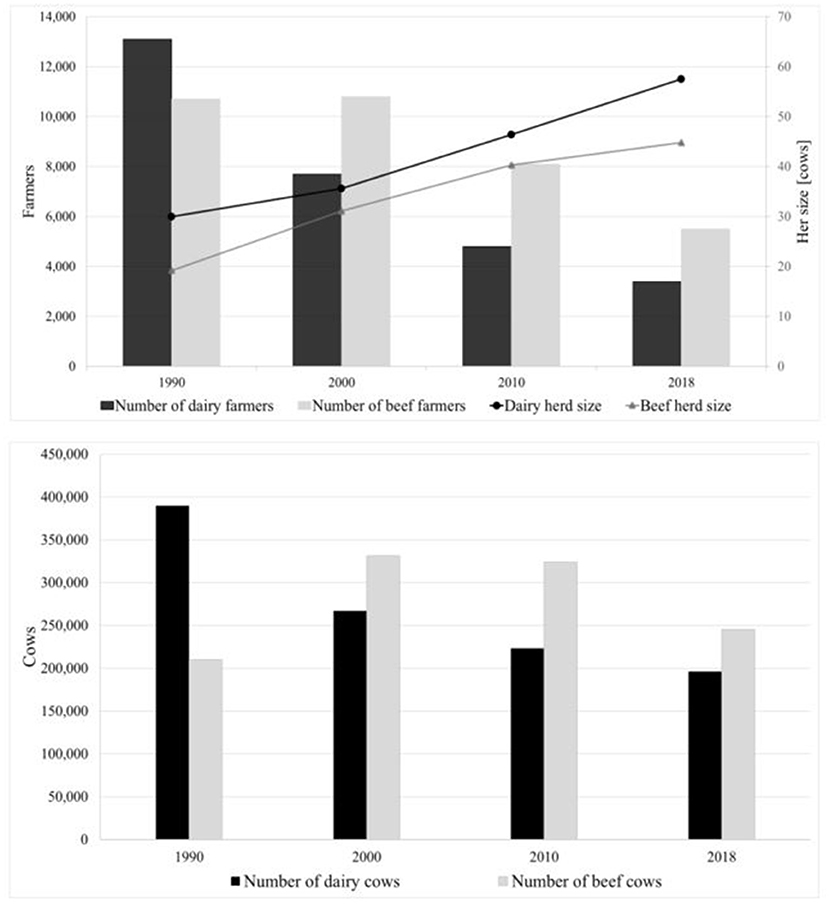
Figure 1. Key features of the Walloon beef and dairy sectors (SPW, 2020).
The most common breeds within the Walloon dairy sector are the Freisen-Holstein (89%), the Bleues mixtes (4%), the Montbéliardes (2%), the Rouge Pie de l'Est (1%), the Normandes (1%) and other breeds (4%) (La Spina, 2017), while the dominant breed in the beef sector is the double-muscled Belgian Blue (83%) followed by French breeds such as the Limousine (6%), the Blonde d'Aquitaine (3%) and Charolaise (2%) (Buron et al., 2014). Dairy production is concentrated in the province of Liège (Région herbagère Liégeoise and Haute Ardenne), while beef production is mainly located in the South of Wallonia, particularly in the province of Luxembourg (SPW, 2020).
Feeding practices have strongly evolved since the 1960s for the dairy sector. Historically, grazed grass was the only feed. Yet, as the milk yield potential of the herds increased significantly through successive selections, pasture was no longer sufficient to fulfill the potential productivity of the cows, leading to changes in feed rations. For the beef sector, feeding practices have evolved with the selection and rearing of the double-muscled Belgian Blue breed in the 1970s. Feeding practices depend strongly on the farm's specialization and concentrates are mostly used during the fattening step. Feed rations in both sectors have a strong influence on milk productivity as well as on zootechnical performance for meat production. Today, they comprise three categories of feed: grazed grass, forages and concentrates.4 Concentrates include maize and sugar-beet pulp but increasingly soybean products as well, usually imported from Brazil, Argentina, Paraguay and the United States (MacLeod and Peyraud, 2020). Between 2013 and 2018, the amount of soy used to feed animals raised in Belgium averaged 880,000 tons per year, nearly doubling from 683,000 tons in 2013 to 1.02 million tons in 2018. Of the total Belgian soy used for animal feed, 19% is used for dairy farming and 2% for beef farming (WWF, 2019).
Agricultural subsidies from the European CAP, including coupled support and green payments, represent a large share of breeders' income. Even though income support payments have been progressively decoupled from specific products, an exemption to this general rule is allowed for suckler cows. Therefore, EU countries may continue to link income support payments to certain products, such as meat or milk (Hocquette and Chatellier, 2011). In 2014, Wallonia applied for an exemption to devote 21.1% of its envelope to coupled support instead of the 13% provided in the European regulation. Since 2015, 21.3% of the Walloon envelope for the first CAP pillar is devoted to coupled support, of which 18.8% is for the beef sector and 1.2% is for the dairy sector (Gavira et al., 2016). The premium for female beef cattle amounts to 180€ per cow, while the premium for dairy cows is about 25€ per cow. Regarding the green payments, subsidies for forage autonomy are granted to farms according to their grazing livestock density. The annual payment is 120€ per hectare if the density of grazing livestock is less than 1.4 livestock unit (LSU) per hectare of area under grass and/or dedicated to forage crops and 60€ per hectare if the density of grazing livestock is less than 1.8 LSU per hectare (Natagriwal, 2014).
Data and Methods
Data
The economic performance of Walloon farms was assessed using the FADN database. This network, established in 1965 by the European Commission, provides standardized micro-economic indicators at the farm level. In Wallonia, the “Direction de l'Analyse Economique Agricole” (DAEA) is in charge of collecting these data. The data cover farm characteristics (e.g. location, number of animals, crop areas) as well as financial and accounting data (e.g. revenues, subsidies, investment liabilities, average farm capital).
We use the data from four survey rounds (2014, 2015, 2016 and 2017). In order to be classified as specialized dairy farms (OTE450) or beef farms (OTE460) in the FADN database, at least 2/3 of the farm's standard outputs must come from dairy or meat activities. As a result, an unbalanced pooled sample of 114 dairy farms and 82 beef farms were selected. We then excluded dairy farms where more than 10% of cows were suckler cows to ensure specialization in milk production. This criterion avoids the inclusion of farms where the boundary between milk and meat production is less marked. As a result, 19 of the 114 farms were excluded.5 For the beef sector, we excluded fattening farms and selected farms with a dominant breed of more than 50%. We then retained farms that raise the double-muscled Belgian Blue and excluded farms that raise French breeds, as they are typically raised organically. It is nearly impossible to produce Belgian Blue meat according to the standards of organic production as the number of cesarean sections is limited to 20% and preventive allopathic treatments and concentrate inputs are restricted. As a result, 17 of the 82 beef farms were excluded. The final sample consisted of an unbalanced panel of 95 dairy farms with 324 observations over the 4 years, and 65 beef farms with 208 observations over the 4 years.
Regression Models
To assess the economic performance of the farms in both sectors, we analyzed Cobb-Douglas type production functions and estimated the following equation:
Where Yit represents the outcome variable of interest of farm i in region j at time t (t = 2014, …, 2017). We used seven outcome variables: (1) milk productivity (in liter of milk per cow) (specific indicator of the dairy sector), (2) gross revenues per hectare6 (total revenues excluding subsidies), (3) operating expenses per hectare (operating costs including cost of salaried labor, work by third parties, total expenses for seeds, plants, fertilizers and control products, veterinary and other expenses), (4) operating profits per hectare (gross revenues minus operating expenses), (5) operating profit margins (operating profits divided by gross revenues), (6) subsidies per hectare, and (7) subsidy dependence (subsidies divided by gross revenues). The values relate to the 12 month period prior to the survey, i.e., values for 2014 correspond to the results obtained during the year 2013. Milk productivity, gross revenues, operating expenses, operating profits and subsidies are expressed in logarithmic values to obtain a normal distribution and hence improve the model fit, and to interpret the results as elasticities. As a robustness check, dependent variables were also expressed per unit of labor and per livestock unit.7
Xit is a vector of independent variables including share of grassland (permanent and temporary grasslands divided over forage area), cattle area (area dedicated to milk or beef production including forage and concentrate areas8), grazing livestock density (livestock units per hectare of forage area), herd size (total livestock units), total concentrate use (in kilograms), concentrate autonomy (share of concentrate produced on-farm) and labor (in number of annual work units,9 including family labor). We refer to feed autonomy as the combination of concentrate autonomy and the share of grassland. These variables were selected based on theoretical production models and similar studies that investigate the economic performance of cattle farms (Thomassen et al., 2009; Ma et al., 2018). They are also strongly linked to the farm-level implications of a protein transition: a reduction of herd size and a shift in feeding practices from more intensive systems that heavily rely on concentrates produced off-farm (such as imported soy feed) to less intensive systems that rely more on grazing and concentrates produced on-farm. We also include year dummies (θt) and region dummies (υj). Seven regions of Wallonia are considered (Limoneuse, Sablo-limoneuse, Condroz, Herbagère, Ardenne, Jura, and Famenne). Finally, αi represents the farm-level fixed effects and εijt the time-varying component of the error term.
We adopted a random effects (RE) model,10 which assumes no correlation between observed explanatory variables and the farm-level fixed effects. As this is a strong assumption, we refrain from making causal statements, but focus instead on correlations that remain significant after controlling for a set of other explanatory variables. We use robust standard errors to solve problems related to heteroscedasticity and auto-correlation. The variance inflation factor is 9.71, indicating that there were no significant multicollinearity issues. All regressions were performed using Stata 16.1 software.
Results
Summary Statistics
In general, all economic variables are better for the dairy sector than the beef sector. In particular, the dairy sector performs better in terms of gross revenues, operating profits and operating profit margins (Table 1). Gross revenues per hectare of the dairy sector are 2,918€ compared to 1,810€ for the beef sector, while operating profits per hectare are 1,599€ and 748€ respectively. The operating profit margin is on average 0.56 for the dairy sector compared to 0.42 for the beef sector. In addition, the dairy sector is less dependent on subsidies, with a subsidy dependence of 28% of total revenues for the beef sector compared to 13% for the dairy sector. Appendix Table A1 in Supplementary Material shows economic indicators expressed per cow and per unit of labor, also confirming a significantly better economic performance in the dairy sector.
A sector comparison reveals beef farms are characterized by statistically significant larger herd sizes with an average of 147 LSUs compared to 121 LSUs for the dairy sector, and a higher grazing livestock density with 2.23 LSU per hectare of fodder area compared to 1.96 for the dairy sector (Table 2). Average area does not differ statistically across sectors and is respectively 65 and 69 hectares for the dairy and beef sectors. The share of grassland is also comparable between the two sectors with 84% of the area dedicated to grassland for the dairy sector and 88% for the beef sector. Regarding concentrates, the dairy sector has a higher total concentrate use (1,010 kg per LSU and per year for the dairy sector against 500 kg per LSU and per year for the beef sector) and a lower concentrate autonomy (0.06 against 0.19). Finally, more labor input is observed in the dairy sector than in the beef sector.
Regression Analysis
Table 3 provides the results of the random effects regression for all economic indicators for the dairy sector. A 10% increase in herd size is associated with 10% increase in gross revenues, 8% in operating expenses and 14% in operating profits, but not with an increase in profit margins. Additionally, a 10% increase in herd size is associated with a 2.8 percentage point (pp) decrease in subsidy dependence. The share of grassland is highly significant for milk productivity, gross revenues, operating expenses and operating profit margin. A 10 pp increase in the share of grassland involves a 4% decrease in milk productivity, a 4% decrease in gross revenues and 8% decrease in operating costs, resulting in a 1.7 pp increase in operating profit margin. Finally, concentrate autonomy is weakly significant for milk productivity, operating expenses and operating profits and highly significant for operating profit margins. Specifically, a 10 pp increase in concentrate autonomy is associated with a 4 unit decrease in milk productivity, a 3% decrease in operating costs and a 4% increase in operating profits. A 10 pp increase in concentrate autonomy is associated with a 1.2 pp increase in operating profit margin. Grazing livestock density is negatively correlated to operating profits and operating profit margin but positively correlated to subsidy dependence. Cattle area is negatively correlated to gross revenues, operating expenses and operating profits but positively related to subsidy dependence. Total concentrate and labor are not significantly correlated with the economic indicators, but labor is positively associated with total subsidies and subsidy dependence.
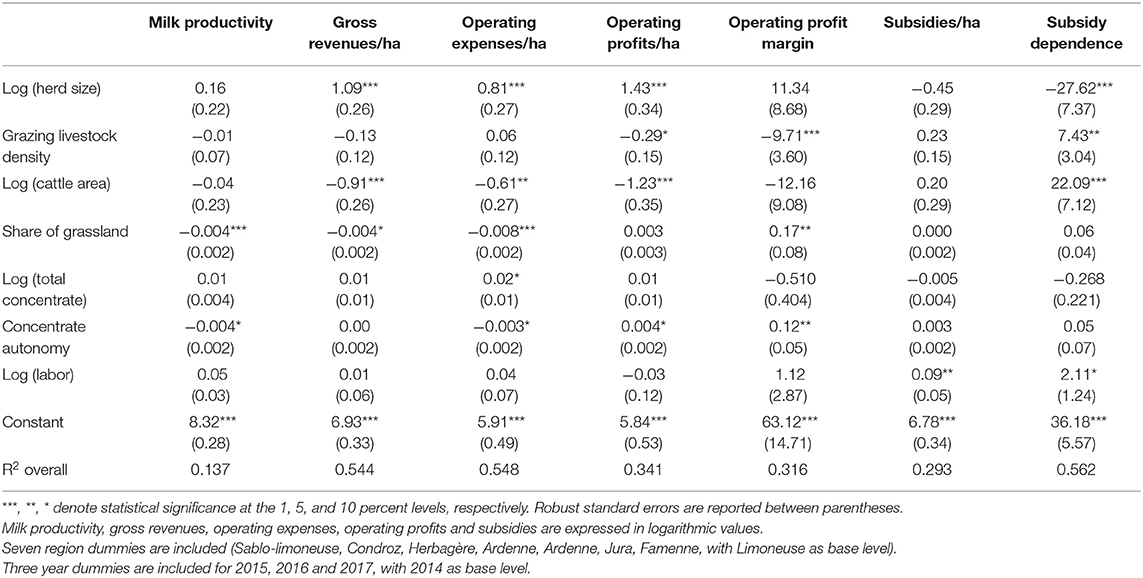
Table 3. Random effects regression analysis for the dairy sector—(dependent variables per hectare) (N = 324).
Table 4 shows the regression results for the beef sector. While few coefficients of our variables of interest are significant for economic performance, a majority are statistically linked to the subsidies. A 10% increase in herd size is associated with a 3 pp decrease in the subsidy dependence. A 10 pp increase in the share of grassland is associated with a 10% decrease in gross revenues and a 15% decrease of operating expenses. Finally, concentrate autonomy has no influence on the dependent variables. We also see a positive correlation between grazing livestock density and subsidy per hectare as well as the subsidy dependence. The same positive correlation is also observed between cattle area and total subsidies as well as subsidy dependence.
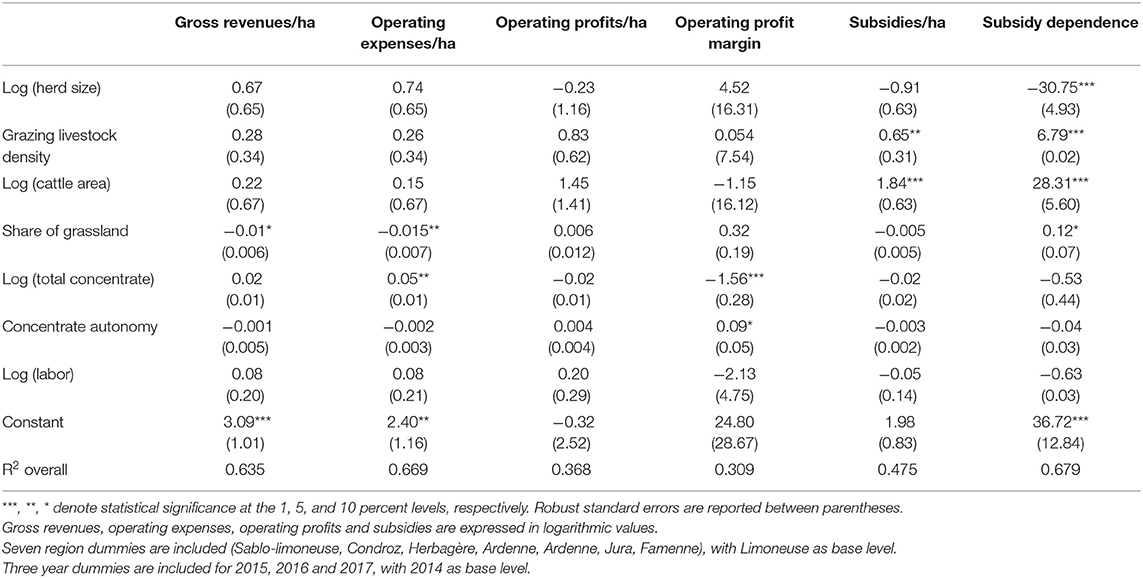
Table 4. Random effects regression analysis for the beef sector—(dependent variables per hectare) (N = 208).
Appendix Tables A2–A5 in Supplementary Material show the regression results for the dependent variables expressed per unit of labor and per livestock unit. Results remain robust as the coefficients signs remain the same and significance levels do not change much. When dependent variables are expressed per cow, herd size and cattle area, they are not significant anymore for the dairy sector but results for the share of grassland and concentrate autonomy remain similar.
Discussion
The results show substantial differences between the dairy and beef sectors, indicating that economic implications of a potential protein transition from meat to more plant-based human diets in Belgium will impact the two sectors differently. In the dairy sector, we find a reduction in herd size is associated with lower gross revenues but also reduced operating costs, leading to lower operating profits but no differences in operating profit margins. Farms with a higher share of pastures and an increased concentrate autonomy have a lower milk productivity and therefore lower gross revenues, but their operating costs are lower, resulting in higher operating profit margins and no significant differences of operating profits. These results suggest a switch to more extensive grazing systems can entail some economic benefits or at least no economic penalty for farmers, which is in line with studies by Meul et al. (2012), Bijttebier et al. (2017), and Ma et al. (2018).
Our results also entail implications for feed autonomy and protein self-sufficiency in dairy farms. We find a better economic performance when concentrate autonomy is increased, which is in line with the conclusions of Lebacq et al. (2015) and Gaudino et al. (2018). Regarding the positive effects of the share of on-farm pasture, the results are in line with other studies (Gillespie et al., 2009; Meul et al., 2012; Hanson et al., 2013; Lebacq et al., 2015). Grazing remains the usual management practice in Wallonia with more than 96% of lactating dairy cows on pastures (Lessire et al., 2019) and 43% of its agricultural area dedicated to pastures (SPW, 2020). Changes to pasture-based systems might be more challenging nutritionally for high-demanding breeds. A switch to breeds more suited to extensive grazing systems requires adaptations at the production and processing level, such as breeding objectives and carcass classification in slaughterhouses. Our results contribute to the larger debate on sustainable intensification by showing that extensification is not correlated to lower incomes in the Belgian dairy sector.
In the beef sector, on the other hand, farm characteristics are highly correlated with subsidies and few farm characteristics are associated with economic indicators. Subsidies are correlated to larger cattle area and higher grazing livestock stocking rates. Farmers are operating under substantial economic losses and their profits depend on the subsidies they receive to cover their losses. This reliance on subsidies from the CAP has been found in other studies (Wolfová et al., 2004; Vrolijk et al., 2010; Hocquette and Chatellier, 2011), and suggests that changes in this sector will rather be induced by changes to the CAP subsidies than by economic parameters. European cattle farmers are embedded in the European food system where they receive subsidies through coupled support11 (Hayden et al., 2019). As a result, the business model of beef farms is rather based on subsidies than on actual market supply and demand factors. More research is needed on how these subsidies can gear a protein transition in which beef farmers are not left behind.
This study entails some limitations. While the FADN data represent the most reliable source on farms' economic indicators, there are some shortcomings. We do not include return on investments as an indicator of economic performance, as the data do not cover this information. This means that long-term effects of switching to more pasture-based extensive systems, for example, cannot be evaluated. In addition, the dataset does not provide detailed information on feeding practices, such as the share of soy in concentrates or the origin of fodder. Therefore, feed autonomy is only assessed at the farm level, while it might be possible that fodder is purchased in the vicinity of the farm and traded with other farmers in the region. In addition, the dataset does not provide information on zero grazing systems and does not allow us to distinguish between different types of subsidies (i.e. coupled, decoupled, and agri-environmental).
From a methodological perspective, our retrospective impact assessment does not consider general equilibrium effects of the protein transition occurring at a larger scale, possibly leading to changes in market prices of both inputs and outputs, but also in subsidies. We also stress that our results hold for the Walloon context, a region with one of the highest livestock densities in Europe and where animal protein consumption is too high compared to national dietary guidelines. More research is needed to investigate the implications of a protein transition, and how subsidies might affect this, in countries where a protein transition is deemed to be beneficial. Such studies should not only look at the micro-level (i.e. at the farm level), but also at the meso-level (i.e. regional and national levels) and the macro-level (i.e. global food system), preferably taking both environmental and economic effects into account. A promising avenue to investigate this at the European level is the integration of sustainability indicators in the FADN, as stipulated by one of the objectives of the EU Farm-to-Fork Strategy.
Conclusion
This study assessed the economic implications of a potential meat to plant-based protein transition for Walloon dairy and beef farms. Using detailed FADN panel data, we pay particular attention to herd size, share of grazing and feed self-sufficiency. We find large differences for the dairy and beef sector, indicating that the role of livestock systems in such a transition is likely to be highly dependent on the sector. In the dairy sector, we find that a reduction in herd size, a higher share of on-farm pastures and an increased concentrate autonomy are correlated with lower operating costs, resulting in higher margins - or similar margins in of the event of herd size reduction. Therefore, a switch to more extensive grazing systems that rely on on-farm fodder production can entail economic benefits or at least no economic penalty for farmers. In the beef sector, on the other hand, farm characteristics are uncorrelated with most economic indicators, but are highly associated with subsidies. Farmers are operating under very strong economic constraints, suggesting that changes in this sector will rather be induced by policy shifts (including subsidy allocation) than by economic incentives. More research is needed on potential scenarios in the context of a protein transition, and the economic and environmental impacts related to those scenarios. Studies could particularly focus on the potential of livestock production to contribute to the protein transition and the impact of different production systems on economic and environmental indicators.
Data Availability Statement
Publicly available datasets were analyzed in this study. Data was provided by the DAEA (Direction de l'Analyse Economique Agricole), the FADN liaison office for Wallonia.
Author Contributions
The study was commissioned by MS. PB and AR were responsible for providing the database and initial data processing. GV and OD performed the statistical analysis. OD wrote the drafts of the manuscript. All authors contributed to manuscript revision, read, and approved the submitted version.
Funding
This work received support from the EU co-funded Eat4Change DEAR project, aiming at the transition towards more sustainable consumption and production in Belgium and Europe, with a special focus on the livestock sector. The Grand Agreement number is: CSO-LA/2020/414-438.
Conflict of Interest
MS was employed by World Wildlife Fund.
The remaining authors declare that the research was conducted in the absence of any commercial or financial relationships that could be construed as a potential conflict of interest.
Publisher's Note
All claims expressed in this article are solely those of the authors and do not necessarily represent those of their affiliated organizations, or those of the publisher, the editors and the reviewers. Any product that may be evaluated in this article, or claim that may be made by its manufacturer, is not guaranteed or endorsed by the publisher.
Supplementary Material
The Supplementary Material for this article can be found online at: https://www.frontiersin.org/articles/10.3389/fsufs.2022.803872/full#supplementary-material
Footnotes
1. ^Including plant-based proteins as well as cellular proteins, new animal proteins and proteins from by-products.
2. ^In other regions, particularly in low-income countries in Asia and Africa, the reverse holds: meat or other major protein sources should be added to mitigate micronutrient deficiencies (Willett et al., 2019).
3. ^Suckler cows are not milked as their calves stay with the herd to suckle. After the production cycle, they are fattened and sold as cull cows before being sent to the slaughterhouse.
4. ^Grass from meadows is a natural and highly nutritional food due to its balance in terms of energy, protein, minerals, antioxidants and fatty acids. Forages are cellulose-rich and include silages (grass, maize, wet pulp, etc), dry fodder (hay, straw), root and tubers (beetroot and potatoes) and brewers' grains. Finally, concentrates have low cellulose and high energy contents. They have a very high nutritional value and are easily digested by ruminants (Petel et al., 2017).
5. ^A similar criterion was applied to the meat sector (exclusion of farms with more than 10% of dairy cows) but this did not concern any farm.
6. ^Economic variables are expressed per hectare of utilized agricultural area (UAA), corresponding to the physical area of the holding.
7. ^The livestock unit is a reference unit for aggregating livestock of different species and ages using specific coefficients initially established on the basis of the nutritional or feed requirements of each type of animal. The standard unit used for calculating the number of livestock units (= 1 LSU) is the grazing equivalent of a dairy cow producing 3000 kg of milk per year, without concentrated feed supplements (Eurostat, 2022).
8. ^Forage area includes grassland and land destined for forage maize and other fodder crops (e.g., alfalfa, clover, forage cereals, secondary forage crops). Concentrate area include land destined to produce cereals and protein crops (on-farm and off-farm).
9. ^A work unit performs 2,000 h of work on a farm in one year.
10. ^Because of limited within-variation, a fixed effects model could not be used.
11. ^27 out of 28 Member States made use of the coupled support between 2014 and 2020.
References
Alvarez, A., del Corral, J., Solís, D., and Pérez, J. A. (2008). Does intensification improve the economic efficiency of dairy farms? J. Dairy Sci. 91, 3693–3698. doi: 10.3168/jds.2008-1123
Antier, C., Kudsk, P., Reboud, X., Ulber, L., Baret, P. V., and Messéa, A. (2020). Glyphosate use in the european agricultural sector and a framework for its further monitoring. Sustainability 12:5682. doi: 10.3390/su12145682
Basset-Mens, C., Ledgard, S., and Boyes, M. (2009). Eco-efficiency of intensification scenarios for milk production in New Zealand. Ecol. Econ., Eco-effici. From Technic. Optimisat. Reflect. Sustain. Anal. 68, 1615–1625. doi: 10.1016/j.ecolecon.2007.11.017
Bava, L., Anna, S., Zucali, M., Guerci, M., and Tamburini, A. (2014). How can farming intensification affect the environmental impact of milk production? J. Dairy Sci. 97, 530. doi: 10.3168/jds.2013-7530
Bijttebier, J., Hamerlinck, J., Moakes, S., Scollan, N., Van Meensel, J., and Lauwers, L. (2017). Low-input dairy farming in Europe: Exploring a context-specific notion. Agric. Syst. 156, 43–51. doi: 10.1016/j.agsy.2017.05.016
Buron, M.-H., Bouquiaux, J.-M., and Marsin, J.-M. (2014). Blanc-bleu belge, Blonde d'Aquitaine, Charolaise, Limousine, les 4 races viandeuses les plus répandues en Wallonie.
Capper, J. L. (2012). Is the grass always greener? comparing the environmental impact of conventional, natural and grass-fed beef production systems. Animals 2, 127–143. doi: 10.3390/ani2020127
Cillero, M. M., Wallace, M., Thorne, F., and Breen, J. (2014). Analyzing the Impact of Subsidies on Beef Production Efficiency in Selected European Union Countries. A Stochastic Metafrontier Approach. Am. J. Agric. Econ. 103, 1903–23.
de Boer, J., and Aiking, H. (2011). On the merits of plant-based proteins for global food security: Marrying macro and micro perspectives. Ecol. Econ., Special Sect. Ecol. Econ. Environ. Hist. 70, 1259–1265. doi: 10.1016/j.ecolecon.2011.03.001
de Boer, J., and Aiking, H. (2017). Pursuing a low meat diet to improve both health and sustainability: how can we use the frames that shape our meals? Ecol. Econ. 142, 238–248. doi: 10.1016/j.ecolecon.2017.06.037
de Boer, J., and Aiking, H. (2018). Prospects for pro-environmental protein consumption in Europe: Cultural, culinary, economic and psychological factors. Appetite 121, 29–40. doi: 10.1016/j.appet.2017.10.042
de Boer, J., and Aiking, H. (2019). Strategies towards healthy and sustainable protein consumption: A transition framework at the levels of diets, dishes, and dish ingredients. Food Qual. Prefer. 73, 171–181. doi: 10.1016/j.foodqual.2018.11.012
Deppermann, A., Havlík, P., Valin, H., Boere, E., Herrero, M., Vervoort, J., et al. (2018). The market impacts of shortening feed supply chains in Europe. Food Secur. 10, 1401–1410. doi: 10.1007/s12571-018-0868-2
Dillon, P., Hennessy, T., Shalloo, L., Thorne, F., and Horan, B. (2008). Future outlook for the Irish dairy industry: a study of international competitiveness, influence of international trade reform and requirement for change. Int. J. Dairy Technol. 61, 16–29. doi: 10.1111/j.1471-0307.2008.00374.x
Dutreuil, M., Wattiaux, M., Hardie, C. A., and Cabrera, V. E. (2014). Feeding strategies and manure management for cost-effective mitigation of greenhouse gas emissions from dairy farms in Wisconsin. J. Dairy Sci. 97, 5904–5917. doi: 10.3168/jds.2014-8082
Escribano, A. J. (2016). “Organic livestock farming—challenges, perspectives, and strategies to increase its contribution to the agrifood system's sustainability—a review,: in Organic Farming-A Promising Way of Food Production, ed Konvalina, P. (London: InTech).
Escribano, A. J. (2018). Organic Feed: A Bottleneck for the Development of the Livestock Sector and Its Transition to Sustainability? Sustainability 10, 2393. doi: 10.3390/su10072393
European Commission (2020). Voluntary coupled support - Review by the Member States of their support decisions applicable as from claim year 2020. European Commission, Brussels.
Eurostat. (2022). Glossary:Livestock Unit (LSU). Eurostat Statistics Explained. Available online at: https://ec.europa.eu/eurostat/statistics-explained/index.php?title=Glossary:Livestock_unit_(LSU)
Foltz, J., and Lang, G. (2005). The adoption and impact of management intensive rotational grazing (MIRG) on Connecticut dairy farms. Renew. Agric. Food Syst. 20, 261–266. doi: 10.1079/RAF2005127
Friel, S., Dangour, A. D., Garnett, T., Lock, K., Chalabi, Z., Roberts, I., et al. (2009). Public health benefits of strategies to reduce greenhouse-gas emissions: food and agriculture. The Lancet 374, 2016–2025. doi: 10.1016/S0140-6736(09)61753-0
Gaudino, S., Reidsma, P., Kanellopoulos, A., Sacco, D., and Van Ittersum, M. K. (2018). Integrated Assessment of the EU's Greening Reform and Feed Self-Sufficiency Scenarios on Dairy Farms in Piemonte, Italy. Agriculture 8, 137. doi: 10.3390/agriculture8090137
Gavira, I. F. T., Burny, P., and Lebailly, P. (2016). Etude d'impact et appui à la mise en oeuvre de la nouvelle réforme de la PAC au niveau Wallon 67.
Gerber, P. J., Steinfeld, H., Henderson, B., Mottet, A., Opio, C., Dijkman, J., et al. (2013). Tackling climate change through livestock: a global assessment of emissions and mitigation opportunities. Rome: Food and Agriculture Organization of the United Nations (FAO).
Gillespie, J., Nehring, R., Hallahan, C., and Sandretto, C. (2009). Pasture-based dairy systems: who are the producers and are their operations more profitable than conventional dairies? J. Agric. Resour. Econ. 34, 412–427. doi: 10.22004/ag.econ.57630
Hanson, J. C., Johnson, D. M., Lichtenberg, E., and Minegishi, K. (2013). Competitiveness of management-intensive grazing dairies in the mid-Atlantic region from 1995 to 2009. J. Dairy Sci. 96, 1894–1904. doi: 10.3168/jds.2011-5234
Hayden, A., Adenaeuer, L., Jansson, T., Höglind, L., and Breen, J. (2019). Possible economic and environmental impacts from changes to the coupled beef support payments for EU beef production.
Hennessy, D. A. (1998). The Production Effects of Agricultural Income Support Policies under Uncertainty. Am. J. Agric. Econ. 80, 46–57. doi: 10.2307/3180267
Hiel, R., Geling, V., de Vries, T., Lan, C.-C., and Sleurink, N. (2018). European soy Monitor - Insights on European Responsible and Deforestation-Free Soy Consumption in 2018. Rome: The Sustainable Trade Initiative.
Hocquette, J.-F., and Chatellier, V. (2011). Prospects for the European beef sector over the next 30 years. Anim. Front. 1, 20–28. doi: 10.2527/af.2011-0014
Houzer, E., and Scoones, I. (2021). Are Livestock Always Bad for the Planet? Rethinking the Protein Transition and Climate Change Debate. PASTRES.
Jan, P., Dux, D., Lips, M., Alig, M., and Dumondel, M. (2012). On the link between economic and environmental performance of Swiss dairy farms of the alpine area. Int. J. Life Cycle Assess. 17, 706–719. doi: 10.1007/s11367-012-0405-z
Jouan, J., Ridier, A., and Carof, M. (2020). Legume production and use in feed: Analysis of levers to improve protein self-sufficiency from foresight scenarios. J. Clean. Prod. 274, 123085. doi: 10.1016/j.jclepro.2020.123085
Karlsson, J. O., and Röös, E. (2019). Resource-efficient use of land and animals—Environmental impacts of food systems based on organic cropping and avoided food-feed competition. Land Use Policy 85, 63–72. doi: 10.1016/j.landusepol.2019.03.035
La Spina, S. (2017). Quelles races bovines pour relever les nouveaux défis de notre agriculture? Potentiel des races mixtes.
Latruffe, L., Bravo-Ureta, B. E., Carpentier, A., Desjeux, Y., and Moreira, V. H. (2016). Subsidies and technical efficiency in agriculture: evidence from European dairy farms. Am. J. Agric. Econ.16, 77. doi: 10.1093/ajae/aaw077
Lebacq, T., Baret, P. V., and Stilmant, D. (2013). Sustainability indicators for livestock farming. A review. Agron. Sustain. Dev. 33, 311–327. doi: 10.1007/s13593-012-0121-x
Lebacq, T., Baret, P. V., and Stilmant, D. (2015). Role of input self-sufficiency in the economic and environmental sustainability of specialised dairy farms. Animal 9, 544–552. doi: 10.1017/S1751731114002845
Lessire, F., Jacquet, S., Veselko, D., Piraux, E., and Dufrasne, I. (2019). Evolution of grazing practices in belgian dairy farms: results of two surveys. Sustainability 11, 97. doi: 10.3390/su11153997
Ma, W., Renwick, A., and Bicknell, K. (2018). Higher intensity, higher profit? empirical evidence from dairy farming in New Zealand. J. Agric. Econ. 69, 739–755. doi: 10.1111/1477-9552.12261
Machovina, B., Feeley, K. J., and Ripple, W. J. (2015). Biodiversity conservation: the key is reducing meat consumption. Sci. Total Environ. 536, 419–431. doi: 10.1016/j.scitotenv.2015.07.022
MacLeod, M., and Peyraud, J.-L. (2020). Future of EU livestock How to contribute to a sustainable agricultural sector? (European commission external study).
Manners, R., Blanco-Gutiérrez, I., Varela-Ortega, C., and Tarquis, A. M. (2020). Transitioning European protein-rich food consumption and production towards more sustainable patterns—strategies and policy suggestions. Sustainability 12, 1962. doi: 10.3390/su12051962
Meul, M., Van Passel, S., Fremaut, D., and Haesaert, G. (2012). Higher sustainability performance of intensive grazing vs. zero-grazing dairy systems. Agron. Sustain. Dev. 32, 629–638. doi: 10.1007/s13593-011-0074-5
Natagriwal (2014). Autonomie fourragère. Les Méthodes Agro-Environnementales et Climatiques (MB 9). Available online at: [WWW Document]. URL https://www.natagriwal.be/fr/mesures-agro-environnementales/liste-des-mae/fiches/details/351
Pais, D. F., Marques, A. C., and Fuinhas, J. A. (2020). Reducing meat consumption to mitigate climate change and promote health: but is it good for the economy? Environ. Model. Assess. 25, 793–807. doi: 10.1007/s10666-020-09710-0
Petel, T., Antier, C., and Baret, P. (2017). Etat des lieux et scénarios à horizon 2050 de la filière lait en Région wallonne 81.
Peyraud, J.-L., Taboada, M., and Delaby, L. (2014). Integrated crop and livestock systems in Western Europe and South America: a review. Eur. J. Agron., Integrated crop-livestock 57, 31–42. doi: 10.1016/j.eja.2014.02.005
Prag, A. A., and Henriksen, C. B. (2020). Transition from animal-based to plant-based food production to reduce greenhouse gas emissions from agriculture—the case of denmark. Sustainability 12, 8228. doi: 10.3390/su12198228
Reinsch, T., Loza, C., Malisch, C. S., Vogeler, I., Klu,ß, C., Loges, R., et al. (2021). Toward Specialized or Integrated Systems in Northwest Europe: On-Farm Eco-Efficiency of Dairy Farming in Germany. Front. Sustain. Food Syst. 5, 614348. doi: 10.3389/fsufs.2021.614348
Resare Sahlin, K., Röös, E., and Gordon, L. J. (2020). ‘Less but better’ meat is a sustainability message in need of clarity. Nat. Food 1, 520–522. doi: 10.1038/s43016-020-00140-5
Riera, A., Antier, C., and Baret, P. (2020). Analyse des performances environnementales et économiques de différents systèmes de production bovins en Région wallonne 147.
Schils, R. L. M., Olesen, J. E., del Prado, A., and Soussana, J. F. (2007). A review of farm level modelling approaches for mitigating greenhouse gas emissions from ruminant livestock systems. Livest. Sci., Recycl. Livestock Manure Whole-Farm Perspective 112, 240–251. doi: 10.1016/j.livsci.2007.09.005
Serra, T., Zilberman, D., and Gil, J. M. (2008). Farms' technical inefficiencies in the presence of government programs*. Aust. J. Agric. Resour. Econ. 52, 57–76. doi: 10.1111/j.1467-8489.2008.00412.x
Smil, V. (2002). Worldwide transformation of diets, burdens of meat production and opportunities for novel food proteins. Enzyme Microb. Technol., Third Int. Sympos. Industr. Prot. 30, 305–311. doi: 10.1016/S0141-0229(01)00504-X
Soder, K. J., and Rotz, C. A. (2001). Economic and Environmental Impact of Four Levels of Concentrate Supplementation in Grazing Dairy Herds. J. Dairy Sci. 84, 2560–2572. doi: 10.3168/jds.S0022-0302(01)74709-1
Soteriades, A. D., Stott, A. W., Moreau, S., Charroin, T., Blanchard, M., Liu, J., et al. (2016). The Relationship of Dairy Farm Eco-Efficiency with Intensification and Self-Sufficiency. evidence from the french dairy sector using life cycle analysis, data envelopment analysis and partial least squares structural equation modelling. PLOS ONE 11, e0166445. doi: 10.1371/journal.pone.0166445
Stanley, P. L., Rowntree, J. E., Beede, D. K., DeLonge, M. S., and Hamm, M. W. (2018). Impacts of soil carbon sequestration on life cycle greenhouse gas emissions in Midwestern USA beef finishing systems. Agric. Syst. 162, 249–258. doi: 10.1016/j.agsy.2018.02.003
Teague, R., and Kreuter, U. (2020). Managing Grazing to Restore Soil Health, Ecosystem Function, and Ecosystem Services. Front. Sustain. Food Syst. 4, 187. doi: 10.3389/fsufs.2020.534187
Tessier, L., Bijttebier, J., Marchand, F., and Baret, P. (2020). Agroecology and Sustainable Food Systems Pathways of action followed by Flemish beef farmers -an integrative view on agroecology as a practice. Agroecol. Sustain. Food Syst. 45, 64. doi: 10.1080/21683565.2020.1755764
Thomassen, M. A., Dolman, M., and Calker, B.oer (2009). Relating life cycle assessment indicators to gross value added for Dutch dairy farms. Ecol. Econ. (2009) 68, 8, 9. doi: 10.1016/j.ecolecon.2009.02.011
Van Zanten, H., Meerburg, B. G., Bikker, P., Herrero, M., and De Boer, I. (2016). The role of livestock in a sustainable diet: a land-use perspective. Animal 16, 547–549. doi: 10.1017/S1751731115002694
Vrolijk, H., Bont, C., Blokland, P., and Soboh, R. (2010). Farm Viability in the European Union : Assessment of the Impact of Changes in Farm Payments.
Watson, C., Atkinson, D., Gosling, P., Jackson, L., et al. (2002). Managing soil fertility in organic farming systems. Soil Use Manag. 18, 239–247. doi: 10.1079/SUM2002131
Willett, W., Rockström, J., Loken, B., Springmann, M., Lang, T., Vermeulen, S., et al. (2019). Food in the Anthropocene: The EAT-Lancet Commission on healthy diets from sustainable food systems. Lancet 393, 447–492. doi: 10.1016/S0140-6736(18)31788-4
Wolfová, M., Wolf, J., Zahrádková, R., Pribyl, J., Dano, J., and Kica, J. (2004). Main sources of the economic efficiency of beef cattle production systems. Czech J. Anim. Sci. 49, 357–372. doi: 10.17221/4320-CJAS
Keywords: livestock production, economic performance, protein transition, intensification, feed self-sufficiency, grazing practices
Citation: Duluins O, Riera A, Schuster M, Baret PV and Van den Broeck G (2022) Economic Implications of a Protein Transition: Evidence From Walloon Beef and Dairy Farms. Front. Sustain. Food Syst. 6:803872. doi: 10.3389/fsufs.2022.803872
Received: 28 October 2021; Accepted: 23 February 2022;
Published: 05 April 2022.
Edited by:
Fred Provenza, Utah State University, United StatesReviewed by:
Marcia Dutra De Barcellos, Federal University of Rio Grande do Sul, BrazilUrs Kreuter, Texas A&M University, United States
Copyright © 2022 Duluins, Riera, Schuster, Baret and Van den Broeck. This is an open-access article distributed under the terms of the Creative Commons Attribution License (CC BY). The use, distribution or reproduction in other forums is permitted, provided the original author(s) and the copyright owner(s) are credited and that the original publication in this journal is cited, in accordance with accepted academic practice. No use, distribution or reproduction is permitted which does not comply with these terms.
*Correspondence: Océane Duluins, oceane.duluins@uclouvain.be