- 1Laboratory of Sustainable Food Processing, Institute of Food, Nutrition and Health, Department of Health Science and Technology, ETH Zurich, Zurich, Switzerland
- 2Department of Civil Engineering, Indian Institute of Technology (IIT) Kanpur, Kanpur, India
Food production for human consumption is a leading cause of environmental damage in the world and yet over two billion people suffer from malnutrition. Several studies have presented evidence that changes in dietary patterns across the world can lead to win-win outcomes for environmental and social sustainability and can complement ongoing technological and policy efforts to improve the efficiency of agricultural production. However, the existing evidence have been compiled in “silos” by a large range of researchers across several disciplines using different indicators. The aim of this quantitative review is to bring together the existing knowledge on heterogeneity of current dietary patterns across the world and how a transition toward healthy diets in different countries can aid in progress toward multiple global Sustainable Development Goals (SDGs). We first summarize the nutritional quality, economic cost, and environmental footprint of current diets of over 150 countries using multiple indicators. Next, we review which shifts in dietary patterns across different world regions can help toward achievement of SDG2 (Zero hunger), SDG3 (Good health and wellbeing), SDG 6 (Clean water and sanitation), SDG13 (Climate action), SDG14 (Life below water), and SDG15 (Life on land). Finally, we briefly discuss how to enable the shift toward sustainable dietary patterns and identify the research and data gaps that need to be filled through future efforts. Our analysis reveals that dietary change is necessary in all countries as each one has unique priorities and action items. For regions such as Sub-Saharan Africa and South Asia, increased intake of nutrient dense foods is needed to address deficiency of essential nutrients like folate, potassium, and vitamin A. For North America and Europe, shifting toward more plant-based diets would be healthier and simultaneously reduce the per capita environmental footprints. The results can be useful for policymakers in designing country-specific strategies for adoption of sustainable dietary behaviors and for food industry to ensure the supply of sustainable food items customized with regions' need.
Introduction
The United Nations Sustainable Development Goals (SDGs) outline the global consensus on social, economic, and environmental targets that humanity is striving to achieve by the year 2030 (United Nations, 2015). Achievement of these 17 SDGs is highly dependent upon the dietary habits of people across the world. This is because over two billion people suffer from malnutrition and dietary factors are one of the main causes of premature mortality and morbidity according to global burden of disease assessment (GBD 2019 Diseases Injuries Collaborators, 2020), thereby hampering the progress toward SDG2 (Zero hunger) and SDG3 (Good health and wellbeing).
Moreover, current agricultural production sector supplying food for human consumption is the leading employer of the people and a main contributor to the environmental problems such as GHG emissions, freshwater scarcity, eutrophication, land degradation and biodiversity loss (Chaudhary et al., 2018a; Willett et al., 2019), thereby hampering the progress toward SDG 6 (Clean water and sanitation), SDG13 (Climate action), SDG14 (Life below water), and SDG15 (Life on land).
A global transition toward sustainable diets that are affordable, nutritionally adequate, and environmentally friendly will be key in achieving several SDGs simultaneously (Roberts and Mattoo, 2019; Garcia et al., 2020; Vågsholm et al., 2020). As of today, this seems a mammoth task because the current diets of most people around the world is either lacking in essential micronutrients, or high in nutrients of health concern or high in environmental footprint or all of the above (Springmann et al., 2018a).
Earlier literature in this field explored the health, nutrition, environment, or economic consequences of a shift from current toward alternative diets such as vegan, vegetarian, Mediterranean, etc. at the national or regional levels (Vanham et al., 2013). This was followed by several global scale studies that compared the intake levels of major food groups in different countries with alternative or WHO recommended levels and quantified the benefits of such a transition on health or environment (Tilman and Clark, 2014). All these studies were confined to a limited number of sustainability indicators such as GHG emissions, freshwater use, disease mortality, etc. (Jalava et al., 2014; Springmann et al., 2016).
This was followed by one of the most comprehensive analysis on this topic to date, where the recent EAT-Lancet commission on healthy diets from sustainable food systems proposed a healthy diet that meets the daily nutritional recommendations and does not transgress the different environmental planetary boundaries (Willett et al., 2019). Later studies calculated the cost of this healthy diet for different countries and reflected upon the economic sustainability or affordability dimension of healthy global diets (Hirvonen et al., 2019).
However, we realized that the published literature on dietary change to improve sustainability outcomes have been spread across a wide range of journals—each catering to a niche audience in silos (Jones et al., 2016). Studies discussing dietary change under a consistent framework of SDGs are almost non-existent. The aim of this study is to fill this research gap.
Here we perform a review to summarize the existing knowledge on how the dietary transformation across the world can help the progress toward multiple sustainable development goals (SDGs). We first summarize how the current diets of different countries look like from the social (nutrition), environmental, and economic dimensions of sustainability. For this, we review the nutritional quality, economic cost and environmental footprint of current national diets calculated by recent studies using several indicators. Next, we review the literature on how a shift in dietary patterns across different world regions can help achievement of SDG2 (Zero hunger), SDG3 (Good health and wellbeing), SDG 6 (Clean water and sanitation), SDG13 (Climate action), SDG14 (Life below water), and SDG15 (Life on land). Finally, we review studies on different policy or behavioral interventions that can help adoption of sustainable diets. We end the review by laying down the limitations of existing dietary sustainability studies and identify research or data gaps that need to be filled through future efforts.
Sustainability Status of Current Diets of Different World Regions
Current Dietary Patterns Vis-à-Vis Dietary Recommendations
Early work on proposing the healthy eating principles and recommended intakes of different food groups were carried out by World Health Organization (WHO) (WHO, 2003, 2020; WHO and FAO, 2004) and other institutions (e.g., Harvard's Healthy Eating Plate) based on the link between food intake and the risk of undernutrition, premature mortality from diet-related non-communicable diseases (NCDs) and obesity. An increasing number of countries have since then established the national food-based dietary guidelines (FBDGs) that fit the country-specific food availability, dietary habits, and nutrition concerns (WHO, 1998).
However, it is possible that a healthy diet has huge environmental impacts and thus its supply would not be sustainable in long term. Reflecting this need to consider the global environmental targets such as planetary boundaries (Steffen et al., 2015) or those agreed upon by several countries in the Paris climate deal (United Nations Framework Convention on Climate Change (UNFCCC), 2015; Rockström et al., 2017), recent guidance on sustainable diets is also emphasizing the need to add environmental sustainability criteria in addition to the nutrition, thereby promoting foods that are not only nutrient dense but also low in their environmental footprints (Behrens et al., 2017; Blackstone et al., 2018; Ritchie et al., 2018; FAO and WHO, 2019; Herforth et al., 2019; Willett et al., 2019; Kesse-Guyot et al., 2020; Springmann et al., 2020).
The reference diet proposed by the EAT-Lancet commission is one such attempt as it considers the dietary risk factors, nutrient adequacy and five environmental planetary boundaries (Willett et al., 2019). Their reference diet provides the mean value along with a range for the intake amounts of 16 food groups. Other global studies have derived country-specific sustainable diets using mathematical optimization algorithms that meet the nutrition, environmental and cultural acceptability constraints (Chaudhary and Krishna, 2019). Certain authors have also derived optimal diets at the national level considering nutrition, environment as well as the economic cost of daily diet in local food market (Gephart et al., 2016; Perignon et al., 2019; Abejón et al., 2020; Eini-Zinab et al., 2020).
Apart from health, nutrition and environmental dimensions, there have been calls to further improve the national dietary guidelines taking into account additional factors such as affordability of food items, subnational differences in food habits, cultural diversities, and income inequality alongside other indicators of food system sustainability to achieve more realistic and feasible dietary transformations (Chaudhary et al., 2018a; He et al., 2018; Batlle-Bayer et al., 2020; Blackstone and Conrad, 2020; Esteve-Llorens et al., 2020; Lassen et al., 2020; Moberg et al., 2020; Raghunathan et al., 2020; Sharma et al., 2020).
A comparison of the current intake of different food items or groups in different countries with the recommended intake levels proposed by dietary guidelines has revealed that a global average daily diet is low in fruits, non-starchy vegetables, legumes, nuts, and seeds, while high in red and processed meat, added sugar and roots and tubers (Afshin et al., 2019; Willett et al., 2019). Figure 1 shows the geographic variability in diets across the world compiled by comparing the current intake levels of major food groups with their EAT-Lancet recommended levels.
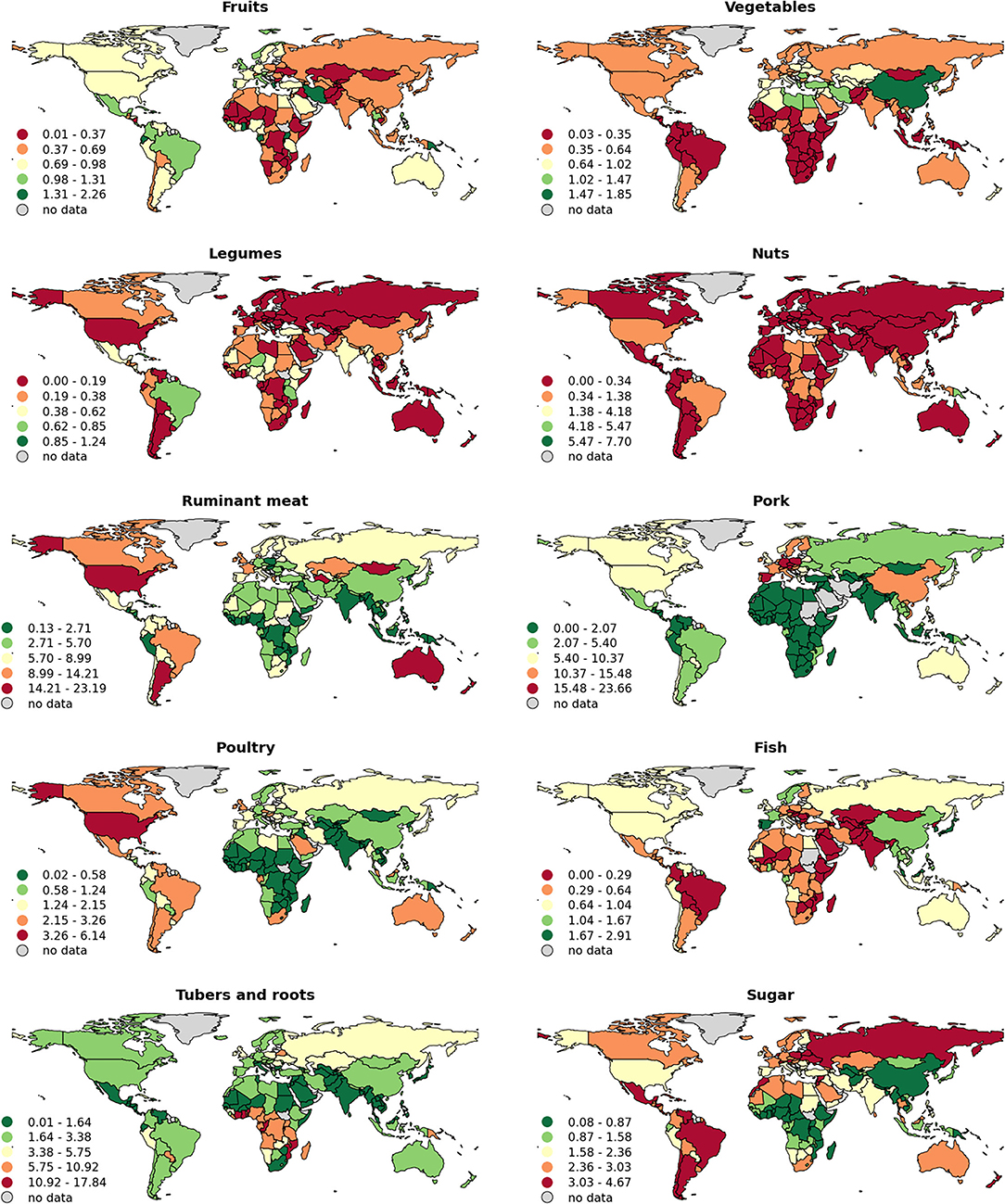
Figure 1. Diets in different countries relative to the EAT-Lancet reference diet. Ratio of current to EAT-Lancet recommended intakes of different food groups per country are shown. We adopted the national average intake estimates (year 2010) from Willett et al. (2019), and the references proposed by EAT-Lancet Commission for the major food groups to calculate the ratios of current to reference intake levels. The darker red color indicates an insufficient intake of sustainable healthy foods or an excessive intake of unsustainable unhealthy foods in the national average diet.
The diet in the low-income regions like Sub-Saharan Africa is primarily composed of cereals, roots and tubers which contain mainly carbohydrates, while the diets in high-income countries source energy primarily from non-staple foods with high quantities of animal-based products (e.g., meat and dairy), sugar and fats (Chaudhary et al., 2018a; Chaudhary and Krishna, 2019; FAO et al., 2020).
Only 55% of the world population has average fruit and vegetable availability above the recommended level of 400 g capita-1 day-1 (Mason-D'Croz et al., 2019a; WHO, 2020). On average, the current global intake of fruits and vegetables is around 57% of the recommended amount and ranges from around 25% in several South Asian and Sub-Saharan African countries to around 95% in certain Mediterranean, Middle East and North African countries such as Armenia, Turkey, Tunisia, Romania, Egypt, and Iran (Afshin et al., 2019; Mason-D'Croz et al., 2019a; Willett et al., 2019; Springmann et al., 2020; Figure 1).
As one of the major plant-based protein sources, the reference diet from EAT-Lancet Commission report proposed an average 75 g per capita daily intake of dry beans, lentils, peas, and soy. Current legume consumption globally is remarkably insufficient compared to the recommended amount. For legume products, Sub-Saharan Africa, Latin America and Caribbean, and India have highest per capita consumption, while Europe and Central Asia has the lowest intake. The average daily consumption of roots and tubers is above the level in EAT-Lancet diet across all regions, with the highest intake in Sub-Saharan Africa and the lowest in South Asia. Current intakes of nuts and seeds are substantially below the recommended levels across all geographical regions (Figure 1).
Globally, the current livestock meat intake is higher than the recommended levels except in South Asia and Sub-Saharan Africa. For red meat, per capita average intake is highest in North America, followed by Europe and Central Asia, China, and Latin America and Caribbean. Mongolia, Argentina, Australia, Turkmenistan, and United States are the top consumers of ruminant meat (beef and lamb) with the highest per capita values. High-intake regions like North America have 15 times more per capita intake than low-intake regions like South Asia (Figure 1). North America also has the highest per capita consumption of dairy products, with around seven times the daily dairy intake of people in Sub-Saharan Africa and East Asia and Pacific. Europe and Central Asia has the second largest dairy intake.
Nutritional Quality of Current National Diets
SDG2 (zero hunger) and SDG3 (Good health and wellbeing) underscore the importance of the nutritional quality of diet for a healthy life. Apart from calories, human body needs several essential nutrients to support the body functions (Fern et al., 2015) including macronutrients such as protein, fiber and poly-unsaturated fatty acids (PUFA); minerals (iron, potassium, phosphorus, calcium, zinc, copper, magnesium, manganese, selenium) and many vitamins. The daily per capita recommended intake levels of essential nutrients for different age groups is available from agencies such as WHO or the U.S. Institute of Medicine (WHO, 2003; Institute of Medicine, 2005). Then there are nutrients of health concern such as saturated fats, sugar, sodium, cholesterol, whose intake above a certain level is associated with negative impacts on the body functioning (Fern et al., 2015).
Many studies have linked the nutrient density (amounts per gram) of individual food items with their daily per capita intake amounts to calculate the current intake levels of nutrients in different countries and compared it with the recommended intake levels (Chaudhary et al., 2018a; Springmann et al., 2018b; Chaudhary and Krishna, 2019). Figure 2 shows the comparison of current and recommended intake levels of selected nutrients with high deficiency prevalence in different regions of the world.
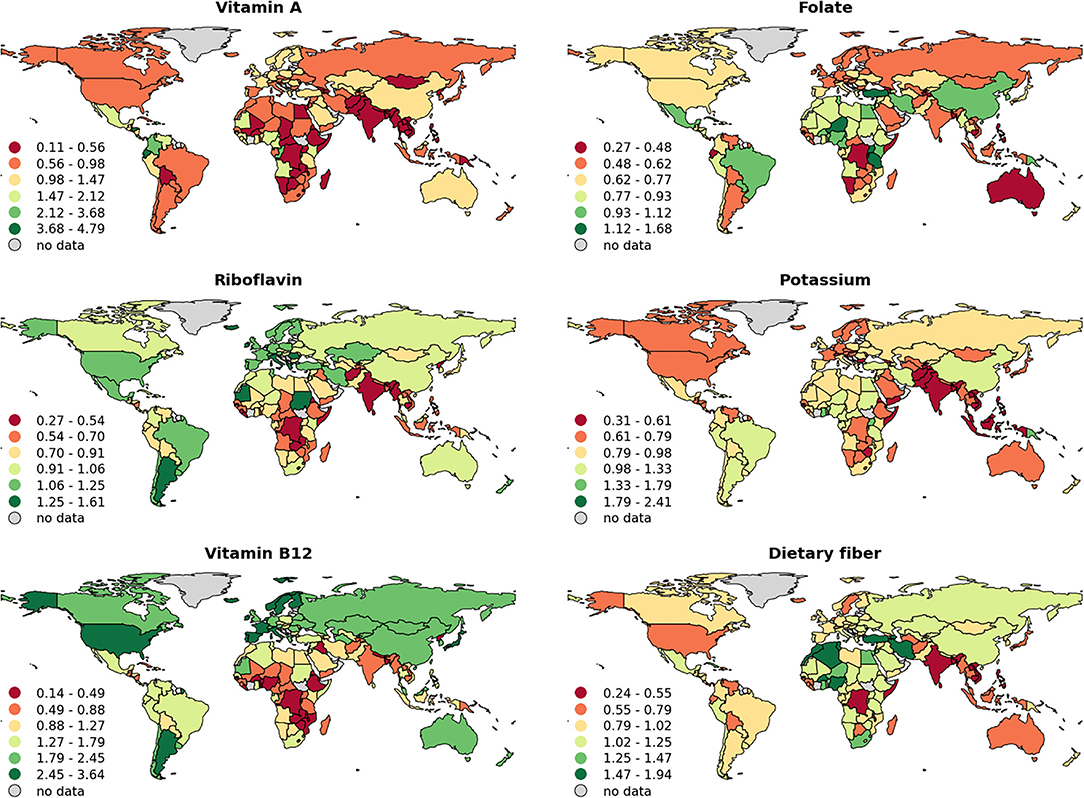
Figure 2. Ratio of current dietary supply to recommended intake for selected nutrients. Recommendations are population level average Reference Nutrient Intake (RNI) per capita per nutrient for each country which are calculated following the methods in Chen et al. (2020). Current intake levels (year 2010) were adopted from Springmann et al. (2018b).
Low intakes of dietary fiber, iron, potassium, vitamin A, folate, vitamin E, riboflavin (vitamin B2), vitamin B12, choline, and vitamin K are most prevalent globally (Beal et al., 2017; Springmann et al., 2018b; Chaudhary and Krishna, 2019).
The diets in the western countries are high in calories, low in several essential micronutrients and very high in nutrients of health concern (cholesterol, total fat, sugar, saturated fat). Diets in Africa and Asia are low in several essential micronutrients but also low in nutrients of health concern. A few countries in Sub-Saharan Africa here have diets low in calories as well.
The global average intake of the calories and the three essential macronutrients (protein, fiber, and polyunsaturated fatty acids) is much closer to their respective recommended levels compared with the intake of essential vitamins and minerals which is rarely adequate. Sub-Saharan Africa and India have the lowest average intake of protein while the intake in North America, Europe, China, and Latin America and Caribbean is way high and surpasses their mean population-level requirements. The intake of dietary fiber is most inadequate in South Asia and East Asia and Pacific. Across geographic regions, the intake of polyunsaturated fatty acids (PUFA) is most inadequate in South Asian countries, followed by Sub-Saharan Africa and East Asia and Pacific. United States and Israel have the highest national average PUFA intakes, twice the amount of their recommended levels.
Regarding vitamins, globally, the population-weighted average intakes are below their requirements for folate, riboflavin, choline, vitamin B12, vitamin K, vitamin E and vitamin A (Chaudhary and Krishna, 2019). The average folate intake is inadequate (ratio < 1) across all regions except China. Rwanda, Cuba, and Burundi have the highest national dietary folate intake while Somalia, Guinea-Bissau, and Sri Lanka are estimated to be most inadequate in their folate intake (Figure 2). Riboflavin (or vitamin B2) in current diets is lower than the recommended amounts for India, South Asia, Sub-Saharan Africa, East Asia and Pacific, and Middle East and North Africa. Democratic Republic of the Congo, India, and Sri Lanka have the lowest riboflavin content in their national average diets, accounting for <40% of their required amounts (Figure 2).
The average vitamin A intake is inadequate in South Asia, India, East Asia and Pacific, Middle East and North Africa, and North America. Diets in South Asia and India contain the lowest vitamin A level and their dietary vitamin A only fulfill 40% of the daily requirement (Figure 2). Indian diets are also the most inadequate in vitamin B12 which is only found in animal-based foods, followed by diets in Sub-Saharan Africa and South Asia (Figure 2). The vitamin C requirements are met by current average diets of all regions except in South Asia. Diets in most world countries (86%) contain less Choline than the reference of 550 mg per capita per day, and people in South Asia and Sub-Saharan Africa have lowest intake level (Chaudhary and Krishna, 2019). Vitamin K in the average diet of Sub-Saharan Africa is the lowest, followed by South Asia and India, barely reaching two third of the required level (Chaudhary and Krishna, 2019). Vitamin E intake on average is inadequate in South Asia, India, Latin America and Caribbean, Sub-Saharan Africa, East Asia and Pacific, and China (Chaudhary and Krishna, 2019).
Regarding minerals, average intake of potassium is lower than the daily recommendation in nearly every region except China that just meets the level. Latin America and Caribbean and Sub-Saharan Africa have relatively higher potassium intakes than other regions. The estimates for year 2011 show that 90% of the global population who were at risk of calcium and zinc deficiency due to insufficient food supply lived in Africa and Asia (Kumssa et al., 2015). Dietary calcium is highest among North America and Europe and Central Asia, while lowest for countries in Sub-Saharan Africa and East Asia and Pacific where the current diets do not meet its recommended levels.
Regarding the intake of nutrients of health concern, the intake of saturated fatty acids exceeds the maximum allowable limit in North America, Europe and Central Asia, China, Latin America and Caribbean. The global consumption of sodium is far higher than the optimal level in many regions. China has the highest sodium intake of 10.7 g per capita per day, followed by East Asia and Pacific countries such as Singapore, Brunei Darussalam, and Japan (Afshin et al., 2019). The global average intake of sugar far exceeds the EAT-Lancet recommended level and the energy-dense and nutrient-poor food products like sugar-sweetened beverages are excessively consumed globally especially in North America and Latin America and Caribbean (Afshin et al., 2019).
Environmental Footprint of Current Diets
Food or feed production for ultimate human consumption causes massive environmental damage in the form of greenhouse gas emissions, eutrophication, water scarcity, land utilization, biodiversity loss etc., thereby hampering the achievement of multiple SDGs (Willett et al., 2019). Globally, food systems contribute to around one third of global GHG anthropogenic emissions from sources including land-use change (e.g., due to conversion of forests into farmland/pasture), the enteric fermentation of ruminants, fertilizer application, and energy use (Vermeulen et al., 2012; Crippa et al., 2021; Hong et al., 2021). Food production utilizes ~40% of global ice-free land surface (Foley et al., 2011), which not only weakens the carbon sinks but also undermines the natural habitats of species and ecosystem intactness (Kastner et al., 2021).
Global food systems in 2010 are responsible for ~5.2 billion tons of carbon dioxide equivalent direct GHG emissions (referring to methane and nitrous oxide), 12.6 million km2 of cropland use, 1,810 km3 freshwater use, 104 teragrams nitrogen and 18 teragrams phosphorus fertilizer application (Springmann et al., 2018a). Among these five environmental impact domains with quantitative planetary boundary estimates for global food systems (Springmann et al., 2018a; Chaudhary and Krishna, 2019), boundaries for GHG emission and nitrogen fertilizer use are more difficult to meet with around 40% of all countries having their average diet-related environmental footprints exceeding the planetary boundaries (Chaudhary and Krishna, 2021). The planetary boundaries reflect the maximum allowable environmental emissions/limits, transgression of which would increase the risk of Earth system at risk of unwanted outcomes due to ecosystem destabilization, jeopardizing human livelihood including the food systems (Steffen et al., 2015).
Figure 3 shows the heterogeneity of environmental impacts linked with the average daily per capita diets of different countries. Sub-Saharan Africa has the lowest per capita food-related environmental impacts on average (Figure 3). Regarding food-related carbon footprints, most regional average diets are above or near the planetary boundary except Middle East and North Africa, and India. Latin America and Caribbean has the largest carbon footprints embedded in their average dietary pattern. Per capita current diets in Uruguay, Montenegro, New Zealand, Serbia, and Australia are associated with the highest food-production related carbon footprints (>11 kg CO2 eq. per capita) (Springmann et al., 2020) which might be related to the consumption of food products such as high-emission ruminant meat (Poore and Nemecek, 2018).
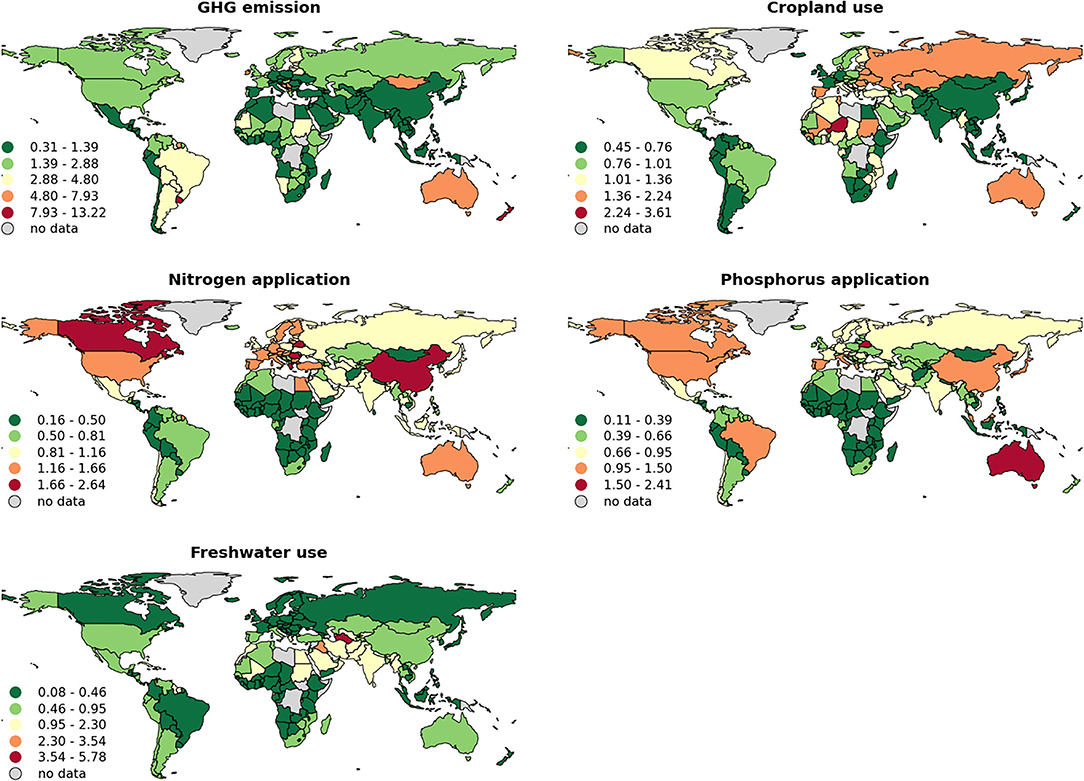
Figure 3. Ratio of the per capita environmental footprints associated with current diets to food-related planetary boundaries. Environmental footprints regarding greenhouse-gas (GHG) emissions, cropland use, freshwater demand, nitrogen, and phosphorus application of national food consumption in year 2010 were estimated by Springmann et al. (2020). Per capita food-related planetary boundaries of global average were based on the estimates of Chaudhary and Krishna (2019) and Springmann et al. (2018a).
Regarding nitrogen and phosphorus fertilizer application, the environmental footprints of national average diet are largest in China, followed by North America (Springmann et al., 2020). The associated impacts are driven by the inefficient fertilizer use and manure management related to the production of staples and livestock products.
Regarding cropland, Europe and Central Asia has the highest footprint per capita, followed by Sub-Saharan Africa and North America. Per capita cropland footprints are high for countries such as Niger, Kazakhstan, Australia, Russian, and Tunisia that source foods from low-yield crops and animals that rely on large amounts of crop feeds (Figure 3). The extensive grazing land demand for livestock-sourced foods contribute to high diet-related land footprints of countries such as Mongolia and Namibia (Chaudhary et al., 2018a).
Regarding freshwater, diets are associated with the highest footprints in South Asia, Middle East and North Africa, and India (Figure 3) where the water use is not efficient especially for products like rice, legumes, and nuts.
Compared to carbon, land, water, and fertilizer use, the impact of national food consumption on biodiversity has been less often assessed in the previous studies. The encroachment of species' natural habitat due to agricultural land use and the pollution from nutrient runoffs into water bodies greatly undermines the terrestrial and aquatic biodiversity of the region and threatens the species with extinction risk. The EAT-Lancet report estimated that the current agriculture driven biodiversity loss rate has already violated the planetary boundary of species extinction rate (Willett et al., 2019).
Other studies have calculated the food production, consumption and trade related national biodiversity footprints and found that high diet-related biodiversity footprint countries are either the ones that have high species richness density, natural habitat conversion and small share of imported food products (such as tropical Central America and Caribbean countries), or the regions with large per capita food consumption (such as North America and European Union) and high dependence on products imported from tropical biodiversity hotspots (Lenzen et al., 2012; Chaudhary and Brooks, 2017; Chaudhary et al., 2018a; Estrada et al., 2019).
Exports from Indonesia, Thailand, India, Australia, and Malaysia embody high biodiversity losses, meanwhile countries such as United States, China, Japan, and Germany imported large quantities of products that threaten the species in the country of production (Chaudhary and Kastner, 2016). Staple crops, sugarcane, palm oil, coconut, and cassava are responsible for the most global cropland use induced biodiversity impact, especially those produced in Southeast Asia (Chaudhary and Kastner, 2016).
Economic Cost of Current Diets
The local food market price is a key determiner of the dietary choices, especially for poor people with a limited income. The affordability of healthy food items for people with low socioeconomic status is important to ensure food security and nutritional health (Darmon and Drewnowski, 2015). The price of foods and the income per household member often together determine whether people have economic access to the food that meet their nutritional needs and dietary preferences. For example, the low intake of fruit and vegetables in low-income countries was found to be associated with their high relative cost in the market (Miller et al., 2016).
To estimate the dietary cost, earlier studies sourced food price or consumer expenditure data from national statistical agencies, retailer records or consumer survey (Wilson et al., 2013; Donati et al., 2016; Chen et al., 2019; Reynolds et al., 2019). More recent global-level studies adopted the nationally representative retail prices of 680 standard food products across 170 countries from the World Bank's International Comparison Program (ICP) (Headey and Alderman, 2019; Hirvonen et al., 2019; Herforth et al., 2020). Using this dataset, United Nations Food and Agriculture Organization recently found that the diets satisfying the daily caloric requirements through cheapest available staple foods are affordable for almost all people in most countries except Sub-Saharan Africa where 15% of population cannot afford even this calorie sufficient diet (Figure 4).
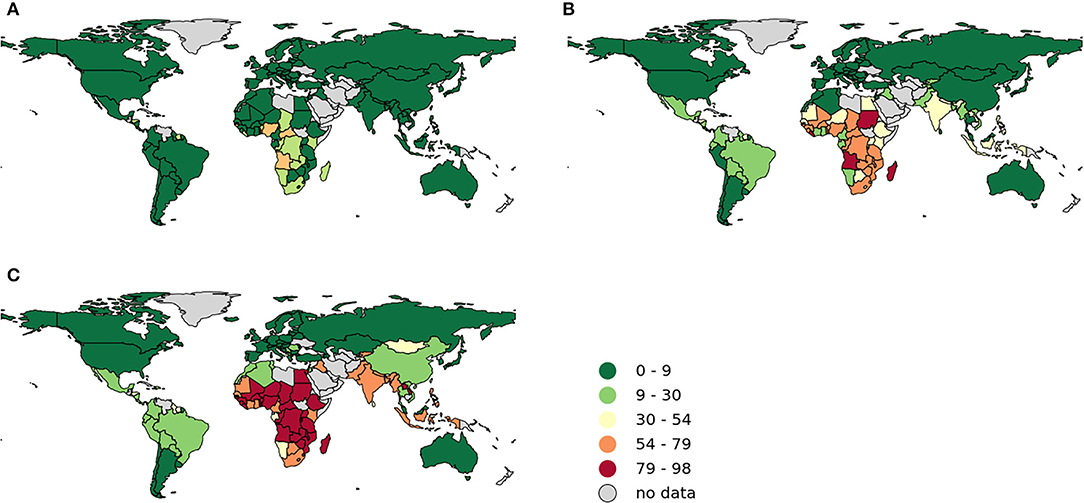
Figure 4. Affordability of (A) energy sufficient diet, (B) nutrient sufficient diet, and (C) healthy diets. Higher values (in red) indicate the food is too expensive and not affordable to local population. The affordability is measured by the percentage of people in each country whose household income cannot afford the cost of the cheapest diets using locally available items, assuming they can spend no more than 63% of their income on food. For a given country, energy sufficient diet provides enough calories and is based on the least-cost staple foods, nutrient sufficient diet provides not only sufficient calories but also adequate amounts of essential nutrients, and healthy diets meet the national food-based dietary guidelines (FBDGs). Values were obtained from Herforth et al. (2020).
However, a nutrient adequate daily diet is rather expensive for many regions as over 60% of population in Sub-Saharan African and 12–21% of people living in Latin America and Caribbean, South Asia, East Asia and Pacific, and Middle East and North Africa cannot afford the least-cost diets which are adequate in terms of 23 macro- and micronutrients (Figure 4). Countries like Sudan, Madagascar, and Liberia would encounter the greatest affordability barrier where more than 85% of national population is unable to afford the food products for fulfilling their daily nutritional requirements (Figure 4).
Achieving the culturally favored healthy diets which follow the national dietary guidelines is even more challenging. Three billion people globally cannot afford such a diet. These recommended diets are often 60% more costly than diets that fulfill the nutritional adequacy and have nearly five times the cost of diets that just provide sufficient calories with cheap starchy staple foods (Figure 4; Herforth et al., 2020). These healthy diets are unaffordable for almost 60% of people in South Asia and sub-Saharan Africa, over 45% of people in South-east Asia and over 20% of population in Latin America (Figure 4). In contrast, the cost of healthy diets account for a small fraction of per capita household income in high income regions such as Europe and Central Asia and North America where just 1–3% of people cannot afford it (Figure 4).
The high economic cost not only creates the barrier for the healthy eating but also hinders the possibility of meeting food related environmental sustainability targets. For example, the reference diet proposed by EAT-Lancet Commission which accounts for both human health and environmental sustainability, is found to be not affordable in many countries. For low-income countries, the mean daily cost of an EAT-Lancet reference diet is estimated to be $2.43 (in 2011 international dollars) accounting for around 89% of the average daily household income per capita (Hirvonen et al., 2019). The cost of such diets would account for the smallest fraction (4.4.%) of average per capita household income for people in North America and the largest portion (73%) for people living in sub-Saharan Africa (Hirvonen et al., 2019). Across food groups, fruits, and vegetables together account for the largest share of dietary cost on average globally. The share of animal sourced foods (i.e., meat, fish, eggs, and dairy) in total cost is highest in low-income countries at 33%.
Risks of unaffordability in terms of having a healthy diet are distributed unevenly among subgroups with socio-economic discrepancy. Even in affluent regions like the European urban area, the low-income households would have financial difficulties accessing the healthy diets complied the national dietary guidelines (Penne and Goedemé, 2020). The case study on dietary changes in India shows that approximately two-thirds of the rural Indian population could not afford a recommended healthy diet in 2011, despite the rapid average income growth in the past two decades (Raghunathan et al., 2020). High price of nutrient dense food items creates the financial barrier to shifting toward recommended healthy dietary pattern. People would need to spend an additional 2.4 US dollars for a diet that in line with the EAT-Lancet references in India, primarily to incorporate increased fruits and dairy products in daily diets (Gupta et al., 2021).
Besides the consumer's food purchase ability, another indicator, the Food Affordability Index considers the vulnerability to the food price shocks into the affordability assessment, indicating that Venezuela, Syria, Madagascar and sub-Saharan countries were most vulnerable to the supply of healthy foods and had the lowest food affordability in 2019 (The Economic Intelligence Unit, 2020).
For many regions and subpopulations, the current cost of healthy diets and nutritious foods is too high in comparison to the household income levels. The unaffordability could be a significant barrier for adopting the healthier and more sustainable diets unless sectors across food systems (e.g., production, trade, and market resilience) can facilitate the supply of the healthy foods such as vegetables, fruits, nuts, and dairy at lower prices (Hirvonen et al., 2019; Bai et al., 2020). For example, most of the cost of healthy diets can be attributed to two types of foods: protein-rich foods and fruits and vegetables accounting for 44 and 40% of the total cost, respectively (Herforth et al., 2020). Financial interventions that target the source of the issue of unaffordability of healthy food items in a country and reduce the price barriers to access of these food commodities could help improve the economic access to healthy diets, especially for the low income and vulnerable population like children and the elderly (Rao et al., 2013; Hirvonen et al., 2019; Vandevijvere et al., 2020).
Dietary Change and Sustainable Development Goals (SDGs)
Dietary Change and SDG2—Zero Hunger
The SDG 2 aims to end hunger and food insecurity (target 2.1) and all forms of malnutrition (target 2.2) by 2030. This means that not only can people have enough dietary calorie intake but also the essential nutrients like protein, fiber, unsaturated fatty acids, vitamins, and minerals. According to the estimates of year 2019, 690 million people global still suffer from hunger meaning their daily diets do not provide sufficient calories. Over two billion people (26% of the global population) suffer from malnutrition meaning that they do not have enough safe, sufficient and nutritious food available (FAO et al., 2020). The number of hungry people has been slowly rising since 2014 despite worldwide commitment and economic progress. The world is not on track to achieve the global nutrition and SDG 2 targets by 2030 if the trends of the last 10 years continue. Today's food systems still face the challenge from the burden of malnutrition shown in forms of undernutrition, micronutrient deficiency, and overnutrition (Popkin et al., 2020). It is clear that dietary change is needed in each country to achieve progress toward global sustainable development goals (SDGs).
Several global and regional studies have presented the required increase or decrease in the intake of individual food items or food groups necessary to achieve the daily nutritional needs for different countries. This includes the EAT-Lancet commissions' report and other studies that have employed optimization algorithm to derive a sustainable diet fulfilling daily nutritional requirements of all essential nutrients (Chaudhary and Krishna, 2019, 2021). They found that the malnutrition reduction effects from adopting healthier diets would entail different strategies and pathways.
For undernourished regions like sub-Saharan Africa and South Asia, the dietary shifts toward an increase in dietary energy and increased intake of almost all food items but especially nutrient dense animal-based products and fruits and vegetables would decrease the prevalence of hunger, undernutrition, and deficiency in essential nutrients. Replacing cereals and root products with diverse non-staple foods could reduce the risk of dietary nutrient deficiencies in Africa for micronutrients such as calcium, zinc, selenium, and iron that are associated with 20–50% inadequate intake among population currently (Joy et al., 2014; Gregory et al., 2017). A moderate intake of nutrient-rich fish or livestock products that are produced respecting the local environmental boundaries would help tackle the hidden hunger issue in undernourished regions (Beal et al., 2017; Chaudhary and Krishna, 2019). For example, the nutrient gaps (deficiency risk >20%) of calcium, iron, zinc, and vitamin A among coastal population in countries like Namibia, Mauritania and Kiribati could be resolved by the fish products (Hicks et al., 2019). To address the iron deficiency and associated anemia, a diet with iron-rich and nutrient-dense foods like lentils, pulses (Chaudhary et al., 2018b; Chaudhary and Tremorin, 2020) or animal-sourced foods is important to ensure the dietary quality and alleviate adverse health outcomes for children and women (Black et al., 2008). Although caution must be exercised as concerns have been reported by some studies on the low iron bioavailability of plant-sourced foods (Haider et al., 2018). Note that increased intake of food in the undernourished regions is likely to increase their environmental footprint as well as expenditure on daily diets (Chaudhary and Krishna, 2021).
For regions with widely sufficient food calorie intakes such as North America, Europe, and Australasia, malnutrition in the form of obesity and diet-related non-communicable disease prevalence have become an increasing concern (Cooper et al., 2019; Swinburn et al., 2019; Hawkes et al., 2020). Here the benefits would result from a restrain of excessive calorie and unhealthy nutrients such as added sugar and saturated fats that are consumed through discretionary products (Hadjikakou, 2017). An increased intake of fruits and vegetables (e.g., 250 g per capita per day more in North America), legumes, and roots, and a substantial reduction in meat, dairy, and eggs are expected to ensure the nutritional and environmental targets are met (Chaudhary and Krishna, 2019).
Adopting an energy-balance and nutrient-adequate diet are crucial to address the increasing coexistence of undernutrition and overnutrition (overweight and obesity) for countries undergoing rapid income growth and food environment transition such as Indonesia and many other Asian and sub-Saharan African countries (Popkin et al., 2020).
It is therefore important that governments pursue SDG2 without ignoring environmental impacts in order to avoid the potential trade-offs between global hunger (as well as “hidden hunger”) eradication and environment-related SDGs (e.g., climate action, preserving life on land and water). Dietary change is often proposed as one indispensable strategy for feeding the projected future population (SDG 2) and achieving the climate change target (e.g., 1.5°C increase limit) simultaneously (Bajželj et al., 2014; Chaudhary et al., 2018a; Clark et al., 2020). Ensuring the availability of culturally-acceptable, low-cost, nutrient dense and low environmental footprint food items is the major challenge ahead for countries in timely achievement of SDG2.
Dietary Change and SDG3—Good Health and Wellbeing
Poor dietary quality is closely associated with the global burden of non-communicable diseases (NCDs) such as cardiovascular diseases, type 2 diabetes, and neoplasms. Globally, 11 million deaths and 255 million disability-adjusted life years (DALYs) were attributable to dietary risk factors including high intake of sodium, low intake of whole grains and fruits (Afshin et al., 2019).
Improving diets can help achieve the SDG 3 that nourish healthy lives. A growing number of studies have revealed the association between the reduced disease risks and the optimal intakes of whole grains, fruits, vegetables, legumes, nuts and seeds, red and processed meat consumption through indicators such as mortality and DALYs (Afshin et al., 2014; Li et al., 2014; Tilman and Clark, 2014; Wang et al., 2014, 2019; Aune et al., 2016; Mayhew et al., 2016; Springmann et al., 2016; Milner et al., 2017; Miller et al., 2020). Studies also found that the adherence to an alternative dietary pattern such as Mediterranean and Vegetarian diets is associated a lower risk of multiple chronic diseases (Orlich et al., 2013; Satija et al., 2016; Dinu et al., 2018). These evidence highlights the potential health benefits from shifting to an energy-balanced diet containing larger portions of plant-based and less-processed food products.
If the global population shifted from current diets toward the dietary pattern that EAT-Lancet Commission proposed, 10–12 million premature deaths due to NCDs could be prevented annually, meaning a 19–24% premature mortality reduction (Table 1; Willett et al., 2019). The mortality reduction from the recommended dietary shifts is projected to be distributed relatively evenly across regions, with slightly larger benefits in Europe and Central Asia, Middle East and North Africa, Latin America and Caribbean, and upper-middle-income countries (Springmann et al., 2018b).
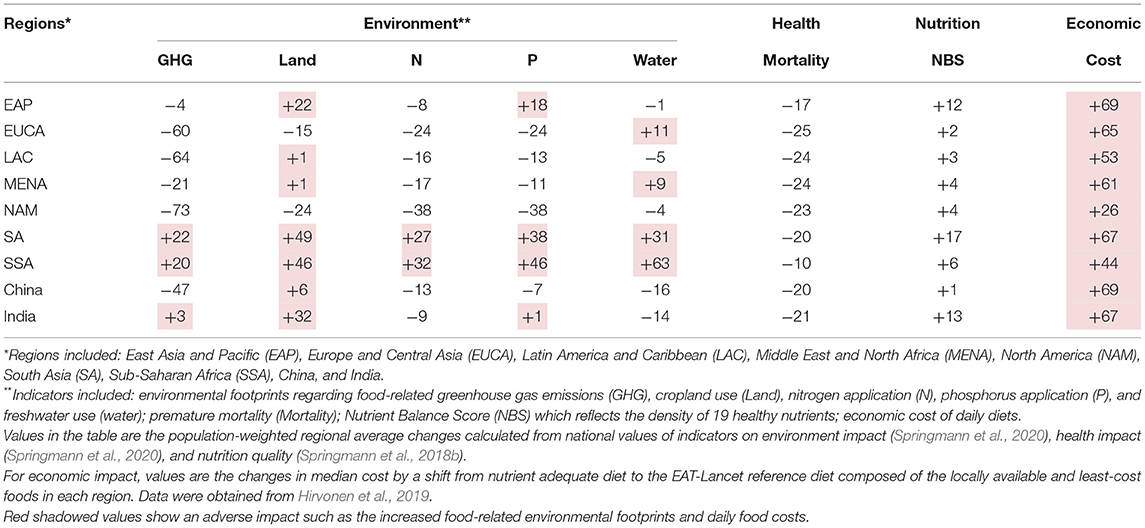
Table 1. Region-specific changes (%) in multiple indicators of sustainability resulting from a transition toward the EAT-Lancet reference diet.
For regions whose current diets are composed of abundant unhealthy foods, adopting the diets that are lower in the meat amounts and filling the gaps for fruits, vegetables, legumes, and nuts (e.g., EAT-Lancet, vegan, vegetarian, pescatarian diets) is projected to bring great population health benefits, with a 23–8% reduction in premature mortality in Europe and Central Asia, North America, Latin and Caribbean, and Middle East and North Africa. Such health benefit estimates are in alignment with the SDG 3 target 3.4 which aims to reduce one third of premature mortality from NCDs through prevention and treatment by 2030 (United Nations, 2021). Shifting to the diets that follow the national food-based dietary guidelines would lead to a reduction in premature mortality from diet-related NCDs as well but with smaller effects comparing to the extensive plant-based options (Springmann et al., 2020).
Indicators that monitor the progress for SDG 2 are also relevant for the mortality rate of maternal, neonatal and children under five (SDG 3). Maternal undernutrition contributes to fetal growth restriction thus increasing the risk of neonatal deaths. Maternal and child undernutrition is attributed to 35% of child deaths and more than 10% of total global disease burden (Black et al., 2008). Ensuring nutrient-adequate and balanced food intake is one of the crucial interventions for lowering disease risks and support children to reach their development potential.
In less-affluent regions such as sub-Sahara Africa and South Asia, shifting from current staple-based dietary patterns toward diets composed of a variety of nutritious food products could improve the energy balance and nutrient adequacy, leading to a reduction in undernutrition-related mortality.
Previous assessments of dietary changes often considered the long-term disease consequences for adults aged 20 year or older (e.g., the reduced premature mortality attributed to cardiovascular diseases) (Springmann et al., 2018b), however, did not explicitly address the burden mitigation effect related to macronutrient and micronutrient deficiency for vulnerable groups such as maternal and children under 5 years old (Gödecke et al., 2018; Willett et al., 2019; Zagmutt et al., 2020). Such effect interconnects with SDG 2 agenda and would have important contribution to the missions of SDG 3 especially for regions with large insufficiency in healthy foods and nutrient consumption.
Dietary Change and SDG 6—Clean Water and Sanitation
A recent review provides a critical examination of SDG 6 and its indicators (Germann and Langergraber, 2022). Current food systems are the largest consumer of global freshwater resources and to achieve the water scarcity reduction (target 6.4 of the SDG 6), there is need to improve their water use efficiency. A transition toward diets composed of foods that demand less water for production could help reduce freshwater use stress and avoid violating the environmental flow requirements for healthy aquatic ecosystems (Jägermeyr, 2020).
Lowering the fertilizer footprints through dietary shifts could also contribute to the achievement of SDG 6 (e.g., target 6.3), because the nutrient runoffs from fertilizer application in agricultural farms is among the leading polluters of rivers and lakes that jeopardizes the supply of safe drinking water. This impact will be discussed in the section on SDG 14 “life below water” as the nutrient pollution effect is linked with biodiversity agenda as well. In this section we will focus on the water scarcity reduction target within SDG 6.
Earlier studies at the continental scale have reported substantially high food-related water footprint reduction (by 20% or more) through a shift toward healthy plant-based options mainly attributed to a large decrease in water-intensive meat products (Vanham et al., 2013; Aleksandrowicz et al., 2016). Water footprints of red meat and farmed fish are on average higher than plant-based substitutes (Mekonnen and Hoekstra, 2011).
However, global assessments like Jalava et al. (2014) have illustrated the limited water saving effect of shifting to healthier diets. A decline of 1% in global freshwater footprint (or blue water footprint) was observed when shifting from conventional diets to the WHO's recommendations on macronutrients, sugar, fruits, and vegetables (WHO, 2003) at the global level. Recent study also showed a modest effect on freshwater use reduction if global population shift toward the EAT-Lancet Commission recommended reference diet, with 1-9% lesser water demand (Willett et al., 2019). According to their model, the reduction in water demand for animal products and feed crops was compromised by the increased demand for healthy foods like nuts, fruits and vegetables, which are relatively water-intensive (Willett et al., 2019). The dietary change effects on freshwater use would vary across regions. For undernourished regions like Sub-Saharan Africa and South Asia, improving dietary quality would be associated with more irrigation water use through increased intake of foods such as fruits, vegetables, nuts, legumes, animal protein (Willett et al., 2019).
In this case, solutions that improve water-use efficiency of food systems are needed (Davis et al., 2016; Rosa et al., 2018; Vanham and Mekonnen, 2021) along with identification of items within each food group that have relatively lower water use than the other items. For example, pistachios and almonds would not be the sustainable options for filling the intake gaps of nuts due to their high water footprints at 7,602 and 3,816 m3/ton, respectively. In contrast, nuts with much lower freshwater demand like Kola nuts (26 m3/ton) and chestnuts (174 m3/ton) (Mekonnen and Hoekstra, 2011) might be better alternatives to meet daily recommended nuts intake amounts. Within animal-sourced foods, the low-food chain animal products such as bivalve mollusks, forage fish, and insects are associated with relatively low freshwater footprint and thus can act as sustainable alternatives to high food chain and high impact meat products such as beef or pork (Jalava et al., 2014; Kim et al., 2019).
Another issue is the origin of crop production as the water use for a particular crop and water scarcity varies widely across the globe. The freshwater footprint of rice production in Pakistan and Iran are highest among the rice producer countries, and one-kilogram of rice produced in India requires more freshwater than rice in other major producers like China, Indonesia, and Bangladesh (Kim et al., 2019). Dietary shifts from water-intensive rice toward more water-efficient whole grain choices like maize, millet, and sorghum could help India in progress toward SDG 6 by mitigating the existing water scarcity while satisfying the national nutrient intakes (Davis et al., 2018; Chaudhary and Krishna, 2021). The unsustainable water use driven by food trade is severe where the regional freshwater resources mismatch their production scale, thus call for more efficient trade to sustain the environmental flows (Dalin et al., 2014; Pastor et al., 2019; Rosa et al., 2019).
Other have demonstrated that a substantial fraction of fruits and vegetables imports of high-income temperate countries come from climate vulnerable and water scarce low-income countries (Scheelbeek et al., 2020). Here expansion of horticulture locally through novel agricultural systems in temperate water-abundant countries and dietary shifts toward more locally grown crops with lesser water use impacts and trade agreements with producer countries that seek to improve the water use efficiency of imported crops through technology transfer or other means can help progress toward SDG 6.
Dietary Change and SDG13—Climate Action
SDG 13 calls for urgent actions to combat climate change and the associated consequences. Growing number of studies have assessed the measures for cutting the greenhouse gas (GHG) emissions associated with food systems. Dietary change is an imperative measure for climate mitigation without sacrificing other sustainability domains (e.g., food security, biodiversity intactness) (Bajželj et al., 2014) as for most of world regions, GHG emissions associated with current per capita diet have transgressed the food-related carbon planetary boundary (Figure 3).
Diets with increasing replacement of emission-intensive foods with plant-based foods would be associated with a decrease in food related GHG emissions and can help in the achievement of SDG 13 and the commitments under Paris Agreement. As shown in Table 2, animal-sourced products in general have higher GHG emissions regardless of the unit used such as per weight, per serving, per calories, or per protein content among alternative food groups (Clune et al., 2017; Poore and Nemecek, 2018; Clark et al., 2019; Willett et al., 2019) and the ruminant meats in particular have emissions more than 20 folds larger than plant-based products per unit calories (Clark et al., 2019).
A median of 22% GHG emission reduction effect was reported across various meat substituted diets including Mediterranean pescatarian, vegetarian, and vegan diets and up to 80% reduction could be achieved by plant-based diets (Hallström et al., 2015; Aleksandrowicz et al., 2016; Springmann et al., 2018a; Broekema et al., 2020; Drew et al., 2020). Transition toward healthy diets in high income and food secure regions has also been shown to massively reduce their environmental footprint, thereby compensating for increased environmental footprint due to transition of regions with high hunger prevalence toward a nutritionally adequate diet (Hasegawa et al., 2018; Willett et al., 2019).
Efforts to reduce diet-related GHG emissions can also bring co-benefits for other SDGs because climate-change brings hazards and uncertainties to global food systems. Increased average temperature, change of water availability and salinity due to elevated carbon dioxide levels in the atmosphere, would have negative impacts on the crop yields (Challinor et al., 2014) and even nutrient content of food crops. For example, Myers et al. (2014) reported reduced levels of zinc, iron, and protein in cereal and legume products under elevated carbon dioxide conditions. Evidence on reduced yields of fruits, vegetables, and legumes due to the increased water scarcity and salinity driven by climate change has also been reported (Scheelbeek et al., 2018; Alae-Carew et al., 2020). Nutrition-sensitive food policy (SDG 2) should therefore take the climate change impacts into account to minimize its negative nutritional consequences.
Dietary Change and SDG14—Life Below Water
SDG 14 focuses on the conservation and sustainable use of oceans, sea, and marine resources. The overapplication of nitrogen and phosphorus fertilizer in agriculture production and the associated runoffs drive the nutrient pollution of aquatic systems, impeding the mission of SDG 14 due to the eutrophication in coastal zones (i.e., target 14.1).
Earlier studies have identified that the replacement of nitrogen-intensive beef to poultry and a reduced consumption of animal- products could lower the nitrogen requirements thus mitigating the associated pollution in ecosystems (Bouwman et al., 2013; Bodirsky et al., 2014). Eutrophication emission impacts on average are high for animal-sourced products and farmed crustaceans (e.g., 300 g PO4eq/kg for beef, 50 g PO4eq/kg for poultry meat) and low for plant-based foods such as fruits, maize, and cassava with emission <5 g PO4eq/kg (Poore and Nemecek, 2018). The eutrophication potential embedded in per capita food intakes would reduce by 10–20% in high-income countries if people follow the national dietary guidelines that generally recommend a large replacement of meat, dairy, sugars, and oils with other crop foods (Behrens et al., 2017). A shift from current diets to the EAT-Lancet reference diet is projected to decrease the nitrogen and phosphorus application needs by around 10% globally (Willett et al., 2019).
Across regions, North America will have the largest environmental savings with a 38% fertilizer application reduction if they transition from their current diet to EAT-Lancet reference diet, followed by Europe and Central Asia with one quarter reduction (Table 1). As with other environmental impact domains, the N and P footprint in Sub-Saharan Africa and South Asia would increase by 30% or more because it entails increased intake amounts of most foods. The dietary shifts in the rest of world regions would reduce around 7–17% fertilizer-associated impact, except the increase in P demand for East Asia and Pacific and India.
Dietary change to mitigate the nutrient-pollution induced eutrophication and acidification and protect the life under aquatic ecosystems (i.e., SDG 14), would complement other on-farm efforts to improve the nitrogen and phosphorus use efficiency of different foods (Metson et al., 2021) by increasing N conversion for livestock production (Lassaletta et al., 2016; Groenestein et al., 2019) and reducing nutrient overuse during the cereal cropping practices especially in hotspot regions like China (Mueller et al., 2012), or to establish vegetative buffers to prevent the run-off from polluting water bodies (Ramesh et al., 2021). In addition, cutting down the food related carbon footprints (SDG 13) through dietary change also interconnects with the targets of SDG 14 as the elevated atmospheric GHG levels drive the ocean acidification (target 14.3) that in turn negatively affects the marine life (Doney et al., 2009; Kroeker et al., 2013; Lade et al., 2019).
Food production and consumption affect the life below water in multiple ways. Fisheries and aquaculture support the provision of fish and shellfish products which are important sources of protein and high bioavailable nutrients like polyunsaturated fatty acids (Wallin et al., 2012; Zheng et al., 2012). The EAT-Lancet Commission recommends 28 grams of daily fish intake, yet the intake in current average diets is below this level across most regions except East Asia and Pacific (Willett et al., 2019). Thus, to close the existing nutrition gaps particularly in regions with high food insecurity, the consumption of fish and other edible aquatic animal products needs to increase across the globe through promotion of sustainable fisheries (Bogard et al., 2018; Hicks et al., 2019).
Currently, the fish sector in food systems is associated with ecosystem damage from overfishing and unsustainable aquaculture practices that result in damage to life under water (Diana, 2009; FAO et al., 2020). The indicator monitoring the SDG target 14.4 shows that fish stocks within biologically sustainable level have deteriorated from 90% of stocks in 1974 to 66% in 2017 and more than 30% have been overfished (FAO, 2020). Rapid expansion of aquaculture also contributes to the resource use and emission associated to fish meal production (Pahlow et al., 2015; Fry et al., 2016). Effective regulation on global fisheries is need of the hour that keeps the fish stocks within biologically sustainable levels and enables the sustainable expansion of aquaculture production to meet the SDG 14 targets.
Another issue to consider while increasing the intake of fish or seafood is the type of species or production systems supplying them because the environmental impacts of fish vary widely. Across fish and seafood species, mollusks from aquaculture can have beneficial impacts on ecosystem services (Naylor et al., 2021) with smallest emissions and energy use, while catfish aquaculture on average was found to be associated with larger environmental damages (Hilborn et al., 2018). Regarding production systems, the recirculating aquaculture and trawling fisheries have higher carbon footprints than other production methods due to fuel use, and fisheries involving bottom trawls have more ecosystem disruption on life under water (Clark and Tilman, 2017). Fish products from integrated agricultural-aquaculture system (e.g., rice-catfish) were found to be associated with relatively low environmental footprints (Clark and Tilman, 2017). Enhancing the supply chain technology including processing and packaging can help improve the environmental, nutritional, economic impacts of extensively integrating fish and seafood resources into diets (Bogard et al., 2018).
Dietary Change and SDG15—Life on Land
Notwithstanding the growing efforts, only one-third of countries are on track to achieve their national biodiversity targets (https://unstats.un.org/sdgs/report/2020/goal-15/). Agriculture is the largest driver of deforestation and land use change and occupies 40% of the global land surface (Foley et al., 2011). The expansion of agricultural land since twentieth century mainly occurred in biodiversity-rich tropical regions while the land area for food production in temperate regions shrunk (Willett et al., 2019; Estrada et al., 2020). As a result, the food-related land use is associated with the destruction or degradation of the natural habitats of many species driving them to the brink of extinction and impeding the progress toward the SDG 15 targets 15.1, 15.2, and 15.5.
Leclère et al. (2020) showed that adjusting dietary choices toward a lower portion of animal-based foods could reduce the pressure on the land for food production while satisfying the dietary energy and nutritional requirements for the growing human population. Reflecting the biodiversity hotspots, a shift toward more plant-rich diets could lower the extinction risks for various mammal and bird species of the tropical South America and the southeast Asia, India, and China (Tilman et al., 2017).
Several studies have shown that per serving of cereals, vegetables, and fruits require less cropland rather than livestock products and thus transitioning from animal-based to plant-based diet can reduce the demand for natural land clearing (Aleksandrowicz et al., 2016; Poore and Nemecek, 2018; Clark et al., 2019; Chaudhary et al., 2022). Others have shown that a shift toward the recommended healthy pattern (SDG 2 and 3) in affluent countries would co-benefit SDG 15 due to a lower land demand than current average footprints (Behrens et al., 2017; Ridoutt et al., 2020).
However, the cropland use reduction was found to be negligible if global food consumption transitioned toward the healthy diet that EAT-Lancet Commission recommended, with only 0-2% lesser land demand than current levels (Willett et al., 2019). According to their model, the reduction in land demand for animal products and feed crops was compensated by the increased demand for filling intake gaps of healthy foods like nuts and legumes, which are relatively low yielding in many national food systems (Willett et al., 2019). South Asia, Sub-Saharan Africa, India, and East Asia and Pacific are likely to have 20–50% increase in the cropland needs for adopting the EAT-Lancet proposed reference diet, while the adoption of such diet would reduce one quarter of land footprints embedded in food consumption for North America, Europe, and Central Asia (Table 1). At the national level, Chaudhary and Krishna (2021) showed that a shift toward sustainable diet in India would entail 33% higher per capita land footprint although this increased land demand can be met if the yield gaps of major crops are filled.
Several studies have explored the consequences of past, current and future human cropland use and individual food items on biodiversity (Machovina et al., 2015; Chaudhary and Kastner, 2016; Chaudhary and Brooks, 2018; Chaudhary et al., 2018c; Green et al., 2019; Willett et al., 2019; Leclère et al., 2020; Williams et al., 2020; Benton et al., 2021; Mair et al., 2021). Animal meat products in human diets are associated with disproportionately large biodiversity footprints and their demand is projected to further increase (Machovina et al., 2015), thus threatening biodiversity across the world (Leclère et al., 2020; Benton et al., 2021). Across crop foods, staples, sugarcane, palm oil, and cocoa exported from biodiverse and less affluent countries including Indonesia, Madagascar, Tanzania, and Philippines are the hotspots of natural habitat loss and degradation with consequent negative biodiversity impacts (Chaudhary and Kastner, 2016; Chaudhary et al., 2018c).
The specific location of cropland use influences the magnitude of the biodiversity impact because species are distributed non-uniformly in the world with higher species density in tropical regions. Transition toward a healthy diet might increase the clearance of natural habitats for agriculture purposes if regional food systems do not consider biodiversity conservation as a goal. It thus calls for complementary strategies such as the optimized location of regional crop production to minimize the conflicts between food provision and biodiversity integrity of ecosystems (Erb et al., 2016).
Apart from the land-use induced habitat loss and threat, nutrient runoff and loading due to fertilizer application is also associated with damage to the ecosystem function and the terrestrial biodiversity jeopardization. Limiting the agricultural nitrogen and phosphorus footprint embedded in dietary choices (SDG 14) could also contribute positively toward the SDG 15.
Discussion
According to current dietary demand trajectories and socio-economic development (e.g., population, income), global food-related environmental footprints are projected to transgress all five planetary boundaries by 2050 in terms of GHG emission, cropland, freshwater, and nitrogen and phosphorus application (Springmann et al., 2018a; Willett et al., 2019). Supply-side efforts such as closing yield gaps, agriculture expansion and intensification are either associated with an increase in resource inputs (e.g., fertilizer, water, land) or not enough to meet the global food demand by 2050 if continuing currents diets (Bajželj et al., 2014; Springmann et al., 2018a). Moreover, currently less affluent regions will expect a necessary growth in the consumption of diverse food products including animal protein for tackling food insecurity and malnutrition. A shift toward healthy and sustainable eating pattern worldwide as a key demand-side measure is therefore imperative for feeding the global population within a safe living space and progress toward SDGs.
The interactions between SDGs are complex and their connections are dynamic for different development levels (Pradhan et al., 2017; Wu et al., 2022). The co-benefits of dietary change for mitigating climate change and improving public health (e.g., obesity, cardiovascular diseases, nutrition) are often highlighted in different global and national modeling studies (Payne et al., 2016; Song et al., 2017). However, the implications for multiple SDGs can be mixed and divergent. For example, sustainable fish consumption needs to address the potential trade-offs between biodiversity in aquatic ecosystem (SDG 15), dietary nutrition (SDG 2, 3), and poverty (SDG 1), especially for the small-scale fishery communities and the coastal localities (Blanchard et al., 2017; Hicks et al., 2019). The decision-making in crop options (e.g., rice vs. sorghum in India) also needs to balance the dietary nutrition provision (SDG 2, 3) and the resilience of climate risks in agricultural production (SDG 13) (DeFries et al., 2016). The affordability (SDG 1) of a low-environmental-footprint (SDG 5, 6) and healthy (SDG 2, 3) diet is worth examination especially when considering the economic challenges for poorer communities (Reynolds et al., 2019; Penne and Goedemé, 2020; Gupta et al., 2021). Overall, our review advocates the need for comprehensive assessments that can reveal the potential trade-offs and support an optimal decision-making. Supplementary Table S1 summarizes the studies looking at the connection between dietary change and sustainable development goals (SDG) indicators.
Here in sections below we briefly discuss how to achieve this dietary transition. No single stakeholders or actors can catalyze the dietary change; the food producers, industries (e.g., packaging, marketing), service providers (e.g., menu in restaurants and school canteens), policy makers (e.g., fiscal interventions), and food-related culture norms (e.g., traditions, habits, cooking skills) and so forth would have to together drive this transition.
Consumer Behavior Change
Consumers make personal choices on what they eat according to individual preference, meanwhile their choices are also shaped by their cultural or religious background as well as the food environment that are composed of various food-related activities such as available foods in local markets, food price, the perceived product properties, marketing, and regulation (Herforth and Ahmed, 2015; Bianchi et al., 2018; Turner et al., 2018).
To broadly achieve a shift from current diets to sustainable healthy dietary choices, the latest scientific knowledge from consumer behavior studies could help design the measures. For example, the social norms including the peer's engagement or positive evaluation on vegetarianism and the self-efficacy meaning a belief in the power of mitigating the adverse environmental effect via dietary shifts are the top two factors that drive the behavioral change in food consumption context (Eker et al., 2019; van Valkengoed and Steg, 2019). For local communities, campaigns that target modifying the social norms on dietary habits may contribute to dietary change behaviors (Rust et al., 2020).
The growing body of scientific evidence reveals the magnitude of benefits from adoption of sustainable diets, and this can strengthen the perceived self-efficacy if the findings can be clearly communicated to different stakeholders and broad audience. For example, consumers tend to underestimate the environmental impact of food products, thus providing them correct impact information through intuitive indicators (e.g., in units like light-bulb minutes or number of cars on road) as product labels could facilitate more sustainable purchase choices (Camilleri et al., 2019; Siegrist and Hartmann, 2019). Particularly in high-income countries, people given health co-benefit information are likely to show more willingness to adopt the environmentally-friendly products or diets (Amelung et al., 2019). For global and national food-based dietary guidelines, consideration of the potential impacts on human health and the environment together is therefore of importance to drive dietary behavior changes (Lazzarini et al., 2018; Howe et al., 2019).
Apart from the conscious determinants of dietary behaviors like values and knowledge, the non-conscious and non-reasoning behavioral processes may also have a discernible impact on individual demand (Marteau, 2017). Reconstructing the physical micro-environments such as reducing meat serving size, providing meat alternative products with educational information, and changing the sensory characteristics of the products, were found to be effective in reducing meat consumption which in turn has sustainability benefits (Bianchi et al., 2018; Rust et al., 2020). Similarly, placing more sustainable and healthy product options on retail shelf and menu and making them visually prominent can increase their consumption (Garnett et al., 2019).
Monetary and Fiscal Interventions
Apart from individual behavior changes, fiscal interventions can also drive the transition from current to sustainable dietary behaviors. A sugary drink tax in Mexico has decreased their consumption by 7.6% with the greatest reduction effects among low-income households that reduced purchases by 11.7% (Colchero et al., 2017). The tax on sugar-sweetened beverages in UK was found to drive product-reformulation by food industries, reducing sugar intake from soft drinks and inducing positive population health outcomes like obesity reduction (Briggs et al., 2017; Bandy et al., 2020).
Fiscal measures like levying carbon tax on food products could be a viable option for addressing the hidden cost associated with the foods and motivate a shift toward sustainable and healthy dietary patterns (Edjabou and Smed, 2013; Pieper et al., 2020). Though varying across regions, a global carbon emission tax would increase the market price of animal source products the most due to their substantially high GHG emissions and consequently the consumption of beef, milk, and lamb would have the most reduction (Springmann et al., 2017). On the other hand, health related taxes on food products which are high in added sugar, unhealthy fats, or carcinogens could also help improve the dietary quality and decrease food related chronic disease risks (Springmann et al., 2018b; Waterlander et al., 2019; Blakely et al., 2020).
However, such taxes should not hinder the progress toward ending hunger and malnutrition (SDG 2), especially for low-income households who will be unable to purchase sufficient and nutritious foods if the price is raised. To avoid this scenario, the revenues of environmental tax could be recycled to the consumers via lowering the price of healthier and sustainable food like fruits, vegetables, and plant-based protein products. This revenue recycling can involve direct subsidization (Olsho et al., 2016; Springmann et al., 2017) or the capital investment on sustainable alternatives (Mason-D'Croz et al., 2019a,b). The combination of taxes and subsidies for respective food categories could help address the underlying dilemma that people living with poor diets cannot afford the recommended nutritious foods like fruits, vegetables, nuts, and legumes (Hirvonen et al., 2019).
Regarding the investments in food systems to achieve SDGs, developing quantitative indicators capable of discerning the sustainability performance of alternative products (Chaudhary et al., 2018b; Chaudhary and Tremorin, 2020; Mair et al., 2021) and the economic valuation of sustainability benefits from dietary change (Springmann et al., 2016) could help to guide the industrial investors and other stakeholders. Governments could remove systemic barriers (e.g., policy instruments, subsidies, etc.) to such investments for creating an environment that cooperates with capital interests and stimulates the business models in favor of human wellbeing and ecosystem integrity.
To adopt the healthy diets, food systems should improve their supply of affordable, palatable, diverse, and acceptable food commodity options (e.g., plant-based protein sources, fruits, vegetables) for people worldwide (Ferreira et al., 2021). Policy in this case could help advance the development priorities such as high yielding crop varieties of insufficient foods [e.g., legumes and nuts in undernourished regions; Ofori et al., 2021], resource-efficient agriculture practices (Davis et al., 2017; Rosa et al., 2018; Rothrock et al., 2019; van Wijk et al., 2020; Jin et al., 2021; Obirikorang et al., 2021), sustainable fisheries management, alternative food and feed (Gold et al., 2018; Caporgno et al., 2020) through fiscal and other interventions.
Current Data Gaps
Previous global assessments often rely on the food balance sheet data from United Nations Food and Agriculture Organization (FAO, 2019) to derive the average dietary intake estimates at the country level. However, the intake amounts of only 98 primary commodities are available here while in reality people consume hundreds of processed food products which has implications for actual nutrient intake as well as environmental footprints (e.g., nutrient content and footprint of pizza or pasta is different from its primary product wheat). Although GENUS database (Smith et al., 2016) improved the resolution to 225 food items, the intake amounts of many processed items remain elusive for most countries. Also, in addition to national average data, the dietary intake data should be available for different age, gender, region, or income groups for a given country to better understand the nutritional inadequacies for devising interventions.
The dietary guidelines should also improve the food item resolution taking into account cultural acceptability of the region (Chaudhary and Krishna, 2019). For example, the EAT-Lancet reference diet includes 16 broad food groups with wide intake ranges and exchangeable options (e.g., a set of animal and plant protein sources), which needs further fine tuning for individual food items to be more useful for personal and policy decision-making. Emerging platforms like Food System Dashboard (Fanzo et al., 2020), Global Burden of Disease (GBD), and Global Dietary Database (Khatibzadeh et al., 2016) have aggregated extensive data-based evidence to provide accessible information on food system and its impacts.
The food composition databases providing nutrient content per unit mass of foods also need to include data for food items in the final form in which they are eaten (e.g., pizza instead of just wheat). Processing of foods through cooking can leads to nutrient loss and thus considering primary food items instead of final processed ones can lead to overestimation of nutrient adequacy of diets.
Huge efforts are still needed to come up with accurate environmental footprints of different food items produced in different parts of the world. Previous studies have done significant works to collect the scattering data points on environmental footprints of foods in different regions and production systems (Mekonnen and Hoekstra, 2011; Clark and Tilman, 2017; Clune et al., 2017; Poore and Nemecek, 2018; Springmann et al., 2018a,b) but gaps remain. For example, emission changes mediated by the soil carbon stock were insufficiently addressed in climate change implications of dietary strategies (Ridoutt et al., 2017). The water footprints should better take into account the local water scarcity situations (Clark et al., 2019).
Other issue is quantifying the impact of diets on multiple domains of the environment rather than on a selected few. Most existing research focuses on climate change, land use, and water use impacts, while other impact categories are rarely addressed (Ridoutt et al., 2017). Environmental burdens such as biodiversity losses (Chaudhary et al., 2015, 2018c), chemical toxicity from pesticide use, air pollution linked with food production (Lelieveld et al., 2015; Blackstone et al., 2018; Domingo et al., 2021) have not been well quantified when evaluating the impact of global dietary changes. For environmental sustainability, the future dietary change studies can utilize recently proposed environmental footprint family that are directly linked with the SDG framework (Vanham et al., 2019).
Finally, a harmonized dataset on price of different food items in different parts of the world is need of the hour. The low affordability of healthy and sustainable diets could be the key barrier of realizing dietary changes around the globe while previous assessment such as EAT-Lancet commission often failed to examine the economic feasibility of recommended dietary transitions due to the lack of robust data. This would entail expanding existing programs such as International Comparison Program (ICP) of World Bank to include more food items and making it open-access or compiling retail food price data from local supermarkets across the globe.
As shown in Supplementary Table S1, indicators used in the past dietary change studies did not always align with global SDG indicator framework (United Nations, 2021). In future, the indicator identification could consider a SMART manner (specific, measurable, attainable, relevant, time-based) and learn from the representative case studies in different regions (Germann and Langergraber, 2022). In the end, all indicative numbers are tools to help capture the benchmark, progress, as well as the complexity of sustainability, with the ultimate goal being locally feasible actions in real world. The scope of this review was to provide an overview of the studies that have shown how changing our current diets can help us make progress toward six different SDGs, but future studies should carry out an in-depth quantitative analysis on the potential of dietary change to contribute toward progress on individual SDG indicators (Germann and Langergraber, 2022).
We acknowledge that SDG 1 (No poverty), SDG 5 (Gender equality), SDG 8 (Decent work and economic growth), SDG 10 (Reduced inequalities), SDG 12 (Responsible consumption and production), and other SDGs are also interconnected with the performance of food systems (Chaudhary et al., 2018a,b,c; Alarcon et al., 2021) but presenting quantitative evidence on their linkages is beyond the scope of this review. Future research efforts should focus on filling this gap and present the implications of dietary change for other SDG targets.
Conclusions
Agricultural production to feed increasing world population with rising income levels and globalization is one of the leading causes of climate change, ongoing biodiversity extinctions, land degradation, water pollution and ecosystem service loss (Kastner et al., 2021). Yet, the diets of almost all nations lack in one or more micronutrients (vitamins, minerals) essential for human wellbeing (Chaudhary and Krishna, 2019; Chen et al., 2021). Widespread economic inequalities and the supply instability risks under emerging disturbances like the pandemic and climate change may increase the risk for inadequate and unsustainable diets in near future and thus calls for urgent research and policy efforts (Kimani-Murage et al., 2021).
Applying a consistent Sustainable Development Goals (SDG) framework, here we carried out a quantitative review of over 200 published articles aiming to inform national policy makers and food business companies on which food items and nutrients should be promoted or discouraged in different countries of the world to achieve nutritional security and meet environmental targets.
We highlighted the existing evidence that dietary changes can lead to win-win outcomes for human society and the planet revealing the heterogeneity of dietary patterns and the associated sustainability impact across the world. The dietary change strategies should consider the diverse needs (e.g., undernutrition vs. diet-related non-communicable diseases) and regional-adaptive solutions (e.g., local food availability, regional production condition, food preferences, trade partnerships).
Unlike past reviews focusing on a particular world region, sustainability aspects, or limited number of metrics, the main strength of our review is the consideration of six SDGs simultaneously as well as the use of several indicators of environmental footprint (GHG emissions, freshwater use, land use, biodiversity loss, nitrogen, and phosphorus application) and nutritional quality for all countries of the world. This enables in identification of geographic hotspots and highlights potential trade-offs in achievement of different SDGs and underscores the need for breaking silos and adopting an interdisciplinary approach to solve these problems. No single stakeholders or actors can catalyze the dietary change toward sustainable food consumption. Contributions for the food producers (farmers), industries (e.g., packaging, marketing), service providers (e.g., restaurants, retail, school canteens), policy makers (e.g., those designing fiscal interventions), and food-related cultural norms (e.g., traditions, habits, cooking skills) and others would together drive this transition.
Author Contributions
CC conceived the ideas, designed the study, compiled data, carried out analysis, and wrote the first draft. AC conceived the ideas, designed the study, and wrote the manuscript. AM provided comments on manuscript. All authors have critically reviewed the manuscript for the intellectual content and approved the final version.
Funding
The research was funded by the National Research Program Sustainable Economy: resource-friendly, future-oriented, innovative (NRP 73) by the Swiss National Science Foundation (Grant Number: 407340_172415) and the Initiation Grant of IIT Kanpur, India (Project Number: 2018386).
Conflict of Interest
The authors declare that the research was conducted in the absence of any commercial or financial relationships that could be construed as a potential conflict of interest.
Publisher's Note
All claims expressed in this article are solely those of the authors and do not necessarily represent those of their affiliated organizations, or those of the publisher, the editors and the reviewers. Any product that may be evaluated in this article, or claim that may be made by its manufacturer, is not guaranteed or endorsed by the publisher.
Supplementary Material
The Supplementary Material for this article can be found online at: https://www.frontiersin.org/articles/10.3389/fsufs.2022.771041/full#supplementary-material
References
Abejón, R., Batlle-Bayer, L., Laso, J., Bala, A., Vazquez-Rowe, I., Larrea-Gallegos, G., et al. (2020). Multi-objective optimization of nutritional, environmental and economic aspects of diets applied to the Spanish Context. Foods 9, 1677. doi: 10.3390/foods9111677
Afshin, A., Micha, R., Khatibzadeh, S., and Mozaffarian, D. (2014). Consumption of nuts and legumes and risk of incident ischemic heart disease, stroke, and diabetes: a systematic review and meta-analysis. Am. J. Clin. Nutr. 100, 278–288. doi: 10.3945/ajcn.113.076901
Afshin, A., Sur, P. J., Fay, K. A., Cornaby, L., Ferrara, G., Salama, J. S., et al. (2019). Health effects of dietary risks in 195 countries, 1990-2017: a systematic analysis for the Global Burden of Disease Study 2017. Lancet 393, 1958–1972. doi: 10.1016/S0140-6736(19)30041-8
Alae-Carew, C., Nicoleau, S., Bird, F. A., Hawkins, P., Tuomisto, H. L., Haines, A., et al. (2020). The impact of environmental changes on the yield and nutritional quality of fruits, nuts and seeds: a systematic review. Environ. Res. Lett. 15, 023002. doi: 10.1088/1748-9326/ab5cc0
Alarcon, P., Dominguez-Salas, P., Fèvre, E. M., and Rushton, J. (2021). The importance of a food systems approach to low and middle income countries and emerging economies: A review of theories and its relevance for disease control and malnutrition. Front. Sustain. Food Syst. 5, 642635. doi: 10.3389/fsufs.2021.642635
Aleksandrowicz, L., Green, R., Joy, E. J. M., Smith, P., and Haines, A. (2016). The impacts of dietary change on greenhouse gas emissions, land use, water use, and health: a systematic review. PLoS ONE 11, e0165797. doi: 10.1371/journal.pone.0165797
Amelung, D., Fischer, H., Herrmann, A., Aall, C., Louis, V. R., Becher, H., et al. (2019). Human health as a motivator for climate change mitigation: results from four European high-income countries. Glob. Environ. Chang. 57, 101918. doi: 10.1016/j.gloenvcha.2019.05.002
Aune, D., Keum, N., Giovannucci, E., Fadnes, L. T., Boffetta, P., Greenwood, D. C., et al. (2016). Whole grain consumption and risk of cardiovascular disease, cancer, and all cause and cause specific mortality: systematic review and dose-response meta-analysis of prospective studies. BMJ 353, i2716. doi: 10.1136/bmj.i2716
Bai, Y., Alemu, R., Block, S. A., Headey, D., and Masters, W. A. (2020). Cost and affordability of nutritious diets at retail prices: evidence from 177 countries. Food Policy 99, 101983. doi: 10.1016/j.foodpol.2020.101983
Bajželj, B., Richards, K. S., Allwood, J. M., Smith, P., Dennis, J. S., Curmi, E., et al. (2014). Importance of food-demand management for climate mitigation. Nat. Clim. Chang. 4, 924–929. doi: 10.1038/nclimate2353
Bandy, L. K., Scarborough, P., Harrington, R. A., Rayner, M., and Jebb, S. A. (2020). Reductions in sugar sales from soft drinks in the UK from 2015 to 2018. BMC Med. 18, 20. doi: 10.1186/s12916-019-1477-4
Batlle-Bayer, L., Bala, A., Albertí, J., Xifré, R., Aldaco, R., and Fullana-i-Palmer, P. (2020). Food affordability and nutritional values within the functional unit of a food LCA. An application on regional diets in Spain. Resour. Conserv. Recycl. 160, 104856. doi: 10.1016/j.resconrec.2020.104856
Beal, T., Massiot, E., Arsenault, J. E., Smith, M. R., and Hijmans, R. J. (2017). Global trends in dietary micronutrient supplies and estimated prevalence of inadequate intakes. PLoS ONE 12, e0175554. doi: 10.1371/journal.pone.0175554
Behrens, P., Kiefte-de Jong, J. C., Bosker, T., Rodrigues, J. F. D., de Koning, A., and Tukker, A. (2017). Evaluating the environmental impacts of dietary recommendations. Proc. Natl. Acad. Sci. U.S.A. 114, 13412–13417. doi: 10.1073/pnas.1711889114
Benton, T. G., Bieg, C., Harwatt, H., Pudasaini, R., and Wellesley, L. (2021). Food System Impacts on Biodiversity Loss. Three Levers for Food System Transformation in Support of Nature. London: Chatham House.
Bianchi, F., Garnett, E., Dorsel, C., Aveyard, P., and Jebb, S. A. (2018). Restructuring physical micro-environments to reduce the demand for meat: a systematic review and qualitative comparative analysis. Lancet Planet. Heal. 2, e384–e397. doi: 10.1016/S2542-5196(18)30188-8
Black, R. E., Allen, L. H., Bhutta, Z. A., Caulfield, L. E., de Onis, M., Ezzati, M., et al. (2008). Maternal and child undernutrition: global and regional exposures and health consequences. Lancet 371, 243–260. doi: 10.1016/S0140-6736(07)61690-0
Blackstone, N. T., and Conrad, Z. (2020). Comparing the recommended eating patterns of the EAT-Lancet Commission and dietary guidelines for americans: implications for sustainable nutrition. Curr. Dev. Nutr. 4, nzaa015. doi: 10.1093/cdn/nzaa015
Blackstone, N. T., El-Abbadi, N. H., McCabe, M. S., Griffin, T. S., and Nelson, M. E. (2018). Linking sustainability to the healthy eating patterns of the Dietary Guidelines for Americans: a modelling study. Lancet Planet. Heal. 2, e344–e352. doi: 10.1016/S2542-5196(18)30167-0
Blakely, T., Cleghorn, C., Mizdrak, A., Waterlander, W., Nghiem, N., Swinburn, B., et al. (2020). The effect of food taxes and subsidies on population health and health costs: a modelling study. Lancet Public Heal. 5, e404–e413. doi: 10.1016/S2468-2667(20)30116-X
Blanchard, J. L., Watson, R. A., Fulton, E. A., Cottrell, R. S., Nash, K. L., et al. (2017). Linked sustainability challenges and trade-offs among fisheries, aquaculture and agriculture. Nat. Ecol. Evol. 1, 1240–1249. doi: 10.1038/s41559-017-0258-8
Bodirsky, B. L., Popp, A., Lotze-Campen, H., Dietrich, J. P., Rolinski, S., Weindl, I., et al. (2014). Reactive nitrogen requirements to feed the world in 2050 and potential to mitigate nitrogen pollution. Nat. Commun. 5, 1–7. doi: 10.1038/ncomms4858
Bogard, J. R., Marks, G. C., Wood, S., and Thilsted, S. H. (2018). Measuring nutritional quality of agricultural production systems: application to fish production. Glob. Food Sec. 16, 54–64. doi: 10.1016/j.gfs.2017.09.004
Bouwman, L., Goldewijk, K. K., Van Der Hoek, K. W., Beusen, A. H. W., Van Vuuren, D. P., Willems, J., et al. (2013). Exploring global changes in nitrogen and phosphorus cycles in agriculture induced by livestock production over the 1900-2050 period. Proc. Natl. Acad. Sci. U.S.A. 110, 20882–20887. doi: 10.1073/pnas.1012878108
Briggs, A. D. M., Mytton, O. T., Kehlbacher, A., Tiffin, R., Elhussein, A., Rayner, M., et al. (2017). Health impact assessment of the UK soft drinks industry levy: a comparative risk assessment modelling study. Lancet Public Heal. 2, e15–e22. doi: 10.1016/S2468-2667(16)30037-8
Broekema, R., Tyszler, M., van 't Veer, P., Kok, F. J., Martin, A., Lluch, A., et al. (2020). Future-proof and sustainable healthy diets based on current eating patterns in the Netherlands. Am. J. Clin. Nutr. 112, 1338–1347. doi: 10.1093/ajcn/nqaa217
Camilleri, A. R., Larrick, R. P., Hossain, S., and Patino-Echeverri, D. (2019). Consumers underestimate the emissions associated with food but are aided by labels. Nat. Clim. Chang. 9, 53–58. doi: 10.1038/s41558-018-0354-z
Caporgno, M. P., Böcker, L., Müssner, C., Stirnemann, E., Haberkorn, I., Adelmann, H., et al. (2020). Extruded meat analogues based on yellow, heterotrophically cultivated Auxenochlorella protothecoides microalgae. Innov. Food Sci. Emerg. Technol. 59, 102275. doi: 10.1016/j.ifset.2019.102275
Challinor, A. J., Watson, J., Lobell, D. B., Howden, S. M., Smith, D. R., and Chhetri, N. (2014). A meta-analysis of crop yield under climate change and adaptation. Nat. Clim. Chang. 4, 287–291. doi: 10.1038/nclimate2153
Chaudhary, A., and Brooks, T. M. (2017). National consumption and global trade impacts on biodiversity. World Dev. 120, 178–187. doi: 10.1016/j.worlddev.2017.10.012
Chaudhary, A., and Brooks, T. M. (2018). Land use intensity-specific global characterization factors to assess product biodiversity footprints. Environ. Sci. Technol. 52, 5094–5104. doi: 10.1021/acs.est.7b05570
Chaudhary, A., Gustafson, D., and Mathys, A. (2018a). Multi-indicator sustainability assessment of global food systems. Nat. Commun. 9, 1–13. doi: 10.1038/s41467-018-03308-7
Chaudhary, A., and Kastner, T. (2016). Land use biodiversity impacts embodied in international food trade. Glob. Environ. Chang. 38, 195–204. doi: 10.1016/j.gloenvcha.2016.03.013
Chaudhary, A., and Krishna, V. (2019). Country-specific sustainable diets using optimization algorithm. Environ. Sci. Technol. 53, 7694–7703. doi: 10.1021/acs.est.8b06923
Chaudhary, A., and Krishna, V. (2021). Region-specific nutritious, environmentally friendly, and affordable diets in India. One Earth 4, 531–544. doi: 10.1016/j.oneear.2021.03.006
Chaudhary, A., Mair, L., Strassburg, B. B. N., Brooks, T. M., Menon, V., and McGowan, P. J. (2022). Sub-national assessment of threats to Indian biodiversity and habitat restoration opportunities. Environ. Res. Lett. 17, 054022. doi: 10.1088/1748-9326/ac5d99
Chaudhary, A., Marinangeli, C. P. F., Tremorin, D., and Mathys, A. (2018b). Nutritional combined greenhouse gas life cycle analysis for incorporating canadian yellow pea into cereal-based food products. Nutrients 10, 490. doi: 10.3390/nu10040490
Chaudhary, A., Mooers, A., Chaudhary, A., and Mooers, A. O. (2018c). Terrestrial vertebrate biodiversity loss under future global land use change scenarios. Sustainability 10, 2764. doi: 10.3390/su10082764
Chaudhary, A., and Tremorin, D. (2020). Nutritional and environmental sustainability of lentil reformulated beef burger. Sustainability 12, 6712. doi: 10.3390/su12176712
Chaudhary, A., Verones, F., De Baan, L., and Hellweg, S. (2015). Quantifying land use impacts on biodiversity: combining species-area models and vulnerability indicators. Environ. Sci. Technol. 49, 9987–9995. doi: 10.1021/acs.est.5b02507
Chen, C., Chaudhary, A., and Mathys, A. (2019). Dietary change scenarios and implications for environmental, nutrition, human health and economic dimensions of food sustainability. Nutrients 11, 856. doi: 10.3390/nu11040856
Chen, C., Chaudhary, A., and Mathys, A. (2020). Nutritional and environmental losses embedded in global food waste. Resour. Conserv. Recycl. 160, 104912. doi: 10.1016/j.resconrec.2020.104912
Chen, C., Chaudhary, A., and Mathys, A. (2021). Nutrient adequacy of global food production. Front. Nutr. 8, 739755. doi: 10.3389/fnut.2021.739755
Clark, M., and Tilman, D. (2017). Comparative analysis of environmental impacts of agricultural production systems, agricultural input efficiency, and food choice. Environ. Res. Lett. 12, 064016. doi: 10.1088/1748-9326/aa6cd5
Clark, M. A., Domingo, N. G., Colgan, K., Thakrar, S. K., Tilman, D., et al. (2020). Global food system emissions could preclude achieving the 1.5 and 2 C climate change targets. Science 370, 705–708. doi: 10.1126/science.aba7357
Clark, M. A., Springmann, M., Hill, J., and Tilman, D. (2019). Multiple health and environmental impacts of foods. Proc. Natl. Acad. Sci. U.S.A. 116, 23357–23362. doi: 10.1073/pnas.1906908116
Clune, S., Crossin, E., and Verghese, K. (2017). Systematic review of greenhouse gas emissions for different fresh food categories. J. Clean. Prod. 140, 766–783. doi: 10.1016/j.jclepro.2016.04.082
Colchero, M. A., Rivera-Dommarco, J., Popkin, B. M., and Ng, S. W. (2017). In Mexico, evidence of sustained consumer response two years after implementing a sugar-sweetened beverage tax. healthaffairs.org. 36, 564–571. doi: 10.1377/hlthaff.2016.1231
Cooper, M. W., Brown, M. E., Hochrainer-Stigler, S., Pflug, G., McCallum, I., Fritz, S., et al. (2019). Mapping the effects of drought on child stunting. Proc. Natl. Acad. Sci. U.S.A. 116, 17219–17224. doi: 10.1073/pnas.1905228116
Crippa, M., Solazzo, E., Guizzardi, D., Monforti-Ferrario, F., Tubiello, F. N., and Leip, A. (2021). Food systems are responsible for a third of global anthropogenic GHG emissions. Nat. Food 2, 198–209. doi: 10.1038/s43016-021-00225-9
Dalin, C., Hanasaki, N., Qiu, H., Mauzerall, D. L., and Rodriguez-Iturbe, I. (2014). Water resources transfers through Chinese interprovincial and foreign food trade. Proc. Natl. Acad. Sci. U.S.A. 111, 9774–9779. doi: 10.1073/pnas.1404749111
Darmon, N., and Drewnowski, A. (2015). Contribution of food prices and diet cost to socioeconomic disparities in diet quality and health: a systematic review and analysis. Nutr. Rev. 73, 643–660. doi: 10.1093/nutrit/nuv027
Davis, K. F., Chiarelli, D. D., Rulli, M. C., Chhatre, A., Richter, B., Singh, D., et al. (2018). Alternative cereals can improve water use and nutrient supply in India. Sci. Adv. 4, eaao1108. doi: 10.1126/sciadv.aao1108
Davis, K. F., Gephart, J. A., Emery, K. A., Leach, A. M., Galloway, J. N., and D'Odorico, P. (2016). Meeting future food demand with current agricultural resources. Glob. Environ. Chang. 39, 125–132. doi: 10.1016/j.gloenvcha.2016.05.004
Davis, K. F., Rulli, M. C., Seveso, A., and D'Odorico, P. (2017). Increased food production and reduced water use through optimized crop distribution. Nat. Geosci. 10, 919–924. doi: 10.1038/s41561-017-0004-5
DeFries, R., Mondal, P., Singh, D., Agrawal, I., Fanzo, J., Remans, R., et al. (2016). Synergies and trade-offs for sustainable agriculture: nutritional yields and climate-resilience for cereal crops in Central India. Glob. Food Sec. 11, 44–53. doi: 10.1016/j.gfs.2016.07.001
Diana, J. S. (2009). Aquaculture production and biodiversity conservation. Bioscience 59, 27–38. doi: 10.1525/bio.2009.59.1.7
Dinu, M., Pagliai, G., Casini, A., and Sofi, F. (2018). Mediterranean diet and multiple health outcomes: an umbrella review of meta-analyses of observational studies and randomised trials. Eur. J. Clin. Nutr. 72, 30–43. doi: 10.1038/ejcn.2017.58
Domingo, N. G. G., Balasubramanian, S., Thakrar, S. K., Clark, M. A., Adams, P. J., Marshall, J. D., et al. (2021). Air quality-related health damages of food. Proc. Natl. Acad. Sci. U.S.A. 118, e2013637118. doi: 10.1073/pnas.2013637118
Donati, M., Menozzi, D., Zighetti, C., Rosi, A., Zinetti, A., and Scazzina, F. (2016). Towards a sustainable diet combining economic, environmental and nutritional objectives. Appetite 106, 48–57. doi: 10.1016/j.appet.2016.02.151
Doney, S. C., Fabry, V. J., Feely, R. A., and Kleypas, J. A. (2009). Ocean acidification: the other CO2 problem. Ann. Rev. Mar. Sci. 1, 169–192. doi: 10.1146/annurev.marine.010908.163834
Drew, J., Cleghorn, C., Macmillan, A., and Mizdrak, A. (2020). Healthy and climate-friendly eating patterns in the New Zealand context. Environ. Health Perspect. 128, 017007. doi: 10.1289/EHP5996
Edjabou, L. D., and Smed, S. (2013). The effect of using consumption taxes on foods to promote climate friendly diet - the case of Denmark. Food Policy 39, 84–96. doi: 10.1016/j.foodpol.2012.12.004
Eini-Zinab, H., Sobhani, S. R., and Rezazadeh, A. (2020). Designing a healthy, low-cost and environmentally sustainable food basket: an optimisation study. Public Health Nutr. 24, 1–10. doi: 10.1017/S1368980020003729
Eker, S., Reese, G., and Obersteiner, M. (2019). Modelling the drivers of a widespread shift to sustainable diets. Nat. Sustain. 2, 725–735. doi: 10.1038/s41893-019-0331-1
Erb, K. H., Lauk, C., Kastner, T., Mayer, A., Theurl, M. C., and Haberl, H. (2016). Exploring the biophysical option space for feeding the world without deforestation. Nat. Commun. 7, 1–9. doi: 10.1038/ncomms11382
Esteve-Llorens, X., Martín-Gamboa, M., Iribarren, D., Moreira, M. T., Feijoo, G., and González-García, S. (2020). Efficiency assessment of diets in the Spanish regions: a multi-criteria cross-cutting approach. J. Clean. Prod. 242, 118491. doi: 10.1016/j.jclepro.2019.118491
Estrada, A., Garber, P. A., and Chaudhary, A. (2019). Expanding global commodities trade and consumption place the world's primates at risk of extinction. PeerJ 7, e7068. doi: 10.7717/peerj.7068
Estrada, A., Garber, P. A., and Chaudhary, A. (2020). Current and future trends in socio-economic, demographic and governance factors affecting global primate conservation. PeerJ 8, e9816. doi: 10.7717/peerj.9816
Fanzo, J., Haddad, L., McLaren, R., Marshall, Q., Davis, C., Herforth, A., et al. (2020). The Food Systems Dashboard is a new tool to inform better food policy. Nat. Food 1, 243–246. doi: 10.1038/s43016-020-0077-y
FAO (2019). Statistical Databases. FAOSTAT. Available online at: http://www.fao.org/faostat/en/#home (accessed March 21, 2018).
FAO, IFAD, UNICEF, WFP, and WHO. (2020). The State of Food Security and Nutrition in the World 2020. Transforming Food Systems for Affordable Healthy Diets. Rome: FAO, IFAD, UNICEF, WFP, and WHO.
Fern, E. B., Watzke, H., Barclay, D. V., Roulin, A., and Drewnowski, A. (2015). The nutrient balance concept: a new quality metric for composite meals and diets. PLoS ONE 10, e0130491. doi: 10.1371/journal.pone.0130491
Ferreira, H., Pinto, E., and Vasconcelos, M. W. (2021). Legumes as a cornerstone of the transition toward more sustainable agri-food systems and diets in Europe. Front. Sustain. Food Syst. 5, 694121. doi: 10.3389/fsufs.2021.694121
Foley, J. A., Ramankutty, N., Brauman, K. A., Cassidy, E. S., Gerber, J. S., Johnston, M., et al. (2011). Solutions for a cultivated planet. Nature 478, 337–342. doi: 10.1038/nature10452
Fry, J. P., Love, D. C., MacDonald, G. K., West, P. C., Engstrom, P. M., Nachman, K. E., et al. (2016). Environmental health impacts of feeding crops to farmed fish. Environ. Int. 91, 201–214. doi: 10.1016/j.envint.2016.02.022
Garcia, S. N., Osburn, B. I., and Jay-Russell, M. T. (2020). One health for food safety, food security, and sustainable food production. Front. Sustain. Food Syst. 4, 1. doi: 10.3389/fsufs.2020.00001
Garnett, E. E., Balmford, A., Sandbrook, C., Pilling, M. A., and Marteau, T. M. (2019). Impact of increasing vegetarian availability on meal selection and sales in cafeterias. Proc. Natl. Acad. Sci. U.S.A. 116, 20923–20929. doi: 10.1073/pnas.1907207116
GBD 2019 Diseases and Injuries Collaborators (2020). Global burden of 369 diseases and injuries in 204 countries and territories, 1990-2019: a systematic analysis for the Global Burden of Disease Study 2019. Lancet 396, 1204–1222. doi: 10.1016/S0140-6736(20)30925-9
Gephart, J. A., Davis, K. F., Emery, K. A., Leach, A. M., Galloway, J. N., and Pace, M. L. (2016). The environmental cost of subsistence: optimizing diets to minimize footprints. Sci. Total Environ. 553, 120–127. doi: 10.1016/j.scitotenv.2016.02.050
Germann, V., and Langergraber, G. (2022). Going beyond global indicators-policy relevant indicators for SDG 6 targets in the context of Austria. Sustainability 14, 1647. doi: 10.3390/su14031647
Gödecke, T., Stein, A. J., and Qaim, M. (2018). The global burden of chronic and hidden hunger: trends and determinants. Glob. Food Sec. 17, 21–29. doi: 10.1016/j.gfs.2018.03.004
Gold, M., Tomberlin, J. K., Diener, S., Zurbrügg, C., and Mathys, A. (2018). Decomposition of biowaste macronutrients, microbes, and chemicals in black soldier fly larval treatment: a review. Waste Manag. 82, 302–318. doi: 10.1016/j.wasman.2018.10.022
Green, J. M. H., Croft, S. A., Durán, A. P., Balmford, A. P., Burgess, N. D., Fick, S., et al. (2019). Linking global drivers of agricultural trade to on-the-ground impacts on biodiversity. Proc. Natl. Acad. Sci. U.S.A. 116, 23202–23208. doi: 10.1073/pnas.1905618116
Gregory, P. J., Wahbi, A., Adu-Gyamfi, J., Heiling, M., Gruber, R., Joy, E. J. M., et al. (2017). Approaches to reduce zinc and iron deficits in food systems. Glob. Food Sec. 15, 1–10. doi: 10.1016/j.gfs.2017.03.003
Groenestein, C. M., Hutchings, N. J., Haenel, H. D., Amon, B., Menzi, H., Mikkelsen, M. H., et al. (2019). Comparison of ammonia emissions related to nitrogen use efficiency of livestock production in Europe. J. Clean. Prod. 211, 1162–1170. doi: 10.1016/j.jclepro.2018.11.143
Gupta, S., Vemireddy, V., Singh, D. K., and Pingali, P. (2021). Ground truthing the cost of achieving the EAT lancet recommended diets: evidence from rural India. Glob. Food Sec. 28, 100498. doi: 10.1016/j.gfs.2021.100498
Hadjikakou, M. (2017). Trimming the excess: environmental impacts of discretionary food consumption in Australia. Ecol. Econ. 131, 119–128. doi: 10.1016/j.ecolecon.2016.08.006
Haider, L. M., Schwingshackl, L., Hoffmann, G., and Ekmekcioglu, C. (2018). The effect of vegetarian diets on iron status in adults: a systematic review and meta-analysis. Crit. Rev. Food Sci. Nutr. 58, 1359–1374. doi: 10.1080/10408398.2016.1259210
Hallström, E., Carlsson-Kanyama, A., and Börjesson, P. (2015). Environmental impact of dietary change: a systematic review. J. Clean. Prod. 91, 1–11. doi: 10.1016/j.jclepro.2014.12.008
Hasegawa, T., Fujimori, S., Havlík, P., Valin, H., Bodirsky, B. L., Doelman, J. C., et al. (2018). Risk of increased food insecurity under stringent global climate change mitigation policy. Nat. Clim. Chang. 8, 699–703. doi: 10.1038/s41558-018-0230-x
Hawkes, C., Fox, E., Downs, S. M., Fanzo, J., and Neve, K. (2020). Child-centered food systems: reorienting food systems towards healthy diets for children. Glob. Food Sec. 27, 100414. doi: 10.1016/j.gfs.2020.100414
He, P., Baiocchi, G., Hubacek, K., Feng, K., and Yu, Y. (2018). The environmental impacts of rapidly changing diets and their nutritional quality in China. Nat. Sustain. 1, 122–127. doi: 10.1038/s41893-018-0035-y
Headey, D. D., and Alderman, H. H. (2019). The relative caloric prices of healthy and unhealthy foods differ systematically across income levels and continents. J. Nutr. 149, 2020–2033. doi: 10.1093/jn/nxz158
Herforth, A., and Ahmed, S. (2015). The food environment, its effects on dietary consumption, and potential for measurement within agriculture-nutrition interventions. Food Secur. 7, 505–520. doi: 10.1007/s12571-015-0455-8
Herforth, A., Arimond, M., Álvarez-Sánchez, C., Coates, J., Christianson, K., and Muehlhoff, E. (2019). A global review of food-based dietary guidelines. Adv. Nutr. 10, 590–605. doi: 10.1093/advances/nmy130
Herforth, A., Bai, Y., Venkat, A., Mahrt, K., Ebel, A., and Masters, W. A. (2020). Cost and Affordability of Healthy Diets Across and Within Countries. Background Paper for the State of Food Security and Nutrition in the World 2020. Rome.
Hicks, C. C., Cohen, P. J., Graham, N. A. J., Nash, K. L., Allison, E. H., D'Lima, C., et al. (2019). Harnessing global fisheries to tackle micronutrient deficiencies. Nature 574, 95–98. doi: 10.1038/s41586-019-1592-6
Hilborn, R., Banobi, J., Hall, S. J., Pucylowski, T., and Walsworth, T. E. (2018). The environmental cost of animal source foods. Front. Ecol. Environ. 16, 329–335. doi: 10.1002/fee.1822
Hirvonen, K., Bai, Y., Headey, D., and Masters, W. A. (2019). Affordability of the EAT-Lancet reference diet: a global analysis. Lancet Glob. Heal. 8, e59–e66. doi: 10.1016/S2214-109X(19)30447-4
Hong, C., Burney, J. A., Pongratz, J., Nabel, J. E. M. S., Mueller, N. D., Jackson, R. B., et al. (2021). Global and regional drivers of land-use emissions in 1961-2017. Nature 589, 554–561. doi: 10.1038/s41586-020-03138-y
Howe, L. C., MacInnis, B., Krosnick, J. A., Markowitz, E. M., and Socolow, R. (2019). Acknowledging uncertainty impacts public acceptance of climate scientists' predictions. Nat. Clim. Chang. 9, 863–867. doi: 10.1038/s41558-019-0587-5
Institute of Medicine (2005). Dietary Reference Intakes for Energy, Carbohydrate, Fiber, Fat, Fatty Acids, Cholesterol, Protein, and Amino Acids (Macronutrients). Washington, DC: National Academies Press.
Jägermeyr, J. (2020). Agriculture's historic twin-challenge toward sustainable water use and food supply for all. Front. Sustain. Food Syst. 4, 35. doi: 10.3389/fsufs.2020.00035
Jalava, M., Kummu, M., Porkka, M., Siebert, S., and Varis, O. (2014). Diet change-a solution to reduce water use? Environ. Res. Lett. 9, 074016. doi: 10.1088/1748-9326/9/7/074016
Jin, S., Zhang, B., Wu, B., Han, D., Hu, Y., Ren, C., et al. (2021). Decoupling livestock and crop production at the household level in China. Nat. Sustain. 4, 48–55. doi: 10.1038/s41893-020-00596-0
Jones, A. D., Hoey, L., Blesh, J., Miller, L., Green, A., and Shapiro, L. F. (2016). A systematic review of the measurement of sustainable diets. Adv. Nutr. An Int. Rev. J. 7, 641–664. doi: 10.3945/an.115.011015
Joy, E. J. M., Ander, E. L., Young, S. D., Black, C. R., Watts, M. J., Chilimba, A. D. C., et al. (2014). Dietary mineral supplies in Africa. Physiol. Plant. 151, 208–229. doi: 10.1111/ppl.12144
Kastner, T., Chaudhary, A., Gingrich, S., Marques, A., Persson, U. M., Bidoglio, G., et al. (2021). Global agricultural trade and land system sustainability: implications for ecosystem carbon storage, biodiversity, and human nutrition. One Earth 4, 1425–1443. doi: 10.1016/j.oneear.2021.09.006
Kesse-Guyot, E., Chaltiel, D., Wang, J., Pointereau, P., Langevin, B., Allès, B., et al. (2020). Sustainability analysis of French dietary guidelines using multiple criteria. Nat. Sustain. 3, 377–385. doi: 10.1038/s41893-020-0495-8
Khatibzadeh, S., Kashaf, M. S., Micha, R., Fahimi, S., Shi, P., Elmadfa, I., et al. (2016). A global database of food and nutrient consumption. Bull. World Health Organ. 94, 931. doi: 10.2471/BLT.15.156323
Kim, B. F., Santo, R. E., Scatterday, A. P., Fry, J. P., Synk, C. M., Cebron, S. R., et al. (2019). Country-specific dietary shifts to mitigate climate and water crises. Glob. Environ. Chang. 62, 101926. doi: 10.1016/j.gloenvcha.2019.05.010
Kimani-Murage, E., Gaupp, F., Lal, R., Hansson, H., Tang, T., Chaudhary, A., et al. (2021). An optimal diet for planet and people. One Earth 4, 1189–1192. doi: 10.1016/j.oneear.2021.08.017
Kroeker, K. J., Kordas, R. L., Crim, R., Hendriks, I. E., Ramajo, L., Singh, G. S., et al. (2013). Impacts of ocean acidification on marine organisms: quantifying sensitivities and interaction with warming. Glob. Chang. Biol. 19, 1884–1896. doi: 10.1111/gcb.12179
Kumssa, D. B., Joy, E. J. M., Ander, E. L., Watts, M. J., Young, S. D., Walker, S., et al. (2015). Dietary calcium and zinc deficiency risks are decreasing but remain prevalent. Sci. Rep. 5, 10974. doi: 10.1038/srep10974
Lade, S. J., Steffen, W., de Vries, W., Carpenter, S. R., Donges, J. F., Gerten, D., et al. (2019). Human impacts on planetary boundaries amplified by Earth system interactions. Nat. Sustain. 3, 119–128. doi: 10.1038/s41893-019-0454-4
Lassaletta, L., Billen, G., Garnier, J., Bouwman, L., Velazquez, E., Mueller, N. D., et al. (2016). Nitrogen use in the global food system: past trends and future trajectories of agronomic performance, pollution, trade, and dietary demand. Environ. Res. Lett. 11, 095007. doi: 10.1088/1748-9326/11/9/095007
Lassen, A. D., Christensen, L. M., and Trolle, E. (2020). Development of a danish adapted healthy plant-based diet based on the EAT-lancet reference diet. Nutrients 12, 738. doi: 10.3390/nu12030738
Lazzarini, G. A., Visschers, V. H. M., and Siegrist, M. (2018). How to improve consumers' environmental sustainability judgements of foods. J. Clean. Prod. 198, 564–574. doi: 10.1016/j.jclepro.2018.07.033
Leclère, D., Obersteiner, M., Barrett, M., Butchart, S. H. M., Chaudhary, A., Palma, A., et al. (2020). Bending the curve of terrestrial biodiversity needs an integrated strategy. Nature 585, 551–556. doi: 10.1038/s41586-020-2705-y
Lelieveld, J., Evans, J. S., Fnais, M., Giannadaki, D., and Pozzer, A. (2015). The contribution of outdoor air pollution sources to premature mortality on a global scale. Nature 525, 367–371. doi: 10.1038/nature15371
Lenzen, M., Moran, D., Kanemoto, K., Foran, B., Lobefaro, L., and Geschke, A. (2012). International trade drives biodiversity threats in developing nations. Nature 486, 109–112. doi: 10.1038/nature11145
Li, M., Fan, Y., Zhang, X., Hou, W., and Tang, Z. (2014). Fruit and vegetable intake and risk of type 2 diabetes mellitus: meta-analysis of prospective cohort studies. BMJ Open 4, e005497. doi: 10.1136/bmjopen-2014-005497
Machovina, B., Feeley, K. J., and Ripple, W. J. (2015). Biodiversity conservation: the key is reducing meat consumption. Sci. Total Environ. 536, 419–431. doi: 10.1016/j.scitotenv.2015.07.022
Mair, L., Bennun, L. A., Brooks, T. M., Butchart, S. H. M., Bolam, F. C., Burgess, N. D., et al. (2021). A metric for spatially explicit contributions to science-based species targets. Nat. Ecol. Evol. 5, 836–844. doi: 10.1038/s41559-021-01432-0
Marteau, T. M. (2017). Towards environmentally sustainable human behaviour: Targeting non-conscious and conscious processes for effective and acceptable policies. Philos. Trans. R. Soc. A Math. Phys. Eng. Sci. 375, 20160371. doi: 10.1098/rsta.2016.0371
Mason-D'Croz, D., Bogard, J. R., Sulser, T. B., Cenacchi, N., Dunston, S., Herrero, M., et al. (2019a). Gaps between fruit and vegetable production, demand, and recommended consumption at global and national levels: an integrated modelling study. Lancet Planet. Heal. 3, e318–e329. doi: 10.1016/S2542-5196(19)30095-6
Mason-D'Croz, D., Sulser, T. B., Wiebe, K., Rosegrant, M. W., Lowder, S. K., Nin-Pratt, A., et al. (2019b). Agricultural investments and hunger in Africa modeling potential contributions to SDG2 - Zero Hunger. World Dev. 116, 38–53. doi: 10.1016/j.worlddev.2018.12.006
Mayhew, A. J., De Souza, R. J., Meyre, D., Anand, S. S., and Mente, A. (2016). A systematic review and meta-analysis of nut consumption and incident risk of CVD and all-cause mortality. Br. J. Nutr. 115, 212–225. doi: 10.1017/S0007114515004316
Mekonnen, M. M., and Hoekstra, A. Y. (2011). The green, blue and grey water footprint of crops and derived crop products. Hydrol. Earth Syst. Sci. 15, 1577–1600. doi: 10.5194/hess-15-1577-2011
Metson, G. S., Chaudhary, A., Zhang, X., Houlton, B., Oita, A., Raghuram, N., et al. (2021). Nitrogen and the food system. One Earth 4, 3–7. doi: 10.1016/j.oneear.2020.12.018
Miller, V., Webb, P., Micha, R., Mozaffarian, D., and Database, G. D. (2020). Defining diet quality: a synthesis of dietary quality metrics and their validity for the double burden of malnutrition. Lancet Planetary Health 4, e352–e370. doi: 10.1016/S2542-5196(20)30162-5
Miller, V., Yusuf, S., Chow, C. K., Dehghan, M., Corsi, D. J., Lock, K., et al. (2016). Availability, affordability, and consumption of fruits and vegetables in 18 countries across income levels: findings from the Prospective Urban Rural Epidemiology (PURE) study. Lancet Glob. Heal. 4, e695–e703. doi: 10.1016/S2214-109X(16)30186-3
Milner, J., Joy, E. J. M., Green, R., Harris, F., Aleksandrowicz, L., Agrawal, S., et al. (2017). Projected health effects of realistic dietary changes to address freshwater constraints in India: a modelling study. Lancet Planet. Heal. 1, e26–e32. doi: 10.1016/S2542-5196(17)30001-3
Moberg, E., Karlsson Potter, H., Wood, A., Hansson, P.-A., and Röös, E. (2020). Benchmarking the Swedish diet relative to global and national environmental targets-identification of indicator limitations and data gaps. Sustainability 12, 1407. doi: 10.3390/su12041407
Mueller, N. D., Gerber, J. S., Johnston, M., Ray, D. K., Ramankutty, N., and Foley, J. A. (2012). Closing yield gaps through nutrient and water management. Nature 490, 254–257. doi: 10.1038/nature11420
Myers, S. S., Zanobetti, A., Kloog, I., Huybers, P., Leakey, A. D. B., Bloom, A. J., et al. (2014). Increasing CO2 threatens human nutrition. Nature 510, 139–142. doi: 10.1038/nature13179
Naylor, R. L., Hardy, R. W., Buschmann, A. H., Bush, S. R., Cao, L., Klinger, D. H., et al. (2021). A 20-year retrospective review of global aquaculture. Nature 591, 551–563. doi: 10.1038/s41586-021-03308-6
Obirikorang, K. A., Sekey, W., Gyampoh, B. A., Ashiagbor, G., and Asante, W. (2021). Aquaponics for improved food security in Africa: A review. Front. Sustain. Food Syst. 5, 705549. doi: 10.3389/fsufs.2021.705549
Ofori, S. A., Cobbina, S. J., and Obiri, S. (2021). Climate change, land, water, and food security: perspectives from Sub-Saharan Africa. Front. Sustain. Food Syst. 5, 680924. doi: 10.3389/fsufs.2021.680924
Olsho, L. E., Klerman, J. A., Wilde, P. E., and Bartlett, S. (2016). Financial incentives increase fruit and vegetable intake among Supplemental Nutrition Assistance Program participants: a randomized controlled trial of the USDA Healthy Incentives Pilot. Am. J. Clin. Nutr. 104, 423–435. doi: 10.3945/ajcn.115.129320
Orlich, M. J., Singh, P. N., Sabaté, J., Jaceldo-Siegl, K., Fan, J., Knutsen, S., et al. (2013). Vegetarian dietary patterns and mortality in adventist health study 2. JAMA Intern. Med. 173, 1230–1238. doi: 10.1001/jamainternmed.2013.6473
Pahlow, M., van Oel, P. R., Mekonnen, M. M., and Hoekstra, A. Y. (2015). Increasing pressure on freshwater resources due to terrestrial feed ingredients for aquaculture production. Sci. Total Environ. 536, 847–857. doi: 10.1016/j.scitotenv.2015.07.124
Pastor, A. V., Palazzo, A., Havlik, P., Biemans, H., Wada, Y., Obersteiner, M., et al. (2019). The global nexus of food-trade-water sustaining environmental flows by 2050. Nat. Sustain. 2, 499–507. doi: 10.1038/s41893-019-0287-1
Payne, C. L., Scarborough, P., and Cobiac, L. (2016). Do low-carbon-emission diets lead to higher nutritional quality and positive health outcomes? A systematic review of the literature. Public Health Nutr. 19, 2654–2661. doi: 10.1017/S1368980016000495
Penne, T., and Goedemé, T. (2020). Can low-income households afford a healthy diet? Insufficient income as a driver of food insecurity in Europe. Food Policy 99, 101978. doi: 10.1016/j.foodpol.2020.101978
Perignon, M., Sinfort, C., El Ati, J., Traissac, P., Drogué, S., Darmon, N., et al. (2019). How to meet nutritional recommendations and reduce diet environmental impact in the Mediterranean region? An optimization study to identify more sustainable diets in Tunisia. Glob. Food Sec. 23, 227–235. doi: 10.1016/j.gfs.2019.07.006
Pieper, M., Michalke, A., and Gaugler, T. (2020). Calculation of external climate costs for food highlights inadequate pricing of animal products. Nat. Commun. 11, 1–13. doi: 10.1038/s41467-020-19474-6
Poore, J., and Nemecek, T. (2018). Reducing food's environmental impacts through producers and consumers. Science 360, 987–992. doi: 10.1126/science.aaq0216
Popkin, B. M., Corvalan, C., and Grummer-Strawn, L. M. (2020). Dynamics of the double burden of malnutrition and the changing nutrition reality. Lancet 395, 65–74. doi: 10.1016/S0140-6736(19)32497-3
Pradhan, P., Costa, L., Rybski, D., Lucht, W., and Kropp, J. P. (2017). A systematic study of sustainable development goal (SDG) interactions. Earth's Future 5, 1169–1179. doi: 10.1002/2017EF000632
Raghunathan, K., Headey, D., and Herforth, A. (2020). Affordability of nutritious diets in rural India. Food Policy 99, 101982. doi: 10.2499/p15738coll2.133638
Ramesh, R., Kalin, L., Hantush, M., and Chaudhary, A. (2021). A secondary assessment of sediment trapping effectiveness by vegetated buffers. Ecol. Eng. 159, 106094. doi: 10.1016/j.ecoleng.2020.106094
Rao, M., Afshin, A., Singh, G., and Mozaffarian, D. (2013). Do healthier foods and diet patterns cost more than less healthy options? A systematic review and meta-analysis. BMJ Open 3, e004277. doi: 10.1136/bmjopen-2013-004277
Reynolds, C. J., Horgan, G. W., Whybrow, S., and Macdiarmid, J. I. (2019). Healthy and sustainable diets that meet greenhouse gas emission reduction targets and are affordable for different income groups in the UK. Public Health Nutr. 22, 1503–1517. doi: 10.1017/S1368980018003774
Ridoutt, B., Anastasiou, K., Baird, D., Garcia, J. N., and Hendrie, G. (2020). Cropland footprints of australian dietary choices. Nutrients 12, 1212. doi: 10.3390/nu12051212
Ridoutt, B. G., Hendrie, G. A., and Noakes, M. (2017). Dietary strategies to reduce environmental impact: a critical review of the evidence base. Adv. Nutr. 8, 933–946. doi: 10.3945/an.117.016691
Ritchie, H., Reay, D. S., and Higgins, P. (2018). The impact of global dietary guidelines on climate change. Glob. Environ. Chang. 49, 46–55. doi: 10.1016/j.gloenvcha.2018.02.005
Roberts, D. P., and Mattoo, A. K. (2019). Sustainable crop production systems and human nutrition. Front. Sustain. Food Syst. 3, 72. doi: 10.3389/fsufs.2019.00072
Rockström, J., Gaffney, O., Rogelj, J., Meinshausen, M., Nakicenovic, N., and Schellnhuber, H. J. (2017). A roadmap for rapid decarbonization. Science 355, 1269–1271. doi: 10.1126/science.aah3443
Rosa, L., Chiarelli, D. D., Tu, C., Rulli, M. C., and D'Odorico, P. (2019). Global unsustainable virtual water flows in agricultural trade. Environ. Res. Lett. 14, 114001. doi: 10.1088/1748-9326/ab4bfc
Rosa, L., Rulli, M. C., Davis, K. F., Chiarelli, D. D., Passera, C., and D'Odorico, P. (2018). Closing the yield gap while ensuring water sustainability. Environ. Res. Lett. 13, 104002. doi: 10.1088/1748-9326/aadeef
Rothrock, M. J. Jr., Gibson, K. E., Micciche, A. C., and Ricke, S. C. (2019). Pastured poultry production in the United States: Strategies to balance system sustainability and environmental impact. Front. Sustain. Food Syst. 3, 74. doi: 10.3389/fsufs.2019.00074
Rust, N. A., Ridding, L., Ward, C., Clark, B., Kehoe, L., Dora, M., et al. (2020). How to transition to reduced-meat diets that benefit people and the planet. Sci. Total Environ. 718, 137208. doi: 10.1016/j.scitotenv.2020.137208
Satija, A., Bhupathiraju, S. N., Rimm, E. B., Spiegelman, D., Chiuve, S. E., Borgi, L., et al. (2016). Plant-based dietary patterns and incidence of type 2 diabetes in US men and women: results from three prospective cohort studies. PLoS Med. 13, e1002039. doi: 10.1371/journal.pmed.1002039
Scheelbeek, P. F. D., Bird, F. A., Tuomisto, H. L., Green, R., Harris, F. B., Joy, E. J. M., et al. (2018). Effect of environmental changes on vegetable and legume yields and nutritional quality. Proc. Natl. Acad. Sci. U.S.A. 115, 6804–6809. doi: 10.1073/pnas.1800442115
Scheelbeek, P. F. D., Moss, C., Kastner, T., Alae-Carew, C., Jarmul, S., Green, R., et al. (2020). United Kingdom's fruit and vegetable supply is increasingly dependent on imports from climate-vulnerable producing countries. Nat. Food 1, 705–712. doi: 10.1038/s43016-020-00179-4
Sharma, M., Kishore, A., Roy, D., and Joshi, K. (2020). A comparison of the Indian diet with the EAT-Lancet reference diet. BMC Public Health 20, 812. doi: 10.1186/s12889-020-08951-8
Siegrist, M., and Hartmann, C. (2019). Impact of sustainability perception on consumption of organic meat and meat substitutes. Appetite 132, 196–202. doi: 10.1016/j.appet.2018.09.016
Smith, M. R., Micha, R., Golden, C. D., Mozaffarian, D., and Myers, S. S. (2016). Global expanded nutrient supply (GENuS) model: a new method for estimating the global dietary supply of nutrients. PLoS ONE 11, e0146976. doi: 10.1371/journal.pone.0146976
Song, G., Li, M., Fullana-i-Palmer, P., Williamson, D., and Wang, Y. (2017). Dietary changes to mitigate climate change and benefit public health in China. Sci. Total Environ. 577, 289–298. doi: 10.1016/j.scitotenv.2016.10.184
Springmann, M., Clark, M., Mason-D'Croz, D., Wiebe, K., Bodirsky, B. L., Lassaletta, L., et al. (2018a). Options for keeping the food system within environmental limits. Nature 562, 519–525. doi: 10.1038/s41586-018-0594-0
Springmann, M., Godfray, H. C. J., Rayner, M., and Scarborough, P. (2016). Analysis and valuation of the health and climate change cobenefits of dietary change. Proc. Natl. Acad. Sci. U.S.A. 113, 4146–4151. doi: 10.1073/pnas.1523119113
Springmann, M., Mason-D'Croz, D., Robinson, S., Wiebe, K., Godfray, H. C. J., Rayner, M., et al. (2017). Mitigation potential and global health impacts from food emission pricing. Nat. Clim. Chang. 7, 69–74. doi: 10.1038/nclimate3155
Springmann, M., Spajic, L., Clark, M. A., Poore, J., Herforth, A., Webb, P., et al. (2020). The healthiness and sustainability of national and global food based dietary guidelines: modelling study. BMJ 370, m2322. doi: 10.1136/bmj.m2322
Springmann, M., Wiebe, K., Mason-D'Croz, D., Sulser, T. B., Rayner, M., and Scarborough, P. (2018b). Health and nutritional aspects of sustainable diet strategies and their association with environmental impacts: a global modelling analysis with country-level detail. Lancet Planet. Heal. 2, e451–e461. doi: 10.1016/S2542-5196(18)30206-7
Steffen, W., Richardson, K., Rockström, J., Cornell, S. E., Fetzer, I., Bennett, E. M., et al. (2015). Planetary boundaries: guiding human development on a changing planet. Science 347, 1259855. doi: 10.1126/science.1259855
Swinburn, B. A., Kraak, V. I., Allender, S., Atkins, V. J., Baker, P. I., Bogard, J. R., et al. (2019). The global syndemic of obesity, undernutrition, and climate change: the Lancet Commission report. Lancet 393, 791–846. doi: 10.1016/S0140-6736(18)32822-8
The Economic Intelligence Unit (2020). Global Food Security Index (GFSI). Available online at: https://foodsecurityindex.eiu.com/ (accessed August 5, 2021).
Tilman, D., and Clark, M. (2014). Global diets link environmental sustainability and human health. Nature 515, 518–522. doi: 10.1038/nature13959
Tilman, D., Clark, M., Williams, D. R., Kimmel, K., Polasky, S., and Packer, C. (2017). Future threats to biodiversity and pathways to their prevention. Nature 546, 73–81. doi: 10.1038/nature22900
Turner, C., Aggarwal, A., Walls, H., Herforth, A., Drewnowski, A., Coates, J., et al. (2018). Concepts and critical perspectives for food environment research: a global framework with implications for action in low- and middle-income countries. Glob. Food Sec. 18, 93–101. doi: 10.1016/j.gfs.2018.08.003
United Nations (2015). Transforming Our World: The 2030 Agenda for Sustainable Development. Report No. A/RES/70/1. New York, NY.
United Nations (2021). Global Indicator Framework for the Sustainable Development Goals and Targets of the 2030 Agenda for Sustainable Development. New York.
Vågsholm, I., Arzoomand, N. S., and Boqvist, S. (2020). Food security, safety, and sustainability-getting the trade-offs right. Front. Sustain. Food Syst. 4, 16. doi: 10.3389/fsufs.2020.00016
van Valkengoed, A. M., and Steg, L. (2019). Meta-analyses of factors motivating climate change adaptation behaviour. Nat. Clim. Chang. 9, 158–163. doi: 10.1038/s41558-018-0371-y
van Wijk, M. T., Merbold, L., Hammond, J., and Butterbach-Bahl, K. (2020). Improving assessments of the three pillars of climate smart agriculture: Current achievements and ideas for the future. Front. Sustain. Food Syst. 4, 558483. doi: 10.3389/fsufs.2020.558483
Vandevijvere, S., Seck, M., Pedroni, C., De Ridder, K., and Castetbon, K. (2020). Food cost and adherence to guidelines for healthy diets: evidence from Belgium. Eur. J. Clin. Nutr. 75, 1142–1151. doi: 10.1038/s41430-020-00815-z
Vanham, D., Hoekstra, A. Y., and Bidoglio, G. (2013). Potential water saving through changes in European diets. Environ. Int. 61, 45–56. doi: 10.1016/j.envint.2013.09.011
Vanham, D., Leip, A., Galli, A., Kastner, T., Bruckner, M., Uwizeye, A., et al. (2019). Environmental footprint family to address local to planetary sustainability and deliver on the SDGs. Sci. Total Environ. 693, 133642. doi: 10.1016/j.scitotenv.2019.133642
Vanham, D., and Mekonnen, M. M. (2021). The scarcity-weighted water footprint provides unreliable water sustainability scoring. Sci. Total Environ. 756, 143992. doi: 10.1016/j.scitotenv.2020.143992
Vermeulen, S. J., Campbell, B. M., and Ingram, J. S. I. (2012). Climate change and food systems. Annu. Rev. Environ. Resour. 37, 195–222. doi: 10.1146/annurev-environ-020411-130608
Wallin, A., Di Giuseppe, D., Orsini, N., Patel, P. S., Forouhi, N. G., and Wolk, A. (2012). Fish consumption, dietary long-chain n-3 fatty acids, and risk of type 2 diabetes: systematic review and meta-analysis of prospective studies. Diabetes Care 35, 918–929. doi: 10.2337/dc11-1631
Wang, D. D., Li, Y., Afshin, A., Springmann, M., Mozaffarian, D., Stampfer, M. J., et al. (2019). Global improvement in dietary quality could lead to substantial reduction in premature death. J. Nutr. 149, 1065–1074. doi: 10.1093/jn/nxz010
Wang, X., Ouyang, Y., Liu, J., Zhu, M., Zhao, G., Bao, W., et al. (2014). Fruit and vegetable consumption and mortality from all causes, cardiovascular disease, and cancer: systematic review and dose-response meta-analysis of prospective cohort studies. BMJ 349, g4490. doi: 10.1136/bmj.g4490
Waterlander, W. E., Jiang, Y., Nghiem, N., Eyles, H., Wilson, N., Cleghorn, C., et al. (2019). The effect of food price changes on consumer purchases: a randomised experiment. Lancet Public Heal. 4, e394–e405. doi: 10.1016/S2468-2667(19)30105-7
WHO (1998). Preparation and Use of Food-Based Dietary Guidelines: Report of a Joint FAO/WHO Consultation. World Health Organization. Geneva.
WHO (2003). Diet, Nutrition, and the Prevention of Chronic Diseases: Report of a Joint WHO/FAO Expert Consultation. Geneva.
WHO (2020). Healthy Diet. World Health Organization. Available online at: https://www.who.int/en/news-room/fact-sheets/detail/healthy-diet (accessed August 5, 2021).
Willett, W., Rockström, J., Loken, B., Springmann, M., Lang, T., Vermeulen, S., et al. (2019). Food in the anthropocene: the EAT-Lancet Commission on healthy diets from sustainable food systems. Lancet 393, 447–492. doi: 10.1016/S0140-6736(18)31788-4
Williams, D. R., Clark, M., Buchanan, G. M., Ficetola, G. F., Rondinini, C., and Tilman, D. (2020). Proactive conservation to prevent habitat losses to agricultural expansion. Nat. Sustain. 4, 314–322. doi: 10.1038/s41893-020-00656-5
Wilson, N., Nghiem, N., Ni Mhurchu, C., Eyles, H., Baker, M. G., and Blakely, T. (2013). Foods and dietary patterns that are healthy, low-cost, and environmentally sustainable: a case study of optimization modeling for New Zealand. PLoS ONE 8, e59648. doi: 10.1371/journal.pone.0059648
Wu, X., Fu, B., Wang, S., Li, Y., Xu, Z., and Liu, J. (2022). Decoupling of SDGs followed by re-coupling as sustainable development progresses. Nat. Sustain. 5, 452–459. doi: 10.1038/s41893-022-00868-x
Zagmutt, F. J., Pouzou, J. G., and Costard, S. (2020). The EAT-Lancet Commission's dietary composition may not prevent noncommunicable disease mortality. J. Nutr. 150, 985–988. doi: 10.1093/jn/nxaa020
Keywords: dietary change, environment, sustainable development goals (SDGs), climate-change, malnutrition
Citation: Chen C, Chaudhary A and Mathys A (2022) Dietary Change and Global Sustainable Development Goals. Front. Sustain. Food Syst. 6:771041. doi: 10.3389/fsufs.2022.771041
Received: 05 September 2021; Accepted: 06 June 2022;
Published: 08 July 2022.
Edited by:
Kathleen L. Hefferon, Cornell University, United StatesReviewed by:
Xuan Li, Food and Agriculture Organization of the United Nations, ThailandAnna Kiss, Hungarian Academy of Sciences (MTA), Hungary
Copyright © 2022 Chen, Chaudhary and Mathys. This is an open-access article distributed under the terms of the Creative Commons Attribution License (CC BY). The use, distribution or reproduction in other forums is permitted, provided the original author(s) and the copyright owner(s) are credited and that the original publication in this journal is cited, in accordance with accepted academic practice. No use, distribution or reproduction is permitted which does not comply with these terms.
*Correspondence: Abhishek Chaudhary, YWJoaXNoZWtjJiN4MDAwNDA7aWl0ay5hYy5pbg==