- Wageningen Food & Biobased Research, Wageningen, Netherlands
During storage, cereals and legumes are vulnerable to insects, rodents, and fungi, which can cause loss of weight, damage or discoloration of products, and/or toxin formation. Hermetic bags can prevent excessive insect infestation, and toxin formation. This paper presents an analysis of the effects of hermetic bags for the storage of maize on food loss reduction by insects and on net greenhouse gas (GHG) emissions, based on practical data from field trials. Their economic feasibility, by optimizing the total income in markets with different price seasonalities in different Sub-Saharan African countries, is analyzed. The data of five field trials were combined and put in classes of 50 days of increasing storage time to get a realistic loss of produce during storage using regular bags (with or without the use of pesticides) and hermetic bags. The maize for storage trials were used as is, bought locally or used direct from the field. Scenario studies with standard storage, standard storage combined with pesticides and hermetic bag storage show significant losses by insects after 100 storage days for standard and standard with pesticides storage, whereas with hermetic bags, product losses are kept to a minimum of 2%. The economic analysis shows less clear-cut outcomes: the interventions' effectiveness depends largely on the rate of seasonal price fluctuation of the commodity. For farmers' personal consumption, when the quality is less critical, the use of hermetic bags is only more economical compared with other methods of storage for produce kept over 100 days. Since the quality of maize is well-preserved by the hermetic bag, the return on the investment is shorter when the maize is sold at the market. However, for countries with a low seasonal price gap, the investment cannot be recouped. As the use of hermetic bags is a good intervention for preventing food loss, it is best promoted not only for providing direct profits to farmers but also for health benefits, as bag use implies a lower need for pesticides and a possible reduction in aflatoxin intake.
Introduction
Post-harvest losses (PHL) of dry food crops are a large problem in the Sub-Saharan region, especially for smallholder farmers. Globally, more than 500 million smallholder farmers grow crops on <10 hectares of land, with most of them located in developing countries (FAO, 2014). Most of the dry food crops (80%) in Sub-Saharan Africa are produced by smallholders. Post-harvest losses by smallholder farmers can be divided into three categories: in the field, during processing, and during storage (Abass et al., 2014). During storage, insect infestation is a significant cause of losses in developing countries (Kamanula et al., 2010; Ndiso et al., 2017). For instance, 11% of maize is lost due to weevil infestation in Ghana (Nabila and Kumah, 2018), which is almost half of the total post-harvest loss reported. In Kenya, comparable results are recorded for maize losses due to insects (Ognakossan et al., 2016).
Weevils, grain borers, red flour beetles and moths are the most common infestation insects (Manandhar et al., 2018), of which the larger grain borer (LGB) has had an increasing impact on PHL. The first outbreak in African countries was in the 1970s and resulting losses have been increasing since then. One report found that some products' weights have reduced by 34%, with 80% of the grain damaged by the LGB (Boxall, 2002).
The problem persists if the crop is planted or stored near old granaries, which is the case with most of Africa's smallholder farmers. The infestation can easily move to and from storage sites. Moreover, using the same bins year after year without proper hygiene measures provides a continuous chain of infestation. Insects can hibernate or even continue to feed on the wooden structures of storage facilities or can hide between holes and cracks in the walls. They can then re-infest the new crop in the same storage facility and resume feeding (Sallam, 1999).
The storage of smallholder farms is still done in traditional canaries or more often in polypropylene (PP) woven bags. To reduce insect-induced PHL, farmers can use insecticides and/or improve their farms' storage facilities. One possibility is the introduction of hermetic storage facilities, such as metal silos, plastic drums or hermetic bags. Hermetic bags require relatively low capital investment, suiting smallholder farmers' capabilities.
The mechanism for reducing storage loss with a hermetic storage system involves reducing the oxygen and increasing the carbon dioxide levels inside the system. This controls the activity and the number of live insects in the product. The oxygen reduction is partly caused by the oxygen use of the insect themselves (Murdock et al., 2012; Njoroge et al., 2018), oxidation of the product, and some fungi growth (H. De Groote et al., 2013). Insecticides appear less effective than using appropriate bags (Kimenju and Groote, 2010); however, they are commonly used despite the health risks associated with them (De Bon et al., 2014).
For smallholder farmers, hermetic bags are the simplest low-cost way to make a hermitic storage facility. A few different types of bags have been tested in the field, such as the Supergrainbags (GrainPro Inc. of Concord, Massachusetts, USA) and the Purdue Improved Crop Storage (PICS) bags (Bern et al., 2013). The latter has been introduced on a large scale, and has been promoted and supported by the Bill & Melinda Gates Foundation. Although the Supergrainbags have a higher barrier for oxygen, the resulting oxygen levels for both systems are comparable as well as their insect infestation reduction performance during storage (Baoua et al., 2013). According to Baoua et al., (Baoua et al., 2013) PICS bags have the advantages of lower cost, wider accessibility, and greater durability. Therefore, the PICS bags can be used for multiple seasons, effectively lowering their cost per unit stored substantially. A PICS bag consists of three layers: two liners of 80 μm high density polyethylene plastic (HDPE) fitted inside a woven sack. The two liners form the oxygen and moisture barrier between the bag and the environment. A third, woven PP layer provides the mechanical strength. Besides the reduction in insect activity, the hermetic bags can also reduce the growth of fungi and the production of aflatoxin, especially when the grain is not completely dry at the start of the storage period (Busso et al., 2016; Tubbs et al., 2016). The working mechanism is also based on reducing the oxygen in the sealed bag. Fungi respiration in maize with 18% or 20% moisture can reduce the oxygen level to almost zero within an hour.
The rollout of an intervention is most easy in the case of a positive business scenario in which the required investments balance the economic benefit of the intervention. In Sub-Saharan Africa, smallholder farmers produce a median of 730 kg maize/farm. A total of 49% of smallholder farmers sells their maize, and a total of 23% of all maize is sold (Jirström et al., 2018). Besides the direct need for cash, the risk of economic devaluation over time can be a large driver for farmers to sell their products directly after harvest. The commonest argument heard to convince farmers to adopt hermetic bags is that they can ensure the safe storage of grain without economic devaluation, and therefore gain more money for their crops as they can sell them later in the season when the prices are higher than during the harvest period (NCBA CLUSA, 2016). The second benefit for the farmers is related to health issues, as the use of pesticides can be reduced and the development of mycotoxin in the maize can be avoided (Ng'ang'a et al., 2016a).
This study focuses on the first argument in order to estimate the additional profit that a farmer can expect to gain by using hermetic bags. This is calculated by taking into account the PHL, market price and quality discounts. In formal trade, national or regional authorities or trading organizations dictate quality grades. Batches with defect percentages above predefined thresholds will be rejected or discounted, resulting in large economic and product loss. Jones et al. (Jones et al., 2018) concluded that beans with levels of 5–10% insect damage can generally be sold with a moderate discount, whereas beans with over 20% insect damage are largely unmarketable. Rejected batches may still be used for farmers' personal consumption or animal feed.
Both arguments are also convincing on a wider environmental level; for one, reducing PHL can contribute in turn to the reduction in greenhouse gas (GHG) emissions. PHL contribute to GHG emissions due to the emissions associated with agricultural production and post-harvest operations. Consequently, reducing PHL may be an efficient strategy for the reduction in food-related GHG emissions. However, PHL reduction interventions also induce additional emissions. Adequate decision making on PHL-reducing interventions should therefore take into consideration their net effect on GHG emissions. Analyzing this trade-off is essential to improve in a nett positive direction (Vagsholm et al., 2020).
The aim of this work is to analyse whether introducing hermetic bags for a relatively low-cost commodity crop such as maize leads to increases in farmers' incomes and food security, and, more widely, a positive effect on total GHG emissions. The study will not analyse the possible health effects. The net effectiveness of the interventions will be based on realistic estimates of the hermetic bags' performance, which will be compared with standard woven polypropylene (PP) bags, with and without the use of insecticides. Furthermore, the influence of maize price and country dependent seasonal price variations on economic benefits for the farmers are analyzed.
Methods and Data
Postharvest Loses
The results of different field trials for maize were used to estimate the benefits of the hermetic bags for food security, GHG emissions and the economic outlook in different Sub-Saharan countries. The average effects of hermetic bags and the use of insecticides on PHL reduction during field trials involving stored maize grain were calculated based on data from five different field studies of the storage of maize in Sub-Saharan Africa: Baoua et al. (2014); Likhayo et al. (2016); Ndegwa et al. (2016); Ng'ang'a et al. (2016b), and Mlambo et al. (2017). Table 1 shows some of the characteristics of these studies. These data were used to determine the PHL, quality and economic value of the maize during storage. In these studies, the weight loss measurements were mostly done indirectly, since weighing the total stored grain is impossible due to sampling and the use of the stored grains for consumption. Therefore, to calculate weight losses, indirect methods were used in the used studies (see Table 1), count and weight, or 100 gram weight describe by (Harris and Lindblad, 1978; Boxall, 1986), and (Bbosa et al., 2017).

Table 1. Studies used for the estimation of PHL during field trials of stored maize grain in Sub-Saharan African countries.
The experimental setup differs between the studies but had at least two things in common to be able to extrapolate the results to normal practice. In contrast to lab experiments the maize was not disinfected and contaminated with specific number of insects prior to the test. So the maize has at the start of the trail a natural fluctuating contamination. Secondly, the maize was stored locally and under local conditions.
To be able to use the data for the calculation of the GHG emission as well as the economic evaluation the PHL the data were combined in classes of 50 storage days, as the lowest storage time was 49 days, starts the first class here. The last class is numbers of storage days above 200 as there were only a limited number of data points between 250 and 300 days.
GHG Emissions Analysis
The effect of PHL on GHG emissions was determined with the use of the Agro-Chain Greenhouse Gas Emissions (ACGE) calculator (Broeze et al., 2019). Next, the GHG emissions of the primary production and storage solutions used by the studies referenced in Table 1 were used as inputs.
The primary production of maize in Sub-Saharan Africa has a GHG emissivity of 1.56 kg CO2-equivalent per kg harvested maize (Porter et al., 2016). We focused on production for local markets, therefore, transport is neglected. Energy use for drying is also neglected, and the use of sun-drying is assumed when needed. In cases where product losses occur during the production, collection or drying stages, they are equal for the three scenarios; therefore, those losses were not taken into account. The storage bags have a capacity of 90 kilograms, dimensions of 1,370 x 700 mm, and can be used for 3 years/cycle. Standard bags and the outer layer of PICS bags are made of one layer of PP of 100 μm thickness, with a 919 kg/m3 density. The PICS bags' inner layers consist of two layers of HDPE of 80 μm thickness, with a 955 kg/m3 density. The pesticide assumed was Actellic 50CE, dosed at 8 ml/MT. GHG emissions related to pesticide production were estimated at 9 kg CO2-eq. per kg Actellic 50CE (Heimpel et al., 2013). The resulting GHG emissions in CO2-eq. were outcomes of the ACGE calculator.
Economic Evaluation: In-house Price and Market Price
In the economic evaluation, two scenarios were calculated for the products: home use, and their sale at local markets for the current market price. The value of the maize at the beginning of storage was calculated as the market price at the harvest period in addition to the costs of the storage system. This is referred to as “In-house price” in USD/kg.
During storage, products will be consumed by insects and may loss or gain moisture, which changes the calculated in-house price in time. For home use, it was assumed that only the weight loss over time increases the in-house price as the number of kilograms decreases. For the weight loss, the weighted data of the studies from Table 1 are taken and aggregated into different storage periods. The quality lost in damaged grain, molds, or the production of mycotoxins are not taken into account. However, when the farmers need to buy from the local market, the maize they purchase will be of a good quality, whereas when they use their own maize, it can be of a lower quality.
In the second scenario, the maize is sold at a local market. For this scenario, weight losses as well as negative effects on the market price due to the lower quality of the maize being sold (Table 3) were taken into account.
The maize samples were graded with a quality classification system based on the fraction of damaged grains, and were classified as either a good product (<10% damage), poor quality product (10–20% damage) or unmarketable for human consumption product (>20% damage). To determine the quality lost, the amount of damaged grain with the standard deviation of every point was used taken from the five field studies. Assuming a normal deviation of the data points, the exceedance chances of 10 and 20% damaged grain were calculated. All the chances of exceedance were added in a weighted form to determine the average amount of exceedance in a certain period.
Only discounts for damaged grain were taken into account. Moldy grain contamination, which can also have a price reduction effect when it exceeds 5% (Jones et al., 2016), and any health effects of improved quality, e.g., aflatoxin reduction, were not taken into account.
The market price of maize fluctuates during the year depending on supply and demand. During harvest time, the price drops and normally increases again until the next harvest. This price fluctuation is called the seasonal gap and is the main driving force behind the storage of grain. A high seasonal gap makes storage more profitable. This seasonal gap, however, differs for every country (Gilbert et al., 2017; Kornher, 2018). For the economic cost and benefit analysis, three different seasonal patterns that exist in Africa were evaluated, represented by Uganda, Malawi and Zambia. Malawi has a high seasonal gap, Uganda harvests twice a year and has a moderate seasonal gap, and Zambia records small variations in price during the year. The typical price fluctuations in the three countries are given in Figure 1. The average yearly market price of 30 $c/kg Maize was considered the same for every country. The penalties due to 10 and 20% damaged grain were combined with the exceedances and the market price at that period to obtain an average sales price for the three selected countries.
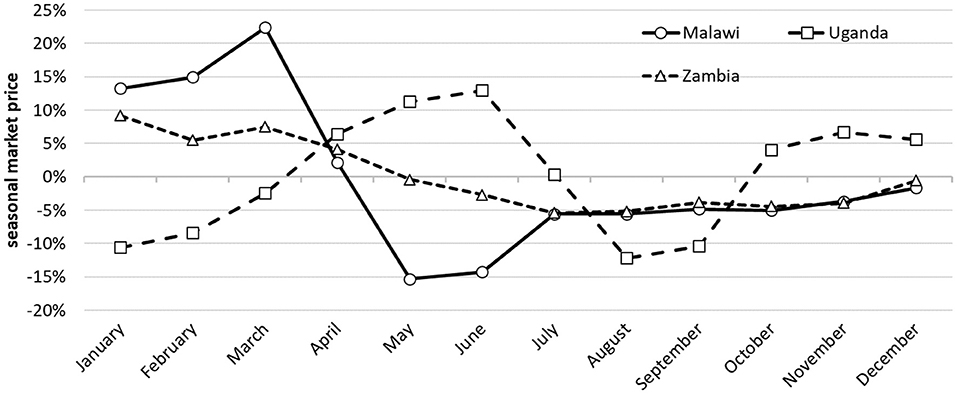
Figure 1. Seasonal price fluctuations of maize, below and above the average year price (30 $c/kg maize) in different countries, data from (Gilbert et al., 2017; Kornher, 2018).
Results
PHL Effect on Food Security
Figure 2 shows the weight losses over time for maize stored in standard bags, hermetic bags, and for when pesticides are used. Through hermetic storage technology, average weight losses of grain were found to be significantly (LDS 5%) reduced between 49 and 99 days of storage (standard bag 4.2 ± 0.3%, hermetic bag 1.2 ± 0.5%),ensuring the increased availability of food-grade grain throughout the year. Table 2 show that hermetic bags can help to reduce losses caused by insects and/or evaporation till an average loss of 1.5 ± 0.4% after more than 200 day of storage. In the reference situation (with polypropylene (PP) woven or jute bags), the average weight losses for small farmers' stored maize at the end of the storage season (>200 days) rose to 36.0 ± 6.2 %. The use of pesticide was also, on average, beneficial compared to the standaard bag, between 49 and 99 days of storage 2.5 ± 0.5% weight loss, and after more than 200 days of storage 12.9 ± 3.8%. Especially for longer storage times, the results of the individual measurements for the standard bag and even more for the use of pesticides were very scattered. And although the average weight loss of the hermetic bag was lower for both the normal bag and the use of pesticides after a storage period of 49 days, data points below the average of the hermetic bag in the same period can still be found, in 6% and 41% for resp. the normal bag and the use of pesticides.
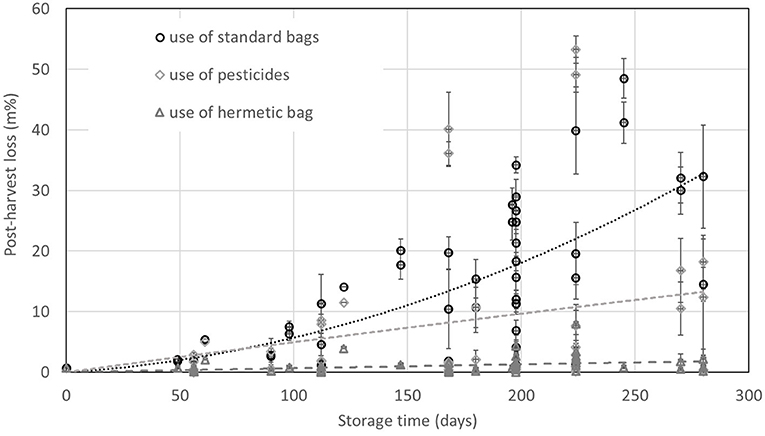
Figure 2. Post-harvest weight loss in % in different storage scenarios [standard bags (circles), standard bags combined with pesticides (diamonds) and hermetic bags (crosses)] in field trials of maize storage, based on data from Likhayo et al. (2016), Ndegwa et al. (2016), Mlambo et al. (2017), Ng'ang'a et al. (2016b), and Baoua et al. (2014). The points are averages from a number of different samples, error bar represent the standard deviation. The regression lines are corrected for the underlying number of samples of the points.
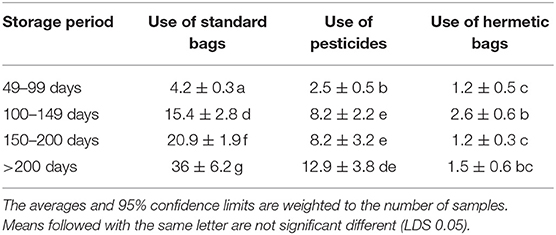
Table 2. Post-harvest average weight loss in % of different storage periods in different storage scenarios in field trials of maize storage.
Depending on the storage location the moisture content of maize stored in the PP or Jute bags could change. Up to 2.5%wb loss and 3.5%wb increase was reported, However, the moisture content did not increase above 14%wb. Maize stored in the Hermetic bags did not show any change in moisture content.
PHL Effect on GHG Emissions
The effect of using any measure to reduce the impact of PHL on GHG emissions required taking into account two aspects. Firstly, any GHG emissions related to the production of the bags and pesticides must be taken into account, which contributes to the GHG emissions associated with the commodity. In the case of the hermetic bags, the PICS bags were taken for GHG calculations. The two HDPE liners of the PICS bag attribute additional GHG emissions compared with a traditional woven polypropylene bag. Secondly, the lost produce must be taken into account, as it increases the GHG emissions per kilogram of maize available for consumption. The impact on GHG emissions was calculated for three scenarios: storage in standard bags, storage in hermetic bags, or in-bag treatment with a pesticide in combination with storage in standard bags. Table 3 gives the GHG emissions for the packaging systems used in the three scenarios. The impact of the packaging system was <0.1% compared with the impact of the primary production per kilogram of maize.

Table 3. GHG emissions for packaging (90 kg) for the different scenarios (kg CO2-eq. emissions per kg food-grade maize).
The GHG emissions were calculated for the whole lifespan and full capacities of the bags, using the storage loss fits presented in Figure 2. The results of the GHG emission analysis for the reference case (storage in standard bags) and the interventions are shown in Figure 3.
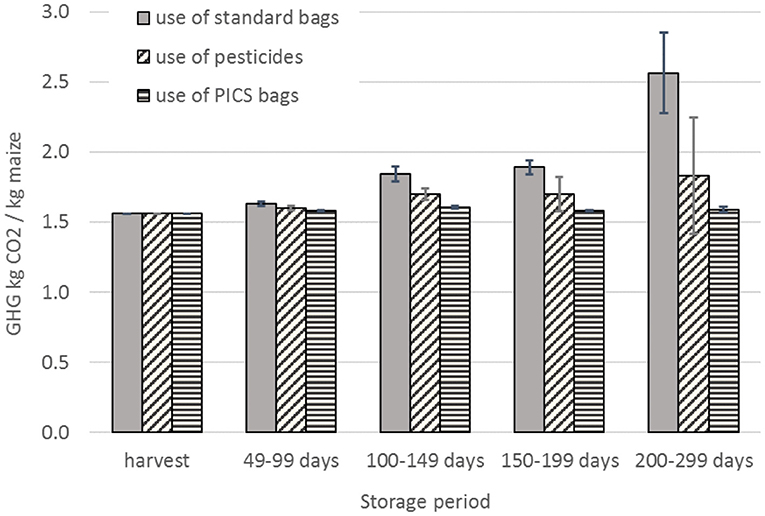
Figure 3. Specification of calculated GHG emissions along the supply chain for the scenarios over time (kg CO2-eq. emissions per kg maize remaining for consumption). Error bars represent 95% confidence limits.
Economic Effect on Costs and Benefits
For the economic calculation of the interventions analyzed in this study, both the home use of maize and its sale at local markets were considered. These calculations took into consideration three aspects: (1) the direct loss of economic value caused by the actual weight loss of maize (see Figure 2), (2) the effect of revenue losses due to quality reductions, and (3) price fluctuations during the year.
Quality of the Grain
The ratios between good, poor and unmarketable maize varies during the storage period and depends on the packaging system used. Based on the quality ratios inside the bags, it can be determined to what extent the full sales price can be obtained. Figure 4 shows that the use of pesticides or hermetic bags leads to much higher market values due to the reduced quantity of damaged grain. In the case of maize stored for shorter than 5 months, the use of the hermetic bag yielded close to zero damage, resulting in full economic benefits from the maize sales. Even after 7 months, for >60% of the volume, the full market price applied. The use of the pesticides also had a clear economic benefit compared with the use of the standard bags, with a close to 80% good product share recorded for maize stored for up to 3 months. In comparison with the hermetic bags, a faster decline in the economic value of the pesticide-treated maize was observed for intermediate storage periods of 3–6 months. A striking reduction in market value was observed for the use of the standard bags during the first 150 days of storage, with <5% of the volume classed as being of a good quality, and >90% assessed as being of an unmarketable quality. The slight improvement in quality observed between 150 and 200 days of storage was due to a number of good quality samples in this period coming from the study of Baoua et al. (Baoua et al., 2014), which were explained by a low infestation rate and very dry product. Storage in standard bags for more than 200 days led to so much damaged grain that all the maize was assessed as being of an unmarketable quality.
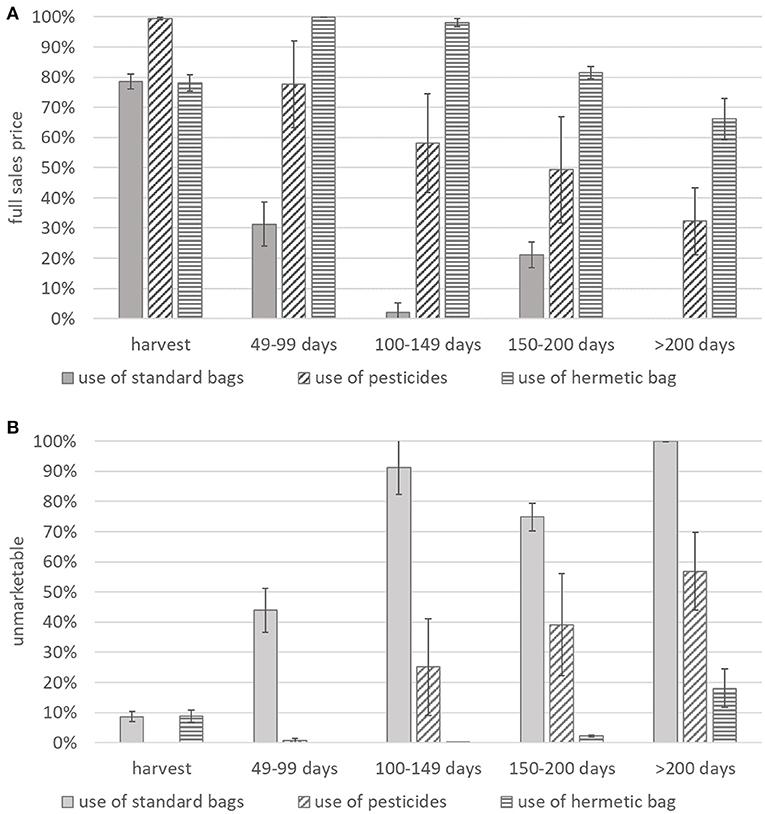
Figure 4. Post-harvest maize quality based on the amount of damaged grain by insects during different storage scenarios. Based on field data from Baoua et al. (2014), Ng'ang'a et al. (2016b), Mlambo et al. (2017). (A) % of full sales price when damaged grain level is <10%. (B) % Unmarketable for human consumption, >20% damaged grain. Error bars represent 95% confidence limits.
Profit for the Farmers
To estimate the economic benefits of different storage strategies for farmers, an in-house price was calculated during the season. The in-house price starts with the market price during the harvest period (Malawi, Zambia: May, Uganda: January and August). The planting, growing and harvest phase cost prices were not taken into account, since the focus was on the effect of the storage method on the farmers' profits. To the market price, the costs of storage are added (depreciations of the bags and pesticide use, Table 4). It was assumed that the bags were used once per year, and the cost of pesticides were determined directly after the harvest and were independent on the storage time. This resulted in the in-house price of the maize at the start of the storage period. During the storage period, some of the maize was lost according to the best-fit curves in Figure 2. This loss resulted in an increase in the in-house price per kilogram. Thus, when a 50% weight loss is recorded at a specific storage period, the in-house price will be twice the price of that at the beginning of the season. The resulting in-house prices per kilogram of maize during storage for the three countries and the different storage methods are presented in Table 5, together with the market prices of the same period. Independent of the country, the in-house price of maize stored in standard bags started lower than for the other storage systems (because these bags have the lowest cost price); however, the in-house price increased for every storage period (because of the high losses suffered). The hermetic bag method started with a higher in-house price, but the price was stable through the whole storage period. Further, for the use of pesticides, some benefits were found compared with the standard bag method for a period of more than 150 days.
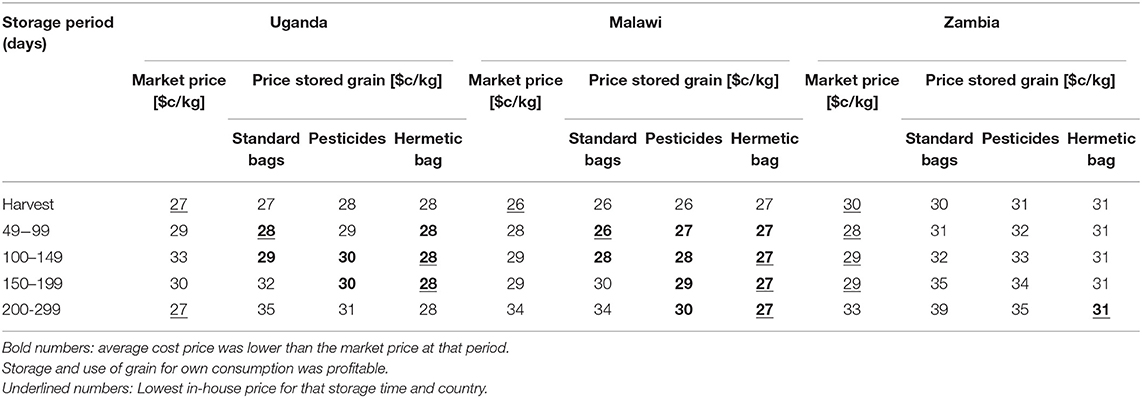
Table 5. Calculated in-house price/kg maize during different types of storage and in different African countries.
When the market price is higher than the in-house price in the same period, it will be cheaper for farmers to use their own maize instead of buying maize from the market to feed their families. In Table 6, the savings for farmers using their own harvest rather than buying from the market are calculated. This table shows that it is not so evident that hermetic bags are beneficial at all storage times. Compared with standard bag storage, hermetic bag storage only resulted in lower cost prices after 100 days. For storage times of up to 3 months, the use of a standard bag seems preferable. In the case of Zambia, it is better not to store the maize at all. For this country, only after long storage times with the hermetic bag can a slightly lower in-house price than the market price be expected.
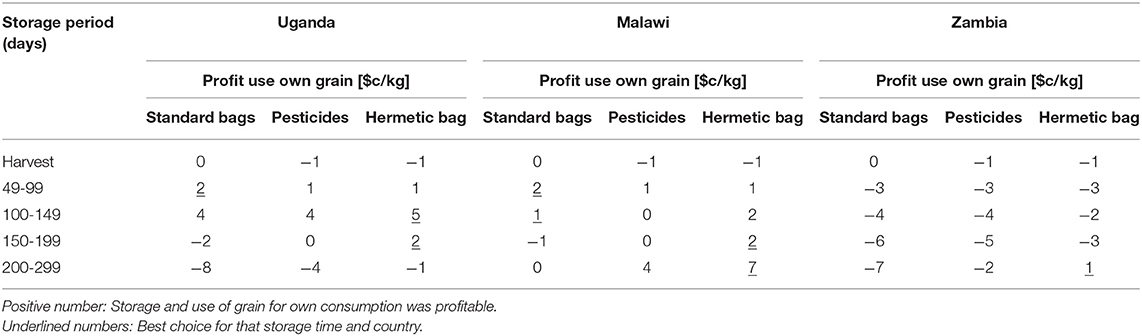
Table 6. Calculated savings for farmers consuming their own maize instead of purchasing maize from local markets during storage with different types of storage methods and in different African countries with a bag lifespan of 3 years.
Since poorer quality maize results in lower sales prices, the quality of the maize is relevant for the profit obtained when a farmer decides to sell maize in a certain period. In Table 7, discounts for damaged grain are taken into account. It shows the extra profit (or losses) farmers can make from the storage of maize by selling it in a certain period.
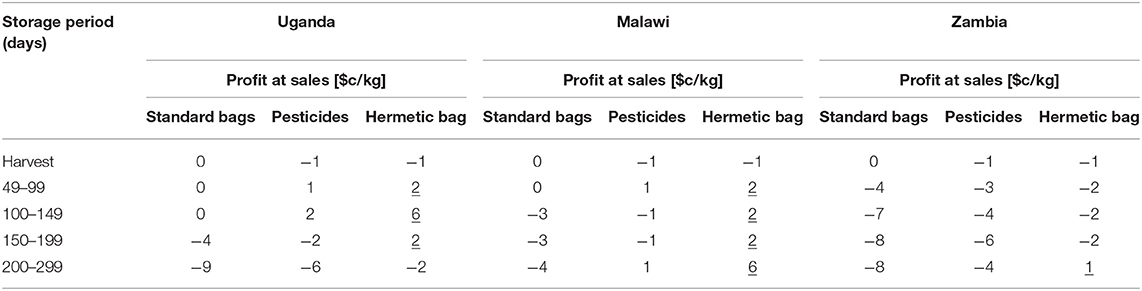
Table 7. Calculated profit for farmer selling their maize during storage with different types of storage methods and in different African countries with a bag lifespan of 3 years.
Discussion
Only data from literature describing practical field trials were used for a realistic comparison. In these studies the bags are used in normal circumstances. The maize was stored without specific predefined storage conditions. Studies on bags' effectiveness under laboratory conditions were excluded. Although these studies, like the study from Affognon et al. (Affognon et al., 2016), give exact results for the possible PHL reduction for applied circumstances and types of infections, they do not provide the expected losses when the grain has been naturally infested. As for maize, several studies with a practical approach are available, and they give a good estimation of the contribution of hermetic bags to PHL reduction in the field. Additionally, field studies that only measured the amount of damaged grain were excluded from the PHL estimations. Weight loss cannot be estimated from the percentage of damaged grain, since the ratio between weight loss and the percentage of weight loss is not uniform (Boxall, 1986). It varies with the type and variety of the grain, insect species, and applied storage time, as can be derived from the data from Jat et al. (Jat et al., 2013).
The recorded weight losses showed a very large scattering (see Figure 2). This is noticeable in case of the use of pesticides, where in some scenarios higher weight losses were recorded than for untreated maize at the same storage times. This is not uncommon, and has been reported by other authors (Stathers et al., 2008; De Groote et al., 2013): some farmers use pesticides in an attempt to save their harvests when their products are showing clear infestations. Despite these efforts, often high recorded weight losses for the pesticide-treated maize resulted, which were originally attributed to the reference maize storage method. However, since this is common practice, these numbers were not removed from the data.
The used field studies do not provide data on visible molded grain during the storage time. Therefore, it was assumed that the grains were well-dried and that no increase in molded grain occurred during storage. This is confirmed by the relatively low percentage of PHL due to mold found in most Sub-Saharan countries, as reported by Shiferaw et al. (Abdoulaye et al., 2016).
The GHG emissions related to PICS bags production were significantly smaller than the impact related to product losses. Thus, the PICS bags contribute to a net reduction in GHG emissions per unit of maize marketed for consumption after a storage time of 30 days and longer.
Using hermetic bags is the best way to make a profit for farmers in Uganda or Malawi to commercialize their maize. Large margins can be realized when the market value is at its highest point. Already after 2 months of storage the reduction in quality loss due to hermetic bags makes this type of storage profitable in Uganda and Malawi. For a Zambian farmer, storing maize is not beneficial at all due to the country's low seasonal gap. When storing maize in a standard bag, it is not likely that a Zambian farmer can make more profit by waiting for increased market prices. The use of pesticides can result in higher profits if the storage time is kept below 150 days in Uganda and below 100 days in Malawi.
The investment costs of hermetic bags are still quite high (see Table 4). Combined with the high variation in quality and weight losses, investments in hermetic bags will not be profitable every year. The cash flow of a farmer, as well as his or her experience with the losses suffered with traditional storage methods, will probably be decisive in their willingness to invest in hermetic bags.
This becomes even more relevant if the lifespan of the bags is reduced from 3 years to 1 year. After one harvest, the hermetic bags showed already some holes in the liners, mostly made by the insects (De Groote et al., 2013; Baoua et al., 2014). Although hermetic bags are still functional with a small number of holes in the liner, they will be more vulnerable to disruptions, thereby losing their efficiency over time. None of the included studies used the bags more than two times; therefore, their lifespan is not exactly known. On the other hand, maize is a relatively cheap product. Using hermetic bags for more expensive crops, like cowpeas, might make the use of hermetic bags for either farmers' own use or for sale more profitable than for maize.
Furthermore, additional profits can be expected if the benefit of reduced mycotoxins can be marketed. Aflatoxin production can be prevented in hermitic storage (Ng'ang'a et al., 2016a), and rural households are prepared to pay up to 38% more for certified aflatoxin-free maize according to De Groote, et al. (De Groote et al., 2016). However, the economic benefits are difficult to calculate as the cost of aflatoxin measurement is high, especially for relatively small harvested quantities. Contamination, especially in high moisture stored maize, is still common, when hermetic bags are repeatedly opened during (Tubbs et al., 2016). If aflatoxin is present before storage it will be maintained during storage (Ng'ang'a et al., 2016a). Furthermore, when storage starts with dry, good quality maize, aflatoxin can already be under control. If the starting contamination is low and the maize is dried to below 14%, the aflatoxin level seems not to cause problems in regular PP bags (Mutambuki and Likhayo, 2021).
Conclusions
From the perspectives of food security and greenhouse gas emissions, use of hermetic bags for maize storage in Sub-Saharan Africa works out positively compared with standard bags or standard bags combined with a pesticide. Up to 99 days of storage, this becomes already significant (LDS 5%). The difference with a standard bag increases to 9% and higher after 100 days of storage.
Differences in maize quality were notable after shorter storage times and onwards. At 49–99 days, 44% ± 7.3 of the maize stored in standard bags was unmarketable, and only 31 ± 7.3% would have been sold at the full market price, while maize stored in hermetic bags did not show any reduction in quality. For storing maize to sell it later in the season, quality is essential, next to minimization of weight loss. Since hermetic bags maintain quality during storage very well, these bags are most suitable for this strategy. In countries with high or moderate seasonal price fluctuations, hermetic bags can already be profitable for a commodity crop after 50 days of storage.
The net economic effect for farmers depends on storage time, seasonal price variations, weight losses and quality. Seasonal price variability differs considerably amongst African countries, affecting the results significantly. When maize is used for farmers' own consumption, standard bags are the best choice until 100-−149 days of storage, while hermetic bags are preferable for longer storage times.
It is most likely that farmers will be more open to investing after having negative experience with insect infestations. Furthermore, uptake will increase if investments are made in education regarding the expected health effects, based on their limitation of insecticides and their possible lowering of aflatoxin daily intake.
Future research could include additional field studies to further validate the results, as well as verification of the findings for other crops and countries. The hurdles of adaptation, and their mitigation, of improved storage methods need further investigation as well.
Data Availability Statement
The original contributions presented in the study are included in the article/supplementary material, further inquiries can be directed to the corresponding author.
Author Contributions
BD idea, implementation, and writing. MV co-writing. JB evaluation of the GHG emissions and reviewing. All authors contributed to the article and approved the submitted version.
Funding
This work was implemented as part of the CGIAR Research Program on Climate Change, Agriculture and Food Security (CCAFS), which is carried out with support from the CGIAR Trust Fund and through bilateral funding agreements. For details please visit https://ccafs.cgiar.org/donors. The views expressed in this document cannot be taken to reflect the official opinions of these organizations.
Conflict of Interest
The authors declare that the research was conducted in the absence of any commercial or financial relationships that could be construed as a potential conflict of interest.
Publisher's Note
All claims expressed in this article are solely those of the authors and do not necessarily represent those of their affiliated organizations, or those of the publisher, the editors and the reviewers. Any product that may be evaluated in this article, or claim that may be made by its manufacturer, is not guaranteed or endorsed by the publisher.
References
Abass, A. B., Ndunguru, G., Maniro, P., Alenkhe, B., Mlingi, N., and Bekunda, M. (2014). Post-harvest food lossed in a maize-based farring system of semi-arid savannah area of Tanzania. J. Stored Prod. Res. 57, 49–57. doi: 10.1016/j.jspr.2013.12.004
Abdoulaye, T, Ainembabazi, JH, Alexander, C, Baributsa, D, Kadjo, D, and Moussa, B (2016). Postharvest Loss of Maize and Grain Legumes in Sub-Saharan Africa. Purdue Extens. EC-807-W, 4.
Affognon, H. D., Njoroge, A. W., Mutungi, C. M., Manono, J., Baributsa, D., and Murdock, L. L. (2016). Storage of pigeonpea grain (Cajanus cajan (L.) Millsp.) in hermetic triplelayer bags prevents losses caused by Callosobruchu s maculatus (F.) (Coleoptera: Bruchidae). Acta. Horticulturae. 245–252. doi: 10.17660/ActaHortic.2016.1120.37
Baoua, I. B., Amadou, L., Ousmane, B., Baributsa, D., and Murdock, L. L. (2014). PICS bags for post-harvest storage of maize grain in West Africa. J. Stored Prod. Res. 58, 20–28. doi: 10.1016/j.jspr.2014.03.001
Baoua, I. B. L, Amadou Lowenberg-DeBoer, J. D., and Murdock, L. L. (2013). Side by side comparison of GrainPro and PICS bags for postharvest preservation of cowpea grain in Niger. J. Stored Prod. Res. 54, 13–16. doi: 10.1016/j.jspr.2013.03.003
Bbosa, D., Brumm, T. J., Bern, C. J., Rosentrater, K. A., and Raman, D. R. (2017). Evaluation of hermetic maize storage in 208 liter (55GAL) steel barrels for smallholder farmers. Transactions of the ASABE. 60, 981–987. doi: 10.13031/trans.12044
Bern, C. J., Yakubu, A., Brumm, T. J., and Rosentrater, K. A. (2013). Hermetic storage systems for maize stored on subsistence farms. Agricultural and Biosystems Engineering Conference Proceedings and Presentations. p. 343. Available online at: https://lib.dr.iastate.edu/abe_eng_conf/343
Boxall, R. A (1986). A Critical Review of the Methodology for Assessing Farm-level Grain Losses After Harvest. Slough, UK: Tropical Development and Research Institute Report G191.
Boxall, R. A (2002). Damage and loss caused by the larger grain borer Prostephanus truncatus. J. Integr. Pest Manag. 7, 105–121. doi: 10.1023/A:1026397115946
Broeze, J., Guo, X., Axmann, H., and Vollebregt, M. (2019). A systemic approach for trade-off analysis of food loss reduction and greenhouse gas emissions. CCAFS Working Paper no. 289. 2019 Wageningen, The Netherlands: CGIAR Research Program on Climate Change, Agriculture and Food Security (CCAFS).
Busso, C. D., Christ, R. M., Coelho, A. S. D., Domenico Frata, M. T., and Hashimoto, E. H. (2016). Occurrence of Aspergillus sp., Fusarium sp., and aflatoxins in corn hybrids with different systems of storage. Acta Scientiarum. Agronomy 38(1), 111-121. doi: 10.4025/actasciagron.v38i1.25621
De Bon, H., Huat, J., Parrot, L., Sinzogan, A., Martin, T., Malézieux, E., et al. (2014). Pesticide risks from fruit and vegetable pest management by small farmers in sub-Saharan Africa. A review. Agron. Sustain. Dev. 34, 723–736. doi: 10.1007/s13593-014-0216-7
De Groote, H., Narrod, C., Kimenju, S. C., Bett, C., Scott, R. P. B., Tiongco, M. M., et al. (2016). Measuring rural consumers' willingness to pay for quality labels using experimental auctions: the case of aflatoxin-free maize in Kenya. Agric. Econ. 47, 33–45. doi: 10.1111/agec.12207
De Groote, H.S. C, Kimenju, P., Likhayo, F., Kanampiu Tefera, T., and Hellin, J. (2013). Effectiveness of hermetic systems in controlling maize storage pests in Kenya. J. Stored Prod. Res. 53, 27–36. doi: 10.1016/j.jspr.2013.01.001
FAO (2014). The state of food and agriculture - Innovation in family farming. Rome: Food and Agriculture Organisation of the United Nations.
Gilbert, C. L., Christiaensen, L., and Kaminski, J. (2017). Food price seasonality in Africa: Measurement and extent. Food Policy. 67, 119–132. doi: 10.1016/j.foodpol.2016.09.016
Harris, K. L., and Lindblad, C. J. (1978). Postharvest grain loss assessment methods. Am. Associat. Cereal Chem. 193.
Heimpel, G. E. Y, Yang, J. D., and Hill Ragsdale, D. W. (2013). Environmental consequences of invasive species: greenhouse gas emissions of insecticide use and the role of biological control in reducing emissions. PLoS ONE. 8, e72293. doi: 10.1371/journal.pone.0072293
Jat, N. R., Rana, B. S., and Jat, S. K. (2013). Estimation of losses due to pulse beetle in chickpea. The Bioscan. 8, 861–863.
Jirström, M., Bustos, M. A., and Loison, S. A. (2018). African Smallholder Farmers on the Move: Farm and Non-Farm Trends for Six Sub-Saharan African Countries, 2002–15. Agriculture, Diversification, and Gender in Rural Africa. Oxford: Oxford University Press. doi: 10.1093/oso/9780198799283.003.0002
Jones, M., Alexander, C., Olynk, N., Ricker-Gilbert, J., and Lowenberg-DeBoer, J. (2016). Do insect and mold damage affect maize prices in Africa? Evidence from Malawi. Modern Economy 7, 1168–1185. doi: 10.4236/me.2016.711115
Jones, M. S., Alexander, C. E., and Smith, B. (2018). Economic consequences of post-harvest insect damage in Rwandan common bean markets. Crop Protection. 104, 92–100. doi: 10.1016/j.cropro.2017.10.015
Kamanula, J.G. W, Sileshi, S. R., Belmain, P., Sola, B. M., Mvumi, G. K. C., et al. (2010). Farmers' insect pest management practices and pesticidal plant use in the protection of stored maize and beans in Southern Africa. Int. J. Pest Manag. 57, 41–49. doi: 10.1080/09670874.2010.522264
Kimenju, S. C., and Groote, H. d. (2010). Economic analysis of alternative maize storage technologies in Kenya. Cape Town, South Africa: Paper presented at the AAAE Third Conference / AESA 48th Conference.
Kornher, L (2018). Maize markets in Eastern and Southern Africa (ESA) in the context of climate change.(SOCO) 2018: Backgruond paper. Rome: FAO.
Likhayo, P. A. Y, Bruce, K., Mutambuki Tefera, T., and Mueke, J. (2016). On-farm evaluation of hermetic technology against maize storage pests in Kenya. J. Econ. Entomol. 109, 1943–1950. doi: 10.1093/jee/tow134
Manandhar, A., Milindi, P., and Shah, A. (2018). An overview of the post-harvest grain storage practices of smallholder farmers in developing countries. Agri.-Basel. 8, 57. doi: 10.3390/agriculture8040057
Mlambo, S. B. M, Mvumi, T., Stathers Mubayiwa, M., and Nyabako, T. (2017). Field efficacy of hermetic and other maize grain storage options under smallholder farmer management. Crop Protect. 98, 198–210. doi: 10.1016/j.cropro.2017.04.001
Murdock, L. L. V, Margam, I., Baoua Balfe, S., and Shade, R. E. (2012). Death by desiccation: effects of hermetic storage on cowpea bruchids. J. Stored Prod. Res. 49, 166–170. doi: 10.1016/j.jspr.2012.01.002
Mutambuki, K., and Likhayo, P. (2021). Efficacy of different hermetic bag storage technologies against insect pests and aflatoxin incidence in stored maize grain. Bull. Entomol. Res. 111, 499–510. doi: 10.1017/S0007485321000213
Nabila, F. A., and Kumah, P. (2018). Determination of postharvest losses in maize production in the upper West region of Ghana. American Scientific Reseach Journal fo Engineering, Technology, and Sciences. 44, 1–18.
NCBA CLUSA (2016). Purdue Increased Crop Storage (Pics) Bags. Available online at: https://ncbaclusa.coop/project/purdue-increased-crop-storage-pics-bags/
Ndegwa, M. K. H, De Groote Gitonga, Z. M., and Bruce, A. Y. (2016). Effectiveness and economics of hermetic bags for maize storage: Results of a randomized controlled trial in Kenya. Crop Protect. 90, 17–26. doi: 10.1016/j.cropro.2016.08.007
Ndiso, J. B. S, Mugo, A. M., Kibe Pathaka, R. S., and Likhayo, P. (2017). Screening Kenya local coastal maize landraces for resistance to maize weevil (Sitophilus Zeamais Motschulsky) and larger grain borer (Prostephanus Truncates). Int. J. Plant Res. 7, 5–11.
Ng'ang'a, J., Mutungi, C., Imathiu, S., and Affognon, H. (2016a). Effect of triple-layer hermetic bagging on mould infection and aflatoxin contamination of maize during multi-month on-farm storage in Kenya. J. Stored Prod. Res. 69, 119–128. doi: 10.1016/j.jspr.2016.07.005
Ng'ang'a, J., Mutungi, C., Imathiu, S. M., and Affognon, H. (2016b). Low permeability triple-layer plastic bags prevent losses of maize caused by insects in rural on- farm stores. Food Secur. 8, 621–633. doi: 10.1007/s12571-016-0567-9
Njoroge, A. W., Mankin, R. W., Smith, B. W., and Baributsa, D. (2018). Oxygen consumption and acoustic activity of adult Callosobruchus maculatus (F.) (Coleoptera: Chrysomelidae: Bruchinae) during hermetic storage. Insects 9, 45. doi: 10.3390/insects9020045
Ognakossan, K. E., Affognon, H. D., Mutungi, C. M., Sila, D. N., Midingoyi, S.-K. G., and Owino, W. O. (2016). On-farm maize storage systems and rodent postharvest losses in six maize growing agro-ecological zones of Kenya. Food Security. 8, 1169–1189. doi: 10.1007/s12571-016-0618-2
Porter, S. D., Reay, D. S., Higgins, P., and Bomberg, E. (2016). A half-century of production-phase greenhouse gas emissions from food loss & waste in the global food supply chain. Sci. Total Environ. 571, 721–729. doi: 10.1016/j.scitotenv.2016.07.041
Sallam, M. N (1999). Insect damage: damage on post-harvest. In D. Insect Daamge: Post-Harvest Operations, Meija (Ed.). Rome: Food and Agricultural Organization of the United Nations. p. 37
Stathers, T. E. W, Riwa, B. M., Mvumi, R., Mosha, L., Kitandu, K., et al. (2008). Do diatomaceous earths have potential as grain protectants for small-holder farmers in sub-Saharan Africa? The case of Tanzania. Crop Protect. 27, 44–70. doi: 10.1016/j.cropro.2007.04.020
Tubbs, T., Baributsa, D., and Woloshuka, C. (2016). Impact of opening hermetic storage bags on grain quality, fungal growth and aflatoxin accumulation. J. Stored Prod. Res. 69, 276–281. doi: 10.1016/j.jspr.2016.10.003
Keywords: food security, losses, hermetic bags, economic analysis, maize, Sub-Saharan Africa
Citation: Dijkink B, Broeze J and Vollebregt M (2022) Hermetic Bags for the Storage of Maize: Perspectives on Economics, Food Security and Greenhouse Gas Emissions in Different Sub-Saharan African Countries. Front. Sustain. Food Syst. 6:767089. doi: 10.3389/fsufs.2022.767089
Received: 30 August 2021; Accepted: 05 January 2022;
Published: 28 January 2022.
Edited by:
Cristobal N. Aguilar, Universidad Autónoma de Coahuila, MexicoReviewed by:
R. Pandiselvam, Central Plantation Crops Research Institute (ICAR), IndiaCristian Torres-León, Universidad Autónoma de Coahuila, Mexico
Copyright © 2022 Dijkink, Broeze and Vollebregt. This is an open-access article distributed under the terms of the Creative Commons Attribution License (CC BY). The use, distribution or reproduction in other forums is permitted, provided the original author(s) and the copyright owner(s) are credited and that the original publication in this journal is cited, in accordance with accepted academic practice. No use, distribution or reproduction is permitted which does not comply with these terms.
*Correspondence: Bert Dijkink, bert.dijkink@wur.nl