- WorldFish, Bayan Lepas, Malaysia
Aquatic foods, or “seafood”, are an integral part of the global food system that contribute significantly to many dimensions of human wellbeing, including livelihoods and food and nutrition security. Fish, molluscs, crustaceans, algae and other aquatic foods are of particular importance in low- and middle-income countries as a source of employment, income, and nutrition for many poor and vulnerable people, including women. Global concern over the ability of fisheries and aquaculture to sustainably meet future seafood demand is driving improvements in technology and management. It has also inspired the emergence of plant-based and cell-based seafood, collectively termed “alternative seafood”. Growing investment, consumer demand, and participation by major food companies in the alternative seafood sector necessitate an evaluation of potential opportunities and challenges alternative seafood poses to food systems. This paper explores key economic, social, and environmental implications associated with production, distribution, and consumption of alternative seafood and its interactions with fisheries and aquaculture over the next decade, with specific emphasis on low- and middle-income countries. Available data on current supply and projected growth suggest that alternative seafood may account for almost eight percent of global seafood supplies destined for human consumption in 2030. Assuming current production techniques and expected technological development, the sector has potential for reduced environmental impacts relative to the existing fisheries and aquaculture sectors. However, its potential to impact livelihoods, food and nutrition security, and the environment remains largely a matter of conjecture due to the lack of robust data. Mechanistically, it is believed that growth of alternative seafood supplies will lessen demand for “conventional” seafood and/or meat, a scenario with implications for livelihoods, food and nutrition security, and the environment. Such changes are contingent on technological development, human and institutional behavior, market forces, and ecological linkages and as such, remain speculative. Nevertheless, as a novel sector, new food, and potential alternative to conventional seafood and/or meat, society has an opportunity to shape the growth of alternative seafood and its contribution to national and global development goals. This paper identifies knowledge gaps that require further research to inform inclusive, equitable, and sustainable development and governance of the emerging alternative seafood sector.
Introduction
Increasing awareness of challenges to our food systems—climate change, public health, ecosystem disruption, environmental degradation, human rights violations, and animal welfare—is driving major changes in food production and consumption. Increased public exposure to shocks like COVID-19 appear to be accelerating consumer demand for change globally (Knight et al., 2020; Love et al., 2021; White et al., 2021), with static or declining national averages of meat and other terrestrial animal-source food consumption in many high-income countries (HICs), albeit with changing composition of food consumption (Godfray et al., 2018; Attwood and Hajat, 2020). Consumers are also demanding greater transparency about provenance, production methods, environmental sustainability, and social responsibility. However, the complexity and decentralization of value chains for conventional aquatic foods (hereafter “seafood”) make this difficult to achieve equitably and effectively (Bailey et al., 2016; McClenachan et al., 2016).
Seafood is an integral part of the global food system that contributes significantly to livelihoods and food and nutrition security, especially in low- and middle-income countries (LMICs) (Hicks et al., 2019; FAO, 2020). However, at a time of significant and unprecedented stress on aquatic ecosystems, concern mounts regarding the sustainability of present supplies and our ability to meet future demand (Barange et al., 2018; GFI, 2019; FAO, 2020). Plant-based and cell-based seafood, collectively termed “alternative seafood”, have rapidly emerged over the past half decade and are often promoted as part of the solution to these concerns. Plant-based seafood products seek to mimic the taste, texture, appearance and/or nutritional properties of conventional seafood, so consumers can enjoy the sensory and/or nutritional experience and reduce their seafood intake. Cell-based seafood is grown directly from cells of aquatic animals and as such, is comprised of the same cell types and may be arranged in the same three-dimensional structures as its conventional counterpart.
The emerging alternative seafood sector lacks statistics and public monitoring systems, but recent projections indicate that plant- and cell-based seafood may claim 0.14 and 7.5% of the conventional seafood market by volume by 2030 (Marwaha et al., 2020). These estimates say more about the perceived disruption value of the sector than the likely impacts on food systems, as it remains to be seen if alternative seafood will be a fad, retain its place as a food for human consumption, or be used differently altogether, such as for animal feed.
Few studies have evaluated the potential of alternative seafood to impact food systems, especially in LMICs. This review explores key economic, social and environmental implications associated with production, distribution, and consumption of alternative seafood and its interactions with fisheries and aquaculture over the next decade, with specific emphasis on LMICs. Our analysis relies on the available evidence in peer-reviewed literature, reports and other gray literature, some of which is extrapolated from research on alternative terrestrial meats. The paper covers the potential of alternative seafood to impact food systems; economic implications, especially regarding trade and livelihoods; social implications, specifically for food and nutrition security; environmental implications, focused on natural resources use and health of aquatic ecosystems; and recommendations for further research.
Alternative Seafood and Its Potential to Impact Food Systems
Traditional (e.g., tofu, tempeh) and whole food (e.g., mushrooms, jackfruit) alternatives to meat and seafood have been eaten for centuries, but their sensory and nutritional attributes differ from conventional meat and seafood (Kyriakopoulou et al., 2019). Alternative seafood often better emulates its conventional counterparts in effort to gain acceptance by conventional seafood consumers, as evidenced by growing investment from public and private sectors, consumer demand and participation by major food companies (Marwaha et al., 2020). Alternative seafood comprises all plant-based, fermentation-derived and cell-based seafood alternatives that mimic the taste, texture, appearance and/or nutritional properties of conventional seafood (Marwaha et al., 2020). Fermentation-derived seafood is considered with plant-based seafood here but does have its own unique characteristics (GFI, 2020a).
Plant-based seafood encompasses structured plant-, algae- or fungus-derived foods designed to replace conventional seafood either as standalone products or within recipes (GFI, 2019; Marwaha et al., 2020). Aquatic plants and algae consumed in their natural form (Aasim et al., 2018) are not discussed here. Plant-based seafood is typically comprised of a combination of legume proteins, soy protein, wheat protein, rice, vegetables, mycoproteins, seaweed, algal oil and plant oils (Table 1a). The plant proteins mainly come from terrestrial sources and are processed with water, flavoring, fat, and binding and coloring agents to mimic the sensory, and to some degree the nutritional, attributes of conventional seafood (Kyriakopoulou et al., 2019). Almost 30 companies across Europe, North America and Asia have emerged with plant-based versions of breaded fish filets and cakes, shredded and raw tuna, smoked and raw salmon, and shrimp, which are available for purchase at major food retailers and online platforms (Table 1a) (Marwaha et al., 2020). These visceral equivalents are often targeted at conventional seafood consumers (Stephens et al., 2018), but public research and regulatory support, especially regarding novel ingredients and production technologies, are needed to widen market acceptability and accessibility, which remains low (Kazir and Livney, 2021).
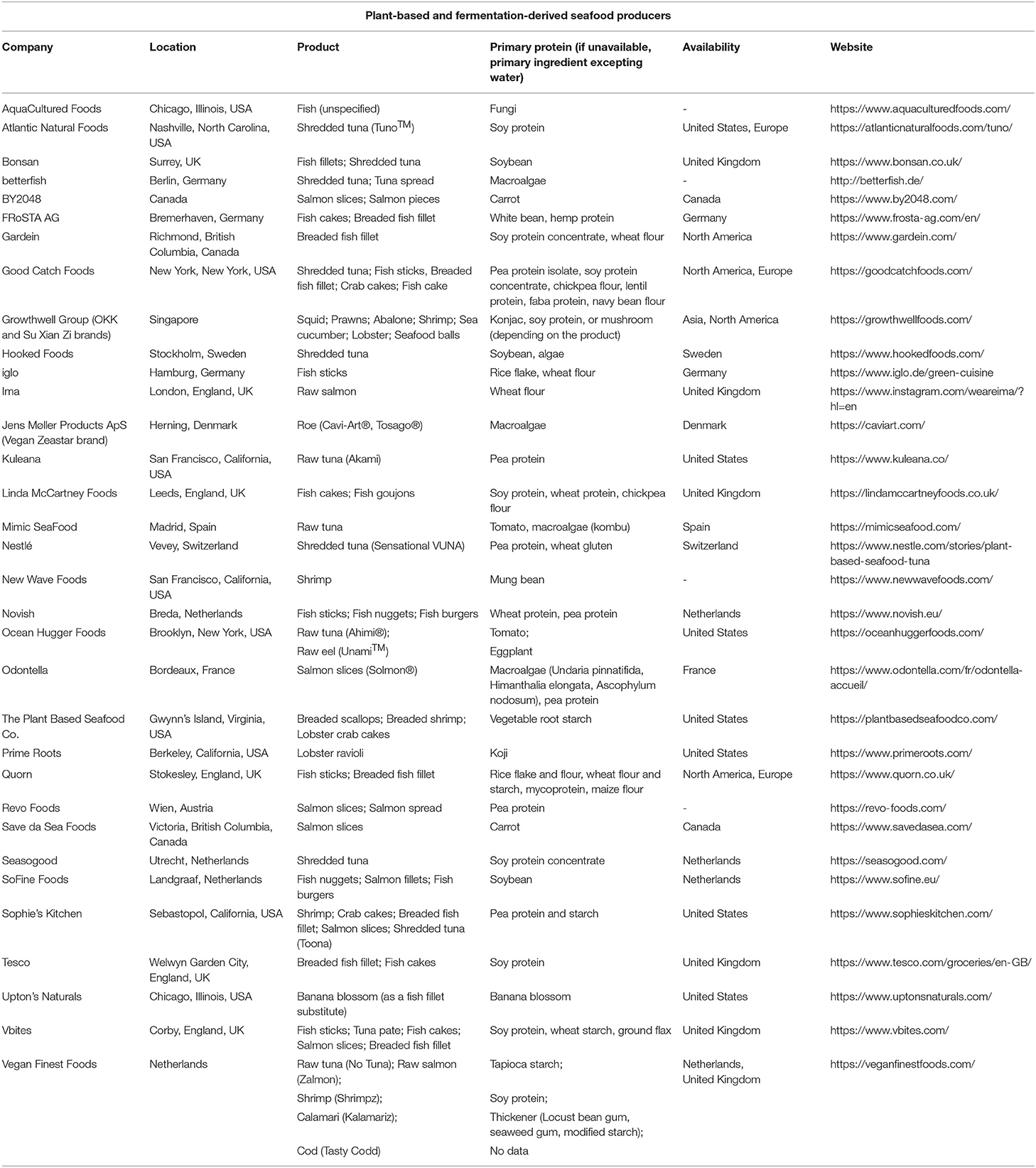
TABLE 1a. Current plant-based and fermentation-derived seafood producers, products, and main protein sources (as of 29 July 2021).
Cell-based seafood is produced through the cultivation of aquatic animal cells, and as such is genuine animal tissue that aims to replicate the sensory and/or nutritional profile of conventional aquatic animal foods (GFI, 2019; Marwaha et al., 2020). Cells are generally grown in bioreactors that regulate temperature, nutrients and other conditions (e.g., dissolved oxygen, pH) to optimize growth, then concentrated and structured to produce commercial products (Rubio et al., 2019). This allows for the isolated production of desired cuts with fewer public health concerns, reduced human and animal welfare issues, and novel opportunities for shorter and more transparent value chains and localized production. Currently, the nine companies producing cell-based seafood are based in North America, Asia, and Europe and focus on higher value species including bluefin tuna, crab, fish maw, grouper, lobster, mahi mahi, salmon, shrimp, and sturgeon (Table 1b). Rapid development of the industry is marked by growing investment, with recent involvement by the public sector (Dolgin, 2020; National Science Foundation, 2020) and partnerships between cell-based seafood producers and major food companies (Marwaha et al., 2020). Although no cell-based seafood products have received regulatory approval, it is anticipated in Singapore by 2022, with sales in Japan and approval in North America, Europe, and Australia expected to follow (Waltz, 2021). Sales will be targeted at wealthier markets (e.g., fine dining, HICs) as extremely high prices preclude wider market penetration. This is mainly due to a range of technological limitations, including optimized and scalable production (e.g., appropriate cell lines and scaffolding, optimized media formulations and cell culture densities, scalable bioreactors), and natural resources use, specifically energy and water (Rubio et al., 2019; Potter et al., 2020). It is widely agreed that public research would create a foundation of shared scientific knowledge to help advance the sector (Potter et al., 2020) and be necessary to bring cell-based seafood to mass markets and poorer consumers (Dolgin, 2019).
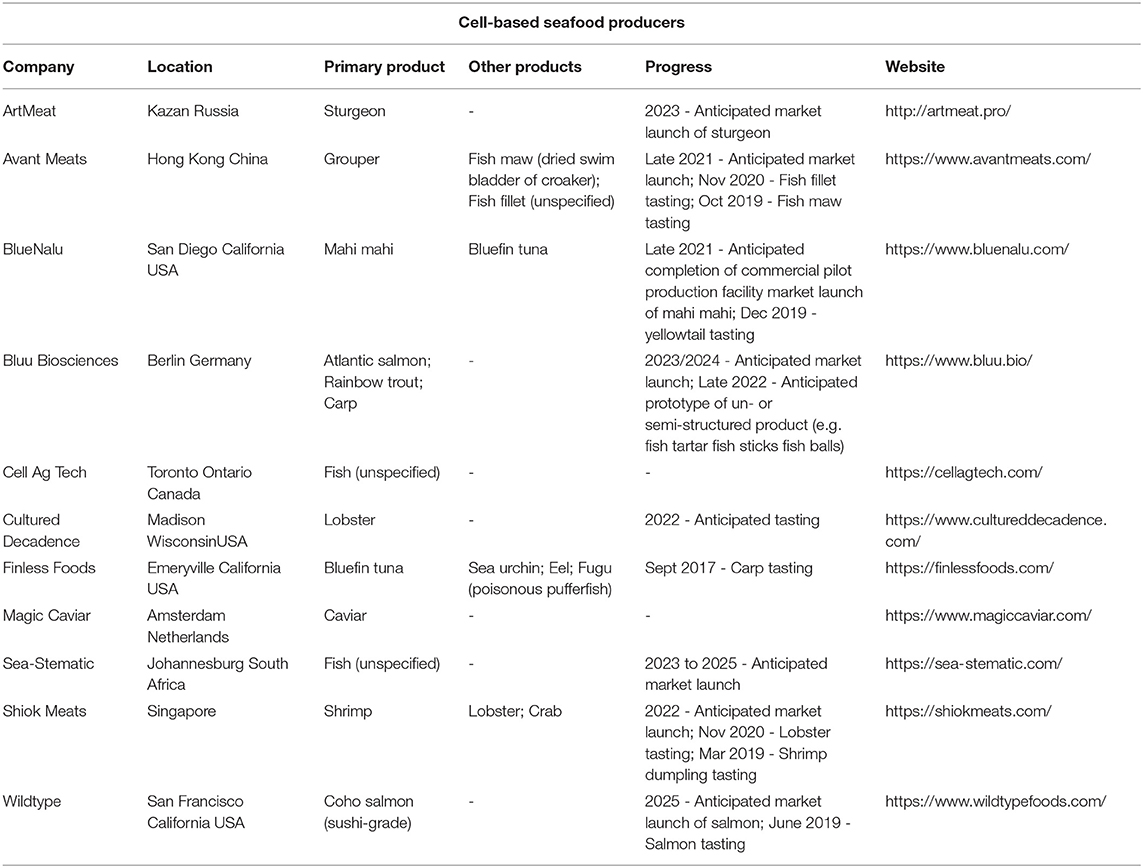
TABLE 1b. Current cell-based seafood producers, products, and progress to date (as of 29 July 2021).
Alternative seafood is promoted for its potential to increase the sustainability and resilience of food systems without requiring significant behavioral change from consumers (GFI, 2019; Wurgaft, 2020). This stance is framed around the continued increase in seafood demand and belief that significant reductions in global seafood consumption are unlikely (FAO, 2020; Wurgaft, 2020). However, impacts on food systems are dependent on many factors ranging from the development of production to methods of consumer adoption. To see positive change, production must be well-governed and uphold social and environmental standards. The adoption of alternative seafood must also be coupled with sufficient disadoption of conventional seafood and/or meat, which itself is influenced by many factors including price, taste and accessibility (Halpern et al., 2021). Although plant-based seafood is mainly accessible in wealthier markets and the introduction of cell-based seafood is expected to be concentrated in wealthier markets, if these products are responsibly produced and the adoption transition happens at scale, the partial replacement of wild or farmed seafood could impact global food systems. These changes will likely be experienced in HICs, but there may be indirect effects on livelihoods, food and nutrition security and the environment in LMICs. However, demand projections for alternative seafood are subject to great uncertainty, largely because the current market share is small, so it remains unclear if the scale of adoption will be significant enough to create measurable improvements (Halpern et al., 2021).
As a novel sector, new food and potential alternative to conventional seafood and/or meat, governments, intergovernmental organizations, and businesses have an opportunity to shape the growth of alternative seafood to contribute to national and international goals for inclusive, equitable, and sustainable food systems (Herrero et al., 2020). This paper primarily explores how alternative seafood might impact food systems if it can indeed augment global seafood production, while acknowledging other methods to sustainably increase seafood supply (Cabral et al., 2020; DeWeerdt, 2020), decrease loss and waste (Kruijssen et al., 2020), and better distribute nutrient-rich foods (Ahern et al., 2021) are likely of more immediate relevance to improving livelihoods, food and nutrition security, and the environment, especially in LMICs.
Economic Implications
The following section explores how current development and future projections for alternative seafood may affect global seafood markets and livelihoods, especially of small-scale actors in the conventional seafood sector.
Global Markets
Significant development and rapid investment in alternative seafood has occurred over the past half decade (GFI, 2021c). Major food companies have invested in or partnered with plant-based producers (e.g., Bumble Bee Foods and Good Catch Foods, Tyson Ventures and New Wave Foods) and others have acquired or started their own lines of plant-based seafood (e.g., Nestle's Vuna, Van Cleve Seafood's The Plant Based Seafood Co.). A few cell-based seafood producers have also seen investment and partnerships from major incumbents (e.g., Cargill, Griffith Foods, Nutreco, Pulmuone, Rich Products Corporation, Sumitomo, Thai Union). Early research on the science of cell-based seafood by the public sector (Benjaminson et al., 2002) provided a starting point for much of the current development by the private sector, but there is renewed advocacy to secure consistent public sector support for both plant- and cell-based seafood (116th Congress, 2020; Dolgin, 2020; National Science Foundation, 2020).
Involvement by incumbents and the concentration of producers in HICs raises concern over increased consolidation in value chains that can perpetuate power disparities (Santo et al., 2020), but it remains unclear how alternative seafood will act in global markets. Before COVID-19, and increasingly so since, we have seen increased consumer demand for alternatives to animal-source foods and better transparency in value chains (Attwood and Hajat, 2020). These trends are expected to continue as consumers improve their food literacy (De Backer et al., 2021). However, at <1% of the conventional seafood market (GFI, 2020b), it is yet to be seen if alternative seafood will play a significant part in this transition.
There may be more opportunity for widespread adoption if price parity with conventional seafood is reached. Although key protein inputs are generally much less expensive than animal-source proteins, plant-based seafood tends to retail for a premium (Rubio et al., 2020). Prices may become more competitive as start-up costs are recovered, input supplies and processing are optimized, and economies of scale are reached (Specht, 2019). The cost of cell-based seafood remains prohibitive largely due to expensive growth factors in cell culture media, though capital expenses can also be significant (Risner et al., 2021; Vergeer et al., 2021). With further research and development it is postulated that cell-based seafood may be produced at less than USD 6 per kg of edible product by 2030, placing it at price parity with many types of conventional seafood (Vergeer et al., 2021). In the meantime, cell-based seafood may prove viable as a minor ingredient in hybrid seafood alternatives or as high-value products (e.g., bluefin tuna, fish maw). However, implications for food systems also depend on how adoption occurs. If it lasts, will alternative seafood compete with conventional seafood, conventional or alternative meat, or other foods, and result in significant substitution? Or, as with the development of aquaculture, will alternative seafood simply expand the global market and supply?
Alternative seafood may also help improve sustainability of other sectors. For example, plant-based seafood and associated production technologies (e.g., fermentation) could be adapted to produce novel, accessible ingredients for aquatic animal feeds that reduce reliance on wild capture fish or improve fish and human nutrition (Cottrell et al., 2020; Marwaha et al., 2020). The development of alternative seafood may also stimulate and support other sectors (e.g., seaweed farming) that, with appropriate management, can help reduce impacts of climate change (Duarte et al., 2017; Froehlich et al., 2019; Roque et al., 2021).
Livelihoods
Alternative seafood production depends on a transdisciplinary group, including farmers, biologists, chemists, engineers, and factory workers. If alternative seafood were to significantly displace conventional seafood production, there may be significant changes in livelihoods, specifically in terms of income and employment, of aquatic food system actors. A mass shift from conventional seafood to alternative seafood could disrupt current employment in seafood production and processing, as well as upstream in the value chain (e.g., vessel construction, gear fabrication). However, downstream employment opportunities (e.g., packaging, transport) may increase as alternative seafood, especially cell-based seafood, will require similar handling as conventional seafood, although the actual distribution of benefits may aggravate existing inequalities (Marwaha et al., 2020).
As alternative seafood grows, research and policies that allow marginalized aquatic food system actors to retain their place in conventional value chains or participate in alternative seafood value chains are needed. Since alternative seafood will likely be mainly available in HICs in the next decade, effects may be felt by actors in LMICs that increasingly produce seafood for export to HICs (FAO, 2020). It is expected that change would be concentrated in industrial fishery or aquaculture operations, which may manifest as increased unemployment or competition for low wage or dangerous jobs, but the focus on alternatives for high value species may also directly affect small-scale fishers and farmers producing high value species (e.g., shrimp, grouper, snapper, yellowtail) (Marwaha et al., 2020). Appropriate measures in LMICs may be necessary to protect workers, support livelihood diversification or conversion (GFI, 2021a), and develop key domestic seafood markets.
If the adoption of alternative seafood in place of conventional seafood reduces pressure on aquatic ecosystems, it may further benefit marginalized actors whose traditional livelihoods have been compromised by industrial fishing operations. However, the scale at which this must occur is unclear and should be further explored (Cottrell et al., 2021). Given current projections for the next decade, it is unlikely that alternative seafood will cause fisheries and aquaculture to reduce their current scale of operation. Rather, this will be due to broader adoption and enforcement of environmental regulations, reduced availability of water and production locations, increasing incidence of aquatic animal diseases, and decreasing productivity gains (FAO, 2020).
Social Implications
The implications of alternative seafood on key social indicators, with an emphasis on nutrition and food security, namely availability, access, utilization, stability, agency, and sustainability, are reviewed here.
Food and Nutrition Security
Plant-based seafood is generally promoted as having comparable nutritional value to conventional counterparts. However, this must be systematically evaluated as there are key nutrients (e.g., vitamin A, vitamin B12, riboflavin, calcium, iron, zinc) that are difficult to adequately secure from solely plant-source foods (Murphy and Allen, 2003). Plant-based seafood may also be developed to help balance diets rather than replace conventional seafood in diets (e.g., high dietary fiber content, vitamin and/or mineral fortification) (Kyriakopoulou et al., 2019). Although there is limited evidence of the nutritional value and health effects of plant-based seafood consumption, plant-based meat generally contains similar nutrient composition (i.e., macronutrients, readily available minerals) as their conventional counterparts (Bohrer, 2019) and can lower several cardiovascular disease risk factors in healthy adults (Crimarco et al., 2020). Nutritional equivalency, however, depends on the specific formulation of the plant-based product and what it replaces in diets. As such, health implications regarding ingredient types, degree of processing and final nutritional profile of plant-based seafood has raised concern, especially for nutritionally vulnerable populations (Monteiro et al., 2019).
There is little information about the nutritional value of cell-based seafood (Potter et al., 2020), though it is often claimed that it will be comparable or superior to their conventional counterpart and can be tailored to meet dietary needs and preferences (Datar and Betti, 2010; Rubio et al., 2020). Seafood is a nutrient-dense source of high quality, highly bioavailable proteins, lipids and micronutrients, however, some compounds (e.g., omega-3 fatty acids, vitamin B12, heme iron) not synthesized by muscle cells must be supplemented in cell-based seafood (Datar and Betti, 2010). More research on the metabolism of essential compounds and methods for supplying these compounds (e.g., media formulation, co-cultures, genetic engineering), including the development of appropriate supply chains for supplemented compounds, is necessary to optimize nutritional value of products (Datar and Betti, 2010; Rubio et al., 2019, 2020; Fraeye et al., 2020). The controlled production process allows for more direct customization, which can be used to refine organoleptic or nutritional properties, and minimization of food safety concerns, including contaminant accumulation and zoonotic diseases (Datar and Betti, 2010; Gauthier, 2015; Johnson and Schantz, 2017). However, novel aspects of cell-based seafood production that can affect food safety, including the necessity of antibiotic use (Thorrez and Vandenburgh, 2019; Post et al., 2020), require further research (Ong et al., 2021).
It is also unclear if alternative seafood will improve key indicators of food security—availability, access, utilization, stability, agency and sustainability (HLPE, 2020). Plant-based seafood shifts proteins up the supply chain by moving protein sources, including some traditionally used for animal feed, toward consumption by humans as ingredients for extending or replacing animal-source proteins (Boland et al., 2013). Cell-based seafood allows for the isolated production of desired cuts, so inputs are directed to the edible portion and not on other developmental or metabolic functions. Additionally, the production of alternative seafood is not dependent on proximity to aquatic environments so there is potential to bring alternative seafood value chains to inland or urban areas which may improve local food and nutrition security (O'Meara et al., 2021), though barriers of economic accessibility and other social or cultural norms must be addressed (Halpern et al., 2021). However, concentration of these products in HICs may limit their availability to a wealthy elite (Rubio et al., 2019), or consumers may simply adopt alternative seafood in addition to their current animal-source food intake or in place of other more sustainable foods. Despite this, supporters claim that based on the size of the conventional seafood industry, if alternative seafood displaces even a small portion of conventional supplies over the next couple decades it could improve aquatic ecosystem health, which might stimulate recovery of coastal and other small-scale fisheries and in turn, improve food and nutrition security in these areas. Although the potential for these ripple effects are debated (Halpern et al., 2021), any transition to achieve them will require support from governments and the alternative and conventional seafood sectors to, for example, promote alternatives for species that would maximize economic, social and environmental benefits (GFI, 2021b), especially in LMICs.
Increased production, decreased loss and waste, and better distribution of nutritious foods are required to ensure adequate food and nutrition security of growing populations (Willett et al., 2019). Information regarding the nutritional value, potential to improve indicators of food security and methods of adoption of alternative seafood is largely speculative. There is no doubt a combination of methods is necessary to sustainably meet growing global demand for nutrient-rich foods, so further research on complementary solutions is merited.
Environmental Implications
The environmental implications of alternative seafood are determined by all segments of the value chain, including input sourcing, production techniques and consumer adoption. The following sections focus on environmental indicators indicative of natural resources use, which is largely associated with production and upstream segments of the value chain, and aquatic ecosystem health, largely associated with consumption patterns. However, many other factors, including eutrophication potential, acidification potential and ozone depletion, should also be accounted for in a balanced environmental assessment (Halpern et al., 2019).
Natural Resources Use
Given the paucity of data specific to alternative seafood, estimates of environmental impact are derived from available assessments of both alternative meat and seafood. Areas where they are expected to differ are noted. Estimates are given per kg of product since the potential nutritional value of alternative seafood is not limited to certain nutrients, like protein.
One study to date has quantified the greenhouse gas emissions associated with plant-based seafood as 1.5 kg CO2e per kg of product (farm to factory gate), though these are fermentation-derived mycoprotein products (Quorn Foods, 2019). The estimate is comparable to those for plant-based meat (range = 0.9–6.94 kg CO2e/kg; median = 2.4 kg CO2e/kg) (Santo et al., 2020). Estimated emissions from plant-based meat vary, but are mainly distributed between inputs, processing and packaging (Santo et al., 2020). Plant-based seafood may also have lower emissions if inputs from aquatic ecosystems (e.g., algae) can be sustainably produced and significantly integrated in products.
Cell-based seafood is expected to have more efficient production processes than conventional counterparts, though no formal environmental impact analyses have yet been published. Anticipatory estimates of greenhouse gas emissions from cell-based meat range from 1.69 to 25.4 kg CO2e per kg of product (median = 5.44 kg CO2e/kg), but rely on simplifications and assumptions regarding inputs, processes and technological development since no facilities are currently producing at scale (Scharf et al., 2019; Santo et al., 2020). The energy required for product manufacture is responsible for a large proportion of emissions from cell-based meat (Santo et al., 2020; Sinke and Odegard, 2021), but cell-based seafood is expected to have lower energy requirements because of the greater tolerance of fish muscle tissue to cooler temperatures, a wider range of pH, and lower oxygen requirements during growth (Rubio et al., 2019), and thus may have lower emissions than cell-based meat.
Median emission estimates for plant- and cell-based meat are lower than that for farmed fish (median = 6.52 kg CO2e/kg) and crustaceans (median = 9.87 kg CO2e/kg), though the actual difference depends on the degree of decarbonization along the value chain, and the estimate for cell-based meat is higher than that of wild tuna (median = 2.86 kg CO2e/kg) (Santo et al., 2020; Sinke and Odegard, 2021). The use of CO2e to compare greenhouse gas emissions is contested, especially in animal agriculture, because of the varying atmospheric lifespans and global warming potentials of different greenhouse gasses (Garnett, 2011; Lynch et al., 2021), so research on appropriate metrics to measure climate impacts in food systems is merited.
No estimates of land use for alternative seafood production are available but estimates for alternative meat range between 0.41 and 5 m2 year per kg of plant-based meat product (median = 2.47 m2/kg) and 0.19–8.03 m2 year per kg of cell-based meat product (median = 1.27 m2/kg). Both median estimates are lower than that of farmed fish (median = 5.6 m2/kg) but higher than that of farmed crustaceans (median = 0.82 m2/kg) (Santo et al., 2020). Improved feed conversion ratios for alternative seafood contribute to land savings, but actual land use will depend on input ingredients, production methods and volumes. For plant-based seafood specifically, dependence on soy, wheat and palm oil could impede sustainability (Santo et al., 2020). However, the potential for local, underused, and/or novel ingredient use has implications for ecosystem health and biodiversity, local income generation, and diet diversification.
Fresh water use for alternative meat production is estimated in fewer studies, ranging from 13.4 to 202.9 L per kg of plant-based meat product (median = 71.6 L/kg) and 106.3–773.2 L per kg of cell-based meat product (median = 397.5 L/kg) (Santo et al., 2020). There are no available estimates for alternative seafood. The median estimate of fresh water use for plant-based meat is lower than those of farmed fish (non-pond, median = 284 L/kg; pond-raised, median = 10,705 L/kg) and farmed crustaceans (median = 9,258.6 L/kg), while the estimate for cell-based meat is lower than those of pond-raised fish and farmed crustaceans (Santo et al., 2020).
Reductions in use of natural resources are likely to be spatially heterogeneous as they will be influenced by patterns of production, trade and consumption. Research regarding the factors and enabling environment for alternative seafood that contribute to reduced natural resources use, and how this might be designed and effectively implemented to fit within the planetary framework (Springmann et al., 2018), is crucial for informing sound interventions for environmentally sustainable food systems.
Aquatic Ecosystem Health
The potential conservation outcomes of plant- and cell-based seafood are discussed collectively since the proposed mechanism is the same, but the probability of these outcomes may differ. Proponents of alternative seafood generally posit it as an additional seafood supply that can help meet growing seafood demand without increasing pressure on aquatic ecosystems, eventually displacing conventional seafood to varying degrees, ranging from a cessation of the most harmful forms of fishing to complete displacement, for further conservation outcomes (GFI, 2019; Marwaha et al., 2020). To achieve conservation outcomes, including reduced human impact on aquatic environments, recovery of fish stocks, and other collateral ocean benefits, a series of sequential conditions must be met. Most notably, consumers must consistently substitute conventional seafood with alternative seafood at sufficient scale to substantially reduce demand for conventional seafood, which must translate to a decrease in price that is passed on to fishers and farmers who as a result, produce less (Farmery et al., 2020; Halpern et al., 2021). Because all the conditions are dependent on interactions between technological development, human and institutional behavior, market forces, and ecological linkages, the potential contribution of alternative seafood to improving aquatic ecosystem health remains speculative (Halpern et al., 2021).
Alternative seafood may have greater influence on aquatic ecosystem health if it augments or displaces the conventional supplies of certain species—namely, species that are overfished, difficult to farm without wild juveniles, or farmed species that rely on fishmeal and fish oil from poorly managed fish stocks (Halpern et al., 2021). However, this outcome is still dependent on the conditions outlined above, including those associated with demand-driven interventions (Roheim et al., 2018).
In LMICs, subsistence fishing and fish farming dominate and are essential for local livelihoods and food and nutrition security, making it unlikely that alternative seafood will have direct conservation outcomes (Halpern et al., 2021). Alternative seafood may, however, have indirect conservation outcomes if it slows the intensification of large-scale commercial fishing efforts and unsustainable aquaculture practices by providing less resource-intensive alternatives (Bell et al., 2017; Halpern et al., 2021).
Research on fish stocks in need of conservation, ecologically meaningful indicators for aquatic ecosystem health, the effect of alternative seafood on conventional seafood demand and potential negative outcomes, especially for displaced fishers or fish farmers, is necessary to characterize the extent of direct and indirect conservation outcomes associated with alternative seafood.
Discussion and Conclusions
Accelerating the development, commercialization and availability of alternative seafood is of growing interest for many who envisage inclusive, equitable, and sustainable food systems. This paper explores the foundation of this association through key economic, social and environmental impacts that are especially relevant to the millions of people in LMICs who depend on fisheries and aquaculture in diverse ways. Available literature and data suggest there is potential for alternative seafood to impact local and global food systems, but the nature and extent of these impacts depends on if and how the sector reaches scale, consumer behavior and governance. Figure 1 presents the discussed potential impacts of alternative seafood on LMIC food systems and offers some preliminary indicators for consideration when assessing the emerging sector, though they are worth revaluating regularly given the pace of development.
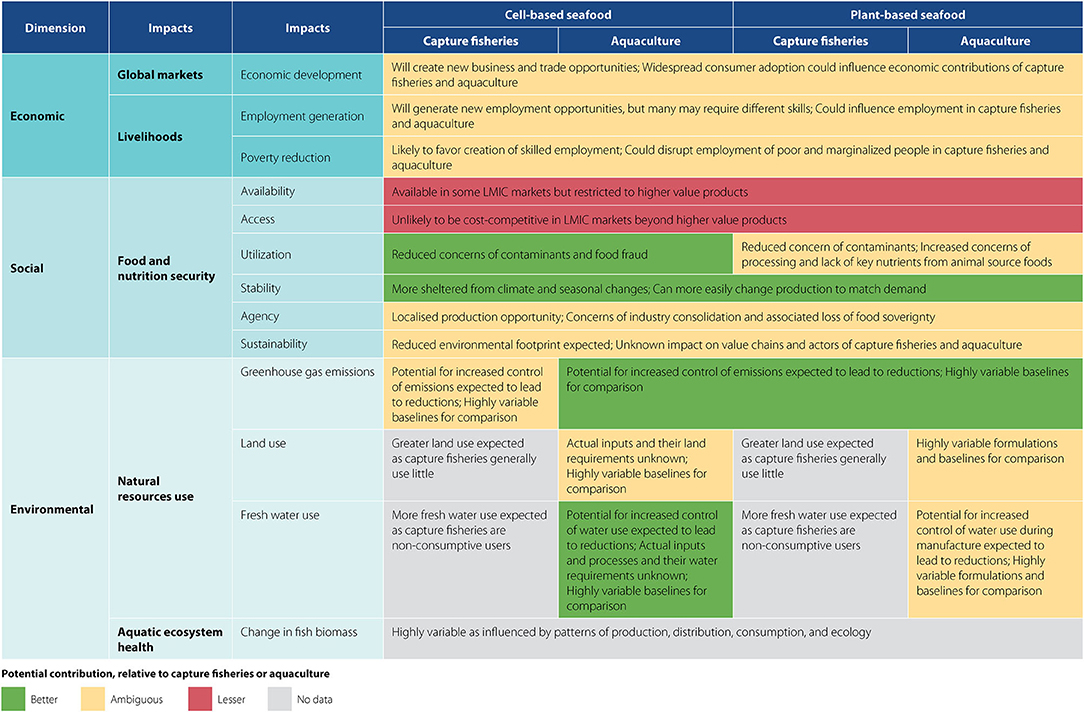
Figure 1. Summary of the economic, social and environmental contributions of alternative seafood production, trade and consumption in LMIC food systems by 2030, as compared to capture fisheries and aquaculture. Source: Authors' interpretation of the literature cited in this review.
Future Research
As an emergent food sector, society can influence the growth of alternative seafood to help achieve positive domestic and international outcomes. Further research on important knowledge gaps should inform appropriate development and governance of the alternative seafood sector. We recognize that some important issues including decent and meaningful livelihoods, social and gender equity, and animal welfare, although little discussed here, need further exploration.
It is essential to understand how markets for plant- and cell-based seafood are likely to develop in different regions, the main drivers of this development, and how these markets will interact with those for conventional seafood. Current growth projections are extrapolated from the alternative meat sector and the trajectories of individual businesses. However, the emerging nature and current small size of the alternative seafood sector makes it difficult to predict how it will interact with other sectors and be adopted by consumers. Government guidance is necessary, especially regarding broader societal impacts, such as production methods that allow for more consistent, reliable, and localized seafood supplies that are more resilient to food system shocks, as most recently and dramatically highlighted by the global COVID-19 pandemic.
Concrete policy and programme recommendations are necessary to guide the development of plant- and cell-based seafood to generate well-governed value chains that maximize societal benefits. This first requires an understanding of how plant- and cell-based seafood value chains are likely to differ from those of conventional seafood and an understanding of the influence of markets, policies or stakeholders on its development. It is also important to determine which areas of plant- and cell-based seafood value chains offer the greatest opportunities for decent employment, especially for women, youth and other marginalized groups.
For plant- and cell-based seafood to contribute to food and nutrition security, they need to successfully enter growing markets. There is first a question of accessibility of plant- and cell-based seafood to consumers in various geographic regions, economic classes, and cultural and social groups. Decisions by plant- and cell-based seafood producers regarding species, product form, and inputs can be made with intentions to reach specific markets. Likewise, there are important questions regarding the nutritional potential of plant- and cell-based seafood, how the nutrient profiles compare with those of conventional seafood, and how they might best be used as nutrient delivery platforms, especially where food-based solutions to hunger and malnutrition are being considered.
Further research around environmental impacts requires an assumption of how plant- and cell-based seafood will be adopted and development of a standardized, transparent assessment methodology that facilitates comparison between wild, farmed, plant-based and/or cell-based seafood. Standardized baseline data of species, product types, production systems, natural resources requirements, including energy, water, and land, and other inputs, including nutrients and feed, are also needed. With regards to aquatic ecosystem health, further research may explore various indicators of recovery and their associated timelines, the impact of species-specific changes in production, and enabling conditions or policies that allow for aquatic ecosystem recovery, including control of overfishing and illegal, unreported, and unregulated catches, especially in areas relied on by small-scale fishers and fish farmers.
Plant- and cell-based seafood may eventually generate similar food system outcomes, but the major differences in production, regulation, and marketability require some separate lines of research. Public and independently funded fundamental research may help level the playing field. Other research areas outside the scope of this review will likely influence the growth of the alternative seafood sector and also merit research, including seafood coproduct valorisation, use of plant-based extenders in seafood, and substitutes or alternative production methods for high quality animal feed ingredients, such as fishmeal and fish oil.
The potential of alternative seafood to contribute to inclusive, equitable, and sustainable food systems remains to be seen. Alternative seafood may complement existing initiatives for sustainable fisheries and aquaculture but could also introduce new stressors on food systems. The longevity of its popularity, its contributions to food and nutrition security, and its potential influence on the conventional seafood sector will help define its place as an emerging fad, common food, or feed ingredient. If, however, alternative seafood is here to stay, it is crucial that its development is supported by sound evidence, social and environmental standards are upheld, and planning and management is integrated with that of fisheries and aquaculture. Since the direct impacts of alternative seafood may be concentrated in HICs, at least initially, it is imperative that other methods to improve livelihoods, food and nutrition security, and the environment in LMICs are realized.
Data Availability Statement
The data generated for this study can be found in the WorldFish Dataverse at https://dataverse.harvard.edu/dataverse/worldfish.
Author Contributions
MP: conceptualization, funding acquisition, and project administration and supervision. NM and MB: literature and data curation. NM, MB, and MP: manuscript preparation. All authors contributed to the article and approved the submitted version.
Funding
This research was funded by National Philanthropic Trust but the review is a product of the authors.
Author Disclaimer
The findings, interpretations and conclusions expressed in this work do not necessarily reflect the views of National Philanthropic Trust, WorldFish, or the CGIAR.
Conflict of Interest
NM, MB, and MP were employed by WorldFish.
Publisher's Note
All claims expressed in this article are solely those of the authors and do not necessarily represent those of their affiliated organizations, or those of the publisher, the editors and the reviewers. Any product that may be evaluated in this article, or claim that may be made by its manufacturer, is not guaranteed or endorsed by the publisher.
Acknowledgments
We wish to thank the many individuals and organizations who generously contributed their time and knowledge to inform our research into the alternative seafood sector. We specifically thank the following people who were involved in our research process: Ahmed Khan (African Development Bank), Alan Tollervey (DFID), Bibi Giyose (NEPAD), Carrie Chan (Avant Meats), Chin Yee Chan and Delvene Boso and Doina Huso and Eddie Allison and Kazi Ahmed Kabir and Rose Komugisha Basiita and Shakuntala Thilsted (WorldFish), Chris Kerr (Unovis Asset Management), Dave Little (University of Stirling), David Benzaquen (Ocean Hugger Foods), David Guthrie and Michele Stanley (The Scottish Association for Marine Science), Esther Garrido and Molly Ahern (FAO), Georg Baunach and Supriya Srinivasan (Hatch), Greg Murphy (BlueNalu), Heather Lahr (University of California, Santa Barbara), Jen Lamy (The Good Food Institute), Jeremiah Johnstone (New Harvest), Jim Leape and Michelle Tigchelaar and Zach Koehn (Stanford Center for Ocean Solutions), Joyce Kinabo (Sokoine University of Agriculture), Ka Yi Ling (Shiok Meats), Keith Wiebe and Nicostrato D. Perez and Rowena Andrea Valmonte-Santos and Timothy Sulser (IFPRI), Kristopher Gasteratos (Cellular Agriculture Society), Maiko van der Meer (Novish), Mariko Powers (Oceankind), Mark Prein (GIZ), Max Troell (Beijer Institute, Stockholm Resilience Centre), Michael Selden and Shannon Cosentino-Roush (Finless Foods), Randall Brummett (World Bank), Robin Simsa (Revo Foods), Shivaun Leonard (USAID), Sloans Chimatiro (Pan-African Policy Research Network for Fisheries & Aquaculture, MwAPATA Institute), and Sophie Wood (World Resources Institute). We also extend thanks to Seong Lee Chua and Florine Lim for their expert figure design.
References
116th Congress. (2020). House Report 116-446 - Agriculture, Rural Development, Food and Drug Administration, and Related Agencies Appropriations Bill, 2021. Washington, DC: U.S. Government Publishing Office.
Aasim, M., Bakhsh, A., Sameeullah, M., Karatas, M., and Khawar, K. M. (2018). “Aquatic plants as human food,” in Global Perspectives on Underutilized Crops, eds M. Ozturk, K. Hakeem, M. Ashraf, and M. Ahmad (Cham: Springer International Publishing), 165–187.
Ahern, M., Thilsted, S. H., Oenema, S., and Kühnhold, H. (2021). The Role of Aquatic Foods in Sustainable Healthy Diets. New York, NY: UN Nutrition.
Attwood, S., and Hajat, C. (2020). How will the COVID-19 pandemic shape the future of meat consumption? Public Health Nutr. 23, 3116–3120. doi: 10.1017/S136898002000316X
Bailey, M., Bush, S. R., Miller, A., and Kochen, M. (2016). The role of traceability in transforming seafood governance in the global South. Curr. Opin. Environ. Sustain. 18, 25–32. doi: 10.1016/j.cosust.2015.06.004
Barange, M., Bahri, T., Beveridge, M. C. M., Cochrane, K. L., Funge-Smith, S., and Poulain, F. (2018). “Impacts of climate change on fisheries and aquaculture: synthesis of current knowledge, adaptation and mitigation options,” in FAO Fisheries and Aquaculture Technical Paper No. 627 (Rome).
Bell, J. D., Watson, R. A., and Ye, Y. (2017). Global fishing capacity and fishing effort from 1950 to 2012. Fish Fish. 18, 489–505. doi: 10.1111/faf.12187
Benjaminson, M. A., Gilchriest, J. A., and Lorenz, M. (2002). In vitro edible muscle protein production system (MPPS): stage 1, fish. Acta Astronaut. 51, 879–889. doi: 10.1016/S0094-5765(02)00033-4
Bohrer, B. M. (2019). An investigation of the formulation and nutritional composition of modern meat analogue products. Food Sci. Hum. Wellness 8, 320–329. doi: 10.1016/j.fshw.2019.11.006
Boland, M. J., Rae, A. N., Vereijken, J. M., Meuwissen, M. P. M., Fischer, A. R. H., van Boekel, M. A. J. S., et al. (2013). The future supply of animal-derived protein for human consumption. Trends Food Sci. Technol. 29, 62–73. doi: 10.1016/j.tifs.2012.07.002
Cabral, R. B., Bradley, D., Mayorga, J., Goodell, W., Friedlander, A. M., Sala, E., et al. (2020). A global network of marine protected areas for food. Proc. Natl. Acad. Sci. U. S. A. 117, 28134–28139. doi: 10.1073/pnas.2000174117
Cottrell, R. S., Blanchard, J. L., Halpern, B. S., Metian, M., and Froehlich, H. E. (2020). Global adoption of novel aquaculture feeds could substantially reduce forage fish demand by 2030. Nat. Food 1, 301–308. doi: 10.1038/s43016-020-0078-x
Cottrell, R. S., Ferraro, D. M., Blasco, G. D., Halpern, B. S., and Froehlich, H. E. (2021). The search for blue transitions in aquaculture-dominant countries. Fish Fish. 22, 1006–1023. doi: 10.1111/faf.12566
Crimarco, A., Springfield, S., Petlura, C., Streaty, T., Cunanan, K., Lee, J., et al. (2020). A randomized crossover trial on the effect of plant-based compared with animal-based meat on trimethylamine-N-oxide and cardiovascular disease risk factors in generally healthy adults: Study With Appetizing Plantfood—Meat Eating Alternative Trial (SWAP-MEAT). Am. J. Clin. Nutr. 112, 1188–1199. doi: 10.1093/ajcn/nqaa203
Datar, I., and Betti, M. (2010). Possibilities for an in vitro meat production system. Innovat. Food Sci. Emerg. Technol. 11, 13–22. doi: 10.1016/j.ifset.2009.10.007
De Backer, C., Teunissen, L., Cuykx, I., Decorte, P., Pabian, S., Gerritsen, S., et al. (2021). An Evaluation of the COVID-19 pandemic and perceived social distancing policies in relation to planning, selecting, and preparing healthy meals: an observational study in 38 countries worldwide. Front. Nutr. 7, 621726. doi: 10.3389/fnut.2020.621726
DeWeerdt, S. (2020). Can aquaculture overcome its sustainability challenges? Nature 588, S60–S62. doi: 10.1038/d41586-020-03446-3
Dolgin, E. (2019). Sizzling interest in lab-grown meat belies lack of basic research. Nature 566, 161–162. doi: 10.1038/d41586-019-00373-w
Dolgin, E. (2020). Will cell-based meat ever be a dinner staple? Nature 588, S64–S67. doi: 10.1038/d41586-020-03448-1
Duarte, C. M., Wu, J., Xiao, X., Bruhn, A., and Krause-Jensen, D. (2017). Can seaweed farming play a role in climate change mitigation and adaptation? Front. Mar. Sci. 4, 100. doi: 10.3389/fmars.2017.00100
FAO (2020). The State of World Fisheries and Aquaculture 2020. Sustainability in Action. Rome: Food and Agriculture Organization of the United Nations.
Farmery, A. K., van Putten, I. E., Phillipov, M., and McIlgorm, A. (2020). Are media messages to consume more under-utilized seafood species reliable? Fish Fish. 21, 844–855. doi: 10.1111/faf.12467
Fraeye, I., Kratka, M., Vandenburgh, H., and Thorrez, L. (2020). Sensorial and nutritional aspects of cultured meat in comparison to traditional meat: much to be inferred. Front. Nutr. 7, 35. doi: 10.3389/fnut.2020.00035
Froehlich, H. E., Afflerbach, J. C., Frazier, M., and Halpern, B. S. (2019). Blue growth potential to mitigate climate change through seaweed offsetting. Curr. Biol. 29, 3087–3093.e3. doi: 10.1016/j.cub.2019.07.041
Garnett, T. (2011). Where are the best opportunities for reducing greenhouse gas emissions in the food system (including the food chain)? Food Policy 36, S23–S32. doi: 10.1016/j.foodpol.2010.10.010
Gauthier, D. T. (2015). Bacterial zoonoses of fishes: a review and appraisal of evidence for linkages between fish and human infections. Vet. J. 203, 27–35. doi: 10.1016/j.tvjl.2014.10.028
GFI (2019). An Ocean of Opportunity: Plant-Based and Cell-Based Seafood for Sustainable Oceans Without Sacrifice. Washington, DC: Good Food Institute.
GFI (2020a). Fermentation: An Introduction to a Pillar of the Alternative Protein Industry. Washington, DC: Good Food Institute.
GFI (2021a). Assemblymember Kalra Introduces Bill to Help Smaller Farms Transition to Sustainable, Plant-Based Agriculture. Good Food Institute. Available online at: https://gfi.org/press/assemblymember-kalra-introduces-bill-to-help-smaller-farms-transition-to-sustainable-plant-based-agriculture/ (accessed March 8, 2021).
GFI (2021b). Decision Matrix for Seafood Target Species Selection. Good Food Institute. Available online at: https://gfi.org/solutions/decision-matrix-for-seafood-target-species-selection/ (accessed April 19, 2021).
GFI (2021c). Record $3.1 Billion Invested in Alt Proteins in 2020 Signals Growing Market Momentum. Good Food Institute. Available online at: https://gfi.org/blog/2020-state-of-the-industry-highlights/ (accessed April 19, 2021).
Godfray, H. C. J., Aveyard, P., Garnett, T., Hall, J. W., Key, T. J., Lorimer, J., et al. (2018). Meat consumption, health, and the environment. Science (80-.). 361. doi: 10.1126/science.aam5324
Halpern, B. S., Cottrell, R. S., Blanchard, J. L., Bouwman, L., Froehlich, H. E., Gephart, J. A., et al. (2019). Putting all foods on the same table: achieving sustainable food systems requires full accounting. Proc. Natl. Acad. Sci. U. S. A. 116, 18152–18156. doi: 10.1073/pnas.1913308116
Halpern, B. S., Maier, J., Lahr, H. J., Blasco, G., Costello, C., Cottrell, R. S., et al. (2021). The long and narrow path for novel cell-based seafood to reduce fishing pressure for marine ecosystem recovery. Fish Fish. 22, 652–664. doi: 10.1111/faf.12541
Herrero, M., Thornton, P. K., Mason-D'Croz, D., Palmer, J., Benton, T. G., Bodirsky, B. L., et al. (2020). Innovation can accelerate the transition towards a sustainable food system. Nat. Food 15, 266–272. doi: 10.1038/s43016-020-0074-1
Hicks, C. C., Cohen, P. J., Graham, N. A. J., Nash, K. L., Allison, E. H., D'Lima, C., et al. (2019). Harnessing global fisheries to tackle micronutrient deficiencies. Nature 574, 95–98. doi: 10.1038/s41586-019-1592-6
Johnson, E. A., and Schantz, E. J. (2017). “Seafood toxins,” in Foodborne Diseases, eds C. E. R. Dodd, T. Aldsworth, R. A. Stein, D. O. Cliver, and H. P. Riemann (Cambridge: Academic Press), 345–366.
Kazir, M., and Livney, Y. D. (2021). Plant-based seafood analogs. Molecules 26, 1559. doi: 10.3390/molecules26061559
Knight, C. J., Burnham, T. L. U., Mansfield, E. J., Crowder, L. B., and Micheli, F. (2020). COVID-19 reveals vulnerability of small-scale fisheries to global market systems. Lancet Planet. Heal. 4, e219. doi: 10.1016/S2542-5196(20)30128-5
Kruijssen, F., Tedesco, I., Ward, A., Pincus, L., Love, D., and Thorne-Lyman, A. L. (2020). Loss and waste in fish value chains: a review of the evidence from low and middle-income countries. Glob. Food Sec. 26, 100434. doi: 10.1016/j.gfs.2020.100434
Kyriakopoulou, K., Dekkers, B., and van der Goot, A. J. (2019). “Plant-based meat analogues,” in Sustainable Meat Production and Processing, ed C. Galanakis (Cambridge: Elsevier), 103–126.
Love, D. C., Allison, E. H., Asche, F., Belton, B., Cottrell, R. S., Froehlich, H. E., et al. (2021). Emerging COVID-19 impacts, responses, and lessons for building resilience in the seafood system. Glob. Food Sec. 28, 100494. doi: 10.1016/j.gfs.2021.100494
Lynch, J., Cain, M., Frame, D., and Pierrehumbert, R. (2021). Agriculture's contribution to climate change and role in mitigation is distinct from predominantly fossil CO2-emitting sectors. Front. Sustain. Food Syst. 4, 518039. doi: 10.3389/fsufs.2020.518039
Marwaha, N., Beveridge, M., Phillips, M. J., Komugisha, B. R., Notere Boso, D., Chan, C. Y., et al. (2020). Alternative Seafood: Assessing Food, Nutrition and Livelihood Futures of Plant-Based and Cell-Based Seafood. Penang: WorldFish.
McClenachan, L., Dissanayake, S. T. M., and Chen, X. (2016). Fair trade fish: consumer support for broader seafood sustainability. Fish Fish. 17, 825–838. doi: 10.1111/faf.12148
Monteiro, C. A., Cannon, G., Lawrence, M., Costa Louzada, M. L., and Pereira Machado, P. (2019). Ultra-Processed Foods, Diet Quality, and Health Using the NOVA Classification System. Rome: FAO.
Murphy, S. P., and Allen, L. H. (2003). Nutritional importance of animal source foods. J. Nutr. 133, 3932S−3935S. doi: 10.1093/jn/133.11.3932S
National Science Foundation (2020). NSF Award Search: Award#2021132. GCR: Laying the Scientific and Engineering Foundation for Sustainable Cultivated Meat Production. Available online at: https://www.nsf.gov/awardsearch/showAward?AWD_ID=2021132&HistoricalAwards=false (accessed September 18, 2020).
O'Meara, L., Cohen, P. J., Simmance, F., Marinda, P., Nagoli, J., Teoh, S. J., et al. (2021). Inland fisheries critical for the diet quality of young children in sub-Saharan Africa. Glob. Food Sec. 28, 100483. doi: 10.1016/j.gfs.2020.100483
Ong, K. J., Johnston, J., Datar, I., Sewalt, V., Holmes, D., and Shatkin, J. A. (2021). Food Safety Considerations and research priorities for the cultured meat and seafood industry. Compr. Rev. Food Sci. Food Saf. 20, 5421–5448. doi: 10.1111/1541-4337.12853
Post, M. J., Levenberg, S., Kaplan, D. L., Genovese, N., Fu, J., Bryant, C. J., et al. (2020). Scientific, sustainability and regulatory challenges of cultured meat. Nat. Food 1, 403–415. doi: 10.1038/s43016-020-0112-z
Potter, G., Smith, A. S. T., Vo, N. T. K., Muster, J., Weston, W., Bertero, A., et al. (2020). A more open approach is needed to develop cell-based fish technology: it starts with zebrafish. One Earth 3, 54–64. doi: 10.1016/j.oneear.2020.06.005
Risner, D., Li, F., Fell, J. S., Pace, S. A., Siegel, J. B., Tagkopoulos, I., et al. (2021). Preliminary techno-economic assessment of animal cell-based meat. Foods 10, 3. doi: 10.3390/foods10010003
Roheim, C. A., Bush, S. R., Asche, F., Sanchirico, J. N., and Uchida, H. (2018). Evolution and future of the sustainable seafood market. Nat. Sustain. 1, 392–398. doi: 10.1038/s41893-018-0115-z
Roque, B. M., Venegas, M., Kinley, R. D., Nys, R., de Duarte, T. L., Yang, X., et al. (2021). Red seaweed (Asparagopsis taxiformis) supplementation reduces enteric methane by over 80 percent in beef steers. PLoS ONE 16, e0247820. doi: 10.1371/journal.pone.0247820
Rubio, N., Datar, I., Stachura, D., Kaplan, D., and Krueger, K. (2019). Cell-based fish: a novel approach to seafood production and an opportunity for cellular agriculture. Front. Sustain. Food Syst. 3, 43. doi: 10.3389/fsufs.2019.00043
Rubio, N. R., Xiang, N., and Kaplan, D. L. (2020). Plant-based and cell-based approaches to meat production. Nat. Commun. 11, 1–11. doi: 10.1038/s41467-020-20061-y
Santo, R. E., Kim, B. F., Goldman, S. E., Dutkiewicz, J., Biehl, E. M. B., Bloem, M. W., et al. (2020). Considering plant-based meat substitutes and cell-based meats: a public health and food systems perspective. Front. Sustain. Food Syst. 4, 134. doi: 10.3389/fsufs.2020.00134
Scharf, A., Breitmayer, E., and Carus, M. (2019). Review and Gap-Analysis of LCA-Studies of Cultured Meat for The Good Food Institute. Hürth: nova-Institute GmbH.
Sinke, P., and Odegard, I. (2021). LCA of Cultivated Meat: Future Projections for Different Scenarios - CE Delft. Delft: CE Delft.
Specht, L. (2019). Why Plant-Based Meat Will Ultimately Be Less Expensive Than Conventional Meat. Good Food Institute. Available online at: https://www.gfi.org/plant-based-meat-will-be-less-expensive (accessed February 8, 2021).
Springmann, M., Clark, M., Mason-D'Croz, D., Wiebe, K., Bodirsky, B. L., Lassaletta, L., et al. (2018). Options for keeping the food system within environmental limits. Nature 562, 519–525. doi: 10.1038/s41586-018-0594-0
Stephens, N., Di Silvio, L., Dunsford, I., Ellis, M., Glencross, A., and Sexton, A. (2018). Bringing cultured meat to market: technical, socio-political, and regulatory challenges in cellular agriculture. Trends Food Sci. Technol. 78, 155–166. doi: 10.1016/j.tifs.2018.04.010
Thorrez, L., and Vandenburgh, H. (2019). Challenges in the quest for ‘clean meat.' Nat. Biotechnol. 37, 215–216. doi: 10.1038/s41587-019-0043-0
Vergeer, R., Sinke, P., and Odegard, I. (2021). TEA of Cultivated Meat: Future Projections of Different Scenarios. Delft: CE Delft.
Waltz, E. (2021). Club-goers take first bites of lab-made chicken. Nat. Biotechnol. 39, 257–258. doi: 10.1038/s41587-021-00855-1
White, E. R., Froehlich, H. E., Gephart, J. A., Cottrell, R. S., Branch, T. A., Agrawal Bejarano, R., et al. (2021). Early effects of COVID-19 on US fisheries and seafood consumption. Fish Fish. 22, 232–239. doi: 10.1111/faf.12525
Willett, W., Rockström, J., Loken, B., Springmann, M., Lang, T., Vermeulen, S., et al. (2019). Food in the Anthropocene: the EAT–Lancet Commission on healthy diets from sustainable food systems. Lancet 393, 447–492. doi: 10.1016/S0140-6736(18)31788-4
Keywords: alternative seafood, plant-based, cell-based, livelihoods, food and nutrition security, environment, aquatic health, biodiversity
Citation: Marwaha N, Beveridge MCM and Phillips MJ (2022) Fad, Food, or Feed: Alternative Seafood and Its Contribution to Food Systems. Front. Sustain. Food Syst. 6:750253. doi: 10.3389/fsufs.2022.750253
Received: 30 July 2021; Accepted: 24 February 2022;
Published: 04 April 2022.
Edited by:
Åsa Strand, Swedish Environmental Research Institute (IVL), SwedenReviewed by:
Richard S. Cottrell, University of California, Santa Barbara, United StatesBarry Antonio Costa-Pierce, Ecological Aquaculture Foundation LLC, United States
Copyright © 2022 Marwaha, Beveridge and Phillips. This is an open-access article distributed under the terms of the Creative Commons Attribution License (CC BY). The use, distribution or reproduction in other forums is permitted, provided the original author(s) and the copyright owner(s) are credited and that the original publication in this journal is cited, in accordance with accepted academic practice. No use, distribution or reproduction is permitted which does not comply with these terms.
*Correspondence: Nisha Marwaha, bm1hcndhaGEmI3gwMDA0MDt1Y2RhdmlzLmVkdQ==