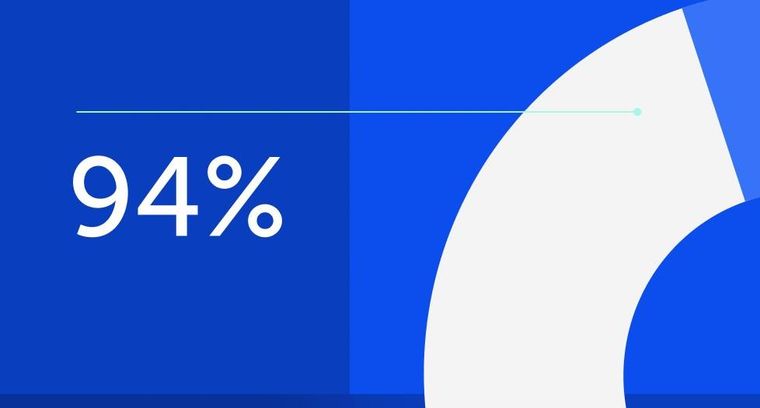
94% of researchers rate our articles as excellent or good
Learn more about the work of our research integrity team to safeguard the quality of each article we publish.
Find out more
ORIGINAL RESEARCH article
Front. Sustain. Food Syst., 01 March 2022
Sec. Agroecology and Ecosystem Services
Volume 6 - 2022 | https://doi.org/10.3389/fsufs.2022.688194
This article is part of the Research TopicEcosystem Services Underpinning Food Security in the African TropicsView all 4 articles
Endosphere and rhizosphere microbes offer plant growth promotion and pest suppression ecosystem services in banana-based agroecosystems. Interest has been growing towards the use of such beneficial microbes in protecting vulnerable tissue culture banana plants against pathogens such as Radopholus similis and Fusarium oxysporum. A screenhouse experiment with potted tissue culture banana plants was conducted using sterile and non-sterile soil to investigate the effect of soil biota on R. similis and F. oxysporum strain V5w2. Plants grown in non-sterile soil had lower damage and R. similis density in roots and rhizosphere, while most plant growth-related parameters including root freshweight, shoot freshweight, total freshweight, plant height, and leaf size were larger compared to those from sterile soil. Shoot dryweight and Mg content were higher in plants from sterile soil, while their leaves developed discolored margins. R. similis-inoculated plants in sterile soil were smaller, had more dead roots, higher nematode density, and produced fewer and smaller leaves, than those from non-sterile soil. For all plant growth-related parameters, nematode density and root damage, no differences were recorded between controls and F. oxysporum V5w2-inoculated plants; and no differences between those inoculated with R. similis only and the ones co-inoculated with the nematode and F. oxysporum V5w2. Banana roots inoculated with F. oxysporum V5w2 were lighter in color than those without the fungus. Independent or combined inoculation of banana plants with F. oxysporum V5w2 and R. similis resulted in lower optical density of root extracts. In vitro assays indicated the presence of Fusarium spp. and other root endophytic microbes that interacted antagonistically with the inoculated strain of F. oxysporum V5w2. It is concluded that, soil sterilization eliminates beneficial microbes that provide natural pest suppression ecosystem services against R. similis and F. oxysporum in the endosphere and rhizosphere of tissue culture banana plants. I recommend the integration of microbiome conservation into tissue culture technology through the proposed “Tissue Culture Microbiome Conservation Technology.”
Banana and plantains are important food crops among many communities in the developing countries around the world (Scott, 2021). The annual global production of banana and plantains has been estimated at about 145 million metric tons (Ploetz and Evans, 2015; Rocha et al., 2021), of which around 30% comes from Africa (Kikulwe and Asindu, 2020). Disease complexes involving plant-parasitic nematodes and Fusarium of Banana Complex (FOBC) remain a major threat to banana production and hence food security in Africa and many other regions around the world (Maryani et al., 2019; Rocha et al., 2020; Bedoya et al., 2021). In Eastern Africa, the production of highland cooking banana “Matooke” (Musa spp. genomic group AAA-EA), which is a very important staple food crop, is threatened by disease interactions between various species of plant parasitic nematodes especially Radopholus similis and the devastating Fusarium of Banana Complex (FOBC) (Kangire et al., 2000; Kangire and Rutherford, 2001; Talwana et al., 2016; Coyne et al., 2018; Van der Veken et al., 2021). R. similis among other nematode species have the potential to inflict as high as 50% yield loss (Speijer and Kajumba, 2000; Ssango et al., 2004), while the highly virulent stains of Fusarium species could just wipe out susceptible banana cultivars (Dita et al., 2018; Maymon et al., 2020). R. similis burrows into the root cortex of banana plants causing necrotic lesions and death of roots (Seenivasan, 2017). The consequences of root damage include impaired nutrient uptake and toppling of the banana trees. Besides this, wounds inflicted by the plant parasitic nematodes allow entry of pathogenic microbes especially the Fusarium species associated with root-rot and wilt diseases (Mai and Abawi, 1987; Speijer and Sikora, 1993; Back et al., 2002). Depending on prevailing conditions such as the availability of nutrients and agro-climatic factors (Gaidashova et al., 2009; Olivares et al., 2021), Fusarium-nematode disease interactions in susceptible plant cultivars are mostly synergistic characterized by increased nematode penetration and multiplication (Back et al., 2002; Rocha et al., 2020), enhanced root tissue and xylem colonization by the fungus (Dita et al., 2018; Pegg et al., 2019), resulting in stunted growth coupled with intensified rotting of roots, rhizomes and pseudostems and the ultimate wilting and death of banana plants (Kangire et al., 2000; Kangire and Rutherford, 2001; Jamil et al., 2019; Liu et al., 2020; Ochieno, 2020).
In the face of the global rise in food demand, ecosystem services, and technologies that limit the damage caused by plant-parasitic nematodes and fungal pathogens in banana and other food crops become of increasing importance. Plant-parasitic nematodes including R. similis and microbial pathogens such as the Fusarium of Banana Complex (FOBC) are readily transmitted through infected planting material (Dita et al., 2018; Jacobsen et al., 2019; Kumar et al., 2021). The highly aseptic tissue culture technology is efficient for mass production of certified clean banana planting material (Kumar et al., 2021). However, stringency in aseptic production of tissue culture banana plants not only eliminates harmful organisms, but also beneficial endophytic microbes that provide various ecosystem services including pest suppression (Dubois et al., 2006). Besides elimination of beneficial endophytic microbes, tissue culture banana plants are propagated in soil sterilized by various methods including steam-sterilization (Ochieno, 2010). This again eliminates beneficial rhizosphere biota associated with various plant growth promoting ecosystem services (Yu et al., 2019). Deficiency in pest-protective endosphere and rhizosphere microbes renders tissue culture banana plants susceptible to re-infestation by plant-parasitic nematodes and microbial pathogens in the field (Jacobsen et al., 2019).
To address this draw-back, the innovative concept of re-introducing beneficial pest-protective endophytic microbes such as Fusarium oxysporum V5w2 into tissue culture banana plants was developed (Dubois et al., 2004; Paparu et al., 2006a,b; Sikora and Pocasangre, 2006; Sikora et al., 2008), while this endophyte-enhanced banana tissue culture technology had already been availed to farmers in Kenya and Uganda from the year 2006 (Dubois et al., 2006, 2011; zum Felde, 2011; Waweru et al., 2014). Induced systemic resistance is the main mechanism through which F. oxysporum V5w2 among other strains suppress various pests and diseases of banana (Vu et al., 2006; Paparu et al., 2007, 2009a,b, 2010, 2013). However, future research is still necessary to elucidate the mechanisms underpinning nematode suppression and increased banana yield observed after treatment with F. oxysporum V5w2 (Kisaakye et al., 2022). Besides this, F. oxysporum V5w2 has been reported to be a non-beneficial endophyte that interacts with R. similis in a wilt disease complex of banana (Ochieno, 2020, 2021). Therefore, more information has still been pending in the registration of F. oxysporum V5w2 as a biopesticide through the Kenya Standing Technical Committee on Imports and Exports of live organisms (KSTCIE) (Hoeschle-Zeledon et al., 2013; Avedi et al., 2014; KEPHIS, 2014; Ochieno, 2020). This is partly because in the year 2008, the concept of using various strains of F. oxysporum as endophytic beneficial microbes in banana and other crops was considered not well-understood (Sikora et al., 2008).
In the year 2010, induced systemic resistance was found to be unlikely mechanism of F. oxysporum V5w2, while its pathogenicity remained unresolved despite the fungus not being genetically very closely related to the three pathogenic strains of F. oxysporum f. sp. cubense (Foc) tropical race 4 that included Fo001 (VCG 0120), JC1 (VCG 01210), and II5 (VCG 01213) (Kurtz, 2010). Furthermore, F. oxysporum V5w2 was among 16 strains originating from Uganda, Kenya, Costa Rica, Cuba and Indonesia that were found not to be compatible with any known vegetative compatibility group (VCG) of F. oxysporum f. sp. cubense or with any non-pathogenic isolate (Niere, 2001; Kurtz, 2010). Also, it was concluded that F. oxysporum V5w2 was a plant growth suppressing microbe (Ochieno, 2010) (Figures 1A,B), which has the potential of invading banana xylem through the rhizosphere just like the known pathogenic strains (Paparu et al., 2009a; Warman and Aitken, 2018; Ochieno, 2020). F. oxysporum V5w2 was therefore considered not to be a beneficial endophyte that offers biological control of banana pests through induced systemic resistance mechanisms (Ochieno, 2020). However, between the year 2010 and 2014, there were more evidences supporting the retention of F. oxysporum V5w2 as an endophytic biological control agent of banana pests (Paparu et al., 2010, 2013; Dubois et al., 2011; zum Felde, 2011; Waweru et al., 2013, 2014). Issues concerning the application of F. oxysporum V5w2 as an endophytic biological control agent have since remained in an open debate requiring further evidences toward consensus (Ochieno, 2020).
Figure 1. Tissue culture banana plants inoculated with strains of Fusarium oxysporum. (A) An experimental plot of East African Highland Cooking Banana plants (genomic group Musa AAA-EA, cv. Kisansa) inoculated with wild-type F. oxysporum V5w2 expressing stuntedness and yellowing of leaves flanked by two plots with relatively healthier plants of the same cultivar. The plants had been transferred to the field on 18th January 2007 and the photograph taken 10 months later on 19th November 2007 (Source: Own work). (B) Potted East African Highland Cooking Banana plants (genomic group Musa AAA-EA, cv. Kibuzi) co-inoculated inoculated with chlorate-resistant F. oxysporum V5w2 and Radopholus similis expressing wilt-like symptoms (2 right) and those inoculated with F. oxysporum V5w2 only (2 left). All plants were grown in sterile beach sand collected from Lake Victoria and supplied with phosphorus-deficient nutrient solution (Source: Own work).
The objective of the present study was to determine the effect of soil sterilization, and hence elimination of soil biota, on the suppression of R. similis and F. oxysporum V5w2 in tissue culture banana plants grown under adequate supply of nutrients. It is hypothesized that under proper nourishment, rhizosphere biota positively interact with banana roots to suppress R. similis and F. oxysporum V5w2 thereby promoting plant growth.
The treatments, viz. R. similis nematode vs. no nematode, F. oxysporum vs. no fungus, sterile vs. non-sterile soil were studied in a full factorial experiment (2 × 2 × 2). This resulted in 8 treatment combinations that comprised the untreated controls, R. similis, F. oxysporum, and R. similis + F. oxysporum, each having been conducted in sterile and non-sterile soil. The eight (8) treatment combinations, each having n=15 plants, were in three (3) replicates and repeated twice (2) over time to result in nine (9) sets of plants per treatment combination. The plants were completely randomized in the screenhouse. One hundred days after the start of the experiment, data were collected on parameters that included (i) biomass (root, shoot dry weight, total biomass), (ii) percentage dead roots, (iii) percentage root necrosis, (iv) R. similis densities in roots and soil, as well as the presence of other nematode species (vi) number of standing leaves, (vii) leaf length and width, (viii) percentage root piece colonization by F. oxysporum, (ix) identities of root-invading microbes and their interactions with F. oxysporum, (x) root color, (xi) optical density of root extracts, and (xii) leaf nutrient content.
Tissue culture banana plants (genomic group AAA-EA, cv. Kibuzi) (Onguso et al., 2004; Akankwasa et al., 2021), were obtained from the Banana Tissue Culture Laboratory at Sendusu Field Station in IITA-Uganda. The plants had been micro-propagated by the shoot-tip culture technique in which plants are produced by multiplication of the meristematic tissue of banana corms (Vuylsteke, 1998). The banana plants had been maintained for 6 weeks in nutrient solution (1 g/L, Polyfeed™, Haifa Chemicals, Israel), after which they were graded into four sizes and distributed to eight groups of 15 plants. Four groups were marked for inoculation with F. oxysporum and the other four were unmarked.
Pre-planting initial plant growth parameters were recorded through a slight modification of the method described by Akello et al. (2007) and Paparu et al. (2009b). Initial plant height (the distance from the base of the plant to the youngest leaf axil), initial number of open leaves, and initial width (widest part of the lamina) and initial length (the distance from the leaf apex to the leaf stalk) of the largest open leaf were recorded using a measuring tape. Initial fresh weight of each plant was measured using a toploading laboratory balance (Mettler Toledo™, Columbus, Ohio, United States), and the number of healthy-looking functional roots recorded.
The fungus used was chlorate-resistant Fusarium oxysporum strain V5w2 that had originally been meant to be an endophytic biological control agent (Niere et al., 1999; Paparu et al., 2009b; Dubois et al., 2011; zum Felde, 2011; Waweru et al., 2013, 2014), but currently considered to be a non-beneficial plant growth suppressing microbe (Ochieno, 2010, 2020). Inoculum was prepared by sprinkling the soil culture onto 90 mm diameter glass Petri dishes containing half-strength PDA (19 g/L, Sigma-Aldrich, Germany). Ten days later, the fungal spores (conidia) and mycelia were scraped from the media into a 500 mL beaker containing sterile water. The spore mixture was sieved (1 mm aperture) and homogenized, and then adjusted to 1.5 × 106 spores/mL.
Radopholus similis were obtained from cultures aseptically maintained on carrot discs at the Nematology Laboratory in Sendusu Field Station of IITA-Uganda (Speijer and De Waele, 1997; Ochieno, 2010). The nematodes were supplied in sterile water suspension containing mixed stages of 250 R. similis /mL.
Loamy top soil (0–10 cm) was collected from a bare 5-year-old banana field at the Sendusu Field Station of IITA-Uganda. The soil at this site has been classified as isohyperthermic Rhodic Kandiudalf (USDA taxonomy) (McIntyre et al., 2000). The soil was sieved (5 mm aperture) and thoroughly mixed. Soil samples were analyzed for chemical properties at the National Agricultural Research Laboratories at Kawanda-Uganda. Available phosphorus (P) and exchangeable potassium (K) were extracted using the Mehlich-3 method (Mehlich, 1984). Phosphorus in the extract was determined using the molybdenum blue colorimetric method and potassium using a flame photometer (Okalebo et al., 2002). Total nitrogen (N) was analyzed by Kjeldahl oxidation and semi-micro Kjeldahl distillation (Bremner, 1960). Organic matter (OM) was determined using the Walkley-Black method (Walkley, 1947). Soil pH was analyzed using de-ionized water with a soil to water ratio of 1:2.5. The soil contained N (0.15%), P (3.6 ppm), K (0.38 cmolc/kg), OM (2.4%) and a pH of 5.1. Part of the loamy soil was steam-sterilized at 100°C for 1 h using an electrode steam conditioner (Model ESC40, Marshall-Fowler, South Africa).
Plants that were marked for F. oxysporum V5w2 treatment were inoculated by the root-dipping technique (Paparu et al., 2006a; Ochieno, 2010), except that in the present study the roots were left undamaged. The roots were immersed for 4 h into a 1.5 L spore suspension contained in tubs (30 × 25 × 15 cm, length × width × height). Control plants were immersed in distilled water.
The banana plants were grown in 2.5 L buckets containing either sterile or non-sterile soil under screenhouse conditions (25 ± 3°C, 70–75% RH, 12L: 12D photoperiod). Post-planting initial height, plus length and width of the youngest open leaf were recorded. The plants were supplied with rain water (100 mL) daily and a complete nutrient solution (CNS, 100 mL) weekly. One liter of CNS contained 1,650 mg NH4NO3, 1900 mg KNO3, 440 mg CaCl2.2H2O, 370 mg MgSO4.7H2O, 170 mg KH2PO4, 37.3 mg Na2EDTA.2H2O, 27.8 mg FeSO4.7H2O, 6.2 mg H3BO3, 22.3 mg MnSO4.4H2O, 8.6 mg ZnSO4.7H2O, 0.83 mg KI, 0.25 mg Na2MoO4.2H2O, 0.025 mg CuSO4.5H2O, and 0.025 mg CoCl2.6H2O (Murashige and Skoog, 1962). Twenty days after planting, three holes (5 cm deep) were made into the soil around the base of each plant, by using a disinfected stick. Each plant was inoculated with 500 R. similis in 2 mL of the nematode suspension distributed across the three holes before covering them with soil.
At 100 days after nematode inoculation, final plant growth parameters that included plant height (the distance from the base of the plant to the youngest leaf axil), number of fully developed leaves, and width (widest part of the lamina) and length (the distance from the leaf apex to the leaf stalk) of the youngest leaf were recorded using a measuring tape based on Akello et al. (2007) and Paparu et al. (2009b). Plants were harvested and the number of dead and healthy roots were recorded and used to calculate the percentage dead roots. The shoots and roots were detached and their fresh weights recorded using a toploading laboratory balance (Mettler Toledo™, Columbus, Ohio, United States). Root and shoot biomasses were combined to obtain total fresh weight. The roots were preserved at 4°C within 3 days. The shoots were oven-dried at 70°C for 14 days to determine dry weight. From these drying banana shoots, two youngest open leaves were pre-selected from seven randomly chosen banana plants for the determination of nutrient contents. Samples for each banana plant were mixed and analyzed separately for nitrogen (N), phosphorus (P), potassium (K), calcium (Ca), magnesium (Mg), and Zinc (Zn) in the Soil and Plant Analytical Laboratories at Kawanda Agricultural Research Institute, Kampala, Uganda.
From the preserved banana roots, 5 roots per plant were randomly selected for assessment of necrosis caused by nematodes, and for estimation of nematode densities. Three roots were randomly selected per plant to estimate the level of colonization by F. oxysporum V5w2 and identify other endophytic microbes.
Assessment of root necrotic lesions caused by R. similis and quantification of nematode densities were undertaken based on the methods of Speijer and De Waele (1997) and Brooks (2004). Each of the five roots selected per plant for necrosis assessment was cut into 10 cm length. The pieces were then split longitudinally and one half selected from each of them. The cumulative length of necrotic lesions of cortical tissue on each 10 cm side of the stele was recorded as a fraction of the total 20 cm length per root. The sum length of the necrotic tissue for five roots per plant was then expressed as percentage necrosis. These roots were chopped into small pieces and 5 g sub-samples were mixed with 50 mL of water, and macerated at medium speed for 20 s using a Waring® laboratory blender. Nematodes were extracted from the suspension using a Baermann tray for 24 h. The extracts were transferred into 100 mL glass bottles and stored at 4°C for 24 h to allow the nematodes to sediment. The water volume was standardized to 25 mL by gentle siphoning from the surface. The suspension was homogenized by stirring, and nematodes quantified on a counting dish at ×10 magnifications from triplicate 2.5 mL pipette sub-samples. The nematode densities were expressed as R. similis counts per 100 g of root material. To investigate whether densities of R. similis varied in the rhizosphere between sterile and non-sterile soil, eight 100 mL soil samples were selected from each soil treatment. This was done for plants that were treated with R. similis only. R. similis and other nematode species in the soil samples were extracted using a Baermann tray over a period of 48 h. The extract was concentrated to 25 mL followed by concurrent morphological identification nematode species and absolute counts of R. similis in the sample based on nematode identification keys (Fortuner, 1988; Luc et al., 2005).
Each of the three roots selected for estimation of colonization by F. oxysporum V5w2 and infection by other endophytic microbes was surface-sterilized by dipping it into 96% ethanol followed by flaming. This ensured that only microbes within the tissues remained alive. Each root was then cut into six pieces. The root pieces were plated on 60 mm glass Petri dishes containing PDA enriched with KClO3 for 7 days. Growth of Fusarium strains including V5w2 on PDA is characterized by whitish mycelia with pink pigmentation (Leslie and Summerell, 2008). The number of root pieces with growth of Fusarium species among six assessed pieces was recorded fungal endophyte colonization. Root piece colonization was the percentage of all root pieces yielding Fusarium among all assessed pieces within a treatment. Other root-invading microbes growing from the root pieces were sub-cultured and morphologically identified through the TSBF-CIAT project on Below-Ground Biodiversity (BGBD) funded by GEF-UNEP at the Soil Microbiology Laboratory, Makerere University, Uganda (Nakintu et al., 2008; Ochieno, 2010). In vitro growth inhibition tests between the microbes and F. oxysporum V5w2 were conducted. Fusarium oxysporum V5w2 was inoculated in the center of a glass Petri dish containing PDA using a sterile needle. The microbes were inoculated on four spots surrounding the fungal pathogen. The fungal pathogen or the test microbe was considered inhibitive if its mycelia grew into the colonies of the other marked with a boundary between them.
It was realized that roots that were stored at 4°C exhibited differences in color after 3 days. Therefore, visual and colorimetric tests on roots and their extracts was conducted to assess possible effects of the experimental treatments on root color. Roots from all plants per treatment were mixed. This was followed by random selection of up to 30 roots from every treatment; the color of the external root surface was visually recorded as either brown or light-brown by comparing with two roots that had been pre-selected as standards for the two colors. For colorimetric assessments, five roots from three plants per treatment were chopped together into a composite sample. This was conducted in triplicate. Ten grams of each composite sample were mixed with 100 mL distilled water, and macerated using a Waring Laboratory Blender (Christison Scientific, Gateshead, UK). The root extracts were diluted (10×) and their optical density (absorbance) measured at 320 nm using a spectrophotometer (Genesys 10 UV, Thermo Fisher Scientific, USA).
Data were analyzed using SAS 9.1 software (SAS, 2004). Diagnostic check for normality was conducted using Proc Univariate. Proc Transreg was used to find appropriate Box-Cox transformations, which included generation of suitable powers or lambda (λ) for data (Y) that required transformation. Percentage dead roots and percentage necrosis (x) were arcsine√(x/100) transformed. No transformation was done on data for other parameters. Proc GLM was used for three-way factorial analyses of variance (ANOVA) among the treatments. Mean separation was done by t-tests (LSD) when there were significant differences among treatment means. Mean separation of optical density of root extracts was done by LS-Means with Bonferroni adjustment for multiple comparisons. Root piece colonization by Fusarium was analyzed by Proc Genmod as data having binomial distribution; Bonferroni-adjusted p-values were obtained by Proc Multtest from raw p-values in Proc Genmod, and used for pairwise comparisons between treatments. Root coloration data were analyzed by Proc Genmod and pairwise comparisons using contrasts.
Pre-planting initial plant growth parameters did not vary between the treatments, and they included fresh weight (7.9 ± 0.13 g), plant height (5.5 ± 0.08 cm), number of leaves (3.4 ± 0.02), leaf length (12.2 ± 0.10 cm), leaf width (4.6 ± 0.05 cm), and number of functional roots (3.6 ± 0.04) (n = 1,084, mean ± SE, F-test, p > 0.05).
At the end of the experiment, rhizosphere populations of Radopholus similis in pots of nematode-inoculated plants were significantly higher in sterile soil than in non-sterile soil (Figure 2). Dead roots and those having necrotic lesions were mainly found in R. similis-inoculated plants (Figure 3A). Percentage dead roots in sterile soil was significantly higher than in non-sterile soil (Table 1). Percentage root necrosis was significantly higher in R. similis-inoculated plants than in plants not inoculated with the nematode (Table 1).
Figure 2. Density of Radopholus similis in the rhizospheres of potted tissue culture banana plants grown in non-sterile soil and sterile soil after 100 days of inoculation with the nematode. Bars with the same letter represent values that are not statistically different (df = 1, F = 24.24, p = 0.0002).
Figure 3. Banana root damage caused by Radopholus similis. (A) Healthy-looking banana roots (left) and those having necrotic lesions associated with Radopholus similis infection (right). (B) Female R. similis in root cortical tissues of banana plants.
Table 1. Effect of soil sterility on infection symptoms of Radopholus similis and Fusarium oxysporum in tissue culture banana plants grown under adequate nutrient supply.
R. similis were mainly found in plants inoculated with the nematode (Figure 3B), and their density in roots from sterile soil was higher than in roots from non-sterile soil (Table 1). Thirteen other nematode species, identified mainly in non-sterile soil among the soil samples, included; the plant parasitic Helicotylenchus multicintus, Meloidogyne spp., Pratylenchus goodeyi, Xiphinema spp., Trophorus spp., Scutellonema spp., Rotylenchus spp., Tylenchus spp., Hemicycliophora spp., Paratrichodorus spp., and Ditylenchus spp. Saprophytic genera included Aphelenchus spp. and Rhabditis spp. among numerous others that could not be identified. The first three nematode species were frequently recorded in root samples from non-sterile soil.
Banana plants in the different treatments showed no visually clear differences in their size (Figure 4). However, leaf margins of all banana plants grown in sterile soil developed a characteristic yellow coloration especially from the older lower leaves toward the upper second youngest open leaves (Figure 4). This discoloration of leaf margins did not occur in banana plants grown in non-sterile soil. R. similis-inoculated plants from non-sterile soil had more biomass (root freshweight, shoot freshweight, total freshweight) than those from sterile soil (Figure 5; Table 1). R. similis-inoculated plants had lower shoot dry weights than those not inoculated with the nematode (Figure 5; Table 1). Plants from non-sterile soil had lower shoot dry weights than those from sterile soil (Figure 5; Table 1).
Figure 4. Tissue culture banana plants grown in potted sterile soil and supplied with completer nutrient solution. (A) Control plants (Left pots labeled P) and those inoculated with Fusarium oxysporum V5w2 (Right pots labeled D) all having been grown in sterile soil. (B) Plants co-inoculated with Radopholus similis and Fusarium oxysporum V5w2 (Left pots labeled A) and those inoculated with Fusarium oxysporum V5w2 only (Right pots labeled M) all having been grown in sterile soil. Leaf margins of all banana plants grown in sterile soil developed a characteristic yellow coloration especially from the older lower leaves toward the upper second youngest open leaves.
Figure 5. Biomasses of potted tissue culture banana plants either inoculated with Radopholus similis or not inoculated with the nematode and grown in either sterile soil or non-sterile soil supplied with complete nutrient solution. (A) Root freshweight, (B) Shoot freshweight, (C) Shoot dryweight, and (D) Total plant freshweight. Bars with the same letter represent values that are not statistically different (p > 0.05; more details in Table 1).
Leaves of R. similis-inoculated plants from sterile soil were fewer, shorter and narrower than for plants from non-sterile soil (Figure 6; Table 1). The R. similis-inoculated plants were shorter than plants that were not inoculated with the nematode (Figure 6; Table 1). Plants in non-sterile soil were taller than plants from sterile soil (Figure 6; Table 1). Fusarium oxysporum V5w2 inoculant did not induce significant effects on all the assessed plant growth-related parameters, R. similis density and root damage by the nematode (Table 1).
Figure 6. Sizes of potted tissue culture banana plants either inoculated with Radopholus similis or not inoculated with the nematode and grown in either sterile soil or non-sterile soil supplied with complete nutrient solution. (A) Plant height, (B) Number of leaves, (C) Leaf length, and (D) Leaf width. Bars with the same letter represent values that are not statistically different (p > 0.05; more details in Table 1).
In the main treatment effects and interactions, leaf tissue nutrient analysis showed that plants grown in non-sterile soil had higher concentrations of N, P, K, Ca and Zn but lower Mg when compared to those grown in sterile soil (Table 2). In the main treatment effects, the concentrations of N, K, Ca, and Mg were higher in R. similis-inoculated plants while P and Zn were lower when compared to those not inoculated with the nematode (Table 2). In the main treatment effects, the concentrations of N, P, K and Zn were higher in F. oxysporum V5w2-inoculated plants while Ca and Mg were lower when compared to those not inoculated with the fungus (Table 2).
Table 2. Nutrient content in banana leave tissues from plants under independent and combined inoculation with Radopholus similis and Fusarium oxysporum V5w2 having grown in sterile or non-sterile soil and supplied with complete nutrient solution.
Fusarium spp. were recorded in roots from all the eight treatments including the controls (Figure 7). The proportion of root pieces that yielded Fusarium spp. was lower than 30%; percentage root piece colonization by Fusarium spp. was lowest in the control in sterile soil and highest in the R. similis + F. oxysporum V5w2 treatment in sterile soil, the other six treatments were not different (Figure 7).
Figure 7. Percentage root piece colonization by Fusarium spp. in potted tissue culture banana plants under independent or combined inoculation with Fusarium oxysporum V5w2 and Radopholus similis, grown in either sterile soil or non-sterile soil supplied with complete nutrient solution. Bars with the same letter represent values that are not statistically different (p > 0.05), with the sample sizes indicated below the letters (df = 7, χ2 = 118.21, p < 0.0001).
Fusarium oxysporum V5w2 could not be visually distinguished from other Fusarium spp. that grew from root pieces that had been plated in KClO3-enriched PDA (Figure 8). Twenty-five other endophytic fungi were isolated from the banana root pieces (Figure 8), 11 genera among them were identified and included; Fusarium spp., Rhizoctonia spp., Trichoderma spp., Sclerotium spp., Bipolaris spp., Curvularia spp., Mucor spp., Rhizopus spp., Papulaspora spp., Pleurophragmium spp., and Trichocladium spp. At least 14 of the microbes that included Rhizoctonia spp., Trichoderma spp., Sclerotium spp., Curvularia spp., Rhizopus spp., Papulaspora spp., Pleurophragmium spp., and Trichocladium spp. inhibited F. oxysporum V5w2 in vitro; seven were inhibited by F. oxysporum V5w2 among them Sclerotium sp. Bipolaris and Mucor among other unidentified microbes showed no negative interactions with F. oxysporum V5w2. Among the Fusarium spp. that were identified, some inhibited F. oxysporum V5w2, others were inhibited while the remaining ones did not interact antagonistically with the inoculated fungus.
Figure 8. Fusarium spp. and other endophytic microbes isolated from potted tissue culture banana plants under independent or combined inoculation with Fusarium oxysporum V5w2 and Radopholus similis, and grown in either sterile soil or non-sterile soil supplied with complete nutrient solution. (A) F. oxysporum V5w2 can be seen with pinkish-white mycelia at the central spot surrounded by an endophytic fungus with brownish mycelia isolated from the banana roots in a Petri dish containing potato dextrose agar (PDA). (B) F. oxysporum V5w2 at the central spot surrounded by Fusarium spp. isolated from non-inoculated plants (third right). (C) Diversity of endophytic microbes isolated from roots of tissue culture banana plants especially those grown in non-sterile soil.
The four groups of F. oxysporum V5w2-inoculated plants varied significantly in frequency of externally light-brown colored roots, with absence of light-brown roots in plants that were not inoculated with the fungus (Figure 9). In both sterile and non-sterile soil, F. oxysporum V5w2-inoculated plants had higher frequency of light-brown roots as compared to those inoculated with R. similis + F. oxysporum V5w2. The frequency of light-brown roots was higher in sterile than in non-sterile soil in both F. oxysporum V5w2 and R. similis + F. oxysporum V5w2 treatments.
Figure 9. Percentage of light-brown roots in potted tissue culture banana plants under independent or combined inoculation with Fusarium oxysporum V5w2 and Radopholus similis, and grown in either sterile soil or non-sterile soil supplied with complete nutrient solution. Bars with the same letter represent values that are not statistically different (p > 0.05), with the sample sizes indicated below the letters (df = 3, χ2 = 59.67, p < 0.0001).
Optical density of root extracts from plants that were treated with the F. oxysporum V5w2 or with R. similis was lower than for control plants (Figure 10). The combination of R. similis and F. oxysporum V5w2 treatment resulted in a similar optical density as any of the two treatments alone. Soil sterility did not affect the optical density of root extracts.
Figure 10. Optical density of root extracts from potted tissue culture banana plants under independent or combined inoculation with Fusarium oxysporum V5w2 and Radopholus similis, and grown in either sterile soil or non-sterile soil supplied with complete nutrient solution. Bars with the same letter represent values that are not statistically different [F(7,16) = 14.79, p < 0.0001].
Under the conditions of the current study, increased necrosis and percentage dead roots were apparent in all banana plants that were inoculated with R. similis resulting in a lower living root biomass. This confirms the pest potential of R. similis (Huang et al., 2019). Density of R. similis and percentage dead roots were lower in banana plants that were grown in non-sterile soil than in sterile soil. This is an indication of the presence of nematode-suppressive biota in the non-sterile soil (Topalović et al., 2020; Jayaraman et al., 2021). It is likely that soil biota such as the root-invading microbes that were identified in samples from non-sterile soil help suppress R. similis and other nematodes. Further suppression of soil populations of R. similis in the present experiment may have been a result of competition by the other identified plant parasitic nematodes namely; H. multicintus, Meloidogyne spp., and P. goodeyi. Competitive interaction between R. similis and other plant parasitic nematodes has been reported (Moens et al., 2006), with R. similis appearing to suppress the population of Meloidogyne spp. in roots of field-grown banana plants (Ochieno, 2010).
Banana plants grown in non-sterile soil exhibited better growth, had heavier roots and more leaves compared to those planted in sterile soil. This plant growth promotion in non-sterile soil has been attributed to root infection by beneficial microbes such as vesicular arbuscular mycorrhizal fungi (AMF) that symbiotically enhance the acquisition of nutrients, and especially phosphorus (Gough et al., 2020). Evidence from the present study shows that leaves from plants grown in non-sterile soil accumulated high concentrations of N, P, K, Ca, and Zn. Such effects on leaf nutrient content were also partly evident in plants inoculated with R. similis or F. oxysporum V5w2, considering that these organisms also are part of the functional biota in the non-sterile soil rhizosphere. The provision of complete nutrient solution may have shifted the function of rhizosphere biota toward improved nutrient acquisition (Ochieno, 2010). However, poor growth of banana plants in steam-sterilized soil may have been due to modification of nutrient compounds to less available forms (Evan et al., 2003; De Deyn et al., 2004; Dietrich et al., 2020). Soil sterilization therefore eliminates the rhizosphere ecosystem services associated with nutrient acquisition and cycling by beneficial microbes (Jacoby et al., 2017; Li et al., 2019).
Interestingly, banana plants grown in sterile soil had higher shoot dryweight than those from non-sterile soil. Although this increased shoot dryweight may be interpreted as better plant growth in sterile soil, the yellowing of banana leaf margins may point to an onset of a nutrient stress possibly related to magnesium deficiency (He et al., 2020). Banana plants growing under Mg deficiency exhibit the accumulation of sugars like sucrose and amylose in the leaves that may have contributed to the increase in shoot dryweight (He et al., 2020; Ogura et al., 2020). However, in the present study, leaf tissues of banana plants grown in sterile soil had higher Mg content than those from non-sterile soil, raising questions on whether these plants were actually Mg deficient. It is possible that the banana plants mobilized Mg2+ from lower older leaves toward the upper younger ones in response to the nutrient deficiency (Ogura et al., 2020). This may partly explain the higher Mg content in the sampled youngest open leaves of banana plants grown in sterile soil. There are varied explanations on the manifestation of Mg deficiency in plants (Hauer-Jákli and Tränkner, 2019; Chaudhry et al., 2021), considering that the associated symptoms may even be expressed in plants growing in soils with high contents of this very important nutrient (Jezek et al., 2015). For instance, Mg deficiency may be induced through antagonistic effects of K+ or Ca2+ ions or decline in soil pH that limit the uptake and utilization of Mg2+ (He et al., 2020; Wang et al., 2020; Chaudhry et al., 2021). Although soil sterilization increases the availability of soil nutrients (De Deyn et al., 2004), this may also create nutrient imbalances that may affect the uptake and utilization of Mg2+ by plants (Raghupathi et al., 2002; Wairegi and van Asten, 2011). This may have been the case in the present study, considering that the banana plants grown in sterile soil kept receiving 100 mL of complete nutrient solution through a microbe-deficient rhizosphere. Rhizosphere microbes in the non-sterile rhizospheres may have shared the supplied nutrients with banana roots while engaging in nutrient cycling activities (Shen et al., 2015). The growing banana plants may also have exhibited an increasing demand for nutrients, which was not properly addressed through the regular supply of 100 mL of complete nutrient solution without considering the size or age of the plants.
Radopholus similis density, root damage and plant growth parameters were not different between plants inoculated with the nematode and those co-inoculated with the nematode and F. oxysporum V5w2. These results are contrary to previous reports that have linked the suppression of R. similis by F. oxysporum V5w2 to its successful in planta establishment and induced resistance activities in banana roots (Niere et al., 1999; Vu et al., 2006; Paparu et al., 2009b; Waweru et al., 2013, 2014). The low optical density of root extracts from F. oxysporum V5w2-inoculated plants, and the light-brown coloration of their roots provide circumstantial evidence on the interaction between banana roots with the fungal pathogen. These observations on color change in roots and their extracts tended toward corroborating Sikora et al. (2008) “we noticed a distinct lack of root-rotting in excised banana roots following dual inoculation of non-pathogenic F. oxysporum and a lesion nematode Pratylenchus goodeyi in roots that we expected to be necrotic in this combination.” The light-brown root color and the low optical density of their extracts could have been subtle browning reactions, probably due to consumption of organic nitrogen from the roots by F. oxysporum V5w2 (Divon et al., 2005, 2006), resulting in higher concentration of unreacted phenolic compounds that are not dark colored (Bittner, 2006). Furthermore, the percentage of root pieces infected with Fusarium spp. was higher when F. oxysporum V5w2 and R. similis were both applied in sterile soil. Fusarium oxysporum V5w2 although not discernible from other Fusarium spp., may have exhibited better colonization of banana roots through wounds and necrotic tissues inflicted by R. similis (Ochieno, 2020). The in vitro tests indicated the presence of Fusarium spp. that did not interact inhibitively with F. oxysporum V5w2, and may be considered related to the inoculated fungus. Therefore, enhanced root piece colonization by Fusarium in roots of co-inoculated plants with F. oxysporum V5w2 and R. similis is an indicator of disease interactions between the two pathogens and other Fusarium spp.
It was evident that banana plants from non-sterile soil exhibited lower percentage root piece colonization by Fusarium spp. than those grown in sterile soil, and particularly those co-inoculated with R. similis and F. oxysporum V5w2. This still indicates the presence of pest-inhibitive biota in non-sterile soil (Kaushal et al., 2020; Zhang et al., 2020), which may have included Trichoderma spp., Rhizoctonia spp., and other Fusarium species that inhibited F. oxysporum V5w2 in vitro, as well as fungivore invertebrates including the saprophytic nematodes that were not identified in the present study. Inoculation of banana plants with F. oxysporum V5w2 has been found to result in wilt disease symptoms, especially when nutrient supply is limited or the plants are older (Ochieno, 2010, 2020, 2021). This was not the case in the present study, probably due to the fact that the banana plants had been maintained under adequate nutrient supply while the experimental period was relatively short.
Results from the current study present contrasting aspects in the improvement of banana plant genetic materials through tissue culture technology. It is well-known that the aseptic tissue culture techniques intended to remove invertebrate pests and microbial pathogens from banana tissues (Van den Houwe et al., 2020), also do eliminate beneficial endophytic biota (Liu et al., 2019). However, in some cases, this view has been held with little attention on the removal of beneficial rhizosphere biota through soil sterilization as part of production of tissue culture banana plantlets (Ochieno, 2010, 2020). This is because soil sterilization is intended to eliminate microbial pathogens and plant parasitic nematodes among other pests (Li et al., 2019). However, the fact that Fusarium spp. were recovered from plants not inoculated with F. oxysporum V5w2 and grown in non-sterile soil points to the risk of spreading the fungus to pathogen-free areas. This is because cross-contamination and recolonization of tissue culture plants and sterilized soil with Fusarium spp. among other microbial agents is a common problem (Kabuye et al., 2011; Warman and Aitken, 2018; Ochieno, 2020).
In the context of ecosystem services, tissue culture technology should be developed toward the multiplication of healthy mother plants without destruction of the beneficial microbes within the endosphere microbiome. This is because the highly stringent aseptic tissue culture techniques eliminate beneficial endophytic microbes (Bubici et al., 2019). The micro-propagated plants multiplied without elimination of beneficial microbes should be grown in healthy soils without destruction of the beneficial rhizosphere microbes. This is because soil sterilization is currently regarded as an unpopular technique because it kills all soil life (Li et al., 2019). Such a proposal would even make more sense with advancement in methods of profiling biota within endophytic and rhizosphere microbiomes of certified tissue culture mother plants. Probably, the concept of “Tissue Culture Microbiome Conservation Technology” could emerge as a new area of focus. Micropropagation techniques utilizing non-sterile explants have already been developed for conservation of endangered plant species (Bowes, 1990, 1999; Bowes and Curtis, 1991). This would link well with the concepts of natural biological control and conservation biological control (van Lenteren et al., 2018), especially as Conservation Agriculture (CA) among other agroecological approaches are increasingly gaining importance within Sustainable Food Systems (Lal, 2020; Nyanga et al., 2020; Page et al., 2020; Ochieno et al., 2021).
On the other hand, the highly stringent aseptic tissue culture techniques including soil sterilization would make more sense if the planting material is propagated from sick mother plants using unhealthy soil substrates. This could be more of a rescue plan for plants with desirable traits, especially those under the threat of extinction posed by invertebrate pest and microbial pathogen infestations. In this case, the rich biodiversity of beneficial endophytic and rhizosphere microbes could be re-introduced through plant and rhizosphere inoculation to restore the ecosystem services (Bubici et al., 2019). Classical biological control or augmentative biological control through inundation and inoculation could be implemented (van Lenteren et al., 2018). This could be done while putting into perspective concepts and technologies aimed at unraveling the composition and function of endophytic and rhizosphere microbiomes for sustainable food systems in agroecosystems (Ahkami et al., 2017; Fernández-González et al., 2019; Berg et al., 2020; Kaushal et al., 2020).
In summary, the present study reveals a root-rot disease complex of banana involving the root parasitic nematode R. similis and pathogenic F. oxysporum V5w2. Tissue culture banana plants exhibit uptake of biota from the rhizosphere to replenish the endophytic microbiomes. Soil sterilization eliminated the plant growth promoting effect of rhizosphere microbes and their natural suppressiveness toward the R. similis-F. oxysporum disease complex of banana plants. In-depth studies are still necessary to determine the actual identity of F. oxysporum V5w2 and conditions under which this fungus affects banana plants. Such information would contribute toward determination of the pending registration of F. oxysporum V5w2 as a biopesticide in line with the International Standards for Phytosanitary Measures and the Laws of Kenya (Wabule et al., 2003; Avedi et al., 2014; KEPHIS, 2014; FAO-ISPM, 2021). It is concluded that, soil sterilization eliminates beneficial microbes that provide natural pest suppression ecosystem services against Radopholus similis and Fusarium oxysporum in the endosphere and rhizosphere of tissue culture banana plants. From analysis of these endosphere and rhizosphere ecosystem services, I recommend the integration of microbiome conservation into tissue culture technology through the proposed “Tissue Culture Microbiome Conservation Technology.”
The datasets presented in this article are not readily available because the data has remained in thesis form as per the conflict related to consensus over the outcomes (Ochieno, 2020). Requests to access the datasets should be directed to ZHdvY2hpZW5vQGdtYWlsLmNvbQ==.
The author confirms not being the sole contributor of this work and has approved it for publication in line with the conflict of interest.
This work was part of my PhD studies that were funded by Wageningen University and Research Centre (WUR) (Project number PE&RC 06034), and the International Institute of Tropical Agriculture (IITA-Uganda) through finances from the German Federal Ministry for Economic Cooperation and Development (BMZ) (GTZ Project number 03.7860.4-001.00). Masinde Muliro University of Science and Technology provided funds for presentation of this research at the X International Symposium on Banana ISHS-ProMusa Symposium, 10–14 October 2016, Montpellier, France.
This work has been disassociated from studies that propose Fusarium oxysporum V5w2 as an Endophytic Biological Control Agent and the matter has already been openly declared (https://doi.org/10.1016/j.sciaf.2020.e00605). Prof. Marcel Dicke, Prof. Arnold van Huis, Dr. Thomas Dubois, Dr. Danny Coyne, and Dr. Piet J. A. van Asten have renounced their co-authorship due to difference of opinion about the interpretation of the data and the conclusions of this study. Masinde Muliro University of Science and Technology (MMUST) with its Centre for African Medicinal Flora and Fauna (CAMNFF) was not involved in the research described in this article. The Scientific Integrity Committee of Wageningen University & Research (CWI) has already advised on these issues.
All claims expressed in this article are solely those of the authors and do not necessarily represent those of their affiliated organizations, or those of the publisher, the editors and the reviewers. Any product that may be evaluated in this article, or claim that may be made by its manufacturer, is not guaranteed or endorsed by the publisher.
The study was originally co-authored as Chapter 5 (Ochieno, 2010), with Dr. Thomas Dubois, Dr. Danny Coyne, Piet J. A. van Asten (IITA-Uganda), and Prof. dr. ir. Arnold van Huis and Prof. dr. Marcel Dicke (Laboratory of Entomology, WUR). Prof. Richard A. Sikora (University of Bonn), Prof. dr. Thomas Kuijper, Prof. dr. Jos Raaijmakers (WUR), and Dr. MNEJ Smit (Private IPM Consultant) reviewed the original manuscript. Thanks to Prof. dr. Joop C. van Lenteren (Laboratory of Entomology-WUR), Dr. Clifford S. Gold (IITA-Uganda), Dr. Ellie Osir (International Centre of Insect Physiology and Ecology-ICIPE), Prof. Micheni Ntiba and Prof. Lucy W. Irungu (School of Biological Sciences, University of Nairobi) for facilitating my recruitment into the great Wageningen Sandwich PhD program. Thanks to Mr. Stephen Mutoro, the Secretary General of the Consumers Federation of Kenya (COFEK), and Member of the University Council (MMUST) for fostering public-participation by representing farmers and other stakeholders interests in the registration of Fusarium oxysporum V5w2 as a biopesticide through the Legislative Process in Kenya.
Ahkami, A. H., White, R. A. III., Handakumbura, P. P., and Jansson, C. (2017). Rhizosphere engineering: enhancing sustainable plant ecosystem productivity. Rhizosphere 3, 233–243. doi: 10.1016/j.rhisph.2017.04.012
Akankwasa, K., Marimo, P., Tumuhimbise, R., Asasira, M., Khakasa, E., Mpirirwe, I., et al. (2021). The East African highland cooking bananas ‘Matooke'preferences of farmers and traders: implications for variety development. Int. J. Food Sci. Technol. 56, 1124–1134. doi: 10.1111/ijfs.14813
Akello, J., Dubois, T., Gold, C. S., Coyne, D., Nakavuma, J., and Paparu, P. (2007). Beauveria bassiana (Balsamo) Vuillemin as an endophyte in tissue culture banana (Musa spp.). J. Invertebr. Pathol. 96, 34–42. doi: 10.1016/j.jip.2007.02.004
Avedi, E. K., Ochieno, D. M. W., Ajanga, S., Wanyama, C., Wainwright, H., Elzein, A., et al. (2014). Fusarium oxysporum f. sp. strigae strain Foxy 2 did not achieve biological control of Striga hermonthica parasitizing maize in Western Kenya. Biol. Control. 77, 7–14. doi: 10.1016/j.biocontrol.2014.05.012
Back, M. A., Haydock, P. P. J., and Jenkinson, P. (2002). Disease complexes involving plant parasitic nematodes and soilborne pathogens. Plant Pathol. 51, 683–697. doi: 10.1046/j.1365-3059.2002.00785.x
Bedoya, E. T., Bebber, D. P., and Studholme, D. J. (2021). Taxonomic revision of the Banana Fusarium wilt TR4 pathogen is premature. Phytopathology 111, 2141–2145. doi: 10.1094/PHYTO-03-21-0089-LE
Berg, G., Rybakova, D., Fischer, D., Cernava, T., Vergès, M. C. C., Charles, T., et al. (2020). Microbiome definition re-visited: old concepts and new challenges. Microbiome 8, 103. doi: 10.1186/s40168-020-00875-0
Bittner, S. (2006). When quinones meet amino acids: chemical, physical and biological consequences. Amino Acids 30, 205–224. doi: 10.1007/s00726-005-0298-2
Bowes, B. (1990). A simple micropropagation technique utilizing non-sterile explants and its potential in conservation. Prof. Hortic. 4, 113–120.
Bowes, B. G., and Curtis, E. W. (1991). Conservation of the British National Begonia collection by micropropagation. New Phytol. 119, 169–181. doi: 10.1111/j.1469-8137.1991.tb01021.x
Bremner, J. M. (1960). Determination of nitrogen in soil by the Kjeldahl method. J. Agric. Sci. 55, 11–33. doi: 10.1017/S0021859600021572
Brooks, F. E. (2004). Plant-parasitic nematodes of banana in American Samoa. Nematropica 34, 65–72. Available online at: https://journals.flvc.org/nematropica/article/view/69688
Bubici, G., Kaushal, M., Prigigallo, M. I., Gómez-Lama, C. C., and Mercado-Blanco, J. (2019). Biological control agents against Fusarium wilt of banana. Front. Microbiol. 10, 616. doi: 10.3389/fmicb.2019.00616
Chaudhry, A. H., Nayab, S., Hussain, S. B., Ali, M., and Pan, Z. (2021). Current understandings on Magnesium deficiency and future outlooks for Sustainable Agriculture. Int. J. Mol. Sci. 22, 1819. doi: 10.3390/ijms22041819
Coyne, D. L., Cortada, L., Dalzell, J. J., Claudius-Cole, A. O., Haukeland, S., Luambano, N., et al. (2018). Plant-parasitic nematodes and food security in Sub-Saharan Africa. Annu. Rev. Phytopathol. 56, 381–403. doi: 10.1146/annurev-phyto-080417-045833
De Deyn, G. B., Raaijmakers, C. E., and Van der Putten, W. H. (2004). Plant community development is affected by nutrients and soil biota. J. Ecol. 92, 824–834. doi: 10.1111/j.0022-0477.2004.00924.x
Dietrich, P., Cesarz, S., Eisenhauer, N., and Roscher, C. (2020). Effects of steam sterilization on soil abiotic and biotic properties. Soil Org. 92, 99–108. doi: 10.25674/so92iss2pp99
Dita, M., Barquero, M., Heck, D., Mizubuti, E. S. G., and Staver, C. P. (2018). Fusarium wilt of banana: current knowledge on epidemiology and research needs toward sustainable disease management. Front. Plant Sci. 9, 1468. doi: 10.3389/fpls.2018.01468
Divon, H. H., Denoyes, B. R., Davydov, O., Di Pietro, A., and Fluhr, R. (2005). Nitrogen-responsive genes are differentially regulated in planta during Fusarium oxysporum f. sp. lycopersici infection. Mol. Plant Pathol. 6, 459–470. doi: 10.1111/j.1364-3703.2005.00297.x
Divon, H. H., Ziv, C., Davydov, O., Yarden, O., and Fluhr, R. (2006). The global nitrogen regulator, FNR1, regulates fungal nutrition-genes and fitness during Fusarium oxysporum pathogenesis. Mol. Plant Pathol. 7, 485–497. doi: 10.1111/j.1364-3703.2006.00354.x
Dubois, T., Coyne, D., Kahangi, E., Turoop, L., and Nsubuga, E. W. N. (2006). Endophyte-enhanced banana tissue culture: technology transfer through public-private partnerships in Kenya and Uganda. ATDF J. 3, 18–24. Available online at: https://hdl.handle.net/10568/91380
Dubois, T., Coyne, D., and zum Felde, A. (2011). Enhanced Protection for Tissue Cultured Banana Plants. SP-IPM Technical Innovation Brief No. 10. CGIAR Systemwide Program on Integrated Pest Management (SP-IPM). Available online at: www.spipm.cgiar.org
Dubois, T., Gold, C. S., Coyne, D., Paparu, P., Mukwaba, E., Athman, S., et al. (2004). Merging biotechnology with biological control: banana Musa tissue culture plants enhanced by endophytic fungi. Uganda J. Agric. Sci. 9, 445–451. Available online at: https://www.ajol.info/index.php/ujas/article/view/134983
Evan, R., Harald, K., Fatos, H., Jafargholi, I., and Konrad, M. (2003). The importance of soil microbial activity for the supply of iron to sorghum and rape. Eur. J. Agron. 19, 487–493. doi: 10.1016/S1161-0301(02)00185-5
FAO-ISPM (2021). International Standards for Phytosanitary Measures. Rome: Secretariat of the International Plant Protection Convection (IPPC); Food and Agriculture Organization (FAO). Available online at: https://assets.ippc.int/static/media/files/publication/en/2021/04/ISPM_List_En_2021-03-17.pdf
Fernández-González, A. J., Villadas, P. J., Gómez-Lama Cabanás, C., Cabanás, C., Valverde-Corredor, A., Belaj, A., et al. (2019). Defining the root endosphere and rhizosphere microbiomes from the World Olive Germplasm Collection. Sci. Rep. 9, 20423. doi: 10.1038/s41598-019-56977-9
Gaidashova, S. V., van Asten, P., De Waele, D., and Delvaux, B. (2009). Relationship between soil properties, crop management, plant growth and vigour, nematode occurrence and root damage in East African Highland banana-cropping systems: a case study in Rwanda. Nematology 11, 883–894. doi: 10.1163/156854109X430310
Gough, E. C., Owen, K. J., Zwart, R. S., and Thompson, J. P. (2020). A systematic review of the effects of arbuscular mycorrhizal fungi on root-lesion nematodes, Pratylenchus spp. Front. Plant Sci. 11, 923. doi: 10.3389/fpls.2020.00923
Hauer-Jákli, M., and Tränkner, M. (2019). Critical leaf magnesium thresholds and the impact of magnesium on plant growth and photo-oxidative defense: a systematic review and meta-analysis from 70 years of research. Front. Plant Sci. 10, 766. doi: 10.3389/fpls.2019.00766
He, H., Jin, X., Ma, H., Deng, Y., Huang, J., and Yin, L. (2020). Changes of plant biomass partitioning, tissue nutrients and carbohydrates status in magnesium-deficient banana seedlings and remedy potential by foliar application of magnesium. Sci. Hortic. 268, 109377. doi: 10.1016/j.scienta.2020.109377
Hoeschle-Zeledon, I., Neuenschwander, P., and Kumar, L. (2013). Regulatory Challenges for Biological Control. Ibadan: CGIAR Systemwide Program on Integrated Pest Management (SP-IPM); International Institute of Tropical Agriculture (IITA). Available online at: https://hdl.handle.net/10568/80836
Huang, X., Xu, C. L., Yang, S. H., Li, J. Y., Wang, H. L., Zhang, Z. X., et al. (2019). Life-stage specific transcriptomes of a migratory endoparasitic plant nematode, Radopholus similis elucidate a different parasitic and life strategy of plant parasitic nematodes. Sci. Rep. 9, 6277. doi: 10.1038/s41598-019-42724-7
Jacobsen, K., Omondi, B. A., Almekinders, C., Alvarez, E., Blomme, G., Dita, M., et al. (2019). Seed degeneration of banana planting materials: strategies for improved farmer access to healthy seed. Plant Pathol. 68, 207–228. doi: 10.1111/ppa.12958
Jacoby, R., Peukert, M., Succurro, A., Koprivova, A., and Kopriva, S. (2017). The role of soil microorganisms in plant mineral nutrition-current knowledge and future directions. Front. Plant Sci. 8, 1617. doi: 10.3389/fpls.2017.01617
Jamil, F. N., Tang, C. N., Saidi, N. B., Lai, K. S., and Baharum, N. A. (2019). “Fusarium wilt in banana: epidemics and management strategies,” in Horticultural Crops, eds H. K. Baimey, N. Hamamouch, and Y. A. Kolombia (London: IntechOpen). doi: 10.5772/intechopen.89469
Jayaraman, S., Naorem, A., Lal, R., Dalal, R. C., Sinha, N. K., Patra, A. K., et al. (2021). Disease-suppressive soils—beyond food production: a critical review. J. Soil Sci. Plant. Nutr. 21, 1437–1465. doi: 10.1007/s42729-021-00451-x
Jezek, M., Geilfus, C.-M., Bayer, A., and Mühling, K-H. (2015). Photosynthetic capacity, nutrient status, and growth of maize (Zea mays L.) upon MgSO4 leaf-application. Front. Plant Sci. 5, 781. doi: 10.3389/fpls.2014.00781
Kabuye, D., Tripathi, L., Niyibigira, E., Tripathi, J., and Okori, P. (2011). Does horizontal gene flow occur in transgenic banana/Fusarium oxysporum (V5W2-9 NH3) associations? Proc. Inter. Conf. Agbiotech. Biosafety Seed Syst. 2011, 49–53. Available online at: https://www.scifode-foundation.org/attachments/article/38/Kabuye-D-et-al-2011a.pdf
Kangire, A., Karamura, E. B., Gold, C., and Rutherford, M. A. (2000). Fusarium wilt of banana in Uganda, with special emphasis on wilt-like symptoms observed on East African Highland Cooking cultivars (Musa Spp., AAA). Acta Hortic. 540, 343–353. doi: 10.17660/ActaHortic.2000.540.40
Kangire, A., and Rutherford, M. (2001). Wilt-like disorder of bananas in Uganda. Musa Disease Factsheet No. 10. Montpellier: International Network for the Improvement of Banana and Plantain (INIBAP), Parc Scientifique Agropolis. Available online at: https://www.bioversityinternational.org/fileadmin/_migrated/uploads/tx_news/Wilt-like_disorder_of_bananas_in_Uganda_721.pdf
Kaushal, M., Swennen, R., and Mahuku, G. (2020). Unlocking the microbiome communities of banana (Musa spp.) under disease stressed (Fusarium wilt) and non-stressed conditions. Microorganisms 8, 443. doi: 10.3390/microorganisms8030443
KEPHIS (2014). Guidelines for Introduction and Use of Bio-Products, Biological Control Agents and Related Products. Kenya Standing Technical Committee on Imports and Exports (KSTCIE). Nairobi: Kenya Plant Health Inspectorate Service (KEPHIS). Available online at: http://www.kephis.org/images/Guidelines/guidelines.pdf
Kikulwe, E. M., and Asindu, M. (2020). A contingent valuation analysis for assessing the market for genetically modified planting materials among banana producing households in Uganda. GM Crops Food 11, 113–124. doi: 10.1080/21645698.2020.1720498
Kisaakye, J., Fourie, H., Haukeland, S., Kisitu, J., Nakimera, S., Cortada, L., et al. (2022). Endophytic non-pathogenic Fusarium oxysporum-derived dual benefit for nematode management and improved banana (Musa spp.) productivity. Agriculture 12, 125. doi: 10.3390/agriculture12020125
Kumar, P. L., Cuervo, M., Kreuze, J. F., Muller, G., Kulkarni, G., Kumari, S. G., et al. (2021). Phytosanitary interventions for safe global germplasm exchange and the prevention of transboundary pest spread: the role of CGIAR Germplasm Health Units. Plants 10, 328. doi: 10.3390/plants10020328
Kurtz, A. (2010). Endophytic Fusarium oxysporum: phylogeny and induced defense responses in banana plants against Radopholus similis (PhD thesis). University of Bonn, Bonn, Germany. Available online at: https://hdl.handle.net/20.500.11811/4221
Lal, R. (2020). Soil science beyond COVID-19. J. Soil Water Conser. 75, 79A–81A. doi: 10.2489/jswc.2020.0408A
Leslie, J. F., and Summerell, B. A. (2008). The Fusarium Laboratory Manual. New Jersey, NY: John Wiley & Sons.
Li, K., DiLegge, M. J., Minas, I. S., Hamm, A., Manter, D., and Vivanco, J. M. (2019). Soil sterilization leads to re-colonization of a healthier rhizosphere microbiome. Rhizosphere 12, 100176. doi: 10.1016/j.rhisph.2019.100176
Liu, S., Li, J., Zhang, Y., Liu, N., Viljoen, A., Mostert, D., et al. (2020). Fusaric acid instigates the invasion of banana by Fusarium oxysporum f. sp. cubense TR 4. New Phytol. 225, 913–929. doi: 10.1111/nph.16193
Liu, Y., Zhu, A., Tan, H., Cao, L., and Zhang, R. (2019). Engineering banana endosphere microbiome to improve Fusarium wilt resistance in banana. Microbiome 7, 74. doi: 10.1186/s40168-019-0690-x
Luc, M., Sikora, R. A., and Bridge, J. (2005). Plant Parasitic Nematodes in Subtropical and Tropical Agriculture. Wallingford: CABI Publishing.
Mai, W. F., and Abawi, G. S. (1987). Interactions among root-knot nematodes and Fusarium wilt fungi on host plants. Annu. Rev. Phytopathol. 25, 317–338. doi: 10.1146/annurev.py.25.090187.001533
Maryani, N., Lombard, L., Poerba, Y. S., Subandiyah, S., Crous, P. W., and Kema, G. H. J. (2019). Phylogeny and genetic diversity of the banana Fusarium wilt pathogen Fusarium oxysporum f. sp. cubense in the Indonesian centre of origin. Stud. Mycol. 92, 155–194. doi: 10.1016/j.simyco.2018.06.003
Maymon, M., Sela, N., Shpatz, U., Galpaz, N., and Freeman, S. (2020). The origin and current situation of Fusarium oxysporum f. sp. cubense tropical race 4 in Israel and the Middle East. Sci. Rep. 10, 1590. doi: 10.1038/s41598-020-58378-9
McIntyre, B. D., Speijer, P. R., Riha, S. J., and Kizito, F. (2000). Effects of mulching on biomass, nutrients, and soil water in banana inoculated with nematodes. Agron. J. 92, 1081–1085. doi: 10.2134/agronj2000.9261081x
Mehlich, A. (1984). Mehlich 3 soil test extractant: a modification of Mehlich 2 extractant. Comm. Soil Sci. Plant Anal. 15, 1409–1416. doi: 10.1080/00103628409367568
Moens, T., Araya, M., Swennen, R., and De Waele, D. (2006). Reproduction and pathogenicity of Helicotylenchus multicinctus, Meloidogyne incognita and Pratylenchus coffeae, and their interaction with Radopholus similis on Musa. Nematology 8, 45–58. doi: 10.1163/156854106776179999
Murashige, T., and Skoog, F. (1962). A revised medium for rapid growth and bioassays with tobacco tissue cultures. Physiol. Plant. 15, 473–497. doi: 10.1111/j.1399-3054.1962.tb08052.x
Nakintu, J., Rwakitarara, M. S., and Ochieno, D. M. W. (2008). Occurrence of Soilborne Microbes Invading Roots of Tissue Culture Bananas Inoculated With Endophytic Fusarium oxysporum V5w2. Special Project, Faculty of Agriculture, Makerere University, Kampala, Uganda.
Niere, B. I. (2001). Significance of non-pathogenic isolates of Fusarium oxysporum Schlecht.: fries for the biological control of the burrowing nematode Radopholus similis (Cobb) Thorne on tissue cultured banana (Ph.D. thesis). University of Bonn, Bonn, Germany.
Niere, B. I., Speijer, P. R., and Sikora, R. A. (1999). A Novel Approach to the Biological Control of Banana Nematodes. Deutscher Tropentag, 1–9. Available online at: https://ftp.gwdg.de/pub/tropentag/proceedings/1999/referate/STD_C5.pdf
Nyanga, P. H., Umar, B. B., Chibamba, D., Mubanga, K. H., Kunda-Wamuwi, C. F., and Mushili, B. M. (2020). “Reinforcing ecosystem services through conservation agriculture in sustainable food systems,” in The Role of Ecosystem Services in Sustainable Food Systems, ed L. Rusinamhodzi (Cambridge, MD: Academic Press), 119–133.
Ochieno, D. M. W. (2010). Endophytic control of Cosmopolites sordidus and Radopholus similis using Fusarium oxysporum V5w2 in tissue culture banana (Ph.D. thesis and Propositions). Wageningen University and Research Centre, Wageningen, Netherlands. Available online at: http://library.wur.nl/WebQuery/wda/lang/1948566
Ochieno, D. M. W. (2020). Towards consensus on the transfer of Fusarium oxysporum V5w2-enhanced tissue culture banana technology to farmers through public-private partnerships in East Africa. Sci. Afr. 10, e00605. doi: 10.1016/j.sciaf.2020.e00605
Ochieno, D. M. W. (2021). Fusarium oxysporum V5w2 is a non-beneficial endophyte that interacts with Radopholus similis in a wilt disease complex of banana. Sci. Afr. 14, e01014. doi: 10.1016/j.sciaf.2021.e01014
Ochieno, D. M. W., Karoney, E. M., Muge, E. K., Nyaboga, E. N., Baraza, D. L., Shibairo, S. I., et al. (2021). Rhizobium-linked nutritional and phytochemical changes under multitrophic functional contexts in Sustainable Food Systems. Front. Sustain. Food Syst. 4, 604396. doi: 10.3389/fsufs.2020.604396
Ogura, T., Kobayashi, N. I., Hermans, C., Ichihashi, Y., Shibata, A., Shirasu, K., et al. (2020). Short-term magnesium deficiency triggers nutrient retranslocation in Arabidopsis thaliana. Front. Plant Sci. 11, 563. doi: 10.3389/fpls.2020.00563
Okalebo, J. K., Gathua, K. W., and Woomer, P. L. (2002). Laboratory Methods of Soil and Plant Analysis: A Working Manual, 2nd Edn. Nairobi: SACRED-Africa.
Olivares, B. O., Rey, J. C., Lobo, D., Navas-Cortés, J. A., Gómez, J. A., and Landa, B. B. (2021). Fusarium wilt of bananas: a review of agro-environmental factors in the Venezuelan production system affecting its development. Agronomy 11, 986. doi: 10.3390/agronomy11050986
Onguso, J. M., Kahangi, E. M., Ndiritu, D. W., and Mizutani, F. (2004). Genetic characterization of cultivated bananas and plantains in Kenya by RAPD markers. Sci. Hortic. 99, 9–20. doi: 10.1016/S0304-4238(03)00082-7
Page, K. L., Dang, Y. P., and Dalal, R. C. (2020). The ability of conservation agriculture to conserve soil organic carbon and the subsequent impact on soil physical, chemical, and biological properties and yield. Front. Sustain. Food Syst. 4, 31. doi: 10.3389/fsufs.2020.00031
Paparu, P., Adele, M., Dubois, T., Coyne, D., and Viljoen, A. (2009a). Efficacy of chemical and fluorescent protein markers in studying plant colonization by endophytic non-pathogenic Fusarium oxysporum isolates. Biol. Control. 54, 709–722. doi: 10.1007/s10526-009-9221-1
Paparu, P., Dubois, T., Coyne, D., and Viljoen, A. (2007). Defense-related gene expression in susceptible and tolerant bananas (Musa spp.) following inoculation with non-pathogenic Fusarium oxysporum endophytes and challenge with Radopholus similis. Physiol. Mol. Plant Pathol. 71, 149–157. doi: 10.1016/j.pmpp.2007.12.001
Paparu, P., Dubois, T., Coyne, D., and Viljoen, A. (2009b). Dual inoculation of Fusarium oxysporum endophytes in banana: effect on plant colonization, growth and control of the root burrowing nematode and the banana weevil. Biocontrol Sci. Technol. 19, 639–655. doi: 10.1080/09583150902972206
Paparu, P., Dubois, T., Coyne, D., and Viljoen, A. (2010). Effect of Fusarium oxysporum endophyte inoculation on the activities of phenylpropanoid pathway enzymes and Radopholus similis numbers in susceptible and tolerant East African highland bananas. Nematology 12, 469–480. doi: 10.1163/138855409X12571623969646
Paparu, P., Dubois, T., Coyne, D., and Viljoen, A. (2013). Differential gene expression in East African highland bananas (Musa spp.): Interactions between non-pathogenic Fusarium oxysporum V5w2 and Radopholus similis. Physiol. Mol. Plant P. 82, 56–63. doi: 10.1016/j.pmpp.2012.10.003
Paparu, P., Dubois, T., Gold, C. S., Niere, B., Adipala, E., and Coyne, D. (2006a). Colonisation pattern of non-pathogenic Fusarium oxysporum, a potential biological control agent, in roots and rhizomes of tissue cultured Musa plantlets. Ann. Appl. Biol. 149, 1–8. doi: 10.1111/j.1744-7348.2006.00064.x
Paparu, P., Dubois, T., Gold, C. S., Niere, B., Adipala, E., and Coyne, D. L. (2006b). Improved colonization of East African highland Musa tissue culture plants by endophytic Fusarium oxysporum. J. Crop Improv. 16, 81–95. doi: 10.1300/J411v16n01_06
Pegg, K. G., Coates, L. M., O'Neill, W. T., and Turner, D. W. (2019). The epidemiology of Fusarium wilt of banana. Front. Plant Sci. 10, 1395. doi: 10.3389/fpls.2019.01395
Ploetz, R. C., and Evans, E. A. (2015). The future of global banana production. Hortic. Rev. 43, 311–352. doi: 10.1002/9781119107781.ch06
Raghupathi, H. B., Reddy, B. M. C., and Srinivas, K. (2002). Multivariate diagnosis of nutrient imbalance in banana. Commun. Soil Sci. Plant Anal. 33, 2131–2143. doi: 10.1081/CSS-120005753
Rocha, A. D. J., Ferreira, M. D. S., Rocha, L. D. S., Oliveira, S. A., Amorim, E. P., Mizubuti, E. S., et al. (2020). Interaction between Fusarium oxysporum f. sp. cubense and Radopholus similis can lead to changes in the resistance of banana cultivars to Fusarium wilt. Eur. J. Plant Pathol. 158, 403–417. doi: 10.1007/s10658-020-02081-y
Rocha, A. D. J. D. J., Soares, J. M. D. S. M. D. S., Nascimento, F. D. S. D. S., Santos, A. S. S., Amorim, V. B. D. O. B. D. O., Ferreira, C. F. F., et al. (2021). Improvements in the resistance of the banana species to Fusarium wilt: a systematic review of methods and perspectives. J. Fungi 7, 249. doi: 10.3390/jof7040249
SAS (2004). Statistical Analysis System User's Guide: Statistics, Version 9.1. SAS Institute, Cary, NC. Available online at: https://support.sas.com/documentation/onlinedoc/91pdf/sasdoc_91/stat_ug_7313.pdf
Scott, G. J. (2021). A review of root, tuber and banana crops in developing countries: past, present and future. Int. J. Food Sci. 56, 1093–1114. doi: 10.1111/ijfs.14778
Seenivasan, N. (2017). Management of Radopholus similis and Helicotylenchus multicinctus in ratoon banana grown under high density planting systems. Int. J. Fruit Sci. 17, 41–62. doi: 10.1080/15538362.2016.1250696
Shen, Z., Ruan, Y., Chao, X., Zhang, J., Li, R., and Shen, Q. (2015). Rhizosphere microbial community manipulated by 2 years of consecutive biofertilizer application associated with banana Fusarium wilt disease suppression. Biol. Fertil. Soils 51, 553–562. doi: 10.1007/s00374-015-1002-7
Sikora, R. A., and Pocasangre, L. (2006). “The concept of a suppressive banana plant: root health management with a biological approach,” in Proceedings of the XVII ACROBAT International Congress, Vol. 1 (Joinville), 241–248.
Sikora, R. A., Pocasangre, L., zum Felde, A., Niere, B., Tam, T. V., and Dababat, A. A. (2008). Mutualistic endophytic fungi and in-planta suppressiveness to plant parasitic nematodes. Biol. Control 46, 15–23. doi: 10.1016/j.biocontrol.2008.02.011
Speijer, P. R., and De Waele, D. (1997). “Screening of Musa germplasm for resistance and tolerance to nematodes, INIBAP technical guidelines 1,” in The International Network for the Improvement of Banana and Plantain (Montpellier). INIBAP-ISBN 2-910810-16-X
Speijer, P. R., and Kajumba, C. (2000). Yield loss from plant parasitic nematodes in east African highland banana (Musa spp. AAA). Acta Hort. 540, 453–459. doi: 10.17660/ActaHortic.2000.540.51
Speijer, P. R., and Sikora, R. A. (1993). “Influence of a complex disease involving Pratylenchus goodeyi and a non-pathogenic strain of Fusarium oxysporum on banana root health,” in Biological and Integrated Control of Highland Banana and Plantain Pests and Diseases: Proceedings of a Research Coordination Meeting, Cotonou, Benin, 12–14 November 1991 (Cotonou), 218–230. Available online at: https://www.cabdirect.org/cabdirect/abstract/19952307862
Ssango, F., Speijer, P. R., Coyne, D. L., and De Waele, D. (2004). Path analysis: a novel approach to determine the contribution of nematode damage to East African Highland banana (Musa spp., AAA) yield loss under two crop management practices in Uganda. Field Crops Res. 90, 177–187. doi: 10.1016/j.fcr.2004.02.018
Talwana, H., Sibanda, Z., Wanjohi, W., Kimenju, W., Luambano-Nyoni, N., Massawe, C., et al. (2016). Agricultural nematology in East and Southern Africa: problems, management strategies and stakeholder linkages. Pest Manag. Sci. 72, 226–245. doi: 10.1002/ps.4104
Topalović, O., Hussain, M., and Heuer, H. (2020). Plants and associated soil microbiota cooperatively suppress plant-parasitic nematodes. Front. Microbiol. 11, 313. doi: 10.3389/fmicb.2020.00313
Van den Houwe, I., Chase, R., Sardos, J., Ruas, M., Kempenaers, E., Guignon, V., et al. (2020). Safeguarding and using global banana diversity: a holistic approach. CABI Agric. Biosci. 1, 15. doi: 10.1186/s43170-020-00015-6
Van der Veken, L., Cabasan, M. N., Teodora, N., Elsen, A., Swennen, R., and De Waele, D. (2021). Effect of single or dual inoculation of the arbuscular mycorrhizal fungus Glomus mosseae and root-nodulating rhizobacteria on reproduction of the burrowing nematode Radopholus similis on non-leguminous and leguminous banana intercrops. J. Plant Dis. Prot. 128, 961–971. doi: 10.1007/s41348-021-00429-y
van Lenteren, J. C., Bolckmans, K., Köhl, J., Ravensberg, W. J., and Urbaneja, A. (2018). Biological control using invertebrates and microorganisms: plenty of new opportunities. Biocontrol 63, 39–59. doi: 10.1007/s10526-017-9801-4
Vu, T. T., Hauschild, R., and Sikora, R. A. (2006). Fusarium oxysporum endophytes induced systemic resistance against Radopholus similis on banana. Nematology 8, 847–852. doi: 10.1163/156854106779799259
Vuylsteke, D. R. (1998). Shoot-Tip Culture for the Propagation, Conservation, and Distribution of Musa germplasm. Ibadan: International Institute of Tropical Agriculture. Available online at: https://hdl.handle.net/10568/95930
Wabule, M. N., Ngaruiya, P. N., Kimmins, F. K., and Silverside, P. J. (2003). “Registration for biocontrol agents in Kenya,” in Proceedings of the Pest Control Products Board/Kenya Agricultural Research Institute/Department for International Development Crop Protection Programme Workshop, Nakuru, Kenya, 14–16 May 2003 (Nairobi; Aylesford: KARI/PCPB; Natural Resources International Ltd). Available online at: https://assets.publishing.service.gov.uk/media/57a08cc040f0b649740013fe/R7299Registration.pdf
Wairegi, L., and van Asten, P. (2011). Norms for multivariate diagnosis of nutrient imbalance in the East African highland bananas (Musa spp. AAA). J. Plant Nutr. 34, 1453–1472. doi: 10.1080/01904167.2011.585203
Walkley, A. (1947). A critical examination of a rapid method for determining organic carbon in soils: effect of variations in digestion conditions and of inorganic soil constituents. Soil Sci. 37, 29–38. doi: 10.1097/00010694-193401000-00003
Wang, Y., Wu, B., Berns, A. E., Xing, Y., Kuhn, A. J., and Amelung, W. (2020). Magnesium isotope fractionation reflects plant response to magnesium deficiency in magnesium uptake and allocation: a greenhouse study with wheat. Plant Soil 455, 93–105. doi: 10.1007/s11104-020-04604-2
Warman, N. M., and Aitken, E. A. B. (2018). The movement of Fusarium oxysporum f.sp. cubense (Sub-Tropical Race 4) in susceptible cultivars of banana. Front. Plant Sci. 9, 1748. doi: 10.3389/fpls.2018.01748
Waweru, B., Turoop, L., Kahangi, E., Coyne, D., and Dubois, T. (2014). Non-pathogenic Fusarium oxysporum endophytes provide field control of nematodes, improving yield of banana (Musa sp.). Biol. Control 74, 82–88. doi: 10.1016/j.biocontrol.2014.04.002
Waweru, B. W., Losenge, T., Kahangi, E. M., Dubois, T., and Coyne, D. (2013). Potential biological control of lesion nematodes on banana using Kenyan strains of endophytic Fusarium oxysporum. Nematology 15, 101–107. doi: 10.1163/156854112X645606
Yu, M., Xie, W., Zhang, X., Zhang, S., Wang, Y., Hao, Z., et al. (2019). Arbuscular mycorrhizal fungi can compensate for the loss of indigenous microbial communities to support the growth of liquorice (Glycyrrhiza uralensis Fisch.). Plants 9, 7. doi: 10.3390/plants9010007
Zhang, Y., Li, S., Li, H., Wang, R., Zhang, K.-Q., and Xu, J. (2020). Fungi–nematode interactions: diversity, ecology, and biocontrol prospects in agriculture. J. Fungi 6, 206. doi: 10.3390/jof6040206
zum Felde, A. (2011). Endophytes: Novel Weapons in the IPM Arsenal. SP-IPM Technical Innovation Brief No. 9. CGIAR Systemwide Program on Integrated Pest Management (SP-IPM). Available online at: www.spipm.cgiar.org
Keywords: biological control, endophyte, Fusarium oxysporum V5w2, Radopholus similis, rhizosphere, tissue culture
Citation: Ochieno DMW (2022) Soil Sterilization Eliminates Beneficial Microbes That Provide Natural Pest Suppression Ecosystem Services Against Radopholus similis and Fusarium Oxysporum V5w2 in the Endosphere and Rhizosphere of Tissue Culture Banana Plants. Front. Sustain. Food Syst. 6:688194. doi: 10.3389/fsufs.2022.688194
Received: 30 March 2021; Accepted: 03 February 2022;
Published: 01 March 2022.
Edited by:
Stacy Michelle Philpott, University of California, Santa Cruz, United StatesReviewed by:
Ghulam Hussain Jatoi, Institute of Plant Protection (CAAS), ChinaCopyright © 2022 Ochieno. This is an open-access article distributed under the terms of the Creative Commons Attribution License (CC BY). The use, distribution or reproduction in other forums is permitted, provided the original author(s) and the copyright owner(s) are credited and that the original publication in this journal is cited, in accordance with accepted academic practice. No use, distribution or reproduction is permitted which does not comply with these terms.
*Correspondence: Dennis M. W. Ochieno, ZG9jaGllbm9AbW11c3QuYWMua2U=; ZHdvY2hpZW5vQGdtYWlsLmNvbQ==; orcid.org/0000-0003-3985-9421
†Present Address: Dennis M. W. Ochieno, Department of Biological Sciences, School of Natural Sciences, Masinde Muliro University of Science and Technology, Kakamega, Kenya; Biodiversity and Ecosystem Services Section, Centre for African Medicinal and Nutritional Flora and Fauna (CAMNFF), Techno Park, Masinde Muliro University of Science and Technology (MMUST), Kakamega, Kenya
Disclaimer: All claims expressed in this article are solely those of the authors and do not necessarily represent those of their affiliated organizations, or those of the publisher, the editors and the reviewers. Any product that may be evaluated in this article or claim that may be made by its manufacturer is not guaranteed or endorsed by the publisher.
Research integrity at Frontiers
Learn more about the work of our research integrity team to safeguard the quality of each article we publish.