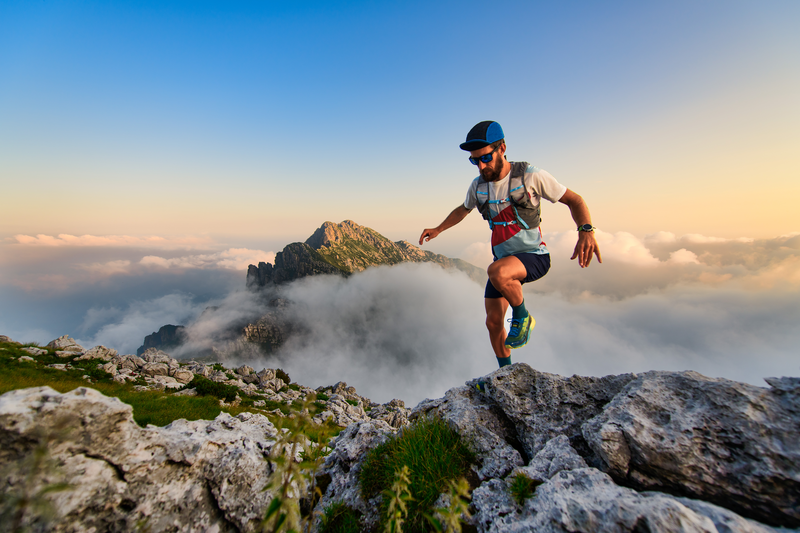
94% of researchers rate our articles as excellent or good
Learn more about the work of our research integrity team to safeguard the quality of each article we publish.
Find out more
ORIGINAL RESEARCH article
Front. Sustain. Food Syst. , 03 February 2022
Sec. Land, Livelihoods and Food Security
Volume 6 - 2022 | https://doi.org/10.3389/fsufs.2022.668335
This article is part of the Research Topic COVID-19: Food System Frailties and Opportunities View all 23 articles
The initial months of the COVID-19 pandemic revealed unique vulnerabilities of the global food system with notable societal consequences, calling for the need to implement resilience strategies to support food security for all. The objective of this study was to elicit perceptions, experiences, and responses of producers of diversified farms in the Northern Great Plains of the United States to the early stage of the COVID-19 pandemic toward identifying factors for strengthening the resilience of agricultural production for supporting livelihoods and food security. Between May and August 2020, a cross-sectional online survey was administered to the emerging community of diversified farm managers in two rural U.S. states, Montana and South Dakota (n = 53), where monocropping and extensive livestock production are prevalent. About two-thirds of surveyed producers (68%) reported that they did not change their farming practices in response to the pandemic up until the survey period in Summer 2020. Almost three-quarters of producers (73%) indicated that access to commodities, farm inputs, and farm labor was not a concern for them during the early stage of the pandemic. Most surveyed producers (88%) were not concerned about their household food security and expressed confidence regarding a long-term increase in the demand for local food. However, almost half of surveyed producers (47%) reported that their anxiety increased because of the pandemic. Findings further highlight that small farms implemented a greater number of practices for enhancing ecological self-regulation while depending strongly on off-farm income compared to larger farms that were economically more autonomous. This study points to the promise of farm system diversification in strengthening the resilience of agricultural systems. We conclude by outlining pathways for increasing diversity on farms toward supporting food security during extreme food system shocks.
The COVID-19 pandemic unprecedentedly impacted food systems around the world (Afshin et al., 2019) in terms of scope and immediateness (Baldwin and Tomiura, 2020), revealing unique vulnerabilities (Altieri and Nicholls, 2020a) as well as resilience (Orden, 2020) across supply chains. On the production side of food systems, lockdown and “stay at home” measures during the initial phase of the COVID-19 pandemic had severe impacts on agricultural production and food commodity prices (Elleby et al., 2020), with repercussions for producers and consumers worldwide. Producer income, purchasing power, planning, access to markets, and emotional wellbeing were impacted (Reissman et al., 2006; Laborde et al., 2020). Recent data shows that the pandemic has been a rare case of a global supply chain disruption due to restricted transportation, unavailability of raw material supply, and shutdowns of food processing, distribution, and sale (Taqi et al., 2020; Shahed et al., 2021). While evidence demonstrates how unprepared food systems were to respond appropriately to the COVID-19 pandemic, emerging research is showing how some communities and sectors of the food system were more resilient than others in their responses (Ahmed et al., 2020).
Failure to learn from previous disasters, laxity to adopt early preparedness strategies from warnings by health organizations and scientists, market globalization, as well as highly interconnected living systems contributed to spreading the pandemic with significant impacts on agriculture (Saqr and Wasson, 2020). COVID-19 affected agriculture in both supply and demand (Siche, 2020). On the supply side, there have been restrictions on travel, transportation, and trade, reinforced sanitary controls, and labor shortages with many food system workers becoming sick or not able to travel to their workplaces (Elleby et al., 2020). During the first few months of the pandemic when this study was conducted, these limitations constrained the distribution and processing of foods, the circulation of farm inputs, and resulted in a vast decay of perishable products; a further enduring effect of COVID-19 on the food system has been the uncertainty farmers confront regarding their production management decisions and consumer demand (Benton, 2020). On the demand side, mobility restrictions and reduced purchasing power due to the related economic slowdown have been impacting consumers worldwide, especially the most vulnerable population groups (Siche, 2020).
An increasing body of evidence demonstrates that elevated levels of agrobiodiversity allow for quicker recovery after environmental disturbances such as hurricanes (Philpott et al., 2008; Rosset et al., 2011) or drought (Murgueitio et al., 2011). Agrobiodiversity is also associated with greater success in achieving food security (Thornton et al., 2019). Given the role of agrobiodiversity for resilience, the study presented here focused on surveying farms with an elevated level of diversification in their farm management. The overall goals of this study were to: (1) identify the challenges and opportunities faced by diversified farms during the initial months of the COVID-19 pandemic; (2) evaluate the resilience and vulnerabilities of diversified farms to the early stages of the COVID-19 pandemic; and (3) characterize responses to the COVID-19 pandemic to identify factors contributing to resilience to inform future preparedness efforts.
To address our study goals, we deployed an online cross-sectional survey to managers of diversified farms in two states of the Northern Great Plains (NGP) in the U.S., Montana (MT) and South Dakota (SD). Survey questions assessed producer perceptions in response to COVID-19 (including impacts on the farms' economy and producers' emotional wellbeing), farm management and crop diversity, and food security concerns of farms and farming communities based on the perceptions of 53 farm managers of diversified farms in MT and SD. We used the agroecosystem resilience assessment framework by Cabell and Oelofse (2012) as well as the rapid C-SCAN (COVID-19 Surveillance Community Action Network for Food Systems) survey (Ahmed et al., 2020) as baselines for developing our survey instrument. We acknowledge that the sample size of this study is relatively small due to: (1) the circumstance that the diversified farming community in MT and SD is small; (2) the travel constraints imposed by the COVID-19 pandemic that did not enable us to travel to administer the surveys and rather relied on a purely online format; (3) the short time window due to the aim of capturing producers' perceptions during the early stages of the COVID-19 pandemic. Despite the relatively small sample size, the survey findings presented here cover a unique time, the first growing season since the start of the pandemic characterized by extreme uncertainty.
To situate the context of this study, the following section provides background on the theoretical foundations of this study regarding the concepts of resilience and agrobiodiversity, as well as a comprehensive review on the impact of the COVID-19 pandemic on agricultural and food systems.
Food system resilience is the capacity of a food system and its units at multiple levels to constantly provide food security, even while facing unforeseen (internal or external) disturbances such as natural, political, social, or economic shocks (Tendall et al., 2015). Food system resilience is characterized by five capacities: (1) capacity to withstand disturbances (robustness); (2) disturbance absorption capacity; (3) capacity to react (rapidity and flexibility); (4) capacity to restore (resourcefulness); and (5) capacity to learn or adaptability (Carpenter et al., 2001; Tendall et al., 2015). In contrast, the vulnerability of a food system is dependent on its propensity or predisposition to external shocks or disturbances. Accordingly, a vulnerable food system is characterized by low decision-making autonomy, connectivity, and diversity (Ericksen et al., 2012; Intergovernmental Panel on Climate Change, 2014).
Agroecosystems are managed to produce, distribute, and consume food, biofuel, and fiber. Boundaries of agroecosystems encompass the physical space dedicated to the production, as well as the resources, infrastructure, markets, institutions, and people operating within these boundaries (Cabell and Oelofse, 2012). This definition characterizes agroecosystems as socio-environmental systems which are part of the production subsystem of food systems (Ericksen, 2008). Agroecosystem resilience is a fundamental component of overall food system resilience. Specifically, it describes the capacity of an agroecosystem to maintain the ability to feed, clothe, and provide energy to people in the face of shocks while building the natural capital base upon which they depend and providing a livelihood for the people who make it function (Cabell and Oelofse, 2012).
Apart from redundancy, diversity is the key attribute for any system's capacity to cope with change, as a greater range of options reduces its vulnerability after the loss of specific elements (Folke et al., 2004; Hodbod and Eakin, 2015). Previous studies have promoted diversification as the core strategy to increase the robustness, absorption capacity, flexibility, resourcefulness, and adaptability of agricultural systems to external shocks (Zimmerer et al., 2019). Two types of diversity enhance resilience: functional diversity, i.e., the number of functionally different groups in a system, and response diversity, i.e., the number of different groups providing the same function (Walker and Salt, 2012). While functional diversity enhances a system's learning and adaptation capacity, response diversity increases its absorption capacity (Elmqvist et al., 2003; Hodbod and Eakin, 2015).
Agrobiodiversity is defined as the diversity of wild and domesticated genetic resources involved in obtaining agricultural products as well as non-harvested species that contribute to the functioning of an agroecosystem (Howard, 2010; Herforth et al., 2019). In general, diversified and sustainable farming systems mimic the biodiversity levels and functioning of local ecosystems (Altieri and Nicholls, 2020b) to generate multiple ecosystem services including increased nutrient and water efficiency; stimulation of the soil microflora and organic matter content; improved resilience to pests and meteorological perturbations; increased per-plant yield; and the sequestration of atmospheric carbon (Gliessman, 1985; Altieri, 1994; Letourneau et al., 2011; Altieri and Nicholls, 2020b). Agrobiodiversity also creates health and nutrition benefits. For example, a variety of crops offers farmers diverse diets comprised of vitamins, minerals, and phytochemicals that support the immune system (Yousafzai et al., 2013). Until the second half of the twentieth century, agricultural systems have been widely characterized by diversified farming (Rosset et al., 2011; Béné et al., 2015).
Despite the advantages of agrobiodiversity, the globalization and industrialization of food systems during the past decades have resulted in the simplification of farming systems and dominance of mechanized, high-input monocropping (Jäger et al., 2019; Hobbs, 2020). Consequently, diversified agriculture at present is widely limited to subsistent farms in low- and middle-income countries (Howard, 2010) and only 15 crops provide over 80% of the world's food calorie intake (Motley et al., 2006). This homogenization of farming systems has negatively impacted the functionality of ecosystem services and weakened their ability to prevent the development and spread of communicable diseases (Altieri and Nicholls, 2020b). For example, habitat simplification due to monocropping has increased human contact rates with disease vectors such as Lyme disease (Pongsiri et al., 2009; Rohr et al., 2019). Large-scale animal production has created opportunities for many viruses to mutate and spread directly to humans (Altieri and Nicholls, 2020b), including the H1N1 virus (causing “swine flu”), the H5N1 virus (cause of “bird flu”), and potentially SARS-CoV-2, the virus that causes COVID-19 (Jackson et al., 2007). The homogenization of farming systems also affects the socio-cultural resilience of farming communities by impacting social organizations, local culture, language, and cuisine (Ebel et al., 2021).
After an increased worldwide focus on restoring agrobiodiversity in the 1990s and early 2000s, the 2008 food crisis resulted in a global call for monocropping of staple crops (Renkow and Byerlee, 2010; Kahane et al., 2013). Nevertheless, a countermovement has continued to demand more local, diversified, and sustainable farming methods that are expanding worldwide, including in high-income countries such as the U.S. (Kolodinsky et al., 2020). However, diversified farms in the U.S. are still limited to niche sectors including certified organic farming and other sustainably managed, often horticultural, systems (Youngberg and DeMuth, 2013; Rosa-Schleich et al., 2019).
The states where this study was carried out, MT and SD, are part of the NGP ecoregion, which includes smaller parts of North Dakota, Wyoming, Nebraska, and southwestern Canada (Padbury et al., 2002). Agriculture is an integral component of the economy, history, and culture of the region (Conant et al., 2018). While only 1.6% of the U.S. population live in the NGP, the region contributes notably to the U.S. food supply, especially to the production of spring and durum wheat, sunflower seed (>70% of U.S. production), barley, lentils, and dry beans (>40% of U.S. production), winter wheat, oats, corn, sugar beets, and cattle, where the region generates over 20% of U.S. production (Ebel and Thornton, under review)1. The NGP are currently experiencing a transition in agricultural land use, mainly the conversion of grass and rangeland to the monocropping of annual crops (Sayre et al., 2012). Historically, wheat has been the dominant crop in the NGP; more recently, monocropping of barley, corn, soybeans, and pulses is gaining prominence (Heinemann et al., 2014; Conant et al., 2018; Herforth et al., 2019; Jäger et al., 2019).
Diversified cropping systems have not played an important role in the NGP during the past 100 years (Padbury et al., 2002; Conant et al., 2018). However, numerous NGP dryland farmers have recently begun to diversify their agricultural portfolio, especially by including pulses into their crop rotations. Also, small-scale diversified horticultural production is emerging in urban areas and fruit-producing regions, particularly in the western NGP (Gough, 1997; Long et al., 2014; Miller et al., 2015; Carlisle, 2016; Stoy et al., 2018; Warne et al., 2019).
The COVID-19 pandemic affected food, fiber, and bioenergy production and the associated industry of most commodities and scales of production (Naja and Hamadeh, 2020; Zurayk, 2020). One of the most immediate consequences of the pandemic was a series of bankruptcies of farms, particularly in the fresh-market horticultural sector (Richards and Rickard, 2020), which was triggered by three core factors: (1) shift toward the consumption of processed items (Altieri and Nicholls, 2020b; Béné, 2020); (2) decreased demand from hotels and restaurants because of health regulations and changes in consumer habits (Nicola et al., 2020); and (3) limited service or closure by food banks and other institutions that provide nutrition assistance and support the food security (Stephens et al., 2020).
In addition to decreasing consumer demand for many foods, farms in the U.S. faced disruptions and price increases in the supply chain for agricultural inputs including seeds and fertilizers (Altieri and Nicholls, 2020b; Béné, 2020). Although farmworkers were classified as essential workers and widely exempt from working and mobility restrictions (Béné, 2020; Benton, 2020), many seasonal foreign farm workers were unable to travel to their workplaces because of virus-related regulation measures as well as visa restrictions and delays (Torero, 2020). Livestock production systems, meat processors and packers, and horticultural producers were most affected by labor shortages (Stephens et al., 2020).
Before the pandemic, small-scale producers of locally marketed foods saw constant growth despite accounting for only 1.5% of the U.S. agricultural production (Low et al., 2015). During the pandemic, small producers, especially in the vegetable and fruit sector, were challenged by social-distancing regulations and decreased availability of farmworkers while production costs increased (Laborde et al., 2020; Orden, 2020). Whereas small-scale horticultural producers who sell their produce close to urban centers benefited from the lockdown measures (as many consumers opted for local food purchases and avoided large food retailers), small producers in rural settings faced economic challenges (Kolodinsky et al., 2020; Westervelt, 2020).
The pandemic created uncertainty among U.S. farmers (Reissman et al., 2006; Laborde et al., 2020), including about how to adapt their operations (farm management, crop choices) and impacts on their sales (Benton, 2020). Producer stress caused by uncertainty can result in unhealthy lifestyle patterns such as dietary choices with long-lasting effects on nutrition and health (Yousafzai et al., 2013). Globally, several studies indicate that the uncertainty related to the COVID-19 pandemic impacted a range of mental health conditions including loneliness, anxiety, stress, insomnia, denial, anger, post-traumatic stress disorder, psychological distress, and depression (Galea et al., 2020; Torales et al., 2020; Xiong et al., 2020) that have instigated an overall increase in substance abuse and domestic violence (Galea et al., 2020; Kalil, 2020).
Farming systems in the NGP were impacted by pandemic mitigation measures carried out during the initial phase of COVID-19. In MT, a stay-at-home order was implemented in April 2020 based on state and federal State of Emergency regulations (State of Montana, 2020a,b,c). In SD, despite the declaration of a state of emergency in March 2020, COVID-19 control measures did not include a stay-at-home order (South Dakota Department of Health, 2020).
Consequences of the lockdown and “stay at home” measures for farmers in the NGP included interrupted supply chains caused by bankruptcies of food distributors and processors, transport limitations (US Department of Agriculture, 2018; Afshin et al., 2019; Pyatt, 2020; Sy, 2020; Taylor, 2020), reduced commodity prices (DeLeon, 2020; Lynch et al., 2020), uncertain export markets (Kerr, 2020), and unpredictable demand due to changes in consumer behavior and spending (Nicosia, 2005; Bhattacharya, 2012). By April 2020, the economy of more than 85% of NGP farm operations was negatively affected by COVID-19 (Grimberg, 2020). While grain and oilseed producers were confronted with minor commodity price and supply chain issues (Brewin, 2020), livestock production was severely hit. Numerous dairy farmers in the region had to pour milk away during the first months of the pandemic (Torero, 2020). While direct sales were an area of opportunity for small-scale farms across the U.S., most NGP farms could not benefit from this trend as they serve commodity markets (Grimberg, 2020).
Despite a later normalization in food transportation and retail, the economy of most farms in the NGP started to recover long after the lockdown ended in May 2020 (Cates-Carney, 2020). In MT, many ranchers postponed selling their calves or feeder cattle for the late Fall months (Belasco, 2020). In contrast, grain sales were more stable (Cates-Carney, 2020). The situation in SD was similar, especially in the livestock sector. Ranchers in SD were not only affected by closings of processing facilities but also by limited exportation opportunities (Birkeland, 2020).
Research was carried out during the first growing season of the COVID-19 pandemic with managers of diversified farms in MT and SD, two states within the Northern Great Plains. Our study focused on producers of diversified farms given that diversification is a core strategy for increasing the resilience of farms facing external shocks (Zimmerer et al., 2019). Specifically, we assessed producer perceptions in response to COVID-19, characterization of farm management practices and crop diversity, and food security of farms and food security concerns of farming communities based on the perceptions of 53 farm managers.
For our study, diversified farms were identified as operations using a whole system approach to agriculture that is based on promoting biodiversity and ecosystem services from field to landscape scales based on one or more of the following types of farm management practices to enhance diversity (UC Berkley, 2020): (1) polycropping (multiple crops and/or varieties); (2) complex crop rotations; (3) holding of different livestock species; (4) integration of fish and/or livestock in plant production; (5) maintenance of hedgerows or live fences around farming areas; and (6) adoption of techniques to increase the biodiversity of a farm's surrounding landscape.
The study inclusion criteria involved: (1) producers (those who own, lease, are hired, or have some other arrangement of working on a farm) working on a farm located in MT or SD; (2) producers who have grown crops or held animals for at least one season; and (3) producers that classify their agricultural systems as diversified farms based on the aforementioned definition.
The development of the survey was informed by two research questions (1) What were producer perceptions, experiences, and responses to the COVID-19 pandemic during its initial phase? and (2) How did the early phase of the COVID-19 pandemic impact crop diversity, farm management practices, the emotional well-being of surveyed producers, and the economy of farms and farming communities?
We used the agroecosystem resilience assessment framework developed by Cabell and Oelofse (2012) as a baseline for developing a self-administered online survey, and for interpreting participant responses. The agroecosystem resilience assessment framework consists of 12 resilience indicators, out of which five were considered suitable to address our study questions and applied in the survey section “Farm management and crop diversity” (Table 1). To address producer perceptions of the impact of COVID-19 on their agroecosystems more broadly, we drew questions from the rapid C-SCAN (COVID-19 Surveillance Community Action Network for Food Systems) survey tool (Ahmed et al., 2020). Furthermore, the survey included the validated 2-item measure to screen for food insecurity by Gundersen et al. (2017).
The survey instrument was subsequently modified and refined through pilot testing for input from field experts. The final tool included a self-administered survey with a total of 31 questions divided into five sections. In this article, data from a subset of 13 survey questions were used (see Supplementary Appendix 1 for survey questions). The survey questions addressed in this article comprise of six single-select multiple-choice questions (all framed as binary yes/no questions with the possibility to specify information using text inputs), three multi-select multiple-choice questions, two open-ended questions, and two three-step Likert-Scale questions (Brown, 2011; Gundersen et al., 2017).
Participation in the study was voluntary and anonymous. Prior informed consent was obtained from all participants. The consent form, study introduction, and survey instrument were approved by the Montana State University Institutional Review Board (approval number SA042720-EX) in April 2020. The introduction to the survey presented the aims and procedures of the survey, including the anonymity and confidentiality of responses, inclusion criteria, and contact details of the research team and Institutional Review Board.
The survey was distributed online and included the study introduction and informed consent using the online platform Qualtrics (SAP, Provo, UT) (Oppenheimer et al., 2011). Data were collected May 1st through August 7th, 2020. The research team distributed the survey using farming listservs in the study area as well as posting a link to the survey and study introduction in various social media venues and newsletters. To encourage responses, a $15 gift card was provided as an incentive for participants upon completion of the survey. Completed surveys were given identifiers for tracking purposes only. We obtained 120 responses (response rate of a~20%) of which 53 were validated and included in the study analysis.
We excluded incomplete and inconsistent responses and the remaining survey data was validated using Cronbach's Alpha Test as a measure for data consistency (Ferketich, 1990; Vaske, 2019). We obtained a value of 0.67, which is adequate for scales in human dimension research (Vaske et al., 2017).
Of the 53 validated responses, 31 were from producers with farms in MT and 22 from producers with farms in SD. Surveyed farms in MT were smaller than in SD (Table 2, Supplementary Appendix 2). In the majority of farms, agriculture generates <75% of the household income and most surveyed producers own their farmland. Surveyed producers with more than 5 years of farming experience tended to work on larger farms (>100 acres), less experienced producers on smaller ones (Supplementary Appendix 3). On larger farms, more than 90% of production was designated for sale, while this share was lower for smaller farms (Supplementary Appendix 3). Thirty-eight surveyed farms were dedicated to plant production (out of which ten can be classified as strictly horticultural producers), four produced livestock, five have a mixed farm, and six producers did not specify their farm products. Only eight farms produced one single commodity (in addition to non-commercial diversification measures such as pollinator strips). The median number of commodities per farm was four. In 2020, the most common crops on surveyed farms were annual vegetables, followed by corn, wheat, berries, dry legumes, oats, and soybean. The most common livestock was cattle (Supplementary Appendix 4).
Table 2. Characteristics of farms managed by surveyed producers: farm size, farm's contribution to household economy, destination of farm outputs, farm ownership, and farming experience of farm manager per state where farm is located.
The survey responses generated quantitative data from multiple-choice and Likert-type questions (these data were also used for the overall assessment of agroecosystem resilience), as well as qualitative data from the responses of the open-ended questions, and the explanations elicited from the multiple-choice questions.
All analyses were conducted using SPSS for Windows, v 26 (IBM, Armonk, NY) (De Sá, 2007). First, we described the perceived impact of COVID-19 on farms, producers, and their communities from the responses to binary (yes/no) survey questions. Responses were transformed into numeric binary values (Brown, 2011). For all data, we conducted descriptive statistics, which included frequency and percentage response distributions as well as dispersion measures.
For the analysis of the farm management practices, we grouped the practices into three categories: (1) sustainable tillage (including conservation tillage or direct seeding), (2) sustainable fertilization (use of compost, compost tea, charcoal, and/or manure), and (3) cropping system diversification (crop rotations, intercropping, and/or cover cropping). Inter-associations were assessed using the two-sided Fishers Exact Test at p < 0.05 (Upton, 1992).
Regarding the impact of COVID-19 on farm management and crop diversity, farm economy, producers' emotional well-being, and producer food insecurity, we used cross-tabulations and chi-square tests to identify significant (p < 0.05) di?erences on how the pandemic affected several characteristics of farm management and food security (Chambers and Skinner, 2003).
To identify factors that influence producers' perceptions of COVID-19, we contrasted the responses of the perceived impact of COVID-19, with data about farm management practices, including diversification. This data originated from multi-option questions, which we converted into ordinal integer numbers (Brown, 2011) with 0 as the lowest value (example question on farm size: 0 for <5 acres; 4 for > 1,000 acres). We conducted univariate association analysis using the Chi-square test for categorical, non-parametric variables. Significance was considered at p < 0.05 (Chambers and Skinner, 2003). To quantify relationships, we applied a two-tailed Cramer's V test, where a value <0.2 indicated a weak association, 0.2 ≤ 0.3 a moderate association, and >0.3 strong association (Santos-Díaz et al., 2019).
For the analysis of open-ended questions, we conducted inductive, undirected content analysis to identify common themes in the responses (Kuckartz, 2014; Saldaña, 2015). The coding process was facilitated by the qualitative software NVivo 12 (QSR International, Doncaster, Australia). We applied inductive coding, where we prioritized in vivo codes, resumed all condensed meaning units, and calculated the frequency of meaning units per code as a percentage of total meaning units per survey question (Leech and Onwuegbuzie, 2011; Saldaña, 2015).
The assessment of agroecosystem resilience (Table 1) was adapted from five parameters of the Cabell and Oelofse (2012) resilience framework as well as three additional parameters for food system resilience and vulnerability (Ericksen et al., 2012; Intergovernmental Panel on Climate Change, 2014). For each parameter, we calculated the respective values as a proxy based on response rates to quantitative survey questions. The overall assessment was calculated on a percentual scale, where 100% represents the highest resilience possible to the pandemic (see Table 3 for equations) and visualized in a radar chart.
Table 3. Equations, adapted from Cabell and Oelofse (2012), processed for the overall assessment of the resilience of the participating farms.
Our study deals with diversified farmers in MT and SD, an emerging community in two states widely characterized by conventional monocropping systems (Sayre et al., 2012; Conant et al., 2018) during the initial stages of the COVID-19 pandemic. To our knowledge, there is no data available on the total number of diversified farms in these states, considering how diversification is defined in our study (UC Berkley, 2020). However, in 2019, there were 171 certified organic farms with annual revenue of < $500,000 in MT, and 63 in SD (US Department of Agriculture, 2020). Although certified organic management was not a selection criterion for our study, based on our survey findings (see Section Characterization of Agroecosystem Management Practices) and field experience, approximately half of the diversified farms in the region are small organic farms. Hence, it can be considered that our sample of 53 surveyed farm managers represents at least 15% of diversified farms in MT and SD combined.
One reason for the relatively low response rate was the difficult working and communication conditions during the first months of the COVID-19 pandemic that limited us to reach producers by email instead of visiting farms to interact with farmers in depth. We acknowledge that our outcomes would have been more significant if obtained through a triangulation of methods, including complementary key informant interviews or mapping. Yet, the results obtained from this study address a sector of agricultural production in the NGP that is emerging and currently understudied.
It was our purpose to obtain a snapshot of the state of diversified farms in the NGP during the early phase of the pandemic, which led to a relatively short time frame for its implementation. We recognize that the short time window of the survey, despite providing important insight into the early impact of the pandemic on diversified farms, may have reduced the validity of our data, especially of aspects not directly related to the pandemic. Yet, our dataset is unique as it allows us to assess a phenomenon for which no previous information exists. For future studies, it may also be interesting to compare the responses of diversified farmers to a stressor like COVID-19 to those of a control group, for example, operators of monocropping farms, while controlling for other variables such as operation size, years of expertise, or annual income from agricultural production.
Almost half the respondents [46%, out of n = 52, no response (NR) = 1] reported that they follow certified organic agriculture guidelines (Figure 1). Overall, the surveyed producers articulated that they are using different combinations of sustainable crop management practices on their farms. Findings indicate that most of the surveyed producers who are not certified organic use crop rotations, cover cropping, and conservation tillage (p < 0.05).
Figure 1. Frequency of compliance with organic standards and use of specific agroecosystem management practices of surveyed producers (% of responses, n = 53). *Conservation Tillage or Direct Seeding. **Use of compost, compost tea, charcoal, and/or manure. ***Crop rotations, intercropping and/or cover cropping.
The majority of surveyed producers (68% out of n = 53) reported they did not undertake changes in their farm management practices because of the COVID-19 pandemic. Also, 75% of producers stated that they did not intend to change their crop choices for 2021 (Table 4). Our findings highlight that those producers who apply sustainable fertilization techniques such as composting, application of manure, and crop rotations were more likely to modify their farm management because of COVID-19 (Tables 5, 6). When asked about drivers of farming management changes before COVID-19, most respondents mentioned markets as the central motive (85%, n = 40), while subsidies (20%, n = 40), and land use (18%, n = 40) played a minor role (Figure 2).
Table 5. Farm management changes due to COVID-19, based on farming practices applied by surveyed producers.
Table 6. Crop choice changes due to COVID-19 in the 2020 and 2021 cropping season, based on farming practices applied by surveyed producers.
Figure 2. Drivers of shifts in farm management. Factors that have influenced farming practice changes in the past (n = 53). *Significant (p < 0.05). **No significant difference between responses.
Out of the 19 producers who changed their crop rotations and/or crop choices because of the pandemic (43% of surveyed producers, n = 53), the majority stated they did so for increasing production to compensate for price decreases. The notion of price-loss compensation was more frequently reported among SD producers, who manage larger and more grain-focused operations, than among MT producers. Specifically, increasing production was most common among corn and soybean producers, followed by producers of small grains other than corn. Three producers reported pandemic-related changes in their livestock production management.
Eighty-nine percent of surveyed producers (out of n = 53) were concerned that the COVID-19 pandemic was impacting the future of farming in general. Most concerns were related to “unstable and shrinking markets for agricultural products.” The majority of producers (62%) were anxious about their community's food security while only 16% expected their access to food to be affected by the pandemic (Table 7). Based on the two-item food insecurity screening tool by Gundersen et al. (2017), one producer (2% of respondents) reported having been food insecure between March and August 2020. Five producers (10% of respondents, n = 53) said they were food insecure for a specific period in 2020. The remaining 43 producers (88% of respondents, n = 53) stated they were not food insecure during the survey period. Almost half of all surveyed producers stated that they did not depend on external food sources, as most of their food comes from their farms, hunting, or gathering (40%, n = 53). Most producers were not apprehensive about access to fresh fruits and vegetables (91%, n = 53). A fifth of producers reported confidence in their local food retailers (21%, n = 53).
Producers who expected a negative impact of the COVID-19 pandemic on their community (62% of all respondents, n = 53) were also those most concerned about the economy of their local fellow producers. Specific pandemic-related concerns shared by producers include (1) disruptions in supply chains and a corresponding shipping cost increase (9 out of the 33 producers who expected a negative community impact); and (2) decreasing consumer demand due to lower income, rising unemployment, and previous stockpiling of food (11 out of 33 producers). Specifically, producers reported anxiety about the local, higher-priced segment of the market (such as CSAs), which several producers expected to shrink. Eleven out of 33 respondents to this question were also concerned about their community's access to food, especially in remote areas of the region where grocery stores are scarce.
Most producers stated that their access to commodities, farm inputs, as well as the availability of farm labor, were not impacted during the early stages of the COVID-19 pandemic (Table 7). However, over half of producers (62%, n = 53) reported that they expected their total farm income to shrink in 2020 (Table 7).
Roughly half of the surveyed producers (47%, n = 53) stated that their overall anxiety increased because of the COVID-19 pandemic. Accordingly, multiple producers articulated an “anxiety of the unknown.” For example, two producers reported that they were having sleepless nights due to the pandemic.
The most frequently mentioned concerns of producers during the pandemic involved financial issues, supply chain disruptions, anxiety about family and loved ones, personal health concerns, how to implement social distancing in farm work, and concerns about the negative impacts of the pandemic on society as a whole.
Out of the producers that did not report increased anxiety because of the pandemic (53%, n = 53), three themes were identified that kept them grounded: (1) faith or religion; (2) confidence in their food self-sufficiency; and (3) expanded market opportunities for their farm operations because of an expected increased consumer demanding for local food.
We identified three factors that shaped producers' perceptions of the effect of COVID-19 on their farming systems and communities: (1) farming experience; (2) farm size; and (3) contribution of farming to household income.
Farming experience had a considerable impact on producers' perceptions of COVID-19. Most “new” producers (those who had been in charge of their farm for <2 years) changed their farm management practices because of the pandemic (80%, n = 42, NR = 9), and 40% of them also altered their crop portfolio in 2020 (Figure 3; see Supplementary Appendix 5 for details). In contrast, most producers with more than 5 years of experience did not shift their farm management practices (84%) or modified their 2020 crop rotations (81%).
Figure 3. Impact of producers' experience on farm management and cultivated crops (% of all responses, n = 42, NR = 9). *Strong relationship [Cramer's V >0.3 ∧ p (Chi-Square) < 0.05].
In terms of farm size, only 21% (n = 42, NR = 9) of surveyed producers operating on farms smaller than 100 acres were concerned that the pandemic would affect the available workforce needed for their farms, while 73% of the managers of larger farms, stated that the availability of farm labor was a concerning issue. Only 10% of managers of small producers were anxious about their household diet, while this share was higher (31%) among operators of larger farms (Figure 4, Supplementary Appendix 6).
Figure 4. Impact of farm size on perceived available farm labor and household diet (% of all responses, n = 42, NR = 9). *Moderate relationship [Cramer's V >0.2 ∧ p (Chi-Square) < 0.1]. **Strong relationship (V > 0.3 ∧ p < 0.05).
The third factor impacting the producers' perception of the impact of COVID-19 was the contribution of farming to a household's overall economy. Where farming provided more than 75% of the household income, 73% of producers (n = 42) were concerned about losses of household income due to the pandemic. As for part-time producers, where farming contributes to <75% of the household economy, 69% stated that they did not expect COVID-19 to seriously affect their household income (Figure 5, Supplementary Appendix 7). Among those part-time producers concerned about their income (31%), almost all lost their off-farm jobs or faced salary decreases in their side jobs.
Figure 5. Impact of contribution of agriculture to total household economy on perceived household income and household diet (% of all responses, n = 42, NR = 9). *Strong relationship (Cramer's V >0.3 ∧p [Chi-Square] < 0.05).
On the basis of the adapted agroecosystem assessment framework (Cabell and Oelofse, 2012), survey responses indicated that shock exposure had the highest resilience score within the 53 farms, specifically, access to commodities, farm inputs, and farm labor. In contrast, two farm-management-related parameters, ecological self-regulation (presence of riparian buffers, pollinator strips, hedgerows, and trees) and connectedness (use of inter- and cover cropping, inter- and intraspecific diversity), revealed a low overall resilience score (Figure 6). The parameter of ecological self-regulation was found to have a lower resilience score for larger farms (>100 acres) than for smaller farms, while larger farms had a higher resilience score for autonomy and local interdependence (the contribution of farming to a household's income) (Figure 7). In terms of food security, access to food was not considered a limiting factor by most producers, which indicates high resilience and self-sufficiency. However, producers expressed considerable concern regarding the overall food security in their communities.
Figure 6. Overall assessment of the perceived resilience of the participating farms (n = 53) based on Cabell and Oelofse (2012) framework and additional food system resilience parameters (Ericksen et al., 2012; Intergovernmental Panel on Climate Change, 2014), 100% represents the highest resilience.
Figure 7. Assessment of the perceived resilience of the participating farms (n = 53) divided into farms larger and smaller than 100 acres based on Cabell and Oelofse (2012) framework and additional food system resilience parameters (Ericksen et al., 2012; Intergovernmental Panel on Climate Change, 2014), 100% represents the highest resilience.
This study highlights that while national and global food systems experienced disruptions during the early stages of the COVID-19 pandemic, the surveyed producers of diversified farms in the NGP expressed long-term confidence regarding local food production from diversified farms during the first growing season of the pandemic. Survey findings demonstrate relatively “mild” responses by farmers to the initial phase of the pandemic compared to other national and international studies administered during this time of heightened uncertainty. The disruptions associated with the COVID-19 pandemic have emphasized the need to strengthen the resilience of food systems to future external disturbances (Orden, 2020). Given that the surveyed producers managed diversified farms to supply local food systems, the results of this study highlight the potential of diversified farming and local food systems for strengthening resilience to support food security in the context of extreme shocks.
The population highlighted in this study, farmers who practice diversified agriculture in the NGP, represents a unique sector of the regional agricultural production. The percentage of small-scale horticultural producers in our sample is disproportionally higher compared to typical farms in the NGP (Gough, 1997; Long et al., 2014; Miller et al., 2015; Carlisle, 2016; Stoy et al., 2018). In addition, the percentage of producers following certified organic farming guidelines is higher in our sample (46%) compared to the region, where it is <3% (US Department of Agriculture, 2019). The percentage of producers following sustainable farming practices such as conservation tillage, use of manure, crop rotations, intercropping, and cover cropping is also elevated among our sample.
A further unique attribute of the surveyed producers is their history as farm owners; 15% of the respondents were recent farmers with 2020 being their second farming season, while 96% of all MT and SD farms are multiple-generation family farms (US Department of Agriculture, 2019). Thus, findings indicate that farming experience strongly impacted producer responses to the COVID-19 pandemic. While 84% of producers with more than 5 years of experience did not change their farm management practices because of the pandemic, 80% of the “newcomers” (<5 years of experience) did change their practices in adaptation to the new situation. The unique features of the surveyed farmers are important to consider in the interpretation of study findings, especially because pandemic-related supply chain disruptions were strongest among meat and dairy NGP producers (Belasco, 2020; Brewin, 2020), and both sectors were underrepresented in our study.
The relatively “milder” responses to the COVID-19 pandemic among the surveyed producers compared to evidence from national (Belasco, 2020; Béné, 2020; Wolfson and Leung, 2020) and international (Benton, 2020; Elleby et al., 2020; Kerr, 2020; Torero, 2020) studies, indicate an elevated level of shock absorption capacity. For example, most surveyed producers did not extensively change their farming practices in response to the COVID-19 pandemic and associated measures, which differs from the strong disruptions of farm management practices due to COVID-19 at the global level (Torero, 2020). Also, the reduced availability of farm labor that challenged producers throughout the U.S. (Béné, 2020; Benton, 2020) was only a prevalent concern among larger-scaled surveyed farms.
We also found relatively “milder” responses regarding emotional well-being compared to other studies administered during the study period. While a more difficult access to markets and a generally perceived uncertainty affected the emotional well-being of most farmers globally (Reissman et al., 2006; Benton, 2020; Laborde et al., 2020), 53% of producers in our survey stated they were not anxious during the initial months of the pandemic. These findings demonstrate a remarkably low shock sensitivity of the surveyed producers during uncertain times. Concerns were stronger among part-time and smaller producers as they feared side-job losses and decreasing household income.
While food insecurity due to COVID-19 skyrocketed in the U.S. (Laborde et al., 2020; Swinnen and McDermott, 2020), including in MT (Montana Food Bank Network, 2020) and SD (Lowrey, 2020), access to food was not considered an alarming issue by 88% of surveyed producers. We hypothesize that there are three reasons for the low perceived food insecurity in our study. First, 40% of surveyed producers do not depend on commercial food for their diet because a considerable part of the food they consume is produced on their farms or obtained in wild food environments, including hunting and gathering in their surroundings. Almost half of the surveyed producers stated that they use at least 10% of their production for family consumption, and a quarter of farms use more than 50% of their production for family subsistence. This reliance on non-commercial food sources of food helps explain the high food security during the pandemic. Second, respondents demonstrated high confidence in their local food retailers to provide accessible food. Lastly, producers showed optimism that the COVID-19 pandemic may increase long-term demand for food produced on diversified farms.
The overall resilience self-assessment, based on producer responses and applying the agroecosystem assessment framework of Cabell and Oelofse (2012), suggested that the producers' relatively moderate response to the pandemic was enabled by diversified farm management practices and influenced by farm size. Larger farms showed a higher economic autonomy compared to smaller farms, mostly because they are operated by full-time producers and consequently do not depend on side jobs. However, larger farms had lower ecological self-regulation indices, which refer to practices such as pollinator strips. The integration of local natural resources (including fertilization with manure and compost), as well as the spatial and temporal farm system heterogeneity (including the frequent use of crop rotations and diverse farm management practices), were found to be high in both large and small farms (Figure 7). Our study suggests that experience with diversification influenced the producers' ability to respond to an external stressor like the COVID-19 pandemic. Farms where multi-crop rotations and sustainable soil fertility management were common before 2020, were more likely to alter crop rotations than farms with a shorter history of sustainable management practices.
The COVID-19 pandemic exposed the fragility of global and national food systems (Altieri and Nicholls, 2020a; Béné, 2020), with severe socio-economic and emotional consequences for food system stakeholders across scales. Diversification is increasingly recognized as a core strategy to increase food system resilience, whether it be short- and mid-termed shocks such as COVID-19, or gradually evolving complex disruptions such as climate change (Rotz and Fraser, 2015; Tendall et al., 2015; Zimmerer et al., 2019). At the individual farm, diversification includes that of plants and animals at the genetic and species levels, as well as diversity-enhancing farm management practices including complex crop rotations or the integration of plant and animal production (Béné et al., 2015; UC Berkley, 2020). During the past 70 years, diversified farms in the U.S., as in other high-income countries, have been widely pushed back by conventional high-input monocropping systems (Jäger et al., 2019; Hobbs, 2020). Nowhere in the U.S. is the simplification of farming systems more visible than in the small-grain producing breadbasket regions of the Midwest and the Great Plains (Bagley et al., 2012), including the NGP. While over the past decade, there has been a resurgence of farm system diversification in the U.S. (Kolodinsky et al., 2020), these farms represent a niche sector within millions of acres of “Big Ag,” and most existing diversified farms are small in size and managed by relatively inexperienced producers. The study presented here revealed that diversified small-scale producer responses to the COVID-19 pandemic differed from those of conventional producers. We found that the majority of surveyed producers did not extensively change their farming practices during the study period comprised of the early months of the pandemic, which contrasts with profound farm management changes witnessed regionally, nationally, and globally (Torero, 2020). Several producers, especially those who operate complex and diverse farms, implemented punctual management and crop rotation changes.
While food insecurity increased across MT (Montana Food Bank Network, 2020) and SD (Lowrey, 2020) in 2020, food security and accessibility were not severe concerns of most surveyed producers. Reasons for the producers' high perceived level of food security during the pandemic include the possibility to access food from their farms and for 40% of them, the procurement of food through hunting and gathering. Additionally, the confidence of the surveyed producers in the local food system to provide sufficient food was high. Hence, this study suggests that diversified farms have an elevated capacity to absorb an external shock as severe as the COVID-19 pandemic. We also found that larger farms were economically more independent than smaller ones. For example, off-farm income allowed large farms to avoid “panic reactions” such as changing their crop rotations, indicating that the economic status and antecedents of a farm sharply determine its capacity to absorb shocks.
This study further examines potential factors that limited the surveyed farms from benefitting more from the diversification of their operations. Producers of small farms in our study were often highly dependent on off-farm income, which impacted their economic stability and emotional well-being. Larger farms that lacked an extensive diversification of their systems could benefit from a greater number of diversification practices to enhance resilience.
Diversification of agroecosystems should be recognized as a process (not a goal) across ecological, social, and institutional dimensions of farming systems (Petersen-Rockney et al., 2021a). The more advanced the level of diversification on a farm, the more effective it is for enhancing resilience (Altieri, 1994). Farms in the NGP should engage in a process of constantly increasing diversification including diversifying farm business and management models, governing institutions and policies, farmer demographics, markets, values and goals, and knowledge systems toward improving adaptive capacity in the context of shocks (Petersen-Rockney et al., 2021b). Policies, market, and education programs are called for to promote the continuous enhancement of farm diversification for enhancing food system resilience. If policies, market, education, and research re-orient their efforts to enable the establishment of an increasing number of diversified farms, a whole region such as the NGP can achieve a greater ability to provide food security to their communities as well as support farmer livelihoods in the context of global challenges including climate change, population growth, and future pandemics. Based on the findings of our study and the literature cited here, we recommend the following pathways for increasing and maintaining diversification and the resilience of agricultural production systems:
(1) Development of evidence-based agroecological farm management plans. Evidence-based agroecological farm management plans that are tailored to different farming scales and agroclimatic regions are needed for enhancing diversification including at the functional diversity and landscape levels. For example, producers in regions such as the NGP that practice wide crop rotations can be encouraged to also conduct mixed cropping. These diversified farms, in turn, will be more resilient to local and global disruptions.
(2) Dissemination of evidence on the benefits of agricultural diversification. Efforts are needed to broadly disseminate evidence on the benefits of agricultural diversification and associated farm management plans to producers and other food system stakeholders through media, extension, and education to enhance the resilience of food systems.
(3) Research on the role of agricultural diversification for producers' emotional wellbeing and personal resilience. Research is called for to examine the potential role of agricultural diversification for producers' emotional wellbeing and personal resilience. The low anxiety rates of the surveyed producers during the initial stages of the pandemic and their optimism regarding the long-term growth of their farm operations suggest that diversity not only enhances the environmental and economic shock absorption capacity but also benefits the emotional well-being of producers.
(4) Policy and incentives to remove barriers for new farmers and for enabling diversification. Policy and programs are necessary to support new farmers to pursue farming full-time while providing enabling conditions for diversification. The high dependence of small-diversified farms on off-farm income lowers their flexibility in responding to shocks and their potential to experiment and observe on their farms. For example, peer-to-peer exchange platforms with experienced farmers can support new farmers build knowledge and enhance their capacity to respond to shocks.
The original contributions presented in the study are included in the article/Supplementary Material, further inquiries can be directed to the corresponding author.
The studies involving human participants were reviewed and approved by Institutional Review Board (IRB) at Montana State University. The patients/participants provided their written informed consent to participate in this study.
SA and MJ led the study conception. SA and TW developed the survey and led the study design. TW piloted the survey and led the acquisition of data. RE led data analysis with support from AM. RE, SA, IG, MJ, and FM contributed to data interpretation. RE led the drafting of the manuscript with essential contributions to writing and revising from all SA, IG, and FM. All authors contributed to the article and approved the submitted version.
This work was supported by the National Science Foundation (NSF; EPSCoR Track II OIA-1632810), the USDA National Institute of Food and Agriculture (NIFA; Project # MONB12440586), and the U.S. National Institute of General Medical Sciences of the National Institutes of Health (NIH NIGMS P20GM103473 and 5P20GM104417).
The authors declare that the research was conducted in the absence of any commercial or financial relationships that could be construed as a potential conflict of interest.
All claims expressed in this article are solely those of the authors and do not necessarily represent those of their affiliated organizations, or those of the publisher, the editors and the reviewers. Any product that may be evaluated in this article, or claim that may be made by its manufacturer, is not guaranteed or endorsed by the publisher.
We are grateful to all producers who completed this survey for sharing their experiences and perceptions as well as for their time. In addition, we are grateful to the organizations who helped distribute this survey including MT AERO, Montana Organic Association, and Open and Local. We thank Alyssa Stewart for supporting the creation and piloting the survey.
The Supplementary Material for this article can be found online at: https://www.frontiersin.org/articles/10.3389/fsufs.2022.668335/full#supplementary-material
1. ^Ebel, R., and Thornton, A. (under review). The Importance of the Food System for Maintaining Rural Population Densities in the Northern Great Plains. Unpublished manuscript.
Afshin, A., Sur, P. J., Fay, K. A., Cornaby, L., Ferrara, G., Salama, J. S., et al. (2019). Health effects of dietary risks in 195 countries, 1990 and 2017: a systematic analysis for the Global Burden of Disease Study 2017. Lancet 393, 1958–1972. doi: 10.1016/S0140-6736(19)30041-8
Ahmed, S., Downs, S. M., Yang, C., Chunlin, L., ten Broek, N., and Ghosh-Jerath, S. (2020). Rapid tool based on a food environment typology framework for evaluating effects of the COVID-19 pandemic on food system resilience. Food Security 12, 773–778. doi: 10.1007/s12571-020-01086-z
Altieri, M. A. (1994). Bases agroecológicas para una producción agrícola sustentable. Agric. Técnica 54, 371–386.
Altieri, M. A., and Nicholls, C. I. (2020a). Agroecology and the emergence of a post COVID-19 agriculture. Agric. Human Values 37, 525–526. doi: 10.1007/s10460-020-10043-7
Altieri, M. A., and Nicholls, C. I. (2020b). Agroecology and the reconstruction of a post-COVID-19 agriculture. J. Peasant Stud. 47, 881–898. doi: 10.1080/03066150.2020.1782891
Bagley, J. E., Desai, A. R., Dirmeyer, P. A., and Foley, J. A. (2012). Effects of land cover change on moisture availability and potential crop yield in the world's breadbaskets. Environ. Res. Lett. 7, 014009. doi: 10.1088/1748-9326/7/1/014009
Baldwin, R., and Tomiura, E. (2020). “Thinking ahead about the trade impact of COVID-19,” in Economics in the Time of COVID-19, eds. R. Baldwin and B. Weder di Mauro (London, UK: Centre for Economic Policy Research), 59–73.
Belasco, E. (2020). Cattle Market Expectations for Fall Under COVID-19. Bozeman MT: Montana State University.
Béné, C. (2020). Resilience of local food systems and links to food security—a review of some important concepts in the context of COVID-19 and other shocks. Food Secur. 12, 805–822. doi: 10.1007/s12571-020-01076-1
Béné, C., Frankenberger, T., and Nelson, S. (2015). Design, monitoring and evaluation of resilience interventions: conceptual and empirical considerations. IDS Working Papers 459, 1–23.
Benton, T. G. (2020). COVID-19 and disruptions to food systems. Agric. Human Values 37, 577–578. doi: 10.1007/s10460-020-10081-1
Bhattacharya, D. (2012). “Trade and health: Emergent paradigms and case studies in infectious diseases,” in Global Health Disputes and Disparities (London: Routledge), 107–125
Birkeland, K. (2020). Impact of COVID-19 on Agriculture with SDFB Executive Director Krystil Smit and Professor Chair of Economics and Decisions Sciences at USD. Huron SD: South Dakota Farm Bureau. Available online at: https://www.youtube.com/watch?v=N0IVBlzDwq8andfeature=youtu.be (accessed Sep 12, 2020).
Brewin, D. (2020). The impact of COVID-19 on the grains and oilseeds sector. Can. J. Agric. Econ. 68, 185–188. doi: 10.1111/cjag.12239
Cabell, J. F., and Oelofse, M. (2012). An indicator framework for assessing agroecosystem resilience. Ecol. Soc. 17:18. doi: 10.5751/ES-04666-170118
Carlisle, L. (2016). Lentil Underground: Renegade Farmers and the Future of Food in America. New York City: Avery.
Carpenter, S., Walker, B., Anderies, J. M., and Abel, N. (2001). From metaphor to measurement: resilience of what to what? Ecosystems 4, 765–781. doi: 10.1007/s10021-001-0045-9
Cates-Carney, C. (2020). Montana Ag Producers Say They Need More COVID-19 Relief. Helena MT: Montana Public Radio. Available online at: https://www.mtpr.org/post/montana-ag-producers-say-they-need-more-covid-19-relief [accessed Sep 12, 2020].
Conant, R. T., Kluck, D., Anderson, M. T., Badger, A., Boustead, B. M., Derner, J. D., et al. (2018). “Northern great plains,” in Global Change Research Program. ed USGS (Washington DC: US Global Change Research Program).
Ebel, R., Menalled, F., Ahmed, S., Gingrich, S., Baldinelli, G. M., and Félix, G. (2021). “How biodiversity loss affects society,” in Handbook on the Human Impact of Agriculture, ed S. Harvey. (Cheltenha, UK: Edward Elgar Publishing).
Elleby, C., Domínguez, I. P., Adenauer, M., and Genovese, G. (2020). Impacts of the COVID-19 pandemic on the global agricultural markets. Environ. Resour. Econ. 76, 1067–1079. doi: 10.1007/s10640-020-00473-6
Elmqvist, T., Folke, C., Nyström, M., Peterson, G., Bengtsson, J., Walker, B., et al. (2003). Response diversity, ecosystem change, and resilience. Front. Ecol. Environ. 1, 488–494. doi: 10.1890/1540-9295(2003)001%5B0488:RDECAR%5D2.0.CO;2
Ericksen, P., Bohle, H.-G., and Stewart, B. (2012). “Vulnerability and resilience of food systems,” in Food Security and Global Environmental Change (London, UK: Routledge), 87–97.
Ericksen, P. J. (2008). Conceptualizing food systems for global environmental change research. Global Environ. Change 18, 234–245. doi: 10.1016/j.gloenvcha.2007.09.002
Ferketich, S. (1990). Internal consistency estimates of reliability. Res. Nurs. Health 13, 437–440. doi: 10.1002/nur.4770130612
Folke, C., Carpenter, S., Walker, B., Scheffer, M., Elmqvist, T., Gunderson, L., et al. (2004). Regime shifts, resilience, and biodiversity in ecosystem management. Annu. Rev. Ecol. Evol. Syst. 35, 571–581. doi: 10.1146/annurev.ecolsys.35.021103.105711
Galea, S., Merchant, R. M., and Lurie, N. (2020). The mental health consequences of COVID-19 and physical distancing: the need for prevention and early intervention. JAMA Intern. Med. 180, 817–818. doi: 10.1001/jamainternmed.2020.1562
Gliessman, S. R. (1985). “Multiple cropping systems: a basis for developing an alternative agriculture,” in Innovative Biological Technologies for Lesser Developed Countries: Workshop Proceedings, ed. OoTA US Congress (Washington, DC: Congress of the USA), 67–83.
Gough, R. (1997). Growing vegetables under the big sky. J. Veg. Crop Prod. 3, 79–91. doi: 10.1300/J068v03n01_10
Grimberg, I. (2020). Western SARE COVID-19 Survey Report. Bozeman, MT: Western SARE. Available online at: https://western.sare.org/resources/responses-to-covid-19-in-western-ag/ (accessed Feb 15, 2021).
Gundersen, C., Engelhard, E. E., Crumbaugh, A. S., and Seligman, H. K. (2017). Brief assessment of food insecurity accurately identifies high-risk US adults. Public Health Nutr. 20, 1367–1371. doi: 10.1017/S1368980017000180
Heinemann, J., Massaro, M., Coray, D., Agapito-Tenfen, S., and Wen, J. D. (2014). Sustainability and innovation in staple crop production in the US Midwest. Int. J. Agric. Sustain. 12, 71–88. doi: 10.1080/14735903.2013.806408
Herforth, A., Johns, T., Creed-Kanashiro, H. M., Jones, A. D., Khoury, C. K., Lang, T., et al. (2019). “Agrobiodiversity and feeding the world: more of the same will result in more of the same,” in Agrobiodiversity: Integrating Knowledge for a Sustainable Future, eds. K.S. Zimmerer and S. de Haan (Cambridge, MA: MIT Press), 185–210.
Hobbs, J. E. (2020). Food supply chains during the COVID-19 pandemic. Can. J. Agric. Econ. 68, 171–176. doi: 10.1111/cjag.12237
Hodbod, J., and Eakin, H. (2015). Adapting a social-ecological resilience framework for food systems. J. Environ. Stud. Sci. 5, 474–484. doi: 10.1007/s13412-015-0280-6
Howard, P. L. (2010). “Culture and agrobiodiversity: understanding the links,” in Nature and Culture: Rebuilding Lost Connections, eds. S. Pilgrim and J. N. Pretty (London, UK: Routledge).
Intergovernmental Panel on Climate Change (2014). “Climate change: Impacts, adaptation, and vulnerability,” in 5th Assessment of the Intergovernmental Panel on Climate Change (Geneva, Switzerland: IPCC).
Jackson, L. E., Pascual, U., and Hodgkin, T. (2007). Utilizing and conserving agrobiodiversity in agricultural landscapes. Agric. Ecosyst. Environ. 121, 196–210. doi: 10.1016/j.agee.2006.12.017
Jäger, M., van Loosen, I., and Giuliani, A. (2019). How Have Markets Affected the Governance of Agrobiodiversity? Cambridge, MA: MIT Press.
Kahane, R., Hodgkin, T., Jaenicke, H., Hoogendoorn, C., Hermann, M., Dyno Keatinge, J., et al. (2013). Agrobiodiversity for food security, health and income. Agron. Sustain. Dev. 33, 671–693. doi: 10.1007/s13593-013-0147-8
Kalil, A. C. (2020). Treating COVID-19—off-label drug use, compassionate use, and randomized clinical trials during pandemics. JAMA 323, 1897–1898. doi: 10.1001/jama.2020.4742
Kerr, W. A. (2020). The COVID-19 pandemic and agriculture—short and long run implications for international trade relations. Can. J. Agric. Econ. 68, 225–229. doi: 10.1111/cjag.12230
Kolodinsky, J., Sitaker, M., Chase, L., Smith, D., and Wang, W. (2020). Food systems disruptions. J. Agric. Food Syst. Commun. Dev. 9, 1–4. doi: 10.5304/jafscd.2020.093.013
Kuckartz, U. (2014). “Three basic methods of qualitative text analysis,” in Qualitative Text Analysis: A Guide to Methods, Practice and Using Software, ed. U. Kuckartz. (London, UK: SAGE), 65–121.
Laborde, D., Martin, W., Swinnen, J., and Vos, R. (2020). COVID-19 risks to global food security. Science 369, 500–502. doi: 10.1126/science.abc4765
Leech, N. L., and Onwuegbuzie, A. J. (2011). Beyond constant comparison qualitative data analysis: Using NVivo. School Psychol. Q. 26:70. doi: 10.1037/a0022711
Letourneau, D. K., Armbrecht, I., Rivera, B. S., Lerma, J. M., Carmona, E. J., Daza, M. C., et al. (2011). Does plant diversity benefit agroecosystems? A synthetic review. Ecol. Appl. 21, 9–21. doi: 10.1890/09-2026.1
Long, J. A., Lawrence, R. L., Miller, P. R., and Marshall, L. A. (2014). Changes in field-level cropping sequences: indicators of shifting agricultural practices. Agric. Ecosyst. Environ. 189, 11–20. doi: 10.1016/j.agee.2014.03.015
Low, S. A., Adalja, A., Beaulieu, E., Key, N., Martinez, S., Melton, A., et al. (2015). Trends in US Local and Regional Food Systems: A Report to Congress. Washington, DC: USDA. Available online at: https://www.ers.usda.gov/publications/pub-details/?pubid=42807 (accessed Feb 15, 2021).
Lowrey, N. (2020, Apri 17). Need for food assistance skyrocketing for South Dakota residents during pandemic. Argus Leader.
Lynch, D. J., Growen, A., and Reiley, L. (2020, May 11). Farmers' hopes for respite from Trump-era struggles fade amid pandemic. Washington Post.
Miller, P. R., Bekkerman, A., Jones, C. A., Burgess, M. H., Holmes, J. A., and Engel, R. E. (2015). Pea in rotation with wheat reduced uncertainty of economic returns in southwest Montana. Agron. J. 107, 541–550. doi: 10.2134/agronj14.0185
Montana Food Bank Network (2020). Montana Food Bank Network's Response to COVID-19. Missoula, MT. Available online at: https://mfbn.org/2020/08/montana-food-bank-networks-response-tocovid-19/ (accessed December 1 2020).
Motley, T. J., Zerega, N., and Cross, H. (2006). Darwin's harvest: new approaches to the origins, evolution, and conservation of crops. New York, NY: Columbia University Press.
Murgueitio, E., Calle, Z., Uribe, F., Calle, A., and Solorio, B. (2011). Native trees and shrubs for the productive rehabilitation of tropical cattle ranching lands. For. Ecol. Manag. 261, 1654–1663. doi: 10.1016/j.foreco.2010.09.027
Naja, F., and Hamadeh, R. (2020). Nutrition amid the COVID-19 pandemic: a multi-level framework for action. Eur. J. Clin. Nutr. 74, 1117–1121. doi: 10.1038/s41430-020-0634-3
Nicola, M., Alsafi, Z., Sohrabi, C., Kerwan, A., Al-Jabir, A., Iosifidis, C., et al. (2020). The socio-economic implications of the coronavirus pandemic (COVID-19): a review. Int. J. Surg. 78, 185–193. doi: 10.1016/j.ijsu.2020.04.018
Nicosia, J. (2005). Avian flu: the consumer costs of preparing for global pandemic. Loy. Consumer L. Rev. 18, 479.
Oppenheimer, A. J., Pannucci, C. J., Kasten, S. J., and Haase, S. C. (2011). Survey says? A primer on web-based survey design and distribution. Plastic Reconstruct. Surg. 128, 299. doi: 10.1097/PRS.0b013e3182174413
Orden, D. (2020). Resilience test of the North American food system. Can. J. Agric. Econ. 2020, 1–3. doi: 10.1111/cjag.12238
Padbury, G., Waltman, S., Caprio, J., Coen, G., McGinn, S., Mortensen, D., et al. (2002). Agroecosystems and land resources of the northern Great Plains. Agron. J. 94, 251–261. doi: 10.2134/agronj2002.0251
Petersen-Rockney, M., Baur, P., Guzman, A., Bender, S. F., Calo, A., Castillo, F., et al. (2021b). Narrow and brittle or broad and nimble? Comparing adaptive capacity in simplifying and diversifying farming systems. Front. Sustain. Food Syst. 5, 56. doi: 10.3389/fsufs.2021.564900
Petersen-Rockney, M., Bowles, T., Ahmed, S., and Baur, P. (2021a). Editorial: diversifying farming systems for adaptive capacity. Front. Sustain. Food Syst. 5, 494. doi: 10.3389/fsufs.2021.806604
Philpott, S. M., Lin, B. B., Jha, S., and Brines, S. J. (2008). A multi-scale assessment of hurricane impacts on agricultural landscapes based on land use and topographic features. Agric. Ecosyst. Environ. 128, 12–20. doi: 10.1016/j.agee.2008.04.016
Pongsiri, M. J., Roman, J., Ezenwa, V. O., Goldberg, T. L., Koren, H. S., Newbold, S. C., et al. (2009). Biodiversity loss affects global disease ecology. Bioscience 59, 945–954. doi: 10.1525/bio.2009.59.11.6
Reissman, D. B., Watson, P. J., Klomp, R. W., Tanielian, T. L., and Prior, S. D. (2006). Pandemic influenza preparedness: adaptive responses to an evolving challenge. J. Homeland Secur. Emerg. Manag. 3, 13. doi: 10.2202/1547-7355.1233
Renkow, M., and Byerlee, D. (2010). The impacts of CGIAR research: a review of recent evidence. Food Policy 35, 391–402. doi: 10.1016/j.foodpol.2010.04.006
Richards, T. J., and Rickard, B. (2020). COVID-19 impact on fruit and vegetable markets. Can. J. Agric. Econ. 68, 189–194. doi: 10.1111/cjag.12231
Rohr, J. R., Barrett, C. B., Civitello, D. J., Craft, M. E., Delius, B., DeLeo, G. A., et al. (2019). Emerging human infectious diseases and the links to global food production. Nat. Sustain. 2, 445–456. doi: 10.1038/s41893-019-0293-3
Rosa-Schleich, J., Loos, J., Mußhoff, O., and Tscharntke, T. (2019). Ecological-economic trade-offs of diversified farming systems—a review. Ecol. Econ. 160, 251–263. doi: 10.1016/j.ecolecon.2019.03.002
Rosset, P. M., Machín Sosa, B., Roque Jaime, A. M., and Ávila Lozano, D. R. (2011). The Campesino-to-Campesino agroecology movement of ANAP in Cuba: social process methodology in the construction of sustainable peasant agriculture and food sovereignty. J. Peasant Stud. 38, 161–191. doi: 10.1080/03066150.2010.538584
Rotz, S., and Fraser, E. D. (2015). Resilience and the industrial food system: analyzing the impacts of agricultural industrialization on food system vulnerability. J. Environ. Stud. Sci. 5, 459–473. doi: 10.1007/s13412-015-0277-1
Santos-Díaz, S., Hensiek, S., Owings, T., and Towns, M. H. (2019). Survey of undergraduate students' goals and achievement strategies for laboratory coursework. J. Chem. Educ. 96, 850–856. doi: 10.1021/acs.jchemed.8b00984
Saqr, M., and Wasson, B. (2020). COVID-19: lost opportunities and lessons for the future. Int. J. Health Sci. 14, 4.
Sayre, N. F., Carlisle, L., Huntsinger, L., Fisher, G., and Shattuck, A. (2012). The role of rangelands in diversified farming systems: innovations, obstacles, and opportunities in the USA. Ecol. Soc. 17. doi: 10.5751/ES-04790-170443
Shahed, K. S., Azeem, A., Ali, S. M., and Moktadir, M. A. (2021). A supply chain disruption risk mitigation model to manage COVID-19 pandemic risk. Environ. Sci. Pollut. Res. 2021, 1–16. doi: 10.1007/s11356-020-12289-4
Siche, R. (2020). What is the impact of COVID-19 disease on agriculture? Sci. Agropec. 11, 3–6. doi: 10.17268/sci.agropecu.2020.01.00
South Dakota Department of Health (2020). Novel Coronavirus (COVID-19) Updates. State of South Dakota. Available online at: https://doh.sd.gov/news/coronavirus.aspx (accessed Sep 11, 2020).
State of Montana (2020a). Directive Requiring Face Coverings in Certain Indoor Spaces and Outdoor Gatherings to Prevent the Spread of COVID-19. Helena, MT: State of Montana. Available online at: https://dphhs.mt.gov/aboutus/news/2020/directiverequiringfacecoverings (accessed Sep 10, 2020).
State of Montana (2020b). Executive order Amending Executive Order 2-2020 and Providing that the State of Emergency Runs Concurrent with the President's Emergency Declaration. Helena, MT: State of Montana.
State of Montana (2020c). Executive Order Declaring a State of Emergency to Exist within the State of Montana Related to the Communicable Disease COVID-19 Novel Coronavirus. Helena, MT: State of Montana.
Stephens, E. C., Martin, G., van Wijk, M., Timsina, J., and Snow, V. (2020). Impacts of COVID-19 on agricultural and food systems worldwide and on progress to the sustainable development goals. Agric. Syst. 183, 102873. doi: 10.1016/j.agsy.2020.102873
Stoy, P. C., Ahmed, S., Jarchow, M., Rashford, B., Swanson, D., Albeke, S., et al. (2018). Opportunities and Trade-offs among BECCS and the Food, Water, Energy, Biodiversity, and Social Systems Nexus at Regional Scales. BioScience 68, 100–111. doi: 10.1093/biosci/bix145
Swinnen, J., and McDermott, J. (2020). COVID-19 and global food security. EuroChoices 19, 26–33. doi: 10.1111/1746-692X.12288
Taqi, H. M., Ahmed, H. N., Paul, S., Garshasbi, M., Ali, S. M., Kabir, G., et al. (2020). Strategies to manage the impacts of the COVID-19 pandemic in the supply chain: implications for improving economic and social sustainability. Sustainability 12, 9483. doi: 10.3390/su12229483
Tendall, D., Joerin, J., Kopainsky, B., Edwards, P., Shreck, A., Le, Q. B., et al. (2015). Food system resilience: defining the concept. Global Food Security 6, 17–23. doi: 10.1016/j.gfs.2015.08.001
Thornton, P. K., Loboguerrero Rodriguez, A. M., Campbell, B. M., Mercado, L., Shackleton, S., and Kavikumar, K. (2019). Rural Livelihoods, Food Security and Rural Transformation Under Climate Change. Rotterdam, Netherlands and Washington DC: Global Commission on Adaptation.
Torales, J., O'Higgins, M., Castaldelli-Maia, J. M., and Ventriglio, A. (2020). The outbreak of COVID-19 coronavirus and its impact on global mental health. Int. J. Social Psychiatry 66, 317–320. doi: 10.1177/0020764020915212
Torero, M. (2020). Without food, there can be no exit from the pandemic. Nature 580, 588. doi: 10.1038/d41586-020-01181-3
UC Berkley (2020). What Are Diversified Farming Systems?. Berkley, CA: UC Berkley. Available online at: https://food.berkeley.edu/about-us/centers/dfs/what-are-diversified-farming-systems/ (accessed Aug 28, 2020).
Upton, G. J. (1992). Fisher's exact test. J. R. Stat. Soc. Ser. A 155, 395–402. doi: 10.2307/2982890
US Department of Agriculture (2018). Wyoming Agricultural Facts. Washington, DC: USDA. Available online at: https://www.nass.usda.gov/Statistics_by_State/Wyoming/Publications/Brochures/Wyoming%20AG%20Profile%20Brochure.pdf (accessed May, 15 2020).
US Department of Agriculture (2019). USDA Economic Research Service State Fact Sheets. Washington, DC: USDA. Available online at: https://www.ers.usda.gov/data-products.aspx (accessed Dec 16, 2019).
US Department of Agriculture (2020). Farms, Land, and Value of Sales on Certified Organic Farms: 2019. Washington, DC: Census of Agriculture.
Vaske, J. J., Beaman, J., and Sponarski, C. C. (2017). Rethinking internal consistency in Cronbach's alpha. Leisure Sci. 39, 163–173. doi: 10.1080/01490400.2015.1127189
Walker, B., and Salt, D. (2012). Resilience practice: Building capacity to absorb disturbance and maintain function. Washington, DC: Island Press.
Warne, T., Ahmed, S., Byker Shanks, C., and Miller, P. (2019). Sustainability dimensions of a North American lentil system in a changing world. Front. Sustain. Food Syst. 3, 88. doi: 10.3389/fsufs.2019.00088
Westervelt, E. (2020). As Food Supply Chain Breaks Down, Farm-To-Door CSAs Take Off. Washington, DC: NPR. Available online at: https://www.npr.org/2020/05/10/852512047/as-food-supply-chain-breaks-down-farm-to-door-csas-take-off (accessed Oct 13, 2020).
Wolfson, J. A., and Leung, C. W. (2020). Food Insecurity and COVID-19: disparities in early effects for US adults. Nutrients 12, 1648. doi: 10.3390/nu12061648
Xiong, J., Lipsitz, O., Nasri, F., Lui, L. M., Gill, H., Phan, L., et al. (2020). Impact of COVID-19 pandemic on mental health in the general population: a systematic review. J. Affective disorders. doi: 10.1016/j.jad.2020.08.001
Youngberg, G., and DeMuth, S. P. (2013). Organic agriculture in the United States: A 30-year retrospective. Renew. Agric. Food Syst. 28, 294–328. doi: 10.1017/S1742170513000173
Yousafzai, A. K., Rasheed, M. A., and Bhutta, Z. A. (2013). Annual research review: improved nutrition–a pathway to resilience. J. Child Psychol. Psychiatry 54, 367–377. doi: 10.1111/jcpp.12019
Zimmerer, K. S., de Haan, S., Jones, A. D., Creed-Kanashiro, H., Tello, M., Carrasco, M., et al. (2019). The biodiversity of food and agriculture (Agrobiodiversity) in the anthropocene: Research advances and conceptual framework. Anthropocene 25, 100192. doi: 10.1016/j.ancene.2019.100192
Keywords: food system shock, agrobiodiversity, food security, organic farming, rural livelihood, resilience assessment
Citation: Ebel R, Ahmed S, Warne T, Moxley A, Grimberg I, Jarchow M and Menalled FD (2022) Perceptions and Responses of Diversified Farm Producers in the Northern Great Plains to the Early Stage of the COVID-19 Pandemic. Front. Sustain. Food Syst. 6:668335. doi: 10.3389/fsufs.2022.668335
Received: 16 February 2021; Accepted: 11 January 2022;
Published: 03 February 2022.
Edited by:
Ivette Perfecto, University of Michigan, United StatesReviewed by:
Karl S. Zimmerer, The Pennsylvania State University (PSU), United StatesCopyright © 2022 Ebel, Ahmed, Warne, Moxley, Grimberg, Jarchow and Menalled. This is an open-access article distributed under the terms of the Creative Commons Attribution License (CC BY). The use, distribution or reproduction in other forums is permitted, provided the original author(s) and the copyright owner(s) are credited and that the original publication in this journal is cited, in accordance with accepted academic practice. No use, distribution or reproduction is permitted which does not comply with these terms.
*Correspondence: Selena Ahmed, c2VsZW5hLmFobWVkQG1vbnRhbmEuZWR1
Disclaimer: All claims expressed in this article are solely those of the authors and do not necessarily represent those of their affiliated organizations, or those of the publisher, the editors and the reviewers. Any product that may be evaluated in this article or claim that may be made by its manufacturer is not guaranteed or endorsed by the publisher.
Research integrity at Frontiers
Learn more about the work of our research integrity team to safeguard the quality of each article we publish.