- CSIR-Crops Research Institute, Kumasi, Ghana
Complex controls and non-linear responses of the climate system to global warming make it difficult to have clear-cut predictions of future precipitation amounts and timelines. It is, however, evident from current observations that some predictions of unusually high rates of flooding and droughts are occurring and threatening food security in sub-Saharan Africa (SSA). The impact of climate change is immense on SSA though it contributes the least to climate change globally. Crops face lots of growth challenges which reduce their productivity under drought and flood conditions. SSA must prepare agricultural soils for the anticipated climate variabilities, to ensure sustainable food availability. The effort to adapt soils to climate change must be a concerted one, using technologies from various facets of science. Stakeholders must adopt water-smart strategies that maintain proper soil-water balance. They should focus on manageable inherent soil properties that control the susceptibility/adaptability of cropping systems to climate change. Conservation agriculture techniques that target improving soil organic matter and maintaining soil life; protecting the soil from compaction and erosion; reducing soil disturbance; enhancing soil infiltration and groundwater recharge capacity, must be applied to our soils. A number of these techniques equip the soils to be better sinks of excess water in flood-prone areas and improve water-holding capacities in drought-prone ones. Governments, farmers, and all stakeholders must also invest in both simple and complex water harvesting/ re-directing infrastructure which conserve water for future use. Water-efficient irrigation systems must be employed by farmers during water scarcity. Most importantly, gaps between research, industry, farmers, and governments must be bridged to for easy flow of information on improved technologies and quick adoption of climate change mitigation strategies.
1. Introduction
Climate change refers to any change in the climate over time, due to natural variability and/or human activity (IPCC, 2007). Such changes in the climate are identified by changes in the mean and/or variability of climatic properties which persists typically over decades or longer (IPCC, 2007). Global warming is the long-term heating of the earth's surface observed since pre-industrial period (1850 to 1900) primarily due to fossil fuel burning and human activities (NASA-GISTEMP, 2022). The activities increase concentrations of greenhouse gases that trap re-radiated heat energy in the earth's atmosphere (NASA-GISTEMP, 2022). Global warming and climate change cause unexpected water stresses (floods and droughts) that limit agricultural productivity (Surendra and Awais, 2019; Bai et al., 2022). To meet global food demand in the face of climate change, the soil must be managed to be productive even under these unexpected conditions. Proactive risk-based soil conservation measures should be employed to enhance the resilience and capacity of the soil to droughts or floods. Usually, such management approaches are often overlooked until a crisis unfolds. Consequently, reactive responses give rise to implementation challenges where interventions are isolated, and the mitigation strategies underperform (FAO, 2021). A holistic approach that targets soil and water conservation, including improving soil water holding, water budgeting, development of water harvesting structures and groundwater recharge and promotion of micro-irrigation, must be integrated into our agricultural systems (Bhattacharyya et al., 2020). Awareness must be created to enlighten policy and decision-makers about the urgency with which these management approaches must be adopted, especially in Ghana and sub-Saharan Africa, because these regions of the world are / will be severely hit by climate change, even though they contribute the least to it (United Nations, 2019; IPCC, 2022). Figure 1 shows areas at risk of climate change on the African continent, which calls for immediate interventions. Though predictions in this map were made in 2007, they hold true to time, particularly for Ghana. Increased frequencies of unexpected droughts in the northern parts of the country (Jarawura, 2014; Adonadaga et al., 2022) and floods in the South (Tengan and Aigbavboa, 2016; Ansah et al., 2020) are shreds of evidence of the predictions in the map. If soil water resources are not used sustainably, sub-Saharan Africa stands the risk of intensified food insecurity, poor economic growth and poverty. In 2022, about 12% of the population (123 million people) of sub-Saharan Africa are reported to be acutely food insecure and malnourished (IMF, 2022). The lack of resilience to climate change, global cereal shortages and fuel price hikes due to the war in Ukraine and post COVID 19 challenges continue to exacerbate food insecurities in SSA. Currently, Ethiopia and Kenya are suffering some of the most severe droughts in recent history and food insecurity in these countries increase by 5–20% points at every drought event (IMF, 2022). In the recent past, climate change has decreased diary yields by 10–25% in South Africa (FAO, 2015). The future looks bleak for SSA, with severe climatic events worsening food shortages in given countries or import sourcing countries; and surging severe consequences for economies (IMF, 2022). There is an urgent need to build the resilience of our agricultural systems to climate change while various stakeholders take charge to strengthen the crumbling economies. In light of the afore-mentioned challenges, the objective of this review is to highlight several soil and water conservation measures that adapt cropping systems to climate change. These measures have yielded crop productivity benefits in some parts of Africa and elsewhere. To better understand the effects of climate change on agriculture and mechanisms behind the measures, the review further assesses crop growth challenges under flood and drought conditions, and factors that influence the susceptibility of cropping systems to water stresses. Soil and water conservation measures outlined in the review are necessary interventions to mitigate climate change and make SSA food secure. The review also emphasizes on feasible water harvesting strategies and water-efficient irrigation methods that use conserved water resources judiciously. It also provides solutions to bridge the disconnection between researchers, governments and farmers for easy flow of climate change adaptation information and quick adoption of improved strategies.
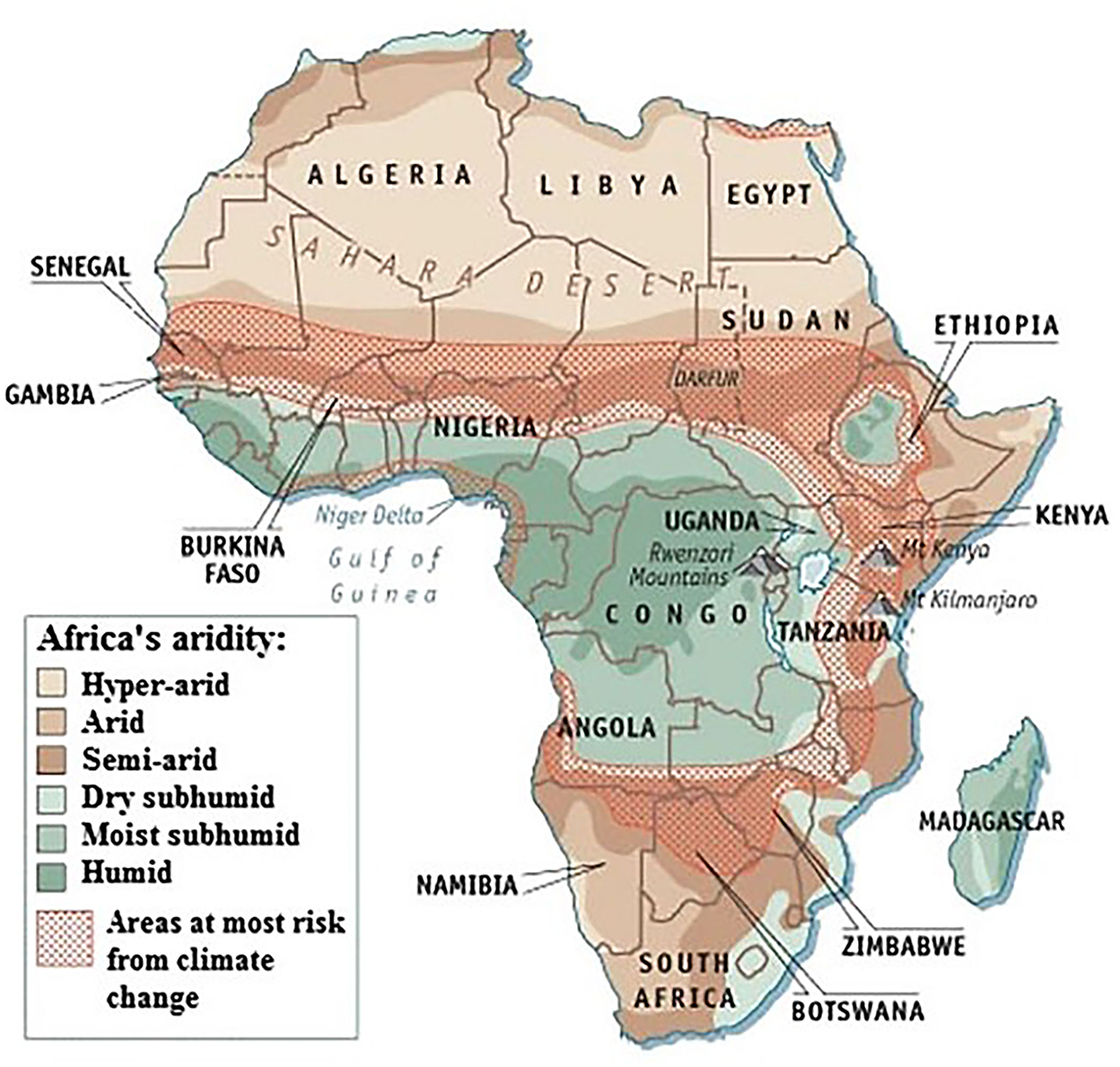
Figure 1. Map of Africa showing countries at risk of climate change facilitated water stresses. Source: http://kenvironews.wordpress.com/2007/08/31/africa-prepares-for-impact-of-climate-change.
2. Review methodology
We used the recently updated PRISMA guidelines (Page et al., 2021) adopted by Kleppel and Frank (2022) as framework for this review. According to the guidelines, we searched and compiled research and review articles describing the effect of climate-change facilitated water stresses on crop growth, yield and food security; and of soil and water conservation measures implemented to mitigate the water stresses.
Literature was searched in peer-reviewed articles, other journals, books and trusted websites with aims, scopes and focuses on soil conservation, soil fertility, agronomy, crop physiology, climate-smart cropping systems, agricultural mechanization, engineering water harvesting infrastructure and efficient irrigation in agriculture. In our search, we accessed original research results published by researchers to demonstrate the effect of precipitation anomalies (unexpected droughts and floods) on crop growth and the coping mechanisms adopted by plants to tolerate such anomalies, which consequently reduces yield. We also accessed research results authored by researchers for professional consumption, on the effect of soil conservation and efficient use of conserved water on crop productivity. Additionally, information in extension service factsheets and international reports of the Food and Agriculture Organization (FAO), United States Department of Agriculture (USDA), United Nations, Intergovernmental Panel on Climate Change (IPCC) and local government agencies among others were used. We ensured that literature that were not necessarily peer-reviewed, but intended for only farmers and the general public were authored by writers who were knowledgeable in their disciplines, and that their information were technically correct according to existing scientific literature. In total, this review is based on 78 published sources.
Conventional search engines and research databases such as google, google scholar, researchgate and web of science were used to search for literature. Search terms like drought OR flood; crop yield AND drought; crop yield AND flood; climate change AND soil conservation; soil conservation measures pdf, drought AND water harvesting infrastructure; flood AND water re-direction infrastructure, among others were keyed in to search for literature. We also accessed our personal libraries and the CSIR-Crops Research Institute library repository for books related to the review topic.
3. Crop growth challenges under flood and drought scenarios
Under flood conditions, agricultural soils become water-logged, accompanied by associated anaerobic conditions prevailing in the soil system. Poor soil aeration affects respiration processes of plant roots and soil microbes, leading to reduced root elongation, reduced mineralization rates, water, and nutrient uptake, carbon dioxide accumulation, reduced plant hormone synthesis, and many other negative effects (Li et al., 2019a). Under such conditions, water and nutrient transport through the plants are affected (Abuarab et al., 2019); crop growth declines are accompanied by yield reductions or total crop failures. Anaerobic conditions slow down organic matter decomposition and mineralization rates due to reduced soil redox potential (Dorau et al., 2018) and microbial activity (Abuarab et al., 2019). Flooded soils are prone to nutrient leaching beyond the root zone (Engelstad et al., 1985). Flooding reduces soil temperature below the optimum range (25–35°C), causing a reduction in nitrification rates and making less available to plants (Havlin et al., 2014). Flooding increases the pH of acid soils by reducing Fe3+ to Fe2+ and decreases the pH of alkaline soils by facilitating the formation of H2CO3 compounds due to CO2 accumulation. As a result, native acid/alkaline tolerant crops growing in such areas begin to fail. Anaerobic soil conditions favor methanogenesis and denitrification processes leading to increased greenhouse gas (CH4 and N2O) emissions (Schlesinger and Bernhardt, 2013), which are major pathways for carbon and nitrogen losses from the soil and soil organic matter degradation. Crop nutrient uptake and utilization are thus affected. Flooding promotes soil compaction which further destroys the soil structure through the breakdown of aggregates, deflocculation of clay, and destruction of cementing agents (Rodriguez et al., 2016). Flooding in coastal areas results in seawater intrusion into arable lands, creates high water tables and increases the salinity of soils (Mahmoodzadeh and Karamouz, 2017) which inhibits nitrogen uptake by crops. The increased chloride concentration in the soil (from the salts) also tends to poison many plants to death. This may give an advantage to salt-tolerant weeds which further undermine target crop yields. Flooding also causes increased runoff velocities and erosion which carry soil nutrients off-site. It makes farm operations with heavy machinery difficult (Adesina, 2018). Previous studies have estimated the effects of floods on agricultural production in real-time. Cape gooseberry plants submerged in floods for more than 0, 2, 4, 6, and 8 days showed symptoms of chlorosis, epinasty, necrosis, and abscission of leaves due to oxygen deprivation in root zones and reduced growth rate and dry matter accumulation after 4 days (Table 1; Aldana et al., 2014). Flooding banana plants for 1, 3, 5, 7, 14, and 24 days induced morphological, molecular, and biochemical changes in their roots which reduced growth and productivity (Teoh et al., 2022). In this study, anaerobic conditions created by flooding redirected the use of photosynthates and energy for the excessive development of adventitious roots and aerenchyma with lots of gas spaces (Figure 2). It also led to the induction of stress adaptation mechanisms such as ethylene production, basic helix-loop-helix, myeloblastosis, plant signal transduction, and carbohydrate metabolism.
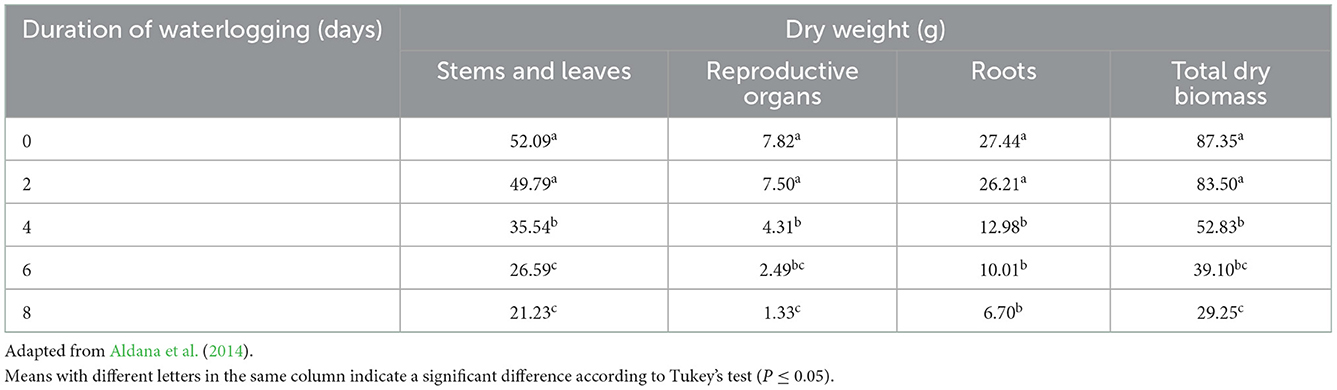
Table 1. Dry weight of stems and leaves, reproductive organs (floral buds, flowers, and fruits), and roots of cape gooseberry (Physalis peruviana L.) subjected at an age of 2 months to waterlogging of different durations at 50 days after waterlogging started.
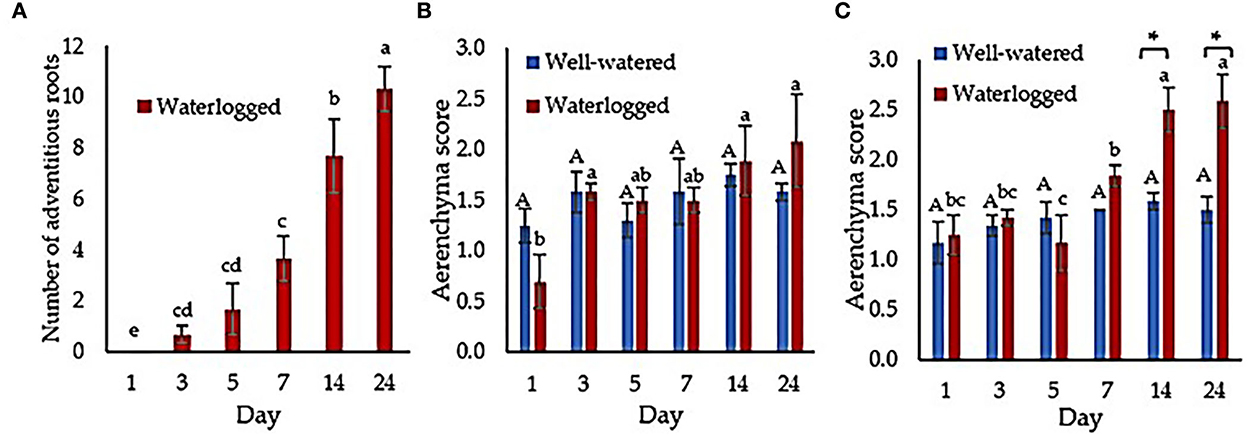
Figure 2. Adventitious root and aerenchyma formation. (A) The number of adventitious roots in well-watered and waterlogged banana plants. The mean aerenchyma score of (B) 5 cm from the root tip and (C) 5 cm from the root base. The results represent the mean ± standard error of the mean of nine independent biological replicates with three technical replicates each. Different capital letters indicate a significant difference between time points within well-watered samples. Different lowercase letters indicate a significant difference between time points within waterlogged samples. Asterisk (*) indicates a significant difference between well-watered and waterlogged samples at the same time point (p < 0.05). Adapted from Teoh et al. (2022).
Many agroecosystem models are unable to effectively predict the depressing effect of excessive rainfall and flooding of cropping systems on crop yields. On the contrary, they often overestimate yield under flood scenarios (Li et al., 2019b). It is therefore important to estimate real-time flooding effects to find solutions to potential climate change-induced floods. Li et al. (2019b) observed up to 34% reduction in maize yield compared to up to 37% reduction in yield under extreme wet and extreme dry conditions, respectively, in different places in the United States, though models predicted increased yield under flood scenarios (Figure 3). This implies equal degrees of devastation of both climate change extremes on cropping systems.
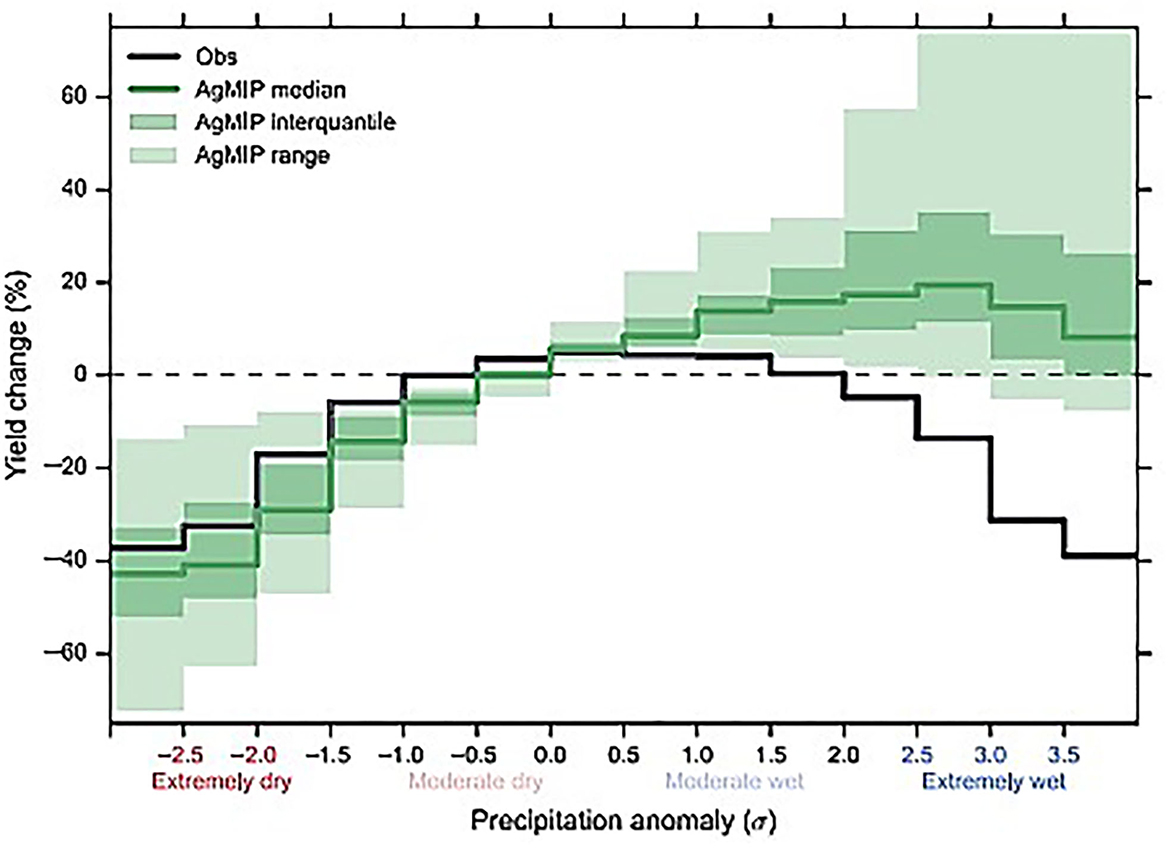
Figure 3. Maize yield response to precipitation anomaly from simulations of 12 global crop models participated in the AgMIP (green solid line and shaded area) compared with the observed response (black solid line). The green solid line shows the multi-model median response and the shaded area shows the model's interquartile range. The AgMIP global crop model data are from the historical simulations of 1981 to 2010 without irrigation, driven by AgMERRA with the default setting. Adapted from Li et al. (2019b).
Under the scenario where global warming leads to drought, there could be massive soil degradation and famine (FAO, 2021). Seasonal or continuous droughts impact agroecosystem carbon and nitrogen cycles. Drought conditions accompanied by increased temperatures reduce the communities of soil bacteria and microbiota functions beneficial to organic matter decomposition. This may result in up to a 93% decline in the rate of organic matter decomposition (Toth et al., 2017). In their study, fungi were found to be less sensitive to drought and increased temperatures. However, whether the anticipated drought conditions due to climate change would increase beneficial fungi communities perpetually or not is not known. Water is the main soil solvent by which nutrients are supplied to both microbes and plants; hence a lack of it limits nutrient availability to these entities (Bista et al., 2017). Under drought conditions, little to no crop responses to farm inputs like fertilizer are observed because water limitation makes nutrients less available to crops. Drought dries the soil and increases its susceptibility to wind erosion, leading to loss of soil and soil nutrients. Plants are less productive in areas subjected to prolonged droughts. Drought reduces the rate of photosynthesis and enhances autotrophic respiration in crop plants leading to cavitation and/or carbon starvation in plants and consequent death of the plants (Chandregowda et al., 2022). The higher predicted temperatures associated with droughts would also place high transpiration demands on crops leading to their wilt and eventual death if the conditions persist. Severe drought (≤30% field capacity) was reported to reduce wheat dry biomass by 50% even with adequate fertilization (Kulczycki et al., 2022). Thus drought poses lots of economic losses to farmers and especially those in sub-Saharan Africa who are financially constrained (Gilbert, 2020; Lottering et al., 2020). Different crops are sensitive to droughts occurring at different stages of growth. Drought at the seedling stage of maize growth leaves perpetual devastating effects that transcend jointing, heading, and grain-filling stages (Song et al., 2019). Song et al. (2019) found that drought imposed on seedlings of maize led to lower leaf area, reduced leaf chlorophyll concentration, and lower unit kernel weight due to reduced intercepted photosynthetically active radiation (IPAR) and radiation-use efficiency (RUE) that occurred throughout the maize growing season, compared to drought imposition at jointing, heading and grain filling stages (Table 2).
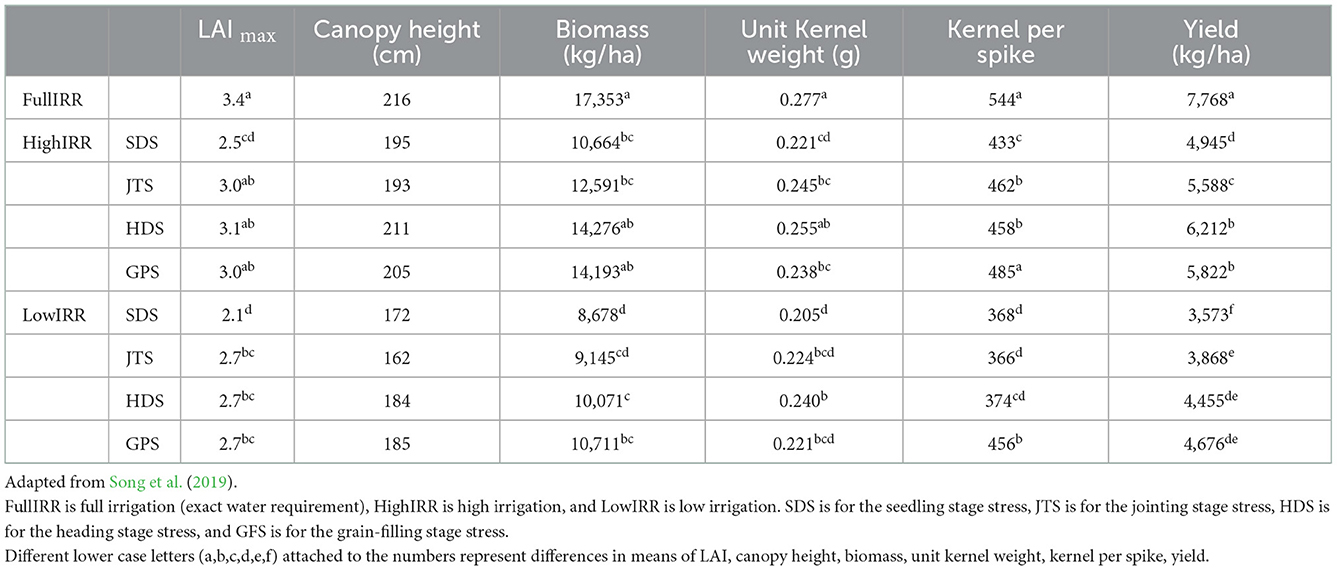
Table 2. Variables of maize growth and yield under water stress (HighIRR and LowIRR) during different maize growth stages and FullIRR during the maize growing season from 2013 to 2016.
Brempong et al. (2022) also observed very poor common bean yield of 15 to 100 kg/ha, compared to the mean 437 kg/ha yield commonly obtained in sub-Saharan Africa, when water stress of 16 and 40% field capacity (FC) and sufficient water at 80% field capacity were applied at first flower stage, even though soil nutrients were not limiting (Figure 4).
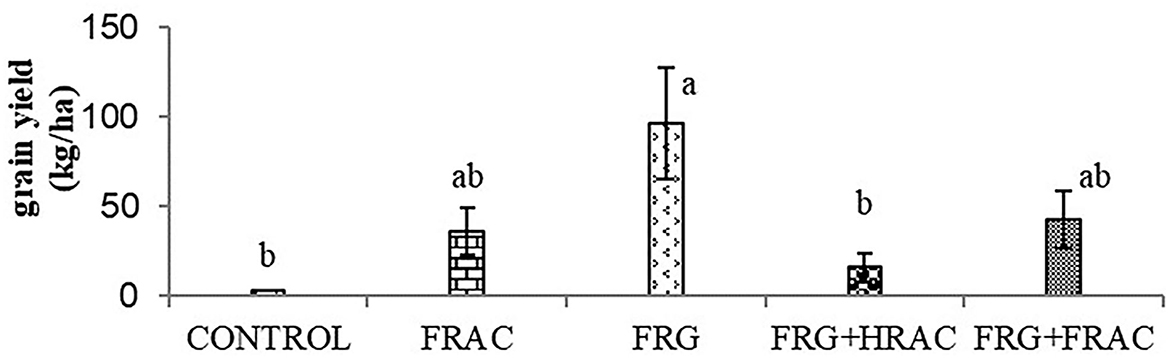
Figure 4. Mean grain yield (kg/ha) affected by fertilizer treatments across 16, 40, and 80 % field capacities moisture. Control is no amendment; FRAC is full-rate compost; FRG is full-rate glycine mix fertilizer; HRAC is half-rate compost. Error bars represent standard errors of the fertilizer treatment means. Different lowercase letters on top of the bars represent significant differences at least significant difference of 5% probability. Adapted from Brempong et al. (2022).
The standardized precipitation evapotranspiration index (SPEI), the standardized precipitation index (SPI), the palmer drought severity index (PDSI), palmer Z index, (Z index SDI), the palmer hydrological drought index (PHDI), the palmer modified drought index (PMDI), and the standardized palmer drought index (SPDI) are drought indices used to estimate the effect of drought on crops yields (Pena-Gallardo et al., 2019). In a study, Pena-Gallardo et al. (2019), using the above indices, found that the magnitude of the effect of drought on wheat and barley yield depended on the region, period of the year, and the drought timescale and magnitude. Since these factors are difficult to precisely predict, soils and cropping systems must be adequately prepared for climate change. Aside from crop growth challenges, animals may suffer higher disease and pest incidence in flooded areas and feed shortages in drought areas.
4. Factors influencing susceptibility of soils to floods and droughts
Soil infiltration and permeability are major factors influencing the soil-water balance (Veerapan and Sayed, 2020) and the susceptibility of the soil to water stresses. Those factors are in turn affected by soil characteristics like texture, structure, porosity, and organic matter content. Flood-prone soils are often characterized by heavy clay texture; poor drainage and poor organic matter content (Adesina, 2018). These characteristics cause a reduction in the soil's pore spaces, biological activity, and the soil's ability to contain fluids (water and air). Such soils are highly susceptible to compaction and erosion which further increase flood cycle frequency. Soil compaction often results from soil compression by heavy machinery (frequent tillage especially on wet soil), humans, animals (uncontrolled grazing), and vehicular traffic. Deforestation and land clearing uncovers the soil and increases the erodibility of the soil, especially on steep slopes. Clearing the forest on steep-sloped lands can increase soil erosion by 5 to 20 times that of flat terrains (Khodadali et al., 2021). Soil compaction increases soil bulk density while decreasing porosity (Chakraborty and Mistri, 2017). Compaction also creates a physical resistance to soil water retention and root growth. Water erosion is another culprit which sediments streams and rivers, causing them to overflow their banks, clog waterways and fill soil pores, increasing the susceptibility of land to flooding.
Plant available water holding capacity (PAWHC) of soil determines its vulnerability to droughts (Shao et al., 2018). PAWHC is the difference in soil water content between field capacity and permanent wilting point. Soil factors that affect the soil-water balance also affect soil erodibility. Soil erodibility depends on the particle size distribution and their interaction with organic matter. Sandy soils with less organic matter have weak aggregate stability and therefore erode more than fine-textured soils in high-intensity rains (Dumbrovsky et al., 2019). However, sandy soils have larger macropores which allow them higher permeability than fine-textured soils. Hence in less intensive rains, sandy soils are slower to erode than fine-textured soils because their infiltration rate is much faster and runoff generation is slower. Very dry soils are also susceptible to wind erosion. This implies that the soil should have the right particle size distribution, structure and organic matter content to balance permeability and erodibility.
5. Soil and water conservation measures that maintain productive soil-water balance in the face of water stresses
From the above scenarios, maintaining a good soil-water balance in the face of climate change-facilitated water stress (floods and droughts) demands improvements in soil permeability, texture, structure, porosity, and organic matter content to reduce the soil's susceptibility to compaction and erosion. Thus, the right soil conservation practices should be aimed at reducing soil compaction and erosion (water and wind) and improving general soil health. The practices should be aimed at improving the water-holding capacity of soil, promoting aggregate stability, and general improvement of soil health. Measures should also be put in place to conserve excess water received through precipitation and/or redirect excess water which may flood cropping systems. The flow chart in Figure 5 summarizes some must-do-practices that could prepare the soil for climate change-induced water stresses (Singh et al., 2016). In this chapter, soil and water conservation measures prescribed in the flow chart are discussed.
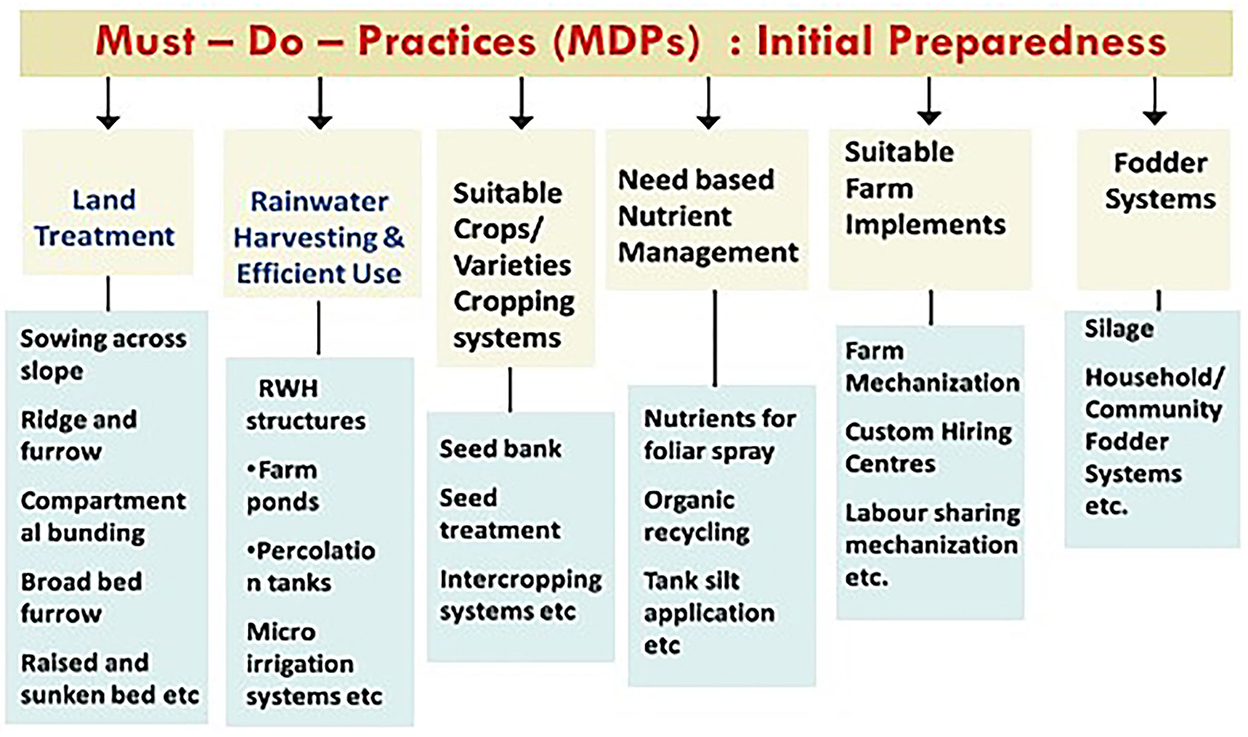
Figure 5. Must-do practices to prepare the soil and cropping systems for climate-induced water stresses. Adapted from Singh et al. (2016).
5.1. Land treatment
Soil conservation measures have been proposed in the flow chart under the “land treatment” section (Figure 5). Some simple land treatment or preparation methods prescribed under this section include planting across the slope to reduce runoff velocities and loss of nutrients due to erosion; and making ridges and raised beds in wet areas and furrows and sunken beds in dry areas to maximize soil moisture. Common soil conservation measures that could also be adopted include, the addition of organic amendments, use of suitable farm implements, reduced tillage practices, contour farming, grassing waterways, crop rotation, strip cropping, cover cropping and agroforestry among others (Diop et al., 2022).
5.2. Use of suitable farm implements
Agricultural machinery which supports soil conservation performs minimum or zero tillage operations during preparation of favorable seedbed conditions for satisfactory crop establishment. Agricultural mechanization in the past included direct drilling machines that negatively impacted soil micro-environments. Today, agricultural machinery have been designed with controlled traffic measures for cost-effective and energy-saving seeding without tilling the soil unnecessarily. Such equipment include sub-soilers, no-till plant drill, zero-till drill, strip-till drill, happy seeder, roto-till drill, rotary slit no-till drill among others (Patel et al., 2018). Selecting the most appropriate machinery based on specific field conditions is important to soil and water conservation. Thus conditions causing a lack of crop productivity must be assessed and addressed accordingly. For example, no-till and zero-tillage practices may not have significant benefits on deep-rooted crops on land with compacted sub-soils (between 30 to 56 cm below the soil surface) (Becker et al., 2022). In this case, sub-soiling is required before the subsequent implementation of soil conservation measures to avoid future compaction. Before using sub-soilers, the soil should first be dug to about 76 cm when it is saturated, and then tested for compaction with a penetrometer (Weilli, 2015). Compacted sub-soil layers restrict water and root movement in the soil. A penetrometer test determines the depth of compaction to inform whether to use simple tillage (for surface compaction) or sub-soiler (for compaction beyond 30 cm depth). Sub-soiling is best done when the soil is dry. A sub-soiling expert should be contracted to do this. During the process, ground contours should be followed to help improve soil permeability and reduce erosion in susceptible areas. Sub-soiling compacted soils improves water retention and reduces soil susceptibility to floods and droughts. Additionally, heavy machinery should not be used on wet or flooded soils as it causes compaction, makes farm operations difficult, and wastes lots of energy. It is recommended that tillage operations are done when the soil is semi-dry. To determine if the soil is in working condition, simply pick a ball of soil and poke it. If it falls apart in your hand, the soil is dry enough to be tilled. Otherwise, it is too wet to be tilled. Also, sandy soils in drought-prone areas should not be tilled too frequently as they are already loose and do not pose compaction threats. These examples are to wit that appropriate farm operations may require the services of an expert or very experienced farmers if soil conservation is of interest. Images of some soil conservation equipment are shown in Figure 6.
5.3. Reduced tillage practices
Conservation agriculture is defined as minimal soil disturbance, diversified crop rotations, and surface residue retention that reduce soil and environmental degradation and sustain crop production. Reduced tillage practices are classified under conservation agricultural practices. Repetitive conventional tillage is a major cause of soil aggregate destruction and organic matter breakdown which leads to soil nutrient loss through greenhouse gas (CO2, CH4, and N2O) emissions (Bista et al., 2017). Reduced tillage practices reduce organic matter degradation. They decrease the over-reliance on frequent tillage and focus on other measures that prevent soil surface crusting and compaction. The practices include no-till, strip tillage, ridge tillage, mulch tillage, and shallow tillage among other variants (Bista et al., 2017). Images of reduced tillage practices are shown in Figure 7.
In no-till agriculture, the soil is left undisturbed by tillage. No-till is usually established after one or few tillage operations after which crop residues are left annually on the soil surface to protect the soil and add organic matter after decomposition. The bed of residue intercepts rainfall intensity, improves infiltrations, protects the soil from erosion and consequent nutrient loss, and increases soil organic matter content. In drought-prone soils, the soil cover reduces excessive evaporation from the soil surface. Some soil and crop yield benefits have been recorded with the no-till practice. After a 2 year experiment, Yang et al. (2020) found higher soil N and P levels of 4,757 and 2,429 kg/ha respectively in the top 20 cm soil layer under no-till, compared to 4726 kg N/ha and 2,412 kg P/ha under conventional tillage. No tillage also reduced the risk of obtaining wheat yields below 1,000 kg/ha and 1,500 kg/ha by 95 and 33.3%, respectively in dry areas of Syria (El-Shater et al., 2020).
In strip tillage, there is a minimum tillage disturbance only at the seed rows which allows improvement in water infiltration around the crop root zone. Strip tillage is best practiced in poorly drained soils that are susceptible to flooding. In ridge tillage, shallow raised beds or ridges are made on the soil on which crops are grown. Ridge tillage provides better drainage in wet soils and reduces the tendency for the soil to flood. In dry or drought-prone soils, rainwater is stored behind the ridges, improving the soil's water storage capacity. Ridge tillage increased mean soil water content by 20% compared to conventional tillage under semi-arid conditions in Ethiopia (Bekele, 2020).
Mulch tillage is a typical conventional tillage practice but leaves crop residues on the soil which is turned in before every growing season. It adds organic matter to the soil and reduces water and wind erosion. The organic matter addition allows the soil to hold more water in drought-prone areas. Shallow tillage is more like mulch tillage, except that the tillage is done at shallower depths with lighter instruments like knives, coulters, or small forward-facing discs. The residue left on the surface adds organic matter to the soil after decomposition, and improves its water-holding capacity in drought susceptible areas (Telkar et al., 2017).
Though reduced tillage practices have demonstrated many soil and crop benefits, there is still a knowledge gap on the minimum number of years they should be practiced before the next conventional tillage, so that sub-surface soil compaction does not become the next limiting factor to crop growth. Norton et al. (2016), observed increased maize yields in 4 years under strip tillage and residue cover in Kenya. However, they observed yield declines after the 4th year, due to sub-soil compaction; and recommended that conventional tillage be done at the end of every 4 years of practicing strip tillage to prevent compaction related yield declines. Similarly, Salem et al. (2020) found higher soil bulk density and higher soil penetration resistance in sub-soil layers under minimum tillage compared to reservoir tillage, which alludes to the fact that there should be a cut-off year when the soil is loosened again or at least, sub-soiled.
Grassed farm waterways with grass or a permanent vegetative cover also allows surface water to move across farmlands without causing significant soil erosion. It prevents the formation of gullies and carries runoff water from the farm without causing flooding. Grassed waterways are conducive for flood-prone areas because they reduce compaction, and improve infiltration and the water-holding capacity of the soil.
6. Cropping systems that enhance soil and water conservation
6.1. Contour farming
Contour farming is the practice of performing farm operations like tillage near or on the contours of the field and not up or down the hill. It is an effective conservation measure that reduces rill development when surface runoff impounds in small depressions (Gathagu et al., 2018). Thus, it minimizes erosion and stores rainwater behind ridges made on the contours. It is effective at conserving water in drought-prone areas.
6.2. Strip cropping
Strip cropping is a crop rotation system in which two or more crops are grown in alternating strips on the same field. The strips are wide enough that they can be managed independently but narrow enough that the strips interact. Legumes are often included in the alternating crops to improve soil nitrogen levels. The crops are closely planted in the strips such that runoff water is impeded and soil is filtered through the strips. In effect, the strips serve as natural dams for water storage (NWRM, 2015), allowing water to percolate slowly into the soil. It is highly effective in drought-prone areas to conserve water. Images of cropping systems are shown in Figure 8.
6.3. Crop rotation
Crop rotation is the practice of growing dissimilar crops in sequential seasons in the same space. The rotation traditionally includes a legume crop which serves as green manure, adding nitrogen and organic matter to the soil. Soil structure could be improved in crop rotation by alternating deep and shallow-rooted crops, which benefits the conservation of soil water in drought-prone areas. Rotating rice with winter crops (wheat, potato, green manure and oilseed rape) produced rice yields of 7.2 to 7.48 Mg/ha compared to 5.93 Mg/ha in mono-culture rice alone fields (Chen et al., 2018). Spring wheat yield was increased by up to 30% under no-till in wheat-turnip-pea rotations and 13% under conventional tillage, compared with mono-culture wheat (Jalli et al., 2021).
6.4. Cover cropping
A cover crop is any living ground cover planted to improve soil fertility, protect the soil from erosion, maintain water quality and help manage weeds and pests. They may act as green manures, improving soil organic matter, and live / residue mulches, conserving soil moisture or catch crops, and reducing nutrient leaching after harvesting the cash crop. After a 12-year study, cover crops were found to increase soil organic carbon (SOC) under no-till, chisel plow and mouldbaord plow practices, though no-till retained the highest SOC (Olson et al., 2014). Type of cover crops included in the cropping system is equally important. Legumes are usually recommended as they fix nitrogen and may serve as an extra source of income. A negative yield effect on the main cash crop may be observed if the right cover crop is not selected. A yield decline of 12.6% in maize was observed in Illinois when maize followed wheat cover crop in rotation compared to no cover crop (Adeyemi et al., 2020).
6.5. Agroforestry
Agroforestry is the intentional integration of trees and/or shrubs into crops and/or animal farming systems for their economic, environmental, and social benefits (USDA, 2021). In an agroforestry system, the leaves of the trees intercept rainwater, and the stems soak some of it and allow the water to trickle down slowly, improving the infiltration rate into the soil. This reduces runoff velocities and volumes which results in floods. The ability of the trees to also intercept some of the solar radiation reaching the soil and reduce soil evaporation is also important to mitigate drought. The roots of trees create macro-pores in the soil and improve the permeability and water-holding capacity of the soil. This allows the soil to hold more water and reduce flooding tendencies in flood-prone areas while it allows the soil to retain enough water in dry areas. Dead leaves and tree debris serve as mulch and a source of organic matter to the soil, also improving soil water holding. For instance, intensifying a maize cropping system with Faidherbia albida leguminous trees increased maize yield by 20% and in Southern Malawi, due to general soil improvement (Amadu et al., 2020).
7. Rainwater harvesting and efficient use
As described in Figure 5, rainwater harvesting and efficient use is a very prudent way of conserving water in high rainfall and flood-prone areas. They range from simple rainwater catchments such as collecting water in containers to complex engineered infrastructure. In a broader sense, the water distribution in such areas could be controlled by putting up water control structures that reduce runoff, waterlogging, and breaking steep slopes where water can pick up speed and cause erosion. Such infrastructure includes drop structures, flood bed stabilizers, water spreading weirs, dikes, sluices, river embankments, and drainage ditches among others. Drop structures transition runoff water between levels to reduce runoff velocity and associated soil displacement. Flood bed stabilizers prevent uncontrolled runoff. Water-spreading weirs reduce erosion and promote the inundation of valley floors and the deposition of organic matter and fine-textured soil. River embankments reduce seepage interflows and flooding of farmlands. Sluices re-direct farm floods to desired locations. Dikes are used to drain and retaining excess water for future use. Drainage ditches are created to channel away excess flood waters for reuse. Since flood-prone areas have high water tables, groundwater could be accessed through relatively shallow local hand-drilled wells or ground-lifting technologies during the dry seasons. This would extend the cropping season and create more room for water absorption by the soil during heavy rains. To help sustain food security and boost the productivity of the flood-prone areas, retained water in the catchment areas could be used for aquaculture and fisheries and waste from the fish would serve as fertilizer for crops. To realize the full potential of these approaches, the government and other stakeholders must be willing to invest in such infrastructure. In times of total crop failures, irrespective of the measures taken by farmers, some form of relief should be given by the government to support these farmers. The following are pictures of some water management structures for flood-prone areas. Images of flood-based infrastructure are shown in Figure 9.
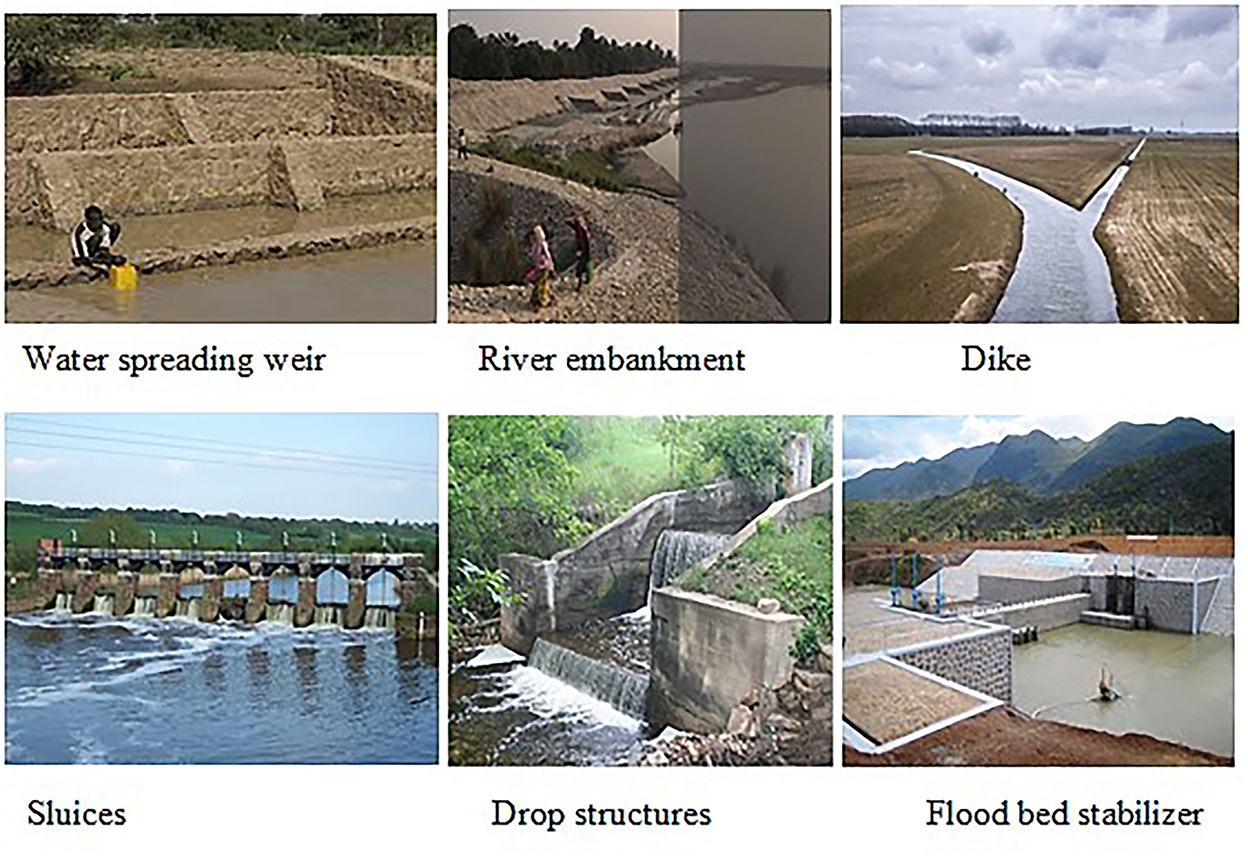
Figure 9. Water re-direction infrastructure for flood-prone areas. Source: https://wle.cgiar.org/solutions/six-ways-increase-productivity-flood-based-farming-systems.
Drought-prone areas also require judicious water use strategies which improve the water-holding capacity of the soil, improve the water use efficiency of crops, and conserve water in preparation for drought. Water harvesting/ catchment structures must be implemented in cropping systems. Structures like farm ponds can be created to store runoff water on the farm to augment groundwater; percolation tanks to recharge groundwater storage; roof water harvesting tanks to harvest rainwater; wells for artificial recharge through; subsurface dams to capture the base flow of groundwater; field soil bunds to slow down run-off and prevent water from moving out of the field; cemented irrigation channels to prevent seepage losses and leveling soil and creating of depressions on the soil surface to retain soil moisture and reduce runoff (Kumawat et al., 2020). Once soil water holding capacity is improved, WUE of crops, and water conservation practices are applied, the effects of prolonged droughts would be offset by these practices. The pictures in Figure 10 are some structures for water conservation and harvesting in drought-prone areas.
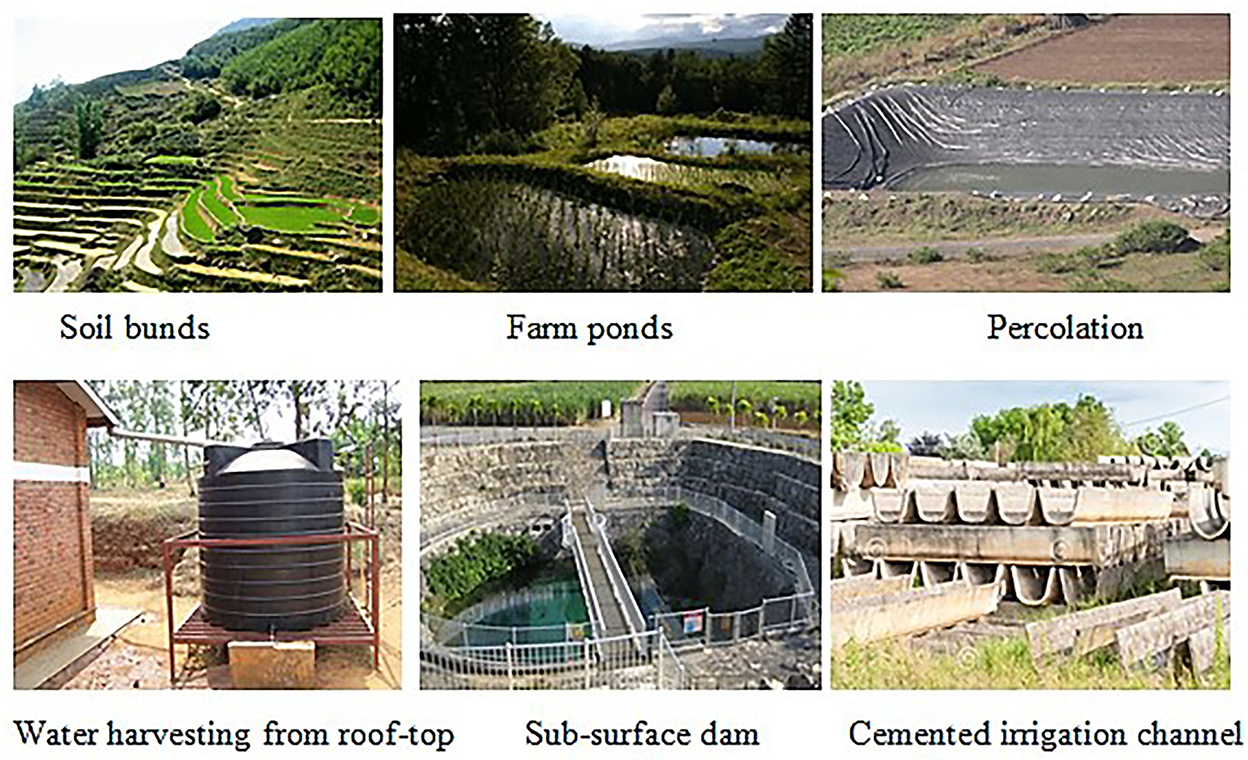
Figure 10. Water catchment infrastructure for drought-prone areas. Pictures collected from google images.
7.1. Efficient use of harvested water through irrigation
After conserving water in the above manner, water-efficient irrigation practices should be applied during water scarcity or drought. Water-efficient irrigation practices enhance the water use efficiency of crops. Micro-irrgation systems like drip and localized irrigation and sub-irrigation systems are very efficient irrigation systems that supply water specifically to crop roots (Khan et al., 2019). The method of water placement is important as drip irrigation is more efficient than sprinkler irrigation and sprinkler irrigation more efficient than furrow/flood irrigation (Khan et al., 2019). Approaches like scheduling irrigation based on evapotranspiration, scheduling irrigation based on soil water content, and using deficit irrigation are ways to avoid over-application and wasting water (Shock et al., 2013). Thus, the amount and timing of irrigation should match actual crop needs and proper water placement. The amount should be based on daily crop water requirements and the growth stage of plants. Some images of efficient irrigation systems are shown in Figure 11.
Drip irrigation was found to significantly increase the yield of four cotton varieties at an average of 2,405 kg/ha over the flood irrigation method (Table 3; Choudhary et al., 2016). In the same study, drip irrigation realized the highest water use efficiency ranging from 0.48 to 0.55 kg/m3 (Figure 12). Additionally, in a semi-arid cropping system, potatoes yielded 51.8 Mg/ha tubers, 16.3 kg/m3 irrigation WUE, and 374 mm of evapotranspiration under drip irrigation compared to 32.6 Mg/ha tuber yield, 14.1 kg/m3 WUE and 556 mm of evapotranspiration under furrow irrigation (Akram et al., 2020). In this experiment, irrigation was scheduled based on evapotranspiration needs. 562 mm of water was required through furrow irrigation while 374 mm of water was required through drip irrigation. These research findings are indications that with the right irrigation method, conserved water in the soil and harvested water can be used judiciously to maximize yield.
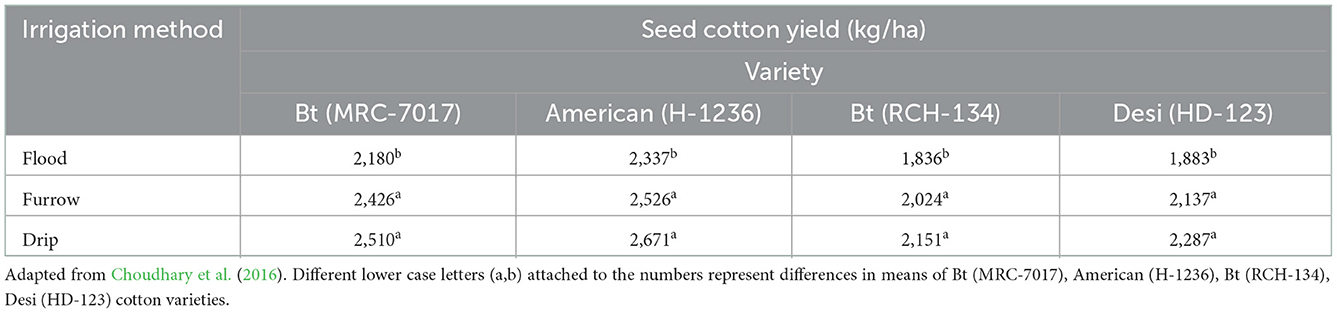
Table 3. Effect of different irrigation methods on seed cotton yield (kg/ha) of different cultivars at 5% probability.
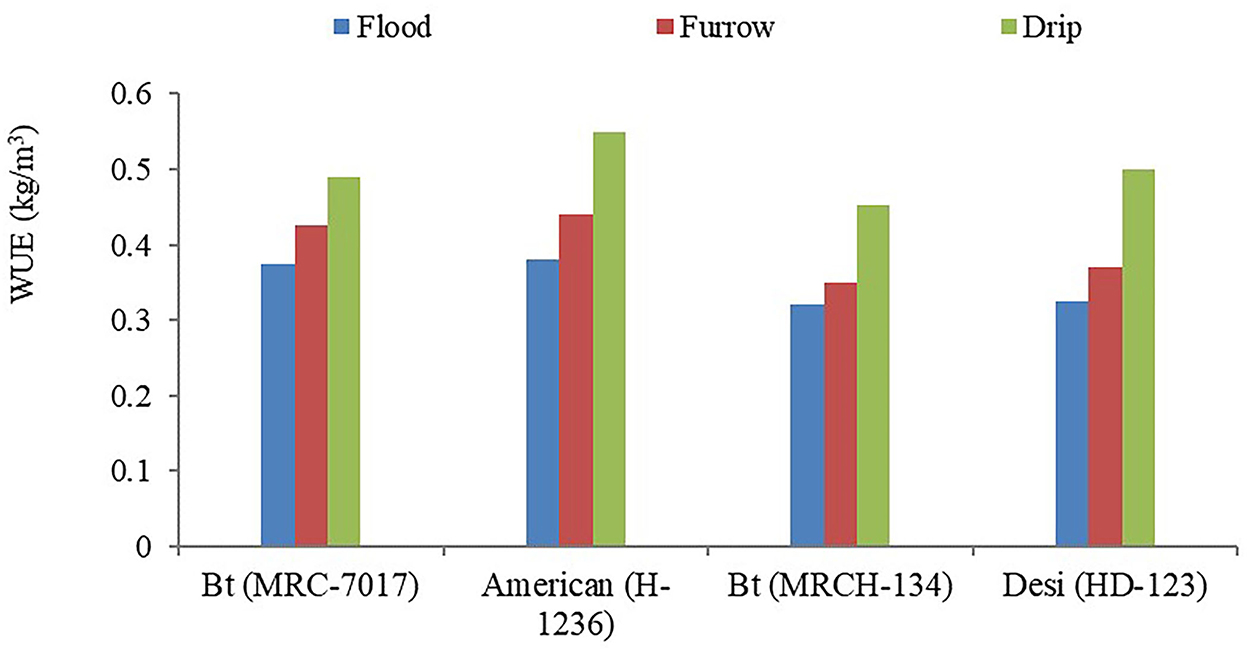
Figure 12. Effect of irrigation methods on WUE of different cotton cultivars. Adapted from Choudhary et al. (2016).
8. Organic recycling
Soils of cropping systems must be improved in preparation for the current and predicted global climate change. Lots of organic matter must be added to the soil to improve its water-holding capacity. In some medium-textured soil, the water holding capacity was increased by 1.03% per 1% increase in soil organic matter (Minansy and McBratney, 2017). Soil organic matter has an affinity for water it binds soil particles together while creating favorable conditions for soil microfauna whose movement and activity create micro and macropores in the soil, creating extra room for water holding (Hoffland et al., 2020).
A decline in soil organic matter (SOM) results from the intensive long-term use of the soil for farming without any organic amendments (manures, composts, crop residue) addition. It causes a reduction in the soil's biological activity and soil pore spaces, reducing the soil's ability to conserve fluids (water and air) (Hoffland et al., 2020). SOM addition could be achieved through crop rotations (Debele, 2020) that include high-residue crops and legumes. Residues can be incorporated into the soil. Cover crops (Debele, 2020), could serve as live soil cover that intercepts high rainfall intensities, reduces runoff velocities and erosion, and could also be incorporated into the soil to add organic matter. When organic amendments are recycled (added to the soil), the resulting organic matter binds soil particles together, improves soil structure and texture, and leaves the soil with more pore spaces. It improves permeability and infiltration and creates favorable conditions for soil micro-fauna whose movement and activity create micro and macro-pores in the soil, creating extra room for water holding (Hoffland et al., 2020). It also serves as a substrate and a source of energy for soil micro and macro-fauna, enhancing their composition, distribution, and activities. In flood-prone areas, the increased soil permeability from organic matter addition reduces runoff volumes and the tendency for the soil to be flooded. In drought-prone areas, the improvement in soil properties and the hydrophilic nature of organic matter (Ankenbauer and Loheide, 2016; Smith et al., 2017) improves and prolongs water-holding and reduces the tendency for crops to reach the permanent wilting point before the next rainfall or an irrigation intervention during drought.
Improving soil organic matter allows crops to take full advantage of natural rainfall and irrigation facilities and improves agricultural water use efficiency (Bhadha et al., 2017). Proper plant nutrition is indispensable when it comes to WUE of crops. With no other limiting factor, a well-nourished plant would produce more biomass and yield per unit of transpired water compared to an under-nourished plant. This is because nutrient deficiencies in plants tend to reduce the hydraulic conductivity of root cortical cells (Coffey et al., 2018). To make good use of the water taken up by plants, farmers must endeavor to supply enough nutrients to plants so that the water is not wasted.
9. Research and implementation accomplishments, gaps, and future needs
The need to meet food security demands in the face of climate change-induced water stresses has directed many scientists to research various agricultural techniques that conserve soil and water, to maximize yield (among others; Farooq and Siddique, 2015; Bista et al., 2018; Bhattacharyya et al., 2020; USDA, 2020; Stanojevic, 2021). In high-income countries, engineering industries are also investing in more complex water-saving infrastructure for agricultural use (Rodriguez et al., 2021; Kakoulas et al., 2022). Today, engineering inventions make it possible to have agricultural machinery designed with controlled traffic measures for cost-effective and energy-saving farm operations without tilling the soil unnecessarily (Patel et al., 2018). In sub-Saharan Africa, some successes have been chalked in the soil and water conservation research space; some of which include reduced tillage practices, high organic residue recycling agriculture, efficient irrigation practices, and even the improvising of simple water harvesters (Linderhof et al., 2022) among others. However, there seems to be a wide gap between research successes and actual implementation in farmer fields. This is because studies in the various disciplines that contribute to the adoption of developed technologies are highly contextualized and isolated. While scientific research and developments are focused on replicable documentation of agronomic conditions, practices, and metrics, it does not consider socio-economic metrics that affect farmer decision-making and adoption of the practices (Hermans et al., 2020). Specific farmer socio-economic conditions may hinder the feasibility of soil conservation techniques on-farm. On the other hand, isolated socio-economic studies often face doubtful internal validity due to farmer heterogeneity and biases in participant selection (Stevenson et al., 2014). It is common to find studies on conservation agriculture practices alone or socio-economic studies alone, however, there are very few that involve farmers in the research and also identify socioeconomic conditions that affect adoption in the same research (Hermans et al., 2020).
Need-based research (what forms of conservation research work where, for whom, and why) and farming systems research (FSR) must be encouraged to address the adoption and implementation problems. We make the following recommendations to bridge the gap between soil and water conservation research and adoption by farmers:
- Soils of cropping systems are diverse and dynamic; hence research should be tailor-made and most often on-farm to include farmers. Including farmers makes them contribute to knowledge generation and interpretation, appreciate the results, and sensitize adoption of the technology.
- Research should integrate ethnographic approaches and local knowledge to broaden farmer perspectives on the need for conservation measures (Beach and Larson, 2022). This is because resistance to technology may be linked to ethno-geographic histories.
- During soil and water conservation research, a wide range of variables within socio-economic and agronomic borders should be collected and made available to farmer platforms in lay-man comprehensive, consistent and comparable formats
- Socio-economic studies should ask open-ended questions about how farmers are going to integrate the new technology into existing farm practices
- Lastly, management must review and assess the performance of the technology frequently to encourage and advice farmers or even correct unforeseen situations that may occur after implementing the practice.
While adhering to the above recommendations, scientists should invest more time and resources into bridging the knowledge gap on the short and long-term impacts of soil and water conservation technologies on soil physical, chemical and biological fertility and water cycles in cultivated soils, using soil physics and hydro-ecology approaches. Scientists should also research more conservation agriculture practices that further reduce greenhouse gas emissions and consequent global warming. All researchers and practitioners associated with soil and water conservation such as soil scientists, geophysicists, hydro-ecologists, environmental statisticians, climate scientists, extension officers, and social scientists should be involved in this research to see to every step of technology development and adoption.
Some studies have observed sub-surface soil compaction after long-term practices of minimum tillage, which eventually limits crop yield. There is therefore the need to find a cut-off year where conventional tillage should be done once again or the soil sub-soiled to loosen it up, before minimum tillage practices continue. Since yield is the main economic product a farmer anticipates, such studies would help to not let farmers totally abandon soil implemented soil conservation measures.
10. Conclusion
Climate change imposes water stresses on the world and sub-Saharan Africa suffers the most from its effect. Droughts and floods induced by climate change pose threats to crop growth, yield, and quality; and call for immediate interventions. In the scope of feasibility, sub-Saharan Africa must integrate technologies that maintain efficient soil water balance such as improving permeability, infiltration, and water holding capacities to maximize yield. Physical barriers to soil water storage like soil compaction and erodibility should be amended. Scientists and engineers should help farmers improvise rainwater catchments in their fields and better yet, put in collective efforts with governments and all stakeholders to invest in more complex water harvesting and re-directing infrastructure. Water harvested through these techniques should be used efficiently through irrigation for crop production. Though many soil and water conservation research successes have been realized in researcher-managed fields, socio-economic conditions limiting their adoption must be considered by researchers to boost their embrace. Farmers may not easily single out and appreciate the benefits of soil and water conservation measures because many other farming practices may have been applied to realize the yields they observe. However, more research into the long and short-term impacts of the technologies on soil water cycles and other far-fetched benefits like reducing global warming may broaden their perspectives on the need for such technologies.
Author contributions
The general idea of the manuscript was conceived and discussed by all authors. MB drafted the first version of the manuscript. PA-Y, SY, EO, KA, AK, AA-D, and JA critically revised the subsequent versions of the manuscript. MB put together the final version of the manuscript which was agreed on by all authors for publication.
Acknowledgments
The authors are grateful to the Council for Scientific and Industrial Research (CSIR)-Crops Research Institute for providing an enabling environment to prepare this manuscript.
Conflict of interest
The authors declare that the research was conducted in the absence of any commercial or financial relationships that could be construed as a potential conflict of interest.
Publisher's note
All claims expressed in this article are solely those of the authors and do not necessarily represent those of their affiliated organizations, or those of the publisher, the editors and the reviewers. Any product that may be evaluated in this article, or claim that may be made by its manufacturer, is not guaranteed or endorsed by the publisher.
References
Abuarab, M. E., El-Mogy, M. M., Hassan, A. M., Abdeldaym, E. A., Abdelkader, N. H., and El-Sawy, M. B. I. (2019). The effects of root aeration and different soil conditioners on the nutritional values, yield, and water productivity of potato in clay loam soil. Agronomy. 9, 418. doi: 10.3390/agronomy9080418
Adesina, O. (2018). Effect of Waterlogging on Soil. Coventry: University of Warwick. doi: 10.13140/RG.2.2.25533.31202
Adeyemi, O., Keshavarz-Afshar, R., Jahanzad, E., Battaglia, M. L., Luo, Y., and Sadeghpour, A. (2020). Effect of wheat cover crop and split nitrogen application on corn yield and nitrogen use efficiency. Agronomy 10, 1081. doi: 10.3390/agronomy10081081
Adonadaga, M. G., Ampadu, B., Ampofo, S., and Adiali, F. (2022). Climate change adaptation strategies towards reducing vulnerability to drought in northern Ghana. Euro. J. Environ. Earth Sci. doi: 10.24018/ejgeo.34.294
Akram, M. M., Asif, M., Rasheed, S., and Rafique, M. A. (2020). Effect of drip and furrow irrigation on yield, water productivity and economics of potato (Solanum tuberosum L.) grown under semi-arid conditions. Sci. Letters. 8, 48–54. doi: 10.47262/SL/8.2.132020004
Aldana, F., Nel Garcia, P., and Fischer, G. (2014). Effect of waterlogging stress on the growth, development and symptomatology of cape gooseberry (Physalis peruviana L.) plants. Rev. Acad. Colomb. Cienc. 38, 393–400. doi: 10.18257/raccefyn.114
Amadu, F. O., Miller, D. C., and McNamara, P. E. (2020). Agroforestry as a pathway to agricultural yield impacts in climate-smart agriculture investments: evidence from Southern Malawi. Ecol Econ. 167, 106443. doi: 10.1016/j.ecolecon.2019.106443
Ankenbauer, K. J., and Loheide, S. P. (2016). The effects of soil organic matter on soil water retention and plant water use in a meadow of the Sierra Nevada, CA. Hydrol. Proc. 31, 891–901. doi: 10.1002/hyp.11070
Ansah, S. O., Ahiataku, M. A., Yorke, C. K., Out-Larbi, F., Yahaya, B., Lamptey, P. N. L., et al. (2020). Meteorological analysis of floods in Ghana. Adv. Meteorol. 2020, 1–14. doi: 10.1155/2020/4230627
Bai, D., Ye, L., Yuan, Z., and Wang, G. (2022). Impact of climate change on agricultural productivity: a combination of spatial Durbin mode and entropy approaches. School of economics. Anhui Univ. Finance. Econ. doi: 10.1108./IJCCSM-02-2022-0016
Beach, D., and Larson, S. (2022). On developments in ethnographic research: the case of two Swedish Universities. Int. J. Qual. Meth. 21, 4432. doi: 10.1177./16094069221084432
Becker, R. K., Barbosa, E. A. A., Giarola, N. F. B., Kochinski, E. G., Povh, F. P., Paula, de., et al. (2022). Mechanical intervention in compacted no-till soil in Southern Brazil: soil physical quality and maize yield. Agronomy, 12, 2281. doi: 10.3390/agronomy12102281
Bekele, D. (2020). The effect of tillage on soil moisture conservation: a review. Int. J. Res. Stud. Comput. 6, 30–41. doi: 10.20431/2454-6224.0610004
Bhadha, J. H., Capasso, J. M., Khatiwada, R., Swanson, S., and Labrode, C. (2017). Raising Soil Organic Matter Content to Improve Water Holding Capacity. Uni Florida: UF, IFAS Extension. doi: 10.32473./edis-ss661-2017
Bhattacharyya, P., Pathak, H., and Pal, S. (2020). “Water management for climate-smart agriculture.” In: Climate Smart Agric. Green Energy and Tech. Springer, Singapore. 57–72. doi: 10.1007./978-981-15-9132-7_5
Bista, D., Heckathorn, S., Jayawardena, D., Mishra, S., and Boldt, J. (2018). Effects of drought on nutrient uptake and the levels of nutrient-uptake proteins in roots of drought-sensitive and -tolerant grasses. Plants 7, 28. doi: 10.3390/plants7020028
Bista, P., Machado, S., Ghimire, R., and Yorgey, G. (2017). Conservation Tillage Systems. Pendleton long-term cropping systems project. Semi-arid cropping systems and soil health. Available online at: http://www.researchgate.net/publication/317648002_Conservation_Tillage_Systems (accessed October 16, 2022).
Brempong, M. B., Yeboah, S., Marno, P., Addo-Danso, A., Keteku, A. K., Agyeman, K., et al. (2022). Enhancing common bean tolerance to short-term droughts at the reproductive stage using a soil fertility management approach. J. Exp. Agric Int. 44, 56–74. doi: 10.9734/JEAI/2022/v44i112053
Chakraborty, K., and Mistri, B. (2017). Estimation of soil compaction from bulk density and its effect on crop production study in Burdwan-I Block. Indian J. Spatial Sci. Available online at: https://www.researchgate.net/publication/319939892_Estimation_of_~soil_compaction-from_bulk_density_and_its_effect_on_crop_production_Study_in_Burdwan-I_Block (accessed October 16, 2022).
Chandregowda, M. H., Tjoelker, M. G., Power, S. A., and Pendall, E. (2022). Drought and warming alter gross primary production allocation and reduce productivity in a widespread pasture grass. Plant Cell Environ. Willey. 45, 2271–2291. doi: 10.1111/pce.14334
Chen, S., Liu, S., Zheng, X., Yin, M., Chu, G., Xu, C., et al. (2018). Effect of various crop rotations on rice yield and nitrogen use efficiency in paddy-upland systems in southeastern China. Crop. J. 3, 7. doi: 10.1016/j.cj.07007
Choudhary, K., Phogat, V. K., and Dahiya, R. (2016). Effect of drip and furrow irrigation methods on yield and water use efficiency in cotton. Res. Crops. 17, 823–828. doi: 10.5958/2348-7542.2016.00139
Coffey, O., Bonfield, R., Corre, F., Sirigiri, J. A., Meng, D., and Frickle, W. (2018). Root and cell hydraulic conductivity, apoplastic barriers and aquaporin gene expression in barley (Hordeum vulgare L.) grown with low supply of potassium. Annals Botany. 54, 110. doi: 10.1093./aob/mcy110
Debele, G. G. (2020). Soil organic matter and its role in soil health and crop productivity improvement. Ethiopian Inst. Agric. Res. Acad. Res J. doi: 10.1466./ARJASR2019.147
Diop, M., Chirinda, N., Beniach, A., El-Gharous, M., and El-Mejahed, K. (2022). Soil and water conservation in Africa: State of play and potential role in tackling soil degradation and building soil health in agricultural lands. Sustainability, 14, 13425. doi: 10.3390/su142013425
Dorau, K., Luster, J., and Mansfeldt, T. (2018). Soil aeration: the relation between air-filled pore volume and redox potential. Eu. J Soil Sci. 69, 1035–1043. doi: 10.1111/ejss.12717
Dumbrovsky, M., Larisova, L., Sobotkova, V., and Kulihova, M. (2019). Comparison of different texture analysis for soil erodibility calculations of Loamy and sandy-loam soils in Moravian Regions. Acta Univ. Agri. et Silviculturae Mende. Brunensis 67, 383–393. doi: 10.11118/actaun201967020383
El-Shater, T., Mugera, A., and Yigezu, Y. A. (2020). Implications of adoption of zero tillage (ZT) on productive efficiency and production risk of wheat production. Sust. MDPI. 12, 3640. doi: 10.3390/su12093640
Engelstad, O. P., Patrick Jr, W. H., Mikkelsen, D. S., and Wells, B. R. (1985). Plant nutrient behaviour in flooded soils. Fert. Technol. Use 3, 250.
FAO (2015). Climate change and food security: risks and responses. Available online at: https://www.fao.org/3/i5188e/I5188E.pdf (accessed October 16, 2022).
FAO (2021). Drought and agriculture. Land and water. Available online at: http://www.fao.org/land-water/overview/en/ (accessed October 16, 2022).
Farooq, M., and Siddique, K. H. M. (2015). Conservation Agriculture. Springer. Available online at: https://link.springer.com/book/10.1007./978-3-319-11620-4 (accessed October 21, 2022).
Gathagu, J. N., Mourad, K. A., and Sang, J. (2018). Effectiveness of contour farming and filter strips on ecosystem services. Water. MDPI. 10, 1312. doi: 10.3390/w10101312
Gilbert, J. R. (2020). “Inorganic fertilizer use among smallholder farmers in sub-Saharan Africa: Implications for input subsidy policies.” In: The Role of Smallholder Farms in Food and Nutrition Security. 81–98. Available online at: https://link.springer.com/chapter/10.1007./978-3-030-42148-9_5
Havlin, J. L., Beaton, J. D., Tisdale, S. L., and Nelson, W. L. (2014). Soil Fertility and Fertilizers; An Introduction to Nutrient Management, 6th Edn. Upper Saddle River, NJ: Prentice Hall.
Hermans, T. D. G., Whitfield, S., Dougill, A. J., and Thierfelder, C. (2020). Bridging the disciplinary gap in conservation agriculture research in Malawi. A review. Agron. Sustain. Dev. 45, 9. doi: 10.1007./s13593-020-0608-9
Hoffland, E., Kuyper, T. W., Comans, R. N. J., and Creamer, R. E. (2020). Plant and soil. Mod. Synth. 455, 1–22. doi: 10.1007/s11104-020-046511-9
IMF (2022). Climate change and chronic food insecurity in sub-Saharan Africa. Eds: Bapista, D., Farid, M., Fayad, D., Kemoe, L. et al. African and Research Departments. International Monetary Fund
IPCC (2007). The IPCC Fourth Assessment Report, “Climate change 2007”, Synthesis Report. Available online at: https://www.ipcc.ch/assessment-report/ar4/ (accessed October 21, 2022).
IPCC (2022). Africa. Authors: Trisos et al. Available online at: https://www.ipcc.ch/report/ar6/wg2/downloads/report/IPCC_AR6_WGII_Chapter09.pdf (accessed October 21, 2022).
Jalli, M., Huusela, E., Jalli, H., Kauppi, K., Niemi, M., Himanen, S., et al. (2021). Effects of crop rotation on spring wheat yield and pest occurrence in different tillage systems: a multi-year experiment in Finnish growing conditions. Front. Sustain. Food Syst. 35, 7335. doi: 10.3389./fsufs.2021.647335
Jarawura, F. X. (2014). Perceptions of drought among rural farmers in the Savelugu district in the northern Savannah of Ghana. Ghana J. Geog. 6, 102–120.
Kakoulas, D. A., Golfinopoulos, S. K., Koumparou, D., and Alexakis, D. E. (2022). The effectiveness of rainwater harvesting infrastructure in a Mediterranean Island. Water. 14, 716. doi: 10.3390/w14050716
Khan, Y. D. I., Reddy, K. S., and Mukate, S. (2019). Micro irrigations systems. In: Sustainable livelihoods and adaptation to climate change (SLACC). 141–154. Center for Natural Res Manage, National Inst Rural Dev. Available online at: https://www.researchgate.net/publication/333660260_Micro_Irrigation_Systems (accessed October 17, 2022).
Khodadali, M., Alewell, C., Mirzaei, M., Ehssan-Malahat, E., Asadzadeh, F., Strauss, P., et al. (2021). Deforestation effects on soil erosion rates and soil physiochemical properties in Iran: a case study of using fallout radionuclides in a Chernobyl contaminated area. Soil Disc. 25, 2. doi: 10.5194./soil-2021-2
Kleppel, G. S., and Frank, D. A. (2022). Structure and functioning of wild and agricultural grazing ecosystems: a comparative review. Front. Sus. Food Syst. 5, 5514. doi: 10.3389./fsufs.2022.945514
Kulczycki, G., Sacala, E., Chohura, P., and Zaluska, J. (2022). Maize and wheat response to drought stress under varied sulphur fertilization. Agronomy. 12, 1076. doi: 10.3390/agronomy12051076
Kumawat, A., Yadav, D., Samadharmama, K., and Rashmi, I. (2020). Soil and Water Conservation Measures for Agricultural Sustainability. Intech Open. doi: 10.5772/intechopen.92895
Li, Y., Guan, K., Schnitkey, G., DeLucia, E., and Peng, B. (2019b). Excessive rainfall leads to maize yield loss of a comparable magnitude to extreme drought in the United States. Glob. Change Biol. 25, 14628. doi: 10.1111/gcb.14628
Li, Y., Niu, W., Cao, X., Wang, J., Zhang, M., Duan, X., et al. (2019a). Effect of soil aeration on root morphology and phoptosynthetic characteristics of potted tomato plants (Solanum lycopersicum) at different NaCl salinity levels. BMC Plant Biol. 19, 331. doi: 10.1186/s12870-019-1927-3
Linderhof, V., Cervi, W. R., van Oosten, C., Duku, C., Witte, E., Derkyi, M., et al. (2022). Rainwater harvesting for irrigation for climate-resilient and circular food systems. The case of Ghana's Bono East Region. Wageningen Uni. Res. Min. Agric. Nat. Food Qual. 5, 7865. doi: 10.18174./567865
Lottering, S., Mafongoya, P., and Lottering, R. (2020). Drought and its impacts on small scale farmers in sub-Saharan Africa: a review. South Af. Geograph Soc. 4, 5914. doi: 10.1080./03736245.2020.1795914
Mahmoodzadeh, D., and Karamouz, M. (2017). Influence of coastal flooding on seawater intrusion in coastal aquifers. World Environ. Water Res. Conf. 12, 66–79. doi: 10.1061./9780784480618.007
Minansy, B., and McBratney, A. B. (2017). Limited effect of organic matter on soil available water capacity. Eu. J. Soil Sci. 5, 12475. doi: 10.1111./ejss.12475
NASA-GISTEMP (2022). GISS Surface Temperature Analysis (GISTEMP), version 4. NASA Goddard Institute for Space Studies. Dataset accessed 20YY-MM-DD. Available online at: https://data.giss.nasa.gov/gistemp/ (accessed October 18, 2022).
Norton, J., Norton, U., and Ashilenje, D. (2016). Conservation agriculture in the Mt. Elgon Highlands of Kenya and Uganda. Successes and limitations. Tropentag. Solidarity in a competing world-fair use of resources. 19–21. Available online at: https://www.tropentag.de/2016/abstracts/abstract.php?code=94CdKPT (accessed October 16, 2022).
NWRM (2015). Strip cropping along contours. Available online at: http://nwrm.eu/sites/default/files/nwrm_ressources/a4_-strip_cropping_along_contours.pdf (accessed October 21, 2022).
Olson, K., Ebelhar, S. A., and Lang, J. M. (2014). Long-term effects of cover crops on crop yields, soil organic carbon stocks and sequestration. Open J. Soil Sci. 4, 284–292. doi: 10.4236/ojss.2014.48030
Page, M. J., McKenzie, J. E., Bossuyt, P. M., Boutron, I., Hoffmann, T. C., Mulrow, C. D., et al. (2021). The PRISMA 2020 statement: an upgraded guideline for reporting systematic reviews. PLoS Med. 3, 3583. doi: 10.1371./journal.pmed.1003583
Patel, A., Singh, R. C., Singh, D., and Shukla, P. (2018). Farm machinery for conservation agriculture. Available online at: https://www.biotecharticles.com/Agriculture-Article/Farm-Machinery-for-Conservation-Agriculture-4462.html (accessed October 17, 2022).
Pena-Gallardo, M., Vicente-Serrano, S. M., Dominguez-Castro, F., and Begueria, S. (2019). The impact of drought on the productivity of two rainfed crops in Spain. Nat. Hazards Earth Syst. Sci. 19, 1215–1234. doi: 10.5194/nhess-19-1215-2019
Rodriguez, D. J., Paltan, H., Garcia, L. E., Ray, P. A., Freeman, S., and St, G. (2021). Water-related infrastructure investmentsin a changing environment: a perspective from the World Bank. Water Policy 23, 31–53. doi: 10.2166/wp.2021.265
Rodriguez, S., Uloa, M., Perez, Y., and Rodriguez, L. (2016). Disturbances caused by floods in three physical properties of a vertisol soil in the east region of Cuba, cultivated with sugarcane (Saccharum spp.). HOLOS. 4, 115–129. doi: 10.15628/holos.2016.4658
Salem, H. M., Munoz, M. A., and Rodriguez, M. G. (2020). Effect of reservoir and minimum tillage practices on soil physical properties, soil water tension monitoring by wireless sensors network. Int. J. Water Res. Arid. Environ. 9, 46–55.
Schlesinger, W. H., and Bernhardt, E. S. (2013). Biogeochemistry: An Analysis of Global Change, 3rd Edn. Salt Lake City, UT: Elsevier Inc. doi: 10.1016/C2010-0-66291-2
Shao, M., Wang, Y., and Xia, Y. (2018). Soil drought and water carrying capacity for vegetation in the critical zone of the Loess Plateau: a review. Vadose Zone J. Available online at: https://access.onlinelibrary.wiley.com/doi/pdf/10.2136/vzj040077
Shock, C. C., Wang, F. X., Flock, R., and Eldredge L Pereira, A. (2013). Successful Potato Irrigation Scheduling. Sustainable Agriculture Techniques. Oregon State University Extension. Available online at: https://agsci.oregonstate.edu/sites/agscid7/files/malheur/attachments/em8911-PotatoIrrigationScheduling.pdf
Singh, S. U. K., Kumudini, S. I. R., Rajmohan, S. V., Kumar, S. R., et al. (2016). Manual for drought management. Dept Agric, Cooperation and Farmers Welfare. Gov't India. Availalble online at: https://agrocoop.nic.in/sites/default/files/Manual%20Drought%202016.pdf (accessed October 16, 2022).
Smith, P., Lutfalla, S., Riley, W. J., Torn, M. S., Schmidt, M. W. I., Soussana, J. F., et al. (2017). The changing faces of soil organic matter research. Eu. J. Soil Sci. 69, 23–30. doi: 10.1111/ejss.12500
Song, L., Jin, J., and He, J. (2019). Effects of severe water stress in maize growth processes in the field. Sustainability 11, 5086. doi: 10.3390/su11185086
Stanojevic, A. B. (2021). Conservation agriculture and its principles. Short Comm. Annals of Environ Sci Toxic. Available online at: http://www.researchgate.net/publication/350900095_Conservation_agriculture_and_its_principles (accessed November 02, 2022).
Stevenson, J. R., Serraj, R., and Cassman, K. G. (2014). Evaluating conservation agriculture for small scale farmers in sub-Saharan Africa and South Asia. Agric Ecosyst. Environ. 187, 1–10. doi: 10.1016/j.agee.0018
Surendra, S. J., and Awais, M. (2019). Climate change and agricultural productivity: a teoritical and empirical review. Glob. J. Econ. Bus. 8, 259–267.
Telkar, S., Kant, K., Pratap, S., and Solanki, S. P. S. (2017). Effect of mulching on soil moisture conservation. Biomolecule Reports. Available online at: http://www.researchgate.net/publication/320356486_Effect_of_mulching_on_Soil_Moisture_Conservation
Tengan, C., and Aigbavboa, C. O. (2016). Addressing flood challenges in Ghana: A case of the Accra metropolis. Conference: 5th Int Conf on Infrastructure Development in Africa. Available online at: https://www.researchgate.net/publication/316093848_ADDRESSING_FOOD_CHALLENGES_IN_GHANA_A_CASE_OF_THE_ACCRA-METROPOLIS
Teoh, E. Y., Teo, C. H., Baharum, N. A., Pua, T. L., and Tan, B. C. (2022). Waterlogging stress induces antioxidant defense responses, aerenchyma formation and alters metabolism of banana plants. Plants. 11, 2052. doi: 10.3390/plants11152052
Toth, Z., Tancsics, B., Kriszt, G., Kroel-Dulay, G., and Hornung, E. (2017). Extreme effects of drought on composition of the soil bacterial community and decomposition of plant tissue. Eur. J. Soil Sci. 68, 504–513. doi: 10.1111/ejss.12429
United Nations (2019). Climate change impacts on Africa's economic growth. Authors: Granadillos et al. African Development Bank. Available online at: https://www.afbd.org/sites/default/files/documents/publications/afbd-economics_of_climate_change_in_africa.pdf (accessed November 02, 2022).
USDA (2020). Soil and water conservation. A celebration of 75 years. Eds: Delgado, J.A., Gamtzer, C.J., Sassenrath, G.F. Available online at: https://www.swcs.org/static/media/cms/75th_Book_online_small_EB8351774A8B2.pdf (accessed October 16, 2022).
USDA (2021). Agroforestry strategic framework: Fiscal Year 2019–2020. Available online at: https://www.usda.gov/topics/forestry/agroforestry (accessed October 16, 2022).
Veerapan, R., and Sayed, S. I. (2020). Urban flood susceptibility zonation mapping using evidential belief function, frequency ratio and fuzzy gamma operator models in GIS: a case study of Greater Mumbai, Maharashtra, India. Geocarto. Int. 3, 1–26. doi: 10.1080./10106049.2020.1730448
Weilli, A. (2015). A guide to successful subsoiling. CETAB+, Victoriaville. 43. Available online at: https://www.cetab.org/publications (accessed November 18, 2022).
Keywords: drought, floods, soil-water balance, conservation tillage, conservation agriculture, efficient irrigation
Citation: Brempong MB, Amankwaa-Yeboah P, Yeboah S, Owusu Danquah E, Agyeman K, Keteku AK, Addo-Danso A and Adomako J (2023) Soil and water conservation measures to adapt cropping systems to climate change facilitated water stresses in Africa. Front. Sustain. Food Syst. 6:1091665. doi: 10.3389/fsufs.2022.1091665
Received: 07 November 2022; Accepted: 12 December 2022;
Published: 06 January 2023.
Edited by:
Daniel Oppong-Sekyere, Bolgatanga Technical University, GhanaReviewed by:
Raja Ben-Laouane, Cadi Ayyad University, MoroccoVesna Radovanović, University of Belgrade, Serbia
Copyright © 2023 Brempong, Amankwaa-Yeboah, Yeboah, Owusu Danquah, Agyeman, Keteku, Addo-Danso and Adomako. This is an open-access article distributed under the terms of the Creative Commons Attribution License (CC BY). The use, distribution or reproduction in other forums is permitted, provided the original author(s) and the copyright owner(s) are credited and that the original publication in this journal is cited, in accordance with accepted academic practice. No use, distribution or reproduction is permitted which does not comply with these terms.
*Correspondence: Mavis Badu Brempong, mbrempong@cropsresearch.org
†ORCID: Mavis Badu Brempong orcid.org/0000-0002-3632-0191