- Department of Sustainable Agriculture, Biodiversity and Ecosystem Management, School of Life Science and Bioengineering (LiSBE), Nelson Mandela African Institution of Science and Technology, Arusha, Tanzania
Globally, the increase in human population continues to threaten the sustainability of agricultural systems. Despite the fast-growing population in Sub-Saharan Africa (SSA) and the efforts in improving the productivity of crops, the increase in the yield of crops per unit area is still not promising. The productivity of crops is primarily constrained by inadequate levels of soil nutrients to support optimum crop growth and development. However, smallholder farmers occasionally use fertilizers, and the amount applied is usually small and does not meet plant requirements. This is due to the unaffordability of the cost of fertilizers, which is enough to suffice the crop requirement. Therefore, there is a need for alternative affordable and effective fertilization methods for sustainable intensification and improvement of the smallholder farming system's productivity. This study was designed to evaluate the symbiotic performance of indigenous soybean nodulating rhizobia in selected agricultural soils of Tanzania. In total, 217 rhizobia isolates were obtained from three agroecological zones, i.e., eastern, northern, and southern highlands. The isolates collected were screened for N2 fixing abilities under in vitro (nitrogen-free medium) and screen house conditions. The results showed varying capabilities of isolates in nitrogen-fixing both under in vitro and screen house conditions. Under in vitro experiment, 22% of soybean rhizobia isolates were identified to have a nitrogen-fixing capability on an N-free medium, with the highest N2-fixing diameter of 1.87 cm. In the screen house pot experiment, results showed that soybean rhizobia isolate significantly (P < 0.001) influenced different plant growth and yield components, where the average shoot dry weight ranged from 2.49 to 10.98 g, shoot length from 41 to 125.27 cm whilst the number of leaves per plant ranged from 20 to 66. Furthermore, rhizobia isolates significantly (P = 0.038) increased root dry weight from 0.574 to 2.17 g. In the case of symbiotic parameters per plant, the number of nodules was in the range of 0.33–22, nodules dry weight (0.001–0.137 g), shoot nitrogen (2.37–4.97%), total nitrogen (53.59–6.72 g), and fixed nitrogen (46.878–0.15 g) per plant. In addition, the results indicated that 51.39% of the tested bacterial isolates in this study were ranked as highly effective in symbiosis, suggesting that they are promising as potential alternative biofertilizers for soybean production in agricultural soils of Tanzania to increase productivity per unit area while reducing production cost.
1. Introduction
The human population is rapidly growing with an estimate of around 9.7 billion people worldwide by 2050 (Vollset et al., 2020; Giller et al., 2021). Based on the study by Giller et al. (2021), the global governments' focus on human fertility and efforts to reduce mortality rates and food shortages have been the drivers for rapid population growth. While the world is realizing rapid population growth, the increase is higher in Sub-Saharan Africa (SSA), with an estimated 3.1 billion people in 2100 compared to 2.4 billion people in 2021 (Giller et al., 2021). The growth in the global human population exceeds the food required in terms of quantities produced, availability, and accessibility not only due to a decrease in fertile, available arable land for food production, which is utilized for the construction of modern infrastructures but also due to the vulnerability of food systems to climate change (Sorvali et al., 2021; Sadigov, 2022), with water and energy becoming the limiting factors (Nassary et al., 2020). At the same insight, global food production needs to be increased by 70% by 2050 to meet the food demand of 9.5 billion people (Kopittke et al., 2019; Wolde et al., 2021). To ensure food security in terms of quantity produced, accessibility, availability, and nutrients, the agricultural output needs to be optimized to meet the global rise in food demand (Aloo et al., 2021; Nakei et al., 2022).
Soybean (Glycine max) is among the common nutritious and high-value leguminous crops grown worldwide. The value of the crop is due to its high protein and oil content. Globally, soybean production covers about 6% of arable land and 50% of legume-growing areas. In different countries of Africa, mostly in Sub-Saharan Africa (SSA), including Tanzania soybean is grown (Wilson, 2013; Santos, 2019; Vanlauwe et al., 2019). In Tanzania, the crop has recently gained popularity and attention due to its nutritional value for human consumption and animal feeds, as well as higher global market demand (Chianu and Mairura, 2019). Soybean is among the leguminous crops which is important for food production and the improvement of agricultural systems. However, soybean productivity in SSA is not promising (Snapp et al., 2018). The low productivity is mainly due to soil fertility depletion caused by continuously growing and harvesting crops, which leads to inadequate levels of nutrients for optimum crop growth for optimum yield (Parveen et al., 2019). For instance, soybean production is estimated to be 1.1 tons/ha in SSA compared to 2.4 tons/ha at the global level.
Nitrogen is the first and most important plant-limiting element in agricultural soils and its deficit is usually remedied mainly by synthetic nitrogenous fertilizers (Simon et al., 2015). In SSA, smallholder farmers are the major producers of food crops, including legumes. Attaining higher crop yield under intensive and smallholder farming systems requires adequate levels of fertilizers to suffice the crop requirement for growth and development due to significant reduction in plant nutrients in agricultural soils as a result of continuous cropping and soil erosion (Elkoca et al., 2015). Smallholder farmers rarely use fertilizers due to the higher cost associated with synthetic fertilizers (Simon et al., 2015). The extensive and indiscriminate use of these artificial N-fertilizers has been linked to environmental pollution and consumer health concerns (Elkoca et al., 2015). Biological nitrogen fixation (BNF), a naturally occurring process, can supply an appreciable quantity of nitrogen to the soil. Biofertilizers made from the BNF process, especially involving native strains, have been reported to be the most effective, cheap, and environmentally friendly (Pirbalouti et al., 2006; Komarek et al., 2017). Leguminous crops largely depend on BNF symbiotic association with rhizobia for fixing nitrogen (Simon et al., 2015).
Soybeans (Glycine max), like other legumes, establish symbiotic relationships with rhizobia to form nodules in their roots, leading to the BNF process. Rhizobia play a fundamental role in N supply to ecosystems through their ability to fix N in symbiosis with legumes and promote the growth of plants as well as increase the productivity of crops (Peoples et al., 2009). This can be witnessed through several notable efforts to increase the productivity of soybean through the use of biofertilizers as an alternative source of nitrogen by some key joint research projects including N2AFRICA, administered by the International Institute of Tropical Agriculture (IITA) under the Consultative Group on International Agricultural Research (CGIAR) (Vanlauwe et al., 2019). Other notable efforts are made by different national research institutions, universities, national soybean improvement programs, and private sectors (Khojely et al., 2018). Through effective symbiosis, soybean annually can fix about 16.4 Tg of N from the atmosphere, about 77% of the total amount of N fixed by legumes (Hartman et al., 2011). Despite having the ability to associate symbiotically with rhizobia to fix nitrogen, soybean associates with a narrow diversity of symbiotically effective rhizobia to fix nitrogen and the efficiency of nitrogen fixation differs with the type of rhizobia species (Nakei et al., 2022). In the areas where the soybean is grown without inoculation with biofertilizers, the nodulation reports have been poor (Agoyi et al., 2017). The inoculation of soybean with symbiotically effective rhizobia results in effective nodulation and N2-fixation; however, newly introduced rhizobia are observed to be outcompeted by well-adapted indigenous rhizobia, which are ineffective in N2-fixation (Ampomah et al., 2008; Mathu et al., 2012; Kim et al., 2014). More effort is required for the isolation of effective indigenous rhizobia and commercialization to produce biofertilizers for easy accessibility and availability to smallholder farmers to enhance the sustainable productivity of soybean in Tanzania and SSA in general.
Despite the adaptation and competitiveness of indigenous rhizobia over commercial strains (Ampomah et al., 2008; Mathu et al., 2012; Kim et al., 2014), the competition is linked to environmental conditions, motility, production of different compounds, and phytohormones, yet, it is difficult to define the genetic basis of competitiveness (Vanlauwe et al., 2019). Although there are commercial strains, such as B. diazoefficiens USDA 110, which can fix nitrogen in a wide range of soils, their assurances still need careful on-farm trials in different soil types, including those with extreme acidic, salinity, and alkalinity. For this case, the use of indigenous rhizobia biofertilizer formulations is gaining the attention of different researchers to obtain indigenous strains of rhizobia that can effectively fix nitrogen in a wide range of soils, as well as the most effective ones in soils with extreme abiotic stresses. The availability of commercialized indigenous rhizobia species will provide easy accessibility to smallholder farmers for sustainable intensification of soybean productivity in their farming systems. The present study focused on isolating indigenous rhizobia from different soils of Tanzania that form symbiotic nodules with a specific soybean variety (Uyole IV) and testing their effectiveness under controlled conditions. The findings of this study enrich the knowledge that the researchers could tap into for the benefit of further studies on searching for the most effective indigenous rhizobia species and even the prospect of site-specific strains, especially those exposed to abiotic stresses.
2. Materials and methods
2.1. Description of the study area
The present study included isolates from nine regions (Iringa, Njombe, Ruvuma, Songwe, Rukwa, Mbeya, Morogoro, Arusha, and Kilimanjaro) of Tanzania and from mainly three agroecological zones. The isolates were collected from major soybean growing regions in the southern highlands zone (Iringa, Njombe, Ruvuma, Songwe, Rukwa, and Mbeya) of Tanzania. This zone is located between latitudes 7°C and 11.5°C S and longitudes 30°C and 38°C E with an elevation ranging from 302 to 2,925 m above sea level (m.a.s.l.). Rainfall is unimodal, falling from November to May with an annual rainfall of 1,650 mm and dry periods ranging from June to September (Mfwango et al., 2018). The mean annual temperature ranges from 7 to 32.2°C. The eastern zone included the Morogoro region, located between latitudes 5° and 9° S and longitudes 35° and 38° E. The mean annual temperature ranges from 15 to 32°C and the average annual rainfall is around 740 mm (Kacholi, 2020). The northern zone, Arusha, and the Kilimanjaro regions were included in the present study. Arusha region lies between latitudes 1 and 4°S and longitudes 34 and 37°E with an average annual rainfall of 873 mm. In contrast, the temperature ranges from 12.1 to 28.8°C. Kilimanjaro region lies between latitudes 2 and 4°S and longitudes 36 and 38°E. The average annual rainfall in the Kilimanjaro region ranges between 700 and 2,000 mm, and the temperature ranges from 12.5 to 27°C. The description of sampling sites in terms of altitudes is presented in Figure 1.
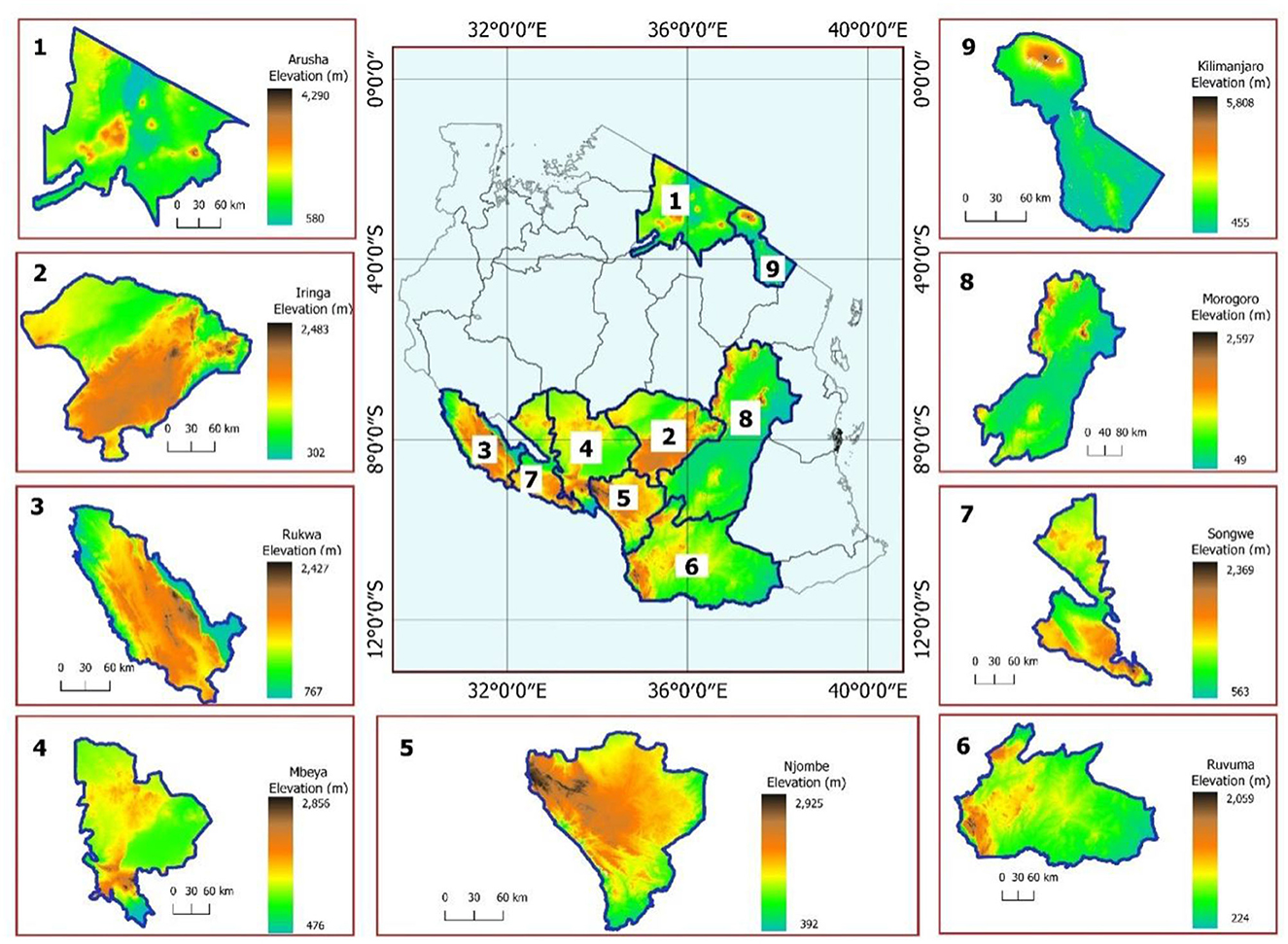
Figure 1. The map showing maximum and minimum elevations of the regions where soils and nodules were sampled.
2.2. Nodules collection and isolation of rhizobia from root nodules
The study sites and the sampling location map were generated using QGIS 3.14.0 software (Figure 2). The collection of nodules was conducted during cropping seasons. In each region, three districts were selected, where three villages in each district were selected, and one field was selected for the collection of nodules in each village. Three plants with nodules at 50% flowering stage were randomly collected from each field, making a total of 243 plants, and treated as separate samples (Somasegaran and Hoben, 2012; Abrar and Letebo, 2017). The roots were cut at the crown level, kept in an icebox (around 4°C), and transported to the laboratory at Nelson Mandela African Institution of Science and Technology (NM-AIST), Arusha, Tanzania, for isolation, testing of effectiveness, and identification of effective rhizobia strains.
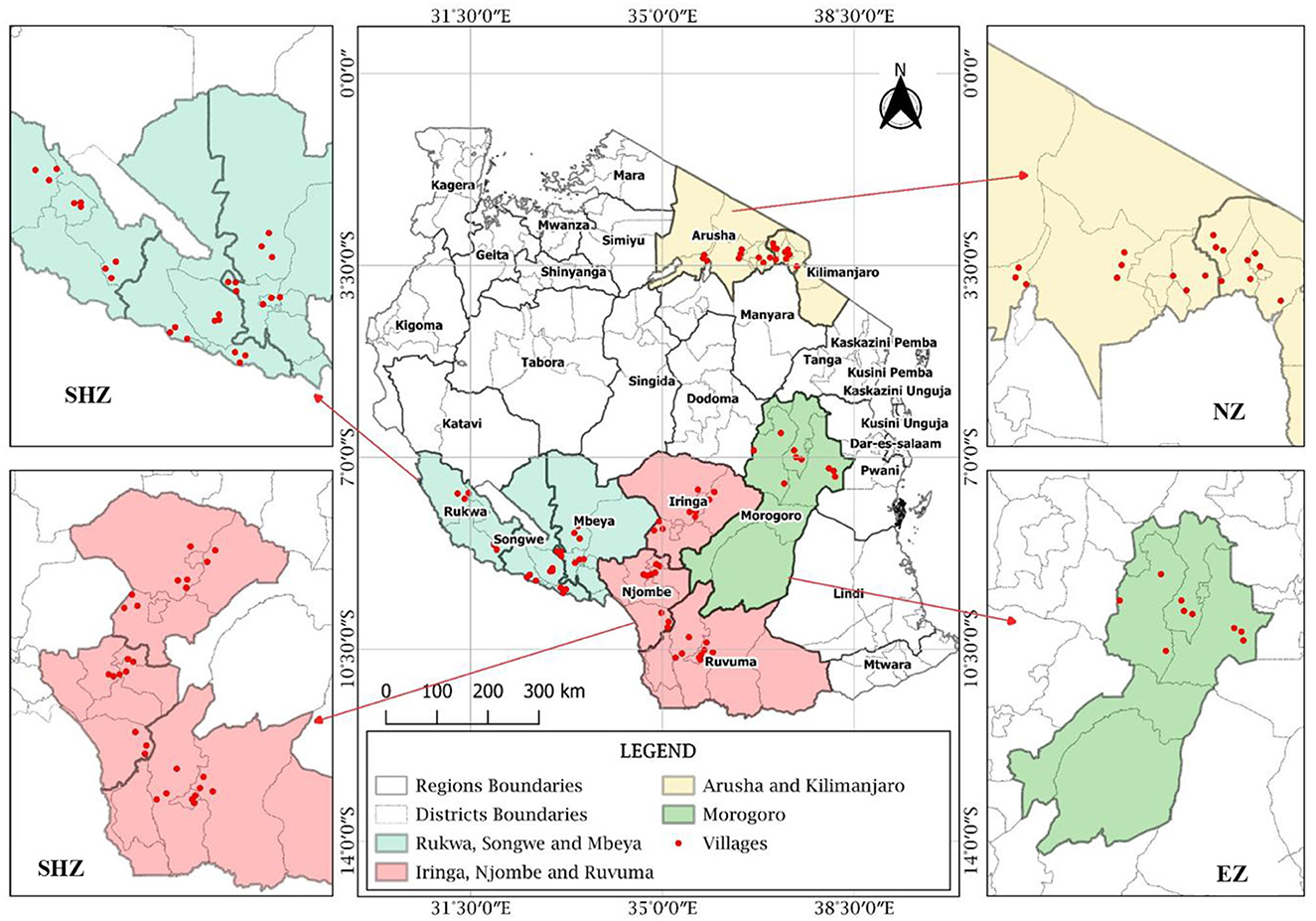
Figure 2. Map showing nodules sampling fields in different regions of Tanzania (SHZ-Southern Highland Zone, EZ-Eastern Zone, and NZ-Northern Zone).
Before isolation of nodules, the media, Yeast Extract Mannitol Agar (YEMA), was prepared by adding 0.5 g of dipotassium phosphate (K2HPO4), 0.2 g of magnesium sulfate (MgSO4.7H2O), 0.1 g of sodium chloride (NaCl), 1 g of calcium carbonate (CaCO3), 0.5 g of yeast extract powder, 10 g of mannitol, 15 g of agar bacteriological, and 0.025 g of Congo red in 1 L of distilled water. The pH of the media was adjusted to 7.0, which is optimum for the growth of rhizobia (Legesse, 2016), and the medium was sterilized by an autoclave at 15 pound-force per square inch (lbs) and 121°C for 15 min.
For isolation, nodules from each plant were detached from the roots and surface-sterilized in 70% ethanol for 30 s, followed by 1% sodium hypochlorite (NaClO) for 30 s and rinsed five times by using sterilized distilled water to remove the adhering sterilizing agents. From the sterilized nodules, one active nodule was selected by dissecting the nodules using a sterile surgical blade to observe the pinkish-red color as the indicator of leghemoglobin presence. Approximately 243 nodules were selected for the isolation of rhizobia. All the selected nodules were crushed separately with sterile toothpicks (Res, 2013) in sterile Eppendorf tubes containing 0.5 μL of 0.9% sodium chloride (NaCl) solution to obtain a milky suspension (Yuan et al., 2016). The suspension was streaked on a solidified YEMA medium; then, the plates were incubated at 28°C for 7 days (Abrar and Letebo, 2017). The plates were placed in an inverted position to avoid contamination by condensation of water. Bacterial growth on plates was observed every day after incubation for 7 days. Pure single colonies were then picked and then used for the N2 fixation test, both under in vitro and screen house conditions.
2.3. In vitro screening for N2 fixation test on solid N-free media
The intrinsic BNF ability of soybean nodulating rhizobia isolates was established on solid N-free media (NFM) in the in vitro assays before the screen house experiment. The NFM was prepared following the procedure described by Baldani et al. (2014). Then, the medium was sterilized using an autoclave at 1.05 kg/cm2 pressure and a temperature of 121°C for 15 min. After sterilization, the medium was left to cool to about 50°C and then poured aseptically into sterile Petri dishes and left to solidify. After solidification, the pure rhizobial cultures were spot-inoculated on the medium. Each culture was considered as a treatment, the inoculation of each treatment was done in triplicates, and a non-inoculated plate was maintained as a control. The cultures were incubated at 28 ± 2°C for 7 days. After 7 days, the colony diameter and the yellow-colored zone around each colony were measured using a ruler and the values were recorded (Akintokun et al., 2019). The color changes in the media were observed to distinguish slow and fast growers.
2.4. Assessing the symbiotic effectiveness of isolates in the glass house
For the assessment of the symbiotic effectiveness of rhizobia in the screen house, the soil samples for the pot experiment were collected from the uncultivated area near the NM-AIST farm, and the fertility status was evaluated for its suitability to support rhizobia activities and growth of soybean plants. Three soil samples were taken from three spotted locations depending on the color of the soil because the area was too small. For each spotted location, about 1,000 g of soil samples were collected from 0 to 30 cm depth. Then, one composite soil sample was prepared by mixing three soil samples and removing the roots and crumbs. Prior to laboratory analysis, the soil was air-dried and sieved through a 2-mm mesh. The soil organic carbon was characterized by Walkley-Black's wet digestion (oxidation) method as described by Nelson and Sommers (1982). Soil pH was measured electrochemically in 1:2.5 (w/v) soil using the water suspensions potentiometric method previously described by Thomas (1982). Total nitrogen was determined by the micro-Kjedahl digestion–distillation method described by Motsara and Roy (2008). Extractable P was determined using the Bray 1 procedure because the soil sample had a pH of <7 (Bray and Kurtz, 1945). Cation exchange capacity was measured at pH 7 with 1 M ammonium acetate (NH4OAc), and exchangeable cations K+ and Na+ were determined by flame photometer. In contrast, Ca2+ and Mg2+, as well as micronutrients iron (Fe), copper (Cu), zinc (Zn), and manganese (Mn), were determined by atomic absorption spectrophotometer (Thomas, 1982; Motsara and Roy, 2008). Moreover, the exchangeable sodium percentage (ESP) was calculated by dividing exchangeable Na by CEC (× 100) (Msanya, 2012). The ratings of physico-chemical parameters were based on the compilation of Motsara and Roy (2008).
For the pot screen house experiment, soybean seeds were sterilized with 70% ethanol for 1 min and soaked in 0.25% NaClO solution for 3 min. Then, the seeds were washed with distilled water five times before sowing to remove the disinfectants (Youseif et al., 2014). The seeds were aseptically transferred into a sterile Petri dish containing 1% water with tissue paper and allowed to germinate for 3 days at 25°C (Yuan et al., 2016). Then, the soil for growing crops was sterilized by oven drying. The germinated seeds (sprouts) were planted in 2-L plastic pots containing sterilized soil (oven dried at 70°C for 48 h (Sinegani and Hosseinpur, 2010). One germinated seed was planted per pot, and the pots were arranged in a complete randomized block design with three replicates. Each seedling was inoculated with 1 ml of 3-day-old rhizobia cultures, whereby the cells were calibrated to 109 ml−1 using a Macfarland scale at an optical density (OD) of 540 nm (Youseif et al., 2014). One positive control (commercial inoculum) and two negative controls (non-inoculated plants) and urea fertilizers at a recommended rate of 20 kg N/ha (Senkoro et al., 2017) were used as a comparison for the test cultures. All plants were given equal treatments by watering with nitrogen-free nutrient solution. The solution composition was CaCl2.2H2O 294 g/l, MgSO4.7H2O 123.3 g/l, KH2PO4 136.1 g/l, and K2SO4 87 g/l for macronutrients. Furthermore, Fe-citrate 6.7 g/l, MnSO4.H2O 0.338 g/l, H3BO3 0.247 g/l, ZnSO4.7 H2O 0.288 g/l, CuSO4.5 H2O 0.1 g/l, COSO4.7H2O 0.056 g/l, and Na2MoO4.2H2O 0.048 g/l were used for supplementation of trace nutrient elements (Maingi and Shisanya, 2006).
At 50% flowering (42 days after sowing), the plants were harvested to assess the presence of nodules in the roots. The isolates that managed to nodulate were proven as soybean-specific rhizobia. To collect the data for above-ground biomass, the shoots were collected by cutting the plants at the ground level. The shoots were placed in paper bags and dried at 70°C for 48 h, as described by Legesse (2016), and their dry weights were recorded. The soil particle and the roots, which adhered to the detached nodules during the uprooting of plants, were separated by sieving through a 0.76-mm mesh and then washed gently with running tap water to clean the nodules and the roots completely. The roots were dried the same way as the shoots, all the nodules collected for each plant were counted, and their dry weight was determined in the same manner as the shoots and roots. The N concentration in the shoot was determined by using a micro-Kjedahl digestion–distillation method (Motsara and Roy, 2008). Total nitrogen (TN) and fixed nitrogen (FN) values per plant were calculated in above-ground biomass as shown in Equations 1 and 2 (Elkoca et al., 2015).
The percentage increase/decrease in response/dependent variables was calculated from rhizobial treatments named Tanzania Soybean Rhizobia (TZSR) to compare the differences in inoculated, N-fertilized, and non-inoculated plants using Equation 3.
The accumulation of shoot dry matter (DM) as the relative percentage of symbiotic effectiveness (S.E.) of isolates was calculated as shown in Equation 4. Nitrogen fixation was rated as highly effective > 85%, effective 55–85%, lowly effective 35–54%, and ineffective <35% as described by Legesse (2016).
2.5. Statistical data analysis
Different statistical methods were applied to analyze the collected data in terms of its distribution and correlation among the studied parameters. Data of all the collected nodules were statistically analyzed by R-software version 4.1.0, GenStat 15th Edition, and Jamovi version 2.3.2.0. The statistical difference for in vitro N2 fixation test, among plant growth, yield components, and symbiotic traits, within and between the isolates was determined by one-way analysis of variance (ANOVA) following the factor effect model as shown in Equation 5. Tukey's-HSD multiple comparison test at a threshold of 5% in GenStat 15th Edition was conducted to separate mean values among replications of the in vitro N2 fixation test, plant growth, yield components, and symbiotic traits. Irrespective of the sample collection sites, some of the isolates did not show symbiosis with the rhizobia strains; hence physiographic effect was excluded from the analysis. Therefore, only one factor, the isolates (i.e., 47 isolates) that were capable of fixing nitrogen, was considered as the fixed main effect whereas replicates were treated as the random effect.
where Yi is the observed response variable in the ith factor; μ is the overall (grand) mean; αi is the main effect of the factor isolates; εi is the random error associated with the observation of the response variable in the ith factor.
To understand the relationship among studied parameters for the tested isolates, Pearson correlation matrix analyses for nodulation, growth, and yield components were performed using Jamovi software version 2.3.2.0. The trends in N2 fixation traits were generated by using R-software version 4.1.0 Simpson (2015).
3. Results
3.1. In vitro nitrogen fixation abilities of soybean nodulating rhizobia isolates on solid nitrogen-free medium
About 217 rhizobia isolates were obtained from 243 nodules collected from different farmers' fields. The isolates were grown on NFM to test their abilities to fix nitrogen. Only 47 isolates (22%) were capable of growing on NFM as an indication of nitrogen fixation, and a change in the color of media from blue to yellow, indicating that the isolates are fast-growing rhizobia (Figure 3), was observed. The diameter of their nitrogen fixation zones on NFM varied significantly (P = 0.001) among 47 isolates (Figure 4), with an average of 1.23 cm. The largest N2 fixation zone was observed in TZSR27A (1.87 cm), closely followed by TZSR25A (1.6 cm), and TZSR39C, TZSR41B, and TZSR56C, all of which had a fixation zone of 1.53 cm. At the same time, the smallest diameter (0.8 cm) was observed in TZSR35C, TZSR48B, TZSR67A, and TZSR72B.
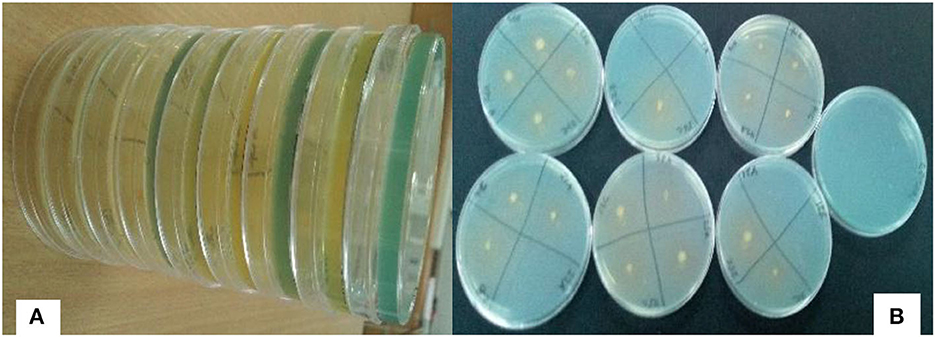
Figure 3. Yellow zones on solid nitrogen-free medium [(A) Color changes from blue to yellow, (B) Yellow zones around the rhizobia colony].
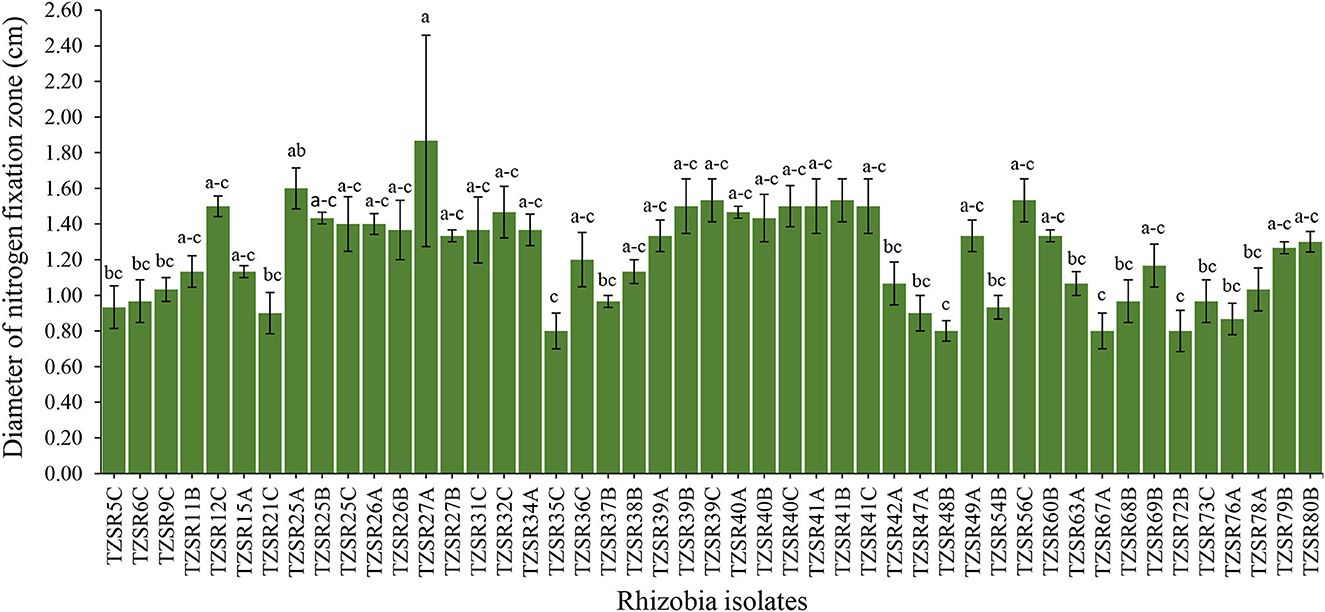
Figure 4. Nitrogen fixation zones of different rhizobia isolates on solid nitrogen-free medium (bars with different letters are statistically significantly different at P < 0.05).
3.2. The physicochemical properties of the soil used for the potting experiment
The physicochemical properties of the soil used for the pot experiment were evaluated and the results are presented in Table 1. The soil was sandy clay loam in texture, with soil reaction ranging from slightly acid (pH = 6.4 in CaCl2) to neutral (pH = 6.6 in water). The percentage of organic carbon (OC) and total nitrogen were 3.2 and 0.36%, rated as very high and medium, respectively. The levels of extractable phosphorus (83.95 mg kg−1) and micronutrients, such as zinc (8.09 mg kg−1), manganese (10.96 mg kg−1), and iron (10.53 mg kg−1) were very high while that of copper (2.28 mg kg−1) was high. Exchangeable bases, such as calcium [14.05 cmol(+) kg−1] and magnesium [3.12 cmol(+) kg−1] were very high, while potassium [0.4 cmol(+) kg−1] was medium and sodium [0.089 cmol(+) kg−1] was very low. In addition, the cation exchange capacity [CEC; 19.12 cmol(+) kg−1] of the experimental soil was medium.
3.3. Nodulation and plant growth, shoot N, N-fixed, and symbiotic effectiveness
Rhizobia isolates were inoculated on soybean seedlings to assess their effects on plant growth parameters, as presented in Table 2, and the formation of symbiotic nodules and the modification of root system architecture in Figures 5A, B. There was a significant (P < 0.001) difference in the number of leaves, shoot length, nodules number, nodules dry weight, shoot dry weight, and total plant dry weight among the tested rhizobia isolates. Root dry weight differed significantly (P = 0.038) while there was a non-significant (P = 0.081) difference in root length among the rhizobia isolates. The highest nodule number per plant (22) was recorded in plants inoculated with isolated TZSR25B, whilst the lowest (0.33) was recorded in those inoculated with isolate TZSR34A. The highest nodule dry weight of 0.137 g was observed in plants inoculated with isolate TZSR41A whilst the lowest (0.001 g) was observed in those inoculated with isolate TZSR34A. The plants inoculated with TZSR41A also recorded the highest shoot dry weight of 10.98 g, corresponding to a 75.82% increase above the control (non-inoculated plants).
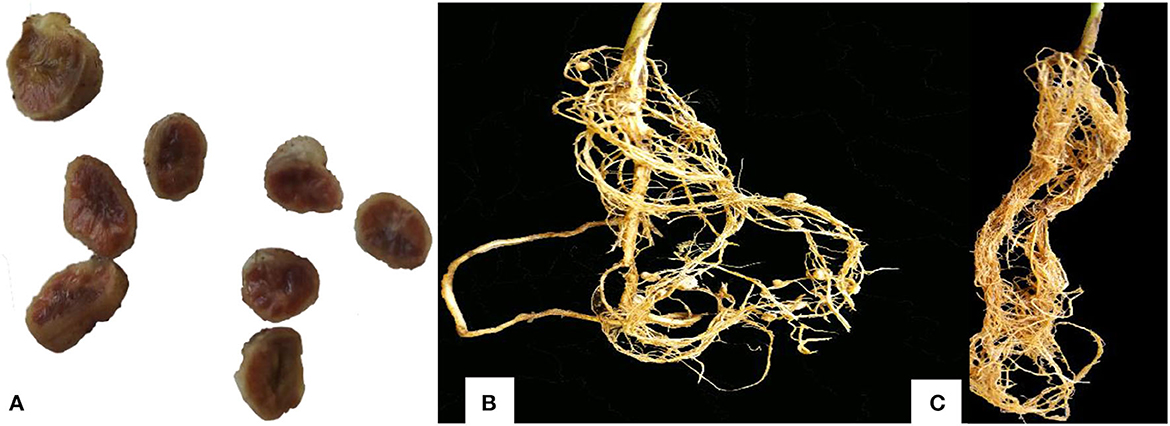
Figure 5. Effective soybean nodules and the root form of inoculated and un-inoculated plants [(A) Effective nodules, (B) root of inoculated plant having nodules, (C) root of un-inoculated plant, which did not form nodules].
On the other hand, the lowest shoot dry weight of 2.49 g, corresponding to −4.73% over un-inoculated plants, was observed in plants inoculated with isolate TZSR21C. Interestingly, the highest root dry weight of 2.17 g corresponding to a 92.49% increase over un-inoculated plants was observed in plants inoculated with isolate TZSR69B, which had very few nodules, 1.33 per plant, but higher root density (Figure 5C). Conversant to this, the plants inoculated with isolate TZSR27B had the lowest average root dry weight, 0.574 g, which is 75.28% higher than un-inoculated plants but having many nodules, about 16.67 per plant.
The largest average total plant dry weight, 12.36 g corresponding to 73.95%, was observed in plants inoculated with isolate TZSR26B with the lowest 3.626 g corresponding to 11.22 % in rhizobia in those inoculated with isolate TZSR21C. The plants inoculated with rhizobia isolate TZSR41A yielded the highest average shoot length, 125.27 cm, corresponding to a 68.19 % increase over un-inoculated control plants. On the other hand, the lowest shoot length of 41 cm, corresponding to a 24.70% increase over un-inoculated plants, was observed in the plants inoculated with isolate TZSR34A. The largest number of leaves, 66 per plant, corresponding to 63.48%, was recorded in plants inoculated with isolate TZSR39B, while the lowest, 20, corresponding to a 52.38% increase, was observed in those inoculated with isolate TZSR39C. Interestingly, the highest root length of 43 cm corresponding to a 27.14% increase was recorded in plants inoculated with isolate TZSR40B whilst the lowest root length, 23.67 cm, corresponding to 22.4% lesser than un-inoculated plants, was recorded in plants inoculated with isolate TZSR63A.
Interestingly, the plants inoculated with two isolates, TZSR39C and TZSR41A, were observed to have consistently higher values for most of the studied plant growth and yield components except for root length and dry weight. On average, inoculated plants performed better than non-inoculated plants. Moreover, compared with positive control treatments, legume fix, and urea, rhizobia isolates performed better on average for all the tested parameters except for plant height and root dry weight, which was higher in plants where urea was applied. Surprisingly, three-quarters (19) of tested isolates (47) performed better than the legume fix except for shoot dry weight. However, half of the isolates performed better than urea except for root dry weight, in which 11 out of 47 isolates were better than urea.
Inoculation of soybean seedlings with rhizobia significantly (P < 0.001) increased shoot concentration of N, total N, fixed N, and symbiotic effectiveness of N (Table 3). The highest N concentration of 4.97% was recorded in plants inoculated with isolate TZSR27B; the lowest, which was 2.37 %, was recorded in those inoculated with isolate TZSR37B. Conversely, inoculation of plants with rhizobia isolates TZSR41A yielded higher averages of total and fixed N levels of 53.59 and 46.878 g, respectively. The lowest level of total N (6.72 g) was recorded in the un-inoculated treatment (NC-0), whereas that of fixed N (0.15 g) was recorded in plants inoculated with isolate TZSR36C. Surprisingly, the plants inoculated with isolates TZSR25A, TZSR27B, and TZSR41A that have the highest fixed N are those with the highest shoot concentration of N and total N. Among the tested, 47 rhizobia isolates, 25 equivalents to 53% isolates were highly effective in symbiosis, 18 (38%) were effective, and 4 (9%) were lowly effective in symbiosis. In addition, among the isolates which are highly effective in symbiosis, TZSR25A (245.46 %) and TZSR26B (242.40 %) were ranked high in symbiotic effectiveness (Table 3).
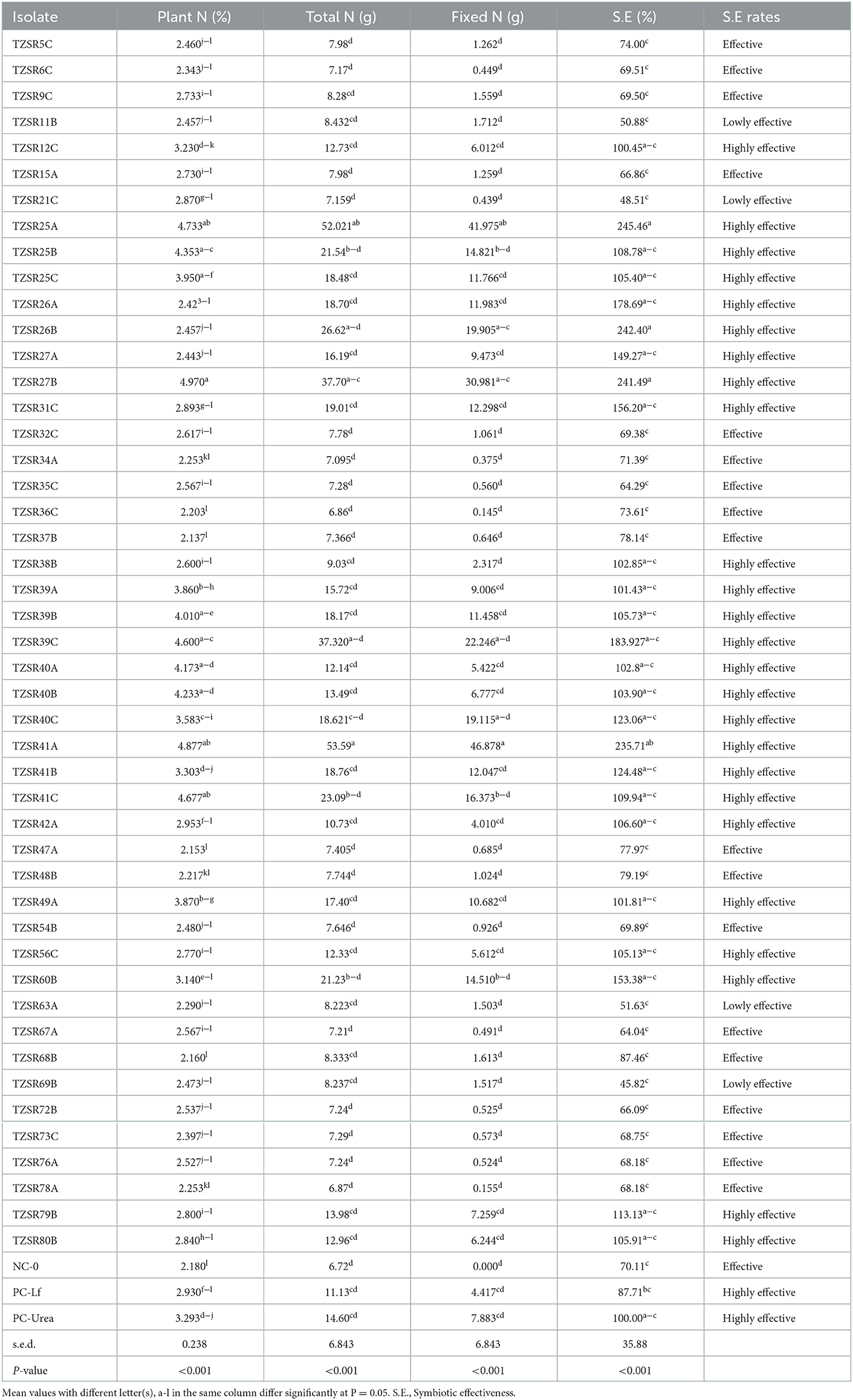
Table 3. Shoot N, fixed N, phosphorus, and symbiotic effectiveness of soybean with rhizobia isolates inoculation.
3.4. Relationship for nodulation and plant growth and trends in N2 fixation traits
The results indicated that nodulation (nodule numbers and dry weight) influenced different plant growth and yield components and interactions between various components (Table 4). There was a positive and significant (P < 0.001) correlation of total plant dry weight with shoot length, number of leaves, shoot dry weight, root dry weight, number of nodules, and nodules dry weight. Nodule dry weight was positively and significantly (P < 0.001) correlated with shoot length, shoot dry weight, and nodule numbers. Conversely, there was a positive and significant correlation (P = 0.004) of nodule dry weight with the number of leaves. There was a positive and significant (P < 0.001) correlation of nodule numbers with shoot length and shoot dry weight and number of leaves (P = 0.007). A significant positive correlation (P < 0.001) was found in shoot dry weight with shoot length and the number of leaves. Moreover, root length was significantly (P = 0.011) correlated with the number of leaves. Furthermore, shoot length had a significantly (P < 0.001) positive relationship with the number of leaves.
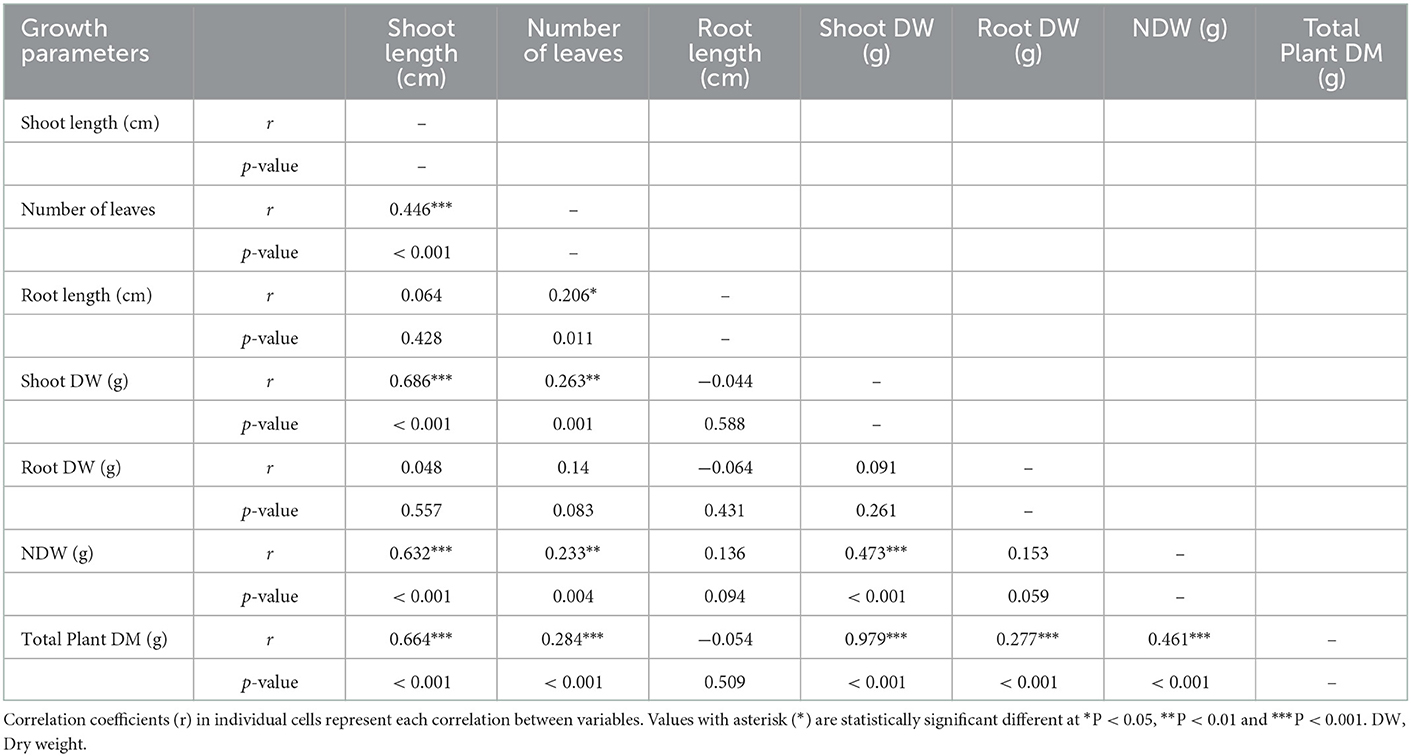
Table 4. Pearson correlation matrix for nodulation parameters (nodule number and dry weight), plant growth (root and shoot dry weight).
A correlation analysis was performed across symbiotic traits identifying their relationships and strength in predicting SNF. All the symbiotic traits had positive significant (P < 0.001) correlations in predicting SNF at the flowering stage (Figure 6). The study found a significant positive correlation for nodule numbers with shoot dry weight (r = 0.5186), percentage plant N (r = 0.5465), fixed N (r = 0.5622), and total N (r = 0.5633). For the case of nodules dry weight, there was a significant relationship between nodules dry weight and shoot dry weight (r = 0.4732), percentage plant N (r = 0.6157), fixed N (r = 0.5637), and total N (r = 0.5617).
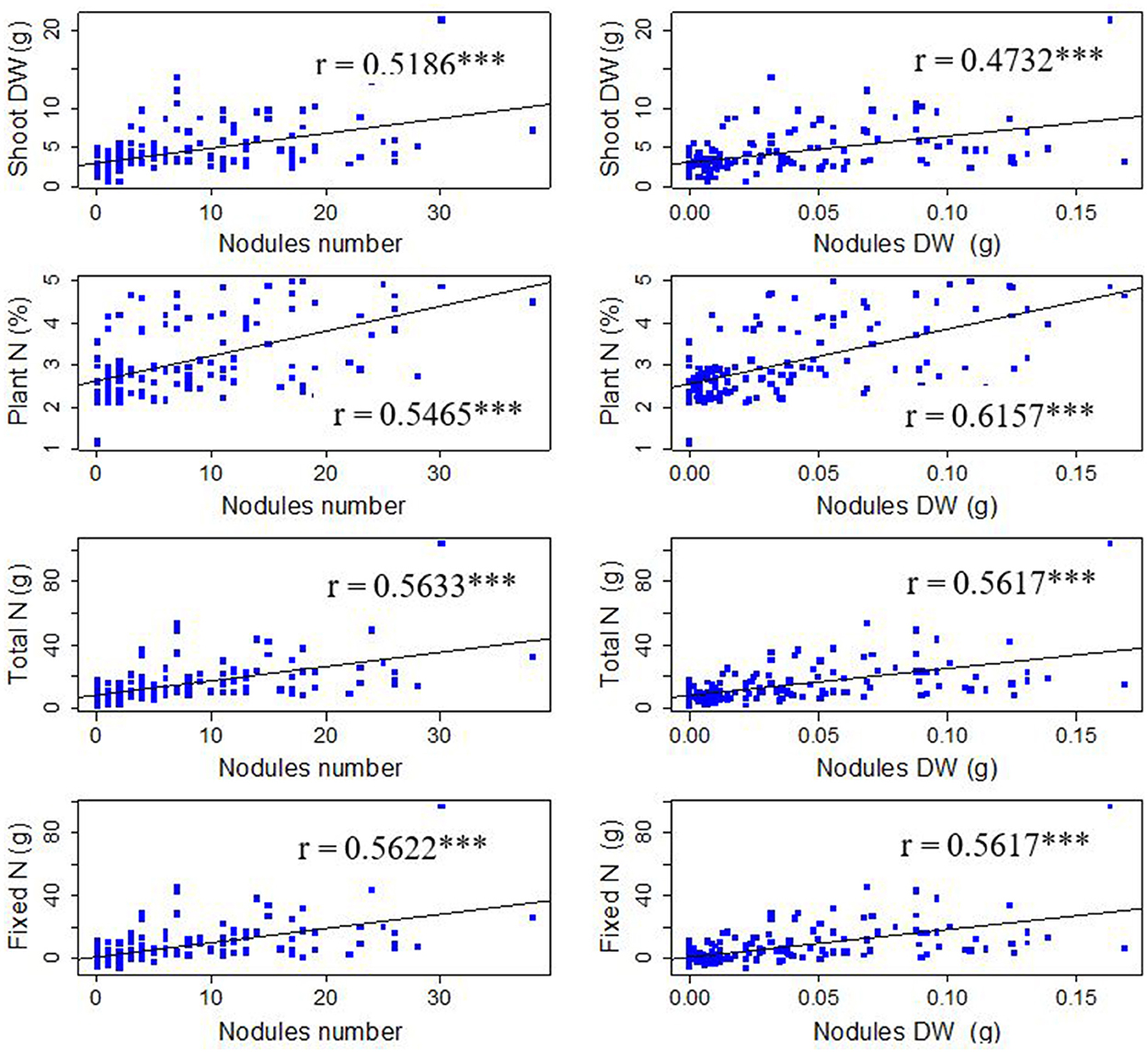
Figure 6. Trends analysis plots for SNF-related traits (*** significant at 0.001; n = 150, P < 0.001). N, Nitrogen; DW, Dry weight.
4. Discussion
4.1. In vitro N2 fixation in nitrogen-free medium
The findings of this study demonstrate the wide variability in the tested indigenous rhizobial isolates' capacity to fix nitrogen on NFM. The varied capacities of rhizobia isolates in N2 fixation are indicated by the difference in the diameter of the N2 fixation zones (Aloo, 2021). The media color around the rhizobia colonies changes from blue to yellow due to the production of acidic metabolites during their growth on NFM (Baldani et al., 2014; Akintokun et al., 2019), which also indicates that the tested rhizobia are fast growers (Purwaningsih et al., 2021). Although there was no statistical significance between TZSR27A, TZSR25A, and the three isolates TZSR39C, TZSR41B, and TZSR56C, but the best isolate, TZSR27A, in terms of larger diameter of N2 fixation zone ~1.87 cm on NFM, would be considered highest, based on previous studies on different types of rhizobia species (Aloo et al., 2021).
4.2. Experimental soil's ability to support rhizobia and growth of soybeans
Soil health, especially soil fertility, is an important factor in the success of the biological nitrogen fixation process. Soil reaction and nutrient availability are among the components of soil fertility, which accounts for the effectiveness of the BNF process. Soil reaction (soil pH) determines the suitability of the given soil for certain rhizobia species. In contrast, different nutrients in the soil have different functions in ensuring the success of the BNF process (Nakei et al., 2022). Before authentication of the abilities of different rhizobial isolates under screen house conditions, different physicochemical characteristics of experimental soil were analyzed. The soil belonged to medium texture, Sandy clay loam (SCL), which is favorable for nodulation compared with heavy and light textured soils (Ali, 2016). The soil reaction of an experimental soil ranged from slightly acidic to neutral soil pH. This describes its suitability for most microbial populations and activities and is ideal for the availability of macronutrients and solubility of micronutrients that support plant growth and development (Zaharan, 1999; Giller, 2001; Zhang et al., 2018). The very high percentage of soil organic carbon and medium total nitrogen determined in this study suggests that the soil was well-enriched with organic matter contents (Giller, 2001). For rhizobial activities, a higher carbon level is important in providing energy, while a medium nitrogen level is sufficient for the biological nitrogen fixation process (Liu et al., 2018; Wang et al., 2020).
A very high level of available phosphorus determined in our experimental soil indicates its sufficiency for energy acquisition, storage, and utilization in the N2 fixation process by the plant (Mmbaga et al., 2014; Abebe, 2017). Higher levels of micronutrients Zn, Mn, and Fe and a higher level of Cu signify the availability of these nutrients for plant uptake as well as serving various functions in the SNF process (González-Guerrero et al., 2014; Kasper et al., 2019). A higher level of zinc is crucial for plants and rhizobia during the SNF process for enhancing the expression of superoxide dismutase required during nodule development (Rubio et al., 2007). Manganese is involved in the initial stages of root colonization by rhizobia through the mediation of rhizobial lectin in binding to the root hair tips; its extreme levels enhance the formation of many symbiotic nodules and hence a more fixed amount of nitrogen (González-Guerrero et al., 2014). Extreme iron levels will facilitate the N2 fixation process because iron is a component of the nitrogenase enzyme and a cofactor for other proteins (cytochromes and leghemoglobin) inside the nodule (Bonilla and Bolaños, 2009). The observed higher level of Cu is crucial for promoting N2 fixation per nodule and raising the levels of N in plant tissues because copper is a member of the cytochromes that mediate energy transduction in bacteroids (Rubio et al., 2007; González-Guerrero et al., 2014).
Exchangeable bases, especially Ca, Mg, and K serve different important roles in soils for rhizobia activities as well as the growth and development of plants. The observed higher level of calcium is important in promoting the abundance of rhizobia and the development of roots, enhances the attachment of rhizobia to root hairs, root infection by rhizobia, and hence nodulation (Miwa et al., 2006; Bonilla and Bolaños, 2009; Capoen et al., 2009; Debona et al., 2017; Dabessa et al., 2018). Magnesium is a co-factor for urease and nitrogenase enzymes (Cakmak and Yazici, 2010; Gransee and Führs, 2013), and its involvement in the stabilization of cell membrane, nucleic acids, and ribosomes of the rhizobia (O'Hara, 2001) will increase the formation of the nodule (Kiss et al., 2004; Ramesh and Winkler, 2010; Peng et al., 2018). Moreover, the observed higher level of potassium will help in the regulation of water by the plant and influence the growth of roots, increasing the chances of infection by rhizobia and hence nodulation (Youssef et al., 2001; Wakeel et al., 2011; Mfilinge et al., 2014). In addition, the cation exchange capacity of an experimental soil was medium, suggesting a balance of the soil's exchangeable bases and acid, forming cations, Al3+ and H+ (de Borja Reis et al., 2021). Exchangeable sodium is very low; however, sodium is required in very small amounts in soil for SNF to avoid salinity stress. Its role in the soil can be replaced by the presence of potassium (Bonilla and Bolaños, 2009; Wakeel et al., 2011).
4.3. Nodulation and plant growth promotion by rhizobia isolates
The significant difference observed in the number of nodules and nodules' dry weight indicates the different abilities of rhizobia in forming symbiotic nodules (Gebrehana and Dagnaw, 2020). Nodulation, especially nodules' dry weight, is an important parameter in determining the competency of rhizobia in fixing nitrogen. Moreover, the composition of nodules, including bacteroids (N2 fixing rhizobia), proteins, enzymes, and nutrients, determines the dry weight of nodules as demonstrated by different isolates in this study. Different components perform different functions to ensure a successful and efficient N2 fixation process (Schwember et al., 2019). However, nodule numbers may not exactly determine the extent of N2 fixation when they miss some components such as leghemoglobin responsible for the supply of oxygen to bacteroids inside the nodules (Ott et al., 2005; Thilakarathna and Raizada, 2017). However, many nodules with higher nodule dry weight are an indication of effective symbiotic nodulation and host specificity (Alam et al., 2015; Gebrehana and Dagnaw, 2020). Similarly, in this study, the plants were inoculated with some isolates, and among them, TZSR41A had many nodules with the highest nodule dry weight. However, in this study, the shoot dry weight observed in plants inoculated with isolate TZSR21C was −4.73%, lesser than un-inoculated plants, which may be attributed to the modification of root system architecture by the inoculated rhizobia (Concha et al., 2020), which formed non-symbiotic nodules, hence, reducing the chances of nutrients uptake which affected the growth of shoot. Furthermore, the highest shoot dry weight and shoot length for a particular isolate suggest its effectiveness in N2 fixation (Hungria and Vargas, 2000; Alam et al., 2015; Schwember et al., 2019).
In this study, the root dry weight differed significantly while there was a non-significant difference in root length among the rhizobia isolates. However, the lack of significant difference in root length may be attributed to the regulation of the growth of roots and modification in root system architecture by rhizobia during root infection and nodule formation (Concha et al., 2020). Furthermore, the reduced need of exploiting nutrients results in reduced root length, as the nutrients are made available around the rhizosphere either through fixed N or through solubilization of other nutrients. Root growth regulation and modification do not imply an absolute decrease in root length but rather balance a proportionality with the shoot for uptake and transportation of nutrients (Elkoca et al., 2015; Concha et al., 2020). Similarly, in this study, the highest root length of 43 cm recorded in TZSR40B is among the isolates with many nodules, indicating a balanced root system architecture and root growth as a result of reduced exploitation of nutrients induced by inoculation with rhizobia (Concha et al., 2020). However, extremely shorter roots, for instance, the one recorded in plants inoculated with TZSR63A, which is −22.4% lesser than un-inoculated plants, may be attributed to the alteration of root growth by promoting the lateral root formation likely through the modulation of auxin signaling by the inoculated rhizobia isolate (Zhao et al., 2018). Conversant to lesser root length, the root dry weight of the plants in the particular treatments have higher roots dry weight over un-inoculated plants. Overall, inoculated plants performed better than non-inoculated plants.
Moreover, compared with positive control treatments, legume fix, and urea, rhizobia isolates performed better for all the tested parameters except for plant height and root dry weight, which was higher in urea. Interestingly, in this study, the plants inoculated with two isolates, TZSR39C and TZSR41A, were observed to have consistently higher average values for most of the studied plant growth parameters except for root length and dry weight. Therefore, the above two isolates are potential in the production of rhizobia-based biofertilizers for intensification and sustainable production of soybean.
Inside the symbiotic nodules is where the rhizobia associate with plants to fix nitrogen and hence promote the growth and development of plants. It is highly likely that many symbiotic nodules with higher dry weight per plant lead to an increased level of fixed nitrogen and nutrient uptake by plants. This is supported by the observations made in this study on the significant positive correlations of nodule numbers and nodule dry weight with other plant growth parameters. Similar to this study, other researchers have reported significant correlations among the mentioned parameters (Appunu and Dhar, 2006; Youseif et al., 2014; Elkoca et al., 2015; Wang et al., 2020). This study showed a strong and significant positive correlation between the number of nodules and nodule dry weight. However, the correlation between nodules number and dry weight may be due to collinearity but many nodules may not necessarily exhibit higher dry weight due to the size and the composition of the nodules (Ott et al., 2005; Thilakarathna and Raizada, 2017). In addition, the observed positive significant correlation between nodulation (nodule numbers and dry) and shoot length, shoot dry weight, and the number of leaves suggests that the increase in nutrient availability is a result of increased symbiotic nodulation upon inoculation and hence above-ground biomass (Elkoca et al., 2015; Gitonga et al., 2021; Ozede and Olubukola, 2021). Although in this study, nodulation (nodules number and dry weight) has been used as a major parameter in measuring SNF, the nodules number is observed to have several limited proxy values in determining effective symbiosis (Hungria et al., 2001; Thilakarathna and Raizada, 2017); hence nodules dry weight is used as a better proxy (Thilakarathna et al., 2021). The observed significant positive correlation among different individual plant growth parameters can be linked to the increased growth and development of healthy plants through the availability of nutrients (Alam et al., 2015; Ntambo et al., 2017) mediated by N2 fixation attributed to inoculation of soybean seedlings with rhizobia (Aloo et al., 2021).
4.4. Symbiotic parameters as influenced by isolates
The significant increase in shoot nitrogen concentration, total N, fixed N, and symbiotic effectiveness observed among the tested isolates clearly demonstrates that the isolates were capable of fixing nitrogen under screen house conditions but in varying abilities. The increase in shoot N concentration corroborates (Iturralde et al., 2019), who found that inoculation increased nitrogen concentration in shoots of soybean plants. On the other hand, Subramanian and Kim (2014) reported an increase in soybean shoot total nitrogen with inoculation by symbiotically effective rhizobia. From this study, the plants inoculated with three isolates, TZSR25A, TZSR27B, and TZSR41A, that have the highest fixed N are those with also the highest shoot concentration of N and total N. In addition, inoculation of plants with two isolates, TZSR25A and TZSR26B, yielded high symbiotic effectiveness, suggesting that fixed N contributed to the total N content of the plant shoot. These findings agree with those of Dashti et al. (1998) that inoculation of soybean with effective rhizobia significantly increased fixed N, which ultimately contributes to the increment of total nitrogen in the shoot of plants. Chibeba et al. (2017) indicated that different rhizobia isolates had different abilities in fixing nitrogen and hence different relative symbiotic effectiveness. The 94% of isolates, which were effective in N2 fixation, implies that most of the isolates tested in this study had a higher capacity to fix nitrogen under controlled conditions. Furthermore, on-farm testing under field conditions is recommended for isolates, which performed well than urea treatment in the screen house potted experiment. This finding also suggests that these effective isolates can potentially be used to improve soybean productivity and N budget, hence contributing to sustainable intensification of the agricultural system, specifically in soybean production.
Nodulation (nodules number and dry weight) is the first symbiotic trait to be observed before measuring other traits, namely shoot dry weight, percentage plant N, and total N. This is because the measurement of other traits is dependent on the extent of nodulation and their influence on the variation of other SNF traits. The significant positive correlation between nodulation (nodules number and nodules dry weight) and shoot dry weight (Unkovich et al., 2010) suggests that inoculation of soybean seedlings with rhizobia increased N2 fixation. These results are in agreement with Alam et al. (2015) and Ntambo et al. (2017) who reported an increase in shoot dry weight as a measurable indicator of increased nitrogen concentration in plants resulting from symbiotic N2 fixation through inoculation. The nitrogen concentration in plant shoot biomass is the determinant of total and fixed nitrogen in plants inoculated with effective rhizobia (Elkoca et al., 2007; Ogutcu et al., 2008). The study conducted by Elkoca et al. (2015) and Chibeba et al. (2017) demonstrated that inoculation of legumes with effective rhizobia increased the concentration of N, fixed N, and total N concentration in plants. Moreover, different rhizobia isolates differ in symbiotic effectiveness, although the difference among some isolates was not statistically significant due to higher variation, but they are numerically different, and this is relative to their abilities to fix nitrogen (Chibeba et al., 2017). According to rating guidelines by Legesse (2016), the isolates with symbiotic effectiveness value of >85% are considered highly effective in nitrogen fixation regardless of their variation as observed in this study.
5. Conclusion and recommendations
The availability of nutrients to suffice crop requirements is crucial in increasing the productivity of crops. Nitrogen, the first and most limiting nutrient for crops is required in larger quantities for growth, development, and optimum yield. The available nutrient sources, synthetic, organic, and commercial biofertilizers, have been there, but still, there is an appreciable deficiency of nutrients including nitrogen in smallholder farming systems. It is high time to explore the effective indigenous species of rhizobia and commercialize their biofertilizer formulations for easy availability and accessibility to smallholder farming systems. The tested indigenous rhizobia species in this study demonstrated varying abilities with some highly effective ones in nodulation and promotion of plant growth and yield components as indicated by nodules and shoot dry weight of up to 0.137 and 10.99 g per plant and the increase in shoot dry weight of 75.82% for the plants inoculated by isolate TZSR41A. Moreover, about 50% of the isolates showed higher nitrogen-fixing abilities as indicated by their symbiotic effectiveness. Enhancement of plant growth and yield components results from increased nutrient availability and uptake. However, on-farm testing under field conditions is recommended for isolates, which performed well in the screen house potted experiments. Nevertheless, the availability of nutrients should be sustainable to ensure sustainable crop production. The two aspects, nutrient availability and crop productivity, should consider factors such as affordability by farmers, environmental safety, and effectiveness to ensure safe and nutritious food security. Therefore, the studied isolates are the potential for formulating biofertilizers for field applications and sustainable soybean productivity in Tanzania upon confirmation under field conditions.
Data availability statement
The raw data supporting the conclusions of this article will be made available by the authors, without undue reservation.
Author contributions
MDN, PAN, and PBV: conceptualization and methodology. MDN: original draft preparation. PAN and PBV: review and editing. All authors have read and agreed to the published version of the manuscript.
Acknowledgments
The authors thank all staff and technical experts from Nelson Mandela African Institution of Science and Technology (NMAIST) and Arusha-Tanzania for their guidance and support during sampling and laboratory and screen house experiments.
Conflict of interest
The authors declare that the research was conducted in the absence of any commercial or financial relationships that could be construed as a potential conflict of interest.
Publisher's note
All claims expressed in this article are solely those of the authors and do not necessarily represent those of their affiliated organizations, or those of the publisher, the editors and the reviewers. Any product that may be evaluated in this article, or claim that may be made by its manufacturer, is not guaranteed or endorsed by the publisher.
References
Abebe, Z. (2017). On-farm yield variability and responses of common bean (phaseolus vulgaris l.) varieties to rhizobium inoculation with inorganic fertilizer rates. J. Anim. Plant Sci. 32, 5120–5133. Available online at: http://www.m.elewa.org/JAPS
Abrar, T. H., and Letebo, A. A. (2017). Isolation and characterization of rhizobia from rhizospher and root nodule of cowpea, elephant and lab lab plants. Int. J. Novel Res. Interdiscipl. Stud. 4, 1–7. Available online at: www.noveltyjournals.com
Agoyi, E. E., Odong, T. L., Tumuhairwe, J. B., Chigeza, G., Diers, B. W., and Tukamuhabwa, P. (2017). Genotype by environment effects on promiscuous nodulation in soybean (Glycine Max L. Merrill). Agri. Food Secur. 6, 1–14. doi: 10.1186/s40066-017-0107-7
Akintokun, A. K., Ezaka, E., Akintokun, P. O., Shittu, O. B., and Taiwo, L. B. (2019). Isolation, screening and response of maize to plant growth promoting Rhizobacteria 50, 181–190. doi: 10.2478/sab-2019-0025
Alam, F., Bhuiyan, M. A. H., Alam, S. S., Waghmode, T. R., Kim, P. J., and Lee, Y. B. (2015). Effect of Rhizobium sp. BARIRGm901 inoculation on nodulation, nitrogen fi xation and yield of soybean (Glycine max) genotypes in gray terrace soil. Biosci. Biotechnol. Biochem. 79, 1660–1668. doi: 10.1080/09168451.2015.1044931
Ali, N. A. (2016). Effect of soil textural classes on the biological nitrogen fixation by bradyrhizobium measured by 15n dilution analysis. Baghdad Sci. J. 13, 0734. doi: 10.21123/bsj.2016.13.4.0734
Aloo, B. (2021). Characterization of rhizobacteria and their formulation into biofertilizers for potato (solanum tuberosum l.) growth promotion in Tanzania (Doctoral dissertation). The Nelson Mandela African Institution of Science and Technolgy, Arusha, Tanzania). Available online at: https://dspace.nm-aist.ac.tz/handle/20.500.12479/1329%0Ahttps://dspace.nm-aist.ac.tz/bitstream/handle/20.500.12479/1329/PhD_LiSe_Becky_Aloo_2021.pdf?sequence=1
Aloo, B. N., Mbega, E. R., and Makumba, B. A. (2021). “Sustainable food production systems for climate change mitigation: Indigenous rhizobacteria for potato bio-fertilization in Tanzania,” in African Handbook of Climate Change Adaptation (Cham: Springer), 1469–1495. doi: 10.1007/978-3-030-45106-6_276
Ampomah, O. Y., Ofori-ayeh, E., Solheim, B., and Svenning, M. M. (2008). Host range, symbiotic effectiveness and nodulation competitiveness of some indigenous cowpea bradyrhizobia isolates from the transitional savanna zone of Ghana. Afr. J. Biotechnol. 7, 988–996. Available online at: http://www.academicjournals.org/AJB
Appunu, C., and Dhar, B. (2006). Symbiotic effectiveness of acid-tolerant Bradyrhizobium strains with soybean in low pH soil. Afr. J. Biotechnol. 5, 842–845. Available online at: http://www.academicjournals.org/AJB
Baldani, J. I., Reis, V. M., Videira, S. S., Boddey, L. H., and Baldani, V. L. D. (2014). The art of isolating nitrogen-fixing bacteria from non-leguminous plants using N-free semi-solid media: a practical guide for microbiologists. Plant Soil 384, 413–431. doi: 10.1007/s11104-014-2186-6
Bonilla, I., and Bolaños, L. (2009). Mineral nutrition for legume-rhizobia symbiosis: B, Ca, N, P, S, K, Fe, Mo, Co, and Ni: a review. Sustain. Agric. Rev. 1, 253–274. doi: 10.1007/978-1-4020-9654-9_13
Bray, R. H., and Kurtz, L. T. (1945). Determination of total, organic and available forms of phosphorous in soils. Soil Sci. 58, 39–45. doi: 10.1097/00010694-194501000-00006
Cakmak, I., and Yazici, A. M. (2010). Magnesium: a forgotten element in crop production. Better Crops 94, 23–25.
Capoen, W., Herder, J. D., Sun, J., Verplancke, C., Keyser, A. D., Rycke, R. D., et al. (2009). Calcium spiking patterns and the role of the calcium/calmodulin-dependent kinase ccamk in lateral root base nodulation of sesbania rostrata. Plant Cell. 21, 1526–1540. doi: 10.1105/tpc.109.066233
Chianu, N., and Mairura, F. (2019). Situation Outlook_Tanzania Soybean Situation and Outlook Analysis: The Case of Tanzania. Gigiri: Tropical Soil Biology and Fertility; Institute of the International Center for Tropical Agriculture.
Chibeba, A. M., Kyei-Boahen, S., Guimarães, M. F., Nogueira, M. A., and Hungria, M. (2017). Isolation, characterization and selection of indigenous Bradyrhizobium strains with outstanding symbiotic performance to increase soybean yields in mozambique. Agric. Ecosyst. Environ. 246, 291–305. doi: 10.1016/j.agee.2017.06.017
Concha, C., Doerner, P., and Gutiérrez, R. (2020). The impact of the rhizobia-legume symbiosis on host root system architecture. J. Exp. Bot. 71, 3902–3921. doi: 10.1093/jxb/eraa198
Dabessa, A., Abebe, Z., and Bekele, S. (2018). Limitations and strategies to enhance biological nitrogen fixation in sub-humid tropics of Western Ethiopia. J. Agric. Biotechnol. Sustain. Dev. 10, 122–131. doi: 10.5897/JABSD2018.0318
Dashti, N., Zhang, F., Hynes, R., Smith, D. L., and Bellevue, S. A. (1998). Plant growth promoting rhizobacteria accelerate nodulation and increase nitrogen fixation activity by field grown soybean [Glycine max (L.) Merr.] under short season conditions. Plant Soil 200, 205–213. doi: 10.1023/A:1004358100856
de Borja Reis, A. F., Moro Rosso, L., Purcell, L. C., Naeve, S., Casteel, S. N., Kovács, P., et al. (2021). Environmental factors associated with nitrogen fixation prediction in soybean. J. Plant Sci. 12, 675410. doi: 10.3389/fpls.2021.675410
Debona, D., Cruz, M. F. A., and Rodrigues, F. A. (2017). Calcium-triggered accumulation of defense-related transcripts enhances wheat resistance to leaf blast. Trop. Plant Pathol. 42, 309–314. doi: 10.1007/s40858-017-0144-6
Elkoca, E., Kantar, F., and Sahin, F. (2007). Influence of nitrogen fixing and phosphorus solubilizing bacteria on the nodulation, plant growth, and yield of chickpea. J. Plant Nutr. 31, 157–171. doi: 10.1080/01904160701742097
Elkoca, E., Kocli, T., Gunes, A., Turan, M., Arietinum, C., and Of, L. C. (2015). The symbiotic performance and plant nutrient uptake of certain nationally registered chickpea (Cicer Arietinum L.) cultivars of Turkey. J. Plant Nutr. 38, 1427–1448. doi: 10.1080/01904167.2014.983123
Gebrehana, Z. G., and Dagnaw, L. A. (2020). Response of soybean to rhizobial inoculation and starter N fertilizer on nitisols of assosa and begi areas, Western Ethiopia. Environ. Syst. Res. 9, 14. doi: 10.1186/s40068-020-00174-5
Giller, K. E. (2001). “Targeting management of organic resources and mineral fertilizers: can we match scientists' fantasies with farmers' realities?,” in Integrated Plant Nutrient Management in Sub-Saharan Africa: From Concept to Practice (Wallingford: CABI Publishing), 155–171.
Giller, K. E., Delaune, T., Silva, J. V., Descheemaeker, K., van de Ven, G., Schut, A. G. T., et al. (2021). The future of farming: who will produce our food? Food Sec. 13, 1073–1099. doi: 10.1007/s12571-021-01184-6
Gitonga, N., Mawira, E. M., Njeru, R. C., and Maingi, J. M. (2021). Bradyrhizobium inoculation has a greater effect on soybean growth, production and yield quality in organic than conventional farming systems. Cogent Food Agric. 7, 1935529. doi: 10.1080/23311932.2021.1935529
González-Guerrero, M., Matthiadis, A., Sáez, Á., and Long, T. A. (2014). Fixating on metals: new insights into the role of metals in nodulation and symbiotic nitrogen fixation. Front. Plant Sci. 5, 45. doi: 10.3389/fpls.2014.00045
Gransee, A., and Führs, H. (2013). Magnesium mobility in soils as a challenge for soil and plant analysis, magnesium fertilization and root uptake under adverse growth conditions. Plant Soil 368, 5–21. doi: 10.1007/s11104-012-1567-y
Hartman, G. L., West, E. D., and Herman, T. K. (2011). Crops that feed the World 2. Soybean—worldwide production, use, and constraints caused by pathogens and pests. Food Sec. 3, 5–17. doi: 10.1007/s12571-010-0108-x
Hungria, M., Campo, R. J., Chueire, L. M. O., Grange, L., and Megías, M. (2001). Symbiotic effectiveness of fast-growing rhizobial strains isolated from soybean nodules in Brazil. Biol. Fertil. Soils 33, 387–394. doi: 10.1007/s003740100338
Hungria, M., and Vargas, M. A. T. (2000). Environmental factors affecting N2 xation in grain legumes in the tropics, with an emphasis on Brazil. Field Crops Res. 65, 151–164. doi: 10.1016/S0378-4290(99)00084-2
Iturralde, E. T., Covelli, J. M., Alvarez, F., Pérez-Giménez, J., Arrese-Igor, C., and Lodeiro, A. R. (2019). Soybean-nodulating strains with low intrinsic competitiveness for nodulation, good symbiotic performance, and stress-tolerance isolated from soybean-cropped soils in Argentina. Front. Microbiol. 10, 1061. doi: 10.3389/fmicb.2019.01061
Kacholi, D. S. (2020). Population structure, harvesting rate and regeneration status of four woody species in Kimboza forest reserve, Morogoro region—Tanzania. Plants Environ. 2, 94–100. doi: 10.22271/2582-3744.2020.sep.94
Kasper, S., Christo, B., Soti, P., and Racelis, A. (2019). Abiotic and biotic limitations to nodulation by leguminous cover crops in south Texas. Agriculture 9, 209. doi: 10.3390/agriculture9100209
Khojely, D. M., Ibrahim, S. E., Sapey, E., and Han, T. (2018). History, current status, and prospects of soybean production and research in sub-Saharan Africa. Crop J. 6, 226–235. doi: 10.1016/j.cj.2018.03.006
Kim, D. H., Kaashyap, M., Rathore, A., Das, R. R., Parupalli, S., Upadhyaya, H. D., et al. (2014). Phylogenetic diversity of mesorhizobium in chickpea. J. Biosci. 39, 513–517. doi: 10.1007/s12038-014-9429-9
Kiss, S. A., Stefanovits-Bányai, E., and Takács-Hájos, M. (2004). Magnesium-content of rhizobium nodules in different plants: the importance of magnesium in nitrogen-fixation of nodules. J. Am. Coll. Nutr. 23, 751–753. doi: 10.1080/07315724.2004.10719422
Komarek, A. M., Drogue, S., Chenoune, R., Hawkins, J., Msangi, S., Belhouchette, H., et al. (2017). Agricultural household effects of fertilizer price changes for smallholder farmers in central Malawi. Agric. Syst. 154, 168–178. doi: 10.1016/j.agsy.2017.03.016
Kopittke, P. M., Menzies, N. W., Wang, P., McKenna, B. A., and Lombi, E. (2019). Soil and the intensification of agriculture for global food security. Environ. Int. 132, 105078. doi: 10.1016/j.envint.2019.105078
Legesse, S. (2016). Isolation, identification and authentication of root nodule bacteria (rhizobia) in promoting sustainable agricultural productivity: a review. Dev. Country Stud. 6, 87–93.
Liu, A., Contador, C. A., Fan, K., Lam, H., Dicenzo, G. C., and Dunn, M. F. (2018). Interaction and regulation of carbon, nitrogen, and phosphorus metabolisms in root nodules of legumes. Front. Plant Sci. 9, 1860. doi: 10.3389/fpls.2018.01860
Maingi, J., and Shisanya, C. (2006). population levels of indigenous bradyrhizobia nodulating promiscuous soybean in two Kenyan soils of the semi-arid and semi-humid agroecological zones. J. Agric. Rural Dev. Trop. Subtrop. 107, 149–159.
Mathu, S., Herrmann, L., Pypers, P., Matiru, V., Lesueur, D., Mwirichia, R., et al. (2012). Soil Science and plant nutrition potential of indigenous bradyrhizobia versus commercial inoculants to improve cowpea (Vigna unguiculata L. walp.) and green gram (Vigna radiata L. wilczek.) yields in Kenya Potential of indigenous bradyrhizobia. Soil Sci. Plant Nutr. 58, 750–763. doi: 10.1080/00380768.2012.741041
Mfilinge, A., Mtei, K., and Ndakidemi, P. A. (2014). Effects of rhizobium inoculation and supplementation with P and K, on growth, leaf chlorophyll content and nitrogen fixation of bush bean varieties. Am. J. Res. Commun. 2, 49–87.
Mfwango, L. H., Mfwango, L. H., and Pranuthi, G. (2018). Application of decision support system for agro technology transfer (DSSAT) to Simulate agronomic practices for cultivation of maize in southern highland of Tanzania. Sci. Res. 9, 910–923. doi: 10.4236/as.2018.97063
Miwa, H., Sun, J., Oldroyd, G. E. D., and Downie, J. A. (2006). Analysis of nod-factor-induced calcium signaling in root hairs of symbiotically defective mutants of lotus japonicus. Mole. Plant-Microb. Interact. 19, 914–923. doi: 10.1094/MPMI-19-0914
Mmbaga, G. W., Mtei, K. M., and Ndakidemi, P. A. (2014). Extrapolations on the use of Rhizobium inoculants supplemented with phosphorus (P) and potassium (K) on growth and nutrition of legumes. Agric. Sci. 05, 1207–1226. doi: 10.4236/as.2014.512130
Motsara, M. R., and Roy, R. N. (2008). Guide to Laboratory Establishment for Plant Nutrient Analysis. Rome: Food And Agriculture Organization of the United Nations.
Msanya, B. M. (2012). Guide to General Rating of Some Chemical and Physical Soil. Morogoro: Department of Soil Science; Faculty of Agriculture; Sokoine University of Agriculture.
Nakei, M. D., Venkataramana, P. B., and Ndakidemi, P. A. (2022). Soybean-nodulating rhizobia: ecology, characterization, diversity, and growth promoting functions. Front. Sustain. Food Syst. 6, 824444. doi: 10.3389/fsufs.2022.824444
Nassary, E. K., Baijukya, F., and Ndakidemi, P. A. (2020). Intensification of common bean and maize production through rotations to improve food security for smallholder farmers. J. Agric. Food Res. 2, 100040. doi: 10.1016/j.jafr.2020.100040
Nelson, D.W., and Sommers, L.E. (1982). “Organic carbon in soils,” in Methods of Soil Analysis. Part 2 Agronomy Monograph No.9, eds A. L. Page, R. H. Miller, and D. R. Keeney (Madison, WI: American Society of Agronomy), 570–571.
Ntambo, M. S., Chilinda, I. S., Taruvinga, A., Hafeez, S., Anwar, T., Sharif, R., et al. (2017). The effect of Rhizobium inoculation with nitrogen fertilizer on growth and yield of soybeans (Glycine max L.). Int. J. Biosci. 10, 163–172. doi: 10.12692/ijb/10.3.163-172
Ogutcu, H., Algur, Ö., Elkoca, E., and Kantar, F. (2008). The determination of symbiotic effectiveness of rhizobium strains isolated from wild chickpeas collected from high altitudes in Erzurum. Turkish J. Agric. For. 32, 241–248. doi: 10.3906/tar-0801-35
O'Hara, G. W. (2001). Nutritional constraints on root nodule bacteria affecting symbiotic nitrogen fixation: a review. Aust. J. Exp. Agric. 41, 417–433. doi: 10.1071/EA00087
Ott, T., van Dongen, J. T., Gu, C., Krusell, L., Desbrosses, G., Vigeolas, H., et al. (2005). Symbiotic leghemoglobins are crucial for nitrogen fixation in legume root nodules but not for general plant growth and development. Curr. Biol. 15, 531–535. doi: 10.1016/j.cub.2005.01.042
Ozede, N. I., and Olubukola, O. B. (2021). Rhizobium and mycorrhizal fungal species improved soybean yield under drought stress conditions. Curr. Microbiol. 78, 1615–1627. doi: 10.1007/s00284-021-02432-w
Parveen, G., Noreen, R., Shafique, H. A., Sultana, V., Ehteshamul-Haque, S., and Athar, M. (2019). Role of rhizobia in suppressing the root diseases of soybean under soil amendment. Planta Daninha 37, 1–8. doi: 10.1590/s0100-83582019370100038
Peng, W. T., Zhang, L. D., Zhou, Z., Fu, C., Chen, Z. C., and Liao, H. (2018). Magnesium promotes root nodulation through facilitation of carbohydrate allocation in soybean. Physiol. Plant 163, 372–385. doi: 10.1111/ppl.12730
Peoples, M. B., Brockwell, J., Herridge, D. F., Rochester, I. J., Alves, B. J. R., Urquiaga, S., et al. (2009). The contributions of nitrogen-fixing crop legumes to the productivity of agricultural systems. Symbiosis 48, 1–17. doi: 10.1007/BF03179980
Pirbalouti, A. G., Allahdadi, I., Akbari, G. A., Golparvar, A. R., and Rostampoor, S. A. (2006). Effects of different strains of Rhizobium legominosarum biovar phaseoli on yield and N2 fixation rate of common bean (Phaseolus vulgaris L.) Iranian cultivars. Pak. J. Biol. Sci. 9, 1738–1743. doi: 10.3923/pjbs.2006.1738.1743
Purwaningsih, S., Agustiyani, D., and Antonius, S. (2021). Diversity, activity, and effectiveness of rhizobium bacteria as plant growth promoting rhizobacteria (PGPR) isolated from Dieng, central Java. Iran. J. Microbiol. 13, 130. doi: 10.18502/ijm.v13i1.5504
Ramesh, A., and Winkler, W. C. (2010). Magnesium-sensing riboswitches in bacteria. RNA Biol. 7, 77–83. doi: 10.4161/rna.7.1.10490
Res, J. M. B. (2013). In Vitro Screening of isolates for its plant growth promoting activities from the rhizosphere of alfalfa (Medicago Sativa). J. Microbiol. Biotechnol. 3:79–88.
Rubio, M. C., Becana, M., Sato, S., James, E. K., Tabata, S., and Spaink, H. P. (2007). Characterization of genomic clones and expression analysis of the three types of superoxide dismutases during nodule development in lotus japonicus. Mol. Plant Microbe Interact. 20, 262–275. doi: 10.1094/MPMI-20-3-0262
Sadigov, R. (2022). Rapid growth of the world population and its socioeconomic results. Sci. World J. 2022, 8110229. doi: 10.1155/2022/8110229
Santos, M. (2019). The State of Soybean in Africa: Soybean Varieties in Sub-Saharan Africa. Urban-Champain, IL: University of Illinois. Available online at: https://farmdocdaily.illinois.edu/2019/08/the-state-of-soybean-in-africa-soybean-varieties-insub-saharan-africa.html
Schwember, R., Schulze, J., Pozo, A., and Cabeza, R. A. (2019). Regulation of symbiotic nitrogen fixation in legume rootroot nodules. Plants 8, 333. doi: 10.3390/plants8090333
Senkoro, C. J., Ley, G. J., Marandu, A. E., Wortmann, C., Mzimbiri, M., Msaky, J., and Lyimo, S. D. (2017). Optimizing fertilizer use within the context of integrated soil fertility management in Tanzania. Fertil. Use Optim. Sub-Saharan Africa. 17, 176–192.
Simon, Z., Mtei, K., Gessesse, A., and Ndakidemi, P. A. (2015). Isolation and characterization of nitrogen fixing rhizobia from cultivated and uncultivated soils of northern Tanzania. Am. J. Plant Sci. 5, 4050–4067. doi: 10.4236/ajps.2014.526423
Simpson, M. G. L. (2015). Functions for Generating Restricted Permutations of Data. Available online at: https://github.com/gavinsimpson/permute
Sinegani, A. A. S., and Hosseinpur, A. (2010). Evaluation of effect of different sterilization methods on soil biomass phosphorus extracted with NaHCO3. Plant Soil Environ. 56, 156–162. doi: 10.17221/86/2009-PSE
Snapp, S., Rahmanian, M., and Batello, C. (2018). Pulse Crops for Sustainable Farms in Sub-Saharan Africa. United Nations. p. 1–57. doi: 10.18356/6795bfaf-en
Somasegaran, P., and Hoben, H. J. (2012). Handbook for Rhizobia Methods in Legume-Rhizobium Technology. New York, NY: Springer-Verlag. p. 1–135. doi: 10.1007/978-1-4613-8375-18
Sorvali, J., Kaseva, J., and Peltonen-Sainio, P. (2021). Farmer views on climate change—a longitudinal study of threats, opportunities and action. Clim. Change 164, 50. doi: 10.1007/s10584-021-03020-4
Subramanian, P., and Kim, K. (2014). Endophytic bacteria improve nodule function and plant nitrogen in soybean on co-inoculation with Bradyrhizobium japonicum. Plant Growth Regul. 76, 327–332. doi: 10.1007/s10725-014-9993-x
Thilakarathna, M. S., and Raizada, M. N. (2017). A meta-analysis of the effectiveness of diverse rhizobia inoculants on soybean traits under field conditions. Soil Biol. Biochem. 105, 177–196. doi: 10.1016/j.soilbio.2016.11.022
Thilakarathna, M. S., Torkamaneh, D., Bruce, R. W., Rajcan, I., Chu, G., Grainger, C. M., et al. (2021). Testing whether pre-pod-fill symbiotic nitrogen fixation in soybean is subject to drift or selection over 100 years of soybean breeding. Front. Agron. 3, 725813. doi: 10.3389/fagro.2021.725813
Thomas, G. W. (1982). Exchangeable Cations. Methods of Soil Analysis. Agronomy Monograph No. 9. Methods of Soil Analysis, Part, 2.
Unkovich, M. J., Baldock, J., and Peoples, M. B. (2010). Prospects and problems of simple linear models for estimating symbiotic N2 fixation by crop and pasture legumes. Plant Soil 329, 75–89. doi: 10.1007/s11104-009-0136-5
Vanlauwe, B., Hungria, M., Kanampiu, F., and Giller, K. E. (2019). The role of legumes in the sustainable intensification of African smallholder agriculture: lessons learnt and challenges for the future. Agric. Ecosyst. Environ. 284, 106–583. doi: 10.1016/j.agee.2019.106583
Vollset, S. E., Goren, E., Yuan, C.-W., Cao, J., Smith, A. E., Hsiao, T., et al. (2020). Fertility, mortality, migration, and population scenarios for 195 countries and territories from 2017 to 2100: a forecasting analysis for the global burden of disease study. Lancet 396, 1285–1306. doi: 10.1016/S0140-6736(20)30677-2
Wakeel, A., Schubert, S., Wakeel, A., Farooq, M., Qadir, M., and Schubert, S. (2011). Critical reviews in plant sciences potassium substitution by sodium in plants. Crit. Rev. Plant Sci. 30, 401–413. doi: 10.1080/07352689.2011.587728
Wang, H., Gu, C., Liu, X., Yang, C., and Li, W. (2020). Impact of soybean nodulation phenotypes and nitrogen fertilizer levels on the rhizosphere bacterial community. Front. Microbiol. 11, 750. doi: 10.3389/fmicb.2020.00750
Wilson, R. T. (2013). The Red Meat Value Chain in Tanzania A report from the Southern Highlands Food Systems Programme. Available online at: www.fao.org/publications (accessed November 26, 2022).
Wolde, Z., Wei, W., Ketema, H., Yirsaw, E., and Temesegn, H. (2021). Indicators of land, water, energy and food (LWEF) nexus resource drivers: a perspective on environmental degradation in the gidabo watershed, southern Ethiopia. Int. J. Environ. Res. Public Health 18, 5181. doi: 10.3390/ijerph18105181
Youseif, S. H., Abd El-Megeed, F. H., Ageez, A., Mohamed, Z. K., Shamseldin, A., and Saleh, S. A. (2014). Phenotypic characteristics and genetic diversity of rhizobia nodulating soybean in Egyptian soils. Eur. J. Soil Biol. 60, 34–43. doi: 10.1016/j.ejsobi.2013.10.008
Youssef, G., Yanni, A., Rizk, Y., Rizk, A., Faiza, K., Abd El-Fattah, A., et al. (2001). The beneficial plant growth-promoting association of Rhizobium leguminosarum bv. trifolii with rice roots. Austral. J. Plant Physiol. 28, 845–870. doi: 10.1071/PP01069
Yuan, K., Miwa, H., Iizuka, M., Yokoyama, T., Fujii, Y., and Okazaki, S. (2016). Genetic diversity and symbiotic phenotype of hairy vetch rhizobia in Japan. Microbes Environ. 31, 121–126. doi: 10.1264/jsme2.ME15184
Zaharan, H. H. (1999). Zahara soil stress conditions for rhizobia activities. Miocrobiol. Mole. Biol. Rev. 63, 968–989.
Zhang, B., Du, N., Li, Y., Shi, P., and Wei, G. (2018). Science of the total environment distinct biogeographic patterns of rhizobia and non-rhizobial endophytes associated with soybean nodules across china. Sci. Total Environ. 643, 569–578. doi: 10.1016/j.scitotenv.2018.06.240
Keywords: biological nitrogen fixation, rhizobial isolates, soybean, soybean-nodulating rhizobia, symbiotic performance
Citation: Nakei MD, Venkataramana PB and Ndakidemi PA (2023) Preliminary symbiotic performance of indigenous soybean (Glycine max)-nodulating rhizobia from agricultural soils of Tanzania. Front. Sustain. Food Syst. 6:1085843. doi: 10.3389/fsufs.2022.1085843
Received: 31 October 2022; Accepted: 28 December 2022;
Published: 26 January 2023.
Edited by:
Benjamin Karikari, University for Development Studies, GhanaReviewed by:
Mustapha Mohammed, University for Development Studies, GhanaRakesh S, National Academy of Agricultural Research Management (ICAR), India
Jacob Ulzen, University of Ghana, Ghana
Copyright © 2023 Nakei, Venkataramana and Ndakidemi. This is an open-access article distributed under the terms of the Creative Commons Attribution License (CC BY). The use, distribution or reproduction in other forums is permitted, provided the original author(s) and the copyright owner(s) are credited and that the original publication in this journal is cited, in accordance with accepted academic practice. No use, distribution or reproduction is permitted which does not comply with these terms.
*Correspondence: Monica D. Nakei, bmFrZWltJiN4MDAwNDA7bm0tYWlzdC5hYy50eg==